Accessibility Links
- Skip to content
- Skip to search IOPscience
- Skip to Journals list
- Accessibility help
- Accessibility Help
Click here to close this panel.

As a society-owned publisher with a legacy of serving scientific communities, we are committed to offering a home to all scientifically valid and rigorously reviewed research. In doing so, we aim to accelerate the dissemination of scientific knowledge and the advancement of scholarly communications to benefit all.
Nano Express supports this mission and actively demonstrates our core values of inclusive publishing and trusted science . To find out more about these values and how they can help you publish your next paper with us, visit our journal scope .
Purpose-led Publishing is a coalition of three not-for-profit publishers in the field of physical sciences: AIP Publishing, the American Physical Society and IOP Publishing.
Together, as publishers that will always put purpose above profit, we have defined a set of industry standards that underpin high-quality, ethical scholarly communications.
We are proudly declaring that science is our only shareholder.
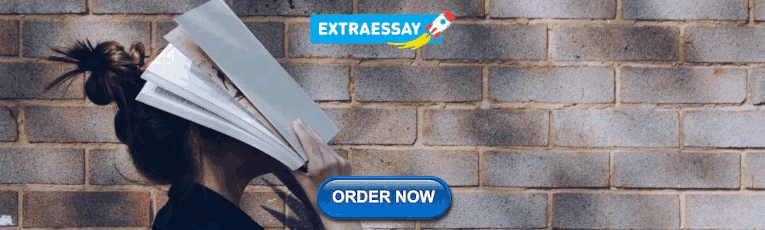
Synthesis of ZnO nanoparticles by two different methods & comparison of their structural, antibacterial, photocatalytic and optical properties
Md Jahidul Haque 1 , Md Masum Bellah 1 , Md Rakibu Hassan 1 and Suhanur Rahman 1
Published 16 March 2020 • © 2020 The Author(s). Published by IOP Publishing Ltd Nano Express , Volume 1 , Number 1 Citation Md Jahidul Haque et al 2020 Nano Ex. 1 010007 DOI 10.1088/2632-959X/ab7a43
Article metrics
29077 Total downloads
Share this article
Author e-mails.
Author affiliations
1 Department of Glass & Ceramic Engineering, Rajshahi University of Engineering & Technology (RUET), Rajshahi-6204, Bangladesh
Md Jahidul Haque https://orcid.org/0000-0001-7945-5937
- Received 23 December 2019
- Revised 3 February 2020
- Accepted 26 February 2020
- Published 16 March 2020
Peer review information
Method : Single-anonymous Revisions: 1 Screened for originality? Yes
Buy this article in print
In this work, two different methods (sol-gel and biosynthesis) were adopted for the synthesis of zinc oxide (ZnO) nanoparticles. The leaf extract of Azadirachta Indica (Neem) was utilized in the biosynthesis scheme. Structural, antibacterial, photocatalytic and optical performances of the two variants were analyzed. Both variants demonstrated a wurtzite hexagonal structure. The biosynthesized variant (25.97 nm) exhibited smaller particles than that of the sol-gel variant (33.20 nm). The morphological analysis revealed that most of the particles of the sol-gel variant remained within the range of 15 nm to 68 nm while for the biosynthesized variant the range was 10–70 nm. The antibacterial assessment was redacted by using the agar well diffusion method in which the bacteria medium was Escherichia coli O157: H7. The zone of inhibition of bacterial growth was higher in the biosynthesized variant (14.5 mm). The photocatalytic performances of the nanoparticles were determined through the degradation of methylene blue dye in which the biosynthesized variant provided better performance. The electron spin resonance (EPR) analysis revealed that the free OH · radicals were the primary active species for this degradation phenomenon. The absorption band of the sol-gel and biosynthesized variants were 363 nm and 356 nm respectively. The optical band gap energy of the biosynthesized variant (3.25 eV) was slightly higher than that of the sol-gel variant (3.23 eV). Nevertheless, the improved antibacterial and photocatalytic responses of the biosynthesized variants were obtained due to the higher rate of stabilization mechanism of the nanoparticles by the organic chemicals (terpenoids) present in the Neem leaf extract.
Export citation and abstract BibTeX RIS
Original content from this work may be used under the terms of the Creative Commons Attribution 4.0 licence . Any further distribution of this work must maintain attribution to the author(s) and the title of the work, journal citation and DOI.
1. Introduction
As a rapidly growing sector in materials science, nanotechnology and nanoscience deal with materials that have particles within a size range of 1 to 100 nm and a high surface-to-volume ratio [ 1 ]. In general form, these particles are termed as nanoparticles (NPs) which exhibit highly controllable physical, chemical and biological properties in the atomic and sub-atomic levels. However, these unique features create opportunities to use them in different sectors such as electronics, optoelectronics, agriculture, communications, and biomedicine [ 2 , 3 ].
Although, several NPs are showing their effectiveness in different sectors of technology, but zinc oxide (ZnO) NPs have gained much more importance in the recent years due to their attractive and outstanding properties such as high chemical stability, high photostability, high electrochemical coupling coefficient and a wide range of radiation absorption [ 4 ]. Again, ZnO NPs are also recognized as n-type multi-functional semiconductor materials that have a wide band gap of 3.37 eV and exciton binding energy up to 60 meV even at room temperature [ 1 ]. Nowadays, ZnO NPs are predominantly used as antimicrobial agents, delivering systems vaccines and anti-cancer systems, photocatalyst, biosensors, energy generators and bio-imaging materials [ 5 – 7 ]. Among themselves, the photocatalytic application of ZnO NPs is significant. However, the photocatalytic performance of ZnO NPs can be significantly enhanced by adopting two ways. The first one involves the reduction of particle sizes by using efficient synthesis methods, while the second one involves the change of structural morphology by the incorporation of several elements (such as metal, non-metal, noble metal, transition metal, etc) into the crystal structure of ZnO NPs. However, in this work, we will proceed by adopting the first one.
Several fabrication techniques are used to produce ZnO NPs such as thermal hydrolysis techniques, hydrothermal processing, sol-gel method, vapor condensation method, spray pyrolysis and thermochemical techniques [ 8 ]. Nevertheless, recently a new synthesis method has been introduced and that is called biosynthesis scheme in which the NPs are prepared by using biological materials having significant reducing and stabilizing features. Moreover, NPs with variable size and shape can be achieved through this process.
Researchers proposed several possible plant extracts and fungal biomasses that were used in the green synthesis of ZnO NPs such as Aloe Barbadensis Miller (Aloe Vera) leaf extract [ 9 ], Poncirus trifoliate leaf extract [ 10 ], Parthenium hysterophorus L. (Carrot grass) leaf extract [ 11 ], Aspergillus aeneus [ 12 ], Calotropis procera latex [ 13 ], Sedum alfredii Hance [ 14 ], Physalis alkekengi L. [ 15 ], etc. However, the smaller particle size of ZnO NPs was observed by using Poncirus trifoliate leaf extract (8.48–32.51 nm), while for others, the results were satisfactory. In addition, another potential element for the preparation of ZnO NPs through the biosynthesis method is considered to be a leaf extract of Azadirachta indica (Neem leaf). The leaf extract contains highly active phytochemicals and enzymes that participate in the oxidation or reduction reactions that occur during the fabrication method and manipulate the bulk ZnO to convert into ZnO NPs [ 16 ]. Moreover, Neem leaf provides significant biological restrictions against bacterial growth and fungal growth [ 17 ].
The present study focused on the preparation of ZnO NPs by two different methods. The first one is the sol-gel method, while the second one is the biosynthesis method in which the Neem leaf extract was used as a mandatory element. A comparison of the properties (structural, antibacterial, photocatalytic and optical) between the two variants of ZnO NPs was performed. Here, the sol-gel synthesized and biosynthesized ZnO nanoparticles were nominated as ZnO A NPs and ZnO B NPs respectively.
2. Methodology
2.1. materials.
All the starting raw materials including zinc acetate dihydrate [Zn(CH 3 COO) 2 .2H 2 O, Merck Specialties, India], sodium hydroxide [NaOH, Merck Specialties, India] and absolute ethanol [CH 3 CH 2 OH, Merck Specialties, Germany) were maintained at a high purity level (>99%). However, in the biosynthesis method, another raw material was also used and that was the leaf of Azadirachta indica (Neem leaf).
2.2. Synthesis of ZnO nanoparticles (ZnO A NPs) by sol-gel method
At first, 20 gm Zn(CH 3 COO) 2 .2H 2 O was mixed into 150 ml distilled water and stirred for 20 min at 35 °C to produce a zinc acetate solution. Again, 80 gm NaOH powder was weighed, mixed into 80 ml water and stirred for around 20 min at 35 °C for producing NaOH solution. After mixing both solutions, the titration reaction was performed by the addition of 100 ml ethanol into the drop-wise manner accompanied by vigorous stirring. The stirring was continued for around 90 min to complete the reaction for obtaining a gel-like product. Then the gel was dried at 80 °C overnight and calcined in an oven at 250 °C for 4 h. Finally, ZnO nanoparticles were prepared. However, the overall chemical reaction for the preparation of ZnO nanoparticles by using NaOH can be expressed as:
2.3. Synthesis of ZnO nanoparticles (ZnO B NPs) by biosynthesis method
At first, the neem (A. Indica) leaves were collected from the Azadirachta Indica trees on the campus of Rajshahi University of Engineering and Technology, Bangladesh. After washing with distilled water, the leaves were dried into a dryer for 24 h. Then 20 gm dried leaves were smashed and mixed with 50 ml distilled water. After that, the mixture was stirred by a magnetic stirrer and heated at 60 °C for 1 h. As the mixture displayed a yellow color, it was filtered using the Whatman TM filter paper. However, the extract solution was used for further preparation of ZnO nanoparticles. The overall process for the preparation of Neem leaf extract is stereotyped in figure 1 .
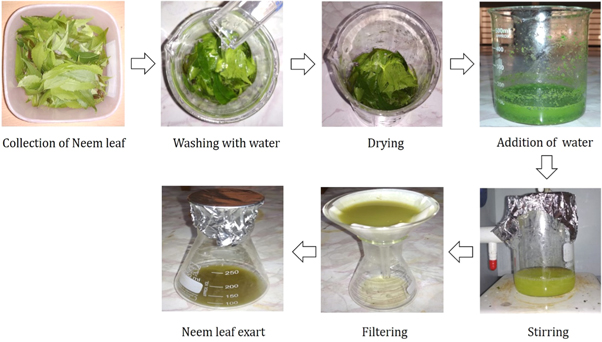
Figure 1. Process flow diagram for the preparation of Neem leaf extract.
Download figure:
The next step included the preparation of the zinc acetate solution. For this, 21.94 gm Zn(CH 3 COO) 2 .2H 2 O was mixed into 50 ml water and stirred for 20 min at 35 °C. Similarly, in order to prepare a NaOH solution, 4 gm NaOH powder was added into 50 ml distilled water and simultaneously stirred for 20 min at 35 °C. Both solutions were then mixed by vigorous stirring. During this stirring process, the neem leaf extract was drop-wise mixed with the solution. As the addition of neem leaf continued, white precipitation of nanoparticles appeared. Then the solution was filtered and the filtered product was dried at 80 °C for 4 h. After that, the dried powder was calcined at 250 °C for 4 h and grounded to obtain the desired ZnO nanoparticles.
2.4. Characterization of ZnO NPs
X-ray diffraction was performed for structural analysis employing 40 kV-40 ma (scanning step of 0.02°) and Cu- K α radiation having wavelengths of K α 1 = 1.54060 Å, K α 2 = 1.54439 Å (Bruker Advance D8, Germany). Morphological characterization was accomplished by scanning electron microscopy (ZEISS EVO 18, UK). The optical properties were determined through UV–vis spectroscopy (SHIMADZU UV/Vis-1650 PC, Japan) into a range of 200–800 nm.
2.5. Antibacterial analysis of ZnO NPs
Escherichia coli bacteria were mainly involved in the determination of the antibacterial performance of ZnO NPs. Initially, the bacteria was stock-cultured in brain heart infusion (BHI) growth medium at −20 °C. Around 3 ml of BHI broth was added to 300 ml of stock-culture and preserved the culture overnight at 36 °C ± 1 °C for 24 h. After 24 h of incubation, dilution of the bacterial suspension (inoculum) was accomplished by using sterile saline. To indicate the bacterial growth during the test, a solution of 2-(4-iodophenyl)−3-(4-nitrophenyl)−5-phenyltetrazolium chloride (INT) in ethanol was added to the bacterial inoculum. Then the inoculum was distributed on a Mueller Hinton Agar Petri Dish in a consistent manner. After that, ZnO A NPs and ZnO B NPs were placed into the wells (prepared by cutting the agar gel) and the systems were preserved at 36 °C ± 1 °C for 24 h to allow successive incubation. After 24 h, the growth of bacteria was monitored and finally, the zone of inhibition for bacterial growth was determined in mm scale.
2.6. Photocatalytic analysis of ZnO NPs
The photocatalytic analysis was performed by monitoring the degradation of Methylene Blue (MB) dye due to ZnO NPs under the influence of UV radiation (having intensity ∼120 μ W cm −2 and wavelength ∼300–400 nm). At first, 5 gm NPs were added into MB solution and mixed properly. The mixture was placed in the dark for 2 h and then irradiated with UV rays with subsequent stirring action and at a variation of time (0, 40, 80, 120, 160, 200 min). The absorbance of the mixture was measured by UV–vis spectroscopy (SHIMADZU UV/Vis-1650 PC, Japan). The efficiency of photodegradation was measured by the following equation:
Where C 0 is the absorption of MB solution before the addition of ZnO NPs and C 1 is the absorption of the mixture solution with respect to time t.
ESR (electron spin resonance) analysis was performed using the EPR spectrometer (Bruker EMX MicroX, Germany) for the identification of the major factor that provides effective photocatalytic performance. During this characterization, DMPO (5,5-dimethyl-1-pyrroline-N-oxide) was used as a spin-trapped reagent in methanol and aqueous state. Moreover, the analysis was performed both in the presence and absence of light irradiation.
3. Results and discussion
3.1. effect analysis of neem leaf extract.
Neem leaf extract contains various phytochemicals such as flavones, quinines, organic acids, aldehyde and ketones which act as reducing agents and significantly reduces the particle sizes. After the successive reduction of particle sizes, the NPs are also affected by the terpenoids. Because of the interaction between the terpenoids and the ZnO NPs become stabilized as terpenoids are effective capping and stabilizing agents. The corresponding mechanism is graphically abstracted into figure 2 . Moreover, the possible seven types of terpenoids that are present in Neem leaf extract are stereotyped in figure 3 .
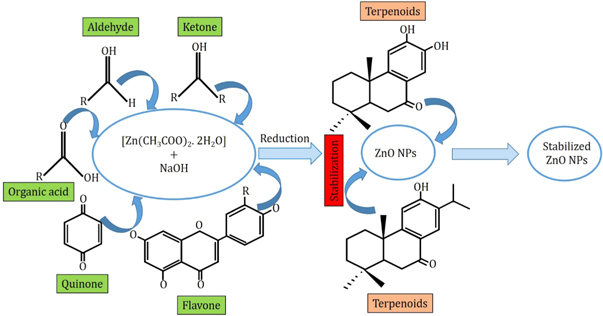
Figure 2. Schematic representation of the mechanism of size reduction and stabilization of ZnO NPs during the biosynthesis fabrication scheme using Neem leaf extract.
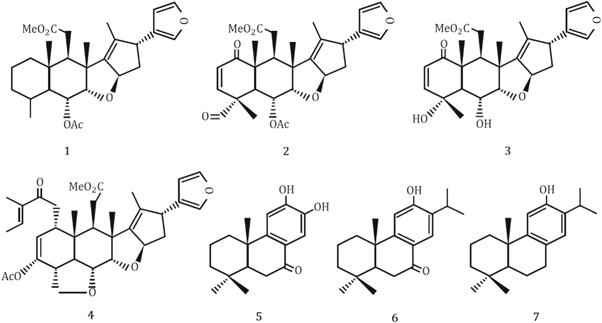
Figure 3. Chemical structures of different types of terpenoids subsisting in the Neem leaf extract.
3.2. X-ray diffraction analysis
Figure 4 represents the corresponding X-ray diffraction patterns of ZnO nanoparticles synthesized by sol-gel and bio-synthesis schemes respectively. The intense peaks at the crystal faces (100), (002), (101), (102), (110) assure the emergence of hexagonal wurtzite structure (as shown in figure 5 ) which belong to the space group of P6 3mc (JCPDS card no. 36-1451) [ 18 ]. The bio-synthesized ZnO nano-particles show more acute diffraction peak value introducing the appearance of the high percentage of crystalline phases. In addition, no impurity phases are present in the samples.
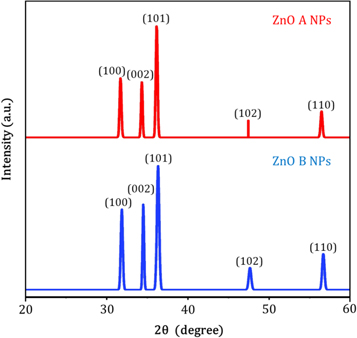
Figure 4. XRD patterns of ZnO A and ZnO B NPs.
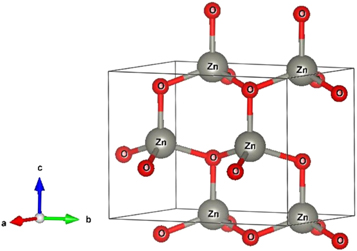
Figure 5. Schematic wurtzite crystal structure of ZnO NPs.
However, considering the most severe diffraction peak (101), the crystallite size (D) can be calculated in accordance with the Debye Scherer formula [ 19 ]:
Hither, β is the Full Width at Half Maxima of the corresponding peak, k is a dimensionless shape factor (∼0.94), while λ is the wavelength of Cu K α radiation (1.54 Å) and ϴ is the Bragg angle. D is mainly the mean size of the ordered domains which is considered to be equal to the particle size (applicable for only particles less than 100 nm). So, the average particle size of ZnO A NPs and ZnO B NPs is 33.20 nm and 25.95 nm respectively [ 19 ]. Again, there remains an inverse relationship between the β and the D which means that narrower peaks are resulted due to larger particles while broader particles are obtained because of smaller particles. The ZnO NPs showed a good agreement with this statement.
Since the crystallite size can be further employed for the determination of defect concentration within the specimen which is designated as the dislocation density ( δ ) and the leading formulae is adopted for this purpose [ 20 ]:
From the exploration of diffraction data, the lattice constant (a & c), inter-planar spacing (d) and unit cell volume (V) of the specimens (table 1 ) can also be enumerated by utilizing the following formulas respectively [ 21 ]:
Where, h, k, l belong to Miller indices.
Table 1. Structural information on ZnO A and ZnO B NPs.
Besides, the lengthening of the stricture (L) between Zn and O can be enumerated by the following equation [ 20 ]:
Where u corresponds to parameterized constant belonging to wurtzite structure and can be expressed as:
In accordance with the Williamson-Hall proposition, the lattice strain was calculated by adopting the undermentioned equation [ 20 ]:
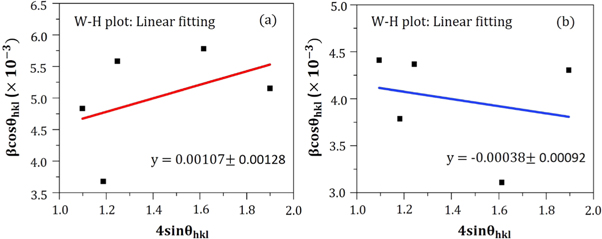
Figure 6. W-H plot of (a) ZnO A NPs and (b) ZnO B NPs for the measurement of lattice strain.
3.3. Morphological analysis
Figures 7 (a) and (b) shows the scanning electron micrographs of ZnO A and ZnO B NPs respectively. From the previous section, we have learned that the average particle size of ZnO B NPs (25.97 nm) is smaller than that of ZnO A NPs (33.20 nm). This can be also caused due to the presence of terpenoids in the Neem leaf extract. The terpenoid act not only as a stabilizing agent but also as a powerful reducing agent that interacts with ZnO NPs and reduces its size significantly [ 8 , 17 ]. Moreover, the maximum particles of ZnO A NPs remain between the range of 15 nm to 68 nm, whereas for ZnO B NPs the range lies from 10 nm to 70 nm.
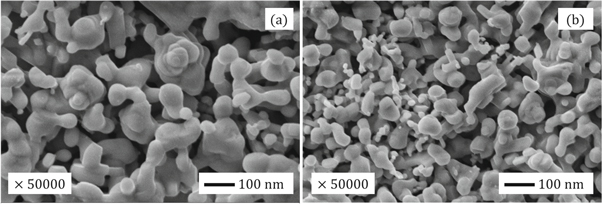
Figure 7. SEM micrographs of (a) ZnO A NPs and (b) ZnO B NPs.
3.4. Antibacterial activity
Antibacterial activity of ZnO A NPs and ZnO B NPs was analyzed by adopting the agar well diffusion method using Escherichia coli O157: H7 as the bacterial medium. Generally, there involve three mechanisms behind the interaction between the bacteria and the NPs. The first one involves the formation of extremely active hydroxyls and the second one involves the deposition of NPs on the bacteria surface. In addition, for the last one, the NPs accumulates in the cytoplasm or in the periplasmic region of bacteria cell which disrupts the cellular operations and simultaneously disorganizes the membrane. However, in consideration of E. coli , ZnO NPs firstly disorganize the membrane of E. coli and enters into the cytoplasmic region. Positioning themselves into the cytoplasm, the NPs neutralizes the respiratory enzymes and increases the emersion of cytoplasmic contents into the outward direction which impairs the membrane and finally kills the E. coli bacteria resulting in a zone of inhibition of bacterial growth around itself [ 3 , 23 ].
From figure 8 , it is observed that the zone of inhibition of bacterial growth due to ZnO A NPs is different from the zone of inhibition that is caused by ZnO B NPs. However, ZnO B NPs introduce a higher zone of inhibition than ZnO A nanoparticles and the measurements of the inhibition zone of bacterial growth are tabulated in table 2 . According to Krishna R Rangupathi, the antibacterial activity of nanoparticles is a size-dependent property and the property enhances with the reduction of particle size [ 23 ]. As the ZnO B NPs have smaller particle size as well as higher surface area, they show more antibacterial potential than that of ZnO A NPs [ 2 ].
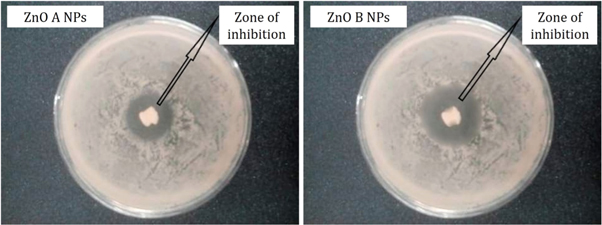
Figure 8. Antibacterial analysis of ZnO NPs showing the zone of inhibition of the growth of Escherichia coli O157: H7.
Table 2. Antibacterial measurements of ZnO A NPs and ZnO B NPs.
3.5. Photocatalytic activity
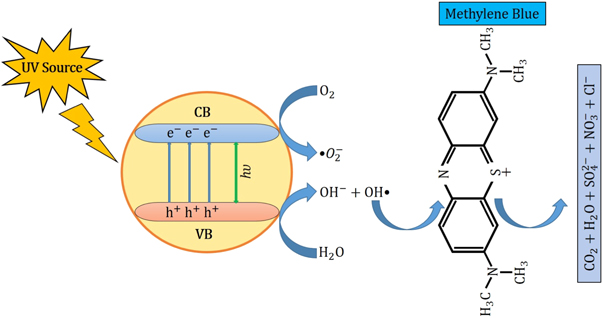
Figure 9. Degradation mechanism of MB dye by ZnO NPs under the influence of UV irradiation.
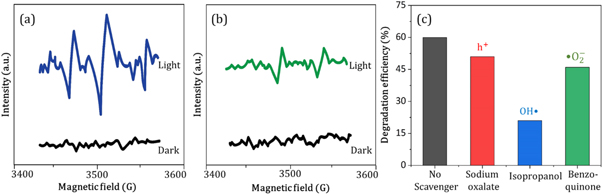
However, the corresponding reactions in the photodegradation scheme can be summarized as below [ 24 , 25 ]:
Figure 11 displays the discoloration of MB dye due to the photocatalytic action of ZnO NPs at different times (0, 40 and 120 min). However, figures 12 (a) and (b) illustrates the absorption spectra of MB dye as a function of wavelength under the influence of UV radiation at a variation of time i.e. 0, 40, 80, 120, 160, 200 min. From the graph, it is observed that the absorption rate of MB containing ZnO B NPs decreases more rapidly than that of ZnO A NPs. Moreover, the degradation efficiency ( η ) of ZnO NPs (biosynthesized and sol-gel synthesized) with respect to time is illustrated in figure 13 . The degradation of MB for sol-gel synthesized ZnO are 35.3%, 45.7%, 56.1% 62.4%, 68.9% at 40, 80, 120, 160 and 200 min respectively. Again, the values for biosynthesized ZnO are 36.9%, 47.5%, 62.7%, 72.1%, and 80.2% at 40, 80, 120, 160 and 200 min respectively. So, MB dye degraded more rapidly in the presence of ZnO B NPs backing the reason for smaller particle sizes than that of ZnO A NPs. As the particles become smaller, the active surface area for the photocatalysis increased which results in enhanced degradation of MB [ 26 ]. Moreover, there remain terpenoids in the neem leaf extract which stabilizes the nanoparticles by capping themselves which also causes in the increment of photocatalytic action [ 27 ].
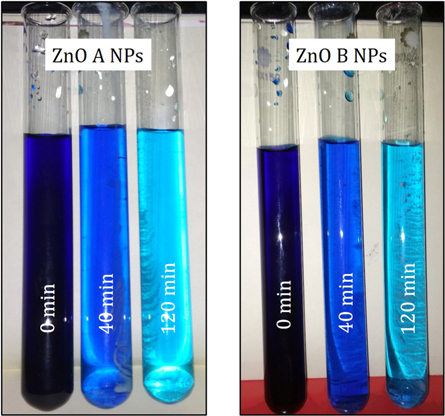
Figure 11. Visual inspection of the degradation phenomenon of MB dye by ZnO NPs.
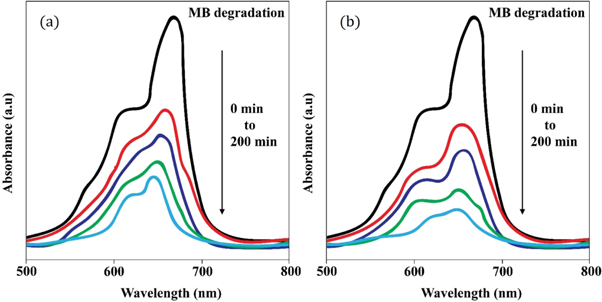
Figure 12. Absorption spectrum of (a) ZnO A NPs and (b) ZnO B NPs as a function of wavelength at 0, 40, 80, 120, 160, 200 min.
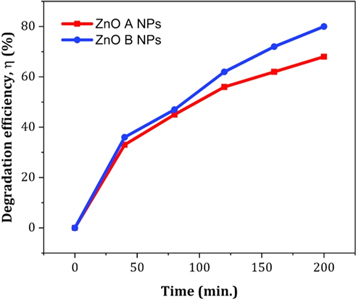
Figure 13. The degradation efficiency of ZnO NPs for methylene blue dye with respect to time.
3.6. Optical analysis
Figures 14 (a) and (b) displays the room temperature absorption spectrum of ZnO nanoparticles fabricated by sol-gel and biosynthesis methods correspondingly. Here, the absorption wavelengths are remaining within the maximum allowable limit of the absorption band of bulk ZnO (∼373 nm). Although the absorption slightly increases up to a wavelength of 363 nm for ZnO A NPs, the maximum incremental value for ZnO B NPs is 356 nm. The slight shift of the absorption peak may be caused due to the variation of particle size and their configuration [ 28 ]. However, this phenomenon results in the presence of a wide range of particle size distribution of ZnO [ 29 ]. Moreover, the redshift of ZnO A NPs compared to ZnO B NPs corresponds to the formation of agglomeration in the specimens significantly. Furthermore, in accordance with Gunanlan Sangeetha et al the shifting of absorption band to the higher wavelength as well as higher energy was associated with the increment of the size of nanoparticles [ 30 ]. Moreover, considering the direct interband transition between the valence band and the conduction band, the absorption band gap energy was measured by adopting the following Tauc's formula [ 31 ]:
Where A is an energy-independent constant, α is the absorption coefficient, h υ is for the photon energy, and E g is the optical band gap energy. The E g of the ZnO NPs was obtained from the ( α h υ ) 2 versus h υ plot (as shown in the inset of figures 14 (a) and (b). Where the extrapolation of the linear segment of the graph to (α h υ ) 2 = 0 provides the value of E g for ZnO NPs. It is observed that the optical band gap energy of ZnO B NPs (3.25 eV) is higher than that of ZnO A NPs (3.23 eV). This incremental phenomenon is mainly attributed to the quantum confinement effect. According to this theory, as the particle size decreases, the electrons in the valence band and the holes in the conduction band confine themselves within a space having a dimension of the de Broglie wavelength. However, this confinement influences the quantization of the energy and the momentum of the corresponding carriers and also enhances the optical transition energy between the valence band and the conduction band resulting in a broad band gap [ 32 ].
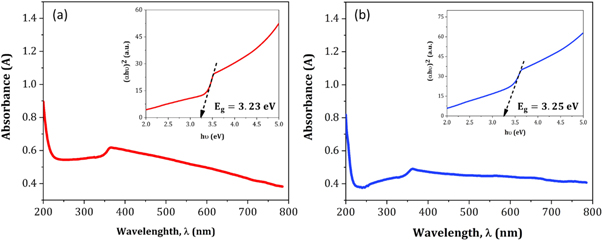
Figure 14. Absorption spectra of (a) ZnO A NPs and (b) ZnO B NPs (inset shows ( α h υ ) 2 versus h υ plot for the determination of band gap energy.
Figure 15 displays the UV visible transmittance spectrum of ZnO A NPs and ZnO B NPs. Here, the transparency of ZnO B NPs is greater than that of ZnO due to the reduced particle size of ZnO B NPs. From the research of Takuya Tsuzuki, it is clear that smaller particles are capable to show higher transparency at the visible range of spectrum [ 33 ]. However, the UV blocking characteristics are almost similar for each of the variants of NPs.
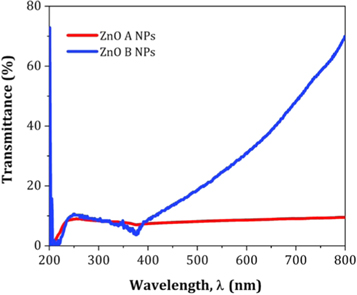
Figure 15. Typical transmittance spectra of ZnO NPs.
4. Conclusion
In summary, ZnO NPs were synthesized by two different methods i.e., sol-gel and biosynthesis method. The green synthesis of ZnO NPs allows avoiding the toxic chemical agents that are used in the sol-gel method for the size reduction. However, the Neem leaf extract possesses some phytochemicals which not only performs in the reduction of the particle sizes but also provide sufficient stabilization. Although, the average particle size of ZnO B NPs (25.97 nm) was smaller than that of ZnO A NPs (33.20 nm), the optical band gap energy of ZnO B NPs was higher than that of ZnO A NPs due to the quantum confinement effect. In addition, the antibacterial and photocatalytic properties of ZnO B NPs were greater than that of ZnO A NPs. Where, the zone of inhibition of bacterial growth for ZnO B NPs was 14.5 mm and for ZnO A NPs, it was 9.3 mm. Moreover, the degradation efficiency of ZnO B NPs at 200 min was 80% while for ZnO A NPs, the corresponding efficiency was 68%. Again, from the ESR analysis, it was proved that the OH · radicals were the main contributing factor for the degradation of MB dye. So, based on the comparison between the properties of the two variants, it is concluded that the biosynthesis method shows more effectiveness than the sol-gel method for the synthesis of ZnO NPs.
Acknowledgments
The authors are grateful to Rajshahi University of Engineering & Technology (RUET) for providing the opportunity to perform various tests. Special thanks go to Tasmia Zaman, Assistant Professor, Department of Glass & Ceramic Engineering, Rajshahi University of Engineering & Technology, Bangladesh for her cordial assistance.

An official website of the United States government
The .gov means it’s official. Federal government websites often end in .gov or .mil. Before sharing sensitive information, make sure you’re on a federal government site.
The site is secure. The https:// ensures that you are connecting to the official website and that any information you provide is encrypted and transmitted securely.
- Publications
- Account settings
Preview improvements coming to the PMC website in October 2024. Learn More or Try it out now .
- Advanced Search
- Journal List
- Antioxidants (Basel)

Green Synthesis and Characterization of ZnO Nanoparticles Using Pelargonium odoratissimum (L.) Aqueous Leaf Extract and Their Antioxidant, Antibacterial and Anti-inflammatory Activities
Ahmed s. abdelbaky.
1 Department of Biochemistry, Faculty of Agriculture, Fayoum University, Fayoum 63514, Egypt
Taia A. Abd El-Mageed
2 Department of Soil and Water, Faculty of Agriculture, Fayoum University, Fayoum 63514, Egypt; ge.ude.muoyaf@00aat
Ahmad O. Babalghith
3 Department of Medical Genetics, College of Medicine, Umm Al-Qura University, P.O. Box 57543, Makkah 21955, Saudi Arabia; as.ude.uqu@htihglababoa
4 Department of Clinical Laboratory Sciences, College of Applied Medical Sciences, Jouf University, Sakaka 72388, Saudi Arabia; as.ude.uj@malasludbas
Abir M. H. A. Mohamed
5 Department of Agricultural Microbiology, Faculty of Agriculture, Fayoum University, Fayoum 63514, Egypt; ge.ude.muoyaf@50hma
Associated Data
The data presented are included within the article.
Nanoparticles (NPs) exhibit distinct features compared to traditional physico-chemical synthesis and they have many applications in a wide range of fields of life sciences such as surface coating agents, catalysts, food packaging, corrosion protection, environmental remediation, electronics, biomedical and antimicrobial. Green-synthesized metal NPs, mainly from plant sources, have gained a lot of attention due to their intrinsic characteristics like eco-friendliness, rapidity and cost-effectiveness. In this study, zinc oxide (ZnO) NPs have been synthesized employing an aqueous leaf extract of Pelargonium odoratissimum (L.) as a reducing agent; subsequently, the biosynthesized ZnO NPs were characterized by ultraviolet-visible spectroscopy (UV-Vis), dynamic light scattering (DLS), Fourier transform infrared (FTIR) spectroscopy, X-ray diffraction (XRD), field emission scanning electron microscopy (FESEM) and energy-dispersive X-ray spectroscopy (EDX), high-resolution transmission electron microscopy (HRTEM) and selected area electron diffraction (SAED). Moreover, aqueous plant leaf extract was subjected to both qualitative and quantitative analysis. Antioxidant activity of ZnO NPs was assessed by DPPH assay, with varying concentrations of ZnO NPs, which revealed scavenging activity with IC 50 = 28.11 μg mL −1 . Furthermore, the anti-bacterial efficacy of the green synthesized ZnO NPs against four foodborne pathogenic bacterial strains was examined using the disk diffusion assay, and Staphylococcus aureus (ATCC 8095), Pseudomonas aeruginosa (ATCC10662) and Escherichia coli (ATCC 25922) were found to be the most sensitive against biosynthesized ZnO NPs, whereas the least sensitivity was shown by Bacillus cereus (ATCC 13753). The anti-inflammatory effect was also evaluated for both ZnO NPs and the aqueous leaf extract of P. odoratissimum through the human red blood cells (HRBC) membrane stabilization method (MSM) in vitro models which includes hypotonicity-induced hemolysis. A maximum membrane stabilization of ZnO NPs was found to be 95.6% at a dose of 1000 μg mL −1 compared with the standard indomethacin. The results demonstrated that leaf extract of P. odoratissimum is suitable for synthesizing ZnO NPs, with antioxidant, antibacterial as well as superior anti-inflammatory activity by improving the membrane stability of lysosome cells, which have physiological properties similar to erythrocyte membrane cells and have no hemolytic activity. Overall, this study provides biosynthesized ZnO NPs that can be used as a safe alternative to synthetic substances as well as a potential candidate for antioxidants, antibacterial and anti-inflammatory uses in the biomedical and pharmaceutical industries.
1. Introduction
Nanotechnology is one of the most quickly evolving fields, potentially forming and underpinning a wide range of technological and biotechnological advancements; as a result, it is seen as the century’s oncoming industrial revolution [ 1 ]. Nanotechnology has been used in different industrial and academic areas, including chemistry, agriculture, biology, medicine, electronics, information technology and physics [ 2 , 3 , 4 ]. Nanomaterials possess great potential in various fields of science due to their excellent physico-chemical and biological characteristics over bulk materials [ 5 ]. Nanoparticles (NPs) have the unique property of having a high surface-to-volume ratio [ 6 ], which means that they are more appropriate candidates for application-oriented performance (e.g., photocatalysis, cosmetics, gas sensing, energy reservoirs, electronics, packaging and environmental remediation) and encourages their incorporation into a wide range of commercial products, biotechnology and biomedical applications [ 7 , 8 , 9 , 10 , 11 , 12 , 13 ].
Among the large variety of NPs available, metal oxide (MO) NPs are thought to be the most promising because they have distinctive physical, chemical, and biological properties like solubility, chemical stability, and adhesiveness [ 8 ]. Additionally, the utilization of harmful compounds for reduction and as a capping agent in the nanoparticle synthesis process causes a variety of adverse effects on the flora life as well as the environment and the living system toxicity. As a result, plant extracts (PEs) are therefore a more promising tool for the easy synthesis of MO NPs through the green route, because this approach is eco-friendly, non-toxic, low cost, environmentally compatible and easy to apply. Additionally, the resultant particles are biocompatible and free of toxic stabilizers compared to classical chemicals. Basically, PEs contain a variety of active biomolecules that aid to reduce and stabilize NPs [ 6 , 12 ].
Zinc oxide (ZnO) is one of the very promising inorganic oxides that has recently attracted the attention of many scientists for the biosynthesis of NPs due to its unique properties and multiple applications such as drug delivery, solar cells, photocatalytic degradation and personal care products like sunscreens and cosmetics [ 14 , 15 , 16 , 17 , 18 , 19 ]. Based on earlier reports in the literature, ZnO NPs have been biosynthesized from several plant extracts such as Cassia auriculata [ 20 ], Aloe vera [ 13 , 21 ], Duranta erecta [ 22 ], Cinnamomum verum [ 23 ], Bauhinia tomentosa [ 24 ], Vitex trifolia [ 25 ], Moringa oleifera [ 26 ], Azadirachta indica [ 27 , 28 ], Artocarpus gomezianus [ 29 ] and Olea europaea [ 30 ]. In biological systems, the overproduction of highly reactive radical species (HRRS) causes oxidative stress, which has been observed in several diseases, i.e., cancer, diabetes, cardiovascular disease, and arthritis [ 31 ]. All biosystems depend heavily on antioxidants to function correctly. As a result, there is an urgent need to search for innovative and safe antioxidants produced from natural sources, which are more effective and less toxic. Additionally, the widespread use of antibacterial and anti-inflammatory drugs has caused resistance, the appearance of new pathogenic strains resistant to antibiotics [ 32 ] and chronic and acute toxicities in several human physiological systems, particularly the immune system. As a result, searching for new, effective antibacterial and anti-inflammatory drugs that can effectively combat drug-resistant bacteria is necessary and does not cause immunosuppression. Biosynthesized NPs have been proposed as an alternate potential approach to address these problems [ 33 ]. Pelargonium odoratissimum (L.) aqueous leaf extract (ALE) was utilized in the present study, for the biosynthesis of ZnO NPs as this is the first report on the use of this plant’s leaves for the green synthesis of NPs. Pelargonium odoratissimum (L.) known as “Apple Geranium” is a perennial and relatively flat-growing shrublet that belongs to the family Geraniaceae, very commonly grown locally in Egypt and is widely utilized for its health benefits [ 34 ]. Essential oils of Pelargonium spp. are in considerable demand in the pharmaceutical, perfumery, and cosmetic industries. Additionally, some reports revealed that essential oils obtained from a variety of Pelargonium spp. possess excellent antioxidant, antibacterial and antifungal properties [ 35 , 36 , 37 , 38 ]
The aerial parts of this Pelargonium spp. are used in traditional medicine for the treatment of wound healing, debility, gastrointestinal disorders (i.e., diarrhea and dysentery), hemorrhage, skin complaints, neuralgia and throat infections due to their various phytochemical constituents such as phenolics, flavonoids, terpenes, saponins and essential oils [ 39 ], which can contribute to their biological activities and facilitate the biosynthesis of NPs by employing them as reducing, capping and stabilizing agents.
Despite the widespread use of Pelargonium species as therapeutic agents, to date, there have been no data on their use for green synthesis of NPs, antioxidant, antibacterial and anti-inflammatory effects from Pelargonium odoratissimum leaf extract.
The aim of this study was to explore the application of P. odoratissimum ALE as a capping and reducing agent for the biosynthesis of ZnO NPs. The biosynthesized ZnO NPs were characterized and confirmed by various spectroscopic and microscopic techniques, i.e., UV-Vis spectroscopy, FTIR, XRD, DLS, HR-TEM, FE-SEM and EDX, in addition, to evaluate the antioxidant effects, as well as the antibacterial activities against some food-borne pathogens strains beside evaluating the anti-inflammatory activities of both ZnO NPs and the ALE of P. odoratissimum .
2. Materials and Methods
2.1. chemicals.
Gallic acid, rutin, 1,1-diphenyl-2-picrylhydrazyl (DPPH, ≥99%), Folin–Ciocalteu’s reagent, L-ascorbic acid (Sigma-Aldrich, St. Louis, MO 63103, USA), aluminum chloride anhydrous (Fluka, Buchs, Switzerland), sodium carbonate (>99%), zinc acetate dihydrate (Advent Chembio PVT. LTD, Mumbai, India), Luria-Bertani (LB) broth medium (Himedia, Mumbai, India) gentamycin (Tody Laboratories Int., 22nd Vadul Moldovei Street, Bucharest, Romania). All chemicals used in this study were of analytical grade.
2.2. Plant Collection and Processing
Fresh leaves of P. odoratissimum (L.) were collected from the Botanical Garden of Fayoum University, Fayoum, Egypt, in March 2021. The taxonomic identification of the plant was identified by Mrs. Therese Labib, Head of the Taxonomy specialists at El-Orman Botanical Garden, Cairo, Egypt. A voucher specimen with number 126 was deposited in the herbarium of the Biochem. Dept. Fac. Agric., Fym. Univ., Fym., Egypt. The leaves were completely air dried in the shade before being ground into a fine powder in a lab mill and sieved using a 24 mesh sieve. The powdered leaves were maintained in an air-tight container at room temperature (28 ± 2 °C) and kept away from light until use.
2.3. Preparation of P. odoratissimum Leaf Extract
The air-dried powder (20 g) of P. odoratissimum leaves was taken and immersed in 400 mL of deionized water (dH 2 O). The extraction process was performed via the ultrasonic-assisted solvent extraction (UASE) method [ 40 ] by placing the conical flask in a Probe Sonicator homogenizer (Benchmark Scientific, USA, 150 W, 25 kHz) at room temperature (35 ± 2 °C) for 30 min. The solvent (d.H 2 O) and powder layer were filtered using muslin cloth first and then Whatman filter paper No.1. The filtrate solution of P. odoratissimum leaf extract was kept in a refrigerator to be utilized for further use.
2.4. Qualitative Phytochemical Screening
The detection of various phytoconstituents present in the ALE of P. odoratissimum was carried out using the standard phytochemical methods [ 41 , 42 , 43 ].
2.5. HPLC-Analysis
The HPLC analysis was carried out using an Agilent 1260 series. The separation was performed using Eclipse C18 column (4.6 mm × 250 mm i.d., 5 μm). The mobile phase consisted of water (A) and 0.05% trifluoroacetic acid (TFA) in acetonitrile (B) at a flow rate of 0.9 mL/min. The mobile phase was programmed consecutively in a linear gradient as follows: 0 min (82% A); 0–5 min (80% A); 5–8 min (60% A); 8–12 min (60% A); 12–15 min (82% A); 15–16 min (82% A) and 16–20 (82%A). The multi-wavelength detector was monitored at 280 nm. The injection volume was 5 μL for each of the sample solutions. The column temperature was maintained at 40 °C.
2.6. Estimation of Total Phenolic and Flavonoid Contents (TPC and TFC)
The determination of both TPC as mg gallic acid equivalents (GAE) mg GAE/g plant extract) and TFC as mg rutin equivalents (RE)/g plant extract were performed spectrophotometrically by the Folin-Ciocalteu reagent [ 44 ] and aluminum chloride methods [ 45 ] respectively.
2.7. Green Synthesis of ZnO Nanoparticles
After heating twenty milliliters of P. odoratissimum leaf extract at 50 °C for 10 min, fifty milliliters of 0.1 M zinc acetate dihydrate (Zn(CH 3 COO) 2 ·2H 2 O) (1.095 g of zinc acetate dihydrate was dissolved in 50 mL of d.H 2 O) was added drop-by-drop to it under stirring at 800 rpm that resulted in cream-colored zinc hydroxide precipitate formation. For the complete reduction in zinc hydroxide, the reaction mixture was left for 30 min. Then the precipitate was centrifuged (Sigma Laborzentrifugen 2k15, Osterode, Germany) at 16,000 rpm for 10 min at 4 °C by dH 2 O followed by ethanol repeatedly in order to remove the impurities. The precipitate was dried overnight in an oven at 100 °C. The obtained dried powder was calcined in a muffle furnace at 600 °C for 2 h and the white powder of ZnO NPs was obtained after calcination as shown in Figure 1 . The resulted powder was used for characterization.

Represent (pictorial) the synthesis of ZnO NPs via P. odoratissimum ALE.
2.8. Characterization Methods of ZnO NPs
2.8.1. uv-vis spectroscopy.
In order to study the optical characteristics of green synthesized ZnO NPs, a known amount of ZnO NPs (0.05 g) was dispersed in 5 mL of ethanol (96%). The absorption spectrum was recorded by using a UV-Vis (U-2900) double beam spectrophotometer (Hitachi, Tokyo, Japan) in between a wavelength scan of 200–800 nm.
2.8.2. Dynamic Light Scattering (DLS)
A particle size analyzer (Zetasizer V 2.2, Worcestershire, Malvern, UK) was utilized to determine the particle size distribution (PSD) of ZnO NPs obtained using ALE. The zeta potential of ZnO NPs was carried out in the water as a dispersant through a Zeta sizer (V 2.3, Worcestershire, Malvern, UK) to identify the stability of the synthesized NPs.
2.8.3. Fourier Transform Infra-Red Spectroscopy (FTIR)
FTIR analysis (Bruker, Berlin, Germany) was employed to identify the functional groups (FGs) involved in biosynthesized ZnO NPs. At a wavelength of 4000–400 cm −1 , the FTIR spectra were scanned with a resolution of 4.0 cm −1 .
2.8.4. X-ray Diffraction (XRD)
The crystalline structure of ZnO NPs was analyzed by an X-ray diffractometer (Bruker D8 DISCOVER, Bruker, Germany) with Cu-Kα radiation (λ = 1.54060 Angstrom). The relative intensity data were collected over a 2θ range of 5°–80°, 2θ values and relative intensities (I/Io) were determined from the chart, and the minerals of core materials were identified with JCPDS carts.
2.8.5. Field Emission-Scanning Electron Microscopy (FE-SEM)
The topography and surface morphology of the biosynthesized ZnO NPs were examined using FE-SEM (Carl- ZEISS Sigma 500 VP, Sigma, Osterode, Germany) equipped with an energy dispersive X-ray spectrometer (EDX, Bruker, Germany) for the element composition present in the powder of ZnO NPs. A portion of the sample was set on a carbon-coated copper (CCC) grid, and the film on the FE-SEM grid was then dried by fixing it under gold for 5 min.
2.8.6. High-Resolution Transmission Electron Microscopy (HRTEM)
The shape and size distribution of powdered ZnO NPs were studied by using HRTEM (JEM-2100, JEOL, Tokyo, Japan) at an accelerated voltage of 200 kV.
2.9. Estimation of Antioxidant Activity—DPPH Radical Scavenging Activity
The ability to scavenge the free radical DPPH of the ALE of P. odoratissimum , biosynthesized ZnO NPs and standard L-ascorbic acid at different concentrations ranging from 3125–100 μg mL −1 were performed using the Brand-Williams et al. method [ 46 ]. Briefly, 2 mL of the DPPH solution (Sigma-Aldrich, 3050 Spruce Street, St. Louis, MO 63103, USA) (25 mg L −1 in methanol) was added to 0.1 mL of different concentrations of each sample and standard L-ascorbic acid (3125–100 μg mL −1 ). After shaking vigorously for 1 min, the reaction mixture was maintained in the dark for 30 min at room temperature (35 ± 2 °C) and the absorbance was recorded at 517 nm using the U-2900 UV-Vis double beam spectrophotometer (Hitachi, Tokyo, Japan). Each measurement was taken in three replications. The free radical scavenging activity (FRSA) of each sample was expressed as percent inhibition of DPPH free radical and was calculated as:
% inhibition (% Anti-radical activity) = [(A control − A sample )/A control ] × 100, where A is the absorbance. The IC 50 values were measured from the relationship curve of FRSA versus concentrations of the respective sample curve.
2.10. Estimation of Antibacterial Activity
2.10.1. bacteria strains.
The antibacterial effect of the biosynthesized ZnO NPs with P. odoratissimum ALE was established against two Gram-positive bacteria (GPB), Bacillus cereus (ATCC13753) and Staphylococcus aureus (ATCC8095), and two Gram-negative bacteria (GNB), Escherichia coli (ATCC25922) and Pseudomonas aeruginosa (ATCC10662). These four strains were acquired from the Microbiol. Dept., Fac. Agric., Fym. Univ., Egypt. The bacterial strains used were maintained in the Luria–Bertani (LB) agar at 30 °C for 24 h and then kept at 4 °C in a refrigerator. During this study, LB media was used for all bacterial cultures.
2.10.2. Antibacterial Assay
The antibacterial effect against the examined bacterial strains was determined using the agar disc diffusion method (ADDM) described by Bauer et al. [ 47 ]. In this method, three different ZnO NPs concentrations (10, 20 and 30 μg mL −1 ) and ALE (20 μg mL −1 ) were dissolved in ethanol and then used to fill sterilized Whatman filter paper discs of approximately 40 μL with the proper volume containing the tested ZnO NPs concentrations and ALE and left to totally dry. A disc containing only solvent was used as a negative control and a disc containing zinc acetate dihydrate was employed. A positive control gentamicin (10 μg mL −1 ) was used. Overnight bacterial cultures were prepared in LB broth for obtaining tested bacterial suspensions for the assay. The discs were then placed on the plates having the tested bacterial cultures and diluted to obtain about 1 × 10 −7 colony-forming unit (CFU). The inoculated plates were incubated at 37 °C for 24 h and then the activity was assayed by measuring the inhibition diameter in millimeters (mm). All tests were performed in triplicate.
2.11. Estimation of Anti-inflammatory Activity
The human red blood cells (HRBCs)-membrane stabilization method (HRBCs-MSM) has been performed for the evaluation of in vitro anti-inflammatory activity according to the procedure outlined by Anosike et al. [ 48 ].
2.12. Statistical Analysis
All of the tests (antioxidant, antibacterial, and anti-inflammatory activity) were performed in triplicates, with the results provided as mean ± SD. Using the statistical software SPSS (SPSS version 21, IBM Corporation, Armonk, NY, USA), the statistical data were examined using the two-way ANOVA technique. The difference in significance was calculated at p < 0.05.
3. Results and Discussion
3.1. qualitative phytochemical screening (qps).
The results of the QPS of P. odoratissimum ALE are summarized in ( Table 1 ), which displays the existence of saponins, phenolics and tannins, flavonoids, carbohydrates and/or glycosides and the absence of steroids, triterpenoids and alkaloids. These present compounds could be responsible for the bio-reduction of the metal salts into nanosize particles [ 49 ].
Qualitative phytochemical screening of P. odoratissimum ALE.
(+): present; (−): absent.
3.2. HPLC-Analysis
HPLC analysis of ALE indicates the presence of sixteen phenolic compounds in appropriate amounts: Gallic acid, Chlorogenic acid, Catechin, Methyl gallate, Caffeic acid, Syringic acid, Rutin, Ellagic acid, Coumaric acid, Ferulic acid, Naringenin, Daidzein, Quercetin, Cinnamic acid, Apigenin and Kaempferol ( Table 2 and Figure 2 , respectively), that may be responsible for the bio-reduction of the metal salts into ZnO-nanosize particles. Additionally, Gallic acid, Syringic acid, Chlorogenic acid, Ferulic acid, Naringenin, Ellagic acid, Rutin and Coumaric acid were found to be highly prevalent among several significant phenolic components identified. Both phenolic acids and flavonoids are known to be potent hydrogen donors [ 50 ], which are responsible for a variety of biological activities because of their functional (carboxyl and hydroxyl) groups. The amounts (µg/g) and structures of polyphenols are illustrated in Table 2 and Figure 3 , respectively.

HPLC chromatogram: ( a ) standard polyphenolic compounds; ( b ) ALE of P. odoratissimum .

Chemical structures of polyphenolic compounds present in P. odoratissimum ALE.
Polyphenolic compounds of P. odoratissimum ALE.
3.3. Characterization of ZnO NPs
3.3.1. visual observation.
The first essential indicator that confirms the biosynthesis of ZnO NPs is visual observation. When the Zn(CH 3 COO) 2 ·2H 2 O, as a precursor for ZnO NPs, was added to the P. odoratissimum leaf extract, the color of the P. odoratissimum leaf extract was changed from light red to cream-colored precipitate ( Figure 4 ). Similar color changes of synthesized ZnO NPs employing Hibiscus subdariffa leaf extract, from light red to cream-colored precipitate, were displayed by Bala et al. [ 16 ], confirming the biosynthesis of ZnO NPs.

The visual observation of colour changes at 0 time ( a ) ( P. odoratissimum ALE) and after 30 min ( b ) ( P. odoratissimum ALE and (Zn(CH 3 COO) 2 ·2H 2 O)).
3.3.2. UV-Vis Spectroscopy
To confirm the synthesis of ZnO NPs, UV/Vis spectrophotometry was performed in order to examine the optical characteristics of green synthesized ZnO NPs using P. odoratissimum ALE. The UV-Vis spectrum recorded the maximum absorbance peak at 370 nm as shown in Figure 5 , which verified the synthesis of ZnO NPs via P. odoratissimum ALE, which is consistent with earlier studies by Senthilkumar et al. [ 51 ], who examined the ability of Tecona grandis (L.) ALE to synthesize ZnO NPs with surface plasmon resonance (SPR) at 370 nm. Additionally, there are no other peaks recorded in the spectrum which means that the biosynthesized ZnO NPs are a pure product. Furthermore, the high absorption band seen at 378 nm might be attributed to ZnO’s inherent band-gap absorption caused by electron transitions from the valence band (E V ) to the conduction band (E C ) (O 2p –Zn 3d ) [ 52 , 53 ]. The formula for calculating the energy bandgap (E G ) of ZnO NPs was used as follows:

UV/Vis spectrum of ZnO NPs biosynthesized using P. odoratissimum ALE.
Where h is Planck’s constant (6.626 × 10 − 34 Js), c is the velocity of light (3 × 10 8 m/s) and λ is the wavelength (378 nm). In total, 3.28 eV was found to be the bandgap energy of ZnO. The significant UV absorption of ZnO NPs demonstrates the product’s suitability for a variety of medicinal applications, including sun-screen protectors and antibacterial ointments [ 54 ].
3.3.3. Dynamic Light Scattering (DLS)
The Z-average diameter (nm) and PSD of the biosynthesized ZnO NPs were measured using the DLS technique. As shown in Figure 6 A, the measurements demonstrated that the average size (nm) of the ZnO NPs with P. odoratissimum ALE was about 76 nm. The result obtained from the PSD profile of the ZnO nanoparticles revealed two notable peaks with intensities of 98.7% and 1.3%. Additionally, the ZnO NPs have a polydispersity index (PDI) of 0.241. This indicated that ZnO nanoparticles are very homogeneous and have a uniform size range [ 55 ]. This finding is completely compatible with Badran, Chen et al. and Putri et al. [ 56 , 57 , 58 ] who reported that PDI values of 0.3 and below are considered to be monodisperse. Because of the hydrodynamical shell, the DLS technique is known to produce significantly higher values than HRTEM size analyses. Additionally, the size of the hydrodynamical shell is influenced by particle structure, particle shape, and roughness [ 59 ].

PSD ( A ) and ZP ( B ) of green synthesized P. odoratissimum -ZnO nanoparticles.
The surface charges and stability of biosynthesized ZnO NPs have been assessed through zeta potential (ZP) analysis. The ZP graph of ZnO nanoparticles is presented in ( Figure 6 B). As shown in Figure 6 B, the ZP was found to be −19.3 mV which indicates the potential stability of the examined NPs [ 51 ]. As a result, the reducing agents (i.e., phenolic and flavonoid components) found in the leaf extract (LE) are probably responsible for the negative charge potential of the produced ZnO NPs. It also confirms that the produced substance contains substantial electrostatic forces [ 60 ].
3.3.4. FTIR Analysis of Biosynthesized ZnO NPs and P. odoratissimum ALE
The FTIR technique was used in order to detect possible FGs present in the ALE of P. odoratissimum that contribute to the reduction in and stabilization of ZnO NPs. Figure 7 a,b represents the FTIR spectra of biosynthesized ZnO nanoparticles and P. odoratissimum leaf extract. The peaks of P. odoratissimum ALE and biosynthesized ZnO nanoparticles are displayed in Table 3 . The broad stretch peak at 3409 cm − 1 and 3417 cm − 1 indicates the presence of an O-H stretch band for the extract and ZnO NPs which are corresponded to the O-H stretching of alcohol, phenolic and flavonoid constituents [ 61 , 62 ]. The low-intensity peaks that arise at 2923 cm − 1 and 2920 cm − 1 were assigned to –CH stretching vibration of the hydroxyl compounds [ 63 , 64 ]. The absorption peaks at 2356 cm − 1 and 2356 cm − 1 were ascribed to O=C=O (stretching vibration) [ 65 ]. The peaks observed at 1616 cm − 1 and 1621 cm − 1 indicate the stretching C=C vibration of the aromatic ring system [ 66 , 67 ]. The absorption peaks at 1400 cm − 1 and 1403 cm − 1 correspond to the C-N stretching vibration of amino acids [ 63 ]. The strong intensity peaks at 1068 cm − 1 and 1072 cm − 1 are due to the C-O stretching bond of the aromatic rings [ 67 ] and may also be related to phenols and flavonoids found in the P. odoratissimum ALE in Table 1 . The bands at 852 cm − 1 and 855 cm − 1 are attributed to –CH stretching vibration of aromatics [ 64 ]. The absorption band observed at 435 cm − 1 confirmed the successful formation of Metal-Oxygen (ZnO). The ZnO absorption peak obtained by FTIR analysis of biosynthesized ZnO NPs has been detected at wavelengths 436 cm − 1 [ 51 ], 442 cm − 1 [ 68 ], 450 cm − 1 [ 69 ] and 485 cm − 1 [ 70 ], in the range 400 to 500 cm − 1 [ 71 ], which are consistent with our findings. The similarity of bands in both P. odoratissimum ALE and P. odoratissimum -synthesized ZnO NPs ( Table 3 ) could be attributable to capped biomolecules on the surface of green synthesized ZnO nanoparticles.

FTIR absorption spectra of ( a ) ZnO NPs and ( b ) ALE of P. odoratissimum .
FTIR spectra of biosynthesized ZnO NPs and P. odoratissimum ALE.
3.3.5. X-ray Diffraction (XRD) Analysis of ZnO NPs
The XRD pattern of biosynthesized ZnO NPs using ALE of P. odoratissimum is illustrated in Figure 8 . The XRD diffraction peaks existed at 2θ angles of 31.85°, 34.55°, 36.35°, 47.69°, 56.75°, 63.09°, 66.56°, 68.17°, 69.29°, 72.87° and 77.21° corresponding to lattice planes (100), (002), (101), (102), (110), (103), (200), (112), (201), (004) and (202), respectively [ 72 ]. These peaks are in accordance with those of (JCPDS card No: 36-1451), which is indicating the confirmation of the hexagonal wurtzite structure of ZnO NPs formation [ 73 ]. The average crystalline size (ACS) of biosynthesized ZnO NPs was calculated using Deby-Scherrer’s formula [ 74 ] and the ACS of the ZnO NPs was estimated to be 14 nm, which is derived from the full width at half maximum (FWHM) of the most intense peak corresponding to (101) plane located at 36.35°. Furthermore, the XRD pattern revealed no additional peaks other than the characteristic ZnO peaks, confirming the purity of the produced ZnO NPs. Additionally, the narrow and strong diffraction peak clearly indicates that the ZnO NPs have an optimal crystalline structure [ 75 , 76 ].

XRD pattern of biosynthesized ZnO NPs via P. odoratissimum L. ALE.
3.3.6. FE-SEM of ZnO NPs
The size and the morphology of the biosynthesized ZnO nanoparticles were imaged via FE-SEM ( Figure 9 ), and the chemical composition of the biosynthesized ZnO nanoparticles was determined using EDX ( Figure 10 ). The FE-SEM image demonstrated that the ZnO NPs were spherical and hexagonal in the morphology shape with good distribution. AN FE-SEM examination showed that the average size of ZnO NPs was 21.6 nm.

FE-SEM image of biosynthesized ZnO NPs.

EDX Spectrum of biosynthesized ZnO NPs.
3.3.7. Energy Dispersive X-ray Analysis (EDX) Spectrum of ZnO NPs
The elemental mapping of the EDX ( Figure 10 ) verified that the examined sample displayed the elemental peaks of zinc and oxygen which are summarized in Table 4 . The EDX analysis proved that the examined sample contained the biosynthesized ZnO NPs.
Elemental constituents of ZnO NPs.
3.3.8. HR-TEM of ZnO NPs
The high-resolution TEM analysis ( Figure 11 a–g) was carried out to confirm the formation of the biosynthesized ZnO NPs. Based on the results obtained, it can be concluded that the pure green ZnO NPs display hexagonal shapes with an average size of 34.12 nm ( Figure 11 i) and also clearly reveal lattice fringes without any distortion, indicating that ZnO NPs have high crystallinity. The selected area electron diffraction (SAED) ( Figure 11 h) pattern revealed a series of rings with bright spots, indicating that ZnO nanoparticles are crystalline in nature [ 74 , 76 ]. Additionally, the hexagonal wurtzite crystalline structure of ZnO NPs is also proven by the diffraction rings on the SAED image and the peaks in the XRD pattern.

( a – g ) HR-TEM images of biosynthesized ZnO NPs, ( h ) SAED pattern and ( i ) histogram of particle size distribution.
3.4. Antioxidant Activity
The antioxidant activity of ZnO NPs, the ALE of P. odoratissimum and L-ascorbic acid are shown in Figure 12 . The results obtained show the DPPH scavenging activity of ZnO NPs, ALE and L-ascorbic acid at six different concentrations (3.125 to 100 μg mL − 1 ) ranging from 10.78 to 76.14%, 23.05 to 89.92% and 14.70 to 83.02% respectively. The DPPH assay showed the scavenging effect of ZnO nanoparticles having an IC 50 value of 28.11 ± 0.01 μg mL − 1 when compared with the IC 50 value of L-ascorbic acid (11.50 ± 0.03 μg mL − 1 ) and aqueous extract (04.56 ± 0.02 μg mL − 1 ). Additionally, the aqueous extract revealed a superior antioxidant potential to traditional reference L-ascorbic acid, which could be due to various bioactive constituents and the higher content of phenolics and flavonoids present in the P. odoratissimum ALE. Moreover, the IC 50 value of P. odoratissimum ALE exhibited higher antioxidants than the aqueous extract of P. graveolens , which had an IC 50 value of 16.59 μg mL − 1 [ 77 ].

DPPH FRSA of ZnO NPs, ALE and L-ascorbic acid at different concentrations.
Generally, phenolic and flavonoid compounds are almost present in all plants in varying proportions and have been reported to act as bio-reductants of metallic ions in an aqueous medium and display a wide range of biological activities such as antioxidant and antimicrobial activity [ 78 ]. Many studies have specified that various OH groups’ presence in phenolic and flavonoids are responsible for the formation and stabilization of metal and metal oxide nanoparticles [ 79 , 80 , 81 ].
As presented in Table 5 , the total phenolic content (TPC) of P. odoratissimum ALE was found to be 21.93 ± 0.01 mg GAE/g of dried leaf extract, while the total flavonoid content (TFC) was recorded to be 17.11 ± 0.001 mg RE/g of dried leaf extract. From the above results, the ALE of P. odoratissimum possesses phytoconstituents that can be used in the formation, capping, stabilization and reduction of zinc acetate salt into ZnO NPs via the green route.
IC 50 , total phenolic (TP), and total flavonoid (TF) contents of P. odoratissimum ALE.
n.d. not determined; values expressed as mean of triplicates ± SD ( p < 0.05). The means of each column with the letters (a–c) differ significantly ( p < 0.05).
3.5. Antibacterial Activity
The antibacterial effect of the biosynthesized ZnO NPs was evaluated by disc diffusion assay against S. aureus (ATCC 8095), B. cereus (ATCC 13753) as GPB, and E. coli (ATCC 25922) and P. aeruginosa (ATCC10662) as GNB. The results are represented in Table 6 and Figure 13 . Generally, the results revealed that the biosynthesized ZnO NPs using P. odoratissimum ALE possessed a significant antibacterial effect against all tested bacterial strains. The significant antibacterial zone of inhibition was recorded in S. aureus (28 ± 0.35 mm) followed by B. cereus (24 ± 0.14 mm), P. aeruginosa (21 ± 0.28 mm) and E. coli (16 ± 0.21 mm). ALE does not observe any zone of inhibition in the tested bacterial strains. Furthermore, compared to gentamycin as a positive control and ALE of P. odoratissimum , biosynthesized ZnO NPs displayed higher antibacterial activity. The antibacterial activities of ZnO NPs differ depending on the cell wall nature of GPB or GNB [ 82 , 83 ]. In the present study, the biosynthesized ZnO NPs showed higher antibacterial activity against GPB ( S. aureus and B. cereus ) compared to GNB ( P. aeruginosa and E. coli ). A similar trend was obtained by Vijayakumar et al. [ 10 ] who stated that ZnO NPs synthesized from Laurus nobilis leaf extract displayed greater antibacterial activity against GPB ( S. aureus ) than GNB ( P. aeruginosa ). This is maybe owing to the structure and the components of GPB (i.e., peptidoglycan layer) and may improve the ZnO NPs’ attachment to the cell wall, while the components of GNP avoid this attachment [ 84 ].

Antibacterial effects (zone of inhibition (mm)) at different concentrations of ZnO NPs (A: 10 μg mL − 1 ; B: 20 μg mL − 1 ; C: 30 μg mL − 1 and D: standard) towards various pathogens.
Evaluation of the antibacterial activity toward pathogenic bacteria.
Values are means ( n = 3). According to LSD (as a post hoc test (PHT) at p ≤ 0.05), the means of ZnO NPs concentrations sharing different capital letters are significantly different. Interactions between each concentration and bacterial strains are indicated with different superscripted small letters and significantly differ according to LSD as a PHT at p ≤ 0.05.
Additionally, the results indicated that the inhibitory effect of biosynthesized ZnO NPs using P. odoratissimum leaf extract increased when the concentration of ZnO NPs was increased. This was in agreement with Gunalan et al. [ 85 ], who reported that increasing the concentration of ZnO NPs in discs and wells consistently increased the growth inhibition due to optimal NPs diffusion in the agar medium.
For the effect of ZnO NPs, there are some proposed bactericidal mechanisms ( Figure 14 ) that have been suggested by scientists. Some suggested that the released Zn from ZnO NPs possess toxic properties that are leading to inhibiting a lot of bacterial cell activities such as bacterial metabolism, and enzyme activity resulting in cell bacterial death [ 86 , 87 ]. The other suggested mechanism is the formation of reactive oxygen species (ROS) that activates oxidative stress which subsequently leads to cell death [ 88 , 89 ]. Another proposed mechanism is the lethal activity of the ZnO NPs due to the attachment of the NPs to the bacterial cell membranes, and the accumulation inside the cytoplasm resulting in damaging the cell membrane integrity and loss of cell contents because of the leakage ending up with cell death [ 90 ].

Various proposed mechanisms of ZnO NPs toxicity against bacteria [ 17 ].
3.6. Anti-inflammatory Activity
During times of inflammation, lysosomes lyse and release their component enzymes, resulting in a variety of disorders. Nonsteroidal anti-inflammatory drugs (NSAIDs) work by either blocking lysosomal enzyme release or stabilizing lysosomal membranes [ 91 ]. When RBCs are exposed to harmful substances such as hypotonic medium, heat, methyl salicylate (MeS) or phenylhydrazine (PhNHNH 2 ), the membranes lyse, resulting in hemolysis and hemoglobin oxidation [ 92 ]. Because the membranes of HRBCs are similar to those of lysosomes [ 91 ], the inhibition of hypotonicity-induced RBCs membrane lysis was used as a measure of the mechanism of the anti-inflammatory effect of ZnO NPs and P. odoratissimum ALE.
From the results obtained in Table 7 , the ZnO NPs and P. odoratissimum ALE have an anti-inflammatory effect that is concentration-dependent, with the percentage of protection increasing as the concentration of the samples increases. At the concentration of 1000 μg mL − 1 , the ZnO NPs significantly ( p ≤ 0.05) produced 95.60% inhibition of RBC hemolysis, and it was comparable to the results achieved with standard indomethacin ( Table 7 ). The hemolytic effect of the hypotonic solution is due to an excessive accumulation of fluid within the cell, which causes the cell membrane to rupture. Damage to the red cell membrane (RCM) increases the cell’s vulnerability to subsequent damage caused by free radical-induced lipid peroxidation [ 93 ]. During a time of increased permeability produced by inflammatory mediators, membrane stability prevents leaking the flow of serum protein and fluids into the tissues [ 94 ]. The ZnO NPs and ALE of P. odoratissimum maybe stabilized the RBC membrane by preventing the release of active mediators of inflammation and lytic enzymes. Furthermore, many studies have revealed that plant flavonoids have anti-inflammatory and antioxidant activity [ 95 , 96 , 97 ]. Their anti-inflammatory properties are thought to be owing to an inhibitory action on enzymes involved in the synthesis of the chemical mediators of inflammation and arachidonic acid metabolism [ 98 , 99 ].
Effect of the biosynthesized ZnO NPs and ALE of P. odoratissimum on hypotonicity-induced hemolysis of HRBCs.
Values are expressed as the mean of triplicates ± SD. Different superscripted small letters significantly differ based on LSD as a post hoc test at p ≤ 0.05.
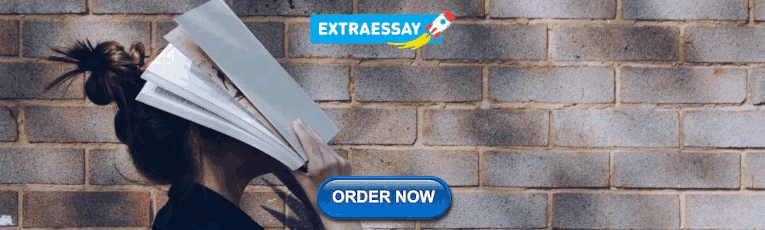
4. Conclusions
This study presents the biosynthesized ZnO NPs for the first time using an ALE of P. odoratissimum via a simple green route. The biosynthesized ZnO NPs showed a characteristic Uv-Vis absorption peak at 370 nm. The XRD pattern also indicated the hexagonal pure Wurtzite structure. FE-SEM coupled with EDX, HR-TEM, FTIR and DLS, confirmed the formation of NPs with an average size of 34.12 nm as obtained from HR-TEM analysis. The DPPH assay revealed that ZnO NPs possess antioxidant activity with an IC 50 value of 28.11 μg mL − 1 . Furthermore, ZnO NPs showed excellent antibacterial effects against both GNB and GPB. In addition, ZnO NPs were found to be more effective as anti-inflammatory via stabilizing the RBCs’ membrane in in vitro models. Our findings suggest the possibility of using the aqueous leaf extract of P. odoratissimum for synthesizing stable ZnO NPs. The biosynthesized ZnO NPs possess a significant antioxidant, antibacterial against foodborne pathogenic bacteria and anti-inflammatory activities that can be used as a safe and stable alternative to synthetic substances in the fields of pharmaceutical and biomedical research.
Funding Statement
This research received no external funding.
Author Contributions
Conceptualization, A.S.A. and A.M.H.A.M.; methodology, A.S.A. and A.M.H.A.M.; biosynthesis and all characterization, A.S.A. and A.M.H.A.M.; validation, A.S.A. and A.M.H.A.M.; data analyzation, A.S.A. and A.M.H.A.M., software.; A.S.A. and A.M.H.A.M., resources., A.S.A. and A.M.H.A.M., data interpretation., A.S.A. and A.M.H.A.M., writing—original draft preparation., A.S.A. and A.M.H.A.M., writing—review and editing., A.S.A., T.A.A.E.-M., A.O.B., S.S. and A.M.H.A.M., visualization, A.S.A. and A.M.H.A.M. All authors have read and agreed to the published version of the manuscript.
Institutional Review Board Statement
Not applicable.
Informed Consent Statement
Data availability statement, conflicts of interest.
The authors declare that they have no conflict of interest.
Publisher’s Note: MDPI stays neutral with regard to jurisdictional claims in published maps and institutional affiliations.
Thank you for visiting nature.com. You are using a browser version with limited support for CSS. To obtain the best experience, we recommend you use a more up to date browser (or turn off compatibility mode in Internet Explorer). In the meantime, to ensure continued support, we are displaying the site without styles and JavaScript.
- View all journals
- My Account Login
- Explore content
- About the journal
- Publish with us
- Sign up for alerts
- Open access
- Published: 03 June 2024
Aloe-inspired eco-friendly synthesis of Ag/ZnO heterostructures: boosting photocatalytic potential
- Nawal Ansar 1 ,
- Wajeehah Shahid 1 ,
- Muhammad Atif Irshad 2 ,
- Samiah Shahid 3 ,
- Rab Nawaz 2 , 4 ,
- Ali Irfan 5 ,
- Muhammad Iftikhar Khan 1 ,
- Aamal A. Al-Mutairi 6 ,
- Maria Khizar 1 ,
- Sami A. Al-Hussain 6 ,
- Sana Ullah 1 &
- Magdi E. A. Zaki 6
Scientific Reports volume 14 , Article number: 12711 ( 2024 ) Cite this article
66 Accesses
Metrics details
- Environmental sciences
- Materials science
- Nanoscience and technology
The current research focuses on the development of Ag–ZnO heterostructures through a “bottom-up” approach involving the assembly and extraction of Aloe barbadensis Miller gel. These heterostructures composed of metals/semiconductor oxide display distinct and notable optical, electrical, magnetic, and chemical properties that are not found in single constituents and also exhibit photocatalytic applications. These synthesized heterostructures were characterized by XRD, FTIR, SEM, and UV–visible spectroscopy. The high peak intensity of the Ag/ZnO composite shows the high crystallinity. The presence of Ag–O, Zn–O, and O–H bonding is verified using FTIR analysis. SEM analysis indicated the formation of spherical shapes of Ag/ZnO heterostructures. The Zn, O, and Ag elements are further confirmed by EDX analysis. Ag–ZnO heterostructures exhibited excellent photocatalytic activity and stability against the degradation of tubantin red 8BL dye under visible light irradiation.
Similar content being viewed by others
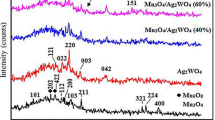
Rapid reduction of nitroarenes photocatalyzed by an innovative Mn3O4/α-Ag2WO4 nanoparticles
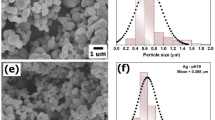
Synthesis of Ag3PO4/Ag4P2O7 by microwave-hydrothermal method for enhanced UV–visible photocatalytic performance
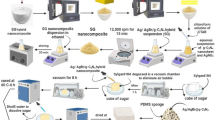
Fabricating a 3D floating porous PDMS − Ag/AgBr decorated g-C3N4 nanocomposite sponge as a re-usable visible light photocatalyst
Introduction.
Silver and zinc oxide nanostructures are the most widely used metallic and metallic oxide nanostructures. Zinc oxide nanostructures have gained significant attention in research due to their increased industrial potential as well as their high piezoelectric properties, large binding energy, and low toxicity. Additionally, owing to their exceptional anti-oxidant activity, zinc oxide nanostructures are among the most widespread inorganic nanostructures. Zinc oxide nanostructures, an n-type semiconductor with a direct band gap of 3.37 eV 1 , have been generally utilized in water decontamination photocatalysis owing to their notable photocatalytic features such as their physically and chemically stable structure, low affordability, biocompatibility, strong oxidizing ability, high photosensitivity, and high availability 2 . On the other hand, due to electron–hole recombination, which results in reduced active species on the photocatalyst surface, the wide band gap in ZnO led to non-optimal photocatalytic activity 3 . Therefore, to improve ZnO photocatalytic activity, it is essential to prevent electron–hole recombination through appropriate modifications. Additionally, they offer potential for environmental and biological applications because of their non-toxic nature. ZnO nanostructures are widely used in several applicable areas, including energy storage 4 , dye-sensitized solar cells 5 , nanosensors 6 , optoelectronic devices 7 , nano-electronics 8 , gas sensors 9 , photocatalysts 10 , spintronics 11 , and biomedical treatment 12 . ZnO nanostructures have been employed as semiconductors in the fabrication of microelectronics and pollutant degradation. ZnO nanostructures are also suitable for UV screening applications due to their greater chemical stability and low toxicity 13 .
Silver nanostructures are attracting much interest due to their carrier properties, which include anti-microbial 14 , anti-inflammatory 15 , anticancer drugs 16 , and antioxidant activities 17 . Unique biological, chemical, and physical properties of silver nanostructures among metallic nanostructures are advantageous, reserving their potentiality in industrial applications. Ag nanostructures have an exceptional wide-spectrum of anti-fungal, anti-bacterial, and anti-oxidant activities that are commonly used in several electronic devices such as washing machines, televisions, and refrigerators, as well as for water and air purification, food packaging, clothing, cosmetics, and medicine 18 , 19 , 20 . Moreover, the morphological features of synthesized Ag nanostructures are significantly affected by their anti-oxidant activity 21 , 22 . In order to exploit the anti-oxidant activity in the fabrication of innovative products, a variety of plant extracts have been utilized to fabricate Ag nanostructures in various shapes and sizes 23 . Ag nanostructures, including those treated with plant extracts, have gained considerable attention, especially owing to their well-documented anti-oxidant and anti-bacterial activities 24 , 25 , 26 , 27 .
Aloe vera is systematically identified as Aloe barbadensis Miller. The Liliaceae family includes Aloe vera 28 . The succulent plant Aloe vera , which has thorns on its branches and waxy coatings, grows readily in dry environments 29 , 30 . Three layers comprise Aloe vera leaf: the exterior portion, which is dense and protective, includes a pretty good amount of cellulose; the central portion is composed of primary flavanone (aloin A and B); and the interior portion contains a fresh gel that is made of sugars, vitamins (A, B, C, and E), proteins, anthraquinones, amino acids, and acetylated glucomannan 31 , 32 , 33 . Aloe vera leaf consists of latex, gel, and rind. Since ancient times, Aloe vera gel has been widely employed as a traditional medicine to heal different skin ailments, inflammations, and minor burns, as well as as a source of vitamins and minerals 34 . The green rind is a consequence of gel extraction, which involves strong active substances that can be implemented to manufacture metal and metallic oxide nanoparticles containing polysaccharides, proteins, tannins, flavonoids, and glycosides such as aloin. The plant Aloe vera and its numerous components, such as leaf extract and gel, have revealed several beneficial uses that facilitate an extensive variety of applications. Acetic acid, citric acid, ascorbic acid, pectin, lignin, polyphenols, hemicellulose, and flavonoids are the primary biomolecules that exist in Aloe vera leaves and are capable of being used as stabilizing and reducing agents in the green fabrication of metallic nanoparticles 35 . A clear mucilaginous constituent named Aloe vera gel is generally composed of water, fibres, and chemicals that help maintain moisture 36 .
The current study focuses on the synthesis of Ag/ZnO heterostructures for biomedical applications via Aloe vera gel extract. We produce the photocatalytic activity of Ag/ZnO heterostructures through the use of Aloe vera extracts. To this end, we take advantage of the fact that Aloe vera gel includes a wide range of different chemicals utilizable as an agent that act on the surface and hinder nucleus accumulation by decreasing overall surface energy. Based on the findings of the present study, we illustrate that the synthesized Ag/ZnO heterostructures are eco-friendly and can be employed as a potent for efficient photocatalytic activity. Finally, we explain how the current work has been successfully utilized in a variety of environmental domains.
Materials and methods
Analytical-grade chemicals and precursors needed in experiments are purchased from Sigma Aldrich Company and used without further purification because all chemicals are 99% pure. Among these chemicals are powdered forms of silver nitrate (AgNO 3 ), zinc nitrate hexahydrate Zn (NO 3 ) 2 .6H 2 O, and sodium hydroxide (NaOH). To synthesize the Ag/ZnO nanocomposites as starting materials, deionized water, ethanol, and fresh Aloe vera leaves have been utilized. Ag/ZnO composites/nanocomposites were synthesized via ex-situ techniques.
Gel extract preparation
Aloe vera fresh leaves were collected from plants and washed completely with water. Then, we dried them in the air for about twenty minutes to prepare the aqueous extract of Aloe vera leaves. The gel was isolated from the leaves. 400 g of gel was divided into chunks and crushed with the help of a pestle and mortar. The gel was blended with a comparable quantity of distilled water and dried for 40 min at 85 °C in an oven. The prepared extract was stored in a refrigerator at room temperature for further experiments and then filtered using filter paper.
Synthesis of silver (Ag) nanostructures
An aqueous solution of AgNO 3 was prepared by mixing 0.5 M of AgNO 3 in 40 mL of distilled water, and the solution was magnetically stirred for 30 min at 85 °C. 40 mL of plant extract, Aloe vera , was gradually added to the AgNO 3 solution, and the above mixture was kept under magnetic stirring for about 1 h. Then, sonicate the abovementioned mixture for 2 h. The final solution was then transferred into a Teflon-lined, sealed vessel of 100 ml capacity under 150 °C conditions for 6 h. The centrifugation technique is used to separate a dark grey precipitation that was continually rinsed with ethanol and deionized water after being dried in an oven for 12 h at 80 °C.
Synthesis of zinc oxide (ZnO) nanostructures
To prepare zinc oxide nanostructures, 0.5 M zinc nitrate hexahydrate Zn (NO 3 ) 2 .6H 2 O was dispersed in 20 mL of deionized water and continued on magnetic stirring at 85 °C for 20 min. We added 20 mL of plant extract, Aloe vera , to the above mixture. Through this procedure, a solution of 0.5 M NaOH in 20 mL of deionized water was made. We further added the NaOH solution drop-wise to the above mixture and left it on magnetic stirring for 1 h. The abovementioned solution was sonicated for 1 h. The solution was transferred to a 100 mL coated Teflon-lined jar and heated at 150 °C for 6 h. The pale-white precipitation was gathered via centrifugation, repetitively scrubbed with ethanol and deionized water, and formerly dried for 12 h in an oven at 80 °C.
Ex-situ synthesis of Ag/ZnO heterostructures
Ag/ZnO heterostructures have been synthesized using an ex-situ approach. In this approach, previously-made nanostructures of ZnO and Ag have been utilized. Initially, in order to prepare the Ag/ZnO heterostructures with a molar ratio of 1:1, 0.2 g of ZnO was mixed in 20 mL of ethanol, and 0.2 g of Ag was mixed in 20 mL of ethanol, and both mixtures were combined together for 30 min with continuous stirring. Then add a drop-wise Ag solution to the ZnO solution and maintain magnetic stirring for 1 h. We then sonicated the aforementioned mixture for 1 h. Later on, the mixture was transferred into the centrifuge tube to gather the particles. Then it was dried in an oven for 6 h at about 80 °C. A dark grey precipitation was formed after grinding.
Photocatalytic activity
The photocatalytic activity was investigated using the Tubantin red 8BL dye. The study was carried out in the presence of a sun simulator named Abet Technologies Sunlight TM solar simulator. Prior to being employed in subsequent degradation experiments, each of these catalysts (ZnO and Ag/ZnO) was disinfected, implementing a range of cleaning and centrifugation methods. In order to estimate the catalyst's reusability and stability, three cycles were performed. The following equation is used to examine the degree of degradation: the pseudo-first-order process is a catalytic decolorization of dye solution 37 , 38 .
Here \(C_{0}\) is the initial concentration, and here is the concentration after a particular amount of time. The degradation was calculated with the help of 50 mL of aqueous Tubantin red 8BL dye (10 ppm) for 10 min, 20 min, 30 min, 40 min, 50 min, and 60 min in the light and for 30 min in the dark, respectively. All the samples were achieved with 0.015 g of the catalyst’s standard pH. The photocatalytic breakdown mechanism was utilized to determine the absorption peak characteristics of tubantin red 8BL dye. In the absence of catalyst, there was particularly no degradation.
Results and Discussion
Xrd analysis.
X-ray diffraction was used to analyze the crystalline nature of the prepared heterostructures. Figure 1 illustrates the XRD pattern of prepared Ag/ZnO heterostructures using Aloe vera gel extract. Sharp diffraction patterns were observed at 2 \(\theta\) = \(31^\circ\) , \(34^\circ\) , \(36^\circ\) , \(47^\circ\) , \(56^\circ\) , \(67^\circ\) , and \(69^\circ\) , which were ascribed to diffraction planes having indices (100), (002), (101), (102), (110), (112), and (201), respectively, that resemble the hexagonal crystal structure of ZnO nanostructures (JCPDS-files: PDF#36-1451). No other impurity peaks were found in the diffraction pattern, showing that all the precursors have been totally transformed into nanostructures, and therefore the resulting ZnO nanostructures are extremely crystalline in nature. The XRD pattern shows that only a single phase exists in ZnO nanostructures with the space group (P63mc). The crystalline character of the prepared specimen presents the sharp and broad nature of diffraction patterns. The comparatively higher intensity (101) peak implies a preferred alignment and anisotropic growth of crystallites. It is obvious from the results of XRD analysis that there were characteristic diffraction patterns with a distinct value of sharp peaks at 2 \(\theta\) = \(38^\circ\) and \(43^\circ\) attained, which correspond to the diffraction forms of (111) and (200) orientations. Based on standard diffraction data card no. (PDF#04-0783), some additional peaks occurred at \(62^\circ\) and \(77^\circ\) were compatible with diffraction planes (220) and (311), respectively. The examined Bragg’s reflection correlating to (111) and (200) may be recorded the prediction of the face-centered cubic crystal structure of Ag nanostructures. This pattern affirmed that there are no additional phases other than the FCC structure of metallic Ag. The confirmation of phase purity and synthesis of elemental silver were clearly investigated for consistency with reference data 39 . Furthermore, the presence of Ag in the ZnO lattice is verified by two additional peaks that have been identified as (111) and (200). Similar findings have been reported for Ag/ZnO heterostructures 39 , 40 , 41 , 42 , 43 , 44 , 45 .

XRD spectrum of Ag, ZnO and Ag/ZnO heterostructures.
FTIR analysis
The purity of samples and identification of functional groups were observed in the range of 500–4000 cm −1 using FTIR analysis. Figure 2 depicts the FTIR spectra of Ag nanostructures, ZnO nanostructures, and Ag/ZnO heterostructures synthesized using Aloe vera gel. The strong absorption band at 3200–3500 cm −1 correlates with the overlaying of the stretching vibrations of O–H in the phenolic group and N–H in the amine group 46 . With the transformation of Ag + ions into Ag and Zn +2 ions into ZnO, the O–H groups in phenols behave as reducing agents 46 . The peaks in the range of 2700–cm −1 are also linked with amide groups, which contain amino acids and proteins present in the gel extract. The stretching vibrations of alkanes, alkenes, and alkynes are attributed to the bands at 2089 cm −1 , 1360 cm −1 , and 2370 cm −1 , respectively 47 . The bands in the region of 1760–1520 cm −1 are ascribed to C=O carboxylic acids. Furthermore, the small vibrations in the region of 1476–1019 cm −1 indicate the presence of C–N groups of aromatic and aliphatic amines, –C–O– or –C–O–C-stretching vibrations, and aliphatic fluoro compounds (C–F) functional groups, which signifies the accessibility of flavonoids and reducing sugars 48 . Broader peaks observed below 1000 cm −1 indicate the occurrence of metal oxide vibrations (M–O). The sharp and dominant peaks were observed at 823.9 cm −1 and 712.2 cm −1 , which might be attributed to Ag–O and Zn–O bonding. The high band intensity of Ag/ZnO heterostructures usually signifies an increase in functional groups connected to the molecular bond, while the shifting of absorption bands designates an alteration in the hybridization state or arrangement of electrons in the molecular bonds. The absorption peak shows the interaction of H-bond formation with the O–H functional groups in Ag/ZnO heterostructures. FTIR measurements illustrate the carboxyl, alcoholic, and Ag–O and Zn–O functional groups, which confirm the fabrication of Ag/ZnO heterostructures 41 , 46 .

Fourier transmission spectra of Ag, ZnO and Ag/ZnO heterostructures.
SEM analysis
The morphological properties of prepared nanostructures were investigated via the SEM technique. The growth mechanism, shape, and surface morphology of Ag nanostructures, ZnO nanostructures, and Ag/ZnO heterostructures are illustrated in Fig. 3 a–c. It is examined that Aloe vera gel extract acts as capping and reducing agents, leading to the fabrication of Ag/ZnO heterostructures in various shapes. The randomly oriented and densely packed spherical shapes of silver nanostructures are clearly observed using SEM analysis in Fig. 3 a. The collected clusters that are distributed over the surface with a huge random empty space were displayed in the SEM image 49 . The nanostructure agglomeration shows that they were in direct contact and indicates the stability of the formation of Ag. The majority of nanostructures exhibit a spherical shape, though some nanostructures demonstrate cubic shapes that are not precisely determined. The synthesized ZnO nanostructure’s morphology and the micrographs are presented in Fig. 3 b. The formation of uniformly dispersed ZnO nanosheets with definite particle boundaries was clearly observed in Fig. 3 b. The 2D nanosheets are interconnected with each other to form a flower configuration. The micrographs indicated the three-dimensional nanoflower-like structures of ZnO nanostructures. Regarding the Aloe vera gel extract, more homogeneity of nanoflowers was examined in ZnO nanostructures 50 . The SEM image revealed that the shape of ZnO nanostructures is somehow nano needle-like morphology 51 . Figure 3 c depicts the SEM analysis of Ag/ZnO heterostructures. The micrograph indicates that the morphology of heterostructures is irregular and non-uniform in shape. The arrangement of particles looks like randomness in the form of clusters. This morphology implies that the composition might not be homogenous and the synthesized procedure might not have been uniformly optimized. It is observed that some spherical shapes are present due to Ag nanostructures on the surface of ZnO 40 . However, a small amount of Ag nanostructures appears to be comparatively tiny in size, whereas a huge amount of Ag agglomerates at the surface of porous and dispersed ZnO 39 . The formation of some irregular nanosheets was due to the presence of Ag nanostructures covering the surface of ZnO nanostructures. This agglomeration is generated because of the densification produced by the small space among the particles. The particles in Ag/ZnO heterostructures are conspicuously more agglomerated, with the creation of tiny particles over bigger clusters, which might be attributed to the fabrication of Ag nanostructures 44 , 52 . Furthermore, different morphologies might be attributed to the gel extract of the bioactive components. The presence of different shapes of nanostructures could be produced due to the bio-component nature of the extract 53 , 54 .

SEM Analysis of ( a ) Ag ( b ) ZnO and ( c ) Ag/ZnO heterostructures, ( d ) Average particle size distribution.
Figure 3 d represented the average particle size distribution of prepared nanostructures. The average size was 160.3 nm for Ag nanoparticles, 258.4 nm for ZnO, and 303.9 nm for Ag/ZnO. From this, we can clearly confirm the fact that average particle size was increased in composite as compared to Ag and ZnO.
EDX analysis
The most effective characterization technique for identifying the synthesis of Ag, ZnO, and Ag/ZnO nanostructures is EDX analysis. EDX measurements may also be employed to investigate the elemental composition. Figure 4 a–c describes the EDX spectrum of Ag, ZnO, and Ag/ZnO heterostructures using Aloe vera gel. The fabrication of Ag nanostructures was confirmed by the strong peak for metallic silver at 3 keV observed in the EDX spectrum in Fig. 4 a. The existence of silver elements is represented by a line in the EDX plot. Therefore, the formation of Ag nanostructures from Aloe vera gel can be confirmed. The total composition of silver is 87.0% (wt%), which is not found in previous literature. In order to observe the chemical composition of ZnO nanostructures, the graph depicts the two sharp and strong peaks associated with zinc and one distinct peak of the oxygen element. Figure 4 b reveals the significant peaks of zinc and oxygen, which verify that these are the two major components in nanostructures. The produced sample contains no impurities of other elements, which strongly confirmed the formation of high-purity ZnO nanostructures. The EDX spectrum of Ag/ZnO heterostructures indicates the specific peaks of Zn, O, and Ag. The distributions of elements in the analysis presented peaks of Zn, O, and Ag with atomic percentages of 21.07%, 70.50%, and 6.82%, respectively. While weight percentages are taken into consideration, the exposed peaks of 41.75% for Zn, 34.19% for O, and 22.32% for Ag are represented in Fig. 4 c. For zinc, there were two prominent signals near 1.5 keV and 8.5 keV. The only significant peak of the oxygen element was generated at 0.6 keV, whereas in the case of silver, two moderate peaks were observed at approximately 0.2 keV and 3.0 keV. Some minor peaks of C and Cl were examined in the EDX spectra. These elements were obtained from the biomolecules that were bound to the surfaces of Ag nanostructures and Ag/ZnO heterostructures in Aloe vera gel 45 , 55 .
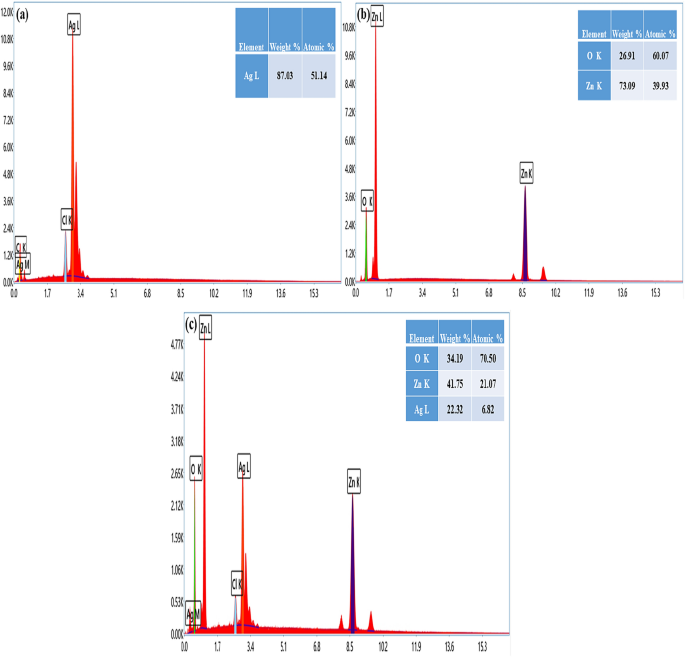
EDX spectra of ( a ) Ag, ( b ) ZnO and ( c ) Ag/ZnO heterostructures.
UV analysis
The optical absorption properties of ZnO, and Ag/ZnO heterostructures prepared via Aloe vera gel extract in the range of 200–800 nm were examined. The equation representing the Tauc relationship 56 was utilized for calculating the prepared samples optical band gap values:
In this equation, “E g ” signifies the optical bandgap energy, “ h ” is the Plank’s constant, “ \(\nu\) ” represents frequency, “ \(\alpha\) ” symbolizes the absorption coefficient and “A” is a constant that depends on transition probability 57 , 58 . Here, n relies on electrons transition and the value of n = 1/2 belongs to direct electrons transition with a direct optical band gap. Figure 5 a,b exhibit a plot between \(\alpha h\nu^{2}\) and \(E_{g}\) that determines the optical band gap energy of prepared nanocomposites. This Tauc plot indicated a band gap of 3.15 eV and 2.96 eV for ZnO and Ag/ZnO heterostructures, respectively. The decrease in energy band gap in Ag/ZnO heterostructures was observed when Ag is embedded into the ZnO. This might be due to n-type conductivity of metallic ions. The oxygen vacancies generated in ZnO, which may capture impurities and possible chemical reactions originated among ZnO and Ag surface could also be involved in narrowing the energy band gap. This contributes the more oxygen vacancies, whereas high electron density was produced in ZnO. Ag + replaces the Zn 2+ sites, leading to increasing electron densities and vacancies due to variation in ionic radius which lowers the Fermi level and brings about narrowing the band gap.
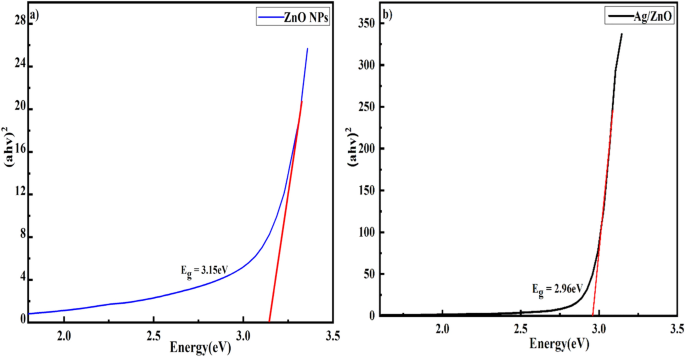
Tauc Plot of ( a ) ZnO and ( b ) Ag/ZnO heterostructures.
Tubantin red 8BL photodegradation
The photocatalytic activity of fabricated ZnO and Ag/ZnO heterostructures was studied by examining the degradation of tubantin red 8BL dye using solar light irradiation. The absorption band of tubantin red 8BL was positioned at 510 nm and reduced subsequently with increasing irradiation time. The photodegradation and absorption bands versus time of Tubantin red 8BL dye acting as a photocatalyst ZnO and Ag/ZnO (1:1) heterostructures at a concentration of 0.015 g are represented in Fig. 6 a,b. The photodegradation method of Tubantin red 8BL dye displays the characteristic band at 510 nm, which is a distinctive standard indicator, and the degradation of synthesized ZnO and Ag/ZnO (1:1) heterostructures was also performed under dark conditions. A catalyst’s degradation of tubantin red 8BL dye was revealed to cause the decrease in concentration over time.

Uv–visible absorption spectrum of the Tubantin red 8BL dye with catalyst ( a ) ZnO and ( b ) Ag/ZnO (1:1) heterostructures.
Figure describes the initial absorption band and the absorption spectrum for the photocatalytic activity of ZnO and Ag/ZnO (1:1) heterostructures after 30 min in the dark and 10 min, 20 min, 30 min, 40 min, 50 min, and 60 min in the light. Figure 7 a indicates the correlation among efficiency (%) and time for the ZnO and Ag/ZnO (1:1) heterostructures. The measured efficiency values of ZnO nanostructures in the presence of light 11.15%, 16.93%, 20.62%, 24.89%, 29.16% and 34.29%; and for Ag/ZnO (1:1) heterostructures efficiency values are 44.08%, 47.41%, 50.73%, 54.04%, 58.97% and 64.55% correspondingly. The efficiency values of ZnO and Ag/ZnO (1:1) heterostructures in 30 min dark conditions is analogously observed around 08.61% and 42.33%, respectively.

( a ) Irradiation time verses efficiency (%); ( b ) degradation ( \(C/C_{o}\) ) over time; ( c )—Ln ( \(C/C_{o}\) ) versus irradiation time; ( d ) % efficiency verses cycle graphs of ZnO and Ag/ZnO (1:1) heterostructures.
The connection between degradation rate ( \(C/C_{0}\) ) and irradiation time (min) is explained in Fig. 7 b. The degradation rate of Ag/ZnO (1:1) heterostructures are 0.5593, 0.5245, 0.4898, 0.4608, 0.4085 and 0.3535 and ZnO nanostructures are 0.8869, 0.8291, 0.7899, 0.7507, 0.7072, and 0.6551 respectively after 10, 20, 30, 40, 50 and 60 min in the presence of light. Likewise after 30 min under dark conditions, the degradation rates of pure and nanocomposites are 0.9116 and 0.57338 respectively. Figure 7 c examines the graph of Ln ( \(C/C_{0}\) ) values over time for the Ag/ZnO (1:1) are 0.5791, 0.6427, 0.7063, 0.7768, 0.8895 and 1.0354 and for the ZnO nanostructures are 0.1114, 0.1868, 0.2313, 0.2854, 0.3418 and 0.4195 respectively. The rate constant values of Ag/ZnO (1:1) heterostructures and ZnO nanostructures are 0.013804 and 0.006477 min −1 accordingly, demonstrating the pseudo-1st order kinetics and has significant photocatalytic activity. The stability of Ag/ZnO (1:1) and ZnO synthesized heterostructures has been confirmed using three cycles as represented in Fig. 7 d. The degradation characteristics and stability of generated heterostructures were obviously visible after being subjected to solar radiation after 60 min.
Conclusions
In the current work, Ag/ZnO heterostructures were successfully synthesized using the hydrothermal method via Aloe vera gel extract. The Ag/ZnO heterostructures are preferentially oriented along the (111) plane, which was verified by using XRD analysis. FTIR measurements exhibited that the absorption bands demonstrate the formation of H-bonds and their interaction with O–H functional groups in Ag/ZnO heterostructures. The SEM analysis reveals the existence of both ZnO and Ag in spherical shapes that could be produced due to the bio-component nature of the gel extract. The EDX spectrum designates the weight percentages of Zn, O, and Ag as 41.75%, 34.19%, and 22.32%, respectively. The energy band gap (E g ) observed in Ag/ZnO heterostructures is 2.96 eV. Ag/ZnO exhibited a maximum photodegradation efficiency of 64.55%, demonstrating that it is a promising material for excellent photocatalytic degradation. The aforementioned findings imply that the synthesized Ag/ZnO heterostructures could be helpful in numerous fields, including the elimination of harmful organic dyes from wastewater, and can be used in various biomedical domains.
Data availability
Data will be provided on request.
Gea, S. et al. Facile synthesis of ZnO–Ag nanocomposite supported by graphene oxide with stabilised band-gap and wider visible-light region for photocatalyst application. JMRT 19 , 2730–2741 (2022).
CAS Google Scholar
Xue, B. & Zou, Y. High photocatalytic activity of ZnO–graphene composite. J. Colloid Interface Sci. 529 , 306–313 (2018).
Article ADS CAS PubMed Google Scholar
Yang, S. J. et al. MOF-derived ZnO and ZnO@ C composites with high photocatalytic activity and adsorption capacity. J. Hazard. Mater. 186 (1), 376–382 (2011).
Article CAS PubMed Google Scholar
Chakraborty, S. & Mary, N. L. Biocompatible supercapacitor electrodes using green synthesised ZnO/Polymer nanocomposites for efficient energy storage applications. J. Energy Storage 28 , 101275 (2020).
Article Google Scholar
Suliman, A. E. et al. Preparation of ZnO nanoparticles and nanosheets and their application to dye-sensitized solar cells. Sol. Energy Mater. 91 (18), 1658–1662 (2007).
Que, M. et al. Progress in ZnO nanosensors. Sensors 21 (16), 5502 (2021).
Article ADS CAS PubMed PubMed Central Google Scholar
Xu, L. et al. Characterization of Ag-doped ZnO thin film for its potential applications in optoelectronic devices. Optik 170 , 484–491 (2018).
Article ADS CAS Google Scholar
Alrajhi, A. H. & Ahmed, N. M. Green synthesis of zinc oxide nanoparticles using salvia officinalis extract. In Handbook of Green and Sustainable Nanotechnology: Fundamentals, Developments and Applications (eds Shanker, U. et al. ) 1–21 (Springer, Cham, 2023).
Google Scholar
Galstyan, V. et al. Nanostructured ZnO chemical gas sensors. Ceram. Int. 41 (10), 14239–14244 (2015).
Article CAS Google Scholar
Noman, M. T. et al. One-pot sonochemical synthesis of ZnO nanoparticles for photocatalytic applications, modelling and optimization. J. Mater. 13 (1), 14 (2019).
Article ADS MathSciNet Google Scholar
Sharma, D. K. et al. A review on ZnO: Fundamental properties and applications. Mater. Today 49 , 3028–3035 (2022).
Islam, F. et al. Exploring the journey of zinc oxide nanoparticles (ZnO-NPs) toward biomedical applications. Materials 15 (6), 2160 (2022).
Kaur, R. et al. Lignin-based metal oxide nanocomposites for UV protection applications: A review. J. Clean. Prod. 317 , 128300 (2021).
Ismail, M. et al. Plant mediated green synthesis of anti-microbial silver nanoparticles—A review on recent trends. Rev. Nanosci. Nanotechnol. 5 (2), 119–135 (2016).
Zhang, X.-F. et al. Silver nanoparticles: Synthesis, characterization, properties, applications, and therapeutic approaches. Int. J. Mol. Sci. 17 (9), 1534 (2016).
Article PubMed PubMed Central Google Scholar
Hussein, H. A. MA Abdullah, Novel drug delivery systems based on silver nanoparticles, hyaluronic acid, lipid nanoparticles and liposomes for cancer treatment. Appl. Nanosci. 12 (11), 3071–3096 (2022).
Ameen, F. et al. Anti-oxidant, anti-fungal and cytotoxic effects of silver nanoparticles synthesized using marine fungus Cladosporium halotolerans . Appl. Nanosci. 13 (1), 623–631 (2023).
Alaallah, N. J. et al. Eco-friendly approach for silver nanoparticles synthesis from lemon extract and their anti-oxidant, anti-bacterial, and anti-cancer activities. J. Turk. chem. soc. 10 (1), 205–216 (2023).
Kumar, S. et al. Plant extract mediated silver nanoparticles and their applications as antimicrobials and in sustainable food packaging: A state-of-the-art review. Trends Food Sci. 112 , 651–666 (2021).
Paiva-Santos, A. C. et al. Plant-mediated green synthesis of metal-based nanoparticles for dermopharmaceutical and cosmetic applications. Int. J. Pharm. 597 , 120311 (2021).
Ahmadi, O. et al. Eco-friendly microwave-enhanced green synthesis of silver nanoparticles using Aloe vera leaf extract and their physico-chemical and antibacterial studies. Green Process. Synth. 7 (3), 231–240 (2018).
Mohammadlou, M., Maghsoudi, H. & Jafarizadeh-Malmiri, H. A review on green silver nanoparticles based on plants: Synthesis, potential applications and eco-friendly approach. Int. Food Res. J. 23 (2), 446 (2016).
Abdel-Aziz, M. S. et al. Antioxidant and antibacterial activity of silver nanoparticles biosynthesized using Chenopodium murale leaf extract. J. Saudi Chem. Soc. 18 (4), 356–363 (2014).
Kim, M. et al. Green-synthesis of anisotropic peptone-silver nanoparticles and its potential application as anti-bacterial agent. Polym. J. 11 (2), 271 (2019).
Baruah, K. et al. Ocimum sanctum mediated green synthesis of silver nanoparticles: A biophysical study towards lysozyme binding and anti-bacterial activity. J. Mol. Liq. 337 , 116422 (2021).
Melkamu, W. W. & Bitew, L. T. J. H. Green synthesis of silver nanoparticles using Hagenia abyssinica (Bruce) JF Gmel plant leaf extract and their antibacterial and anti-oxidant activities. J. Mol. Liq. 7 (11), e08459 (2021).
Mohanta, Y. K. et al. Phyto-assisted synthesis of bio-functionalised silver nanoparticles and their potential anti-oxidant, anti-microbial and wound healing activities. IET Nanobiotechnol. 11 (8), 1027–1034 (2017).
Burange, P. J. et al. Synthesis of silver nanoparticles by using Aloe vera and Thuja orientalis leaves extract and their biological activity: A comprehensive review. BNRC 45 , 1–13 (2021).
Nandal, U. & Bhardwaj, R. L. Aloe vera : A valuable wonder plant for food, medicine and cosmetic use-a review. Int J Pharm Sci Rev Res. 13 (1), 59–67 (2012).
Chow, J.T.-N. et al. Chemical characterization of the immunomodulating polysaccharide of Aloe vera L. Carbohydr. Res. 340 (6), 1131–1142 (2005).
Reynolds, T. & Dweck, A. C. Aloe vera leaf gel: A review update. J. Ethnopharmacol. 68 (1–3), 3–37 (1999).
Anju, T. et al. Green synthesis of silver nanoparticles from Aloe vera leaf extract and its antimicrobial activity. Mater. Today Proc. 43 , 3956–3960 (2021).
Zaidan, M. et al. In vitro screening of five local medicinal plants for antibacterial activity using disc diffusion method. Trop. Biomed. 22 (2), 165–170 (2005).
CAS PubMed Google Scholar
Rathor, N. et al. Acute effect of Aloe vera gel extract on experimental models of pain. Inflammation 35 , 1900–1903 (2012).
Article PubMed Google Scholar
Zhang, Y. et al. Biosynthesis of silver nanoparticles at room temperature using aqueous aloe leaf extract and antibacterial properties. Colloids Surf. A Physicochem. Eng. Asp. 423 , 63–68 (2013).
Kumar, D. et al. A review of immunomodulators in the Indian traditional health care system. J. Microbiol. 45 (3), 165–184 (2012).
Shahid, W. et al. Ex situ synthesis and characterizations of MoS 2 /WO 3 heterostructures for efficient photocatalytic degradation of RhB. Nanomaterials 12 (17), 2974 (2022).
Article CAS PubMed PubMed Central Google Scholar
Ullah, S. et al. Advancing photocatalysis: Innovative approaches using novel V 2 O 5 /ZnO nanocomposites for efficient photocatalytic degradation of tubantin red. J. Saudi. Chem. Soc. 27 , 101766 (2023).
Slathia, S., Gupta, T. & Chauhan, R. Green synthesis of Ag–ZnO nanocomposite using Azadirachta indica leaf extract exhibiting excellent optical and electrical properties. Matter. Phys. Rev. B Condens. 621 , 413287 (2021).
Jadhav, P. et al. Green AgNPs decorated ZnO nanocomposites for dye degradation and antimicrobial applications. Eng. Sci. 12 , 79–94 (2020).
Panchal, P. et al. Biogenic mediated Ag/ZnO nanocomposites for photocatalytic and antibacterial activities towards disinfection of water. J. Colloid Sci. 563 , 370–380 (2020).
Nagaraju, G. et al. Electrochemical heavy metal detection, photocatalytic, photoluminescence, biodiesel production and antibacterial activities of Ag–ZnO nanomaterial. Mater. Res. Bull. 94 , 54–63 (2017).
Jobe, M. C. et al. Biosynthesis of zinc oxide and silver/zinc oxide nanoparticles from Urginea epigea for antibacterial and antioxidant applications. Heliyon 8 (12), e12243 (2022).
Alharthi, F. A. et al. Facile one-pot green synthesis of Ag–ZnO Nanocomposites using potato peeland their Ag concentration dependent photocatalytic properties. Sci. Rep. 10 (1), 20229 (2020).
Mtavangu, S. G. et al. In situ facile green synthesis of Ag–ZnO nanocomposites using Tetradenia riperia leaf extract and its antimicrobial efficacy on water disinfection. Sci. Rep. 12 (1), 15359 (2022).
Das, B. et al. Understanding the antifungal mechanism of Ag@ ZnO core-shell nanocomposites against Candida krusei . Sci. Rep. 6 (1), 36403 (2016).
Article ADS MathSciNet CAS PubMed PubMed Central Google Scholar
Sohal, J. K. et al. Determination of antioxidant potential of biochemically synthesized silver nanoparticles using Aloe vera gel extract. Plant Sci. Today. 6 (2), 208–217 (2019).
PP, V. J. B. In vitro biocompatibility and antimicrobial activities of zinc oxide nanoparticles (ZnO NPs) prepared by chemical and green synthetic route—a comparative study. BNSC 10 , 112–121 (2020).
Gecer, E. N. et al. Green synthesis of silver nanoparticles from Echinacea purpurea (L.) Moench with antioxidant profile. JPST 40 (1), 50–57 (2022).
Mohan, S. et al. Hydrothermal synthesis and characterization of zinc oxide nanoparticles of various shapes under different reaction conditions. Nano. Exp. 1 (3), 030028 (2020).
Article ADS Google Scholar
Venkataraju, J. L. et al. Synthesis, characterization and evaluation of antimicrobial activity of zinc oxide nanoparticles. J. Biochem. Tech. 3 (5), 151–154 (2014).
Khan, M. S. et al. Ultrasound-assisted green synthesis of ag-decorated ZnO nanoparticles Using Excoecaria agallocha leaf extract and evaluation of their photocatalytic and biological activity. ChemistrySelect. 5 (41), 12660–12671 (2020).
SJ, L. J. R. et al. Green synthesis, characterizations, and antibacterial activity of silver nanoparticles from Themeda quadrivalvis , in conjugation with macrolide antibiotics against respiratory pathogens. RAMS 62 (1), 20220301 (2023).
Medda, S. et al. Biosynthesis of silver nanoparticles from Aloe vera leaf extract and antifungal activity against Rhizopus sp. and Aspergillus sp. Appl. Nanosci. 5 , 875–880 (2015).
Yuharmon, N. et al. Scanning electron microscopy of soxhlet extracted Aloe vera gel for electrolyte application. JPCS 1123 , 012070 (2018).
Feng, Y. et al. Can Tauc plot extrapolation be used for direct-band-gap semiconductor nanocrystals?. J. Appl. Phys. 117 (12), 125701 (2015).
Yusof, K. N. et al. Parkia speciosa as reduction agent in green synthesis silver nanoparticles. ChemistrySelect 3 (31), 8881–8885 (2018).
Maraj, M. et al. Mo-doped CuO nanomaterial for photocatalytic degradation of water pollutants under visible light. Catalysts 11 (10), 1198 (2021).
Download references
Acknowledgements
The authors express their gratitude to the Deanship of Scientific Research, Imam Mohammad Ibn Saud Islamic University, Saudi Arabia to support this research work. The authors also thankful to The University of Lahore, Lahore, Pakistan.
Author information
Authors and affiliations.
Department of Physics, The University of Lahore, Lahore, 54000, Pakistan
Nawal Ansar, Wajeehah Shahid, Muhammad Iftikhar Khan, Maria Khizar & Sana Ullah
Department of Environmental Sciences, The University of Lahore, Lahore, 54000, Pakistan
Muhammad Atif Irshad & Rab Nawaz
Institute of Molecular and Biology and Biotechnology, The University of Lahore, Lahore, 54000, Pakistan
Samiah Shahid
Faculty of Engineering and Quantity Surveying, INTI International University, 71800, Nilai, Negeri Sembilan, Malaysia
Department of Chemistry, Government College University Faisalabada, Faisalabad, Pakistan
Department of Chemistry, College of Science, Imam Mohammad Ibn Saud Islamic University (IMSIU), 11623, Riyadh, Saudi Arabia
Aamal A. Al-Mutairi, Sami A. Al-Hussain & Magdi E. A. Zaki
You can also search for this author in PubMed Google Scholar
Contributions
N.A.: methodology, formal analysis, validation, writing—original draft. W.S.: conceptualization, supervision, writing—original draft, resources, writing—review and editing. Project administration, M.A.I.: writing—original draft, investigation, writing—review and editing. S.S.: formal analysis, data curation, writing—review and editing. R.N.: conceptualization, methodology, validation, writing—original draft. A.I.: data curation, visualization, funding acquisition, writing—review and editing. M.I.K.: resources, validation, software, writing—review and editing. A.A.A.: data curation, formal analysis, writing—review and editing. M.K.: visualization, data curation. Writing—review and editing, S.A.A.; validation, funding acquisition, visualization, data curation. Methodology, writing—original draft, writing—review and editing. S.U. validation, data curation. Writing—review and editing, M.E.A.Z.: funding acquisition, project administration, investigation, formal analysis, writing—review and editing. All authors have read and agreed to the published version of the manuscript.
Corresponding authors
Correspondence to Wajeehah Shahid , Ali Irfan or Magdi E. A. Zaki .
Ethics declarations
Competing interests.
The authors declare no competing interests.
Additional information
Publisher's note.
Springer Nature remains neutral with regard to jurisdictional claims in published maps and institutional affiliations.
Rights and permissions
Open Access This article is licensed under a Creative Commons Attribution 4.0 International License, which permits use, sharing, adaptation, distribution and reproduction in any medium or format, as long as you give appropriate credit to the original author(s) and the source, provide a link to the Creative Commons licence, and indicate if changes were made. The images or other third party material in this article are included in the article's Creative Commons licence, unless indicated otherwise in a credit line to the material. If material is not included in the article's Creative Commons licence and your intended use is not permitted by statutory regulation or exceeds the permitted use, you will need to obtain permission directly from the copyright holder. To view a copy of this licence, visit http://creativecommons.org/licenses/by/4.0/ .
Reprints and permissions
About this article
Cite this article.
Ansar, N., Shahid, W., Irshad, M.A. et al. Aloe-inspired eco-friendly synthesis of Ag/ZnO heterostructures: boosting photocatalytic potential. Sci Rep 14 , 12711 (2024). https://doi.org/10.1038/s41598-024-61466-9
Download citation
Received : 16 January 2024
Accepted : 06 May 2024
Published : 03 June 2024
DOI : https://doi.org/10.1038/s41598-024-61466-9
Share this article
Anyone you share the following link with will be able to read this content:
Sorry, a shareable link is not currently available for this article.
Provided by the Springer Nature SharedIt content-sharing initiative
By submitting a comment you agree to abide by our Terms and Community Guidelines . If you find something abusive or that does not comply with our terms or guidelines please flag it as inappropriate.
Quick links
- Explore articles by subject
- Guide to authors
- Editorial policies
Sign up for the Nature Briefing newsletter — what matters in science, free to your inbox daily.

Phytofabrication of biocompatible zinc oxide nanoparticle using Gymnema sylvestre and its potent in vitro antibacterial, antibiofilm, and cytotoxicity against human breast cancer cells (MDA-MB-231)
- Research Paper
- Published: 31 May 2024
Cite this article
- R. Kavipriya 1 &
- R. Ramasubburayan 2
37 Accesses
Explore all metrics
The increasing incidence of breast cancer and bacterial biofilm in medical devices significantly heightens global mortality and morbidity, challenging synthetic drugs. Consequently, greener-synthesized nanomaterials have emerged as a versatile alternative for various biomedical applications, offering new therapeutic avenues. This study explores the synthesis of biocompatible zinc oxide (ZnONPs) nanoparticles using Gymnema sylvestre and its antibacterial, antibiofilm, and cytotoxic properties. Characterization of ZnONPs inferred that UV–Vis spectra exhibited a sharp peak at 370 nm. Fourier transform infrared spectroscopical analysis revealed the presence of active functional groups such as aldehyde, alkyne, cyclic alkene, sulfate, alkyl aryl ether, and Zn–O bonds. X-ray diffraction analysis results confirmed the crystalline nature of the nanoparticle. Scanning electron microscope analysis evidenced hexagonal morphology, and energy-dispersive X-ray analysis confirmed zinc content. High-resolution transmission electron microscope analysis showed hexagonal and rod-shaped ZnONPs with a size of 5 nm. Zeta potential results affirmed the stability of nanoparticles. The ZnONPs effectively inhibited gram-positive (18–20 mm) than gram-negative (12–18 mm) bacterial pathogens with lower bacteriostatic and higher bactericidal values. Biofilm inhibitory property inferred ZnONPs were more effective against gram-positive (38–94%) than gram-negative bacteria (27–86%). The concentration of ZnONPs to exert 50% biofilm-inhibitory is lower against gram-positive bacteria (179.26–203.95 μg/mL) than gram-negative bacteria (201.46–236.19 μg/mL). Microscopic visualization inferred that at 250 μg/mL, ZnONPs strongly disrupted biofilm formation, as evidenced by decreased biofilm density and altered architecture. The cytotoxicity of ZnONPs against breast cancer cells showed a dose-dependent reduction in cell viability with an IC 50 value of 19.4 µg/mL. AO/EB staining indicated early and late apoptotic cell death of breast cancer cells under fluorescence microscopy. The results of hemolytic activity validated the biocompatibility of the ZnONPs. Thus, the unique properties of the green-synthesized ZnONPs suggest their potential as effective drug carriers for targeted delivery in cancer therapy and the treatment of biofilm-related infections.
This is a preview of subscription content, log in via an institution to check access.
Access this article
Price includes VAT (Russian Federation)
Instant access to the full article PDF.
Rent this article via DeepDyve
Institutional subscriptions
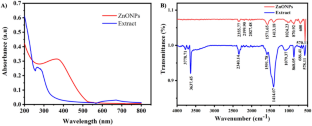
Similar content being viewed by others
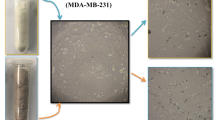
In vitro anticancer and antibacterial performance of biosynthesized Ag and Ce co-doped ZnO NPs

Arthrospira platensis-Mediated Green Biosynthesis of Silver Nano-particles as Breast Cancer Controlling Agent: In Vitro and In Vivo Safety Approaches

Biosynthesis of Zinc Oxide-Zerumbone (ZnO-Zer) Nanoflakes Towards Evaluating Its Antibacterial and Reactive Oxygen Species (ROS)-Dependent Cytotoxic Activity
Availability of data and materials.
Data availability will be made upon request.
Kumar B, Sharma D, Sharma P, Katoch VM, Venkatesan K, Bisht D (2013) Proteomic analysis of Mycobacterium tuberculosis isolates resistant to kanamycin and amikacin. J Proteomics 94:68–77
Article CAS PubMed Google Scholar
Chen M, Yu Q, Sun H (2013) Novel strategies for the prevention and treatment of biofilm related infections. Int J Mol Sci 14:18488–18501
Article PubMed PubMed Central Google Scholar
Rahim MI, Rohde M, Rais B, Seitz JM, Mueller PP (2016) Susceptibility of metallic magnesium implants to bacterial biofilm infections. J Biomed Mater ResA 104:1489–1499
Article CAS Google Scholar
Zheng Y, He L, Asiamah TK, Otto M (2018) Colonization of medical devices by staphylococci . J Appl Environ Microbiol 20:3141–3153
Google Scholar
Oliveira WF, Silva PMS, Silva RCS, Silva GMM, Machado G, Coelho L, Correia MTS (2018) Staphylococcus aureus and Staphylococcus epidermidis infections on implants. J Hosp Infect 98:111–117
Sung H, Ferlay J, Siegel RL, Laversanne M, Soerjomataram I, Jemal A, Bray F (2021) Global Cancer Statistics 2020: GLOBOCAN Estimates of Incidence and Mortality Worldwide for 36 Cancers in 185 Countries. CA Cancer J Clin 71:209–249
Article PubMed Google Scholar
Bengoechea JA, Bamford CG (2020) SARS-CoV-2, bacterial co-infections, and AMR: the deadly trio in COVID-19? EMBO Mol Med 12(7):e12560
Article CAS PubMed PubMed Central Google Scholar
Maldiney T, Pineau V, Neuwirth C, Ouzen L, Eberl I, Jeudy G et al (2022) Endotracheal tube biofilm in critically ill patients during the COVID-19 pandemic: description of an underestimated microbiological compartment. Sci Rep 12(1):22389
Liao D, Zhang W, Gupta P, Lei ZN, Wang JQ, Cai CY, De Vera AA, Zhang L, Chen ZS, Yang DH (2019) Tetrandrine interaction with ABCB1 reverses multidrug resistance in cancer cells through competition with anti-cancer drugs followed by downregulation of ABCB1 expression. Molecules 24:4383
Pelgrift RY, Friedman AJ (2013) Nanotechnology as a therapeutic tool to combat microbial resistance. Adv Drug Deliv Rev 65:1803–1815
Gudkov SV, Burmistrov DE, Serov DA, Rebezov MB, Semenova AA, Lisitsyn AB (2021) A mini review of antibacterial properties of ZnO nanoparticles. Front Phys 9:641481
Article Google Scholar
Vinotha V, Iswarya A, Thaya R, Govindarajan M, Alharbi NS, Kadaikunnan S, Khaled JM, Al-Anbr MN, Vaseeharan B (2019) Synthesis of ZnO nanoparticles using insulin-rich leaf extract: anti-diabetic, antibiofilm and anti-oxidant properties. J Photochem Photobiol B: Biol 197:111541
Ma DD, Yang WX (2016) Engineered nanoparticles induce cell apoptosis: Potential for cancer therapy. Oncotarget 7:40882
Malaikozhundan B, Vinodhini J, Kalanjiam MAR, Vinotha V, Palanisamy S, Vijayakumar S, Vaseeharan B, Mariyappan A (2020) High synergistic antibacterial, antibiofilm, antidiabetic and antimetabolic activity of Withania somnifera leaf extract-assisted zinc oxide nanoparticle. Bioproc Biosyst Eng 43:1533–1547
Khan F, Sarker MMR, Ming LC, Mohamed IN, Zhao C, Sheikh BY, Tsong HF, Rashid MA (2019) Comprehensive review on phytochemicals, pharmacological and clinical potentials of Gymnema sylvestre . Front Pharmacol 10:1223
Tiwari P, Mishra BN, Sangwan NS (2014) Phytochemical and pharmacological properties of Gymnema sylvestre : an important medicinal plant. Biomed Res Int 2014:830285. https://doi.org/10.1155/2014/830285
Muddappur U, Manjunath S, Alqathani YS, Shaikh IA, Khan AA, Mannasaheb BA, Yaraguppi D, More SS (2024) Exploring bioactive phytochemicals in Gymnema sylvestre: biomedical uses and computational investigations. Separations 11(2):50
Singh N, Singh R, Shah K, Pramanik BK (2023) Green synthesis of zinc oxide nanoparticles using lychee peel and its application in anti-bacterial properties and CR dye removal from wastewater. Chemosphere 327:138497
Ramasubburayan R, Prakash S, Iyapparaj P, Sumathi S, Titus S, Immanuel G, Palavesam A (2017) Isolation, screening and evaluation of antifouling activity of mangrove associated bacterium, Bacillus subtilis subsp. subtilis RG. Proc. Natl. Acad Sci India Sect B Biol Sci 87:1015–1024
CAS Google Scholar
Devilakshmi M, Ramasubburayan R (2023) Green synthesis of TiO 2 nanoparticles using Terminalia chebula and its potent antibiofilm activity against dental caries-associated bacterium Streptococcus mutans . Biocatal Agric Biotechnol 54:102953
Prakash S, Pattukumar V, Thirumurugan D, Sarvanan R, Ravinder Singh C, Immanuel G, Ramasubburayan R (2023) Exploring biotechnological approaches on in vitro callus induction and evaluation of biomedicinal properties of Cleome gynandra L. Proc Biochem 134:16
Mosmann T (1983) Rapid colorimetric assay for cellular growth and survival: application to proliferation and cytotoxicity assays. J Immunol Methods 65(1–2):55–63
Baskić D, Popović S, Ristić P, Arsenijević NN (2006) Analysis of cycloheximide-induced apoptosis in human leukocytes: fluorescence microscopy using annexin V/propidium iodide versus acridin orange/ethidium bromide. Cell Biol Int 30:924–932
Prakash S, Ramasubburayan R, Sri Ramkumar V, Kannapiran E, Palavesam E, Immanuel G (2016) In vitro – Scientific evaluation on antimicrobial, antioxidant, cytotoxic properties and phytochemical constituents of traditional coastal medicinal plants. Biomed Pharamacother 83:648–657
Umavathi S, Mahboob S, Govindarajan M, Al-Ghanim KA, Ahmed Z, Virik P, Norah Al-Mulhm N, Subash M, Gopinath K, Kavitha C (2021) Green synthesis of ZnO nanoparticles for antimicrobial and vegetative growth applications: a novel approach for advancing efficient high quality health care to human wellbeing. Saudi J Biol Sci 28:1808–1815
Hameed H, Waheed A, Sharif MS, Saleem M, Afreen A, Tariq M, Kamal A, Al-onazi W, Al Farraj DA, Ahmad S, Mahmoud RM (2023) Green synthesis of Zinc Oxide (ZnO) nanoparticles from green algae and their assessment in various biological applications. Micromachines 14:928
Stan M, Popa A, Toloman D, Silipas TD, Vodnar DC (2016) Antibacterial and Antioxidant Activities of ZnO Nanoparticles Synthesized Using Extracts of Allium sativum , Rosmarinus officinalis and Ocimum basilicum . Acta Metall Sin (Eng Lett) 29:228–236
Kuchekar SR, Patil MP, Gaikwad V, Sung- Hwan H (2017) Synthesis and characterization of silver nanoparticles using Azadirachta indica (Neem) leaf extract. Int J Eng Sci Invention Res Dev 6:47–55
Geetha MS, Nagabhushana H, Shivananjaiah HN (2016) Green mediated synthesis and characterization of ZnO nanoparticles using Euphorbia Jatropa latex as reducing agent. J Sci Adv Mater Devices 1:301–310
Naseer M, Aslam U, Khalid B, Chen B (2020) Green route to synthesize Zinc Oxide Nanoparticles using leaf extracts of Cassia fistula and Melia azadarach and their antibacterial potential. Sci Rep 10:9055
Nagajyothi PC, Sreekanth TVM, Tetty CO, Jun YI, Mook SH (2014) Characterization, antibacterial, antioxidant and cytotoxic activities of ZnO nanoparticles using Coptidis rhizoma . Biorg Med Chem Lett 24(7):4298–4303
Iqbal J, Abbasi BA, Yaseen T, Zahra SA, Shahbaz A, Shah SA et al (2021) Green synthesis of zinc oxide nanoparticles using Elaeagnus angustifolia L. leaf extracts and their multiple in vitro biological applications. Sci Rep 11(1):20988
Kalaimurgan D, Lalitha K, Govindarajan RK, Unban K, Shivakumar MS, Venkatesan S et al (2023) Biogenic synthesis of zinc oxide nanoparticles using Drynaria quercifolia tuber extract for antioxidant, antibiofilm, larvicidal, and photocatalytic applications. Biomass Convers Biorefin 0:1–17. https://doi.org/10.1007/s13399-023-04751-3
Abdelmigid HM, Hussien NA, Alyamani AA, Morsi MM, AlSufyani NM, Kadi HA (2022) Green synthesis of zinc oxide nanoparticles using pomegranate fruit peel and solid coffee grounds vs chemical method of synthesis, with their biocompatibility and antibacterial properties investigation. Molecules 27:1236
Honary S, Zahir F (2013) Effect of zeta potential on the properties of nano-drug delivery systems – A review (Part 2). Trop J Pharm Res 32(4):711
El-Fallal AA, Elfayoumy RA, El-Zahed MM (2023) Antibacterial activity of biosynthesized zinc oxide nanoaprticles using Kombucha extract. SN Applied Sci 5:332
Siddiqi KS, Rahman A, Husen A (2018) Properties of zinc oxide nanoparticles and their activity against microbes. Nanoscale Res Lett 13:141
Shkodenko L, Kassirov I, Koshel E (2020) Metal oxide nanoparticles against bacterial biofilms: perspectives and limitations. Microorganisms 8:1545
Kumar L, Bisen M, Harjai K, Chhibber S, Azizov S, Lalhlenmawia H, Kumar D (2023) Advances in Nanotechnology for Biofilm Inhibition. ACS Omega 8(24):21391–21409
Gupta K, Chhibber S (2019) Biofunctionalization of Silver Nanoparticles with Lactonase Leads to Altered Antimicrobial and Cytotoxic Properties. Front Mol Biosci 6:63
Abdelghafar A, Yousef N, Askoura M (2022) Zinc oxide nanoparticles reduce biofilm formation, synergize antibiotics action and attenuate Staphylococcus aureus virulence in host; an important message to clinicians. BMC Microbiol 22:1–17
Mendes CR, Dilarri G, Forsan CF, Sapata VDMR, Lopes PRM, de Moraes PB, Montagnolli RN, Ferreira H, Bidoia ED (2022) Antibacterial action and target mechanisms of zinc oxide nanoparticles against bacterial pathogens. Sci Rep 12:2658
Tabrez S, Khan AU, Hoque M, Suhail M, Khan MI, Zughaibi TA (2022) Biosynthesis of ZnO NPs from pumpkin seeds extract and elucidation of its anticancer potential against breast cancer. Nanotechnol Rev 11:2714–2725
Alqahtani AA, El Raey MA, Abdelsalam E, Ibrahim AM, Alqahtani O, Torky ZA, Attia HG (2022) The biosynthesized Zinc Oxide nanoparticles’ antiviral activity in combination with Pelargonium zonale extract against the human Corona 229E virus. Molecules 27:8362
Anitha R, Ramesh KV, Ravishankar TN, Kumar KS, Ramakrishnappa T (2018) Cytotoxicity, antibacterial and antifungal activities of ZnO nanoparticles prepared by the Artocarpus gomezianus fruit mediated facile green combustion method. J Sci Adv Mater Devices 3:440–451
Vahabirad M, Daei S, Abbasalipourkabir R, Ziamajidi N (2024) Anticancer Action of Silver Nanoparticles in SKBR3 Breast Cancer Cells through Promotion of Oxidative Stress and Apoptosis. Biomed Res Int 2024:7145339. https://doi.org/10.1155/2024/7145339
Stepankova H, Swiatkowski M, Kruszynski R, Svec P, Michalkova H et al (2021) The anti-proliferative activity of coordination compound-based ZnO nanoparticles as a promising agent against triple negative breast cancer cells. Int J Nanomedicine 1(16):4431–4449
Akhtar MJ, Ahamed M, Kumar S, Khan MM, Ahmad J, Alrokayan SA (2012) Zinc oxide nanoparticles selectively induce apoptosis in human cancer cells through reactive oxygen species. Int J Nanomedicine 7:845–857
CAS PubMed PubMed Central Google Scholar
Kc B, Paudel SN, Rayamajhi S, Karna D, Adhikari S, Shrestha BG, Bisht G (2016) Enhanced preferential cytotoxicity through surface modification: synthesis, characterization and comparative in vitro evaluation of TritonX-100 modified and unmodified zinc oxide nanoparticles in human breast cancer cell (MDA-MB-231). Chem Cen J 10:1–10
Razak NA, Abu N, Ho W, Zamberi NR, Tan SW, Alitheen NB, Long K, Yeap SK (2016) Cytotoxicity of eupatorine in MCF-7 and MDA-MB-231 human breast cancer cells via cell cycle arrest, anti-angiogenesis and induction of apoptosis. Sci Rep 9(1):1514
Abu-Huwaij R, Abbas MM, Al-Shalabi R, Almasri FN (2022) Synthesis of transdermal patches loaded with greenly synthesized zinc oxide nanoparticles and their cytotoxic activity against triple negative breast cancer. Appl Nanosci 12:69–78
Gurushankar K, Gohulkumar M, Prasad NR, Krishnakumar N (2013) Synthesis, characterization and in vitro anti-cancer evaluation of hesperetin-loaded nanoparticles in human oral carcinoma (KB) cells. Adv Nat Sci Nanosci Nanotechnol 5(1):01500
Umar H, Kavaz D, Rizaner N (2019) Biosynthesis of zinc oxide nanoparticles using Albizia lebbeck stem bark, and evaluation of its antimicrobial, antioxidant, and cytotoxic activities on human breast cancer cell lines. Int J Nanomedicine 2019:87–100
Ali KH, Ibraheem SA, Jabir MS, Ali KA, Taqi ZJ, Dan FM (2019) Zinc oxide nanoparticles induces apoptosis in human breast cancer cells via caspase-8 and P53 pathway. Nano Biomed Eng 11:35–43
Anjum S, Hashim M, Malik SA, Khan M, Lorenzo JM, Abbasi BH, Hano C (2021) Recent advances in zinc oxide nanoparticles (ZnO NPs) for cancer diagnosis, target drug delivery, and treatment. Cancers 13(18):4570
Wahab A, Waqas M, Urooj S, Tariq H, Abbasi ZY, Ali M, Abbasi BH (2023) Synthesis of zinc oxide nanoparticles (ZnONPs) from leaf extract of Carthamus oxycantha and its in vitro biological applications. Austin J Anal Pharm Chem 10(2):1159
Download references
Acknowledgements
The authors are grateful to Saveetha Dental College and Hospitals, Saveetha Institute of Medical and Technical Sciences, Saveetha University for providing necessary facilities to carry out the research work.
Author information
Authors and affiliations.
Saveetha Medical College, Saveetha Institute of Medical and Technical Sciences (SIMATS), Saveetha University, Chennai, Tamil Nadu, India
R. Kavipriya
Department of Prosthodontics, Saveetha Dental College and Hospitals, Saveetha Institute of Medical and Technical Sciences (SIMATS), Saveetha University, Chennai, Tamil Nadu, India
R. Ramasubburayan
You can also search for this author in PubMed Google Scholar
Contributions
RK: Methodology and Resources, RR: Conceptualization, Writing, Data Validation and Supervision.
Corresponding author
Correspondence to R. Ramasubburayan .
Ethics declarations
Conflict of interest.
The authors declare that they have no conflict of interest.
Ethical approval
The manuscript does not include any human or animal studies. Hence ethical approval is not applicable.
Additional information
Publisher's note.
Springer Nature remains neutral with regard to jurisdictional claims in published maps and institutional affiliations.
Supplementary Information
Below is the link to the electronic supplementary material.
Supplementary file1 (DOCX 667 KB)
Rights and permissions.
Springer Nature or its licensor (e.g. a society or other partner) holds exclusive rights to this article under a publishing agreement with the author(s) or other rightsholder(s); author self-archiving of the accepted manuscript version of this article is solely governed by the terms of such publishing agreement and applicable law.
Reprints and permissions
About this article
Kavipriya, R., Ramasubburayan, R. Phytofabrication of biocompatible zinc oxide nanoparticle using Gymnema sylvestre and its potent in vitro antibacterial, antibiofilm, and cytotoxicity against human breast cancer cells (MDA-MB-231). Bioprocess Biosyst Eng (2024). https://doi.org/10.1007/s00449-024-03035-y
Download citation
Received : 31 December 2023
Accepted : 21 May 2024
Published : 31 May 2024
DOI : https://doi.org/10.1007/s00449-024-03035-y
Share this article
Anyone you share the following link with will be able to read this content:
Sorry, a shareable link is not currently available for this article.
Provided by the Springer Nature SharedIt content-sharing initiative
- Gymnema sylvestre
- Biofilm inhibition
- Breast cancer cells
- Cytotoxicity
- Biocompatible
Advertisement
- Find a journal
- Publish with us
- Track your research

RSC Advances
Polyalthia longifolia -mediated green synthesis of zinc oxide nanoparticles: characterization, photocatalytic and antifungal activities †.

* Corresponding authors
a Interdisciplinary Nanotechnology Centre, Zakir Husain College of Engineering and Technology, Aligarh Muslim University, Aligarh 202002, India E-mail: [email protected]
b Unit for Environmental Sciences and Management, North-West University (Mafikeng Campus), Mmabatho 2735, South Africa
c Department of Plant Protection, Aligarh Muslim University, Aligarh 202002, India
d SSLD Varshney Institute of Management & Engineering, Aligarh 202001, India
e Department of Botany, Aligarh Muslim University, Aligarh 202002, India E-mail: [email protected]
f Department of Zoology, Aligarh Muslim University, Aligarh 202002, India
g Department of Botany, University of Delhi, New Delhi 110007, India
h Department of Biology, College of Science, King Khalid University, Abha 61413, Saudi Arabia
i Department of Botany and Microbiology, Faculty of Science, Assiut University, Assiut 71516, Egypt
The biological synthesis of zinc oxide nanoparticles (ZnO NPs) from plant extracts has emerged as a novel method for producing NPs with great scalability and biocompatibility. The present study is focused on bio-fabricated zinc oxide nanomaterial characterization and investigation of its photocatalytic and antifungal activities. ZnO NPs were biosynthesized using the leaf extract of Polyalthia longifolia without using harmful reducing or capping chemicals, which demonstrated fungicidal activity against Fusarium oxysporum f. sp. ciceris . The results showed that the inhibition of the radial growth of F . oxysporum f. sp. ciceris was enhanced as the concentration increased from 100 ppm to 300 ppm. The effectiveness of the photocatalytic activity of biosynthesized ZnO NPs was analyzed using MB dye degradation in aqueous medium under ultraviolet (UV) radiation and natural sunlight. After four consecutive cycles, the photocatalytic degradation of MB was stable and was 84%, 83%, 83%, and 83%, respectively, during natural sunlight exposure. Under the UV sources, degradation reached 92%, 89%, 88%, and 87%, respectively, in 90 minutes. This study suggests that the ZnO NPs obtained from plant extract have outstanding photocatalytic and antifungal activities against F. oxysporum f. sp. ciceris and have the potential for application as a natural pest control agent to reduce pathogenesis.
Supplementary files
- Supplementary information PDF (199K)
Article information

Download Citation
Permissions.

Polyalthia longifolia -mediated green synthesis of zinc oxide nanoparticles: characterization, photocatalytic and antifungal activities
A. Raza, P. Malan, I. Ahmad, A. Khan, M. Haris, Z. Zahid, Mohd. Jameel, A. Ahmad, C. S. Seth, T. A. Y. Asseri, M. Hashem and F. Ahmad, RSC Adv. , 2024, 14 , 17535 DOI: 10.1039/D4RA01035C
This article is licensed under a Creative Commons Attribution-NonCommercial 3.0 Unported Licence . You can use material from this article in other publications, without requesting further permission from the RSC, provided that the correct acknowledgement is given and it is not used for commercial purposes.
To request permission to reproduce material from this article in a commercial publication , please go to the Copyright Clearance Center request page .
If you are an author contributing to an RSC publication, you do not need to request permission provided correct acknowledgement is given.
If you are the author of this article, you do not need to request permission to reproduce figures and diagrams provided correct acknowledgement is given. If you want to reproduce the whole article in a third-party commercial publication (excluding your thesis/dissertation for which permission is not required) please go to the Copyright Clearance Center request page .
Read more about how to correctly acknowledge RSC content .
Social activity
Search articles by author, advertisements.
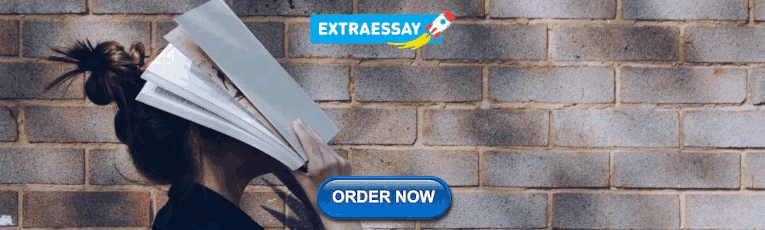
IMAGES
VIDEO
COMMENTS
The green synthesis of ZnO, CuO, and NiO nanoparticles was assessed by the rapid change in color of Azadirachta indica (neem) leaf extract solution from light green to yellowish, dark brown, and dark green within 15 min of the addition of ZnSO 4.7H 2 O, CuSO 4.5H 2 O, and Ni(NO 3) 2.6H 2 O, respectively. UV-Vis spectra could provide useful ...
A number of studies have shown that extracts from leaves, peels, roots, flowers, fruits, and seeds of plants can act as both reducing and stabilization agents for the synthesis of ZnO NPs . 5-16 The green synthesis of ZnO NPs from plant extracts results in excellent antibacterial activity against a variety of bacteria, 17-19 greater than ...
The green synthesis of nanoparticles offers a sustainable and environmentally friendly approach to nanomaterial production. However, its scalability prospects are contingent on addressing challenges related to resource availability, standardization, cost-effectiveness, regulatory compliance, technological advancements, and economic viability ...
This paper presents an efficient, environmentally friendly, and simple approach for the green synthesis of ZnO nanoparticles (ZnO NPs) using orange fruit peel extract. This approach aims to both minimize the use of toxic chemicals in nanoparticle fabrication and enhance the antibacterial activity and biomedical applications of ZnO nanoparticles.
Nanoparticles (NPs) exhibit distinct features compared to traditional physico-chemical synthesis and they have many applications in a wide range of fields of life sciences such as surface coating agents, catalysts, food packaging, corrosion protection, environmental remediation, electronics, biomedical and antimicrobial. Green-synthesized metal NPs, mainly from plant sources, have gained a lot ...
pattern of ZnO nanoparticles by green synthesis method. Broad diffraction peaks were observed for eco- friendly method with one extra peak attributed to plane (001) obtained at 2θ o value of 27. ...
Green synthesis of nanoparticles using plant extract is gaining importance as an alternative to conventional chemical and physical method of synthesis, because of its simplicity and environmental friendliness. In this research we synthesized Zinc oxide nanoparticle (ZnO.NPs) using plant extracts and deposited using spin- coating technique.
2.4. Synthesis of ZnO nanoparticles using aqueous broccoli extract. ZnO nanoparticles were prepared following a modification of a reported procedure (Citation 11). About 20 mL of the aqueous extract of broccoli and 0.05 M ZnCl 2 ·7H 2 O were continuously stirred for about 30 min. The solution was then transferred to a 100 mL conical flask and ...
However, when Aloe barbadensis 15 and Ocimum tenuiflorum 11 were used as reducing agent for the green synthesis of ZnO nanoparticles, the average nanoparticle sizes were 25-40 nm and 13.86 nm ...
The biosynthesis of algal-based zinc oxide (ZnO) nanoparticles has shown several advantages over traditional physico-chemical methods, such as lower cost, less toxicity, and greater sustainability. In the current study, bioactive molecules present in Spirogyra hyalina extract were exploited for the biofabrication and capping of ZnO NPs, using zinc acetate dihydrate and zinc nitrate hexahydrate ...
In the present work, bioaugmented zinc oxide nanoparticles (ZnO-NPs) were prepared from aqueous fruit extracts of Myristica fragrans. The ZnO-NPs were characterized by different techniques such as X-ray diffraction (XRD), Fourier transform infrared (FTIR) spectroscopy, ultraviolet (UV) spectroscopy, scanning electron microscopy (SEM), transmission electron microscopy (TEM), dynamic light ...
Zinc oxide (ZnO) has broad applications in various areas. Green synthesis is an alternative to conventional physical and chemical methods. Green synthesis of nanoparticles is gaining importance due to its cost-effectiveness, reduction of toxic chemicals and extensive antimicrobial activity. Herein, we have discovered synthesis of zinc oxide nanoparticles (ZnONPs) using agro waste materials ...
This study consists of a reliable process for synthesizing ZnO NPs by green method. Here, Eucalyptus globulus Labill. leaf extract is utilized as an efficient chelating and capping agent for synthesizing ZnO NPs from zinc nitrate hexahydrate salt. The plant ingredients, structure, morphology, thermal behavior, chemical composition and optical properties of ZnO nanoparticles were investigated ...
Iqbal, J. et al. synthesis of green and cost effective cobalt oxide nanoparticles using Geranium wallichianum leaves extract and evaluation of in vitro antioxidant, antimicrobial, cytotoxic and ...
The thesis is based on the synthesis and the main physical properties of pure ZnO ... quasi-spherical ZnO nanoparticles with an average crystallite size of 24.6 nm (at 300 °C), 27.2 nm (at 400 °C), 27.6 nm (at 500 °C), and 28.5 nm (at 600 °C) were found. ... needle-like leaves that were used in the green synthesis of ZnO nanoparticles ...
Keywords: green synthesis; ZnO NPs; characterization; antioxidant; ... harmful compounds for reduction and as a capping agent in the nanoparticle synthesis process causes a variety of adverse effects on the flora life as well as the environment and the living system toxicity. As a result, plant extracts (PEs) are therefore a more promising ...
"Green synthesis" of nanoparticles makes use of environmental friendly, non-toxic and safe reagents. To the best of our knowledge, biological approach using milky latex of Calotropis procera has been used for the first time as a reducing material as well as surface stabilizing agent for the synthesis of spherical-shaped ZnO-NPs.
The synthesis of Zinc oxide nanoparticles using a plant-mediated approach is presented in this paper. The nanoparticles were successfully synthesized using the Nitrate derivative of Zinc and plant extract of the indigenous medicinal plant Cayratia pedata. 0.1 mM of Zn (NO 3) 2.6H 2 O was made to react with the plant extract at different concentrations, and the reaction temperature was ...
[13] Vidya C et al. 2013 Green synthesis of ZnO nanoparticles by Calotropis Gigantea Int J Curr Eng Technol 28 2012-4. Go to reference in article; Crossref; Google Scholar [14] Qu J, Luo C and Hou J 2011 Synthesis of ZnO nanoparticles from Zn-hyperaccumulator (Sedum alfredii Hance) plants Micro Nano Lett. 6 174-6. Go to reference in article ...
In this paper, antibacterial activities of zinc oxide (ZnO) nanoparticles synthesized through green method using garlic bulb ( Allium sativum ), ginger ( Zingiber officinale ) extracts, and their mixture are reported. The synthesized ZnO NPs were characterized by X-ray diffraction (XRD), ultraviolet visible (UV-vis), photoluminescence (PL), spectroscopy and Fourier transform infrared (FTIR ...
Green Synthesis of ZnO Nanoparticles After heating twenty milliliters of P. odoratissimum leaf extract at 50 °C for 10 min, fifty milliliters of 0.1 M zinc acetate dihydrate (Zn(CH 3 COO) 2 ·2H 2 O) (1.095 g of zinc acetate dihydrate was dissolved in 50 mL of d.H 2 O) was added drop-by-drop to it under stirring at 800 rpm that resulted in ...
The biological synthesis of zinc oxide nanoparticles (ZnO NPs) from plant extracts has emerged as a novel method for producing NPs with great scalability and biocompatibility. The present study is focused on bio-fabricated zinc oxide nanomaterial characterization and investigation of its photocatalytic and antifungal activities.
The primary benefits of creating nanoparticles at room temperature and using plant extracts are that they help to partially complete a green synthesis (Gopinath et al. 2012; Singh et al. 2017). Physical, biological, chemical, and recently green methods create metal and metal oxide nanoparticles. Zinc oxide and ZnONPs have a significant
Khan, M. S. et al. Ultrasound-assisted green synthesis of ag-decorated ZnO nanoparticles Using Excoecaria agallocha leaf extract and evaluation of their photocatalytic and biological activity ...
Green synthesis method; provides a faster metallic nanoparticle production by offering an environmentally friendly, simple, economical and reproducible approach. Given the wide range of ...
The increasing incidence of breast cancer and bacterial biofilm in medical devices significantly heightens global mortality and morbidity, challenging synthetic drugs. Consequently, greener-synthesized nanomaterials have emerged as a versatile alternative for various biomedical applications, offering new therapeutic avenues. This study explores the synthesis of biocompatible zinc oxide (ZnONPs ...
(Ni-Mn) co-doped ZnO nanoparticles were synthesized for different doping concentrations (1 %, 2 %, and 3 %) and compared with the purely synthesized ZnO nanoparticles. Green synthesis route was followed to fabricate both pure and co-doped ZnO nanoparticles and the orange peel extract was used as the reducing agent. The main objectives of this research were to reduce the use of toxic ...
@article{Yadav2024SyzygiumAG, title={Syzygium aromaticum-Mediated Green Synthesis of Iron Oxide Nanoparticles for Efficient Heavy Metal Removal from Aqueous Solutions}, author={Jyoti Yadav and Pratima Chauhan and Ravindra Kumar Rawat and Saurabh Kumar Pathak and Sudhakar Srivastava}, journal={Journal of the Indian Chemical Society}, year={2024 ...
The biological synthesis of zinc oxide nanoparticles (ZnO NPs) from plant extracts has emerged as a novel method for producing NPs with great scalability and biocompatibility. The present study is focused on bio-fabricated zinc oxide nanomaterial characterization and investigation of its photocatalytic and antifungal activities.
Figure 5 (a) displays the FE-SEM image, which clearly depict the growth of spherical shaped ZnO nanoparticles on the RGO surface. The RGO sheets act as matrix for the nucleation and growth of ZnO nanoparticles at available anchoring sites. To determine the average particle size of the ZnO nanoparticles, ImageJ software is employed.