Coronavirus (COVID-19): Latest Updates | Visitation Policies Visitation Policies Visitation Policies Visitation Policies Visitation Policies | COVID-19 Testing | Vaccine Information Vaccine Information Vaccine Information
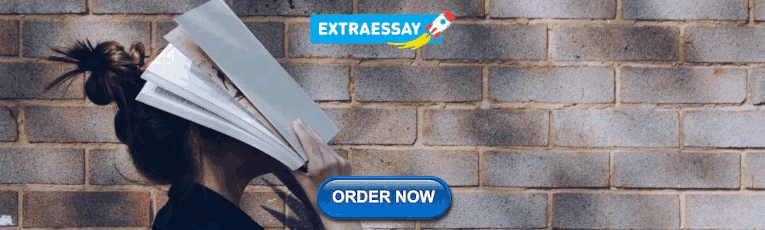
URMC & UR Research Labs
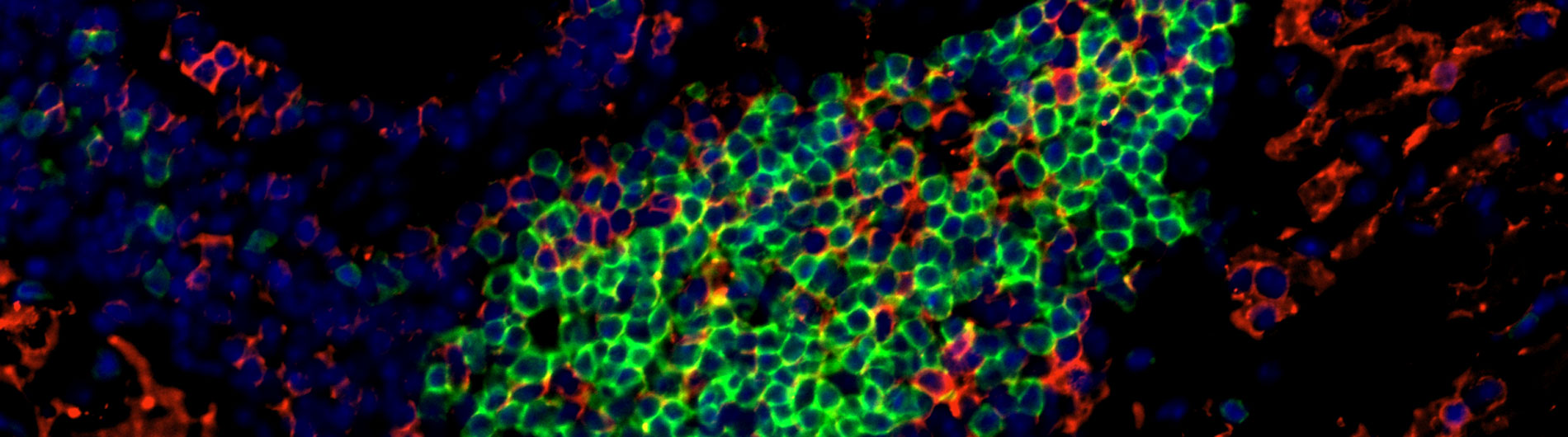
Explore Our URMC & UR Research Labs
The University of Rochester Medical Center and University of Rochester are home to more than 3,000 researchers and 500+ labs dedicated to scientific research. Our researchers study common and rare illnesses - from cancer and heart disease and Parkinson’s to pandemic Influenza and COVID-19. These efforts have led to therapies that have saved countless lives and improved human health locally, in the region, and across the globe.
Our research makes the world a better place.
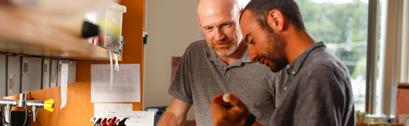
Alphabetical Listing of Labs
A B C D E F G H I J K L M N O P Q R S T U V W X Y Z
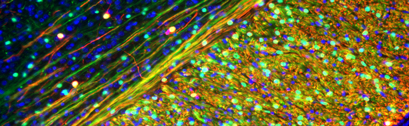
Labs Department & Center Listing
Research news.

Masks Strongly Recommended but Not Required in Maryland, Starting Immediately
Due to the downward trend in respiratory viruses in Maryland, masking is no longer required but remains strongly recommended in Johns Hopkins Medicine clinical locations in Maryland. Read more .
- Vaccines
- Masking Guidelines
- Visitor Guidelines
Understanding Clinical Trials
Clinical research: what is it.
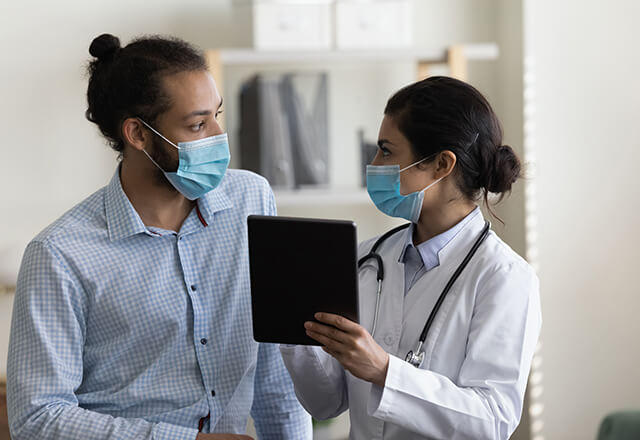
Your doctor may have said that you are eligible for a clinical trial, or you may have seen an ad for a clinical research study. What is clinical research, and is it right for you?
Clinical research is the comprehensive study of the safety and effectiveness of the most promising advances in patient care. Clinical research is different than laboratory research. It involves people who volunteer to help us better understand medicine and health. Lab research generally does not involve people — although it helps us learn which new ideas may help people.
Every drug, device, tool, diagnostic test, technique and technology used in medicine today was once tested in volunteers who took part in clinical research studies.
At Johns Hopkins Medicine, we believe that clinical research is key to improve care for people in our community and around the world. Once you understand more about clinical research, you may appreciate why it’s important to participate — for yourself and the community.
What Are the Types of Clinical Research?
There are two main kinds of clinical research:
Observational Studies
Observational studies are studies that aim to identify and analyze patterns in medical data or in biological samples, such as tissue or blood provided by study participants.
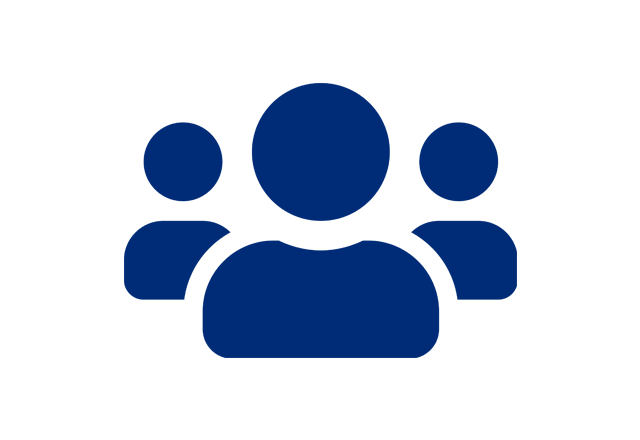
Clinical Trials
Clinical trials, which are also called interventional studies, test the safety and effectiveness of medical interventions — such as medications, procedures and tools — in living people.
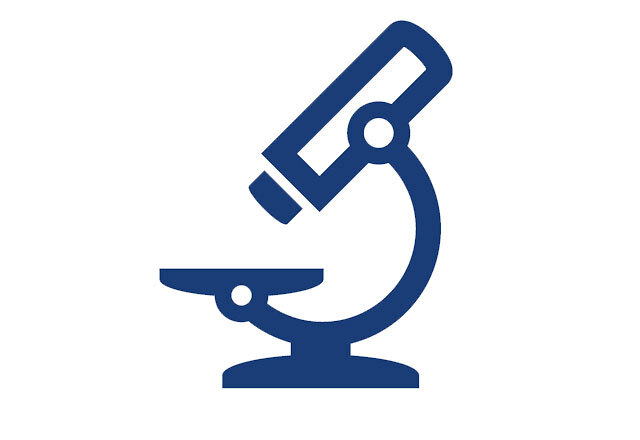
Clinical research studies need people of every age, health status, race, gender, ethnicity and cultural background to participate. This will increase the chances that scientists and clinicians will develop treatments and procedures that are likely to be safe and work well in all people. Potential volunteers are carefully screened to ensure that they meet all of the requirements for any study before they begin. Most of the reasons people are not included in studies is because of concerns about safety.
Both healthy people and those with diagnosed medical conditions can take part in clinical research. Participation is always completely voluntary, and participants can leave a study at any time for any reason.
“The only way medical advancements can be made is if people volunteer to participate in clinical research. The research participant is just as necessary as the researcher in this partnership to advance health care.” Liz Martinez, Johns Hopkins Medicine Research Participant Advocate
Types of Research Studies
Within the two main kinds of clinical research, there are many types of studies. They vary based on the study goals, participants and other factors.
Biospecimen studies
Healthy volunteer studies.
Clinical trials study the safety and effectiveness of interventions and procedures on people’s health. Interventions may include medications, radiation, foods or behaviors, such as exercise. Usually, the treatments in clinical trials are studied in a laboratory and sometimes in animals before they are studied in humans. The goal of clinical trials is to find new and better ways of preventing, diagnosing and treating disease. They are used to test:
Drugs or medicines
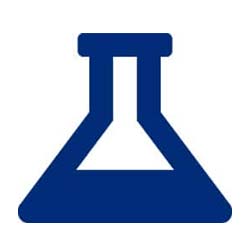
New types of surgery
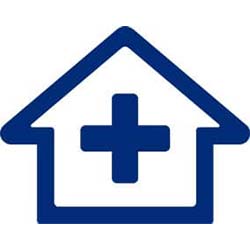
Medical devices
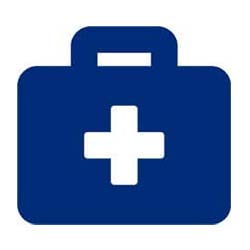
New ways of using current treatments
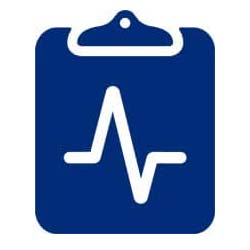
New ways of changing health behaviors
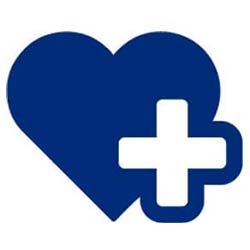
New ways to improve quality of life for sick patients
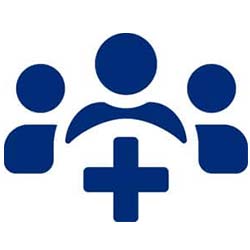
Goals of Clinical Trials
Because every clinical trial is designed to answer one or more medical questions, different trials have different goals. Those goals include:
Treatment trials
Prevention trials, screening trials, phases of a clinical trial.
In general, a new drug needs to go through a series of four types of clinical trials. This helps researchers show that the medication is safe and effective. As a study moves through each phase, researchers learn more about a medication, including its risks and benefits.
Is the medication safe and what is the right dose? Phase one trials involve small numbers of participants, often normal volunteers.
Does the new medication work and what are the side effects? Phase two trials test the treatment or procedure on a larger number of participants. These participants usually have the condition or disease that the treatment is intended to remedy.
Is the new medication more effective than existing treatments? Phase three trials have even more people enrolled. Some may get a placebo (a substance that has no medical effect) or an already approved treatment, so that the new medication can be compared to that treatment.
Is the new medication effective and safe over the long term? Phase four happens after the treatment or procedure has been approved. Information about patients who are receiving the treatment is gathered and studied to see if any new information is seen when given to a large number of patients.
“Johns Hopkins has a comprehensive system overseeing research that is audited by the FDA and the Association for Accreditation of Human Research Protection Programs to make certain all research participants voluntarily agreed to join a study and their safety was maximized.” Gail Daumit, M.D., M.H.S., Vice Dean for Clinical Investigation, Johns Hopkins University School of Medicine
Is It Safe to Participate in Clinical Research?
There are several steps in place to protect volunteers who take part in clinical research studies. Clinical Research is regulated by the federal government. In addition, the institutional review board (IRB) and Human Subjects Research Protection Program at each study location have many safeguards built in to each study to protect the safety and privacy of participants.
Clinical researchers are required by law to follow the safety rules outlined by each study's protocol. A protocol is a detailed plan of what researchers will do in during the study.
In the U.S., every study site's IRB — which is made up of both medical experts and members of the general public — must approve all clinical research. IRB members also review plans for all clinical studies. And, they make sure that research participants are protected from as much risk as possible.
Earning Your Trust
This was not always the case. Many people of color are wary of joining clinical research because of previous poor treatment of underrepresented minorities throughout the U.S. This includes medical research performed on enslaved people without their consent, or not giving treatment to Black men who participated in the Tuskegee Study of Untreated Syphilis in the Negro Male. Since the 1970s, numerous regulations have been in place to protect the rights of study participants.
Many clinical research studies are also supervised by a data and safety monitoring committee. This is a group made up of experts in the area being studied. These biomedical professionals regularly monitor clinical studies as they progress. If they discover or suspect any problems with a study, they immediately stop the trial. In addition, Johns Hopkins Medicine’s Research Participant Advocacy Group focuses on improving the experience of people who participate in clinical research.
Clinical research participants with concerns about anything related to the study they are taking part in should contact Johns Hopkins Medicine’s IRB or our Research Participant Advocacy Group .
Learn More About Clinical Research at Johns Hopkins Medicine
For information about clinical trial opportunities at Johns Hopkins Medicine, visit our trials site.
Video Clinical Research for a Healthier Tomorrow: A Family Shares Their Story
Clinical Research for a Healthier Tomorrow: A Family Shares Their Story
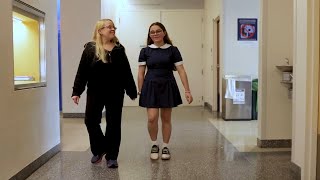
What can we help you find?
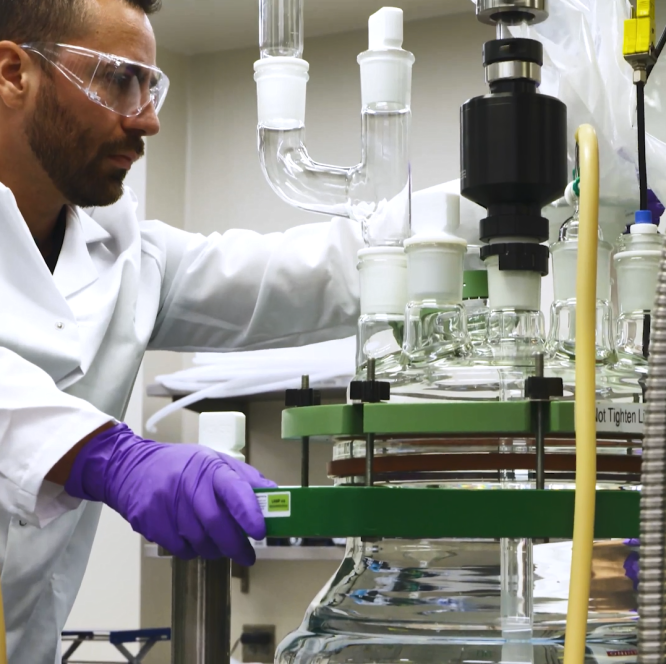
We run to, not from, the world’s biggest health challenges.
The journey to discovery is guided by science – and inspired by patients
We use the power of leading-edge science to save and improve lives around the world. The path to discovery is often unclear, but we are tireless in seeking solutions for some of the world’s most difficult health challenges.
Our work in numbers
people employed in research and development
invested in R&D in 2023
Our areas of focus
We focus on scientific innovation to deliver medicines and vaccines that may help millions of people around the world.
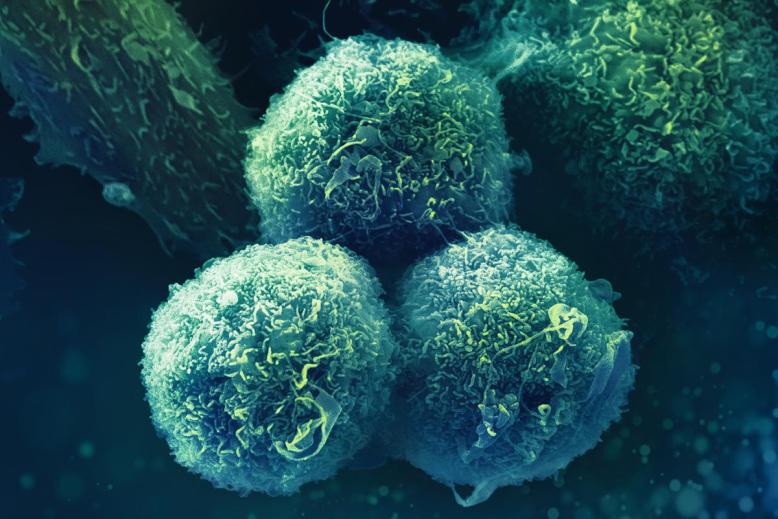
Our team of researchers and scientists are pushing the boundaries of cancer research to discover more effective anticancer therapies.
Our work in oncology
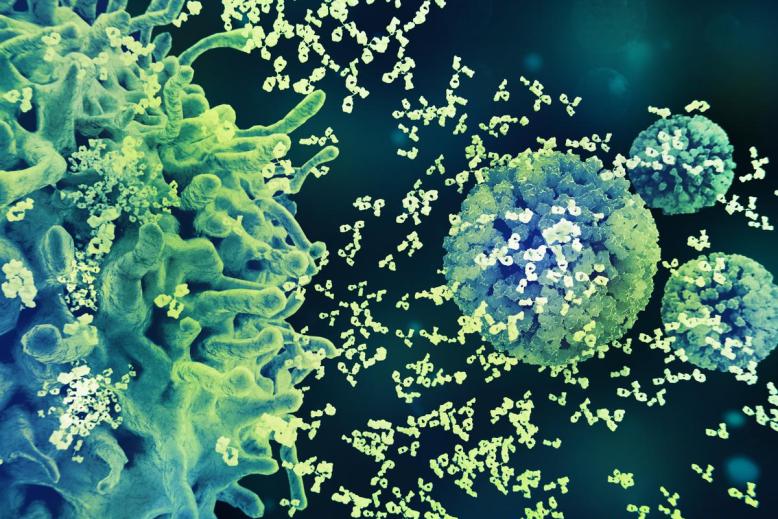
Vaccines are one of the greatest public health success stories – and we’ve been discovering, developing, supplying and delivering vaccines to help prevent disease for over 130 years.
Our work in vaccines
Infectious diseases
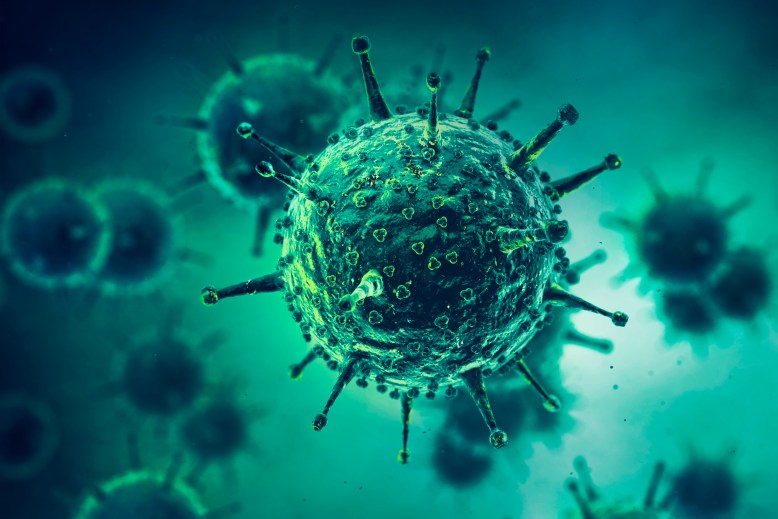
Our focus has always been on the prevention and treatment of diseases that threaten people and communities around the world.
Our work in infectious diseases
Cardio-metabolic disorders
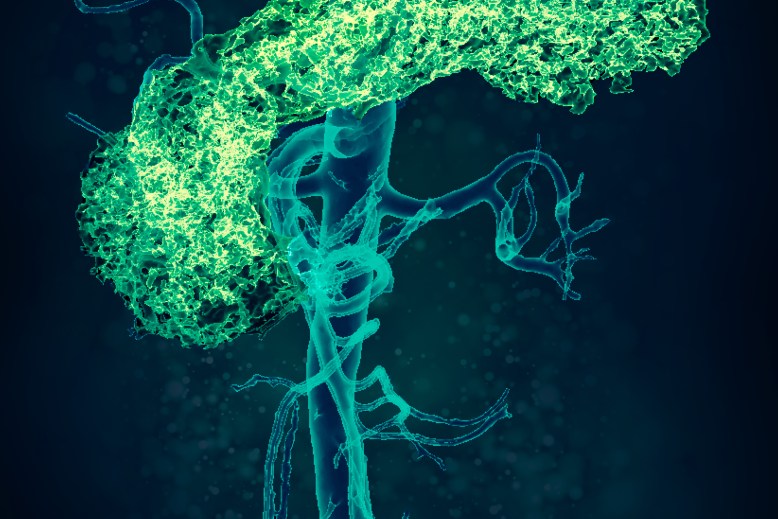
We have a long history of making an impact in cardio-metabolic disorders, such as type 2 diabetes and cardiovascular disease.
Our work in cardio-metabolic disorders
Discovery & development
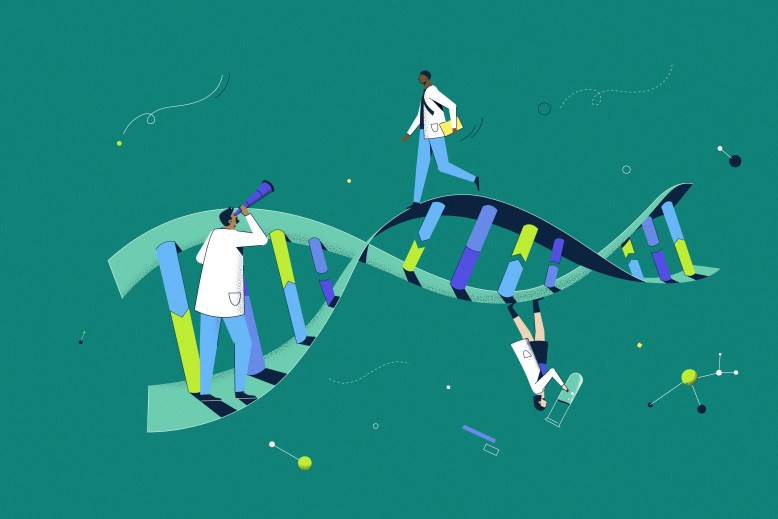
We follow the science where we can make the greatest difference, now and in the future.
More about discovery and development
We address the world’s most difficult health challenges, following leading-edge science
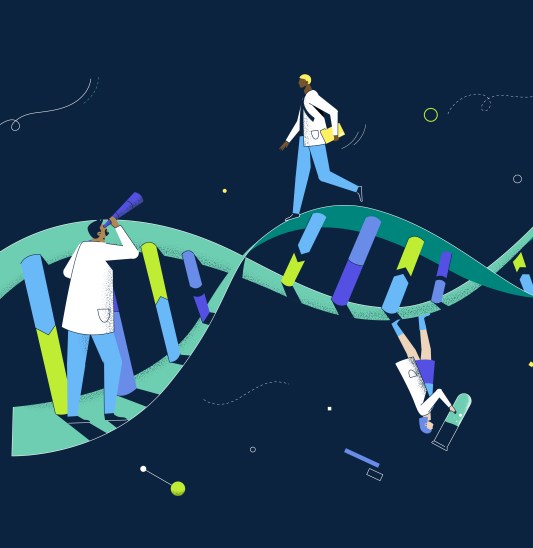
Step inside Merck Research Laboratories (MRL) where our quest to save and improve lives through research and development begins
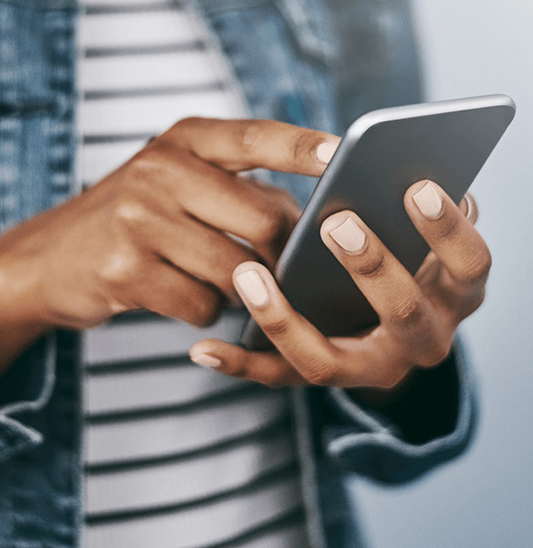
We’re focused on inventing new medicines and vaccines to help more people
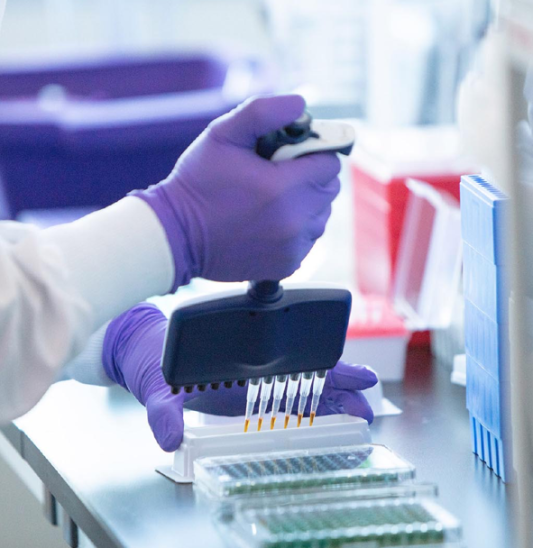
Our focus on breakthrough science is working
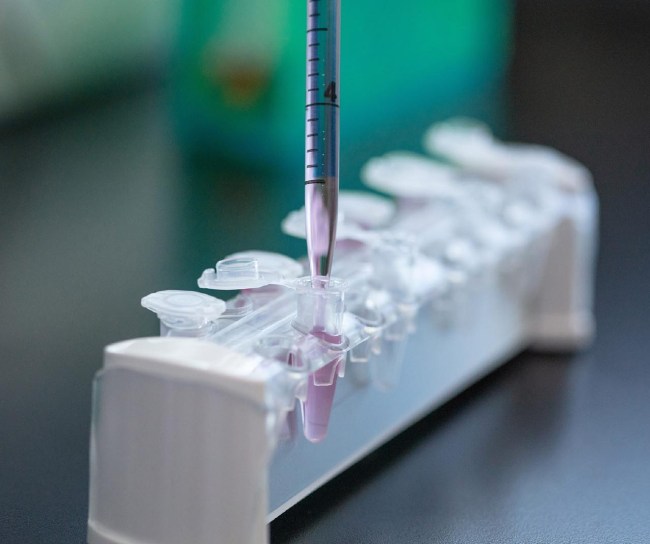
Clinical trials
Our progress is due in large part to the important and tough scientific questions we set out to answer with our trials and collaborations. We are grateful to the thousands of volunteers who participate in our clinical trials — making this all possible.
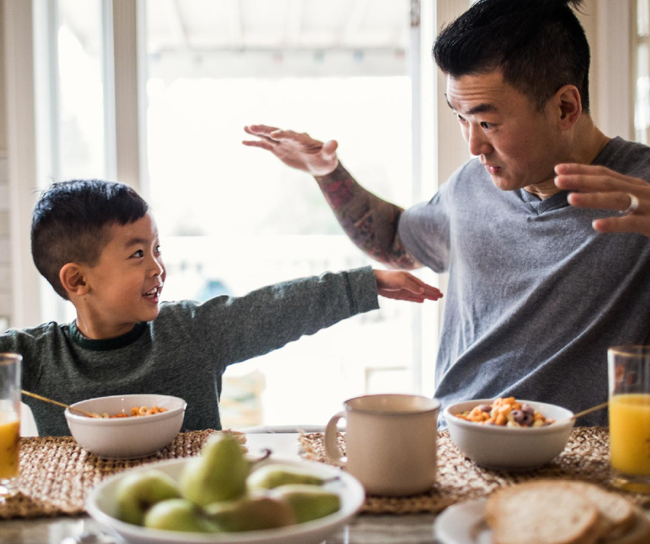
Our commitment to patients is unwavering
As long as there are still patients in hospitals, doctors and nurses desperate to add years to the lives of their patients, and a world where treatments aren’t accessible to all, we will be here, fighting with all we have to deliver more, sooner.
Discovery starts here
Our scientists are applying the latest groundbreaking research technologies and revolutionizing how we discover and develop medicines and vaccines
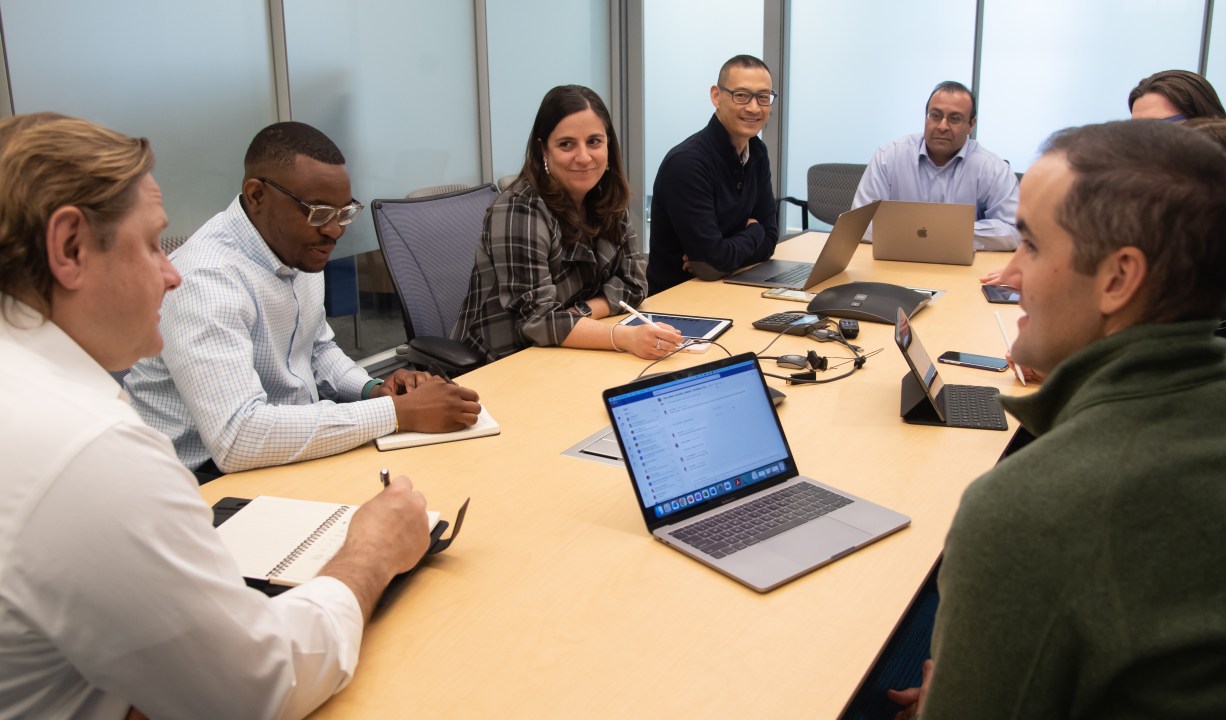
What is One Pipeline?
How we’re advancing the best internal and external science to progress our pipeline for patients
Next: What is One Pipeline?
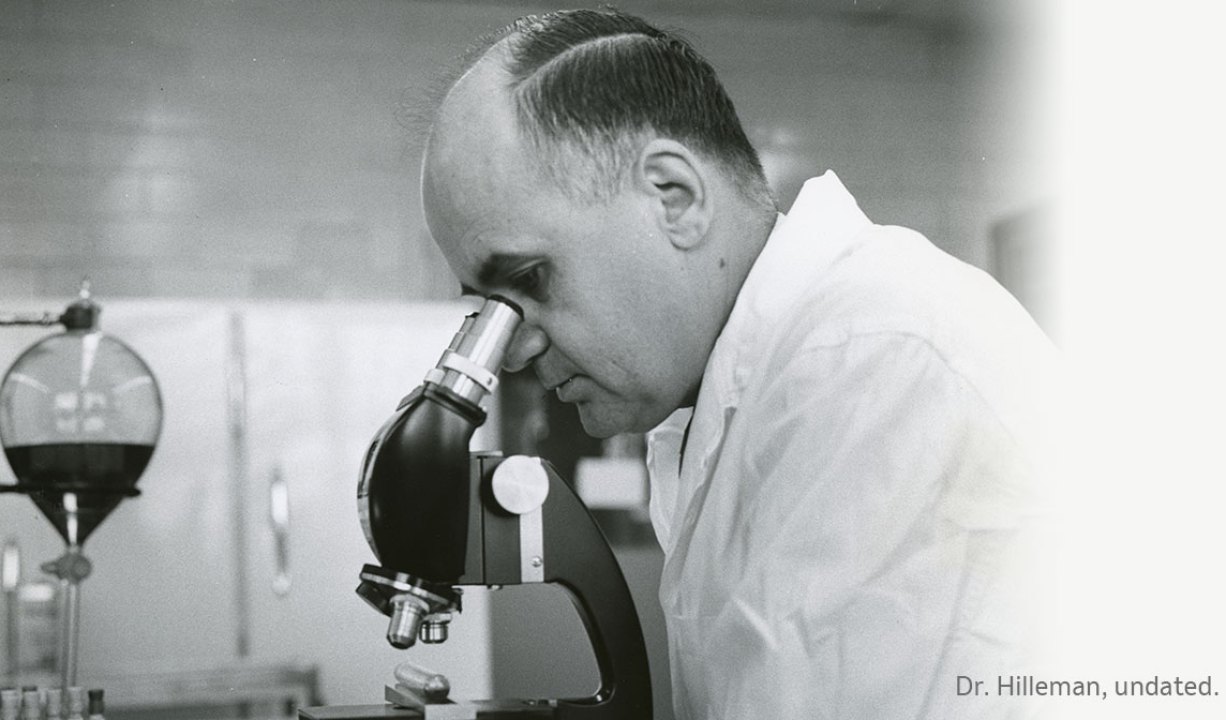
Vaccine inventors, creators and innovators
Dr. Maurice Hilleman was among the pioneering scientists who made strides in vaccine history and the fight against infectious disease
Next: Vaccine inventors, creators and innovators
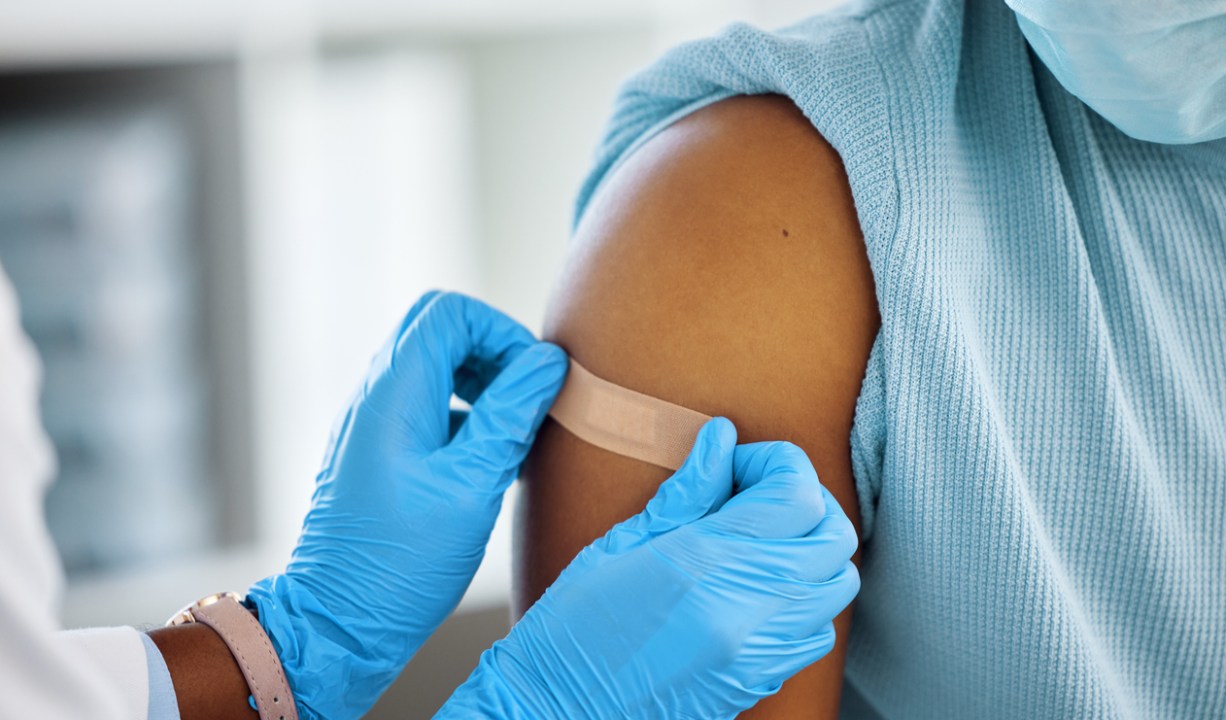
Vaccines: Our history, our legacy
We've been working to discover and develop vaccines for more than a century
Next: Vaccines: Our history, our legacy
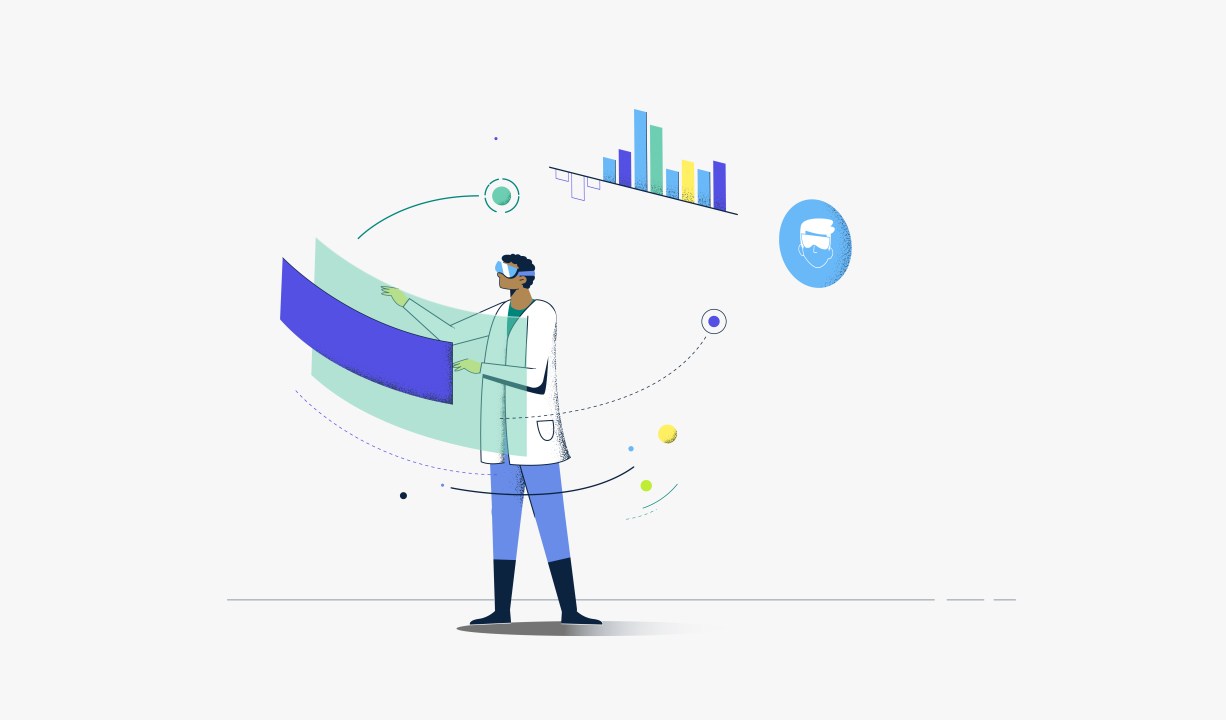
Our Q1 2024 earnings report
Merck’s (NYSE: MRK) Q1 2024 results reflect continued strong growth in oncology and vaccines. Our company announced Q1 worldwide sales of $15.8 billion, an increase of 9% from Q1 2023. “Merck has begun 2024 with continuing momentum in our business. We are harnessing the power of innovation to advance our deep pipeline and are maximizing […]
Next: Our Q1 2024 earnings report
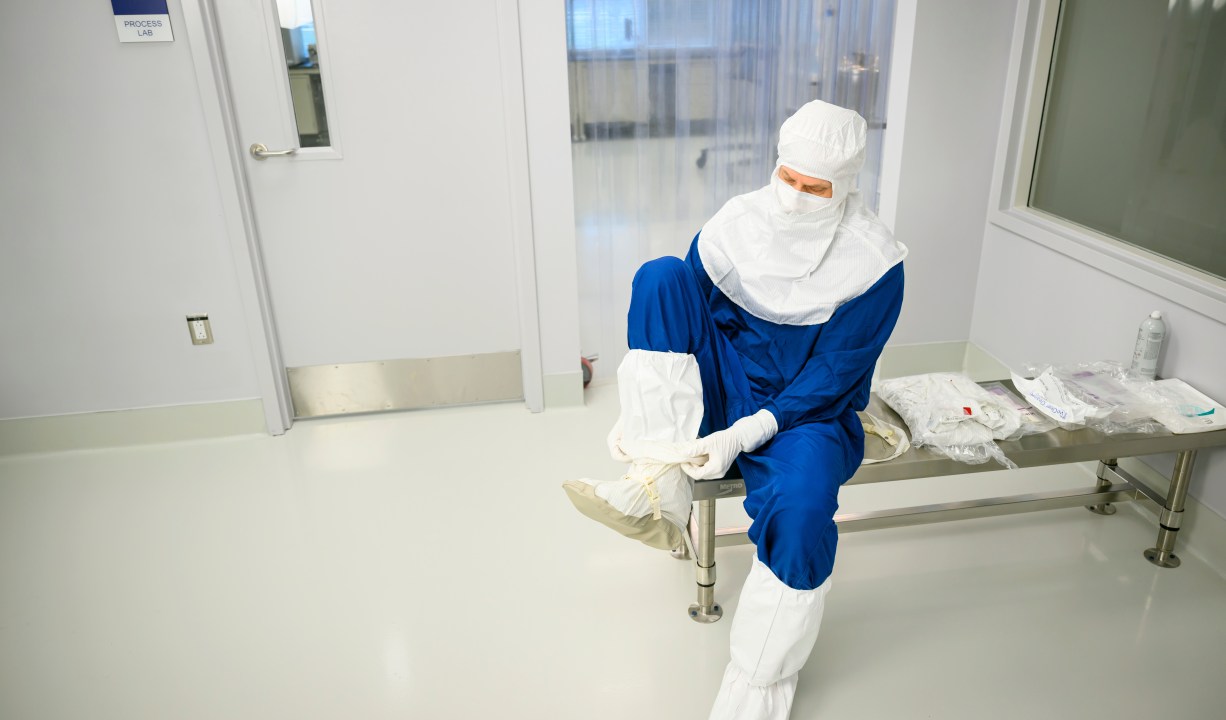
A new foundation for future talent
We’re partnering with America’s largest HBCU to launch a collaborative biotechnology learning center
Next: A new foundation for future talent
Safety and quality go hand in hand
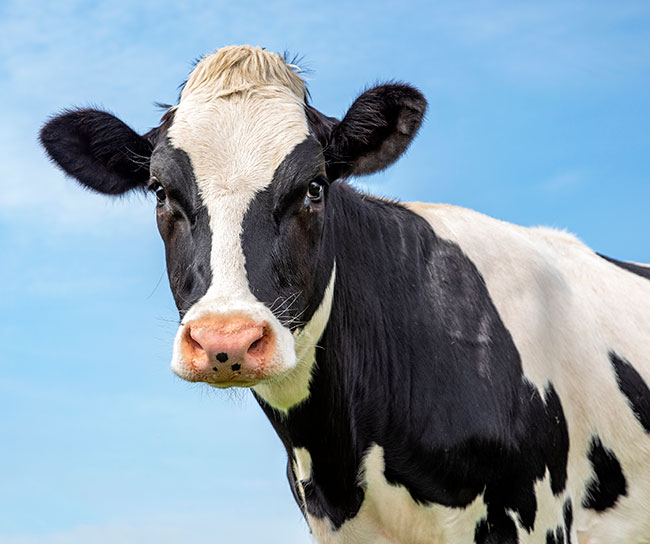
Protecting animal health
Our Animal Health business is dedicated to preserving and improving the health, well-being and performance of animals and the people who care for them.
Related links
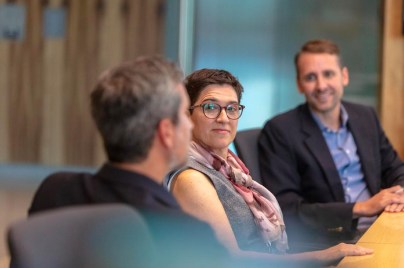
Business development & licensing
We work with many partners, from early-stage science to clinical-stage programs, to deliver life-changing therapies.
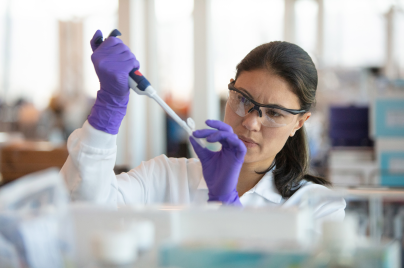
Find our latest financials, events & presentations, news, stock information, and contacts
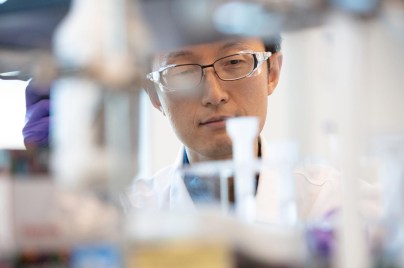
We aspire to be the premier research-intensive biopharmaceutical company.
Forward-looking statement of Merck & Co., Inc., Rahway, N.J., USA
This website of Merck & Co., Inc., Rahway, N.J., USA (the “company”) includes “forward-looking statements” within the meaning of the safe harbor provisions of the U.S. Private Securities Litigation Reform Act of 1995. These statements are based upon the current beliefs and expectations of the company’s management and are subject to significant risks and uncertainties. There can be no guarantees with respect to pipeline candidates that the candidates will receive the necessary regulatory approvals or that they will prove to be commercially successful. If underlying assumptions prove inaccurate or risks or uncertainties materialize, actual results may differ materially from those set forth in the forward-looking statements. Risks and uncertainties include but are not limited to, general industry conditions and competition; general economic factors, including interest rate and currency exchange rate fluctuations; the impact of pharmaceutical industry regulation and health care legislation in the United States and internationally; global trends toward health care cost containment; technological advances, new products and patents attained by competitors; challenges inherent in new product development, including obtaining regulatory approval; the company’s ability to accurately predict future market conditions; manufacturing difficulties or delays; financial instability of international economies and sovereign risk; dependence on the effectiveness of the company’s patents and other protections for innovative products; and the exposure to litigation, including patent litigation, and/or regulatory actions. The company undertakes no obligation to publicly update any forward-looking statement, whether as a result of new information, future events or otherwise. Additional factors that could cause results to differ materially from those described in the forward-looking statements can be found in the company’s Annual Report on Form 10-K for the year ended December 31, 2023 and the company’s other filings with the Securities and Exchange Commission (SEC) available at the SEC’s Internet site (www.sec.gov). No Duty to Update The information contained in this website was current as of the date presented. The company assumes no duty to update the information to reflect subsequent developments. Consequently, the company will not update the information contained in the website and investors should not rely upon the information as current or accurate after the presentation date.
You are leaving Merck.com
Welcome to merck.com.
By continuing, you will be directed to a site intended only for residents of the United States and Canada. We are called MSD everywhere, except in the United States and Canada where we are known as Merck & Co Inc, Rahway, NJ USA.
Research Labs
Our Health Science campus is home to an array of research laboratories. We have featured information about the major laboratory spaces on our campus here. Some additional laboratories are located at other sites on our campus and at our other clinical partners.
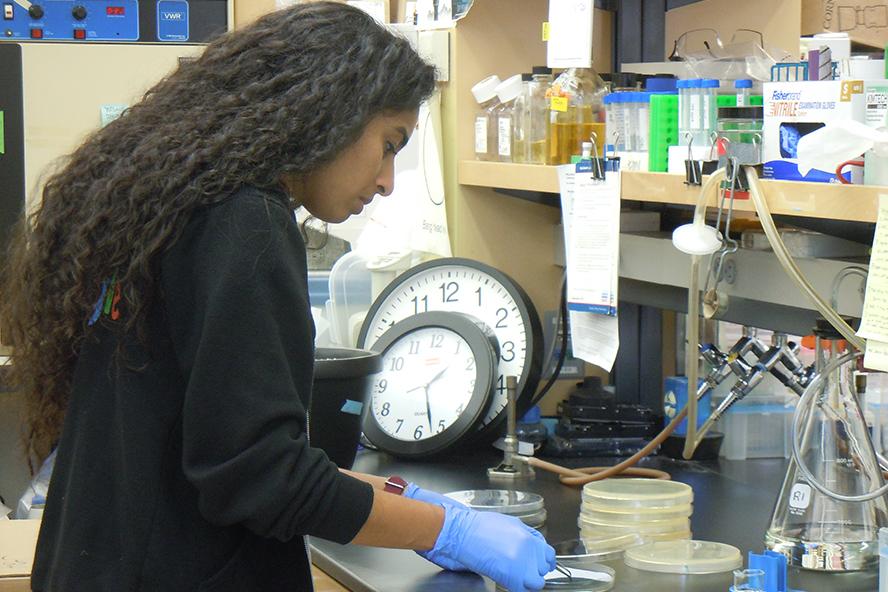
The Jaharis Family Center for Biomedical and Nutrition Research
Tufts School of Medicine researchers enjoy open, flexible, modern laboratory spaces in the 165,000 square foot Jaharis Center. Located at 150 Harrison Avenue on our Health Science Campus, this facility is home to over fifty different laboratories focused on basic and translational approaches to important disease problems.
Graduate students, medical students, postdoctoral scholars and other professional degree students come together here to advance our research mission.
The Biomedical Research and Public Health Building
Following major renovations beginning nearly a decade ago, laboratory spaces in the Biomedical Research and Public Health Building feature newly designed laboratories supporting over fifty laboratories. The 265,000 square foot complex features research laboratories as well as several important teaching facilities, bringing our students and researchers into close proximity. This facility is also home to our BSL-3 facility which supports work on tuberculosis and other pathogens.
Located at 136 Harrison Avenue on the Health Science Campus, this complex serves both basic and translational research endeavors as well as public health research and education across all our training programs. This facility is a center of activity on our campus.
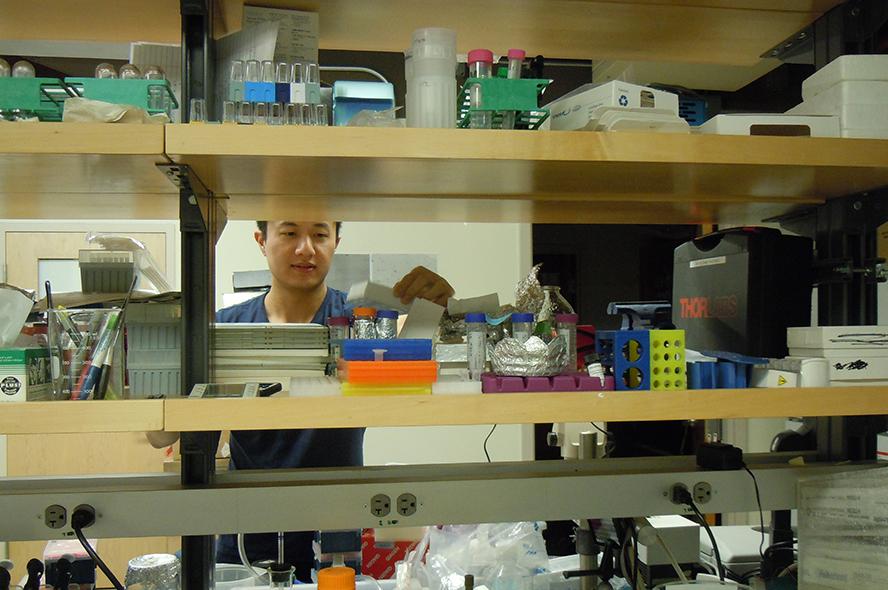
Research Laboratories at Tufts Medical Center
Tufts Medical Center, joined to Tufts School of Medicine by multiple bridges, plays an integral role in our research. Over 100,000 square feet of research space is home to the scientists and physician-scientists at this facility. These include the Tupper Building at 15 Kneeland Street.
These facilities are home to several important research institutes on our campus, including the Molecular Cardiology Research Institute and the Mother Infant Research Institute. These laboratories provide important spaces for our students to explore research.
- Awards and honors
- Graduate programs
- Events and trainings
- Facilities and resources
- Faculty Resources
- Volunteer for Research Studies
Powering the innovators and innovations that transform health.
Scientifically rich.
UW Medicine ranks among the top academic research institutions to receive National Institutes of Health (NIH) funding. The UW Medicine research powerhouse is driven by outstanding collaboration and interdisciplinary programs.
Award-Winning Faculty
UW Medicine's faculty includes many members of the National Academy of Sciences, the National Academy of Medicine and the Howard Hughes Medical Institute as well as recipients of Nobel, Gairdner, Lasker and Shaw prizes.
Diverse Graduate Programs
We are training the next wave of innovative scientists. Our internationally acclaimed graduate and postdoctoral training programs prepare students to advance science as members of an inclusive research community .
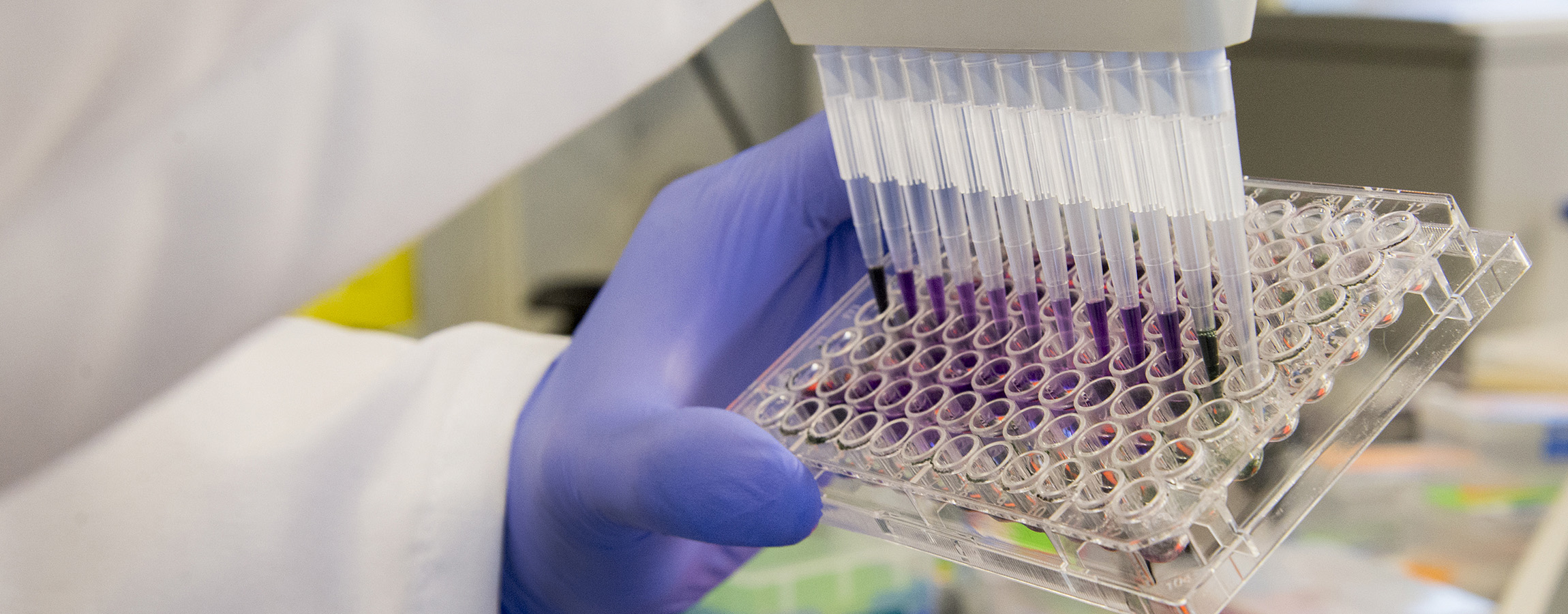
Outstanding collaborators.
By any measure, UW Medicine is leading the discovery of new health findings and the development of new approaches to health. Our team is recognized internationally for its research achievements, including No. 7 for biological sciences and No. 9 for clinical medicine in the Academic Ranking of World Universities (ARWU). In 2022, UW ranked No. 18 globally.
- MyResearch Project Lifecycle
- Scientific Resources Directory
About UW Research
- Research awards
- Facilities and Resources
- Biomedical graduate programs
Clinical Trials
You can help make history by participating in research. Working together, we can improve healthcare for years to come.
- Information for participants
Join a trial
Power the future of medicine
Medical Research
Scientists and physicians in academic medicine conduct groundbreaking biomedical research that improves our knowledge of human health and promotes the development of treatments from bench to bedside to community.
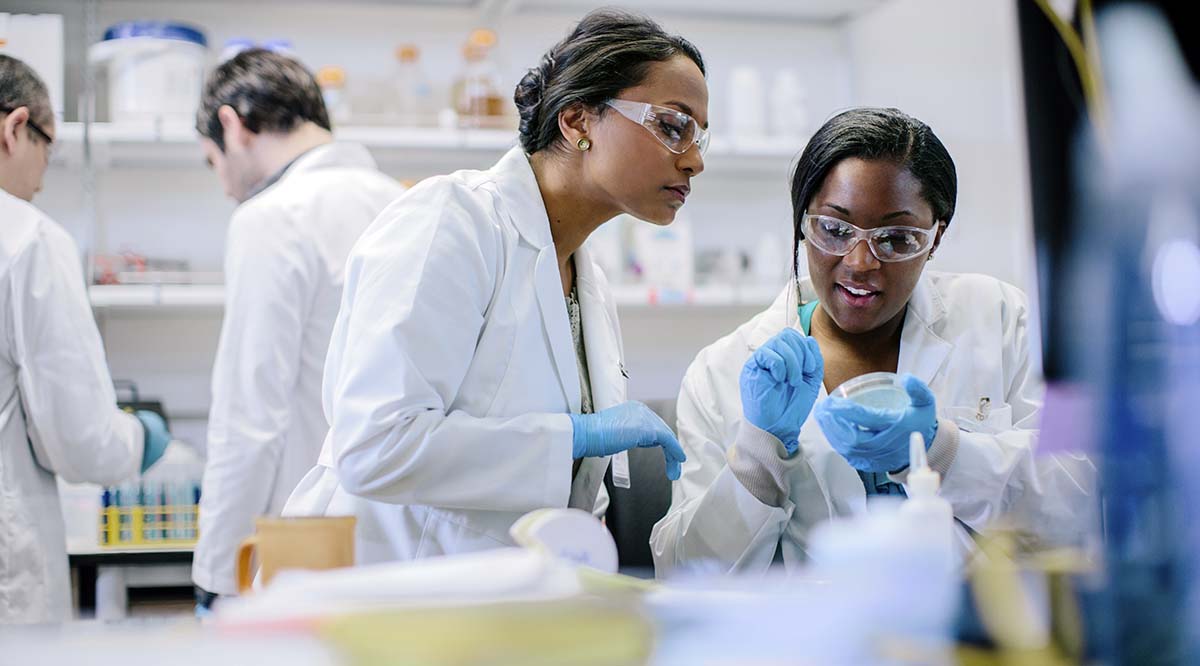
Through policy and advocacy initiatives, data and research projects, professional learning and networking opportunities, and the development of tools and resources, the AAMC supports the entire spectrum of medical research from basic discovery and translational science to clinical and population health research, research policies and regulations that promote robust and ethical science and minimize administrative burden and a diverse biomedical research workforce and an inclusive and equitable research environment across all career stages.
On this page:
Science policy updates .
AAMC updates on federal science policy and regulatory topics impacting institutions and researchers can be found below.
The AAMC sent a letter to Senate HELP Committee Ranking Member Bill Cassidy, MD, (R-La.), in response to RFI on the regulation of Laboratory Developed Tests.
- April 2, 2024
The House passed VA spending bill increases research and medical care services.
- March 8, 2024
The NIH ACD Working Group on Re-envisioning NIH-Supported Postdoctoral Training released their recommendations on Dec. 15, 2023.
- Jan. 12, 2024
For more on the latest legislative and regulatory activities affecting academic medicine, check out Washington Highlights . For the latest news, current trends, and ongoing conversations about the most important topics in academic medicine, visit AAMCNews .
Connect with Colleagues
The AAMC convenes several affinity groups focused on connecting individuals who work in biomedical research or support researchers at their institution. Affinity groups include councils, professional development groups, and other organizations that provide individuals at member institutions access to professional growth, leadership development, networking, and collaboration opportunities.
Find information about how to join each group on the pages below.
The GDI unites expertise, experience, and innovation to guide the advancement of diversity, equity, and inclusion throughout medicine and biomedical sciences.
CFAS represents the collective interests of medical school faculty and academic societies on a range of cross-cutting issues.
GRAND convenes research leaders in discussion of issues critical to the research enterprise and linking research advancements with improvements to health.
GREAT provides a forum for discussion of the research training enterprise with leadership of biomedical graduate, postdoctoral, and MD-PhD programs.
FOCI provides a national forum for leadership who oversee and manage conflict of interest related to research, medical education, and clinical decision-making.
The research subgroup of COF promotes compliance and ethical conduct in academic medicine with a specific focus on research and laboratory issues.
Attend an Event
The AAMC is committed to providing professional learning opportunities for biomedical researchers in every stage of their career. Find information about the AAMC’s webinars, meetings, and more below.
Related AAMC Initiatives
The AAMC engages in other work that may be of interest to researchers and scientists:
The Center sparks community-centered, multi-sector research, collaboration, and action to make the case for policies and practices that ensure all communities have an equal opportunity to thrive.
An optimal research environment that drives impactful biomedical discovery is supportive, diverse, equitable, and inclusive.
We are drawn to the difficult problems, the ones for which prevailing science does not yet have a framework to understand. Our strength is in developing new paradigms to tackle these problems and opening new avenues to transform human health
Psychiatry's new frontiers
The new issue of Stanford Medicine magazine reports on emerging research and innovative treatments to improve mental health

Generative AI develops potential new drugs for antibiotic-resistant bacteria

Excellence in Scientific Discovery
A commitment to scientific discovery is one of Stanford Medicine's defining strengths. In ways that cannot be anticipated, the knowledge generated by unfettered exploration yields the building blocks for tomorrow's revolutionary clinical applications.
Explore Departments: Basic science | Clinical science
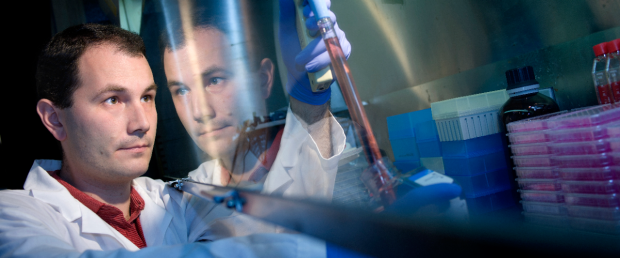
Bench-to-Bedside Research
Researchers and clinician-scientists at Stanford Medicine work across disciplines to expand the frontiers of scientific understanding while moving the most promising breakthroughs into tangible health benefits through clinical trials .
Learn about our translational research Search clinical trials Core Research Facilities

Interdisciplinary Collaboration
With access to the resources of Stanford University -- including the Schools of Engineering, Law, Business, Humanities & Sciences and Education -- Stanford Medicine enables close interactions between physicians and scientists, faculty and trainees, and basic science and clinical care.
Learn about our institutes & initiatives
Our bacteria are more personal than we thought, Stanford Medicine-led study shows

Brain organoids and assembloids are new models for elucidating, treating neurodevelopmental disorders

Stanford Medicine-led study shows why women are at greater risk of autoimmune disease

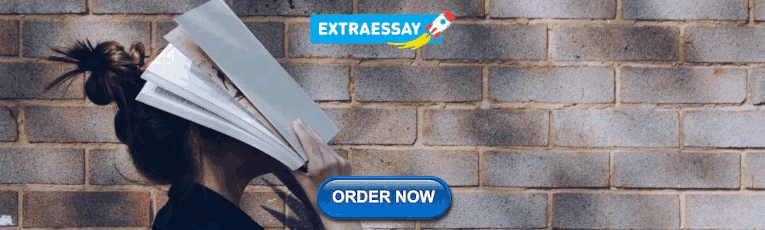
Discovery & Innovation to Improve Human Health
Faculty research.
Faculty research across the basic and clinical sciences is helping to unlock to morrow's medical breakthroughs.
Find Stanford Medicine faculty, students and staff
Research news

Risk of secondary cancers after CAR-T cell therapy low, according to large Stanford Medicine study

Stanford Medicine trial: 15-day Paxlovid regimen safe but adds no clear long-COVID benefit

Leanne Williams receives $18 million National Institutes of Health grant to diagnose and treat depression

Howard Chang awarded the 2024 Lurie Prize in Biomedical Sciences
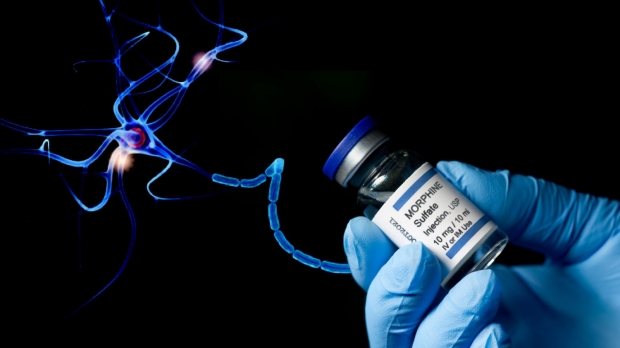
Myelination in the brain may be key to ‘learning’ opioid addiction
Explore our news topics:.
- Cardiovascular health
- Neuroscience
- Stem cells
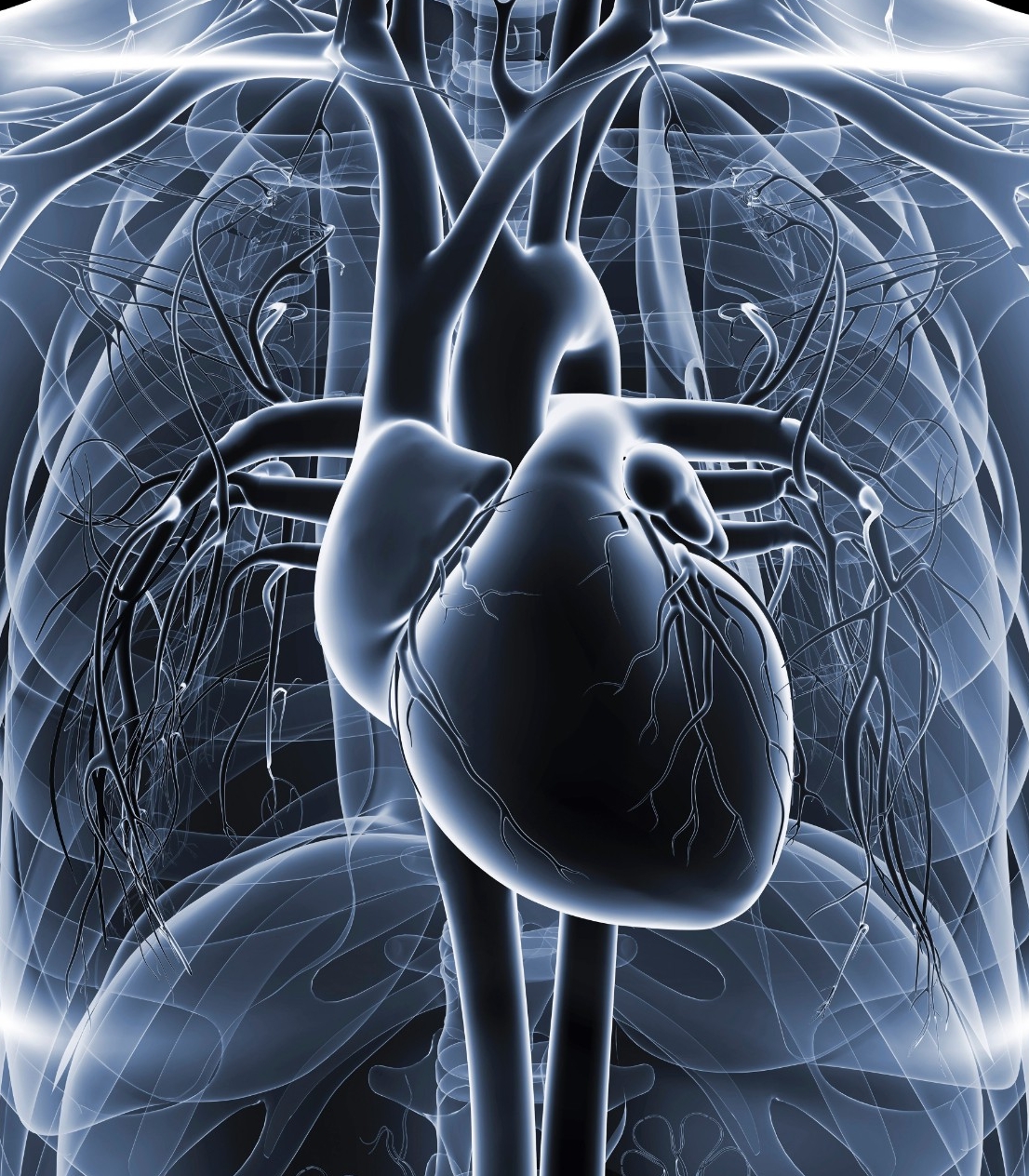
Leading in Precision Health
Stanford Medicine is leading the biomedical revolution in precision health and developing the next generation of care that is proactive, predictive and precise.
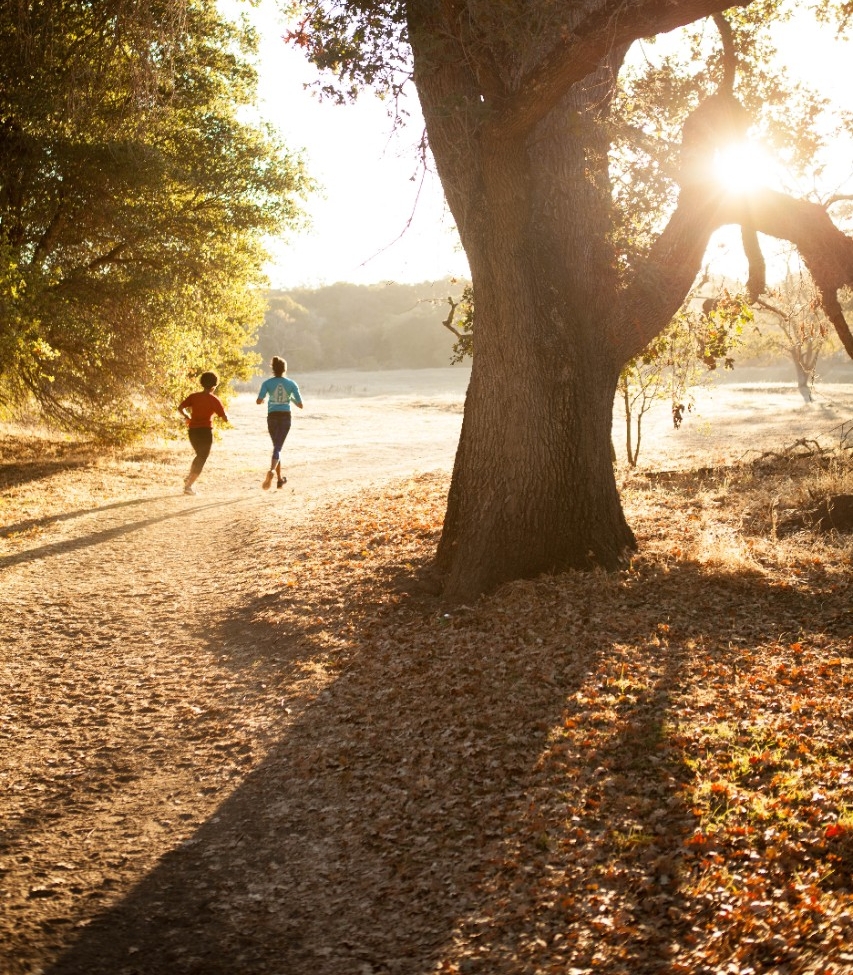
Support our Research
With your gift, you make an investment in leading-edge research that advances understanding and enhances life for generations to come.
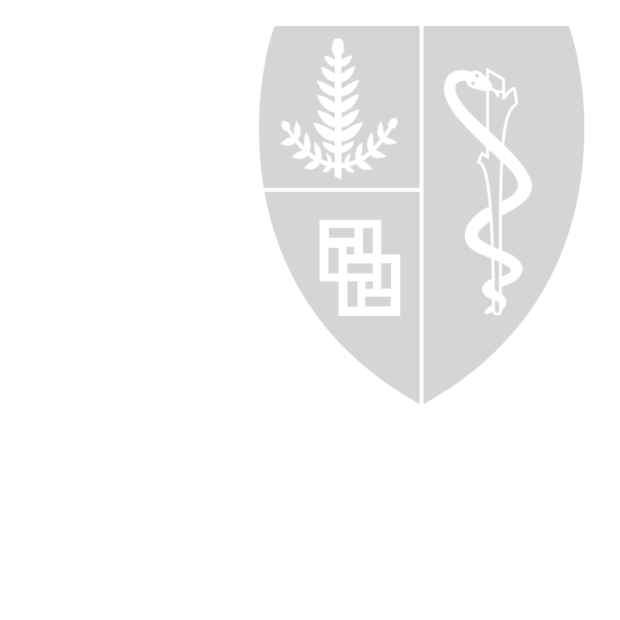
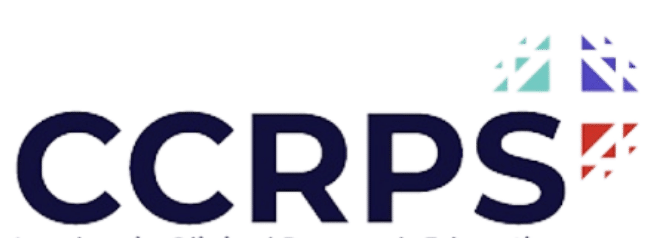
Clinical Research vs Lab Research: An In-depth Analysis
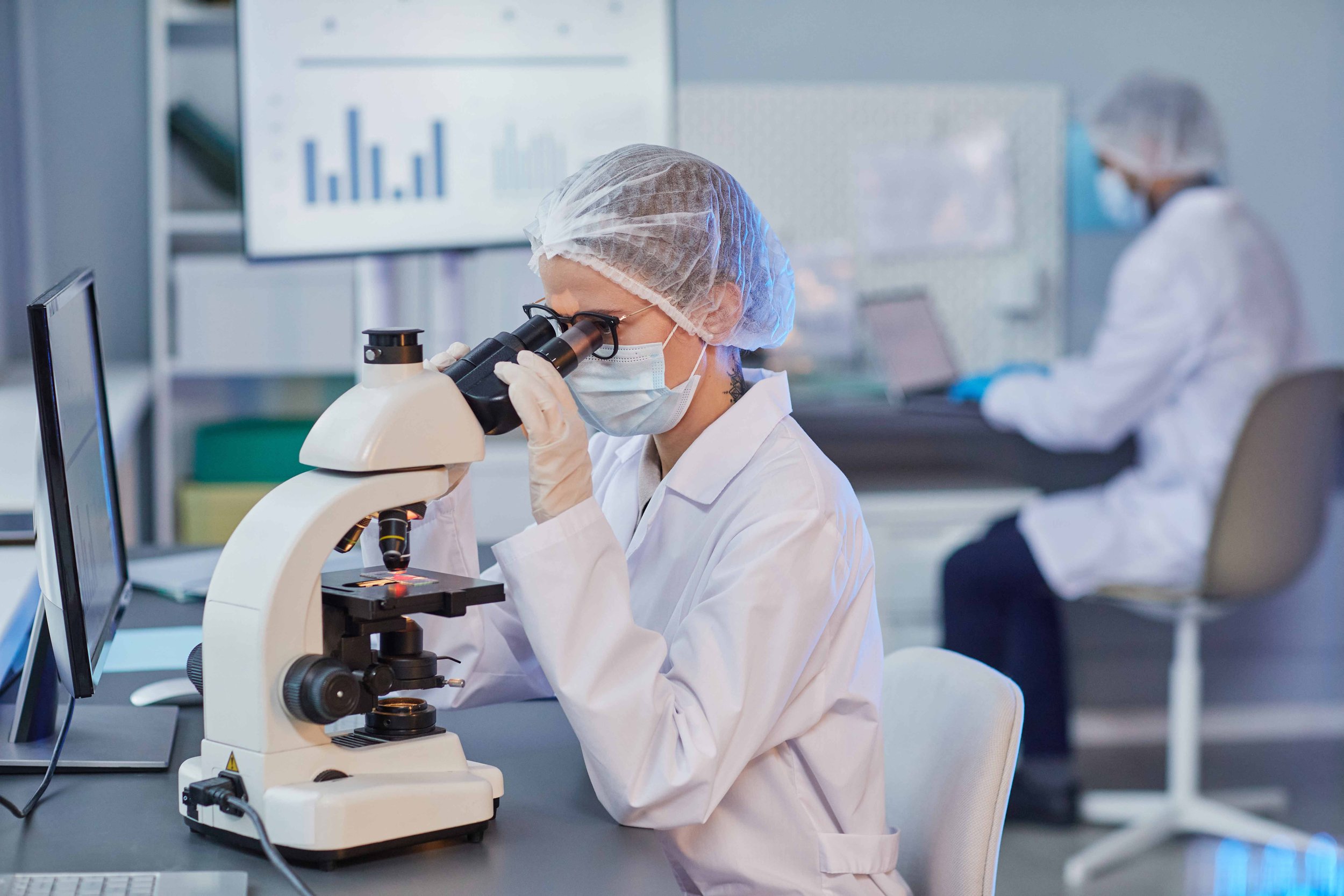
Clinical research , a cornerstone in advancing patient care, involves human subjects to test the safety and effectiveness of new treatments , ranging from drugs to diagnostic tools. Unlike clinical research , laboratory research focuses on the foundational science behind medicine without direct human involvement , contributing significantly to medical lab science.
The contrast between clinical research vs lab research highlights the diverse approaches in the scientific pursuit of better healthcare, where every medical advancement once relied on volunteer participation in clinical studies 1 . Bridging these two fields promises to accelerate the translation of lab discoveries into practical medical applications, underscoring the importance of collaboration in future developments in medical lab science 1 2 .
The Evolution of Clinical Research
The evolution of clinical research traces its origins back to ancient times, with the world's first recorded clinical tria l found in the "Book of Daniel" where a dietary intervention was observed to improve health after 10 days. This historical milestone was followed by significant advancements including Avicenna's rules for drug testing in his ‘ Canon of Medicine’ and Ambroise Pare's accidental trial in 1537 , which introduced a novel therapy for wounded soldiers. The modern era of clinical trials was marked by James Lind's controlled trial on scurvy in 1747 , laying the foundational principles for contemporary clinical research methodologies. The progression from these early experiments to the structured, ethical, and scientifically rigorous trials of today highlights the dynamic nature of clinical research. This evolution was further shaped by the introduction of the placebo in the early 1800s and the establishment of ethical frameworks , starting with the Hippocratic Oath and later formalized by the Nuremberg Code in 1947 . The development of clinical research has been instrumental in advancing medical science, with each phase of clinical trials meticulously designed to ensure the safety and efficacy of new treatments for the benefit of patient care.
Key Components of Laboratory Research
Clinical Research Facility Sciences, pivotal in the realm of medical lab science, leverage laboratory data and services extensively for disease diagnosis, monitoring, and treatment 2 4 . These sciences are underpinned by professionals who, after obtaining a Bachelor's degree in fields such as clinical research facility science or biomedical sciences from NAACLS-accredited programs, perform crucial laboratory tests, analyze specimens, and furnish healthcare providers with critical insights into the results' significance and validity 2 . Notably, these activities are conducted in laboratory settings without involving human subjects, emphasizing the distinction between clinical and laboratory research 2 .
The infrastructure of laboratories is meticulously designed to support the complex and sensitive nature of laboratory tests and analyses. This includes sturdy tables and ample counter space for heavy equipment, overhead and adjustable shelving for efficient space utilization, and cabinets and drawers for organized storage. Additionally, the deployment of fume hoods, customized for specific research needs, is essential for the safe handling of chemicals. Compliance with safety regulations and proper storage of flammable items underscore the operational standards necessary for high-quality testing and analysis in medical breakthroughs 6 .
The scientific process in laboratory research unfolds through several key steps: hypothesis formulation, experiment design, data collection, data analysis, and report writing. This structured approach begins with formulating a tentative explanation for a phenomenon, followed by planning and conducting experiments using appropriate methods and tools. The subsequent collection and analysis of data facilitate testing the hypothesis, culminating in the documentation of the entire process and findings in a formal report or paper 7 . This systematic methodology underscores the rigorous and methodical nature of laboratory research, contributing significantly to advancements in medical lab science.
Bridging the Gap: Collaboration between Clinical and Laboratory Research
Bridging the gap between clinical and laboratory research involves fostering collaborative environments that leverage the strengths of both fields to advance medical science. Medical scientific studies bifurcate into clinical laboratory scientists, who interpret critical data for healthcare professionals, and clinical researchers, who lay the groundwork for medical education and understanding 4 . This collaboration is pivotal for both building the future of medicine and administering its current benefits 4 . Enhanced operational efficiency is achieved through cross-departmental synergy, reducing redundancies in resource and personnel utilization, and fostering faster adoption of best practices and innovations across the lab 8 . These collaborations are exemplified by real-world success stories from renowned institutions like Mayo Clinic and Stanford Health Care, which have demonstrated the profound impact of integrated efforts on medical advancements 8 .
Key strategies for effective collaboration include regular meetings to address challenges, the integration of digital communication platforms with lab databases for swift sharing of results, and the establishment of clear guidelines for consistency in sample collection and result dissemination 8 . Unified objectives ensure that despite methodological differences, the end goals of improving patient care and advancing medical knowledge remain aligned 8 . Furthermore, the adoption of cloud-based data systems and AI technologies not only facilitates seamless data sharing but also automates routine tasks, thereby enhancing productivity and enabling the discovery of new insights 9 .
Challenges such as competition, ethics reviews, insufficient research funds, and the recruitment of project managers underscore the complexities of collaborative efforts 9 . However, the benefits, including improved reputation, publication quality, knowledge transfer, and acceleration of the research process, often outweigh the costs and risks associated with collaboration 9 . Collaborative relationships in Translational Medical Research (TMR) among clinicians highlight a strong willingness to collaborate, with preferences varying across different stages of research and between preferring independent and interdependent relationships 9 . This willingness to collaborate is crucial for bridging the gap between clinical and laboratory research, ultimately leading to groundbreaking advancements in medical science.
Future Trends in Clinical and Laboratory Research
The future of clinical and laboratory research is poised for transformative changes, driven by technological advancements and evolving healthcare needs. Notably:
Greater Efficiency through Automation : The integration of automation in research processes promises to streamline workflows, reducing manual labor and enhancing precision 13 .
Collaboration and Capacity Sharing : Partnerships between research institutions will facilitate shared resources and expertise, optimizing research outputs 13 .
Remote Sample Support and Diagnostic Data Interoperability : These advancements will enable more inclusive research and improved patient care by allowing data to flow seamlessly between different healthcare systems 13 .
Artificial Intelligence and Machine Learning : AI and machine learning are set to revolutionize both clinical and laboratory research by providing advanced data analysis, predictive modeling, and personalized medicine approaches 13 14 .
Staffing Solutions and Digital Workflows : Addressing staffing shortages through innovative solutions, alongside the adoption of digital workflows, will be crucial for maintaining research momentum 14 .
New Diagnostic Technologies : The development of novel diagnostic methods and technologies, including next-generation sequencing and biomarker-based screenings, will enhance disease diagnosis and treatment 14 .
Regulatory Changes and Patient-Centric Approaches : Increased FDA oversight of laboratory-developed tests and a shift towards patient-centric research models will ensure safer and more effective healthcare solutions 14 16 .
Precision Medicine and Big Data Analytics : The focus on precision medicine, supported by real-world evidence and big data analytics, will tailor treatments to individual patient needs, improving outcomes 15 .
Decentralized Clinical Trials and Digital Health Technologies : The rise of decentralized trials and digital health tools, including remote monitoring, will make research more accessible and patient-friendly 15 .
Innovation in Testing and Consumer Health : Laboratories will explore new frontiers in diagnostics, such as multi-drug-of-abuse testing and T-cell testing, while also responding to consumer health trends with at-home testing services 14 18 .
These trends underscore a dynamic shift towards more efficient, patient-centered, and technologically advanced clinical and laboratory research, setting the stage for groundbreaking discoveries and innovations in healthcare 13 14 15 16 18 .
Through this detailed exploration, we have seen the distinct yet intertwined roles that clinical and laboratory research play in the advancement of medical science and patient care. By comparing their methodologies, evolution, and collaborative potential, it becomes clear that both domains are crucial for fostering innovations that can bridge the gap between theoretical knowledge and practical healthcare solutions. The synergy between clinical and laboratory research, as highlighted by various examples and future trend predictions, establishes an essential framework for the continual improvement of medical practices and patient outcomes.
As we look toward the future, the significance of embracing technological advancements, enhancing collaboration, and adopting patient-centric approaches cannot be overstressed. These elements are pivotal in navigating the challenges and leveraging the opportunities within clinical and laboratory research landscapes. The potential impacts of such advancements on the field of medicine and on societal health as a whole are immense, underscoring the imperative for ongoing research, dialogue, and innovation in bridging the gap between the laboratory bench and the patient's bedside.
What distinguishes clinical research from laboratory research? Clinical research involves studies that include human participants, aiming to understand health and illness and answer medical questions. Laboratory research, on the other hand, takes place in environments such as chemistry or biology labs, typically at colleges or medical schools, and does not involve human subjects. Instead, it focuses on experiments conducted on non-human samples or models.
How does a clinical laboratory differ from a research laboratory? Clinical laboratories are specialized facilities where laboratory information and services are utilized to diagnose, monitor, and treat diseases. Research laboratories, in contrast, are settings where scientific investigation is conducted to study illness and health in humans to answer medical and behavioral questions.
In what ways do clinical research and scientific research differ? Clinical research is a branch of medical research that directly applies knowledge to improve patient care, often through the study of human subjects. Scientific research, including basic science research, aims to understand the mechanisms of diseases and biological processes, which may not have immediate applications in patient care.
Can you outline the various types of medical research analysis? Medical research can be categorized into three primary types based on the study's nature: basic (experimental) research, clinical research, and epidemiological research. Clinical and epidemiological research can be further divided into interventional studies, which actively involve treating or intervening in the study subjects, and noninterventional studies, which observe outcomes without intervention.
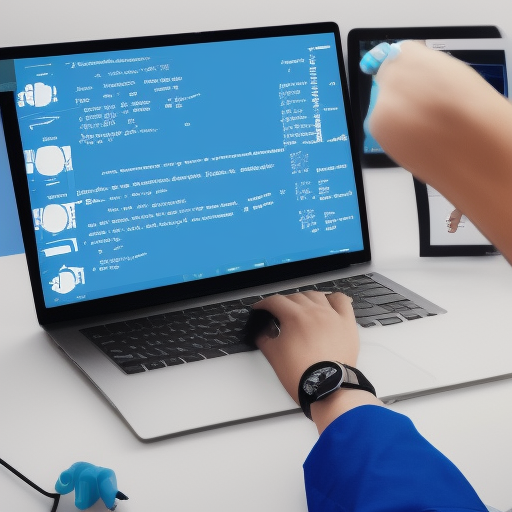
Prevent CRA Fraud: 5 Strategies to Protect Your CRO Team
Everything you need to know about clinical research studies.
- U.S. Department of Health & Human Services

- Virtual Tour
- Staff Directory
- En Español
You are here
Nih clinical research trials and you, finding a clinical trial, around the nation and worldwide.

NIH conducts clinical research trials for many diseases and conditions, including cancer , Alzheimer’s disease , allergy and infectious diseases , and neurological disorders . To search for other diseases and conditions, you can visit ClinicalTrials.gov.
ClinicalTrials.gov [ How to Use Search ] This is a searchable registry and results database of federally and privately supported clinical trials conducted in the United States and around the world. ClinicalTrials.gov gives you information about a trial's purpose, who may participate, locations, and phone numbers for more details. This information should be used in conjunction with advice from health care professionals.
Listing a study does not mean it has been evaluated by the U.S. Federal Government. Read the disclaimer on ClinicalTrials.gov for details.
Before participating in a study, talk to your health care provider and learn about the risks and potential benefits.
At the NIH Clinical Center in Bethesda, Maryland

Search NIH Clinical Research Studies The NIH maintains an online database of clinical research studies taking place at its Clinical Center, which is located on the NIH campus in Bethesda, Maryland. Studies are conducted by most of the institutes and centers across the NIH. The Clinical Center hosts a wide range of studies from rare diseases to chronic health conditions, as well as studies for healthy volunteers. Visitors can search by diagnosis, sign, symptom or other key words.
Join a National Registry of Research Volunteers
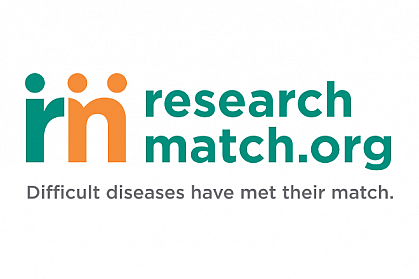
ResearchMatch This is an NIH-funded initiative to connect 1) people who are trying to find research studies, and 2) researchers seeking people to participate in their studies. It is a free, secure registry to make it easier for the public to volunteer and to become involved in clinical research studies that contribute to improved health in the future.
This page last reviewed on November 6, 2018
Connect with Us
- More Social Media from NIH
Faculty Research Labs

In Dell Medical School’s research environment — which spans the campuses of Dell Med, the Dell Pediatric Research Institute, The University of Texas at Austin and other locations in the city — faculty using similar techniques and equipment work near each other and share expertise from diverse fields.
This fosters transdisciplinary discourse and operational efficiencies that bolster the school’s research output. Researchers and lab members benefit from opportunities to gather with colleagues in related areas of expertise to connect on their work. The result of this model? A strong team, focused on transforming health.
Areas of Research
Explore a listing of research lab websites managed by Dell Med-affiliated investigators. Faculty lab sites provide details on active work, opportunities to collaborate, openings and people working within them.
Not all research faculty members have lab sites or are represented on this page. To learn more about research taking place at Dell Med, view the latest news and discoveries , or explore individual departments, centers and institutes .
- Brumback Lab | neurophysiological mechanisms of autism, electrophysiology, optogenetics
- Neural Circuits and Behavioral Development Lab | stress, neurodevelopment
- Melamed Lab | multiple sclerosis, neuroinflammation
- MS.io Lab | neuroimaging, outcome prediction, equity
- Howard Lab | cellular neurophysiology, translational epilepsy, synaptic transmission and plasticity
- COPE Lab | memory, aging, caregiver roadmap and support
- CNBI | brain-machine interfaces, translational neuroscience, neurorehabilitation
- Developmental Cognitive Neuroscience Lab | development of executive functions
- Dobbs Lab | neural circuits, animal models, polysubstance abuse
- Gaudet Lab | neuroinflammation, spinal cord injuries, biological clocks
- Gonzales-Lima lab | behavioral neuroscience
- Hamilton Lab | translational neuroscience, computational neuroscience
- Marinelli Lab | addiction, motivation
- Memory and Aging Lab | cognitive neuroscience
- Messing Lab | translational neuroscience, addiction, pain, neuroimmune signaling
- Noble-Haeusslein Lab | translational neuroscience, traumatic brain injuries, spinal cord injuries, neurorehabilitation
- Orsini Lab | behavioral neuroscience, decision-making, sex differences, substance use disorders
- Technology to Assist With Neurocognitive Symptoms (TechANS) Lab | memory, aging, technology
- CNBI | neural engineering, machine learning, artificial intelligence, closed-loop neuromodulation, human-robot interaction, BCI, imaging
- Willsey Lab | brain-computer interfaces, computational neuroscience, neuromodulation, neural engineering, machine learning
- The Physiological Laboratories | mathematical models, machine learning, physiological sensors, rehabilitation, electroceuticals
- Speech Disorders and Technology Lab | speech science
- Swallow Modulation Lab (SML) | speech production, swallowing disorders, neuromodulation
- Center for Computational Oncology | tumor model construction
- Stephen Yi Laboratory | systems biology, bioinformatics, multi-omics networks
- Gray Lab | musculoskeletal development and homeostasis
- EdEN Lab | educational gardening
- TherapeUTex | drug formulation, delivery and production
Population Health
- Burgermaster Lab | nutrition, health equity, biomedical informatics
- Khurshid Labs | data and information systems
- Harpak Lab | statistical genomics, population genetics
- Mackert Lab | traditional and new digital media, health messaging
- Texas PRIDE Health Collaborative | sexual health, mental health
- Urban Information Lab | urban informatics
Psychiatry & Behavioral Sciences
- Developmental Behavioral Genetics Lab | developmental roots of social inequality
- Beer Self-Regulation Lab | emotion and cognition
- Lifespan Development Lab | cognitive function
- Anxiety and Health Behaviors Lab | anxiety disorder treatment and intervention
- Clinical Neuroendocrinology Lab | endocrine basis of mood anxiety disorders
- Laboratory for the Study of Anxiety Disorders | nature and treatment of anxiety disorders
- Mood Disorders Lab | depression
- Main Lab | bipolar disorder, alcohol use disorder, stress
- Laboratory of Affective Neurogenetics | clinical and behavioral neuroscience, mood and effective disorders
- Pediatric Coping and Language Lab | adaptation to stress
- Women’s Reproductive Mental Health of Texas | reproductive psychiatry
- Fenno Lab | molecular engineering, systems neuroscience
- Learning, Memory and Emotion Lab | neuroimaging, cognitive neuroscience, PTSD
- LewPeaLab | cognitive neuroscience
- Morikawa Lab | addiction
- Translational and Clinical Neuroscience (TrAC Neuro) Lab | stress, neuromodulation, neuroimaging
- Neurocircuitry of Trauma and PTSD (NeuroTap) Lab | neurocircuitry, PTSD, treatment
- Osier Lab | brain trauma
Resources for Researchers
The office of research at dell med works to advance the pace and efficiency of research and innovation through a variety services..
Esta página está disponible en español .
Managing Your Health
- Shop for Health Tests
- Explore Women's Health
- Annual Wellness Guidelines
Diseases & Specialties
- Cancer & Oncology
- Rheumatology
Treatment Methods & Product Testing
- Cell & Gene Therapies
- Precision Medicine
Lab Disciplines & Services
- Genetics & Genomics
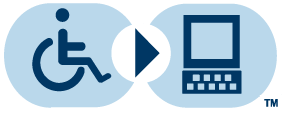
- Resource Center
Patients & Individuals
Appointments & Locations
- Search Test Menu
- New & Updated Tests
- Provider Portal Login
- Create a Provider Portal Account
Organizations
- Managed Care Portal Login
- Diseases & Conditions
- Educational Resources
View Biopharma
- Order Trial Test Kit
- Print UPS Label
- Become a Clinical Trial Site
Find Locations
Find your nearest lab location and schedule an appointment using the search below.
To make an appointment or get detailed lab information use the search below. Walk-ins are also welcome. Please note that not all lab locations offer all services.
*All fields are required, except ones marked as optional.

Purchase over 40 different health tests, on demand.
Labcorp makes managing your health more convenient by letting you purchase the same lab tests trusted by doctors, online.
Shop All Tests
- Mayo Clinic Careers
- Anesthesiology
- Dermatology
- Emergency Medicine
- Family Medicine
- Internal Medicine
- Lung Transplant
- Psychiatry & Psychology
- Nurse Practitioner & Physician Assistant
- Ambulance Service
- Clinical Labs
- Med Surg RN
- Radiology Imaging
- Clinical Research Coordinator
- Respiratory Care
- Senior Care
- Surgical Services
- Travel Surgical Tech
- Practice Operations
- Administrative Fellowship Program
- Administrative Internship Program
- Career Exploration
- Nurse Residency and Training Program
- Nursing Intern/Extern Programs
- Residencies & Fellowships (Allied Health)
- Residencies & Fellowships (Medical)
- SkillBridge Internship Program
- Training Programs & Internships
- Diversity, Equity & Inclusion
- Employees with Disabilities
You're using Internet Explorer - therefore, some pages or features may not display properly. We recommend switching to a modern browser such as Chrome, Microsoft Edge, or Firefox for a smoother experience.
Search life-changing careers..
Search by Role or Keyword
Enter Location
- United States Applicants
- United Kingdom Applicants
- United Arab Emirate Applicants
- Current Employees
RTP-RESEARCH FELLOW - The Medical Microrobots Lab
- Scottsdale, AZ
Not ready to apply? Join our talent community
The Medical Microrobots Lab at the Mayo Clinic Arizona Campus is seeking a highly motivated Postdoctoral Fellow to join our innovative team of scientists, engineers, and medical doctors.
We are passionate about tiny, soft-bodied robots and unleashing their disruptive potential, pushing the boundaries of targeted therapies and minimally invasive procedures. Our lab is uniquely positioned to develop translational microrobots, using human tissues, small and large animal disease models as primary testbeds.
This position is initially offered for 1 year. The funding is available for up to 3 years after satisfactorily meeting the academic performance expectations.
Key Responsibilities:
- Develop and test micro/milli/soft robotic medical devices
- Contribute to developing testbeds for microrobot validation using in vitro phantoms, and animal models (rats and rabbits)
- Create and refine research ideas, projects and study protocols
- Participate in weekly research meetings, journal clubs and mentoring activities
- Report to and visit our research partners located across Rochester and Jacksonville Campuses
- Collaborate with institutional bodies to ensure compliance with safety regulations and efficient use of research labs and shared spaces
- Represent our lab by presenting high-impact research at the leading international conferences.
Training and Career Development: Dr. Hakan Ceylan (PI) is committed to supporting the career development of the fellow. Individual career development plans will be tailored to include training in advanced career skills, such as
- Project management
- Grant writing
- Team leadership
- Manuscript preparation and peer review
- Networking skills toward academic and professional independence
A Research Fellow position in Life Sciences (LS) will require knowledge of either clinical-based research or laboratory-based research often obtained from a postdoctoral program. A Research Fellow at Mayo Clinic is a temporary position intended to provide training and education in research. Individuals will train in the research program of a Mayo Clinic principal investigator. Qualified individuals will demonstrate the potential for research as evidenced by their training and peer-reviewed publications and should become competitive for national research grants. Proof of English proficiency is required for J-1 Short-Term Scholars, Research Scholars, Professors, Specialists, and Student Interns sponsored by Mayo Clinic.
Excellent communication and teamwork skills.
Ph.D. in a relevant field, such as materials science, biomaterials, drug delivery
Strong background with a demonstrable track record in at least one of these skills: in vitro pharmacokinetic testing, primary cell culture, controlled drug release systems
Experience with rat, rabbit, or pig animal models is a big advantage. Otherwise, willingness to learn and perform experiments with animals.
“Can-do” attitude, a strong willingness to build up a career in soft robots, microrobots, medical device development
Demonstrated ability to conduct high-impact research and present findings at conferences.
About our location
Scottsdale, Arizona
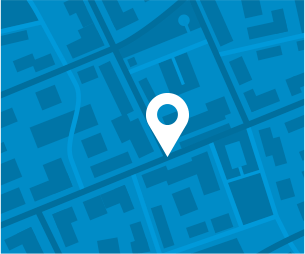
We would love to connect with you.
Click the button for a list of our upcoming events.
Join Our Talent Community
Sign up, stay connected and get opportunities that match your skills sent right to your inbox
Email Address
Phone Number
Upload Resume/CV (Must be under 1MB) Remove
Job Category* Select One Advanced Practice Providers Business Education Engineering Executive Facilities Support Global Security Housekeeping Information Technology Internship Laboratory Nursing Office Support Patient Care - Other Pharmacy Phlebotomy Physician Post Doctoral Radiology Imaging Research Scientist Surgical Services Therapy
Location Select Location Albert Lea, Minnesota Arcadia, Wisconsin Austin, Minnesota Barron, Wisconsin Bloomer, Wisconsin Caledonia, Minnesota Cannon Falls, Minnesota Chandler, Arizona Chippewa Falls, Wisconsin Decorah, Iowa Duluth, Minnesota Eau Claire, Wisconsin Fairmont, Minnesota Holmen, Wisconsin Jacksonville, Florida La Crosse, Wisconsin Lake City, Minnesota London, England Mankato, Minnesota Menomonie, Wisconsin Minneapolis-St. Paul-Bloomington, Minnesota New Prague, Minnesota Onalaska, Wisconsin Osseo, Wisconsin Owatonna, Minnesota Phoenix, Arizona Prairie du Chien, Wisconsin Red Wing, Minnesota Rice Lake, Wisconsin Rochester, Minnesota Saint Cloud, Minnesota Saint James, Minnesota Scottsdale, Arizona Sparta, Wisconsin Tomah, Wisconsin Waseca, Minnesota Zumbrota, Minnesota
Area of Interest Select One Nursing Radiology Research Laboratory Medicine & Pathology Physical Medicine & Rehabilitation Licensed Practical Nurse (LPN) Cardiovascular Medicine Facilities Surgery Neurology Psychiatry & Psychology Finance Pharmacy Emergency Medicine Respiratory Therapy Ambulance Services General Services Surgical Technician Anesthesiology & Perioperative Medicine Environmental Services Family Medicine Gastroenterology & Hepatology Radiation Oncology Social Work Orthopedics Hospital Internal Medicine Mayo Clinic Laboratories Mayo Collaborative Services Administration Medical Oncology Obstetrics & Gynecology Information Technology Cardiovascular Surgery Hematology Housekeeping Patient Scheduling International Pediatrics Senior Care Transplant Global Security Linen & Central Services Education General Internal Medicine Oncology Ophthalmology Engineering Hospice & Palliative Care Office Support Community Internal Medicine Critical Care Dermatology Desk Operations Nephrology & Hypertension Physiology & Biomedical Engineering Rheumatology Urology Artificial Intelligence & Informatics Biochemistry & Molecular Biology Clinical Genomics Endocrinology Otolaryngology (ENT) Pulmonary/Sleep Medicine Health Care Delivery Research Healthcare Technology Management Neurosciences Quality Regenerative Biotherapeutics Sports Medicine Cancer Center Development/Philanthropy Digital Epidemiology Immunology Infectious Diseases Legal Molecular Medicine Molecular Pharmacology & Experimental Therapeutics Neurologic Surgery Primary Care Surgical Assistant Business Development Clinical Trials & Biostatistics Communications Informatics Mayo Clinic Platform Media Support Services Pain Medicine Spiritual Care Allergic Diseases Bariatric Medicine Cancer Biology Center for Individualized Medicine Comparative Medicine Dental Specialities Geriatric Medicine & Gerontology Human Resources Information Security Marketing Occupational/Preventative Medicine Spine Center Strategy Travel Urgent Care Volunteer Services
- Research, Scottsdale, Arizona, United States Remove
Confirm Email
By submitting your information, you consent to receive email communication from Mayo Clinic.
Join our talent community.
Join our global talent community to receive alerts when new life-changing opportunities become available.
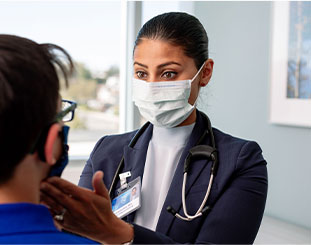
If you want to know what it's really like at Mayo Clinic, just ask. You'll find that our pride–in where we work, and in what we do–is a common trait. You will also find a lot of inspiring stories about lives changed for the better.
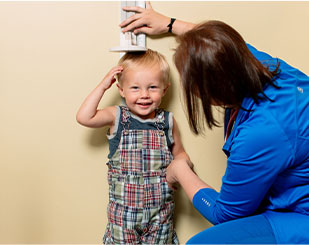
Nurse Residency Program
The Nurse Residency Program (NRP) is for all nurses with less than 12 months of experience, to be completed within the first year. NRP provides a framework for a successful transtion to a professional nurse by promoting educational and personal advancement.
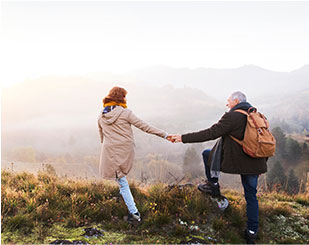
As your career evolves, our compensation and benefits packages are designed to change with you — meeting needs now, and anticipating what comes next. We know that when Mayo Clinic takes care of you, you can take better care of our patients.
Equal opportunity
All qualified applicants will receive consideration for employment without regard to race, color, religion, sex, gender identity, sexual orientation, national origin, protected veteran status, or disability status. Learn more about "EEO is the Law." Mayo Clinic participates in E-Verify and may provide the Social Security Administration and, if necessary, the Department of Homeland Security with information from each new employee's Form I-9 to confirm work authorization.
Reasonable accommodations
Mayo Clinic provides reasonable accommodations to individuals with disabilities to increase opportunities and eliminate barriers to employment. If you need a reasonable accommodation in the application process; to access job postings, to apply for a job, for a job interview, for pre-employment testing, or with the onboarding process, please contact HR Connect at 507-266-0440 or 888-266-0440.
Job offers are contingent upon successful completion of a post offer placement assessment including a urine drug screen, immunization review and tuberculin (TB) skin testing, if applicable.
Recruitment Fraud
Learn more about recruitment fraud and job scams
Advertising
Mayo Clinic is a not-for-profit organization and proceeds from Web advertising help support our mission. Mayo Clinic does not endorse any of the third party products and services advertised.
Advertising and sponsorship policy | Advertising and sponsorship opportunities
Reprint permissions
A single copy of these materials may be reprinted for noncommercial personal use only. "Mayo," "Mayo Clinic," "MayoClinic.org," "Mayo Clinic Healthy Living," and the triple-shield Mayo Clinic logo are trademarks of Mayo Foundation for Medical Education and Research.
Any use of this site constitutes your agreement to the Terms and Conditions and Privacy Policy linked below.
Terms and Conditions | Privacy Policy | Notice of Privacy Practices | Notice of Nondiscrimination
© 1998-2024 Mayo Foundation for Medical Education and Research (MFMER). All rights reserved.
COLUMBIA UNIVERSITY IN THE CITY OF NEW YORK

Clinical Research Coordinator
- Columbia University Medical Center
- Opening on: Jun 15 2024
- Job Type: Officer of Administration
- Bargaining Unit:
- Regular/Temporary: Regular
- End Date if Temporary:
- Hours Per Week: 35
- Standard Work Schedule:
- Salary Range: $70,000- $80,000
Position Summary
Reporting to the Principal Investigator of the Ultrasound Elasticity Imaging laboratory, the Clinical Research Coordinator will act as a cardiovascular sonographer and coordinator of patient-related research. As part of the sonography responsibilities, the candidate will work with our team of researchers to acquire high-quality transthoracic cardiac and vascular images in human subjects and large animal studies. Responsibilities also include participation in clinical study monitoring, data maintenance, and recruitment and consenting of subjects. The ideal candidate will possess excellent scanning abilities, strong anatomical knowledge. Organization, time management, and the ability to prioritize the collection of sonographic data for a variety of research projects is required, as well as strong interpersonal skills to recruit and consent research subjects. The candidate will have diverse responsibilities in the laboratory imaging and electronic health record (EHR) human subjects data collection, data organization, quality assessment and reporting, computer-based database management (Epic, Excel, etc.). Additional duties will relate to operations of the laboratory including ordering and management of equipment and supplies, assist with IRB protocol submissions, website updates and assistance with preparation of publications and grant applications. The Clinical Research Coordinator will work closely with the PI, as well as with other faculty, staff members and trainees within the department.
Responsibilities
- Perform 2D and 3D sonography on research human subjects and large animals (canine/swine);
- Assist the PI in the preparation of clinical study and large animal study planning;
- HS recruitment, pre-consent, and informed consent prior to scanning;
- Safely handle study information and informed consent forms;
- Maintains and organizes imaging and EHR human subjects data collection;
- Performs quality assessment and reporting for the laboratory;
- Conducts computer-based database management (Epic, Excel, etc.);
- Assists the PI with the preparation of publications and grant applications.
- Assists with the preparation of institutional review board (IRB) forms, material transfer agreements (MTAs), data use agreements (DUA) and maintenance of study documentation;
- Works closely with PI to coordinate clinical trials associated with MRI and/or other imaging;
- Maintains and organizes study documentation and records. Compiles data and assists in consolidating /analyzing data for presentation;
- Collaborates with investigators and the research team on the development of new research projects, databases and protocols or new department /service initiatives;
- Serves as the contact person for all investigators and research coordinators within radiology and from other departments who are interested in utilizing radiology imaging data for clinical research purposes. This includes proactively addressing any potential issues, facilitating database enhancements, and overseeing/managing special requests;
- Performs additional duties related to the operations of the laboratory including ordering and management of equipment and supplies, and website updates.
- Perform other duties as assigned.
Minimum Qualifications
- Bachelor’s degree or equivalent in education, training, and experience.
Preferred Qualifications
- Graduate of an accredited diagnostic medical ultrasound program in Sonography
- Registered Diagnostic Cardiac Sonographer (RDCS) from ARDMS or
- Registered Cardiac Sonographer (RCS) from CCI
Other Requirements
- Comfort working with computer-based applications is required.
- Ability to take initiative and work independently is required.
- Experience with ultrasound and related imaging modalities
- Excellent communication, interpersonal, and organizational skills are a must.
- Working knowledge of MS Office (Word, Excel) and database is needed.
Equal Opportunity Employer / Disability / Veteran
Columbia University is committed to the hiring of qualified local residents.
Commitment to Diversity
Columbia university is dedicated to increasing diversity in its workforce, its student body, and its educational programs. achieving continued academic excellence and creating a vibrant university community require nothing less. in fulfilling its mission to advance diversity at the university, columbia seeks to hire, retain, and promote exceptionally talented individuals from diverse backgrounds. , share this job.
Thank you - we'll send an email shortly.
Other Recently Posted Jobs
Associate Director of Development
Customer service rep, coding specialist.
Refer someone to this job

- ©2022 Columbia University
- Accessibility
- Administrator Log in
Wait! Before you go, are you interested in a career at Columbia University? Sign up here!
Thank you, for sharing your information. A member of our team will reach out to you soon!

This website uses cookies as well as similar tools and technologies to understand visitors' experiences. By continuing to use this website, you consent to Columbia University's usage of cookies and similar technologies, in accordance with the Columbia University Website Cookie Notice .

An official website of the United States government
The .gov means it’s official. Federal government websites often end in .gov or .mil. Before sharing sensitive information, make sure you’re on a federal government site.
The site is secure. The https:// ensures that you are connecting to the official website and that any information you provide is encrypted and transmitted securely.
- Publications
- Account settings
Preview improvements coming to the PMC website in October 2024. Learn More or Try it out now .
- Advanced Search
- Journal List
- Clin Infect Dis

Severe Acute Respiratory Syndrome Coronavirus 2 (SARS-CoV-2)–Specific T Cells and Antibodies in Coronavirus Disease 2019 (COVID-19) Protection: A Prospective Study
Ivan a molodtsov.
Clinical City Hospital named after I. V. Davydovsky, Moscow Department of Healthcare, Moscow, Russia
Evgenii Kegeles
Genome Engineering Laboratory, Moscow Institute of Physics and Technology, Dolgoprudniy, Russia
Alexander N Mitin
National Research Center–Institute of Immunology Federal Medical-Biological Agency of Russia, Moscow, Russia
Olga Mityaeva
Oksana e musatova.
Shemyakin-Ovchinnikov Institute of Bioorganic Chemistry of the Russian Academy of Sciences, Moscow, Russia
Anna E Panova
National Medical Research Center for Phthisiopulmonology and Infectious Diseases of the Ministry of Health of the Russian Federation, Moscow, Russia
Mikhail V Pashenkov
Iuliia o peshkova.
National Medical Research Center of Hematology, Moscow, Russian Federation (Russia)
Almaqdad Alsalloum
Walaa asaad, anna s budikhina, alexander s deryabin, inna v dolzhikova.
Federal State Budget Institution “National Research Centre for Epidemiology and Microbiology named after Honorary Academician N. F. Gamaleya” of the Ministry of Health of the Russian Federation, Moscow, Russia
Ioanna N Filimonova
Alexandra n gracheva, oxana i ivanova.
A. I. Yevdokimov Moscow State University of Medicine and Dentistry, Moscow, Russia
Anastasia Kizilova
Viktoria v komogorova, anastasia komova.
Research Institute of Personalized Medicine, National Center for Personalized Medicine of Endocrine Diseases, National Medical Research Center for Endocrinology, Moscow, Russia
Natalia I Kompantseva
Ekaterina kucheryavykh.
Government of Moscow, Moscow, Russia
Denis А Lagutkin
Yakov a lomakin, alexandra v maleeva, elena v maryukhnich, afraa mohammad, vladimir v murugin, nina e murugina, anna navoikova, margarita f nikonova, leyla a ovchinnikova, yana panarina, natalia v pinegina, daria m potashnikova, elizaveta v romanova, aleena a saidova, anastasia g samoilova, yana serdyuk, naina t shakirova, nina i sharova, saveliy a sheetikov, anastasia f shemetova, liudmila v shevkova, alexander v shpektor, anna trufanova, anna v tvorogova, valeria m ukrainskaya, anatoliy s vinokurov, daria a vorobyeva, ksenia v zornikova, grigory a efimov, musa r khaitov.
Pirogov Russian National Research Medical University, Moscow, Russia
Ilya A Kofiadi
Alexey a komissarov, denis y logunov, nelli b naigovzina, yury p rubtsov, irina a vasilyeva, pavel volchkov, elena vasilieva, associated data.
In a prospective study involving 5340 individuals, humoral and cellular responses revealed magnitude-dependent protection from COVID-19. Antibodies alone significantly decreased infection rates; isolated cellular response provided an intermediate level of protection. The lowest COVID-19 incidence was in the double-positive group.
During the ongoing coronavirus disease 2019 (COVID-19) pandemic, many individuals were infected with and have cleared the virus, developing virus-specific antibodies and effector/memory T cells. An important unanswered question is what levels of T-cell and antibody responses are sufficient to protect from the infection.
In 5340 Moscow residents, we evaluated anti–severe acute respiratory syndrome coronavirus 2 (SARS-CoV-2) immunoglobulin M (IgM)/immunoglobulin G (IgG) titers and frequencies of the T cells specific to the membrane, nucleocapsid, and spike proteins of SARS-CoV-2, using interferon gamma (IFN-γ) enzyme-linked immunosorbent spot (ELISpot) assay. Additionally, we evaluated the fractions of virus-specific CD4 + and CD8 + T cells using intracellular staining of IFN-γ and interleukin 2 followed by flow cytometry. We analyzed the COVID-19 rates as a function of the assessed antibody and T-cell responses, using the Kaplan–Meier estimator method, for up to 300 days postinclusion.
We showed that T-cell and antibody responses are closely interconnected and are commonly induced concurrently. Magnitudes of both responses inversely correlated with infection probability. Individuals positive for both responses demonstrated the highest levels of protectivity against the SARS-CoV-2 infection. A comparable level of protection was found in individuals with antibody response only, whereas the T-cell response by itself granted only intermediate protection.
Conclusions
We found that the contribution of the virus-specific antibodies to protection against SARS-CoV-2 infection is more pronounced than that of the T cells. The data on the virus-specific IgG titers may be instructive for making decisions in personalized healthcare and public anti–COVID-19 policies.
Clinical Trials Registration. {"type":"clinical-trial","attrs":{"text":"NCT04898140","term_id":"NCT04898140"}} NCT04898140 .
Severe acute respiratory syndrome coronavirus 2 (SARS-CoV-2) was identified as a causative agent of a new coronavirus disease 2019 (COVID-19). Individuals who have cleared the virus or who have been vaccinated develop an adaptive immune response including virus-specific T cells and antibodies [ 1–3 ], which have been shown to protect from reinfection [ 4–8 ]. However, the antibody and T-cell response levels vary considerably from person to person and substantially decrease over time [ 9 , 10 ]. These facts raise an important question: What levels of T-cell response and immunoglobulin G (IgG) titers are sufficient to protect from the infection? The definitive answer requires a population-level study of the immune response to SARS-CoV-2 followed by the tracing of infection rates.
Here, we report on a prospective study based on evaluation of the virus-specific immunoglobulin levels and virus-specific T cells in a cohort of 5340 Moscow residents. Specifically, we evaluated the anti-SARS-CoV-2 immunoglobulin M (IgM)/IgG titers and the frequencies of the T cells specific to membrane (M), nucleocapsid (N), and spike (S) proteins of SARS-CoV-2, using interferon gamma (IFN-γ) enzyme-linked immunosorbent spot (ELISpot) assay. Furthermore, we assessed the fractions of the virus-specific IFN-γ– and interleukin 2 (IL-2)–producing CD4 + and CD8 + T cells using flow cytometry. Finally, we monitored the participants for up to 300 days and analyzed the postinclusion COVID-19 rates as a function of the antibody and T-cell response levels.
This study was approved by the Moscow City Ethics Committee and performed according to the Helsinki Declaration. All participants provided written informed consent. The study was registered at ClinicalTrials.gov (identifier: {"type":"clinical-trial","attrs":{"text":"NCT04898140","term_id":"NCT04898140"}} NCT04898140 ). Individuals enrolled in the study were Moscow residents >18 years old who voluntarily visited Moscow city clinics for routine testing for COVID-19 antibodies and agreed to participate. No specific inclusion or exclusion criteria were applied. The Moscow State COVID-19 registry was used to extract information about participants’ vaccination status and previous polymerase chain reaction (PCR)–confirmed COVID-19.
Peripheral blood was collected into two 8-mL Vacutainer Cell Preparation Tube tubes with sodium citrate (BD). Peripheral blood mononuclear cells (PBMCs) were isolated according to the manufacturer’s protocol within 2 hours after venipuncture (for details, see Supplementary Material 1 ). For serum isolation, peripheral blood was collected into S-Monovette 7.5-mL Z tubes (Sarstedt, Germany).
SARS-CoV-2–specific antibodies were evaluated using an automated CL-series chemiluminescent immunoassay analyzer with compatible reagent kits (Mindray, China). The assay detects an integrated pool of antibodies specific to full-length N protein, as well as receptor-binding-domain fragment of the S protein (see Supplementary material ). According to the manufacturer, the assay units can be converted into the World Health Organization standard binding antibody units/mL by dividing by 1.32 (for details, see Supplementary Material 1 ). Virus-neutralizing activity of plasma was analyzed with a microneutralization assay using a SARS-CoV-2 strain (hCoV-19/Russia/Moscow_PMVL-1/2020) in a 96-well plate and a 50% tissue culture infective dose of 100 as described in [ 6 ], with plasma dilutions of 10, 20, 40, 80, 160, 320, 640, and 1280 times.
Flow cytometry was performed on freshly isolated PBMCs stimulated with a mixture of SARS-CoV-2 PepTivator S, S1, S+, N, and M peptide pools (1 μg/mL each, Miltenyi Biotec, Germany). After 14–16 hours of stimulation, cells were stained with a panel of antibodies against surface markers and cytokines and then analyzed with flow cytometry ( Supplementary Figure 1 ). Data were analyzed using FlowJo software (BD Biosciences) (for details, see Supplementary Material 1 ). IFN-γ ELISpot assay was performed on freshly isolated PBMCs using the IFN-γ Single-Color ELISPOT kit (CTL). For each donor, 5 wells were tested without replicates: a negative control well without stimulation, a positive control well nonspecifically stimulated with 10 µg/mL phytohemagglutinin, and 3 experimental wells stimulated with PepTivator peptide pools covering the M, N, or S protein of SARS-CoV-2. Spots were visualized and counted using an automated spot counter CTL ImmunoSpot Analyzer and ImmunoSpot software (CTL) (for details, see Supplementary Material 1 ). It should be noted that there were no replicates in our ELISpot protocol; undoubtedly, it represents a limitation of the study. However, we believe that the large number of samples analyzed allowed us to mitigate the variability of the method.
Statistical analysis was performed with the Python3 programming language with numpy , scipy , pandas , and lifelines packages (for details, see Supplementary Material 1 ). Serology positivity thresholds were set according to the assay manufacturer’s instructions at 10 AU/mL for IgG and 1 cutoff index for IgM, respectively. For IFN-γ ELISpot and flow cytometry assays, positivity criteria were developed individually (see Supplementary Material 2 ).
Cohort Characteristics
In total, 5340 individuals from the Moscow general population were included in the study. All of them were tested for SARS-CoV-2–specific IgG/IgM titers, while virus-specific T cells in peripheral blood were estimated for 156 participants using ELISpot, for 1640 participants using flow cytometry, and for 1629 participants using both assays ( Figure 1 A A ). The difference in these numbers resulted from cases in which the peripheral blood amount was not enough to perform both T-cell assays, or from which samples were excluded from the analysis because of failed controls. Cohort recruitment lasted from October to December 2020; the age and sex distribution of the participants is presented in Figure 1 B B . Accordingly, 854 participants (17%) with previously PCR-confirmed COVID-19 infection were included, 81 (2%) COVID-19 cases were diagnosed at the time of inclusion, and 496 (10%) COVID-19 cases were registered postinclusion ( Figure 1 C C ). The postinclusion observation continued until the end of August 2021; the distribution of all COVID-19 cases in time is presented in Figure 1 D D . The cohort recruitment took place before the onset of the public vaccination program in Moscow. However, among enrolled participants, there were 175 individuals who had participated in the Sputnik V vaccine clinical trial and thus had received either vaccine or placebo.

Study overview and experimental cohort description. ( A ), Schematic study design. We tested volunteers for severe acute respiratory syndrome coronavirus 2–specific antibodies (blue circle) and virus-specific T cells using interferon-γ enzyme-linked immunosorbent spot (ELISpot) assay (pink circle) and flow cytometry with intracellular staining (green circle) (Figure was created using Biorender.com). ( B ), Age and sex distribution of volunteers included in the study. ( C ), Coronavirus disease 2019 (COVID-19) status of volunteers included in the study according to the Moscow State COVID-19 registry provided by the Moscow Department of Healthcare. ( D ), COVID-19 cases among study participants per week from April 2020 to August 2021.
Correlation Between Antibody and T-Cell Responses
At the time of inclusion, 1382 (26%) individuals were positive for SARS-CoV-2–specific IgM and 2455 (46%) for IgG ( Figure 2 A A and and2 2 B B and Supplementary Figure 2 ). By analyzing a subgroup of 854 participants with confirmed previous COVID-19, we found that IgM titers considerably decreased 60 days post–disease onset ( Figure 2 C C ), whereas IgG titers stayed relatively high and unaltered up to 270 days post–disease onset ( Figure 2 D D ). Among 180 randomly selected individuals, we detected a strong correlation between the virus-neutralizing activity (VNA) of plasma and integrated IgG titers, as well as S and N protein–specific antibodies ( Supplementary Material 3 and Supplementary Figure 3 ).

Evaluation of coronavirus disease 2019 (COVID-19)–specific antibody immunity. ( A ), Percentages of patients positive for virus-specific immunoglobulin M (IgM) and immunoglobulin G (IgG). ( B ), Venn diagram showing the number of participants positive for severe acute respiratory syndrome coronavirus 2–specific IgG (green), IgM (red), and both antibody types (orange). ( C ) and ( D ), Time dependence of the IgM and IgG levels among a subgroup of 854 nonvaccinated participants who had previous polymerase chain reaction–confirmed coronavirus disease 2019 (COVID-19). Each dot represents a single patient. Time is counted from the date of disease onset according to the Moscow State COVID-19 registry to the day of inclusion in the study. Time interval presented in each boxplot is 30 days. Abbreviation: COI, cutoff index.
We analyzed the frequencies of the T cells specific to the M, N, and S proteins of SARS-CoV-2 in peripheral blood, using the IFN-γ ELISpot assay; we also analyzed the frequencies of IL-2– and IFN-γ–producing virus-specific CD4 + and CD8 + T cells, with flow cytometry. For this purpose, we used a stimulation protocol described elsewhere [ 2 , 11 , 12 ]. Both ELISpot and flow cytometry assays showed that approximately half of the individuals analyzed had a T-cell response against SARS-CoV-2 antigens, which was consistent with the level of the specific antibodies in the cohort ( Figure 3 A A and and3 3 B B ). Overall, 1145 (64.1%) individuals had SARS-CoV-2–specific T-cell responses to at least 1 of the SARS-CoV-2 proteins (M, N, or S), including 692 (38.8%) with T-cell responses to all 3 proteins ( Figure 3 C C ). Flow cytometry revealed that 2217 (67.8%) participants had SARS-CoV-2–specific CD4 + T cells expressing IL-2, IFN-γ, or both cytokines, with 1095 (33.5%) participants having all 3 cell populations ( Figure 3 D D ). All of the metrics of T-cell immunity appeared to be relatively stable up to 270 days after disease onset ( Figure 3 E E and and3 3 F F and Supplementary Figure 4 ).

Evaluation of coronavirus disease 2019 (COVID-19)–specific T-cell immunity. Freshly isolated peripheral blood mononuclear cells (PBMCs) were stimulated with peptide pools covering severe acute respiratory syndrome coronavirus 2 (SARS-CoV-2) proteins, and cytokine responses were assessed with enzyme-linked immunosorbent spot (ELISpot) assay or flow cytometry. The percentages of patients exceeding the positivity threshold for M, N, and S proteins in the ELISpot assay ( A ) or exceeding the percentage of cells expressing both interleukin 2 (IL-2) and interferon gamma (IFN-γ), or either of these cytokines, in the flow cytometry assay ( B ) are shown. Venn diagrams showing relation in positivity between different SARS-CoV-2 proteins in the ELISpot assay ( C ) or between expression of different cytokines in response to activation with SARS-CoV-2 proteins in the flow cytometry assay ( D ). The time dependence of the spot-forming units (SFU) per 10 6 PBMC for S protein in the ELISpot assay is shown in ( E ) and that of the fraction of CD4 + T cells expressing IL-2 out of total CD4 + cells in the flow cytometry assay is shown in ( F ). Each dot represents a single participant. Time is counted from the date of disease onset according to the Moscow State COVID-19 registry to the day of inclusion in the study, and thus serology testing. Time interval presented in each boxplot is 30 days. The dashed line represents a positivity threshold for ELISpot. For flow cytometry, the positivity threshold was variable (see Supplementary Material 2 ).
We observed a strong correlation between the frequencies of SARS-CoV-2–specific T cells detected with ELISpot and those detected with flow cytometry; also, a strong correlation between IgG titers and T-cell frequencies was determined ( Supplementary Figure 5 ). This correlation was found in the cases of M, N, and S protein–specific T cells, as well as for different populations of CD4 + T cells.
Protectivity of Different Immune Responses Against SARS-CoV-2 Infection
To evaluate the effects of antibody and T-cell responses on susceptibility to SARS-CoV-2 infection, we analyzed the postinclusion COVID-19 rates as functions of the assessed parameters. To avoid possible bias, we excluded from the analysis 175 individuals who had participated in the Sputnik V clinical trial and 81 individuals who were already infected at the moment of blood collection. Vaccinated participants were withdrawn from the study on the day of vaccination. Since we have subjects who were excluded from the dataset during observation and have >2 groups in all comparisons, we employed the nonparametric Kaplan–Meier estimator method for initial exploration and the Cox proportional hazards (CPH) model for further quantitative assessment of observed effects. Accordingly, among the 3989 participants who were eligible for the postinclusion observation, 420 postinclusion COVID-19 cases were registered. For each of the immune parameters, participants were divided by the quantiles depending on the levels of their responses, and corresponding Kaplan-Meier curves for each quantile were analyzed and CPH models were built ( Supplementary Material 4 ).
For all the immune response metrics, we found an inverse correlation with the SARS-CoV-2 infection rates. Thus, at the end of the observation individuals with IgG titers <0.29 AU/mL (quantile [Q] 1) were characterized by a 22% chance of becoming infected ( Figure 4 A A ). For individuals in Q2 and Q3 (IgG titers 0.29–0.97 and 0.97–8.23 AU/mL, respectively), age-adjusted log-hazard ratios (HRs) compared with Q1 were significantly below zero: −0.3 (95% confidence interval [CI], −.5 to −.03) and −0.33 (95% CI, −.6 to −.1), respectively. Individuals representing Q4 and Q5 (IgG titers: 8.23–66.5 AU/mL and >66.5 AU/mL, respectively) had the lowest infection chances: log(HRs), −1.5 (95% CI, −1.9 to −1.2) and −2.4 (95% CI, −3 to −2), respectively. We found that Q4 and Q5, which demonstrated the highest protection, were at the same time characterized by the highest VNA ( Supplementary Figure 3 B ). Surprisingly, Q3, with infection chances in the intermediate range, also had VNA significantly higher than Q1, demonstrating an absence of protectivity. There was no difference in VNA between Q1 and Q2.

Evaluation of the effects of antibody and T-cell immune responses on coronavirus disease 2019 (COVID-19) infection rates. The patients were split into 5 nearly equal groups by quantiles of immunoglobulin G (IgG) levels ( A , top) or by S protein–specific spot-forming units estimated from enzyme-linked immunosorbent spot (ELISpot) assay ( B , top) from quartile (Q) 1 to Q5. Additionally, participants were split into 4 groups ( C , top): positive only by antibodies (A + T − ), positive only by S protein–specific T cells estimated from ELISpot (A − T + ), double-positive (A + T + ), and double-negative (A − T − ). Corresponding Kaplan–Meier curves were generated for each group, and COVID-19 rates were analyzed. A – C (bottom), Age-adjusted Cox proportional hazard models were fitted (with age measured in decades for ease of representation) and hazard ratios in comparison with either Q1 or the A − T − group were calculated together with the model concordance index ( c -index). ✢, decades were used as units for age measurements. * p < 0.05; ** p < 0.01; *** p < 0.001; **** p < 0.0001. Abbreviations: CI, confidence interval; HR, hazard ratio; IgG, immunoglobulin G; PBMC, peripheral blood mononuclear cells; Q, quartile; SFU, spot-forming units.
An almost binary relationship was observed between infection chances and the frequencies of virus-specific T cells identified by ELISpot ( Figure 4 B B and Supplementary Figure 6 A–C ). For all of the SARS-CoV-2 proteins analyzed, individuals in Q4 and Q5 were characterized by the highest levels of protection against the infection, whereas Q1–Q3 were similar and demonstrated no considerable protection. For example, the maximal protection was achieved when the number of S protein–specific spot-forming units per 10 6 PBMCs exceeded 67. In contrast to ELISpot, the results of the T-cell response analysis using flow cytometry revealed a gradual relationship between the frequencies of T cells producing IFN-γ, IL-2, or both cytokines, and infection chances ( Supplementary Figure 6 D–H ). However, when CD4 + T cells expressing different cytokines were combined, the relationship with infection chances transformed into a binary one.
A similar strategy was employed to separate the effects of cellular and antibody responses on protection against SARS-CoV-2 infection. The participants were split into 4 groups: positive only by antibody response (A + T − ), positive only by any metric of the T-cell response (A − T + ), double-positive (A + T + ), and double-negative (A – T – ), according to the previously estimated positivity criteria ( Supplementary Material 2 ). Such analysis was performed for all metrics of the T-cell response, except for CD8 + T cells since it was impossible to develop a reliable positivity criterion. The group size depended on the T-cell immune response metric used; for example, for S protein–specific T cells ( Figure 4 C C ), the numbers of participants at the start of observation were 446, 113, 147, and 609 for A + T + , A + T − , A − T + , and A − T − , respectively. For all of the T-cell response metrics, the A − T − group had the highest infection chances, while the strongest protection was observed for A + T + and A + T − groups, the latter two groups being statistically indistinguishable ( Figure 4 C C and Supplementary Figure 7 ). For example, for S protein–specific T cells, age-adjusted log-HRs for all other groups compared with the A − T − group were significantly above zero: for A − T + : log(HR), −0.7 (95% CI, −1.3 to −.1); for A + T − : log(HR), −1.8 (95% CI, −2.8 to −.8); and for A + T + : log(HR), −2.6 (95% CI, −3.3 to −1.9). For all studied metrics, the A − T + group demonstrated an intermediate protection that was significantly higher than in the A − T − group, but was lower than in the A + T + and A + T − groups. In particular, the protection provided by the T cells in the absence of antibodies was observed when the response was estimated from the numbers of N and S protein–specific T cells with ELISpot ( Figure 4 C C and Supplementary Figure 7 A–C ). The trend for increased protection was observed for the CD4 + T cells producing IFN-γ, IL-2, both cytokines, and, especially, these populations combined ( Supplementary Figure 7 D–G ). It is noteworthy that individuals single-positive for N- and S protein–specific T cells, as well as for virus-specific CD4 + T cells, were characterized by higher IgG levels than individuals of the A – T – group, although the antibody levels were below the positivity cutoff value of 10 AU/mL ( Supplementary Figure 8 ).
With the progression of the COVID-19 epidemic, a growing number of individuals develop immune responses against SARS-CoV-2. Prospective studies in humans [ 13–15 ] and studies using primate models with SARS-CoV-2 rechallenge [ 16–18 ] have demonstrated that an acquired post–COVID-19 immune response provides protection from reinfection. The goal of our study was to evaluate what metrics of the antibody and T-cell immune responses against SARS-CoV-2 correlate with protection against infection in humans in the context of the COVID-19 epidemic in Moscow between October 2020 and August 2021.
As expected, we found a strong correlation between frequencies of SARS-CoV-2–specific T cells evaluated with ELISpot and with flow cytometry, since these methods detect cytokine expression in activated T cells [ 19 ]. IgG titers strongly correlated with the frequencies of SARS-CoV-2–specific T cells, confirming that antibody and cellular responses are closely interconnected and induced concurrently. This correlation existed even at IgG values below the seropositivity cutoff.
From April 2021 in Russia, the B.1.1 lineage of SARS-CoV-2 predominated [ 20 , 21 ], while from April to August the vast majority of SARS-CoV-2 variants detected belonged to the B.1.617 (Delta and derivatives) lineage [ 22 ]. However, we found that IgG titers and parameters of the T-cell response negatively correlated with infection probabilities regardless of the predominant virus variant. T-cell response was characterized by a binary relationship between response level and infection probabilities, as measured with ELISpot. This means that for all individuals with a frequency of SARS-CoV-2–specific T cells surpassing a particular threshold, protection against SARS-CoV-2 infection was the same. A different pattern was observed for IgG titers. We identified 3 groups of individuals characterized by different infection chances. Individuals with very low IgG titers were characterized by the highest infection chances, while high titers were associated with the lowest infection chances. Meanwhile, infection chances for individuals with intermediate IgG titers were also intermediate, notwithstanding the fact that these titers were below the seropositivity cutoff. Moreover, we found significant VNA among these individuals. Given the strong correlation between antibody and T-cell responses found in the study, the protection observed in these individuals might be T-cell dependent. We surmise that this group may consist of individuals who developed a COVID-19–specific response after previous asymptomatic infection [ 23 , 24 ], or after infection with either cross-reactive “common cold” coronaviruses [ 11 ] or other pathogens [ 25 , 26 ]. The low-level humoral response could nevertheless be indicative of successful formation of memory B cells, as it is known that SARS-CoV-2 induces the formation of durable B-cell memory [ 27–29 ].
Depending on estimated T-cell and antibody responses, we split the participants into 4 groups and analyzed the protection against the SARS-CoV-2 infection. Two groups were characterized by the highest protection: individuals positive for both types of responses and those with antibody response only. Apparently, these groups contain individuals with previous COVID-19 that had not been confirmed by PCR for some reasons. It is noteworthy that even though the reinfection rates in these groups were very small, few cases were still detected. Individuals with T-cell response alone demonstrated intermediate protection levels that nevertheless were higher than levels in individuals without either type of immunity. Statistically significant protection was observed for N and S protein–specific T-cell responses. Individuals single-positive for these cellular response metrics had higher IgG titers than individuals without either type of immunity, although the titers were below the positivity cutoff. Taken together, our results demonstrated that antibodies better correlated with protection against the SARS-CoV-2 infection, indicating that IgG evaluation is a more precise method for prediction of infection chances than virus-specific T cells. However, the most important role of T cells might be not in protection from the infection but rather in viral clearance and managing disease severity [ 30–35 ]. Moreover, rhesus macaque models [ 17 , 36 ] and recent human studies [ 37 , 38 ] have supported that T-cell protection becomes important as neutralizing antibodies decline.
Our study has several limitations. The cohort analyzed is likely to be nonrepresentative and includes only individuals who have visited outpatient clinics for COVID-19 antibody tests and who agreed to participate in the study. Some cases of COVID-19 infections, especially asymptomatic, were inevitably missed as they were not reported to the Moscow State COVID-19 registry, though we do not expect any nonrandom distribution of unreported cases between different groups. Additionally, our study was focused on the systemic immune responses detected in peripheral blood, while local concentrations of antibodies and tissue-resident T cells in the mucosa and respiratory system may differ from blood levels; this issue deserves thorough investigation.
In summary, our data suggest that serological testing is advantageous for the prediction of protection against SARS-CoV-2 infection. Our data on the specific IgG titers may be instructive for making decisions in personalized healthcare and for development of public anti–COVID-19 policies.
Supplementary Data
Supplementary materials are available at Clinical Infectious Diseases online. Consisting of data provided by the authors to benefit the reader, the posted materials are not copyedited and are the sole responsibility of the authors, so questions or comments should be addressed to the corresponding author.
Author contributions. G. A. E., I. A. K., A. A. K., M. R. K., D. Y. L., N. B. N., Y. P. R., A. V. S., I. A. V., P. V., and E. V. designed and coordinated the study. I. A. M. performed the statistical analysis and, together with E. K., A. N. M., O. M., O. E. M., A. E. P., M. V. P., and I. O. P., performed initial data interpretation. A. A., W. A., A. S. B., A. S. D., I. V. D., I. N. F., A. N. G., O. I. I., A. K., V. V. K., A. K., N. I. K., D. A. L., Y. A. L., A. V. M., E. V. M., A. M., V. V. M., N. E. M., A. N., M. F. N., L. A. O., N. V. P., D. M. P., E. V. R., A. A. S., N. S., A. G. S., Y. S., N. T. S., N. I. S., S. A. S., A. F. S., L. S., A. T., A. V. T., V. M. U., A. S. V., D. A. V., and K. V. Z. performed the experiments. E. K. and Y. P. provided the information from the Moscow State COVID-19 registry. Every author contributed to the initial draft of the manuscript and agreed on submission for publication. All authors reviewed the manuscript and approved the final version.
Acknowledgments. The authors thank Dr Leonid Margolis (Eunice Kennedy Shriver National Institute of Child Health and Human Development, National Institutes of Health, Bethesda, Maryland) and Professor Michael M. Lederman (Case Western Reserve University/University Hospitals Cleveland Medical Center, Cleveland, Ohio) for their valuable comments and suggestions during experimental design, discussion of the results, and manuscript preparation; and Dr Barry Alpher for assistance in editing and improving the English style of the manuscript. The authors also thank the Moscow Department of Healthcare for help in organization of the study.
Financial support. This study was supported by Moscow Department of Healthcare (DC number DZM-30-3, payments made to institution). T-cell response analysis using ELISpot was supported in part by the Ministry of Science and Higher Education of the Russian Federation (agreement number 075-15-2020-899, payments made to institution). T-cell response analysis using flow cytometry was supported in part by an A. I. Yevdokimov Moscow State University of Medicine and Dentistry grant (agreement number KNP-06/21, purchase and supply of study materials).
Supplementary Material
Ciac278_supplementary_data, contributor information.
Ivan A Molodtsov, Clinical City Hospital named after I. V. Davydovsky, Moscow Department of Healthcare, Moscow, Russia.
Evgenii Kegeles, Genome Engineering Laboratory, Moscow Institute of Physics and Technology, Dolgoprudniy, Russia.
Alexander N Mitin, National Research Center–Institute of Immunology Federal Medical-Biological Agency of Russia, Moscow, Russia.
Olga Mityaeva, Genome Engineering Laboratory, Moscow Institute of Physics and Technology, Dolgoprudniy, Russia.
Oksana E Musatova, Shemyakin-Ovchinnikov Institute of Bioorganic Chemistry of the Russian Academy of Sciences, Moscow, Russia.
Anna E Panova, National Medical Research Center for Phthisiopulmonology and Infectious Diseases of the Ministry of Health of the Russian Federation, Moscow, Russia.
Mikhail V Pashenkov, National Research Center–Institute of Immunology Federal Medical-Biological Agency of Russia, Moscow, Russia.
Iuliia O Peshkova, National Medical Research Center of Hematology, Moscow, Russian Federation (Russia)
Almaqdad Alsalloum, Genome Engineering Laboratory, Moscow Institute of Physics and Technology, Dolgoprudniy, Russia.
Walaa Asaad, Genome Engineering Laboratory, Moscow Institute of Physics and Technology, Dolgoprudniy, Russia.
Anna S Budikhina, National Research Center–Institute of Immunology Federal Medical-Biological Agency of Russia, Moscow, Russia.
Alexander S Deryabin, Shemyakin-Ovchinnikov Institute of Bioorganic Chemistry of the Russian Academy of Sciences, Moscow, Russia.
Inna V Dolzhikova, Federal State Budget Institution “National Research Centre for Epidemiology and Microbiology named after Honorary Academician N. F. Gamaleya” of the Ministry of Health of the Russian Federation, Moscow, Russia.
Ioanna N Filimonova, Shemyakin-Ovchinnikov Institute of Bioorganic Chemistry of the Russian Academy of Sciences, Moscow, Russia.
Alexandra N Gracheva, National Medical Research Center for Phthisiopulmonology and Infectious Diseases of the Ministry of Health of the Russian Federation, Moscow, Russia.
Oxana I Ivanova, Clinical City Hospital named after I. V. Davydovsky, Moscow Department of Healthcare, Moscow, Russia. A. I. Yevdokimov Moscow State University of Medicine and Dentistry, Moscow, Russia.
Anastasia Kizilova, Genome Engineering Laboratory, Moscow Institute of Physics and Technology, Dolgoprudniy, Russia.
Viktoria V Komogorova, National Research Center–Institute of Immunology Federal Medical-Biological Agency of Russia, Moscow, Russia.
Anastasia Komova, Genome Engineering Laboratory, Moscow Institute of Physics and Technology, Dolgoprudniy, Russia. Research Institute of Personalized Medicine, National Center for Personalized Medicine of Endocrine Diseases, National Medical Research Center for Endocrinology, Moscow, Russia.
Natalia I Kompantseva, National Medical Research Center for Phthisiopulmonology and Infectious Diseases of the Ministry of Health of the Russian Federation, Moscow, Russia.
Ekaterina Kucheryavykh, Government of Moscow, Moscow, Russia.
Denis А Lagutkin, National Medical Research Center for Phthisiopulmonology and Infectious Diseases of the Ministry of Health of the Russian Federation, Moscow, Russia.
Yakov A Lomakin, Shemyakin-Ovchinnikov Institute of Bioorganic Chemistry of the Russian Academy of Sciences, Moscow, Russia.
Alexandra V Maleeva, National Medical Research Center of Hematology, Moscow, Russian Federation (Russia)
Elena V Maryukhnich, Clinical City Hospital named after I. V. Davydovsky, Moscow Department of Healthcare, Moscow, Russia. A. I. Yevdokimov Moscow State University of Medicine and Dentistry, Moscow, Russia.
Afraa Mohammad, Genome Engineering Laboratory, Moscow Institute of Physics and Technology, Dolgoprudniy, Russia.
Vladimir V Murugin, National Research Center–Institute of Immunology Federal Medical-Biological Agency of Russia, Moscow, Russia.
Nina E Murugina, National Research Center–Institute of Immunology Federal Medical-Biological Agency of Russia, Moscow, Russia.
Anna Navoikova, Genome Engineering Laboratory, Moscow Institute of Physics and Technology, Dolgoprudniy, Russia.
Margarita F Nikonova, National Research Center–Institute of Immunology Federal Medical-Biological Agency of Russia, Moscow, Russia.
Leyla A Ovchinnikova, Shemyakin-Ovchinnikov Institute of Bioorganic Chemistry of the Russian Academy of Sciences, Moscow, Russia.
Yana Panarina, Government of Moscow, Moscow, Russia.
Natalia V Pinegina, Clinical City Hospital named after I. V. Davydovsky, Moscow Department of Healthcare, Moscow, Russia. A. I. Yevdokimov Moscow State University of Medicine and Dentistry, Moscow, Russia.
Daria M Potashnikova, Clinical City Hospital named after I. V. Davydovsky, Moscow Department of Healthcare, Moscow, Russia. A. I. Yevdokimov Moscow State University of Medicine and Dentistry, Moscow, Russia.
Elizaveta V Romanova, Clinical City Hospital named after I. V. Davydovsky, Moscow Department of Healthcare, Moscow, Russia.
Aleena A Saidova, Clinical City Hospital named after I. V. Davydovsky, Moscow Department of Healthcare, Moscow, Russia.
Nawar Sakr, Genome Engineering Laboratory, Moscow Institute of Physics and Technology, Dolgoprudniy, Russia.
Anastasia G Samoilova, National Medical Research Center for Phthisiopulmonology and Infectious Diseases of the Ministry of Health of the Russian Federation, Moscow, Russia.
Yana Serdyuk, National Medical Research Center of Hematology, Moscow, Russian Federation (Russia)
Naina T Shakirova, National Medical Research Center of Hematology, Moscow, Russian Federation (Russia)
Nina I Sharova, National Research Center–Institute of Immunology Federal Medical-Biological Agency of Russia, Moscow, Russia.
Saveliy A Sheetikov, National Medical Research Center of Hematology, Moscow, Russian Federation (Russia)
Anastasia F Shemetova, National Medical Research Center for Phthisiopulmonology and Infectious Diseases of the Ministry of Health of the Russian Federation, Moscow, Russia.
Liudmila V Shevkova, Genome Engineering Laboratory, Moscow Institute of Physics and Technology, Dolgoprudniy, Russia. Research Institute of Personalized Medicine, National Center for Personalized Medicine of Endocrine Diseases, National Medical Research Center for Endocrinology, Moscow, Russia.
Alexander V Shpektor, Clinical City Hospital named after I. V. Davydovsky, Moscow Department of Healthcare, Moscow, Russia. A. I. Yevdokimov Moscow State University of Medicine and Dentistry, Moscow, Russia.
Anna Trufanova, Genome Engineering Laboratory, Moscow Institute of Physics and Technology, Dolgoprudniy, Russia.
Anna V Tvorogova, Clinical City Hospital named after I. V. Davydovsky, Moscow Department of Healthcare, Moscow, Russia.
Valeria M Ukrainskaya, Shemyakin-Ovchinnikov Institute of Bioorganic Chemistry of the Russian Academy of Sciences, Moscow, Russia.
Anatoliy S Vinokurov, National Medical Research Center for Phthisiopulmonology and Infectious Diseases of the Ministry of Health of the Russian Federation, Moscow, Russia.
Daria A Vorobyeva, Clinical City Hospital named after I. V. Davydovsky, Moscow Department of Healthcare, Moscow, Russia. A. I. Yevdokimov Moscow State University of Medicine and Dentistry, Moscow, Russia.
Ksenia V Zornikova, National Medical Research Center of Hematology, Moscow, Russian Federation (Russia)
Grigory A Efimov, National Medical Research Center of Hematology, Moscow, Russian Federation (Russia)
Musa R Khaitov, National Research Center–Institute of Immunology Federal Medical-Biological Agency of Russia, Moscow, Russia. Pirogov Russian National Research Medical University, Moscow, Russia.
Ilya A Kofiadi, National Research Center–Institute of Immunology Federal Medical-Biological Agency of Russia, Moscow, Russia. Pirogov Russian National Research Medical University, Moscow, Russia.
Alexey A Komissarov, Clinical City Hospital named after I. V. Davydovsky, Moscow Department of Healthcare, Moscow, Russia. A. I. Yevdokimov Moscow State University of Medicine and Dentistry, Moscow, Russia.
Denis Y Logunov, Federal State Budget Institution “National Research Centre for Epidemiology and Microbiology named after Honorary Academician N. F. Gamaleya” of the Ministry of Health of the Russian Federation, Moscow, Russia.
Nelli B Naigovzina, A. I. Yevdokimov Moscow State University of Medicine and Dentistry, Moscow, Russia.
Yury P Rubtsov, Shemyakin-Ovchinnikov Institute of Bioorganic Chemistry of the Russian Academy of Sciences, Moscow, Russia.
Irina A Vasilyeva, National Medical Research Center for Phthisiopulmonology and Infectious Diseases of the Ministry of Health of the Russian Federation, Moscow, Russia.
Pavel Volchkov, Genome Engineering Laboratory, Moscow Institute of Physics and Technology, Dolgoprudniy, Russia. Research Institute of Personalized Medicine, National Center for Personalized Medicine of Endocrine Diseases, National Medical Research Center for Endocrinology, Moscow, Russia.
Elena Vasilieva, Clinical City Hospital named after I. V. Davydovsky, Moscow Department of Healthcare, Moscow, Russia. A. I. Yevdokimov Moscow State University of Medicine and Dentistry, Moscow, Russia.
Renovations approved to improve safety and efficiency of classrooms, research laboratories
The Indiana University Board of Trustees approved one architectural design and several facilities projects during its June meeting in Bloomington, including:
- The architectural designs for the IU Indianapolis Science Laboratory expansion and renovation project.
- Renovations to the IU School of Medicine’s VanNuys Medical Science Building Laboratory on the Indianapolis campus.
- Renovation and rehabilitation to chemistry teaching labs on the IU Bloomington campus.
- Replacement, upgrades and expansion of cooling capacity and distribution on the IU Bloomington campus.
- The 2025 Repair and Rehabilitation Plan for all IU campuses.
- 2025 Regional Campus Deferred Maintenance projects at five regional campuses.
IU Indianapolis Science Laboratory Building
The architectural designs for the IU Indianapolis Science Laboratory Building will introduce a cutting-edge, multidisciplinary research facility spanning 52,000 square feet, strategically positioned at the intersection of North Blackford and West New York streets in the IU Indianapolis Science and Technology Corridor.

The architecture of the new building is designed to complement the existing Science and Engineering Laboratory Building at IU Indianapolis and address the pressing need for enhanced research infrastructure to accommodate the campus’s growth.
The trustees approved this $60 million expansion and renovation project at their June 15, 2023, meeting and the project was also approved for state funding during the 2023 Indiana General Assembly as part of IU’s 2023-25 Capital Appropriation Request.
IU School of Medicine VanNuys Medical Science Building Laboratory
This project will renovate academic spaces across areas of the first, second and third floors of the North Wing of the IU School of Medicine’s VanNuys Medical Science Building at IU Indianapolis to convert existing office and classroom space into new research laboratories.
The anticipated opening of IU’s new Medical Education and Research Building provides opportunities to renovate existing office and classroom space in the VanNuys building to meet IU’s research laboratory goals. These new units in the VanNuys building will allow the university to explore how common research equipment can be efficiently leveraged by placing researchers with similar interests or approaches in close proximity.
In addition to academic space, renovations will include interior structural, mechanical and infrastructure updates and installation of new laboratory utilities, HVAC, plumbing, fire protection, power, data and finishes as required for new laboratory layouts. In all, the project will cover a total area of 28,099 square feet.
Chemistry teaching labs at IU Bloomington
This project will renovate eight teaching laboratories and related support areas in the Chemistry Building on the IU Bloomington campus, as well as replace four air handling units that serve these laboratories and related areas in the building.
Upgrades to more than 31,000 square feet — including more than 19,000 square feet of academic space — will be made in support of IU Bloomington Provost Rahul Shrivastav’s Project Inspire, a multiyear effort to systematically improve and update instructional spaces on the Bloomington campus.
The chemistry lab project will reconfigure and modernize 1980s-era teaching laboratories to improve safety, sightlines and accessibility, enabling flexible and collaborative instruction. Nearly 100 new fume hoods — which make use of high-efficiency, low-flow technology for significant energy savings, as well as improved laboratory safety — will be installed as well. The project will replace the building’s central heating, ventilation and air conditioning systems.
Work will be completed in two phases, allowing for uninterrupted instructional delivery during the construction process.
Cooling capacity and distribution at IU Bloomington
Phase one of a two-phase project will replace two aging chillers at the Main Chilled Water Plant and add one additional chiller in support of growing energy management and utility distribution needs on the Bloomington campus.
Since 2015, more than 2.1 million gross square feet of space has been added to the central chilled water system. This increase, especially during periods of extreme or prolonged periods of heat, has significantly increased cooling demand. New higher-capacity replacement chillers will operate more efficiently while largely making use of existing plant infrastructure.
In addition to the new chillers, this project will connect the standalone Union Street plant to the Forest Quad plant, adding Union Street to the central system and expanding reliability and diversity of cooling capacity campus-wide. The new chiller equipment as well as the physical connection of the Union Street plant will add a net increase of more than 3,200 tons of additional capacity — a 16% increase — to IU Bloomington’s central chilled water loop.
2025 Repair and Rehabilitation Plan
Funded by state appropriation and student fees, the 2025 Repair and Rehabilitation Plan includes repairs or replacement of roofing; windows; elevators; electrical, fire protection, mechanical and plumbing systems; steam, utilities, and electricity distribution systems; and classroom and site improvements at IU Bloomington, IU Indianapolis and all regional campuses.
2025 Regional Campus Deferred Maintenance
The Regional Campus Deferred Maintenance projects impact 14 buildings on five campuses and will provide safe, effective and efficient learning and work environments for students, faculty and staff through repairs and renovations of facilities and infrastructure.
Renovations will include replacing or updating building heating systems, mechanical systems and controls, and will continue to address work begun in Phases I-V of the Regional Campus Deferred Maintenance requests. This project was previously funded by the Indiana General Assembly in the 2023-2025 State Budget and was part of Indiana University’s 2023-25 Capital Appropriation Request.
Marah Yankey
Filed under:, more stories.

IU South Bend preparing for celestial event with on-campus activities

History Museum in South Bend honors Dé Bryant with African American Legacy Award
Social media.
- Facebook for IU
- Linkedin for IU
- Twitter for IU
- Instagram for IU
- Youtube for IU
Additional resources
Indiana university.
- About Email at IU
- People Directory
- Non-discrimination Notice
- Email Newsletters & Press Releases
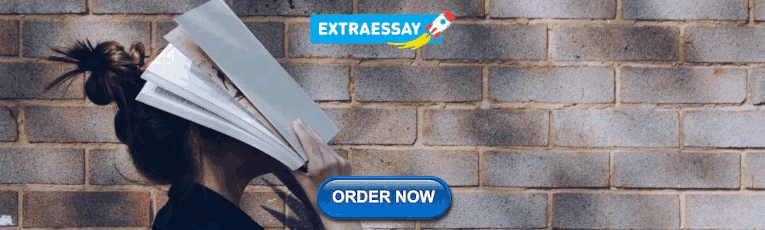
IMAGES
VIDEO
COMMENTS
At the foundation of Johns Hopkins Medicine is research — from basic research, where scientists study cells and mechanisms, to clinical research that builds on those findings using trials, to translational research that takes information learned from trials to the patient bedside. Browse Research Topics.
Explore Our URMC & UR Research Labs. The University of Rochester Medical Center and University of Rochester are home to more than 3,000 researchers and 500+ labs dedicated to scientific research. Our researchers study common and rare illnesses - from cancer and heart disease and Parkinson's to pandemic Influenza and COVID-19.
Research Lab Results. Clinical Trials. For Patients: Understanding Clinical Trials. About Our Faculty. About Our Faculty. Back to Research Main Menu. Overview. Discoveries for a Better Tomorrow. ... Search for a research area, principal investigator, or keyword. Search. Browse an A-Z Listing. A
Clinical research is the comprehensive study of the safety and effectiveness of the most promising advances in patient care. Clinical research is different than laboratory research. It involves people who volunteer to help us better understand medicine and health. Lab research generally does not involve people — although it helps us learn ...
At Michigan Medicine research happens first in the lab. Find labs below organized by Medical School categories. Lab Directory - Basic Sciences Baldridge Lab (Ryan Baldridge, PhD) ... Biomechanics Research Laboratory (James Ashton-Miller, PhD) Baker Lab (Brendon Baker, PhD) Virtual Physiological Rat Project (Daniel Beard, PhD)
Protecting animal health. Our Animal Health business is dedicated to preserving and improving the health, well-being and performance of animals and the people who care for them. At Merck, we follow the science. Learn about Merck's research and products by getting to know the science, strategy and brains behind the innovations.
NIH Institutes. National Cancer Institute (NCI) — Est. 1937 NCI leads a national effort to eliminate the suffering and death due to cancer. Through basic and clinical biomedical research and training, NCI conducts and supports research that will lead to a future in which we can prevent cancer before it starts, identify cancers that do develop at the earliest stage, eliminate cancers through ...
The labs at Michigan Medicine are at the heart of our research work. With 600+ labs in operation, we work across the realm of cutting-edge science. Throughout 20 clinical and nine basic science departments, teaching, research and clinical care often cross traditional departmental boundaries. Our interdisciplinary centers, institutes and ...
Research Laboratories at Tufts Medical Center. Tufts Medical Center, joined to Tufts School of Medicine by multiple bridges, plays an integral role in our research. Over 100,000 square feet of research space is home to the scientists and physician-scientists at this facility. These include the Tupper Building at 15 Kneeland Street.
Research in NIH Labs & Clinics. Research on the NIH campuses. NIH Clinical Center. Research in Action. Careers. Commercializing Inventions. News and Events. Office of Intramural Research. This page last reviewed on January 18, 2017.
The UW Medicine research powerhouse is driven by outstanding collaboration and interdisciplinary programs. Award-Winning Faculty UW Medicine's faculty includes many members of the National Academy of Sciences, the National Academy of Medicine and the Howard Hughes Medical Institute as well as recipients of Nobel, Gairdner, Lasker and Shaw prizes.
Medical Research. Scientists and physicians in academic medicine conduct groundbreaking biomedical research that improves our knowledge of human health and promotes the development of treatments from bench to bedside to community. Through policy and advocacy initiatives, data and research projects, professional learning and networking ...
Medical research often requires access to specialized instruments and complex technologies most individual labs can't afford. The RUSH core laboratories provide both RUSH and outside investigators with access to facilities that offer leading-edge tools, equipment and technologies essential to many modern research projects.
With access to the resources of Stanford University -- including the Schools of Engineering, Law, Business, Humanities & Sciences and Education -- Stanford Medicine enables close interactions between physicians and scientists, faculty and trainees, and basic science and clinical care. Learn about our institutes & initiatives.
Clinical research, a cornerstone in advancing patient care, involves human subjects to test the safety and effectiveness of new treatments, ranging from drugs to diagnostic tools.Unlike clinical research, laboratory research focuses on the foundational science behind medicine without direct human involvement, contributing significantly to medical lab science.
This is an NIH-funded initiative to connect 1) people who are trying to find research studies, and 2) researchers seeking people to participate in their studies. It is a free, secure registry to make it easier for the public to volunteer and to become involved in clinical research studies that contribute to improved health in the future.
Faculty Research Labs. In Dell Medical School's research environment — which spans the campuses of Dell Med, the Dell Pediatric Research Institute, The University of Texas at Austin and other locations in the city — faculty using similar techniques and equipment work near each other and share expertise from diverse fields.
Basic medical research (otherwise known as experimental research) includes animal experiments, cell studies, biochemical, genetic and physiological investigations, and studies on the properties of drugs and materials. ... Laboratory conditions cannot always be directly transferred to normal clinical practice and processes in isolated cells or ...
Find your nearest lab location and schedule an appointment using the search below. To make an appointment or get detailed lab information use the search below. Walk-ins are also welcome. Please note that not all lab locations offer all services. *All fields are required, except ones marked as optional. Locate Me OR. Reason for your visit. Radius.
Responsibilities. The Medical Microrobots Lab at the Mayo Clinic Arizona Campus is seeking a highly motivated Postdoctoral Fellow to join our innovative team of scientists, engineers, and medical doctors. We are passionate about tiny, soft-bodied robots and unleashing their disruptive potential, pushing the boundaries of targeted therapies and ...
Here are 10 careers you can pursue in the field of medical research: 1. Clinical laboratory scientist. National average salary: $89,291 per year Primary duties: A clinical laboratory scientist is a scientist who specializes in using lab equipment to perform tests on biological specimens. This can involve extracting and testing bodily fluids to ...
At a glance: Researchers have created a cervix-on-a-chip that models the structure and function of the human cervix. They plan to use the model to investigate and develop better treatments for diseases of the female reproductive tract. Models like this and the recently developed vagina-on-a-chip can counter a historical research gap in women ...
1. Enroll in a program. To become a medical laboratory technician, you'll need to complete a college certificate program in medical laboratory science. This type of program will prepare you for an entry-level job. In many cases, if you decide to advance your health care career, your credits will transfer toward earning a bachelor's degree in ...
The Clinical Research Coordinator will work closely with the PI, as well as with other faculty, staff members and trainees within the department. Responsibilities. Perform 2D and 3D sonography on research human subjects and large animals (canine/swine); Assist the PI in the preparation of clinical study and large animal study planning;
Severe acute respiratory syndrome coronavirus 2 (SARS-CoV-2) was identified as a causative agent of a new coronavirus disease 2019 (COVID-19). Individuals who have cleared the virus or who have been vaccinated develop an adaptive immune response including virus-specific T cells and antibodies [], which have been shown to protect from reinfection [].
Performs routine scientific and medical research work in science area such as biology, chemistry, physics, or other related scientific field. Assembles and/or operates various laboratory equipment and apparatus. Records research data in accordance with prescribed procedures, discussing results with supervisor.
The architecture of the new building is designed to complement the existing Science and Engineering Laboratory Building at IU Indianapolis and address the pressing need for enhanced research infrastructure to accommodate the campus's growth. The trustees approved this $60 million expansion and renovation project at their June 15, 2023 ...
The Institute of Medical Parasitology and Tropical Medicine named after E. Martsinovskii is the educational base of the department of Tropical Medicine and parasitic diseases which founded as a part of the Faculty of Preventive Medicine. The institute owns a large collection of agents of parasitic diseases and arthropod-carriers of diseases ...
Research Centre for Medical Genetics is a federal nonprofit academic research institution that leads in medical genetics field in Russia. Researchers and physician-scientists work side by side to transform scientific discoveries into breakthrough diagnostic methods and therapies in patient care. ... Laboratories of the centre diagnose more than ...
Rebecca Alvarado ('21,'23) was accepted into Harvard Medical School's Bernardo Sabatini Laboratory as a research assistant. Alvarado, who holds both a bachelor's and master's degrees in psychology from TAMIU, hopes to use this experience as she works to apply for an MD/PhD—program in Neuroscience. "Anything is possible if you ...