Log In | Join AACT | Renew Membership
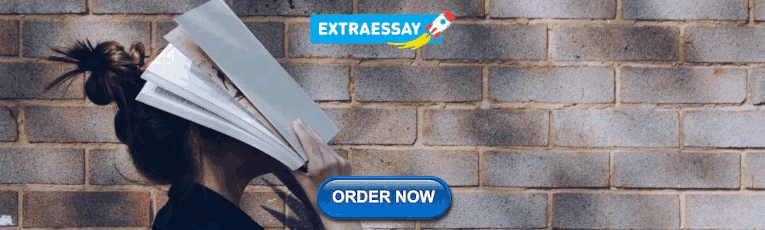
Save Your Favorite AACT Resources! ×
Log in or join now to start building your personalized "My Favorites" page. Easily save all the resources you love by logging in and clicking on the star icon next to any resource title.
- AACT member benefits »
- Forgot User Name or Password?
Radiocarbon Dating and Willard Libby Mark as Favorite (7 Favorites)
LESSON PLAN in History , Half Lives , Radioactive Isotopes , ACS National Historic Chemical Landmarks Program . Last updated August 31, 2022.
In this lesson, students will learn about the development and application of radiocarbon dating through an article reading. There are a series of activities to help promote literacy in the science classroom related to the reading. This lesson could be easily used as plans for a substitute teacher, as most of the activities are self-guided.
Grade Level
High School
NGSS Alignment
This lesson will help prepare your students to meet the performance expectations in the following standards:
- HS-PS1-8: Develop models to illustrate the changes in the composition of the nucleus of the atom and the energy released during the processes of fission, fusion, and radioactive decay.
- Using Mathematics and Computational Thinking
- Constructing Explanations and Designing Solutions
- Engaging in Argument from Evidence
- Obtaining, Evaluating, and Communicating Information
By the end of this lesson, students should be able to:
- Understand how carbon dating works.
- Learn the concept of half-lives.
- Recognize the factors that contribute to isotopes.
Chemistry Topics
This lesson supports students’ understanding of:
- Nuclear Chemistry
- Radioactive Isotopes
- Carbon Dating
Teacher Preparation : 10 minutes Lesson : Approximate times for students to complete each activity in the lesson:
- Anticipation guide: 10 minutes
- Reading: 20 minutes
- Timeline: 10–15 minutes
- Graphic organizer and writing: 15–20 minutes
- Nuclear equations: 20–30 minutes
- Jigsaw summary: 15–30 minutes
- Reading document and desired handouts to accompany the reading.
- A computer with internet access for the Jigsaw summary.
- No specific safety precautions need to be observed for this activity.
Teacher Notes
Background:
- This lesson plan was originally developed through the American Chemical Society’s National Historic Chemical Landmarks Program . Under this program, ACS grants Landmark status to seminal achievements in the history of the chemical sciences and provides a record of their contributions to chemistry and society in the United States.
Lesson Overview :
The lesson includes multiple components as outlined individually below. The Reading is essential for all of the activities. Teachers can choose to do one or all of the included activities. Student handouts and corresponding answer keys are provided for each item described below:
- Have students identify whether they agree or disagree with the ten statements. After they complete the reading, they can adjust their answers and rephrase “disagree” statements so they read true.
- Ask students to share when they think the method of radiocarbon dating was developed and what technical obstacles the scientist who developed it might have had to overcome.
- After this discussion, invite students to read the article to find more details about how Willard Libby developed the method of radiocarbon dating and how the method can be used.
- Reading : Radiocarbon Dating and Willard Libby
- Students are asked to develop a timeline by arranging events in chronological order. Students could do this before the reading and then adjust the order of events once they read the article and learn more about the discovery of 14 C.
- Students sort artifacts into those that can and can’t be dated using radiocarbon dating methods, then they identify how different kinds of scientists might use radiocarbon dating. Finally, they identify challenges and assumptions that Libby had to make when doing his work.
- Students write nuclear equations for the formation and decay of 14 C and extend that understanding to other types of nuclear equations.
- After reading the article, students work in groups on one of the suggested projects, then each group summarizes their results to share with the class in a 1-minute presentation
- Activity: The Demise of Frosty
- Activity: Why are Some Isotopes Radioactive?
- Activity: Radiological Applications of Isotopes
- Activity: Using Stable Isotopes to Determine Material Origin
- Activity: Graphical Analysis of Nuclear Decay
- Activity: Radioactive Decay and Seafloor Data
- What are Isotopes?
- National Historic Chemical Landmark: Willard Libby and Radiocarbon Dating
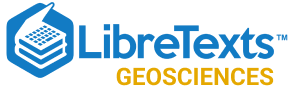
- school Campus Bookshelves
- menu_book Bookshelves
- perm_media Learning Objects
- login Login
- how_to_reg Request Instructor Account
- hub Instructor Commons
Margin Size
- Download Page (PDF)
- Download Full Book (PDF)
- Periodic Table
- Physics Constants
- Scientific Calculator
- Reference & Cite
- Tools expand_more
- Readability
selected template will load here
This action is not available.
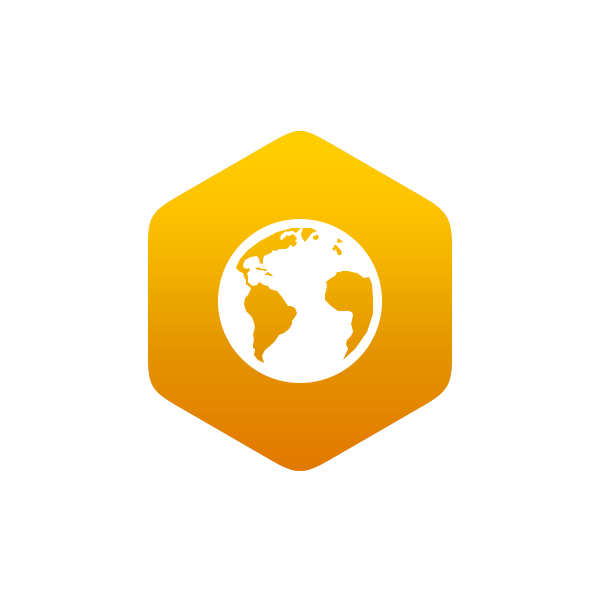
1.42: Radiocarbon Dating and Relative Dating
- Last updated
- Save as PDF
- Page ID 9764

- Miracosta Oceanography 101
\( \newcommand{\vecs}[1]{\overset { \scriptstyle \rightharpoonup} {\mathbf{#1}} } \)
\( \newcommand{\vecd}[1]{\overset{-\!-\!\rightharpoonup}{\vphantom{a}\smash {#1}}} \)
\( \newcommand{\id}{\mathrm{id}}\) \( \newcommand{\Span}{\mathrm{span}}\)
( \newcommand{\kernel}{\mathrm{null}\,}\) \( \newcommand{\range}{\mathrm{range}\,}\)
\( \newcommand{\RealPart}{\mathrm{Re}}\) \( \newcommand{\ImaginaryPart}{\mathrm{Im}}\)
\( \newcommand{\Argument}{\mathrm{Arg}}\) \( \newcommand{\norm}[1]{\| #1 \|}\)
\( \newcommand{\inner}[2]{\langle #1, #2 \rangle}\)
\( \newcommand{\Span}{\mathrm{span}}\)
\( \newcommand{\id}{\mathrm{id}}\)
\( \newcommand{\kernel}{\mathrm{null}\,}\)
\( \newcommand{\range}{\mathrm{range}\,}\)
\( \newcommand{\RealPart}{\mathrm{Re}}\)
\( \newcommand{\ImaginaryPart}{\mathrm{Im}}\)
\( \newcommand{\Argument}{\mathrm{Arg}}\)
\( \newcommand{\norm}[1]{\| #1 \|}\)
\( \newcommand{\Span}{\mathrm{span}}\) \( \newcommand{\AA}{\unicode[.8,0]{x212B}}\)
\( \newcommand{\vectorA}[1]{\vec{#1}} % arrow\)
\( \newcommand{\vectorAt}[1]{\vec{\text{#1}}} % arrow\)
\( \newcommand{\vectorB}[1]{\overset { \scriptstyle \rightharpoonup} {\mathbf{#1}} } \)
\( \newcommand{\vectorC}[1]{\textbf{#1}} \)
\( \newcommand{\vectorD}[1]{\overrightarrow{#1}} \)
\( \newcommand{\vectorDt}[1]{\overrightarrow{\text{#1}}} \)
\( \newcommand{\vectE}[1]{\overset{-\!-\!\rightharpoonup}{\vphantom{a}\smash{\mathbf {#1}}}} \)
Radiocarbon Dating
Radiocarbon dating is one of the most used method of absolute dating because of its useful dating window encompassing the past 100,000 years (it is especially useful for studying archeological features and young sedimentary deposits). 14 C (isotope carbon -14) is a unstable radioactive isotope (radionuclide). Radiocarbon dating (using ratios of the isotopes of radioactive isotope 14 C to stable isotopes 12 C and 13 C derived from buried or isolated organic or carbonate materials. The half life of 14 C [unstable isotope carbon-14] is about 5,730 years . Radiocarbon dating has extensively used in archeological investigation and the study of climate change over the last several hundred thousand years, and precision methods now available make radiocarbon dating highly reliable. Radiocarbon dating is highly effective for extracting ages of organic materials (bone, tissues, wood, etc.) that have been isolated by burial and is effective for dating materials materials from ancient human activities going back for many thousands of years.
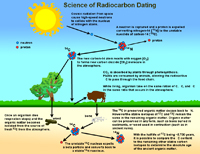
Relative Dating
Relative dating is the science of determining the relative order of past events, without necessarily determining their absolute age (see above). Relative dating involved the study of fossils and the correlation or comparison of fossils of similar ages but from different regions where their age is known. Microfossils derived from sediments and cores from wells help in the subsurface exploration for oil and gas. Relative dating is useful and relatively easy compared with absolute dating • Not all rocks can be dated with radioactivity (see above). • This is the way we tell the ages of rock layers relative to each other.
Basic Geologic Principles Used For Relative Dating
These basic principles are easily observed in geologic outcrops, but have value for any number of scientific and technical applications beyond geology. Figure 1.105 and 1-106 illustrates the four laws that are used in resolving the age of rocks and the order in which they formed or geologic events occurred. The three laws are as follows: Law of Original Horizontality —this law states that most sediments, when originally formed, were generally laid down horizontally. However, many layered rocks are no longer horizontal. Law of Superposition —this law states that in any undisturbed sequence of rocks deposited in layers, and the oldest on bottom the youngest layer is on top. Each layer being younger than the one beneath it and older than the one above it. Law of Cross-Cutting Relationships —this law states that a body of igneous rock (an intrusion), a fault, or other geologic feature must be younger than any rock across which it cuts through. Law of Inclusions • An inclusion is a piece of rock within another rock. • The rock containing the inclusion is younger
Radiocarbon Dating and Chronology
Radiocarbon dating is one of most important techniques used by archaeologists throughout the world. Here at Penn State, we are extremely privileged to have an accelerated mass spectrometry radiocarbon dating lab at the Institutes for Energy and the Environment (IEE) Energy and Environmental Sustainability Laboratories (EESL). Our lab fully engages with extensive use of this resource for dating specific materials that facilitate building archaeological and paleoenvironmental chronologies. In addition, we are involved in constructing databases and methodological resources that facilitate using sets of radiocarbon dates as part of meta-data analyses for various purposes including reconstruction of demographic processes, population dynamics, and migration processes over time.
Collaborators
- Dr. Brendan Culleton , Penn State
- Dr. Michael H. Price , Santa Fe Institute


Radiocarbon dating

Vol: 1 (2004) > Issue: 1 (October)
Primer | DOI: 10.1038/s43586-021-00058-7
- NEIF Radiocarbon Laboratory, Scottish Universities Environmental Research Centre, East Kilbride, UK
- Department of Integrated Biosciences, Graduate School of Frontier Sciences, The University of Tokyo, Chiba, Japan
- The University Museum, The University of Tokyo, Tokyo, Japan
- INFN (National Institute for Nuclear Physics) — Lecce Section, Lecce, Italy
- Radiocarbon Laboratory, Research School of Earth Sciences, The Australian National University, Canberra, Australian Capital Territory, Australia
- CEDAD — Centre for Applied Physics, Dating and Diagnostics, Department of Mathematics and Physics ‘Ennio de Giorgi’, University of Salento, Lecce, Italy
- Laboratory of Tree-Ring Research, University of Arizona, Tucson, AZ, USA
- Laboratory of Ion Beam Physics, ETH Zurich, Zurich, Switzerland
- Cell and Molecular Biology, Karolinska Institutet, Stockholm, Sweden
- Department of Geosciences, University of Arizona, Tucson, AZ, USA
- School of Anthropology, University of Arizona, Tucson, AZ, USA

Radiocarbon dating uses the decay of a radioactive isotope of carbon ( 14 C) to measure time and date objects containing carbon-bearing material. With a half-life of 5,700 ± 30 years, detection of 14 C is a useful tool for determining the age of a
Radiocarbon dating uses the decay of a radioactive isotope of carbon ( 14 C) to measure time and date objects containing carbon-bearing material. With a half-life of 5,700 ± 30 years, detection of 14 C is a useful tool for determining the age of a specimen formed over the past 55,000 years. In this Primer, we outline key advances in 14 C measurement and instrument capacity, as well as optimal sample selection and preparation. We discuss data processing, carbon reservoir age correction, calibration and statistical analyses. We then outline examples of radiocarbon dating across a range of applications, from anthropology and palaeoclimatology to forensics and medical science. Reproducibility and minimum reporting standards are discussed along with potential issues related to accuracy and sensitivity. Finally, we look forwards to the adoption of radiocarbon dating in various fields of research thanks to continued instrument improvement.
Figures ( 0 ) & Videos ( 0 )
Fig. 1 : Life cycle of 14C.
Fig. 2 : AMS radiocarbon analysis process.
Fig. 3 : Calibration curve and precision of calendar ages.
Fig. 4 : Calibration of radiocarbon ages.
Fig. 5 : Bayesian modelling in radiocarbon dating.
Fig. 6 : Radiocarbon dating of sedimentary records.
Fig. 7 : Distribution of 14C in the human body.
Fig. 8 : Radiocarbon analysis of cultural heritage items and detection of forgeries.
Experimental Specifications
Other keywords.

Citations (81)
Associated articles.
- Supplementary information
- Ruben, S. & Kamen, M. D. Radioactive carbon of long half-life. Phys. Rev. 57 , 549 (1940).
- Anderson, E. C. et al. Natural radiocarbon from cosmic radiation. Phys. Rev. 72 , 931–936 (1947).
- Arnold, J. R. & Libby, W. F. Age determinations by radiocarbon content — checks with samples of known age. Science 110 , 678–680 (1949).
- Libby, W. F., Anderson, E. C. & Arnold, J. R. Age determination by radiocarbon content — world-wide assay of natural radiocarbon. Science 109 , 227–228 (1949).
- Rom, W. et al. A detailed 2-year record of atmospheric (CO)-C-14 in the temperate northern hemisphere. Nucl. Instrum. Methods Phys. Res. Sect. B-Beam Interact. Mater. At. 161 , 780–785 (2000).
- Kutschera, W. Applications of accelerator mass spectrometry. Int. J. Mass. Spectrom. 349 , 203–218 (2013).
- Kutschera, W. The half-life of 14 C — why is it so long? Radiocarbon 61 , 1135–1142 (2019).
- Reimer, P. J. et al. The IntCal20 northern hemisphere radiocarbon age calibration curve (0–55 cal kBP). Radiocarbon 62 , 725–757 (2020).
- Hogg, A. G. et al. SHCal20 southern hemisphere calibration, 0–55,000 years cal BP. Radiocarbon 62 , 759–778 (2020).
- Heaton, T. J. et al. Marine20 — the marine radiocarbon age calibration curve (0–55,000 cal BP). Radiocarbon 62 , 779–820 (2020).
- Santos, G., Southon, J., Griffin, S., Beaupre, S. & Druffel, E. Ultra small-mass AMS 14 C sample preparation and analyses at KCCAMS/UCI facility. Nucl. Instrum. Methods Phys. Res. Sect. B: Beam Interact. Mater. At. 259 , 293–302 (2007).
- Ruff, M. et al. A gas ion source for radiocarbon measurements at 200 kV. Radiocarbon 49 , 307–314 (2007).
- Smith, A., Hua, Q., Williams, A., Levchenko, V. & Yang, B. Developments in micro-sample C-14 AMS at the ANTARES AMS facility. Nucl. Instrum. Methods Phys. Res. Sect. B-Beam Interact. Mater. At. 268 , 919–923 (2010).
- Steier, P., Liebl, J., Kutschera, W., Wild, E. M. & Golser, R. Preparation methods of µg carbon samples for 14 C measurements. Radiocarbon 59 , 803 (2017).
- Eglinton, T. I., Aluwihare, L. I., Bauer, J. E., Druffel, E. R. & McNichol, A. P. Gas chromatographic isolation of individual compounds from complex matrices for radiocarbon dating. Anal. Chem. 68 , 904–912 (1996).
- Reimer, P. J., Brown, T. A. & Reimer, R. W. Discussion: Reporting and calibration of post-bomb C-14 data. Radiocarbon 46 , 1299–1304 (2004).
- Stuiver, M. & Polach, H. A. Reporting of C-14 data — discussion. Radiocarbon 19 , 355–363 (1977).
- Mook, W. G. & van der Plicht, J. Reporting C-14 activities and concentrations. Radiocarbon 41 , 227–239 (1999).
- van der Plicht, J. & Hogg, A. A note on reporting radiocarbon. Quaternary Geochronol. 1 , 237–240 (2006).
- Stenström, K. E., Skog, G., Georgiadou, E., Genberg, J. & Johansson, A. A Guide to Radiocarbon Units and Calculations Department of Physics Internal Report 1–17 (Lund University, 2011).
- Paterne, M., et al. in Paleoclimatology (eds Ramstein, G. et al.) 51–71 (Springer International, 2020).
- Libby, W. F. Radiocarbon dating. Chem. Brit 5 , 548–552 (1955).
- Kromer, B. & Muennich, K. O. in Radiocarbon After Four Decades. An Interdisciplinary Perspective (eds Taylor, R. E., Long, A. & Kra, R. S.) 184–197 (Springer Verlag, 1992).
- Polach, H. A. in Radiocarbon After Four Decades. An Interdisciplinary Perspective (eds Taylor, R. E., Long, A. & Kra, R. S.) 198–213 (Springer Verlag, 1992).
- Povinec, P. P., Litherland, A. & von Reden, K. F. Developments in radiocarbon technologies: from the Libby counter to compound-specific AMS analyses. Radiocarbon 51 , 45–78 (2009).
- Tudyka, K. et al. A low level liquid scintillation spectrometer with five counting modules for 14 C, 222 Rn and delayed coincidence measurements. Radiat. Meas. 105 , 1–6 (2017).
- Theodórsson, P. et al. in LSC 2008, Advances in Liquid Scintillation Spectrometry (eds Eikenberg, J., Jäggi, M., Beer, H. & Baehrle, H.) 253–260 (Packard Instrument Company, 2009).
- Quiles, A., Kamal, N. S., Abd’el Fatah, M. & Mounir, N. The IFAO Radiocarbon Laboratory: a status report. Radiocarbon 59 , 1157 (2017).
- Gudelis, A., Gaigalaitė, L. & Gvozdaitė, R. Application of the absolute method for determination of tritium and radiocarbon in groundwater from radioactive waste facility. J. Radioanalytical Nucl. Chem. 322 , 1391–1396 (2019).
- Feng, B. et al. Application of synthetic benzoic acid technology in environmental radiocarbon monitoring. J. Environ. Radioactivity 216 , 106188 (2020).
- Cuchet, A. et al. Authentication of the naturalness of wintergreen ( Gaultheria genus ) essential oils by gas chromatography, isotope ratio mass spectrometry and radiocarbon assessment. Ind. Crop. Products 142 , 111873 (2019).
- Purser, K. H. et al. An attempt to detect stable N-ions from a sputter ion source and some implications of the results for the design of tandems for ultra-sensitive carbon analysis. Rev. de. Phys. Appliquee 12 , 1487–1492 (1977).
- Nelson, D. E., Korteling, R. G. & Stott, W. R. Carbon-14: direct detection at natural concentrations. Science 198 , 507–508 (1977).
- Bennett, C. L. et al. Radiocarbon dating using electrostatic accelerators: negative ions provide the key. Science 198 , 508–510 (1977).
- Litherland, A., Zhao, X. L. & Kieser, W. Mass spectrometry with accelerators. Mass. Spectrometry Rev. 30 , 1037–1072 (2011).
- Synal, H.-A., Jacob, S. & Suter, M. The PSI/ETH small radiocarbon dating system. Nucl. Instrum. Methods Phys. Res. Sect. B: Beam Interact. Mater. At. 172 , 1–7 (2000).
- Synal, H.-A., Dobeli, M., Jacob, S., Stocker, M. & Suter, M. Radiocarbon AMS towards its low-energy limits. Nucl. Instrum. Methods Phys. Res. Sect. B: Beam Interact. Mater. At. 223–224 , 339–345 (2004).
- Synal, H. A., Schulze-Konig, T., Seiler, M., Suter, M. & Wacker, L. Mass spectrometric detection of radiocarbon for dating applications. Nucl. Instrum. Methods Phys. Res. Sect. B-Beam Interact. Mater. At. 294 , 349–352 (2013).
- Salehpour, M., Håkansson, K., Possnert, G., Wacker, L. & Synal, H.-A. Performance report for the low energy compact radiocarbon accelerator mass spectrometer at Uppsala University. Nucl. Instrum. Methods Phys. Res. Sect. B: Beam Interact. Mater. At. 371 , 360–364 (2016).
- Seiler, M., Maxeiner, S., Wacker, L. & Synal, H.-A. Status of mass spectrometric radiocarbon detection at ETHZ. Nucl. Instrum. Methods Phys. Res. Sect. B: Beam Interact. Mater. At. 361 , 245–249 (2015).
- Fahrni, S., Wacker, L., Synal, H.-A. & Szidat, S. Improving a gas ion source for 14 C AMS. Nucl. Instrum. Methods Phys. Res. Sect. B: Beam Interact. Mater. At. 294 , 320–327 (2013).
- Wacker, L. et al. A versatile gas interface for routine radiocarbon analysis with a gas ion source. Nucl. Instrum. Methods Phys. Res. Sect. B-Beam Interact. Mater. At. 294 , 315–319 (2013).
- Wacker, L., Lippold, J., Molnar, M. & Schulz, H. Towards radiocarbon dating of single foraminifera with a gas ion source. Nucl. Instrum. Methods Phys. Res. Sect. B-Beam Interact. Mater. At. 294 , 307–310 (2013).
- Haghipour, N. et al. Compound-specific radiocarbon analysis by elemental analyzer–accelerator mass spectrometry: precision and limitations. Anal. Chem. 91 , 2042–2049 (2019).
- Leonard, A., Castle, S., Burr, G. S., Lange, T. & Thomas, J. A wet oxidation method for AMS radiocarbon analysis of dissolved organic carbon in water. Radiocarbon 55 , 545–552 (2013).
- Molnar, M. et al. C-14 analysis of groundwater down to the millilitre level. Nucl. Instrum. Methods Phys. Res. Sect. B-Beam Interact. Mater. At. 294 , 573–576 (2013).
- Welte, C. et al. Laser ablation–accelerator mass spectrometry: an approach for rapid radiocarbon analyses of carbonate archives at high spatial resolution. Anal. Chem. 88 , 8570–8576 (2016).
- Freeman, S. P., Shanks, R. P., Donzel, X. & Gaubert, G. Radiocarbon positive-ion mass spectrometry. Nucl. Instrum. Methods Phys. Res. Sect. B: Beam Interact. Mater. At. 361 , 229–232 (2015).
- Bayliss, A. Rolling out revolution: using radiocarbon dating in archaeology. Radiocarbon 51 , 123–147 (2009).
- van der Plicht, J., Scott, E., Alekseev, A. & Zaitseva, G. Radiocarbon, the calibration curve and Scythian chronology. Impact Environ. Hum. Migr. Eurasia 42 , 45–61 (2004).
- Jull, A. Accelerator radiocarbon dating of art, textiles, and artifacts. Nucl. N. 41 , 30–38 (1998).
- Aitken, M. J. Archaeological dating using physical phenomena. Rep. Prog. Phys. 62 , 1333–1376 (1999).
- Boaretto, E. Radiocarbon and the archaeological record: an integrative approach for building an absolute chronology for the Late Bronze and Iron Ages of Israel. Radiocarbon 57 , 207–216 (2015).
- Brock, F., Higham, T. & Ramsey, C. B. Pre-screening techniques for identification of samples suitable for radiocarbon dating of poorly preserved bones. J. archaeological Sci. 37 , 855–865 (2010).
- D’Elia, M. et al. Evaluation of possible contamination sources in the 14 C analysis of bone samples by FTIR spectroscopy. Radiocarbon 49 , 201–210 (2007).
- Brock, F. et al. Testing the effectiveness of protocols for removal of common conservation treatments for radiocarbon dating. Radiocarbon 60 , 35–50 (2018).
- Devièse, T. et al. A multi-analytical approach using FTIR, GC/MS and Py-GC/MS revealed early evidence of embalming practices in Roman catacombs. Microchem. J. 133 , 49–59 (2017).
- Yizhaq, M. et al. Quality controlled radiocarbon dating of bones and charcoal from the early Pre-Pottery Neolithic B (PPNB) of Motza (Israel). Radiocarbon 47 , 193–206 (2005).
- Sponheimer, M. et al. Saving old bones: a non-destructive method for bone collagen prescreening. Sci. Rep. 9 , 1–7 (2019).
- Naito, Y. I., Yamane, M. & Kitagawa, H. A protocol for using attenuated total reflection Fourier-transform infrared spectroscopy for pre-screening ancient bone collagen prior to radiocarbon dating. Rapid Commun. Mass. Spectrometry 34 , e8720 (2020).
- Brock, F., Higham, T., Ditchfield, P. & Ramsey, C. B. Current pretreatment methods for AMS radiocarbon dating at the Oxford Radiocarbon Accelerator Unit (ORAU). Radiocarbon 52 , 103–112 (2010).
- Hajdas, I. The radiocarbon dating method and its applications in Quaternary studies. Quaternary Sci. J. -Eiszeitalt. und Ggw. 57 , 2–24 (2008).
- Ingalls, A. E. & Pearson, A. Compound-specific radiocarbon analysis. Oceanography 18 , 18–31 (2005).
- Ingalls, A. E. et al. HPLC purification of higher plant-derived lignin phenols for compound specific radiocarbon analysis. Anal. Chem. 82 , 8931–8938 (2010).
- Ishikawa, N. F. et al. Improved method for isolation and purification of underivatized amino acids for radiocarbon analysis. Anal. Chem. 90 , 12035–12041 (2018).
- Blattmann, T. M., Montluçon, D. B., Haghipour, N., Ishikawa, N. F. & Eglinton, T. I. Liquid chromatographic isolation of individual amino acids extracted from sediments for radiocarbon analysis. Front. Mar. Sci. 7 , 174 (2020).
- Mann, W. B. An international reference material for radiocarbon dating. Radiocarbon 25 , 519–527 (1983).
- Rozanski, K. et al. The IAEA 14 C intercomparison exercise 1990. Radiocarbon 34 , 506–519 (1992).
- Scott, E. M., Naysmith, P. & Cook, G. T. Why do we need 14 C inter-comparisons?: the Glasgow- 14 C inter-comparison series, a reflection over 30 years. Quaternary Geochronol. 43 , 72–82 (2018).
- Huysecom, E., Hajdas, I., Renold, M.-A., Synal, H.-A. & Mayor, A. The “enhancement” of cultural heritage by AMS dating: ethical questions and practical proposals. Radiocarbon 59 , 559–563 (2017).
- Hajdas, I. et al. Radiocarbon dating and the protection of cultural heritage. Radiocarbon 61 , 1133–1134 (2019).
- Freedman, J., van Dorp, L. & Brace, S. Destructive sampling natural science collections: an overview for museum professionals and researchers. J. Nat. Sci. Collect. 5 , 21–34 (2018).
- American Institute for Conservation of Historic & Artistic Works (AIC). Code of Ethics and Guidelines for Practice. American Institute for Conservation (1994). https://www.culturalheritage.org/about-conservation/code-of-ethics
- Séguin, F. H., Schneider, R. J., Jones, G. A. & Karl, F. Optimized data analysis for AMS radiocarbon dating. Nucl. Instrum. Methods Phys. Res. Sect. B: Beam Interact. Mater. At. 92 , 176–181 (1994).
- Rom, W. et al. Systematic investigations of 14 C measurements at the Vienna Environmental Research Accelerator. Radiocarbon 40 , 255–263 (1997).
- McNichol, A. P., Jull, A. J. T. & Burr, G. S. Converting AMS data to radiocarbon values: considerations and conventions. Radiocarbon 43 , 313–320 (2001).
- Wacker, L., Christl, M. & Synal, H. A. BATS: a new tool for AMS data reduction. Nucl. Instrum. Methods Phys. Res. Sect. B-Beam Interact. Mater. At. 268 , 976–979 (2010).
- Craig, H. Carbon-13 in plants and the relationships between carbon-13 and carbon-14 variations in nature. J. Geol. 62 , 115–149 (1954).
- Welte, C. et al. Towards the limits: analysis of microscale 14 C samples using EA–AMS. Nucl. Instrum. Methods Phys. Res. Sect. B: Beam Interact. Mater. At. 437 , 66–74 (2018).
- Santos, G. M. et al. AMS C-14 sample preparation at the KCCAMS/UCI facility: status report and performance of small samples. Radiocarbon 49 , 255–269 (2007).
- Sookdeo, A. et al. Quality dating: a well-defined protocol implemented at ETH for high-precision 14 C-dates tested on late glacial wood. Radiocarbon 62 , 891–899 (2020).
- Alves, E. Q., Macario, K., Ascough, P. & Bronk Ramsey, C. The worldwide marine radiocarbon reservoir effect: definitions, mechanisms, and prospects. Rev. Geophysics 56 , 278–305 (2018).
- Stuiver, M., Pearson, G. W. & Braziunas, T. Radiocarbon age calibration of marine samples back to 9000 cal yr BP. Radiocarbon 28 , 980–1021 (1986).
- Ascough, P., Cook, G. & Dugmore, A. Methodological approaches to determining the marine radiocarbon reservoir effect. Prog. Phys. Geogr. 29 , 532–547 (2005).
- Reimer, P. J. & Reimer, R. W. A marine reservoir correction database and on-line interface. Radiocarbon 43 , 461–463 (2001).
- Philippsen, B. The freshwater reservoir effect in radiocarbon dating. Herit. Sci. 1 , 1–19 (2013).
- Keaveney, E. M. & Reimer, P. J. Understanding the variability in freshwater radiocarbon reservoir offsets: a cautionary tale. J. Archaeological Sci. 39 , 1306–1316 (2012).
- Cook, G. T. et al. Best practice methodology for 14 C calibration of marine and mixed terrestrial/marine samples. Quaternary Geochronol. 27 , 164–171 (2015).
- Arneborg, J. et al. Change of diet of the Greenland Vikings determined from stable carbon isotope analysis and 14 C dating of their bones. Radiocarbon 41 , 157–168 (1999).
- MacAvoy, S. E., Macko, S. A. & Garman, G. C. Tracing marine biomass into tidal freshwater ecosystems using stable sulfur isotopes. Sci. Nat. 85 , 544–546 (1998).
- Beesley, L. S. et al. New insights into the food web of an Australian tropical river to inform water resource management. Sci. Rep. 10 , 1–12 (2020).
- Ascough, P. L. et al. Radiocarbon reservoir effects in human bone collagen from northern Iceland. J. Archaeological Sci. 39 , 2261–2271 (2012).
- Fernandes, R., Millard, A. R., Brabec, M., Nadeau, M.-J. & Grootes, P. Food Reconstruction Using Isotopic Transferred Signals (FRUITS): a Bayesian model for diet reconstruction. PLoS ONE 9 , e87436 (2014).
- Cheng, H. et al. Atmospheric 14 C/ 12 C changes during the last glacial period from Hulu Cave. Science 362 , 1293–1297 (2018).
- Reimer, P. J. et al. Selection and treatment of data for radiocarbon calibration: an update to the International Calibration (IntCal) criteria. Radiocarbon 55 , 1923–1945 (2013).
- Lechleitner, F. A. et al. Hydrological and climatological controls on radiocarbon concentrations in a tropical stalagmite. Geochimica Cosmochimica Acta 194 , 233–252 (2016).
- Yeman, C. et al. Unravelling quasi-continuous 14 C profiles by laser ablation AMS. Radiocarbon 62 , 453–465 (2020).
- Ramsey, C. OxCal 4.4. University of Oxford (2021). https://c14.arch.ox.ac.uk/oxcal.html
- Stuiver, M., Reimer, P. J. & Reimer, R. W. CALIB 8.2. IntCal (2021). http://calib.org/
- Santos, G. M., Granato-Souza, D., Barbosa, A. C., Oelkers, R. & Andreu-Hayles, L. Radiocarbon analysis confirms annual periodicity in Cedrela odorata tree rings from the equatorial Amazon. Quaternary Geochronol. 58 , 101079 (2020).
- Hua, Q., Barbetti, M. & Rakowski, A. Z. Atmospheric radiocarbon for the period 1950–2010. Radiocarbon 55 , 2059–2072 (2013).
- Buck, C. E., Kenworthy, J. B., Litton, C. D. & Smith, A. F. Combining archaeological and radiocarbon information: a Bayesian approach to calibration. Antiquity 65 , 808 (1991).
- Ramsey, C. B. Bayesian analysis of radiocarbon dates. Radiocarbon 51 , 337–360 (2009).
- van de Schoot, R. et al. Bayesian statistics and modelling. Nat. Rev. Methods Prim. 1 , 1 (2021).
- Hajdas, I. & Michczynski, A. Age-depth model of Lake Soppensee (Switzerland) based on the high-resolution C-14 chronology compared with varve chronology. Radiocarbon 52 , 1027–1040 (2010).
- Buck, C. E. & Meson, B. On being a good Bayesian. World Archaeol. 47 , 567–584 (2015).
- Ramsey, C. B. Radiocarbon calibration and analysis of stratigraphy: the OxCal program. Radiocarbon 37 , 425–430 (1995).
- Buck, C. E., Christen, J. A. & James, G. N. BCal: an on-line Bayesian radiocarbon calibration tool. Internet Archaeology 7 , 1192–1201 (1999).
- Lougheed, B. & Obrochta, S. MatCal: open source Bayesian 14 C age calibration in MatLab. J. Open Res. Softw. 4 , e42 (2016).
- Lanos, P. & Dufresne, P. ChronoModel version 2.0 User manual. scanR (2019). https://scanr.enseignementsup-recherche.gouv.fr/publication/hal-02427428
- Renfrew, C. Before Civilization (Random House, 2011).
- Ramsey, C. B., Schulting, R., Bazaliiskii, V., Goriunova, O. & Weber, A. Spatio-temporal patterns of cemetery use among Middle Holocene hunter-gatherers of Cis-Baikal, East Siberia. Archaeol. Res. Asia 25 , 100253 (2021).
- Wood, R. From revolution to convention: the past, present and future of radiocarbon dating. J. Archaeological Sci. 56 , 61–72 (2015).
- Wood, R. et al. Testing the ABOx-SC method: dating known-age charcoals associated with the Campanian Ignimbrite. Quaternary Geochronol. 9 , 16–26 (2012).
- Jacobi, R. M., Higham, T. F. G. & Ramsey, C. B. AMS radiocarbon dating of Middle and Upper Palaeolithic bone in the British Isles: improved reliability using ultrafiltration. J. Quaternary Sci. 21 , 557–573 (2006).
- Cersoy, S. et al. Radiocarbon dating minute amounts of bone (3–60 mg) with ECHoMICADAS. Sci. Rep. 7 , 1–8 (2017).
- Fewlass, H. et al. Size matters: radiocarbon dates of Radiocarbon 60 , 425–439 (2017).
- Fewlass, H. et al. Pretreatment and gaseous radiocarbon dating of 40–100 mg archaeological bone. Sci. Rep. 9 , 1–11 (2019).
- Douka, K., Hedges, R. E. & Higham, T. F. Improved AMS 14 C dating of shell carbonates using high-precision X-ray diffraction and a novel density separation protocol (CarDS). Radiocarbon 52 , 735–751 (2010).
- Marom, A., McCullagh, J. S., Higham, T. F., Sinitsyn, A. A. & Hedges, R. E. Single amino acid radiocarbon dating of Upper Paleolithic modern humans. Proc. Natl Acad. Sci. USA 109 , 6878–6881 (2012).
- Deviese, T. et al. Increasing accuracy for the radiocarbon dating of sites occupied by the first Americans. Quaternary Sci. Rev. 198 , 171–180 (2018).
- Higham, T. et al. The timing and spatiotemporal patterning of Neanderthal disappearance. Nature 512 , 306–309 (2014).
- Fewlass, H. et al. A 14 C chronology for the Middle to Upper Palaeolithic transition at Bacho Kiro Cave, Bulgaria. Nat. Ecol. Evolution 4 , 794–801 (2020).
- Spindler, L. et al. Dating the last Middle Palaeolithic of the Crimean Peninsula: new hydroxyproline AMS dates from the site of Kabazi II. J. Hum. Evolution 156 , 102996 (2021).
- Berstan, R. et al. Direct dating of pottery from its organic residues: new precision using compound-specific carbon isotopes. Antiquity 82 , 702–713 (2008).
- Casanova, E. et al. Accurate compound-specific 14 C dating of archaeological pottery vessels. Nature 580 , 506–510 (2020).
- Zuo, X. et al. Dating rice remains through phytolith carbon-14 study reveals domestication at the beginning of the Holocene. Proc. Natl Acad. Sci. USA 114 , 6486–6491 (2017).
- Boaretto, E. Dating materials in good archaeological contexts: the next challenge for radiocarbon analysis. Radiocarbon 51 , 275–281 (2009).
- Santos, G. M. et al. The phytolith 14 C puzzle: a tale of background determinations and accuracy tests. Radiocarbon 52 , 113–128 (2010).
- Santos, G. M., Masion, A. & Alexandre, A. When the carbon being dated is not what you think it is: insights from phytolith carbon research. Quaternary Sci. Rev. 197 , 162–174 (2018).
- Charlton, S. et al. Finding Britain’s last hunter-gatherers: a new biomolecular approach to ‘unidentifiable’ bone fragments utilising bone collagen. J. Archaeological Sci. 73 , 55–61 (2016).
- Devièse, T. et al. Compound-specific radiocarbon dating and mitochondrial DNA analysis of the Pleistocene hominin from Salkhit Mongolia. Nat. Commun. 10 , 1–7 (2019).
- Zeidler, J. A., Buck, C. E. & Litton, C. D. Integration of archaeological phase information and radiocarbon results from the Jama River Valley, Ecuador: a Bayesian approach. Latin American Antiquity 9 , 160–179 (1998).
- Crema, E. R. & Kobayashi, K. I. A multi-proxy inference of Jōmon population dynamics using bayesian phase models, residential data, and summed probability distribution of 14 C dates. J. Archaeological Sci. 117 , 105136 (2020).
- Gakuhari, T. et al. Radiocarbon dating of one human and two dog burials from the Kamikuroiwa rock shelter site, Ehime Prefecture. Anthropol. Sci. 123 , 87–94 (2015).
- Bayliss, A. et al. Getting to the bottom of it all: a Bayesian approach to dating the start of Çatalhöyük. J. World Prehistory 28 , 1–26 (2015).
- Regev, J. et al. Radiocarbon dating and microarchaeology untangle the history of Jerusalem’s Temple Mount: a view from Wilson’s Arch. PLoS ONE 15 , e0233307 (2020).
- Piotrowska, N., Blaauw, M., Mauquoy, D. & Chambers, F. M. Constructing deposition chronologies for peat deposits using radiocarbon dating. Mires Peat 7 , 1–14 (2011).
- Francisquini, M. I. et al. Cold and humid Atlantic Rainforest during the last glacial maximum, northern Espírito Santo state, southeastern Brazil. Quaternary Sci. Rev. 244 , 106489 (2020).
- Staff, R. et al. The multiple chronological techniques applied to the Lake Suigetsu SG06 sediment core, central Japan. Boreas 42 , 259–266 (2013).
- Broecker, W. et al. Ventilation of the glacial deep Pacific Ocean. Science 306 , 1169–1172 (2004).
- Sikes, E. L., Samson, C. R., Guilderson, T. P. & Howard, W. R. Old radiocarbon ages in the southwest Pacific Ocean during the last glacial period and deglaciation. Nature 405 , 555–559 (2000).
- Joerin, U., Nicolussi, K., Fischer, A., Stocker, T. & Schluchter, C. Holocene optimum events inferred from subglacial sediments at Tschierva Glacier, Eastern Swiss Alps. Quaternary Sci. Rev. 27 , 337–350 (2008).
- Bolton, A., Goodkin, N. F., Druffel, E. R. M., Griffin, S. & Murty, S. A. Upwelling of Pacific intermediate water in the South China Sea revealed by coral radiocarbon record. Radiocarbon 58 , 37–53 (2016).
- Hirabayashi, S. et al. Insight into Western Pacific circulation from South China Sea coral skeletal radiocarbon. Radiocarbon 61 , 1923–1937 (2019).
- Hua, Q. et al. Robust chronological reconstruction for young speleothems using radiocarbon. Quaternary Geochronol. 14 , 67–80 (2012).
- Akers, P. D. et al. Integrating U-Th, 14 C, and 210 Pb methods to produce a chronologically reliable isotope record for the Belize River Valley Maya from a low-uranium stalagmite. Holocene 29 , 1234–1248 (2019).
- Therre, S. et al. Climate-induced speleothem radiocarbon variability on Socotra Island from the Last Glacial Maximum to the Younger Dryas. Clim. Past. 16 , 409–421 (2020).
- Moska, P., Jary, Z., Adamiec, G. & Bluszcz, A. Chronostratigraphy of a loess–palaeosol sequence in Biały Kościół, Poland using OSL and radiocarbon dating. Quaternary Int. 502 , 4–17 (2019).
- Pigati, J. S., McGeehin, J. P., Muhs, D. R., Grimley, D. A. & Nekola, J. C. Radiocarbon dating loess deposits in the Mississippi Valley using terrestrial gastropod shells (Polygyridae, Helicinidae, and Discidae). Aeolian Res. 16 , 25–33 (2015).
- Moine, O. et al. The impact of Last Glacial climate variability in west-European loess revealed by radiocarbon dating of fossil earthworm granules. Proc. Natl Acad. Sci. USA 114 , 6209–6214 (2017).
- Bond, G. et al. Correlations between climate records from North-Atlantic sediments and Greenland ice. Nature 365 , 143–147 (1993).
- Grimm, E. C. et al. Evidence for warm wet Heinrich events in Florida. Quaternary Sci. Rev. 25 , 2197–2211 (2006).
- Prokopenko, A. A., Williams, D. F., Karabanov, E. B. & Khursevich, G. K. Continental response to Heinrich events and Bond cycles in sedimentary record of Lake Baikal, Siberia. Glob. Planet. Change 28 , 217–226 (2001).
- Obrochta, S. et al. Mt. Fuji Holocene eruption history reconstructed from proximal lake sediments and high-density radiocarbon dating. Quaternary Sci. Rev. 200 , 395–405 (2018).
- Vandergoes, M. et al. A revised age for the Kawakawa/Oruanui tephra, a key marker for the Last Glacial Maximum in New Zealand. Quaternary Sci. Rev. 74 , 195–201 (2013).
- Sevink, J., Bakels, C., Van Hall, R. & Dee, M. Radiocarbon dating distal tephra from the Early Bronze Age Avellino eruption (EU-5) in the coastal basins of southern Lazio (Italy): uncertainties, results, and implications for dating distal tephra. Quaternary Geochronol. 63 , 101154 (2021).
- Danišík, M. et al. Sub-millennial eruptive recurrence in the silicic Mangaone Subgroup tephra sequence, New Zealand, from Bayesian modelling of zircon double-dating and radiocarbon ages. Quaternary Sci. Rev. 246 , 106517 (2020).
- Nicolussi, K., Spötl, C., Thurner, A. & Reimer, P. J. Precise radiocarbon dating of the giant Köfels landslide (Eastern Alps, Austria). Geomorphology 243 , 87–91 (2015).
- Bertolini, G., Casagli, N., Ermini, L. & Malaguti, C. Radiocarbon data on Lateglacial and Holocene landslides in the Northern Apennines. Nat. Hazards 31 , 645–662 (2004).
- Borgatti, L. & Soldati, M. Landslides as a geomorphological proxy for climate change: a record from the Dolomites (northern Italy). Geomorphology 120 , 56–64 (2010).
- Talling, P. J. Fidelity of turbidites as earthquake records. Nat. Geosci. 14 , 113–116 (2021).
- Shirahama, Y. et al. Detailed paleoseismic history of the Hinagu fault zone revealed by the high-density radiocarbon dating and trenching survey across a surface rupture of the 2016 Kumamoto earthquake, Kyushu, Japan. Isl. Arc 30 , e12376 (2021).
- Ishizawa, T. et al. Sequential radiocarbon measurement of bulk peat for high-precision dating of tsunami deposits. Quaternary Geochronol. 41 , 202–210 (2017).
- Bondevik, S., Stormo, S. K. & Skjerdal, G. Green mosses date the Storegga tsunami to the chilliest decades of the 8.2 ka cold event. Quaternary Sci. Rev. 45 , 1–6 (2012).
- Williams, M. et al. Late Quaternary floods and droughts in the Nile valley, Sudan: new evidence from optically stimulated luminescence and AMS radiocarbon dating. Quaternary Sci. Rev. 29 , 1116–1137 (2010).
- Shen, H., Yu, L., Zhang, H., Zhao, M. & Lai, Z. OSL and radiocarbon dating of flood deposits and its paleoclimatic and archaeological implications in the Yihe River Basin, East China. Quaternary Geochronol. 30 , 398–404 (2015).
- Miyake, F., Nagaya, K., Masuda, K. & Nakamura, T. A signature of cosmic-ray increase in AD 774–775 from tree rings in Japan. Nature 486 , 240–242 (2012).
- Miyake, F. et al. Search for annual C-14 excursions in the past. Radiocarbon 59 , 315–320 (2017).
- Miyake, F., Masuda, K. & Nakamura, T. Another rapid event in the carbon-14 content of tree rings. Nat. Commun. 4 , 1–6 (2013).
- Miyake, F. et al. Large C-14 excursion in 5480 BC indicates an abnormal sun in the mid-Holocene. Proc. Natl Acad. Sci. USA 114 , 881–884 (2017).
- Quarta, G. et al. Identifying the 993–994 CE Miyake event in the oldest dated living tree in Europe. Radiocarbon 61 , 1317–1325 (2019).
- Park, J., Southon, J., Fahrni, S., Creasman, P. P. & Mewaldt, R. Relationship between solar activity and Δ 14 C peaks in AD 775, AD 994, and 660 BC. Radiocarbon 59 , 1147–1156 (2017).
- Dee, M. W. & Pope, B. J. Anchoring historical sequences using a new source of astro-chronological tie-points. Proc. R. Soc. A: Mathematical, Phys. Eng. Sci. 472 , 20160263 (2016).
- Wacker, L. et al. Radiocarbon dating to a single year by means of rapid atmospheric C-14 changes. Radiocarbon 56 , 573–579 (2014).
- Oppenheimer, C. et al. Multi-proxy dating the ‘Millennium Eruption’ of Changbaishan to late 946 CE. Quaternary Sci. Rev. 158 , 164–171 (2017).
- Mazaud, A. et al. Geomagnetic-assisted stratigraphy and sea surface temperature changes in core MD94-103 (Southern Indian Ocean): possible implications for north–south climatic relationships around H4. Earth Planet. Sci. Lett. 201 , 159–170 (2002).
- Cooper, A. et al. A global environmental crisis 42,000 years ago. Science 371 , 811–818 (2021).
- Lister, A. M. & Stuart, A. J. The extinction of the giant deer Megaloceros giganteus (Blumenbach): new radiocarbon evidence. Quaternary Int. 500 , 185–203 (2019).
- Kosintsev, P. et al. Evolution and extinction of the giant rhinoceros Elasmotherium sibiricum sheds light on late Quaternary megafaunal extinctions. Nat. Ecol. Evolution 3 , 31–38 (2019).
- Orlova, L. A., Kuzmin, Y. V. & Dementiev, V. N. A review of the evidence for extinction chronologies for five species of Upper Pleistocene megafauna in Siberia. Radiocarbon 46 , 301–314 (2004).
- Roberts, R. G. et al. New ages for the last Australian megafauna: continent-wide extinction about 46,000 years ago. Science 292 , 1888–1892 (2001).
- Miller, G. H. et al. Ecosystem collapse in Pleistocene Australia and a human role in megafaunal extinction. Science 309 , 287–290 (2005).
- Gillespie, R., Brook, B. W. & Baynes, A. Short overlap of humans and megafauna in Pleistocene Australia. Alcheringa: An. Australasian J. Palaeontology 30 , 163–186 (2006).
- Stewart, M., Carleton, W. C. & Groucutt, H. S. Climate change, not human population growth, correlates with Late Quaternary megafauna declines in North America. Nat. Commun. 12 , 1–15 (2021).
- Turnbull, J. C. et al. Sixty years of radiocarbon dioxide measurements at Wellington, New Zealand: 1954–2014. Atmos. Chem. Phys. 17 , 14771–14784 (2017).
- Levin, I. & Kromer, B. The tropospheric (CO 2 )-C-14 level in mid-latitudes of the northern hemisphere (1959–2003). Radiocarbon 46 , 1261–1272 (2004).
- Levin, I. et al. Observations and modelling of the global distribution and long-term trend of atmospheric 14 CO 2 . Tellus B: Chem. Phys. Meteorol. 62 , 26–46 (2010).
- Hammer, S. & Levin, I. Monthly mean atmospheric D 14 CO 2 at Jungfraujoch and Schauinsland from 1986 to 2016. Tellus B 65 , 20092 (2017).
- Hua, Q. & Barbetti, M. Influence of atmospheric circulation on regional (CO 2 )-C-14 differences. J. Geophys. Res. Atmos. 112 , D19102 (2007).
- Levin, I. & Hesshaimer, V. Radiocarbon — a unique tracer of global carbon cycle dynamics. Radiocarbon 42 , 69–80 (2000).
- Hua, Q., Barbetti, M., Worbes, M., Head, J. & Levchenko, V. Review of radiocarbon data from atmospheric and tree ring samples for the period 1945–1997 AD. Iawa J. 20 , 261–283 (1999).
- Santos, G. M., Linares, R., Lisi, C. S. & Tomazello Filho, M. Annual growth rings in a sample of Paraná pine ( Araucaria angustifolia ): toward improving the 14 C calibration curve for the southern hemisphere. Quaternary Geochronol. 25 , 96–103 (2015).
- Ancapichún, S. et al. Radiocarbon bomb-peak signal in tree-rings from the tropical Andes register low latitude atmospheric dynamics in the southern hemisphere. Sci. Total. Environ. 774 , 145126 (2021).
- Graven, H. D., Gruber, N., Key, R., Khatiwala, S. & Giraud, X. Changing controls on oceanic radiocarbon: new insights on shallow-to-deep ocean exchange and anthropogenic CO 2 uptake. J. Geophys. Res. Oceans 117 , C1005 (2012).
- Graven, H. D. Impact of fossil fuel emissions on atmospheric radiocarbon and various applications of radiocarbon over this century. Proc. Natl Acad. Sci. USA 112 , 9542–9545 (2015).
- Levin, I., Hammer, S., Kromer, B. & Meinhardt, F. Radiocarbon observations in atmospheric CO 2 : determining fossil fuel CO 2 over Europe using Jungfraujoch observations as background. Sci. Total. Environ. 391 , 211–216 (2008).
- Gamnitzer, U. et al. Carbon monoxide: a quantitative tracer for fossil fuel CO 2 ? J. Geophys. Res. Atmos. (2006). https://doi.org/10.1029/2005JD006966
- Graven, H., Hocking, T. & Zazzeri, G. Detection of fossil and biogenic methane at regional scales using atmospheric radiocarbon. Earth’s future 7 , 283–299 (2019).
- Xiong, X. et al. Time series of atmospheric Δ 14 CO 2 recorded in tree rings from Northwest China (1957–2015). Chemosphere 272 , 129921 (2021).
- Szidat, S. et al. Contributions of fossil fuel, biomass-burning, and biogenic emissions to carbonaceous aerosols in Zurich as traced by 14 C. J. Geophys. Res. Atmos. (2006). https://doi.org/10.1029/2005JD006590
- Lim, S. et al. Fossil-driven secondary inorganic PM2. 5 enhancement in the North China Plain: evidence from carbon and nitrogen isotopes. Environ. Pollut. 266 , 115163 (2020).
- Huang, L. et al. Application of the ECT9 protocol for radiocarbon-based source apportionment of carbonaceous aerosols. Atmos. Meas. Tech. 14 , 3481–3500 (2021).
- Dias, C. M. et al. 14 CO 2 dispersion around two PWR nuclear power plants in Brazil. J. Environ. Radioactivity 100 , 574–580 (2009).
- Major, I. et al. Source identification of PM2.5 carbonaceous aerosol using combined carbon fraction, radiocarbon and stable carbon isotope analyses in Debrecen, Hungary. Sci. Total Environ. 782 , 146520 (2021).
- Pabedinskas, A. et al. Assessment of the contamination by 14 C airborne releases in the vicinity of the Ignalina Nuclear Power Plant. Radiocarbon 61 , 1185–1197 (2019).
- Trumbore, S. Radiocarbon and soil carbon dynamics. Annu. Rev. Earth Planet. Sci. 37 , 47–66 (2009).
- Shi, Z. et al. The age distribution of global soil carbon inferred from radiocarbon measurements. Nat. Geosci. 13 , 555–559 (2020).
- Trumbore, S. E. & Zheng, S. Comparison of fractionation methods for soil organic matter 14 C analysis. Radiocarbon 38 , 219–229 (1996).
- Rethemeyer, J. et al. Transformation of organic matter in agricultural soils: radiocarbon concentration versus soil depth. Geoderma 128 , 94–105 (2005).
- Hemingway, J. D. et al. Mineral protection regulates long-term global preservation of natural organic carbon. Nature 570 , 228–231 (2019).
- Kuzyakov, Y., Bogomolova, I. & Glaser, B. Biochar stability in soil: decomposition during eight years and transformation as assessed by compound-specific 14 C analysis. Soil. Biol. Biochem. 70 , 229–236 (2014).
- Munksgaard, N. C. et al. Partitioning of microbially respired CO 2 between indigenous and exogenous carbon sources during biochar degradation using radiocarbon and stable carbon isotopes. Radiocarbon 61 , 573–586 (2019).
- Estop-Aragonés, C. et al. Assessing the potential for mobilization of old soil carbon after permafrost thaw: a synthesis of 14 C measurements from the northern permafrost region. Global Biogeochem. Cycles 34 , e2020GB006672 (2020).
- Moore, S. et al. Deep instability of deforested tropical peatlands revealed by fluvial organic carbon fluxes. Nature 493 , 660–663 (2013).
- Basu, S. et al. Estimating US fossil fuel CO 2 emissions from measurements of 14 C in atmospheric CO 2 . Proc. Natl Acad. Sci. USA 117 , 13300–13307 (2020).
- Cartwright, I., Currell, M. J., Cendón, D. I. & Meredith, K. T. A review of the use of radiocarbon to estimate groundwater residence times in semi-arid and arid areas. J. Hydrol. 580 , 124247 (2020).
- Hobbie, E. A., Weber, N. S., Trappe, J. M. & Van Klinken, G. J. Using radiocarbon to determine the mycorrhizal status of fungi. N. Phytologist 156 , 129–136 (2002).
- Newsham, K. K., Garnett, M. H., Robinson, C. H. & Cox, F. Discrete taxa of saprotrophic fungi respire different ages of carbon from Antarctic soils. Sci. Rep. 8 , 1–10 (2018).
- Briones, M. J. I. & Ineson, P. Use of 14 C carbon dating to determine feeding behaviour of enchytraeids. Soil Biology and Biochemistry 34 , 881–884 (2002).
- Ishikawa, N. F. et al. Combined use of radiocarbon and stable carbon isotopes for the source mixing model in a stream food web. Limnol. Oceanogr. 65 , 2688–2696 (2020).
- Wilson, S. A. et al. Assessing capture of atmospheric CO 2 within mine tailings using stable isotopes and C-14. Geochimica Cosmochimica Acta 74 , A1135–A1135 (2010).
- Wilson, S. A. et al. Offsetting of CO 2 emissions by air capture in mine tailings at the Mount Keith Nickel Mine, Western Australia: rates, controls and prospects for carbon neutral mining. Int. J. Greenh. Gas. Control. 25 , 121–140 (2014).
- Power, I. M. et al. Magnesite formation in playa environments near Atlin, British Columbia, Canada. Geochimica Cosmochimica Acta 255 , 1–24 (2019).
- Broecker, W. S. & Peng, T.-H. in Tracers in the Sea 690 (Eldigio, 1982).
- Broecker, W., Gerard, R., Ewing, M. & Heezen, B. C. Natural radiocarbon in the Atlantic Ocean. J. Geophys. Res. 65 , 2903–2931 (1960).
- Druffel, E. M. Radiocarbon in annual coral rings from the eastern tropical Pacific Ocean. Geophys. Res. Lett. 8 , 59–62 (1981).
- Nydal, R. Radiocarbon in the ocean. Radiocarb. 42 , 81–98 (2000).
- Key, R. M. et al. A global ocean carbon climatology: results from Global Data Analysis Project (GLODAP). Global Biogeochem. Cycles (2004). https://doi.org/10.1029/2004GB002247
- Guilderson, T. P., Caldeira, K. & Duffy, P. B. Radiocarbon as a diagnostic tracer in ocean and carbon cycle modeling. Global Biogeochem. Cycles 14 , 887–902 (2000).
- Barker, S., Knorr, G., Vautravers, M. J., Diz, P. & Skinner, L. C. Extreme deepening of the Atlantic overturning circulation during deglaciation. Nat. Geosci. 3 , 567–571 (2010).
- Skinner, L. C., Fallon, S., Waelbroeck, C., Michel, E. & Barker, S. Ventilation of the deep Southern Ocean and deglacial CO 2 rise. Science 328 , 1147–1151 (2010).
- Walczak, M. H. et al. Phasing of millennial-scale climate variability in the Pacific and Atlantic Oceans. Science 370 , 716 (2020).
- Burke, A. & Robinson, L. F. The Southern Ocean’s role in carbon exchange during the last deglaciation. Science 335 , 557–561 (2012).
- Guilderson, T. P. & Schrag, D. P. Abrupt shift in subsurface temperatures in the tropical Pacific associated with changes in El Niño. Science 281 , 240–243 (1998).
- McNichol, A. P. & Aluwihare, L. I. The power of radiocarbon in biogeochemical studies of the marine carbon cycle: insights from studies of dissolved and particulate organic carbon (DOC and POC). Chem. Rev. 107 , 443–466 (2007).
- Williams, P. M. & Druffel, E. R. Radiocarbon in dissolved organic matter in the central North Pacific Ocean. Nature 330 , 246–248 (1987).
- Bauer, J. E., Williams, P. M. & Druffel, E. R. 14 C activity of dissolved organic carbon fractions in the north-central Pacific and Sargasso Sea. Nature 357 , 667–670 (1992).
- Druffel, E. R. & Bauer, J. E. Radiocarbon distributions in Southern Ocean dissolved and particulate organic matter. Geophys. Res. Lett. 27 , 1495–1498 (2000).
- Ishikawa, N. F., Butman, D. & Raymond, P. A. Radiocarbon age of different photoreactive fractions of freshwater dissolved organic matter. Org. Geochem. 135 , 11–15 (2019).
- Beaupré, S. R., Walker, B. D. & Druffel, E. R. M. The two-component model coincidence: evaluating the validity of marine dissolved organic radiocarbon as a stable-conservative tracer at Station M. Deep. Sea Res. Part. II: Topical Stud. Oceanography 173 , 104737 (2020).
- Druffel, E. R. & Williams, P. M. Identification of a deep marine source of particulate organic carbon using bomb 14 C. Nature 347 , 172–174 (1990).
- Druffel, E. R., Bauer, J. E., Griffin, S. & Hwang, J. Penetration of anthropogenic carbon into organic particles of the deep ocean. Geophys. Res. Lett. (2003). https://doi.org/10.1029/2003GL017423
- Verdugo, P. et al. The oceanic gel phase: a bridge in the DOM–POM continuum. Mar. Chem. 92 , 67–85 (2004).
- Spalding, K. L., Bhardwaj, R. D., Buchholz, B. A., Druid, H. & Frisen, J. Retrospective birth dating of cells in humans. Cell 122 , 133–143 (2005).
- Bergmann, O. et al. The age of olfactory bulb neurons in humans. Neuron 74 , 634–639 (2012).
- Bhardwaj, R. D. et al. Neocortical neurogenesis in humans is restricted to development. Proc. Natl Acad. Sci. USA 103 , 12564–12568 (2006).
- Huttner, H. B. et al. The age and genomic integrity of neurons after cortical stroke in humans. Nat. Neurosci. 17 , 801–803 (2014).
- Spalding, K. L. et al. Dynamics of hippocampal neurogenesis in adult humans. Cell 153 , 1219–1227 (2013).
- Ernst, A. et al. Neurogenesis in the striatum of the adult human brain. Cell 156 , 1072–1083 (2014).
- Yeung, M. S. et al. Dynamics of oligodendrocyte generation and myelination in the human brain. Cell 159 , 766–774 (2014).
- Réu, P. et al. The lifespan and turnover of microglia in the human brain. Cell Rep. 20 , 779–784 (2017).
- Bergmann, O. et al. Evidence for cardiomyocyte renewal in humans. Science 324 , 98–102 (2009).
- Bergmann, O. et al. Dynamics of cell generation and turnover in the human heart. Cell 161 , 1566–1575 (2015).
- Spalding, K. L. et al. Dynamics of fat cell turnover in humans. Nature 453 , 783–787 (2008).
- Huttner, H. B. et al. Meningioma growth dynamics assessed by radiocarbon retrospective birth dating. Ebiomedicine 27 , 176–181 (2018).
- Mold, J. E. et al. Cell generation dynamics underlying naive T-cell homeostasis in adult humans. PLoS Biol. 17 , e3000383 (2019).
- Arner, P. et al. Dynamics of human adipose lipid turnover in health and metabolic disease. Nature 478 , 110–113 (2011).
- Spalding, K. L. et al. Impact of fat mass and distribution on lipid turnover in human adipose tissue. Nat. Commun. 8 , 1–9 (2017).
- Arner, P. et al. Adipose lipid turnover and long-term changes in body weight. Nat. Med. 25 , 1385–1389 (2019).
- Libby, W. F. Radiocarbon dates. 2. Science 114 , 291–296 (1951).
- Jull, A. J. T. et al. Radiocarbon dating and intercomparison of some early historical radiocarbon samples. Radiocarbon 60 , 535–548 (2018).
- Kasso, T. M., Oinonen, M. J., Mizohata, K., Tahkokallio, J. K. & Heikkilä, T. M. Volumes of worth — delimiting the sample size for radiocarbon dating parfchment. Radiocarb. 63 , 105–120 (2021).
- Brock, F. Radiocarbon dating of historical parchments. Radiocarbon 55 , 353–363 (2013).
- Brock, F., Ostapkowicz, J., Wiedenhoeft, A. C. & Bull, I. D. Radiocarbon dating wooden carvings and skeletal remains from Pitch Lake, Trinidad. Radiocarbon 59 , 1447–1461 (2017).
- Liccioli, L., Fedi, M., Carraresi, L. & Mando, P. A. Characterization of the chloroform-based pretreatment method for C-14 dating of restored wooden samples. Radiocarbon 59 , 757–764 (2017).
- Solís, C. et al. AMS 14 C dating of the Mayan codex of Mexico revisited. Radiocarbon 62 , 1543–1550 (2020).
- Ringbom, A., Lindroos, A., Heinemeier, J. & Sonck-Koota, P. 19 Years of mortar dating: learning from experience. Radiocarbon 56 , 619–635 (2014).
- Urbanová, P., Boaretto, E. & Artioli, G. The state-of-the-art of dating techniques applied to ancient mortars and binders: a review. Radiocarbon 62 , 503–525 (2020).
- Michalska, D., Czernik, J. & Goslar, T. Methodological aspect of mortars dating (Poznań, Poland, MODIS). Radiocarbon 59 , 1891–1906 (2017).
- Caroselli, M., Hajdas, I. & Cassitti, P. Radiocarbon dating of Dolomitic mortars from the Convent Saint John, Müstair (Switzerland): first results. Radiocarbon 62 , 601–615 (2020).
- Barrett, G. T. et al. Ramped pyroxidation: a new approach for radiocarbon dating of lime mortars. J. Archaeological Sci. 129 , 105366 (2021).
- Derrick, M. R., Stulik, D. & Landry, J. M. Infrared Spectroscopy in Conservation Science 236 (Getty Publications, 2000).
- Ford, T., Rizzo, A., Hendriks, E., Frøysaker, T. & Caruso, F. A non-invasive screening study of varnishes applied to three paintings by Edvard Munch using portable diffuse reflectance infrared Fourier transform spectroscopy (DRIFTS). Herit. Sci. 7 , 1–13 (2019).
- Vitali, F. et al. The vernacular sculpture of Saint Anthony the Abbot of Museo Colle del Duomo in Viterbo (Italy). Diagnostic and wood dating. J. Cultural Herit. 48 , 299–304 (2021).
- Colombini, M. P. & Modugno, F. Organic Mass Spectrometry in Art and Archaeology 483 (Wiley, 2009).
- Hendriks, L. et al. Microscale radiocarbon dating of paintings. Applied Phys. A 122 , 1–10 (2016).
- Quarta, G., D’Elia, M., Paparella, S., Serra, A. & Calcagnile, L. Characterisation of lead carbonate white pigments submitted to AMS radiocarbon dating. J. Cultural Herit. 46 , 102–107 (2020).
- Fiorillo, F., Hendriks, L., Hajdas, I., Vandini, M. & Huysecom, E. The rediscovery of Jan Ruyscher and its consequence. J. Am. Inst. Conserv. (2021). https://doi.org/10.1080/01971360.2020.1822702
- Hajdas, I. et al. Bomb C-14 on paper and detection of the forged paintings of T’ang Haywen. Radiocarbon 61 , 1905–1912 (2019).
- Hüls, M., Grootes, P. M. & Nadeau, M.-J. Sampling iron for radiocarbon dating: influence of modern steel tools on 14 C dating of ancient iron artifacts. Radiocarbon 53 , 151–160 (2011).
- Claggett, D. et al. Modern or medieval? Analysis of an iron anchor from Camuscross, Scotland and direct dating methods for metal artefacts. J. Archaeological Science: Rep. 25 , 144–152 (2019).
- Hüls, C. M., Petri, I. & Föll, H. Absolute dating of early iron objects from the Ancient Orient: radiocarbon dating of Luristan Iron Mask Swords. Radiocarbon 61 , 1229–1238 (2019).
- Durier, M. et al. Radiocarbon dating of legacy music instrument collections: example of traditional indian Vina from the Musée De La Musique, Paris. Radiocarbon 61 , 1357–1366 (2019).
- Patrut, A. et al. The demise of the largest and oldest African baobabs. Nat. Plants 4 , 423–426 (2018).
- Patrut, A. et al. African baobabs with false inner cavities: the radiocarbon investigation of the Lebombo Eco Trail baobab. PLoS ONE 10 , e0117193 (2015).
- Patrut, A. et al. The growth stop phenomenon of baobabs ( Adansonia spp.) identified by radiocarbon dating. Radiocarb. 59 , 435–448 (2017).
- Piovesan, G. et al. Dating old hollow trees by applying a multistep tree-ring and radiocarbon procedure to trunk and exposed roots. MethodsX 5 , 495–502 (2018).
- Wild, E. M. First 14 C results from archaeological and forensic studies at the Vienna Environmental Research Accelerator. Radiocarbon 40 , 273–281 (1998).
- Jørkov, M. L. S., Heinemeier, J. & Lynnerup, N. The petrous bone — a new sampling site for identifying early dietary patterns in stable isotopic studies. Am. J. Phys. Anthropology 138 , 199–209 (2009).
- Spalding, K. L., Buchholz, B. A., Bergman, L. E., Druid, H. & Frisen, J. Forensics: age written in teeth by nuclear tests. Nature 437 , 333–334 (2005).
- Speller, C. F. et al. Personal identification of cold case remains through combined contribution from anthropological, mt DNA, and bomb-pulse dating analyses. J. Forensic Sci. 57 , 1354–1360 (2012).
- Alkass, K., Buchholz, B., Druid, H. & Spalding, K. Analysis of 14 C and 13 C in teeth provides precise birth dating and clues to geographical origin. Forensic Sci. Int. 209 , 34–41 (2011).
- Alkass, K. et al. Age estimation in forensic sciences: application of combined aspartic acid racemization and radiocarbon analysis. Mol. Cell. Proteom. 9 , 1022–1030 (2010).
- Cook, G. T., Dunbar, E., Black, S. M. & Xu, S. A preliminary assessment of age at death determination using the nuclear weapons testing 14 C activity of dentine and enamel. Radiocarbon 48 , 305–313 (2006).
- Buchholz, B. & Spalding, K. Year of birth determination using radiocarbon dating of dental enamel. Surf. Interface Analysis: An. Int. J. devoted Dev. application Tech. Anal. surfaces, interfaces thin films 42 , 398–401 (2010).
- Alkass, K. et al. Analysis of radiocarbon, stable isotopes and DNA in teeth to facilitate identification of unknown decedents. PLoS ONE 8 , e69597 (2013).
- Saitoh, H. et al. Estimation of birth year by radiocarbon dating of tooth enamel: approach to obtaining enamel powder. J. Forensic Leg. Med. 62 , 97–102 (2019).
- Kjeldsen, H., Heinemeier, J., Heegaard, S., Jacobsen, C. & Lynnerup, N. Dating the time of birth: a radiocarbon calibration curve for human eye-lens crystallines. Nucl. Instrum. Methods Phys. Res. Sect. B-Beam Interact. Mater. At. 268 , 1303–1306 (2010).
- Lynnerup, N., Kjeldsen, H., Heegaard, S., Jacobsen, C. & Heinemeier, J. Radiocarbon dating of the human eye lens crystallines reveal proteins without carbon turnover throughout life. PLoS ONE 3 , e1529 (2008).
- Uno, K. T. et al. Bomb-curve radiocarbon measurement of recent biologic tissues and applications to wildlife forensics and stable isotope (paleo)ecology. Proc. Natl Acad. Sci. USA 110 , 11736 (2013).
- Cerling, T. E. et al. Radiocarbon dating of seized ivory confirms rapid decline in African elephant populations and provides insight into illegal trade. Proc. Natl Acad. Sci. USA 113 , 13330–13335 (2016).
- Schmidberger, A., Durner, B., Gehrmeyer, D. & Schupfner, R. Development and application of a method for ivory dating by analyzing radioisotopes to distinguish legal from illegal ivory. Forensic Sci. Int. 289 , 363–367 (2018).
- Quarta, G., D’Elia, M., Braione, E. & Calcagnile, L. Radiocarbon dating of ivory: potentialities and limitations in forensics. Forensic Sci. Int. 299 , 114–118 (2019).
- Wild, E. M., Kutschera, W., Meran, A. & Steier, P. 14 C bomb peak analysis of African elephant tusks and its relation to cites. Radiocarbon 61 , 1619–1624 (2019).
- Dormontt, E. E. et al. Forensic timber identification: it’s time to integrate disciplines to combat illegal logging. Biol. Conserv. 191 , 790–798 (2015).
- Lowe, A. J. et al. Opportunities for improved transparency in the timber trade through scientific verification. BioScience 66 , 990–998 (2016).
- Retief, K., West, A. G. & Pfab, M. F. Can stable isotopes and radiocarbon dating provide a forensic solution for curbing illegal harvesting of threatened cycads? J. Forensic Sci. 59 , 1541–1551 (2014).
- Köhler, P. Using the Suess effect on the stable carbon isotope to distinguish the future from the past in radiocarbon. Env. Res. Lett. 11 , 124016 (2016).
- Quarta, G., Calcagnile, L., Giffoni, M., Braione, E. & D’Elia, M. Determination of the biobased content in plastics by radiocarbon. Radiocarbon 55 , 1834–1844 (2013).
- Dijs, I. J., Van der Windt, E., Kaihola, L. & van der Borg, K. Quantitative determination by 14 C analysis of the biological component in fuels. Radiocarbon 48 , 315–323 (2006).
- Varga, T. et al. High-precision biogenic fraction analyses of liquid fuels by 14 C AMS at HEKAL. Radiocarbon 60 , 1317–1325 (2018).
- Palstra, S. W. L., Rabou, L. P. L. M. & Meijer, H. A. J. Radiocarbon-based determination of biogenic and fossil carbon partitioning in the production of synthetic natural gas. Fuel 157 , 177–182 (2015).
- Calcagnile, L., Quarta, G., D’Elia, M., Ciceri, G. & Martinotti, V. Radiocarbon AMS determination of the biogenic component in CO 2 emitted from waste incineration. Nucl. Instrum. Methods Phys. Res. Sect. B: Beam Interact. Mater. At. 269 , 3158–3162 (2011).
- Quarta, G. et al. AMS- 14 C determination of the biogenic-fossil fractions in flue gases. Radiocarbon 60 , 1327–1333 (2018).
- Bronić, I. K., Barešić, J., Horvatinčić, N. & Sironić, A. Determination of biogenic component in liquid fuels by the 14 C direct LSC method by using quenching properties of modern liquids for calibration. Radiat. Phys. Chem. 137 , 248–253 (2017).
- ISO. ISO 13833:2013: stationary source emissions – determination of the ratio of biomass (biogenic) and fossil-derived carbon dioxide–radiocarbon sampling and determination. ISO (2013). https://www.iso.org/standard/54332.html
- ASTM International. ASTM D6866-21: standard test methods for determining the biobased content of solid, liquid, and gaseous samples using radiocarbon analysis. ASTM International (2021). https://www.astm.org/Standards/D6866.htm
- Nilsson, M. et al. Analysis of the putative remains of a European Patron Saint — St. Birgitta. PLoS ONE 5 , e8986 (2010).
- Damon, P. E. et al. Radiocarbon dating of the Shroud of Turin. Nature 337 , 611–615 (1989).
- De Vries, H. & Oakley, K. P. Radiocarbon dating of the Piltdown skull and jaw. Nature 184 , 224–226 (1959).
- De Groote, I. et al. New genetic and morphological evidence suggests a single hoaxer created ‘Piltdown man’. R. Soc. Open. Sci. 3 , 160328 (2016).
- Caforio, L. et al. Discovering forgeries of modern art by the C-14 bomb peak. Eur. Phys. J. Plus 129 , 1–5 (2014).
- Petrucci, F. et al. Radiocarbon dating of twentieth century works of art. Appl. Phys. A 122 , 983 (2016).
- Hendriks, L. et al. Uncovering modern paint forgeries by radiocarbon dating. Proc. Natl Acad. Sci. USA 116 , 13210–13214 (2019).
- Olsson, I. U. Radiocarbon dating history: early days, questions, and problems met. Radiocarbon 51 , 1–43 (2009).
- Scott, E. M., Cook, G. T. & Naysmith, P. The Fifth International Radiocarbon Intercomparison (VIRI): an assessment of laboratory performance in stage 3. Radiocarbon 52 , 859–865 (2010).
- Naysmith, P. et al. A cremated bone intercomparison study. Radiocarbon 49 , 403–408 (2007).
- Kuzmin, Y. V. et al. A laboratory inter-comparison of AMS 14 C dating of bones of the Miesenheim IV elk (Rhineland, Germany) and its implications for the date of the Laacher See eruption. Quaternary Geochronology 48 , 7–16 (2018).
- Scott, E., Cook, G., Naysmith, P. & Staff, R. Learning from the wood samples in ICS, TIRI, FIRI, VIRI and SIRI. Radiocarbon 61 , 1293–1304 (2019).
- Quiles, A. et al. Second Radiocarbon Intercomparison Program for the Chauvet-Pont D’arc Cave, Ardeche, France. Radiocarbon 56 , 833–850 (2014).
- Millard, A. R. Conventions for reporting radiocarbon determinations. Radiocarbon 56 , 555–559 (2014).
- Bayliss, A. Quality in Bayesian chronological models in archaeology. World Archaeol. 47 , 677–700 (2015).
- Johnson, F. Radiocarbon dating lists and their use. Am. Antiquity 21 , 312–313 (1956).
- Johnson, F. J. R. A bibliography of radiocarbon dating. Radiocarbon 1 , 199–214 (1959).
- Hedges, R., Housley, R., Ramsey, C. B. & Van Klinken, G. J. A. Radiocarbon dates from the Oxford AMS system: archaeometry datelist 18. Archaeometry 36 , 337–374 (1994).
- Broecker, W. S., Kulp, J. L. & Tucek, C. S. Lamont natural radiocarbon measurements. 3. Science 124 , 154–165 (1956).
- Olson, E. A. & Broecker, W. S. Lamont natural radiocarbon measurements. 5. Am. J. Sci. 257 , 1–28 (1959).
- Broecker, W. S. & Olson, E. A. Lamont radiocarbon measurements VIII. Radiocarbon 3 , 176–204 (1961).
- Vermeersch, P. M. Radiocarbon Palaeolithic Europe database: a regularly updated dataset of the radiometric data regarding the Palaeolithic of Europe, Siberia included. Data Brief. 31 , 105793–105793 (2020).
- Loftus, E., Mitchell, P. J. & Ramsey, C. B. An archaeological radiocarbon database for southern Africa. Antiquity 93 , 870–885 (2019).
- Gajewski, K. et al. The Canadian Archaeological Radiocarbon Database (CARD): archaeological 14 C dates in North America and their paleoenvironmental context. Radiocarbon 53 , 371–394 (2011).
- Bryson, R., Bryson, R. & Ruter, A. A calibrated radiocarbon database of late Quaternary volcanic eruptions. eEarth Discuss. 1 , 123–134 (2006).
- Lawrence, C. R. et al. An open-source database for the synthesis of soil radiocarbon data: International Soil Radiocarbon Database (ISRaD) version 1.0. Earth Syst. Sci. Data 12 , 61–76 (2020).
- Herrando-Pérez, S. Bone need not remain an elephant in the room for radiocarbon dating. R. Soc. Open. Sci. 8 , 201351 (2021).
- van der Voort, T. S. et al. MOSAIC (Modern Ocean Sediment Archive and Inventory of Carbon): a (radio)carbon-centric database for seafloor surficial sediments. Earth Syst. Sci. Data 13 , 2135–2146 (2021).
- Sánchez Goñi, M. F. et al. The ACER pollen and charcoal database: a global resource to document vegetation and fire response to abrupt climate changes during the last glacial period. Earth Syst. Sci. Data 9 , 679–695 (2017).
- Guilderson, T. P., Reimer, P. J. & Brown, T. A. The boon and bane of radiocarbon dating. Science 307 , 362–364 (2005).
- Svetlik, I. et al. The best possible time resolution: how precise could a radiocarbon dating method be? Radiocarbon 61 , 1729–1740 (2019).
- Ramsey, C. B., van der Plicht, J. & Weninger, B. ‘Wiggle matching’ radiocarbon dates. Radiocarbon 43 , 381–389 (2001).
- Mol, D. et al. Results of the CERPOLEX/Mammuthus Expeditions on the Taimyr Peninsula, Arctic Siberia, Russian Federation. Quaternary Int. 142–143 , 186–202 (2006).
- Maruccio, L., Quarta, G., Braione, E. & Calcagnile, L. Measuring stable carbon and nitrogen isotopes by IRMS and 14 C by AMS on samples with masses in the microgram range: performances of the system installed at CEDAD — University of Salento. Int. J. Mass. Spectrom. 421 , 1–7 (2017).
- McIntyre, C. P. et al. Online C-13 and C-14 gas measurements by EA–IRMS–AMS at ETH Zurich. Radiocarbon 59 , 893–903 (2017).
- Blattmann, T. M. & Ishikawa, N. F. Theoretical amino acid-specific radiocarbon content in the environment: hypotheses to be tested and opportunities to be taken. Front. Mar. Sci. (2020). https://doi.org/10.3389/fmars.2020.00302
- Deviese, T., Comeskey, D., McCullagh, J., Bronk Ramsey, C. & Higham, T. New protocol for compound-specific radiocarbon analysis of archaeological bones. Rapid Commun. Mass. Spectrometry 32 , 373–379 (2018).
- Hamady, L. L., Natanson, L. J., Skomal, G. B. & Thorrold, S. R. Vertebral bomb radiocarbon suggests extreme longevity in white sharks. PLoS ONE 9 , e84006 (2014).
- Nielsen, J. et al. Eye lens radiocarbon reveals centuries of longevity in the Greenland shark ( Somniosus microcephalus ). Science 353 , 702–704 (2016).
- Loader, N. J. et al. Tree ring dating using oxygen isotopes: a master chronology for central England. J. Quaternary Sci. 34 , 475–490 (2019).
- Sigl, M. et al. Timing and climate forcing of volcanic eruptions for the past 2,500 years. Nature 523 , 543–549 (2015).
- Brehm, N. et al. Eleven-year solar cycles over the last millennium revealed by radiocarbon in tree rings. Nat. Geosci. 14 , 10–15 (2021).
- Land, A., Kromer, B., Remmele, S., Brehm, N. & Wacker, L. Complex imprint of solar variability on tree rings. Environ. Res. Commun. 2 , 101003 (2020).
- Libby, W. F. in Radiocarbon dating (ed. Libby, W. F.) 34–42 (University of Chicago Press, 1952).
- Godwin, H. Half-life of radiocarbon. Nature 195 , 984 (1962).
- Buck, C. E. & Sahu, S. K. Bayesian models for relative archaeological chronology building. J. R. Stat. Soc. Ser. C-Applied Stat. 49 , 423–440 (2000).
- Ramsey, C. B. Deposition models for chronological records. Quaternary Sci. Rev. 27 , 42–60 (2008).
- Hajdas, I., Sojc, U., Ivy-Ochs, S., Akçar, N. & Deline, P. Radiocarbon dating for the reconstruction of the 1717 CE Triolet rock avalanche in the Mont Blanc Massif, Italy. Front. Earth Sci. (2021). https://doi.org/10.3389/feart.2020.580293
- Yamamoto, S. et al. Compound-specific radiocarbon analysis of organic compounds from Mount Fuji Proximal Lake (Lake Kawaguchi) sediment, Central Japan. Radiocarbon 62 , 439–451 (2020).
- Gierga, M. et al. Long-stored soil carbon released by prehistoric land use: evidence from compound-specific radiocarbon analysis on Soppensee lake sediments. Quaternary Sci. Rev. 144 , 123–131 (2016).
- Heinemeier, K. M., Schjerling, P., Heinemeier, J., Magnusson, S. P. & Kjaer, M. Lack of tissue renewal in human adult Achilles tendon is revealed by nuclear bomb 14 C. FASEB J. 27 , 2074–2079 (2013).
- Heinemeier, K. M. et al. Radiocarbon dating reveals minimal collagen turnover in both healthy and osteoarthritic human cartilage. Sci. Transl. Med. 8 , 346ra390–346ra390 (2016).
- Ubelaker, D. H. Radiocarbon analysis of human remains: a review of forensic applications. J. Forensic Sci. 59 , 1466–1472 (2014).
- Castro, K. et al. Multianalytical approach to the analysis of English polychromed alabaster sculptures: μRaman, μEDXRF, and FTIR spectroscopies. Anal. Bioanal. Chem. 392 , 755–763 (2008).
- Casadio, F., Daher, C. & Bellot-Gurlet, L. in Analytical Chemistry for Cultural Heritage (ed. Mazzeo, R.) 161–211 (Springer International, 2017).
- Švarcová, S., Kočí, E., Bezdička, P., Hradil, D. & Hradilová, J. Evaluation of laboratory powder X-ray micro-diffraction for applications in the fields of cultural heritage and forensic science. Anal. Bioanal. Chem. 398 , 1061–1076 (2010).
- Marzaioli, F. et al. Characterisation of a new protocol for mortar dating: 14 C evidences. Open J. Archaeometry 2 , 55–59 (2014).
- Yates, T. Studies of non-marine mollusks for the selection of shell samples for radiocarbon dating. Radiocarbon 28 , 457–463 (1986).
- Gianfrate, G. et al. Qualitative application based on IR spectroscopy for bone sample quality control in radiocarbon dating. Nucl. Instrum. Methods Phys. Res. Sect. B: Beam Interact. Mater. At. Accelerator Mass. Spectrometry - Proc. Tenth Int. Conf. Accelerator Mass Spectrometry 259 , 316–319 (2007).
- Zazzo, A., Saliège, J.-F., Person, A. & Boucher, H. Radiocarbon dating of calcined bones: where does the carbon come from? Radiocarbon 51 , 601–611 (2009).
- Alon, D., Mintz, G., Cohen, I., Weiner, S. & Boaretto, E. The use of Raman spectroscopy to monitor the removal of humic substances from charcoal: quality control for 14 C dating of charcoal. Radiocarbon 44 , 1–11 (2002).
- Ospitali, F., Smith, D. C. & Lorblanchet, M. Preliminary investigations by Raman microscopy of prehistoric pigments in the wall-painted cave at Roucadour, Quercy, France. J. Raman Spectroscopy: An. Int. J. Original Work. all Asp. Raman Spectroscopy, Including High. Order Processes, also BrillouRayleigh Scattering 37 , 1063–1071 (2006).
- Boaretto, E. et al. Radiocarbon dating of charcoal and bone collagen associated with early pottery at Yuchanyan Cave, Hunan Province, China. Proc. Natl Acad. Sci. USA 106 , 9595–9600 (2009).
- Lahlil, S. et al. The first in situ micro-Raman spectroscopic analysis of prehistoric cave art of Rouffignac St-Cernin, France. J. Raman Spectrosc. 43 , 1637–1643 (2012).
- Hendriks, L. et al. Selective dating of paint components: radiocarbon dating of lead white pigment. Radiocarbon 61 , 473–493 (2019).
- Bird, M., Ascough, P., Young, I., Wood, C. & Scott, A. X-ray microtomographic imaging of charcoal. J. Archaeological Sci. 35 , 2698–2706 (2008).
- Huang, Y., Li, B., Bryant, C., Bol, R. & Eglinton, G. Radiocarbon dating of aliphatic hydrocarbons a new approach for dating passive-fraction carbon in soil horizons. Soil. Sci. Soc. Am. J. 63 , 1181–1187 (1999).
- Santos, G., De La Torre, H., Boudin, M., Bonafini, M. & Saverwyns, S. Improved radiocarbon analyses of modern human hair to determine the year-of-death by cross-flow nanofiltered amino acids: common contaminants, implications for isotopic analysis, and recommendations. Rapid Commun. Mass. Spectrometry 29 , 1765–1773 (2015).
- Van Strydonck, M. et al. 14 C-dating of the skeleton remains and the content of the lead coffin attributed to the Blessed Idesbald (Abbey of the Dunes, Koksijde, Belgium). J. Archaeological Science: Rep. 5 , 276–284 (2016).
- Teetaert, D., Boudin, M., Saverwyns, S. & Crombé, P. Food and soot: organic residues on outer pottery surfaces. Radiocarbon 59 , 1609 (2017).
- Cersoy, S., Daheur, G., Zazzo, A., Zirah, S. & Sablier, M. Pyrolysis comprehensive gas chromatography and mass spectrometry: a new tool to assess the purity of ancient collagen prior to radiocarbon dating. Anal. Chim. Acta 1041 , 131–145 (2018).
- Wagner, T. V. et al. Molecular characterization of charcoal to identify adsorbed SOM and assess the effectiveness of common SOM-removing pretreatments prior to radiocarbon dating. Quaternary Geochronol. 45 , 74–84 (2018).
- Casanova, E., Knowles, T. D., Williams, C., Crump, M. P. & Evershed, R. P. Use of a 700 MHz NMR microcryoprobe for the identification and quantification of exogenous carbon in compounds purified by preparative capillary gas chromatography for radiocarbon determinations. Anal. Chem. 89 , 7090–7098 (2017).
- Boudin, M., Boeckx, P., Vandenabeele, P., Mitschke, S. & Strydonck, M. V. A. N. Monitoring the presence of humic substances in wool and silk by the use of nondestructive fluorescence spectroscopy: quality control for 14 C dating of wool and silk. Radiocarbon 53 , 429–442 (2011).
- Bird, M. I. et al. Radiocarbon dating of “old” charcoal using a wet oxidation, stepped-combustion procedure. Radiocarbon 41 , 127–140 (1999).
- Broecker, W. S. & Farrand, W. R. Radiocarbon age of the Two Creeks forest bed, Wisconsin. Geol. Soc. Am. Bull. 74 , 795–802 (1963).
- Nemec, M., Wacker, L., Hajdas, I. & Gaggeler, H. Alternative methods for cellulose preparation for AMS measurement. Radiocarbon 52 , 1358–1370 (2010).
- Czimczik, C. I., Trumbore, S. E., Xu, X., Carbone, M. S. & Richardson, A. D. Extraction of nonstructural carbon and cellulose from wood for radiocarbon analysis. Bio Protoc. 4 , e1169–e1169 (2014).
- Hajdas, I., Hendriks, L., Fontana, A. & Monegato, G. Evaluation of preparation methods in radiocarbon dating of old woods. Radiocarbon 59 , 727–737 (2017).
- Eglinton, T. I. et al. Compound specific radiocarbon analysis as a tool to quantitatively apportion modern and fossil sources of polycyclic aromatic hydrocarbons in environmental matrices. Abstr. Pap. Am. Chem. Soc. 212 , 65–ENVR (1996).
- Dahl, K. A. et al. Terrigenous plant wax inputs to the Arabian Sea: implications for the reconstruction of winds associated with the Indian Monsoon. Geochimica Cosmochimica Acta 69 , 2547–2558 (2005).
- Ohkouchi, N., Eglinton, T., Hughen, K., Roosen, E. & Keigwin, L. Radiocarbon dating of alkenones from marine sediments: III. Influence of solvent extraction procedures on C-14 measurements of foraminifera. Radiocarbon 47 , 425–432 (2005).
- Sun, S. W. et al. C-14 blank assessment in small-scale compound-specific radiocarbon analysis of lipid biomarkers and lignin phenols. Radiocarbon 62 , 207–218 (2020).
- Ya, M., Wu, Y., Wang, X., Li, Y. & Su, G. The importance of compound-specific radiocarbon analysis in source identification of polycyclic aromatic hydrocarbons: a critical review. Crit. Rev. Environ. Sci. Technol. (2020). https://doi.org/10.1080/10643389.2020.1843305
- Hendriks, L. et al. The ins and outs of C-14 dating lead white paint for artworks application. Anal. Chem. 92 , 7674–7682 (2020).
- Beck, L. et al. Thermal decomposition of lead white for radiocarbon dating of paintings. Radiocarbon 61 , 1345–1356 (2019).
- Toffolo, M. B. et al. Structural characterization and hermal decomposition of lime binders allow accurate radiocarbon age determinations of aerial lime plaster. Radiocarbon 62 , 633–655 (2020).
- McGeehin, J. et al. Stepped-combustion C-14 dating of sediment: a comparison with established techniques. Radiocarbon 43 , 255–261 (2001).
- Hajdas, I., Maurer, M. & Röttig, M. B. Development of 14 C dating of mortars at ETH Zurich. Radiocarbon 62 , 591–600 (2020).
- Hua, Q. et al. Progress in radiocarbon target preparation at the ANTARES AMS Centre. Radiocarbon 43 , 275–282 (2001).
- Aerts-Bijma, A. T., van der Plicht, J. & Meijer, H. Automatic AMS sample combustion and CO 2 collection. Radiocarbon 43 , 293–298 (2001).
- Nemec, M., Wacker, L. & Gaggeler, H. Optimization of the graphitization process at AGE-1. Radiocarbon 52 , 1380–1393 (2010).
- Salazar, G., Zhang, Y., Agrios, K. & Szidat, S. Development of a method for fast and automatic radiocarbon measurement of aerosol samples by online coupling of an elemental analyzer with a MICADAS AMS. Nucl. Instrum. Methods Phys. Res. Sect. B: Beam Interact. Mater. At. 361 , 163–167 (2015).
- Steier, P. et al. A new UV oxidation setup for small radiocarbon samples in solution. Radiocarbon 55 , 373–382 (2013).
- Murseli, S. et al. The preparation of water (DIC, DOC) and gas (CO 2 , CH 4 ) samples for radiocarbon analysis at AEL-AMS, Ottawa, Canada. Radiocarbon 61 , 1563–1571 (2019).
- Burr, G. S., Edwards, R. L., Donahue, D. J., Druffel, E. R. M. & Taylor, F. W. Mass-spectrometric C-14 and U–Th measurements in coral. Radiocarbon 34 , 611–618 (1992).
- Wacker, L., Fulop, R., Hajdas, I., Molnar, M. & Rethemeyer, J. A novel approach to process carbonate samples for radiocarbon measurements with helium carrier gas. Nucl. Instrum. Methods Phys. Res. Sect. B-Beam Interact. Mater. At. 294 , 214–217 (2013).
- Garnett, M. & Murray, C. Processing of CO 2 samples collected using zeolite molecular sieve for 14 C analysis at the NERC Radiocarbon Facility (East Kilbride, UK). Radiocarbon 55 , 410–415 (2013).
- Gaudinski, J. B., Trumbore, S. E., Davidson, E. A. & Zheng, S. Soil carbon cycling in a temperate forest: radiocarbon-based estimates of residence times, sequestration rates and partitioning of fluxes. Biogeochemistry 51 , 33–69 (2000).
- Russ, J., Hyman, M., Shafer, H. J. & Rowe, M. W. Radiocarbon dating of prehistoric rock paintings by selective oxidation of organic carbon. Nature 348 , 710–711 (1990).
- Rowe, M. et al. Application of supercritical carbon dioxide-co-solvent mixtures for removal of organic material from archeological artifacts for radiocarbon dating. J. Supercrit. Fluids 79 , 314–323 (2013).
- Vogel, J. S., Southon, J. R., Nelson, D. E. & Brown, T. A. Performance of catalytically condensed carbon for use in accelerator mass-spectrometry. Nucl. Instrum. Methods Phys. Res. Sect. B-Beam Interact. Mater. At. 233 , 289–293 (1984).
- Wacker, L., Nemec, M. & Bourquin, J. A revolutionary graphitisation system: fully automated, compact and simple. Nucl. Instrum. Methods Phys. Res. Sect. B-Beam Interact. Mater. At. 268 , 931–934 (2010).
- Marzaioli, F. et al. Zinc reduction as an alternative method for AMS radiocarbon dating: process optimization at CIRCE. Radiocarbon 50 , 139–149 (2008).
- Xu, X. M., Gao, P. & Salamanca, E. G. Ultra small-mass graphitization by sealed tube zinc reduction method for AMS C-14 measurements. Radiocarbon 55 , 608–616 (2013).
- Rinyu, L., Orsovszki, G., Futo, I., Veres, M. & Molnar, M. Application of zinc sealed tube graphitization on sub-milligram samples using EnvironMICADAS. Nucl. Instrum. Methods Phys. Res. Sect. B-Beam Interact. Mater. At. 361 , 406–413 (2015).
- Janovics, R., Futó, I. & Molnár, M. Sealed tube combustion method with MnO 2 for AMS 14 C measurement. Radiocarbon 60 , 1347–1355 (2018).
- Siegenthaler, U., Heimann, M. & Oeschger, H. C-14 variations caused by changes in the global carbon-cycle. Radiocarbon 22 , 177–191 (1980).
- Suess, H. E. Radiocarbon concentration in modern wood. Science 122 , 415–417 (1955).
- Levin, I., Kromer, B. & Hammer, S. Atmospheric Δ(CO 2 )-C-14 trend in Western European background air from 2000 to 2012. Tellus B Chem. Phys. Meteorol. 65 , 20092 (2013).
- Barisevičiūtė, R. et al. Distribution of radiocarbon in sediments of the cooling pond of RBMK type Ignalina Nuclear Power Plant in Lithuania. PLoS ONE 15 , e0237605 (2020).
- Rakowski, A. Z., Kuc, T., Nakamura, T. & Pazdur, A. Radiocarbon concentration in urban area. Geochronometria 24 , 63–68 (2005).
- Svetlik, I. et al. Radiocarbon in the air of central Europe: long-term investigations. Radiocarbon 52 , 823–834 (2010).
- Hancock, G. J., Tims, S. G., Fifield, L. K. & Webster, I. T. The release and persistence of radioactive anthropogenic nuclides. Stratigraphical Basis for the Anthropocene 395 , 265–281 (2014).
- Waters, C. N. et al. Can nuclear weapons fallout mark the beginning of the Anthropocene Epoch? Bull. At. Scientists 71 , 46–57 (2015).
Advertisement
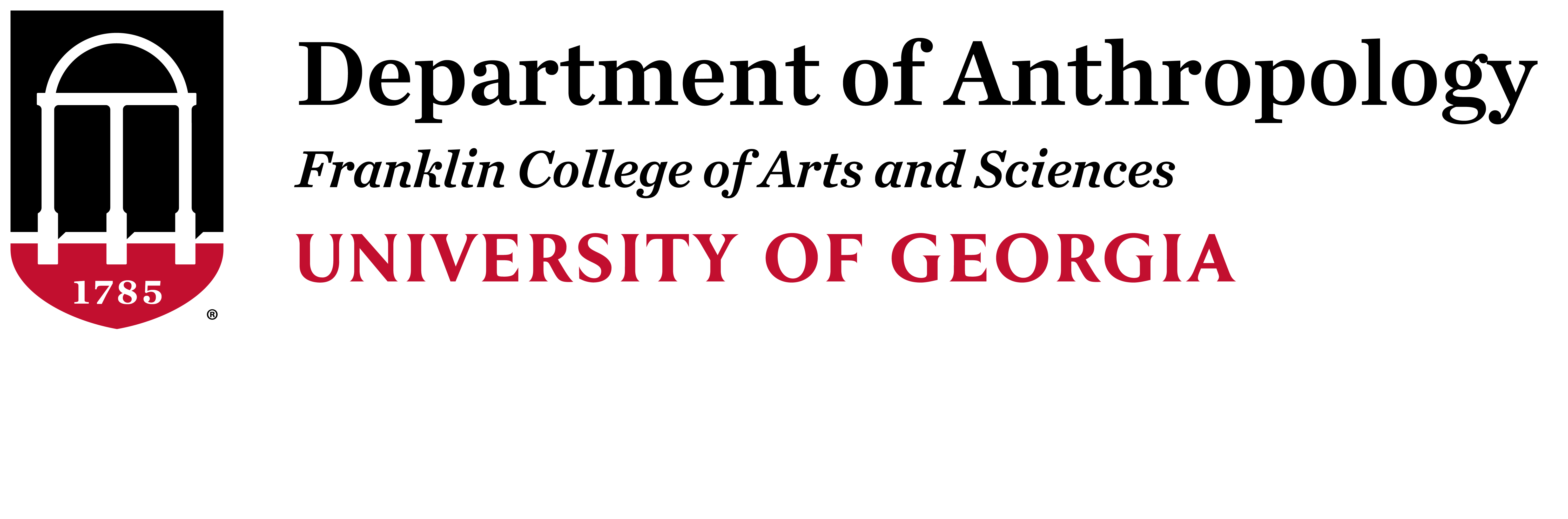
Collaborative research reveals how radiocarbon dating can rewrite history in the American Southeast
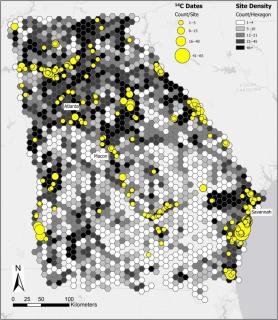
Stemming from the collaboration between faculty, researchers, and current and former graduate students at the Department of Anthropology, a newly published article sheds light on the importance of radiocarbon dating in the American Southeast, emphasizing its role in refining regional chronologies. Placing radiocarbon dating at the center of collective archaeological practice , born from a graduate seminar taught by Dr. Thompson at the University of Georgia, delves into the challenges and potential solutions associated with the current understanding of chronological frameworks in the region. The collaborative effort, which evolved beyond the seminar, highlights the uneven distribution of radiocarbon dates across time and space in Georgia. The authors argue for the widespread adoption of radiocarbon dating, particularly in cultural resource management (CRM) projects, suggesting its significant impact on creating more accurate chronologies. The article concludes by emphasizing the shared goals across different sectors of archaeology in writing accurate and meaningful histories.
Support Anthropology at UGA
Your support helps bring in speakers of note, provides student research funding, assists in student fieldwork and conference travel, and creates new resources to further enrich each learner's experience. Learn more about how you can support the Department of Anthropology .
Every dollar given has a direct impact upon our students and faculty.
Radiocarbon dating
- Tree-Ring Research, Laboratory of
- Anthropology, School of
- Geosciences
- Global Change - GIDP
Research output : Contribution to journal › Review article › peer-review
Radiocarbon dating uses the decay of a radioactive isotope of carbon ( 14 C) to measure time and date objects containing carbon-bearing material. With a half-life of 5,700 ± 30 years, detection of 14 C is a useful tool for determining the age of a specimen formed over the past 55,000 years. In this Primer, we outline key advances in 14 C measurement and instrument capacity, as well as optimal sample selection and preparation. We discuss data processing, carbon reservoir age correction, calibration and statistical analyses. We then outline examples of radiocarbon dating across a range of applications, from anthropology and palaeoclimatology to forensics and medical science. Reproducibility and minimum reporting standards are discussed along with potential issues related to accuracy and sensitivity. Finally, we look forwards to the adoption of radiocarbon dating in various fields of research thanks to continued instrument improvement.
ASJC Scopus subject areas
- General Medicine
- General Biochemistry, Genetics and Molecular Biology
Access to Document
- 10.1038/s43586-021-00058-7
Other files and links
- Link to publication in Scopus
Fingerprint
- Radiometric Dating Medicine & Life Sciences 100%
- Carbon Medicine & Life Sciences 50%
- Radioisotope Chemical Compounds 27%
- Forensic Sciences Medicine & Life Sciences 24%
- Reaction Half Life Chemical Compounds 24%
- Carbon Atom Chemical Compounds 23%
- Anthropology Medicine & Life Sciences 22%
- Calibration Medicine & Life Sciences 17%
T1 - Radiocarbon dating
AU - Hajdas, Irka
AU - Ascough, Philippa
AU - Garnett, Mark H.
AU - Fallon, Stewart J.
AU - Pearson, Charlotte L.
AU - Quarta, Gianluca
AU - Spalding, Kirsty L.
AU - Yamaguchi, Haruka
AU - Yoneda, Minoru
N1 - Publisher Copyright: © 2021, Springer Nature Limited.
PY - 2021/12
Y1 - 2021/12
N2 - Radiocarbon dating uses the decay of a radioactive isotope of carbon (14C) to measure time and date objects containing carbon-bearing material. With a half-life of 5,700 ± 30 years, detection of 14C is a useful tool for determining the age of a specimen formed over the past 55,000 years. In this Primer, we outline key advances in 14C measurement and instrument capacity, as well as optimal sample selection and preparation. We discuss data processing, carbon reservoir age correction, calibration and statistical analyses. We then outline examples of radiocarbon dating across a range of applications, from anthropology and palaeoclimatology to forensics and medical science. Reproducibility and minimum reporting standards are discussed along with potential issues related to accuracy and sensitivity. Finally, we look forwards to the adoption of radiocarbon dating in various fields of research thanks to continued instrument improvement.
AB - Radiocarbon dating uses the decay of a radioactive isotope of carbon (14C) to measure time and date objects containing carbon-bearing material. With a half-life of 5,700 ± 30 years, detection of 14C is a useful tool for determining the age of a specimen formed over the past 55,000 years. In this Primer, we outline key advances in 14C measurement and instrument capacity, as well as optimal sample selection and preparation. We discuss data processing, carbon reservoir age correction, calibration and statistical analyses. We then outline examples of radiocarbon dating across a range of applications, from anthropology and palaeoclimatology to forensics and medical science. Reproducibility and minimum reporting standards are discussed along with potential issues related to accuracy and sensitivity. Finally, we look forwards to the adoption of radiocarbon dating in various fields of research thanks to continued instrument improvement.
UR - http://www.scopus.com/inward/record.url?scp=85124591754&partnerID=8YFLogxK
UR - http://www.scopus.com/inward/citedby.url?scp=85124591754&partnerID=8YFLogxK
U2 - 10.1038/s43586-021-00058-7
DO - 10.1038/s43586-021-00058-7
M3 - Review article
AN - SCOPUS:85124591754
SN - 2662-8449
JO - Nature Reviews Methods Primers
JF - Nature Reviews Methods Primers
Carbon Dating Worksheet Packet (18 Assignments)
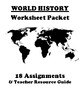
Description
This Packet Includes 18 Assignments that will help supplement and differentiate your topic coverage. Assignments range from writing to creative ones.
*View the Preview for an example of each assignment to make sure this packet is a fit for your classroom
Packet Includes:
Pyramid Summary Worksheet
History Brochure
Three Truths and a Lie Worksheet w/word search
Five Fact Summary Worksheet
Historic Adjectives Worksheet
Create your own Book Cover Worksheet
Create your own Cell Phone Worksheet
Historic Blogging Worksheet
Commemorative Coin Worksheet
Historic News Article Worksheet
Oral Report Reference Cards
Puzzle Worksheet
R.A.C.E Writing Assignment
Ticket to Leave Slips
T-Shirt Worksheet
History Quick Write Slips
Unknown Vocabulary Log Worksheet
Who, What, Where, When & Why Graphic Organizer
Teacher Resource Links
Questions & Answers
Best educational consulting.
- We're hiring
- Help & FAQ
- Privacy policy
- Student privacy
- Terms of service
- Tell us what you think
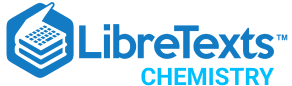
- school Campus Bookshelves
- menu_book Bookshelves
- perm_media Learning Objects
- login Login
- how_to_reg Request Instructor Account
- hub Instructor Commons
Margin Size
- Download Page (PDF)
- Download Full Book (PDF)
- Periodic Table
- Physics Constants
- Scientific Calculator
- Reference & Cite
- Tools expand_more
- Readability
selected template will load here
This action is not available.
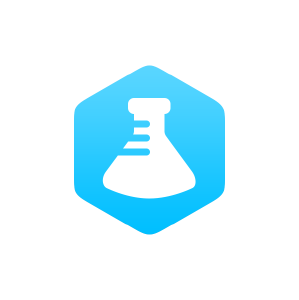
Radiocarbon Dating
- Last updated
- Save as PDF
- Page ID 1479
\( \newcommand{\vecs}[1]{\overset { \scriptstyle \rightharpoonup} {\mathbf{#1}} } \)
\( \newcommand{\vecd}[1]{\overset{-\!-\!\rightharpoonup}{\vphantom{a}\smash {#1}}} \)
\( \newcommand{\id}{\mathrm{id}}\) \( \newcommand{\Span}{\mathrm{span}}\)
( \newcommand{\kernel}{\mathrm{null}\,}\) \( \newcommand{\range}{\mathrm{range}\,}\)
\( \newcommand{\RealPart}{\mathrm{Re}}\) \( \newcommand{\ImaginaryPart}{\mathrm{Im}}\)
\( \newcommand{\Argument}{\mathrm{Arg}}\) \( \newcommand{\norm}[1]{\| #1 \|}\)
\( \newcommand{\inner}[2]{\langle #1, #2 \rangle}\)
\( \newcommand{\Span}{\mathrm{span}}\)
\( \newcommand{\id}{\mathrm{id}}\)
\( \newcommand{\kernel}{\mathrm{null}\,}\)
\( \newcommand{\range}{\mathrm{range}\,}\)
\( \newcommand{\RealPart}{\mathrm{Re}}\)
\( \newcommand{\ImaginaryPart}{\mathrm{Im}}\)
\( \newcommand{\Argument}{\mathrm{Arg}}\)
\( \newcommand{\norm}[1]{\| #1 \|}\)
\( \newcommand{\Span}{\mathrm{span}}\) \( \newcommand{\AA}{\unicode[.8,0]{x212B}}\)
\( \newcommand{\vectorA}[1]{\vec{#1}} % arrow\)
\( \newcommand{\vectorAt}[1]{\vec{\text{#1}}} % arrow\)
\( \newcommand{\vectorB}[1]{\overset { \scriptstyle \rightharpoonup} {\mathbf{#1}} } \)
\( \newcommand{\vectorC}[1]{\textbf{#1}} \)
\( \newcommand{\vectorD}[1]{\overrightarrow{#1}} \)
\( \newcommand{\vectorDt}[1]{\overrightarrow{\text{#1}}} \)
\( \newcommand{\vectE}[1]{\overset{-\!-\!\rightharpoonup}{\vphantom{a}\smash{\mathbf {#1}}}} \)
Learning Objectives
- Identify the age of materials that can be approximately determined using Radiocarbon dating.
When we speak of the element Carbon, we most often refer to the most naturally abundant stable isotope 12 C. Although 12 C is definitely essential to life, its unstable sister isotope 14 C has become of extreme importance to the science world. Radiocarbon dating is the process of determining the age of a sample by examining the amount of 14 C remaining against its known half-life, 5,730 years. The reason this process works is because when organisms are alive, they are constantly replenishing their 14 C supply through respiration, providing them with a constant amount of the isotope. However, when an organism ceases to exist, it no longer takes in carbon from its environment and the unstable 14 C isotope begins to decay. From this science, we are able to approximate the date at which the organism lived on Earth. Radiocarbon dating is used in many fields to learn information about the past conditions of organisms and the environments present on Earth.
The Carbon-14 Cycle
Radiocarbon dating (usually referred to simply as carbon-14 dating) is a radiometric dating method. It uses the naturally occurring radioisotope carbon-14 ( 14 C ) to estimate the age of carbon-bearing materials up to about 58,000 to 62,000 years old. Carbon has two stable, nonradioactive isotopes: carbon-12 ( 12 C) and carbon-13 ( 13 C). There are also trace amounts of the unstable radioisotope carbon-14 ( 14 C) on Earth. Carbon-14 has a relatively short half-life of 5,730 years, meaning that the fraction of carbon-14 in a sample is halved over the course of 5,730 years due to radioactive decay to nitrogen-14. The carbon-14 isotope would vanish from Earth's atmosphere in less than a million years were it not for the constant influx of cosmic rays interacting with molecules of nitrogen (N 2 ) and single nitrogen atoms (N) in the stratosphere. Both processes of formation and decay of carbon-14 are shown in Figure 1.
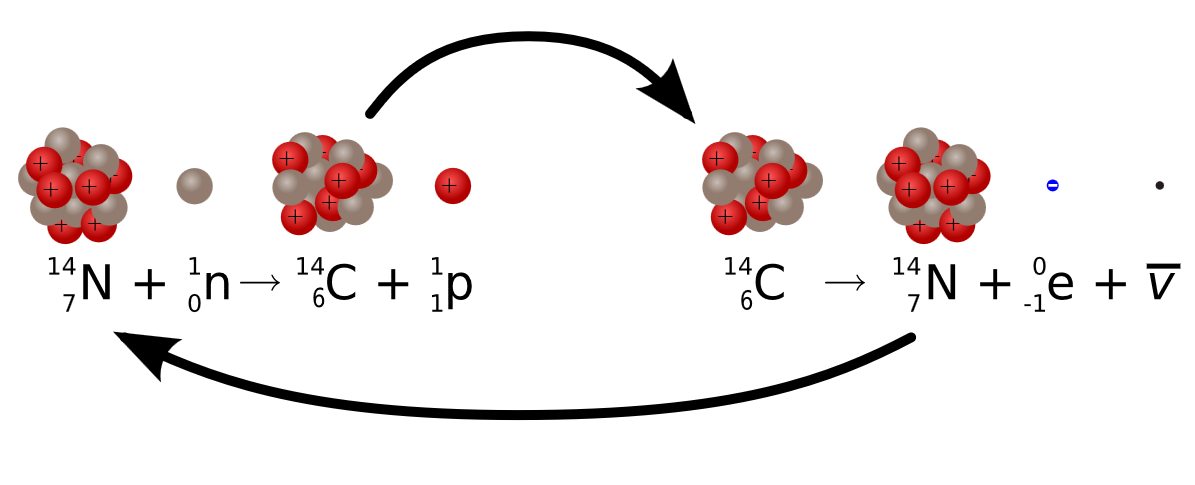
When plants fix atmospheric carbon dioxide (CO 2 ) into organic compounds during photosynthesis, the resulting fraction of the isotope 14 C in the plant tissue will match the fraction of the isotope in the atmosphere (and biosphere since they are coupled). After a plant dies, the incorporation of all carbon isotopes, including 14 C , stops and the concentration of 14 C declines due to the radioactive decay of 14 C following.
\[ \ce{ ^{14}C -> ^{14}N + e^-} + \mu_e \label{E2}\]
This follows first-order kinetics :
\[N_t= N_o e^{-kt} \label{E3}\]
- \(N_0\) is the number of atoms of the isotope in the original sample (at time t = 0, when the organism from which the sample is derived was de-coupled from the biosphere).
- \(N_t\) is the number of atoms left after time \(t\).
- \(k\) is the rate constant for the radioactive decay.
The half-life of a radioactive isotope (usually denoted by \(t_{1/2}\)) is a more familiar concept than \(k\) for radioactivity, so although Equation \(\ref{E3}\) is expressed in terms of \(k\), it is more usual to quote the value of \(t_{1/2}\). The currently accepted value for the half-life of 14 C is 5,730 years. This means that after 5,730 years, only half of the initial 14 C will remain; a quarter will remain after 11,460 years; an eighth after 17,190 years; and so on.
The equation relating rate constant to half-life for first order kinetics is
\[ k = \dfrac{\ln 2}{ t_{1/2} } \label{E4}\]
so the rate constant is then
\[ k = \dfrac{\ln 2}{5.73 \times 10^3} = 1.21 \times 10^{-4} \text{year}^{-1} \label{E5}\]
and Equation \(\ref{E2}\) can be rewritten as
\[N_t= N_o e^{-\ln 2 \;t/t_{1/2}} \label{E6}\]
\[t = \left(\dfrac{\ln \dfrac{N_o}{N_t}}{\ln 2} \right) t_{1/2} = 8267 \ln \dfrac{N_o}{N_t} = 19035 \log_{10} \dfrac{N_o}{N_t} \;\;\; (\text{in years}) \label{E7}\]
The sample is assumed to have originally had the same 14 C/ 12 C ratio as the ratio in the atmosphere, and since the size of the sample is known, the total number of atoms in the sample can be calculated, yielding \(N_0\), the number of 14 C atoms in the original sample. Measurement of N, the number of 14 C atoms currently in the sample, allows the calculation of \(t\), the age of the sample, using the Equation \(\ref{E7}\).
Deriving Equation \(\ref{E7}\) assumes that the level of 14 C in the atmosphere has remained constant over time. However, the level of 14 C in the atmosphere has varied significantly, so time estimated by Equation \(\ref{E7}\) must be corrected by using data from other sources.
Example 1: Dead Sea Scrolls
In 1947, samples of the Dead Sea Scrolls were analyzed by carbon dating. It was found that the carbon-14 present had an activity (rate of decay) of d/min.g (where d = disintegration). In contrast, living material exhibit an activity of 14 d/min.g. Thus, using Equation \(\ref{E3}\),
\[\ln \dfrac{14}{11} = (1.21 \times 10^{-4}) t \nonumber\]
\[t= \dfrac{\ln 1.272}{1.21 \times 10^{-4}} = 2 \times 10^3 \text{years} \nonumber\]
From the measurement performed in 1947, the Dead Sea Scrolls were determined to be 2000 years old, giving them a date of 53 BC, and confirming their authenticity. This discovery is in contrast to the carbon dating results for the Turin Shroud that was supposed to have wrapped Jesus’ body. Carbon dating has shown that the cloth was made between 1260 and 1390 AD. Thus, the Turin Shroud was made over a thousand years after the death of Jesus.
Describes radioactive half-life and how to do some simple calculations using half-life.
The technique of radiocarbon dating was developed by Willard Libby and his colleagues at the University of Chicago in 1949. Emilio Segrè asserted in his autobiography that Enrico Fermi suggested the concept to Libby at a seminar in Chicago that year. Libby estimated that the steady-state radioactivity concentration of exchangeable carbon-14 would be about 14 disintegrations per minute (dpm) per gram. In 1960, Libby was awarded the Nobel Prize in chemistry for this work. He demonstrated the accuracy of radiocarbon dating by accurately estimating the age of wood from a series of samples for which the age was known, including an ancient Egyptian royal barge dating from 1850 BCE. Before Radiocarbon dating was discovered, someone had to find the existence of the 14 C isotope. In 1940, Martin Kamen and Sam Ruben at the University of California, Berkeley Radiation Laboratory did just that. They found a form, an isotope, of Carbon that contained 8 neutrons and 6 protons. Using this finding, Willard Libby and his team at the University of Chicago proposed that Carbon-14 was unstable and underwent a total of 14 disintegrations per minute per gram. Using this hypothesis, the initial half-life he determined was 5568, give or take 30 years. The accuracy of this proposal was proven by dating a piece of wood from an Ancient Egyptian barge, the age of which was already known. From that point on, scientists have used these techniques to examine fossils, rocks, and ocean currents; as well as to determine age and event timing. Throughout the years, measurement tools have become more technologically advanced, allowing researchers to be more precise. We now use what is known as the Cambridge half-life of 5730+/- 40 years for Carbon-14. Although it may be seen as outdated, many labs still use Libby's half-life in order to stay consistent in publications and calculations within the laboratory. From the discovery of Carbon-14 to radiocarbon dating of fossils, we can see what an essential role Carbon has played and continues to play in our lives today.
The entire process of Radiocarbon dating depends on the decay of carbon-14. This process begins when an organism is no longer able to exchange Carbon with its environment. Carbon-14 is first formed when cosmic rays in the atmosphere allow for excess neutrons to be produced, which then react with Nitrogen to produce a constantly replenishing supply of carbon-14 to exchange with organisms.
- Carbon-14 dating can be used to estimate the age of carbon-bearing materials up to about 58,000 to 62,000 years old.
- The carbon-14 isotope would vanish from Earth's atmosphere in less than a million years were it not for the constant influx of cosmic rays interacting with atmospheric nitrogen.
- One of the most frequent uses of radiocarbon dating is to estimate the age of organic remains from archeological sites.
- Hua, Quan. "Radiocarbon: A Chronological Tool for the Recent Past." Quaternary Geochronology 4.5(2009):378-390. Science Direct . Web. 22 Nov. 2009.
- Petrucci, Ralph H. General Chemistry: Principles and Modern Applications 9th Ed. New Jersey: Pearson Education Inc. 2007.
- "Radio Carbon Dating." BBC- Homepage . 25 Oct. 2001. Web. 22 Nov. 2009. http://www.bbc.co.uk .
- Willis, E.H., H. Tauber, and K. O. Munnich. "Variations in the Atmospheric Radiocarbon Concentration Over the Past 1300 Years." American Journal of Science Radiocarbon Supplement 2(1960) 1-4. Print.
- If, when a hippopotamus lived, there was a total of 25 grams of Carbon-14, how many grams will remain 5730 years after he is laid to rest? 12.5 grams, because one half-life has occurred.
- How many grams of Carbon-14 will be present in the hippopotamus' remains after three half-lives have passed? 3.125 grams of Carbon-14 will remain after three half-lives.
Contributors and Attributions
- Template:ContribBarron
Boundless (www.boundless.com)
NOTIFICATIONS
Radiocarbon dating.
- + Create new collection
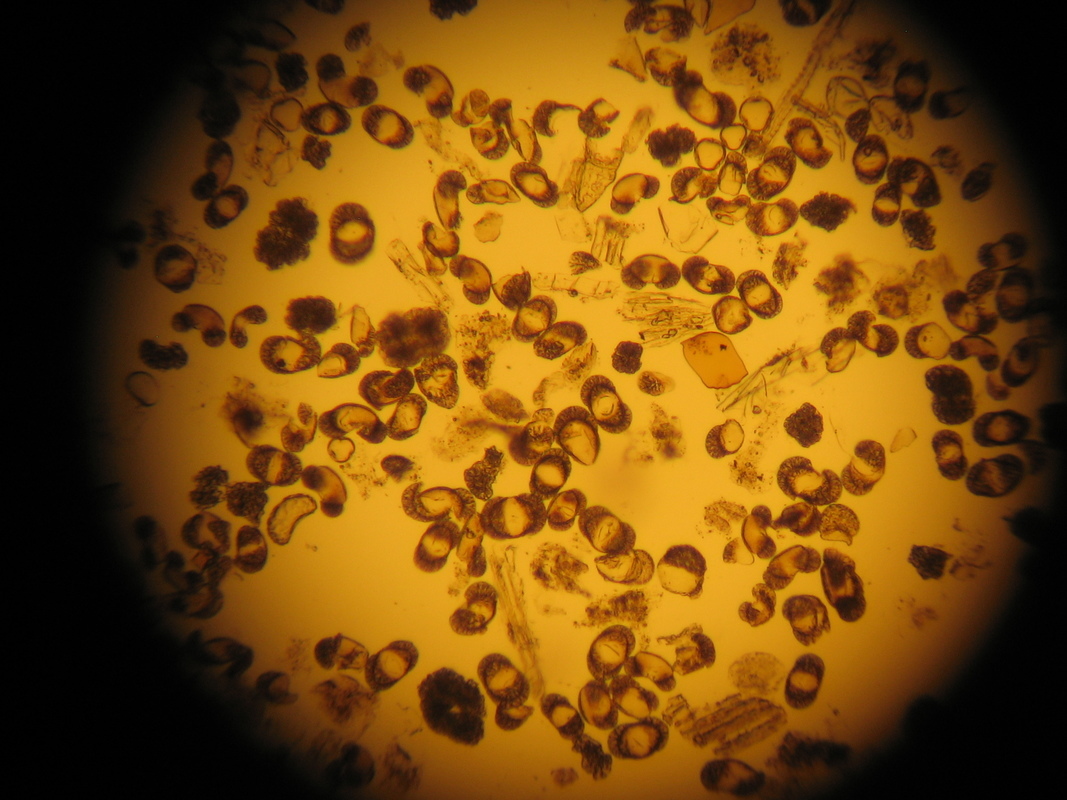
When an organism dies, it no longer absorbs carbon-14. The C-14 it does contain in its tissues starts to decay at a constant rate.
Name : Radiocarbon dating
Material used : Organic remains such as wood and seeds
Age range : Younger than 60,000 years ago
How it works : Measures the amount of radioactive carbon-14 in the organic remains of living things

Absolute dating methods
Absolute dating methods give rocks an actual date or date range in numbers of years. This interactive explores four different methods used in absolute dating.
See our newsletters here .
Would you like to take a short survey?
This survey will open in a new tab and you can fill it out after your visit to the site.
- Search Menu
- Browse content in Arts and Humanities
- Browse content in Archaeology
- Anglo-Saxon and Medieval Archaeology
- Archaeological Methodology and Techniques
- Archaeology by Region
- Archaeology of Religion
- Archaeology of Trade and Exchange
- Biblical Archaeology
- Contemporary and Public Archaeology
- Environmental Archaeology
- Historical Archaeology
- History and Theory of Archaeology
- Industrial Archaeology
- Landscape Archaeology
- Mortuary Archaeology
- Prehistoric Archaeology
- Underwater Archaeology
- Urban Archaeology
- Zooarchaeology
- Browse content in Architecture
- Architectural Structure and Design
- History of Architecture
- Residential and Domestic Buildings
- Theory of Architecture
- Browse content in Art
- Art Subjects and Themes
- History of Art
- Industrial and Commercial Art
- Theory of Art
- Biographical Studies
- Byzantine Studies
- Browse content in Classical Studies
- Classical History
- Classical Philosophy
- Classical Mythology
- Classical Literature
- Classical Reception
- Classical Art and Architecture
- Classical Oratory and Rhetoric
- Greek and Roman Papyrology
- Greek and Roman Epigraphy
- Greek and Roman Law
- Greek and Roman Archaeology
- Late Antiquity
- Religion in the Ancient World
- Digital Humanities
- Browse content in History
- Colonialism and Imperialism
- Diplomatic History
- Environmental History
- Genealogy, Heraldry, Names, and Honours
- Genocide and Ethnic Cleansing
- Historical Geography
- History by Period
- History of Emotions
- History of Agriculture
- History of Education
- History of Gender and Sexuality
- Industrial History
- Intellectual History
- International History
- Labour History
- Legal and Constitutional History
- Local and Family History
- Maritime History
- Military History
- National Liberation and Post-Colonialism
- Oral History
- Political History
- Public History
- Regional and National History
- Revolutions and Rebellions
- Slavery and Abolition of Slavery
- Social and Cultural History
- Theory, Methods, and Historiography
- Urban History
- World History
- Browse content in Language Teaching and Learning
- Language Learning (Specific Skills)
- Language Teaching Theory and Methods
- Browse content in Linguistics
- Applied Linguistics
- Cognitive Linguistics
- Computational Linguistics
- Forensic Linguistics
- Grammar, Syntax and Morphology
- Historical and Diachronic Linguistics
- History of English
- Language Evolution
- Language Reference
- Language Acquisition
- Language Variation
- Language Families
- Lexicography
- Linguistic Anthropology
- Linguistic Theories
- Linguistic Typology
- Phonetics and Phonology
- Psycholinguistics
- Sociolinguistics
- Translation and Interpretation
- Writing Systems
- Browse content in Literature
- Bibliography
- Children's Literature Studies
- Literary Studies (Romanticism)
- Literary Studies (American)
- Literary Studies (Asian)
- Literary Studies (European)
- Literary Studies (Eco-criticism)
- Literary Studies (Modernism)
- Literary Studies - World
- Literary Studies (1500 to 1800)
- Literary Studies (19th Century)
- Literary Studies (20th Century onwards)
- Literary Studies (African American Literature)
- Literary Studies (British and Irish)
- Literary Studies (Early and Medieval)
- Literary Studies (Fiction, Novelists, and Prose Writers)
- Literary Studies (Gender Studies)
- Literary Studies (Graphic Novels)
- Literary Studies (History of the Book)
- Literary Studies (Plays and Playwrights)
- Literary Studies (Poetry and Poets)
- Literary Studies (Postcolonial Literature)
- Literary Studies (Queer Studies)
- Literary Studies (Science Fiction)
- Literary Studies (Travel Literature)
- Literary Studies (War Literature)
- Literary Studies (Women's Writing)
- Literary Theory and Cultural Studies
- Mythology and Folklore
- Shakespeare Studies and Criticism
- Browse content in Media Studies
- Browse content in Music
- Applied Music
- Dance and Music
- Ethics in Music
- Ethnomusicology
- Gender and Sexuality in Music
- Medicine and Music
- Music Cultures
- Music and Media
- Music and Religion
- Music and Culture
- Music Education and Pedagogy
- Music Theory and Analysis
- Musical Scores, Lyrics, and Libretti
- Musical Structures, Styles, and Techniques
- Musicology and Music History
- Performance Practice and Studies
- Race and Ethnicity in Music
- Sound Studies
- Browse content in Performing Arts
- Browse content in Philosophy
- Aesthetics and Philosophy of Art
- Epistemology
- Feminist Philosophy
- History of Western Philosophy
- Metaphysics
- Moral Philosophy
- Non-Western Philosophy
- Philosophy of Language
- Philosophy of Mind
- Philosophy of Perception
- Philosophy of Science
- Philosophy of Action
- Philosophy of Law
- Philosophy of Religion
- Philosophy of Mathematics and Logic
- Practical Ethics
- Social and Political Philosophy
- Browse content in Religion
- Biblical Studies
- Christianity
- East Asian Religions
- History of Religion
- Judaism and Jewish Studies
- Qumran Studies
- Religion and Education
- Religion and Health
- Religion and Politics
- Religion and Science
- Religion and Law
- Religion and Art, Literature, and Music
- Religious Studies
- Browse content in Society and Culture
- Cookery, Food, and Drink
- Cultural Studies
- Customs and Traditions
- Ethical Issues and Debates
- Hobbies, Games, Arts and Crafts
- Lifestyle, Home, and Garden
- Natural world, Country Life, and Pets
- Popular Beliefs and Controversial Knowledge
- Sports and Outdoor Recreation
- Technology and Society
- Travel and Holiday
- Visual Culture
- Browse content in Law
- Arbitration
- Browse content in Company and Commercial Law
- Commercial Law
- Company Law
- Browse content in Comparative Law
- Systems of Law
- Competition Law
- Browse content in Constitutional and Administrative Law
- Government Powers
- Judicial Review
- Local Government Law
- Military and Defence Law
- Parliamentary and Legislative Practice
- Construction Law
- Contract Law
- Browse content in Criminal Law
- Criminal Procedure
- Criminal Evidence Law
- Sentencing and Punishment
- Employment and Labour Law
- Environment and Energy Law
- Browse content in Financial Law
- Banking Law
- Insolvency Law
- History of Law
- Human Rights and Immigration
- Intellectual Property Law
- Browse content in International Law
- Private International Law and Conflict of Laws
- Public International Law
- IT and Communications Law
- Jurisprudence and Philosophy of Law
- Law and Politics
- Law and Society
- Browse content in Legal System and Practice
- Courts and Procedure
- Legal Skills and Practice
- Primary Sources of Law
- Regulation of Legal Profession
- Medical and Healthcare Law
- Browse content in Policing
- Criminal Investigation and Detection
- Police and Security Services
- Police Procedure and Law
- Police Regional Planning
- Browse content in Property Law
- Personal Property Law
- Study and Revision
- Terrorism and National Security Law
- Browse content in Trusts Law
- Wills and Probate or Succession
- Browse content in Medicine and Health
- Browse content in Allied Health Professions
- Arts Therapies
- Clinical Science
- Dietetics and Nutrition
- Occupational Therapy
- Operating Department Practice
- Physiotherapy
- Radiography
- Speech and Language Therapy
- Browse content in Anaesthetics
- General Anaesthesia
- Neuroanaesthesia
- Clinical Neuroscience
- Browse content in Clinical Medicine
- Acute Medicine
- Cardiovascular Medicine
- Clinical Genetics
- Clinical Pharmacology and Therapeutics
- Dermatology
- Endocrinology and Diabetes
- Gastroenterology
- Genito-urinary Medicine
- Geriatric Medicine
- Infectious Diseases
- Medical Toxicology
- Medical Oncology
- Pain Medicine
- Palliative Medicine
- Rehabilitation Medicine
- Respiratory Medicine and Pulmonology
- Rheumatology
- Sleep Medicine
- Sports and Exercise Medicine
- Community Medical Services
- Critical Care
- Emergency Medicine
- Forensic Medicine
- Haematology
- History of Medicine
- Browse content in Medical Skills
- Clinical Skills
- Communication Skills
- Nursing Skills
- Surgical Skills
- Browse content in Medical Dentistry
- Oral and Maxillofacial Surgery
- Paediatric Dentistry
- Restorative Dentistry and Orthodontics
- Surgical Dentistry
- Medical Ethics
- Medical Statistics and Methodology
- Browse content in Neurology
- Clinical Neurophysiology
- Neuropathology
- Nursing Studies
- Browse content in Obstetrics and Gynaecology
- Gynaecology
- Occupational Medicine
- Ophthalmology
- Otolaryngology (ENT)
- Browse content in Paediatrics
- Neonatology
- Browse content in Pathology
- Chemical Pathology
- Clinical Cytogenetics and Molecular Genetics
- Histopathology
- Medical Microbiology and Virology
- Patient Education and Information
- Browse content in Pharmacology
- Psychopharmacology
- Browse content in Popular Health
- Caring for Others
- Complementary and Alternative Medicine
- Self-help and Personal Development
- Browse content in Preclinical Medicine
- Cell Biology
- Molecular Biology and Genetics
- Reproduction, Growth and Development
- Primary Care
- Professional Development in Medicine
- Browse content in Psychiatry
- Addiction Medicine
- Child and Adolescent Psychiatry
- Forensic Psychiatry
- Learning Disabilities
- Old Age Psychiatry
- Psychotherapy
- Browse content in Public Health and Epidemiology
- Epidemiology
- Public Health
- Browse content in Radiology
- Clinical Radiology
- Interventional Radiology
- Nuclear Medicine
- Radiation Oncology
- Reproductive Medicine
- Browse content in Surgery
- Cardiothoracic Surgery
- Gastro-intestinal and Colorectal Surgery
- General Surgery
- Neurosurgery
- Paediatric Surgery
- Peri-operative Care
- Plastic and Reconstructive Surgery
- Surgical Oncology
- Transplant Surgery
- Trauma and Orthopaedic Surgery
- Vascular Surgery
- Browse content in Science and Mathematics
- Browse content in Biological Sciences
- Aquatic Biology
- Biochemistry
- Bioinformatics and Computational Biology
- Developmental Biology
- Ecology and Conservation
- Evolutionary Biology
- Genetics and Genomics
- Microbiology
- Molecular and Cell Biology
- Natural History
- Plant Sciences and Forestry
- Research Methods in Life Sciences
- Structural Biology
- Systems Biology
- Zoology and Animal Sciences
- Browse content in Chemistry
- Analytical Chemistry
- Computational Chemistry
- Crystallography
- Environmental Chemistry
- Industrial Chemistry
- Inorganic Chemistry
- Materials Chemistry
- Medicinal Chemistry
- Mineralogy and Gems
- Organic Chemistry
- Physical Chemistry
- Polymer Chemistry
- Study and Communication Skills in Chemistry
- Theoretical Chemistry
- Browse content in Computer Science
- Artificial Intelligence
- Computer Architecture and Logic Design
- Game Studies
- Human-Computer Interaction
- Mathematical Theory of Computation
- Programming Languages
- Software Engineering
- Systems Analysis and Design
- Virtual Reality
- Browse content in Computing
- Business Applications
- Computer Security
- Computer Games
- Computer Networking and Communications
- Digital Lifestyle
- Graphical and Digital Media Applications
- Operating Systems
- Browse content in Earth Sciences and Geography
- Atmospheric Sciences
- Environmental Geography
- Geology and the Lithosphere
- Maps and Map-making
- Meteorology and Climatology
- Oceanography and Hydrology
- Palaeontology
- Physical Geography and Topography
- Regional Geography
- Soil Science
- Urban Geography
- Browse content in Engineering and Technology
- Agriculture and Farming
- Biological Engineering
- Civil Engineering, Surveying, and Building
- Electronics and Communications Engineering
- Energy Technology
- Engineering (General)
- Environmental Science, Engineering, and Technology
- History of Engineering and Technology
- Mechanical Engineering and Materials
- Technology of Industrial Chemistry
- Transport Technology and Trades
- Browse content in Environmental Science
- Applied Ecology (Environmental Science)
- Conservation of the Environment (Environmental Science)
- Environmental Sustainability
- Environmentalist Thought and Ideology (Environmental Science)
- Management of Land and Natural Resources (Environmental Science)
- Natural Disasters (Environmental Science)
- Nuclear Issues (Environmental Science)
- Pollution and Threats to the Environment (Environmental Science)
- Social Impact of Environmental Issues (Environmental Science)
- History of Science and Technology
- Browse content in Materials Science
- Ceramics and Glasses
- Composite Materials
- Metals, Alloying, and Corrosion
- Nanotechnology
- Browse content in Mathematics
- Applied Mathematics
- Biomathematics and Statistics
- History of Mathematics
- Mathematical Education
- Mathematical Finance
- Mathematical Analysis
- Numerical and Computational Mathematics
- Probability and Statistics
- Pure Mathematics
- Browse content in Neuroscience
- Cognition and Behavioural Neuroscience
- Development of the Nervous System
- Disorders of the Nervous System
- History of Neuroscience
- Invertebrate Neurobiology
- Molecular and Cellular Systems
- Neuroendocrinology and Autonomic Nervous System
- Neuroscientific Techniques
- Sensory and Motor Systems
- Browse content in Physics
- Astronomy and Astrophysics
- Atomic, Molecular, and Optical Physics
- Biological and Medical Physics
- Classical Mechanics
- Computational Physics
- Condensed Matter Physics
- Electromagnetism, Optics, and Acoustics
- History of Physics
- Mathematical and Statistical Physics
- Measurement Science
- Nuclear Physics
- Particles and Fields
- Plasma Physics
- Quantum Physics
- Relativity and Gravitation
- Semiconductor and Mesoscopic Physics
- Browse content in Psychology
- Affective Sciences
- Clinical Psychology
- Cognitive Psychology
- Cognitive Neuroscience
- Criminal and Forensic Psychology
- Developmental Psychology
- Educational Psychology
- Evolutionary Psychology
- Health Psychology
- History and Systems in Psychology
- Music Psychology
- Neuropsychology
- Organizational Psychology
- Psychological Assessment and Testing
- Psychology of Human-Technology Interaction
- Psychology Professional Development and Training
- Research Methods in Psychology
- Social Psychology
- Browse content in Social Sciences
- Browse content in Anthropology
- Anthropology of Religion
- Human Evolution
- Medical Anthropology
- Physical Anthropology
- Regional Anthropology
- Social and Cultural Anthropology
- Theory and Practice of Anthropology
- Browse content in Business and Management
- Business Ethics
- Business Strategy
- Business History
- Business and Technology
- Business and Government
- Business and the Environment
- Comparative Management
- Corporate Governance
- Corporate Social Responsibility
- Entrepreneurship
- Health Management
- Human Resource Management
- Industrial and Employment Relations
- Industry Studies
- Information and Communication Technologies
- International Business
- Knowledge Management
- Management and Management Techniques
- Operations Management
- Organizational Theory and Behaviour
- Pensions and Pension Management
- Public and Nonprofit Management
- Strategic Management
- Supply Chain Management
- Browse content in Criminology and Criminal Justice
- Criminal Justice
- Criminology
- Forms of Crime
- International and Comparative Criminology
- Youth Violence and Juvenile Justice
- Development Studies
- Browse content in Economics
- Agricultural, Environmental, and Natural Resource Economics
- Asian Economics
- Behavioural Finance
- Behavioural Economics and Neuroeconomics
- Econometrics and Mathematical Economics
- Economic History
- Economic Systems
- Economic Methodology
- Economic Development and Growth
- Financial Markets
- Financial Institutions and Services
- General Economics and Teaching
- Health, Education, and Welfare
- History of Economic Thought
- International Economics
- Labour and Demographic Economics
- Law and Economics
- Macroeconomics and Monetary Economics
- Microeconomics
- Public Economics
- Urban, Rural, and Regional Economics
- Welfare Economics
- Browse content in Education
- Adult Education and Continuous Learning
- Care and Counselling of Students
- Early Childhood and Elementary Education
- Educational Equipment and Technology
- Educational Strategies and Policy
- Higher and Further Education
- Organization and Management of Education
- Philosophy and Theory of Education
- Schools Studies
- Secondary Education
- Teaching of a Specific Subject
- Teaching of Specific Groups and Special Educational Needs
- Teaching Skills and Techniques
- Browse content in Environment
- Applied Ecology (Social Science)
- Climate Change
- Conservation of the Environment (Social Science)
- Environmentalist Thought and Ideology (Social Science)
- Natural Disasters (Environment)
- Social Impact of Environmental Issues (Social Science)
- Browse content in Human Geography
- Cultural Geography
- Economic Geography
- Political Geography
- Browse content in Interdisciplinary Studies
- Communication Studies
- Museums, Libraries, and Information Sciences
- Browse content in Politics
- African Politics
- Asian Politics
- Chinese Politics
- Comparative Politics
- Conflict Politics
- Elections and Electoral Studies
- Environmental Politics
- European Union
- Foreign Policy
- Gender and Politics
- Human Rights and Politics
- Indian Politics
- International Relations
- International Organization (Politics)
- International Political Economy
- Irish Politics
- Latin American Politics
- Middle Eastern Politics
- Political Behaviour
- Political Economy
- Political Institutions
- Political Methodology
- Political Communication
- Political Philosophy
- Political Sociology
- Political Theory
- Politics and Law
- Politics of Development
- Public Policy
- Public Administration
- Quantitative Political Methodology
- Regional Political Studies
- Russian Politics
- Security Studies
- State and Local Government
- UK Politics
- US Politics
- Browse content in Regional and Area Studies
- African Studies
- Asian Studies
- East Asian Studies
- Japanese Studies
- Latin American Studies
- Middle Eastern Studies
- Native American Studies
- Scottish Studies
- Browse content in Research and Information
- Research Methods
- Browse content in Social Work
- Addictions and Substance Misuse
- Adoption and Fostering
- Care of the Elderly
- Child and Adolescent Social Work
- Couple and Family Social Work
- Developmental and Physical Disabilities Social Work
- Direct Practice and Clinical Social Work
- Emergency Services
- Human Behaviour and the Social Environment
- International and Global Issues in Social Work
- Mental and Behavioural Health
- Social Justice and Human Rights
- Social Policy and Advocacy
- Social Work and Crime and Justice
- Social Work Macro Practice
- Social Work Practice Settings
- Social Work Research and Evidence-based Practice
- Welfare and Benefit Systems
- Browse content in Sociology
- Childhood Studies
- Community Development
- Comparative and Historical Sociology
- Economic Sociology
- Gender and Sexuality
- Gerontology and Ageing
- Health, Illness, and Medicine
- Marriage and the Family
- Migration Studies
- Occupations, Professions, and Work
- Organizations
- Population and Demography
- Race and Ethnicity
- Social Theory
- Social Movements and Social Change
- Social Research and Statistics
- Social Stratification, Inequality, and Mobility
- Sociology of Religion
- Sociology of Education
- Sport and Leisure
- Urban and Rural Studies
- Browse content in Warfare and Defence
- Defence Strategy, Planning, and Research
- Land Forces and Warfare
- Military Administration
- Military Life and Institutions
- Naval Forces and Warfare
- Other Warfare and Defence Issues
- Peace Studies and Conflict Resolution
- Weapons and Equipment

- < Previous chapter
- Next chapter >

Radiocarbon Dating in Rock Art Research
Fiona Petchey is Deputy Director of the radiocarbon facility at the University of Waikato, Waikato.
- Published: 06 March 2017
- Cite Icon Cite
- Permissions Icon Permissions
Radiocarbon dating has had a significant impact on rock art research, but an initial enthusiasm for this dating method by archaeologists has been replaced by a degree of scepticism. Radiocarbon dates undertaken directly on rock art or on associated mineral crusts have often reinforced such scepticism, in part because organic carbon-based materials are present in small quantities and their composition is of such variable composition that the technique is stretched to its limits. For the researcher planning to obtain radiocarbon dates, it is essential to have an understanding of the dating options available, limitations of the technique, the potential impact of their own bias, and the value of a dating programme that is fully integrated within a larger project. This chapter outlines the various materials and methods used to radiocarbon date rock art. It includes some recent examples and highlights some advances as well as shortfalls in the dating of rock art.
Introduction
Radiocarbon dating remains the most widely applicable, accurate, and reliable chronometric dating technique available to archaeologists. Radiocarbon (also called 14 C) dating measures the concentration of naturally occurring radioactive 14 C that is formed in the upper atmosphere. 14 C is an unstable, or radioactive, isotope because it contains extra neutrons in its nucleus and, as a consequence, will eventually return to a more stable 14 N isotope by the emission of a negatively charged beta particle. 14 C becomes incorporated into all living things through the nutrients they ingest, and the level of 14 C in a plant or animal is therefore maintained in near equilibrium until an organism dies. The decay rate is considered to be constant, and it is therefore possible to estimate the time since death by comparing the amount of 14 C in a dead organism to modern levels. The technique can date back to around 60,000 years ago, but this varies depending on the material, laboratory, and experimental conditions (for a detailed introduction to 14 C use in archaeology, see Taylor & Bar Yosef 2014 ).
The ability to use 14 C dating was out of the reach of most rock art researchers until the introduction of accelerator mass spectrometer (AMS) dating in the late 1970s. This technique has enabled samples as small as a grain of rice to be routinely dated, which in turn results in minimal damage to the artefact or surface sampled while also expanding the range of materials that could be investigated. Despite this advantage, it was more than a decade beforeHedges et al. ( Hedges, Housley, Law, Perry, & Gowlett 1987 ) and van der Merwe et al. ( van der Merwe, Sealy, & Yates 1987 ) first applied 14 C to rock art. Since then, advances in the AMS technique have enabled ever-smaller quantities (now micrograms) of carbon to be dated routinely, as well as previously untested materials. Such refinements have not been undertaken without considerable effort and associated uncertainty, and critical questions should be addressed by those wishing to demonstrate the reliability of their dates. These questions include: What is the source and nature of the organic material to be 14 C-dated?What is the reproducibility of 14 C values derived from a given sample? And, what is the relationship of that sample to the event in question? Unfortunately, researchers investigating rock art have not always adequately addressed these issues.
In the study of rock art, the identification of exactly what material is being dated is difficult because most samples are small, amorphous, and of uncertain chemistry. Consequently, it can be argued that the most significant recent advances in rock art dating actually fall outside the realm of 14 C, instead focussing on material characterization ( Bonneau, Brock, Higham, Pearce, & Pollard 2011 ; Livingston, Robinson, & Armitage 2009 ; López-Montalvo, Villaverde, Roldán, Murcia, & Badal 2014 ; Mori et al. 2006 ; Vazquez, Maier, Parera, Yacobaccio, & Sola 2008 ). Rather than being driven by the need to demonstrate 14 C reliability, the use of chemical characterization techniques such as gas chromatography, X-ray fluorescence, and Raman spectroscopy has been fuelled by an interest in the provenance of raw materials and the technical processes used to prepare the artworks. The introduction of portable machines with improved detection limits, especially X-ray fluorescence, has enabled the characterization of material in the field prior to sampling ( Beck et al. 2013 ; McDonald et al. 2014 ). This in itself promises greater control and more sample selection opportunities to those investigating the age of rock art. It also highlights the importance of participation by specialists in each specific field of analysis from the earliest stages of sample selection.
The reproducibility and accuracy of rock art dates can also be problematic. Many of the preparation methodologies used to remove contaminants are, by necessity, very general, while others—designed specifically for a particular sample type—are experimental. There is a large body of literature discussing disputed results (e.g., Combier & Jouve 2012 ; Gillespie 1997 ; Nelson 1993 ; Pettitt & Bahn 2003 ; Watchman 1999 ; Valladas & Clottes 2003 ), in part because of the limited amount of (published) information on sampling methodology, location, and sample chemistry pre- and post-treatment, but also because of failure to demonstrate the ability to replicate results with blind and repeated tests ( Gowlett & Hedges 1986 ). Although researchers have only relatively recently started to comprehensively address these issues, it should be stressed that the process for the majority of rock art samples is no more “non-routine” or “experimental” ( Pettit & Pike 2007 :37) than other dating endeavours.
Last, the relationship of the sample to the target event is critical. This is entirely the responsibility of the person collecting and submitting the sample for dating and, by implication, necessitates the researcher understanding the limitations of the preparation methodologies applied. Unfortunately, disassociation between the event and date is the most common form of anomaly between 14 C age and expected age, usually because of the complexity of achieving such a goal when dealing with dynamic and complex contexts ( Taylor & Bar Yosef 2014 :132–136). Whether it is “premature to construct grand schemes or make meaningful generalizations” ( Pettitt & Pike 2007 :28) is open to debate, but this attitude may in part explain the slow uptake of Bayesian methodologies that could enable further refinement of chronologies by rock art researchers.
Relative and Associative Dating
Ultimately, the best way to establish the age of rock art is to utilize a holistic approach that incorporates all dating evidence, both chronometric and relative (e.g., superimposition of art works, stratigraphic sequence, stylistic attributes or diagnostic subject matter, differential weathering and archaeological associations by excavation) ( Aubert 2012 ; Bednarik 2002 ). While this multifaceted approach is commonplace for archaeological investigations generally, these associations are not always obvious for rock art ( Whitley 2012 ), and perceived stylistic relationships, while useful, can be contentious with interpretations limited by our own constructs ( Aubert 2012 ).
Regardless, such evaluation has become a valued component of rock art projects, such as at Nawarla Gabarnmang—a large sandstone shelter in central-western Arnhem Land, Australia, where occupation dates from the past few hundred years to back beyond 45,000 cal bp ( David et al. 2011 ). To provide a robust chronological sequence, Gunn et al. ( Gunn, David, Delannoy, & Katherine 2017 ) plotted patterns of superimposition for overlapping designs found on three ceiling panels by cross-correlating common artistic traits. They were able to combine beeswax radiocarbon dates from the identified micro-layers of superimposed pigment artworks with additional dating information obtained from a panel that displayed a painted motif thought to represent a horse, which would have been unknown on the plateau before ad 1845 (Figure 1 ). Using these relative and chronometric dating methods, three periods were established for the artworks: that older than circa ad 1430; between circa ad 1430 and circa ad 1640; and between circa ad 1640 and ad 1930. This work demonstrated that the recent artistic repertoire at Nawarla Gabarnmang included a number of changes in colour and motif types.

Nawarla Gabarnmang horse motif from Panel D1
An extension of this dating program was undertaken using excavated materials from sediment at the base of the rock art and spalls from the art itself to provide terminus ante or post quem ages for the formation of that art. At Nawarla Gabarnmang, a small fragment of a painted slab was found at stratigraphic levels containing mixed 14 C dates between 21,252 cal bp and 36,270 cal bp ( David et al. 2013 ), and a sample of charcoal-rich ash adhering to the back of the pained rock returned an age of 26,913–28,348 cal bp . To demonstrate the interrelationship of the art and each stratigraphic level, the Nawarla Gabarnmang dates were coupled with a geomorphological investigation of the cave structure. This resulted in a detailed understanding of the relative chronology of roof-fall events, the evolution of the cave, and past interactions of people within the space as they modified the environment. On this basis, it was concluded that the painting itself was made shortly prior to a major roof–fall event and dated to circa 28,000–27,000 cal bp (David et al. 2013 , 2014 ).
Direct Dating
Most rock art dating has been undertaken on black organic pigments of which charcoal is assumed to be the primary ingredient, but a wide range of inorganic pigments can be found, including red, orange, and brown ochre; iron oxides; and black manganese oxide or hydroxide. These can be dated if an organic binder is present in the paint matrix. The interpretation of dates from any material is complicated by the fact that we are not necessarily dating the painting event, but the uptake of 14 C prior to death by the organism used in the paint. In most cases, the difference between the age of the targeted event and that of the material dated is small, but large offsets are possible, as was the case for bird track designs from the Canning Stock Route in the Western Desert of Australia. These designs were expected to date to the time of early nineteenth- and twentieth-century droving activity along the route, but ages of 12,970±270 bp , 12,620±460 bp , and 5520±290 bp suggested to McDonald et al. (2014 :200) that the paints used contained a petroleum-based binder resulting in values that reflected this ancient 14 C source.
Regardless of the material, when selecting samples for dating, it is essential that the extraction of organic carbon and subsequent purification is free from contamination. For rock art samples, this is nearly impossible because of the large surface area, amorphous nature of the paint, and small sample sizes; the researcher has to consider the potential inclusion of fungi, algae, lichens and microbes, insects, rootlets, dust, and soil ( Ridges, Davidson, & Tucker 2000 ), all of which can be found naturally on rock surfaces, while the very presence of humans will often accelerate the growth of a wide range of organisms on the applied paint ( Valladas 2003 ). There is also the possibility of subsequent animal and anthropogenic activity. This can include both ancient (e.g., at Cova Remigia in Spain, where a two-colour paint combination was due to ancient retouching and produced art work that deviated from the more typical monochromatic drawings of the region [ López-Montalvo et al. 2014 ]) and modern activities (e.g., the application of kerosene during modern times to aid photography at Great Gallery, Horseshoe Canyon, Utah [ Chaffee, Hyman, & Rowe 1994 ]). Often the impact of recent, chemically diverse contamination may be complex, and care needs to be taken when evaluating the impact of different contaminants (e.g., burning of tyres could result in either old or young results depending on whether they were manufactured from fossil petrochemical sources or natural modern materials; Steelman, Carrera Ramírez, Fábregas Valcarce, Guilderson, & Rowe [2005 :387] argued that a young age for site M10 at Coto dos Mouros, northwest Iberia, was caused by contamination from burning tyres made from natural rubber products).
Protocols for sampling and reporting rock art dates are well documented (e.g., Steelman & Rowe 2012 :572–573; Watchman 1999 ) and are not repeated here. The primary concern of these authors has been to ensure minimal damage to the artwork while maximizing sample size and the retention of valuable information. These works are undoubtedly useful guides, but the wide variety of datable materials and environmental conditions encountered mean that they can only be taken as a rough indication of what is required. Without prior knowledge of a site, the artwork, and potential contaminants, it is very difficult to sample effectively, as demonstrated by the complexity encountered when dating artwork from along the Canning Stock Route ( McDonald et al. 2014 ). Here, where possible, the sampling strategy included the measurement of replicates from a single motif or superimposed paint layers, as well as rock samples from adjacent unpainted areas to investigate natural levels of organic contamination. Of the “charcoal” drawings sampled, there was only a 53% dating success rate, and few of the collected charcoal samples produced sufficient carbon to enable results to be duplicated adequately or at all (e.g., duplicate samples from a black snake outline at Pinpi 5, in the Jilakurru Ranges, weighing 6 milligrams and 8 milligrams, returned results of 225±40 bp and 470±270 bp , respectively. The large error range on the 8 milligram sample is indicative of extremely low levels of carbon).
Charcoal and Carbon Black
Charcoal is a very popular material for dating. This stems from the fact that it has a high carbon content and is relatively chemically stable. Charcoal dating, is not, however, without issues. Of particular concern when dealing with charcoal, and derivatives such as soot, is the old wood and/or old charcoal effect (also called inbuilt age ) that can add an unknown degree of older carbon into a sample depending on the age of the plant combusted. Related to this is a storage effect by which charcoal or other materials may have been curated for some time before being utilized in the paint ( Schiffer 1987 ). In both cases, the exact magnitude of error is impossible to predict because the impact depends on the age of the art and contaminants. An extreme example of this has been put forward by Combier and Jovue (2012) , who suggested that the addition of fossil carbon in the form of lignite or bitumen caused artificially old ( circa 32,000 bp) dates from Chauvet-Pont-d’Arc Cave in the Ardèche region of southern France. Such a hypothesis can be controversial and difficult to prove either way, in part because of complexities associated with small sample size and contaminant removal that can result in disparate duplicate analyses, but also because of complexities with site interpretation. Indeed, this criticism has been put forward for the Chauvet Cave chronology despite the fact that it has been subjected to an intensive dating regime involving multiple dating techniques, test excavations, and superimposition studies that give confidence to the chronological evaluation ( Clottes & Geneste 2012 ; see also Pettitt & Bahn 2015 ; Quiles et al. 2016 ).
The pretreatment of charcoal commonly involves a succession of ‘acid-base-acid’ washes that vary in length and intensity according to sample size and material robusticity. These pretreatments theoretically remove humic acids (a major organic component of soil made up of a mixture of different acids formed from the decay of plant and microbial remains), carbonates (introduced via groundwater contact with limestone and other carbonaceous sediments), fulvic acids (humic acids of lower molecular weight and higher oxygen content), and atmospheric carbon dioxide (CO 2 ) that has been absorbed onto the surface of the sample. A low-temperature ( circa 300°C) combustion step may also be added to remove the most liable fraction ( Bird et al. 2010 ; Sand et al. 2006 ; Valladas 2003 ). Success is, however, not guaranteed: chemical characterization of pretreated samples has demonstrated that contaminants may remain that cannot always be adequately accounted for ( Alon, Mintz, Cohen, Weiner, & Boaretto 2002 ; Livingston et al. 2009 ).
Most black rock art samples submitted for 14 C analysis are highly amorphous, burnt organic materials of unknown origin. Commonly called carbon black , this terminology hides the fact that this material can originate from a wide variety of sources, including incomplete combustion products of substances such as wood, charcoal, fruit, bone, resins, oil, and fat (also variously termed ‘vegetable or plant black’, ‘ivory/bone black’, or ‘lampblack’) ( Bonneau et al. 2011 ). Such materials are less stable chemically than charcoal and often completely dissolve if routine pretreatment methods are used. Moreover, contaminants, including carbon-containing oxalate minerals (CaC 2 O 4 ) derived from some rock surfaces (see the later section on Mineral Accretions), may become preferentially concentrated in the sample during pretreatment ( Hedges et al. 1998 :36–37).
Many experimental pretreatments have been devised to forgo or minimize these wet chemistry steps. One such technique is plasma-oxidation ashing . This uses a low-temperature, low-pressure oxygen plasma that is excited by an oscillating electric field. The temperature of the gas components is increased by elastic collisions between the electrons and the gas, which result in the electrons becoming sufficiently energetic to break molecular bonds while the gas remains at temperatures below 150°C (see Russ, Hyman, Shafer, & Rowe 1990 , 1991 and Rowe 2009 for methodology). Theoretically, this methodology prevents the decomposition of carbonate and oxalate minerals that occur at the higher temperatures ( circa 800°C) used during more traditional radiocarbon combustion techniques. This process can be used to remove organic contaminants by the controlled reduction of a percentage of the starting mass prior to recombustion at a higher temperature ( Bird et al. 2010 ) or to selectively remove and date tiny amounts of organic binder from the surface of a sample ( Rowe 2009 ). Researchers are divided on the reliability of plasma-oxidation. Steelman and Rowe (2012 :577–578) concluded that replicate measurements on the same image suggested an uncertainty of ±250 years and that their tests were more successful in limestone environments than in sandstone environments. Investigations by Bird et al. (2010) also indicated that plasma-oxidation could not discriminate between similar organic components. This means that the wet chemistry approaches used to remove humics prior to combustion are still recommended if possible (cf. McDonald et al. 2014 ).
One novel approach to removing contaminants was implemented by Bonneau et al. (2011) who used a density separation technique previously used on shells ( Douka, Hedges, & Higham 2010 ) to remove calcium oxalates (density of 2.0–2.2 g/cm 3 ) from black pigments (density 1.5 g/cm 3 ). Although Fourier transform infrared (FTIR) spectroscopy indicated that the black pigment was free from contaminants, the expense of the heavy liquid (sodium polytungstate) and the possibility that the calcium carbonates and oxalates had chemically bonded to the black pigment meant that the technique was not considered to be viable. Experimentation with different preparation methodologies to find an uncontaminated form of autochthonous carbon is sure to continue.
Confirmation of minimal post-depositional contamination using chemical characterization of both the paint and unpainted rock substrateis becoming more common in rock art dating. Bonneau et al. (2011) used both Raman spectroscopy and FTIR to evaluate the presence of calcium oxalates in black, noncharcoal pigments from RSATYN2, a painted rockshelter in the Drakensberg Mountains (South Africa). They evaluated the success of different pretreatments to ensure the removal of calcium oxalate (Raman peak at 1,474 cm -1 ) and gypsum (peak at 1,005 cm -1 ) prior to dating. They were able to confirm by Raman peaks at ~1,360 cm -1 and ~1,580 cm -1 that the samples were typical of amorphous carbon, while the absence of characteristic calcium oxalate (3,600–3,400 cm -1 , 1,616 cm -1 , 1,315 cm -1 ) and calcium carbonate (1,429 cm -1 , 877 cm -1 , and 713 cm -1 ) peaks in the FTIR spectra after pretreatment indicated the success of the acid treatment (Figure 2 ).
Fourier transform infrared (FTIR) spectra of rock art sample taken from RSATYN2, in the Drakensberg Mountains showing progressive contaminant removal after different acid treatments (H 2 SO 4 and HCl) (x: Wavenumber cm -1 ; y: FTIR intensity)
As noted earlier, improvements in analytical techniques have seen the advent of portable systems that enable chemical evaluation in the field prior to sampling. Beck et al. (2013) tested a portable X-ray fluorescence (XRF) elemental analyser and a Raman spectrometer to investigate art from the caves of Villars and Rouffignac in the Dordogne region of France. Although XRF cannot detect carbon directly, the absence of heavier elements such as manganese or iron—which are black and therefore difficult to distinguish from carbon—lent support to the carbon nature of the black pigments and enabled the researchers to isolate and sample scorch marks on the rock surface that are thought to have originated from torches. Raman spectrometry, on the other hand, enabled the detection of organic matter and could be used to separate carbon additions from black inorganic pigments.
Other Inclusions
Inclusions in paints and other dated surfaces (e.g., mineral accretions) are rare, but can include plant fibres, pollen, spores, insects, and microorganisms. Generally, inclusions will originate from a heterogeneous range of processes and sources that are unrelated to the artwork, potentially making date interpretation complex. It is unusual to find undisputed evidence of deliberate additions such as those found at the Yam Camp site near Laura, Australia. Here, Watchman and Cole (1993) recovered a quantity of matted plant fibres mixed with the quartz-kaolinite paint. Typically inclusions are few and careful evaluation of context prior to sampling is essential to ensure no cross-contamination has occurred via contact with visitor’s hands and clothes, or animals ( Bednarik 2002 :12).
Pigment Binders
The dating of non–charcoal-derived binders, including animal fats, egg whites, blood and honey, is highly contentious. These materials often do not survive pretreatment, or indeed environmental conditions, and/or it can be difficult to chemically characterize and distinguish them from contaminants ( Bednarik 2014 :230; Gillespie 1997 ; Livingston et al. 2009 ). Of note is the oft-reported dating of a dark red pigment at Laurie Creek (Northern Territory, Australia)—identified as human blood—that returned a 14 C age of 20,320 +3100/−2300 bp ( Loy et al. 1990 ). Subsequent tests on the sandstone surface indicated that datable organic matter was also present in unpainted areas of the shelter ( Nelson 1993 ), while the small sample size and, in hindsight, processing of the protein using ultrafiltration techniques that are prone to humectant contamination (see Bronk Ramsey, Higham, Bowles, & Hedges 2004 ) could also have contributed to the old apparent age (see Gillespie 1997 ).
Quantitative analysis of these compounds (e.g., lipids, proteins, or carbohydrates) by gas or liquid chromatography mass spectrometry provides greater detail when dealing with complex mixtures of organic materials and has been used to confirm the presence and/or removal of degradation products prior to dating (e.g., Livingston et al. 2009 ; Mori et al. 2006 ). Vázquez et al. (2008) demonstrated the potential of such characterization. They removed two black (manganese and iron oxide) samples from camelid figures at Alero Hornillos 2, Argentina. By isolating lipids using chloroform-methanol and subsequent analysis by gas chromatography mass spectrometry, they were able to identify saturated fatty acids characteristic of degraded animal fats, most probably from a ruminant animal source. While no 14 C dates were obtained, such works hold promise for future avenues of 14 C dating fraction-specific materials.
Surprisingly, beeswax figures are the most commonly dated art media in Australia (~48% of radiocarbon dates obtained on rock art), despite its limited geographic distribution to the northern and western regions of the country ( Langley & Taçon 2010 ). Typically, ‘beeswax’ is a variable mixture of plant resins, gums, and wax. The dating of this material has gained popularity because it is easy to sample and has few interpretative limitations because the age of the wax will approximate the age of creation by bees, it is produced seasonally, and use and collection are concurrent since it becomes brittle and unusable over time. Theoretically, this material is also poorly soluble and is resistant to microbial, bacterial, and fungal growth. Tests on the same sample (BW-4 from the site of Gunbilngmurrung, western Arnhem Land, Australia), but utilizing different preparation methodologies (HCl/NaOH/HCl [ Nelson, Chaloupka, Chippindale, Alderson, & Southon 1995 ] vs. acidified (KMnO 4 ) permanganate [ Watchman & Jones 2002 ]) gave ages 400 years apart (4040±90 bp and 4460±80 bp , respectively). This discrepancy remains unsolved but may be related to differences in sample preparation or to the survival characteristics of the beeswax ( Bednarik 2002 :11). The antiquity of these dates is also anomalous in that most beeswax sampled from northern Australia date to less than 2,000 years ago ( Taçon et al. 2010 :2; Watchman & Jones 2002 :146).
A combination of superimposition studies with the dating of beeswax figures has resulted in a re-evaluation of early European contact events. Taçon et al. (2010) took a total of 10 beeswax samples from the Djulirri art site in the Wellington Range, Arnhem Land, Australia, including two samples from a human figure covered by a yellow/orange emu painting; two beeswax pellets over a painting of a European tall ship; one piece of beeswax from a figure with hands on hips and wearing a hat; two pieces from a snake that overlays a large yellow painting of a prau (assumed to be of a southeast Asian sailing vessel); one piece from a female human-like figure over a white painting of a prau ; a sample from a line that was above both the beeswax snake and over the white prau ; and a sample from an unidentifiable design under the tall ship. The results enabled Taçon et al. (2010) to determine that the painting of the yellow prau was made prior to ad 1664 and was therefore not only the oldest dated contact rock art depiction from Australia, but also some of the earliest evidence for Southeast Asian visits to northern Australia. Similarly, the dates for the beeswax human figure wearing a hat with hands on hips and the painting of the tall ship indicated that a close encounter between local Aboriginal people and Europeans probably occurred in the ad 1700s, much earlier than ad 1818 as presupposed, opening up the possibility of Dutch rather than British contact at this time.
Indirect Stratigraphic Dating
Indirect stratigraphic dating refers to the dating of rock art using materials or objects from above or below the artwork (e.g., laminates such as oxalate skins and calcite [CaCO 3 ] crusts or flowstone and insect nests). Such dating, although not directly related to the painting event, is often used to bracket the age of artworks and is, therefore, often characterized by broad age estimates that are further complicated by the carbon source of the dated materials. It does, however, provide information when there are few other dating options available.
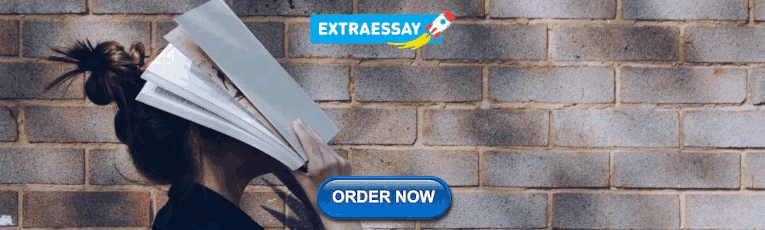
Mineral Accretions
When dating flowstone, it is important to recognize the possibility of the incorporation of “dead-carbon” derived from ancient carbonate rocks, plus any “rejuvenation” that may have occurred: a process by which new carbon can be added to the cave surface through the dissolution and re-precipitation of calcite associated with changes in wet/dry conditions, possibly resulting in younger ages or a mixture of ages ( Plagnes et al. 2003 ). In an attempt to account for such an offset in a nested diamond engraving at Billasurgam Cave in southern India, Taçon et al. (2013) dated what appeared to be a recently precipitated dry shawl of calcite in a nearby section of the cave. The resultant 900 ± 30 bp age indicated the magnitude of offset due to ancient carbon input. This correction was applied to dates on flowstone that bracketed the engraving and resulted in an age that was consistent with similar engravings of Mesolithic age nearby.
Oxalate minerals (whewellite and weddellite) that can form as crusts over artwork or can contaminate carbon samples, as already discussed, can also provide dating opportunities. Oxalates are isolated in the laboratory by treatment in warm, acidified permanganate, which turns the oxalate into CO 2 but leaves carbon from other sources such as amino acids, peptides, and carbonates ( Hedges et al. 1998 ; method reported in Gillespie 1997 ). Therefore, this extraction methodology is more selective than plasma-oxidation or traditional combustion methodologies. Watchman (1987) reported the first dates on oxalate crusts from rockart sites in Australia. The methodology was subsequently improved by isolating individual microscopic layers in stratigraphic sequence to provide additional dating control and limit cross-contamination ( Ruiz et al. 2012 ; Watchman 1993 ; Watchman & Campbell 1996 ).
Most researchers assume that metabolic processes in algae and microorganisms form oxalate minerals, but this remains speculative. Consequently, the age of carbon in these accretions may be near contemporaneous with initial formation or could take place over many years and theoretically could include both rejuvenation and re-deposition of carbon ( Bonneau et al. 2011 ; Watchman 1993 :468; Watchmen & Campbell 196:411). Regardless, calcium oxalate dating can be a valuable tool for confirming chronological limits for pictographs that cannot be otherwise dated directly (e.g., the iron oxide painted “eye-idols” at Abrigo de los Oculados, Spain [ Ruiz et al. 2012 ] or engraved artworks such as at Yiwarlarlay in the Northern Territory, Australia [ Watchman, David, McNiven, & Flood 2000 ]).
Insect Nests
Mud-dauber wasp and termite nests have also been used to provide age-delimiting radiocarbon ages for artwork ( Bednarik 2014 ; Brady, Thorn, McNiven, & Evans 2010 ). Both types of insect gather mud that contains pollen and other organics from nearby environments, and their nests are particularly common in tropical or subtropical locations. In some instances, silica or oxalate minerals that can also be dated may mineralize the nests over time. Often, however, in these instances the interval separating the age of the dating event and the mineralization of the nest may be so great as to be of limited use. This was the case for an artwork dated by Bednarik (2014 :230) at Princess Charlotte Bay, north Queensland, Australia. Here, a silicified mud wasp nest underlay the white kaolinite painted lines of artwork documenting early pearling activities towards the end of the nineteenth century. The resultant 14 C age for organics removed from the nest could only support an age for the artwork sometime in the past 19,000 years!
Date Evaluation: Bayesian Methodologies
The use of Bayesian statistical methodologies for the evaluation of 14 C dates and context is the latest revolution in 14 C dating ( Bayliss 2009 ). The use of Bayesian modelling allows the statistical scatter on the radiocarbon dates to be taken into account, enables the dating to become integrated with archaeological observations, and provides a useful model that can be tested rather than relying on often biased visual inspection. When correctly applied, Bayesian modelling of radiocarbon dates has demonstrably reduced the timescales under discussion (e.g., Bayliss, Bronk Ramsey, van der Plich, & Whittle 2007 ). However, the development of Bayesian models is not easy and requires considerable effort to be expended on simulation and model-building, thus requiring a programme of gradual and progressive dating to infill and test various aspects of the model. Moreover, the radiocarbon dates that constitute these models are tightly linked to pre-existing archaeological opinions (i.e., “prior beliefs”), so, more than ever before, the onus is on the archaeologist to ensure that those beliefs are valid and to recognize these models for what they are—models that require further testing ( Bayliss 2009 :127). Comprehensive guidelines for archaeologists selecting samples for Bayesian evaluation are given in Bayliss (2015) .
The progressive dating methodology and rigorous sample selection protocols recommended by Bayliss ( 2009 , 2015 ) do not mould well with the small sample sizes and complex and often ambiguous superimpositions encountered in rock art. Consequently, most rock art chronologies are still largely based on graphic interpretation of individual radiocarbon-calibrated age ranges. The potential value of Bayesian chronologies has been highlighted by work at the Billasurgam Cave complex, southern India. Here, Taçon et al. (2013) dated five pieces of flowstone associated with an engraving consisting of three interlinked concentric diamond patterns (Figure 3A ). Samples C1, C2, and C3 overlay the engraving, C2 was thought to be younger than C1, and C3 older. C4 was taken from above these, whereas C5 was taken from a surface that was considered to underlie the painting (though about 1 metre away) ( Taçon et al. 2013 :1791). To refine the age of the engraving, the authors then integrated the relative age sequence they had identified into a Bayesian model using the program OxCal ( Bronk Ramsey 2001 ). In the model, sample C5 was designated as predating the engraving and C4 as postdating it. The other three ages were incorporated within a single phase that Taçon et al. thought correlated most closely to the period when the engraving was created. This sequence produced a probable age for the engraving of between 5400 and 5000 cal bp , a significant refinement when compared to visual inspection of the calibrated age ranges of the unmodelled dates (Figure 3B ). 1 However, they noted that C2 produced a very low individual agreement index (A; an index that gives a measure of how well the date fits within the model. The threshold for a good agreement is 60%), suggesting that the date was too young, most likely because of rejuvenation and/or contamination.
Calibrated radiocarbon ages for dated samples from Billasurgam Cave.
Bayesian age model of the results, incorporating the relative sequence information as discussed in the text. Grey = original age distribution; Black = age distribution based on model constraints. TPQ is the acronym for terminus post quem
Radiocarbon dating rock art is difficult; it pushes every parameter of the technique, requiring attention to detail by the archaeologist and the collaborating 14 C scientists. Over the past few decades, a range of processing techniques for rock art dates have been developed, but there is still no consensus on how to handle the diverse range of materials incorporated into these art works, with alternative approaches favoured by different laboratories and researchers. While most studies of rock art recognize the need for replication of dates or comparison of sample preparation methodologies, the science is still hampered by sample size and sample chemistry. Advances in chemical characterization, especially the use of portable technologies and improved analytical detection, combined with the dating of sample-specific fractions, nevertheless promises to open up new avenues of 14 C enquiry.
The relationship of a radiocarbon date to the artistic event of interest is also often difficult to interpret because many dates are of materials that either pre-or postdate the activity in question. While this problem is not specific to rock art dating, the microscopic vertical scale of superimpositions often increases the difficulties of interpretation. Ultimately, researchers must come to terms with the idea that radiocarbon dating does not provide an absolute solution to the dating question; rather, it is a tool from which we can develop chronological models in association with other information gathered from the rock art site.
Acknowledgements
I wish to thank the many collaborators who have given me the opportunity to be involved in a diverse and interesting array of thought-provoking projects, in particular the Jawoyn Association and Margaret Katherine, the senior traditional owner of Nawarla Gabarnmang. Adelphine Bonneau kindly gave permission for the reproduction of Figure 2 . I would also like to thank the editors of the Oxford Handbook of the Archaeology and Anthropology of Rock Art for the opportunity to put forward my opinions and discuss the many issues that should be considered before radiocarbon dating images. Last, but not least, I would also like to give special mention to David Petchey who was my mentor in all things and inspired my interest in archaeology and solving problems.
Alon, D. , Mintz, G. , Cohen, I. , Weiner, S. , & Boaretto, E. ( 2002 ). The use of Raman spectroscopy to monitor the removal of humic substances from charcoal: Quality control for 14C dating of charcoal. Radiocarbon , 44 , 1–11.
Google Scholar
Aubert, M. ( 2012 ). A review of rock art dating in the Kimberley, Western Australia. Journal of Archaeological Science , 39 , 573–577.
Bayliss, A. ( 2009 ). Rolling out revolution: Using radiocarbon dating in archaeology. Radiocarbon , 51 (1), 123–147.
Bayliss, A. ( 2015 ). Quality in Bayesian chronological models in archaeology. World Archaeology , 47 (4), 677–700. doi:10.1080/0043(8243).(2015).1067640 10.1080/0043(8243).(2015).1067640
Bayliss, A. , Bronk Ramsey, C. , van der Plicht, J. , & Whittle, A. ( 2007 ). Bradshaw and Bayes: Towards a timetable for the Neolithic. Cambridge Archaeological Journal , 17 (1), 1–28.
Bednarik, R. G. ( 2002 ). The dating of rock art: A critique. Journal of Archaeological Science , 29 (11), 1213–1233.
Bednarik, R. G. ( 2014 ). Mud-wasp nests and rock art. Rock Art Research , 31 (2), 225–231.
Beck, L. , Genty, D. , Lahlil, S. , Lebon, M. , Tereygeol, F. , Vignaud, C. , … Paillet. P. ( 2013 ). Non-destructive portable analytical techniques for carbon in situ screening before sampling for dating prehistoric rock paintings. Radiocarbon , 55 (2–3), 436–444.
Bird, M. I. , Charville-Mort, P. D. J. , Ascough, P. L. , Wood, R. , Higham, T. , & Apperley, D. ( 2010 ). Assessment of oxygen plasma ashing as a pre-treatment for radiocarbon dating. Quaternary Geochronology , 5 , 435–442.
Bonneau, A. , Brock, F. , Higham, T. , Pearce, D. G. , & Pollard, A. M. ( 2011 ). An improved pretreatment protocol for radiocarbon dating black pigments in San rock art. Radiocarbon , 53 (3), 419–428.
Brady, L. M. , Thorn, A. , McNiven, I. J. , & Evans, T. A. ( 2010 ). Rock art conservation and termite management in Torres Strait, NE Australia. Rock Art Research , 27 , 19–34.
Bronk Ramsey, C. ( 2001 ). Development of the radiocarbon calibration program OxCal. Radiocarbon , 43 (2A), 355–363.
Bronk Ramsey, C. , Higham, T. , Bowles, A. , & Hedges, R. ( 2004 ). Improvements to the pretreatment of bone at Oxford. Radiocarbon , 46 (1), 155–163.
Chaffee, S. D. , Hyman, M. , & Rowe, M. W. ( 1994 ). Vandalism of rock art for enhanced photography. Studies in Conservation , 39 (3), 161–168.
Clottes, J. , & Geneste, J.-M. ( 2012 ). Twelve years of research in Chauvet Cave: Methodology and main results. In D. McDonald & P. Veth (Eds.), A companion to rock art . (pp. 583–604). Chichester, UK: John Wiley & Sons. doi:10.1002/9781118253892.ch33 10.1002/9781118253892.ch33
Google Preview
Combier, J. , & Jouve, G. ( 2012 ). Chauvet Cave’s art is not Aurignacian: A new examination of the archaeological evidence and dating procedures. Quartär , 59 , 131–152.
David, B. , Barker, B. , Delannoy, J.-J. , Geneste, J.-M. , Petchey, F. , & Lamb, L. ( 2014 ). A Pleistocene charcoal drawing or painting from northern Australia. INORA , 69 , 18–22.
David, B. , Barker, B. , Petchey, F. , Delannoy, J.-J. , Geneste, J.-M. , Rowe, C. , … Whear, R. ( 2013 ). A 28,000 year old excavated painted rock from Nawarla Gabarnmang, northern Australia. Journal of Archaeological Science , 40 , 2493–2501.
David, B. , Geneste, J.-M. , Whear, R. L. , Delannoy, J.-J. , Katherine, M. , Gunn, R. G. , … Matthews, J. ( 2011 ). Nawarla Gabarnmang, a 45,180±910 cal BP site in Jawoyn country, southwest Arnhem Land plateau. Australian Archaeology , 73 , 73–77.
Douka, K. , Hedges, R. E. M. , & Higham, T. F. G. ( 2010 ). Improved AMS 14 C dating of shell carbonates using high-precision X-ray diffraction (XRD) and a novel density separation protocol (CarDS). Radiocarbon , 52 (2), 735–751.
Gillespie, R. ( 1997 ). On human blood, rock art and calcium oxalate: Further studies on organic carbon content and radiocarbon age of materials relating to Australian rock art. Antiquity, 71(272), 430–437.
Gowlett, J. A. J. , & Hedges, R. E. M. ( 1986 ). Lessons of context and contamination in dating the Upper Palaeolithic. In Gowlett & Hedges (Ed.), Archaeological results from accelerator dating (pp. 63–71). Monograph 11. Oxford: Oxford University Committee for Archaeology.
Gunn, R. G. , David, B. , Delannoy, J.-J. , & Katherine, M. ( 2017 , in press). The past 500 years at Nawarla Gabarnmang, central-western Arnhem Land. In B. David , P. Taçon , J.-J. Delannoy , & J.-M. Geneste (Eds.), The archaeology of rock art in western Arnhem Land, Australia . Canberra, AUS: ANU Press.
Hedges, R. E. M. , Bronk Ramsey, C. , van Klinen, G. J. , Pettitt, P. B. , Nielsen-March, C. , Etchegoyen, A. , Fernandex Biello, J. O. , Boschin M. T. , Llamazares, A. M. ( 1998 ). Methodological issues in the 14 C dating of rock paintings. Radiocarbon , 40 (1), 35–44.
Hedges, R. E. M. , Housley, R. A. , Law, I. A. , Perry, C. , & Gowlett, J. A. J. ( 1987 ). Radiocarbon dates from the Oxford AMS system: Archaeometry datelist 6. Archaeometry , 29 (2), 289–306.
Langley, M. C. , & Taçon, P. S. C. ( 2010 ). The age of Australian rock art: A review. Australian Archaeology , 71 , 70–73.
Livingston, A. , Robinson, E. , & Armitage, R. A. ( 2009 ). Characterizing the binders in rock paintings by THM-GC-MS: La Casa de Las Golondrinas, Guatemala, a cautionary tail for radiocarbon dating. International Journal of Mass Spectrometry , 284 , 142–151.
López-Montalvo, E. , Villaverde, V. , Roldán, C. , Murcia, S. , & Badal, E. ( 2014 ). An approximation to the study of black pigments in Cova Remigia (Castellón, Spain). Technical and cultural assessments of the use of carbon-based black pigments in Spanish Levantine Rock Art. Journal of Archaeological Science , 52 , 535–545.
Loy, T. , Jones, R. , Nelson, D. , Meehan, B. , Vogel, J. , Southon, J. , & Cosgrove, R. ( 1990 ). Accelerator radiocarbon dating of human blood proteins in pigments from late Pleistocene art sites in Australia. Antiquity , 64 , 110–116.
McDonald, J. , Steelman, K. L. , Veth, P. , Mackey, J. , Loewen, J. , Thurber, C. R. , & Guilderson, T. P. ( 2014 ). Results from the first intensive dating program for pigment art in the Australian arid zone: Insights into recent social complexity. Journal of Archaeological Science , 46 , 195–204.
Mori, F. , Ponti, R. , Messina, A. , Flieger, M. , Havlicek, V. , & Sinibaldi, M. ( 2006 ). Chemical characterization and AMS radiocarbon dating of the binder of a prehistoric rock pictograph at Tadrart Acacus, southern west Libya. Journal of Cultural Heritage , 7 , 344–349.
Nelson, D. E. ( 1993 ). Second thoughts on a rock-art date. Antiquity , 67 , 893–895.
Nelson, D. E. , Chaloupka, G. , Chippindale, C. , Alderson, M. S. , & Southon, J. R. ( 1995 ). Radiocarbon dates for beeswax figures in the prehistoric rock art of northern Australia. Archaeometry , 37 , 151–156.
Pettitt, P. , & Bahn, P. ( 2003 ). Current problems in dating Palaeolithic cave art: Candamo and Chauvet. Antiquity , 77 , 134–141.
Pettitt, P. , & Bahn, P. ( 2015 ). An alternative chronology for the art of Chauvet cave. Antiquity , 89 , 542–553. doi:10.15184/aqy.(2015).21 10.15184/aqy.(2015).21
Pettitt, P. , & Pike, A. ( 2007 ). Dating European Palaeolithic cave art: Progress, prospects, problems. Journal of Archaeological Method and Theory , 14 (1), 27–47.
Plagnes, V. , Causse, C. , Fontugne, M. , Valladas, H. , Chazine, J.-M. , & Fage, L.-H. ( 2003 ). Cross dating (Th/U- 14 C) of calcite covering prehistoric paintings in Borneo. Quaternary Research , 60 , 172–179.
Quiles, A. , Valladas, H. , Bocherens, H. , Delqué-Koliˇc, E. , Kaltnecker, E. , van der Plicht, J. , … Geneste, J-M. ( 2016 ). A high-precision chronological model for the decorated Upper Paleolithic cave of Chauvet-Pont d’Arc, Ardèche, France. Proceedings of the National Academy of Sciences 113(17), 4670–4675. doi:10.1073/pnas.1523158113 10.1073/pnas.1523158113
Ridges, M. , Davidson, I. , & Tucker, D. ( 2000 ). The organic environment of paintings on rock. In G. K. Ward & C. Tuniz (Eds.), Advances in dating Australian rock-markings (pp. 61–70). Occasional AURA Publication 10. Melbourne: Australian Rock Art Research Association.
Rowe, M. W. ( 2009 ). Radiocarbon dating of ancient rock paintings. Analytical Chemistry , 81 , 1728–1735.
Ruiz, J. F. , Hernanz, A. , Armitage, R. A. , Rowe, M. W. , Viñas, R. , Gavira-Vallejo, J. M. , & Rubio, A. ( 2012 ). Calcium oxalate AMS 14 C dating and chronology of post-Palaeolithic rock paintings in the Iberian Peninsula. Two dates from Abrigo de los Oculados (Henarejos, Cuenca, Spain). Journal of Archaeological Science , 39 (8), 2655–2667.
Russ, J. , Hyman, M. , Shafer, H. J. , & Rowe, M. W. ( 1990 ). Radiocarbon dating of prehistoric rock paintings by selective oxidation of organic carbon. Nature , 348 , 710–711.
Russ, J. , Hyman, M. , Shafer, H. J. , & Rowe, M. W. ( 1991 ). 14 C dating of ancient rock art: A new application of plasma chemistry. Plasma Chemistry and Plasma Processing , 11 (4), 515–527.
Sand, C. , Valladas, H. , Cachier, H. , Tisnérat-Laborde, N. , Arnold, M. , Bolé, J. , & Ouetcho, A. ( 2006 ). Oceanic rock art: First direct dating of prehistoric stencils and paintings from New Caledonia (Southern Melanesia ). Antiquity , 80 , 523–529. doi:10.1017/S0003598X0009400X 10.1017/S0003598X0009400X
Schiffer, M. B. ( 1987 ). Formation processes of the archaeological record (pp. xxii + 428). Albuquerque: University of New Mexico Press.
Steelman, K. L. , Carrera Ramírez, F. , Fábregas Valcarce, R. , Guilderson, T. , & Rowe, M. W. ( 2005 ). Direct radiocarbon dating of megalithic paints from north-west Iberia. Antiquity , 79 , 379–389.
Steelman, K. L. , & Rowe, M. W. ( 2012 ). Radiocarbon dating of rock paintings: Incorporating pictographs into the archaeological record. In J. McDonald & P. Veth (Eds.), A companion to rock art (pp. 565–582). Chichester, UK: John Wiley & Sons. doi:10.1002/978111825(3892).ch32 10.1002/978111825(3892).ch32
Taçon, P. S. C. , Boivin, N. , Petraglia, M. , Blinkhorn, J. , Chivas, A. , Roberts, G. , … Zhao, J. -X. ( 2013 ). Mid-Holocene age obtained for nested diamond pattern petroglyph in the Billasurgam Cave complex, Kurnool District, southern India. Journal of Archaeological Science , 40 , 1787–1796.
Taçon, P. S. C. , May, S. K. , Fallon, S. J. , Travers, M. , Wesley, D. , & Lamilami, R. ( 2010 ). A minimum age for early depictions of southeast Asian praus in the rock art of Arnhem Land, Northern Territory. Australian Archaeology , 71 , 1–10.
Taylor, R. E. , & Bar-Yosef, O. ( 2014 ). Radiocarbon dating: An archaeological perspective (2nd ed.). Walnut Creek, CA: Left Coast Press.
Valladas, H. ( 2003 ). Direct radiocarbon dating of prehistoric cave paintings by accelerator mass spectrometry. Measurement Science and Technology , 14 , 1487–1492.
Valladas, H. , & Clottes, J. ( 2003 ). Style, Chauvet and radiocarbon. Antiquity , 77 , 142–145.
Van der Merwe, N. J. , Sealy, J. , & Yates, R. ( 1987 ). First accelerator carbon-14 date for pigment from a rock painting. South African Journal of Science , 83 (1), 56–57.
Vázquez, C. , Maier, M. S. , Parera, S. D. , Yacobaccio, H. , & Solá, P. ( 2008 ). Combining TXRF, FT-IR and GC-MS information for identification of inorganic and organic components in black pigments of rock art from Alero Hornillos 2 (Jujuy, Argentina ). Analytical and Bioanalytical Chemistry , 391 , 1381–1387.
Watchman, A. ( 1999 ). A universal standard for reporting the ages of petroglyphs and rock paintings. In M. Strecker & P. Bahn (Eds.), Dating and the earliest known rock art (pp. 1–3). Oxford: Oxbow.
Watchman, A. L. ( 1987 ). Preliminary determinations of the age and composition on mineral salts on rock art surfaces in the Kakadu National Park. In W. R. Ambrose & J. M. U. Mummery (Eds.), Archaeometry: Further Australasian studies (pp. 36–42). Canberra, AUS: Australian National University.
Watchman, A. L. ( 1993 ). Evidence of a 25,000 year old pictograph in northern Australia. Geoarchaeology , 8 , 465–473.
Watchman, A. L. , & Campbell, J. ( 1996 ). Microstratigraphic analyses of laminated oxalate crusts in northern Australia. In M. Realini & I. Toniolo (Eds.), Oxalate films in the conservation of works of art. Proceedings of the 11th International Symposium. Milan (pp. 409–422). Bologna: Editeam.
Watchman, A. L. , & Cole, S. ( 1993 ). Accelerator radiocarbon dating of plant-fibre binders in rock paintings from north-eastern Australia. Antiquity , 67 , 355–358.
Watchman, A. L. , David, B. , McNiven, I. J. , & Flood, J. M. ( 2000 ). Micro-archaeology of engraved and painted rock surface crusts at Yiwarlarlay (the Lightning Brothers site), Northern Territory, Australia. Journal of Archaeological Science , 27 , 315–315.
Watchman, A. L. , & Jones, R. ( 2002 ). An independent confirmation of the 4ka antiquity of a beeswax figure in Western Arnhem Land, northern Australia. Archaeometry , 44 (1), 145–153.
Whitley, D. S. ( 2012 ). In suspect terrain: Dating rock engravings. In D. McDonald & P. Veth (Eds.), A companion to rock art (pp. 605–624). Chichester, UK: John Wiley & Sons. doi:10.1002/978111825(3892) 10.1002/978111825(3892)
This value is further qualified by an associated age offset because of the presence of a possible dead carbon fraction (see text). Taçon et al. (2013 :1792) suggest a conservative terminus ante quem of circa 4100 cal bp .
- About Oxford Academic
- Publish journals with us
- University press partners
- What we publish
- New features
- Open access
- Institutional account management
- Rights and permissions
- Get help with access
- Accessibility
- Advertising
- Media enquiries
- Oxford University Press
- Oxford Languages
- University of Oxford
Oxford University Press is a department of the University of Oxford. It furthers the University's objective of excellence in research, scholarship, and education by publishing worldwide
- Copyright © 2024 Oxford University Press
- Cookie settings
- Cookie policy
- Privacy policy
- Legal notice
This Feature Is Available To Subscribers Only
Sign In or Create an Account
This PDF is available to Subscribers Only
For full access to this pdf, sign in to an existing account, or purchase an annual subscription.
Radiocarbon Dating
Cite this chapter.
- R. E. Taylor 6
Part of the book series: Advances in Archaeological and Museum Science ((AAMS,volume 2))
1004 Accesses
39 Citations
This chapter reviews the basic elements of the radiocarbon ( 14 C) dating method and summarizes three generations of 14 C studies in archaeology. It considers in greater detail several major advances in 14 C research including the extension of the calibration of the 14 C time scale into the late Pleistocene, further detailed characterization of Holocene short-term perturbations (de Vries effects), and the development of accelerator mass spectrometry.
This is a preview of subscription content, log in via an institution to check access.
Access this chapter
- Available as PDF
- Read on any device
- Instant download
- Own it forever
- Available as EPUB and PDF
- Compact, lightweight edition
- Dispatched in 3 to 5 business days
- Free shipping worldwide - see info
- Durable hardcover edition
Tax calculation will be finalised at checkout
Purchases are for personal use only
Institutional subscriptions
Unable to display preview. Download preview PDF.
Similar content being viewed by others
Radiocarbon Dating: Development of a Nobel Method
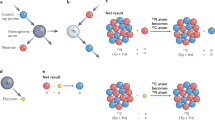
Radiocarbon dating
Radiocarbon Dating in African Archaeology
Aardsma, G.E. 1991 Radiocarbon and the Genesis Flood . El Cajon (California), Institute for Creation Research.
Google Scholar
Ajie, H.O., Kaplan, I.R., Hauschka, P.V., Kirner, D., Slota, P.J. Jr., and Taylor, R.E. 1992 Radiocarbon dating of bone osteocalcin: isolating and characterizing a non-collagen protein. Radiocarbon 34: 296–305.
Alvarez, W. 1981 The early days of accelerator mass spectrometry. In Henning, W., Kutschera, W., Smither, R.K. and Yntema, J.L. eds., Symposium on Accelerator Mass Spectrometry . Argonne, Argonne National Laboratory: 1–15.
Anbar, M. 1978 The limitations of mass spectrometric radiocarbon dating using CN ions. In Gove, H.E. ed., Proceedings of the First Conference on Radiocarbon Dating with Accelerators , Gove, H.E. ed., Rochester, University of Rochester: 152–155.
Arnold, J.R. and Libby, W.F. 1949 Age determinations by radiocarbon content. Checks with samples of known age. Science 110: 678–680.
Article Google Scholar
Arnold, J.R. and W.F. Libby 1950 Radiocarbon dates (September 1,1950) . Chicago, University of Chicago, Institute for Nuclear Studies.
Arnold, J.R. and WE Libby 1951 Radiocarbon dates. Science 113: 111–120.
Atkinson, R.J.C. 1975 British prehistory and the radiocarbon revolution. Antiquity 49: 173–177.
Bard, E., Arnold, M., Fairbanks, R.G., and Hamelin, B. 1993a 230 Th- 234 U and 14 C ages obtained by mass spectrometry on corals. Radiocarbon 35: 191–199.
Bard, E., Stuiver, M. and Shackleton N.J. 1993b How accurate are our chronologies of the past? In Eddy, A. and Oeschger, H. eds., Global Changes in the Perspective of the Past . New York, John Wiley and Sons.
Barker, H. and Mackey, J. 1961 British Museum natural radiocarbon measurements III. Radiocarbon 3: 39–45.
Becker, B. 1993 An 11,000-year German oak and pine dendrochronology for radiocarbon calibration. Radiocarbon 35: 210–213.
Bennett, C.L., Beukens, R.P., Clover, M.R., Gove, H.E., Lieben, R.B., Litherland, A.E., Purser, K.H. and Sondheim W.E. 1977 Radiocarbon dating using accelerators: Negative ions provide the key. Science 198: 508–509.
Bennett, C. L., Beukens, R.P., Clover, M.R., Elmore, D., Gove, H.E., Kilius, L., Litherland, A.E. and Purser, K.H. 1978 Radiocarbon dating with electrostatic accelerators: Dating of milligram samples. Science 201: 345–347.
Berger, R. 1992 Libby’s UCLA Radiocarbon Laboratory: Contributions to archaeology. In Taylor, E., Long, A., Kra, R.S., eds., Radiocarbon After Four Decades An Interdisciplinary Perspective . New York, Springer-Verlag: 421–434.
Chapter Google Scholar
Bertsche, K.J., Friedman, P.G., Morris, D.E., Muller, R.A. and Welch, J.J. 1987 Status of the Berkeley small cyclotron AMS project. Nuclear Instruments and Methods B29: 105–109.
Bertsche, K.J. 1989 A small low energy cyclotron for radioisotope measurements. Ph.D. dissertation, University of California, Berkeley.
Book Google Scholar
Broecker, W.S. and Kulp J.L. 1956 The radiocarbon method of age determination. American Antiquity 22: 1–11.
Bronk, C.R. and Hedges, R.E.M. 1987 A gas ion source for radiocarbon dating. Nuclear Instruments and Methods B29: 45–49.
Brooks, S., Brooks, R.H., Kennedy, G.E., Austin, J., Firby, J.R., Payen, L.A., Prior, C. A., Slota, P.J., Jr., and Taylor, R. E. The Haverty Human Skeletons: Morphologial, Depositional and Geochronological Characteristics. Journal of California and Great Basin Anthropology 12: 60-83.
Brown, R.H. 1983 The interpretation of carbon-14 age data. In Coffin, H.G. with Brown, R.H., eds., Origin by design . Washington, D.C., Review and Herald Publishing Association: 309–329.
Brown, R.H. 1986 14 C depth profiles as indicators of trends in climate and 14 C/ 12 C ratios. Radiocarbon 28: 350–357.
Bruns, M., Levin, I., Munnish, K.O., Hubberten, H.W. and Fillipakis, S. 1980 Regional sources of volcanic carbon dioxide and their influence on 14 C content of present-day plant material. Radiocarbon 22: 532–536.
Burky, R.R. 1996 Radiocarbon dating archaeologically significant bone using gamma-carboxyglutamic acid (Gla) and alpha-carboxyglycine (aminomalonate). Ph.D. disseration, University of Califronia, Riverside.
Chen, M., Li, D., Xu, S., Chen, G., Shen, L., Lu, X., Zhang, W., Zhang, Y., Zhong, Z., Zhang, Y. 1995 Breakthrough of the mini-cyclotron mass spectrometer for 14 C analysis. Radiocarbon 37: 675–682.
Clark, G. 1970 Aspects of prehistory . Berkeley, University of California Press.
Cremo, M.A. and Thompson, R.L. 1993 Forbidden archeology, The hidden history of the human race . San Diego (California), Bhaktivedanta Institute.
Damon, P.E., Donahue, D.J., Gord, B.H., Hatheway, A.L. Jull, A.J.T., Linick, T.W., Sercelo, P.J., Toolin, L.J., Bronk, C.R., Hall, E.T., Hedges, R.E.M., Housley, R., Law, I.A., Perry, C., Bonani, G., Trumbore, S., Wolfli, W., Ambers, J.C., Bowman, S.G.E., Leese, M.N. and Tite, M.S. 1989 Radiocarbon dating the shroud of Turin. Nature 337: 611–615.
Damon, P. E. and J. I. Jirikowic 1992 Solar forcing of global climate change? In Taylor, R.E., Long, A., and Kra, R. eds., Radiocarbon after four decades: An Interdisciplinary Perspective . New York, Springer-Verlag: 177–179.
Daniel, G. 1967 The origins and growth of archaeology . New York, Crowell.
Daniel, G. 1972 Editorial. Antiquity 46: 265.
Dillehay T.D. and Meltzer, D.J., eds. 1991 The First Americans: Search and Research . Baca Raton: CRC Press.
Eddy, J. 1976 The Maunder minimum. Science 192: 1189–1202.
Edwards, R.L. 1993 A large drop in atmospheric 14 C/ 12 C and reduced melting in the Younger Dryas, documented with 230 Th ages of corals. Science 260: 962–968.
Erlenkeuser, H. 1979 A thermal diffusion plan for radiocarbon isotope enrichment from natural samples. In Berger, R. and Suess, H.E., eds., Radiocarbon dating . Berkeley, University of California Press: 216–237.
Gittins, G.O. 1984 Radiocarbon chronometry and archaeological thought . Ph.D. disseration, University of California, Los Angeles.
Gove, H.E. 1992 The history of AMS, its advantages over decay counting: Applications and Prospects. In Taylor, R.E., Long, A., Kra, R.S., eds., Radiocarbon After Four Decades An Interdisciplinary Perspective . New York, Springer-Verlag: 214–229.
Grootes, P.M., Mook, W.G., Vogel, J.C., de Vries, A.E., Haring, A. and Kismaker, J. 1975 Enrichment of radiocarbon for dating samples up to 75,000 years. Zeitschrift fixer Naturforschung 30A: 1–14.
Harbottle, G., Sayre, E.V. and Stoenner, R.W. 1979 Carbon 14 dating of small samples by proportional counting. Science 206: 683–685.
Hedges, R.E.M. and Gowlett, J.A.J. 1986 Radiocarbon dating by accelerator mass spectrometry. Scientific American 254: 100–107.
Johnson, F., 1965 The impact of radiocarbon ating upon archaeology. In Chatters, R.M. and Olson, E.A., eds., Proceedings of the Sixth International Conference radiocarbon and Tritium Dating . Springfield (Virginia): Clearinghouse for Federal Science and Technical Information: 762-780.
Johnson, F. and MacNeish, R.S. 1972 Chronology of the Tehuacan Valley. In Byers, D.S., ed., Prehistory of the Techuacan Valley , vol. 4, Austin, University of Texas Press.
Johnson, F. and Willis, E.H. 1970 Reconciliation of radiocarbon and sideral years in Meso-American chronology. In Olsson, I.U., ed., Radiocarbon variations and absolute chronology . Stockholm, Almqvist & Wiksell: 93–104.
Kaufman, T.S. 1980 Early prehistory of the Clear Lake area, Lake County, California. Ph.D. dissertation, University of California, Los Angeles.
Kirner, D., Taylor, R.E. and Southon, J.R. 1995 Reduction in backgrounds of microsamples for AMS 14 C dating. Radiocarbon 37: 697–704.
Kirner, D., Southon, J.R., Hare, P.E. and Taylor, R.E. 1996 Acclerator mass spectrometry radiocarbon measurement of submilligram samples. In Orna, M. V., ed., Archaeological Chemistry Organic, Inorganic, and Biochemical Analysis . Washington, D.C., American Chemical Society: 434–442.
Kirner, D., Burky, R., Taylor, R.E., and Southon, J.R. 1997 Radiocarbon dating organic residues at the microgram level. Nuclear Instruments and Methods in Physics Research , in press.
Klein, J., Lerman, J.C., Damon, P.E. and Ralph, E.K. 1982 Calibration of radiocarbon dates: Tables based on the consensus data of the owrkshop on calibrating the radiocarbon time scale. Radiocarbon 24: 103–150.
Kromer, B. and Becker, B. 1993 German Oak and pine 14 C calibration, 7200-9439 BC. Radiocarbon 35: 125–135.
Levin, I., Bosinger, R., Bonani, G., Francey, R.J., Kromer, B., Munnich, K.O., Suter, M., Trivett, N.B.A. and Wolfli, W. 1992 Radiocarbon in atmopsheric carbon dioxide and methane: Gloval Distribution and Trends. In Taylor, R.E., Long, A., and Kra, R., eds., Radiocarbon after four decades: An Interdisciplinary Perspective . New York, Springer-Verlag: 503–518.
Libby, W.F. 1963 Accuracy of radiocarbon dates. Antiquity 37: 7–12.
Linick, T.W., Damon, P.E., Donahue, D.J. and Jull, A.J.T. 1989 Accelerator mass spectrometry: The new revolution in radiocarbon dating. Quaternary International 1: 1–6.
Long, A., Benz, B.F., Donahue, D.J., Jull, A.J.T., and Toolin, L.J. 1989 First direct AMS dates on early Maize from Tehuacán, Mexico. Radiocarbon 31: 1035–1040.
Long, A and Miller, A.B. 1981 Arizona radiocarbon dates X. Radiocarbon 23: 191–217.
Lynch, T.F. 1990 Glacial-age man in South America: A critical review American Antiquity 55: 12–36.
Mast, T.S. and Muller, R.A. 1980 Radioisotope detection and dating with accelerators. Nuclear Science Applications 1: 7–32.
Mazaud, A., Laj, C., Bard, E., Arnold, M. and Tric, E. 1992 A geomagnetic calibration of the radiocarbon time-scale. In Bard, E. and Broecker, W.S., eds., The Last Deglaciation: Absolute and Radiocarbon Chronologies . Berlin: Springer-Verlag: 163–169.
Meltzer, D.J., Adovasio, J.M. and Dillehay, T.D. 1994 On a Pleistocene human occupation at Pedra Furada, Brazil. Antiquity 68: 695–714.
Muller, R.A. 1977 Radioisotope dating with a cyclotron. Science 196: 489–494.
Muller, R.A. 1979 Radioisotope dating with accelerators. Physics Today 32(2): 23–30.
Muller, R.A., Stephenson, E.J. and Mast, T.S. 1978 Radioisotope dating with an accelerator: A blind measurement. Science 201: 347–348.
Nelson, D.E., Korteling, R.G. and Scott, W.R. 1977 Carbon-14: Direct detection at natural concentrations. Science 198: 507–508.
Neustupny, E. 1970 The accuracy of radiocarbon dating. In Olsson, I. U., ed., Radiocarbon variations and absolute chronology . Stockholm, Almqvist & Wiksell: 22–34.
Nobel Foundation 1964 Nobel Lectures, Chemistry 1942–1962 . Amsterdam, Elsevier.
Oeschger, H., Houtermans, J., Loosli, H., and Wahlen, M. 1970 The constancy of cosmic radidation from isotope studies in meteorities and on the earth. In Olsson, I.U. ed., Radiocarbon variations and absolute chronology . Stockholm, Almqvist & Wiksell: 471–496.
Otlet, R.L., Huxtable, G., Evans, G.V., Humphreys, D.G., Short, T.D. and Conchie, S.J. 1983 Development and operation of the Harwell small counter facility for the measurement of 14 C in very small samples. Radiocarbon 25: 565–575.
Payen, L.A. 1982 The pre-Clovis of North America: Temporal and artifactual evidence. Ph.D. dissertation, University of California, Riverside.
Ralph, E.K., Michael, H.N. and Han, M.C. 1973 Radiocarbon dates and reality. MASCA Newsletter 9: 1–20.
Rowe, J.H. 1965 An interpretation of radiocarbon measurements on archaeological samples from Peru. In Chatters, R.M. and Olson, E.A. eds., Proceedings of the Sixth International Conference Radiocarbon and Tritium . Springfield (Virignia), Clearinghouse for Federal Scientific and Technical Information: 187-198.
Renfrew, C. 1973 Before civilization: the radiocarbon revolution and prehistoric Europe . New York: Alfred A. Knopf.
Saupe, F., Strappa, O., Coppens, R., Guillet, B., and Jaegy, R. 1980 A possible source of error in 14 C dates: Volcanic emanations (examples from the Monte Amiata District, Provinces of Gorsseto and Sienna, Italy) Radiocarbon 22: 525–531.
Sayre, E.V., Harbottle, G., Stoenner, R.W., Otlet, R.L. and Evans, G.V. 1981 The use of the small gas proportional counters for the carbon 14 measurement of very small samples. IAEA Proceedings on Methods of Low Level Counting and Spectrometry Vienna, International Atomic Energy Agency: 393.
Schmidt, F.H., Balsley, D.R. and Leach, D.D. 1987 Early expectations of AMS: Greater ages and tiny fraction. One failure?-One success. In Gove, H.E.A.E. Litherland, A.E. and Elmore, D. eds., Accelerator Mass Spectrometry . Amsterdam, North-Holland Physics Publishing: 97–99.
Scott, E.M., Long, A., and Kra, R., eds. 1990 Proceedings of the International Workshop on Intercomparison of Radiocarbon Laboratories. Radiocarbon 32: 253–397.
Southon, J.R., Deino, A.L, Orsi, G., Terrasi, R., and Campajola, L. 1995 Calibration of the radiocarbon time scale at 37Ka BP. Abstract of Papers , 209th American Chemical Society National Meeting, Part 2, p. 10.
Stafford, T.W. Jr, Jull, A.J.T., Brendel, K., Duhamel, R.C. and Donahue, D. 1987 Study of bone radiocarbon dating accuracy at the University of Arizona NSF accelerator facility for radioisotope analysis. Radiocarbon 29: 24–44.
Stafford, T.W., Hare, P.E., Currie, L., Jull, A.J.T. and Donahue, D.J. 1990 Accuracy of North American human skeleton ages. Quaternary Research 34: 111–120.
Stafford, T.W., Hare, P.E., Currie, L., Jull, A.J.T. and Donahue, D.J. 1991 Accelerator radiocarbon dating at the molecular level. Journal of Archaeological Science 18: 35–72.
Stuiver, M. and Braziunas, T.G. 1993 Modeling atmospheric 14 C influences and 14 C ages of marine samples to 10,000 BC. Radiocarbon 35: 137–189.
Stuiver, M. and Reimer, P.J. 1993 Extended 14 C data base and revised CALIB 3.0 14 C age calibration program. Radiocarbon 35: 215–230.
Stuiver, M., Heusser, C.H. and Yang, I.C. 1978 North American galcial history extended to 75,000 years ago. Science 200: 16–21.
Stuiver, M., Long, A. and Kra, R.S., eds. Calibration 1993 Radiocarbon 35: 1–244.
Stuiver, M. and Polach, H.A. 1977 Discussion: Repoorting of 14 C data. Radiocarbon 19: 355–363.
Stuiver, M. and Suess, H.E. 1966 On the relationship between radiocarbon dates and true sample ages. Radiocarbon 8: 534–540.
Suess, H.E. 1970 Bristlecone-pine calibration of radiocarbon time 5200 B.C. to present. In Olsson, I.U., ed., Radiocarbon variations and absolute chronology . Stockholm, Almqvist & Wiksell: 303–312.
Taylor, R.E. 1987 Radiocarbon dating An archaeological perspective . San Diego, Academic Press.
Taylor, R.E. 1990 Radiocarbon dating. Encyclopedia of Physical Science and Technology , 1990 Yearbook. New York, Academic Press: 499–504.
Taylor, R.E. 1991 Radioisotope dating by accelerator mass spectrometry: archaeological and paleoan-thropological perspectives. In Göksu, H.Y., Oberhofer, M., Regulloi, D., eds., Scientific Dating Methods . Dordrecht (Netherlands), Kluwer Academic Publishers: 37–54.
Taylor, R.E. 1992 Radiocarbon dating of bone: To collagen and beyond. In Taylor, R.E., Long, A., and Kra, eds., Radiocarbon after four decades: An Interdisciplinary Perspective . New York, Springer-Verlag: 375–402.
Taylor, R.E. 1994 Radiocarbon dating of bone using accelerator mass spectrometry: Current discussions and future directions. In Bonnichsen, R. and Steele, D.G. eds., Method and Theory for Investigating the Peopling of the Americas . Corvallis, Center for the Study of the First Americans, Oregon State University: 27-44.
Taylor, R.E., Long, A., and Kra, R. eds. 1992 Radiocarbon After Four Decades: An Interdisciplinary Perspective . New York, Springer-Verlag.
Taylor, R.E., Donahue, D.J., Zabel, T.H., Damon, P.E. and Jull, A.T.J. 1984 Radiocarbon dating by particle accelerators: An archaeological perspective. In Lambert, J.B. ed., Archaeological Chemistry III . Washington, D.C., American Chemical Society: 333–356.
Taylor, R.E., Payen, L.A. and Slota, P.J., Jr. 1992 The age of the Calaveras Skull: Dating the “Piltdown Man” of the New World. American Antiquity 57: 269–275.
Taylor, R.E., Haynes, C. V., Jr. and Stuiver, M. 1996 Clovis and Folsom age estimates: stratigraphic context and radiocarbon calibration. Antiquity 70: 515–525.
Taylor, R.E., Stuiver, M., and Reimer, P.J. 1996 Development and extension of the calibration of the radiocarbon time scale: Archaeological applications. Quaternary Science Reviews (Quaternary Geochronology) 15: 655–668.
Thomas, D.H. 1978 The awful truth about statistics in archaeology. American Antiquity 43: 231–244.
Vries, H. de 1958 Variations in concentration of radiocarbon with time and location on earth. Proceedings, Nederlandsche Akademie van Wetenschappen , Series B61: 1.
Vogel, J.S., Southon, J.R., Nelson, D.E. and Brown, T.A. 1984 Performance of catalytically condensed carbon for use in accelerator mass spectrometry. Nuclear Instruments and Methods in Physics Research B52: 301–305.
Vogel, J.S., Nelson, D.E. and Southon, J.R. 1987 14 C background levels in an accelerator mass spectrometry system. Radiocarbon 29: 323–333.
Welch, J.J., Bertsche, K.J., Firedman, P.G., Morris, D.E., Muller, R.A. and Tans, P.P. 1984 A 40 keV cyclotron for radioisotope dating Nuclear Instruments and Methods in Physics Research B5: 230–232.
Willis, E.H., Tauber, H. and Munnich, K.O. 1960 Variations in the atmospheric radiocarbon concentration over the past 1300 years. Radiocarbon 2: 1–4.
Wilson, H.W. 1979 Possibility of measurement of 14 C by mass spectrometer techniques. In Berger, R. and H. E. Suess, eds., Radiocarbon Dating . Berkeley, University of California Press: 238–245.
Download references
Author information
Authors and affiliations.
Radiocarbon Laboratory, Department of Anthropology, Institute of Geophysics and Planetary Physics, University of California, Riverside, California, 92521, USA
R. E. Taylor
You can also search for this author in PubMed Google Scholar
Editor information
Editors and affiliations.
Radiocarbon Laboratory, Department of Anthropology, Institute of Geophysics and Planetary Physics, University of California, Riverside, California, 95251, USA
Research Laboratory for Archaeology, Oxford University, OX1 3QJ, UK
Martin J. Aitken
Rights and permissions
Reprints and permissions
Copyright information
© 1997 Springer Science+Business Media New York
About this chapter
Taylor, R.E. (1997). Radiocarbon Dating. In: Taylor, R.E., Aitken, M.J. (eds) Chronometric Dating in Archaeology. Advances in Archaeological and Museum Science, vol 2. Springer, Boston, MA. https://doi.org/10.1007/978-1-4757-9694-0_3
Download citation
DOI : https://doi.org/10.1007/978-1-4757-9694-0_3
Publisher Name : Springer, Boston, MA
Print ISBN : 978-1-4757-9696-4
Online ISBN : 978-1-4757-9694-0
eBook Packages : Springer Book Archive
Share this chapter
Anyone you share the following link with will be able to read this content:
Sorry, a shareable link is not currently available for this article.
Provided by the Springer Nature SharedIt content-sharing initiative
- Publish with us
Policies and ethics
- Find a journal
- Track your research

An official website of the United States government
The .gov means it’s official. Federal government websites often end in .gov or .mil. Before sharing sensitive information, make sure you’re on a federal government site.
The site is secure. The https:// ensures that you are connecting to the official website and that any information you provide is encrypted and transmitted securely.
- Publications
- Account settings
Preview improvements coming to the PMC website in October 2024. Learn More or Try it out now .
- Advanced Search
- Journal List

Radiocarbon dating the Greek Protogeometric and Geometric periods: The evidence of Sindos
Stefanos gimatzidis.
1 Austrian Academy of Sciences, Austrian Archaeological Institute, Vienna, Austria
Bernhard Weninger
2 Institute of Prehistory, University Cologne, Köln, Germany
Associated Data
All relevant data are within the manuscript.
Mediterranean Early Iron Age chronology was mainly constructed by means of Greek Protogeometric and Geometric ceramic wares, which are widely used for chronological correlations with the Aegean. However, Greek Early Iron Age chronology that is exclusively based on historical evidence in the eastern Mediterranean as well as in the contexts of Greek colonisation in Sicily has not yet been tested by extended series of radiocarbon dates from well-dated stratified contexts in the Aegean. Due to the high chronological resolution that is only achievable by (metric-scale) stratigraphic 14 C-age-depth modelling, the analysis of 21 14 C-AMS dates on stratified animal bones from Sindos (northern Greece) shows results that immediately challenge the conventional Greek chronology. Based on pottery-style comparisons with other sites, the new dates for Sindos not only indicate a generally higher Aegean Early Iron Age chronology, but also imply the need for a revised understanding of the Greek periodisation system that will foreseeably have a major impact on our understanding of Greek and Mediterranean history.
Introduction
In contrast to the Near East, where ancient cities often have the form of tell mounds, even the best excavated settlements in central and southern Greece have rarely yielded the long and continuous vertical stratigraphies that in other regions so readily support typo-chronological studies of their material inventories, at high temporal resolution. In Greece, the continuous settlement stratigraphies with well-dated successive layers, that cover many hundreds of years, are a privilege of the ‘northern periphery’ of the Aegean. In this region, dense networks of tell-based settlements developed continuously during the Bronze and Early Iron Age ( Fig 1 ).

Based on free vector and raster map data from @naturalearthdata.com. Constructed with Globalmapper ® Version 11 using Lambert Conformal Conic Projection.
In spite of the steadily increasing number of excavations, in central and southern Greece the Early Iron Age is still better known through cemeteries than settlements. This unfortunate deficiency in Greek archaeology has partly to do with the fact that the architecture of Early Iron Age settlements is indeed often badly preserved, e.g. at Athens and Corinth. Another relevant explanation is that the Early Iron Age settlements have attracted far less archaeological interest, if only because they seldom yield such impressive finds as their contemporaneous necropolises. In consequence, not only is there a general lack of interest in applying radiocarbon dating to the Aegean Early Iron Age, but even the well-excavated settlement sites–suffering as they do from short stratigraphic sequences–are lacking in the main archaeological requirement for high-resolution 14 C-age modelling, which is the availability of an extended sequence of pottery data that would support either quantitative age-depth or pottery-based seriation of the 14 C-measurements. In this respect, and what is sometimes overlooked, even highest-precision single 14 C-measurements on short-lived samples (e.g. from well-defined burial or other contexts) cannot by themselves provide the envisioned high-resolution archaeological chronology. This would require advanced processing of multi-dimensional statistical data (i.e. interdisciplinary research), and ultimately the combination with quantitative pottery data, at best on some kind of metric-scale. Such metric-scaling (alias ‘ quantitative sequencing’ ) is possible, trivially, by direct counting of tree-ring growth-sequences, but also for 14 C-ages that are sequenced according to the time-factor derived from Correspondence Analysis (see below). A particular use of metric 14 C-sequencing is by probabilistic (Monte Carlo) analysis of stratified 14 C-ages from tell stratigraphies, as applied in the present paper [ 1 ] [ 2 ] [ 3 ]. In contrast, when based on ordinal-scaled (‘older-younger’) archaeological 14 C-sequencing, as is the case for the majority of published Bayesian applications in archaeological research, the achieved chronology immediately runs danger of age-distortion due to the uncorrected convolution properties of the 14 C-age calibration curve. As can also be derived from theoretical considerations, the more precise the archaeological 14 C-ages are measured, the stronger their associated artificial age-distortions are likely to become, with actual values strongly depending on the contents of the archaeological sequence in relation to the shape of the calibration curve. A first confirmation for this forecasting is shown in Fig 2 , where the application of Bayesian sequencing to a series of highly-precise 14 C-ages from Assiros [ 4 ] that was measured on bone (N = 27) and combined with the 14 C-ages on two tree-ring sequences has apparently produced an entirely artificial gap of at least 50 yrs length between phases 3 and 4. Presumably, this specific distortion is due to the inhomogeneity of the dataset, hence–ultimately–to the choice of an invalid Bayesian prior. Whatever its cause, the existence of this distortion due to inappropriate age-modelling invalidates the proposed radiometric updating of the Protogeometric vase from Assiros, which is of the very same magnitude. At the other methodological extreme, however, the solution cannot be the (assumed) model-neutral use of calibrated single 14 C-ages, since in this case all that is achieved is repetitive stacking of the one-and-the-same calendric-scale interval, over and over again, with no achieved enhancement of the dating precision. In mathematical terms, the problem at stake is the non-commutativity of the underlying algebra of 14 C-calibration, which complicates the analysis, in both cases, by effectively eliminating the otherwise so advantageous possibility of data-averaging with error-reduction [ 5 ]. The complications of (ordinal-scale) Bayesian age-modelling can be avoided, at large, by application of (metric-scale) stratigraphic age-depth modelling. This will be demonstrated below for the Sindos data.

The modelled probability distributions for Phase 4 and 3 indicate a major hiatus in the stratigraphic sequence, which in reality does not exist.
In Early Iron Age research, if we now switch to the historical perspective, there are further reasons for the general reluctance towards using dating methods such as Radiocarbon or Correspondence Analysis. The main reason is the continuing confidence placed in the absolute dates that were gained by means of textual (historiographic) evidence. In historical terms, this confidence was the outcome of an early enthusiasm and a strong belief in the historicity of the written evidence, and which was eventually (and mainly unintentionally) transformed to become the supposedly scientific base of the now modern Greek chronology. This altogether quite disciplinary tradition in Aegean archaeology contrasts strongly with the highly intense debates on chronology in the Near East. During the last two to three decades, Near Eastern archaeologists, in particular in Israel, have undertaken a wide range of radiocarbon studies, which in many cases have led to the partial or even complete rejection of the authority of the textual evidence. This approach challenges the direct correlation of archaeological and historical data [ 6 ] [ 7 ] [ 8 ] [ 9 ], an approach yet to be customarily pursued in Aegean archaeology.
The historical chronology of the Greek Early Iron Age
The scientific foundation of the conventional Greek Early Iron Age chronology has been, and still is, a much discussed and disputed issue. The beginning of the Protogeometric period, and the tripartite chronological definition of this, as well as the following Geometric period, is based to some large part on highly disputable historical evidence from the eastern Mediterranean. The method to assign absolute age-values to the Greek relative chronological system, which was based on pottery assemblages mostly from tomb contexts, was initially simple: single pottery finds from sites such as Tel Abu Hawam, Megiddo, Samaria, Hama [ 10 ], and more recently Tel Rehov [ 11 ] [ 12 ], were ascribed an absolute date that was obtained by the historical dating of the destruction layers of the respective site they were found in.
While there is practically no evidence that would support the chronological definition of the tripartite Protogeometric period, the transition from the Late Protogeometric to the Early Geometric is unceasingly based on a couple of sherds from a layer at Tel Abu Hawam, which has been variably dated. Similarly, a mere handful of sherds from Megiddo and Samaria has been used to describe, and date, the transitions from Early to Middle Geometric I (850 BC), Middle Geometric I to II (800 BC), Middle Geometric II to Late Geometric (760/750 BC). The same method, already partly used in construction of the Greek periodization by German scholars having worked in the necropolis of Kerameikos at Athens [ 13 ], was firmly established in Classical archaeology, following the comprehensive studies of Greek Protogeometric and Geometric pottery by Vincent R.d’A Desborough and Nicolas Coldstream [ 14 ] [ 15 ].
Aegean archaeology barely took into consideration the continuing discussions in Near Eastern archaeology about the historical dating of at least some of these sites that brought some large ambiguity about the validity of the evidence used in definition of the Greek Early Iron Age chronology. Although some of the eastern Mediterranean sites that yielded Greek pottery have recently also provided long series of precise 14 C-determinations, such as Megiddo and Tel Rehov [ 16 ] [ 17 ] [ 18 ], these sites can still not be taken as safe anchor points for the Greek absolute chronology. This is typically due to the unclear contextual provenance of the randomly discovered Greek pottery finds [ 19 ].
Higher value is often placed on the chronological evidence that derives from the foundation dates of the Greek colonies in Sicily, which are still today widely used as anchor points for the absolute chronology of the Late Geometric and early Archaic period. This was due to an unwavering trust in the credibility of Thucydides, the most respected ancient Greek historiographer, who furnished both the foundation dates of some of the earliest Greek colonies, as well as the potential for correlation of the very same dates with the assumed earliest ‘colonial’ pottery found in the Greek establishments on Sicily [ 20 ] [ 21 ]. Single archaeological finds such as a scarab of the Egyptian king Bocchoris in a tomb at Pithekoussai may support the validity of this method, in certain cases [ 22 ] [ 23 ]. However, unexpectedly ‘earlier’ Greek pottery was soon found to have been used at some of those colonial sites, which was explained as the outcome of ‘precolonial’ contacts. Furthermore, there is still no consensus concerning the actual historicity and the sources of information available to Thucydides, who presents historical data concerning events that took place at least three centuries before his time [ 24 ]. Despite these open questions, the Sicilian evidence is usually considered as safer for the definition of the Late Geometric and Early Archaic periods than the available data from the eastern Mediterranean for the earlier Protogeometric and Geometric periods.
The construction of the relative and absolute chronology of the Greek Early Iron Age, which was the major outcome of Desborough’s and Coldstream’s studies, was not only based on the sequencing of Greek pottery by means of contextual approaches. Also integrated were the results of art historical methods according to the Zeitgeist. A good example, in this respect, is the main argument for the definition of the first part of the Late Geometric that predates the Greek colonisation. This was defined by Coldstream according to the artistic output of a specific Painter, the so-called Dipylon Master: Late Geometric Ia was thought to cover his early work and was therefore given a time span of ten years from 760 to 750 BC. The closely following Late Geometric Ib (750–735 BC) was associated with his late work, with the longer time span of 15 years given, if only to allow for his work together with other painters. While the earlier phases of the Greek Geometric period are based on ambiguous (but: at least) empirical ceramic evidence from the eastern Mediterranean, the beginning of Late Geometric and practically the entire Protogeometric periods are defined according to archaeological intuition [ 14 ] [ 15 ].
Radiocarbon inconsistencies in the Greek Early Iron Age
Unfortunately, the radiocarbon evidence from the Aegean is neither consistent, nor itself sufficiently precise, to support the existence of proposed discrepancies between the historical and the 14 C-based chronological systems. Although there are a number of 14 C-dates from Submycenaean and Early to Middle Protogeometric contexts available at other sites apart from Assiros, e.g. at Kastanas [ 25 ] and Torone [ 26 ] in the northern Aegean, as well as at few other sites in the central and southern Aegean [ 27 ], if we take a closer look at the achieved dating precision (in statistical terms) or otherwise at the sampling and documentation methods (in archaeological terms), the quality of these 14 C-series is insufficient to support further discussion.
The radiocarbon dates of Sindos
The tell mound of Sindos is located some 20 km west of Thessaloniki in northern Greece (40°42’01°N and 22°47’35°E) ( Fig 3 ). This is one of the largest and most complex formed tell settlements in central Macedonia. A settlement system comprising almost exclusively tells developed in the river valleys and coastal plains of this region during the Bronze and Early Iron Age. These settlement sites have the form of conical mounds, known as toumbas in regional archaeology, during the Bronze Age. From the beginning of the Early Iron Age onwards new and larger settlement mounds emerged. They have the form of extended and elevated plateaus that are known as tables. These later tells emerged either independently as new settlement sites or adjacent to older toumbas as means to expand habitation surface.

The two conical structures to the left are modern (photo taken in 2001).
Excavations conducted in the 1990’s and early 2000’s at several parts of the ancient settlement of Sindos revealed long stratigraphic sequences–particularly of the Early Iron Age. These excavations highlighted all major episodes in this tell’s formation: adjacent to an ancient core settlement that initially—during the Bronze Age—probably had the form of a conical tell (toumba), in the following Early Iron Age there emerged not one but two large tells, both with flat surfaces (tables) and both significantly expanding the settlement’s habitation area. Such complex processes in the formation of Macedonian tells, that typically involve extensive levelling and other major earthworks, mainly the construction of extensive terraces made of clay, are not only documented at Sindos, but are analytically presented in the final publication of the excavations, and hence available in all detail necessary for further research [ 28 ].
Complexities in tell formation and depositional history
The formation of a tell mound is a complex depositional process that may hide pitfalls in age-depth modelling due to deep stratigraphic reworking. Sindos presents several characteristic cases of such complex processes in tell formation. For example, the deposition of Late Bronze Age layers immediately below the superficial Late Archaic layers at the top of the toumba may seem puzzling, if someone considers that long sequences of Early Iron Age levels were attested at almost all other parts of the settlement. Apparently debris and layers of this period were missing from the top of the toumba due to continuous levelling works. Furthermore, according to conventional pottery dates, following phase 4 at Sindos we might expect the existence of a major hiatus that dates from the end of the 8 th century BC. Further, since the layers of the immediately overlying phase 3 were deposited after the mid-6 th century BC, acceptance of the conventional dates would imply that a 150 yrs long hiatus marked the settlement history of Sindos during the Archaic period. Below, we will return to our many suspicions that there is something wrong with the conventional historical chronology, when applied to Sindos, and this is evident without application of radiocarbon dating. For the moment, let us begin by analysing the strengths and weaknesses of radiocarbon dating, quite specifically, in the context of a complex tell stratigraphy.
One main concern for application of radiocarbon dating at tell sites pertains to the possibility of stratigraphic reworking of the dated samples. In this respect what immediately comes to mind is the often encountered (upward) reworking of older materials to younger levels. Indeed, since this upward reworking runs parallel to the growth direction of the tell, this would appear the most ‘natural’ direction for the large majority of disturbances. Further, such upwards directional-modelling of tell deposits also corresponds to what may be called the main ‘axiom’ of stratigraphic analysis, that is: the deposits are best dated by their ‘youngest’ inventary. Equally possibly, however, is the stratigraphic re-working in the opposite direction i.e. from younger to older levels, and this would be the immediate (and equally ‘natural’) consequence of the often large-scale and systematic site-management activities of the tell-community. At the Neolithic site of Shir in Syria, for example, some ~45% of all (N = 40) 14 C-dated grain samples from settlement pits have ages 100–200 yrs younger than the 14 C-ages of (incorrectly assumed) ‘contemporaneous’ (i.e. same depth) settlement layers [ 29 ]. Actually, such an unexpected inversion of the more accustomed upwards direction of re-deposition may help to explain one of the major still-existing discrepancies between calibrated 14 C-ages and historical dates. Namely, given the accumulation rate of ~1–2 cm/yr for a typical tell-mound, in combination with the depth of ~1 m for a typical storage pit, such secondary re-deposition of dated materials in younger-> older direction would provide a ‘natural’ explanation at least in quantitative terms for the observed offset of ~100 yrs between the Egyptian historical chronology and the calibrated 14 C-ages from Tell el Daba [ 30 ]. In a nut-shell, resolving such issues is of importance, since enforcing a chronology at fault in one field of research has immediate consequences for other research fields.
Of course, we cannot exclude the occurrence of either sedimentational effects (upwards or downwards) also at Sindos, after having indeed identified a number of possible cases. Nevertheless, it is difficult to imagine that any such material re-deposition would explain all the discrepancies with the historical chronology that we observe at Sindos, and which would allow us to (artificially) maintain its validity vis-a-vis the emerging evidence of its faults (cf. below). Maintaining its validity has consequences, for example, it would imply (ad hoc ) that the (historically dated) pottery sherds and the ( 14 C-dated) animal bones–which show an excellent linear sequence (see below)–would have undergone some kind of systematic vertical separation, in the order of ~1 m, but for which there is no presently ascertained physical or cultural process. The point hereby is that, even if there did exist some unrecognised (say ~1 m deep) storage pits, and even if these pits were in-filled from above, this would not affect the original association of the (historically dated) pottery sherds with the ( 14 C-dated) animal bones. The same argument would apply, similarly, even for the alternative case that the pottery and bones do not derive from some unrecognised deep-storage pit but from some other of the many types of tell-management deposits. We may expect the original association of pottery and bones to remain intact, under the large majority of depositional conditions, unless we assume the existence of some material-discriminating physical or cultural process that would act differently for sherds and broken bones (but which is unlikely, as noted above, if only with the exception that dogs seldom eat sherds). Another point-at-stake is that–in clear contrast to the aforementioned case of Shir–the 14 C-data at Sindos do not show any unusually wide spread of 14 C-ages, that would be indicative for the postulated inter-level (or inter-phase) sample movement.
Nonetheless, an important issue in age-modelling is to critically contrast the primary (sedimentological) site-formation processes with the (cultural) definition of settlement-formation according to archaeological phases/periods. Inevitably, the sedimentological sequence of layers will seldom find a match in the temporal sequence of phases/periods. At Kastanas, for example, a site in close vicinity to Sindos, some buildings were destroyed only a few years or decades after erection, whereas others (e.g. level 12) have a life-span of more than a century before being levelled for purposes of rebuilding [ 31 ]. In contrast to the applied linear age-depth modelling, at Sindos the actual tell-formation is indeed (possibly) not well-represented as series of continuous, successive, and equilength sedimentological episodes.
Sampling methods and strategies
Despite all complexities in tell formation, archaeological exploration at such settlement sites is often accompanied with the welcome opportunity of working with successive destruction layers, that have clearly distinct ash and other burnt materials covering well preserved artefacts on surfaces of use. The house-floor assemblages that were found directly beneath thick layers of ashes and collapsed walls do not necessarily provide direct comparisons with the Pompeii-like systemic inventories that have both rapid and abrupt depositional qualities (Pompeii premise). They may nevertheless be taken as assemblages of artefacts, archaeobotanical and faunal material that were deposited and probably also used in the same period. At Sindos, such near-ideal stratigraphic conditions for radiocarbon sampling are given in a number of instances. An example is the fire-destruction of phase 7, from which six short-lived bone samples were sampled (cf. Table 1 ).
Although a reconstruction of the Early Iron Age settlement plan is not possible at Sindos, as at few other Aegean Geometric sites such as Zagora, Sindos offers the unique opportunity for studying the Aegean material culture by means of a 13 m deep stratigraphy, which shows more than 16 settlement phases, at least 13 of which are successive ( Fig 4 ). The large amount of pottery from its stratified contexts allows the comparative study of several Aegean pottery styles, in a region where pottery sequences of the Early Iron Age have until now been known almost exclusively by means of burial contexts. The finds from Sindos include non-local ceramic wares from many different Aegean micro-regions, such as Euboea, Attica and Corinth, and of course Macedonia. Regional correlations between the chronologies of the central and southern Aegean were already developed by Coldstream, as demonstrated in his most influential book on the Greek Geometric Pottery [ 15 ]. At that time any synchronization with the northern ‘periphery’ was still unthinkable. The Greek relative chronological system still suffers from all biases and ambiguities that resulted from its construction by means of burial contexts given that evidence from stratigraphies was practically missing. The fact that the inhabitants of this Aegean gateway to the Balkans made use of pottery from several parts of central and southern Greece makes Sindos a most appropriate place to achieve a major geographic expansion of the Aegean Early Iron Age relative chronology, and this now applies–due to its many Aegean contacts–to essentially the entire Mediterranean (see below).

An additional benefit of the long stratigraphy at Sindos is that it allows the newly developed Early Iron Age pottery chronology sequence to be dated by a series of N = 21 short-lived 14 C-samples, that were all measured to high-precision (σ < 25 BP) by the Mannheim 14 C-AMS laboratory (Lab-Code MAMS). The series covers essentially almost the entire cultural sequence of the Aegean Early Iron Age, beginning with Late Protogeometric, through Early Geometric I and II, Middle Geometric I and II, up to the Late Geometric Ia and Ib ( Table 1 ).
All 21 samples of animal bone for the radiocarbon analysis were taken from good and relatively well-dated contexts at the stratigraphic trench A.0 that was excavated from 2000 to 2002 at the south-eastern side of the high table. The successive layers sloping to the south on this trench’s profiles reflect long process of debris accumulation at that side of the Early Iron Age table ( Fig 4 ). While some of the earliest excavations at Sindos were conducted with the method of arbitrary layers, the most recent trenches including trench A.0 were excavated with the method of single context recording. Nevertheless, there is no archaeological procedure (known to us) to determine whether bones from allegedly ‘undisturbed’ contexts could be residual from earlier layers, or not. Such re-depositional processes may however be traced by means of pottery , especially in assemblages that have been statistically and typologically studied in such detail as those of Sindos. From these studies we know, for example, that the floors and contexts at trench A.0 of Sindos, where the animal bone samples were finally deposited, did not yield any noticeable residual ceramic or other artefacts.
The sampled bones of domestic animals (oxen/cows, pigs, sheep/goats) come from six successive phases of the settlement (phase 11 to 6) that date according to the conventional relative chronology from the Late Protogeometric (950–900 histBC) to the Late Geometric Ib (750–735 histBC). Note that samples were purposely also taken from phases 6, 7, and 8, despite the threatening expectation that their 14 C-ages would have readings on the Hallstatt plateau (~800–400 calBC) of the 14 C-age calibration curve.
The two latest samples were collected from the floor of a house of phase 6. Two of the six bones analysed from phase 7 were collected from the floor below the collapsed mudbrick wall and the roof of an earlier house. The other four bones of the same phase were found in contexts mixed with burnt material from the same house close to this wall. A thick layer of ash containing burnt clay probably from a house roof was covering the three bone samples together with other artefacts that were found on a floor of phase 8. Part of this destroyed settlement phase was levelled with debris that may have been brought from another part of the contemporary settlement. In any case no samples were collected from that context. The three bone samples from phase 9 were collected above the surface of a thin layer of yellow clay that used to cover the debris of an earlier phase and formed a new surface of use. All four bone samples of phase 10 were collected just above and within two successive thin layers of black earth that represent surfaces of use and relate to them. These were well-defined contexts that contained burnt material and large quantities of pottery and sea shells close to a wall that used to support a layer of yellow clay (terrace). The two sampled bones from phase 11 come from a similar context. This was a burnt layer with a lot of pottery and sea shells deposited on the surface of use, close to a massive and better preserved terrace wall. A more detailed description of the contexts of the bone samples can be taken from the section profiles and plans, which are available and analytically commented in the final publication of Sindos’ excavations [ 28 ].
To complete our brief review of the excavations, we note that the archaeozoological as well as archaeological material from the excavations at the settlement of Sindos is stored within the facilities of the Ephorate of the city of Thessaloniki. All necessary permits (ΥΠΟΠΑΙΘ/ΓΔΑΠΚ/ΔΣΑΝΜ/ΤΕΕ/Φ77/164609/3721) were obtained by the Greek Ministry of Culture for the described study, which complied with all relevant regulations.
Introduction of the Sindos 14 C-data
Based on minimal age-modelling (i.e. albeit model-neutral, but not distortion-free; cf. below), Fig 5 provides an overview of the contents of the 14 C-database ( Table 1 ). In joint context with the INTCAL13-calibration curve and the high-precision laboratory data used in INTCAL-13 construction, Fig 5 shows the 14 C-histogram and the summed calibrated 14 C-age probability distribution of the summed Sindos data (N = 21), both in context with their individual BarCode-ages (small vertical lines on the calendric time-scale). The applied numbering of samples and Sindos-phases is useful for first screening of potential outliers (cf. Fig 5 , lowest line), but this is more efficiently achieved by stratigraphic (metric) age-depth modelling (see below). The Barcode-ages are pragmatically defined as central values of the 95%-confidence intervals. For this kind of data-representation, aimed only on achieving a first summary of the overall data spread, it is important to note that the calendric-scale position of the barcodes is practically always strongly offset, in relation to the (unknown) calendric age. The horizontal age-distortion of single 14 C-ages can be quite strong, in all variables (i.e. not only those evident in this graph), and is typically in the order of– 100 to + 100 yrs, but often more. This distortion is often only attributed to the existence of multiple calibration curve readings. However, from a more fundamental mathematical perspective, there are many such effects (including e.g. the decadal-scale clustering of Barcode-values, as can be taken from Fig 5 ), and all caused by one and the same factor, that is the non-commutative probabilistic algebra that is underlying the statistical properties of the 14 C-age calibration curve. As goes for the present Sindos-series, the strongest age-distortion applies to the youngest date (ID2, MAMS-27019: 2552 ± 23 BP). This is easily recognisable, both from the extreme length of its error-bar as well as from the strong age-shift of the corresponding Barcode-line. Note that, in Fig 5 , in order not to clutter up the picture, we have decided to show the error bars only at 68% confidence.

As goes for the Hallstatt Plateau (~800–400 calBC), an essentially flat region of the calibration curve that archaeologists prefer to avoid, it has its cause in the chance compensation of the increase in the natural atmospheric production of 14 C and its decrease due to radioactive decay in parallel to its oceanic uptake. In consequence, when samples are taken from this time-window, by conventional wisdom all 14 C-ages for the Middle Geometric II (800–760 histBC), Late Geometric Ia (760–750 hist BC) and Late Geometric Ib (750–735 histBC) would be expected to give the same 14 C-age of 2480± 30 BP. As it turned out, none of the samples from Sindos have this 14 C-content ( Fig 5 ), with the exception of the obviously only quasi-distorted sample ID2, from the very youngest 14 C-dated phase 6 at Sindos. Knowing that phase 6 follows immediately after phase 7, from the intercept of the 14 C-age for ID2 with the INTCAL13 curve we can immediately provide a most precise (first) estimated reading of 780 ± 25 calBC for this phase. This is confirmed, later, by the explicit age-modelling (see below). Going back in time, all nine samples from phase 7 and phase 8 came from layers that were destroyed by fire, as evidenced by the large amounts of ashes, charcoal, and collapsed mud brick walls on the settlements floors. Even such apparently ‘safe’ contexts, however, do not entirely eliminate the possibility that some of the animals, whose bones were deposited in these two (or any of the other settlement phases), may actually have been consumed in an earlier phase. There are however reasons to think that such biases are less possible to have affected our sampling (see above).
Stratigraphic 14 C-based age-depth modelling at Sindos
For age-depth modelling at Sindos we have applied the method of Gaussian Monte Carlo Wiggle Matching (GMCWM or GaussWM) [ 2 ]. The achieved chronological results are shown in Fig 6 , with corresponding modelling uncertainties shown in Fig 7 . The numeric modelling values are provided in Table 2 . Before continuing with the archaeological discussion of these results, let us take a moment to evaluate the applied method itself. In historical perspective, GMWCM is an extended and today largely automated version of the wiggle-matching method that was first introduced in the year 1986 by Gordon Pearson in support of the (preliminary) dating of floating tree-ring chronologies based on matching a series of 14 C-ages to the calibration curve [ 32 ]. Also in 1986 essentially the same (Chi-squared) method was applied to stratified 14 C-ages from Tell Dipsis (Bulgaria), Niederwil (Switzerland) and Arslantepe (Turkey), as well as to historically seriated 14 C-data from the Egyptian 1 st Dynasty [ 33 ]. An important drawback of these earliest applications, however, was the difficulty in determining the statistical uncertainties of the dating results, but which can be overcome by including a Monte-Carlo simulation of the different error sources [ 34 ]. Hence, despite a steadily increasing number of extensions and modifications applied over the years (e.g. [ 1 ] [ 2 ] [ 3 ] [ 34 ]), the GMWCM-procedure is still today based on essentially the same method as it was, some 30 years ago. As illustrated (schematically) in Eq (1) , the approach taken is to minimize the statistical distance (on the 14 C-scale) between the discretely measured sequence of tree-ring (or archaeological) samples that have 14 C-ages D i ± σ(D) i [BP], but unknown calendric ages, and the continuous (e.g. splined) calibration curve that has 14 C-ages K(t) ± σ(K) i [BP]) at certain known-age calendric years t.

The samples are arranged in stratigraphic order, with stratigraphically highest (‘youngest’) sample (Hd-27019) at table top, and stratigraphically lowest (‘oldest’) sample (Hd-27034) at table bottom. The Monte Carlo results are provided with measured marginal uncertainties (noted at 95%-confidence), without numeric rounding. During run-time, a Gaussian-shaped random error of ± 10 [a] was added to the numeric age of each sample derived from the (itself randomised) depth-position. The intention hereby is to include not only (possible) phase-internal but also phase-overlapping sample re-location. Note that, due to the Monte Carlo procedures, the overall dating results are given, not for each sample ( per se ) but for the associated stratigraphic sample position. Further quadratic addition of 20 yrs error (95%-confidence) to each of these stratigraphic positions would appear advisable, to account for unrecognised error components, but which cannot be proven to exist.
Accordingly, there exists a statistically best-fitting year t, not only for 14 C-ages measured on tree-ring series (as in the application by Gordon Pearson), but similarly for many other kinds of seriated, sequenced, or otherwise stratified sample series. All that is needed is that the series has a temporal structure (call it an ‘age model’). Potential applications for the required age-modelling can be based on known (metric-scale) historical time-spans, as for the Egyptian historical chronology mentioned above, but also on sequences of ordinal-scale (older/younger) settlement phases. The method is also applicable to archaeological tell-stratigraphies, as in the present paper, in which case the age-modelling can be based on the measured (metric-scale) stratigraphic sample depth. The special advantage of applying the χ2-method to metric-scaled sample sequences is that the required numeric modelling values are immediately available (as measured depths) and forthwith only require rescaling (depth->age). Of course, in practical applications, the necessary depth->age rescaling requires a fair amount of statistical processing (cf. below). But, from a mathematical viewpoint, there are only minor differences between the different Chi-squared approaches. For example, whereas in dendro-studies the distances between the 14 C-dated (annual growth) samples have small sampling errors (0–2 yrs), in archaeological studies the distances between dated samples can be quite large (for settlement phases: 10–50 yrs). This makes the necessary modelling estimates, in archaeological studies, inherently much more error-prone than in the dendrochronological application. In consequence, although archaeological GMWCM-studies require no fundamental change in the mathematical algorithms, they do require further attention in terms of error analysis. Next to such technical aspects, the really important advantage of applying GMWCM to tell-stratigraphies (e.g. Sindos) is that the validity of the modelling assumptions can be checked, namely, by direct comparison of the model-ages achieved for each sample, with the results based on the respective (unmodelled) single calibrated 14 C-ages. This also applies vice-versa .
Nevertheless, although in concept simple, the advantages of metric age-modelling are not received for free. Rather more, the application of metric modelling to real archaeological data is immediately complicated by the need for more advanced (and higher quality-level) requirements already during archaeological sampling, and similarly during the statistical data processing, hereby in terms of data input/output procedures, age-modelling algorithms, application of randomization requirements, calculation of probabilities, and graphic output routines. In the course of the methodological extensions of the GMCWM-method described in [ 29 ] [ 34 ], today the technical procedures are largely automated.
The application of GMCWM to the Sindos 14 C-series is illustrated by a screenshot ( Fig 8 ) of the most recent GaussWM-dialog, which is integrated in CalPal-software (Version 2020.2). In terms of hardware, the results were obtained using workstation Celsius W530 ® with Xeon E3-1281v3 ® 3.7 GHz CPU. In terms of software, CalPal is written in programming language Fortran 95, with compilation by Intel ® Parallel Studio XE Fortran Compiler, in combination with Winteracter ® 13 and IMSL6.0 ® libraries. Data transfer in Excel © -format is achieved through the ODBC © -Interface (Open Database Connectivity). Such ODBC-compliancy greatly simplifies the analysis of archaeological 14 C-data, due to the possibility of user-convenient external data-editing.

Left : spreadsheet with input of data and results; program functions; tools for age-model construction; tools for statistical analysis. Right Upper : Graph showing on-screen (Monte Carlo run-time animation) the presently achieved best-fit position of the archaeological 14 C-series in context with the selected calibration curve. Right Middle : Histogram showing the dating probability and precision of the step-wise expanded 14 C-series. Right Lower : Statistical fit parameters are given as run-time series of the stepwise expanded sequence. Red = dating probability; Blue = dating precision; Green = simultaneously optimized precision and probability. Note: During run-time the GaussWM tables and graphs are continually refreshed (every ~3 secs). An explorative model analysis requires ca. 1–5 min run-time. Typical runs require 6–16 hours.
An important amendment of the GMCWM procedure, used in the Sindos-analysis, is the application of a refined data/curve fitting method, based on the non-central Chi-squared distribution instead of Chi-squared. This does not change the GMCWM results, but makes the analysis less sensitive to slightly asymmetric 14 C-data, as may be caused by chance, or small (decadel-scale) 14 C-reservoir deviations and interlaboratory offsets. The use of non-central Chi-squared probabilities require calculations of the incomplete Gamma function, which were conveniently performed (in Fortran 95) by a call to the IMSL ® -subroutine CSNDF. The advantage of using CSNDF, in comparison to GAMMQ, is that allows passing of a variable noncentrality parameter λ. In the Sindos analysis we applied λ = 5 (for λ = 0 CSNDF converges to a Chi-squared test). As shown schematically in Eq (2) ) the calculation of best-fitting probabilities based on CSNDF follows directly after the Chi-squared distance calculations.
Run time parameters
The final Sindos results are based on a GMCWM run with the following parameters and program settings. The model was run for a total of 1000*50*60 = 3*10 6 random iterations. These included, (1) a fixed number of 60 steps in the sequence expansion, (2) a number of 50 Monte Carlo iterations for phase-internal re-ordering, and (3) a total number of 1000 complete run repetitions. In effect, therefore, the modelling results shown in Table 2 (Column: Results ) are based on 1000 independent measurements., each of which was obtained as best-result of an extended search. During run-time, in parallel to the phase-internal randomized sample order ( Table 2 , Column: Random Depth Position ), the sample distances were also randomized, with additional (squared additive) Gaussian calendric-scale distance errors set to σ = 10 yrs ( Table 2 , Column: Random Age ). Finally, as a final error component, applied prior to each model-expansion, the calibration curve (INTCAL13) was re-splined, with the new curve in each case based on the original INTCAL13 raw data, but re-measured with Gaussian 14 C-scale errors set to σ = 10 BP. The run-time of the final Sindos age-modelling run was 16 hours.
In a nutshell, the best-fitting position of the Sindos 14 C-data series on the INCAL13 calibration curve (shown in Fig 6 ) was identified by systematic stepwise linear-expansion of the sample sequence. The applied linear-stratigraphic age-model was constructed according to the measured sample depth. The validity of this age-model, with modelling errors in der of range of 10–20 yrs (95% confidence), is confirmed due to the reproducibility of the chronological results when only the single 14 C-ages are used i.e our interpretations are altogether independent of the assumptions (whether critical or not) that are at the base of the age-depth modelling. The modelling results are nonetheless useful. They allow the derivation of a simple linear equation, as shown in Fig 7 , that conveniently supports the dating (with associated errors) for any requested tell-depth.
Although the main aim of GMCWM in stratigraphic studies is, naturally, to identify the best-fitting length and age-position of the 14 C-dated sample sequence on the 14 C-age calibration curve (here: INTCAL13), what is actually challenging–as mentioned above—is the derivation of (albeit) realistic dating errors. Of special interest, in this respect, is to derive the marginal probabilities that are assigned (here) to the different Sindos phases. In the present GMWCM–application to the Sindos data, as it turns out, the (calculated) marginal dating uncertainties are quite small (annual-scale) for the youngest phases (7 and 8), but increase strongly for the older phases ( Fig 7 ). Understandably, this is the immediate consequence of the shape of the calibration curve in the time-window under study (1100–700 calBC), which shows a rather wiggly section for ages 1100–800 calBC, followed by a major increase in slope, if only for some 20 yrs (~800–780 calBC). Lucky are those archaeologists, in terms of achievable supra-precision, whose 14 C-dated samples have readings into this time-window. Yet, this time-window–wide as it may appear ( Fig 5 )–is narrower than it looks (from a statistical perspective), as well as representing a most strongly wobbling target (from the view-point of tell-related sedimentation processes).
Note that, with this intention, we must foremost (quantitatively) allow for possible stratigraphic reworking of samples. In the present application, this is attempted both by controlled intra-phase Monte Carlo randomization of the stratigraphic sample position (for multiple-dated phases i.e. phases 7–10), as well as by Gaussian inter-phase spreading of samples, for all phases. Whereas the Monte Carlo randomization is achieved by application of a random-position algorithm only to samples from the multiple-dated phases, the Gaussian sample spreading is achieved by applying an additional (depth-controlled) Gaussian age-distribution, with chosen width of ± 10 yrs (68% confidence) to all sample depths. Even under such, at least, intentionally realistic Monte Carlo conditions, what we actually observe for the younger phases are calculated dating uncertainties smaller than 10 yrs. Given that 14 C-AMS interlaboratory offsets are presently estimated to have values of ± 10 BP, at best, we have accordingly enlarged the calculated errors to this value (on the calendric time scale: ± 10 yrs) for all phases. Nonetheless, Figs Figs6 6 and and7 7 show the original (uncorrected) uncertainty values, if only for purposes of critical interrogation. Put differently, we believe we have the reserve in dating caution that is necessary for targeting the window of supra-high dating precision, as noted above. However, what is equally if not more critical to demonstrate is the stratigraphic integrity of the 14 C-dated samples, and this in combination with the archaeological finds. To this aim, on the following, we provide a detailed archaeological description of the site. Our focus is on the pottery inventory, which is to be used for wider synchronisms, and which is presented in a phase-by-phase manner, from old to young.
Relative pottery-based chronology at Sindos
The relative chronology of the stratigraphic sequence at Sindos was achieved after some quite exhaustive statistical, typological and technological analysis of its large pottery assemblage that comprised 4897 rim-sherds and numerous other wall and other fragments from well-stratified contexts. The study of pottery technology took place independently from its typological analysis. By means of a x40 stereoscope pottery fabrics were macroscopically described and classified in 32 major ceramic ware groups and further subgroups (plain handmade wares not included). Macroscopic fabric descriptions and ware characterisations were supported by an extensive Neutron Activations Analysis project that was conducted in cooperation with Hans Mommsen (forthcoming). The typological study of the ceramic material of Sindos resulted after detailed contextual analysis and cross-comparison with other contexts in the Aegean and Mediterranean, where pottery shapes and fabrics of the same type as those at Sindos were also in use. Finally, statistical analysis based exclusively on rim sherd count of 4897 fragments from the best stratified settlement contexts [ 28 ] [ 35 ].
Well-dated, non-local pottery facilitated correlation with other regional chronological systems in the Aegean, which was further achieved through analytical studies and cross-checking of local ceramic types. The origin of the local and non-local (mainly Euboean and Attic) pottery types that were used at Sindos has been scientifically defined by means of Neutron Activation Analysis of a representative pottery sample (see above). The non-local pottery used in certain settlement phases comprised a considerable part of the total ceramic assemblage consumed at the site. In particular, 5% of the total pottery in the settlement phase 8 was not local; in phases 7 and 6 the rate was 7% and 9% respectively. The majority of the non-local pottery at Sindos came from Euboea: 92% of the imported wares from phase 7 (169 individuals) came from that island [ 28 ]. The use of such large quantities of non-local pottery can barely support its perception as commodity of particular symbolic or other value that may have remained in use for some considerably longer period of time than it did in its place of origin/production. The assumption of overall short-use is further supported by the fact that broken vessels of non-local origin were never repaired–as otherwise usually happened with similar wares in other non-Greek contexts, where bore holes are common on Greek pots–but were immediately rejected. Finally, most of the non-local pots do not show traces of intense use such as chipping and wearing of the paint. It is thus reasonable to assume that the time from production to final deposition of these pots was not considerably different between the place of origin and place of consumption.
In the lowest 14 C-dated phase 11 at Sindos, for the first time in the stratigraphy, we find pottery sherds of wheel-made vases with concentric semicircles. At Kastanas and Lefkandi, this motive appears for the first time during the Middle Protogeometric period. Nonetheless, phase 11 of Sindos probably does not date that early, since a skyphos fragment with a group of zig-zag lines in the handle zone from this phase has numerous parallels in phase 10 at Kastanas that has been firmly dated to Late Protogeometric [ 28 ] [ 36 ].
The pottery from the immediately following phase 10 of Sindos points to a correlation with the Early Geometric or Subprotogeometric I–II periods in southern and central Greece. In this settlement phase appear at Sindos for the first time–at least in considerable numbers–the Thessalo-macedonian cantharoi of type I. This phase has also yielded the earliest fragments of pendent semicircle skyphoi at Sindos as well as numerous fragments of northern Aegean Transport Amphoras of transitional type. Although production of most of these pottery types began in the Late Protogeometric, and continued into the next period, phase 10 can be dated in the Early Geometric or Subprotogeometric I–II phases, i.e. in the first half of the 9 th century according to the conventional chronology, by means of a sherd from a bowl with offset rim that finds good parallels in a closed burial context of the Subprotogeometric I–II at Lefkandi according to the local ceramic sequence [ 28 ].
The overall picture for the ceramic assemblage of phase 9 leaves no doubt that its wheel- and handmade pottery belongs to a period earlier than Middle Geometric II. Especially two ceramic sherds from closed contexts offer a firm date in Middle Geometric I/Subprotogeometric IIIa. On the one hand, the terminus ante quem is set by a fragment of a pendent semicircle skyphos of type 2, which was not produced any more after the end of the Middle Geometric I/Subprotogeometric period IIIa. The terminus post quem for the date of phase 9, on the other hand, is offered by a sherd of Euboean crater with monochrome conical foot decorated with horizontal bulges. This fragment comes from a crater of type II, which cannot be earlier than Middle Geometric I/Subprotogeometric IIIa.
In settlement phase 8 considerable quantities of imported ceramic wares were now used at Sindos for the first time. The most common non-local wares were pendent semicircle skyphoi of the types 4 and 5 that are usually dated in Middle Geometric II, i.e. first half of the 8 th century according to the conventional chronology. This relative chronology of phase 8 is confirmed by several other imported Euboean and Attic wares, which cannot typologically date before Middle Geometric II or after the beginning of Late Geometric. Buildings and other structures of the two best pottery-dated phases predating the Late Geometric phase 7 were excavated on the upper as well as lower table of the settlement [ 28 ].
With phase 7 the settlement of Sindos reached its largest extent (5 ha), and also experienced some remarkable transformations in material culture, including significant innovations in its pottery technology and consumption [ 35 ]. Phase 7 is securely dated by means of Attic and Euboean pottery to Late Geometric Ia, a chronological sub-period that allegedly occupied a single decade, which would make it by far the shortest settlement phase at Sindos, according to the conventional chronology [ 28 ]. During phase 7 deep Euboean skyphoi decorated with concentric circles, dashes and other linear motives on the high lip as well as panels with meanders or hooks and chevrons ( Fig 9 ) or metopes with birds and quatrefoils in the handle zone–all of which are typical of the Late Geometric I period–appeared for the first time at Sindos. At the same time some very characteristic and well-dated Attic vases of Late Geometric Ia with exact typological parallels in the well-defined seriations of Attic pottery were also imported and used at Sindos ( Fig 10 ).

After the destruction of phase 7 by fire, the lower table was not occupied again until the middle of the 6 th century BC, according to the conventional Aegean chronology. The new settlement of phase 6 was now restricted to the smaller plateau of the higher table. The datable pottery of this phase comes mostly from Euboea and continues the previous tradition of the deep skyphoi with the characteristic motives on the lip and the decoration of the handle zone with metopes and panels with hatched hooks and meanders that disappeared only in Late Geometric II. This settlement phase has been subsequently dated to the sub-period Late Geometric Ib, which was defined in Athens by means of a sequence of burial contexts in combination with typological criteria. The next two habitation phases at Sindos, which both predate the 7 th and early 6 th century hiatus, both belong to the Late Geometric period. Their pottery shows features of the later typological developments in the Euboean ceramic sequence that leave no doubt for an assignment to Late Geometric II [ 28 ].
Implications of Sindos stratigraphy for the Greek relative chronology of the Early Iron Age
The study of the pottery finds at Sindos has strongly enhanced our understanding of the temporal changes in ceramic styles not only in the northern but also in the central Aegean, especially during the Geometric period [ 28 ]. Already for this period, there are eight successive phases at Sindos, two of which–phases 7 and 8 –have been excavated to some large extent. Thanks to the continuous stratigraphy of Sindos, it was possible to elucidate for the first time the typo-chronological development of local ceramic categories such as the Protogeometric and Geometric Transport Amphoras and the K 22-Ware as well as certain categories of Euboean wares, such the pendent semicircle skyphoi, the chevron skyphoi ( Fig 9 ) and other types of Atticising and Euboeaning Geometric pottery.
Another significant outcome of pottery studies at Sindos is that certain ceramic wares, previously identified as hallmarks of the pottery production at microregions in central Greece such as Euboea were apparently also locally produced in Macedonia. What is also important, the Macedonian pottery production does not simply imitate the allegedly innovative pottery styles of these regions, which are usually perceived as ‘centres’ with more complex social and economic organisation. Even the local Macedonian wares were part of a common pottery tradition that was dominant everywhere in the north-western Aegean, from Chalkidike and central Macedonia to eastern Thessaly, Phthiotis, Euboea and northern Cyclades [ 37 ]. We recognise that, for example, locally produced skyphoi with pendent semicircles of the same types were produced and used at the same time in Macedonia and Euboea. In clear contradiction to earlier art historical conceptualisations of ancient pottery production and exchange, the recent typo-chronological analysis of the pendent semicircle skyphoi, based on the new finds from Sindos and other sites in central and western Macedonia, shows that the invention of this type in Euboea should not be taken for granted. We should thus keep distance from views that regard the production of many well-known ceramic types in the Aegean ‘periphery’ as later adaptions to stylistic innovations that originated at certain ‘centres’. The use of essentially identical pottery types of both Euboean and Macedonian origin took place, as it now appears, at the same time at Sindos. This conclusion is significant for the purposes of the present study since it allows to argue also by means of local pottery for the correlation of northern and central Aegean relative chronological systems [ 28 ].
It would be fair to state that the stratigraphy of Sindos has proven as helpful for our comprehension of the temporal development of the many non-local ceramic wares, as the excavations conducted at their assumed place of origin. This is true for certain categories of Euboean Middle and Late Geometric pottery that were widely circulating and used in other regions of the Mediterranean, the typological development of which was a much-disputed topic. The most recent excavations at Eretria and their subsequent publication have added much knowledge for the typological development of these wares, but a detailed pottery sequence is still missing in Euboea itself, since the Eretrian pottery contexts are only broadly datable into two or more chronological periods [ 38 ] [ 39 ]. The fine stratigraphy at Sindos provided more detailed information concerning the typological development of Euboean pottery, especially during Middle and Late Geometric [ 28 ].
There are two main reasons that Euboean and Corinthian pottery of the Geometric period has attracted so much scholarly interest in the past decades: first, these are some of the earliest Aegean wares that were circulated and massively used in the Mediterranean after a long break following the end of the Late Bronze Age. Second, the same wares provide what is perceived through a culture-historical perspective as hard evidence for the Greek colonisation.
Even when viewed from the traditional centre-periphery perspective that has dominated historical interpretations for the last two centuries, Sindos offers detailed data not only for the ‘regional’ Macedonian pottery sequence, but also to comprehend the developments at certain ‘centres’ of the central and southern Aegean. In conclusion, due to its long and continuous stratigraphic sequence and its potential for both long- and short-distance correlation of pottery styles the site of Sindos is one of the few places in the Aegean, where it is possible to test the historical chronology of the Early Iron Age. In the present paper this is now accomplished by a series of radiocarbon dates that were measured on a long stratigraphic sequence of short-lived bone samples.
Revision of the Greek Early Iron Age chronology by means of the new radiocarbon dates from Sindos
The new radiocarbon dates from Sindos have important implications for the Greek Early Iron Age chronology, in particular for the periods older than Late Geometric Ib. The dates for the end of the Late Geometric and the beginning of the Archaic period are less affected. A comparison of the newly achieved 14 C-based absolute chronology from Sindos with the conventional chronology is provided in Fig 11 .

It is noteworthy that the traditional sequence of Protogeometric and Geometric periods is well-confirmed by the stratified 14 C-ages from Sindos. Already the satisfactory coherence of the relative pottery sequence, as established at Sindos in high stratigraphic resolution, speaks for the wider applicability of the new chronology. This, of course, requires further confirmation.
The first major implication of the new chronology relates to our understanding of the Late Geometric I period, which is usually perceived as a transformative phase not only for Greece but also for the Mediterranean through an intensification of contacts between the Aegean and the Levant, and the beginning of Greek ‘colonial’ expansion towards the West. All these events are thought to have taken place within a single generation, from 760 to 735 BC (see analytically above). Eight 14 C determinations from phases 6 and 7 at Sindos dating in Late Geometric Ib and Ia respectively present new solid evidence for a redefinition of this historically significant phase. Hints for a higher chronology of the Late Geometric I period from problematic contexts in central and western Mediterranean such as those of Carthage and others, where random Geometric pottery sherds were found [ 40 ] [ 41 ], are confirmed by the new 14 C-series from the northern Aegean, which show that this period may have been longer than usually thought. At Sindos it has empirically measured time span of ca. 130/140 years. Interestingly, the limit between Late Geometric Ia and Ib is now set at 790 calBC and beginning of Late Geometric I at 870 calBC. Settlement phase 7 of Sindos, which dates to the allegedly only short period of Late Geometric Ia, is the best sampled (N = 6 14 C-ages) settlement phase of the entire sequence. In addition, since the 14 C-ages from phase 7 reach well into the steep section of the calibration curve, Late Geometric Ia is also the most precisely dated period at Sindos. In consequence, Late Geometric Ia should not be taken any more as the shortest chronological phase of the Greek Early Iron Age as originally perceived by Coldstream. It covers a time-span much longer than that of other Geometric periods ( Fig 11 ), just as suggested based on the many discernible cultural changes and innovations that took place during this period, all around the Mediterranean.
Three 14 C-determinations from phase 8 raise the beginning of Middle Geometric II from 800 histBC to 930 calBC, a result which agrees well with the recently published radiocarbon dates for seeds from a secure context at Utica in Tunisia, which contained plentiful amounts of Middle Geometric II pottery [ 42 ].
Three further 14 C-ages from settlement phase 9 place Middle Geometric I well into the 10th century, while five 14 C determinations from phase 10 may raise the Early Geometric period–again by around 100 to 150 years–into the second half of the 11th century BC.
Finally, two radiocarbon dates from phase 11 at Sindos that probably dates to Late Protogeometric seem to place this phase of the relative Greek chronology into the first half of the 11th century BC.
Due to missing scientific evidence we may only assume that the underlying Early and Middle Protogeometric would date somewhere before the end of the 12th and beginning of the 11th century BC. To this point it is important to remember the conjectural character of the tripartite definition of the Protogeometric period. Strongly in need of clarification, in relative terms, is the definition of its Middle phase, which is exclusively based on some few mortuary contexts in combination with some not well-defined typological sequences in central and southern Greece. It is mainly for these reasons that the Middle Protogeometric is an extremely elusive phase, especially within settlement contexts.
Sindos is the first Aegean site for which a continuous sequence of 14 C-ages on short-lived samples (animal bones) from the Early Iron Age is now available. Its main amenity is to support critical evaluation of the orthodox historical chronology for the Protogeometric and Geometric periods in north, central and southern Greece. Classical archaeology has long relished a quite unique privilege throughout the circum-Mediterranean chronological systems, in that the underlying absolute dates are based on comprehensive faith in the validity of the antique historiography. The proposed revision of the Greek Early Iron Age (absolute) chronology is in accordance with previous analyses and studies in the northern Aegean as well as in the eastern and western Mediterranean that support a higher chronology [ 4 ] [ 9 ] [ 40 ] [ 41 ] [ 42 ] [ 43 ], if only within given error limits.
Interestingly, the ascertained discrepancies appear to have their largest (joint) cause in the very short time-span (~10 yrs) that is traditionally assigned to Late Geometric Ia. Once introduced–apparently within ±10 yrs of the beginning of the Late Geometric–from that point in time backwards the dating offset remains effectively constant (in the order of 50–80 yrs) for several hundreds of years. At Sindos this ‘down-core’ propagation (wrongly younger) of a nominally constant error (cf. nearly parallel diagonal lines in Fig 11 ) is observable for all phases 7–11, with the notable exception of phase 6 (Late Geometric Ib). A memory-effect for a propagated dating error with this (actually quite small) magnitude would readily explain many of the observed discrepancies between the different chronologies, which are typically of given magnitude, and in particular the proposed updating of the Early Protogeometric at Assiros [ 4 ]. Unfortunately, it is not the initial occurrence of the dating error itself (in Late Geometric Ib), nor its first propagation steps (through the Middle Geometric), but rather more its down-core arrival in the Protogeometric that is difficult to judge. Namely, as can be taken from Fig 11 , for ages older than ca.1000 calBC the GaussWM-derived dating errors from Sindos have values of ±50 yrs (95%) and higher. For all phases of the Protogeometric, this imprecision effectively hampers further meaningful comparisons.
Conclusions
Implications of the revision of greek chronology on aegean and mediterranean archaeology.
It is especially for the younger sections of the Aegean Early Iron Age, in particular for the Late Protogeometric and Geometric periods, that the new data from Sindos may have an impact in our general perception of the cultural and social transformations that took place in the Mediterranean. After a long period of time interregional contacts between Greece and the eastern Mediterranean began again from the Late Protogeometric onwards, while exchanges between the Aegean and Italy were restored in the following Geometric periods. From an archaeological perspective, these contacts are not only recognisable but also best-dated by means of pottery synchronisms. For example, circulation and consumption of drinking cups of the Middle Geometric and Late Geometric I periods overseas are in certain quarters perceived as indicators of Greek and Phoenician ‘pre- or early colonial’ activity. During those periods the Greeks, and probably also other people, are usually thought to have adopted the alphabet from the Phoenicians, appropriated new cultural and social habits such as the symposion, and prepared the ground for one of the most influential events in Mediterranean history, the ‘apoikismos’. According to the conventional chronology all this is thought to have taken place within a period of two generations, from 800 to 735 BC. Particularly significant in this respect is the allegedly short Late Geometric Ia phase, but which probably contains the bulk of the so-called ‘pre-colonial’ pottery in western Mediterranean. The proposed changes in the absolute Greek and consequently Mediterranean chronology may thus change our understanding of the timing and duration of (short) historical events, or (long) cultural processes that took place during the Early Iron Age. One of the rising questions is, for example, whether the transfer of writing really did take place within the time-span of only one generation, as is often assumed, and whether its adoption really did occur at some time in the 8th century calBC, or not already in the second half of the 9 th century calBC. Furthermore, the archaeologically visible restoration of contacts between the Aegean and the western Mediterranean, following the end of the Late Bronze Age, may not date to the first half of the 8th century calBC, but this occurred instead–based on the new evidence from Sindos–much earlier.
Any attempt to revise a well-established and widely respected–despite its deficiencies–chronological system is a major challenge. Dating revisions even on the seemingly small (multi-decadal) scale proposed here will naturally be perceived as inconvenient, in many respects, but herein especially due to their implications for the chronological systems and historical narratives of the Early Iron Age that are accepted as authoritative on a supra-regional scale. Instead, we put forward for the first time a series of radiocarbon dates for the Protogeometric and Geometric periods in the Aegean. The results contradict many of the interpretations that were based on, in our view, some largely ambiguous historiographic and archaeological dating methods. What is certainly needed are many further series of 14 C-ages that derive from secure and well-published contexts in the Aegean and other regions, where Greek pottery was used, at best from long tell-stratigraphies and in combination with large-scale statistical pottery dating, based e.g. on Correspondence Analysis [ 34 ].
Acknowledgments
Problems of the Greek and Mediterranean Late Bronze and Early Iron Age were discussed with many colleagues. We are particularly grateful to José Luis Lopez Castro, Alfredo Mederos Martín, Gunnar Lehmann and Francisco J. Nuñez. Special thanks are also to Alfred Galik, who carefully examined the animal bones from Sindos. The comments and suggestions made by the anonymous reviewers of this paper were critical, as well as substantial and helpful in many aspects, and also deserve our particular accreditation. The permission for sampling and 14 C-analysis was kindly issued by the Greek authorities.
Funding Statement
The dating project was funded within the budgets of the stand-alone FWF projects P 26150 and P 30475 that were implemented by Stefanos Gimatzidis ( https://www.fwf.ac.at/de/ ). The funders had no role in study design, data collection and analysis, decision to publish, or preparation of the manuscript.
Data Availability
Using Graphic Organizers for Writing Essays, Summaries and Research
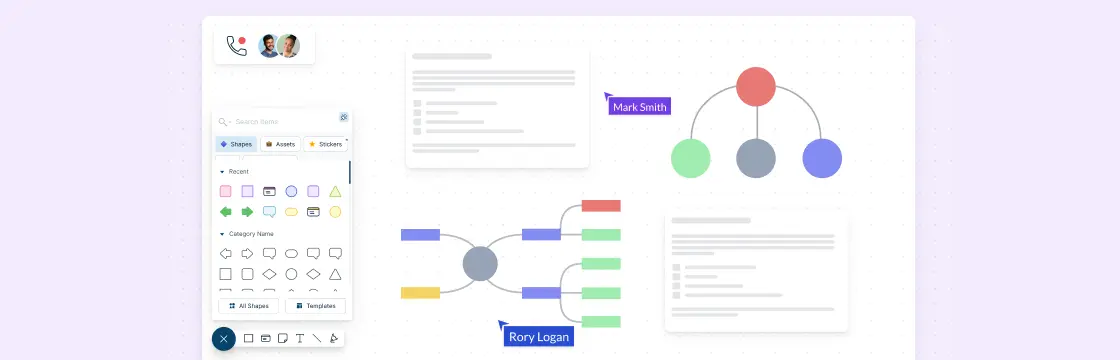
Ask any student – essay writing is one of the most despised tasks of their educational career. Perhaps there is so much displeasure associated with the task because it’s perceived as too linear – there isn’t enough visual and creative appeal. But if you use graphic organizer for writing essays then you can make writing enjoyable – or at least less terrible.
Not only enjoyable but graphic organizers (or diagrams) can make the writing process a snap. They’ll help you think outside the box, draw conclusions you wouldn’t normally observe, and make the entire process faster and more efficient.
Why Use Graphic Organizers for Writing
The phrase “graphic organizer” is just a fancy way of saying “diagram” or “visual aid.” Basically, they are a visual representation of the information you’ve acquired in the research process. There are quite a few reasons why you should use them when writing essays or summaries.
- Helps you visualize your research and how elements connect with each other
- Enhance your essays, summaries and research papers with visual elements
- Track correlations between your thoughts, observations, facts or general ideas
When it comes to essay writing, the most common graphic organizers are webs, mind maps, and concept maps .
Using Webs for Brainstorming
Webbing is a great way to see how various topics are interrelated. This graphic organizer is particularly useful during the brainstorming step of the writing process.
A web can sometimes get a bit messy. Usually, there are lots of arrows to connect overlapping ideas. However, even with lines crisscrossing every which way, it is still a great way to visualize your thoughts. If you’re using an online diagramming software like Creately you can overcome some of this because we automatically arrange the object for you.
Once you’ve created a map to document all your ideas and establish connections, you can easily transition to other forms of diagramming to better organize the information.
For example if you’re writing a research paper about the food web of the Australian bushes you can start creating a food web diagram similar to the one below. This way you can easily visualize the web while writing the paper. This is a simple example but graphic organizers become even more important when the subject gets complex.
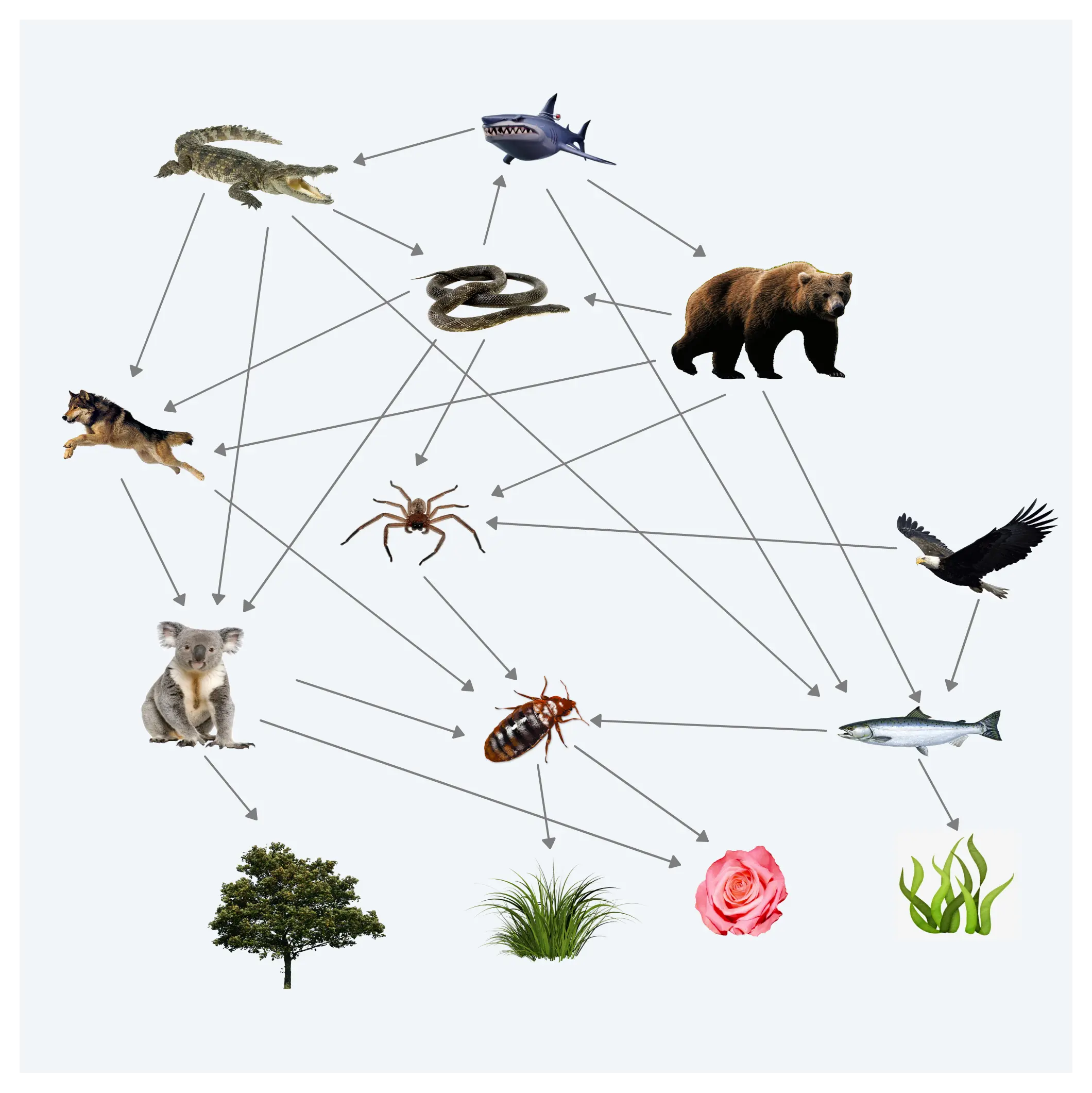
Although simple this example shows the importance of using graphic organizers for writing summaries. A comprehensive diagram pretty much does the summation for you.
Using Mind Maps as Graphic Organizers
Mind maps are a great way to depict a hierarchy. What is hierarchical organization ? The concept is simple: a singular topic dominates with each subsequent idea decreasing in importance.
Usually, the mind map starts with the thesis (or main idea) at the center. From there, you can branch out with your supporting evidence.
Use this process to replace your traditional note taking technique – note cards, outlines, whatever. You’ll quickly realize a mind map is a great way to formulate the structure of your essay. The thing to note here is that the nature of the mind maps force you think about sub topics and how to organize your ideas. And once the ideas are organized writing the essay become very easy.
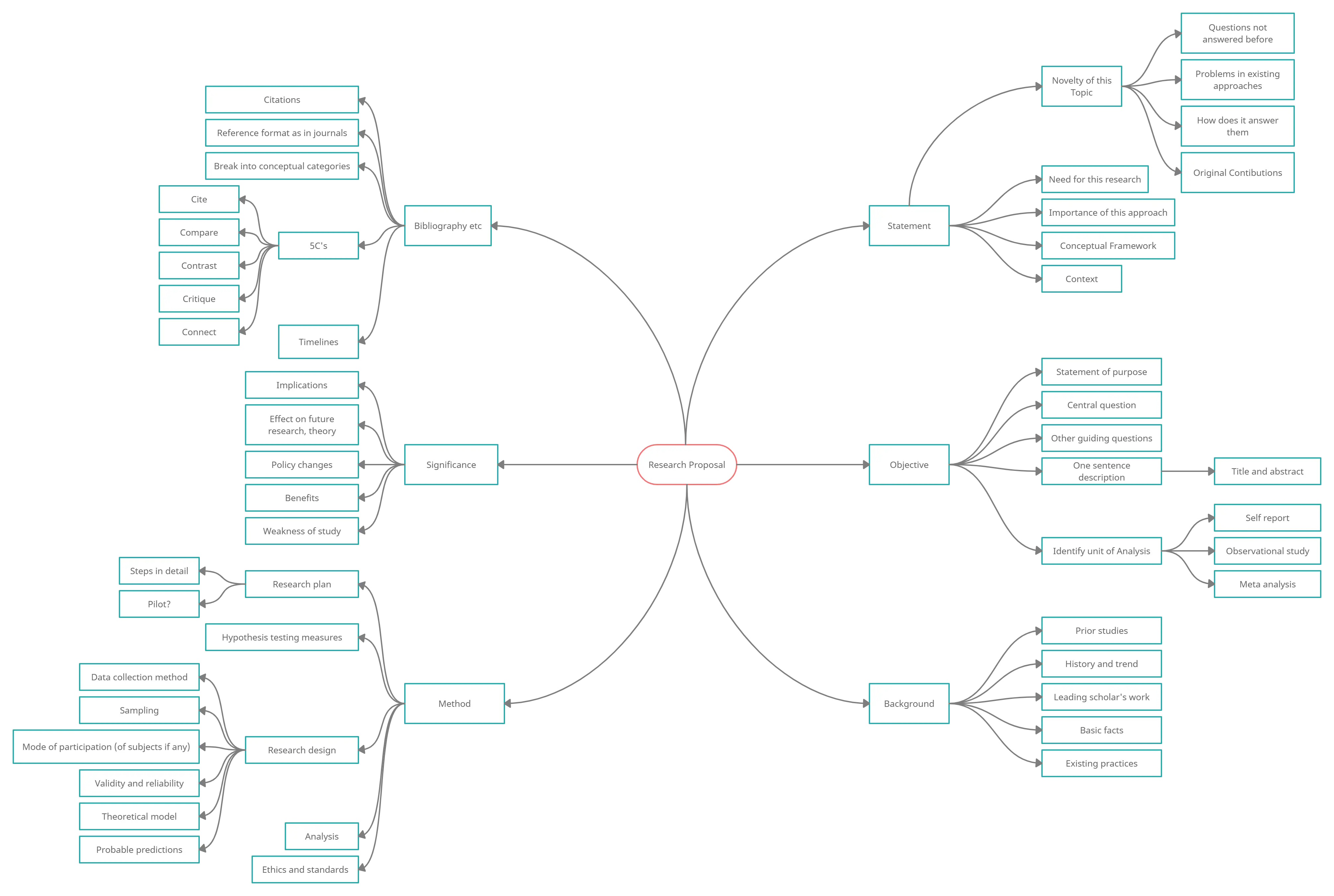
Above is a mind map of a research proposal. Click on it to see the full image or you can see the fully editable template via this link . As you can see in this mind map the difference areas of the research proposal is highlighted. Similarly when your writing the research paper you can use a mind map to break it down to sub topics. We have more mind map templates for you to get started.
Concept Maps
A concept map will help you visualize the connection between ideas. You can easily see cause and effect – how one concept leads to another. Often times, concept mapping includes the use of short words or phrases to depict the budding relationship between these concepts.
If you look closely you can see that its very similar to a mind map. But a concept maps gives more of a free reign compares to the rigid topic structure of a mind map. I’d say it’s the perfect graphic organizer for writing research papers where you have the license to explore.
By creating a concept map , you can also see how a broad subject can be narrowed down into specific ideas. This is a great way to counter writers block. Often, we look at the big picture and fail to see the specifics that lead to it. Identifying contributing factors and supporting evidence is difficult. But with a concept map, you can easily see how the smaller parts add up to the whole.
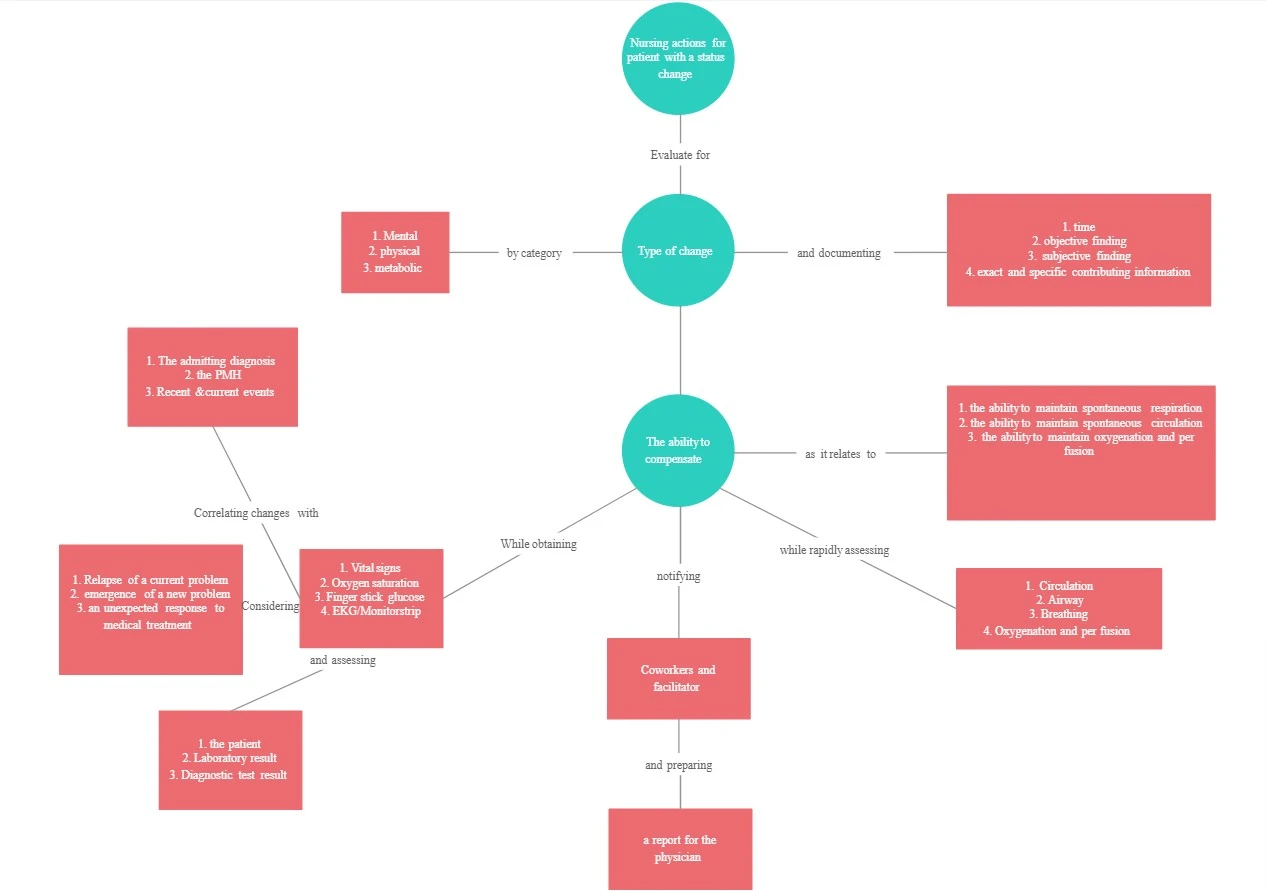
Why Bother With Graphic Organizers?
If you already detest the writing process, adding another step might seem insane. However, there really are several advantages of using them. If you haven’t already accepted the benefits of each individual diagram style, here are some more perks of graphic organizers in general:
- Quality essays are based on detail. No one is going to accept your opinions and reasoning just because you say so. You’ll need proof. And organizing that proof will require attention to detail. Graphic organizers can help you see that detail and how it contributes to the overall concept.
- Graphic organizers are flexible. You don’t need one of those giant pink erasers. You don’t need to restructure your outline. All you have to do is draw a few arrows and bam – the relationship has totally changed.
- No matter what you are writing about, a graphic organizer can help. They can be used to structure an essay on the Great Wall, theoretical physics, or Spanish speaking countries.
- If you write an outline, can you easily see how point A influences point X? Probably not. But if little thought bubble A is sitting out there all by itself, you can visualize the way it ties into point R, T and X.
- Some of us find it difficult to put our opinions, thoughts, and ideas into writing. However, communicating our feelings with little doodles and sketches is far less threatening.
- As a writer, our brain often feels like a 2-year-old’s toy box – a big jumbled mess. Taking that mess and putting it onto paper with some semblance of organization is challenging. Rather than trying to take your thoughts from total chaos to a perfectly structured list, just try to get them out of your brain and onto paper in the form of a diagram.
- A graphic organizer helps you establish validity and relevance. You can easily nix the ideas that don’t support or enhance your thesis.
The next time you are faced with a writing project, take a few minutes to explore the efficiency of graphic organizers. You can find a wealth of templates here.
Have you ever used a graphic organizer to structure an essay? How did it go? Do you have a diagram suggestion for the writing process that wasn’t mentioned here? Let us know!
Join over thousands of organizations that use Creately to brainstorm, plan, analyze, and execute their projects successfully.
More Related Articles
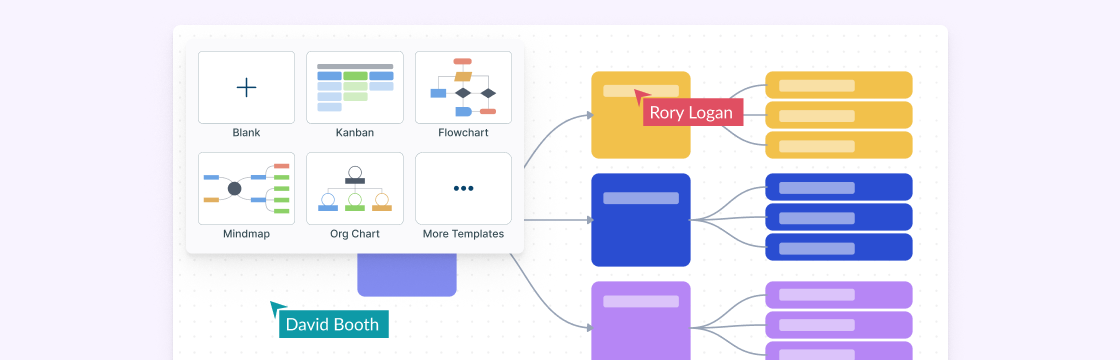
These are awesome guest posts contributed by our users and technology enthusiasts. Do you have something interesting to share? Want to get exposed to a massive tech audience? Check out our Guest Posting Guidelines to how to proceed.
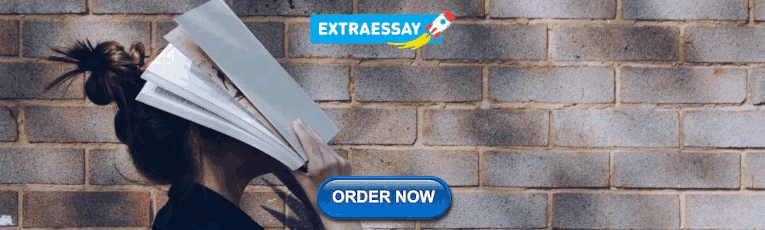
IMAGES
VIDEO
COMMENTS
Activity: Graphic Organizer and Writing Assignment. Students sort artifacts into those that can and can't be dated using radiocarbon dating methods, then they identify how different kinds of scientists might use radiocarbon dating. Finally, they identify challenges and assumptions that Libby had to make when doing his work.
Graphic Organizers (Semantic Maps) dealing with: Radiometric Dating (Absolute Dating & Age) Carbon-14 Radiocarbon Dating; Potassium-40 K-Ar Dating; Isotopes & Radioisotopes ; Nuclides & Radionuclides; Parent atoms & Daughter atoms; Radioactive Decay - Alpha, Beta & Gamma; and. Half-life; 2) Complete the counting & coloring of Parent & Daughter ...
graphic organizer exercise - Free download as Word Doc (.doc / .docx), PDF File (.pdf), Text File (.txt) or view presentation slides online. Scribd is the world's largest social reading and publishing site.
c. Carbon 14 (Radiocarbon) • Used for organic matter • Short half-life (5,730 years) • Useful only in dating objects accurately back to 40,000 years. • Fundamentally different from parent-daughter systems because 14C is continuously created in the atmosphere by bombardment of nitrogen by cosmic rays -Cosmic radiation bombards nitrogen.
The Carbon-14 Cycle. Radiocarbon dating (usually referred to simply as carbon-14 dating) is a radiometric dating method. It uses the naturally occurring radioisotope carbon-14 (14 C) to estimate the age of carbon-bearing materials up to about 58,000 to 62,000 years old.Carbon has two stable, nonradioactive isotopes: carbon-12 (12 C) and carbon-13 (13 C).
Radiocarbon Dating. Radiocarbon dating is one of the most used method of absolute dating because of its useful dating window encompassing the past 100,000 years (it is especially useful for studying archeological features and young sedimentary deposits). 14 C (isotope carbon -14) is a unstable radioactive isotope (radionuclide). Radiocarbon dating (using ratios of the isotopes of radioactive ...
Radiocarbon dating is one of most important techniques used by archaeologists throughout the world. Here at Penn State, we are extremely privileged to have an accelerated mass spectrometry radiocarbon dating lab at the Institutes for Energy and the Environment (IEE) Energy and Environmental Sustainability Laboratories (EESL). Our lab fully engages with extensive use of this resource for dating ...
Radiocarbon dating uses the decay of a radioactive isotope of carbon (14 C) to measure time and date objects containing carbon-bearing material.With a half-life of 5,700 ± 30 years, detection of 14 C is a useful tool for determining the age of a specimen formed over the past 55,000 years. In this Primer, we outline key advances in 14 C measurement and instrument capacity, as well as optimal ...
This chapter reviews the key events associated with the development of the radiocarbon (14 C) dating method immediately following World War II by Willard F. Libby (1909-1980) and his collaborators, James R. Arnold (1923-2013), and Ernest C. Anderson (1920-2013).It also considers the historical background and earlier discoveries that Libby and others drew upon in forming the concepts that ...
All air is evacuated from the vacuum line because it has C-14 in it and is a potential contaminant. Then a stream of oxygen is added into the system and the sample is combusted. It is during this stage that the carbon present in the sample is converted into carbon dioxide. The carbon dioxide is collected and bubbled through various chemicals in ...
The authors argue for the widespread adoption of radiocarbon dating, particularly in cultural resource management (CRM) projects, suggesting its significant impact on creating more accurate chronologies. The article concludes by emphasizing the shared goals across different sectors of archaeology in writing accurate and meaningful histories.
Radiocarbon dating uses the decay of a radioactive isotope of carbon (14 C) to measure time and date objects containing carbon-bearing material.With a half-life of 5,700 ± 30 years, detection of 14 C is a useful tool for determining the age of a specimen formed over the past 55,000 years. In this Primer, we outline key advances in 14 C measurement and instrument capacity, as well as optimal ...
1. Introduction. Consider two ways that we may date artifacts and samples. First, traditional methods of historical analysis and archaeology enable us to date artifacts; and the counting of tree rings enables us to date wood from ancient trees. Second, radiocarbon dating provides another means of dating these samples.
This Packet Includes 18 Assignments that will help supplement and differentiate your topic coverage. Assignments range from writing to creative ones.*View the Preview for an example of each assignment to make sure this packet is a fit for your classroomPacket Includes:Pyramid Summary WorksheetHisto...
The Carbon-14 Cycle. Radiocarbon dating (usually referred to simply as carbon-14 dating) is a radiometric dating method. It uses the naturally occurring radioisotope carbon-14 ( 14C) to estimate the age of carbon-bearing materials up to about 58,000 to 62,000 years old. Carbon has two stable, nonradioactive isotopes: carbon-12 (12C) and carbon ...
1. Every living thing is made of: carbon. 2. How is Carbon 12 different from Carbon 14? carbon 14 is unstable. 3. How can scientists determine how long it has been (in thousands of years) since an animal has died? b. by measuring the ratio of Carbon 14 to Carbon 12.
When an organism dies, it no longer absorbs carbon-14. The C-14 it does contain in its tissues starts to decay at a constant rate. Name: Radiocarbon dating. Material used: Organic remains such as wood and seeds. Age range: Younger than 60,000 years ago. How it works: Measures the amount of radioactive carbon-14 in the organic remains of living things
The writing propels the reader through an adventure following dating successes and controversies around the ... development of AMS radiocarbon dating are covered near the end of the chapter. Chapter 9 provides the reader with a guide to bibliographic sources for radiocarbon dating. General issues, bibliographies, the journal Radiocarbon ...
Radiocarbon dating remains the most widely applicable, accurate, and reliable chronometric dating technique available to archaeologists. Radiocarbon (also called 14 C) dating measures the concentration of naturally occurring radioactive 14 C that is formed in the upper atmosphere. 14 C is an unstable, or radioactive, isotope because it contains extra neutrons in its nucleus and, as a ...
This chapter reviews the basic elements of the radiocarbon (14 C) dating method and summarizes three generations of 14 C studies in archaeology.It considers in greater detail several major advances in 14 C research including the extension of the calibration of the 14 C time scale into the late Pleistocene, further detailed characterization of Holocene short-term perturbations (de Vries effects ...
The new radiocarbon dates from Sindos have important implications for the Greek Early Iron Age chronology, in particular for the periods older than Late Geometric Ib. The dates for the end of the Late Geometric and the beginning of the Archaic period are less affected. A comparison of the newly achieved 14 C-based absolute chronology from ...
In addition, the postmortem interval based on weathering is inaccurate and very difficult if not impossible to assess with any accuracy. Therefore, traditional methods such as skeletal weathering are not recommended. Thus, we recommend adopting radiocarbon dating as standard forensic practice.
The phrase "graphic organizer" is just a fancy way of saying "diagram" or "visual aid.". Basically, they are a visual representation of the information you've acquired in the research process. There are quite a few reasons why you should use them when writing essays or summaries. Helps you visualize your research and how elements ...