Boosting renewable energy growth through responsible value chains: A case study from China
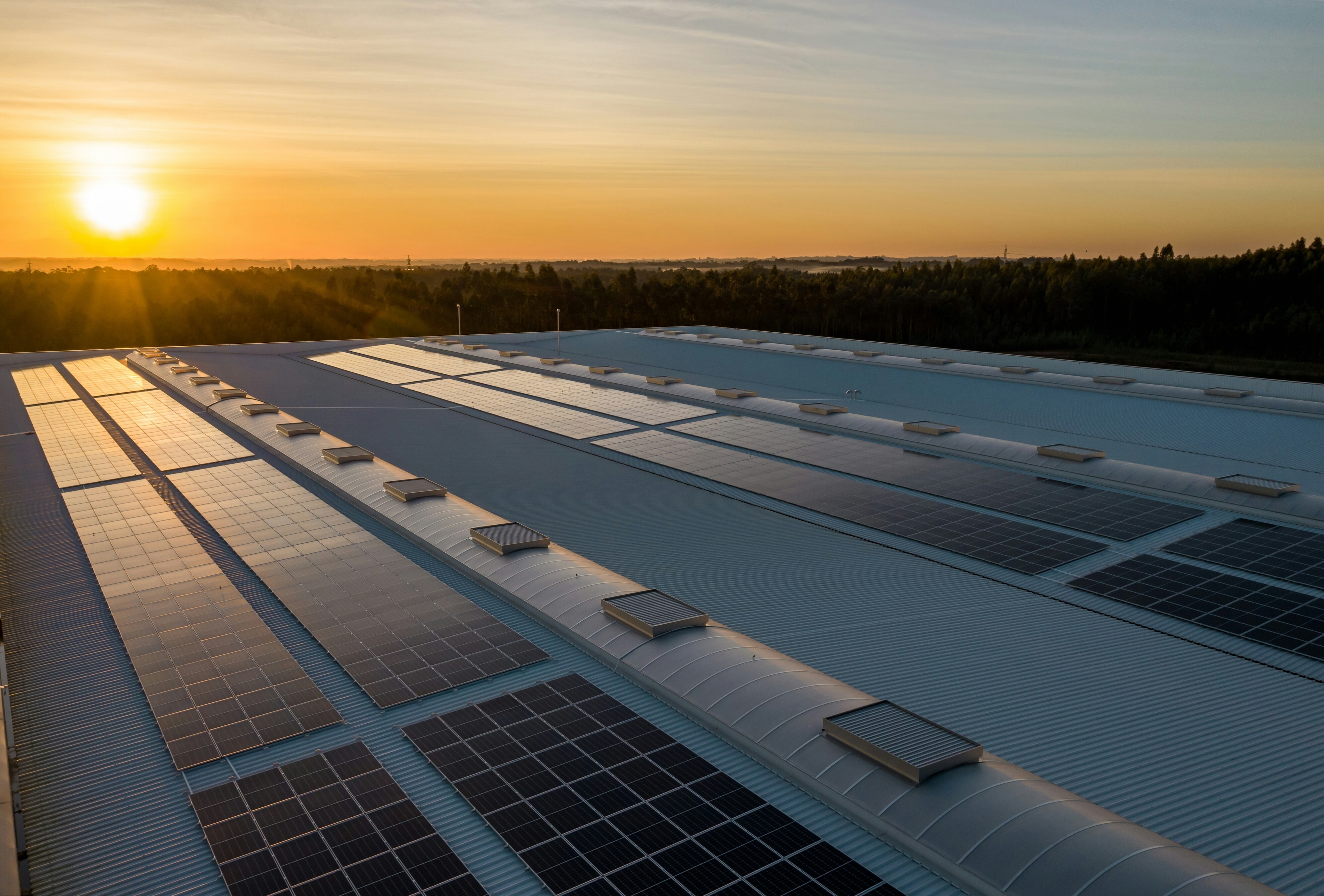
Over half of the carbon emissions in the renewable value chain comes from upstream activities. Image: Unsplash/Nuno Marques
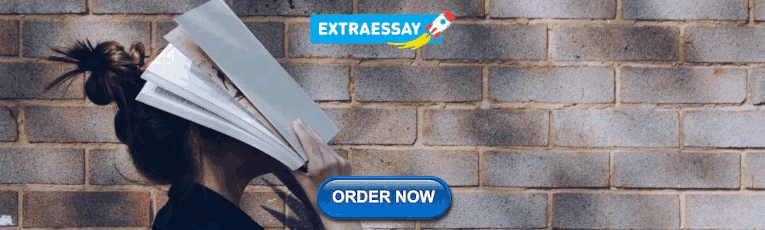
.chakra .wef-1c7l3mo{-webkit-transition:all 0.15s ease-out;transition:all 0.15s ease-out;cursor:pointer;-webkit-text-decoration:none;text-decoration:none;outline:none;color:inherit;}.chakra .wef-1c7l3mo:hover,.chakra .wef-1c7l3mo[data-hover]{-webkit-text-decoration:underline;text-decoration:underline;}.chakra .wef-1c7l3mo:focus,.chakra .wef-1c7l3mo[data-focus]{box-shadow:0 0 0 3px rgba(168,203,251,0.5);} Vee Li
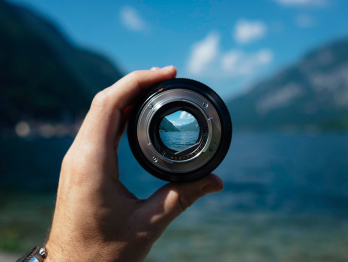
.chakra .wef-1nk5u5d{margin-top:16px;margin-bottom:16px;line-height:1.388;color:#2846F8;font-size:1.25rem;}@media screen and (min-width:56.5rem){.chakra .wef-1nk5u5d{font-size:1.125rem;}} Get involved .chakra .wef-9dduvl{margin-top:16px;margin-bottom:16px;line-height:1.388;font-size:1.25rem;}@media screen and (min-width:56.5rem){.chakra .wef-9dduvl{font-size:1.125rem;}} with our crowdsourced digital platform to deliver impact at scale
Stay up to date:, transitioning industrial clusters towards net zero.
- Meeting the Paris Agreement’s 1.5 degrees Celsius ambition requires a profound shift in energy production and consumption, with renewable energy transitions contributing over a quarter of all efforts.
- Over half of the carbon emissions in the renewable energy sector come from upstream activities. As global renewable energy capacity rapidly expands, responsibly delivering net-zero, trusted and circular energy is becoming a crucial growth driver for the sector.
- The World Economic Forum’s Centre for Energy and Materials working group – Scaling up Renewable Energy in China – with the International Energy Community China and the Clean Power, Grids and Electrification initiative, analyzes China’s renewable energy value chain with recommendations for companies to achieve net-zero emissions.
Recent research indicates that the global average temperature in 2023 may have already exceeded 1.5 degrees Celsius above pre-industrial levels. This underscores the urgency for all governments, particularly major emitters, to enhance their nationally determined contributions for emission reduction, in order to meet the goals of the Paris Agreement.
At the 2023 United Nations Climate Change Conference (COP28), parties achieved the United Arab Emirates Consensus on Global Stocktake . This milestone includes dialogue around the transition from fossil fuels with targets to triple current renewable energy and double energy efficiency.
China’s carbon emission in 2023 was among the top country emitters and was the leading producer of renewable energy. Its emissions reached a staggering 12.6 gigatons , accounting for 35% of the global total . Furthermore, China’s installed renewable energy capacity surged to 1.45 terawatts by the end of 2023, surpassing thermal power installed capacity for the first time.
As China is well into its 14th five-year plan and works towards its long-term goals for 2035, the acceleration of renewable energy development is a critical step towards achieving its ambitious carbon neutrality target by 2060. However, despite renewable energy’s low carbon nature, emissions associated with its value chain must not be overlooked amid its rapid expansion.
Have you read?
Working together to reach net zero: how to transform manufacturing and value chains, clean energy deployment along the value chain is key to china’s industry decarbonization. 3 business leaders explain why, why cooperation is key to decarbonizing value chains, according to a clean energy expert, the energy value chain and emissions.
Over half of the carbon emissions in the renewable energy value chain stem from upstream activities.
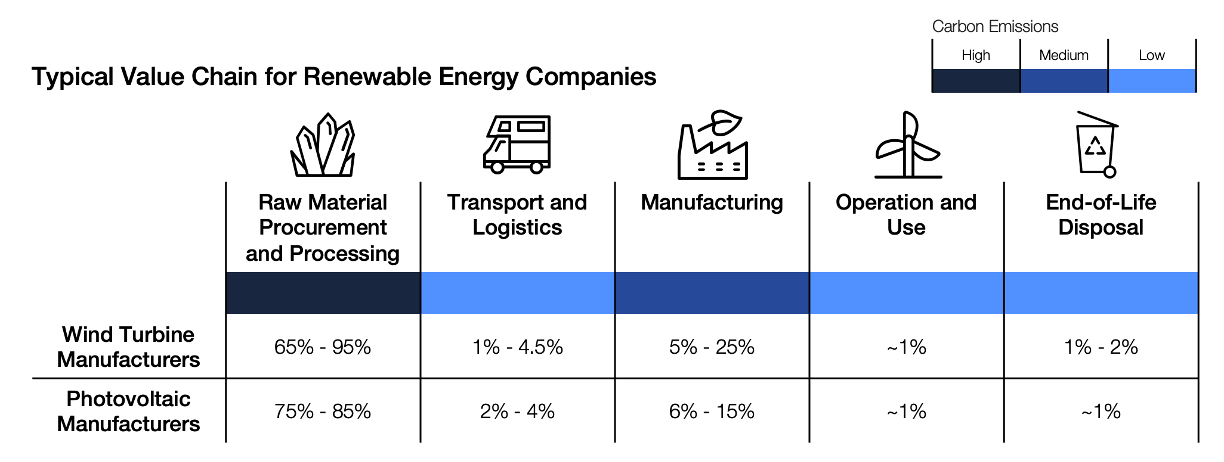
- Upstream: During upstream activities, raw material procurement, processing, and equipment manufacturing are critical stages contributing to increased carbon emissions. These processes typically involve high energy consumption and reliance on non-renewable energy sources.
- Midstream: While carbon emissions from energy production, operations, and distribution are relatively lower in the midstream segment, the energy consumption associated with operations, maintenance and long-distance transmission should still be addressed.
- Downstream: End-user consumption at the downstream end of the value chain significantly influences the product’s lifecycle carbon emissions. Inefficient battery usage and inadequate recycling of waste materials are primary factors contributing to elevated carbon emissions.
Building a responsible renewable energy value chain
Forging a responsible value chain for renewable energy companies is a pivotal catalyst for competitive advantage and a vital contribution to global climate goals.
Reaching net-zero emissions across the value chain is complex and demanding, requiring renewable energy companies to dedicate themselves to strategy planning, operational optimization and ecosystem partnerships.
The Scaling up Renewable Energy in China working group under the World Economic Forum’s Centre for Energy and Materials recommends that these companies take immediate action to enhance renewables’ low-carbon nature, accelerate responsible transformations in the value chain and align the transition to renewable energy with economic growth and decarbonization goals.
1. Measure and report on Scope 3 emissions
- Establish an emissions baseline: Set a clear baseline for emissions covering the entire value chain activities, including scope three emissions.
- Align standards: Ensure the alignment with internationally recognized standards, such as the Greenhouse Gas Protocol (GHG Protocol), to increase the data accuracy and comparability.
- Improve transparency and communication: Regularly publish reports on value chain emissions and maintain transparent communication with stakeholders, including investors, consumers and regulators.
2. Engage suppliers to decarbonize collaboratively
- Strengthen building supply chain partnerships: Establish collaborative relationships with suppliers to set shared emissions reduction targets.
- Provide training and technical support: Offer training and technical assistance in low-carbon transformation, share best practices with suppliers, help them improve energy efficiency, and increase the adoption of clean technologies.
- Establish incentive mechanisms: Motivate suppliers to reduce emissions through incentives such as preferential pricing and extended contracts and prioritize suppliers actively engaged in decarbonization efforts.
3. Improve energy efficiency and transit to 100% renewable
- Improve energy efficiency: Innovate and optimize processes to decrease energy consumption intensity in production and operations.
- Procure renewable energy: Transit to 100% renewable energy by increasing the use of wind and solar power.
- Secure renewable energy certificates: Purchase recognized renewable energy certificates to support the development of renewable energy projects.
Leading by example
Some successful results accelerating decarbonization can already be seen as a result of collaborative actions across the value chain.
Science-based targets
“As a renewable energy company, if we operate in a responsible way, producing solar with solar, manufacturing green with green, it will create a tremendous force for good in business,” says Chenchen Yao, head of environment, social and governance at JinkoSolar.
JinkoSolar leads in the the global photovoltaic sector as it is the first in its sector to have its short-term, long-term and net-zero emission reduction targets validated by the Science Based Targets initiative (SBTi). SBTi is an organization that helps companies determine how much and how quickly they need to reduce their greenhouse gas emissions to prevent the worst effects of climate change.
JinkoSolar aims to fully adopt renewable energy by 2030 and is actively working with its partners to reduce emissions throughout its value chain. Specifically, the company seeks to decrease emissions from purchased goods and services by 58.2% per megawatt of solar-related products produced by the year 2032.
JinkoSolar explores effective approaches to reducing the environmental impact of products throughout their entire lifecycle – including research and development, procurement, manufacturing, transport and recycling – and embeds greenhouse gas emissions into supplier admission, management and risk assessment processes to drive supply chain sustainability improvement for the renewable energy industry.
As a renewable energy company, if we operate in a responsible way, producing solar with solar, manufacturing green with green, it will create a tremendous force for good in business.
Dual carbon goals
“Sunwoda is dedicated to the deep recycling of materials. We collaborate with ecosystem partners in technology innovation and battery recycling management, offering solutions for diverse renewable energy application scenarios, driving progress and development in the renewable energy industry,” says Rui Liang of the company where he is vice president and chief sustainability officer.
Sunwoda has defined its sustainability strategy around China’s dual-carbon goals . The company is committed to reaching a carbon peak by 2029 and neutrality by 2050 in operations, and it aims to reduce societal carbon emissions by 6.84 million tons by 2030 and 42.37 million tons in transportation by 2040.
The company implements a complete lifecycle carbon reduction strategy, powering manufacturing with renewable energy via photovoltaic and storage systems and pursuing technological advancements in raw materials, battery data management and recycling with partners to lower carbon across the battery supply chain and support the energy shift for various industries.
Sunwoda is dedicated to the deep recycling of materials. We collaborate with ecosystem partners in technology innovation and battery recycling management, offering solutions for diverse renewable energy application scenarios, driving progress and development in the renewable energy industry.
Future opportunities
Regions with potential for renewable energy are typically areas rich in biodiversity. Mitigating the impacts of the renewable energy value chain on nature and biodiversity – from risks associated with raw material extraction to daily operation effects on marine and terrestrial habitats – is crucial for renewable energy companies dedicated to shifting from net-zero to net-positive.
Amidst the competitive landscape of the renewable energy sector, leveraging value chain synergies to establish a responsible ecosystem gives industry players a strategic advantage. As the world transitions towards net-zero emissions, the renewable energy industry must stand poised to lead with innovation, driving sustainable growth and fundamentally transforming the global energy paradigm.
Don't miss any update on this topic
Create a free account and access your personalized content collection with our latest publications and analyses.
License and Republishing
World Economic Forum articles may be republished in accordance with the Creative Commons Attribution-NonCommercial-NoDerivatives 4.0 International Public License, and in accordance with our Terms of Use.
The views expressed in this article are those of the author alone and not the World Economic Forum.
The Agenda .chakra .wef-n7bacu{margin-top:16px;margin-bottom:16px;line-height:1.388;font-weight:400;} Weekly
A weekly update of the most important issues driving the global agenda
.chakra .wef-1dtnjt5{display:-webkit-box;display:-webkit-flex;display:-ms-flexbox;display:flex;-webkit-align-items:center;-webkit-box-align:center;-ms-flex-align:center;align-items:center;-webkit-flex-wrap:wrap;-ms-flex-wrap:wrap;flex-wrap:wrap;} More on Energy Transition .chakra .wef-17xejub{-webkit-flex:1;-ms-flex:1;flex:1;justify-self:stretch;-webkit-align-self:stretch;-ms-flex-item-align:stretch;align-self:stretch;} .chakra .wef-nr1rr4{display:-webkit-inline-box;display:-webkit-inline-flex;display:-ms-inline-flexbox;display:inline-flex;white-space:normal;vertical-align:middle;text-transform:uppercase;font-size:0.75rem;border-radius:0.25rem;font-weight:700;-webkit-align-items:center;-webkit-box-align:center;-ms-flex-align:center;align-items:center;line-height:1.2;-webkit-letter-spacing:1.25px;-moz-letter-spacing:1.25px;-ms-letter-spacing:1.25px;letter-spacing:1.25px;background:none;padding:0px;color:#B3B3B3;-webkit-box-decoration-break:clone;box-decoration-break:clone;-webkit-box-decoration-break:clone;}@media screen and (min-width:37.5rem){.chakra .wef-nr1rr4{font-size:0.875rem;}}@media screen and (min-width:56.5rem){.chakra .wef-nr1rr4{font-size:1rem;}} See all
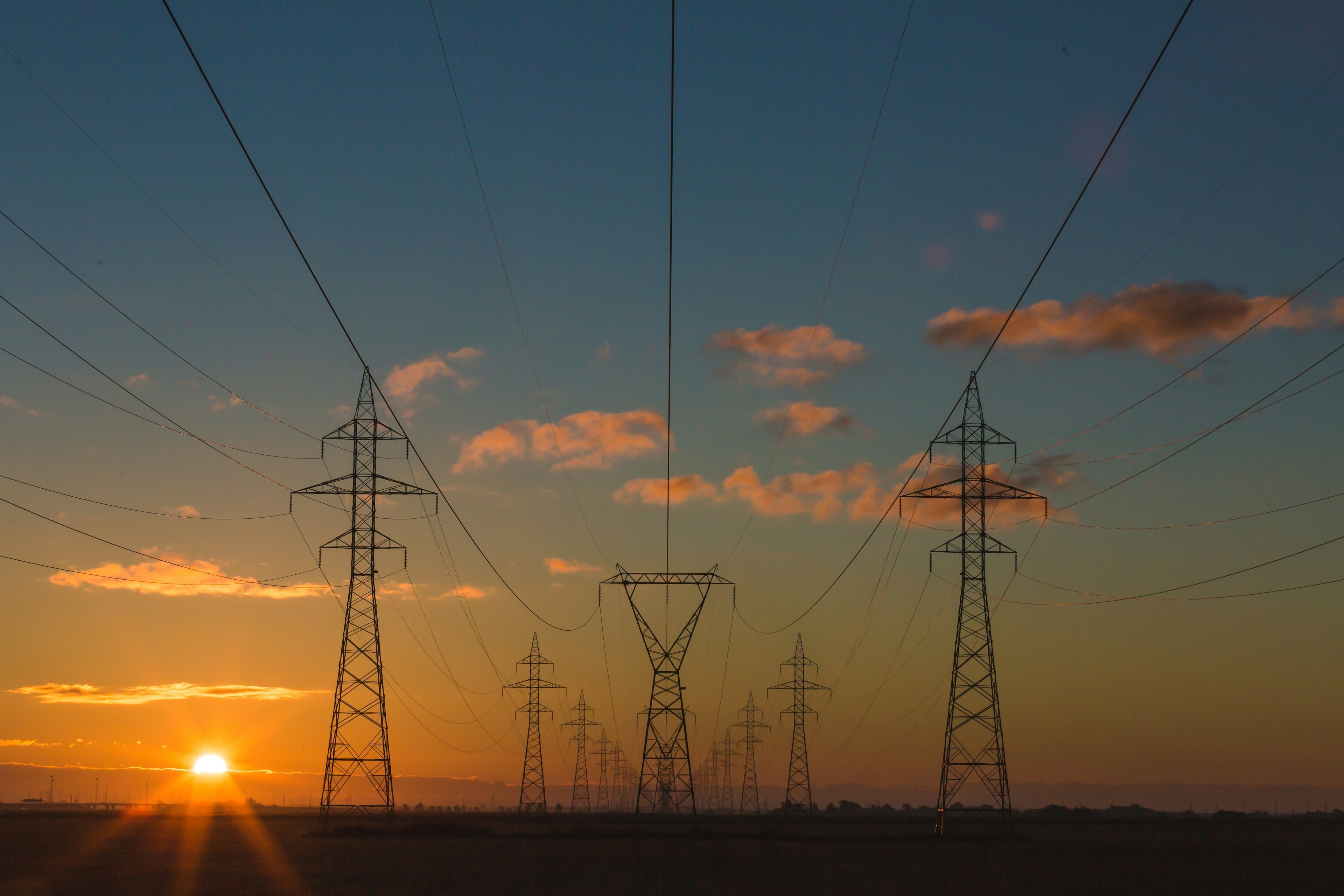
What is needed for inclusive and sustainable global economic growth? Four leaders share their thoughts
Liam Coleman
May 24, 2024
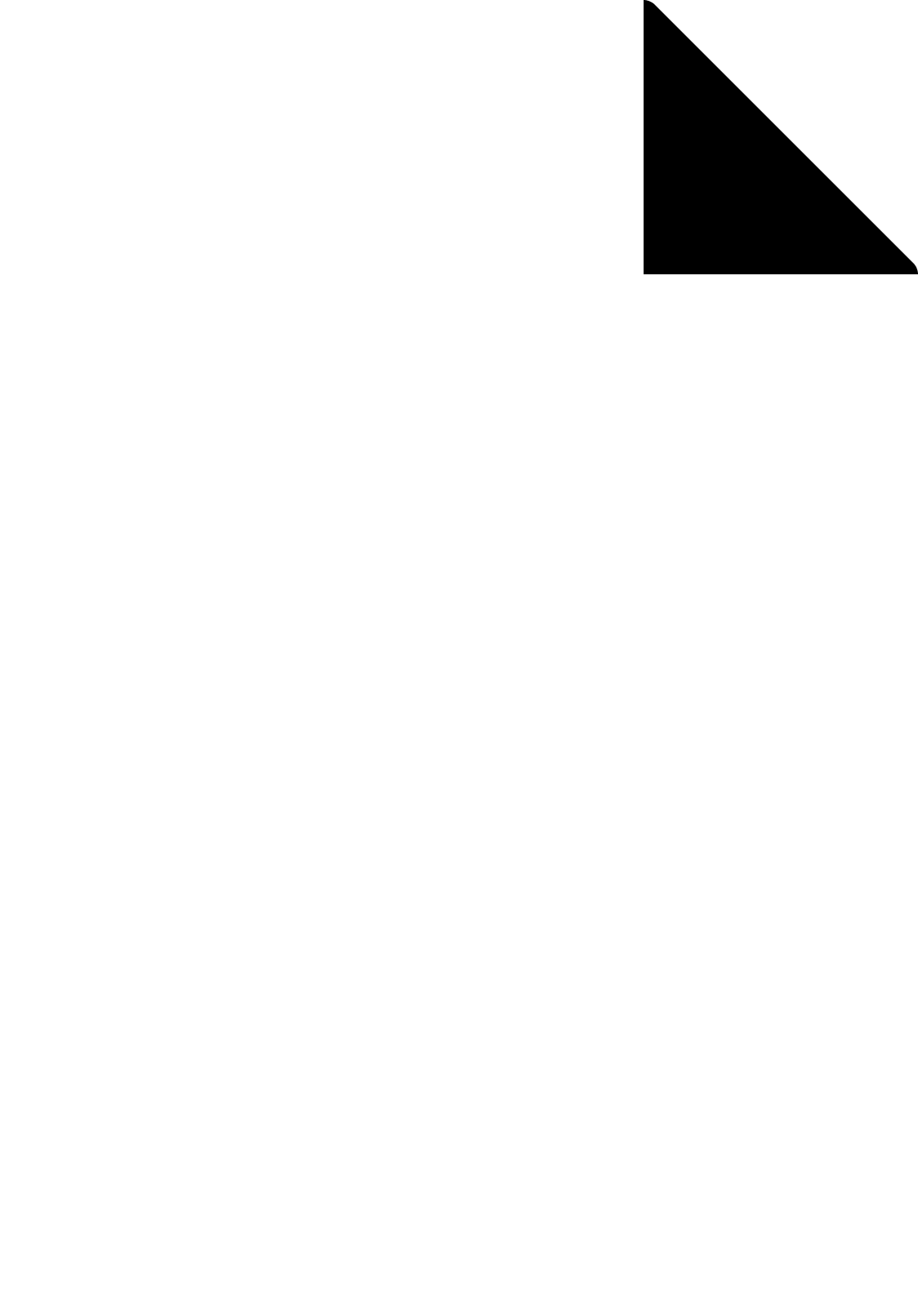
Translating Critical Raw Material Trade into Development Benefits
How trade friction is slowing down the world’s shift to clean energy
Sverre Alvik
May 23, 2024
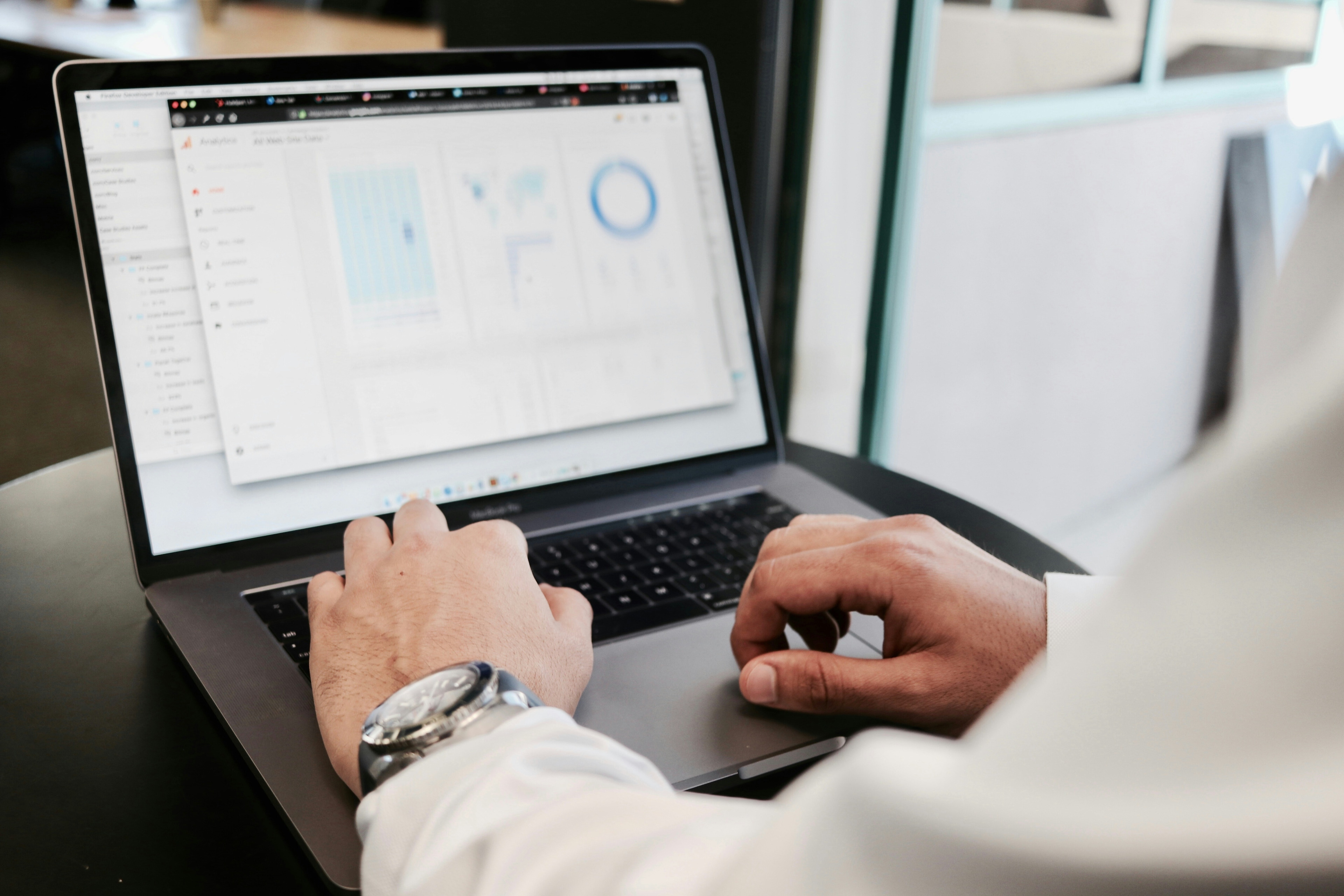
Data volume is soaring. Here’s how the ICT sector can sustainably handle the surge
Bart Valkhof, Eleni Kemene and Justin Stark
May 22, 2024
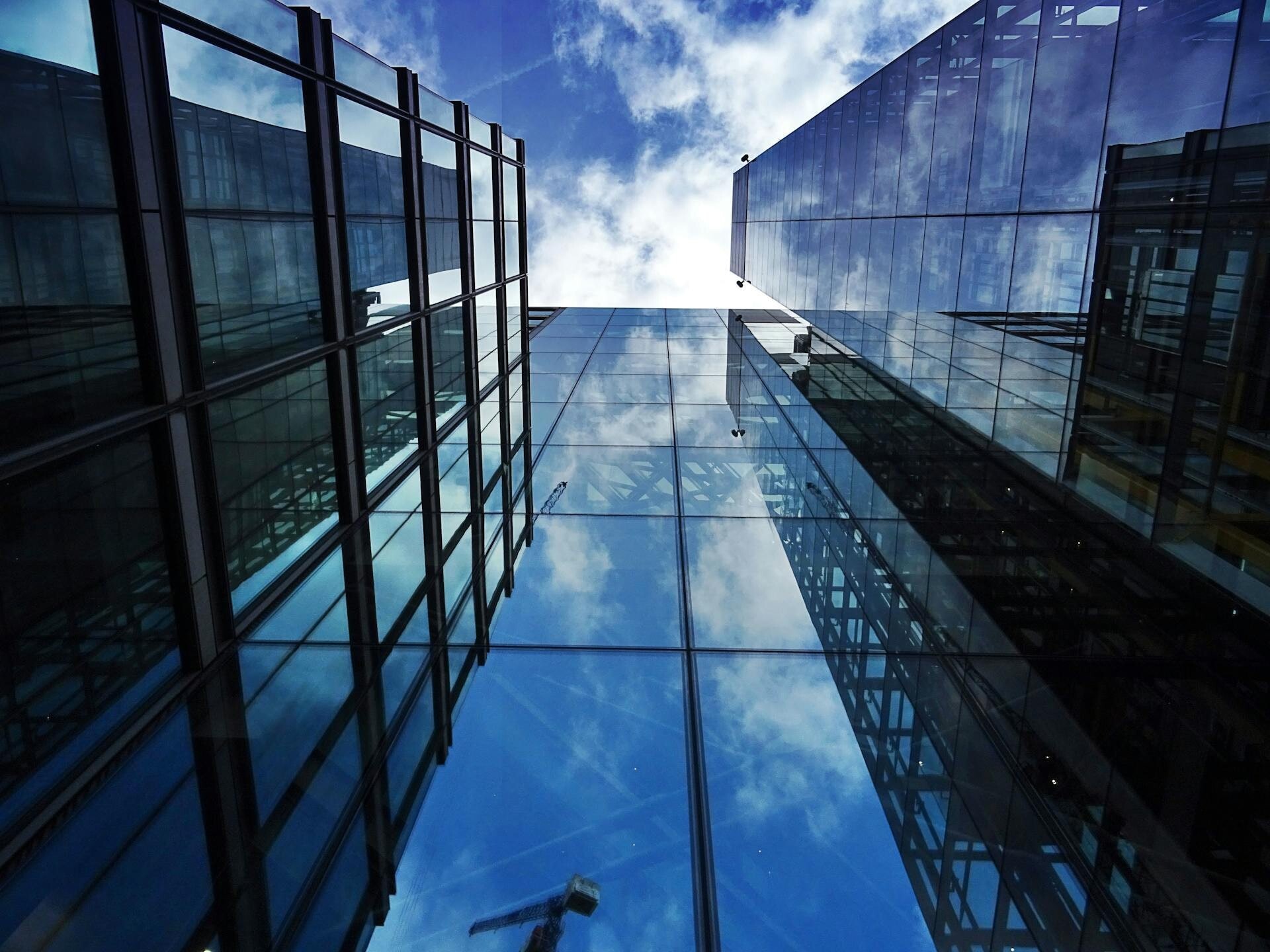
European financial institutions are confronting new challenges. Here's how
Kalin Anev Janse and Kim Skov Jensen
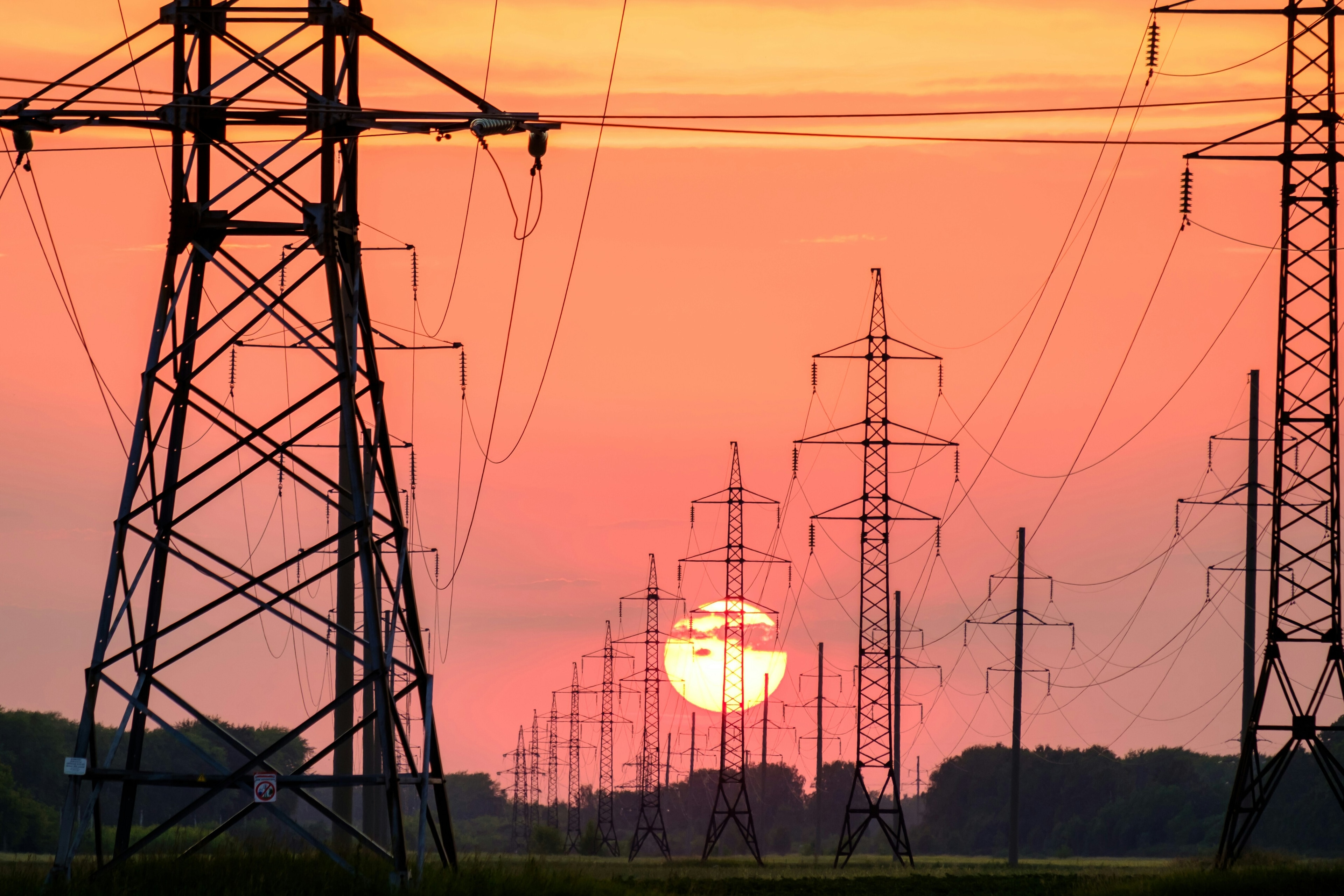
India facing record power shortfall for June, and other top energy stories this month
Roberto Bocca
May 21, 2024
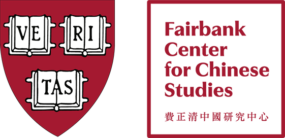
Home » Blog » How China is Winning the Race for Clean Energy Technology
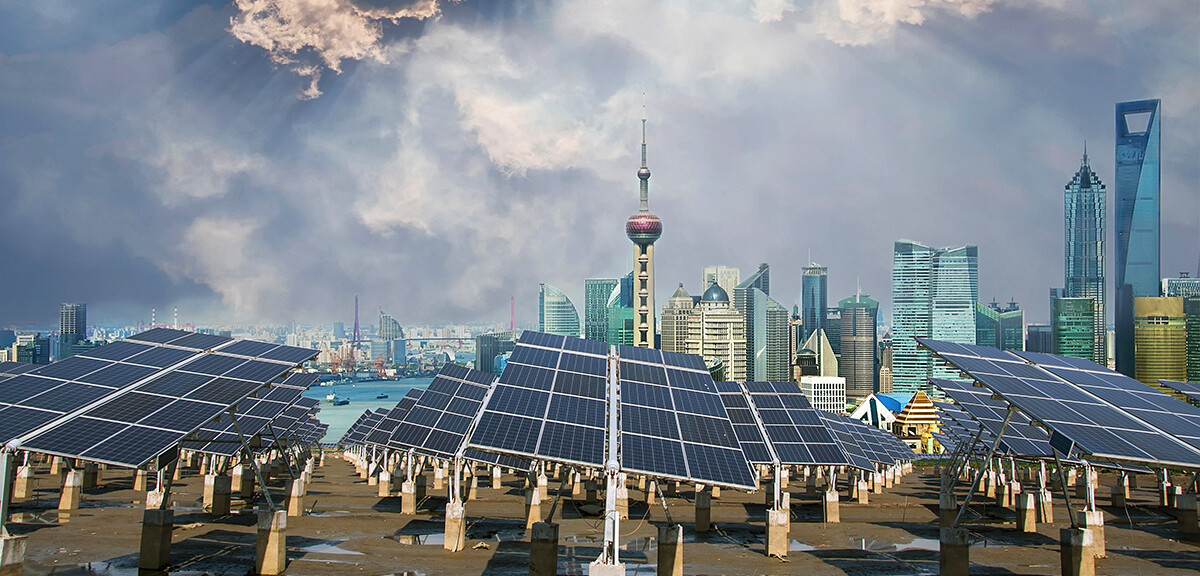
How China is Winning the Race for Clean Energy Technology
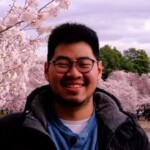
For the past two decades, a clean energy revolution has been quietly taking place across the globe. More recently, a heated global race has begun with China at the head of the pack.
Judging from the emissions data, you wouldn’t think China is ahead. China’s carbon emissions have yet to peak, while the United States and the European Union have drastically lowered their carbon emissions over the last decade. The UK is now at 44% below its 1990 levels and plans to reduce annual carbon emissions to 70% below 1990 levels by 2045. Having peaked its carbon emissions in 2007, the United States has reduced its carbon emissions to 2% above its 1990 levels. China plans to peak its emissions by 2030. But recent investments in fossil fuel have cast doubt on that scenario.
At the same time, however, China has been making massive investments in energy transition. “China saw an economic opportunity in low-carbon industries,” according to Kelly Sims Gallagher, Director at the Tufts University Center for International Environment and Resource Policy, “and they formed industrial policy.” While Europe was an early mover in clean energy, China has harnessed its competitive advantage in policy consistency and lower-cost capital to lead the market in solar, wind, and EV technology exports. With the passage of the CHIPS, Infrastructure, and Inflation reduction bills in 2022, the United States has finally significantly accelerated clean energy investment. But is it too late for the Americans to catch up? At a recent Critical Issues Confronting China talk hosted by the Fairbank Center for Chinese Studies, Gallagher gave an update on the current state of the global race for Leadership in low-carbon tech and provided her outlook on the future of clean energy.
Five key takeaways:
China’s clean energy innovation was driven by economic opportunism.
By 2009, China had made strong political commitments to renewable energy development and there was a noted government acceptance of climate science. But it was rather recognition of the immense economic potential in the green energy innovation that drove China past its tipping point. In 2009, China was already on track to pass the US as the world’s largest market for wind turbines and had begun pitting state owned power companies against each other to see which could build solar plants fastest. According to Gallagher, China initially made forays into clean energy technology as a means for export, but was able to adroitly set up a domestic market when conditions for outward investment and trade turned sour. Today, China is a veritable green power. It leads the world in renewable energy production figures and is the world’s largest producer of wind and solar energy, as well as the largest domestic and outbound investor in renewable energy.
A fear of China drove the U.S. to act on clean energy
Nothing exemplifies the return of Great Power politics more than the race for clean energy. Where decades of activism and warnings from scientists had failed to push significant policy change, fear of falling behind China has created a bipartisan effort to invest in clean energy transition. Together, the CHIPS and Science Act, Infrastructure Deal, and Inflation Reduction Act have brought close to half a trillion dollars in fiscal incentives and investments into the growth of clean energy and low-carbon technologies in the US. However, while the bills will boost research and development, they bring in limited regulation. Gallagher pointed out that according to the Department of Energy’s own estimates, the U.S. is “not competitive” in most clean energy sectors. It is still too early to tell if the massive influx of capital will be enough for the U.S. to win the clean energy race. “It’s a little daunting for the U.S.,” Gallagher said. Speaking of China’s dominance in the supply chains for low-carbon technologies, she added, “China is a planning economy, and that has really paid off.”
While China maintains an advantage in the race, the U.S. still has some areas where it might have a slight edge.
China seems to have an all-round lead on the U.S. in terms of trade balance, R&D investment, and patenting. On the investment front, China had surpassed the U.S. by the late 2000s and Europe by 2012 to be the world leader in low carbon technology investment, pumping in annually at least US$100 billion into innovation by 2014. As of 2018, Chinese investment in clean energy technology was almost double that of the U.S. (The scenario changes when accounting for nuclear investments by the U.S.) At the same time, China’s State-Owned Enterprises are still making massive investments in fossil fuels that entrench the high-carbon economy and signal a bumpy energy transition for China in the coming future. Still, China’s clean energy leadership is signaled not just by its robust investment, trade, and patent figures but rather the number of clean energy jobs. Arguing that a competitive economy is shown through job creation, Gallagher noted that China has created many jobs in the clean energy sector.
A case study in solar energy:
Nowhere is China’s leading position clearer than in the solar energy sector. China has an estimated 2.7 million people employed in the solar energy sector, making up more than half of the world’s 4.3 million solar jobs. How did China do it? Initially China’s solar industry began in the 1990s to supply Germany’s high demand. The Chinese government provided tax incentives and credits to lure in investors and scoured the globe for supplies of machinery and polysilicon. This vertically integrated supply chain in China created a mammoth solar manufacturing industry that produced more solar panels for less. When western countries imposed tariffs (charging dumping), the Chinese government was able to quickly develop a domestic market. In contrast, the U.S. outsourced its solar production to China. In 2014, the U.S. had a dozen factories producing Photovoltaic wafers and ingots. Today, none exist. According to Gallagher, the disassembling of clean energy infrastructure is making it difficult for the U.S. to claw its way back.
The outlook for the U.S. is grim
Gallagher concluded that U.S. competitiveness in low carbon technology had completely eroded and that Chinese dominance in the clean energy supply chain will not soon go away. China’s carefully operated state machinery, political commitment, and industrial backing will let it keep its advantage in the clean energy race for the years to come. Gallagher characterized China’s recent investments in fossil fuels as a response to a temporary response to global energy instability. But the direction and momentum of China’s industrial policy will continue China on the path of clean energy dominance. There is one bright spot of hope for the U.S. however: U.S. climate-related spending is due to take off in the next 5 years and will focus on the next step of the clean energy transition: electricity and energy systems integration.
Thank you for visiting nature.com. You are using a browser version with limited support for CSS. To obtain the best experience, we recommend you use a more up to date browser (or turn off compatibility mode in Internet Explorer). In the meantime, to ensure continued support, we are displaying the site without styles and JavaScript.
- View all journals
- My Account Login
- Explore content
- About the journal
- Publish with us
- Sign up for alerts
- Open access
- Published: 10 January 2022
Assessing the energy transition in China towards carbon neutrality with a probabilistic framework
- Shu Zhang ORCID: orcid.org/0000-0003-1977-0446 1 &
- Wenying Chen ORCID: orcid.org/0000-0001-9376-5579 1
Nature Communications volume 13 , Article number: 87 ( 2022 ) Cite this article
42k Accesses
173 Citations
25 Altmetric
Metrics details
- Climate-change mitigation
- Energy modelling
- Energy policy
- Projection and prediction
A profound transformation of China’s energy system is required to achieve carbon neutrality. Here, we couple Monte Carlo analysis with a bottom-up energy-environment-economy model to generate 3,000 cases with different carbon peak times, technological evolution pathways and cumulative carbon budgets. The results show that if emissions peak in 2025, the carbon neutrality goal calls for a 45–62% electrification rate, 47–78% renewable energy in primary energy supply, 5.2–7.9 TW of solar and wind power, 1.5–2.7 PWh of energy storage usage and 64–1,649 MtCO 2 of negative emissions, and synergistically reducing approximately 80% of local air pollutants compared to the present level in 2050. The emission peak time and cumulative carbon budget have significant impacts on the decarbonization pathways, technology choices, and transition costs. Early peaking reduces welfare losses and prevents overreliance on carbon removal technologies. Technology breakthroughs, production and consumption pattern changes, and policy enhancement are urgently required to achieve carbon neutrality.
Similar content being viewed by others
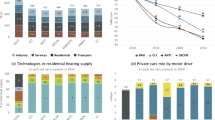
An assessment of energy system transformation pathways to achieve net-zero carbon dioxide emissions in Switzerland
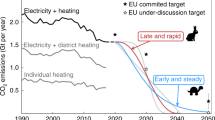
Early decarbonisation of the European energy system pays off
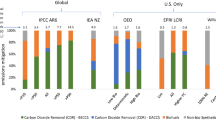
Drivers and implications of alternative routes to fuels decarbonization in net-zero energy systems
Introduction.
The Paris Agreement requires that countries progressively update their nationally determined contributions (NDCs) and reach a balance between sources and sinks of greenhouse gases (GHGs), implying the need to achieve net-zero emissions at some point in time 1 . China is currently the world’s largest energy consumer and CO 2 emitter, accounting for 23% and 29% of global energy consumption and CO 2 emissions in 2019, respectively 2 . A reduction in China’s CO 2 emissions would constitute an incomparable contribution to climate change mitigation. China updated its climate goal in September 2020 to strive to achieve peak CO 2 emissions by 2030 and work towards carbon neutrality by 2060. However, considerable uncertainties in the national cumulative carbon budget and technology evolution have produced broad variability in technology choice, transition costs, and the feasibility of different decarbonization pathways towards carbon neutrality, which have different consequences for national development and global temperature control. Decision-making under high uncertainty presents a difficult challenge to policymakers 3 , 4 , 5 , 6 . A roadmap that comprehensively considers these uncertainties is critically needed to guide this transition 7 .
Most current energy-environment-economy models adopt a scenario analysis approach to make a priori assumptions about future trends. The variety of projections for future development in different studies frequently produce widely divergent and puzzling results. Although some consensus can be obtained through model comparisons, it is challenging to capture the impacts of policy and technology development uncertainty in a probabilistic manner 8 . Additionally, it is difficult to obtain stable and reliable results using traditional sensitivity analysis in the context of multidimensional uncertainty 9 . Monte Carlo analysis (MCA) is a classical approach for climate change assessment under uncertainty 10 , 11 and is widely used with energy system models 12 , 13 , 14 , 15 , 16 .
In this work, we combine MCA with the China-TIMES model, which has been extensively used in energy planning and climate change mitigation studies 17 , 18 , 19 , 20 , 21 , 22 , 23 , 24 , 25 , 26 , 27 , 28 , 29 , to develop an improved version named China-TIMES-MCA (Fig. 1 ) and quantify the effects of technological development and cumulative carbon budget uncertainty. With the help of the advanced data analysis tools, the model delineates numerous possible sectoral decarbonization pathways. The results reflect the unique impacts of China’s cumulative carbon budget and emission peak time on technology choice and transition costs. Over 160 GW per year of variable renewable energy on average must be deployed by 2050, irrespective of the cumulative carbon budget. Hydrogen and bioenergy with carbon capture and storage (BECCS) should be deployed on a large scale after 2040. The installed capacity and cost changes associated with these technologies significantly impact the transition cost. Deep decarbonization brings about shifts in production and consumption patterns, and the significant synergistic effects of CO 2 mitigation on air pollutant reductions are observed. Based on the model results, we urge enhanced policy, decisive actions, further technological innovation, and cross-sectoral collaboration in the future.
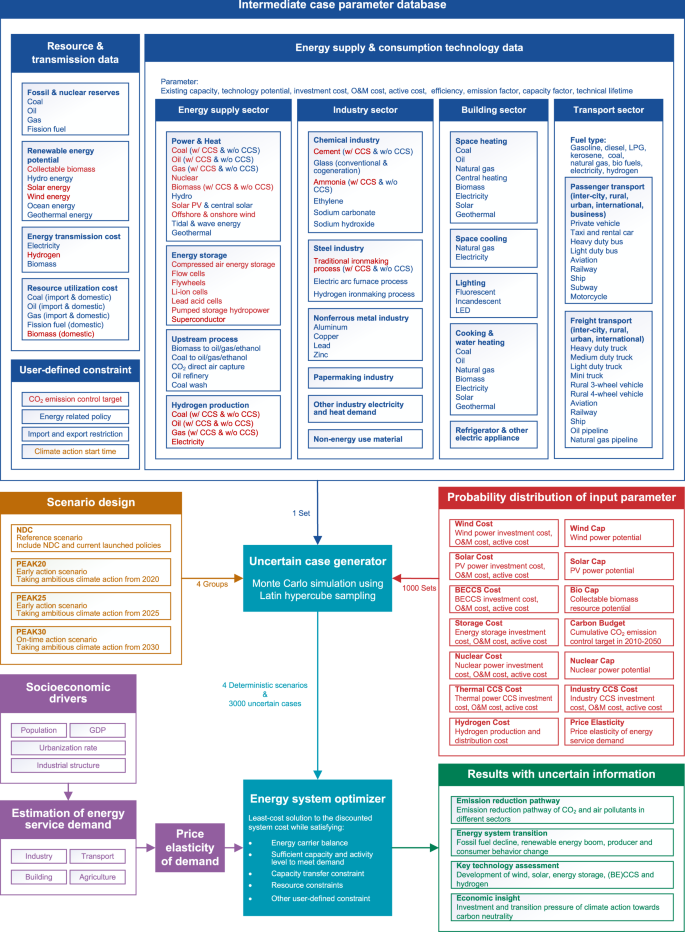
This figure shows the necessary information about the China-TIMES-MCA model. The cyan part is the uncertain case generator and energy system optimizer. The blue part shows the parameters obtained from the statistical data and literature for the intermediate case. The brown part illustrates the scenario design of the study. The purple part is the end-use demand considering price elasticity. The red part is the fourteen input parameters with the corresponding probability distributions. The green part is the model result, which corresponds to the main findings in this paper. In this figure, w/ CCS means that this technology is equipped with carbon capture and storage, while w/o CCS means that it is not equipped.
CO 2 emission reduction pathways
CO 2 emissions are the bulk of China’s GHG emissions and the focus of this study. Three mitigation scenarios (PEAK20, PEAK25, and PEAK30) with different emission peak times are designed with 1000 cases each to highlight the urgency of an early peak. Series of least-cost technology and fuel mixes that meet the energy service demand are obtained. Figure 2 reports the variations in CO 2 emission reduction pathways resulting from the differences in the peak time and cumulative emissions. If the existing NDC target is tracked, emissions will peak at 10.4 GtCO 2 in 2030 and then decline steadily to 7.3 GtCO 2 in 2050, with China’s cumulative emissions totaling 381.1 GtCO 2 from 2010 to 2050. Deep decarbonization is required to reach emission pathways consistent with the carbon neutrality goal. If no further climate action is taken by 2030 (the PEAK30 scenario), then a plunge in emissions between 2030 and 2050 is inevitable, accompanied by −0.9 to 1.6 GtCO 2 of emissions in 2050. The cumulative emissions of the feasible cases (675 out of 1000) are all above 259 GtCO 2 , suggesting that it will be difficult to reach the 1.5-degree goal without substantial negative emission technologies (NETs) in the second half of this century, which is in line with previous multimodel comparison results 30 , 31 . Peaking earlier and lower can alleviate the pressure associated with the subsequent transition, but also frames the great challenge of near-term mitigation actions. For the pathway with an emission peak at 10.3 GtCO 2 in 2025, we estimate an emission reduction of 18% (over a 13–23% range) over 2025–2030 and the majority of the cases will achieve near-zero emissions (−0.9 to 2.7 GtCO 2 ) in 2050. Peaking in 2020 corresponds to the most intense near-term pressure with a 20–33% reduction from NDC in 2030, but almost no net-zero emissions are required in 2050 to support a cumulative emission of 240 GtCO 2 in the PEAK20 scenario. A paradox can be observed in the trade-off between early mitigation actions (greater mitigation contribution) and long-term dependence on NETs (earlier achievement of carbon neutrality).
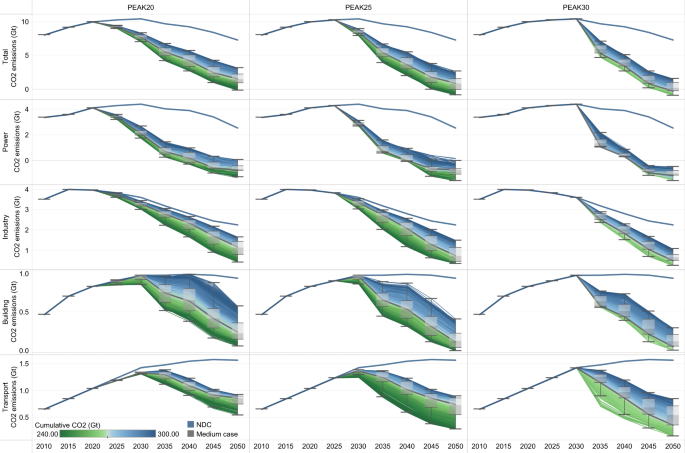
The box plot shows the first quantile, intermediate range (IQR), and third quantile of all the results, where the data range within 1.5 times the IQR is denoted with whiskers. The thick blue line represents the emission pathway of the NDC scenario, and the thick gray line represents the emission pathway for the intermediate case in each scenario. The divergent color from blue to green reflects the increasing stringency of the cumulative carbon budget. The cumulative CO 2 parameter corresponds to the absolute value of China’s cumulative carbon budget for 2010–2050.
These aggregate pathways, however, neglect the dynamics of the emission reduction processes among sectors. Taking the emission peak time as an example, the power sector, the current largest emitter, has the greatest near-term mitigation potential, and the timing of its peak determines the timing of the total CO 2 emissions peak. Except fossil fuel and industrial (FFI) emissions from the industry sector which might reach a peak at 4.6 GtCO 2 by 2020 (of which 4.0 GtCO 2 are energy-related emissions), the emissions from the building sector and transport sector are expected to peak in 2025–2030 at 0.8–1.0 GtCO 2 and in 2030–2035 at 1.3–1.4 GtCO 2 , respectively. After peaking, we observe steep emission reductions from the power sector until 2035 with little variation across cases. Although the emission peak time of the power sector varies considerably in different scenarios, one highly consistent finding is that power sector emissions typically become negative in 2040–2045, with emissions of −1.3 to 0.1, −1.6 to 0.2, −1.6 to −0.5 GtCO 2 in 2050 for the PEAK20, PEAK25, and PEAK30 scenarios. This finding highlights the need to decarbonize the power sector in a timely manner. The later we act, the less time we have left to transition the power system. Turning to the demand sectors, the motivation to reduce emissions is mainly from cumulative emission limitations, and a remarkable spectrum of emission reduction pathways exists. Through the development of alternative materials, popularization of carbon capture and storage (CCS) technology and declining demand for energy-intensive products, as seen from the result for 2050, energy-related emissions from the industry sector will drop to 0.4–1.7 (PEAK20), 0.3–1.5 (PEAK25), and 0.3–1.1 GtCO 2 (PEAK30), and industrial process emissions will decrease by 72–91% compared to the emissions in 2020 to as low as 0.1 GtCO 2 . The building and transport sectors have quite different statuses. Sluggish growth in building floor area and a high electrification rate in the building sector, while the continuous growth of the transport demand and less than 4% electrification rate in the transport sector, has resulted in very divergent development trends. The later the action is taken, the greater the range of 2050 emissions in the building sector (0.1–0.6 GtCO 2 for PEAK20 and 0.0–0.3 GtCO 2 for PEAK30), while the opposite is true for the transport sector (0.6–0.9 GtCO 2 for PEAK20 and 0.2–0.9 GtCO 2 for PEAK30). It is also worth noting that the share of transport sector emissions amongst all demand sectors will rise from 18% in 2020 to 46% (over a 32–59% range) in 2050 under the PEAK25 scenario, bringing it close to or above the industry sector’s share. This finding suggests that the building sector needs to act faster than the transport sector in the near term, but transport sector emission reductions require full attention in the long term.
For China’s carbon neutrality goal of net-zero GHG emissions from all economic sectors by 2060, a significant reduction in non-CO 2 GHGs is imperative. Given the extreme uncertainty of land use, land-use change and forestry (LULUCF) emissions, China’s CH 4 , N 2 O and F-gas emissions, excluding LULUCF, were 2.4 GtCO 2 e in 2020, and at least a 50% reduction is needed by 2050 to meet stringent climate targets 32 . Since non-CO 2 GHG emissions are considered difficult to completely remove based on current perceptions, more ambitious CO 2 reductions are required to achieve a 1.5-degree and China’s carbon neutrality target.
Energy supply decarbonization
Behind the emission reductions are profound changes in the energy system, especially in the energy supply sector. The consistent results of all scenarios reflect that the basis of China’s energy system transformation is eliminating fossil fuel consumption and substantially increasing the use of new and renewable energy (Fig. 3 ). Considering the PEAK25 scenario as an example, both primary energy and coal consumption peak in 2025 and then fall rapidly. The share of renewable energy in primary energy supply rises from 10% in 2020 to nearly 28% (range 22–34%) in 2035 and then further to 59% (range 47–78%) in 2050, with photovoltaic (PV) and wind power playing unique roles. The elimination of fossil fuels occurs faster in PEAK20 than in PEAK25 and slower in PEAK30 than in PEAK25.
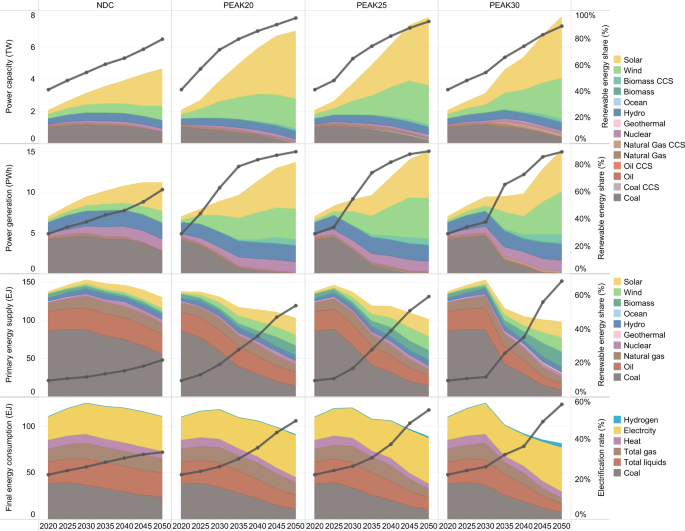
For the power installed capacity, the stacked area chart shows different power plant types, and the black line represents the proportion of the renewable energy contribution to the total capacity. For the power generation, the stacked area chart shows the annual power generation of different types of power generation technologies. The black line represents the proportion of renewable energy power generation to the total power generation. In the legend, the fuel names ending in CCS indicate power plants equipped with CCS, and otherwise indicate power plants without CCS. For the primary energy mix, the stacked area chart represents different types of primary energy supply, and the black line represents the renewable energy share. The calorific value calculation method is applied for primary energy statistics. For the final energy mix, the stacked area chart shows the end-use of different energy types, and the black line represents the electrification rate.
Beyond wind and solar power, nuclear power and CCS technologies are considered promising solutions to achieve a zero-carbon energy system in the future. To better understand the effects of the large uncertainties associated with these technologies themselves or externally on their development, we designed elaborate Monte Carlo simulations. Figure 4 shows the technology development trends and the uncertain influential factors. Figure 5 shows the probability distributions. In 2030, the installed capacity of wind and solar reaches 1.2 TW under the NDC scenario and ~2 TW under the PEAK20 and PEAK25 scenarios, suggesting that renewable energy development is not on track for achieving carbon neutrality based on the current NDC target. In 2050, the explosive growth of solar (4.3 TW, for a 3.0–5.5 TW range) and wind (2.4 TW, for a 1.9–3.1 TW range) will lead to ~90% of the installed capacity and electricity output of the power system from renewable sources. The solar and wind capacities are affected by the national cumulative carbon budget, investment cost, and economic potential of resources (Fig. 4a–d ). Since PV and wind power are in high demand in all mitigation scenarios, they remain the largest investment component in the energy supply sector, despite their rapidly declining costs. From 2020 to 2050, for the 25 th –75 th percentile cases in the PEAK25 scenario, 1.5–1.8 and 2.7–3.1 trillion US dollars are required for investment in PV and wind power. If emissions peak in 2020, investments in PV and wind power will need to increase by 19–25% over the next 10 years compared to those for PEAK25, with the interquartile range spreading between 1.3 and 1.4 trillion US dollars. Additionally, we note that the elasticity of the PV and wind capacities to all uncertain input parameters are lower for the PEAK30 scenario than for the other two scenarios, suggesting that the early peak gains time for energy supply decarbonization, thereby relieving pressure on extensive wind and solar deployment beyond 2030.
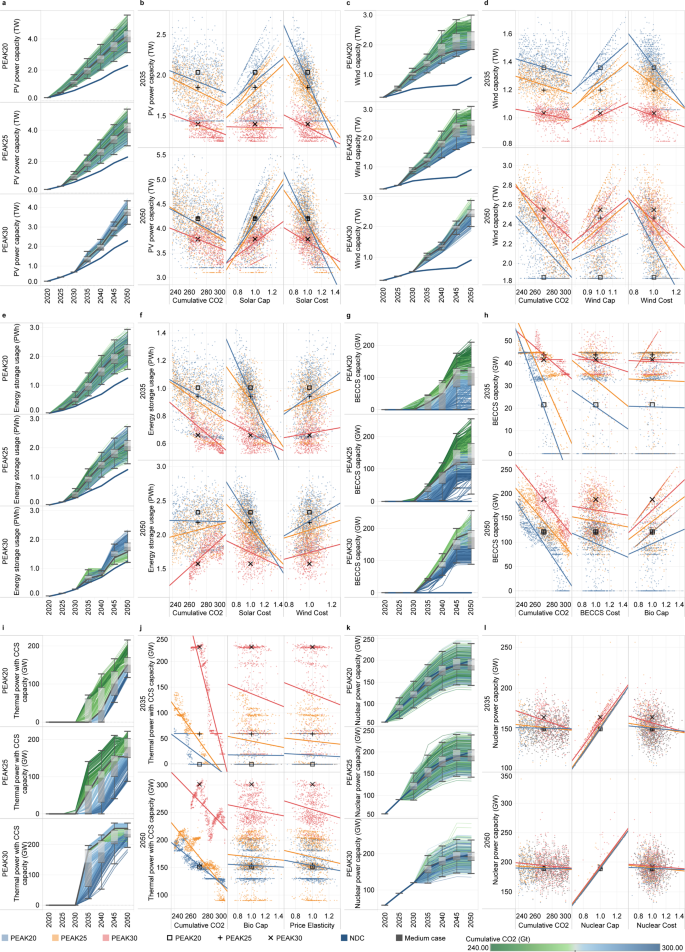
a The PV power installed capacity (unit: TW). b The three most critical uncertain parameters for PV power. c The wind power installed capacity (unit: TW). d The three most critical uncertain parameters for wind power. e The annual energy storage technology usage (unit: PWh). f The three most critical uncertain parameters for annual energy storage technology usage. g The BECCS power installed capacity (unit: GW). h The three most critical uncertain parameters for BECCS power. i The thermal power with CCS installed capacity (unit: GW). j The three most critical uncertain parameters for thermal power with CCS. k The nuclear power installed capacity (unit: GW). l The three most critical uncertain parameters for nuclear power. The box plot shows the first quantile, intermediate range (IQR), and third quantile of all the results, where the data range within 1.5 times the IQR is denoted with whiskers. The thick blue line represents the pathway of the NDC scenario, and the thick gray line represents the pathway for the intermediate case in each scenario. The divergent color from blue to green reflects the increasing stringency of the cumulative carbon budget. The cumulative CO 2 parameter corresponds to the absolute value of China’s cumulative carbon budget for 2010–2050. The scatter plots depict the relationship between the development of each technology and uncertain input parameters, as fitted by a linear function. Three parameters with significant impacts among the fourteen uncertain input parameters are shown for each technology. The remaining results are shown in Supplementary Figs. 5 – 10 . The variables (except for the cumulative carbon budget) represent multiples of the intermediate cases for each scenario. The intermediate cases of PEAK20, PEAK25, and PEAK30 are denoted with square, plus, and multiplication signs, respectively. PV photovoltaic, BECCS bioenergy with carbon capture and storage, CCS carbon capture and storage.
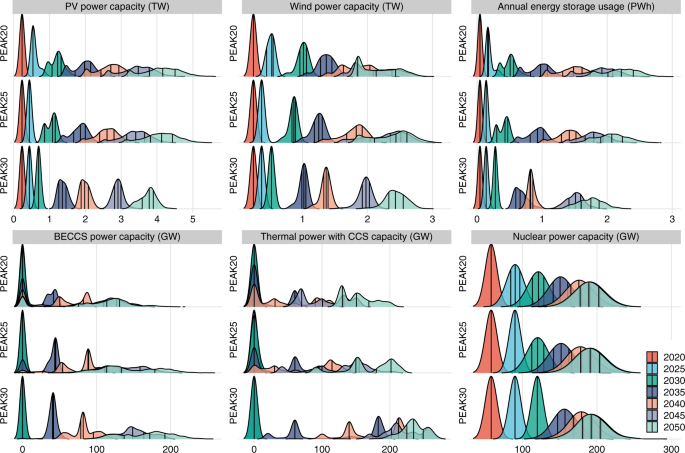
Figure shows the kernel density estimation results for the PV power installed capacity (unit: TW), wind power installed capacity (unit: TW), annual energy storage technology usage (unit: PWh), BECCS power installed capacity (unit: GW), thermal power with CCS installed capacity (unit: GW) and nuclear power installed capacity (unit: GW). The black line in the graph represents the first quantile, intermediate range (IQR), and third quantile for all the cases. PV photovoltaic, BECCS bioenergy with carbon capture and storage, CCS carbon capture and storage.
The high penetration of variable renewable energy creates an enormous demand for energy storage. Figure 4e and f show that energy storage usage rapidly increases until 2045, followed by a slowdown in growth to ~15% of total generation in 2050, requiring 183–220 billion US dollars in investment during 2020–2050. The complementary relationship between energy storage technologies and PV power is shown in Supplementary Fig. 1 , and an interesting shift in the role played by wind power is observed in our study. Wind power and PV power with energy storage have a clear complementary relationship, jointly replacing fossil fuel before 2040. After 2040, wind power competes with PV power with energy storage for the night-time load supply (Supplementary Figs. 1 and 2 ). We also note that hydrogen production and electric vehicle (EV) charging might affect the load characteristics, such that load management can significantly reduce the demand for energy storage.
Rapid development in renewable energy is projected to occur with the phase-out of coal. If emissions do not peak before 2030, the coal share in primary energy supply needs to sharply decline from 57% in 2030 to 10% in 2050. Significant heterogeneity between power generation and the installed capacity reflects the rapidly declining capacity factor of coal-fired power, from 0.4 in 2025, to 0.3 in 2030 and 0.1 in 2035, further exacerbating the urgent issue of thermal power retirement and transition. The cautionary tale is that coal-fired power is rebounding at a time when coal-fired power needs to be controlled for development. Compared to pre-pandemic levels, coal-fired power generation growth in China outpaced wind and solar power in the first half of 2021, and China’s energy supply sector did not achieve “green recovery”, as hoped 33 . With 292 GW of new coal-fired power plants currently announced, permitted, shelved, and under construction in China 34 , stakeholders need to reassess the long-term risks involved.
Carbon lock-in and asset impairment issues for existing coal-fired power units can be effectively mitigated through flexibility retrofits, carbon capture retrofits, or upgrading to BECCS units 35 . BECCS retrofits can be made to existing thermal power plants, and valuable negative emissions can be generated. Due to the high investment and operation cost, BECCS deployment depends largely on the national cumulative carbon budget (Fig. 4g, h ). For mitigation scenarios, the application of BECCS begins in 2035 and is promoted on a large scale after 2040. Weak near- and mid-term action will cause BECCS reliance, as reflected by a comparatively higher capacity and an earlier start of large-scale deployment. Our estimate suggests that the full utilization of biomass resources suitable for power generation can generate the maximum negative emissions of 1.6 GtCO 2 (Supplementary Figs. 3 and 4 ). Increasing upfront action would reduce the biomass demand to half of the biomass potential. Changes in the biomass potential and BECCS investment cost can significantly influence the prospects for BECCS technology development. Despite being a stop-gap measure, thermal power with CCS also displays growth opportunities beyond 2035, providing power system flexibility and some heating needs. The development of thermal power with CCS units corresponds to smooth gradient of increasing capacity while decreasing the cumulative carbon budget (Fig. 4i, j ). For the 25 th –75 th percentile cases, thermal power with CCS capacity reaches 151 GW (over a 130–169 GW range) for PEAK20 and 154 GW (over a 150–202 GW range) for PEAK25 in 2050, most of which is retrofitted from existing thermal power. For the PEAK30 scenario, 225–249 GW of thermal power with CCS is needed in 2050, and most of it will be gas-fired power with CCS to further reduce emissions.
Nuclear power, with a high capacity factor and excellent supply reliability, will grow rapidly in the next 20 years as the ballast of the power system. There are 31 coastal sites and 46 inland sites available for nuclear power construction; in other words, ~200 GW and ~250 GW of coastal and inland nuclear power can be built 36 . Limited site location is a major constraint of nuclear power development (Fig. 4k, l ). For the intermediate cases in all scenarios, the installed capacity of coastal nuclear power reaches 176 GW in 2040, which is 3.5 times higher than that in 2020. By 2050, the installed capacity rises slightly to 190 GW, and the sites available for nuclear power construction along the coast are largely built out. Variation among cases is the result of the choice of nuclear unit capacity at each site. The results also show that inland nuclear power has the potential to reach a modest scale of construction (less than 150 GW) if extremely stringent cumulative emission targets are established, renewable energy development is hindered, and nuclear power costs rapidly decline in the PEAK30 scenario. Moreover, in China’s most recent 14 th Five-Year Plan, inland nuclear power development is not discussed. Thus, the future advancement of inland nuclear power may be limited.
Through an analysis of a large number of cases, the following robust findings were obtained. First, renewable energy should and will grow fastest among the energy supply technologies in mitigation scenarios compared to the NDC scenario (without further policies). Second, after 2030, coal-fired power without CCS will rapidly diminish, and BECCS will gradually gain popularity after 2035 and become crucial by the middle of this century. Third, thermal power with CCS and energy storage technologies that can provide power system stability and reliability in the future will receive increased development attention. Fourth, with the exception of nuclear power, which has limited potential due to siting constraints, all other technological developments are greatly influenced by the commitment to climate action.
Energy demand decarbonization
While the energy transition is more urgent in the energy supply sector, the transitions in the demand sectors cannot be downplayed. Production mode and consumption pattern shifts, technological efficiency improvements and fuel substitution are the three pillars of decarbonization in the demand sectors.
As a result of the first two factors, final energy consumption peaks by 2030 in all scenarios (Fig. 3 ). The production mode strongly influences the industry sector’s energy service demand, and the consumption pattern or consumer behavior heavily impacts the energy service demand in the building and transport sectors. The decline in energy service demand is indicative of shifts in production modes as well as changes in consumption patterns (Fig. 6 ). For example, for the 25 th –75 th percentile cases in the PEAK30 scenario compared to those in the NDC scenario, due to industrial restructuring, energy-intensive industry production decreases rapidly, with cement and glass falling by 15–19%, steel by 11–19%, chemicals by 23–30%, and non-ferrous metals by 20–28% in 2050. The useful energy demand for heating and cooling in 2050 is estimated to decrease by 13–21% due to energy conservation technology improvements, building envelope upgrades, and modest per-capita floor space growth. The turnover of light-duty vehicles in 2050 could decrease by up to 9% in reference to the trend in the NDC scenario. Uncertainty in price elasticity has a greater impact on industry and transport sectors but a smaller impact on the building sector probably due to the comparatively longer life span of equipment in the building sector. The closer we get to carbon neutrality, the greater the uncertainty of demand changes. This result stems from stringent climate policies increasing the cost of energy services in high-emitting sectors, thus reducing the demand due to the price rise; additionally, as society has evolved, there have become more alternative options to meet energy service demands, thus significantly increasing price elasticity. More elastic demand, while reducing energy consumption and the costs of achieving deep emission reductions to a greater extent, entails a faster rise in welfare losses (Supplementary Fig. 11 ). The transition caused by price changes is far from comparable to that caused by social changes, and a significant social cost could be observed. Profound changes in lifestyles and consumption concepts due to remote work, information connectivity, and the sharing economy will aid in decarbonizing energy consumption.
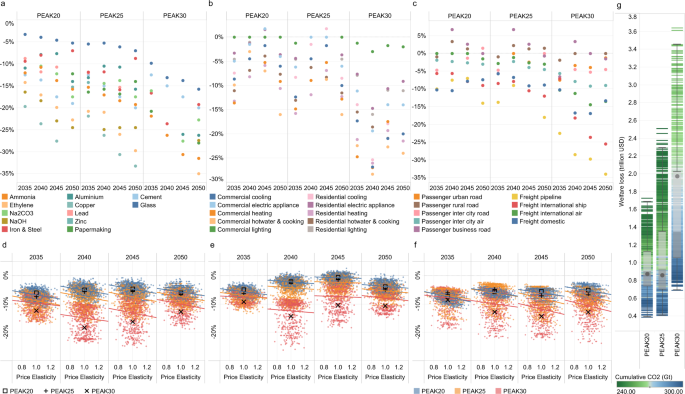
a–c show the declines in the rate of energy service demand for the industry, building, and transport sectors due to price elasticity relative to the NDC scenario, respectively. d–f represent the relationship between the energy service demand for the industry, building, and transport sectors and uncertain price elasticity, respectively. The intermediate cases of PEAK20, PEAK25, and PEAK30 are denoted with square, plus, and multiplication signs, respectively. g summarizes the total discounted welfare loss relative to the value in the NDC scenario for 2020–2050 (unit: trillion US dollar). The black points in panel g denote the welfare loss for the intermediate case in each scenario. The box plot shows the first quantile, intermediate range (IQR), and third quantile of all the results, where the data range within 1.5 times the IQR is denoted with whiskers. The divergent color from blue to green reflects the increasing stringency of the cumulative carbon budget. The cumulative CO 2 parameter corresponds to the absolute value of China’s cumulative carbon budget for 2010–2050. Na 2 CO 3 sodium carbonate, NaOH sodium hydroxide.
Electricity and hydrogen are commonly recognized as alternative energy sources in energy demand sectors. Electricity substitution is the major way to decarbonize all demand sectors. Under the PEAK25 scenario, the electrification rate reaches 55% (range 45–62%) in 2050, where the highest contributions are from electric vehicles in roadway passenger transport (from 2% today to 93% in 2050), electric space heating (from 17% today to 85% in 2050), and electric furnace steel production (from 11% today to 60% in 2050). The large gap between the present and the future suggests that electrification should be accelerated and enhanced in the future, as noted in the literature 37 . Although many studies have suggested that hydrogen is an indispensable option for decarbonization in a short period of time, different studies have not yet reached a consistent conclusion on the quantities of hydrogen energy that can be used in the demand sectors, mainly due to the high production costs and high infrastructure investments required for hydrogen transportation and distribution. Figure 7 reports our uncertainty analysis for hydrogen energy. First, we find that climate goals are the most important factors that influence the expansion of hydrogen energy. Specifically, the use of hydrogen energy exhibits a significant exponential relationship with CO 2 emissions, and a decrease in the cost of hydrogen energy can increase the demand for hydrogen energy to some extent. In 2050, hydrogen consumption (excluding industrial feedstock) ranges from 0.4–2.3 EJ (PEAK20), 0.4–5.4 EJ (PEAK25) and 0.4–6.0 EJ (PEAK30). The later the emission peak is reached, the lower the emissions in 2050, and the higher the demand for hydrogen energy. Second, unless the cost of electrolytic hydrogen production falls more than expected (70% lower than today by 2050), hydrogen energy will gain momentum primarily in areas lacking low-cost abatement options. For example, more than 80% of hydrogen energy is used in the transport sector, with approximately 10% used in the industry sector, and the rest used in the power and building sectors. For the 25 th –75 th percentile cases in the PEAK25 scenario, hydrogen fuel cell vehicles account for 33% (over a 10–64% range) of roadway freight transport (Supplementary Fig. 13 ), and hydrogen direct reduced iron (DRI) technology accounts for 8% (over a 4–37% range) of iron production (Supplementary Fig. 14 ). For the PEAK25 scenario, although hydrogen-powered aircraft may emerge after 2035, even under the strictest carbon budget constraints, the corresponding share will not exceed 41% in 2050 (Supplementary Fig. 15 ). Third, for the source of hydrogen, the model yields very consistent results. In China, electrolytic hydrogen production from renewable energy sources (green hydrogen) will become mainstream.
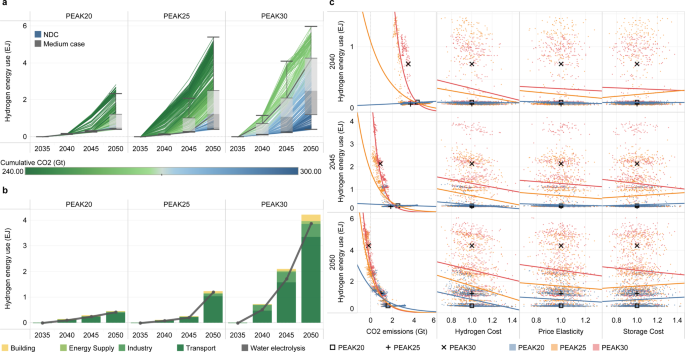
a shows the development of hydrogen energy (unit: EJ). The box plot shows the first quantile, intermediate range (IQR), and third quantile of all the results, where the data range within 1.5 times the IQR is denoted with whiskers. The thick blue line represents the pathway of the NDC scenario, and the thick gray line represents the pathway for the intermediate case in each scenario. The divergent color from blue to green reflects the increasing stringency of the cumulative carbon budget. The cumulative CO 2 parameter corresponds to the absolute value of China’s cumulative carbon budget for 2010–2050. b presents a decomposition of the different uses of hydrogen energy (stacked bar chart) and the production of hydrogen from water electrolysis (black line) for the intermediate cases. c represents the relationship between the development of the hydrogen energy and uncertain input parameters and CO 2 emissions. Three parameters with significant impacts among the fourteen uncertain input parameters are shown for each technology. The remaining results are shown in Supplementary Fig. 12 . The variables (except for the CO 2 emissions) represent multiples of the intermediate cases for each scenario. The intermediate cases of PEAK20, PEAK25, and PEAK30 are denoted with square, plus, and multiplication signs, respectively.
For the steel and cement industries, carbon capture and storage (CCS) technology is a low-cost option for decarbonization. In 2050, the CCS penetration rate in ironmaking reaches 67% (over a 2–80% range) and 77% (over a 25–86% range) of cement production applies CCS technology in the PEAK25 scenario (Supplementary Figs. 14 and 16 ). Since CCS technologies require the extensive construction of pipelines and infrastructure, given the high expectations for CCS technology in both the energy supply and industry sectors, the appropriate layout of the pipeline network and commercial promotion is essential for CCS popularization.
Transition costs and co-benefits
Understanding that the energy transition will create a huge need for investment and technological innovation that drives economic growth, but also brings economic burden and welfare loss. The aggregate results show that the energy supply sector requires an investment of 4.9–7.8 trillion US dollars during 2020–2050 to kickstart the zero-carbon transition, representing an increase of at least 65% over that in the NDC scenario (Fig. 8 ). The 2020–2030 investment will increase, by 43% (over a 21–67% range), if emissions in China peak in 2025 rather than in 2030, but the welfare loss of more than five times the new investment can be avoided (Fig. 6g and Supplementary Fig. 17 ). Furthermore, if emissions in China peak in 2020, for the 25 th –75 th percentile cases, the energy supply sector requires a 99–162 billion US dollars investment increase in the next decade compared to that in PEAK25, which is still a huge jump despite investment reduction in coal-fired power.
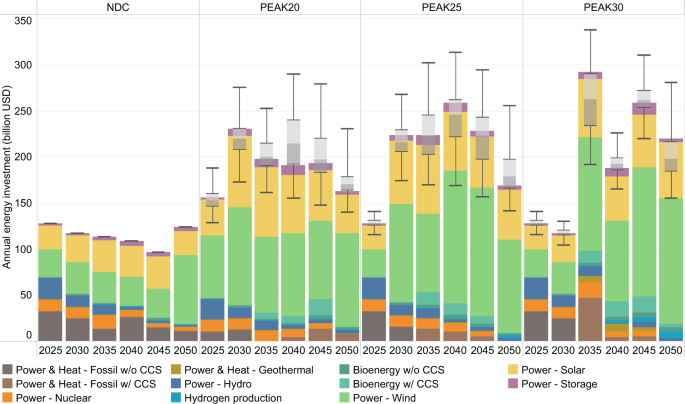
The annual investment in biomass utilization, hydrogen production, energy storage, fossil fuel power generation and heating, solar power generation, wind power generation, and other renewable energy power generation from 2020 to 2050 for the intermediate case in each scenario is shown in the stacked bar chart. The box plot represents the uncertainty range of annual total energy supply investment. The box plot shows the first quantile, intermediate range (IQR), and third quantile of all the results, where the data range within 1.5 times the IQR is denoted with whiskers. The uncertainty in the cumulative investment for each type of technology for 2010–2050 is presented in Supplementary Fig. 17 . In this figure, w/ CCS means that this technology is equipped with carbon capture and storage, while w/o CCS means that it is not equipped.
Considering the whole energy system, on average, the cumulative GDP loss for 2020–2050 due to energy system investment, maintenance and operation costs in the mitigation scenarios is 3.3–3.6%, compared to 3.2% for the NDC scenario. Significant reductions in operating costs offset most of the increasing investment costs. The small increase in total energy system costs also suggests that China’s energy transition is achievable.
As illustrated in Fig. 6g , the welfare loss reflects the utility changes of end users. Similar values of welfare loss are observed for the PEAK20 (0.4–1.7 trillion US dollars) and PEAK25 (0.4–2.3 trillion US dollars), and these values are significantly lower than the 1.9 trillion US dollars (over a 0.7–3.5 trillion US dollar range) for PEAK30. Such high growth in welfare loss for PEAK30 is due to insufficient emission reductions before 2030 compared to those in the other two scenarios, leading to excessive pressure to meet the national cumulative carbon budget, requiring not only the considerable deployment of advanced technologies but also a massive decline in the energy service demand. Combining the results of investment and welfare losses, we find that an earlier peak aids in achieving a complete energy transition and reduces the transition burden, resulting in a win-win for both economic growth and climate governance.
We find that the marginal abatement cost (narrowly defined as the social cost of carbon), is subject to significant uncertainty (Fig. 9a, b ). The interquartile range of the marginal abatement cost is 89–251 US dollars in 2035 and 174–492 US dollars in 2050 under the PEAK30 scenario but is below 151 and 258 US dollars in 2050 for PEAK20 and PEAK25, respectively. The PEAK20 scenario has the lowest marginal abatement cost in 2050, mainly because the substantial effort in the short term reduces the pressure for subsequent mitigation. Figure 9c shows that the marginal abatement cost increases exponentially with the abatement rate. The marginal abatement cost is often equivalent to the optimal carbon price, and therefore policymakers can observe the carbon price needed to achieve the corresponding level of emission reductions in different periods. As shown in Supplementary Fig. 18 , the PEAK30 scenario is significantly more sensitive to future technological potential and costs than the other two scenarios. Cost reduction in CCS and hydrogen energy, can significantly reduce the marginal abatement costs in 2050, reflecting the importance of technological innovation in reducing the policy costs of climate governance.
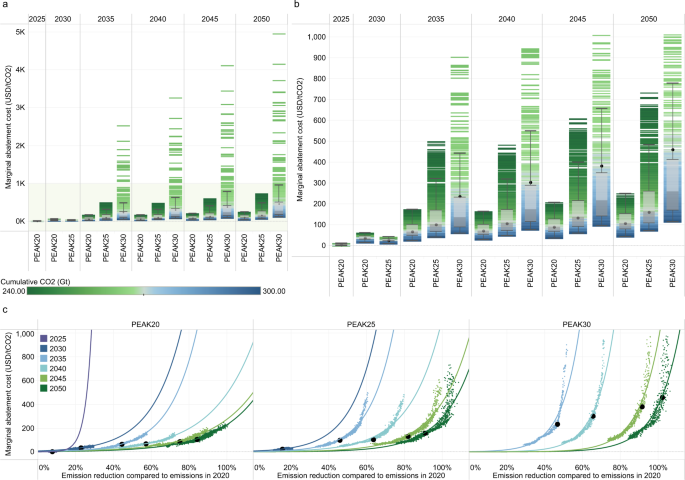
a represents the marginal abatement cost of all feasible cases. b enlarges the concentrated data in panel a (green shadow) corresponding to all cases with carbon prices below 1,013 US dollars per ton CO 2 by 2050 (90% of the total feasible cases for PEAK30). c Relationship between the marginal abatement cost and the CO 2 emission reduction in reference to emissions in 2020 (as fitted by an exponential function). The black points represent the marginal abatement costs for the intermediate cases in each scenario. The box plot shows the first quantile, intermediate range (IQR), and third quantile of all the results, where the data range within 1.5 times the IQR is denoted with whiskers. The divergent color from blue to green reflects the increasing stringency of the cumulative carbon budget. The cumulative CO 2 parameter corresponds to the absolute value of China’s cumulative carbon budget for 2010–2050. Supplementary Fig. 18 shows the relationships between the marginal abatement cost and other uncertain parameters.
Energy transitions have created synergies in local air pollutant reduction. Even without further air pollutant control measures and relying on carbon emission reductions alone, PEAK25 is associated with decreases in the emissions of sulfur dioxide (SO 2 ), nitrogen oxide (NO X ), particulate matter with an aerodynamic diameter no greater than 10 μm (PM 10 ) and particulate matter with an aerodynamic diameter no greater than 2.5 μm (PM 2.5 ) by 14–27%, 18–26%, 13–23% and 12–22%, respectively, in 2030 compared to the present levels, while the corresponding reductions in PEAK30 are only 6%, 12%, 7% and 7%. In 2050, local air pollutant emissions for almost all scenarios fall to only one-fifth of the current values (Fig. 10 ). The results are very consistent with the previous study’s result when carbon neutrality is achieved 38 .
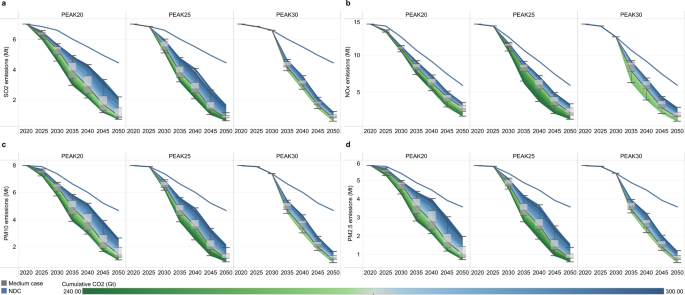
a – d shows sulfur dioxide (SO 2 ), nitrogen oxide (NO X ), particulate matter with an aerodynamic diameter no greater than 10 μm (PM 10 ) and particulate matter with an aerodynamic diameter no greater than 2.5 μm (PM 2.5 ) emissions, respectively. The control measures of SO 2 , NO X , PM 10 and PM 2.5 are based on the current levels; that is, only considering the synergy of CO 2 emission reduction, not considering the effects of subsequent local air pollutant control measures. The box plot shows the first quantile, intermediate range (IQR), and third quantile of all the results, where the data range within 1.5 times the IQR is denoted with whiskers. The thick blue line represents the pathway for the NDC scenario, and the thick gray line represents the pathway for the intermediate case in each scenario. The divergent color from blue to green reflects the increasing stringency of the cumulative carbon budget. The cumulative CO 2 parameter corresponds to the absolute value of China’s cumulative carbon budget for 2010–2050.
A probabilistic representation of technology choice and fuel switching dynamics for the energy transition shows that China’s carbon neutrality goal is not impossible but requires a cross-sectoral, collaborative effort. Although the future of policy and technology development are full of uncertainties, some important consensus can be obtained from this study. Most importantly, decisive action and clear goals enable us to realize carbon neutrality. In particular, clean energy supply, low-carbon energy consumption, and green energy investment go hand in hand on the road to carbon neutrality.
Net-zero emission targets should not distract from the urgent need for near-term emission reductions. Understanding that energy system transition cannot be accomplished overnight, decisive actions facilitate an earlier emission decline, thus buying time for China’s transition to a low-carbon economy at the domestic level. If China’s CO 2 peak occurs in 2025 instead of 2030, welfare losses will be reduced by at least 50%, marginal abatement costs in 2050 will be kept within reasonable limits and heavy reliance on carbon removal technologies will be avoided. At the international level, these actions lay a foundation for China to make an ambitious contribution to mitigating global climate change. With a peak in 2030, it is almost impossible for China to achieve cumulative emissions below 250 Gt in 2010–2050, meaning that either the 1.5-degree target called for in many studies will be difficult to achieve or China will have to generate significant negative emissions for a long time in the second half of this century. Recently, more than 150 parties, including China, submitted their new or updated NDCs, and most have raised their targets to increase their ambition to address climate change. For China, an enhanced NDC would boost climate mitigation while facilitating a domestic transition. It is wise for China to implement the enhanced NDC, aiming to reach an earlier and lower emission peak. Achieving a carbon peak near 2025 with a peak ~10.3 GtCO 2 and declining to ~9 GtCO 2 in 2030, is the possessive choice to combine near-term and long-term transition pressures.
Our study suggests that a concrete and transparent total carbon emission control mechanism can largely reduce uncertainty. Policymakers need to consider setting total emission control targets and cumulative emission targets rather than carbon emission intensity reduction targets alone to encourage progressive emission reductions. Net-zero emission targets alone, while cumulative emissions remain highly uncertain, will ultimately lead to large differences in emission reduction pathways, technology choices and transition costs. For China, an appropriate total carbon emission control target should be established in conjunction with the announced carbon neutrality target in a way that does not impose an excessive burden on society, appropriately carries international responsibility and contributes to the 1.5-degree target.
Key technologies for achieving carbon neutrality have not been fully developed, and it is important to cultivate diverse technologies and build a portfolio of carbon-neutral technology reserves. Innovation in renewables and advanced technologies is a critical backbone of the energy transition. Rapid reductions in PV and wind power costs have already changed the landscape for addressing climate change. Considering the massive demand for variable renewable energy, further technological progress in wind, solar, and energy storage will powerfully impact the transition cost. Hydrogen, biomass, and CCS technologies, which in the past have often been used as back-up resources, will be important pillars of carbon neutrality due to their indispensable roles in a net-zero and carbon-negative world; hence, there is an urgent need for sustained R&D investments and industrial pilots for these high-cost technologies.
While the energy supply sector has considerable potential for emission reduction with the popularization of renewable energy and the elimination of coal in the near term, other sectors still need to take action without delay. The continued elimination of energy-intensive industries and an increase in the value of products are needed in the industry sector. Fuel substitution in the building and transport sectors fundamentally alters energy use patterns and requires public acceptance. Increased public awareness of carbon neutrality helps to consciously adopt a low-carbon lifestyle and reduce energy consumption and the carbon footprint.
The carbon neutrality goal requires us to examine the current financial system and shift investments from carbon-intensive assets to green assets. Investment is the vane of future development. The results have clearly indicated that the existing thermal power is associated with a high risk of capital stranding. Hence, we suggest a moratorium on new thermal power, and thermal power plants under construction should be capture ready. In the context of carbon neutrality, it is imperative to reduce fossil energy investments, support renewable energy expansion, and promote international cooperation. The launch of China’s national emission trading market further reasonably shares the mitigation cost and encourages decarbonization at the enterprise level.
Overall, the significant uncertainties in the emission peak time, technological development and cumulative carbon budget lead to multiple pathways for China towards carbon neutrality. In our study, robust conclusions regarding emission pathways, energy transitions, consumer behavior shifts, transition costs and co-benefits are obtained for policymakers to deploy national mitigation strategies. At present, over 130 countries have announced their carbon neutrality goals, which is a major step forward; however, more attention should be given to near-term actions, emission reduction pathways towards carbon neutrality, and the remaining national cumulative carbon budget required.
China-TIMES model
The China-The Integrated MARKAL-EFOM System (China-TIMES), a dynamic linear programming energy system optimization model, was developed for 5-year intervals extending from 2010 to 2050 on the basis of the China MARKAL model 39 , 40 , 41 . The base year for the China-TIMES model is 2015. The model uses 5% as the discount rate. The model incorporates the full range of energy processes including exploitation, conversion, transmission, distribution and end-use. Over 500 existing and advanced energy supply and demand technologies are introduced in the model. Three demand sectors, industry 19 , 20 , 21 , building (divided into commercial, urban residential and rural residential) 22 and transport 23 , are considered and further divided into over 40 subsectors. Daytime and night-time are modeled separately, and intraday and interseason load differences are fully considered.
For the power sector, we consider different fuel types (coal, oil, gas, wind, solar, nuclear, hydropower, marine, biomass and geothermal). Different thermal power types have different cost and efficiency settings depending on the technology category (ultra-supercritical, supercritical, subcritical, ultra-high pressure, circulating fluidized bed, pressurized fluidized bed, gasification combined cycle, etc.) and cooling method (air cooling, direct water cooling, or circulating water cooling). For renewables, the model includes hydropower, centralized PV, building integrated PV (BIPV), concentrating solar power (CSP), offshore and onshore wind power, biomass energy, geothermal energy, tidal energy, and wave energy at different scales and development levels. In this version of China-TIMES, we refine the description of biomass power generation, which includes biomass gasification, direct combustion, and co-combustion with coal. For thermal and biomass power generation, we consider various CCS technologies available for existing unit retrofits and new construction. The installed capacity, efficiency, and capacity factor of each type of power generation technology are set according to real data from the China Electricity Council.
Stock-based material flow analysis approach, discrete choices method, Gompertz model etc. are used to project energy service demands for the subsectors according to given socioeconomic development scenarios. The socioeconomic driver settings such as the gross domestic product (GDP), population, urbanization level, and industrial structure are listed in Supplementary Table 1 . The projected energy service demands for more than 40 end-use subsectors are met by abundant energy end-use demand technologies, thereby driving energy system optimization. In the industry sector we focused on the production of steel, cement, glass, nonferrous metals, paper, and chemicals (ethylene, sodium hydroxide, sodium carbonate and ammonia) and created process-level descriptions. In addition to emissions from fuel combustion, we considered emissions from the cement production process, which are generated by the decomposition of raw materials. The future penetration of hydrogen, electrical energy and CCS in the industry sector is fully considered. For the building sector, space heating, cooling, cooking, water heating, lighting, and electric appliances are considered. Taking space heating as an example, in addition to modeling different heating methods and heating needs for China’s five climate zones, energy efficient building standards for different periods are also considered. Future clean and efficient technologies, such as solar thermal, geothermal, and air source heat pumps, are also considered. The transport sector is divided into passenger and freight transport, and further split into aviation, navigation, railway, highway (urban, rural, and intercity), and pipeline (for freight); additionally, many different types of vehicles are considered. The model portrays the role of fuel economy improvements, fuel substitution, and travel mode shifts in a balanced way.
Energy storage technologies (including pumped hydro storage, compressed air storage, flywheel storage, and various types of electrochemical storage) can bridge the gap between the energy supply and demand at different times. In this study, we focus on intraday energy storage, but the different characteristics of energy storage technologies in the model and the many demand-side management tools (dynamic hydrogen production, energy demand balancing between seasons, etc.) are able to provide a good understanding of energy storage demand.
Local air pollutants 24 , 25 such as SO 2 , NO X , PM 10 , PM 2.5 as well as energy-related water consumption 26 can also be simulated with the China-TIMES model. The emission factors for CO 2 are set according to IPCC guidelines 42 and remain constant over time. The emission factors for local air pollutants are calibrated with official statistics and kept constant over time to correctly reflect the synergistic effects of CO 2 emission reductions.
China-TIMES-MCA model
The China-TIMES-MCA model (Fig. 1 ) implements coupling with Monte Carlo analysis (MCA) on the basis of the China-TIMES model to perform large-scale uncertainty analysis. We use the Latin hypercube sampling (LHS) method 43 , 44 to replace the random sampling method used in the classical Monte Carlo simulation. LHS is a stratified sampling method that approximates random sampling. Multivariate parameters are sampled using a relatively small number of sampling points to resolve the aggregation problem that can occur in random sampling and ensure that low-probability events are accurately represented in the model.
According to the nature of different parameters, we establish the uniform distribution, lognormal distribution, and normal distribution, while ensuring that the median of each distribution is 1. Different parameters are randomly generated in the same scenario. The sample probability distributions and statistics summary are shown in Supplementary Table 2 . The parameters of cases with the same order number are identical for different scenarios (such as the 1 st case for PEAK20 and the 1 st case for PEAK25), to effectively compare the differences among scenarios.
Generation of uncertain input parameters
We use predetermined probability distributions to model the factors that can significantly impact China’s energy system transformation and climate change mitigation. The fourteen selected key parameters are the cumulative carbon budget (Carbon Budget), technological cost of BECCS (BECCS Cost), utilizable biomass resource potential (Bio Cap), PV power cost (Solar Cost), PV power economic installed capacity (Solar Cap), wind power cost (Wind Cost), wind power economic installed capacity (Wind Cap), energy storage cost (Storage Cost), nuclear power cost (Nuclear Cost), nuclear power economic installed capacity (Nuclear Cap), thermal power with CCS cost (Thermal CCS Cost), industry CCS cost (Industry CCS Cost), hydrogen production, storage and transportation cost (Hydrogen Cost), and price elasticity of energy service demands (Price Elasticity).
Except for the cumulative carbon budget, the parameters of the intermediate case are based on the IEA reports, industry statistics, and existing literature (see Supplementary Table 3 ) 2 , 45 , 46 , 47 , 48 , 49 , 50 , 51 , 52 , 53 . The parameters for other cases are generated using adjustment multipliers based on those used for the intermediate case. The cumulative carbon budget is an important indicator of a country’s contribution to global climate change mitigation. Various studies have shown that considerable uncertainty remains in the remaining carbon budget 54 , 55 . Since China’s carbon budget varies significantly under different allocation principles, we set an uncertain input parameter to reflect the efforts of China’s emission reduction. The carbon budget parameter is the absolute value of China’s cumulative carbon budget from the beginning of 2010 to the end of 2050. The carbon budget parameter considers only energy-related CO 2 emissions and does not include LULUCF emissions. We integrate various fairness principles to construct the carbon budget parameter, which is centered at 270 Gt and uniformly distributed from 240 to 300 Gt, which covers the median carbon quotas for achieving the 2- and 1.5-degree targets 27 , 56 , 57 , 58 , 59 .
Scenario design and simulation
A deterministic reference scenario and three groups of uncertain mitigation scenarios of which 1000 independent cases each are included in this study. The reference for the mitigation scenarios is NDC, which includes China’s NDCs and climate-related policies published before 2020. The three groups of mitigation scenarios are all oriented towards China’s carbon neutrality goal and represent different emission peak times and time at which a carbon neutrality-oriented emission reduction trajectory is observed through from 2020 (PEAK20), 2025 (PEAK25), and 2030 (PEAK30). The same socioeconomic assumptions are used in all scenarios to generate the energy service demand for end-use sectors and subsectors in the China-TIMES-MCA model. This model integrates resource availability, technology cost and technology availability on both the supply and demand sides to seek the lowest cost technology and fuel mix to meet the energy service demand and carbon constraints. The price elasticity of the energy service demand for each end-use demand subsector is introduced to consider emission reductions based on changes in the production mode and consumption pattern. The choice of price elasticity of the intermediate case is referenced from previous study 60 . The early retirement of units in energy-intensive industry sectors, and thermal power and heat generation units without CCS is allowed in the model. On a PC with an Intel i7-9700, 32 GB RAM, and 1 TB SSD, a round of optimization using CPLEX takes 6–8 h, and the performance of the computer and solver can have a significant impact on the computing time. For the PEAK30 scenario, 325 out of 1000 cases are not included because optimization is infeasible.
Reporting summary
Further information on research design is available in the Nature Research Reporting Summary linked to this article.
Data availability
The energy system portion of China-TIMES-MCA was calibrated with the China Energy Statistics Yearbook, which is a public publication. Local air pollutants were calibrated according to the “Second National Pollution Source Census Bulletin”, which can be viewed on the website of the Ministry of Ecology and Environment ( http://www.mee.gov.cn/xxgk2018/xxgk/xxgk01/202006/t20200610_783547.html ). The input data for the key uncertain parameters discussed in the text are disclosed in the Supplementary Information. China-TIMES-MCA model output data generated in this study are provided with this paper in the Source data. Additional data that support the findings of this study are available from the corresponding author upon reasonable request. Source data are provided with this paper.
Code availability
The code and documentation of the TIMES model are open source and available on GitHub ( https://github.com/etsap-TIMES/TIMES_model ). Latin hypercube sampling used for generating uncertain input parameters, model data transfer, model input file generation and some visualization tasks, we used FORTRAN, MATLAB and R, the code can be found at https://doi.org/10.5281/zenodo.5717886 61 . For other visualization tasks, we used Tableau and Visio, with no programming involved.
UNFCCC. Adoption of the Paris Agreement (FCCC, United Nations, 2015).
International Energy Agency. World Energy Outlook 2020 . (International Energy Agency, 2020).
Matthews, H. D. et al. Opportunities and challenges in using remaining carbon budgets to guide climate policy. Nat. Geosci. 13 , 769–779 (2020).
Article CAS ADS Google Scholar
Yalew, S. G. et al. Impacts of climate change on energy systems in global and regional scenarios. Nat. Energy 5 , 794–802 (2020).
Article ADS Google Scholar
Anadón, L. D., Baker, E. & Bosetti, V. Integrating uncertainty into public energy research and development decisions. Nat. Energy 2 , 17071 (2017).
van Vuuren, D. P. et al. The costs of achieving climate targets and the sources of uncertainty. Nat. Clim. Change 10 , 329–334 (2020).
Rogelj, J., Geden, O., Cowie, A. & Reisinger, A. Net-zero emissions targets are vague: three ways to fix. Nature 591 , 365–368 (2021).
Article CAS PubMed ADS Google Scholar
Keilman, N. Modelling education and climate change. Nat. Sustain. 3 , 497–498 (2020).
Article Google Scholar
Huppmann, D., Rogelj, J., Kriegler, E., Krey, V. & Riahi, K. A new scenario resource for integrated 1.5 °C research. Nat. Clim. Change 8 , 1027–1030 (2018).
Mastrandrea, M. D. & Schneider, S. H. Probabilistic integrated assessment of “dangerous” climate change. Science 304 , 571–575 (2004).
Schneider, S. H. & Mastrandrea, M. D. Probabilistic assessment of “dangerous” climate change and emissions pathways. Proc. Natl Acad. Sci. USA 102 , 15728–15735 (2005).
Article CAS PubMed PubMed Central ADS Google Scholar
Bastien-Olvera, B. A. & Moore, F. C. Use and non-use value of nature and the social cost of carbon. Nat. Sustain. 4 , 101–108 (2021).
Gillingham, K. et al. Modeling uncertainty in integrated assessment of climate change: a multimodel comparison. J. Assoc. Environ. Reso. 5 , 791–826 (2018).
Google Scholar
Baležentis, T. & Streimikiene, D. Multi-criteria ranking of energy generation scenarios with Monte Carlo simulation. Appl. Energy 185 , 862–871 (2017).
Marcucci, A., Panos, E., Kypreos, S. & Fragkos, P. Probabilistic assessment of realizing the 1.5 °C climate target. Appl. Energy 239 , 239–251 (2019).
Babonneau, F., Haurie, A., Loulou, R. & Vielle, M. Combining stochastic optimization and Monte Carlo simulation to deal with uncertainties in climate policy assessment. Environ. Model. Assess. 17 , 51–76 (2012).
Chen, W., Yin, X. & Zhang, H. Towards low carbon development in China: a comparison of national and global models. Clim. Change 136 , 95–108 (2016).
Wang, H., Chen, W., Zhang, H. & Li, N. Modeling of power sector decarbonization in China: comparisons of early and delayed mitigation towards 2-degree target. Clim. Change 162 , 1843–1856 (2020).
Yin, X. & Chen, W. Trends and development of steel demand in China: a bottom-up analysis. Resour. Policy 38 , 407–415 (2013).
Li, N., Ma, D. & Chen, W. Quantifying the impacts of decarbonisation in China’s cement sector: a perspective from an integrated assessment approach. Appl. Energy 185 , 1840–1848 (2017).
Article CAS Google Scholar
Chen, W., Yin, X. & Ma, D. A bottom-up analysis of China’s iron and steel industrial energy consumption and CO 2 emissions. Appl. Energy 136 , 1174–1183 (2014).
Shi, J., Chen, W. & Yin, X. Modelling building’s decarbonization with application of China TIMES model. Appl. Energy 162 , 1303–1312 (2016).
Zhang, H., Chen, W. & Huang, W. TIMES modelling of transport sector in China and USA: Comparisons from a decarbonization perspective. Appl. Energy 162 , 1505–1514 (2016).
Li, N. et al. Air quality improvement co-benefits of low-carbon pathways toward well below the 2 °C climate target in China. Environ. Sci. Technol. 53 , 5576–5584 (2019).
Ma, D., Chen, W., Yin, X. & Wang, L. Quantifying the co-benefits of decarbonisation in China’s steel sector: an integrated assessment approach. Appl. Energy 162 , 1225–1237 (2016).
Huang, W., Ma, D. & Chen, W. Connecting water and energy: assessing the impacts of carbon and water constraints on China’s power sector. Appl. Energy 185 , 1497–1505 (2017).
Roelfsema, M. et al. Taking stock of national climate policies to evaluate implementation of the Paris Agreement. Nat. Commun. 11 , 2096 (2020).
Tang, H., Zhang, S. & Chen, W. Assessing representative CCUS layouts for China’s power sector toward carbon neutrality. Environ. Sci. Technol. 55 , 11225–11235 (2021).
Zhang, S. & Chen, W. China’s energy transition pathway in a carbon neutral vision. Engineering , https://doi.org/10.1016/j.eng.2021.09.004 (2021).
Duan, H. et al. Assessing China’s efforts to pursue the 1.5 °C warming limit. Science 372 , 378–385 (2021).
van Soest, H. L., den Elzen, M. G. J. & van Vuuren, D. P. Net-zero emission targets for major emitting countries consistent with the Paris Agreement. Nat. Commun. 12 , 2140 (2021).
Article PubMed PubMed Central ADS Google Scholar
Institute of Climate Change and Sustainable Development Tsinghua University. China’s Long-term Low-carbon Development Strategies and Pathways Comprehensive Report . (China Environment Publishing Group, 2021).
Jones, D., Fulghum, N. & Tunbridge, P. Global Electricity Review: H1-2021 . (Ember, 2021).
Global Energy Monitor. Global Coal Plant Tracker (July 2021) . (Global Energy Monitor, 2021).
Li, J. et al. Unit-level cost-benefit analysis for coal power plants retrofitted with biomass co-firing at a national level by combined GIS and life cycle assessment. Appl. Energy 285 , 116494 (2021).
Xiao, X. & Jiang, K. China’s nuclear power under the global 1.5 °C target: Preliminary feasibility study and prospects. Adv. Clim. Chang. Res. 9 , 138–143 (2018).
Fujimori, S. et al. A framework for national scenarios with varying emission reductions. Nat. Clim. Change 11 , 472–480 (2021).
Cheng, J. et al. Pathways of China’s PM2.5 air quality 2015–2060 in the context of carbon neutrality. Natl. Sci. Rev. , https://doi.org/10.1093/nsr/nwab078 (2021).
Chen, W. The costs of mitigating carbon emissions in China: findings from China MARKAL-MACRO modeling. Energy Policy 33 , 885–896 (2005).
Chen, W., Wu, Z., He, J., Gao, P. & Xu, S. Carbon emission control strategies for China: a comparative study with partial and general equilibrium versions of the China MARKAL model. Energy 32 , 59–72 (2007).
Chen, W., Li, H. & Wu, Z. Western China energy development and west to east energy transfer: application of the Western China Sustainable Energy Development Model. Energy Policy 38 , 7106–7120 (2010).
Intergovernmental Panel on Climate Change. Revised 1996 IPCC Guidelines for National Greenhouse Gas Inventories: The Workbook (Volume 2) . (Intergovernmental Panel on Climate Change, 1996).
Helton, J. C. & Davis, F. J. Latin hypercube sampling and the propagation of uncertainty in analyses of complex systems. Reliab. Eng. Syst. Safe. 81 , 23–69 (2003).
Webster, M. et al. Uncertainty analysis of climate change and policy response. Clim. Change 61 , 295–320 (2003).
China Electricity Council. China Power Industry Annual Development Report 2020 . (China Building Materials Press, 2020).
International Energy Agency. Energy Technology Perspectives 2020 . (International Energy Agency, 2020).
International Energy Agency. Projected Costs of Generating Electricity 2020 . (International Energy Agency, 2020).
International Renewable Energy Agency. Electricity Storage Valuation Framework: Assessing system value and ensuring project viability . (International Renewable Energy Agency, 2020).
Kang, Y. et al. Bioenergy in China: Evaluation of domestic biomass resources and the associated greenhouse gas mitigation potentials. Renew. Sust. Energy Rev. 127 , 109842 (2020).
Hanssen, S. V. et al. The climate change mitigation potential of bioenergy with carbon capture and storage. Nat. Clim. Change 10 , 1023–1029 (2020).
IEA Greenhouse Gas R&D Programme. Towards zero emissions CCS in power plants using higher capture rates of biomass . (IEA Greenhouse Gas R&D Programme, 2019).
IEA Greenhouse Gas R&D Programme. CCS in energy and climate scenarios. (IEA Greenhouse Gas R&D Programme, 2019).
Zhang, P. Evaluation of the Techno-Economics of Nuclear Hydrogen Production using HTGR (China) . (International Atomic Energy Agency, 2018).
Rogelj, J., Forster, P. M., Kriegler, E., Smith, C. J. & Seferian, R. Estimating and tracking the remaining carbon budget for stringent climate targets. Nature 571 , 335–342 (2019).
Rogelj, J. et al. Zero emission targets as long-term global goals for climate protection. Environ. Res. Lett. 10 , 105007 (2015).
Pan, X., Chen, W., Clarke, L. E., Wang, L. & Liu, G. China’s energy system transformation towards the 2 °C goal: Implications of different effort-sharing principles. Energy Policy 103 , 116–126 (2017).
Pan, X., den Elzen, M., Hoehne, N., Teng, F. & Wang, L. Exploring fair and ambitious mitigation contributions under the Paris Agreement goals. Environ. Sci. Policy 74 , 49–56 (2017).
Chen, H. et al. The global impacts of US climate policy: a model simulation using GCAM-TU and MAGICC. Clim. Policy 18 , 852–862 (2018).
Robiou du Pont, Y., Jeffery, M. L., Gütschow, J., Christoff, P. & Meinshausen, M. National contributions for decarbonizing the world economy in line with the G7 agreement. Environ. Res. Lett. 11 , 054005 (2016).
Kesicki, F. & Anandarajah, G. The role of energy-service demand reduction in global climate change mitigation: combining energy modelling and decomposition analysis. Energy Policy 39 , 7224–7233 (2011).
Zhang, S. & Chen, W. Code and data for Nature Communications paper “Assessing the energy transition in China towards carbon neutrality with a probabilistic framework”. Zenodo , https://doi.org/10.5281/zenodo.5717886 (2021).
Download references
Acknowledgements
This study was supported by the National Natural Science Foundation of China (71690243 and 51861135102 to W.C.) and the Ministry of Science and Technology of the People’s Republic of China (2018YFC1509006 to W.C.).
Author information
Authors and affiliations.
Institute of Energy, Environment and Economy, Tsinghua University, 100084, Beijing, PR China
Shu Zhang & Wenying Chen
You can also search for this author in PubMed Google Scholar
Contributions
S.Z. and W.C. conceived the study, performed the analysis, and contributed to writing the paper.
Corresponding author
Correspondence to Wenying Chen .
Ethics declarations
Competing interests.
The authors declare no competing interests.
Peer review information
Nature Communications thanks Fei Guo, Gang He, and the other, anonymous, reviewer(s) for their contribution to the peer review of this work. Peer reviewer reports are available.
Additional information
Publisher’s note Springer Nature remains neutral with regard to jurisdictional claims in published maps and institutional affiliations.
Supplementary information
Supplementary information, peer review file, reporting summary, source data, source data, rights and permissions.
Open Access This article is licensed under a Creative Commons Attribution 4.0 International License, which permits use, sharing, adaptation, distribution and reproduction in any medium or format, as long as you give appropriate credit to the original author(s) and the source, provide a link to the Creative Commons license, and indicate if changes were made. The images or other third party material in this article are included in the article’s Creative Commons license, unless indicated otherwise in a credit line to the material. If material is not included in the article’s Creative Commons license and your intended use is not permitted by statutory regulation or exceeds the permitted use, you will need to obtain permission directly from the copyright holder. To view a copy of this license, visit http://creativecommons.org/licenses/by/4.0/ .
Reprints and permissions
About this article
Cite this article.
Zhang, S., Chen, W. Assessing the energy transition in China towards carbon neutrality with a probabilistic framework. Nat Commun 13 , 87 (2022). https://doi.org/10.1038/s41467-021-27671-0
Download citation
Received : 10 April 2021
Accepted : 30 November 2021
Published : 10 January 2022
DOI : https://doi.org/10.1038/s41467-021-27671-0
Share this article
Anyone you share the following link with will be able to read this content:
Sorry, a shareable link is not currently available for this article.
Provided by the Springer Nature SharedIt content-sharing initiative
This article is cited by
Sustained growth of sulfur hexafluoride emissions in china inferred from atmospheric observations.
- Ronald G. Prinn
- Matthew Rigby
Nature Communications (2024)
Climate change will impact the value and optimal adoption of residential rooftop solar
- Michael T. Craig
Nature Climate Change (2024)
Sustainable astronomy: A comparative life cycle assessment of off-grid hybrid energy systems to supply large telescopes
- Isabelle Viole
- Sabrina Sartori
The International Journal of Life Cycle Assessment (2024)
Public–private partnerships for energy transition: studying role of economic change and energy restructuring over the time
- Shuili Yang
Economic Change and Restructuring (2024)
Urban–rural income gap and economic sustainability: how does energy transition matters?
- Junjiang Li
By submitting a comment you agree to abide by our Terms and Community Guidelines . If you find something abusive or that does not comply with our terms or guidelines please flag it as inappropriate.
Quick links
- Explore articles by subject
- Guide to authors
- Editorial policies
Sign up for the Nature Briefing newsletter — what matters in science, free to your inbox daily.

Morningside Campus Access Updates
Updated May 22, 2024
All Columbia-based affiliates from all campuses, including Morningside, Manhattanville, CUIMC, Baker Athletics Complex, Lamont, and Nevis have access to the Morningside campus. Read more
Explore Our Work
Our work is committed to independent and nonpartisan research that meets the high standards of academic integrity and quality at Columbia University.
- See All Work
- Meet Our Scholars
- Climate Change
- Critical Minerals
- Energy for Development
- Energy Justice
- Energy Markets
- Energy Policy
- Energy & Public Health
- Energy Security
- Finance & Economics
- Fossil Energy
- Geopolitics
- Industrial Decarbonization
- Innovation & Technology
- Natural Gas
- Nuclear Energy
- Power Sector
- Renewable Energy
- Transportation
- Asia + Pacific
- Latin America
- Middle East + North Africa
- Sub-Saharan Africa
- North America
- Op-eds & Essays
- Testimonies & Speeches
- External Publications
Initiatives
Our initiatives and programs are designed to address critical needs in key focus areas around energy and climate policy.
- Women in Energy
- Carbontech Development Initiative (CDI)
- Carbon Management Research
- Critical Materials
- Energy Opportunity Lab
- Energy Systems Modeling
- India Program
- Nuclear for Climate Impact
- Book Series
- All Initiatives
- Distinguished Visiting Fellows
- Columbia Global Energy Fellows
- Energy Journalism Fellows
- Visiting Faculty Program
- All Programs
Partnerships
- The Lancet Countdown 2020
- Future Power Markets Forum
- All Partnerships
Explore our expert insights and analysis in leading energy and climate news stories.
The U.S. Should Stop Playing the Victim Over China Trade
Energy explained.
Get the latest as our experts share their insights on global energy policy.
Gauging the Impact of New US Tariffs on Imports from China
Three CGEP scholars weigh in on the Biden Administration’s recent decision to increase tariffs on imports from China in strategic sectors vital to US economic interests and national...
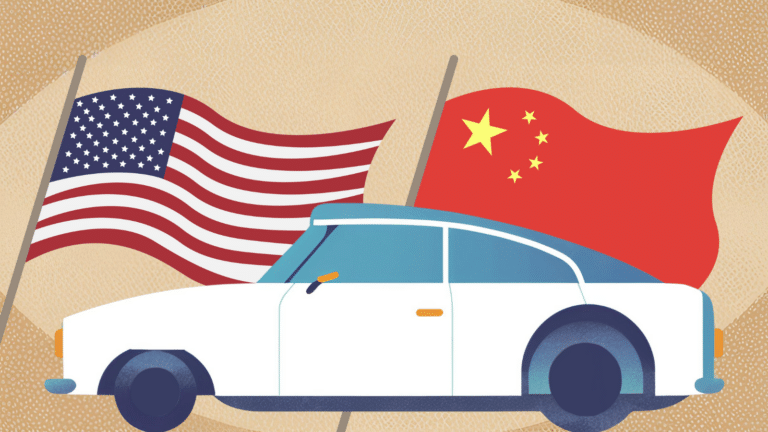
Hear in-depth conversations with the world’s top energy and climate leaders from government, business, academia, and civil society.
Can Industry Eliminate Its Carbon Pollution?
Industry accounts for one-third of global greenhouse gas emissions, a share that will only increase in the coming years. Other high-emission sectors, like electric power and transportation, are...
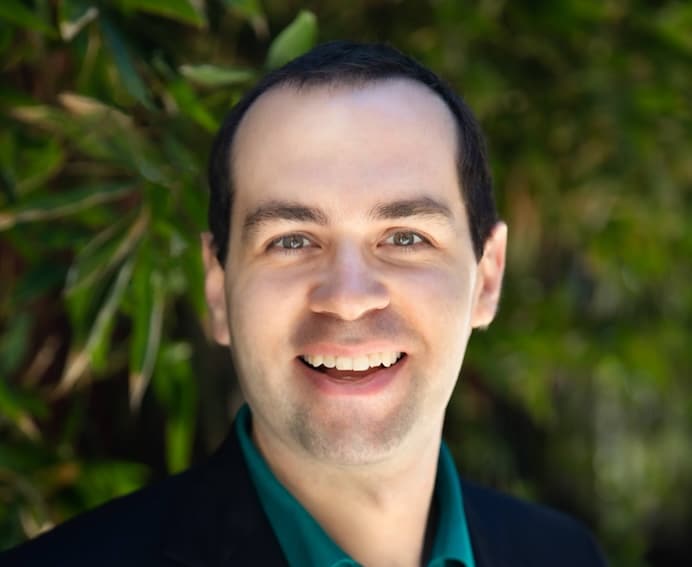
Find out more about our upcoming and past events.
We are the premier hub and policy institution for global energy thought leadership. Energy impacts every element of our lives, and our trusted fact-based research informs the decisions that affect all of us.
- Advisory Board
- Faculty Advisory Committee
- Independent Research
- Columbia University Partners
- Careers & Student Opportunities
The Center on Global Energy Policy is committed to independent and nonpartisan research that meets the high standards of academic integrity and quality at Columbia University.
We advance smart, actionable, and evidence-based energy and climate solutions through research, education, and dialogue.
Meet our staff and senior energy experts from government, academia, industry, and nongovernmental organizations.
- FEATURED CELEBRATING A DECADE
- Publications OUR WORK
- Our People MEET OUR EXPERTS
- INITIATIVES ALL INITIATIVES & PROGRAMS
- NEWS ALL NEWS
- UPDATES Events
- About Overview
Trends and Contradictions in China’s Renewable Energy Policy
Commentary by Anders Hove • August 28, 2020
China is the world’s leader in wind and solar power, although new capacity is being added more slowly than several years ago. Meanwhile, a wave of coal power plant approvals and fewer public mentions of urban air pollution and climate change have raised questions about the future of China’s renewable power sector in the wake of Covid-19. [1]
In this commentary, Anders Hove examines China’s recent energy policy announcements and their implications for the 14th Five-Year Plan, which will set energy policy for the period from 2021–2025. He argues that the future of renewable energy deployment in China will be shaped by an ongoing contradiction within the power sector between long-term market-oriented reforms on the one hand and short-term administrative planning on the other. This contradiction is well represented in two draft laws issued in April and June 2020: the draft Energy Law and the draft Guiding Opinion on Establishing a Clean Energy Consumption Long-Term Mechanism. The former states that the country should prioritize development of renewable energy by opening the market to more players, while the latter puts grid companies, provincial officials, and incumbent generation companies in charge of all aspects of planning and target-setting.
The ways in which this contradiction will be resolved are unclear. China’s central government remains focused on promoting markets in the longer term, in part based on market models that have worked in Europe and the US, so international lessons and experiences could play a role. China’s power reform could benefit from a greater focus on including consumers and other market players, and greater emphasis of long-term over short-term planning. Such a shift would help ameliorate the current trend of over-investment in unneeded and uneconomic coal capacity at the expense of renewables.
China leads the world in terms of total installed wind and solar capacity, as well as in annual installations of wind and solar. These two sources combined provided around 9 percent of China’s total electricity generated in 2019, a proportion that has grown by about 1% each year. [2] China continues to add wind and solar power capacity, but below their peak rates: for example, wind installations in 2019 were 26 gigawatts (GW), versus a peak installation of 33 GW in 2015, and photovoltaic (PV) installations in 2019 were 30 GW, down from a peak of 53 GW in 2017. [3] A June 2020 guidance on energy security jointly issued by the National Development and Reform Commission (NDRC) and the National Energy Administration (NEA) mentions a 2020 target of 240 GW each for wind and solar PV, implying additions of 30 GW of wind and 36 GW of solar in 2020—in other words, stable or moderate growth for these sources. [4]
The peak and decline of wind and solar installations relates mainly to less generous subsidy policies. [5] In the early period of wind and solar development in China, the country experienced boom and bust cycles — with booms due to declines in wind and solar capital costs that outpaced adjustments to administratively set feed-in tariffs and busts following downward revisions in feed-in tariffs. Unexpected installation booms that led to deficits in the fund used to pay for subsidized feed-in tariffs also raised issues for both developers and policy makers. [6] Such funds are derived from surcharges on retail electricity sales, and the government stopped increasing these surcharges after 2016. [7]
Policy makers increasingly require wind and solar to compete with coal on an unsubsidized basis. In 2018, the NDRC, which has overall responsibility for regulating electricity prices, signaled that wind and solar feed-in tariffs would be phased out completely by around 2020. [8] In mid-2018, a sudden announcement that no further quotas would be available for solar feed-in tariffs led to a sharp drop in solar installations; [9] that market has only partially recovered. For 2020, project approvals for any facilities that benefit from feed-in tariffs must be strictly controlled and limited to the anticipated increase in surcharge funds, which rise in proportion to electricity demand growth. [10] Since January 2019, a new category of wind and solar projects, known as grid parity projects, have been granted fixed 20-year contracts provided the price is at or below the local coal tariff and provided planners consider that the provincial grid has sufficient ability to absorb the new renewable capacity [11] .
Shift in Focus to Output and Consumption Rather Than Capacity Additions
Analysts often focus on GW of new additions as the main indicator of the transition to renewable energy, and Chinese energy planners had traditionally relied on administratively set capacity targets to guide the country’s energy sector, including renewable energy. During the period of the 12th Five-Year Plan (2011–2015), the target for total 2015 solar installations was adjusted upwards five times from 2011 to 2013, from 5 GW initially to 35 GW. Because feed-in tariffs were adjusted infrequently and may not have kept pace with declining costs, installations handily exceeded even these targets, [12] creating a sense of euphoria in the industry. For the 13th Five-Year Plan, however, capacity targets were set at modest levels and not revised upward. By mid-2017, solar had already surpassed the 110 GW target for 2020, and wind exceeded its target of 210 GW in 2019.
Figure 1: China wind (left) and PV (right) capacity from 2005-2019, and target for 2020

Source: Various sources [13]
The 13th Five-Year Plan for the first time established energy generation targets for wind and solar, underlining the importance placed on integrating renewable energy rather than just building new plants: The target for wind was set at 420 TWh, and the solar target at 150 TWh. [14] Wind is on track to meet this target in 2020, whereas solar surpassed its electricity production target in 2018.
Instead of spurring ever more renewable capacity, policy makers have come to focus more on resolving persistent problems that impede the efficient integration of renewable energy. One metric of the renewable integration problem is known as the curtailment rate, defined as the percentage of electricity from wind or solar that could have been produced but which the grid would not accept. Overbuilding of wind and solar in remote regions with inadequate transmission was one issue, but many analysts considered physical barriers less important than those related to China’s institutional and regulatory systems. [15] Grid companies did not have to compensate renewable energy producers for curtailed energy and were responsible for upholding fixed annual operating hours contracts with coal generators. [16] Many coal plants also serve as combined heat and power plants, providing local district heating in winter, another cause of inflexibility. [17]
Policy makers recognized that the goal of removing subsidies for wind and solar would be difficult if curtailment were left unresolved, given that curtailment reduces revenue and hurts overall project economics. The solution has been a combination of market-oriented reforms and administrative measures.
Four measures have played a role in resolving the country’s renewable integration challenges:
- Guaranteed consumption of renewable energy: China’s Renewable Energy Law initially guaranteed full purchase of renewable energy, but in practice dispatch did not prioritize renewable sources. In 2016, China reiterated the requirement for full purchase of renewable energy and issued minimum dispatch quotas for provincial grid companies. Subsequently, in 2018, these requirements were reemphasized and strengthened. Guaranteed purchase amounts are set based on provincial capacity factors, which in turn depend on local wind and solar resources. [18]
- Binding targets for reducing curtailment: In 2018, national energy authorities established a rule stating that provinces should basically resolve curtailment by 2020. Provinces were compelled to develop plans to reduce curtailment to below 5 percent by that year. [19]
- Compensation of curtailment: The initial Renewable Energy Law and subsequent feed-in tariff for wind and solar were unclear as to whether wind and solar would receive compensation for all energy they could produce or only for the energy accepted by the local grid. [20] In practice, curtailed electricity production was not compensated, marking a difference between China’s energy system and that of Germany, whose feed-in tariff China largely imitated. [21] In 2016, China issued Rule 625, mandating compensation for curtailed energy, [22] and in late 2019 the NDRC reiterated that such compensation was mandatory. [23] Clarifying that grid companies would bear the cost of curtailment introduced real, systematic economic incentives to reduce curtailment.
- Generation rights trading: In the past, coal plants were generally dispatched under fixed monthly and annual operating hours contracts. Gradually, these have been replaced by monthly and annual bilateral power purchase contracts, but the principle of maintaining dispatch plans on a monthly and annual basis, thereby providing coal plant owners with certainty on revenue, has remained. Allowing these plants to receive compensation from other plants in exchange for generation hours has incentivized generators to accept dispatch on a more economic basis. Trading of generation rights between provinces has allowed marketing of renewable energy that would otherwise be curtailed. [24]
As a result of these measures, wind curtailment has fallen from a peak of 17 percent in 2016 to 4 percent in 2019, while solar curtailment has fallen from 11 percent in 2015 to 2 percent in 2019. [25] Wind production has doubled since 2015 and solar production has grown by a factor of five in the same period. [26]
Policy makers also introduced a variety of measures that could contribute to greater uptake of renewable energy by consumers. These include a 2018 release of the Clean Energy Consumption Action Plan; voluntary green certificates for purchase by companies or individuals in 2017; and a renewable obligation for provinces, grid companies, and large energy consumers.
- The NDRC published the Clean Energy Consumption Action Plan in December 2018, stating a goal of “basically resolving clean energy integration problems by 2020” and setting targets to reduce wind and solar curtailment below 5 percent in all provinces by that date. It outlines the responsibility of grid companies to improve grid infrastructure and fully act as a resource allocation platform. The plan also calls for “prioritization of models for clean energy green consumption,” specifying that “renewable consumption obligations should be extended to electricity consumers; grid companies and electricity retail companies should introduce green electricity packages; and end-users should be encouraged to take up clean energy.” [27]
- On July 1, 2017, the NEA launched a voluntary green certificate market, mainly to reduce the government’s subsidy payment obligation by persuading corporates or individuals to purchase the certificates, which transferred the subsidy obligation for existing projects rather than creating a market for projects that would provide additional renewable energy beyond that already existing. For this reason, the green certificate market never took off. [28]
- The renewable obligation (RO) went through three drafts in 2018 before adoption. China’s RO specifies consumption targets for just three years (including the current year), rather than setting targets for the provinces or market to aim for over the long-term. Indeed, the provincial obligation for 2020 was adjusted in June of this year to more closely reflect output. This means that the obligation resembles an administrative planning quota and does little to promote market-based investments in clean energy over the long-term. [29]
Figure 2: 2020 minimum provincial non-hydro renewable obligation, percentage of generation

Source: National Energy Administration, June 2020
China’s 2020 Renewable Energy Policies
2020 is the last year of the 13th Five-Year Plan and the key time for developing the 14th Five-Year Plan. Several policies that appear to lay out the basic framework for the 14th Five-Year Plan have now been released. They include a draft Energy Law, a draft policy on clean energy consumption, a target for 2020 renewable energy capacity, and updated policies and guidance on provincial coal plant approvals.
In April 2020, China’s National Energy Administration published a new draft Energy Law. [30] The measure goes beyond prior energy law by clearly stating that renewable energy has priority for development in China’s energy system. The measure explicitly calls for development of a low-carbon energy system, for non-fossil energy to gradually replace fossil fuel energy sources, and for finding substitutes for natural gas and oil. The measure mentions various renewable energy sources more than any individual fossil fuel. The draft mentions promoting clean coal — which in China refers to newer, more efficient coal plants with low emissions of particulate matter, NOx, and SO2, including supercritical and ultra-supercritical coal plants — but also emphasizes efficiency, ecological protection, and environmental taxes. The priority attached to low-carbon energy, the requirement that energy planners consider environmental costs and damage, the emphasis on reducing emission of greenhouse gases and other pollutants, and the call for prices to include the cost of emissions and environmental damage are all encouraging signs that could indicate greater attention to these factors in energy sector policy in the future.
The draft Energy Law contains several important measures, but also contains contradictions. For example, while the measure reiterates that the market shall play a decisive role in allocating energy resources, it contains relatively few concrete clauses related to markets. The word “market” is mentioned 44 times, whereas the word “planning” is mentioned 74 times. Substantively, 21 of the 117 articles focus on planning, 17 articles on regulation or supervision, and only six articles discuss the market. No major market-related initiatives or targets are included in the draft.
The draft Energy Law codifies existing policies into law rather than instituting new policies. For example, the law mentions existing provincial renewable obligations, green certificates, and guaranteed consumption of renewable energy, but does not modify these policies or establish a long-term plan for how they might be coordinated or integrated.
One promising article explicitly encourages public participation in energy planning, stating that planners should seek the opinions of institutions, experts, and the public. The draft also indicates room for participation of more players in a competitive energy system—in contrast to the present system in which provincial authorities and major state-owned energy giants dominate planning and market guidance: “A well-regulated energy market system open to diverse players promotes orderly competition and achieves the optimal allocation of energy resources over a wider area.”
Clean Energy Consumption Draft
In May 2019, China’s National Energy Administration issued a draft Guiding Opinion on Establishing a Sound, Long-term Clean Energy Consumption. [31] The Clean Energy Consumption draft includes sections on planning, markets, flexibility, technology innovation, and monitoring, and states China’s overall strategy to put clean energy consumption at the core—rather than new capacity.
The policy starts primarily with content on administrative planning, which includes “scientific targets” for renewable consumption and “strengthened analysis of renewable consumption capacity.” The latter emphasizes that renewable energy developers should strictly consider local grid capacity to absorb renewable energy. The draft promotes a list of innovative fields and technologies, including energy storage, multi-energy complementarity, and uptake of renewable electricity through port electrification, electric vehicle charging, hydrogen production, as well as heating and cooling.
Like the Energy Law draft, the Clean Energy Consumption draft vaguely mentions the need to coordinate renewable obligations and green certificates. It suggests accelerating the adoption of spot and ancillary service markets to incentivize renewable energy producers, grid operators, power companies, and provinces to contribute to an efficient and cost-effective energy market and support the integration of renewable resources.
However, it fails to mention the need for coordinating renewable energy markets and green certificates markets with carbon markets. Furthermore, the draft does not include the word carbon and does not repeat the language from the draft Energy Law stating that China will prioritize development of renewable energy. Rather, it recommends “using the provincial power grid’s clean energy utilization target as a constraint,” and strictly limiting renewable project approvals to prevent overbuilding. Although the draft’s title refers to a long-term mechanism for supporting clean energy consumption, the only timeframe it gives for analysis of clean energy consumption is the three-year planning process for provinces and grid companies to determine provincial capacity for absorbing clean energy. Given that earlier short-term planning has produced over-investment in coal capacity that has a high likelihood of economic stranding, this omission could exacerbate this over-investment problem.
Although the draft is related to consumption, planning remains in the hands of large, state-owned producers including mainly grid companies, provincial energy planners, and large generators. This appears to contradict earlier calls for modernized systems of governance in the energy sector and the draft Energy Law’s call for opening the energy sector to public participation and new market players, as well as the document’s own preamble, which reiterates President Xi’s call for a revolution in energy consumption and production. In the US, consumers have played an important role in commercializing utility-scale solar and wind through the signing of power purchase agreements. Similarly, consumer groups have played a central role in advancing policies that reduced unneeded investment in long-lived fossil fuel infrastructure in favor of more economical and cleaner alternatives, including efficiency, demand response, and clean energy. The relative openness and transparency of power sector planning and regulation in the US has facilitated participation of the public and consumers in guiding and leading the energy transition.
Another interesting aspect of the Clean Energy Consumption draft is how it allocates responsibility for integrating renewable energy. Whereas the 2018 Clean Energy Consumption Action Plan placed responsibility for renewable integration squarely on the grid companies, the new draft prioritizes “grid-friendly” clean energy and mentions hybrid renewable plants that incorporate storage as a strategy for increasing renewable consumption. The draft does not explicitly provide new methods for promoting renewables paired with energy storage, nor does it rule them out. Notably, several provinces have begun requiring new wind plants to come equipped with storage for 20 percent of installed capacity, without offering additional compensation. [32] It is unclear whether such requirements represent the most economical path to integrating more renewables into the grid. In a 2019 study by the International Energy Agency of China’s energy system, analysts recommended prioritizing flexibility, demand response, and trading electricity between provinces over requiring grid-scale energy storage. [33]
Guidance and Targets for 2020 Renewables
The renewable targets set for 2020 appear to signal a return to growth for renewable energy, while falling short of the rapid growth of years prior. As noted above, in June 2020, the NEA set year-end capacity targets of 240 GW each for wind and solar. [34] At the end of 2019, China had 210 GW of wind installed and 204 GW of solar, [35] so this implies growth of 30 GW for wind and 36 GW of solar, which is roughly in line with total annual wind and solar installations in the past three years. The Ministry of Finance has also cut the amount allocated for PV subsidies from RMB 3 billion in 2019 to RMB 2.63 billion in 2020, while wind will receive subsidies of RMB 2.94 billion, down 30 percent from RMB 4.24 billion allocated in 2019. [36]
Meanwhile, both central government planners and grid companies have released calculations on their ability to absorb renewable energy, which serves as a cap on new additions. The NEA has calculated China could absorb 85 GW of new additions in 2020. [37] State Grid, which serves most of China where most wind and solar capacity is located, estimated its territory can absorb 68 GW of new wind and solar. [38] Both these figures would be higher than the year-end capacity targets noted above, but shouldn’t be taken as targets.
Wind and solar installations so far in 2020 were affected by the Covid-19 disruption, but the data suggest stable installation rates rather than a sudden collapse or dramatic increase. A total of 17.8 GW of wind and solar projects were completed in the first six months of 2020 (6.3 GW of wind and 11.4 GW of solar). This is a decline of 13 percent from the combined 20.5 GW added in the first half of 2018, which consisted of 9.1 GW of wind and 11.5 GW of solar. [39] Wind installations in particular have been affected by supply chain disruptions but are expected to rise toward the end of the year as the deadline for feed-in tariff subsidies approaches. [40] In June, the China Photovoltaic Industry Association forecast that China would add around 45 GW of solar in 2020, [41] higher than in 2019 but in line with installations in 2018. In 2020, China’s regulators have also approved 33.1 GW of subsidy-free solar projects and 11.4 GW of wind for connection in 2020. [42] Overall, these figures indicate that wind and solar are likely to see stable or modestly higher capacity installations this year.
Policies on Coal
Given China’s reliance on coal for the majority of electricity production and the continued build-out of new coal capacity in China, policies on renewable energy are inextricably linked to policies on coal. Prior to the Covid-19 pandemic, which resulted in a temporary drop in coal generation, China was moving to accelerate the construction of new coal capacity. In past years, provinces had sought to accelerate coal plant construction as an economic development strategy, despite low utilization of existing coal plants and long-running warnings of overcapacity and poor financial performance. This resulted in a tug-of-war between provincial announcements and measures issued by central authorities, which sought to rationalize coal capacity, improve integration of renewable energy, and boost electricity trade among provinces.
Though provinces have retained authority over new coal plant approvals, starting in 2016, China’s NEA began a new traffic light system that effectively halted new coal plant construction in provinces deemed to have insufficient environmental capacity, resource capacity, or electricity demand to absorb new coal plants. The NEA’s first traffic light announcement labeled all but three provinces as either red or orange on one of these three categories. [43] Each year since then, the number of provinces granted a green light for new coal capacity has increased, and the latest NEA traffic light system, issued in February of 2020, included just five provinces labeled red or orange for the binding capacity adequacy metric (shown at left on the maps below). [44]
Figure 3: NEA traffic light alert system for new coal plant approvals, 2021–2023

The result has been a surge in new coal plant construction and approvals at the provincial level. As of June 2020, there are reportedly 46 GW of new coal plants under construction, and an additional 42 GW of new coal plant approvals. The new coal plant approvals appear linked to lowered ambition on clean energy. For example, in May, Hunan province issued an announcement that provincial grid authorities would not permit any new utility-scale solar PV plants in the next four years due to insufficient grid capacity, [45] but just two days later, Hunan announced approval of a new 2 GW coal plant. [46] At least two other provinces have announced they have no ability to absorb new renewables in 2020, including Henan and Shanxi. [47]
Perhaps in response to the new coal plant approvals, in June the NDRC issued a policy reiterating that provinces with 2023 red or orange traffic lights should not build new coal and overall coal capacity should remain within a 1,100 GW cap set for 2020. While the policy states that provinces should “in principle” prioritize renewable energy, demand side management, and imports over new coal, if these sources are insufficient to meet peak load then new coal plants can be permitted. (This principle had also been stated in the text of the coal traffic light policy issued in prior years.) Since no new mandatory limits are described, the policy largely reiterates current policy and leaves provinces in the driver’s seat regarding their preferred balance between new coal versus alternatives. [48]
In the past two years, China has placed a high priority on reducing electricity prices and improving energy security. [49] Although policy makers in China may see coal as cheap and secure, many analysts view coal as economically risky and likely to be supplanted by cheaper renewables, which use inherently secure domestic wind and solar power and which are largely manufactured within China. The Energy Research Institute of the NDRC and its China National Renewable Energy Centre forecast that prioritizing renewable energy and halting coal additions would save RMB 7.2 trillion through 2050. [50] A 2019 study published in Nature forecasts that China could generate over 60 percent of its electricity from renewables by 2030 at 11 percent lower cost than a business-as-usual scenario in which coal capacity continues to grow. [51] A 2019 Nature Energy article estimates that solar energy is already cheaper than retail electricity prices in most of China’s 344 prefecture and above cities and lower than desulfurized coal feed-in tariffs in 22 percent of those cities. [52]
These estimates also track with international trends. A recent International Renewable Energy Agency (IRENA) analysis of renewable project data worldwide shows that the majority of wind projects are cheaper than the lowest cost fossil fuel alternative, and power purchase agreements for solar for construction in 2021 are on average 42 percent cheaper than the lowest cost fossil fuel alternative. [53] IRENA expects cost declines in renewable energy to continue through the 2020s, with energy storage costs alone declining as much as 65 percent. This implies that even in parts of China where wind, solar, or hybrid renewable energy plants are not yet at parity with coal tariffs, delaying further coal investments and procuring cheaper renewable energy later would save money.
Implications for the 14th Five-Year Plan
China’s 14th Five-Year Plan for renewable energy will not only incorporate elements of the detailed policies described above but also take place in the context of major long-term guidance on energy policy. These directives include broad guidance for the overall energy sector, such as the power market reforms begun in 2015, the vision for a revolution in energy consumption and production, the principle of markets playing a decisive role in allocation of energy and environmental resources, and President Xi’s overall vision for a Beautiful China, which incorporates low-carbon development and construction of an ecological civilization.
More concretely, the plan will incorporate past targets for non-fossil energy to provide 20 percent of primary energy production by 2030 and for China’s total carbon emissions to peak by around 2030. Importantly, none of the policy announcements so far in 2020 imply any increased ambition on either of these targets. Rather, China appears to be focused on keeping the annual installations of renewable energy broadly stable, while leaving room for new fossil energy development led by provincial authorities.
Prior to the Covid-19 crisis, 2020 was shaping up to be a watershed year for climate negotiations, and China’s role appeared particularly central given that this year’s COP would coincide with development of China’s 14th Five-Year Plan. Covid-19 has had several impacts on energy sector developments. First, reduced energy demand temporarily hit production of electricity from coal and seems likely to reduce returns on capital-intensive energy projects across the energy space. However, Covid-19 has also strengthened calls to boost China’s energy security and local economic stability, and coal figures in both of these. In China, domestic coal has often been mentioned in the context of energy security, whereas variable renewable energy often isn’t. While China’s central government hasn’t announced a specific economic stimulus plan in the wake of Covid-19, it has opened up local lending, some of which will support coal power projects.
Given that renewable energy can now compete with fossil fuels in most of the world, even without considering carbon prices or environmental externalities, and given that China has already led the world for several years in the manufacture and installation of wind and solar, many climate analysts had hoped that China’s 14th Five-Year Plan would signal new ambition on renewable energy. Instead, the measures announced so far this year imply a trend of consolidation and conservatism, aimed at balancing the demands of provinces for new coal capacity with sustaining markets for clean energy and holding on to the gains made in integrating the renewable capacity already on the grid.
There is no doubt that China will continue to promote clean energy of all types, and the policies so far in 2020 have emphasized that China will continue to innovate in areas such as energy storage, hydrogen, floating solar, demand response, and electric vehicle charging. The continuing fall in costs for wind, solar, and energy storage are likely to make these resources all but inevitable winners in the long run. But for now, although China’s energy policies continue to include language favoring renewables and market reforms in principle, the specific details focus more on short-term administrative planning targets and stable markets for both renewable energy and coal. The green light for new coal plant construction, constraints on renewable development in the Clean Energy Consumption guiding opinion, and provincial-level caps on renewable energy indicate a conservative approach in the near-term. From these signals, it appears unlikely that the 14th Five-Year Plan will shake up the status quo of China’s energy markets.
[1] For an overview of renewable capacity by country, see Table 2, “Renewables 2020 Global Status Report,” Ren21, March 2020, https://www.ren21.net/gsr-2020/tables/table_02/table_02/ . See also Lauri Myllyvirta, “China’s CO2 emissions surged past pre-coronavirus levels in May,” Carbon Brief, June 29, 2020, https://www.carbonbrief.org/analysis-chinas-co2-emissions-surged-past-pre-coronavirus-levels-in-mayhttps://twitter.com/LiShuo_GP/status/1273791019331379202 .
[2] “中电联发布2019-2020年度全国电力供需形势分析预测报告,” China Electricity Council, January 21, 2020, http://www.cec.org.cn/guihuayutongji/gongxufenxi/dianligongxufenxi/2020-01-21/197090.html .
[3] 2019 wind capacity figures: “2019年风电并网运行情况,” National Energy Administration, February 28, 2020, http://www.nea.gov.cn/2020-02/28/c_138827910.htm . 2019 solar capacity figures: “2018 年可再生能源并网运行情况介绍,” National Energy Administration, January 28, 2019, http://www.nea.gov.cn/2019-01/28/c_137780519.htm . 2015 wind capacity figures: “2015年全国风电产业继续保持强劲增长势头,” National Energy Administration, February 2, 2016, http://www.gov.cn/xinwen/2016-02/02/content_5038302.htm . 2017 solar capacity figures: “2017年光伏发电新增装机5306万千瓦 居可再生能源之首,” National Energy Administration, January 24, 2018, at http://fjb.nea.gov.cn/news_view.aspx?id=28662 .
[4] For 2020 cumulative installation targets, see: “关于做好2020年能源安全保障工作的指导意见, 发改运行〔2020〕900号,” National Development and Reform Commission and National Energy Administration, 12 June 2020, https://www.ndrc.gov.cn/xxgk/zcfb/tz/202006/t20200618_1231501.html . 2019 wind capacity figures: “2019年风电并网运行情况,” National Energy Administration, February 28, 2020, at http://www.nea.gov.cn/2020-02/28/c_138827910.htm . 2019 solar capacity figures: “2018 年可再生能源并网运行情况介绍,” National Energy Administration, January 28, 2019, at http://www.nea.gov.cn/2019-01/28/c_137780519.htm .
[5] China’s peak installations of solar PV was in 2017; cuts in feed-in tariffs and FIT quotas for solar feed-in tariffs led to a drop in solar installations in both 2018 and 2019. See “China still most attractive renewables market despite subsidy cuts – EY,” Reuters, September 12, 2019, https://www.euronews.com/2019/05/15/china-still-most-attractive-renewables-market-despite-subsidy-cuts-eyhttp://m.opinion.caixin.com/m/2019-11-26/101487343.html . Solar feed-in tariff subsidies for both utility-scale and distributed projects had fallen since 2016. “Breaking: China looking to dramatically cut solar PV FIT,” PV Magazine, November 29, 2016, https://www.pv-magazine.com/2016/09/29/breaking-china-looking-to-dramatically-cut-solar-pv-fit_100026296/ . Wind installations peaked at 30.5 GW in 2015 before declining due to tariff cuts. See “China’s wind power industry faces slowdown as tariff cuts loom,” South China Morning Post, October 20, 2016, https://www.scmp.com/business/companies/article/2038694/chinas-wind-power-industry-faces-slowdown-tariff-cuts-loom . Total wind and solar installations peaked in 2018 and declined in 2019; falling solar installations were only partially offset by rising wind installations.
[6] For a good overview of the history of feed-in tariff subsidies and their gradual phase-out, see Yuki Yu, “China’s Renewable Power Price and Subsidy: “New” Design in 2020?,” Energy Iceberg, January 29, 2020, https://energyiceberg.com/china-renewable-power-price/ .
[7] “How to Harvest From China’s Renewable Asset Sales: Subsidy-Not-Receivable the Biggest Threat,” Energy Iceberg, accessed August 9, 2020, https://energyiceberg.com/china-renewable-subsidy-deficit/ .
[8] Starting in 2015, China has begun a transition towards wholesale power markets. To date, most market transactions fall into a category known as mid- to-long-term contracts, which refers to bilateral contracts between large electricity consumers and generation companies. The contracts are for periods of one month, one year, or sometimes more than one year. Several provinces have begun spot power market pilots, but these are mostly performing simulated trading. The Guangdong provincial spot market pilot is the most advanced in terms of implementation, but trades account for a small proportion of total electricity consumption. See Yuyan Cao, Ruosida Lin, Daniel Wetzel, “Tracking China’s Provincial Spot Market Designs,” Rocky Mountain Institute, December 2019, https://rmi.org/insight/tracking-chinas-provincial-spot-market-designs/ .
[9] “关于2018年光伏发电有关事项的通知, 发改能源〔2018〕823号,” National Development and Reform Commission, Ministry of Finance and National Energy Administration, May 31, 2018, http://www.nea.gov.cn/2018-06/01/c_137223460.htm .
[10] “可再生能源电价附加资金管理办法, 财建〔2020〕5号,” Ministry of Finance, National Energy Administration and National Development and Reform Commission, January 20, 2020, http://www.gov.cn/zhengce/zhengceku/2020-02/05/content_5474795.htm .
[11] See “国家发展改革委 国家能源局关于积极推进风电、光伏发电无补贴平价上网有关工作的通知,” National Development and Reform Commission, January 9, 2019, https://www.ndrc.gov.cn/xxgk/zcfb/tz/201901/t20190109_962365.html . For the most recent policy, see: “国家发展改革委办公厅 国家能源局综合司关于公布2020年风电、光伏发电平价上网项目的通知,” National Development and Reform Commission, August 5, 2020, https://www.ndrc.gov.cn/xxgk/zcfb/tz/202008/t20200805_1235592.html .
[12] “China boost 2015 solar capacity target to 21 GW – report,” Renewables Now, July 2, 2012, https://renewablesnow.com/news/china-boost-2015-solar-capacity-target-to-21-gw-report-286220/ .
Nilima Choudhury, “China increases solar target by 67% – yet again,” PV Tech, February 1, 2013, https://www.pv-tech.org/news/china_increases_solar_target_by_67_yet_again .
[13] “中国可再生能源及非化石能源利用数据一览表,” China National Renewable Energy Centre, March 13, 2019;“关于做好2020年能源安全保障工作的指导意见,” National Development and Reform Commission (NDRC) and National Energy Administration (NEA), June 12, 2020, https://www.ndrc.gov.cn/xxgk/zcfb/tz/202006/t20200618_1231501_ext.htmlhttp://www.nea.gov.cn/2020-02/28/c_138827923.htm .
[14] “能源发展“十三五”规划, 发改能源[2016]2744号, ” National Development and Reform Commission and National Energy Administration, December 26, 2016, http://www.nea.gov.cn/2017-01/17/c_135989417.htm .
[15] Michael R. Davidson, “Creating Markets for Wind Electricity in China: Case Studies in Energy Policy” (PhD Dissertation, Massachusetts Institute of Technology, June 2018), https://globalchange.mit.edu/sites/default/files/Davidson_PhD_2018.pdf .
[16] Michael Davidson, “Technology Integration in China’s Electricity System: Central Targets and Local Challenges,” in Loren Brandt and Thomas G Rawski eds., Policy, Regulation and Innovation in China’s Electricity and Telecom Industries (Cambridge: Cambridge University Press, 2019), 150–151.
[17] Qi Ye, Lu Jiaqi, and Zhu Mengye, “Wind Curtailment in China and Lessons from the United States,” Brookings-Tsinghua Center for Public Policy, March 2018, https://www.brookings.edu/wp-content/uploads/2018/03/wind-curtailment-in-china-and-lessons-from-the-united-states.pdf .
[18] “国家能源局关于做好2016年度风电消纳工作有关要求的通知” [NEA Notice on Implementing 2016 Wind Integration Work], National Energy Administration, March 18, 2016, http://www.gov.cn/xinwen/2016-03/18/content_5055168.htmhttp://www.gov.cn/xinwen/2019-05/16/content_5392082.htm .
[19] “清洁能源消纳行动计划(2018-2020年), 发改能源规〔2018〕1575号 [Clean energy consumption action plan [2018-2020], NDRC Energy Planning (2018) No. 1575],” National Development and Reform Commission and National Energy Administration, October 30, 2018, https://www.ndrc.gov.cn/xxgk/zcfb/ghxwj/201812/t20181204_960958.html . See also Max Dupuy and Wang Xuan, “China’s String of New Policies Addressing Renewable Energy Curtailment: An Update,” Regulatory Assistance Project, April 8, 2016, https://www.raponline.org/blog/chinas-string-of-new-policies-addressing-renewable-energy-curtailment-an-update/ .
[20] Max Dupuy and Wang Xuan, “China’s String of New Policies Addressing Renewable Energy Curtailment: An Update,” Regulatory Assistance Project, April 8, 2016, https://www.raponline.org/blog/chinas-string-of-new-policies-addressing-renewable-energy-curtailment-an-update/ .
[21] Max Dupuy, Frederick Weston, and Anders Hove, “Deepening reform to reduce emissions, improve air quality, and promote economic growth,” Paulson Institute, 2015, http://www.paulsoninstitute.org/wp-content/uploads/2015/09/2-Power-Sector-EN-Final.pdfhttps://www.bmwi.de/Redaktion/EN/Downloads/renewable-energy-sources-act-2017.pdf%3F__blob%3DpublicationFile%26v%3D3 . “The German Feed-in Tariff,” Future Policy.org, accessed August 10, 2020, at https://www.futurepolicy.org/climate-stability/renewable-energies/the-german-feed-in-tariff/ .
[22] “可再生能源发电全额保障性收购管理办法, 发改能源 [2016]625号 [Renewable Electricity Full Purchase Guarantee Management Act, NDRC (2016) No. 625],” National Development and Reform Commission Number 625, March 24, 2016, http://www.nea.gov.cn/2016-03/28/c_135230445.htm .
[23] “电网企业全额保障性收购可再生能源电量监管办法(修订) (征求意见稿) [Grid Company Full Purchase of Renewable Electricity Supervision Act Amendment (Revised) (Draft for Comment)],” National Development and Reform Commission, November 22, 2019, https://www.ndrc.gov.cn/xwdt/tzgg/201911/t20191122_1204501.html .
[24] “关于印发《东北区域省间电能交易办法》的通知,” Northeast Supervision Bureau of National Energy Administration, September 26, 2016, http://shoudian.bjx.com.cn/news/20161024/782849.shtml .
[25] “2019年风电并网运行情况,” National Energy Agency, February 28, 2020, at http://www.nea.gov.cn/2020-02/28/c_138827910.htmhttp://www.nea.gov.cn/2020-02/28/c_138827923.htm .
[26] In 2015, solar produced 39.2 TWh; see “能源局公布2015年光伏发电相关统计数据,” National Energy Administration, February 5, 2016, http://www.gov.cn/xinwen/2016-02/05/content_5039508.htm . In 2019, solar produced 224 TWh;see Ding Qiating, “2019年我国风电发电量首次突破4000亿千瓦时!相当于四个三峡电站发电量,” Beijixing, March 3, 2020, http://news.bjx.com.cn/html/20200303/1050046.shtml . In 2015, wind produced 186.3 TWh; see “2015年中国风电开发主要数据汇总,” China 5e, June 3, 2016, https://www.china5e.com/news/news-946629-1.html . In 2019, wind produced 405.7 TWh, Ding Qiating, “2019年我国风电发电量首次突破4000亿千瓦时!相当于四个三峡电站发电量,” Beijixing, March 3, 2020, http://news.bjx.com.cn/html/20200303/1050046.shtml .
[27] See Section 6, article 13, in “关于印发《清洁能源消纳行动计划(2018-2020年)》的通知,” National Development and Reform Commission, December 4, 2018, https://www.ndrc.gov.cn/xxgk/zcfb/ghxwj/201812/t20181204_960958.html .
[28] “关于试行可再生能源绿色电力证书核发及自愿认购交易制度的通知,” National Energy Administration, February 6, 2017, http://www.nea.gov.cn/2017-02/06/c_136035626.htm .
“绿证认购陷入“停滞”,如何助推自愿认购型绿证?,” Yiyou Village PV, September 12, 2018, https://www.jianshu.com/p/90e4035fc901 .
[29] “关于实行可再生能源电力配额制的通知 (征求意见稿) [Notice on Implementing Renewable Electricity Obligation (Draft for Public Comment)],” China National Energy Administration, November 15, 2018, http://www.nea.gov.cn/2018-11/15/c_137607356.htm .
“关于印发各省级行政区域2020年可再生能源电力消纳责任权重的通知” [Renewable Energy Electricity Consumption Obligation in 2020 for Each Provincial Administrative Region China National Energy Administration], June 1, 2020, http://www.nea.gov.cn/2020-06/01/c_139105253.htm .
[30] “Energy Law of the People’s Republic of China [Draft for Comments],” National Energy Administration, April 10, 2020.
[31] “Draft Guidance on Establishing a Sound, Long-term Mechanism for Clean Energy Consumption,” National Energy Administration, May 19, 2019.
[32] You Feng, “湖南风电项目被要求配20%储能,” Nengjian Eknower, April 13, 2020, http://news.bjx.com.cn/html/20200413/1062659.shtml .
[33] “China Power System Transformation – Assessing the benefit of optimised operations and advanced flexibility options,” International Energy Agency, February 26, 2019, https://webstore.iea.org/china-power-system-transformation .
[34] “国家发展改革委 国家能源局 关于做好2020年能源安全保障工作的指导意见,” National Development and Reform Commission, 12 June 2020, at https://www.ndrc.gov.cn/xxgk/zcfb/tz/202006/t20200618_1231501.html .
[35] 2019 wind capacity figures: “2019年风电并网运行情况,” National Energy Administration, February 28, 2020, http://www.nea.gov.cn/2020-02/28/c_138827910.htm . 2019 solar capacity figures: “2018 年可再生能源并网运行情况介绍,” National Energy Administration, January 28, 2019, http://www.nea.gov.cn/2019-01/28/c_137780519.htm .
[36] “关于促进非水可再生能源发电健康发展的若干意见” [Opinions on Promoting the Healthy Development of Non-hydro Renewable Energy Power Generation], Ministry of Finance, January 20, 2020, http://www.gov.cn/zhengce/zhengceku/2020-02/03/content_5474144.htmhttps://m.energytrend.com/news/view/15888.html .
[37] “全国新能源消纳监测预警中心关于发布2020年全国风电、光伏发电新增消纳能力的公告,” National Monitoring and Early Warning Center for New Energy Consumption, May 25, 2020, https://www.nengapp.com/news/detail/3881478 .
[38] “国家电网有限公司关于发布2020年风电、光伏发电新增消纳能力的公告,” State Grid, May 19, 2020, http://news.bjx.com.cn/html/20200520/1073929.shtml .
[39] “2020 Q2 electricity & other energy statistics,” China Energy Portal, July 21, 2020; “2020年上半年风电并网运行情况,” China National Energy Administration, July 31, 2020 http://www.nea.gov.cn/2020-07/31/c_139254298.htmhttp://www.nea.gov.cn/2020-07/31/c_139254346.htm .
[40] “Coronavirus pandemic expected to slow down wind industry in China, says GlobalData,” Global Data, April 1, 2020, https://www.globaldata.com/coronavirus-pandemic-expected-to-slow-down-wind-industry-in-china-says-globaldata/https://www.energyglobal.com/wind/07082020/china-to-add-251-gw-of-new-wind-capacity-by-2029/ .
[41] “广发证券:2020年国内新增光伏装机有望达到甚至超过45GW,” SolarZoom, June 27, 2020, https://mp.weixin.qq.com/s/xwHFS_z04syiExwWAU80XQ .
[42] “《国家发展改革委办公厅 国家能源局综合司关于公布2020年风电、光伏发电平价上网项目的通知》政策解读,” National Development and Reform Commission, August 5, 2020, https://www.ndrc.gov.cn/xxgk/jd/jd/202008/t20200805_1235591.html .
[43] “国家能源局关于建立煤电规划建设风险预警机制暨发布2019年煤电规划建设风险预警的通知,” National Energy Administration, May 9, 2016, https://fgw.hebi.gov.cn/sfgw/638836/639672/639684/1446097/index.html .
[44] “国家能源局关于发布2023年煤电规划建设风险预警的通知 [NEA Notice on 2023 Coal Planning and Construction Risk Warning],” National Energy Administration, February 26, 2020, http://www.nea.gov.cn/2020-02/26/c_138820419.htm .
[45] “湖南宣布无消纳空间,暂停新增普通地面光伏项目备,” Nengapp.com, May 27, 2020, https://www.nengapp.com/news/detail/3881913 .
[46] “关于长安益阳发电有限公司三期(2×1000MW)扩建工程社会稳定风险评估公众参与信息公示,” Yiyang City Government, May 29, 2020, May 29, 2020, http://www.yiyang.gov.cn/yiyang/2/3/4/content_1189176.html .
[47] “河南无储能将无消纳空间 已有九省份鼓励新能源配储能,” Beijixing Energy Storage Net, May 26, 2020, http://chuneng.bjx.com.cn/news/20200526/1075498.shtml .
[48] “2020 年煤电化解过剩产能工作要点” [2020 coal overcapacity work key points], National Development and Reform Commission, June 18, 2020, https://www.ndrc.gov.cn/xxgk/zcfb/tz/202006/P020200618375022908090.pdf .
[49] Keqian Chen, “全国两会降低工商业电价、保障能源安全受关注,” China Southern Grid News, May 27, 2020, http://shupeidian.bjx.com.cn/html/20200527/1075973.shtml .
[50] “2050年我国高比例可再生能源发展的宏观经济影响评价,” Energy Research Institute of NDRC and China National Renewable Energy Center, September 15, 2019, https://wenku.baidu.com/view/45a02eac32d4b14e852458fb770bf78a65293af6.html .
[51] Gang He, et al., “Rapid cost decrease of renewables and storage accelerates the decarbonization of China’s power system,” Nature Communications, 11, 2486 (May 2020), https://www.nature.com/articles/s41467-020-16184-x .
[52] Jingyue Yan et al., ”City-level analysis of subsidy-free solar photovoltaic electricity price, profits and grid parity in China,” Nature Energy, 4 (August 2019) 709–717, https://www.nature.com/articles/s41560-019-0441-z.epdf .
[53] “Renewable Power Generation Costs in 2019,” International Renewable Energy Agency, June 2020, https://www.irena.org/publications/2020/Jun/Renewable-Power-Costs-in-2019 .
Relevant Publications
Sunspot: the rise of renewables in the gulf.
The six Gulf Cooperation Council (GCC) countries hold 2.5% of global electricity generation,[1] are some of the sunniest in the world, have set a string of records for...
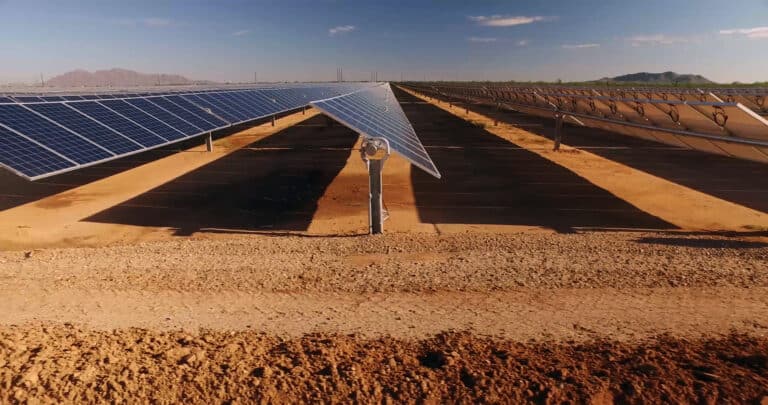
Testimony on Grid Modernization and Clean Energy Standards
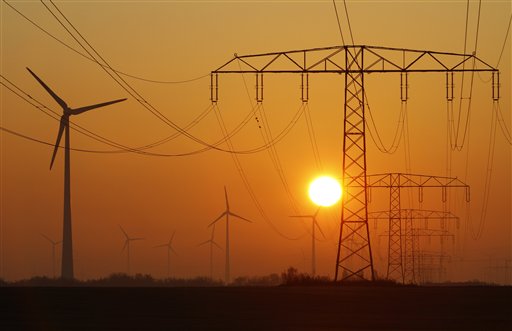
Approaches to include energy trade in TIMES energy system modelling – practices, recommendations and further research needs
Full report
Sign Up Today
Get the latest news and research on energy & climate policy.
- Get involved
- Energy Access Case Study 6 – Going Renewable: China’s Success Story in Capacity Development
Related Publications
- Towards an 'Energy Plus' Approach for the Poor
- Towards an ‘Energy Plus’ Approach for the Poor: An agenda for action for Asia and the Pacific
- English pdf (0.2 MB)
- View All Publications in the Series
Energy Access Case Study 06 – Going Renewable: China’s Success Story in Capacity Development
July 27, 2015.
This case study documents the Capacity Building for the Rapid Commercialization of Renewable Energy project (CRE) in China. The CRE has promoted widespread adoption of renewable energy technologies by strengthening the capacities of public and private organizations, facilitating and supporting new policy initiatives, developing a regulatory system, and providing technical and co-financing support for high-value commercial pilot projects.
Document Type
Regions and countries, publications, a turbulent future: how climate change impacts young peop....
The paper elucidates how the rapid rise in sea levels, extreme weather events, and environmental degradation disproportionately impact the younger population, l...
Poverty and the Household Economy of Myanmar: a Disappear...
The decline in incomes and limited income-generating capacity are pushing households to adopt negative coping mechanisms, such as cutting non-food expenditure. ...
Guidebook on how to Access Climate Finance for Member Sta...
The technical guidebook provides an overview of climate finance in the region and sources of climate finance flows, as well as the project development process t...
People and Planet: Addressing the Interlinked Challenges ...
Despite being at the halfway point for achieving the SDGs, progress has stalled, achieving only 15 per cent of the targets under the 17 Goals. Most worryingly, ...
SDG 16: Peace, justice and strong institutions: promote p...
SDG 16 (highlights the need for peaceful societies, access to justice and effective, accountable and inclusive institutions at all levels. Its realization is es...
Making Our Future: New Directions for Human Development i...
The 2024 Asia-Pacific Human Development Report Making our Future: New Directions for Human Development in Asia and the Pacific, argues that unmet aspirations, h...
- Berkeley Lab
- Energy Technologies Area
China Energy Program
We work collaboratively with researchers in China and around the world to understand the dynamics of China's energy system. Our research focuses on the analysis of energy and related emissions trends, technologies and policies on various sectors in China's economy.
The China Energy Program was founded in 1988 and works collaboratively with partners in China and around the world to understand the dynamics of China's energy system, energy use, and associated impacts on the environment and global climate, and to identify ways to address energy, environment, and climate challenges. Research is conducted at the intersection of science, technology, policy, and economics with collaborators around the world.
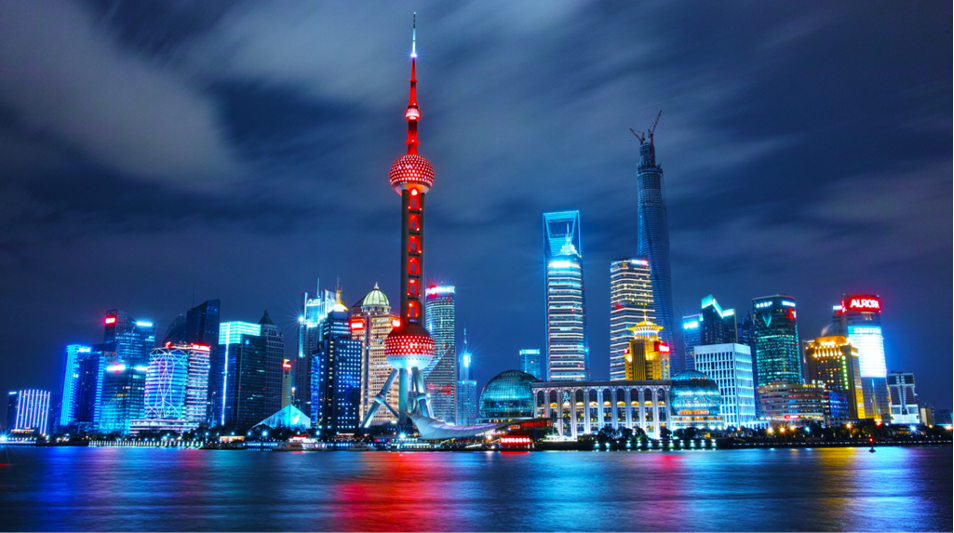
We focus on capturing emerging opportunities in four key sectors: industry, buildings, transportation, and power. We conduct innovative research on effective paths and implementation strategies for these sectors to achieve net zero emissions. In addition, we encompass a wide range of important cross-sector topics including subnational decarbonization, bilateral government climate cooperation, international knowledge transfer, just energy transitions, non-CO 2 impacts, and technology innovation policies to achieve zero emissions.
The next 30 years will be a critical period for China to achieve peaking of carbon dioxide emissions and embark on a pathway to achieve mid-century carbon neutrality. With a long and well-established network of partners in China and with our partner's strong trust, Berkeley Lab’s China Energy Program is uniquely well-equipped to continue to have a beneficial impact on achieving these goals.
The China Energy Outlook (CEO) provides a detailed review of China's energy use and trends. China is the world’s largest consumer and producer of primary energy as well as the world’s largest emitter of energy-related carbon dioxide (CO 2 ). China surpassed the U.S. in primary energy consumption in 2010 and in CO 2 emissions in 2006. In 2018, China was responsible for 21% of total global primary energy use and about 29% of global energy-related CO 2 emissions.
CEO 2020 available for download: eta-publications.lbl.gov/publications/china-energy-outlook-understanding
Contacts: Nina Khanna , Nan Zhou
- China Energy Databook, Version 9.0
- Key China Energy Statistics 2016
- Key China Energy Statistics 2014
- Key China Energy Statistics 2012
- Key China Energy Statistics 2011
Contacts: Hongyou Lu , Nan Zhou
Net-Zero Roadmap for Steel Industry
The iron and steel industry accounts for over 7% of global anthropogenic greenhouse gas (GHG) emissions and around a quarter of GHG emissions from the industrial sector worldwide. Global steel production has more than doubled between 2000 and 2018, dominated by China which produces half of the world’s steel. Energy use and GHG emissions of the steel industry are likely to continue to increase because the growing demand for steel, particularly in developing countries, is outpacing the incremental decreases in energy and carbon dioxide (CO 2 ) emissions intensity of steel production that are happening under current policy and technology regimes.
In this project, we are developing technology pathways that include demand reduction, energy efficiency, fuel switching, technology shift, and carbon capture, utilization, and storage to assess the potential of each decarbonization strategy in order to achieve carbon neutrality in China’s steel sector. We also engage with a number of Chinese collaborators, including industry associations, universities, government think tanks, and research institutes to identify and develop policy support to accelerate the energy transition in China’s steel industry.
Industry Cross-Cutting System Analysis
China’s industrial production is responsible for 70% of the country’s and 28% of the world’s energy-related carbon dioxide (CO 2 ) emissions. Decarbonization efforts typically focus on industry-specific technologies. Data on cross-cutting systems, such as steam system, process heating systems, and motors system are not publicly available for Chinese industries.
Berkeley Lab researchers developed a proxy method to quantify the energy flow by fuel types in China’s most energy intensive industries: steel, chemicals, cement, petroleum refining, and aluminum. We developed the first-ever energy flow Sankey diagrams to illustrate the energy flow-in and flow-out within each of these industries. The data can be used to support cross cutting system analysis, understanding system-level energy use and efficiency, as well as potential to decarbonize the energy use at the system-level.
Industrial Heat Decarbonization
Industrial heat accounted for about 30% of global final energy demand and 21% of global CO 2 emissions in 2018. Widely viewed as one of the most hard-to-abate sectors, industry accounted for more than one third of global GHG emissions. Industrial heat, representing for about 30% of global final energy use in 2018, primarily (81%) comes from burning of fossil fuels. CO 2 emissions associated with industrial heat production represent 21% of global CO 2 emissions, as shown in the Figure below. Renewable heat and electricity provided heat only contributed to 9% and 10% to the industrial heat production in 2018, respectively.
Figure 1: Contribution of Industrial Heat to Global Final Energy Demand and CO 2 Emissions in 2018 Sources: BloombergNEF and WBCSD, 2021; Lovins, 2021; Rissman et al.2020; Thiel and Stark, 2021.
Berkeley Lab conducts technological assessments and techno-economic analysis of key technologies to decarbonize industrial heat, including assessing industrial heat characteristics, technology potential and opportunities, barriers, and potential solutions to implement and scale up technological adoption.
Building Materials Decarbonization
Embodied emissions from building materials contributed to 17% of China’s total CO 2 emissions, emitting more than 1.4 Gt of CO 2 emissions per year. As China pledges to peak its CO 2 emissions before 2030 and reach carbon neutrality before 2060, few studies have analyzed the whether and how China can fully decarbonize its embodied emissions in the buildings sector.
Berkeley Lab developed an integrated model that combines the demand and supply of key building materials, investigated specific measures in energy efficiency, material efficiency, fuel switching, electrification, and carbon capture and storage, and quantified embodied energy and CO 2 emission pathways.
Industry Decomposition Analysis
Industry sector accounted for 65% of China’s total primary energy use and about 70% of its energy-related CO 2 emissions in 2020. It is critical to transform China’s industry in order to achieve China’s “Dual-Carbon” goals and the updated Nationally Determined Contributions (NDCs).
For many years, the Chinese government has been calling for “structural shift” as one of the key ways to modernize its economy. However, the share of gross domestic product (GDP) from the Chinese secondary (industry) sector has remained relatively flat, at around 40% from 1991 to 2019, significantly higher than other advanced countries, such as Japan (29%), Germany (28%), United States (18%), and the OECD countries (average 22%). While the Chinese tertiary (service) sector share of GDP seems to be on track to meet its goal of 56% by 2020, the most significant “structural shift” in China over the past 15 years has been the declining contribution from the primary (agricultural) sector, rather than secondary (industry) sector, as shown in the Figure below. Industry’s share of total energy use as well as energy-related CO 2 emissions in China has stayed at around 70% since 1980.
Figure 2. Share of Sectoral Value Added in China’s GDP and Value-added by Sector Sources: NBS, various years; NBS, 2019c; NBS, 2013.
Note: The secondary sector in China is defined as mining, manufacturing (not including repair of metal products, machinery) and equipment), construction, and production and supply of power, heat, natural gas and water. Primary sector includes agriculture, forestry, farming, and fishing. Tertiary sector refers to the service industry.
Berkeley Lab conducted analysis to identify drivers of China’s recent industry energy and CO 2 emissions trends, evaluated the impact of key drivers using decomposition analysis, conducted interviews with Chinese industry experts and policymakers, and provided policy recommendations to support China’s industry transition.
Contact: Hongyou Lu , Bo Shen , Nan Zhou
Codes and Standards
Mandatory or voluntary building energy codes and standards for new or existing buildings can play an important role in energy conservation. Studies indicate that efficiency standards can generate energy reductions of 20 to 40% or more. Since the 1990’s, Berkeley Lab has been working with emerging economies on building energy codes and standards development. Our work has involved training in the use of DOE-2 , EnergyPlus , and other building energy simulation software, as well as assistance in the drafting and implementation of building energy standards.
In Association of Southeast Asian Nations (ASEAN), Berkeley Lab worked with the U.S. Agency for International Development (USAID) to support commercial building energy efficiency standard development, with a focus on Indonesia, the Philippines, Singapore, and Thailand. For more, visit the ASEAN-USAID Building Energy Conservation Project .
Currently, Berkeley Lab is conducting research to support next-generation outcome-based and net/nearly zero energy building (NZEB) codes and standards. Click here for a case-study-driven review of NZEB in hot and humid climates with technology choices and design features to support future codes and standards development.
At the urban scale, we collaborated with China Green Buildings Council (GBC) to jointly develop low carbon district standards. The Chinese green district standard’s performance was compared with U.S. Leadership in Energy and Environmental Design (LEED) standards .
Benchmarking and Retrofit Targeting
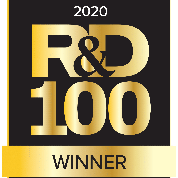
Winner of a 2020 R&D 100 Award and the 2020 Berkeley Lab Director's Award for Exceptional Achievement in Technology Transfer , BETTER utilizes minimal data inputs to benchmark a building’s or portfolio’s energy use against peers; quantify energy, cost, and GHG reduction potential for investors; and recommend measures to decarbonize and electricity individual buildings or portfolios, targeting specific savings levels. Click here to access the BETTER web application.
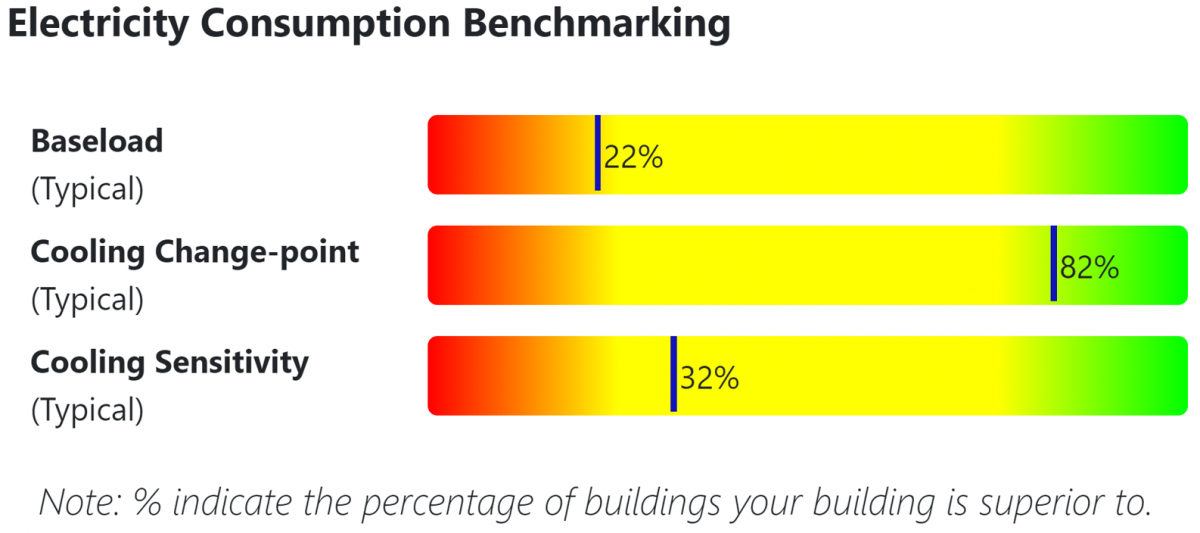
Building Sector Policy Development
Berkeley Lab is working with China, India, and other emerging economies on different aspects of building energy efficiency policy development by drawing on its expertise in the areas of: building sector modeling, electrification, operational and embodied CO 2 emissions, and voluntary program design.
Building Sector Modeling
Berkeley Lab works with emerging country national and local governments to develop, evaluate, and model the long-term impact of building energy efficiency on their economies. In 2016, Berkeley Lab developed building sector models with China National Development and Reform Commission, Energy Research Institute, and Rocky Mountain Institute for the groundbreaking Reinventing Fire China study. The modeling framework was subsequently applied to support additional cities to calculate CO 2 emission reduction potentials and to establish low emission development pathways. Berkeley Lab has also collaborated with the C40 Climate Leadership Group to investigate policy advancement in Chinese cities to decarbonize their building sector . For more on our modeling capabilities, visit Energy Modeling and Pathways .
Electrification
Berkeley Lab also develops electrification scenarios to enhance renewables adoption and decarbonize the building sector. Recently, we conducted an in-depth analysis of how electrification can help decarbonize China’s building sector. It was determined that by switching cooking, water heating, and space heating fossil fuel energy to clean energy electricity, China could significantly reduce its building sector CO 2 emission from 4,000 metric tons to 1,000 metric tons and achieve a sectoral electrification rate approximately 75%.
Embodied CO 2 Emissions
In addition to analysis of energy and CO 2 emissions from building operations, Berkeley Lab works actively to analyze and address emerging economies’ building sector embodied CO 2 emissions from materials and construction. For more on this work, see a recent joint study with Tsinghua University which found that embodied CO 2 emissions from building construction and materials in China was approximately 1600 metric tons and accounted for 17% of the country’s total CO 2 emissions in 2015. When combined with building operations, China’s total building sector CO 2 emission grew to 3900 metric tons and accounted for 41% of China’s total CO 2 emissions in 2015.
Voluntary Program Design
Best practices for high performance buildings are demonstrated and disseminated through Berkeley Lab’s international building sector research network. The Better Buildings Challenge (BBC) - China program is an example of a program Berkeley Lab helped establish to facilitate building owners and managers to voluntarily set and achieve ambitious energy savings targets by implementing operational and technological best practices.
High Performance Building Design and Operation
Berkeley Lab is home to the world’s most advanced integrated building and grid technologies testbed: the Facility for Low Energy Experiments in Buildings (FLEXLAB), which allows users to develop and test energy-efficient building and grid technologies individually or as an integrated system, under real-world conditions. Berkeley Lab supported the development of an international version of FLEXLAB in collaboration with the Singapore Building Construction Authority (BCA). The testbed, called Skylab , is designed to experiment with integration of high-performance technologies in buildings and to provide researchers and the public with data and visibility into the built environment.
Berkeley Lab also works with partners in emerging economies to assess sustainable design and low carbon building operation. See our recent publication, Model for China’s Future , which provides a case study of the Shenzhen Institute of Building Research (IBR) Headquarters - a living laboratory and has proven to be a model building for sustainable design throughout the world.
Direct Current (DC) Power and Renewable Integration
As solar renewable energy and battery storage are all operated in DC power, developing a DC power distribution system to better integrate with renewable energy in buildings is becoming increasingly important. Berkeley Lab works collaboratively with industry and researchers to develop DC power pilot buildings in the U.S. and internationally. Studies have shown that DC power distribution in buildings has less system wide energy loss , and can also yield other benefits such as power safety, smart control and making buildings grid-friendly. Visit our publications page to learn more about our work in DC power and renewable energy integration.
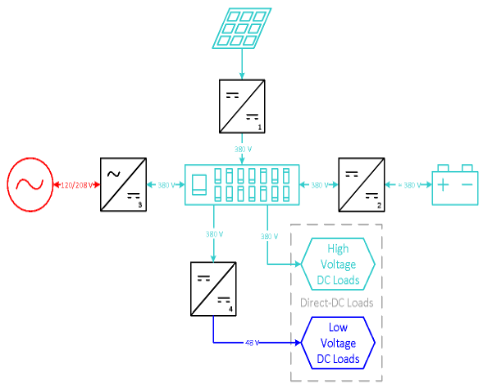
District Energy Systems
Berkeley Lab developed a district energy planning tool, known as DEEP, for campus and small district scale energy planning to benefit the United States and emerging economies. The tool integrates heating, cooling, and electric loads with low energy resources. It calculates the most suitable technologies for district solutions. Equipment cost and heating and cooling pipelines are considered to help users optimize the topology of the system and find the best technological solutions for a community. DEEP provides free cloud-based online computational solutions.
Indoor Environmental Quality
As numerous emerging economies are experiencing severe air quality issues, mitigating indoor environmental pollutants and protecting occupants has become a critical component of high performance buildings research at Berkeley Lab. One person spends 90% of his/her time indoors. Thus, providing occupants, especially vulnerable groups like children and elderly, with good environmental air quality is extremely important.
Berkeley Lab conducts research to apply air filtration technologies to the ventilation system in buildings to reduce indoor occupants’ exposure to particulate matter. We also conduct research to develop air-cleaning technologies to remove pollutants such as Volatile Organic Components (VOC). In response to the COVID-19 threat, Berkeley Lab is also studying the drivers of airborne contaminants and particle transmission in the indoor environment. High resolution numerical simulations and experiment tests are being conducted to learn the contaminant distributions and transport mechanism. For more, visit Berkeley Lab’s Indoor Environment Group .
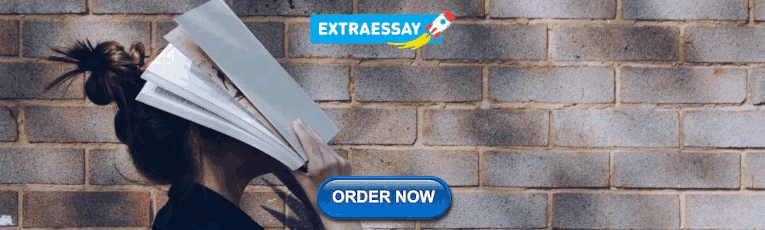
U.S.-China Clean Energy Research Center for Building Energy Efficiency (CERC-BEE)
The U.S. Department of Energy (DOE) U.S.-China Clean Energy Research Center for Building Energy Efficiency (CERC-BEE) program is a ten-year initiative directed by Berkeley Lab to support leading scientists from the United States and China in research to accelerate the development and deployment of advanced building technologies for real world impact.
CERC-BEE involves over 20 U.S. companies and 60 world-class scientists and focuses on technology innovation, evaluation, and real-building field validation. Through 2020, CERC-BEE has delivered 17 new products, 20 new copyrighted software tools, and 84 peer-reviewed publications. Numerous CERC-BEE technologies have earned prestigious awards, including: 2020, 2016, and 2013 R&D 100 Awards, a 2020 Berkeley Lab Director’s Award for Technology Transfer, a 2019 Keeling Curve Prize Honorable Mention, a 2019 HIVE 50 Award, a 2018 Best of Design Award for Digital Fabrication and a 2016 Gold Edison Award.
For more information, visit the CERC-BEE website .
Passive Houses and Net-Zero Energy/Emission Buildings
We provide policy strategies to enhance the deployment of sustainable solutions in buildings through energy-efficient design and renewable energy integration. Buildings account for almost 30% of global CO 2 emissions. Large savings in energy use (75% or higher) are possible in new buildings through better designs. The passive house standard is the most rigorous energy efficient building code today. Passive house standard provides a premise for meeting net-zero energy/emission building goals.
Offsite Construction
As buildings become increasingly efficient, the embodied emissions generated from the production of building materials and construction processes will become more prominent. For highly energy-efficient buildings, the share of embodied GHG emissions over buildings’ life cycle could escalate to 45-50% and surpasses 90% in extreme cases. One of the ways to reduce embodied GHG emissions is by employing the offsite construction (OC) approach. OC offers opportunities to reduce waste, test sustainable materials, and produce highly energy-efficient products and units in a factory-controlled environment.
Viewing OC as a catalyzer to accelerate building decarbonization, we provide comprehensive research programs to help address industry barriers. These include laboratory testing for new design, product, and construction management processes, start-up incubation, firm innovation pattern analysis, financing models, policy design and evaluation, and life-cycle analysis for carbon emissions and costs, etc.
Contact: Wei Feng , Carolyn Szum , Jingjing Zhang , Nan Zhou
A clean-power future benefits the U.S., China and the world.
The China Energy Group conducts joint technical research, pilot demonstrations, and policy analysis on pathways to clean power system, power sector market reform, demand response (DR) and demand-side management (DSM), integration of renewable energy, distributed energy resources (DER), and microgrids with partners in both the U.S. and China.
Pathways of clean power transition: In this research area, we assess technically feasible and economically viable pathways for transitioning to a zero-emissions power sector in China. One of our recent studies finds that more than 60% of China's electricity could come from non-fossil sources by 2030 at a cost that is about 10% lower than achieved through a business-as-usual approach (figure below), given rapid cost decrease of renewable energy technologies.
We have also examined the resource, economic, and institutional implications of reducing and replacing coal generation in China with mostly renewable energy by 2040. We find that, to do so, it will require a rapid scale-up of zero-emission resources, a transformation of planning, market, and regulatory institutions in its electricity sector, and a policy commitment to restrict investment in new coal generation after 2020.
Power sector reform: the electricity market is likely to play an increasingly important role in more efficiently allocating resources, integrating renewable energy, and shaping future investment decisions as China considers its transition to a zero emission power system. In the area of power sector reform collaboration, our research, sponsored by the State Department’s Bureau of Energy Resources and others, focuses on relevant U.S. and Chinese experiences on renewable energy utilization, DR, and wholesale and retail market choices, with a view towards China's replicating/adopting lessons learned across provinces. The activities included information-gathering on, sharing of international best practices, and visit/exchanges that explore current conditions and challenges in each pilot province/city, assessing specific pilot challenges, recommendations, and joint development of policy recommendations on the pilots and broader, market-opening reforms in China's power sector. Some of our recent studies have examined the economic and environmental benefits of the electricity market in China’s southern grid region and the Guangdong province . Our recent collaborative research also examined the need to coordinate the Chinese carbon market and the renewable certificate market.
The China Energy Group works closely with China National Energy Administration (NEA) on its microgrid and distribution generation policies. During the 12th Five Year Plan, LBNL worked with NAE’s affilated Chinese institutes to develop a microgrid development technical guidance and policy recommendation for microgrid demonstration projects.The U.S. and international microgrid experience was extracted and analyzed as an example for China to develop its own policy.
During the 13th FYP, based on LBNL and its partners’ policy and technical research, NEA issued a policy to build nationwide “Internet+ Smart Energy DemonstrationsProject”. We are working with China to conduct benefits analysis of microgrid demonstration projects. We are also using Berkeley Lab's Distributed Energy Resource Customer Adoption Model (DER-CAM) and District Energy Planning (DEEP) to help optimize design and operation of microgrids, especially in building and campus.
LBNL also actively integrates microgrid research with other smart grid areas such as demand response. Research showed that a demand response enabled microgrid can dispatch clean energy efficiently and bring benefits to the microgrid and the whole power grid.
Demand-Side Management (DSM) and Demand Response (DR)
Demand-side management (DSM) has been one of the areas of China's electric power research that we have focused on. In recent years, our focus has been on improving grid flexibility through demand response (DR) and demand-side resources. LBNL researchers have been studying international best practices and effective mechanisms to promote development of DR. We have also conducted joint end-to-end DR automation testing to demonstrate the integration of utility DR management systems, DR products, and end-use control sequences with standards-based DR signals to accelerate acceptance of manufactured DR products in both countries (sponsored by Department of Energy’s Office of Electricity).
LBNL worked with State Grid and Southern Power Grid through the U.S.-China Climate Change Working Group (CCWG) since 2013. In the CCWG platform, LBNL and its Chinese counterparts developed a smart grid benefit analysis method to calculate techno-economics of smart grid investment at power distribution level. A joint smart grid collaboration white paper is published. In recent CCWG collaboration, LBNL worked with State Grid and Southern Power Grid to test demand response technologies and protocols. The U.S. and Chinese partners tested typical demand response related technologies including: Variable Frequency Drive (VFD), Thermostat, Lighting and smart meters. LBNL tested DR performance in a typical building setup using its Flexlab testbed.
In this area, we are also conducting studies to develop recommendations on enhancing DR value by integrating DR into power system dispatch procedures, maximizing DR potential through tapping opportunities beyond peak load management, and creating roles for load aggregators to deliver greater DR value. Our research in this area also focuses on examining how effective tariffs and compensation incentives help increase DR in the US and China. Recently, in collaboration with Chinese researchers, we have provided a series of recommendations for the formulation of China’s 14th Five-Year Plan demand-side management work plan.
A number of articles and research reports reflect our diverse research in this area, including: Integrated DR in intermittent wind power generation and local power energy demand; enabling demand response in district energy systems for energy retail and wholesale; sharing demand-side resources among multiple prosumers, applying frequency regulation demand response in industrial load and demand dispatch . Resilient modeling of community energy system integrated with demand response.
Contact: Bo Shen , Wei Feng , Jiang Lin
China's fast-growing transport sector is a key driver of the growth of national energy demand, particularly for petroleum products, despite significant progress in efficiency improvements and fuel switching. While China leads in electrifying its passenger vehicle fleet, decarbonizing heavy duty trucks remains a key challenge. Our team explored and quantified the potential impacts of different vehicle and operational efficiency, electrification and other fuel switching strategies for reducing diesel demand, and related CO 2 emissions and air pollutants, from China’s heavy-duty trucking sector.
Heavy-duty trucks in the road freight sector are a growing area of focus for reducing transportation-related oil consumption and greenhouse gas emissions because of this sector’s disproportionate environmental impacts and the technical challenges of mitigating them. Read more:
Near and long‑term perspectives on strategies to decarbonize China’s heavy‑duty trucks through 2050
Contacts: Nina Khanna , Nan Zhou
We employ rigorous modeling and social science methodologies, as well as program implementation to inform energy and climate policies that help to accelerate building and urban sustainability transitions.
Subnational climate action support
Over the past 35 years, we have undertaken both analytical and on-the-ground program implementation to develop and introduce clean energy and climate mitigation resources to urban researchers and policymakers. This included training over 600 researchers and officials from more than 20 Chinese cities in the application of our guidebooks, strategies, and tools. China Energy Program also served as the U.S. Secretariat for The Climate-Smart / Low Carbon Cities Initiative of the US-China Climate Change Working Group (CCWG). The U.S. and China have both announced their own carbon neutrality targets in 2020. To realize these ambitious goals, implementing climate actions at the subnational levels will be key to success in the 2020s. Based on our earlier work developing China Green Low-Carbon City Index for more than 100 Chinese cities, we will continue to develop data infrastructure, research, and peer-learning programs to accelerate carbon-neutral urban transformation in both countries.
Energy innovation decision science
We apply surveys, interviews, and econometric analysis approaches to support the development and deployment of cleantech sector strategy by analyzing:
- Technology learning and policy diffusion: how and why sustainable technology, practices, and policies diffuse into the society over time;
- Users and adoption: understanding human behaviors and patterns in adopting demand-side innovations, including commercial demand response programs and energy efficiency home upgrades;
- Innovation database: we compile and maintain a comprehensive innovation database to support energy and climate modeling and decision making, particularly focusing on technological and social innovations that help to reduce and manage energy demand.
Contact: Jingjing Zhang , Stephanie Siehr , Nan Zhou
Energy Efficiency Financing
Today, China has the most comprehensive policy package in the world to advance green financing. Despite this, due to historical reliance on grants and subsidies to advance China’s energy and emissions reduction goals, there are still significant barriers to leveraging private capital for energy efficiency, resulting in the absence of energy efficiency as an asset class and slowing market adoption of advanced energy efficiency technologies.
Berkeley Lab is working with China in the financing space to:
- Uncover market and technical barriers to energy-efficiency financing
- Build financial institution capacity on advanced building technologies that represent business and financial opportunities
- Develop and pilot innovative energy-efficiency financial products
- Analyze data on the performance of energy-efficiency financial products in order to continue to improve product performance and market uptake
Berkeley Lab’s leadership on building energy efficiency financial product research and development was recognized with a 2019 Keeling Curve Prize Honorable Mention for innovation in financing tools and techniques. For more on this work, read one of our recent publications.
Contact: Bo Shen , Carolyn Szum
Since coal accounts for more than half of China's energy mix, the Chinese coal industry plays a pivotal role in influencing the trajectory of climate change. Driving the transformation of the Chinese coal industry will be key to achieving China’s carbon neutrality goals and Paris Agreement targets. However, unlike industrialized countries where the coal industry's dwindling share of energy production makes the impact of coal phase-out more manageable, China today employs millions of people in coal-related industries and many Chinese cities depend on coal related activities. If the coal industry lags behind in China's ongoing energy transition, millions of jobs will be affected. Therefore, promoting a just transition is crucial for China, especially its coal industry.
The coal industry occupies an important place in China's energy system, and failure to integrate the industry into energy transition efforts could lead to coal industry resistance to change, creating challenges for transition. Our researchers have been working with institutions in the Chinese coal industry to introduce global best practices in policy, technology, marketization, and implementation to drive a just energy transition. We are also providing technical assistance to support Chinese coal industry's efforts to create a bottom-up and insider approach that can help accelerate the transformation of China's coal industry, as it enables the coal industry to align its transition needs with China's carbon neutrality goals.
Contact: Bo Shen
The U.S.-China Climate Change Working Group (CCWG), first launched in April 2013, is a comprehensive framework for facilitating constructive U.S.-China cooperation and dialogue on climate change.
international.lbl.gov/CCWGcities
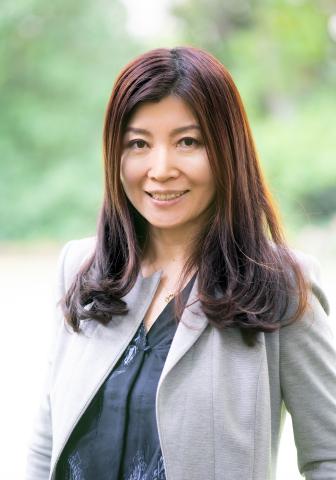
- Open access
- Published: 18 January 2024
Renewable energy as a connecting spot between China and Central and Eastern European countries: status, directions and perspectives
- Nevena Šekarić Stojanović 1 &
- Katarina Zakić 1
Energy, Sustainability and Society volume 14 , Article number: 10 ( 2024 ) Cite this article
1543 Accesses
1 Citations
1 Altmetric
Metrics details
Considering energy- and climate-related policies adopted, the European Union and the People’s Republic of China are expected to be on the same trajectory of reducing pollution, aiming for carbon neutrality in 2050 and 2060, respectively. However, although they share a common goal of more sustainable development, their targets and means often collide. The main objective of the study is to identify the main similarities and differences in approaches to energy and climate policies in the European Union and the People’s Republic of China, with special attention given to the scope, past, present, and future of Chinese investments in renewable energy projects in the countries of the Central and Eastern European region, and to reveal the prevailing factors of the (un)successful renewable energy projects in those countries eventually. The methods used are literature review and qualitative content analysis of the European Union’s and the People’s Republic of China’s energy- and climate-related policies according to the prescribed indicators (from 2005 onwards) and in-depth exploratory desk research of cooperation in renewable energy projects between the People’s Republic of China and 14 Central and Eastern European Countries (from 2014 onwards).
The study showed that despite the significant alignment of the European Union’s and the People’s Republic of China’s energy- and climate-related policies on a normative level, renewable energy cooperation between the People’s Republic of China and Central and Eastern European countries on a practical level is moderate. This state of play mainly results from political factors, such as rising levels of Sino-scepticism and the overall deterioration of the European Union–People’s Republic of China relations.
Conclusions
The study showed that political rather than economic or legal factors had a great impact on the Chinese presence in the domain of renewable energy in the countries of the Central and Eastern European region. However, the significant alignment of the European Union’s and the People’s Republic of China’s energy- and climate-related policies and dedication to common energy transition targets offer room for improving renewable energy cooperation. Overcoming political and economic divergences imposes a condition for achieving better cooperation in the renewable energy domain.
With the post-pandemic environment and, more recently, the warfare started by the Russian invasion of Ukraine, the energy transition on both global and regional scales started to take the connotation of urgency. Decarbonisation is a complex and long-term development process where a significant role belongs to renewable energy sources (RES), making them one of the main pillars of sustainable growth in the future. Increased interest in their usage, especially after 2022, resulted in gaining the status of a new geopolitical asset and one of the crucial tools in the new energy competition, so they could be observed as “a game changer for interstate energy relations” [ 1 ], presenting thus one of the most exploited themes not only in the domain of energy and industry but also in political and security context [ 2 ]. Within this context, one cannot disregard the geopolitical repercussions of the energy transition—critical materials that run renewable energy infrastructure (rare earth materials, nickel, cobalt, lithium, etc.) become a source of rivalry among powers. As Criekemans [ 3 ] stated, “cooperation is possible, but never a given”.
However, although having a strong international competition impetus, some studies have shown that renewable energy sources have a solid potential to “de-geopoliticalise international relations and allow states to transcend the ‘zero-sum’ thinking in their pursuit of energy security” [ 4 ]. In this context, traditionally marked as colliding, the European Union–People’s Republic of China (EU–PR China) energy-based relations started to emerge as aligning with each other due to significant commitments to renewable energy (RE) and energy transition targets. As stated in Liu et al. [ 5 ], the EU and PR China are responsible for approximately one-third of global greenhouse gas (GHG) emissions together, which makes their partnership one of the most important in the ongoing energy transition, with consequences of global importance. Since both actors are among the key players in contemporary energy and climate global governance and are undergoing the energy transition, analysing their relationship in these areas from diverse perspectives becomes very important.
Renewable energy sources have been increasingly used since the launch of the Paris Climate Agreement in 2015. While RES in power generation took 2.8% of global energy consumption in 2015 [ 6 ], they reached almost 13% in power generation in 2021 [ 7 ]. Within this context, PR China remained the main driver of solar and wind capacity growth during 2021, accounting for about 36% and 40% of the global capacity additions, respectively [ 7 ]. On the other hand, the share of renewable energy more than doubled between 2004 and 2021 in the EU, reaching 21.8% of energy consumed in 2021 [ 8 ]. More significant is that RES generated 38% of the EU’s electricity in 2020, overtaking fossil-fired power generation for the first time, which fell to 37% [ 9 ]. With a 20% fall in coal power generation and a 4% fall in gas power generation in 2020, Europe’s electricity was 29% “cleaner” than in 2015 [ 9 ]. This renewable energy source’s rise in electricity production was mostly powered by wind or solar energy. In addition, European policies see RES as mutually beneficial: while presenting one of the primary tools in energy transition and a goal per se , they are also seen as one of the mechanisms to guarantee the security of supply in the context of dwindling reserves of fossil fuels [ 10 , 11 ] which gained added value in the context of the ongoing Russia–Ukraine conflict. Bearing in mind its ambitious climate agenda, where the status of the first carbon-neutral continent by 2050 is one of the most critical goals, the recent integration of the EU’s energy and climate policies should be considered as a result of the evolution of the EU’s actions aimed at achieving energy transition goals [ 12 ]. Nevertheless, it is not negligible that certain European countries historically, as one of the leaders of the industrial revolution, contributed most to the GHG emissions and continue to be among the biggest emitters at present [ 13 ].
PR China had long based its policy on the developing country card and “right to develop” regarding GHG emissions [ 13 ]. However, domestic pollution from industry activities was the main reason for PR China to redirect its activities towards greener options [ 14 ]. Already high levels of urbanisation, rapid economic development, and PR China’s opening to the world, brought environmental issues very high on the agenda. When observing data on the global level, PR China has the most extensive carbon dioxide emissions coming from the energy sector, generating almost more than 31% of all global emissions in 2021 [ 7 ]. On the other hand, PR China is the most prominent leader in RE investments. In 2022, PR China invested 546 billion dollars in clean energy, far surpassing the EU with 180 billion and the United States of America (USA) with 141 billion dollars [ 15 ]. Within the 2014–2022 period, PR China invested 121.3 billion dollars in renewable energy projects, as stated in China Global Investment Tracker (CGIT) [ 16 ], which made it one of the global leaders in the domain of RE investments.
Since the USA’s withdrawal from the Paris Agreement, the burden of the fight against climate change has been transferred to the EU and PR China as powers with significant economies and ecological footprints. Energy transition and renewable energy are seen as integrative tools for the future reconstruction of sectoral politics, and there are obvious aspirations in the EU and PR China to push the energy transition through other departments, such as industry, transport, construction, food, etc. Bearing this in mind, the common denominator of the EU’s and PR China’s XXI century energy policies became a low-carbon future or, more precisely, carbon neutrality, with the EU aiming to reach it by 2050 and PR China by 2060. Besides, their mid-term goals showed significant commitments towards energy transition—both the EU and PR China plan to increase the share of renewable energy in total energy consumption to 45% and 25%, respectively, by 2030 [ 17 ]. Nevertheless, the energy transition and proposed targets are facing many challenges. Significant reliance on imported fossil fuels, further complicated by the ongoing Russia–Ukraine conflict and polarisation of the world when it comes to this issue, questioned the planned dynamics of the energy transition of both actors. Within this context, energy and climate cooperation between the EU and PR China could be observed as a challenging but mostly desirable type of cooperation with significant potential to contribute to mitigating geopolitical tensions as it is based on a common goal of reducing GHG on a global level.
When discussing the potential alignment of the EU’s and PR China’s energy- and climate-related policies and strengthening their cooperation based on RES, it is worth noting that PR China develops relations with the EU as a supranational organisation but also with its individual member states. One of the possible ways to map PR China’s cooperation based on RES with individual European states is to analyse their relationships within the PR China–Central and Eastern European Countries (CEEC) cooperation framework, which was established by PR China in 2012. Namely, this cooperation framework should be understood as a part of a broader Chinese pragmatic strategy [ 18 ] aimed at achieving Chinese economic and environmental goals while simultaneously presenting a group of EU Member States or candidate states, meaning they are functioning (or tend to do so) according to the EU energy acquis communautaire . Thus, this cooperation framework could serve as a solid background for questioning the current state of play , challenges, and perspectives of PR China–CEEC renewable energy cooperation and, consequently, cooperation on Chinese renewable energy projects across Europe.
Official documents and statements recognise the importance of a mutual Sino-European relationship [ 19 , 20 , 21 ], with the area of renewable energy included in this notion. However, this normative cooperation seems far from the one in practice. Therefore, this article aims to explore the current state of play and challenges in the EU’s (CEEC) and PR China’s renewable energy cooperation at present and in the future. A review and comparative analysis of the EU’s and PR China’s energy and climate-related policies should serve as a base for concluding whether their policies tend to align on a renewable energy basis, observing from this normative level. On a practical level, this alignment will be tested on the PR China–CEEC cooperation framework.
The study is focused on two research questions regarding the (potential) normative alignment of the EU’s and PR China’s energy- and climate-related policies in the domain of RE and its influence on the renewable energy cooperation between PR China and CEEC on a practical level. Since the aim of the study demands different methodological tools to answer two research questions, the main methods of data collection and analysis used are literature review, qualitative content analysis, and in-depth exploratory desk research of cooperation in renewable energy projects between PR China and CEEC.
Literature review and qualitative content analysis refer to the analysis of the contemporary EU’s and PR China’s energy- and climate-related policies to identify their possible alignment on the normative level, led by several indicators: targets of the energy transition process, energy transition deadlines, the role of renewable energy sources in energy transition, and methods of achieving energy transition targets. The concept of alignment in this study should be understood as a rather content-neutral concept that does not “prejudge conceptually the type of cooperation involved”, i.e. as “an all-encompassing ‘umbrella’ concept” [ 22 ] that offers an analytical environment for studying different types of cooperation.
Considering the specific scope and aim of this study, the alignment, in this particular case, entails renewable energy cooperation between PR China and CEEC on two levels. On a normative level, this cooperation was assessed by analysing the EU’s and PR China’s energy- and climate-related policies to reveal the main similarities and differences in their renewable energy approaches through prescribed indicators. On a practical level, the renewable energy cooperation was evaluated by conducting an in-depth case study on PR China’s renewable energy investments in Central and Eastern Europe (CEE) region. This region was chosen as a case study for two main reasons. Firstly, PR China established the PR China–CEEC cooperation framework in 2012 as a prominent part of its geo-economic strategy of being more present in this part of Europe. Secondly, the CEE region comprises the EU Member States, as well as candidate states, that base their national legislation and policy approaches on the EU energy acquis communautaire , so the EU’s energy approach is considered a prevalent context within which the CEEC and other external actors interact. This diversity of European countries, which exists within the same or approximately similar energy context under the EU energy and climate umbrella and simultaneously belongs to the PR China–CEEC cooperation framework, were the main reasons to question the scope, past, present, and future of PR China–CEEC renewable energy cooperation.
The second method refers to the case study based on in-depth exploratory desk research on PR China–CEEC renewable energy cooperation. To answer the second research question, Zakić and Šekarić study on Chinese energy investments in CEEC [ 18 ] was updated and subsequently subjected to a qualitative, descriptive analysis to discuss the results of Chinese renewable energy investments in CEEC. This study thus follows a hypothetico-deductive pathway arguing that dedication towards energy transition targets and alignment of strategies based on RES should bring higher levels of practical cooperation between PR China and CEEC. As already stated, PR China–CEEC cooperation is chosen as a case study due to CEEC’s diverse structure, comprising EU Member States and candidate countries, so any change in PR China’s involvement in renewable infrastructure investments towards these two groups could be empirically evaluated.
For the first research question, a review and comparative analysis of the contemporary EU’s and PR China’s energy- and climate-related policies Footnote 1 were conducted and existing cooperation mechanisms were tackled to identify potential alignment according to the prescribed indicators. The first step was to target the main strategic documents regarding energy and climate issues, as believed that they could describe mid- to long-term commitments in the best way due to their strategic nature. Since the end of the 1990s and the beginning of the XXI century, together with the Kyoto Protocol (1997), the Stern Review on the Economics of Climate Change (2007), and the Paris Agreement (2015), which marked the promotion of RES as the main tool in achieving energy transition, this period is considered in the context of the EU’s and PR China’s energy- and climate-related policies.
More precisely, the analysis covered the period from 2005 onwards, as the first concrete activity in this area—the Joint Declaration on Climate Change—has formally marked the EU–PR China relationship in the domain of global climate governance. The cooperation between the EU and PR China in this domain started with a sectoral energy dialogue in 1994. However, it was in 2005, after the 8th EU–China summit meeting, that the dialogue was institutionalised with the establishment of the EU–China Partnership on Climate Change. Besides, concrete energy- and climate-related policies undertaken by both individually were those starting from the years mentioned.
Secondly, an analysis was conducted to trace the representation of “renewable energy sources/renewable energy/renewables” within those documents, particularly in connection with the external dimension of their policies. By searching for similarities, potential differences and points of contention were also explored in order to map the main challenges in cooperation between these two subjects in the domain of RE.
After a brief historical overview of the development of their policies and cooperation, the study focused on the main similarities between the EU’s and PR China’s contemporary energy- and climate-related policies in order to identify possible points of alignment.
For the second research question, PR China–CEEC cooperation in the domain of energy infrastructure investments was examined, with a particular focus on RE investments. This analysis covered a 9-year-long research period—from 2014 (when the PR China–CEEC cooperation framework started to operate) to 2022. Collected data were sourced using relevant national and international sources, and the research sample consists of 14 states in the CEE region—the Republic of Albania, Bosnia and Herzegovina, the Republic of Bulgaria, the Czech Republic, the Republic of Croatia, Greece, Hungary, Montenegro, the Republic of North Macedonia, Poland, Romania, the Republic of Serbia, the Slovak Republic, and the Republic of Slovenia. It should be noted that the Baltic States withdrew from this cooperation framework and were not included in this research. Although Greece was not part of the original PR China–CEEC cooperation framework, there are many projects in the renewable energy field on which the Greece and PR China cooperated, which put Greece into the analysis.
The starting point for the analysis was CGIT, a database founded by the American Enterprise Institute and the Heritage Foundation on Chinese investments abroad. However, it is important to highlight certain database limitations for objective reasons. First of all, since the CGIT has flaws and does not register all transactions, a new database that will be more precise and cover even smaller RE investments was proposed (see “ Appendix ”). In addition, the background of every disputable or failed RE project was investigated to identify the causes of such outcomes, where applicable. In conducting research, all available primary, and secondary sources (official announcements from different governments, media reports in local languages and English, scholarly articles, and existing databases) were used to ensure the accuracy of the analysis.
Renewable energy in the EU’s and PR China’s energy- and climate-related policies
Considering the first research question regarding the (potential) normative alignment of the EU’s and PR China’s energy- and climate-related policies in the domain of RE, this subsection is dedicated to presenting results from a conducted literature review and qualitative content analysis of their policies and cooperation mechanisms.
The literature has offered significant insights into existing and future cooperation between the EU and PR China when it comes to the RE sector. Some authors stated that common elements of the EU’s and PR China’s environmental policies are determined by international processes, acknowledgment of the necessity of dealing with global environmental issues, and international contracts; still, the main differences come from the social and economic development circumstances [ 23 ]. In a similar manner, Altun and Ergenc [ 24 ] describe Sino-European relations in the renewable sector as a dialectical “collaboration–competition nexus” consisting of both consensus and contention. For Dupont [ 25 ], Sino-European relations are deeply grounded in “climate geopolitics” and are determined by the “rhetoric of competition”.
While some authors emphasised irreconcilable differences in their philosophies and political approaches [ 26 , 27 ], others highlighted the necessity of fostering EU–PR China energy and climate cooperation [ 17 ]. Liu et al. [ 5 ], thus, see the necessity of stronger energy cooperation between the EU and PR China in the future “for meaningful climate change mitigation and adaptation”, regardless of the many political and economic differences. In addition, Stensdal and Heggelund [ 28 ] see energy and climate as two main opportunities for increased cooperation between the EU and PR China. While the above-mentioned differences arguably cannot be neglected, the latter views generally rest on the benefits of the energy transition and common interests that go far beyond any particular or (supra)national interest.
A summary of the main EU’s and PR China’s energy- and climate-related policies historically (starting from 2000) as one of the outcomes of this study could be seen in Table 1 .
As could be seen, starting in 2005, the EU and PR China have adopted relevant policies in connection with RE almost simultaneously. This is somewhat expected due to their commitments contained in international agreements in the RE field. However, this renewable energy policy development was not linear from the beginning. While the official start of the development of the EU’s climate-related policies could be traced back to the 1970s (though with very limited concrete objectives and mechanisms to achieve them [ 29 ]), the beginning of the XXI century was significant for PR China in the context of the rapid development of renewable energy policies. Although some rudimentary forms of renewable energy could be traced back to the early 1950s with the development of the first small hydropower plant (HPP), there were no systematic renewable energy policies in PR China until the late 1990s, when PR China’s renewable energy policies started to make “some long-term plans, and to formulate specific development goals” [ 30 ]. Severe floods that hit PR China in 1998 in the Yangtze River basin, for instance, were an “urgent call” to protect the natural environment and to undertake necessary measures [ 31 ].
With the arrival of the XXI century, encouraging messages on the usage of RES within several politics have been replaced by concrete actions towards achieving a greater share of RES in PR China’s industry. More specifically, with the “10th Five-Year Plan for the Development of New Energy and Renewable Energy Industry” released in 2002 and with the introduction of the Renewable Energy Law in 2005 (and its revision in 2009), PR China progressively undertook supporting policies and measures in order to achieve sustainable development in years to come [ 32 ]. This Renewable Energy Law made RES a priority from the legal standpoint, made renewable energy a “preferential area for energy development” in PR China [ 33 ], and is very often marked as an initial step of the upcoming period of rapid development of Chinese renewable energy policies [ 30 ].
Ecological civilisation philosophy, introduced in 2007 by President Hu Jintao, became one of the leitmotifs of Chinese environmental policies in the upcoming period. The Chinese government’s “Opinions of the Central Committee of the Communist Party of China and the State Council on Accelerating the Construction of Ecological Civilization” from 2015 put the philosophy concept into concrete actions and integrated it into all sectors of society [ 34 ]. In 2020, the National Energy Administration (NEA) released a draft of the Energy Law of the PRC that should establish a legal base for the development of PR China’s energy sector towards more green and low-carbon options. However, this draft has been largely criticised due to its non-transparency of the data used, its long-term preparation (13 years at the moment of its release), and its unclear outlook towards the future of fossil fuels [ 35 ]. Through these legislative frameworks, combat against climate change, energy transition, and sustainable growth have been given national priority.
Some studies showed the significant impact of domestic policies on external actions linked to energy and climate issues [ 36 , 37 ]. As the Chinese energy- and climate-related policies entered a new development stage after 2005, the two five-year plans (FYP) for National Economic and Social Development that followed showed the importance of RES for energy transition in PR China, with profound consequences for its external energy and climate actions. The first one was the 11th FYP (2006–2010), with objectives of reducing energy intensity and achieving a greater share of RES in energy consumption objectives. The second one was the 12th FYP (2011–2015), which imposed a reduction of carbon intensity per capita GDP as a binding target for PR China’s economy. As an instance, in 2019, CO 2 emissions per capita GDP were reduced by 48% from 2005 levels; for the same period (2005–2019), coal consumption dropped from 72.4% to 57.7%, while non-fossil fuels in primary energy rose from 7.4 to 15.3% [ 34 ]. Since then, each subsequent FYP has had to include this target as the number one priority. The 13th FYP (2016–2020) followed previous ones in energy conservation and carbon reduction targets and established vast tax and financial incentives for environmental actions. The so-called “Goal 3060”, aimed at peaking CO 2 emissions before 2030 and becoming a carbon-neutral country by 2060, was the latest ambitious goal launched in 2020 by Chinese President Xi Jinping as part of updated Nationally Determined Contributions (NDC). This is often called a “new normal” era in PR China’s development [ 5 ] that relies on more sustainable growth. In addition, the 14th FYP (2021–2025), released in March 2021, set the goal of reducing 18% of carbon dioxide emissions per unit of GDP by 2025 [ 38 ]. However, it is important to stress that this new plan has no targets for a coal phase-out. It clearly states that it will promote “the replacement of coal with electricity” and “reasonably control the intensity of coal development” [ 38 ] but without a clear statement for coal phase-out.
Shortly after, at the 76th Session of the United Nations General Assembly in September 2021, the Chinese President promised that “China will step up support for other developing countries in developing green and low-carbon energy and will not build new coal-fired power projects abroad” [ 39 ]. Yet, at the COP26 in November 2021, PR China stirred up the public with its protesting against the term “phase out” and advocating the term “phase down” carbon emissions [ 40 ], which explicitly reflected its national interest in gradual energy shifts. In a similar manner, PR China’s “14th Five-Year Plan for a Modern Energy System”, released at the beginning of 2022, is sending mixed messages—while promoting renewable energy development, it clearly states the importance of coal in ensuring its energy security in the following years. As believed, this springs from “the government’s attempts to find a balance between ensuring short-term supply security and laying the foundation for the longer-term transition” [ 41 ].
Quite the opposite, the EU was one of the pioneers in the domain of renewable energy policies; as stated in the literature, the EU Member States “have historically been at the forefront of climate policy initiatives” [ 25 ], and today they are among the leaders in renewable technology development—according to the UNCTAD report [ 42 ], seven European countries were among the 20 top countries with the most foreign direct investment (FDI) outflows in renewable energy in 2021 and 2022. Namely, the EU started to develop its environmental legislation during the 1970s. At the same time, the protection of the natural environment was also the subject of the EU’s fundamental treaties. As stated in the literature, before the 1990s, the promotion of RES came mainly “from national programmes in a few pioneering EC countries”, such as Germany, Denmark, and the Netherlands [ 43 ]. The following period was shaped by many contextual factors that paved the way for developing the EU’s energy- and climate-related policies, including global order shifts and the securitisation of many issues. Thus, the beginning of the 1990s saw greater concerns over climate change, resulting in more promotion of RES on a supranational level. A key turning point in the evolution of EU renewable energy policy was the release of the 1996 Commission Green Paper on RES, followed by a White Paper in 1997 entitled “Energy for the Future: Renewable Sources of Energy” [ 43 ], by setting the first goals and mechanisms for introducing RES into different sectoral politics.
In years to come, especially from the 2000s onwards, the rise in oil and gas prices, increased energy dependence, and securitisation of climate change have brought RES as a response to those challenges and set safeguarding environmental sustainability as one of the primary targets. One of the first EU’s strategies that introduced a new perspective on European energy security was “Green Paper: A European Strategy for Sustainable, Competitive and Secure Energy”, which stated that sustainable development should be one of six key areas that require necessary actions toward the challenges the EU faces [ 10 ]. For the EU, RES has begun to be seen as a tool for an “integrated European energy market and a common EU voice in international relations” [ 44 ], strengthening its energy- and climate-leader role in international relations. Between 2008 and 2021, the EU adopted three packages or frameworks for climate and energy policy [ 45 ]. The so-called “2020 climate and energy package” (2008) pressured the EU to achieve a 20% cut in GHG emissions (from 1990 levels), 20% of energy from RES, and a 20% improvement in energy efficiency. Those targets were supplemented to reduce GHG emissions to 80–95% below 1990 levels by 2050, set by the “Energy Roadmap 2050” [ 46 ], with mid-term goals oriented towards reducing GHG emissions up to 40% by 2030 and achieving a share of RES of around 30% at the same time [ 47 ].
As could be seen, the last two decades resulted in a significant increase in RE policies in the EU as climate issues started to be an issue of global concern, Footnote 2 culminating with the 2019 European Commission’s European Green Deal as an overarching set of measures aiming to reduce net EU emissions to zero by 2050 and to reduce emissions from 1990 levels by 55 percent by 2030, as set by “Fit for 55” [ 48 ]. The subsequent European Climate Law (2021) made energy transition targets mandatory. In addition, the REPowerEU Plan, launched in 2022, aims to end the EU’s energy dependence on Russian fossil fuels and address the transition to clean energy as one of the priorities [ 49 ]. A series of directives that the EU has adopted during this period, addressing diverse sectors of society and specific time objectives show its long-term dedication to renewable energy development. As stated in the literature, the EU has progressed during this period from idea-driven leadership to a more pragmatic leadership role [ 45 ].
The EU’s and PR China’s roles in combating climate change evolved through the years and became even more critical with the USA’s withdrawal from the Paris Agreement in 2017. While the EU and Chinese officials have been meeting for annual energy dialogues to cooperate on energy issues at the ministerial level since 1994, the EU and PR China have strengthened energy and climate cooperation in several rounds from 2005 onwards: in 2010, they established a ministerial dialogue mechanism and EU–China Environmental Governance Program; in 2012, the EU–China Environmental Sustainability Program; in 2015, the “EU–China Joint Statement on Climate Change”; in 2016, the “EU–China Roadmap on Energy Cooperation (2016–2020)”; and in 2018, the “China EU Leaders’ Statement on Climate Change and Clean Energy”. In 2019, the EU–China Energy Cooperation Platform was established to enhance energy cooperation between the two and contribute to the global transition to clean energy, respectively. Within this period, they agreed to expand cooperation in areas such as low-carbon development, protecting the environment, addressing climate change, and encouraging clean energy development [ 50 ]. Established cooperation mechanisms thus helped to deepen Chinese presence in the EU’s energy sector.
While some studies have shown the attractiveness of the EU’s market for PR China’s energy investments in terms of gaining access to the EU energy companies’ know-how, technologies, and running operations [ 51 ], others have revealed increased flows of renewable investments into the EU, mainly in the solar and wind sectors in recent years, with Germany being the most popular destination for those investments [ 52 ].
So far, the EU has achieved targets set in the 20–20–20 package in the form of reduced GHG emissions, an increased share of RES, and improved energy efficiency [ 53 ]. The EU, thus, imposed itself as a normative leader in the energy transition with strict objectives to follow, so it served as a good role model for PR China on how to work on the issue of climate change and energy transition in terms of creating policies and technology improvement [ 54 ]. However, it is important to note that the EU’s 2030 renewable energy target is at risk “due to low ambition in Central and Eastern European countries” [ 55 ]. On the other hand, PR China was acting more flexibly during this period. In this context, the literature highlights the pragmatism of PR China’s external politics (and thus actorness related to energy and climate) over revisionism and the wish for changing the established order [ 56 ], its gradual energy policy shifting towards environmental stewardship [ 36 ], which made it wholeheartedly accept the carbon neutrality target as a “new source of performance legitimacy” [ 37 ].
Although largely imposed by international agreements, energy transition targets for PR China were adopted when they were publicly perceived to significantly impact the domestic level. China thus emphasised its partiality towards “a voluntary climate regime that respects state sovereignty and different pathways towards tackling climate change and climate (in)justice” [ 13 ]. Nevertheless, it seems that it went through the path of changing identities from being a “developing country” to “a leading developing country”, admitting the necessity of reducing carbon emissions and the importance of the ecological civilisation concept as well [ 28 ] which, inevitably, mirrored in its energy- and climate-related policies from being responsible for major GHG emissions to being a more ecologically responsible country. In other words, the last two decades showed a gradual shift in PR China’s perception from a country focused solely on economic growth towards more sustainable development. In that sense, China’s energy- and climate-related policies could place it among environmentally aware countries—for illustration, PR China took second place among the 20 top countries with the most FDI outflows in the renewable energy sector in 2021 and 2022, right behind the USA [ 42 ].
The review and comparative analysis of the current EU’s and PR China’s climate- and energy-related policies resulted in some similarities: both recognise the profound role of RES in the energy transition process; both are undoubtedly dedicated towards energy transition and reducing carbon emissions by the similar deadlines; mid-term policies aim at similar targets; both recognise similar challenges in the energy transition such as energy dependency from third countries and the necessity of a greater share of RES in energy consumption; both recognise the necessity of international cooperation in contribution to global energy transition. In addition, some of the established cooperation mechanisms exist, and there is room for improvement in their cooperation, normatively speaking.
In contrast, the review and comparative analysis of their policies and previous actions showed some differences. Despite being the leader in the field of RE investments, PR China still heavily relies on coal in its energy consumption, with no official goal of cutting down coal-fired power plants, domestically or abroad. Additionally, the general impression is that the EU is oriented towards simultaneous processes of cutting off fossil fuels and increasing its share of RES, while PR China tends to establish renewable energy systems before the total phase-out of coal and other hydrocarbons. In this context, it could be said that PR China’s national interests are reflected mainly in its external dimension. The main points of contention thus spring from different perspectives when it comes to environmental responsibility. While the EU calls for an urgent reaction, prioritising green financing and a total phase-out of carbon emissions, PR China’s camp stands for a more nuanced approach towards ambitious energy transition goals that will favour national needs of a country.
PR China’s renewable energy investments in CEEC: state of play and future considerations
Since the analysis of the EU’s and PR China’s energy- and climate-related policies showed significant alignment in the RE domain, it was necessary to examine how this normative alignment reflected on a more practical level. The analysis of Chinese renewable energy investments in CEEC was thus chosen as a case study. In light of PR China’s speedy progress in its renewable energy investments both at home and internationally [ 57 ], the need to examine if this trend is mirrored in the CEEC example has emerged as significant. For this purpose, desk research on the example of 14 countries of the CEE region was conducted. The results of this work are presented in Tables 2 and 3 , while “ Appendix ” provides a comprehensive table with extensive data (see “ Appendix ”). One of the most challenging aspects of this research was obtaining financial details about each project, which resulted in some transactions being labelled as N/A (non-available).
Table 2 presents the number of projects in different RE fields in order to see in which RES subsectors PR China had the most success. Based on the data collected, the solar energy subsector has emerged as the leading subsector with 12 projects. The wind energy subsector follows closely behind with nine projects, while the hydro energy subsector has four major projects. If one takes into account each small hydro energy project separately instead of lumping them together with larger projects, such as the three small HPPs on the River Drina and the three small HPPs on Bistrica in Bosnia and Herzegovina, the number would be even higher. While there were occasional transactions in the geothermal energy subsector or gas cycle power plant, there were no regularities to report.
The structure of RE projects in CEEC in which PR China invested is shown in Table 3 . Countries not listed in this table, such as Albania, Bulgaria, North Macedonia, Slovakia, and Slovenia, did not have any RE projects so far. Bulgaria is the exception in this group because it had one project in the making, in which Dongfang Electric was one of the partners. That was the construction of a refuse-derived fuel plant that uses waste, and as such, it can be considered as an RE project. However, the Bulgarian Supreme Court recently annulled this project [ 58 ]. It is also worth noting that, currently, there are no Chinese-funded RE projects in either Poland or the Czech Republic, and all past investments, rights, and acquisitions have been sold.
When analysing the number of active or completed projects, Bosnia and Herzegovina is the leader in this field of RE cooperation, with six of them. Following closely behind are Poland and Hungary, with five projects each, while Romania and the Republic of Serbia have three. Greece, Montenegro, and Croatia have two projects each, and the Czech Republic has one. So far, Bosnia and Herzegovina and Poland have achieved the most diversified cooperation results.
When looking at the value of realised/active projects, the results are slightly different. Despite having only two projects, Greece has the highest value of approximately €1.74 billion, which ranks it first. Bosnia and Herzegovina come in second with a total project value at around €1.1 billion. Since values are not available for all projects in Poland and Hungary, they could not be ranked. Therefore, according to available data, the Republic of Serbia is ranked third.
Chinese companies were involved in most of the RE projects, either as investors or construction and design companies (see “ Appendix ”). Only two projects were financed by Chinese loans: the hydropower plants on the Drina River in Bosnia and Herzegovina, worth 460 million euros, and in the Republic of Serbia, the construction of a hot water pipeline linking the Obrenovac thermal power plant and the Novi Beograd heating plant, worth 165 million euros. An additional project in Bosnia and Herzegovina, which may also be partially financed by a Chinese loan, is currently under negotiations. This is the case for three small-scale HPPs on the Bistrica River, worth 103 million euros. Comparing these findings with the Zakić and Šekarić study [ 18 ], it was noted that coal-related projects in the traditional energy sector in CEEC were all financed by loans in previous years, while most RE projects were not. Thus, it appears that Chinese financing patterns in the traditional and renewable energy sectors in CEEC differ.
When analysing RE projects in CEEC that involve Chinese investments, there have often been examples of negotiated projects that failed before the construction began. Because of this, it was important to investigate the background of each project because the reasons behind the successful and unsuccessful examples were often more related to politics than to sustainable development issues. In this sense, the concept of Sino-scepticism was a helpful tool in mapping political reasons for collapsing relations established and/or closing down agreed projects between PR China and a specific country from the CEE region. This is due to the general rise of negative sentiment toward PR China across Europe in recent years [ 59 ], which resulted in Lithuania, Latvia, and Estonia stepping out of the framework in 2021 and 2022 and, in certain cases, worsening of bilateral relations with PR China [ 60 ]. For that purpose, the data and information surrounding each project were analysed to identify factors contributing to its outcome. Specifically, CEEC was divided into three groups according to the number of realised and unsuccessful RE projects: those with no projects currently, those with a moderate level of cooperation (1–3 projects), and those with a high level cooperation (more than three projects).
Countries with no Chinese RE projects
Currently, the following 7 countries do not have RE projects connected to PR China: Albania, Bulgaria, the Czech Republic, North Macedonia, Poland, Slovakia, and Slovenia. Within this group, Bulgaria tried, and Poland succeeded in establishing cooperation, but that was not the case with the rest of the group. Poland is a specific case amongst studied countries because it started the RE cooperation strongly and had several successful projects and transactions before it all suddenly stopped. Chinese companies sold all of their RE portfolio in 2021, and this situation coincided with the rise of Sino-scepticism in Poland [ 61 , 62 ] provoked by several factors: the EU’s worsened political relations with PR China [ 63 ], the pandemic, limited economic results in cooperation with PR China [ 64 , 65 ], bilateral diplomatic tensions and the latest one was Chinese relations with the Russian Federation in the context of the ongoing warfare in Ukraine [ 63 ]. PR China tried several times to conduct RE projects in Bulgaria [ 66 , 67 ] with no results, and no particular or out-of-the-ordinary reasons for those failures could be found. The Czech Republic had just one successful Chinese-financed project. After that, mainly due to political changes in this country (ruling parties) and the rise of Sino-scepticism [ 64 ] as a result of PR China’s domestic human rights issues and the PR China–Taiwan dispute [ 68 ], almost all cooperation ceased. Slovakia is a country in which the same process (the rise of Sino-scepticism) and results (or lack thereof) can be interpreted as in the case of Poland and the Czech Republic. In this case, the only difference is that PR China negotiated with Slovakia to construct one HPP (on the river Ipel), and that one was unsuccessful [ 64 ]. On the other hand, in the case of Slovenia, official information about possible cooperation in RES was not available, so a definite conclusion about the reasons for the lack of cooperation in this field could not be reached. Albania, as a country dedicated to its EU path, regardless of good diplomatic relations with PR China, did not want to jeopardise its potential EU membership and its relations with the USA, so it chose not to interact with PR China in the field of investments [ 69 ]. Similarly, North Macedonia was not open to cooperation with PR China, especially since governmental changes in 2017 [ 58 ].
Countries with a moderate level of cooperation with PR China in RE projects
Several countries have had moderate levels of cooperation with PR China in the RE field, including Montenegro, Croatia, Greece, the Republic of Serbia, and Romania. Montenegro, so far, has only one Chinese project that it has finalised until the present day, with one project (an HPP) on hold. Montenegro was not motivated to pursue joint projects in the RE field due to complicated PR China–Montenegro relations regarding the loan and construction of the high-speed road between Bar and Boljare in Montenegro, for which Montenegro had to ask the EU to help it in restructuring it, so that it could pay the loan [ 70 ].
Croatia realised two projects in the solar energy field, which took some time for legal reasons [ 71 ], but in the end, they were successful. However, due to Croatia’s limited engagement with PR China and the absence of official information regarding further cooperation in RES, it was not possible to definitively ascertain the reasons for the halt in cooperation. Although their good diplomatic relations are not strained, Croatia, in this instance, is following the EU example of keeping good relations but with a limited number of Chinese investments.
According to the number of Chinese RE projects, Greece has a medium level of cooperation, but according to their values, it is in the lead. The main reasons for this were the acquisition of 75% of wind farms within the company Copelouzos, worth 1.45 billion euros, and the construction of the MINOS concentrated solar power plant, worth 286 million euros [ 18 ]. Greece has very diversified cooperation with PR China in general. PR China is very interested in cooperation in the RE field because Greece, as an EU country, could apply for many RE funds to increase carbon neutrality, which is attractive to Chinese investors.
As a non-EU Member State, the Republic of Serbia pursued intensive cooperation with PR China, mainly in infrastructure. Until recently, RE projects were not at the top of the Republic of Serbia’s agenda regarding Chinese investments, and if that were the case, the number of projects would be much higher. Interestingly, PR China proposed many RE projects, but the Republic of Serbia was not interested in them, mainly because of other infrastructural and fossil fuel priorities, as clearly seen by the volume and number of those kinds of projects for which the Republic of Serbia applied [ 14 , 18 ]. Chinese private companies have been joining forces with the EU companies for RE projects in the Republic of Serbia, such as solar park Agrosolar [ 72 ], which is currently under construction, and the wind farm Maestrale Ring and the wind park Vetrozelena [ 73 , 74 ] where the construction process will start in 2024.
The last country in this group is Romania, which has had three smaller solar projects since 2014, and in times before that, it cooperated with PR China mainly in the wind energy field. Romania’s relations with PR China have been restrained for various reasons, including constant changes of ruling political parties and the increased level of Sino-scepticism surrounding leading politicians, similar to those in the Czech Republic.
Countries with a high level of cooperation with PR China in RE projects
The final group is the one in which PR China developed the most diversified RE cooperation (with more than three projects), including Hungary and Bosnia and Herzegovina. Even though both countries successfully cooperate with PR China, their ways of cooperating and the projects on which they are working are different.
For example, Hungary introduced legislation in 2016 that prohibits the installation of wind turbines within a 12-km radius of populated areas [ 75 ], which meant there was no space for building new wind farms, so PR China could only invest in solar and geothermal energy projects in Hungary. However, there has been no news about new Chinese RE projects in Hungary since 2021, which could indicate that these two countries are pursuing collaboration in other fields. Despite the above, PR China is heavily investing in the production of lithium batteries in Hungary, to be worth around 9 billion euros in the upcoming years [ 76 , 77 ], so it probably means that PR China is re-focusing its attention on more lucrative RE-related projects in Hungary.
In Bosnia and Herzegovina, PR China was focused on pursuing HPP projects. However, there are legal problems that are putting those projects on hold. For example, in the case of an HPP on the River Bistrica, according to the plans, the PR China National Aero-technology International Engineering Corporation is in charge of constructing three small HPPs. Still, the work was stopped due to annulled construction permission issued by a local court in the city of Banja Luka [ 78 ]. The main problem was the expired environmental permit for construction, which is obligatory for projects that have an invasive impact on the environment. Legal issues surrounding the work on HPPs on the River Buk Bijela keep the project in the early stage of construction, even though it began in 2019. Furthermore, the legal framework surrounding RE projects in Bosnia and Herzegovina is susceptible to illegal actions, which is demonstrated in practice through many suspicious transactions [ 79 ]. Among many other problems, companies that want to be involved in solar or wind energy projects can only be concessionaires for a limited period, and the law forbids them to be the owners. As a result, many projects changed the concession rights several times, and usually, the first holder of these rights gets concession rights at a relatively low price, but subsequent buyers pay those rights at several times higher prices [ 79 ].
Based on data collected from the 14 countries of the CEE region, the study showed that political factors had a greater impact than economic and legal ones regarding the Chinese presence in CEEC in the RE field. This is in line with the findings of Sattich et al. [ 4 ] that “increasing recourse to policy choices based on national priorities today creates obstacles to further cooperation” regarding the EU’s and PR China’s energy- and climate-related policy alignment. In addition, the context of the current Chinese presence in CEEC in the RE field is shaped by the overall stagnation of the EU–PR China partnership due to increased Sino-scepticism in Europe, the harmful effects of PR China’s security policies on European companies in PR China, and PR China’s neutrality over Russia’s invasion of Ukraine, among other factors [ 63 ].
To prove such conclusions, narratives surrounding the political orientation of CEEC towards PR China along the spectrum of Sino-scepticism vs. Sino-optimism were further explored. During the 11 years of the PR China–CEEC cooperation framework, the battle of Chinese versus Western narratives was stark concerning the reasons for PR China’s rapid and intense economic and political involvement in the CEEC. PR China [ 80 , 81 , 82 ] considers this move a pragmatic way to deepen primarily economic cooperation. As Liu [ 81 ] pointed out, it was part of circumstances shaped by, at that time, the situation both in PR China and CEEC:
“Presented with favourable chances, such as the lack of time for the core European countries to take care of the CEE region, and the CEE countries wishing to develop more extensive foreign cooperation in order to overcome economic difficulties, China seized the opportunity of this time frame to develop cooperation with CEE countries and successfully initiated the China–CEEC cooperation”.
On the other hand, the Western countries view this situation differently. Analysing frequently used Western narratives associated with the PR China–CEEC cooperation framework, Mitić [ 83 ] concluded that narratives are mostly negative, viewing this cooperation framework as part of PR China’s geopolitical strategy. Without going too deep into this significant topic, it should be stated that views and opinions regarding this cooperation are stark on both spectrums (positive vs. negative), with a small number of those trying to take moderate positions.
For the purpose of this analysis, CEEC was divided into four groups according to the level of Sino-scepticism/Sino-optimism (Fig. 1 ). This categorisation helps demonstrate each country’s prevailing narratives and political opinions regarding cooperation with PR China. Since this study employed exploratory in-depth desk research of the outlined case study, diverse textual sources referring to each concrete project were analysed in order to reveal possible reasons for their (un)success. In other words, the categorisation of CEEC according to the level of Sino-scepticism/Sino-optimism results from the authors’ opinions shaped by the literature review and media article analysis, Footnote 3 and data collected over several years of research on PR China, and it should be approached cautiously, as it is based on the authors’ interpretations of empirical data.
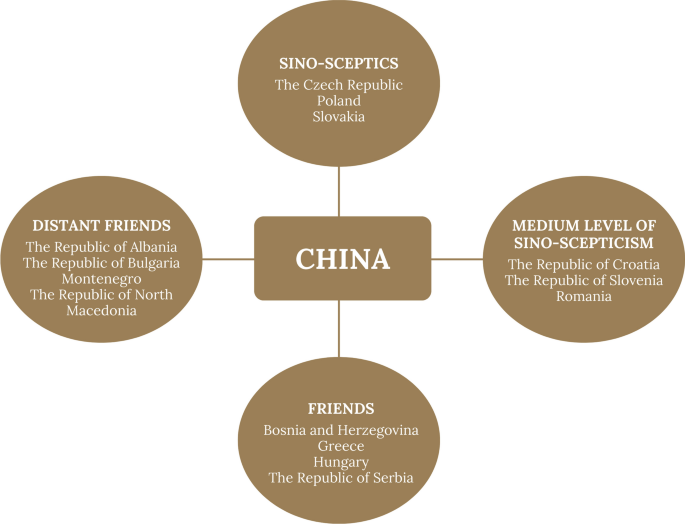
PR China and CEEC: levels of Sino-scepticism (Sino-optimism). Source: authors
When comparing the results of cooperation in the RE sector within the PR China–CEEC cooperation framework (Tables 2 and 3 and Fig. 1 ), it becomes clear that the negative vs. positive orientation of CEEC towards cooperation with PR China significantly affected the success of RE projects. Political factors, such as the rising level of Sino-scepticism due to PR China’s domestic human rights and disputes regarding Taiwan, followed by strained EU–PR China relations, were among those that proved to be the most severe obstacles. Owing to PR China’s extensive experience in renewable energy and competitive project prices, economic factors were not an obstacle to cooperation in most cases. Legal procedures related to environmental factors only affected small HPPs, and those were found only in Bosnia and Herzegovina. Therefore, the level of CEEC’s Sino-scepticism/Sino-optimism seriously affected the results of PR China–CEEC RE cooperation.
Although previous analysis showed significant alignment of the EU’s and PR China’s energy- and climate-related policies on the basis of RES, case study analysis within the PR China–CEEC cooperation framework proved that this alignment did not always lead to successful results in reality. Accordingly, as Gippner and Torney [ 36 ] highlighted, policy alignment of two actors “is a necessary but not sufficient condition for stimulating Chinese investment in EU clean technology sectors”. Therefore, overcoming political and economic divergences imposes a condition for achieving better cooperation in the renewable energy domain.
This study questioned the normative alignment of the EU’s and PR China’s energy- and climate-related policies in the domain of RE and its influence on the renewable energy cooperation between PR China and CEEC on a practical level. More specifically, it tested the hypothesis that dedication towards energy transition targets and alignment of strategies on the basis of RES should result in higher levels of practical cooperation.
According to the conducted analysis, Sino-European relations in this area are intertwined in several domains: first of all, they are both core actors in climate and energy-related issues with the same goal of reaching carbon–neutral status; second, their core energy-related policies became the basis for other climate actions; third, PR China develops relations with the EU as a supranational organisation but also with its individual member states. In this context, the review and comparative analysis of contemporary the EU’s and PR China’s energy- and climate-related policies indicate a significant level of policy alignment on the basis of RE. The main connecting spots thus spring from:
mutual recognition of the significant role of renewable energy sources in the energy transition process,
similar deadlines for achieving energy transition targets,
similar goals of their mid-term policies,
recognition of similar challenges in the energy transition, and
the necessity of international cooperation in contribution to the global energy transition.
The critical point of contention, however, comes from different perspectives regarding environmental responsibility. While the EU calls for an urgent reaction, prioritising green financing and a total phase-out of carbon emissions, PR China’s camp stands for a more nuanced approach towards ambitious energy transition goals that will favour the national needs of a country.
The case study of PR China–CEEC RE cooperation was conducted to test if the alignment of the EU’s and PR China’s energy- and climate-related policies was reflected on a practical level. This region, comprising 14 countries, was chosen as a case study for two reasons. Firstly, it is part of the PR China–CEEC cooperation framework, presenting a prominent part of PR China’s geo-economic strategy. Secondly, this region has a diverse structure comprising European Union Member States and candidate countries that interact with other external actors within the context of the prevailing European Union energy approach.
The study has shown moderate results of Chinese investments in RE projects in CEEC. Solar and wind energy projects are still leading, while hydropower plants have the biggest potential, especially in the Western Balkans. The main reasons for unsuccessful projects were political, stemming from rising levels of Sino-scepticism. Legal reasons, such as the specific legal procedures and norms for RE projects, were also a factor, followed by economic reasons, such as project costs. Those were found in a minority of cases. However, one should not forget the numerous upcoming RE projects involving Chinese and European companies in joint work, which will come to fruition in the next couple of years, thus suggesting that cooperation can be improved.
The transnational nature of climate change consequences requires unity to answer this challenge. This becomes especially important in the context of an unstable geopolitical environment. Once achieved, energy transition goals bring common benefits, global cooperation, and shared action imposed as a necessity in this process. The question is whether the speed of accomplishing the energy transition goals will be a priority over the political and economic differences between the EU and PR China. Sino-European cooperation in the domain of renewable energy has evolved in the XXI century, and a normative basis for its strengthening certainly exists. However, the effectiveness of achieving targeted energy and climate goals depends mainly on overcoming barriers on both political and economic levels. Besides, the two are expected to reach their renewable energy targets as leaders of the energy transition process, so there is a pressure to act accordingly.
In the post-pandemic environment and especially with the start of the conflict between the Russian Federation and Ukraine, energy transition became the raison d'être of the EU’s energy operations, as it has stopped relying on its main external energy partner. The EU’s ambitious energy transition targets reflect its urgent need to be energy independent. On the other hand, PR China, burdened with its own energy and environmental requirements, also strives for sustainable growth based on energy transition. This mutual need should be utilised in a manner that will bring the parties closer to achieving renewable energy targets.
Considering the importance of the EU and PR China in the mitigation of climate change, energy transition success will greatly affect the global level. Significant challenges in this cooperation, in turn, could slow down the process of energy transition. As for future sustainability concerns, the worth of the notion is the fact that the cooperation on climate and sustainability is seen as one of the priority areas for EU–PR China relations in the forthcoming decade. Looking to the future, it is important to be aware of the capacity for strengthening Sino-European cooperation in the domain of renewable energy.
Availability of data and materials
All data generated or analysed during this study are included in this published article and its “ Appendix ”.
Regarding PR China’s energy- and climate-related policies, due to the language barrier, it should be stated that the analysis of those documents that did not contain the English version was conducted relying on secondary data and a literature review with reference to primary documents.
Besides climate changes, the security of supply, actualized by the 2005/2006 and 2008/2009 gas crisis between the Russian Federation and Ukraine, and the 2014 Ukraine crisis, became an additional issue in combating energy threats and challenges in the EU.
Textual sources that were analysed could be found in the References section.
Abbreviations
Central and Eastern Europe
- Central and Eastern European Countries
China Global Investment Tracker
- European Union
Foreign direct investment
Five-year plan
Greenhouse gas
Hydropower plant
Nationally Determined Contributions
National Energy Administration
- People’s Republic of China
- Renewable energy
Renewable energy sources
United Nations Conference on Trade and Development
United States of America
Scholten D (2018) The geopolitics of renewables: an introduction and expectations. In: Scholten D (ed) The geopolitics of renewables. Lecture notes in energy, vol 61. Springer, Cham. https://doi.org/10.1007/978-3-319-67855-9_1
Chapter Google Scholar
Šekarić Stojanović N (2022) The geopolitics of renewables and the place of the Western Balkans. Rev Int Affairs 73(1186):105–124. https://doi.org/10.18485/iipe_ria.2022.73.1186.5
Article Google Scholar
Criekemans D (2021) ‘Geotechnical ensembles’: how new technologies change geopolitical factors and contexts in economy, energy and security. In: Criekemans D (ed) Geopolitics and international relations grounding world politics Anew geopolitics and international relations, vol 1. Brill Nijhoff. https://doi.org/10.1163/9789004432086_004
Sattich T, Freeman D, Scholten D, Yan S (2021) Renewable energy in EU-China relations: policy interdependence and its geopolitical implications. Energy Policy 156:112456. https://doi.org/10.1016/j.enpol.2021.112456
Liu L, Wu T, Wan Z (2019) The EU-China relationship in a new era of global climate governance. Asia Eur J 17:243–254. https://doi.org/10.1007/s10308-018-00530-2
British Petroleum (2016) Statistical Review of World Energy, June 2016. https://www.bp.com/en/global/corporate/energy-economics/statistical-review-of-world-energy.html#tab_sr-2016 . Accessed 15 July 2023.
British Petroleum (2022) Statistical Review of World Energy, 71st edition. https://www.bp.com/en/global/corporate/energy-economics/statistical-review-of-world-energy.html#tab_sr-2016 . Accessed 15 July 2023.
Eurostat. https://ec.europa.eu/eurostat/statistics-explained/index.php?title=Renewable_energy_statistics . Accessed 15 July 2023.
Jones D (2021) EU Power Sector in 2020. https://ember-climate.org/insights/research/eu-power-sector-2020/ . Accessed 16 July 2023.
European Commission (2006) Green Paper: A European Strategy for Sustainable, Competitive and Secure Energy, COM(2006) 105 final. https://europa.eu/documents/comm/green_papers/pdf/com2006_105_en.pdf . Accessed 17 July 2023.
European Commission. (2015). Energy Union Package, COM (2015) 80 final. https://eur-lex.europa.eu/resource.html?uri=cellar:1bd46c90-bdd4-11e4-bbe1-01aa75ed71a1.0001.03/DOC_1&format=PDF . Accessed 17 July 2023.
Šekarić Stojanović N (2022b) Energetska bezbednost Evropskog regionalnog bezbednosnog kompleksa: sekuritizacija ruskog prirodnog gasa i potencijali za transformaciju kompleksa. Dissertation, University of Belgrade.
von Lucke F (2023) The EU and China in the climate regime: exploring different pathways towards climate justice. Asia Eur J 21:429–435. https://doi.org/10.1007/s10308-022-00654-6
Stanojević N, Zakić K (2023) Ekonomski uspon Kine—strategija i dinamika međunarodnih odnosa. Institute of International Politics and Economics, Belgrade
Google Scholar
Schonhardt S (2023) China Invests $546 Billion in Clean Energy, Far Surpassing the U.S. https://www.scientificamerican.com/article/china-invests-546-billion-in-clean-energy-far-surpassing-the-u-s/ . Accessed 18 July 2023.
China Global Investment Tracker. https://www.aei.org/china-global-investment-tracker/ . Accessed 20 July 2023.
Yu Y (2022) The great significance and broad prospects of China-CEEC renewable energy cooperation. Working Paper No.12. https://china-cee.eu/working_papers/the-great-significance-and-broad-prospects-of-china-ceec-renewable-energy-cooperation/ . Accessed 25 July 2023.
Zakić K, Šekarić N (2021) China’s energy cooperation within the 17+1. Međunarodni problemi 73(1):7–38. https://doi.org/10.2298/MEDJP2101007Z
EU-China 2020 Strategic Agenda for Cooperation (2013) https://eeas.europa.eu/archives/docs/china/docs/eu-china_2020_strategic_agenda_en.pdf . Accessed 22 July 2023.
Mission of the PRC to the EU (2018) China’s Policy Paper on the European Union. http://eu.china-mission.gov.cn/eng/zywj/zywd/201812/t20181219_8301603.htm . Accessed 22 July 2023.
European Commission (2020) Von der Leyen following EU-China Summit: We must make progress, one that implies reciprocity and trust. https://ec.europa.eu/commission/presscorner/detail/en/ac_20_1170 . Accessed 22 July 2023.
Chidley C (2014) Towards a framework of alignment in international relations. Politikon South Afr J Polit Stud 41(1):141–157. https://doi.org/10.1080/02589346.2014.885682
Todić D (2015) Kina i Evropska unija – sličnosti i razlike u pravu i politici životne sredine i članstvo u međunarodnim ugovorima. Strani pravni život 3:63–77
Altun S, Ergenc C (2023) The EU and China in the global climate regime: a dialectical collaboration–competition relationship. Asia Eur J 21:437–457. https://doi.org/10.1007/s10308-023-00664-y
Dupont B (2022) China and the EU in Climate Geopolitics. https://eias.org/publications/op-ed/china-and-the-eu-in-climate-geopolitics%EF%BF%BC/ . Accessed 23 July 2023.
Bosce AM (2018). The EU and China: Prospects of Cooperation on Climate and Energy. https://www.europenowjournal.org/2018/06/04/the-eu-and-china-prospects-of-cooperation-on-climate-and-energy/ . Accessed 25 July 2023.
Sattich T, Freeman D (2019) The EU and China: energy policy interdependence: interactions between internal energy policies of the EU and China. In: Hefele P, Palocz-Andresen M, Rech M, Kohler JH (eds) Climate and energy protection in the EU and China. Springer, Cham. https://doi.org/10.1007/978-3-319-99837-4_2
Stensdal I, Heggelund G (2023) Changes in China’s climate justice perceptions: domestic and international consequences. Asia Eur J 21:459–480. https://doi.org/10.1007/s10308-023-00678-6
Rayner T, Szulecki K, Jordan A, Oberthür S (2023) The global importance of EU climate policy: an introduction. In: Rayner T, Szulecki K, Jordan A, Oberthür S (eds) Handbook on European Union climate change policy and politics. Political science and public policy 2023. Edward Elgar Publishing, Cheltenham. https://doi.org/10.4337/9781789906981.00011
Fan, J, Wang J, Wei S, Zhang X (2018) The development of China’s renewable energy policy and implications to Africa. In: IOP conference series: materials science and engineering. https://doi.org/10.1088/1757-899X/394/4/042034 . Accessed 27 July 2023.
Zhang K, Wen Z, Peng L (2007) Environmental policies in China: evolvement, features and evaluation. Chn Popul Res Envi 17(2):1–7
Zhang, V (2020) Renewable Energy Law and Regulation in China. https://cms.law/en/int/expert-guides/cms-expert-guide-to-renewable-energy/china . Accessed 27 July 2023.
International Energy Agency. (2021). Renewable energy law of the People’s Republic of China. https://www.iea.org/policies/3080-renewable-energy-law-of-the-peoples-republic-of-china . Accessed 27 July 2023.
He J, Li Z, Zhang X (2021) China’s long-term low-carbon development strategies and pathways. Springer, Berlin
Jingjing Z, Wang W (2020) China’s energy law could help address the Belt and Road’s climate impact. https://chinadialogue.net/en/energy/chinas-energy-law-could-help-address-the-belt-and-roads-climate-impact/ . Accessed 28 July 2023.
Gippner O, Torney D (2017) Shifting policy priorities in EU-China energy relations: implications for Chinese energy investments in Europe. Energy Policy 101:649–658. https://doi.org/10.1016/j.enpol.2016.09.043
Teng F, Wang P (2021) The evolution of climate governance in China: drivers, features, and effectiveness. Environ Polit 30(1):141–161. https://doi.org/10.1080/09644016.2021.1985221
Center for Security and Emerging Technology. (2021). Outline of the People's Republic of China 14th five-year plan for national economic and social development and long-range objectives for 2035 . https://cset.georgetown.edu/wp-content/uploads/t0284_14th_Five_Year_Plan_EN.pdf . Accessed 30 July 2023.
MFA of the People’s Republic of China. (2021). Xi Jinping attends the general debate of the 76th session of the United Nations general assembly and delivers an important speech. https://www.fmprc.gov.cn/mfa_eng/wjb_663304/zzjg_663340/gjs_665170/gjsxw_665172/202109/t20210923_9580159.html . Accessed 30 July 2023.
Yifan J (2021) China at COP26: Coal, 1.5C and short-term actions. https://chinadialogue.net/en/climate/coal-1-5c-and-short-term-actions-china-at-cop26/ . Accessed 30 July 2023.
Yifan J, Baiyu G, Geall S (2022) China’s Five Year Plan for energy: One eye on security today, one on a low-carbon future. https://chinadialogue.net/en/climate/chinas-five-year-plan-for-energy-one-eye-on-security-today-one-on-a-low-carbon-future/ . Accessed 30 July 2023.
United Nations Conference on Trade and Development (2023) World Investment Report 2023: investing in sustainable energy for all—overview. https://unctad.org/system/files/official-document/wir2023_overview_en.pdf . Accessed 2 Dec 2023.
Solorio I, Bocquillon P (2017) EU renewable energy policy: a brief overview of its history and evolution. In: Solorio I, Jörgens H (eds) A guide to EU renewable energy policy: comparing Europeanization and domestic policy change in EU member states. Social and political science 2017. Edward Elgar Publishing, Cheltenham. https://doi.org/10.4337/9781783471560.00011
Piebalgs A (2007) Andris Piebalgs Energy Commissioner EU’s response to the global energy challenges Speech at the Vilnius Energy Security Conference Vilnius. https://ec.europa.eu/commission/presscorner/detail/en/speech_07_623 . Accessed 2 Aug 2023.
Bäckstrand K (2022) Towards a climate-neutral union by 2050? The European green deal, climate law, and green recovery. In: Bakardjieva Engelbrekt A, Ekman P, Michalski A, Oxelheim L (eds) Routes to a resilient European Union: interdisciplinary European studies. Palgrave Macmillan, Cham. https://doi.org/10.1007/978-3-030-93165-0_3
European Commission (2011) Energy Roadmap 2050, COM(2011) 885 final. https://eur-lex.europa.eu/LexUriServ/LexUriServ.do?uri=COM:2011:0885:FIN:EN:PDF . Accessed 2 Aug 2023.
European Commission (2013) Green paper: a 2030 framework for climate and energy policies, COM(2013) 169 final. https://eur-lex.europa.eu/LexUriServ/LexUriServ.do?uri=COM:2013:0169:FIN:en:PDF . Accessed 2 Aug 2023.
Radovanović M, Filipović S, Vukadinović S, Trbojević M, Podbregar I (2022) Decarbonisation of eastern European economies: monitoring, economic, social and security concerns. Energy Sustain Soc 12:1–15. https://doi.org/10.1186/s13705-022-00342-8
European Commission (2022) REPowerEU Plan, COM (2022) 230 final. https://eur-lex.europa.eu/resource.html?uri=cellar:fc930f14-d7ae-11ec-a95f-01aa75ed71a1.0001.02/DOC_1&format=PDF . Accessed 3 Aug 2023.
European Commission (2016) EU-China Roadmap on energy cooperation (2016–2020). https://energy.ec.europa.eu/system/files/2016-06/FINAL_EU_CHINA_ENERGY_ROADMAP_EN_0.pdf . Accessed 3 Aug 2023.
Liedtke S (2017) Chinese energy investments in Europe: an analysis of policy drivers and approaches. Energy Policy 101:659–669. https://doi.org/10.1016/j.enpol.2016.09.037
Curran L, Lv P, Spigarelli F (2017) Chinese investment in the EU renewable energy sector: Motives, synergies and policy implications. Energy Policy 101:670–682. https://doi.org/10.1016/j.enpol.2016.09.018
European Environment Agency (2021) EU achieves 20–20–20 climate targets, 55 % emissions cut by 2030 reachable with more efforts and policies. https://www.eea.europa.eu/highlights/eu-achieves-20-20-20 . Accessed 3 Aug 2023.
Gippner O (2014) Framing it right: China–EU relations and patterns of interaction on climate change. Chin J Urban Environ Stud 2(1):1450003. https://doi.org/10.1142/S2345748114500031
Popp R, Czyzak P (2023) Eastern EU countries will make or break the bloc’s 2030 renewables goal. https://www.e3g.org/news/eastern-eu-countries-will-make-or-break-the-bloc-s-2030-renewables-goal/ . Accessed 1 Dec 2023.
Gueldry M, Liang W (2016) China’s global energy diplomacy: behavior normalization through economic interdependence or resource neo-mercantilism and power politics? J Chin Polit Sci 21:217–240. https://doi.org/10.1007/s11366-016-9405-3
Hayley A (2023) China’s Belt and Road energy projects set for “greenest” year, research shows. https://www.reuters.com/business/energy/chinas-belt-road-energy-projects-set-greenest-year-research-2023-08-02/ . Accessed 3 Aug 2023.
Shopov V (2022) Let a thousand contacts bloom: How China competes for influence in Bulgaria. https://ecfr.eu/publication/let-a-thousand-contacts-bloom-how-china-competes-for-influence-in-bulgaria/ . Accessed 5 ust 2023.
Pew Research Center. https://www.pewresearch.org/global/2022/06/29/negative-views-of-china-tied-to-critical-views-of-its-policies-on-human-rights/ . Accessed 5 Aug 2023.
Andrijauskas K (2022) The Sino-Lithuanian Crisis: Going beyond the Taiwanese Representative Office Issue. https://www.ifri.org/sites/default/files/atoms/files/andrijauskas_sino_lithuanian_crisis_2022.pdf . Accessed 2 Dec 2023.
Turcsanyi RQ (2017) Central European attitudes towards Chinese energy investments: the cases of Poland, Slovakia, and the Czech Republic. Energy Policy 101:711–722. https://doi.org/10.1016/j.enpol.2016.09.035
Bachulska A (2020) Sino-Polish relations: From hyper-optimism to scepticism. https://mapinfluence.eu/pl/sino-polish-relations-from-hyper-optimism-to-scepticism-2/ . Accessed 6 Aug 2023.
van Wieringen K (2022) EU-China 2030: European expert consultation on future relations with China. www.europarl.europa.eu/RegData/etudes/STUD/2022/739240/EPRS_STU(2022)739240_EN.pdf . Accessed 1 Dec 2023.
Turcsányi RQ (2020) China and the Frustrated region: central and Eastern Europe’s repeating troubles with great powers. China Report 56(1):60–77. https://doi.org/10.1177/0009445519895626
Przychodniak M (2021) The rough “strategic relationship” between Poland and China. https://chinaobservers.eu/the-rough-strategic-relationship-between-poland-and-china/ . Accessed 1 Dec 2023.
Global Data (2023) Sunpower Grp—biomass power plant 16 MW—Bulgaria. https://www.globaldata.com/store/report/sunpower-grp-biomass-power-plant-16-mw-bulgaria-profile-snapshot/ . Accessed 25 Nov 2023.
Ivanova T (2023) Sofia heating utility terminates waste incinerator tender. https://seenews.com/news/sofia-heating-utility-terminates-waste-incinerator-tender-814803 . Accessed 27 Nov 2023.
Karásková I (2022) Chinese Influence in the Czech Republic. https://cepa.org/comprehensive-reports/chinese-influence-in-the-czech-republic/ . Accessed 6 Aug 2023.
Musabelliu M (2022) Albania’s Demeanour in the China-CEEC cooperation: overcoming the “3S” dilemma. In: Horvat L (ed) Results and challenges: 10 years of China-CEEC cooperation. Euro-Asia Center, Budapest, pp 60–79
Tomović P (2022) Kineske ’lekcije’ za nastavak autoputa u Crnoj Gori. https://www.slobodnaevropa.org/a/crna--gora-kinezi-autoput/31982292.html . Accessed 7 Aug 2023.
Markušić D (2017) Kineska tvrtka preuzima tvrtku za gradnju vjetroelektrane kod Senja. https://direktno.hr/razvoj/kineska-tvrtka-preuzima-tvrtku-za-gradnju-vjetroelektrane-kod-senja-105628/ . Accessed 27 Nov 2023.
Spasić V (2022) Srpsko-kinesko-italijanski konzorcijum gradi prvi agrosolarni park u Kuli. https://balkangreenenergynews.com/rs/srpsko-kinesko-italijanski-konzorcijum-gradi-prvi-agrosolarni-park-u-kuli/ . Accessed 9 Aug 2023.
Plutonlogistics (2023) Novi poslovi u transportu vetrenjača iz Kine – U Subotici niče najveći evropski vetropark na kopnu. https://plutonlogistics.com/logistika/novi-poslovi-u-transportu-vetrenjaca-iz-kine-u-subotici-nice-najveci-evropski-vetropark-na-kopnu/ . Accessed 7 Aug 2023.
Peljto S (2023) Kineski kapital ulazi u srpske OIE i najveću vetroelektranu u zemlji. https://rs.bloombergadria.com/biznis/kompanije/27706/kinezi-ulaze-u-srpske-oie-i-najvecu-vetroelektranu/news . Accessed 9 Aug 2023.
Szőke E (2023) Hungary to loosen its rules on deployment of wind turbines. https://ceenergynews.com/renewables/hungary-to-loosen-its-rules-on-deployment-of-wind-turbines/ . Accessed 9 Aug 2023.
Contemporary Amperex Technology Co. Limited (2022) CATL announces its second European battery plant in Hungary. https://www.catl.com/en/news/983.html . Accessed 12 Aug 2023.
Gyori B (2023) Chinese battery maker EVE Power to build plant in Hungary. https://www.reuters.com/business/autos-transportation/chinese-battery-maker-eve-power-build-plant-hungary-2023-05-09/ . Accessed 12 Aug 2023.
Slobodna Evropa (2023) Poništena građevinska dozvola za jednu od tri ‘kineske hidrocentrale’ na istoku BiH. https://www.slobodnaevropa.org/a/ponistena-dozvola-kineska-hidrocentrala-bistrica-foca/32258659.html . Accessed 13 ust 2023.
Maksimović D (2022) Karbonizacija zelene energije u BiH. https://www.dw.com/bs/karbonizacija-zelene-energije-u-bih/a-62830620 . Accessed 13 Aug 2023.
Kong T (2015) 16+1 cooperation framework: Genesis, characteristics and prospect. Međunarodni problemi 67(2–3):167–183. https://doi.org/10.2298/MEDJP1503167T
Liu Z (2021) History and prospects of cooperation between China and countries in Central and Eastern Europe. Rev Int Affairs 72(1181):43–54. https://doi.org/10.18485/iipe_ria.2021.72.1181.3
Qichao W, Wang J (2022) China and Japan in CEEC: competition, cooperation and co-existence? J Contemporary East Asia Stud 11(1):86–106. https://doi.org/10.1080/24761028.2022.2049028
Mitić A (2022) Mapping the Obstructive narratives on China-CEEC cooperation. In: Horvath L (ed) Results and challenges: 10 years of China-CEEC cooperation. Eurasia Center of John Von Neumann University, Budapest, pp 32–58
Download references
Acknowledgements
Manuscript has been translated by a professional translator Tanja Paunović, Republic of Serbia.
The study presents the findings of a study developed as part of the research project entitled ‘Serbia and Challenges in International Relations in 2024’, financed by the Ministry of Science, Technological Development and Innovation of the Republic of Serbia, and conducted by the Institute of International Politics and Economics, Belgrade.
Author information
Authors and affiliations.
Institute of International Politics and Economics, 25 Makedonska Street, Belgrade, 11000, Republic of Serbia
Nevena Šekarić Stojanović & Katarina Zakić
You can also search for this author in PubMed Google Scholar
Contributions
NŠS and KZ prepared concept of the study, analysis, and interpretation of the results. NŠS was the major contributor in the literature and renewable energy policies review. KZ was a major contributor in data collection and formulation of the database. Both authors participated in manuscript editing and approved the final manuscript.
Corresponding author
Correspondence to Nevena Šekarić Stojanović .
Ethics declarations
Ethics approval and consent to participate.
Not applicable.
Consent for publication
Competing interests.
The authors declare that they have no competing interests.
Additional information
Publisher's note.
Springer Nature remains neutral with regard to jurisdictional claims in published maps and institutional affiliations.
Appendix: Chinese renewable energy projects in CEEC, from 2014–2022 (million €)
This table contains seven rows of information on each RE project, including country, project (hydropower plant (HPP), solar park, wind park, etc.), Chinese partner/investor in the project (and, in some cases, information about a local partner), type of the project (e.g., FDI, loan, construction, design, etc.), renewable energy subsector (in which it was invested), status of the project (active, on hold, finalised, etc.) and the project’s value (million €).
Rights and permissions
Open Access This article is licensed under a Creative Commons Attribution 4.0 International License, which permits use, sharing, adaptation, distribution and reproduction in any medium or format, as long as you give appropriate credit to the original author(s) and the source, provide a link to the Creative Commons licence, and indicate if changes were made. The images or other third party material in this article are included in the article's Creative Commons licence, unless indicated otherwise in a credit line to the material. If material is not included in the article's Creative Commons licence and your intended use is not permitted by statutory regulation or exceeds the permitted use, you will need to obtain permission directly from the copyright holder. To view a copy of this licence, visit http://creativecommons.org/licenses/by/4.0/ . The Creative Commons Public Domain Dedication waiver ( http://creativecommons.org/publicdomain/zero/1.0/ ) applies to the data made available in this article, unless otherwise stated in a credit line to the data.
Reprints and permissions
About this article
Cite this article.
Šekarić Stojanović, N., Zakić, K. Renewable energy as a connecting spot between China and Central and Eastern European countries: status, directions and perspectives. Energ Sustain Soc 14 , 10 (2024). https://doi.org/10.1186/s13705-024-00439-2
Download citation
Received : 09 October 2023
Accepted : 05 January 2024
Published : 18 January 2024
DOI : https://doi.org/10.1186/s13705-024-00439-2
Share this article
Anyone you share the following link with will be able to read this content:
Sorry, a shareable link is not currently available for this article.
Provided by the Springer Nature SharedIt content-sharing initiative
- Energy- and climate-related policies
- Sino-scepticism
Energy, Sustainability and Society
ISSN: 2192-0567
- General enquiries: [email protected]
- Bahasa Indonesia
- Bahasa Malaysia
Show all news, opinion, videos and press releases matching →
About Eco-Business
Sustainable development goals.
- Press Releases
International Edition
Interview: China’s renewables ‘pave the way to rapidly reduce coal reliance’
China installed a record 293 gigawatts (gw) of wind and solar in 2023 – pushing its total capacity to 1,050gw, according to a new report..
The report , published by Australia-based think tank Climate Energy Finance , says that, if this rate of renewables growth is maintained, then China could reach its “ dual carbon ” climate goals earlier than planned.
Here, Carbon Brief interviews the author of the report, Xuyang Dong. The questions and Dong’s answers are reproduced in full, below.
(An abridged version of this interview was published in the 16 May edition of China Briefing , Carbon Brief’s fortnightly email newsletter focusing on climate and energy developments relating to China. Sign up for free .)
Carbon Brief: Your report concluded that China’s coal power output will soon peak and decline – despite rising coal capacity – thanks to the rapid rise of clean energy sources. How widely do you think that potential tipping point is understood, both within China and internationally?
Xuyang Dong: This potential is not being understood or acknowledged enough both within China and internationally. China is prioritising energy security over the need to reduce coal-use. It has positioned thermal power as the backup energy source to ensure energy security, as electricity demand continues to grow and boost the economy.
This strategy is being emphasised after the downturn in hydropower generation last year caused by droughts, as well as blackouts in different parts of the country due to the unmet rising electricity demand. I think there is a pressure domestically of not wanting to admit it, as they really want to ensure energy security first. Concurrently, China is increasing renewable energy capacity at a staggering pace that far outstrips every other nation on the planet.
Internationally, news headlines continue to emphasise that China is building new coal-fired power plants, leading to a lack of confidence about China’s commitment to decarbonising its national electricity grid, although the expansion in renewable energy additions in China is at an unprecedented speed and scale.
However, the picture is more positive when we look at installed capacity. At the end of March this year, 53 per cent of China’s installed capacity is zero-emissions. This paves the way for China to reduce its reliance on coal and to do so rapidly – as we map in our report. China now needs to be more ambitious in its climate targets and it is well positioned to do so.
CB: If China is to announce more ambitious climate goals and expand renewable energy like you suggested in the report, in your opinion, what are the barriers?
XD: We are aware there are concerns over China’s land-use as a major constraint for building more wind and solar farms. We have run a case study on a 1.5 gigawatt (GW) solar project being built in the Tengger Desert in Ningxia province.
The project has 3.5m solar modules installed and only took up 0.1 per cent of the total desert. In our model, we estimate that China needs to install a total of 5,405GW of new solar capacity to reach its dual-carbon targets and that may require only 11 per cent of a total land area of the Gobi Desert, a neighbouring desert to Tengger.
The real challenge is that more transmission lines are needed. China recently started construction of an ultra-high-voltage power line project , which will cover three provinces – Shaanxi, Hubei and Anhui – to send 36 terawatt hours (TWh) of electricity to Anhui per annum and help boost renewable energy consumption by more than 18TWh per annum.
More transmission lines like this are needed to maximise the renewable energy generation potential of China’s desert areas and to resolve China’s land use constraints in the east coast.
CB: What do you think about policy support?
XD: I think being more ambitious in the overall climate target would be a good start because China has the capacity, the money and the technology to deploy the renewable energy at the speed and scale it requires.
Considering its political system is “top-down”, a more ambitious target could help the central government to give out more mandates, build better transmission lines and distribute the generated power into the areas that are needed.
Internationally, China needs to align with other developed countries to take its responsibilities as the leading renewable superpower and the carbon price would be an important policy lever. The external incentives and penalties, such as [having a Chinese version of] the EU’s carbon border adjustment mechanism (CBAM), would also help.
CBAM encourages the EU’s trading partners, especially China, to reduce the emissions of their exports. A further driver would be for other nations to also catch up with China’s staggering renewable expansion and start to emulate its speed and scale, so there will be no excuse left for China to do less.
CB: Speaking of CBAM, your report recommended China to have one of its own. Can you explain how introducing one would help, politically, to enable greater ambition from China’s leaders?
XD: Having one itself could incentivise China to increase the price of carbon, which currently is significantly lower than the EU and the rest of the developed world, although there is a deflation in China and most of the commodities are a lot lower than the rest of the world.
But a higher price on carbon could be a good incentive, especially limiting the emission in the manufacturing industry. It could also match China’s carbon price with the EU and lead to less trade barriers. For other trading partners, for example, Australia. Australia has been exporting raw materials. It could also incentivise Australia to do an embedded decarbonisation on its exports as well.
CB: Wouldn’t you worry the CBAM would make trading with some countries, such as developing countries, harder with China?
XD: The Chinese CBAM could list different categories and for different countries. It can have a higher standard for the developed countries and encourage the developed world to help the emerging market and developing economy to decarbonise. In the Asia-Pacific region, China, Japan, South Korea and Australia could work together to decarbonise.
CB: In the future you described, what role do you think solar and wind energy will play?
XD: As our report mapped out, wind and solar will be the leading energy sources in the future. China’s manufacturing capacity is driving down the cost for solar panels, modules and wind turbines.
The cost of deploying them is lower, too. In the meantime, they have the world’s leading technology, which can increase the utilisation rates. However, it needs to be accompanied by a better sort of combination in the energy storage system, and better energy storage, so the renewable energy it generates doesn’t go to waste and it can also help with China’s entire curtailment issues .
CB: What do you think about the current energy storage situation in China?
XD: It has become a priority compared to a year ago. Most of the policy before is supporting more build out for solar and wind power projects, progressively, and now we can see more documents focusing on storage systems. In fact, there is more and more manufacturing capacity for batteries as well, so we can see a dropping price in the batteries, which will be beneficial for a larger deployment of energy storage systems.
CB: Speaking of solar and battery, what do you think about China’s “new three” – solar, batteries and EV – and how they help China in energy transition and economy?
XD: The “ new three ” has played a very huge part in China’s economic growth. In 2023, 40 per cent of China’s total 5.2 per cent GDP growth last year was driven by it. [Read more on Carbon Brief’s analysis on clean energy and China’s economic growth in 2023]. This is very significant, especially because China is facing multiple headwinds in different areas, including the housing sector, population decline and deflation.
According to the International Energy Agency (IEA), the first quarter of 2024 saw China sell nearly 1.9m electric cars, more than the rest of the world combined. I think it’s inevitable that China’s solar manufacturing overcapacity continues to lead the global renewable market.
China’s solar manufacturing overcapacity has been a big topic and it is posing a threat to the industry as it is resulting in price slump for the solar panels and making a lot of business non-profitable. However, there are still some major players remaining financially healthy.
I know there are a lot of concerns about this overcapacity in the industry, such as in the EU and the US, and I think for China to address the concerns over industrial overcapacity, it needs to, first, stimulate domestic demand and deployment of solar and windfarms, energy storage systems buildout and EV sales.
Secondly, China could use its cheap renewable exports to help emerging markets and developing economies to build more renewable energy capacity, boosting and accelerating the global energy transition. Finally, it should be collaborating on joint ventures with European and US investors to build local factories.
CB: You mentioned there are some “financially healthy” Chinese companies and they have often been accused of using state subsidies to win “unfair” competition. What’s your view on the accusations?
XD: It’s a very classic way of the Chinese government doing things. When they see an opportunity, they build the capacity first and they will even run at a loss-making state to just dominate the market. Once they have taken over the market, they can profit from that. China has shown this sort of a pattern of doing business in the past.
However, in the meantime, China has shown it has the labour capacity, resources and the capital to deploy or develop the manufacturing capacity at this rate. It drives down the prices of a solar panel and module, wind turbine, as well as battery and EV prices, so I think it is good news to the global energy transition overall, especially for countries from the emerging market and developing economies when they really need more capital and more cost-efficient materials for them. So I guess it really depends on how you look at it and how you work with China instead of working against it.
This story was published with permission from Carbon Brief .
Like this content? Join our growing community.
Your support helps to strengthen independent journalism, which is critically needed to guide business and policy development for positive impact. Unlock unlimited access to our content and members-only perks.
Related to this story
- Carbon & Climate
- Policy & Finance
- clean energy
- electric vehicles
- electricity
- energy efficiency
- energy intensity
- energy security
- fossil fuels
- infrastructure
- power plants
- renewable energy
- decarbonisation
- energy transition
- electrification
- 9. Infrastructure
- 13. Climate
- 17. Partnerships
Most popular

News / Waste Management
Singapore’s world-first integrated waste and water treatment facility hit by construction delays.

News / Food & Agriculture
Why do environmentalists oppose genetically modified ‘golden rice’.

News / Energy
Wind and solar are ‘fastest-growing electricity sources in history’.

News / Green Buildings
Real estate advisory jll appoints teanna tan to lead asia pacific sustainability.

Opinion / Carbon & Climate
Strengthening asia’s voice for sustainable development.

News / Policy & Finance
From rhino bonds to debt-for-nature swaps: can these financing instruments protect asia’s biodiversity, featured events, undp report launch: biodiversity and the business and human rights agenda, unlocking capital for sustainability 2024 - indonesia, unpacking eu sustainability-related policies, kuala lumpur, wasted | malaysia film premiere, unlocking capital for sustainability 2024 - malaysia, guangzhou, guangdong, 2024 solar pv & energy storage world expo (formerly pv guangzhou 2024).
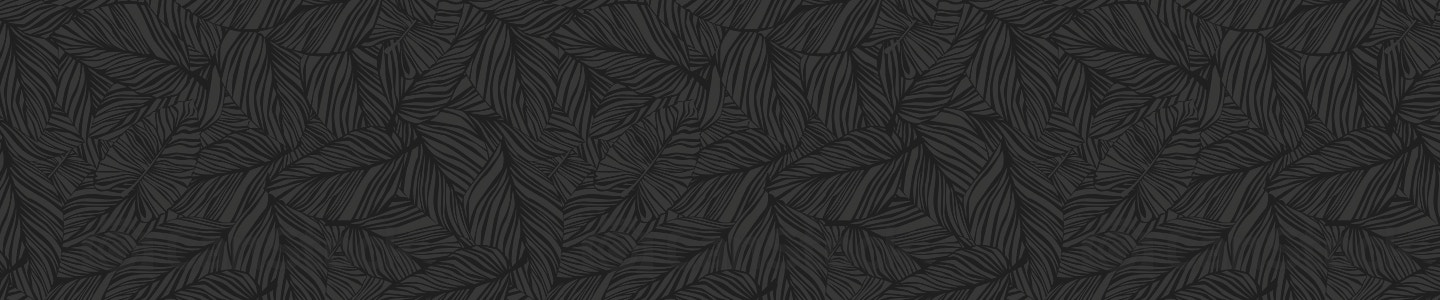
Transforming Innovation for Sustainability Join the Ecosystem →
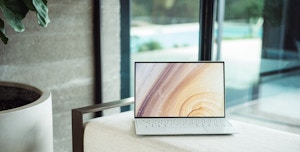
Receive the latest news in sustainability, daily or weekly.
Strategic organisations, support our independent journalism. #newsthatimpacts.
Advertisement
Supported by
How China Rose to Lead the World in Cars and Solar Panels
Heavy subsidies for industry, together with weak sales in China, have set the stage for an export boom, raising fears of factory job losses elsewhere.
- Share full article

By Keith Bradsher
Reporting from Beijing
For decades, China has moved methodically to dominate ever more industries, from toys and clothing in the 1980s to semiconductors and renewable energy today. China now produces a third of the world’s manufactured goods — more than the United States, Germany, Japan, South Korea and Britain combined. Its trade surplus in these goods is equal to a tenth of the entire Chinese economy.
And those exports keep increasing, causing alarm about China’s manufacturing “overcapacity” among its biggest trading partners. Top leaders in the United States and Europe have begun calling on China to dial back how much it sells to the world, and to increase its imports. On Tuesday, President Biden raised U.S. tariffs sharply on imports from China of electric cars , solar panels and other high-tech manufactured goods.
China’s industrial policies had a consistent focus.
Almost a decade ago, China launched an ambitious program called Made in China 2025 . The plan was for China to replace key imports in 10 advanced manufacturing industries by making its own products. The state-controlled banking system directed loans to those key sectors.
Fast forward 10 years, and China’s domestic economy is hurting mostly because of a housing market crash. Leaders in Beijing have ordered increased lending for many of the same manufacturing sectors to compensate for slower consumer spending, and are stepping up exports.
For China’s economic policymakers, the strategy is familiar.
It works like this: Regulators restrict the investment options of Chinese households, which have little choice but to deposit enormous sums of money into banks at low interest rates. The banks then lend the money at low rates to start-ups and other businesses. According to China’s central bank, net lending for industry swelled to $670 billion last year from $83 billion in 2019.
Beijing instructs local governments to help the chosen industries. The assistance takes the form of cheap land for factories, new highways for freight trucks, bullet train lines and other infrastructure.
The Kiel Institute for the World Economy in Kiel, Germany, calculated in a study that more than 99 percent of Chinese companies whose stock traded publicly received direct government subsidies in 2022.
China keeps factory wages low, which helps the competitiveness of its manufacturers. Residence permits limit the ability of rural families to move permanently to cities, where they would qualify for better job benefits. Independent labor unions are barred, and would-be organizers are detained by the police.
Those programs have helped China grow in many industries, fanning fears in the United States and elsewhere that factory jobs could be lost. American tariffs are now targeting exports in some of China’s largest and fastest-growing industries.
Car exports rose fast.
The auto sector is a prime example of how China has been able to move so fast to gain manufacturing dominance.
Just four years ago, China was a weakling in car exports, shipping one million low-priced cars a year mainly to less affluent markets in the Mideast and elsewhere. China has since surpassed Japan and Germany by a wide margin to become the world’s largest car exporter. Shipments are running at an annual pace of nearly six million cars, sport utility vehicles, pickups and vans.
Three-quarters of these exports, particularly to Russia and to developing countries, are cars with gasoline engines, which fewer buyers in China want. Battery electric cars are cheaper to buy in China, and electricity to charge them is cheaper than gasoline.
China’s top leaders have heavily subsidized the research and production of battery electric cars for the past 15 years .
Companies are ramping up their manufacturing of battery electric cars and building a fleet of ships to export them to distant markets, particularly in Europe. Automakers are introducing 71 models of electric cars in China this year, many of them loaded with advanced features and selling for less than comparably equipped cars in the West.
China now leads in producing electric car batteries.
China started off far behind the West in electric car batteries — and Chinese officials knew it.
By 2011, Beijing had begun requiring Western companies to transfer key technologies to operations in China if they wanted consumers in China to receive the same subsidies for imported electric cars that were being offered for cars made in China. Without the subsidies, automakers like General Motors and Ford Motor could not compete with electric cars made in China.
Multinational automakers responded by pressuring their South Korean suppliers, which at the time led the electric car battery industry to build factories in China. Beijing went further in 2016 and declared that even electric cars made in China would qualify for consumer subsidies only if they used batteries from factories owned by Chinese companies . Even automakers like South Korea’s Hyundai abandoned the Chinese factories of South Korean battery manufacturers and switched their contracts to Chinese battery companies like CATL.
Chinese companies now produce the majority of the world’s electric car batteries. Technological breakthroughs over the last several years have meant the cars can achieve greater range.
According to a new report from the Atlantic Council, a research group in Washington, China’s exports of lithium-ion batteries leaped to $65 billion last year from $13 billion in 2019. Nearly two-thirds of these exports went to Europe and North America. Much of the rest went to East Asia, where the batteries are often assembled into products that end up being sold to Europe or North America.
China turned to solar to reduce reliance on oil imports.
China has long made solar panels a top priority to limit its dependence on imports of oil and other fossil fuels along sea lanes controlled by the United States or India, another geopolitical rival. A tenfold expansion of China’s solar panel manufacturing capacity from 2008 to 2012 caused the world price of solar panels to drop about 75 percent. Many American and European factories closed.
Three of China’s largest solar panel producers suffered financial collapses of their own as prices plunged, saddling banks with losses on loans. Smaller rivals in China were able to buy their factories for fractions of the original construction cost. This second generation of companies was then able to make panels more cheaply and invest in cutting-edge research.
Chinese companies make almost all of the world’s solar panels. The country’s exports of solar cells, which the Biden administration is raising tariffs on, have more than doubled in the past four years, to $44 billion last year. China is ramping up twice as fast its exports of solar wafers, a key component.
U.S. limits on chips promoted a China shift.
Export controls by the United States have limited the sale to China of the most advanced semiconductors, which make up about 5 percent of the market, and the technologies to manufacture them. But Chinese companies, benefiting from enormous government subsidies, have become more competitive in the other 95 percent of the market.
The chips made by China are used in a range of equipment in the West, including many cars. Even gasoline engines in cars are controlled by semiconductor often made in China.
Why is the West acting now?
The November election has put political pressure on President Biden to show that he is taking a tough stand toward China .
Trade issues have also become enmeshed with security concerns. Russia’s war in Ukraine is showing that wars may be decided in part by which side can make more drones, artillery shells and vehicles.
China contends that its rising trade surpluses are the legitimate result of the competitiveness of Chinese companies.
Jorge Toledo Albiñana, the European Union’s ambassador to China, disagreed. “In Europe,” he said in a speech last week, “there is increasing pressure to react to what is widely seen as a worsening lack of level playing for our companies and investors.”
Keith Bradsher is the Beijing bureau chief for The Times. He previously served as bureau chief in Shanghai, Hong Kong and Detroit and as a Washington correspondent. He has lived and reported in mainland China through the pandemic. More about Keith Bradsher

An official website of the United States government
The .gov means it’s official. Federal government websites often end in .gov or .mil. Before sharing sensitive information, make sure you’re on a federal government site.
The site is secure. The https:// ensures that you are connecting to the official website and that any information you provide is encrypted and transmitted securely.
- Publications
- Account settings
Preview improvements coming to the PMC website in October 2024. Learn More or Try it out now .
- Advanced Search
- Journal List
- v.10(10); 2024 May 30
- PMC11112324

Developing a conceptual partner selection framework for matching public–private partnerships of rural energy internet project using an integrated fuzzy AHP approach for rural revitalization in China
a School of Ethnology and Sociology, Minzu University of China, Beijing 100081, China
Mingxuan Zhang
b College of Economics and Management, Hebei Agricultural University, Baoding, 071001, China
c College of Humanities and Social Sciences, Hebei Agricultural University, Baoding, 071000, China
Tahir Mahmood
d Department of Mathematics and Statistics, International Islamic University Islamabad, Pakistan
Associated Data
The data that support the findings of this study are available from the corresponding author upon reasonable request.
The development of rural clean energy is the key to cope with the shortage of traditional energy supply in the rural revitalization strategy and improve the sustainability of rural energy supply. Under the background of digital age, the development and utilization of rural clean energy Internet has become the focus of rural economic development. The government partners of the Rural clean Energy Internet PPP project (RCEIPPPP) are the key to promoting the green and intelligent development of rural energy. In this paper, the index system of project partner selection is constructed, and the problem of government partner selection for RCEIPPPP is studied by AHP and fuzzy comprehensive evaluation. The results of this study are as follows: 1) Partners' financial ability, technical ability, management ability, performance experience, corporate reputation, cooperation ability and risk management are the influencing factors for government partner selection of rural clean energy Internet PPP projects (RCEIPPPPs); 2) Compared with other factors, financial ability, technical ability, management ability and performance experience are the four key factors that are more important in choosing partners; 3) The empirical research shows that AHP, fuzzy comprehensive evaluation and the index system constructed by this research can be applied to the practice of government partner selection for RCEIPPPPs. This study puts forward the evaluation system of government cooperation selection of energy Internet PPP projects from the theoretical level, improves the existing research methods, and makes the theoretical system in this field more complete. From the practical level, it provides scientific basis and suggestions for the government to make decisions on energy Internet PPP projects, and improves the engineering efficiency and quality of rural clean energy Internet construction. This study demonstrates the complexity of clean energy projects, the need for an integrated approach to decision-making, and the need for project managers to actively manage communication and collaboration with partners to ensure successful project implementation.
1. Introduction
Since the implementation of China's rural revitalization strategy, although there have been no small development and breakthroughs in rural economy, humanities and other aspects, but the rigid constraints of resources and environment are tightening, the traditional driving forces supporting farmers' income are gradually weakening, and new driving forces need to be cultivated. Clean energy can significantly reduce the dependence on traditional energy, thereby reducing greenhouse gas emissions and other environmental pollution, and comprehensively contribute to rural revitalization [ 1 ]. Therefore, the development of rural clean energy has become the key to improve the sustainability of rural energy supply, which has also been widely concerned at home and abroad. With the rapid development of China's rural economy and the acceleration of urbanization, the energy demand in rural areas is also growing. However, due to the problems of environmental pollution and resource consumption in traditional energy utilization, the development and utilization of rural clean energy has become very important, and promoting the development of rural clean energy is an effective way to improve farmers' quality of life and reduce air pollution. Rural areas are often relatively short of resources, and excessive use of traditional energy will not only lead to resource depletion, but also pose a threat to the health of farmers. However, clean energy can provide sustainable energy supply for rural areas due to its inexhaustible use [ 2 ]. According to the comparison of two national agricultural censuses, the energy consumption structure has changed to a diversified energy consumption structure that combines clean energy such as electricity and natural gas with traditional solid energy, and the proportion of rural household energy and electricity has increased by 57.8 %. The proportion of gas increased by 37.4 %, the proportion of firewood decreased by 16 %, and the proportion of coal decreased by 2.2 %. The use of clean energy met the growing energy demand in rural areas and promoted the sustainable development of rural economy [ 3 ]. In addition, the rural infrastructure construction is relatively lagging, the traditional energy supply is unstable, resulting in residential -electricity difficulties, insufficient lighting and other problems, rural clean energy has become a strong support for rural revitalization and agricultural and rural modernization of energy development outlet and important means, rural areas to promote clean energy has become the top priority to solve the energy problem.
On July 4, 2023, the National Development and Reform Commission and other jointly issued the “Guidance on the implementation of rural power grid consolidation and upgrading Project” stressed that “the construction of intelligent and open modern rural power grid, fully carrying the development and utilization of distributed renewable energy and local consumption”, so the construction of rural clean energy Internet has become the focus of national attention. The construction of rural clean energy Internet is an important direction of China's energy development at present, and it is also an inevitable choice to realize the strategy of rural revitalization. First of all, the construction of rural clean energy Internet can promote the development of rural communities and improve the quality of life of residents. By increasing the use of renewable energy and realizing the stability and intelligence of power supply, it can not only improve the energy supply situation in rural areas, but also provide more convenient, efficient and environmentally friendly services. Secondly, the construction of clean energy Internet can promote the transformation of rural energy structure, reduce dependence on traditional energy, reduce greenhouse gas emissions, improve air quality, and protect the ecological environment. The renewable energy (such as solar energy, wind energy, etc.) used in the Internet system is a green and clean form of energy that does not produce toxic waste and pollution. Relevant research results show that, from the perspective of the whole industry chain, a multi-phase, market-oriented rural energy business model is proposed, and there are certain difficulties in the development of rural energy. It is essential to fully unleash the initiative of both supply and demand. Users should actively engage in demand response efforts to enhance the alignment between supply and demand, thereby fostering the development of the entire energy supply chain. This approach will help optimize the profitability trajectory of the business model [ 4 ]. Finally, the construction of rural clean energy Internet helps to improve the stability and supply capacity of energy supply in rural areas. The use of local renewable energy resources, such as photovoltaic power plants, wind power generation, etc., can provide reliable power supply for rural areas to meet the needs of agricultural production, rural industry and residents' lives. In addition, the development of the clean energy industry chain will also drive employment growth, technological innovation and economic growth in related industries, promote the upgrading of rural industries and improve farmers' incomes. So, how to efficiently build a rural clean energy Internet? As a new form of project promotion in recent years, PPP project has become the main content of this paper.
PPP project is a public-private cooperation mode, which aims to realize the construction, operation and maintenance of public infrastructure through the resources, technology and experience of the government and private sector. With the continuous practice and evolution of the mode, the PPP mode has gradually formed the characteristics of diversified funding sources, high efficiency and flexibility, risk sharing and strong innovation ability. The importance of the rural clean Energy Internet PPP project (RCEIPPPP) is to promote the development of rural clean energy, improve the reliability of energy supply, and promote the development of rural economy and improve the quality of life of residents. On the one hand, the RCEIPPPP can promote the development and utilization of rural clean energy, and integrate various energy resources, including solar energy, wind energy, bioenergy, etc., to meet rural energy needs. Through the construction of clean energy power stations, distributed energy systems, etc., we can reduce the dependence on traditional energy sources, reduce carbon emissions, and achieve the goal of energy conservation and emission reduction [ [ 5 ]]. On the other hand, due to the lag of power supply facilities in rural areas, there is the problem of unstable power supply, which affects the normal life and production of rural residents. The RCEIPPPP can use advanced smart grid technology to achieve two-way networking of clean energy and traditional energy, form a diversified energy supply model, and improve the reliability and stability of rural energy supply. The construction of RCEIPPPPs will drive the development of related industrial chains, such as photovoltaic cells, wind power generation equipment, energy storage technology, etc., while creating job opportunities, enhance the vitality and competitiveness of the rural economy, PPP projects will become an irreplaceable necessary link in the construction of rural clean energy Internet [ [ 6 ]]. Therefore, it is a particularly critical issue to study what factors will affect the construction of PPP projects for rural clean energy Internet, and then put forward reasonable suggestions for promoting rural energy intelligence.
According to the existing literature, there are abundant researches on the selection of government partners for energy Internet and PPP projects. The construction of energy Internet can solve the problem of energy security in China from the perspectives of supply channels and import channels, and the establishment of the world energy network will help realize the optimal distribution of energy resources [ [ 7 ]]. We should not only pay attention to top-level planning and path design, but also carry out scientific analysis and evaluation on the feasibility of investment and operation of specific projects as well as the risks they face. In order to rebuild and optimize key strategic factors, dynamic capabilities must be available and play a decisive role in these factors. China's geopolitical influence and global green and low-carbon development path should also be taken into account [ [ 8 ]]. The academic community has conducted in-depth research on energy Internet from different perspectives and cases, taking the electrical and electrical appliance manufacturing industry in Shandong Province as an example, to explore the role and performance of environmental identification, organizational change, learning innovation and comprehensive coordination ability in the strategic transformation of enterprises. In essence, the strategic transformation of enterprises is to achieve the match between internal factors and external environment. Throughout the exploration process of government partner selection in PPP projects, it can be seen that Zhang et al. [ [ 9 ]] summarized the dimensions and evaluation indicators of PPP private partner selection through the method of literature frequency survey, and empowered each evaluation dimension and index with the method of entropy weight. Through the research, it was found that In the evaluation of the selection of private partners for PPP infrastructure projects, the financial capacity and business reputation of the private sector occupy a large proportion. Cong et al. [ [ 10 ]] established a collaborative partner selection and decision model of social capital alliance based on the characteristics of the cloud model that qualitative and quantitative expressions can be mutually transformed, as well as the characteristics of grey correlation method, such as high information utilization efficiency and simple data processing. The example analysis proves that the optimization decision index system and model can effectively evaluate and predict the project and relationship factors, and can reasonably determine the priority order of various alternative partners, so as to provide a decision basis for the selection of alternative partners. Li et al. [ [ 11 ]] studied three different PPP project payment methods (government payment method, user payment method and feasibility gap subsidy method), and based on this, established a mixed market PPP model including private enterprises and state-owned enterprises, and analyzed the efficiency of government-enterprise cooperation from the perspective of maximizing social welfare. Furthermore, the selection of the optimal project partner and project mode for the government is discussed, but there is a lack of specific discussion on the mechanism of rural clean energy Internet and practical research on the government's choice of cooperation objects.
Therefore, this paper focuses on the selection of government partners for RCEIPPPPs, in order to supplement the existing literature. This paper attempts to study the selection of government partners for RCEIPPPP to reveal the impact of the selection of government partners in PPP mode on rural clean energy Internet, so as to enrich the analysis perspective of PPP mode and government partner selection for energy Internet. This paper uses the literature reading method to give the government partner selection index system, and classifies the rural clean energy Internet energy enterprises, involving qualitative indicators and quantitative indicators. The entropy weight method is used to standardize the qualitative and quantitative indicators in a unified manner, and the government partner indicators of each bidder are jointly evaluated to obtain the comprehensive evaluation coefficient of each enterprise. Through the robustness test of the coefficient, the selection index of the government partner selection of the rural energy Internet PPP project is finally obtained.
The contribution of this paper is to put forward the government cooperation selection evaluation system for energy Internet PPP projects, and for the first time innovatively use AHP and fuzzy evaluation methods to select and make comprehensive decisions on PPP project partners, improve the existing research methods, and make the theoretical system in this field more complete. It also provides scientific basis and suggestions for practical decision-making of energy Internet PPP projects. In the process of combining the realistic case analysis of the rural energy engineering project, the decision-making opinions obtained by the evaluation system constructed in this paper are consistent with the decision-making results of the realistic case, and the PPP project promotes the leapfrog development of the economy and society of the county where it is located, and gets a good response, which also reflects the rigor and science of this paper to a certain extent, and also reflects a certain reference significance.
The remaining structure of this paper is as follows. Section 2 is a literature review from three aspects: energy Internet PPP project management, PPP project partner selection criteria and PPP project partner selection methods. Section 3 introduces the evaluation factors of PPP project partner selection and the partner selection system of RCEIPPPP in detail. Section 4 is the method presentation part of this paper, which introduces the five steps of PPP project partner selection and evaluation; Section 5 is the case analysis, using the real data to implement the method, and the case results are discussed; Section 6 is the conclusion of this paper.
2. Literature review
2.1. energy internet ppp project management.
The research on the energy Internet PPP project in foreign theoretical circles originates from the PPP model proposed by the British government in the 19th century. Since the further promotion of economic globalization, the PPP development of energy Internet has been highly concerned and valued by various academic circles at home and abroad, and has become a hot spot of concern for many scholars, and more research results have been achieved. These achievements can be summarized from three aspects: energy PPP project management, Internet PPP project management and Internet basic project management.
The research on energy PPP project management can be divided into two aspects: the application of energy PPP and the extension of energy PPP. PPP model is a new model of energy project financing. China introduced the PPP model for the first time in 1995. Chen and Chen [[ 12 ]] analyzed the PPP model of the energy-saving partnership development model of the US Virgin Islands and the integrated development model of the Dutch Voluntary environmental Agreement from the perspective of foreign countries, and proposed that the PPP model of energy-saving emission reduction in China needs to clarify the government's positioning, grasp technological innovation and application. The key points that need to be paid attention to when promoting the PPP mode of energy saving and emission reduction are emphasized. Bai and Tan [ [ 13 ]] introduced in detail the connotation and development process of PPP model in broad and narrow sense, and pointed out the construction and operation status of new energy electric vehicle charging facilities. PPP model is a new financing model, which aims to introduce social capital to carry out value-added services through PPP model. It is conducive to improving the construction and operation efficiency of new energy vehicle charging facilities, but there is a lack of PPP financial policy guarantee. Cao et al. [ [ 14 ]] discussed the optimization of the service mode of the integrated energy system. From the perspective of project investment and construction, they deeply analyzed the operation links and modes of the integrated energy project under different service modes, summarized and proposed the service mode suitable for the integrated energy system, and proposed the selection method of the mode. The service model evaluation system based on improved fuzzy comprehensive evaluation method is established. In the era of energy Internet, it is urgent for energy enterprises represented by power grid enterprises to adapt to the reform of the power system by transforming to comprehensive energy service providers and actively carrying out comprehensive energy services. Song et al. [ [ 15 ]] expanded the pricing method of asset securitization in PPP mode of new energy power, and conducted a case study using binary tree option pricing model for option-adjusted spread method. The study increased the financing efficiency of new energy power construction and promoted the market flow of asset securitization in PPP mode. Yang [ [ 16 ]] discusses the impact of social capital control on PPP project investment, and proposes that the higher the social capital control in China's energy PPP projects, the greater the investment in PPP projects; The longer the contract term of energy PPP projects, the less the investment of PPP projects, etc. This study provides a reference for social capital to participate in the investment decision of energy PPP projects. Wang [ [ 17 ]] combined the EMC-PPP model, took the energy-saving renovation project of an old residential area as the project, combined the energy-saving service management technology of an energy-saving company with the funds of social capital, solved the problem of difficult financing in EMC mode, and realized the complementary advantages of all participants. Based on the above, this paper proposes improvement methods for energy PPP projects: first, clarify the role of the government, relevant departments should improve the implementation system of energy PPP model; The second is to promote the expansion and innovation of the energy PPP model to promote the continuous innovation of technology.
The research on the management of Internet PPP projects can be divided into two aspects: the origin of Internet PPP projects and the expansion of Internet PPP projects under different backgrounds. There are few researches on Internet PPP projects abroad, but the research on this project in China comes from the application of PPP projects in Internet. Zeng [ [ 18 ]] analyzed the protocol structure and operation mechanism, and described its application in remote access technology. Keywords: remote access server, whose research and development is of great significance. These two studies opened up the history of China's PPP into the Internet and laid the foundation for the further development of Internet PPP projects. Ju [ [ 19 ]] analyzed the operation strategy of the “Internet + PPP” model from the perspective of SWOT model, formulated the strategic plan of the “Internet + PPP” model under the background of new urbanization, and constructed the PPP Internet platform was of great practical significance for promoting the construction of new urbanization and maintaining the steady development of China's economy. However, the analysis from this perspective is not profound enough, and some scholars have conducted in-depth exploration of “Internet + PPP” from different perspectives. Tang and Rui [ [ 20 ]] after analyzing the driving mechanism of Internet + collaborative integration, financing innovation model and appropriate evaluation method of VFM, proposed that the Internet + financing innovation model should be introduced when promoting the PPP model, which has a profound impact on the allocation of economic, cultural, environmental and social resources. Zhang and Yang [ [ 21 ]] summarized the relevant experience of Germany and the United States in applying the SPV-based PPP model to accelerate R&D breakthroughs in the field of integration and innovation, so as to compare the existing problems in China. However, the SPV model is too simple, and no effective solutions have been obtained for specific situations that are not applicable, and the foreign experience also has certain inapplicability in China. Shen et al. [ [ 22 ]] established the risk degree evaluation index system; The improved operator (ICUOWGA) is used to aggregate each index for many times, and the final comprehensive risk measurement value and the weight ranking of main factors are obtained. This method also introduces fuzzy semantic quantization operator and normalized decision matrix formula, which overcomes the shortcoming of the traditional method. Based on the above, this paper puts forward some improvement methods for Internet PPP project management: First, adopt foreign Internet PPP model according to the actual situation of our country; The second is to establish a risk evaluation index system to control risks and avoid subjective decision misjudgment.
The research on Internet infrastructure is relatively rich, mainly reflected in the analysis based on a certain perspective. ShiltonK [ [ 23 ]] argues that the participatory observations of a team developing Internet protocols show that the difficulty in defining stakeholders in infrastructure and the tension between local and global perspectives are complex value reflections, suggesting that Internet architects tend to equate the core value of interoperability with value neutrality. Ma et al. [ [ 24 ]] proposed to study the protection of Internet infrastructure against link flooding attacks from the perspective of technological economy, arguing that mitigating and defeating LFA is particularly challenging and global networks are increasingly threatened, and proposed two novel mechanisms to mitigate flooding attacks. Cooperation among autonomous systems is stimulated by incentive design and Nash bargaining. SajaniJ et al. [ [ 25 ]] established a hierarchical conceptual model to understand the preemptive strategy in PPP projects and its relationship to management issues. The study confirmed that the research results confirmed the importance of stakeholder participation in alleviating problems in PPP projects and related strategies. Lynch [ [ 26 ]] used CWNGuifi.net in Barcelona as a research object to explore how the sharing of Internet infrastructure relies on the practice of knowledge sharing, which complicates the imagination of the free flow of information without abandoning the possibility of an Internet-based public space. The research is conducive to making the Internet a digital public place and bringing a more just and equitable future for innovation in network technology. Kou and Xu [ [ 27 ]] focused their research on the practical problem of how Internet infrastructure affects carbon emission efficiency. Based on the global Malmquist-Luenberger index model of relaxation variables, static panel and panel quantile regression models were used to estimate carbon emission efficiency. The study reveals how local government intervention and market segmentation can break the bottleneck of the impact of Internet infrastructure on carbon emissions, and how governments can continuously optimize the external environment to ensure the positive impact of Internet infrastructure on carbon emissions performance. Based on the above, the improvement method proposed in this paper for the study of Internet infrastructure is to consider establishing scientific values and strengthening the interaction between Internet infrastructure and local government intervention and market segmentation.
2.2. PPP project partner selection criteria
In recent years, the research on PPP project partner selection criteria has been highly concerned and valued by academic circles at home and abroad, and rich research results have been achieved. These results are mainly from the focus of PPP project partner selection and research examples. Wang et al. [ [ 28 ]] used literature research and expert interviews and other methods to build an indicator system for partner selection, determined the weight of indicators with ANP method, and proposed reasonable allocation of weights according to their importance, which played a certain guiding role in the selection of partners in practice. Chen et al. [ [ 29 ]] analyzed the role of contract functions and information transparency in PPP project cooperation, and believed that the efficient selection of partners could be promoted by strengthening trust among procurement partners. Hu et al. [ [ 30 ]] identify the financial risks of PPP projects to compensate for the failure in financial risks between the government and SPV due to the lack of control over financial risks. The study found that risk analysis, identification and management to deal with uncertainties and contingencies in scope, time, cost and quality is the best choice for the financial risk management of PPP projects, and the study is conducive to further improving the financial risk of public-private partnerships and their funding sources for successful public-private partnership projects. Li et al. [ [ 31 ]] proposed that the PPP + EPC model provides an effective way to solve the investment and financing problems of large-scale public infrastructure construction and improve the construction quality of complex infrastructure projects. Taking the Yiwu Mall Tunnel project in Zhejiang Province as an example, the organizational structure, operation process and responsibilities of construction participants of the design-led PPP + EPC project were analyzed. Summarizing the experience and practice of design-led PPP + EPC mode has great reference value for the implementation of related projects. Nair and Zhang [ [ 32 ]] proposed that EUG Energy chose more partners for the key links of smart grid projects, including smart meters, unified network platform, and assisting enterprises to manage the implementation of smart grid and utility transformation. Grondys and Malinauskaite are also suggested that the enterprise ecosystem helps customers realize the full potential of their sustainability and energy management programs [ [ 33 ],[ 34 ]. Hu [ [ 35 ]] In order to better analyze the performance and improve the design of industrial alarm systems, several industrial case studies were conducted based on alarm data collected from oil sands mining plants operated by Suncor Energy Company in northern Alberta, Canada. This study shows the practicability and usefulness of advanced alarm management tools for alarm rationalization and daily alarm management. Andreas et al. [ [ 36 ]] (2016) studied the energy saving potential of the battery-assisted fleet of trolleybuses. This project was completed jointly with an industrial partner and received financial support from the Swiss Federal Energy Agency (SFOE), thus the partnership improved the operation effect of the trolleybuses. Kazemilari et al. [ [ 37 ]] present an initial analysis of renewable energy companies listed on the stock exchange using the minimum spanning trees approach. Aba-Sah [ [ 38 ]] proposed that waste-to-energy projects require complex value chains to operate effectively. In order to identify business partners, plant operators need to establish links with organizations whose strategic objectives are consistent with their own, and conclude that supplier organizations need to find out their position in the value chain and explain the partnership based on the value of the partner organization. Raghuvanshi et al. [ [ 39 ]] argue that some countries around the world are pursuing energy security to ensure the smooth running of their economies and clean energy supply, and this study provides a multi-dimensional tool that can help build successful strategic alliances to achieve energy security. In addition, the study could facilitate different stakeholders to measure the success of any strategic alliance in the energy sector. Based on the above, this paper proposes improvement methods for the selection criteria of PPP project partners: First, multi-level partners to promote the steady progress of all major links in PPP projects; The second is to understand the value concept of partners and encourage their technological innovation to improve the cooperation compatibility of PPP projects.
2.3. PPP project partner selection method
The criterion of PPP project partner selection provides reference for the PPP project partner selection method, and the discussion of its selection method by senior students at home and abroad has been deepened. Xu et al. [ [ 40 ]] introduced the concept of resource confidence and proposed a cross-enterprise project partner selection model considering resource confidence, so as to minimize the risk of cross-enterprise project failure and delay caused by the uncertainty of partner resources. Within a certain range, this model can find the optimal solution in a short period of time with a high probability. He [ [ 41 ]], aiming at the problems of PPP partner selection and investment incentive contract in the medium or operation period of public project investment, established a cooperative and joint investment contract and subsidy model under existing conditions, analyzed the impact of government management efficiency on cooperative investment contract, and provided a basis for decision-making on PPP partnership investment. Song and Xu [ [ 42 ]], in order to solve the problem that the excessive satisfaction level of individual decision makers leads to the uncoordinated decision-making behaviors of individual decision makers in PPP project group decision-making, designed the scheme by reducing and correcting the satisfaction level of each decision subject, and obtained the satisfactory solution under multi-objective group decision-making by using iterative algorithms. Yan [ [ 43 ]] analyzed the implementation of PPP from the perspective of audit, emphasizing the possible problems in performance responsibility, bidding procedures, contract management, operation and implementation of partnership, so as to effectively promote the better application and development of PPP. Zhang et al. [9] considered the whole process of PPP project construction, built an evaluation index system for partner selection of infrastructure projects under the PPP model, introduced the entropy weight theory to determine the weights of each index, established a multi-level fuzzy comprehensive evaluation model, and cited examples to verify the practicability and scientificity of the model. Wu and Wu [ [ 44 ]] took the real estate projects of pension institutions as research objects, analyzed the characteristics of PPP projects of pension institutions in terms of service level, liability risk, government supervision and other aspects, as well as the advantages and disadvantages of potential partners with the government, so as to provide decision-making reference for the government to choose suitable partners. Yi [ [ 45 ]], taking the selection of private partners for PPP rail transit projects as the research object, proposed a three-stage selection mechanism including access regulation, selection procedure and negotiation through literature research and reference to two-stage selective bidding for international projects. The conclusion is objective and effective. Cong et al. [10] built a partner selection and decision model of social capital consortium based on qualitative description and quantitative representation and grey correlation method through the system integration cloud model, focusing on the partner selection and evaluation of social capital consortium under the PPP model. Tong et al. [ [ 46 ]] elaborated the formation mechanism of resource complementarity from the perspective of cooperative game, proposed a solution method based on expected return constraint selection, and constructed a project partner selection model based on resource complementarity with two-layer programming. Feng et al. [ [ 47 ]] studied the matching relationship between government and enterprises, applied grey target decision theory to quantitatively process the evaluation information of various indicators between government and enterprises in PPP projects, and finally built a public-enterprise matching model for PPP projects, which is conducive to the expansion and extension of public-enterprise partner selection methods for PPP projects. Wang et al. [ [ 48 ]] pointed out that in the early stage of PPP projects, the indicators selected by private partners would be uncertain and fuzzy, and adopted the binary semantic method to integrate and evaluate quantitative and qualitative indicators in a unified manner to obtain the functional evaluation value (comprehensive evaluation value) of each enterprise's corresponding indicators. In combination with each scheme, the partner with the highest value coefficient is selected as the most priority for the PPP project. Bisello et al. [ [ 49 ]] The urban energy district project introduces technological innovation in the energy system and brings immaterial benefits to the city, which are not properly understood and considered. This study investigates the group knowledge and group thinking on this topic through the method of the world cafe, laying a foundation for the change of end-user behavior and intangible assets. Shan and Xu [ [ 50 ]] Tax incentives play an important role in the promotion of PPP model. The tax relationship between PPP partners is an important economic lever to adjust the interest balance of partners. The impact of tax incentives on the financial balance of PPP projects is analyzed from the perspective of net present value. Zhang et al. [ [ 51 ]] analyzed the motivation and selection tendency of energy-using institutions in selecting EPC project partners, assumed the selection tendency of partners in different procurement environments, verified through successful EPC cases, and put forward appropriate approaches and relevant suggestions for energy-using institutions' partner selection. Li [ [ 52 ]] studied the measurement scale of the impact of partner selection indicators on performance, and built the impact model of selection indicators, which provided certain references for scientific allocation of PPP partner selection indicator weights and improvement of PPP project performance. However, it only included static analysis and lacked certain path quantitative reasoning. Through descriptive statistical analysis method, Yin and Zhao [53] the driving mechanism of rural new energy connection is deeply discussed to provide guidance for optimizing the construction of rural new energy system. Yin and Zhang [54] analyzed the pressure-state-response (PSR) model of digital technology to promote green innovation in manufacturing industry, and built the measurement system of digital green innovation in manufacturing industry according to the PSR framework, which promoted the green intelligent development of manufacturing industry. Yu and Yin [55 , 56] explores and clarifies the entanglement mechanism between new energy enterprises and village collective, constructs the quantum evolutionary game model of new energy enterprises and venture capital enterprises, and introduces a set of quantum strategies for both sides, clarifies the entanglement mechanism between new energy enterprises and village collective, and promotes the smooth implementation of regional ecological environment planning and planning process. Li et al. [11] took cooperation efficiency as the starting point, introduced three types of PPP payment models, including government payment, user payment and feasibility gap subsidy, built a mixed-market PPP model, analyzed the efficiency of government-enterprise cooperation from the perspective of social welfare maximization, and discussed the selection of optimal government PPP project partners. Based on this, this paper proposes improving methods for PPP project partner selection: first, strengthen the practicability of PPP project partner selection model; The second is to enrich the dynamic model research of PPP project partner selection method.
3. Analysis of energy internet PPP project partner selection factors
3.1. energy internet ppp project partner role analysis.
Partnership is the highest level of cooperation between people or between companies, it refers to the full communication on the basis of mutual trust, challenging improvement plans for the common goal, and strict criteria to measure cooperation performance and continuous improvement. In the whole life cycle of construction and operation, the rural clean energy Internet project using the PPP model involves multiple stages including financing, design and construction. At each stage, private partners from different sectors will participate in the project operation and share risks and benefits. Therefore, the roles of private sector partners should include project financing roles, technical roles, management roles, cooperation roles, and risk management roles.
- (1) Project financing role. The promotion of the rural clean energy Internet PPP model can effectively alleviate the financial pressure caused by the lack of national funds while reducing the environmental pollution and resource consumption caused by the traditional energy model. Among them, the introduction of the PPP model is mainly completed by energy Internet enterprises, so enterprises should not only have a good asset situation and capital liability ratio, but also develop a clean energy Internet fund use plan, and ensure the rationality of the financing plan.
- (2) Technical role of the project. In the engineering construction of the energy Internet, the technical role plays a core role, and the embodiment of the technical role also includes many aspects, including the rural human resources of the enterprise, the construction management, maintenance and operation of the clean energy Internet, core technologies, and facility transfer schemes. Internet construction projects in rural areas are facing more complex situations, so in the process of introducing the PPP model, attention should be paid to partners' control and application of technology, and technical solutions should be formulated for possible problems.
- (3) Project management roles. The infrastructure construction of clean energy Internet in rural areas has a huge investment, a long construction period, and a large number of participants. Project managers must conduct comprehensive management of all aspects of the project from investment decision, design, construction to operation. To this end, enterprises should be able to fully cooperate and communicate in the implementation process of PPP projects, and ensure the rationality of the organizational structure of rural PPP projects, the perfection of the clean energy Internet management system and the ability to operate and maintain the clean energy Internet, so as to achieve the expected purpose of the project and undertake the construction task of the project.
- (4) Project risk management roles. In the process of construction of rural clean energy Internet project, the risk in the construction process of Internet project is an inevitable objective phenomenon, and reasonable risk allocation is of great significance for the achievement of project objectives and the improvement of project performance. The private partnership introduced by the government should have the Internet risk management system process specifications to avoid the loss of project cost caused by excessive project cost.
3.2. Energy internet PPP project partner selection evaluation dimension analysis
PPP project operation process usually includes project initiation, preliminary preparation, design, bidding and tendering, project construction, acceptance and completion of six stages. Partners selected for RCEIPPPPs must have comprehensive capabilities and qualities. According to the literature review, domestic and foreign scholars have different views on the dimensions of partner selection in the PPP model. Wang et al. [27] divided the evaluation system into a preliminary evaluation stage and a detailed evaluation stage. In the preliminary evaluation stage, the partners of operational infrastructure PPP projects were evaluated from four dimensions, including qualification, financing and performance, and in the detailed evaluation stage, the partners were evaluated from seven dimensions, including finance, service, experience and reputation. Cao et al. [14] proposed two index systems, quantitative and qualitative, focusing on the detailed division of project risks from eight dimensions, such as political risk, financing risk, legal risk and force majeure risk. Wang et al. [48] constructed a private partner evaluation system from five aspects, including finance, technology, management, similar experience, and corporate reputation, and selected partners through a model based on binary semantics and value engineering. This paper uses a multi-level fuzzy comprehensive evaluation model, classifies the existing literature on the selection of contractors and concessioners in PPP projects, and identifies the dimensions of evaluation indicators. Through statistics, it is found that most scholars' views focus on finance, reputation, construction and operation capacity, risk management and control, contract management and other aspects, which can provide reference for the evaluation of PPP project partners. From the perspective of PPP project operation process and the role of rural clean energy Internet partners, this paper divides the selection and evaluation of partners into seven dimensions: financial capability, technical capability, management capability, performance experience, corporate reputation, cooperation capability and risk management. The corresponding relationship of each dimension is shown in Fig. 1 .

Energy Internet PPP project partner selection evaluation system division.
In the above figure, corporate reputation and risk management run through the construction process of the entire RCEIPPPP. Good business reputation can be used as an intangible resource for private partners in the construction process of clean energy Internet in rural areas. To some extent, it can effectively predict the Internet construction behavior of partners. This is very important for the decision of the project, and the risk management ability is reflected in every key link in the whole process. In the process of building clean energy Internet facilities, financing and fund management require the financing capacity of partners; Bidding and project construction require technical ability and management ability to ensure the smooth progress of project construction; The implementation of large-scale PPP projects inevitably requires strong cooperation and risk management capabilities. The specific evaluation system and indicators included are shown in Table 1 below:
- (1) Financial ability. This is a key factor to measure the strength of rural energy Internet PPP project partners, if the rural clean energy Internet project capital management ability is poor, can not carry out reasonable planning of funds, can not carry out effective financing of funds, resulting in a funding gap, which will have a great impact on the construction of Internet facilities. Therefore, the financial capacity of the enterprise includes: the rationality of the PPP project financing plan, the assets of energy Internet enterprises, the asset-liability ratio of energy Internet enterprises, the price competitiveness of rural PPP partners, the solvency of rural PPP cooperation Huoan, and the use of clean energy Internet funds.
- (2) Technical ability. Internet infrastructure construction investment is huge, the project is complex, the whole process of construction and operation management requirements are difficult, partners make full use of their technical advantages, to provide customers with high-quality public goods or services. Specific indicators specifically include the following: rural human resources, clean energy Internet construction management plan, clean energy Internet maintenance and operation plan, clean energy Internet core technology resources and clean energy Internet facility transfer plan.
- (3) Management ability. In the construction process of clean energy Internet infrastructure in rural areas, the project manager must conduct comprehensive management of all aspects of the project from investment decision, design, construction to operation, so as to achieve the expected purpose of the project and undertake the construction task of the project. Specific indicators include the following: rural PPP project cooperation and communication ability, the rationality of the organizational structure of rural PPP projects, the perfection of the clean energy Internet management system, and the clean energy Internet maintenance and management ability.
- (4) Performance experience. It is of great significance to select the right partner and focus on rural clean energy Internet infrastructure with the advantages brought by its experience in PPP project management and energy Internet facility construction. Specific experience includes: clean energy operation experience, energy Internet construction experience, rural PPP project financing experience and rural PPP project owner satisfaction.
- (5) Corporate reputation. In PPP projects, the link between the public and private sectors is not only the contracts signed by the two parties, but more importantly, the corporate credibility owned by the private sector. Therefore, in PPP projects, choosing the private sector with a good reputation as a partner can reduce transaction costs, improve construction efficiency and improve project performance. The qualification of the enterprise can reflect the operation ability of the partner from one side; Enterprise credit rating, in the past project, PPP project contract performance rate, breach of contract litigation record, enterprise energy Internet qualification level and other quality and safety records that affect the project period should be the object of key review.
- (6) Cooperation ability. Clean energy Internet infrastructure PPP projects require a higher level of collaboration capacity than ordinary public-private partnerships. Among them, the consistency of the strategic objectives of the PPP project, the experience of rural communication and coordination of the partners, the satisfaction of farmers in the whole cycle of the clean energy Internet, and the strategic compatibility of the whole life cycle of the clean energy Internet are the main characteristics.
- (7) Risk management. PPP projects have the characteristics of complex financing structure, huge investment scale, numerous participants, and complex relationship coordination. There are many uncertainties in the implementation and operation of rural clean energy Internet projects. The risk allocation of project parties is an important reason for the success or failure of PPP projects. Be able to effectively identify risks in the project and coordinate the relationship between project parties during the project implementation process. Among them, the financial resilience of PPP partners, the rationality of the Internet risk-sharing plan and the process specification of the Internet risk management system are all important indicators to measure the risk management level of private partners.
RCEIPPPP partner selection system.
4. Methodology
4.1. energy internet evaluation index system construction.
This paper adopts the literature frequency statistics method, and integrates the relevant opinions of middle and senior management personnel, technical personnel, supervision engineers of enterprises who have participated in large-scale PPP infrastructure projects, as well as public sector personnel with experience in PPP infrastructure projects, so as to comprehensively consider the comprehensiveness and scientificity of evaluation indicators. Finally, the evaluation index system of partner selection for infrastructure projects under the PPP model is constructed, and the specific contents are shown in Table 1 above.
4.2. Energy internet evaluation method
Since most of the index factors are fuzzy, it is difficult to describe them quantitatively, so as to provide decision-making basis for the partner selection of PPP projects. On this basis, a method based on multi-layer fuzzy comprehensive evaluation is proposed, and the effectiveness of the method is verified by an example:
- (1) Set of comments to determine the evaluation object
When determining the set of comments of the evaluation object, it can be divided into certain levels. Here, from the perspective of “expert scoring”, the evaluation level is divided into five levels of “10 points”, “8 points”, “6 points”, “4 points” and “2 points”, and when choosing partners for RCEIPPPP, the comments set is expressed as V={10 points, 8 points, 6 points, 4 points, 2 points} , The evaluation connotation of the review set is V={benchmark level, lean level, standard level, growth level, initial level} .
- (2) Determine the membership of each indicator u i to the r ij of the comment in V.
In this paper, the membership r ij was determined by the evaluation committee scoring method. If there are n people in the evaluation committee, then for a certain cooperative enterprise, the membership degree of an indicator in the index layer belonging to a comment in V is expressed as:
Since the 30 indicators in C are divided into seven categories according to the seven criteria of financial ability U 1 ,technical ability U 2 , management ability U 3 , performance experience U 4 , corporate reputation U 5 , cooperation ability U 6 and risk management U 7 , the evaluation matrix is constructed by taking the elements in each category as a whole. For example, in U 1 (financial capacity), “Rationality of PPP project financing plan”, “Asset situation of energy Internet enterprises”, “asset-liability ratio of energy Internet enterprises”, “Price competitiveness of rural PPP partners”, “solvency of rural PPP partners”, and “clean energy Internet fund use Plan” are the five levels in the comments set V . The above definition gives the 6 × 5 matrix R 1 , and the same is true for R 2 , R 3 , R 4 , R 5 , R 6 , R 7. The results are equations (1) , (2) , (3) .
- (3) Fuzzy analytic Hierarchy Process to determine the weight
In the comprehensive evaluation system given in Table 1 , the importance of the seven criteria and 30 indicators in the comprehensive evaluation is different. If the status is important, greater weight should be given; On the contrary, less weight should be given.
The basic principle of fuzzy analytic hierarchy process starts from the hierarchical structure of the comprehensive evaluation system, applies the knowledge, wisdom, information and values of experts to compare and contrast the indicators in the same layer or the same domain, and constructs the judgment matrix D=(d ij ) m×n according to the 1–9 judgment scale and meaning. Then, the organizer calculates the largest eigenroot λ max of the comparison judgment matrix D , and has λ max solve the eigenequation: D x =λ max x to obtain the eigenvector x=(x 1 , x 2 , …, x n ) T corresponding to λ max . Finally, the normalization process is equation (4) .
Before determining the final weight vector, the consistency test of the judgment matrix D is needed. The weight vectors determined by any of the methods are equations (5) , (6) , (7) , (8) .
- (4) Comprehensive evaluation of PPP project partners is equation (9) .
The normalization results are equation (10) .
The results show that C 1 % of the judges think that 10 points can be scored for the RCEIPPPP partner selection, C 2 % of people think that 8 points can be scored, C 3 % of people think that 6 points can be scored, C 4 % of people think that 4 points can be scored, C 5 % of people think that 2 points.
Further, 2 points, 4 points, 6 points, 8 points, 10 points as five grades. Let Y= (2,4,6,8,10) T , so the comprehensive evaluation of the PPP project partners is divided into: Z=BOY , and “O” takes the operator M(∙,+) to get equation (11) .
- (5) Description of fuzzy multi-attribute decision theory
Fuzzy multi-attribute decision theory is developed on the basis of classical multi-attribute decision theory, and it can be expressed as: Given A scheme set A={A 1 ,A 2 , …, A m } and the corresponding set of attributes (also known as the index set) C=(C 1 ,C 2 , …, C n ) for each scheme, and given the set of weights w=(w 1 ,w 2 , …, w n ) of relative importance for each attribute. The known attribute index, weight size and data structure are represented as fuzzy subsets or fuzzy numbers in the decision space, and the fuzzy index value matrix is obtained, denoted as F=(f ij ) m×n . Then the generalized fuzzy synthesis operator is used to transform the fuzzy weight vector w and the fuzzy index value matrix F , and the fuzzy decision matrix D: D=wⒽF is obtained. The elements in D are sorted by the fuzzy discount medium decision method, so as to select the optimal scheme in A i (i=1,2, …, m) .
5. Case analysis
This paper takes an Internet PPP project as an example, the bidding announcement is publicly released by the media after the approval of the relevant government departments, and finally the three consortia of A , B and C submit the plan in accordance with the requirements. This data was obtained by expert survey, which to a certain extent represents the opinions of authoritative institutions on the selection of private sector partners for infrastructure projects under the PPP model.
5.1. Gets the energy internet membership matrix
According to the RCEIPPPP partner selection system established in Table 1 , the three private enterprises A, B and C are scored through the method of expert scoring. The scoring rules are divided into five levels, which are “10 points”, “8 points”, “6 points”, “4 points” and “2 points”. The set of comments is expressed as V={10 points, 8 points, 6 points, 4 points, 2 points} , and the evaluation connotation is V={initial level, growth level, standard level, lean level, benchmarking level} . The membership matrix is constructed with enterprise A as the operation case in Table 2 .
Statistical table of expert scores in Enterprise A .
According to the proportion of experts for each indicator in Table 2 , where 1 is 0.11 experts, the fuzzy evaluation matrix is constructed.
R C11 =( 0.11, 0.11, 0.33, 0.22, 0.22 )
R C12 =( 0.00, 0.22, 0.11, 0.44, 0.22 )
R C13 =( 0.11, 0.11, 0.33, 0.22, 0.22 )
R C14 =( 0.22, 0.11, 0.33, 0.22, 0.11 )
R C15 =( 0.11, 0.11, 0.33, 0.33, 0.11 )
R C16 =( 0.00, 0.33, 0.33, 0.33, 0.00 )
Membership matrix of R C1 is composed of its corresponding matrices R C11 , R C12 , R C13 , R C14 , R C15 and R C16 . Similarly, membership matrix R C2 , membership matrix R C3 , membership matrix R C4 , membership matrix R C5 , membership matrix R C6 and membership matrix R C7 can be obtained, and fuzzy operations can be performed on them. The results are equation (12) .
5.2. AHP index weight determination
In this paper, the government partners of RCEIPPPP choose the weight of the evaluation index system, through the method of expert evaluation, with the help of the knowledge and experience of relevant experts, the pairwise comparison of various indicators of the system, the 1–9 scale method is used to score, multiple judgment matrices are constructed, and the weight of each system index is calculated through verification and adjustment. The judgment matrix is in Table 3 , Table 4 , Table 5 , Table 6 , Table 7 , Table 8 , Table 9 , Table 10 .
A-C judgment matrices, weights and consistency tests.
C 1 –C 1i judgment matrices, weights and consistency tests.
C 2 –C 2i judgment matrices, weights and consistency tests.
C 3 –C 3i judgment matrices, weights and consistency tests.
C 4 –C 4i judgment matrices, weights and consistency tests.
C 5 –C 5i judgment matrices, weights and consistency tests.
C 6 –C 6i Judgment matrices, weights and consistency tests.
C 7 –C 7i Judgment matrices, weights and consistency tests.
Note: The above tables are calculated according to AHP index.
Therefore, the weight vector of each indicator layer is shown as follows:
W A =(wc 1 ,wc 2 ,wc 3 ,wc 4 ,wc 5 ,wc 6 ,wc 7 )=( 0.3343, 0.2091, 0.1142, 0.1116, 0.0829, 0.0582, 0.0597 )
W C1 =( 0.106, 0.3058, 0.2598, 0.1716, 0.0944, 0.0624 )
W C2 =( 0.3331, 0.0733, 0.01589, 0.3072, 0.1276 )
W C3 =( 0.1899, 0.4203, 0.2685, 0.1276 )
W C4 =( 0.1297, 0.0917, 0.4829, 0.2957 )
W C5 =( 0.1671, 0.4531, 0.2616, 0.1182 )
W C6 =( 0.434, 0.098, 0.2856, 0.1825 )
W C7 =( 0.5499, 0.2098, 0.2402 )
5.3. Fuzzy comprehensive evaluation operation
Membership matrix R C1 is composed of its corresponding submatrices R C11 , R C12 , R C13 , R C14 , R C15 and R C16 , and the results are equation (13) .
Membership matrices R C2 , R C3 , R C4 , R C5 , R C6 and R C7 can be obtained in the same way. Fuzzy operation is performed on the membership matrix, and the results are shown as follows:
B C1 ]W C1 •R C1 =( 0.0893, 0.159, 0.2654, 0.3076, 0.1788 )
B C2 ]W C2 •R C2 =( 0.0388, 0.1317, 0.3035, 0.2227, 0.1603 )
B C3 ]W C3 •R C3 =( 0.1145, 0.1613, 0.3231, 0.28, 0.1275 )
B C4 ]W C4 •R C4 =( 0.1111, 0.1357, 0.2943, 0.3149, 0.144 )
B C5 ]W C5 •R C5 =( 0.1216, 0.1986, 0.283, 0.2911, 0.1057 )
B C6 ]W C6 •R C6 =( 0.0941, 0.1911, 0.1735, 0.3822, 0.1593 )
B C7 ]W C7 •R C7 =( 0.0878, 0.1577, 0.3333, 0.3066, 0.1145 )
R A is composed of its corresponding submatrix B C1 , B C2 , B C3 , B C4 , B C5 , B C6 and B C7 , which can be obtained by fuzzy calculation formula:
B A = W A • R A =(0.0910, 0.1594, 0.2950, 0.2996, 0.1599), the fuzzy matrix B A and the comments set V={2,4,6,8,10} by fuzzy calculation, we can get the comprehensive score of A project is 6.59, and the project level of A enterprise is “lean level”.
Expert scoring method can know the expert scoring situation of enterprise B and C projects, and the results are shown in Table 11 and Table 12 .
B enterprise experts score statistics table.
C enterprise experts score statistics table.
According to the above calculation process, it can be obtained that B B = W A • R A =(0.1026, 0.148, 0.3089, 0.2590, 0.1217) . By fuzzy calculation of fuzzy matrix B and comment set V={2,4,6,8,10} , the comprehensive score of B item can be obtained as 5.95. The project level of enterprise B is “standard level”; B C = W A • R A =(0.0787, 0.1919, 0.2701, 0.2957, 0.1045) , the fuzzy matrix B C and the comments set V={2,4,6,8,10} by fuzzy calculation, the comprehensive score of C project can be obtained as 5.96, and the project level of C enterprise is “lean level”.
Fig. 2 presents the fuzzy evaluation matrix of the three enterprises in the form of a line chart to visually compare the membership matrix of the three enterprises. B A = W A • R A =(0.0910, 0.1594, 0.2950, 0.2996, 0.1599 ). It can be seen from Fig. 2 that A has the largest probability value between 8 partitions (=0.2996), so it is finally defined as “lean level”. B B = W A • R A =(0.1091, 0.1592, 0.3211, 0.2817, 0.1300) , B has the largest probability value between 6 partitions (= 0.3211 ), so it is finally defined as “standard level”; B C = WA• RA=(0.0815, 0.1986, 0.2948, 0.3097, 0.1163) , C has the largest probability value between 8 partitions (= 0.3097 ), so it is finally defined as “lean level”.

Calculation of PPP project partner selection results.
According to the fuzzy evaluation matrix and the comments set V={2,4,6,8,10} , the final scores of A, B and C enterprises are 6.59 , 6.32 and 6.37 respectively. The grades of enterprise A and enterprise C are in 8 partitions (lean level), and enterprise A 's fuzzy calculation score of 6.59 is higher than that of enterprise C 's fuzzy calculation score of 6.37 .
According to the above calculation process, it can be obtained that B B = WA• RA=(0.1091, 0.1592, 0.3211, 0.2817, 0.1300) . By fuzzy calculation of fuzzy matrix B and comment set V={2,4,6,8,10} , the comprehensive score of item B can be obtained as 6.32 . The project level of enterprise B is “standard level”; B C = W A • R A =(0.0815, 0.1986, 0.2948, 0.3097, 0.1163) , the fuzzy matrix B C and the comments set V={2,4,6,8,10} by fuzzy calculation, the comprehensive score of C project can be obtained as 6.37 , and the project level of C enterprise is “lean level".
Based on the comparison of the above results, it can be seen that the comprehensive evaluation level of enterprise A and enterprise C is “lean level”, and the project score of enterprise A is higher than that of enterprise C . Therefore, according to the comprehensive evaluation results, the first company (A) should be selected to participate in the construction of RCEIPPPP.
5.4. Discussion
According to the actual situation, enterprise A won the bid in the B475# rural energy project, which is consistent with the analysis in this paper. Zhangzhuang Village is powered by 10 kV thunder 2 board, and the superior power supply is 35 kV thunder set substation. The existing public distribution of 3 units, a total capacity of 1000 kV A, electricity customers 470 households, households with 2 . 13 kV A capacity. The village has 18 rooftop photovoltaic households, with a total installed capacity of 340 kW, 9 orderly electric charging piles in the village committee square, 3 V2G charging piles in the village cadre training school, 7 households with mechanical Wells irrigation, and AC/DC flexible energy storage systems are built in the village.
The data show that with the advancement of the rural energy revolution, the low-carbon and green transformation in rural areas has achieved remarkable results: the proportion of non-fossil energy consumption in the county has increased from 22 % to 75 %, the proportion of electric energy in the terminal energy consumption has increased from 37.5 % to 64.5 % , and the electricity generation of renewable energy in the whole society has increased from 21 % to 94 % . At the same time, due to the advancement of the energy revolution, the harmless treatment rate of rural household waste and livestock and poultry feces has increased from 30 % to 100 % and 96 % , respectively. Through energy conservation and energy intelligence, the imbalance and inadequacy between urban and rural areas have been greatly improved. With the energy industry as the forerunner, Lankao has realized the leap-forward development of the economy and society of the whole county. The county's GDP and tax revenue grew at an average annual rate of 9.6 % and 36.2 % , respectively. The role of industry in promoting farmers' income is very significant: the per capita disposable income of urban residents has reached 29,900 yuan, an increase of 7.8 % , while the per capita disposable income of rural residents has reached 16,800 yuan, an increase of 10 % , providing more than 7000 jobs for the rural population, so that energy development and economic development are coordinated. Lankao, with its strong financial resources and strong financial resources, has effectively promoted the improvement of the economic and social development level of the county. The rural energy Internet platform with “one library and three centers” as the core is supported by the reform of rural energy production, consumption, technology, and system, so that rural energy transformation has embarked on the “fast track".
Reviewing the selection process of RCEIPPPP partners, this part uses actual cases, analyzes and calculates the data in the case through AHP and fuzzy comprehensive evaluation method, and concludes that among enterprises A, B and C , the comprehensive score of project A is 6.16, and the project grade of enterprise A is “lean”. The overall score of project B is 5.95, and the project grade of Enterprise B is “standard level”; The comprehensive score of project C is 5.96, and the project grade of enterprise C is “lean”. Therefore, enterprise A is finally selected to participate in the construction of the rural clean energy Internet project.
At the same time, there are limitations in this study, which only evaluates and makes decisions for three enterprises, and lacks many real life cases to verify the feasibility of the evaluation indicators. However, this case analysis is based on the application of scientific methods, and it is impossible to know the implementation results of the three bidding enterprises respectively. However, the construction of partner selection index in this study is the extension and modification of the existing research and the practical application of the evaluation system, which has certain scientific and practical.
The conclusions of this study are as follows: First, partners' financial ability, technical ability, management ability, performance experience, corporate reputation, cooperation ability and risk management are influencing factors for the selection of government partners for RCEIPPPPs. Compared with Ramli et al. [30], which focused on financial capability, six multi-dimensional evaluation indicators, including technical capability, management capability and performance experience, were added to implement a more rigorous and comprehensive evaluation of PPP projects. Second, compared with other factors, financial capability, technical capability, management capability and performance experience are the four key factors that are more important in selecting partners. Compared with Li [52], the direct impact of force input, technical capability, management capability and safety and environmental protection on the performance of PPP projects can be seen. The specific indicators of strength input and safety and environmental protection can be included in the indicators of performance experience and corporate reputation in this paper. On this basis, financial capability is added as a key factor in this paper. Thirdly, the empirical research shows that AHP and fuzzy comprehensive evaluation method as well as the index system constructed by this research can be applied to the practice of government partner selection for RCEIPPPPs. Compared with Zhang et al. [51], this paper is more rigorous and scientific in selecting partner selection tendency through cooperation motivation coding.
6. Conclusions and future prospect
6.1. conclusions.
This paper summarizes the dimensions and evaluation indicators of PPP partner selection for rural clean energy Internet, and uses analytic hierarchy process to empower each evaluation dimension and indicator and combines the fuzzy evaluation matrix to comprehensively evaluate the research results of the three enterprises as follows:
- (1) From the perspective of PPP project operation process and the role of rural clean energy Internet partners, the selection and evaluation of partners are divided into seven dimensions: financial capability, technical capability, management capability, performance experience, corporate reputation, cooperation capability and risk management.
- (2) In the evaluation of the selection of private partners for PPP infrastructure projects, the financial capacity, technical capacity and management capacity of private enterprises occupy a large proportion. In addition, due to the large weight of performance experience, in the selection of partners, in addition to the rationality of construction plans and the level of construction control concerned by traditional projects, we should also pay attention to performance experience.
- (3) The comprehensive evaluation level of enterprise A and Enterprise C is “lean level”, and the project score of enterprise A is higher than that of enterprise C. Therefore, according to the comprehensive evaluation results, the first company ( A ) should be selected to participate in the construction of RCEIPPPP.
6.2. Enlightenment
The research of this paper has theoretical significance and management significance. (1) This paper constructs a partner selection system for RCEIPPPPs. This study shows that the multi-objective fuzzy comprehensive evaluation method is a powerful tool for dealing with complex partner selection problems. It can effectively take into account multiple objectives and uncertainties, providing strong support for decision-making. (2) The selection process of RCEIPPPPs is affected by many factors, including technology, economy, system and other aspects, which indicates the complexity of clean energy projects and the need for a comprehensive decision-making method. This study highlights the importance of partner selection over the life cycle of a project, and that clean energy Internet projects may need to constantly adapt and reevaluate their partners to adapt to changing market and technological conditions. (4) Managers may consider adopting multi-objective fuzzy comprehensive evaluation as a decision support tool to help them weigh different objectives and constraints in partner selection. (5) The project management team shall implement ongoing monitoring and evaluation measures to ensure that the selected partners are able to meet the project needs and objectives at different stages of the project. (6) Building a healthy partnership is essential, and project managers need to actively manage communication and cooperation with partners to ensure the successful implementation of the project.
Because the construction of the rural clean energy Internet usually lasts a long time, and needs to be oriented to the development of relatively backward rural groups, if the selected private partners occur the capital chain break, backward technology, loose management, then the progress and quality of the project can not be guaranteed [ 53 ]. Therefore, in the high-quality development of Chinese economy today, have strong financial strength, excellent technology and strong project organization and management ability, all these are the keys of whether the project can succeed or not. According to the regional characteristics of different rural areas, the government should conceive the implementation policy of clean energy Internet PPP projects, and then build a set of characteristic partner selection evaluation index system, and continue to improve it.
The index system and evaluation model of public sector partner selection under the PPP model constructed in this paper have a certain guiding role for government departments to select public sector partners. In terms of partner selection for RCEIPPPPs, the results of this study provide valuable insights for decision makers [ 54 ]. The multi-objective fuzzy comprehensive evaluation method proves to be a useful tool and can be applied to similar project selection. In addition, the project management team should recognize that project partner selection is a dynamic process that requires ongoing attention and management. Ultimately, successful project implementation depends not only on the selection of partners, but also on the establishment of good partnerships and the appropriate management measures to cope with the changing environment. These findings provide practical guidelines for the successful implementation of RCEIPPPPs.
6.3. Future outlook
There are certain limitations in this paper. Only three bidding enterprises of B475# rural energy engineering project are taken as realistic cases for analysis, evaluation and decision accuracy verification, and there is a lack of more relevant energy Internet engineering project cases for feasibility analysis and accuracy test of the evaluation system. With the continuous promotion of rural clean energy Internet projects in the future, The author will carry out a large number of continuous verification and improvement of the constructed evaluation system through a large number of practical cases. However, the construction of partner selection index in this study is the extension and modification of the existing research and the practical application of the evaluation system, which has certain scientific and practical. This paper designs a multi-dimensional evaluation index system for the selection of RCEIPPPP partners, provides a scientific and effective selection and decision-making method, and hopes to provide some ideas and methodological guidance for rural clean energy Internet and rural infrastructure construction [ [55] , [56] ].
Ethics statement
All procedures performed in this study were in accordance with the ethical standards of the university. Ethical clearance and approval were granted by Hebei Agricultural University (jgy20230505). All participants provided informed consent before joining the study.
This research was funded by The National Social Science Fund of China grant number [22CJY043].
Data availability statement
Credit authorship contribution statement.
Renjie Li: Writing – review & editing, Software, Resources, Methodology. Mingxuan Zhang: Writing – review & editing, Resources, Project administration. Shi Yin: Writing – review & editing, Writing – original draft, Methodology. Nan Zhang: Writing – review & editing, Formal analysis, Data curation. Tahir Mahmood: Writing – review & editing, Methodology, Funding acquisition.
Declaration of competing interest
The authors declare that they have no known competing financial interests or personal relationships that could have appeared to influence the work reported in this paper.
Potential and health impact assessment of air pollutant emission reduction: a case study of China
- Original Paper
- Published: 23 May 2024
Cite this article
- Yuanying Chi 1 ,
- Yangyi Zhang 1 ,
- Xufeng Zhang 1 &
- Baoliu Liu 1 , 2
Explore all metrics
Air pollution has become the world’s largest human health and environmental risk factor. This study used the greenhouse gas and air pollution interactions and synergies model to analyze the emissions, emission reduction potential, and health impacts of atmospheric pollutants (SO 2 , NO x , and PM2.5) in 31 regions of China (excluding Hong Kong, Macau, and Taiwan). Meanwhile, the spatial aggregation of atmospheric pollutants was studied by combining the spatial autocorrelation Moran index. Research results indicate that under the background of implementing pollution control policies and advancing emission reduction technologies, the emissions of SO 2 and NO x are expected to significantly decrease by 2030, demonstrating significant potential for emission reduction. Moreover, some regions in East China, such as Zhejiang, Shandong, and Jiangsu, with significant emission reduction and potential, exhibit relatively high GDP and power generation. This finding also includes regions with abundant and intensive energy reserves such as Shanxi. In addition, significant agglomeration effects and spatial regional differences in atmospheric pollutant emissions are observed. The results of spatial autocorrelation analysis show that by 2030, the spatial clustering level of SO 2 emissions will be strengthened, whereas that of NO x emissions will be reduced. Furthermore, in terms of health impacts, a significant reduction in PM2.5 emissions and attributable deaths is forecasted by 2030. Among them, Guangdong Province has the highest reduction in PM2.5 attributable deaths, reaching 48,332 people, whereas Hainan Province has the highest reduction rate in PM2.5 attributable deaths, reaching 92%. Therefore, we should formulate reasonable emission reduction measures to promote high-quality economic and social development in the region.
Graphical abstract
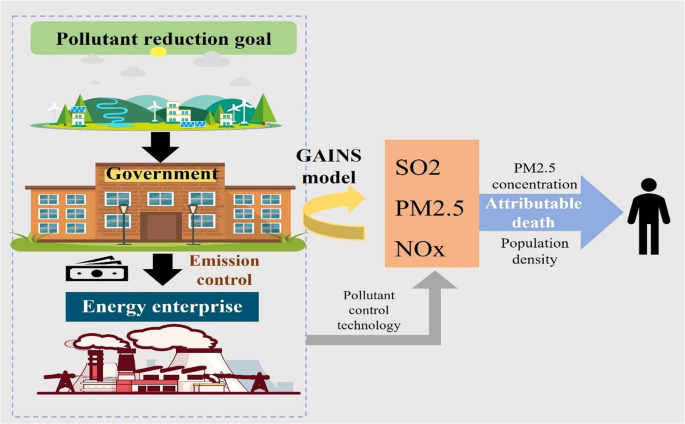
This is a preview of subscription content, log in via an institution to check access.
Access this article
Price includes VAT (Russian Federation)
Instant access to the full article PDF.
Rent this article via DeepDyve
Institutional subscriptions
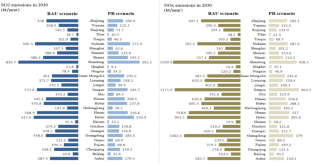
Similar content being viewed by others
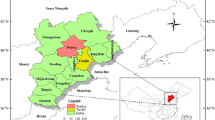
Socioeconomic driving factors of PM2.5 emission in Jing-Jin-Ji region, China: a generalized Divisia index approach
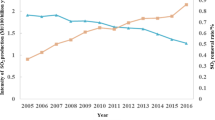
Spatial effects of air pollutants reduction on CO2 emissions
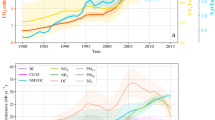
An integrated view of correlated emissions of greenhouse gases and air pollutants in China
Data availability.
No datasets were generated or analysed during the current study.
An D, Xing X, Tang Z, Li Y, Sun J (2022) Concentrations, distribution and potential health risks of organic ultraviolet absorbents in street dust from Tianjin, a megacity in northern China. Environ Res 204:112130. https://doi.org/10.1016/j.envres.2021.112130
Article CAS Google Scholar
Bone C, Wulder MA, White JC, Robertson C, Nelson TA (2013) A GIS-based risk rating of forest insect outbreaks using aerial overview surveys and the local Moran’s I statistic. Appl Geogr 40:161–170. https://doi.org/10.1016/j.apgeog.2013.02.011
Article Google Scholar
Buntaine M, Greenstone M, He G, Liu M, Wang S, Zhang B (2022) Does the squeaky wheel get more grease? The direct and indirect effects of citizen participation on environmental governance in China (No. w30539). Natl Bur Econ Res. https://doi.org/10.3386/w3053
Burnett RT, Pope CA III, Ezzati M, Olives C, Lim SS, Mehta S, Shin HH, Singh G, Hubbell B, Brauer M, Ross Anderson H, Smith KR, Balmes JR, Bruce NG, Kan H, Laden F, Prüss-Ustün A, Turner MC, Gapstur SM, Diver WR, Cohen A (2014) An integrated risk function for estimating the global burden of disease attributable to ambient fine particulate matter exposure. Environ Health Perspect 122(4):397–403. https://doi.org/10.1289/ehp.1307049
Carrijo TB, da Silva AR (2017) Modified Moran’s I for small samples. Geogr Anal 49(4):451–467. https://doi.org/10.1111/gean.12130
Deng QS, Cuesta L, Alvarado R, Murshed M, Tillaguango B, Işık C, Rehman A (2023) Nexus between government stability and environmental pollution. J Clean Prod 434:140061. https://doi.org/10.1016/j.jclepro.2023.140061
Ding J, Liu B, Shao X (2022) Spatial effects of industrial synergistic agglomeration and regional green development efficiency: evidence from China. Energy Econ. 112:106156. https://doi.org/10.1016/j.eneco.2022.106156
Dong H, Dai H, Dong L, Fujita T, Geng Y, Klimont Z et al (2015) Pursuing air pollutant co-benefits of CO2 mitigation in China: a provincial leveled analysis. Appl Energy 144:165–174. https://doi.org/10.1016/j.apenergy.2015.02.020
Ezzati M, Lopez AD, Rodgers A, Vander Hoorn S, Murray CJ (2002) Selected major risk factors and global and regional burden of disease. The Lancet 360(9343):1347–1360. https://doi.org/10.1016/S0140-6736(02)11403-6
Guo K, Cao Y, He S, Li Z (2024) Evaluating the efficiency of green economic production and environmental pollution control in China. Environ Impact Assess Rev 104:107294. https://doi.org/10.1016/j.eiar.2023.107294
Lee CC, Wang CW, Hong PH, Lin W (2023) Environmental policy stringency and bank risks: does green economy matter? Int Rev Finan Anal 91:103040. https://doi.org/10.1016/j.irfa.2023.103040
Li Z, Yim SHL, He X, Xia X, Ho KF, Yu JZ (2024) High spatial resolution estimates of major PM2.5 components and their associated health risks in Hong Kong using a coupled land use regression and health risk assessment approach. Sci Total Environ 907:167932. https://doi.org/10.1016/j.scitotenv.2023.167932
Liao L, Du M, Chen Z (2023) Environmental pollution and socioeconomic health inequality: evidence from China. Sustain Cities Soc 95:104579. https://doi.org/10.1016/j.scs.2023.104579
Lin ZW, Wang P, Ren SY et al (2022) Assessment of economic, environmental and health benefits of the energy transition path—take the Guangdong-Hong Kong-Macao greater Bay area as an example. Clim Change Res 06:777–790
Google Scholar
Liu L, Wang K, Wang S, Zhang R, Tang X (2018) Assessing energy consumption, CO2 and pollutant emissions and health benefits from China’s transport sector through 2050. Energy Policy 116:382–396. https://doi.org/10.1016/j.enpol.2018.02.019
Liu B, Cifuentes-Faura J, Ding CJ, Liu X (2023a) Toward carbon neutrality: how will environmental regulatory policies affect corporate green innovation? Econ Anal Policy 80:1006–1020. https://doi.org/10.1016/j.eap.2023.09.019
Liu B, Ding CJ, Hu J, Su Y, Qin C (2023b) Carbon trading and regional carbon productivity. J Clean Prod 420:138395. https://doi.org/10.1016/j.jclepro.2023.138395
Lu J, Zhou Q, Qi W, Qu S, Bi J (2023) The heterogeneous effects of Chinese industrial parks on environmental pollution. Sci Total Environ 896:165279. https://doi.org/10.1016/j.scitotenv.2023.165279
Moutinho V, Madaleno M, Macedo P (2020) The effect of urban air pollutants in Germany: eco-efficiency analysis through fractional regression models applied after DEA and SFA efficiency predictions. Sustain Cities Soc 59:102204. https://doi.org/10.1016/j.scs.2020.102204
Pan LJ, Xie YB, Li W (2013) An analysis of emission reduction of chief air pollutants and greenhouse gases in Beijing based on the LEAP model. Proc Environ Sci 18:347–352. https://doi.org/10.1016/j.proenv.2013.04.045
Pu Y, Song J, Dong L, Yang W, Wang S (2019) Estimating mitigation potential and cost for air pollutants of China’s thermal power generation: a GAINS-China model-based spatial analysis. J Clean Prod 211:749–764. https://doi.org/10.1016/j.jclepro.2018.11.213
Ren FR, Cui Z, Ding X, Zhang XR, Li RH, Yao Q, Liu XY (2023) The co-benefit of emission reduction efficiency of energy, CO2and atmospheric pollutants in China under the carbon neutrality target. Energ Strat Rev 49:101125. https://doi.org/10.1016/j.esr.2023.101125
Shi X, Lei Y, Xue W, Liu X, Li S, Xu Y et al (2023) Drivers in carbon dioxide, air pollutants emissions and health benefits of China’s clean vehicle fleet 2019–2035. J Clean Prod 391:136167. https://doi.org/10.1016/j.jclepro.2023.136167
Vinayagam S, Sathishkumar K, Ayyamperumal R, Natarajan PM, Ahmad I, Saeed M et al (2023) Distribution and transport of contaminants in soil through mining processes and its environmental impact and health hazard assessment: a review of the prospective solutions. Environ Res. https://doi.org/10.1016/j.envres.2023.117473
Wan YF, Cui YY, Wu XF et al (2022) Characteristics of carbon dioxide emission in Beijing-Tianjin-Hebei region and its synergistic reduction potential with air pollutants. J Capital Norm Univ (nat Sci Ed) 43:46–52. https://doi.org/10.19789/j.1004-9398.2022.04.008
Yang L, Hong S (2023) Impact of the clean energy structure of building operation on the co-benefits of CO2 and air pollutant emission reductions in Chinese provinces. J Clean Prod 413:137400. https://doi.org/10.1016/j.jclepro.2023.137400
Yang J, Wang Y, Tang C, Zhang Z (2024) Can digitalization reduce industrial pollution? Roles of environmental investment and green innovation. Environ Res 240:117442. https://doi.org/10.1016/j.envres.2023.117442
Yong L, Qin L, Xiuge Z et al (2020) PM(2.5) pollution health burden of our country and the impact of economic loss. J Environ Sci (04):1688–1695
Yu Y, Li K, Duan S, Song C (2023) Economic growth and environmental pollution in China: new evidence from government work reports. Energy Econ 124:106803. https://doi.org/10.1016/j.eneco.2023.106803
Yuan Y, Cave M, Zhang C (2018) Using Local Moran’s I to identify contamination hotspots of rare earth elements in urban soils of London. Appl Geochem 88:167–178. https://doi.org/10.1016/j.apgeochem.2017.07.011
Yue T, Liu Q, Tong Y, Gao J, Zheng Y, Li G, Li R (2023) Analysis of emission evolution and synergistic reduction effect of air pollutants and CO2 from Chinese coal-fired power plants. Atmos Pollut Res 15:102001. https://doi.org/10.1016/j.apr.2023.102001
Zhao K, Zhang Y, Shang J, Schauer JJ, Huang W, Tian J et al (2023a) Impact of Beijing’s “Coal to Electricity” program on ambient PM2.5 and the associated reactive oxygen species (ROS). J Environ Sci 133:93–106. https://doi.org/10.1016/j.jes.2022.06.038
Zhao X, Zhang Z, Xu J, Gao J, Cheng S, Zhao X et al (2023b) Impacts of aerosol direct effects on PM2.5 and O3 respond to the reductions of different primary emissions in Beijing-Tianjin-Hebei and surrounding area. Atmos Environ 309:119948. https://doi.org/10.1016/j.atmosenv.2023.119948
Zheng J, Jiang P, Qiao W, Zhu Y, Kennedy E (2016) Analysis of air pollution reduction and climate change mitigation in the industry sector of Yangtze River Delta in China. J Clean Prod 114:314–322. https://doi.org/10.1016/j.jclepro.2015.07.011
Download references
This is a part research accomplishment of the National Natural Science Foundation of China Key Project “Research on the Construction of China’s Economic Transformation Model for Carbon Neutrality” (72140001).
Author information
Authors and affiliations.
School of Economics and Management, Beijing University of Technology, Beijing, 100124, China
Yuanying Chi, Yangyi Zhang, Xufeng Zhang & Baoliu Liu
Institute of Eco-Civilization Studies, Beijing University of Technology, Beijing, 100124, China
You can also search for this author in PubMed Google Scholar
Contributions
Yuanying Chi and Yangyi Zhang wrote the main manuscript text and Xufeng Zhang, Baoliu Liu prepared receipt revisions. All authors reviewed the manuscript.
Corresponding author
Correspondence to Baoliu Liu .
Ethics declarations
Conflict of interest.
The authors declare that they have no conflict of interest.
Additional information
Publisher's note.
Springer Nature remains neutral with regard to jurisdictional claims in published maps and institutional affiliations.
Rights and permissions
Springer Nature or its licensor (e.g. a society or other partner) holds exclusive rights to this article under a publishing agreement with the author(s) or other rightsholder(s); author self-archiving of the accepted manuscript version of this article is solely governed by the terms of such publishing agreement and applicable law.
Reprints and permissions
About this article
Chi, Y., Zhang, Y., Zhang, X. et al. Potential and health impact assessment of air pollutant emission reduction: a case study of China. Clean Techn Environ Policy (2024). https://doi.org/10.1007/s10098-024-02880-5
Download citation
Received : 09 February 2024
Accepted : 06 May 2024
Published : 23 May 2024
DOI : https://doi.org/10.1007/s10098-024-02880-5
Share this article
Anyone you share the following link with will be able to read this content:
Sorry, a shareable link is not currently available for this article.
Provided by the Springer Nature SharedIt content-sharing initiative
- Emission reduction potential
- Health impact
- Spatial autocorrelation
- GAINS model
- Find a journal
- Publish with us
- Track your research
In the tech world and beyond, new 5G applications are being discovered every day. From driverless cars to smarter cities, farms, and even shopping experiences, the latest standard in wireless networks is poised to transform the way we interact with information, devices and each other. What better time to take a closer look at how humans are putting 5G to use to transform their world.
What is 5G?
5G (fifth-generation mobile technology is the newest standard for cellular networks. Like its predecessors, 3G, 4G and 4G LTE, 5G technology uses radio waves for data transmission. However, due to significant improvements in latency, throughput and bandwidth, 5G is capable of faster download and upload speeds than previous networks.
Since its release in 2019, 5G broadband technology has been hailed as a breakthrough technology with significant implications for both consumers and businesses. Primarily, this is due to its ability to handle large volumes of data that is generated by complex devices that use its networks.
As mobile technology has expanded over the years, the number of data users generate every day has increased exponentially. Currently, other transformational technologies like artificial intelligence (AI), the Internet of Things (IoT ) and machine learning (ML) require faster speeds to function than 3G and 4G networks offer. Enter 5G, with its lightning-fast data transfer capabilities that allow newer technologies to function in the way they were designed to.
Here are some of the biggest differences between 5G and previous wireless networks.
- Physical footprint : The transmitters that are used in 5G technology are smaller than in predecessors’ networks, allowing for discrete placement in out-of-the-way places. Furthermore, “cells”—geographical areas that all wireless networks require for connectivity—in 5G networks are smaller and require less power to run than in previous generations.
- Error rates : 5G’s adaptive Modulation and Coding Scheme (MCS), a schematic that wifi devices use to transmit data, is more powerful than ones in 3G and 4G networks. This makes 5G’s Block Error Rate (BER)—a metric of error frequency—much lower.
- Bandwidth : By using a broader spectrum of radio frequencies than previous wireless networks, 5G networks can transmit on a wider range of bandwidths. This increases the number of devices that they can support at any given time.
- Lower latency : 5G’s low latency , a measurement of the time it takes data to travel from one location to another, is a significant upgrade over previous generations. This means that routine activities like downloading a file or working in the cloud is going to be faster with a 5G connection than a connection on a different network.
Like all wireless networks, 5G networks are separated into geographical areas that are known as cells. Within each cell, wireless devices—such as smartphones, PCs, and IoT devices—connect to the internet via radio waves that are transmitted between an antenna and a base station. The technology that underpins 5G is essentially the same as in 3G and 4G networks. But due to its lower latency, 5G networks are capable of delivering faster download speeds—in some cases as high as 10 gigabits per second (Gbps).
As more and more devices are built for 5G speeds, demand for 5G connectivity is growing. Today, many popular Internet Service Providers (ISPs), such as Verizon, Google and AT&T, offer 5G networks to homes and businesses. According to Statista, more than 200 million homes and businesses have already purchased it with that number expected to at least double by 2028 (link resides outside ibm.com).
Let’s take a look at three areas of technological improvement that have made 5G so unique.
New telecom specifications
The 5G NR (New Radio) standard for cellular networks defines a new radio access technology (RAT) specification for all 5G mobile networks. The 5G rollout began in 2018 with a global initiative known as the 3rd Generation Partnership Project (3FPP). The initiative defined a new set of standards to steer the design of devices and applications for use on 5G networks.
The initiative was a success, and 5G networks grew swiftly in the ensuing years. Today, 45% of networks worldwide are 5G compatible, with that number forecasted to rise to 85% by the end of the decade according to a recent report by Ericsson (link resides outside ibm.com).
Independent virtual networks (network slicing)
On 5G networks, network operators can offer multiple independent virtual networks (in addition to public ones) on the same infrastructure. Unlike previous wireless networks, this new capability allows users to do more things remotely with greater security than ever before. For example, on a 5G network, enterprises can create use cases or business models and assign them their own independent virtual network. This dramatically improves the user experience for their employees by adding greater customizability and security.
Private networks
In addition to network slicing, creating a 5G private network can also enhance personalization and security features over those available on previous generations of wireless networks. Global businesses seeking more control and mobility for their employees increasingly turn to private 5G network architectures rather than public networks they’ve used in the past.
Now that we better understand how 5G technology works, let’s take a closer look at some of the exciting applications it’s enabling.
Autonomous vehicles
From taxi cabs to drones and beyond, 5G technology underpins most of the next-generation capabilities in autonomous vehicles. Until the 5G cellular standard came along, fully autonomous vehicles were a bit of a pipe dream due to the data transmission limitations of 3G and 4G technology. Now, 5G’s lightning-fast connection speeds have made transport systems for cars, trains and more, faster than previous generations, transforming the way systems and devices connect, communicate and collaborate.
Smart factories
5G, along with AI and ML, is poised to help factories become not only smarter but more automated, efficient, and resilient. Today, many mundane but necessary tasks that are associated with equipment repair and optimization are being turned over to machines thanks to 5G connectivity paired with AI and ML capabilities. This is one area where 5G is expected to be highly disruptive, impacting everything from fuel economy to the design of equipment lifecycles and how goods arrive at our homes.
For example, on a busy factory floor, drones and cameras that are connected to smart devices that use the IoT can help locate and transport something more efficiently than in the past and prevent theft. Not only is this better for the environment and consumers, but it also frees up employees to dedicate their time and energy to tasks that are more suited to their skill sets.
Smart cities
The idea of a hyper-connected urban environment that uses 5G network speeds to spur innovation in areas like law enforcement, waste disposal and disaster mitigation is fast becoming a reality. Some cities already use 5G-enabled sensors to track traffic patterns in real time and adjust signals, helping guide the flow of traffic, minimize congestion, and improve air quality.
In another example, 5G power grids monitor supply and demand across heavily populated areas and deploy AI and ML applications to “learn” what times energy is in high or low demand. This process has been shown to significantly impact energy conservation and waste, potentially reducing carbon emissions and helping cities reach sustainability goals.
Smart healthcare
Hospitals, doctors, and the healthcare industry as a whole already benefit from the speed and reliability of 5G networks every day. One example is the area of remote surgery that uses robotics and a high-definition live stream that is connected to the internet via a 5G network. Another is the field of mobile health, where 5G gives medical workers in the field quick access to patient data and medical history. This enables them to make smarter decisions, faster, and potentially save lives.
Lastly, as we saw during the pandemic, contact tracing and the mapping of outbreaks are critical to keeping populations safe. 5G’s ability to deliver of volumes of data swiftly and securely allows experts to make more informed decisions that have ramifications for everyone.
5G paired with new technological capabilities won’t just result in the automation of employee tasks, it will dramatically improve them and the overall employee experience . Take virtual reality (VR) and augmented reality (AR), for example. VR (digital environments that shut out the real world) and AR (digital content that augments the real world) are already used by stockroom employees, transportation drivers and many others. These employees rely on wearables that are connected to a 5G network capable of high-speed data transfer rates that improve several key capabilities, including the following:
- Live views : 5G connectivity provides live, real-time views of equipment, events, and even people. One way in which this feature is being used in professional sports is to allow broadcasters to remotely call a sporting event from outside the stadium where the event is taking place.
- Digital overlays : IoT applications in a warehouse or industrial setting allow workers that are equipped with smart glasses (or even just a smartphone) to obtain real-time insights from an application. This includes repair instructions or the name and location of a spare part.
- Drone inspections : Right now, one of the leading causes of employee injury is inspection of equipment or project sites in remote and potentially dangerous areas. Drones, which are connected via 5G networks, can safely monitor equipment and project sites and even take readings from hard-to-reach gauges.
Edge computing , a computing framework that allows computations to be done closer to data sources, is fast becoming the standard for enterprises. According to this Gartner white paper (link resides outside ibm.com), by 2025, 75% of enterprise data will be processed at the edge (compared to only 10% today). This shift saves businesses time and money and enables better control over large volumes of data. It would be impossible without the new speed standards that are generated by 5G technology.
Ultra-reliable edge computing and 5G enable the enterprise to achieve faster transmission speeds, increased control and greater security over massive volumes of data. Together, these twin technologies will help reduce latency while increasing speed, reliability and bandwidth, resulting in faster, more comprehensive data analysis and insights for businesses everywhere.
5G solutions with IBM Cloud Satellite
5G presents significant opportunities for the enterprise, but first, you need a platform that can handle its speed. IBM Cloud Satellite® lets you deploy and run apps consistently across on-premises, edge computing and public cloud environments on a 5G network. And it’s all enabled by secure and auditable communications within the IBM Cloud®.
Get the latest tech insights and expert thought leadership in your inbox.
Get our newsletters and topic updates that deliver the latest thought leadership and insights on emerging trends.
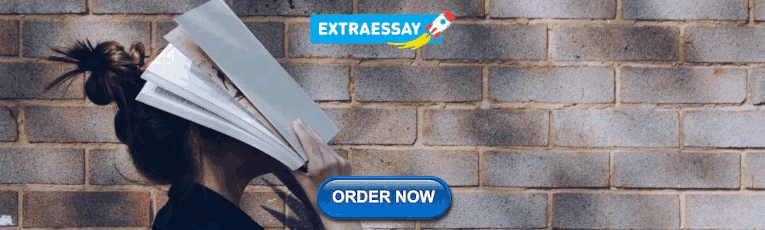
IMAGES
COMMENTS
Boosting renewable energy growth through responsible value chains: A case study from China. May 13, 2024. Over half of the carbon emissions in the renewable value chain comes from upstream activities. Image: Unsplash/Nuno Marques. Vee Li. Energy Sector Lead, China Climate Action,World Economic Forum. Zhang Xun.
A case study in solar energy: Nowhere is China's leading position clearer than in the solar energy sector. China has an estimated 2.7 million people employed in the solar energy sector, making up more than half of the world's 4.3 million solar jobs. How did China do it? Initially China's solar industry began in the 1990s to supply Germany ...
In this work, we combine MCA with the China-TIMES model, which has been extensively used in energy planning and climate change mitigation studies 17,18,19,20,21,22,23,24,25,26,27,28,29, to develop ...
The resilience performance of China's energy system varied according to different disruptive events. (3) Without carbon reduction measures, China's energy technical resilience is projected to decline by 2.21% between 2020 and 2060. In contrast, adopting the carbon neutrality target could lead to a significant improvement of 7.73%.
Based on this, a VAR model was constructed to study the interrelations between China's DT and energy sustainability. This study had the following conclusions: first, China's DT has developed rapidly since 2002. Second, the rapid development of China's DT has significantly advanced sustainable energy development.
In a 2019 study by the International Energy Agency of China's energy system, analysts recommended prioritizing flexibility, demand response, and trading electricity between provinces over requiring grid-scale energy storage. ... "Creating Markets for Wind Electricity in China: Case Studies in Energy Policy" (PhD Dissertation ...
4 Acknowledgements This study was prepared by a core team led by Smita Kuriakose (Senior Economist and Task Team Leader, Trade and Competitiveness GP) and comprising Joanna Lewis (Professor, Georgetown
The transition pathway of energy supply systems towards carbon neutrality based on a multi-regional energy infrastructure planning approach: A case study of China. Energy 2022, 238, 122037. [Google Scholar] National Bureau of Statistic. China Energy Statistical Yearbook; China Statistics Press: Beijing, China, 2023; Volume 2022.
The results showed that China's digital technology level has improved significantly since 2002. This has effectively promoted sustainable energy development, as reflected in the growth of the output of the energy industry, drop in energy supply losses, reduced energy consumption, and decrease in energy-related environmental pollution.
This case study documents the Capacity Building for the Rapid Commercialization of Renewable Energy project (CRE) in China. The CRE has promoted widespread adoption of renewable energy technologies by strengthening the capacities of public and private organizations, facilitating and supporting new policy initiatives, developing a regulatory system, and providing technical and co-financing ...
Subsequently, the panel data model was used to quantitatively study the influence factors of the integrated development of China's digital and energy industries. The study revealed that the fusion ...
The China Energy Outlook (CEO) provides a detailed review of China's energy use and trends. China is the world's largest consumer and producer of primary energy as well as the world's largest emitter of energy-related carbon dioxide (CO 2).China surpassed the U.S. in primary energy consumption in 2010 and in CO 2 emissions in 2006. In 2018, China was responsible for 21% of total global ...
line. Similarly, many companies in China and elsewhere lack an integrated view of how energy yields, energy output, and energy consumption combine to affect their operations. Some measure these factors only in a superficial way. Seizing China's energy-efficiency opportunity: A case study Improving energy efficiency in industrial
The case study of PR China-CEEC RE cooperation was conducted to test if the alignment of the EU's and PR China's energy- and climate-related policies was reflected on a practical level. This region, comprising 14 countries, was chosen as a case study for two reasons.
China is the world's largest energy consumer and CO2 emitter, facing a great challenge of CO2 emission mitigation. In order to identify driving factors for prefecture-level carbon emissions in western China, a case study of Haixi was performed. Time series analysis was undertaken in this study to provide a holistic picture of the historical trend of energy-related CO2 emissions. The ...
DOI: 10.23977/erej.2023.070701 Corpus ID: 265447790; Opportunities and Challenges from Energy Transition: Based on Carbon Neutrality Strategy Case Study in China @article{Long2023OpportunitiesAC, title={Opportunities and Challenges from Energy Transition: Based on Carbon Neutrality Strategy Case Study in China}, author={Junxian Long and Wenyue Chen and Zhike Zhang}, journal={Environment ...
The construction of new-type power system is the basis for achieving China's dual-carbon goal, and integrated energy service is an important way to ensure the sustainable operation of the new-type power system. At present, the development of integrated energy service in China is still in its infancy, and the primary issue has been how to tap the market's potential. However, most of the ...
China is prioritising energy security over the need to reduce coal-use. It has positioned thermal power as the backup energy source to ensure energy security, as electricity demand continues to grow and boost the economy. ... We have run a case study on a 1.5 gigawatt (GW) solar project being built in the Tengger Desert in Ningxia province. The ...
To analyze the factors driving and impeding sustainability, this article provides an overview of China's energy sector and policies. Through case studies of the Energy Corporation and other prominent renewable energy companies, the study showcases a range of demonstration projects, financing models, and management technologies that have the ...
Case Study Beijing Capital International Airport Co.,Ltd. 1 Beijing Capital International ... Energy Review and Planning Case Study Snapshot Industry Airport Product/Service Aviation business; Non-aviation business Location Beijing, China Energy Management System GB/T 23331 (ISO 50001) Energy Performance Improvement Period 2014-2016
Thus, it is crucial to study China's energy consumption pathways effectively and efficiently, for reasonable reference significance on energy consumption improvement and transition. ... Embodied energy in export flows along Global value chain: a case study of China's export trade. Front Energy Res, 9 (2021), Article 649163. View in Scopus ...
Reporting from Beijing. For decades, China has moved methodically to dominate ever more industries, from toys and clothing in the 1980s to semiconductors and renewable energy today. China now ...
Nuclear energy - 0.8%. Renwables - 1.2%. - Construction of clean coal plants. - A small experimental facility near Beijing to remove carbon dioxide from power station emissions and the use to provide carbonation for beverages. Coal - 68.4%. Oil - 17.6%. Hydro-electricity - 7.1%.
Study with Quizlet and memorize flashcards containing terms like what was China's main source of energy in 2012 ?, why did China stop exporting and start importing ?, what did China do to maintain energy security ? and more. ... IGCSE Geography Case Study - 3.1 - Water Supply In A Country Or Area. 15 terms. vdavya. Preview. Geography GCSE: The ...
To obtain the influence of anisotropy and energy evolution characteristics on wellbore stability, the acoustic and mechanical anisotropy characteristics of shales are studied through various experiments, including scanning electron microscopy, ultrasonic pulse transmission, and uniaxial compression experiments, with the Longmaxi Formation shale in the southern area of the Sichuan Basin as the ...
The construction of rural clean energy Internet is an important direction of China's energy development at present, and it is also an inevitable choice to realize the strategy of rural revitalization. ... Song et al. [ ] expanded the pricing method of asset securitization in PPP mode of new energy power, and conducted a case study using binary ...
In China, rural energy refers to energy used for both production and life in rural areas, and belongs to the category of energy construction and industry management (Yang, 1988), which is an essential component of China's Energy Strategy System and the equalization of basic public services in rural and urban areas (Tian, 2014).As defined by the National Energy Administration, rural energy ...
Air pollution has become the world's largest human health and environmental risk factor. This study used the greenhouse gas and air pollution interactions and synergies model to analyze the emissions, emission reduction potential, and health impacts of atmospheric pollutants (SO2, NOx, and PM2.5) in 31 regions of China (excluding Hong Kong, Macau, and Taiwan). Meanwhile, the spatial ...
IBM Cloud Satellite® lets you deploy and run apps consistently across on-premises, edge computing and public cloud environments on a 5G network. And it's all enabled by secure and auditable communications within the IBM Cloud®. A look at the applications and use cases that 5G is enabling to transform the world.
Studying the energy use behavior of occupants is crucial for accurately predicting building energy consumption. However, few studies have considered the impact of occupant behaviors on energy consumption in university dormitories. The objective of this study is to establish an agent-based model of energy consumption for university dormitories based on energy use behavior. The dormitories of a ...