
An official website of the United States government
The .gov means it’s official. Federal government websites often end in .gov or .mil. Before sharing sensitive information, make sure you’re on a federal government site.
The site is secure. The https:// ensures that you are connecting to the official website and that any information you provide is encrypted and transmitted securely.
- Publications
- Account settings
Preview improvements coming to the PMC website in October 2024. Learn More or Try it out now .
- Advanced Search
- Journal List
- Cancer Control
- v.28; Jan-Dec 2021
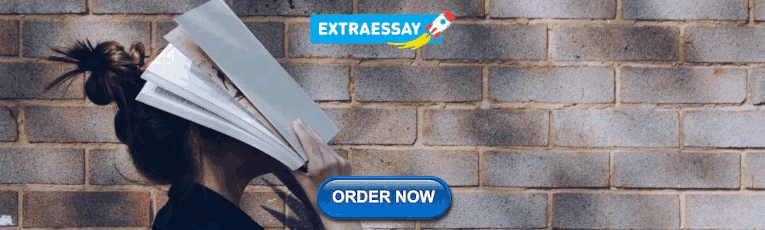
Cancer Biology, Epidemiology, and Treatment in the 21st Century: Current Status and Future Challenges From a Biomedical Perspective
Patricia piña-sánchez.
1 Oncology Research Unit, Oncology Hospital, Mexican Institute of Social Security, Mexico
Antonieta Chávez-González
Martha ruiz-tachiquín, eduardo vadillo, alberto monroy-garcía, juan josé montesinos, rocío grajales.
2 Department of Medical Oncology, Oncology Hospital, Mexican Institute of Social Security, Mexico
Marcos Gutiérrez de la Barrera
3 Clinical Research Division, Oncology Hospital, Mexican Institute of Social Security, Mexico
Hector Mayani
Since the second half of the 20th century, our knowledge about the biology of cancer has made extraordinary progress. Today, we understand cancer at the genomic and epigenomic levels, and we have identified the cell that starts neoplastic transformation and characterized the mechanisms for the invasion of other tissues. This knowledge has allowed novel drugs to be designed that act on specific molecular targets, the immune system to be trained and manipulated to increase its efficiency, and ever more effective therapeutic strategies to be developed. Nevertheless, we are still far from winning the war against cancer, and thus biomedical research in oncology must continue to be a global priority. Likewise, there is a need to reduce unequal access to medical services and improve prevention programs, especially in countries with a low human development index.
Introduction
During the last one hundred years, our understanding of the biology of cancer increased in an extraordinary way. 1 - 4 Such a progress has been particularly prompted during the last few decades because of technological and conceptual progress in a variety of fields, including massive next-generation sequencing, inclusion of “omic” sciences, high-resolution microscopy, molecular immunology, flow cytometry, analysis and sequencing of individual cells, new cell culture techniques, and the development of animal models, among others. Nevertheless, there are many questions yet to be answered and many problems to be solved regarding this disease. As a consequence, oncological research must be considered imperative.
Currently, cancer is one of the illnesses that causes more deaths worldwide. 5 According to data reported in 2020 by the World Health Organization (WHO), cancer is the second cause of death throughout the world, with 10 million deaths. 6 Clearly, cancer is still a leading problem worldwide. With this in mind, the objective of this article is to present a multidisciplinary and comprehensive overview of the disease. We will begin by analyzing cancer as a process, focusing on the current state of our knowledge on 4 specific aspects of its biology. Then, we will look at cancer as a global health problem, considering some epidemiological aspects, and discussing treatment, with a special focus on novel therapies. Finally, we present our vision on some of the challenges and perspectives of cancer in the 21 st century.
The Biology of Cancer
Cancer is a disease that begins with genetic and epigenetic alterations occurring in specific cells, some of which can spread and migrate to other tissues. 4 Although the biological processes affected in carcinogenesis and the evolution of neoplasms are many and widely different, we will focus on 4 aspects that are particularly relevant in tumor biology: genomic and epigenomic alterations that lead to cell transformation, the cells where these changes occur, and the processes of invasion and metastasis that, to an important degree, determine tumor aggressiveness.
Cancer Genomics
The genomics of cancer can be defined as the study of the complete sequence of DNA and its expression in tumor cells. Evidently, this study only becomes meaningful when compared to normal cells. The sequencing of the human genome, completed in 2003, was not only groundbreaking with respect to the knowledge of our gene pool, but also changed the way we study cancer. In the post-genomic era, various worldwide endeavors, such as the Human Cancer Genome Project , the Cancer Genome ATLAS (TCGA), the International Cancer Genome Consortium, and the Pan-Cancer Analysis Working Group (PCAWG), have contributed to the characterization of thousands of primary tumors from different neoplasias, generating more than 2.5 petabytes (10 15 ) of genomic, epigenomic, and proteomic information. This has led to the building of databases and analytical tools that are available for the study of cancer from an “omic” perspective, 7 , 8 and it has helped to modify classification and treatment of various neoplasms.
Studies in the past decade, including the work by the PCAWG, have shown that cancer generally begins with a small number of driving mutations (4 or 5 mutations) in particular genes, including oncogenes and tumor-suppressor genes. Mutations in TP53, a tumor-suppressor gene, for example, are found in more than half of all cancer types as an early event, and they are a hallmark of precancerous lesions. 9 - 12 From that point on, the evolution of tumors may take decades, throughout which the mutational spectrum of tumor cells changes significantly. Mutational analysis of more than 19 000 exomes revealed a collection of genomic signatures, some associated with defects in the mechanism of DNA repair. These studies also revealed the importance of alterations in non-coding regions of DNA. Thus, for example, it has been observed that various pathways of cell proliferation and chromatin remodeling are altered by mutations in coding regions, while pathways, such as WNT and NOTCH, can be disrupted by coding and non-coding mutations. To the present date, 19 955 genes that codify for proteins and 25 511 genes for non-coding RNAs have been identified ( https://www.gencodegenes.org/human/stats.html ). Based on this genomic catalogue, the COSMIC (Catalogue Of Somatic Mutations In Cancer) repository, the most robust database to date, has registered 37 288 077 coding mutations, 19 396 fusions, 1 207 190 copy number variants, and 15 642 672 non-coding variants reported up to August 2020 (v92) ( https://cosmic-blog.sanger.ac.uk/cosmic-release-v92/ ).
The genomic approach has accelerated the development of new cancer drugs. Indeed, two of the most relevant initiatives in recent years are ATOM (Accelerating Therapeutics for Opportunities in Medicine), which groups industry, government and academia, with the objective of accelerating the identification of drugs, 13 and the Connectivity Map (CMAP), a collection of transcriptional data obtained from cell lines treated with drugs for the discovery of functional connections between genes, diseases, and drugs. The CMAP 1.0 covered 1300 small molecules and more than 6000 signatures; meanwhile, the CMAP 2.0 with L1000 assay profiled more than 1.3 million samples and approximately 400 000 signatures. 14
The genomic study of tumors has had 2 fundamental contributions. On the one hand, it has allowed the confirmation and expansion of the concept of intratumor heterogeneity 15 , 16 ; and on the other, it has given rise to new classification systems for cancer. Based on the molecular classification developed by expression profiles, together with mutational and epigenomic profiles, a variety of molecular signatures have been identified, leading to the production of various commercial multigene panels. In breast cancer, for example, different panels have been developed, such as Pam50/Prosigna , Blue Print , OncotypeDX , MammaPrint , Prosigna , Endopredict , Breast Cancer Index , Mammostrat, and IHC4 . 17
Currently, the genomic/molecular study of cancer is more closely integrated with clinical practice, from the classification of neoplasms, as in tumors of the nervous system, 18 to its use in prediction, as in breast cancer. 17 Improvement in molecular methods and techniques has allowed the use of smaller amounts of biological material, as well as paraffin-embedded samples for genomic studies, both of which provide a wealth of information. 19 In addition, non-invasive methods, such as liquid biopsies, represent a great opportunity not only for the diagnosis of cancer, but also for follow-up, especially for unresectable tumors. 20
Research for the production of genomic information on cancer is presently dominated by several consortia, which has allowed the generation of a great quantity of data. However, most of these consortia and studies are performed in countries with a high human development index (HDI), and countries with a low HDI are not well represented in these large genomic studies. This is why initiatives such as Human Heredity and Health in Africa (H3Africa) for genomic research in Africa are essential. 21 Generation of new information and technological developments, such as third-generation sequencing, will undoubtedly continue to move forward in a multidisciplinary and complex systems context. However, the existing disparities in access to genomic tools for diagnosis, prognosis, and treatment of cancer will continue to be a pressing challenge at regional and social levels.
Cancer Epigenetics
Epigenetics studies the molecular mechanisms that produce hereditable changes in gene expression, without causing alterations in the DNA sequence. Epigenetic events are of 3 types: methylation of DNA and RNA, histone modification (acetylation, methylation, and phosphorylation), and the expression of non-coding RNA. Epigenetic aberrations can drive carcinogenesis when they alter chromosome conformation and the access to transcriptional machinery and to various regulatory elements (promoters, enhancers, and anchors for interaction with chromatin, for example). These changes may activate oncogenesis and silence tumor-suppressor mechanisms when they modulate coding and non-coding sequences (such as micro-RNAs and long-RNAs). This can then lead to uncontrolled growth, as well as the invasion and metastasis of cancer cells.
While genetic mutations are stable and irreversible, epigenetic alterations are dynamic and reversible; that is, there are several epigenomes, determined by space and time, which cause heterogeneity of the “epigenetic status” of tumors during their development and make them susceptible to environmental stimuli or chemotherapeutic treatment. 22 Epigenomic variability creates differences between cells, and this creates the need to analyze cells at the individual level. In the past, epigenetic analyses measured “average states” of cell populations. These studies revealed general mechanisms, such as the role of epigenetic marks on active or repressed transcriptional states, and established maps of epigenetic composition in a variety of cell types in normal and cancerous tissue. However, these approaches are difficult to use to examine events occurring in heterogeneous cell populations or in uncommon cell types. This has led to the development of new techniques that permit marking of a sequence on the epigenome and improvement in the recovery yield of epigenetic material from individual cells. This has helped to determine changes in DNA, RNA, and histones, chromatin accessibility, and chromosome conformation in a variety of neoplasms. 23 , 24
In cancer, DNA hypomethylation occurs on a global scale, while hypermethylation occurs in specific genomic loci, associated with abnormal nucleosome positioning and chromatin modifications. This information has allowed epigenomic profiles to be established in different types of neoplasms. In turn, these profiles have served as the basis to identify new neoplasm subgroups. For example, in triple negative breast cancer (TNBC), 25 and in hepatocellular carcinoma, 26 DNA methylation profiles have helped to the identification of distinct subgroups with clinical relevance. Epigenetic approaches have also helped to the development of prognostic tests to assess the sensitivity of cancer cells to specific drugs. 27
Epigenetic traits could be used to characterize intratumoral heterogeneity and determine the relevance of such a heterogeneity in clonal evolution and sensitivity to drugs. However, it is clear that heterogeneity is not only determined by genetic and epigenetic diversity resulting from clonal evolution of tumor cells, but also by the various cell populations that form the tumor microenvironment (TME). 28 Consequently, the epigenome of cancer cells is continually remodeled throughout tumorigenesis, during resistance to the activity of drugs, and in metastasis. 29 This makes therapeutic action based on epigenomic profiles difficult, although significant advances in this area have been reported. 30
During carcinogenesis and tumor progression, epigenetic modifications are categorized by their mechanisms of regulation ( Figure 1A ) and the various levels of structural complexity ( Figure 1B ). In addition, the epigenome can be modified by environmental stimuli, stochastic events, and genetic variations that impact the phenotype ( Figure 1C ). 31 , 32 The molecules that take part in these mechanisms/events/variations are therapeutic targets of interest with potential impact on clinical practice. There are studies on a wide variety of epidrugs, either alone or in combination, which improve antitumor efficacy. 33 However, the problems with these drugs must not be underestimated. For a considerable number of epigenetic compounds still being under study, the main challenge is to translate in vitro efficacy of nanomolar (nM) concentrations into well-tolerated and efficient clinical use. 34 The mechanisms of action of epidrugs may not be sufficiently controlled and could lead to diversion of the therapeutic target. 35 It is known that certain epidrugs, such as valproic acid, produce unwanted epigenetic changes 36 ; thus the need for a well-established safety profile before these drugs can be used in clinical therapy. Finally, resistance to certain epidrugs is another relevant problem. 37 , 38

Epigenetics of cancer. (A) Molecular mechanisms. (B) Structural hierarchy of epigenomics. (C) Factors affecting the epigenome. Modified from Refs. 31 and 32 .
As we learn about the epigenome of specific cell populations in cancer patients, a door opens to the evaluation of sensitivity tests and the search for new molecular markers for detection, prognosis, follow-up, and/or response to treatment at various levels of molecular regulation. Likewise, the horizon expands for therapeutic alternatives in oncology with the use of epidrugs, such as pharmacoepigenomic modulators for genes and key pathways, including methylation of promoters and regulation of micro-RNAs involved in chemoresponse and immune response in cancer. 39 There is no doubt that integrated approaches identifying stable pharmagenomic and epigenomic patterns and their relation with expression profiles and genetic functions will be more and more valuable in our fight against cancer.
Cancer Stem Cells
Tumors consist of different populations of neoplastic cells and a variety of elements that form part of the TME, including stromal cells and molecules of the extracellular matrix. 40 Such intratumoral heterogeneity becomes even more complex during clonal variation of transformed cells, as well as influence the elements of the TME have on these cells throughout specific times and places. 41 To explain the origin of cancer cell heterogeneity, 2 models have been put forward. The first proposes that mutations occur at random during development of the tumor in individual neoplastic cells, and this promotes the production of various tumor populations, which acquire specific growth and survival traits that lead them to evolve according to intratumor mechanisms of natural selection. 42 The second model proposes that each tumor begins as a single cell that possess 2 functional properties: it can self-renew and it can produce several types of terminal cells. As these 2 properties are characteristics of somatic stem cells, 43 the cells have been called cancer stem cells (CSCs). 44 According to this model, tumors must have a hierarchical organization, where self-renewing stem cells produce highly proliferating progenitor cells, unable to self-renew but with a high proliferation potential. The latter, in turn, give rise to terminal cells. 45 Current evidence indicates that both models may coexist in tumor progression. In agreement with this idea, new subclones could be produced as a result of a lack of genetic stability and mutational changes, in addition to the heterogeneity derived from the initial CSC and its descendants. Thus, in each tumor, a set of neoplastic cells with different genetic and epigenetic traits may be found, which would provide different phenotypic properties. 46
The CSC concept was originally presented in a model of acute myeloid leukemia. 47 The presence of CSCs was later proved in chronic myeloid leukemia, breast cancer, tumors of the central nervous system, lung cancer, colon cancer, liver cancer, prostate cancer, pancreatic cancer, melanoma, and cancer of the head and neck, amongst others. In all of these cases, detection of CSCs was based on separation of several cell populations according to expression of specific surface markers, such as CD133, CD44, CD24, CD117, and CD15. 48 It is noteworthy that in some solid tumors, and even in some hematopoietic ones, a combination of specific markers that allow the isolation of CSCs has not been found. Interestingly, in such tumors, a high percentage of cells with the capacity to start secondary tumors has been observed; thus, the terms Tumor Initiating Cells (TIC) or Leukemia Initiating Cells (LIC) have been adopted. 46
A relevant aspect of the biology of CSCs is that, just like normal stem cells, they can self-renew. Such self-renewal guarantees the maintenance or expansion of the tumor stem cell population. Another trait CSCs share with normal stem cells is their quiescence, first described in chronic myeloid leukemia. 49 The persistence of quiescent CSCs in solid tumors has been recently described in colorectal cancer, where quiescent clones can become dominant after therapy with oxaliplatin. 50 In non-hierarchical tumors, such as melanoma, the existence of slow-cycling cells that are resistant to antimitogenic agents has also been proved. 51 Such experimental evidence supports the idea that quiescent CSCs or TICs are responsible for both tumor resistance to antineoplastic drugs and clinical relapse after initial therapeutic success.
In addition to quiescence, CSCs use other mechanisms to resist the action of chemotherapeutic drugs. One of these is their increased numbers: upon diagnosis, a high number of CSCs are observed in most analyzed tumors, making treatment unable to destroy all of them. On the other hand, CSCs have a high number of molecular pumps that expulse drugs, as well as high numbers of antiapoptotic molecules. In addition, they have very efficient mechanisms to repair DNA damage. In general, these cells show changes in a variety of signaling pathways involved in proliferation, survival, differentiation, and self-renewal. It is worth highlighting that in recent years, many of these pathways have become potential therapeutic targets in the elimination of CSCs. 52 Another aspect that is highly relevant in understanding the biological behavior of CSCs is that they require a specific site for their development within the tissue where they are found that can provide whatever is needed for their survival and growth. These sites, known as niches, are made of various cells, both tumor and non-tumor, as well as a variety of non-cellular elements (extracellular matrix [ECM], soluble cytokines, ion concentration gradients, etc.), capable of regulating the physiology of CSCs in order to promote their expansion, the invasion of adjacent tissues, and metastasis. 53
It is important to consider that although a large number of surface markers have been identified that allow us to enrich and prospectively follow tumor stem cell populations, to this day there is no combination of markers that allows us to find these populations in all tumors, and it is yet unclear if all tumors present them. In this regard, it is necessary to develop new purification strategies based on the gene expression profiles of these cells, so that tumor heterogeneity is taken into account, as it is evident that a tumor can include multiple clones of CSCs that, in spite of being functional, are genetically different, and that these clones can vary throughout space (occupying different microenvironments and niches) and time (during the progression of a range of tumor stages). Such strategies, in addition to new in vitro and in vivo assays, will allow the development of new and improved CSC elimination strategies. This will certainly have an impact on the development of more efficient therapeutic alternatives.
Invasion and Metastasis
Nearly 90% of the mortality associated with cancer is related to metastasis. 54 This consists of a cascade of events ( Figure 2 ) that begins with the local invasion of a tumor into surrounding tissues, followed by intravasation of tumor cells into the blood stream or lymphatic circulation. Extravasation of neoplastic cells in areas distant from the primary tumor then leads to the formation of one or more micrometastatic lesions which subsequently proliferate to form clinically detectable lesions. 4 The cells that are able to produce metastasis must acquire migratory characteristics, which occur by a process known as epithelial–mesenchymal transition (EMT), that is, the partial loss of epithelial characteristics and the acquirement of mesenchymal traits. 55

Invasion and metastasis cascade. Invasion and metastasis can occur early or late during tumor progression. In either case, invasion to adjacent tissues is driven by stem-like cells (cancer stem cells) that acquire the epithelial–mesenchymal transition (EMT) (1). Once they reach sites adjacent to blood vessels, tumor cells (individually or in clusters) enter the blood (2). Tumor cells in circulation can adhere to endothelium and extravasation takes place (3). Other mechanisms alternative to extravasation can exist, such as angiopelosis, in which clusters of tumor cells are internalized by the endothelium. Furthermore, at certain sites, tumor cells can obstruct microvasculature and initiate a metastatic lesion right there. Sometimes, a tumor cells that has just exit circulation goes into an MET in order to become quiescent (4). Inflammatory signals can activate quiescent metastatic cells that will proliferate and generate a clinically detectable lesion (5).
Although several of the factors involved in this process are currently known, many issues are still unsolved. For instance, it has not yet been possible to monitor in vivo the specific moment when it occurs 54 ; the microenvironmental factors of the primary tumor that promote such a transition are not known with precision; and the exact moment during tumor evolution in which one cell or a cluster of cells begin to migrate to distant areas, is also unknown. The wide range of possibilities offered by intra- and inter-tumoral heterogeneity 56 stands in the way of suggesting a generalized strategy that could resolve this complication.
It was previously believed that metastasis was only produced in late stages of tumor progression; however, recent studies indicate that EMT and metastasis can occur during the early course of the disease. In pancreatic cancer, for example, cells going through EMT are able to colonize and form metastatic lesions in the liver in the first stages of the disease. 52 , 57 Metastatic cell clusters circulating in peripheral blood (PB) are prone to generate a metastatic site, compared to individual tumor cells. 58 , 59 In this regard, novel strategies, such as the use of micro-RNAs, are being assessed in order to diminish induction of EMT. 60 It must be mentioned, however, that the metastatic process seems to be even more complex, with alternative pathways that do not involve EMT. 61 , 62
A crucial stage in the process of metastasis is the intravasation of tumor cells (alone or in clusters) towards the blood stream and/or lymphatic circulation. 63 These mechanisms are also under intensive research because blocking them could allow the control of spreading of the primary tumor. In PB or lymphatic circulation, tumor cells travel to distant parts for the potential formation of a metastatic lesion. During their journey, these cells must stand the pressure of blood flow and escape interaction with natural killer (NK) cells . 64 To avoid them, tumor cells often cover themselves with thrombocytes and also produce factors such as VEGF, angiopoietin-2, angiopoietin-4, and CCL2 that are involved in the induction of vascular permeability. 54 , 65 Neutrophils also contribute to lung metastasis in the bloodstream by secreting IL-1β and metalloproteases to facilitate extravasation of tumor cells. 64
The next step in the process of metastasis is extravasation, for which tumor cells, alone or in clusters, can use various mechanisms, including a recently described process known as angiopellosis that involves restructuring the endothelial barrier to internalize one or several cells into a tissue. 66 The study of leukocyte extravasation has contributed to a more detailed knowledge of this process, in such a way that some of the proposed strategies to avoid extravasation include the use of integrin inhibitors, molecules that are vital for rolling, adhesion, and extravasation of tumor cells. 67 , 68 Another strategy that has therapeutic potential is the use of antibodies that strengthen vascular integrity to obstruct transendothelial migration of tumor cells and aid in their destruction in PB. 69
Following extravasation, tumor cells can return to an epithelial phenotype, a process known as mesenchymal–epithelial transition and may remain inactive for several years. They do this by competing for specialized niches, like those in the bone marrow, brain, and intestinal mucosa, which provide signals through the Notch and Wnt pathways. 70 Through the action of the Wnt pathway, tumor cells enter a slow state of the cell cycle and induce the expression of molecules that inhibit the cytotoxic function of NK cells. 71 The extravasated tumor cell that is in a quiescent state must comply with 2 traits typical of stem cells: they must have the capacity to self-renew and to generate all of the cells that form the secondary tumor.
There are still several questions regarding the metastatic process. One of the persisting debates at present is if EMT is essential for metastasis or if it plays a more important role in chemoresistance. 61 , 62 It is equally important to know if there is a pattern in each tumor for the production of cells with the capacity to carry out EMT. In order to control metastasis, it is fundamental to know what triggers acquisition of the migratory phenotype and the intrinsic factors determining this transition. Furthermore, it is essential to know if mutations associated with the primary tumor or the variety of epigenetic changes are involved in this process. 55 It is clear that metastatic cells have affinity for certain tissues, depending on the nature of the primary tumor (seed and soil hypothesis). This may be caused by factors such as the location and the direction of the bloodstream or lymphatic fluid, but also by conditioning of premetastatic niches at a distance (due to the large number of soluble factors secreted by the tumor and the recruitment of cells of the immune system to those sites). 72 We have yet to identify and characterize all of the elements that participate in this process. Deciphering them will be of upmost importance from a therapeutic point of view.
Epidemiology of Cancer
Cancer is the second cause of death worldwide; today one of every 6 deaths is due to a type of cancer. According to the International Agency for Research on Cancer (IARC), in 2020 there were approximately 19.3 million new cases of cancer, and 10 million deaths by this disease, 6 while 23.8 million cases and 13.0 million deaths are projected to occur by 2030. 73 In this regard, it is clear the increasing role that environmental factors—including environmental pollutants and processed food—play as cancer inducers and promoters. 74 The types of cancer that produce the greatest numbers of cases and deaths worldwide are indicated in Table 1 . 6
Total Numbers of Cancer Cases and Deaths Worldwide in 2020 by Cancer Type (According to the Global Cancer Observatory, IARC).
Cases | ||
---|---|---|
Both sexes | Women | Men |
Breast (2.26 million) | Breast (2.26 million) | Lung (1.43 million) |
Lung (2.20 million) | Colorectal (865 000) | Prostate (1.41 million) |
Colorectal (1.93 million) | Lung (770 000) | Colorectal (1.06 million) |
Prostate (1.41 million) | Cervical (604 000) | Stomach (719 000) |
Stomach (1.08 million) | Thyroid (448 000) | Liver (632 000) |
Deaths | ||
---|---|---|
Both sexes | Women | Men |
Lung (1.79 million) | Breast (684 000) | Lung (1.18 million) |
Colorectal (935 000) | Lung (607 000) | Liver (577 000) |
Liver (830 000) | Colorectal (419 000) | Colorectal (515 000) |
Stomach (768 000) | Cervical (341 000) | Stomach (502 000) |
Breast (684 000) | Stomach (266 000) | Prostate (375 000) |
Data presented on this table were obtained from Ref. 6.
As shown in Figure 3 , lung, breast, prostate, and colorectal cancer are the most common throughout the world, and they are mostly concentrated in countries of high to very high human development index (HDI). Although breast, prostate, and colorectal cancer have a high incidence, the number of deaths they cause is proportionally low, mostly reflecting the great progress made in their control. However, these data also reveal the types of cancer that require further effort in prevention, precise early detection avoiding overdiagnosis, and efficient treatment. This is the case of liver, lung, esophageal, and pancreatic cancer, where the difference between the number of cases and deaths is smaller ( Figure 3B ). Social and economic transition in several countries has had an impact on reducing the incidence of neoplasms associated with infection and simultaneously produced an increase in the types related to reproductive, dietary, and hormonal factors. 75

Incidence and mortality for some types of cancer in the world. (A) Estimated number of cases and deaths in 2020 for the most frequent cancer types worldwide. (B) Incidence and mortality rates, normalized according to age, for the most frequent cancer types in countries with very high/& high (VH&H; blue) and/low and middle (L&M; red) Human Development Index (HDI). Data include both genders and all ages. Data according to https://gco.iarc.fr/today , as of June 10, 2021.
In the past 3 decades, cancer mortality rates have fallen in high HDI countries, with the exception of pancreatic cancer, and lung cancer in women. Nevertheless, changes in the incidence of cancer do not show the same consistency, possibly due to variables such as the possibility of early detection, exposure to risk factors, or genetic predisposition. 76 , 77 Countries such as Australia, Canada, Denmark, Ireland, New Zealand, Norway, and the United Kingdom have reported a reduction in incidence and mortality in cancer of the stomach, colon, lung, and ovary, as well as an increase in survival. 78 Changes in modifiable risk factors, such as the use of tobacco, have played an important role in prevention. In this respect, it has been estimated that decline in tobacco use can explain between 35% and 45% of the reduction in cancer mortality rates, 79 while the fall in incidence and mortality due to stomach cancer can be attributed partly to the control of Helicobacter pylori infection. 80 Another key factor in the fall of mortality rates in developed countries has been an increase in early detection as a result of screening programs, as in breast and prostate cancer, which have had their mortality rates decreased dramatically in spite of an increase in their incidence. 76
Another important improvement observed in recent decades is the increase in survival rates, particularly in high HDI countries. In the USA, for example, survival rates for patients with prostate cancer at 5 years after initial diagnosis was 28% during 1947–1951; 69% during 1975–1977, and 100% during 2003–2009. Something similar occurred with breast cancer, with a 5-year survival rate of 54% in 1947–1951, 75% in 1975–1977, and 90% in 2003–2009. 81 In the CONCORD 3 version, age-standardize 5-year survival for patients with breast cancer in the USA during 2010–2014 was 90%, and 97% for prostate cancer patients. 82 Importantly, even among high HDI countries, significant differences have been identified in survival rates, being stage of disease at diagnosis, time for access to effective treatment, and comorbidities, the main factors influencing survival in these nations. 78 Unfortunately, survival rates in low HDI countries are significantly lower due to several factors, including lack of information, deficient screening and early detection programs, limited access to treatment, and suboptimal cancer registration. 82 It should be noted that in countries with low to middle HDI, neoplasms with the greatest incidence are those affecting women (breast and cervical cancer), which reflects not only a problem with access to health services, but also a serious inequality issue that involves social, cultural, and even religious obstacles. 83
Up to 42% of incident cases and 47% of deaths by cancer in the USA are due to potentially modifiable risk factors such as use of tobacco, physical activity, diet, and infection. 84 It has been calculated that 2.4 million deaths by cancer, mostly of the lung, can be attributed to tobacco. 73 In 2020, the incidence rate of lung cancer in Western Africa was 2.2, whereas in Polynesia and Eastern Asia was 37.3 and 34.4, respectively. 6 In contrast, the global burden of cancer associated with infection was 15.4%, but in Sub-Saharan Africa it was 30%. 85 Likewise, the incidence of cervical cancer in Eastern Africa was 40.1, in contrast with the USA and Canada that have a rate of 6.2. This makes it clear that one of the challenges we face is the reduction of the risk factors that are potentially modifiable and associated with specific types of cancer.
Improvement of survival rates and its disparities worldwide are also important challenges. Five-year survival for breast cancer—diagnosed during 2010-2014— in the USA, for example, was 90%, whereas in countries like South Africa it was 40%. 82 Childhood leukemia in the USA and several European countries shows a 5-year survival of 90%, while in Latin-American countries it is 50–76%. 86 Interestingly, there are neoplasms, such as pancreatic cancer, for which there has been no significant increase in survival, which remains low (5–15%) both in developed and developing countries. 82
Although data reported on global incidence and mortality gives a general overview on the epidemiology of cancer, it is important to note that there are great differences in coverage of cancer registries worldwide. To date, only 1 out of every 3 countries reports high quality data on the incidence of cancer. 87 For the past 50 years, the IARC has supported population-based cancer registries; however, more than one-third of the countries belonging to the WHO, mainly countries of low and middle income (LMIC), have no data on more than half of the 18 indicators of sustainable development goals. 88 High quality cancer registries only cover 4% of the population in Africa, 8% in Asia, and 7% in Latin America, contrasting with 83% in the USA and Canada, and 33% in Europe. 89 In response to this situation, the Global Initiative for Cancer Registry Development was created in 2012 to generate improved infrastructure to permit greater coverage and better quality registries, especially in countries with low and middle HDI. 88 It is expected that initiatives of this sort in the coming years will allow more and better information to guide strategies for the control of cancer worldwide, especially in developing regions. This will enable survival to be measured over longer periods of time (10, 15, or 20 years), as an effective measure in the control of cancer. The WHO has established as a target for 2025 to reduce deaths by cancer and other non-transmissible diseases by 25% in the population between the ages of 30–69; such an effort requires not only effective prevention measures to reduce incidence, but also more efficient health systems to diminish mortality and increase survival. At the moment, it is an even greater challenge because of the effects of the COVID-19 pandemic which has negatively impacted cancer prevention and health services. 90
Oncologic Treatments
A general perspective.
At the beginning of the 20th century, cancer treatment, specifically treatment of solid tumors, was based fundamentally on surgical resection of tumors, which together with other methods for local control, such as cauterization, had been used since ancient times. 91 At that time, there was an ongoing burst of clinical observations along with interventions sustained on fundamental knowledge about physics, chemistry, and biology. In the final years of the 19 th century and the first half of the 20th, these technological developments gave rise to radiotherapy, hormone therapy, and chemotherapy. 92 - 94 Simultaneously, immunotherapy was also developed, although usually on a smaller scale, in light of the overwhelming progress of chemotherapy and radiotherapy. 95
Thus began the development and expansion of disciplines based on these approaches (surgery, radiotherapy, chemotherapy, hormone therapy, and immunotherapy), with their application evolving ever more rapidly up to their current uses. Today, there is a wide range of therapeutic tools for the care of cancer patients. These include elements that emerged empirically, arising from observations of their effects in various medical fields, as well as drugs that were designed to block processes and pathways that form part of the physiopathology of one or more neoplasms according to knowledge of specific molecular alterations. A classic example of the first sort of tool is mustard gas, originally used as a weapon in war, 96 but when applied for medical purposes, marked the beginning of the use of chemicals in the treatment of malignant neoplasms, that is, chemotherapy. 94 A clear example of the second case is imatinib, designed specifically to selectively inhibit a molecular alteration in chronic myeloid leukemia: the Bcr-Abl oncoprotein. 97
It is on this foundation that today the 5 areas mentioned previously coexist and complement one another. The general framework that motivates this amalgam and guides its development is precision medicine, founded on the interaction of basic and clinical science. In the forecasts for development in each of these fields, surgery is expected to continue to be the fundamental approach for primary tumors in the foreseeable future, as well as when neoplastic disease in the patient is limited, or can be limited by applying systemic or regional elements, before and/or after surgical resection, and it can be reasonably anticipated for the patient to have a significant period free from disease or even to be cured. With regards to technology, intensive exploration of robotic surgery is contemplated. 98
The technological possibilities for radiotherapy have progressed in such a way that it is now possible to radiate neoplastic tissue with an extraordinary level of precision, and therefore avoid damage to healthy tissue. 99 This allows administration of large doses of ionizing radiation in one or a few fractions, what is known as “radiosurgery.” The greatest challenges to the efficacy of this approach are related to radio-resistance in certain neoplasms. Most efforts regarding research in this field are concentrated on understanding the underlying biological mechanisms of the phenomenon and their potential control through radiosensitizers. 100
“Traditional” chemotherapy, based on the use of compounds obtained from plants and other natural products, acting in a non-specific manner on both neoplastic and healthy tissues with a high proliferation rate, continues to prevail. 101 The family of chemotherapeutic drugs currently includes alkylating agents, antimetabolites, anti-topoisomerase agents, and anti-microtubules. Within the pharmacologic perspective, the objective is to attain a high concentration or activity of such molecules in specific tissues while avoiding their accumulation in others, in order to achieve an increase in effectiveness and a reduction in toxicity. This has been possible with the use of viral vectors, for example, that are able to limit their replication in neoplastic tissues, and activate prodrugs of normally nonspecific agents, like cyclophosphamide, exclusively in those specific areas. 102 More broadly, chemotherapy also includes a subgroup of substances, known as molecular targeted therapy, that affect processes in a more direct and specific manner, which will be mentioned later.
There is no doubt that immunotherapy—to be explored next—is one of the therapeutic fields where development has been greatest in recent decades and one that has produced enormous expectation in cancer treatment. 103 Likewise, cell therapy, based on the use of immune cells or stem cells, has come to complement the oncologic therapeutic arsenal. 43 Each and every one of the therapeutic fields that have arisen in oncology to this day continue to prevail and evolve. Interestingly, the foreseeable future for the development of cancer treatment contemplates these approaches in a joint and complementary manner, within the general framework of precision medicine, 104 and sustained by knowledge of the biological mechanisms involved in the appearance and progression of neoplasms. 105 , 106
Immunotherapy
Stimulating the immune system to treat cancer patients has been a historical objective in the field of oncology. Since the early work of William Coley 107 to the achievements reached at the end of the 20 th century, scientific findings and technological developments paved the way to searching for new immunotherapeutic strategies. Recombinant DNA technology allowed the synthesis of cytokines, such as interferon-alpha (IFN-α) and interleukin 2 (IL-2), which were authorized by the US Food and Drug Administration (FDA) for the treatment of hairy cell leukemia in 1986, 108 as well as kidney cancer and metastatic melanoma in 1992 and 1998, respectively. 109
The first therapeutic vaccine against cancer, based on the use of autologous dendritic cells (DCs), was approved by the FDA against prostate cancer in 2010. However, progress in the field of immunotherapy against cancer was stalled in the first decade of the present century, mostly due to failure of several vaccines in clinical trials. In many cases, application of these vaccines was detained by the complexity and cost involved in their production. Nevertheless, with the coming of the concept of immune checkpoint control, and the demonstration of the relevance of molecules such as cytotoxic T-lymphocyte antigen 4 (CTLA-4), and programmed cell death molecule-1 (PD-1), immunotherapy against cancer recovered its global relevance. In 2011, the monoclonal antibody (mAb) ipilimumab, specific to the CTLA-4 molecule, was the first checkpoint inhibitor (CPI) approved for the treatment of advanced melanoma. 110 Later, inhibitory mAbs for PD-1, or for the PD-1 ligand (PD-L1), 111 as well as the production of T cells with chimeric receptors for antigen recognition (CAR-T), 112 which have been approved to treat various types of cancer, including melanoma, non-small cell lung cancer (NSCLC), head and neck cancer, bladder cancer, renal cell carcinoma (RCC), and hepatocellular carcinoma, among others, have changed the paradigm of cancer treatment.
In spite of the current use of anti-CTLA-4 and anti-PD-L1 mAbs, only a subgroup of patients has responded favorably to these CPIs, and the number of patients achieving clinical benefit is still small. It has been estimated that more than 70% of patients with solid tumors do not respond to CPI immunotherapy because either they show primary resistance, or after responding favorably, develop resistance to treatment. 113 In this regard, it is important to mention that in recent years very important steps have been taken to identify the intrinsic and extrinsic mechanisms that mediate resistance to CPI immunotherapy. 114 Intrinsic mechanisms include changes in the antitumor immune response pathways, such as faulty processing and presentation of antigens by APCs, activation of T cells for tumor cell destruction, and changes in tumor cells that lead to an immunosuppressive TME. Extrinsic factors include the presence of immunosuppressive cells in the local TME, such as regulatory T cells, myeloid-derived suppressor cells (MDSC), mesenchymal stem/stromal cells (MSCs), and type 2 macrophages (M2), in addition to immunosuppressive cytokines.
On the other hand, classification of solid tumors as “hot,” “cold,” or “excluded,” depending on T cell infiltrates and the contact of such infiltrates with tumor cells, as well as those that present high tumor mutation burden (TMB), have redirected immunotherapy towards 3 main strategies 115 ( Table 2 ): (1) Making T-cell antitumor response more effective, using checkpoint inhibitors complementary to anti-CTLA-4 and anti-PD-L1, such as LAG3, Tim-3, and TIGT, as well as using CAR-T cells against tumor antigens. (2) Activating tumor-associated myeloid cells including monocytes, granulocytes, macrophages, and DC lineages, found at several frequencies within human solid tumors. (3) Regulating the biochemical pathways in TME that produce high concentrations of immunosuppressive molecules, such as kynurenine, a product of tryptophan metabolism, through the activity of indoleamine 2,3 dioxygenase; or adenosine, a product of ATP hydrolysis by the activity of the enzyme 5’nucleotidase (CD73). 116
Current Strategies to Stimulate the Immune Response for Antitumor Immunotherapy.
Strategies | T cells | Myeloid cells | TME |
---|---|---|---|
Lymph node | Anti-CTLA4 | TNF-α | |
To improve tumor antigen presentation by APCs | Anti-CD137 | IFN-α | |
To optimize effector T-cell activation | Anti-OX40 | IL-1 | |
Anti-CD27/CD70 | GM-CSF | ||
HVEM | CD40L/CD40 | ||
GITR | CDN | ||
L-2 | ATP | ||
IL-12 | HMGB1 | ||
TLR | |||
STING | |||
RIG-1/MDA-5 | |||
Blood vessel | CX3CL1 | ||
To improve T-cell traffic to tumors | CXCL9 | ||
To favor T-cell infiltration into tumors | CXCL10 | ||
Transference of T cells bearing antigen-specific receptor | CCL5 | ||
LFA1/ICAM1 | |||
Selectins | |||
CAR-T cell | |||
TCR-T cell | |||
Tumor | Anti-PD-L1 | Anti-CSF1/CSF1R | Anti-VEGF |
To improve tumor antigen uptake by APCs | Anti-CTLA-4 | Anti-CCR2 | Inhibitors of IDO anti-CD73 |
To improve recognition and killing of tumor cells by T cells | Anti-LAG-3 | PI3Kγ | ARs antagonists |
Anti-TIM-3 | |||
Anti-TIGIT | |||
TNFR-agonists | |||
IL-2 | |||
IL-10 |
Abbreviations: TME, tumor microenvironment; IL, interleukin; TNF, Tumor Necrosis Factor; TNFR, TNF-receptor; CD137, receptor–co-stimulator of the TNFR family; OX40, member number 4 of the TNFR superfamily; CD27/CD70, member of the TNFR superfamily; CD40/CD40L, antigen-presenting cells (APC) co-stimulator and its ligand; GM-CSF, granulocyte-macrophage colony-stimulating factor; IFN, interferon; STING, IFN genes-stimulator; RIG-I, retinoic acid inducible gene-I; MDA5, melanoma differentiation-associated protein 5; CDN, cyclic dinucleotide; ATP, adenosine triphosphate; HMGB1, high mobility group B1 protein; TLR, Toll-like receptor; HVEM, Herpes virus entry mediator; GITR, glucocorticoid-induced TNFR family-related gene; CTLA4, cytotoxic T lymphocyte antigen 4; PD-L1, programmed death ligand-1; TIGIT, T-cell immunoreceptor with immunoglobulin and tyrosine-based inhibition motives; CSF1/CSF1R, colony-stimulating factor-1 and its receptor; CCR2, Type 2 chemokine receptor; PI3Kγ, Phosphoinositide 3-Kinase γ; CXCL/CCL, chemokine ligands; LFA1, lymphocyte function-associated antigen 1; ICAM1, intercellular adhesion molecule 1; VEGF, vascular endothelial growth factor; IDO, indolamine 2,3-dioxigenase; TGF, transforming growth factor; LAG-3, lymphocyte-activation gene 3 protein; TIM-3, T-cell immunoglobulin and mucin-domain containing-3; CD73, 5´nucleotidase; ARs, adenosine receptors; Selectins, cell adhesion molecules; CAR-T, chimeric antigen receptor T cell; TCR-T, T-cell receptor engineered T cell.
Apart from the problems associated with its efficacy (only a small group of patients respond to it), immunotherapy faces several challenges related to its safety. In other words, immunotherapy can induce adverse events in patients, such as autoimmunity, where healthy tissues are attacked, or cytokine release syndrome and vascular leak syndrome, as observed with the use of IL-2, both of which lead to serious hypotension, fever, renal failure, and other adverse events that are potentially lethal. The main challenges to be faced by immunotherapy in the future will require the combined efforts of basic and clinical scientists, with the objective of accelerating the understanding of the complex interactions between cancer and the immune system, and improve treatment options for patients. Better comprehension of immune phenotypes in tumors, beyond the state of PD-L1 and TME, will be relevant to increase immunotherapy efficacy. In this context, the identification of precise tumor antigenicity biomarkers by means of new technologies, such as complete genome sequencing, single cell sequencing, and epigenetic analysis to identify sites or subclones typical in drug resistance, as well as activation, traffic and infiltration of effector cells of the immune response, and regulation of TME mechanisms, may help define patient populations that are good candidates for specific therapies and therapeutic combinations. 117 , 118 Likewise, the use of agents that can induce specific activation and modulation of the response of T cells in tumor tissue, will help improve efficacy and safety profiles that can lead to better clinical results.
Molecular Targeted Therapy
For over 30 years, and based on the progress in our knowledge of tumor biology and its mechanisms, there has been a search for therapeutic alternatives that would allow spread and growth of tumors to be slowed down by blocking specific molecules. This approach is known as molecular targeted therapy. 119 Among the elements generally used as molecular targets there are transcription factors, cytokines, membrane receptors, molecules involved in a variety of signaling pathways, apoptosis modulators, promoters of angiogenesis, and cell cycle regulators. 120
Imatinib, a tyrosine kinase inhibitor for the treatment of chronic myeloid leukemia, became the first targeted therapy in the final years of the 1990s. 97 From then on, new drugs have been developed by design, and today more than 60 targeted therapies have been approved by the FDA for the treatment of a variety of cancers ( Table 3 ). 121 This has had a significant impact on progression-free survival and global survival in neoplasms such as non-small cell lung cancer, breast cancer, renal cancer, and melanoma.
FDA Approved Molecular Targeted Therapies for the Treatment of Solid Tumors.
Drug | Therapeutic target | Indications | Biomarkers |
---|---|---|---|
Abemaciclib | CDK4/6 inhibitor | Breast cancer | ER+/PR+ |
Abiraterone | Anti-androgen | Prostate cancer | AR+ |
Afatinib | TKI anti-ErbB, EGFR (ErbB1), HER2 (ErbB2), ErbB3, ErbB4 | NSCLC | EGFR mutated |
Deletion of exon 19 | |||
Substitution in exon 21 (L858R) | |||
Aflibercept | Anti-VEGF fusion protein | Colorectal cancer | |
Alectinib | Anti-ALK TKI | NSCLC | ALK+ |
Alpelisib | PI3K inhibitor | Breast cancer | PI3K mutated |
Apalutamide | Anti-androgen | Prostate cancer | AR+ |
Atezolizumab | Anti-PD-L1 mAb | Breast cancer | PD-L1 |
Hepatocellular carcinoma | |||
NSCLC | |||
Bladder cancer | |||
Avapritinib | Kinase inhibitor | GIST | PDGFRA mutated in exon 18 (D842V) |
Avelumab | Anti-PD-L1 mAb | Renal cancer | PD-L1 |
Bladder cancer | |||
Neuroendocrine tumors | |||
Axitinib | Anti-VEGF TKI | Renal cancer | |
Bevacizumab | Anti-VEGF mAb | CNS tumors | |
Ovarian cancer | |||
Cervical cancer | |||
Colorectal cancer | |||
Hepatocellular carcinoma | |||
NSCLC | |||
Renal cancer | |||
Brigatinib | Anti-ALK TKI | NSCLC | ALK+ |
Cabozantinib | TKR inhibitor: anti-MET, anti-VEGF, anti-RET, ROS1, MER, KIT | Renal cancer | |
Hepatocellular carcinoma | |||
Thyroid cancer | |||
Ceritinib | Anti-ALK TKI | NSCLC | ALK+ |
Cetuximab | Anti-EGFR mAb | Colorectal cancer | KRAS |
Head and Neck cancer | EGFR+ | ||
Crizotinib | Anti-ALK TKI | NSCLC | ALK+, ROS1+ |
Dabrafenib | BRAF inhibitor | NSCLC | BRAF-V600E, V600K |
Thyroid cancer | |||
Melanoma | |||
Dacomitinib | Anti-EGFR TKI | NSCLC | EGFR+ |
Darolutamide | Anti-androgen | Prostate cancer | AR+ |
Durvalumab | Anti-PD-L1 mAb | NSCLC | PD-L1 |
Bladder cancer | |||
Encorafenib | BRAF inhibitor | Colorectal cancer | BRAF-V600E |
Melanoma | |||
Entrectinib | Anti-ROS1 TKI | NSCLC | ROS1+ |
Enzalutamide | Anti-androgen | Prostate cancer | AR+ |
Erdafitinib | Anti-FGFR-1 TKI | Bladder cancer | |
Erlotinib | Anti-EGFR TKI | NSCLC | EGFR mutated |
Pancreatic caner | Deletion of exon 19 | ||
Substitution in exon 21 (L858R) | |||
Everolimus | mTOR inhibitor | CNS tumors | |
Pancreatic cancer | |||
Breast cancer | |||
Renal cancer | |||
Fulvestrant | ER antagonist | Breast cancer | ER+/PR+ |
Gefitinib | Anti-EGFR TKI | NSCLC | EGFR mutated |
Deletion of exon 19 | |||
Substitution in exon 21 (L858R) | |||
Imatinib | Anti-KIT TKI | GIST | KIT+ |
Dermatofibroma protuberans | |||
Ipilimumab | Anti-CTLA-4 mAb | Colorectal cancer | |
Hepatocellular carcinoma | |||
NSCLC | |||
Melanoma | |||
Renal cancer | |||
Lapatinib | TKI: anti-EGFR, anti-HER2 | Breast cancer | ERBB2 over-expression or amplification |
Lenvatinib | TKR: anti-VEGF, VEGFR1 (FLT1), VEGFR2 (KDR) y VEGFR3 (FLT4); (FGF) FGFR1, 2, 3 y 4, PDGF, PDGFRA, KIT, RET | Endometrial cancer | |
Hepatocellular carcinoma | |||
Renal cancer | |||
Thyroid cancer | |||
Lorlatinib | TKI: anti-ALK, anti-ROS2 | NSCLC | ALK+, ROS1+ |
Necitumumab | Anti-EGFR mAb | NSCLC | EGFR+ |
Neratinib | Anti-HER2 TKI | ||
Anti-EGFR | Breast cancer | ERBB2 over-expression or amplification | |
Niraparib | PARP inhibitor | Ovarian cancer | BRCA1/2 mutations |
Fallopian tube cancer | Homologous recombination deficiency | ||
Peritoneal cancer | |||
Nivolumab | Anti-PD-1 mAb | Colorectal cancer | PD-1 |
Esophageal cancer | |||
Hepatocellular carcinoma | |||
NSCLC | |||
Melanoma | |||
Renal cancer | |||
Bladder cancer | |||
Head and Neck cancer | |||
Olparib | PARP inhibitor | Breast cancer | BRCA1/2 mutations |
Ovarian cancer | |||
Pancreatic cancer | |||
Prostate cancer | |||
Osimertinib | Anti-EGFR TKI | NSCLC | EGFR-T790M |
Palbociclib | CDK4/6 inhibitor | Breast cancer | RE+/RP+ |
Pantitumumab | Anti-EGFR mAb | Colorectal cancer | KRAS |
EGFR+ | |||
Pazopanib | TKI: Anti-VEGF, anti-PDGFR, anti-FGFR, anti-cKIT | Renal cancer | |
Soft tissues sarcoma | |||
Pembrolizumab | PD-1 inhibitor | Cervical cancer | PD-1 |
Endometrial cancer | |||
Esophageal cancer | |||
Gastric cancer | |||
Hepatocellular carcinoma | |||
NSCLC | |||
Bladder cancer | |||
Head and Neck cancer | |||
Pertuzumab | Anti-HER2 mAb | Breast cancer | ERBB2 over-expression or amplification |
Ramucirumab | Anti-VEGF mAb | Colorectal cancer | |
Esophageal cancer | |||
Gastric cancer | |||
Hepatocellular carcinoma | |||
NSCLC | |||
Regorafenib | Anti-cKIT TKI | Colorectal cancer | KIT+ |
Hepatocellular carcinoma | |||
GIST | |||
Ribociclib | CDK4/6 inhibitor | Breast cancer | ER+/PR+ |
Ripretinib | TKI: anti-KIT, anti-PDGFR | GIST | KIT+ |
Rucaparib | PARP inhibitor | Prostate cancer | BRCA1/2 mutations |
Ovarian cancer | |||
Fallopian tube cancer | |||
Peritoneal cancer | |||
Sacituzumab-Govitecan | Conjugated Ab anti-trop-2 | Breast cancer | RE- RP- HER2- |
Selpercatinib | Kinase inhibitor | NSCLC | RET+ |
Thyroid cancer | |||
Sorafenib | Multi-kinase inhibitor: anti-PDGFR, VEGFR, cKIT, TKR | Renal cancer | |
Hepatocellular carcinoma | |||
Thyroid cancer | |||
Sunitinib | Multi-kinase inhibitor: anti-PDGFR, VEGFR, cKIT, TKR | Renal cancer | |
Pancreatic cancer | |||
GIST | |||
Tamoxifeno | SERM | Breast cancer | ER+/PR+ |
Talazoparib | PARP inhibitor | Breast cancer | BRCA1/2 mutations |
Temsirolimus | mTOR inhibitor | Renal cancer | |
Trametinib | BRAF inhibitor | NSCLC | BRAF-V600E, V600K |
Thyroid cancer | |||
Melanoma | |||
Trastuzumab | Anti-HER2 mAb | Gastric cancer | ERBB2 over-expression of amplification |
Gastro-esophageal junction cancer | |||
Breast cancer | |||
Trastuzumab-Deruxtecan | Anti-HER2 conjugated Ab | Breast cancer | ERBB2 over-expression of amplification |
Trastuzumab-Emtansine | Anti-HER2 conjugated Ab | Breast cancer | ERBB2 over-expression of amplification |
Tucatinib | Anti-HER2 TKI | Breast cancer | ERBB2 over-expression of amplification |
Vandetanib | TKI: anti-VEGF, anti-EGFR | Thyroid cancer | EGFR+ |
Vemurafenib | BRAF inhibitor | Melanoma | BRAF-V600E |
Abbreviations: mAb, monoclonal antibody; ALK, anaplastic lymphoma kinase; CDK, cyclin-dependent kinase; CTLA-4, cytotoxic lymphocyte antigen-4; EGFR, epidermal growth factor receptor; FGFR, fibroblast growth factor receptor; GIST, gastrointestinal stroma tumor; mTOR, target of rapamycine in mammal cells; NSCLC, non-small cell lung carcinoma; PARP, poli (ADP-ribose) polimerase; PD-1, programmed death protein-1; PDGFR, platelet-derived growth factor receptor; PD-L1, programmed death ligand-1; ER, estrogen receptor; PR, progesterone receptor; TKR, tyrosine kinase receptors; SERM, selective estrogen receptor modulator; TKI, tyrosine kinase inhibitor; VEGFR, vascular endothelial growth factor receptor. Modified from Ref. [ 127 ].
Most drugs classified as targeted therapies form part of 2 large groups: small molecules and mAbs. The former are defined as compounds of low molecular weight (<900 Daltons) that act upon entering the cell. 120 Targets of these compounds are cell cycle regulatory proteins, proapoptotic proteins, or DNA repair proteins. These drugs are indicated based on histological diagnosis, as well as molecular tests. In this group there are multi-kinase inhibitors (RTKs) and tyrosine kinase inhibitors (TKIs), like sunitinib, sorafenib, and imatinib; cyclin-dependent kinase (CDK) inhibitors, such as palbociclib, ribociclib and abemaciclib; poli (ADP-ribose) polimerase inhibitors (PARPs), like olaparib and talazoparib; and selective small-molecule inhibitors, like ALK and ROS1. 122
As for mAbs, they are protein molecules that act on membrane receptors or extracellular proteins by interrupting the interaction between ligands and receptors, in such a way that they reduce cell replication and induce cytostasis. Among the most widely used mAbs in oncology we have: trastuzumab, a drug directed against the receptor for human epidermal growth factor-2 (HER2), which is overexpressed in a subgroup of patients with breast and gastric cancer; and bevacizumab, that blocks vascular endothelial growth factor and is used in patients with colorectal cancer, cervical cancer, and ovarian cancer. Other mAbs approved by the FDA include pembolizumab, atezolizumab, nivolumab, avelumab, ipilimumab, durvalumab, and cemiplimab. These drugs require expression of response biomarkers, such as PD-1 and PD-L1, and must also have several resistance biomarkers, such as the expression of EGFR, the loss of PTEN, and alterations in beta-catenin. 123
Because cancer is such a diverse disease, it is fundamental to have precise diagnostic methods that allow us to identify the most adequate therapy. Currently, basic immunohistochemistry is complemented with neoplastic molecular profiles to determine a more accurate diagnosis, and it is probable that in the near future cancer treatments will be based exclusively on molecular profiles. In this regard, it is worth mentioning that the use of targeted therapy depends on the existence of specific biomarkers that indicate if the patient will be susceptible to the effects of the drug or not. Thus, the importance of underlining that not all patients are susceptible to receive targeted therapy. In certain neoplasms, therapeutic targets are expressed in less than 5% of the diagnosed population, hindering a more extended use of certain drugs.
The identification of biomarkers and the use of new generation sequencing on tumor cells has shown predictive and prognostic relevance. Likewise, mutation analysis has allowed monitoring of tumor clone evolution, providing information on changes in canonic gene sequences, such as TP53, GATA3, PIK3CA, AKT1, and ERBB2; infrequent somatic mutations developed after primary treatments, like SWI-SNF and JAK2-STAT3; or acquired drug resistance mutations such as ESR1. 124 The study of mutations is vital; in fact, many of them already have specific therapeutic indications, which have helped select adequate treatments. 125
There is no doubt that molecular targeted therapy is one of the main pillars of precision medicine. However, it faces significant problems that often hinder obtaining better results. Among these, there is intratumor heterogeneity and differences between the primary tumor and metastatic sites, as well as intrinsic and acquired resistance to these therapies, the mechanisms of which include the presence of heterogeneous subclones, DNA hypermethylation, histone acetylation, and interruption of mRNA degradation and translation processes. 126 Nonetheless, beyond the obstacles facing molecular targeted therapy from a biological and methodological point of view, in the real world, access to genomic testing and specific drugs continues to be an enormous limitation, in such a way that strategies must be designed in the future for precision medicine to be possible on a global scale.
Cell Therapy
Another improvement in cancer treatment is the use of cell therapy, that is, the use of specific cells as therapeutic agents. This clinical procedure has 2 modalities: the first consists of replacing and regenerating functional cells in a specific tissue by means of stem/progenitor cells of a certain kind, 43 while the second uses immune cells as effectors to eliminate malignant cells. 127
Regarding the first type, we must emphasize the development of cell therapy based on hematopoietic stem and progenitor cells. 128 For over 50 years, hematopoietic cell transplants have been used to treat a variety of hematologic neoplasms (different forms of leukemia and lymphoma). Today, it is one of the most successful examples of cell therapy, including innovative modalities, such as haploidentical transplants, 129 as well as application of stem cells expanded ex vivo . 130 There are also therapies that have used immature cells that form part of the TME, such as MSCs. The replication potential and cytokine secretion capacity of these cells make them an excellent option for this type of treatment. 131 Neural stem cells can also be manipulated to produce and secrete apoptotic factors, and when these cells are incorporated into primary neural tumors, they cause a certain degree of regression. They can even be transfected with genes that encode for oncolytic enzymes capable of inducing regression of glioblastomas. 132
With respect to cell therapy using immune cells, several research groups have manipulated cells associated with tumors to make them effector cells and thus improve the efficacy and specificity of the antitumor treatment. PB leckocytes cultured in the presence of IL-2 to obtain activated lymphocytes, in combination with IL-2 administration, have been used in antitumor clinical protocols. Similarly, infiltrating lymphocytes from tumors with antitumor activity have been used and can be expanded ex vivo with IL-2. These lymphocyte populations have been used in immunomodulatory therapies in melanoma, and pancreatic and kidney tumors, producing a favorable response in treated patients. 133 NK cells and macrophages have also been used in immunotherapy, although with limited results. 134 , 135
One of the cell therapies with better projection today is the use of CAR-T cells. This strategy combines 2 forms of advanced therapy: cell therapy and gene therapy. It involves the extraction of T cells from the cancer patient, which are genetically modified in vitro to express cell surface receptors that will recognize antigens on the surface of tumor cells. The modified T cells are then reintroduced in the patient to aid in an exacerbated immune response that leads to eradication of the tumor cells ( Figure 4 ). Therapy with CAR-T cells has been used successfully in the treatment of some types of leukemia, lymphoma, and myeloma, producing complete responses in patients. 136

CAR-T cell therapy. (A) T lymphocytes obtained from cancer patients are genetically manipulated to produce CAR-T cells that recognize tumor cells in a very specific manner. (B) Interaction between CAR molecule and tumor antigen. CAR molecule is a receptor that results from the fusion between single-chain variable fragments (scFv) from a monoclonal antibody and one or more intracellular signaling domains from the T-cell receptor. CD3ζ, CD28 and 4-1BB correspond to signaling domains on the CAR molecule.
Undoubtedly, CAR-T cell therapy has been truly efficient in the treatment of various types of neoplasms. However, this therapeutic strategy can also have serious side effects, such as release of cytokines into the bloodstream, which can cause different symptoms, from high fever to multiorgan failure, and even neurotoxicity, leading to cerebral edema in many cases. 137 Adequate control of these side effects is an important medical challenge. Several research groups are trying to improve CAR-T cell therapy through various approaches, including production of CAR-T cells directed against a wider variety of tumor cell-specific antigens that are able to attack different types of tumors, and the identification of more efficient types of T lymphocytes. Furthermore, producing CAR-T cells from a single donor that may be used in the treatment of several patients would reduce the cost of this sort of personalized cell therapy. 136
Achieving wider use of cell therapy in oncologic diseases is an important challenge that requires solving various issues. 138 One is intratumor cell heterogeneity, including malignant subclones and the various components of the TME, which results in a wide profile of membrane protein expression that complicates finding an ideal tumor antigen that allows specific identification (and elimination) of malignant cells. Likewise, structural organization of the TME challenges the use of cell therapy, as administration of cell vehicles capable of recognizing malignant cells might not be able to infiltrate the tumor. This results from low expression of chemokines in tumors and the presence of a dense fibrotic matrix that compacts the inner tumor mass and avoids antitumor cells from infiltrating and finding malignant target cells.
Further Challenges in the 21st Century
Beyond the challenges regarding oncologic biomedical research, the 21 st century is facing important issues that must be solved as soon as possible if we truly wish to gain significant ground in our fight against cancer. Three of the most important have to do with prevention, early diagnosis, and access to oncologic medication and treatment.
Prevention and Early Diagnosis
Prevention is the most cost-effective strategy in the long term, both in low and high HDI nations. Data from countries like the USA indicate that between 40-50% of all types of cancer are preventable through potentially modifiable factors (primary prevention), such as use of tobacco and alcohol, diet, physical activity, exposure to ionizing radiation, as well as prevention of infection through access to vaccination, and by reducing exposure to environmental pollutants, such as pesticides, diesel exhaust particles, solvents, etc. 74 , 84 Screening, on the other hand, has shown great effectiveness as secondary prevention. Once population-based screening programs are implemented, there is generally an initial increase in incidence; however, in the long term, a significant reduction occurs not only in incidence rates, but also in mortality rates due to detection of early lesions and timely and adequate treatment.
A good example is colon cancer. There are several options for colon cancer screening, such as detection of fecal occult blood, fecal immunohistochemistry, flexible sigmoidoscopy, and colonoscopy, 139 , 140 which identify precursor lesions (polyp adenomas) and allow their removal. Such screening has allowed us to observe 3 patterns of incidence and mortality for colon cancer between the years 2000 and 2010: on one hand, an increase in incidence and mortality in countries with low to middle HDI, mainly countries in Asia, South America, and Eastern Europe; on the other hand, an increase in incidence and a fall in mortality in countries with very high HDI, such as Canada, the United Kingdom, Denmark, and Singapore; and finally a fall in incidence and mortality in countries like the USA, Japan, and France. The situation in South America and Asia seems to reflect limitations in medical infrastructure and a lack of access to early detection, 141 while the patterns observed in developed countries reveal the success, even if it may be partial, of that which can be achieved by well-structured prevention programs.
Another example of success, but also of strong contrast, is cervical cancer. The discovery of the human papilloma virus (HPV) as the causal agent of cervical cancer brought about the development of vaccines and tests to detect oncogenic genotypes, which modified screening recommendations and guidelines, and allowed several developed countries to include the HPV vaccine in their national vaccination programs. Nevertheless, the outlook is quite different in other areas of the world. Eighty percent of the deaths by cervical cancer reported in 2018 occurred in low-income nations. This reveals the urgency of guaranteeing access to primary and secondary prevention (vaccination and screening, respectively) in these countries, or else it will continue to be a serious public health problem in spite of its preventability.
Screening programs for other neoplasms, such as breast, prostate, lung, and thyroid cancer have shown outlooks that differ from those just described, because, among other reasons, these neoplasms are highly diverse both biologically and clinically. Another relevant issue is the overdiagnosis of these neoplasms, that is, the diagnosis of disease that would not cause symptoms or death in the patient. 142 It has been calculated that 25% of breast cancer (determined by mammogram), 50–60% of prostate cancer (determined by PSA), and 13–25% of lung cancer (determined by CT) are overdiagnosed. 142 Thus, it is necessary to improve the sensitivity and specificity of screening tests. In this respect, knowledge provided by the biology of cancer and “omic” sciences offers a great opportunity to improve screening and prevention strategies. All of the above shows that prevention and early diagnosis are the foundations in the fight against cancer, and it is essential to continue to implement broader screening programs and better detection methods.
Global Equity in Oncologic Treatment
Progress in cancer treatment has considerably increased the number of cancer survivors. Nevertheless, this tendency is evident only in countries with a very solid economy. Indeed, during the past 30 years, cancer mortality rates have increased 30% worldwide. 143 Global studies indicate that close to 70% of cancer deaths in the world occur in nations of low to middle income. But even in high-income countries, there are sectors of society that are more vulnerable and have less access to cancer treatments. 144 Cancer continues to be a disease of great social inequality.
In Europe, the differences in access to cancer treatment are highly marked. These treatments are more accessible in Western Europe than in its Eastern counterpart. 145 Furthermore, highly noticeable differences between high-income countries have been detected in the cost of cancer drugs. 146 It is interesting to note that in many of these cases, treatment is too costly and the clinical benefit only marginal. Thus, the importance of these problems being approached by competent national, regional, and global authorities, because if these new drugs and therapeutic programs are not accessible to the majority, progress in biomedical, clinical and epidemiological research will have a limited impact in our fight against cancer. We must not forget that health is a universal right, from which low HDI countries must not be excluded, nor vulnerable populations in nations with high HDI. The participation of a well-informed society will also be fundamental to achieve a global impact, as today we must fight not only against the disease, but also against movements and ideas (such as the anti-vaccine movement and the so-called miracle therapies) that can block the medical battle against cancer.
Final Comments
From the second half of the 20th century to the present day, progress in our knowledge about the origin and development of cancer has been extraordinary. We now understand cancer in detail in genomic, molecular, cellular, and physiological terms, and this knowledge has had a significant impact in the clinic. There is no doubt that a patient who is diagnosed today with a type of cancer has a better prospect than a patient diagnosed 20 or 50 years ago. However, we are still far from winning the war against cancer. The challenges are still numerous. For this reason, oncologic biomedical research must be a worldwide priority. Likewise, one of the fundamental challenges for the coming decades must be to reduce unequal access to health services in areas of low- to middle income, and in populations that are especially vulnerable, as well as continue improving prevention programs, including public health programs to reduce exposure to environmental chemicals and improve diet and physical activity in the general population. 74 , 84 Fostering research and incorporation of new technological resources, particularly in less privileged nations, will play a key role in our global fight against cancer.
Declaration of Conflicting Interests: The author(s) declared no potential conflicts of interest with respect to the research, authorship, and/or publication of this article.
Funding: The author(s) received no financial support for the research, authorship, and/or publication of this article.
Hector Mayani https://orcid.org/0000-0002-2483-3782
What Is Cancer?
Get email updates from NCI on cancer health information, news, and other topics
Get email updates from NCI
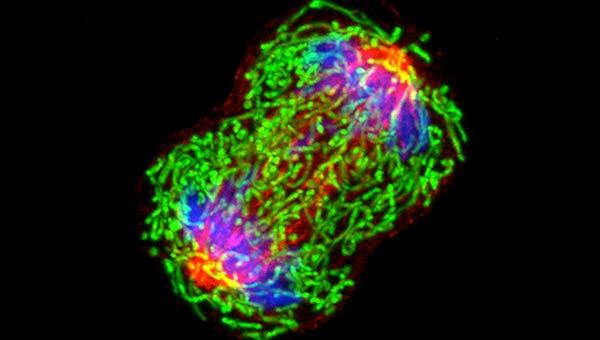
A dividing breast cancer cell.
The Definition of Cancer
Cancer is a disease in which some of the body’s cells grow uncontrollably and spread to other parts of the body.
Cancer can start almost anywhere in the human body, which is made up of trillions of cells. Normally, human cells grow and multiply (through a process called cell division) to form new cells as the body needs them. When cells grow old or become damaged, they die, and new cells take their place.
Sometimes this orderly process breaks down, and abnormal or damaged cells grow and multiply when they shouldn’t. These cells may form tumors, which are lumps of tissue. Tumors can be cancerous or not cancerous ( benign ).
Cancerous tumors spread into, or invade, nearby tissues and can travel to distant places in the body to form new tumors (a process called metastasis ). Cancerous tumors may also be called malignant tumors. Many cancers form solid tumors, but cancers of the blood, such as leukemias , generally do not.
Benign tumors do not spread into, or invade, nearby tissues. When removed, benign tumors usually don’t grow back, whereas cancerous tumors sometimes do. Benign tumors can sometimes be quite large, however. Some can cause serious symptoms or be life threatening, such as benign tumors in the brain.
Differences between Cancer Cells and Normal Cells
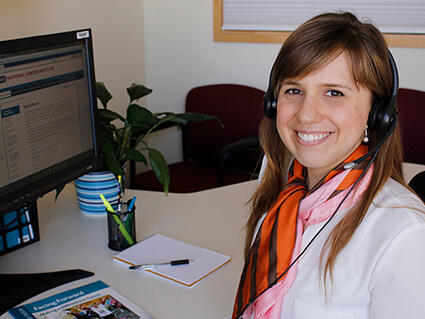
Get Answers >
Have questions? Connect with a Cancer Information Specialist for answers.
Cancer cells differ from normal cells in many ways. For instance, cancer cells:
- grow in the absence of signals telling them to grow. Normal cells only grow when they receive such signals.
- ignore signals that normally tell cells to stop dividing or to die (a process known as programmed cell death , or apoptosis ).
- invade into nearby areas and spread to other areas of the body. Normal cells stop growing when they encounter other cells, and most normal cells do not move around the body.
- tell blood vessels to grow toward tumors. These blood vessels supply tumors with oxygen and nutrients and remove waste products from tumors.
- hide from the immune system . The immune system normally eliminates damaged or abnormal cells.
- trick the immune system into helping cancer cells stay alive and grow. For instance, some cancer cells convince immune cells to protect the tumor instead of attacking it.
- accumulate multiple changes in their chromosomes , such as duplications and deletions of chromosome parts. Some cancer cells have double the normal number of chromosomes.
- rely on different kinds of nutrients than normal cells. In addition, some cancer cells make energy from nutrients in a different way than most normal cells. This lets cancer cells grow more quickly.
Many times, cancer cells rely so heavily on these abnormal behaviors that they can’t survive without them. Researchers have taken advantage of this fact, developing therapies that target the abnormal features of cancer cells. For example, some cancer therapies prevent blood vessels from growing toward tumors , essentially starving the tumor of needed nutrients.
How Does Cancer Develop?
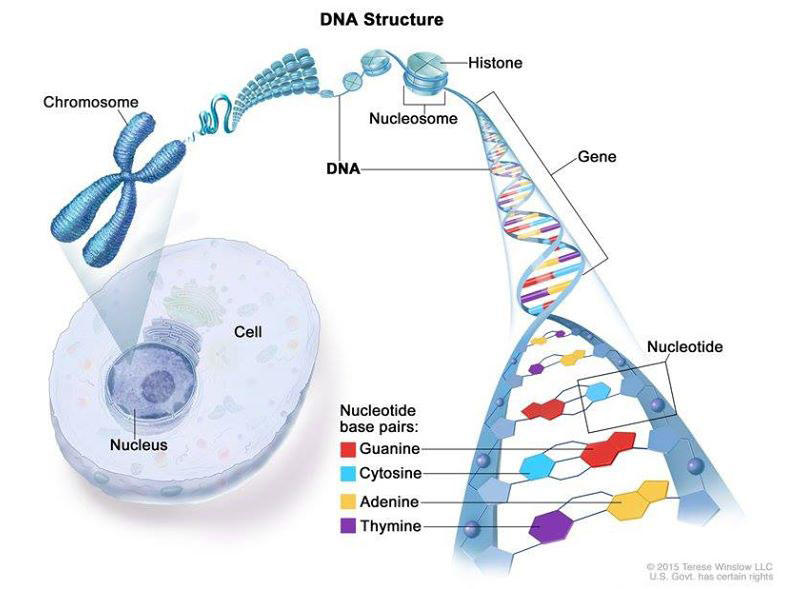
Cancer is caused by certain changes to genes, the basic physical units of inheritance. Genes are arranged in long strands of tightly packed DNA called chromosomes.
Cancer is a genetic disease—that is, it is caused by changes to genes that control the way our cells function, especially how they grow and divide.
Genetic changes that cause cancer can happen because:
- of errors that occur as cells divide.
- of damage to DNA caused by harmful substances in the environment, such as the chemicals in tobacco smoke and ultraviolet rays from the sun. (Our Cancer Causes and Prevention section has more information.)
- they were inherited from our parents.
The body normally eliminates cells with damaged DNA before they turn cancerous. But the body’s ability to do so goes down as we age. This is part of the reason why there is a higher risk of cancer later in life.
Each person’s cancer has a unique combination of genetic changes. As the cancer continues to grow, additional changes will occur. Even within the same tumor, different cells may have different genetic changes.
Fundamentals of Cancer
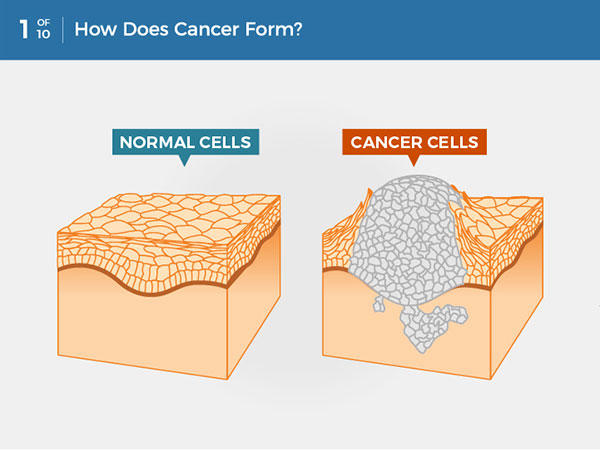
Cancer is a disease caused when cells divide uncontrollably and spread into surrounding tissues.
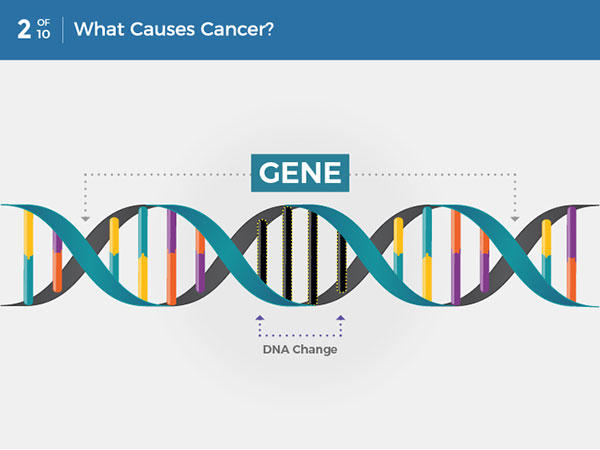
Cancer is caused by changes to DNA. Most cancer-causing DNA changes occur in sections of DNA called genes. These changes are also called genetic changes.
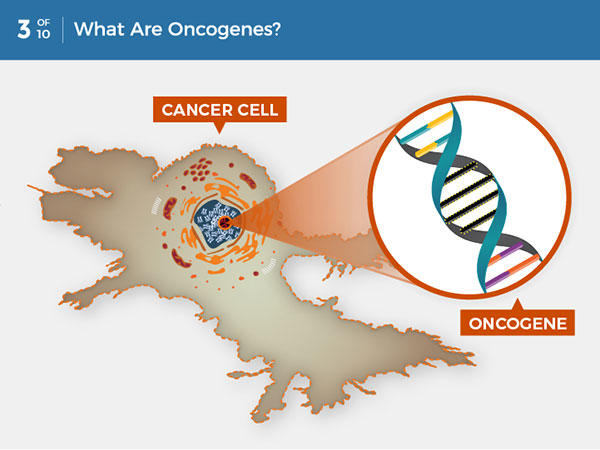
A DNA change can cause genes involved in normal cell growth to become oncogenes. Unlike normal genes, oncogenes cannot be turned off, so they cause uncontrolled cell growth.
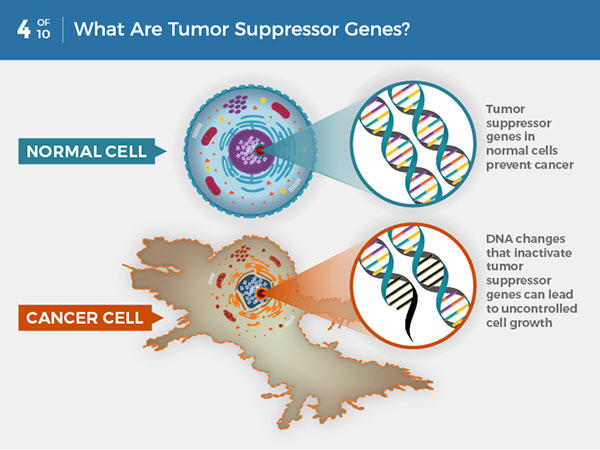
In normal cells, tumor suppressor genes prevent cancer by slowing or stopping cell growth. DNA changes that inactivate tumor suppressor genes can lead to uncontrolled cell growth and cancer.
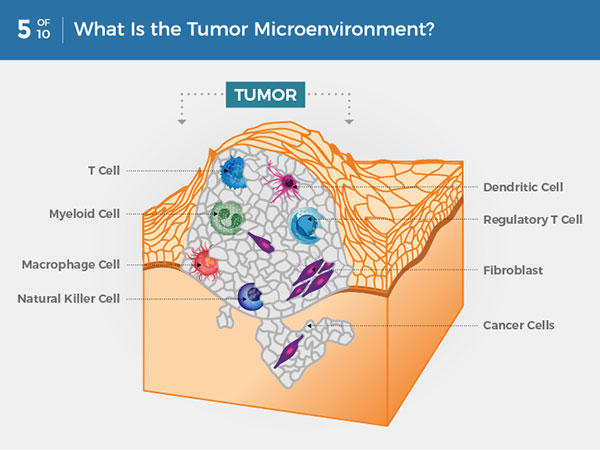
Within a tumor, cancer cells are surrounded by a variety of immune cells, fibroblasts, molecules, and blood vessels—what’s known as the tumor microenvironment. Cancer cells can change the microenvironment, which in turn can affect how cancer grows and spreads.
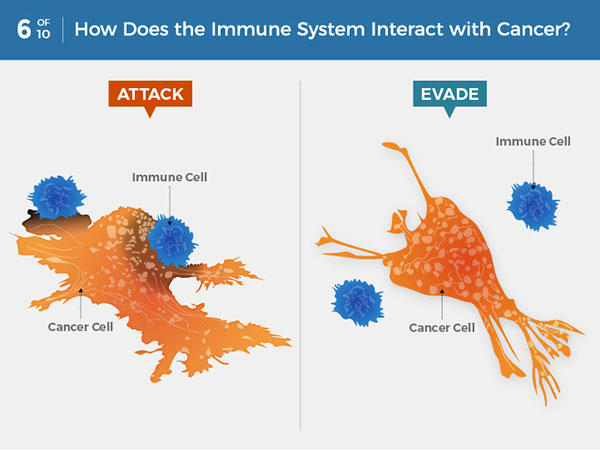
Immune system cells can detect and attack cancer cells. But some cancer cells can avoid detection or thwart an attack. Some cancer treatments can help the immune system better detect and kill cancer cells.
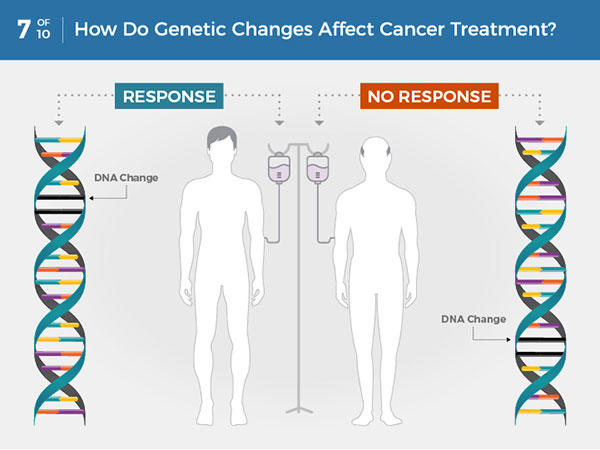
Each person’s cancer has a unique combination of genetic changes. Specific genetic changes may make a person’s cancer more or less likely to respond to certain treatments.
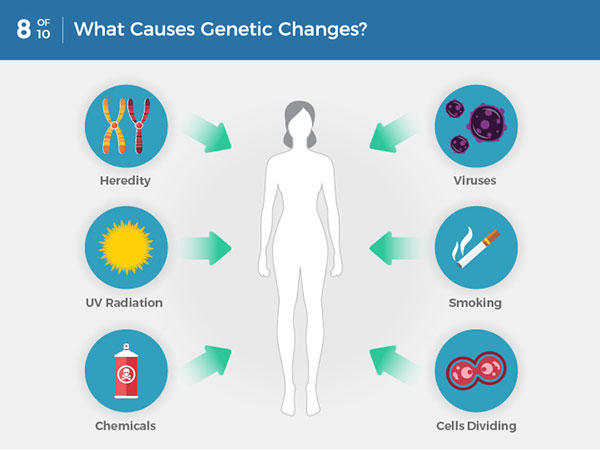
Genetic changes that cause cancer can be inherited or arise from certain environmental exposures. Genetic changes can also happen because of errors that occur as cells divide.
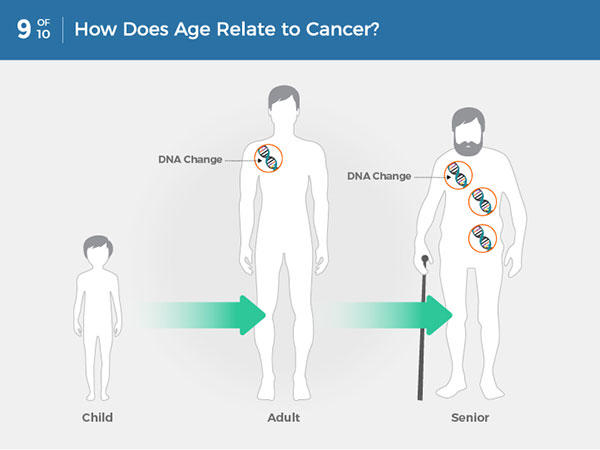
Most often, cancer-causing genetic changes accumulate slowly as a person ages, leading to a higher risk of cancer later in life.
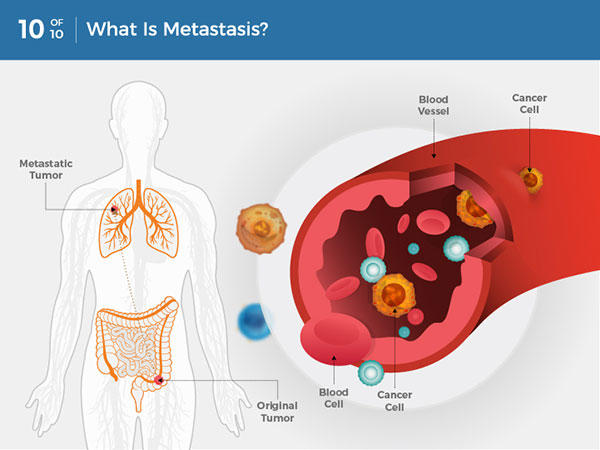
Cancer cells can break away from the original tumor and travel through the blood or lymph system to distant locations in the body, where they exit the vessels to form additional tumors. This is called metastasis.
Types of Genes that Cause Cancer
The genetic changes that contribute to cancer tend to affect three main types of genes— proto-oncogenes , tumor suppressor genes , and DNA repair genes. These changes are sometimes called “drivers” of cancer.
Proto-oncogenes are involved in normal cell growth and division. However, when these genes are altered in certain ways or are more active than normal, they may become cancer-causing genes (or oncogenes), allowing cells to grow and survive when they should not.
Tumor suppressor genes are also involved in controlling cell growth and division. Cells with certain alterations in tumor suppressor genes may divide in an uncontrolled manner.
DNA repair genes are involved in fixing damaged DNA. Cells with mutations in these genes tend to develop additional mutations in other genes and changes in their chromosomes, such as duplications and deletions of chromosome parts. Together, these mutations may cause the cells to become cancerous.
As scientists have learned more about the molecular changes that lead to cancer, they have found that certain mutations commonly occur in many types of cancer. Now there are many cancer treatments available that target gene mutations found in cancer . A few of these treatments can be used by anyone with a cancer that has the targeted mutation, no matter where the cancer started growing .
When Cancer Spreads
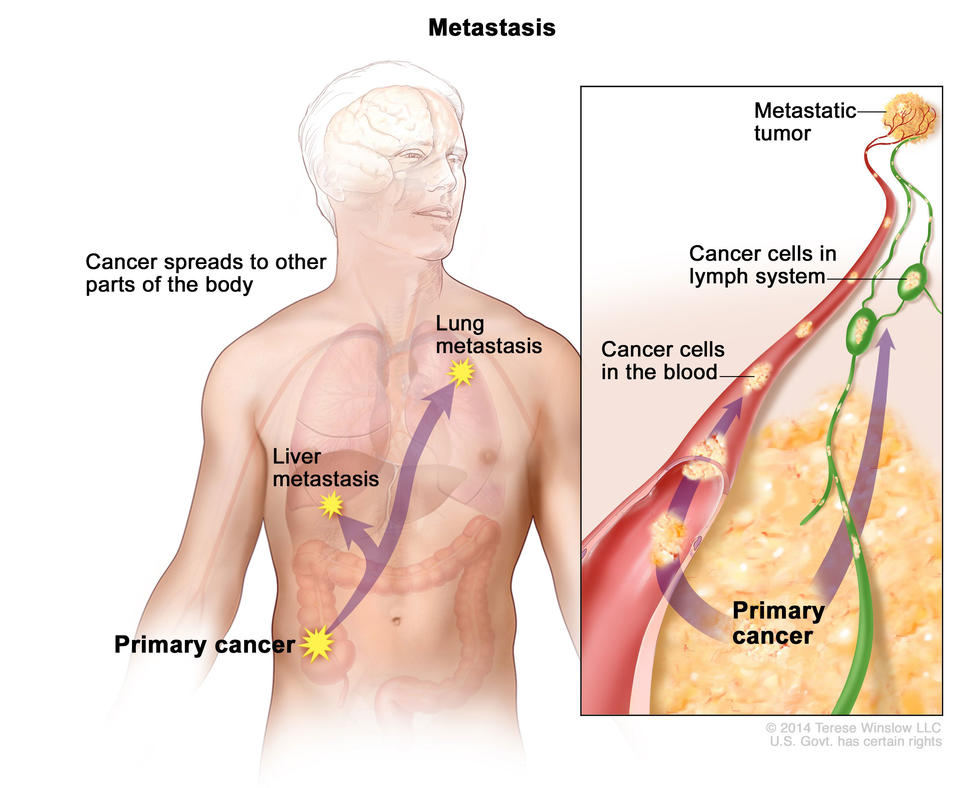
In metastasis, cancer cells break away from where they first formed and form new tumors in other parts of the body.
A cancer that has spread from the place where it first formed to another place in the body is called metastatic cancer. The process by which cancer cells spread to other parts of the body is called metastasis.
Metastatic cancer has the same name and the same type of cancer cells as the original, or primary, cancer. For example, breast cancer that forms a metastatic tumor in the lung is metastatic breast cancer, not lung cancer.
Under a microscope, metastatic cancer cells generally look the same as cells of the original cancer. Moreover, metastatic cancer cells and cells of the original cancer usually have some molecular features in common, such as the presence of specific chromosome changes.
In some cases, treatment may help prolong the lives of people with metastatic cancer. In other cases, the primary goal of treatment for metastatic cancer is to control the growth of the cancer or to relieve symptoms it is causing. Metastatic tumors can cause severe damage to how the body functions, and most people who die of cancer die of metastatic disease.
Tissue Changes that Are Not Cancer
Not every change in the body’s tissues is cancer. Some tissue changes may develop into cancer if they are not treated, however. Here are some examples of tissue changes that are not cancer but, in some cases, are monitored because they could become cancer:
- Hyperplasia occurs when cells within a tissue multiply faster than normal and extra cells build up. However, the cells and the way the tissue is organized still look normal under a microscope. Hyperplasia can be caused by several factors or conditions, including chronic irritation.
- Dysplasia is a more advanced condition than hyperplasia. In dysplasia, there is also a buildup of extra cells. But the cells look abnormal and there are changes in how the tissue is organized. In general, the more abnormal the cells and tissue look, the greater the chance that cancer will form. Some types of dysplasia may need to be monitored or treated, but others do not. An example of dysplasia is an abnormal mole (called a dysplastic nevus ) that forms on the skin. A dysplastic nevus can turn into melanoma, although most do not.
- Carcinoma in situ is an even more advanced condition. Although it is sometimes called stage 0 cancer, it is not cancer because the abnormal cells do not invade nearby tissue the way that cancer cells do. But because some carcinomas in situ may become cancer, they are usually treated.
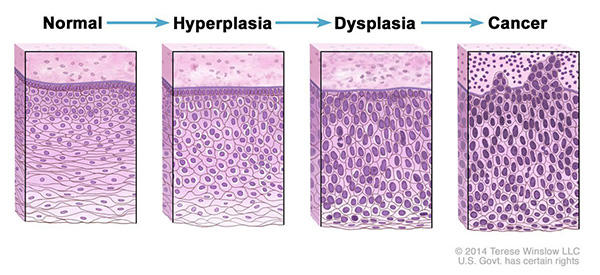
Normal cells may become cancer cells. Before cancer cells form in tissues of the body, the cells go through abnormal changes called hyperplasia and dysplasia. In hyperplasia, there is an increase in the number of cells in an organ or tissue that appear normal under a microscope. In dysplasia, the cells look abnormal under a microscope but are not cancer. Hyperplasia and dysplasia may or may not become cancer.
Types of Cancer
There are more than 100 types of cancer. Types of cancer are usually named for the organs or tissues where the cancers form. For example, lung cancer starts in the lung, and brain cancer starts in the brain. Cancers also may be described by the type of cell that formed them, such as an epithelial cell or a squamous cell .
You can search NCI’s website for information on specific types of cancer based on the cancer’s location in the body or by using our A to Z List of Cancers . We also have information on childhood cancers and cancers in adolescents and young adults .
Here are some categories of cancers that begin in specific types of cells:
Carcinomas are the most common type of cancer. They are formed by epithelial cells, which are the cells that cover the inside and outside surfaces of the body. There are many types of epithelial cells, which often have a column-like shape when viewed under a microscope.
Carcinomas that begin in different epithelial cell types have specific names:
Adenocarcinoma is a cancer that forms in epithelial cells that produce fluids or mucus. Tissues with this type of epithelial cell are sometimes called glandular tissues. Most cancers of the breast, colon, and prostate are adenocarcinomas.
Basal cell carcinoma is a cancer that begins in the lower or basal (base) layer of the epidermis, which is a person’s outer layer of skin.
Squamous cell carcinoma is a cancer that forms in squamous cells, which are epithelial cells that lie just beneath the outer surface of the skin. Squamous cells also line many other organs, including the stomach, intestines, lungs, bladder, and kidneys. Squamous cells look flat, like fish scales, when viewed under a microscope. Squamous cell carcinomas are sometimes called epidermoid carcinomas.
Transitional cell carcinoma is a cancer that forms in a type of epithelial tissue called transitional epithelium, or urothelium. This tissue, which is made up of many layers of epithelial cells that can get bigger and smaller, is found in the linings of the bladder, ureters, and part of the kidneys (renal pelvis), and a few other organs. Some cancers of the bladder, ureters, and kidneys are transitional cell carcinomas.
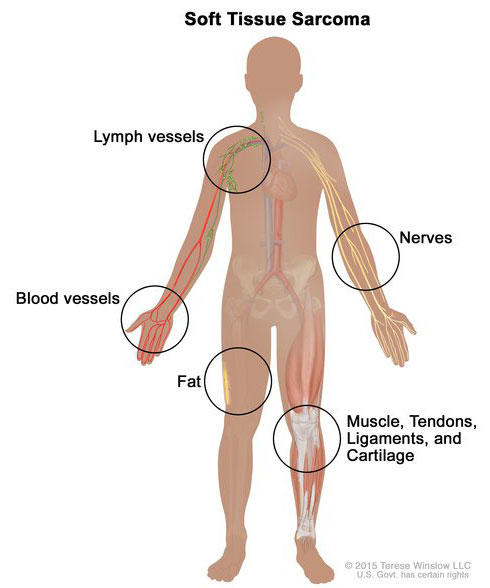
Soft tissue sarcoma forms in soft tissues of the body, including muscle, tendons, fat, blood vessels, lymph vessels, nerves, and tissue around joints.
Sarcomas are cancers that form in bone and soft tissues, including muscle, fat, blood vessels, lymph vessels , and fibrous tissue (such as tendons and ligaments).
Osteosarcoma is the most common cancer of bone. The most common types of soft tissue sarcoma are leiomyosarcoma , Kaposi sarcoma , malignant fibrous histiocytoma , liposarcoma , and dermatofibrosarcoma protuberans .
Our page on soft tissue sarcoma has more information.
Cancers that begin in the blood-forming tissue of the bone marrow are called leukemias. These cancers do not form solid tumors. Instead, large numbers of abnormal white blood cells (leukemia cells and leukemic blast cells) build up in the blood and bone marrow, crowding out normal blood cells. The low level of normal blood cells can make it harder for the body to get oxygen to its tissues, control bleeding, or fight infections.
There are four common types of leukemia, which are grouped based on how quickly the disease gets worse (acute or chronic) and on the type of blood cell the cancer starts in (lymphoblastic or myeloid). Acute forms of leukemia grow quickly and chronic forms grow more slowly.
Our page on leukemia has more information.
Lymphoma is cancer that begins in lymphocytes (T cells or B cells). These are disease-fighting white blood cells that are part of the immune system. In lymphoma, abnormal lymphocytes build up in lymph nodes and lymph vessels, as well as in other organs of the body.
There are two main types of lymphoma:
Hodgkin lymphoma – People with this disease have abnormal lymphocytes that are called Reed-Sternberg cells. These cells usually form from B cells.
Non-Hodgkin lymphoma – This is a large group of cancers that start in lymphocytes. The cancers can grow quickly or slowly and can form from B cells or T cells.
Our page on lymphoma has more information.
Multiple Myeloma
Multiple myeloma is cancer that begins in plasma cells , another type of immune cell. The abnormal plasma cells, called myeloma cells, build up in the bone marrow and form tumors in bones all through the body. Multiple myeloma is also called plasma cell myeloma and Kahler disease.
Our page on multiple myeloma and other plasma cell neoplasms has more information.
Melanoma is cancer that begins in cells that become melanocytes, which are specialized cells that make melanin (the pigment that gives skin its color). Most melanomas form on the skin, but melanomas can also form in other pigmented tissues, such as the eye.
Our pages on skin cancer and intraocular melanoma have more information.
Brain and Spinal Cord Tumors
There are different types of brain and spinal cord tumors. These tumors are named based on the type of cell in which they formed and where the tumor first formed in the central nervous system. For example, an astrocytic tumor begins in star-shaped brain cells called astrocytes , which help keep nerve cells healthy. Brain tumors can be benign (not cancer) or malignant (cancer).
Our page on brain and spinal cord tumors has more information.
Other Types of Tumors
Germ cell tumors.
Germ cell tumors are a type of tumor that begins in the cells that give rise to sperm or eggs. These tumors can occur almost anywhere in the body and can be either benign or malignant.
Our page of cancers by body location/system includes a list of germ cell tumors with links to more information.
Neuroendocrine Tumors
Neuroendocrine tumors form from cells that release hormones into the blood in response to a signal from the nervous system. These tumors, which may make higher-than-normal amounts of hormones, can cause many different symptoms. Neuroendocrine tumors may be benign or malignant.
Our definition of neuroendocrine tumors has more information.
Carcinoid Tumors
Carcinoid tumors are a type of neuroendocrine tumor. They are slow-growing tumors that are usually found in the gastrointestinal system (most often in the rectum and small intestine). Carcinoid tumors may spread to the liver or other sites in the body, and they may secrete substances such as serotonin or prostaglandins, causing carcinoid syndrome .
Our page on gastrointestinal neuroendocrine tumors has more information.
If you're seeing this message, it means we're having trouble loading external resources on our website.
If you're behind a web filter, please make sure that the domains *.kastatic.org and *.kasandbox.org are unblocked.
To log in and use all the features of Khan Academy, please enable JavaScript in your browser.
AP®︎/College Biology
Course: ap®︎/college biology > unit 4.
- Cell cycle control
- Cell cycle checkpoints
- Cell cycle regulators
- Loss of cell cycle control in cancer
Cancer and the cell cycle
- Regulation of cell cycle

Introduction
What’s wrong with cancer cells, how cancer develops, cell cycle regulators and cancer, tumor suppressors.
- Two spontaneous mutations affecting the two different alleles may occur in the same cell over time.
- A person may inherit one "bad" allele of the tumor suppressor from a parent, then lose activity of the other allele through a spontaneous mutation.
Check your understanding: viruses and cancer
- (Choice A) E6 activates p53 A E6 activates p53
- (Choice B) E6 makes p53 bind DNA more strongly B E6 makes p53 bind DNA more strongly
- (Choice C) E6 triggers apoptosis through p53 C E6 triggers apoptosis through p53
- (Choice D) E6 marks p53 for degradation (destruction) D E6 marks p53 for degradation (destruction)
- If E6 made p53 more active, caused it to bind DNA more strongly, or triggered apoptosis, these changes would tend to decrease the chances of cancer development.
- In contrast, if E6 marked p53 for degradation, p53 activity would decrease (because protein levels would be low) and the chances of uncontrolled cell division would increase, potentially leading to cancer.
Attribution:
Works cited:.
- Hanahan, D. and Weinberg, R. A. (2011). Hallmarks of cancer: the next generation. Cell , 144 (5), 647. http://dx.doi.org/10.1016/j.cell.2011.02.013.647
- Hanahan, D. and Weinberg, R. A. (2011). Hallmarks of cancer: the next generation. Cell , 144 (5), 649. http://dx.doi.org/10.1016/j.cell.2011.02.013 .
- Bartlett, Zane. (2014, November 14). The Hayflick limit. In The Embryo Project Encyclopedia . Retrieved from https://embryo.asu.edu/pages/hayflick-limit .
- Hanahan, D. and Weinberg, R. A. (2011). Hallmarks of cancer: the next generation. Cell , 144 (5), 651. http://dx.doi.org/10.1016/j.cell.2011.02.013.647
- Hanahan, D. and Weinberg, R. A. (2011). Hallmarks of cancer: the next generation. Cell , 144 (5), 647, 659-660. http://dx.doi.org/10.1016/j.cell.2011.02.013.647
- Vogelstein, B. and Kinzler, K. W. (2004). Cancer genes and the pathways they control. Nature Medicine , 10 , 790. http://dx.doi.org/10.1038/nm1087 .
- Reece, J. B., Urry, L. A., Cain, M. L., Wasserman, S. A., Minorsky, P. V., and Jackson, R. B. (2011). The cell cycle. In Campbell biology (10th ed.). San Francisco, CA: Pearson, 247
- Vogelstein, B. and Kinzler, K. W. (2004). Cancer genes and the pathways they control. Nature Medicine , 10 , 789. http://dx.doi.org/10.1038/nm1087 .
- Hanahan, D. and Weinberg, R. A. (2011). Hallmarks of cancer: the next generation. Cell , 144 (5), 658. http://dx.doi.org/10.1016/j.cell.2011.02.013.647
- Lodish, H., Berk, A., Zipursky, S. L., Matsudaira, P., Baltimore, D., and Darnell, J. (2000). Proto-oncogenes and tumor suppressor genes. Molecular cell biology (4th ed., section 24.2). New York, NY: W. H. Freeman. Retrieved from http://www.ncbi.nlm.nih.gov/books/NBK21662/#_A7092_ .
- Roberts, P. J. and Der, C. J. (2007). Targeting the Raf-MEK-ERK mitogen-activated protein kinase cascade for the treatment of cancer. Oncogene , 26 , 3292. http://dx.doi.org/10.1038/sj.onc.1210422 .
- Santarpia, L., Lippman, S. L., and El-Naggar, A. K. (2012). Targeting the mitogen-activated protein kinase RAS-RAF signaling pathway in cancer therapy. Expert Opin. Ther. Targets 16 (1), 103-119. http://www.ncbi.nlm.nih.gov/pmc/articles/PMC3457779/#S6title .
- Raven, P. H., Johnson, G. B., Mason, K. A., Losos, J. B., and Singer, S. R. (2014). Cancer is a failure of cell cycle control. In Biology (10th ed., AP ed., pp. 202-204). New York, NY: McGraw-Hill.
- Vogelstein, B., Sur, S. & Prives, C. (2010). p53: the most frequently altered gene in human cancers. Nature Education , 3 . Retrieved from http://www.nature.com/scitable/topicpage/p53-the-most-frequently-altered-gene-in-14192717 .
- Vogelstein, B., Lane, D., and Levine, A.J. (2000). Surfing the p53 network. Nature , 408 , 307-310. http://dx.doi.org/10.1038/35042675 .
Additional references:
Want to join the conversation.
- Upvote Button navigates to signup page
- Downvote Button navigates to signup page
- Flag Button navigates to signup page


The Biology of Cancer
- 1
- | 2
- | 3
- | 4
- | 5
- | 6
- | 7
- | 8
- | 9
- | 10
- | 11
- | 12

All Modules
Characteristics of Cancer Cells
Cancer cells grow and divide at an abnormally rapid rate, are poorly differentiated, and have abnormal membranes, cytoskeletal proteins, and morphology. The abnormality in cells can be progressive with a slow transition from normal cells to benign tumors to malignant tumors.

In 2000 cancer biologists Robert Weinberg and Douglas Hanahan published an article entitled "The Hallmarks of Cancer." [Cell 2000;100(1):57-70] While they recognized that cancers occurred through a series of mutations in any of many genes. Despite this, they listed six essential alterations in cell physiology that characterized malignancy.
- Self-sufficiency in growth signals: cancer cells acquire an autonomous drive to proliferate - pathological mitosis - by virtue of the activation of oncogenes such as ras or myc.
- Insensitivity to growth-inhibitory (antigrowth) signals: cancer cells inactivate tumor suppressor genes, such as Rb, that normally inhibit growth.
- Evasion of programmed cell death (apoptosis): cancer cells suppress and inactivate genes and pathways that normally enable cells to die.
- Limitless replication potential: cancer cells activate specific gene pathways that render them immortal even after generations of growth.
- Sustained angiogenesis: cancer cells acquire the capacity to draw out their own supply of blood and blood vessels - tumor angiogenesis.
- Tissue invasion and metastasis: cancer cells acquire the capacity to migrate to other organs, invade other tissues, and colonize these organs, resulting in their spread throughout the body.
return to top | previous page | next page
Content ©2016. All Rights Reserved. Date last modified: May 4, 2016. Created by Wayne W. LaMorte, MD, PhD, MPH,
Fact sheets
- Facts in pictures
- Publications
- Questions and answers
- Tools and toolkits
- HIV and AIDS
- Hypertension
- Mental disorders
- Top 10 causes of death
- All countries
- Eastern Mediterranean
- South-East Asia
- Western Pacific
- Data by country
- Country presence
- Country strengthening
- Country cooperation strategies
- News releases
- Feature stories
- Press conferences
- Commentaries
- Photo library
- Afghanistan
- Cholera
- Coronavirus disease (COVID-19)
- Greater Horn of Africa
- Israel and occupied Palestinian territory
- Disease Outbreak News
- Situation reports
- Weekly Epidemiological Record
- Surveillance
- Health emergency appeal
- International Health Regulations
- Independent Oversight and Advisory Committee
- Classifications
- Data collections
- Global Health Estimates
- Mortality Database
- Sustainable Development Goals
- Health Inequality Monitor
- Global Progress
- Data collection tools
- Global Health Observatory
- Insights and visualizations
- COVID excess deaths
- World Health Statistics
- Partnerships
- Committees and advisory groups
- Collaborating centres
- Technical teams
- Organizational structure
- Initiatives
- General Programme of Work
- WHO Academy
- Investment case
- WHO Foundation
- External audit
- Financial statements
- Internal audit and investigations
- Programme Budget
- Results reports
- Governing bodies
- World Health Assembly
- Executive Board
- Member States Portal
- Fact sheets /
- Cancer is a leading cause of death worldwide, accounting for nearly 10 million deaths in 2020, or nearly one in six deaths.
- The most common cancers are breast, lung, colon and rectum and prostate cancers.
- Around one-third of deaths from cancer are due to tobacco use, high body mass index, alcohol consumption, low fruit and vegetable intake, and lack of physical activity.
- Cancer-causing infections, such as human papillomavirus (HPV) and hepatitis, are responsible for approximately 30% of cancer cases in low- and lower-middle-income countries.
- Many cancers can be cured if detected early and treated effectively.
Cancer is a generic term for a large group of diseases that can affect any part of the body. Other terms used are malignant tumours and neoplasms. One defining feature of cancer is the rapid creation of abnormal cells that grow beyond their usual boundaries, and which can then invade adjoining parts of the body and spread to other organs; the latter process is referred to as metastasis. Widespread metastases are the primary cause of death from cancer.
The problem
Cancer is a leading cause of death worldwide, accounting for nearly 10 million deaths in 2020 (1). The most common in 2020 (in terms of new cases of cancer) were:
- breast (2.26 million cases);
- lung (2.21 million cases);
- colon and rectum (1.93 million cases);
- prostate (1.41 million cases);
- skin (non-melanoma) (1.20 million cases); and
- stomach (1.09 million cases).
The most common causes of cancer death in 2020 were:
- lung (1.80 million deaths);
- colon and rectum (916 000 deaths);
- liver (830 000 deaths);
- stomach (769 000 deaths); and
- breast (685 000 deaths).
Each year, approximately 400 000 children develop cancer. The most common cancers vary between countries. Cervical cancer is the most common in 23 countries.
Cancer arises from the transformation of normal cells into tumour cells in a multi-stage process that generally progresses from a pre-cancerous lesion to a malignant tumour. These changes are the result of the interaction between a person's genetic factors and three categories of external agents, including:
- physical carcinogens, such as ultraviolet and ionizing radiation;
- chemical carcinogens, such as asbestos, components of tobacco smoke, alcohol, aflatoxin (a food contaminant), and arsenic (a drinking water contaminant); and
- biological carcinogens, such as infections from certain viruses, bacteria, or parasites.
WHO, through its cancer research agency, the International Agency for Research on Cancer (IARC), maintains a classification of cancer-causing agents.
The incidence of cancer rises dramatically with age, most likely due to a build-up of risks for specific cancers that increase with age. The overall risk accumulation is combined with the tendency for cellular repair mechanisms to be less effective as a person grows older.
Risk factors
Tobacco use, alcohol consumption, unhealthy diet, physical inactivity and air pollution are risk factors for cancer and other noncommunicable diseases.
Some chronic infections are risk factors for cancer; this is a particular issue in low- and middle-income countries. Approximately 13% of cancers diagnosed in 2018 globally were attributed to carcinogenic infections, including Helicobacter pylori, human papillomavirus (HPV), hepatitis B virus, hepatitis C virus, and Epstein-Barr virus (2).
Hepatitis B and C viruses and some types of HPV increase the risk for liver and cervical cancer, respectively. Infection with HIV increases the risk of developing cervical cancer six-fold and substantially increases the risk of developing select other cancers such as Kaposi sarcoma.
Reducing the burden
Between 30 and 50% of cancers can currently be prevented by avoiding risk factors and implementing existing evidence-based prevention strategies. The cancer burden can also be reduced through early detection of cancer and appropriate treatment and care of patients who develop cancer. Many cancers have a high chance of cure if diagnosed early and treated appropriately.
Cancer risk can be reduced by:
- not using tobacco;
- maintaining a healthy body weight;
- eating a healthy diet, including fruit and vegetables;
- doing physical activity on a regular basis;
- avoiding or reducing consumption of alcohol;
- getting vaccinated against HPV and hepatitis B if you belong to a group for which vaccination is recommended;
- avoiding ultraviolet radiation exposure (which primarily results from exposure to the sun and artificial tanning devices) and/or using sun protection measures;
- ensuring safe and appropriate use of radiation in health care (for diagnostic and therapeutic purposes);
- minimizing occupational exposure to ionizing radiation; and
- reducing exposure to outdoor air pollution and indoor air pollution, including radon (a radioactive gas produced from the natural decay of uranium, which can accumulate in buildings — homes, schools and workplaces).
Early detection
Cancer mortality is reduced when cases are detected and treated early. There are two components of early detection: early diagnosis and screening.
Early diagnosis
When identified early, cancer is more likely to respond to treatment and can result in a greater probability of survival with less morbidity, as well as less expensive treatment. Significant improvements can be made in the lives of cancer patients by detecting cancer early and avoiding delays in care.
Early diagnosis consists of three components:
- being aware of the symptoms of different forms of cancer and of the importance of seeking medical advice when abnormal findings are observed;
- access to clinical evaluation and diagnostic services; and
- timely referral to treatment services.
Early diagnosis of symptomatic cancers is relevant in all settings and the majority of cancers. Cancer programmes should be designed to reduce delays in, and barriers to, diagnosis, treatment and supportive care.
Screening aims to identify individuals with findings suggestive of a specific cancer or pre-cancer before they have developed symptoms. When abnormalities are identified during screening, further tests to establish a definitive diagnosis should follow, as should referral for treatment if cancer is proven to be present.
Screening programmes are effective for some but not all cancer types and in general are far more complex and resource-intensive than early diagnosis as they require special equipment and dedicated personnel. Even when screening programmes are established, early diagnosis programmes are still necessary to identify those cancer cases occurring in people who do not meet the age or risk factor criteria for screening.
Patient selection for screening programmes is based on age and risk factors to avoid excessive false positive studies. Examples of screening methods are:
- HPV test (including HPV DNA and mRNA test), as preferred modality for cervical cancer screening; and
- mammography screening for breast cancer for women aged 50–69 residing in settings with strong or relatively strong health systems.
Quality assurance is required for both screening and early diagnosis programmes.
A correct cancer diagnosis is essential for appropriate and effective treatment because every cancer type requires a specific treatment regimen. Treatment usually includes surgery, radiotherapy, and/or systemic therapy (chemotherapy, hormonal treatments, targeted biological therapies). Proper selection of a treatment regimen takes into consideration both the cancer and the individual being treated. Completion of the treatment protocol in a defined period of time is important to achieve the predicted therapeutic result.
Determining the goals of treatment is an important first step. The primary goal is generally to cure cancer or to considerably prolong life. Improving the patient's quality of life is also an important goal. This can be achieved by support for the patient’s physical, psychosocial and spiritual well-being and palliative care in terminal stages of cancer.
Some of the most common cancer types, such as breast cancer, cervical cancer, oral cancer, and colorectal cancer, have high cure probabilities when detected early and treated according to best practices.
Some cancer types, such as testicular seminoma and different types of leukaemia and lymphoma in children, also have high cure rates if appropriate treatment is provided, even when cancerous cells are present in other areas of the body.
There is, however, a significant variation in treatment availability between countries of different income levels; comprehensive treatment is reportedly available in more than 90% of high-income countries but less than 15% of low-income countries (3).
Palliative care
Palliative care is treatment to relieve, rather than cure, symptoms and suffering caused by cancer and to improve the quality of life of patients and their families. Palliative care can help people live more comfortably. It is particularly needed in places with a high proportion of patients in advanced stages of cancer where there is little chance of cure.
Relief from physical, psychosocial, and spiritual problems through palliative care is possible for more than 90% of patients with advanced stages of cancer.
Effective public health strategies, comprising community- and home-based care, are essential to provide pain relief and palliative care for patients and their families.
WHO response
In 2017, the World Health Assembly passed the Resolution Cancer prevention and control in the context of an integrated approach (WHA70.12) that urges governments and WHO to accelerate action to achieve the targets specified in the Global Action Plan for the prevention and control of NCDs 2013-2020 and the 2030 UN Agenda for Sustainable Development to reduce premature mortality from cancer.
WHO and IARC collaborate with other UN organizations, inlcuing the International Atomic Energy Agency, and partners to:
- increase political commitment for cancer prevention and control;
- coordinate and conduct research on the causes of human cancer and the mechanisms of carcinogenesis;
- monitor the cancer burden (as part of the work of the Global Initiative on Cancer Registries);
- identify “best buys” and other cost-effective, priority strategies for cancer prevention and control;
- develop standards and tools to guide the planning and implementation of interventions for prevention, early diagnosis, screening, treatment and palliative and survivorship care for both adult and child cancers;
- strengthen health systems at national and local levels to help them improve access to cancer treatments;
- set the agenda for cancer prevention and control in the 2020 WHO Report on Cancer;
- provide global leadership as well as technical assistance to support governments and their partners build and sustain high-quality cervical cancer control programmes as part of the Global Strategy to Accelerate the Elimination of Cervical Cancer;
- improve breast cancer control and reduce avoidable deaths from breast cancer, focusing on health promotion, timely diagnosis and access to care in order to accelerate coordinated implementation through the WHO Global Breast Cancer Initiative;
- support governments to improve survival for childhood cancer through directed country support, regional networks and global action as part of the WHO Global Initiative for Childhood Cancer using the Cure All approach;
- increase access to essential cancer medicines, particularly through the Global Platform for Access to Childhood Cancer Medicines; and
- provide technical assistance for rapid, effective transfer of best practice interventions to countries.
(1) Ferlay J, Ervik M, Lam F, Colombet M, Mery L, Piñeros M, et al. Global Cancer Observatory: Cancer Today. Lyon: International Agency for Research on Cancer; 2020 ( https://gco.iarc.fr/today , accessed February 2021).
(2) de Martel C, Georges D, Bray F, Ferlay J, Clifford GM. Global burden of cancer attributable to infections in 2018: a worldwide incidence analysis. Lancet Glob Health. 2020;8(2):e180-e190.
(3) Assessing national capacity for the prevention and control of noncommunicable diseases: report of the 2019 global survey. Geneva: World Health Organization; 2020.
Cervical cancer
Cervical cancer country profiles
Cervical Cancer Knowledge Repository
Essay on Cancer for Students and Children
500+ words essay on cancer.
Cancer might just be one of the most feared and dreaded diseases. Globally, cancer is responsible for the death of nearly 9.5 million people in 2018. It is the second leading cause of death as per the world health organization. As per studies, in India, we see 1300 deaths due to cancer every day. These statistics are truly astonishing and scary. In the recent few decades, the number of cancer has been increasingly on the rise. So let us take a look at the meaning, causes, and types of cancer in this essay on cancer.
Cancer comes in many forms and types. Cancer is the collective name given to the disease where certain cells of the person’s body start dividing continuously, refusing to stop. These extra cells form when none are needed and they spread into the surrounding tissues and can even form malignant tumors. Cells may break away from such tumors and go and form tumors in other places of the patient’s body.

Types of Cancers
As we know, cancer can actually affect any part or organ of the human body. We all have come across various types of cancer – lung, blood, pancreas, stomach, skin, and so many others. Biologically, however, cancer can be divided into five types specifically – carcinoma, sarcoma, melanoma, lymphoma, leukemia.
Among these, carcinomas are the most diagnosed type. These cancers originate in organs or glands such as lungs, stomach, pancreas, breast, etc. Leukemia is the cancer of the blood, and this does not form any tumors. Sarcomas start in the muscles, bones, tissues or other connective tissues of the body. Lymphomas are the cancer of the white blood cells, i.e. the lymphocytes. And finally, melanoma is when cancer arises in the pigment of the skin.
Get the huge list of more than 500 Essay Topics and Ideas
Causes of Cancer
In most cases, we can never attribute the cause of any cancer to one single factor. The main thing that causes cancer is a substance we know as carcinogens. But how these develop or enters a person’s body will depend on many factors. We can divide the main factors into the following types – biological factors, physical factors, and lifestyle-related factors.
Biological factors involve internal factors such as age, gender, genes, hereditary factors, blood type, skin type, etc. Physical factors refer to environmental exposure of any king to say X-rays, gamma rays, etc. Ad finally lifestyle-related factors refer to substances that introduced carcinogens into our body. These include tobacco, UV radiation, alcohol. smoke, etc. Next, in this essay on cancer lets learn about how we can treat cancer.
Treatment of Cancer
Early diagnosis and immediate medical care in cancer are of utmost importance. When diagnosed in the early stages, then the treatment becomes easier and has more chances of success. The three most common treatment plans are either surgery, radiation therapy or chemotherapy.
If there is a benign tumor, then surgery is performed to remove the mass from the body, hence removing cancer from the body. In radiation therapy, we use radiation (rays) to specially target and kill the cancer cells. Chemotherapy is similar, where we inject the patient with drugs that target and kill the cancer cells. All treatment plans, however, have various side-effects. And aftercare is one of the most important aspects of cancer treatment.
Customize your course in 30 seconds
Which class are you in.

- Travelling Essay
- Picnic Essay
- Our Country Essay
- My Parents Essay
- Essay on Favourite Personality
- Essay on Memorable Day of My Life
- Essay on Knowledge is Power
- Essay on Gurpurab
- Essay on My Favourite Season
- Essay on Types of Sports
Leave a Reply Cancel reply
Your email address will not be published. Required fields are marked *
Download the App
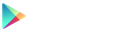
- Type 2 Diabetes
- Heart Disease
- Digestive Health
- Multiple Sclerosis
- Diet & Nutrition
- Supplements
- Health Insurance
- Public Health
- Patient Rights
- Caregivers & Loved Ones
- End of Life Concerns
- Health News
- Thyroid Test Analyzer
- Doctor Discussion Guides
- Hemoglobin A1c Test Analyzer
- Lipid Test Analyzer
- Complete Blood Count (CBC) Analyzer
- What to Buy
- Editorial Process
- Meet Our Medical Expert Board
Cancer Cells vs. Normal Cells: How Are They Different?
- Basic Differences
How Do Cells Become Cancerous?
- Technical Differences
- Differences Among Cancer Cells
Cancer cells are different from normal cells in how they grow, how they look, and what they do in the body. Even though cancer is common, there are actually many steps that a normal cell has to go through to become a cancerous cell.
This article will explain how cancer cells and normal, healthy cells are different. It covers the basic differences between cancer cells and normal cells, like how they grow and communicate. It also goes a bit deeper, covering technical differences between normal cells and cancer cells, including how they invade tissue in the body and "hide" from cancer treatments.
Basic Differences Between Cancer Cells and Normal Cells
There are many differences between cancer cells and normal cells in noncancerous (benign) or cancerous (malignant) tumors.
The major differences between normal cells and cancer cells relate to growth, communication, cell repair and death, "stickiness" and spread, appearance, maturation, evasion of the immune system, function and blood supply.
Normal Cells | Cancerous Cells | |
---|---|---|
Growth | Stop when there's enough | Uncontrolled growth |
Communication | Respond to signals from other cells | Do not respond to signals from other cells |
Cell repair/death | Aged/damaged cells are repaired or replaced | Cells are neither repaired or replaced |
Stickiness/spread | Stay together in assigned area | Can travel solo and throughout the body |
Appearance | Uniform look under a microscope | Varied sizes, larger and darker center under a microscope |
Maturation | Reach maturity | Do not reach maturity |
Evasion of immune system | Can be targeted and eliminated | Can "hide" and grow uninterruputed |
Function | Perform designated tasks | Fail to perform designated tasks |
Blood supply | Blood vessels grow to feed normal growth and aid in repairs | Blood vessels grow regardless, constantly "feeding" a tumor |
It's these differences that account for how cancerous tumors grow and respond differently to their surroundings than benign tumors.
Normal cells stop growing (reproducing) when enough cells are present. For example, if cells are being produced to repair a cut in the skin, new cells are no longer produced when there are enough cells present to fill the hole (when the repair work is done).
In contrast, cancer cells don’t stop growing when there are enough cells present. Cancer cells reproduce rapidly before they have had a chance to mature. This continued replication often results in a tumor (a cluster of cancer cells) being formed.
Each gene in the body carries a blueprint that codes for a different protein. Some of these proteins are growth factors—chemicals that tell cells to grow and divide.
If the gene that codes for one of these proteins is stuck in the “on” position by a mutation (an oncogene ) the growth factor proteins continue to be produced. In response, the cells continue to grow.
Click Play to Learn About the Start and Characteristics of Cancer Cells
This video has been medically reviewed by Doru Paul, MD
Communication
Cancer cells don’t interact with other cells as normal cells do. Normal cells respond to signals sent from other nearby cells that say, “you’ve reached your boundary.” When normal cells “hear” these signals they stop growing. Cancer cells do not respond to these signals.
Cell Repair and Cell Death (Homeostasis)
Homeostasis is the body's way of ensuring all its processes are working properly. Homeostasis is one area where normal cells and cancer cells are very different.
To maintain homeostasis, normal cells are either repaired or die (undergo apoptosis ) when they are damaged or get old. Cancer cells are either not repaired or do not undergo apoptosis.
For example, one protein called p53 has the job of checking to see if a cell is too damaged to repair, and if so, advise the cell to kill itself. If this protein p53 is abnormal or inactive (for example, from a mutation in the p53 gene ), then old or damaged cells are allowed to reproduce.
The p53 gene is one type of tumor suppressor gene that code for proteins that suppress the growth of cells.
Normal cells secrete substances that make them stick together in a group. Cancer cells fail to make these substances, and can “float away” to locations nearby, or through the bloodstream or system of lymph channels to distant regions in the body.
Normal cells stay in the area of the body where they belong. For example, lung cells remain in the lungs. Some cancer cells may lack the adhesion molecules that cause stickiness, and are able to detach and travel via the bloodstream and lymphatic system to other regions of the body—they have the ability to spread ( metastasize ).
Once they arrive in a new region (such as lymph nodes , the lungs, the liver, or the bones) they begin to grow, often forming tumors far removed from the original tumor.
Under a microscope, normal cells and cancer cells may look quite different. In contrast to normal cells, cancer cells often exhibit much more variability in cell size—some are larger than normal and some are smaller than normal.
In addition, cancer cells often have an abnormal shape—both the cell and the nucleus (the “brain” of the cell.) The nucleus appears both larger and darker than normal cells.
The reason for the darkness is that the nucleus of cancer cells contains excess DNA. Up close, cancer cells often have an abnormal number of chromosomes that are arranged in a disorganized fashion.
Normal cells mature. Cancer cells, because they grow rapidly and divide before cells are fully mature, remain immature. Doctors use the term undifferentiated to describe immature cells (in contrast to differentiated to describe more mature cells.)
Another way to explain this is to view cancer cells as cells that don’t “grow up” and specialize to adult cells. The degree of maturation of cells corresponds to the grade of cancer. Cancers are graded on a scale from 1 to 3 with 3 being the most aggressive.
Evasion of the Immune System
When normal cells become damaged, the immune system (via cells called lymphocytes ) identifies and removes them.
Cancer cells are able to evade (trick) the immune system long enough to grow into a tumor by either escaping detection or by secreting chemicals that inactivate immune cells that come to the scene. Some of the newer immunotherapy medications address this aspect of cancer cells.
Normal cells perform the function they are meant to perform, whereas cancer cells may not be functional.
For example, normal white blood cells help fight off infections. In leukemia , the number of white blood cells may be very high, but since the cancerous white blood cells are not functioning as they should, people can be more at risk for infection even with an elevated white blood cell count.
The same can be true of substances produced. For example, normal thyroid cells produce thyroid hormones. Cancerous thyroid cells ( thyroid cancer ) may not produce thyroid hormone. In this case, the body may lack enough thyroid hormone ( hypothyroidism ) despite an increased amount of thyroid tissue.
Blood Supply
Angiogenesis is the process by which cells attract blood vessels to grow and feed the tissue. Normal cells undergo a process called angiogenesis only as part of normal growth and development and when new tissue is needed to repair damaged tissue.
Cancer cells undergo angiogenesis even when growth is not necessary. One type of cancer treatment involves the use of angiogenesis inhibitors—medications that block angiogenesis in the body in an effort to keep tumors from growing.
There are proteins in the body that regulate cell growth. Your DNA carries genes that are the blueprint for proteins produced in the body.
Some of these proteins are growth factors—chemicals that tell cells to divide and grow. Other proteins work to stop (suppress) growth.
Mutations in particular genes—for example, those caused by tobacco smoke, radiation, ultraviolet radiation, and other carcinogens—can result in the abnormal production of proteins. Too many might be produced or not enough. Sometimes, the proteins are abnormal and function differently.
Normally, roughly three billion cells divide in the body every single day. When “accidents” in the reproduction of these cells happen during any of those divisions (for example, caused by genes or environmental carcinogens ), it may create a cell that can mutate more and develop into a cancer cell.
That said, there are several “checkpoints” that need to be bypassed for a cell to become cancerous:
- The cell needs to have growth factors that prompt it to grow even when growth is not necessary.
- The cells have to evade proteins that direct cells to stop growing and die when they become abnormal.
- The cell needs to evade signals from other cells,
- The cells need to lose the normal “stickiness” (adhesion molecules) that normal cells produce.
It takes a combination of abnormalities to make a cancerous cell happen, rather than a single mutation or protein abnormality. It's actually very difficult for a normal cell to become cancerous, which may seem surprising considering that one in three people will develop cancer in their lifetime.
A Deeper Dive Into the Technical Differences
This list contains further differences between healthy cells and cancer cells.
Evading Growth Suppressors
Normal cells are controlled by growth (tumor) suppressors. There are three main types of tumor suppressor genes that code for proteins that suppress growth.
- One type tells cells to slow down and stop dividing.
- Another type is responsible for fixing changes in damaged cells.
- A third type is in charge of the apoptosis noted above.
Mutations that result in any of these tumor suppressor genes being inactivated allow cancer cells to grow unchecked.
Invasiveness
Normal cells listen to signals from neighboring cells and stop growing when they encroach on nearby tissues (something called contact inhibition). Cancer cells ignore these cells and invade nearby tissues.
Benign (noncancerous) tumors have a fibrous capsule. They may push up against nearby tissues but they do not invade/intermingle with other tissues.
Cancer cells, in contrast, don’t respect boundaries and invade tissues. This results in the fingerlike projections that are often noted on radiologic scans of cancerous tumors. The word cancer, in fact, comes from the Latin word for crab used to describe the crablike invasion of cancers into nearby tissues.
Energy Source
Normal cells get most of their energy (in the form of a molecule called ATP) through a process called the Krebs cycle, and only a small amount of their energy through a different process called glycolysis .
Many types of cancer cells produce their energy through glycolysis despite the presence of oxygen (Warburg phenomenon). Thus, the reasoning behind hyperbaric oxygen therapy is flawed. Sometimes hyperbaric oxygen may induce cancer growth.
Mortality/Immortality
Normal cells are mortal, that is, they have a lifespan. Cells aren’t designed to live forever, and just like the humans they are present in, cells grow old. Researchers are beginning to look at something called telomeres , structures that hold DNA together at the end of the chromosomes, for their role in cancer.
One of the limitations to growth in normal cells is the length of the telomeres. Every time a cell divides, the telomeres get shorter. When the telomeres become too short, a cell can no longer divide and the cell dies.
Cancer cells have figured out a way to renew telomeres so that they can continue to divide. An enzyme called telomerase works to lengthen the telomeres so that the cell can divide indefinitely—essentially becoming immortal.
Ability to "Hide"
Many people wonder why cancer can recur years, and sometimes decades after it appears to be gone (especially with tumors such as estrogen receptor-positive breast cancers ). There are several theories about why cancers may recur.
In general, it's thought that there is a hierarchy of cancer cells, with some cells (cancer stem cells) having the ability to resist treatment and lie dormant. This is an active area of research, and extremely important.
Genomic Instability
Normal cells have normal DNA and a normal number of chromosomes. Cancer cells often have an abnormal number of chromosomes and the DNA becomes increasingly abnormal as it develops a multitude of mutations.
Some of these are driver mutations, meaning they drive the transformation of the cell to be cancerous. Many of the mutations are passenger mutations, meaning they don’t have a direct function for the cancer cell.
For some cancers, determining which driver mutations are present (through genetic testing ) allows providers to use targeted medications that specifically target cancer growth.
The development of targeted therapies such as EGFR inhibitors for cancers with EGFR mutations is one of the more rapidly growing and progressing areas of cancer treatment.
Can Cancer Cells Differ From Other Cancer Cells?
Given the many differences between cancer cells and normal cells, you might be wondering if there are differences between cancer cells themselves. That there may be a hierarchy of cancer cells—some having different functions than others—is the basis of discussions looking at cancer stem cells as discussed above.
Scientists still don't understand how cancer cells can seemingly hide for years or decades and then reappear. It's thought by some that the "generals" in the hierarchy of cancer cells referred to as cancer stem cells may be more resistant to treatments and have the ability to lie dormant when other soldier cancer cells are eliminated by treatments such as chemotherapy.
We currently treat all the cancer cells in a tumor as being identical, but it's likely that in the future, treatments will take some of the differences in cancer cells in an individual tumor into account.
Some of the differences between normal cells and cancer cells are well known, whereas others have only been recently discovered and are less well understood.
Many people become frustrated, wondering why we haven't yet found a way to stop all cancers in their tracks. Understanding the many changes a cell undergoes in the process of becoming a cancer cell can help explain some of the complexity. There is not one step, but rather many, that are currently being addressed in different ways.
For researchers, understanding how cancer cells function differently from normal cells lays the foundation for developing treatments designed to rid the body of cancer cells without damaging normal cells.
In addition, cancer isn't a single disease, but rather hundreds of different diseases. And even two cancers that are the same with regard to type and stage, can behave very differently. If there were 200 people with the same type and stage of cancer in a room, they would have 200 different cancers from a molecular standpoint.
A Word From Verywell
As more is learned about what makes a cancer cell a cancer cell, more insight into how to stop that cell from reproducing—and perhaps even making the transition to becoming a cancer cell in the first place—is gained.
Progress is already being made in that arena, as targeted therapies are being developed which discriminate between cancer cells and normal cells in their mechanism.
Research on immunotherapy is just as exciting, as experts are finding ways to "stimulate" the immune system to do what it already know how to do—find and eliminate invaders.
Figuring out the ways in which cancer cells "disguise" themselves and hide has resulted in better treatments, and uncommonly, complete remissions, for some people with the most advanced solid tumors.
Mercadante AA, Kasi A. Genetics, Cancer Cell Cycle Phases .
Vanneman M, Dranoff G. Combining immunotherapy and targeted therapies in cancer treatment . Nat Rev Cancer . 2012;12(4):237-51. doi:10.1038/nrc3237
Chandran R, Hakki M, Spurgeon S. Infections in Leukemia . In: Azevedo L, editor. Sepsis: An Ongoing and Significant Challenge . IntechOpen; 2012. doi:10.5772/50193
Carter Y, Sippel RS, Chen H. Hypothyroidism after a cancer diagnosis: etiology, diagnosis, complications, and management . Oncologist . 2014;19(1):34-43. doi:10.1634/theoncologist.2013-0237
Alexandrov LB, Ju YS, Haase K, et al. Mutational signatures associated with tobacco smoking in human cancer . Science . 2016;354(6312):618-622. doi:10.1126/science.aag0299
National Cancer Institute. Cancer-Causing Substances in the Environment .
American Cancer Society. Lifetime Risk of Developing or Dying From Cancer .
Moen I, Stuhr LE. Hyperbaric oxygen therapy and cancer--a review . Target Oncol. 2012;7(4):233-42. doi:10.1007/s11523-012-0233-x
Artandi SE, Depinho RA. Telomeres and telomerase in cancer . Carcinogenesis . 2010;31(1):9-18. doi:10.1093/carcin/bgp268
Wheeler DL, Dunn EF, Harari PM. Understanding resistance to EGFR inhibitors-impact on future treatment strategies . Nat Rev Clin Oncol. 2010;7(9):493-507. doi:10.1038/nrclinonc.2010.97
DeBaradinis, R. et al. The biology of cancer: metabolic reprogramming fuels cell growth and proliferation . Cell Metabolism . 2008;7(1):11-20.
National Cancer Institute. SEER Training Module. Cell Biology of Cancer .
National Cancer Institute. What is Cancer?
Nio, K., Yamashita, T., and S. Kaneko. The Evolving Concept of Liver Cancer Stem Cells . Molecular Cancer . 2017;16(1):4.
By Lynne Eldridge, MD Lynne Eldrige, MD, is a lung cancer physician, patient advocate, and award-winning author of "Avoiding Cancer One Day at a Time."
Thank you for visiting nature.com. You are using a browser version with limited support for CSS. To obtain the best experience, we recommend you use a more up to date browser (or turn off compatibility mode in Internet Explorer). In the meantime, to ensure continued support, we are displaying the site without styles and JavaScript.
- View all journals
- Explore content
- About the journal
- Publish with us
- Sign up for alerts
- Review Article
- Open access
- Published: 12 March 2020
Molecular principles of metastasis: a hallmark of cancer revisited
- Jawad Fares ORCID: orcid.org/0000-0002-5368-8764 1 , 2 ,
- Mohamad Y. Fares 3 , 4 ,
- Hussein H. Khachfe 3 , 4 ,
- Hamza A. Salhab 3 , 4 &
- Youssef Fares 4
Signal Transduction and Targeted Therapy volume 5 , Article number: 28 ( 2020 ) Cite this article
177k Accesses
1045 Citations
93 Altmetric
Metrics details
Metastasis is the hallmark of cancer that is responsible for the greatest number of cancer-related deaths. Yet, it remains poorly understood. The continuous evolution of cancer biology research and the emergence of new paradigms in the study of metastasis have revealed some of the molecular underpinnings of this dissemination process. The invading tumor cell, on its way to the target site, interacts with other proteins and cells. Recognition of these interactions improved the understanding of some of the biological principles of the metastatic cell that govern its mobility and plasticity. Communication with the tumor microenvironment allows invading cancer cells to overcome stromal challenges, settle, and colonize. These characteristics of cancer cells are driven by genetic and epigenetic modifications within the tumor cell itself and its microenvironment. Establishing the biological mechanisms of the metastatic process is crucial in finding open therapeutic windows for successful interventions. In this review, the authors explore the recent advancements in the field of metastasis and highlight the latest insights that contribute to shaping this hallmark of cancer.
Similar content being viewed by others
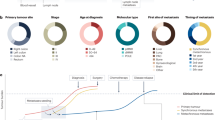
Mechanisms of metastatic colorectal cancer
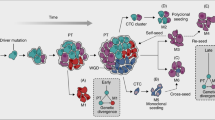
Genomic control of metastasis

Cell-intrinsic and microenvironmental determinants of metastatic colonization
Introduction.
The development of secondary tumors in a part of the body that is far from the original primary cancer is termed “metastasis.” Despite metastasis being the key cause of failure of cancer therapy and mortality, it remains poorly understood. In patients with cancer, large numbers of cancer cells are released in circulation daily; however, melanoma studies in animal models suggest that <0.1% of tumor cells metastasize. 1 The development of metastases requires cancer cells to leave their primary site, circulate in the bloodstream, endure pressure in blood vessels, acclimate to new cellular surroundings in a secondary site, and escape deadly combat with immune cells. 2 , 3 Hanahan and Weinberg 4 specify that “activating invasion and metastasis” is a hallmark of cancer. Indeed, invasion of nearby tissue and seeding at distant sites to form metastases remains a central feature of cancer malignancy (Fig. 1 ). After all, metastasis constitutes the primary cause of death for >90% of patients with cancer. 5 Understanding the dynamics of this process will help identify targets for molecular therapies that may halt or possibly reverse cancer growth and metastasis. Here, the authors review the recent advancements in the field of metastasis and highlight insights that contribute to shaping this hallmark of cancer.
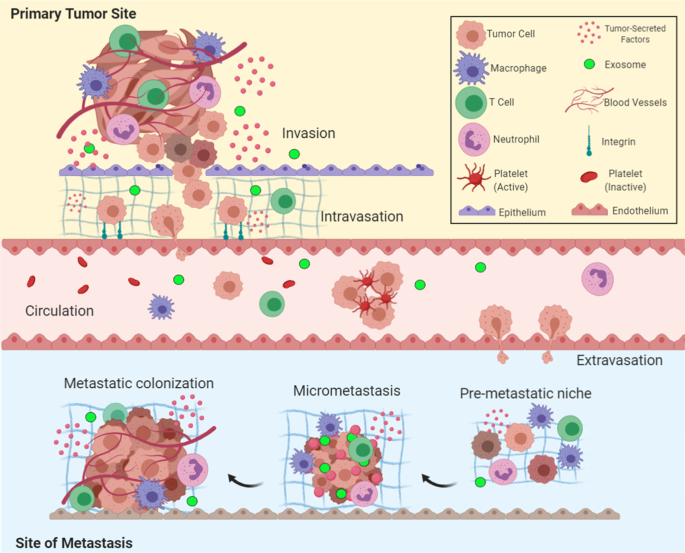
Overview of the metastatic cascade: The five key steps of metastasis include invasion, intravasation, circulation, extravasation, and colonization
Dissemination and invasion
Chromosomal instability: the initial trigger.
Dissemination of cancer cells precedes the initial steps of the invasion-metastasis cascade. 6 The cascade is the consequence of chromosomal instability that is caused by continuous errors in chromosome segregation during mitosis (Fig. 2 ). Faults in chromosome segregation cause the rupture of micronuclei and the secretion of genomic DNA into the cytosol, which subsequently activates cytosolic DNA-sensing pathways (cyclic GMP-AMP synthase–stimulator of interferon (IFN) genes) and downstream nuclear factor κ-light-chain-enhancer of activated B (NF-κB) signaling. 7
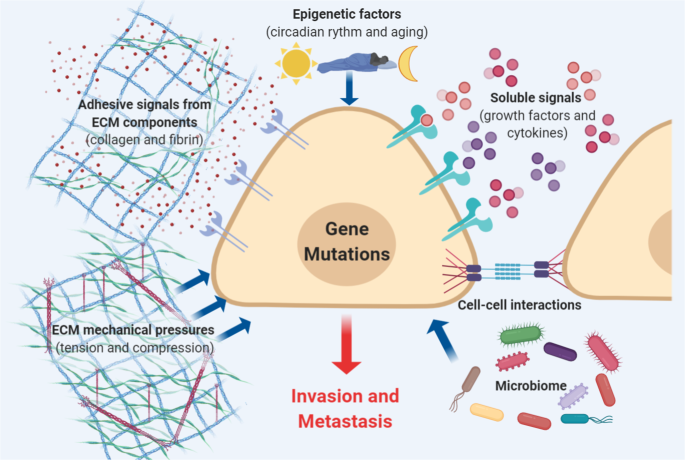
Determinants of metastasis: The activation of invasion and metastasis is triggered by epigenetic factors that are induced by environmental stimuli, such as aging and circadian disruptions; adhesive signals from extracellular matrix (ECM) components, such as collagen and fibrin; ECM mechanical pressures, including tension and compression; cell–cell interactions; soluble signals, such as growth factors and cytokines; and the intratumoral microbiota
Studies suggest that the nature of the primary seeding cancer cell determines the different metastatic properties with respect to growth and response to therapy. 8 , 9 In vivo and in vitro studies show that metastatic cancer cells migrate individually. 10 In humans, however, it is believed that seeding requires the joint action of a cluster of tumor cells moving together, 11 which brings epithelial–mesenchymal transition (EMT) into the picture.
Epithelial–mesenchymal transition: what is new?
EMT is the transdifferentiation process through which transformed epithelial cells develop the ability to invade, resist stress, and disseminate. 4 Epithelial cells are immotile and tightly bound to each other and to the neighboring extracellular matrix (ECM). 12 EMT governs the reversible biochemical alterations that permit a specific epithelial cell to attain a mesenchymal phenotype and confers epithelial–mesenchymal plasticity upon epithelial cells, 13 which is crucial for cancer progression and metastasis (Fig. 3 ). However, not all cells that originate from the primary tumor site contribute to the development of metastasis. Studying the determinants of metastatic potential in a mouse model of breast cancer revealed that asparagine synthetase, a metabolic enzyme, is correlated with metastasis development. 14 Decreasing the levels of asparagine through ʟ-asparaginase treatment or through dietary restriction decreased metastatic spread. As such, asparagine availability promoted EMT. 14
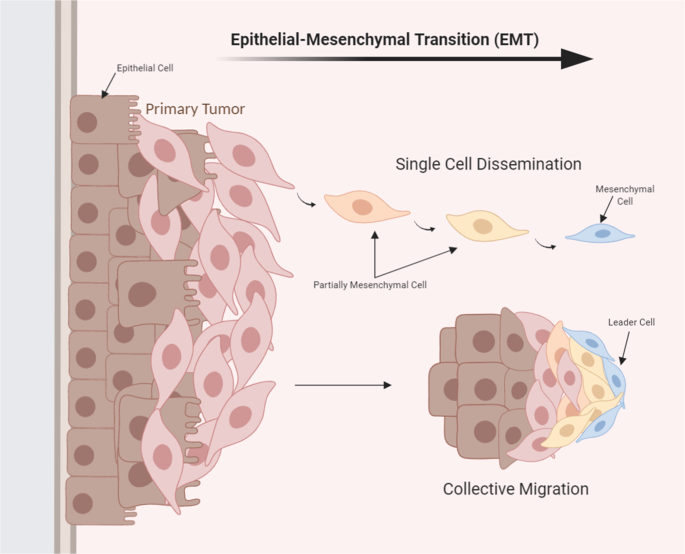
Epithelial–mesenchymal transition (EMT): EMT occurs through single-cell dissemination or through collective migration. The process consists of several transition stages between the initial epithelial cell and the invasive mesenchymal cell
Recently, it has become broadly understood that the EMT program is a spectrum of transitional stages between the epithelial and mesenchymal phenotypes, in contrast to a progression that involves a binary choice between full-epithelial and full-mesenchymal phenotypes. 15 The transition of one state to another is governed by a number of growth factors 16 and signaling pathways. 17 Spontaneous EMT in primary tumor cells shifts between different intermediate stages with different invasive, metastatic, and differentiation characteristics. 18 Tumor cells that express a mix of epithelial and mesenchymal phenotypes are more effective in circulation, colonization at the secondary site, and the development of metastasis. 18 Moreover, transcriptional, chromatin, and single-cell RNA sequencing show that the various stages possess diverse cellular characteristics, chromatin landscapes, and gene expression signatures that are regulated by common and distinct transcription factors and signaling pathways. Moreover, the various EMT stages are situated in diverse microenvironments and are in contact with diverse stromal cells. 18 For example, metastatic cells with the most pronounced mesenchymal phenotype proliferate near endothelial and inflammatory cells. These tumor cells release large quantities of chemokines and proteins to attract immune cells and stimulate angiogenesis, thus promoting the development of a unique inflammatory and highly vascularized niche. 18 Cancer-associated fibroblasts have also been shown to drive and direct cancer cell migration through fibronectin alignment. 19 In addition, hypoxia, 20 metabolic stressors, and matrix stiffness 21 trigger the EMT program in cancer cells. Transitioning is often driven by transcription factors that are programmed to repress epithelial genes and activate mesenchymal genes. 22 Epigenetic and posttranslational modulators also play a vital role in controlling the EMT process. 15
In recent years, there has been an important debate on whether EMT has a central role in cancer metastasis and resistance to chemotherapy. 17 , 23 , 24 , 25 Research in lung and pancreatic cancers shows that even though EMT might not be essential for metastasis, it does contribute to chemoresistance. 23 , 24 Nevertheless, more evidence is needed to completely and clearly elucidate the role of EMT in cancer progression and the metastatic process.
Although EMT might be required for metastasis initiation, the opposite process of mesenchymal–epithelial transition (MET) is needed for metastatic progression. In bone metastasis, E-selectin in the bone vasculature induces MET and WNT activation in cancer cells to drive metastatic tumor formation. 26
Genetic profile of metastatic cells
Metastatic cancer encompasses a diverse collection of cells that possess different genetic and phenotypic characteristics, which differentially drive progression, metastasis, and drug resistance. 27 Hundreds of genes have been reported to determine invasive potential, suggesting that primary tumor cells exhibit a metastatic genetic signature. 28 , 29 , 30 However, specific mutations can still promote invasion and metastasis in the context of some homozygous allelic expressions. Integrative clinical genomics showed that the most predominant genes that were somatically changed in metastasis included tumor protein p53 ( TP53 ), cyclin-dependent kinase inhibitor 2A ( CDKN2A ), phosphatase and tensin homolog ( PTEN ), phosphatidylinositol-4,5-bisphosphate 3-kinase catalytic subunit alpha ( PIK3CA ), and retinoblastoma ( RB1 ). 31 , 32 Putative pathogenic germline variants were present in 12.2% of cases, of which 75% were associated with DNA repair defects. 31
Markers that predict metastatic progression showed that advanced cancers arise from diverse cell types, which deeply affects the eventual genetic and epigenetic alterations that promote metastatic progression. 33 Metastatic small cell lung cancer (SCLC) cells differed in the genes that they expressed. 33 This might explain why some cancer cells respond to treatment, whereas others do not. As such, understanding intertumoral heterogeneity among different cancers can reveal the mechanisms of metastatic progression and how the cell type of origin contributes to tumor development. In colorectal cancer, cells expressing L1 cell adhesion molecule (L1CAM) confer metastasis-initiating abilities and chemoresistance. L1CAM hijacks the regenerative capacity of intestinal cells to promote metastasis. 34 In addition, the cytotoxic immune signature and the presence of lymphatic vessels play an important role in the generation of distant metastases, regardless of genomic instability. 35
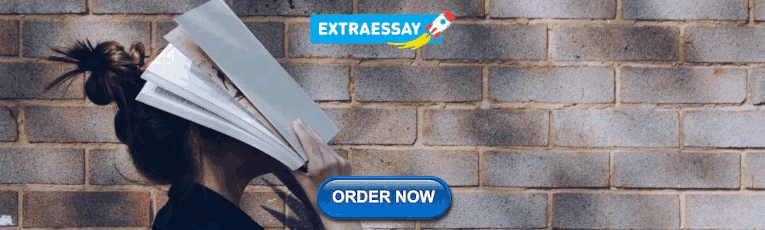
Metabolic profile of metastatic cells
Genetic expression that is involved in different biological processes related to metastasis is also affected by oxygen homeostasis in the tumor microenvironment. 36 Hypoxia‐inducible factors (HIF) permit cancer cells to adapt to their cellular environment by regulating angiogenesis, EMT, invasion, metastasis, and energy metabolism. 4 , 37 , 38 , 39 , 40 Furthermore, AXL, a receptor tyrosine kinase, has been identified as a vital mediator of HIF-dependent invasion and metastasis. In addition, HIF signaling drives the secretion of lysyl oxidase (LOX), LOX-like proteins, and exosomes, to establish a prometastatic environment within the lung and bones of patients with breast cancer. 20 Tumor hypoxia is associated with poor prognosis in clinical scenarios; 37 , 41 , 42 , 43 , 44 HIF‐1α and HIF‐2α expression is linked to patient mortality. 41 , 42 In general, these hypoxic factors, along with others, are associated with tumor aggressiveness and resistance to therapy. 38 Moreover, tumors with more extensive hypoxic and anoxic areas exhibit higher rates of metastasis. 45
Metabolic differences among cancer cells lead to differences in metastatic potential. Metastatic cancer cells depend on monocarboxylate transporter 1 (MCT1) to deal with oxidative stress. MCT1 plays a major role in circulating lactate, which is a prominent energy source for metastasizing cells. 46 As such, highly metastatic cells have increased levels of MCT1, whereas the inhibition of MCT1 decreases lactate uptake by metastatic cells and, thus, reduces their metastatic capability. 46
Changes in ATP/ADP and ATP/AMP ratios also promote metastatic behavior. In pancreatic ductal adenocarcinoma, ECM remodeling through cellular adhesion and compression affects these ratios. 47 Metabolomics shows that such alterations increase phosphocreatine production, which has a role in the invasive migration, chemotaxis, and liver metastasis of cancer cells. 47
Priming the premetastatic niche
Secondary sites do not receive invading cancer cells passively. In fact, the host microenvironment, termed the premetastatic niche (PMN), is selectively primed by the primary tumor even before the initiation of metastasis. 48 The development of a PMN is a multistep process involving secretory factors and extracellular vesicles that induce vascular leakage, ECM remodeling, and immunosuppression. 48 High-definition microscopes have obtained images of cancer cells sharing biological material with less malignant cells, making these cells more cancerous. 49 Cancer cells release vesicles that carry messenger RNA transcribed from genes that are involved in cell migration and metastasis, which are then accepted by other cells. 49 , 50 After host cells engulf these vesicles, human cells that did not express a malignant phenotype start to migrate faster. The transferred genes also enhance the ability of cells to invade other organs. 49 As such, metastatic characteristics can be transferred through extracellular vesicle exchange. 49
Primary tumors release significant amounts of exosomes that transfer invasion-promoting factors, such as microRNAs (miRNAs), to tumorigenic cancer cells. 51 , 52 , 53 For example, miR-10b is carried and released by exosomes and drives metastatic properties in breast cancer cells. 54 In addition, signaling factors mediated by exosomes activate epidermal growth factor receptor (EGFR) signaling to support cancer metastasis. 55 Exosomes that express EGFR ligands, such as amphiregulin, tissue-type plasminogen activator, and/or annexin II, considerably increase cancer cell invasion. 56 , 57 , 58 , 59 Moreover, exosomes secrete EMT inducers that stimulate EMT progression in host epithelial cells, providing them with the ability to invade and metastasize. 60 , 61 , 62 , 63 , 64 , 65 Furthermore, exosomes have the ability to remodel the ECM by interacting with fibroblasts, stromal cells, and endothelial cells to degrade protease-associated components such as collagen, laminin, and fibronectin. 66 Exosome-altered ECM exhibits increased stromal cell proliferation, cancer cell migration and survival, and tumor cell resistance to apoptotic signals. This, along with the effect of chemokines and growth factors, leads to the formation of a new microenvironment for cancer cells, immune cells, and other stromal constituents that is referred to as the PMN, 67 , 68 , 69 , 70 where metastatic cells may arrest, extravasate, and ultimately colonize. 71 , 72 , 73
In addition to their role in priming the PMN, exosomes exhibit properties that drive cancer cell organotropism. This metastatic bias towards certain organs stems from exosomal avidity for specific host cells. 60 Studying the exosomal proteomic expression of bone cancer showed different integrin patterns, whereby the exosomal integrins α6β4 and α6β1 were correlated with lung metastasis, whereas exosomal integrin αvβ5 was associated with liver metastasis. 74 Uptake of integrins in the secondary site led to the phosphorylation of Src and the expression of the proinflammatory gene S100. 74 Targeting those integrins decreased exosomal uptake, and lung and liver metastasis. 74 Other membrane proteins and lipids that are associated with ECM properties and adhesion influence the specific targeting of exosomes to their specific host cells. 74 , 75 , 76 , 77 , 78 In addition, exosomal internalization by target host cells activates heterogeneous endocytic pathways such as clathrin, lipid raft, and caveolin-mediated uptake. 65 , 79 , 80
Exosome-mediated metastasis is not solely dependent upon tumor-released exosomes. In fact, astrocyte-derived exosomes mediate the intercellular transfer of miRNAs that target the PTEN tumor suppressor gene to metastatic cancer cells, promoting invasion and brain metastasis. 81 This, in turn, leads to the increased secretion of chemokine ligand 2 (CCL2), which recruits myeloid cells, enhancing the outgrowth of brain metastatic cells and reducing the effect of apoptotic signaling. 81 Inhibition of astrocytic exosomal release prevents PTEN loss and suppresses brain metastasis. 81
Can metastasis be driven by epigenetic factors?
Age-related physical changes in the ECM promote or inhibit tumor cell motility, invasion, and metastasis. Alterations in the motility of immune cells lead to changes in the immune microenvironment. 82 Elderly patients with melanoma tend to develop fewer metastases in proximal lymph nodes but have more distal metastases, with worse survival than that of younger cohorts. 83 Through in vitro analysis, increased lymphatic permeability of endothelial membranes was shown to be the reason for this phenomenon, as lymph nodes of older patients exhibited less ECM complexity in comparison with that of lymph nodes of younger patients with metastatic melanoma. 83 Further analysis revealed that hyaluronan and proteoglycan link protein 1 ( HAPLN1 ) is responsible for controlling endothelial permeability. 82 , 83 Gene knockout increased endothelial permeability and the invasive ability of disseminating melanoma cells. 83 Other factors, such as decreased vascular endothelial-cadherin-dependent cell–cell adhesion and weak cell–ECM adhesion through α1 and β1 integrins, play a role in increasing lymph node permeability. 83
Chromatin mutations have recently come to light as important mediators of cancer development. Chromatin alterations induce cells to gain full oncogenic characteristics. 84 Furthermore, genetic, environmental, and metabolic conditions influence chromatin to become permissive or restrictive. 84 Epigenetic plasticity is exhibited when permissive chromatin induces oncogenic expression to promote metastatic development. 84
How does the microbiome contribute to cancer metastasis?
The concept of the “tumor microbiome” originates from the fact that bacteria have been detected within tumors themselves. Although no links to patient outcomes and survival have been established, microbes have been reported to confer vulnerability to specific cancers. 85
Bacterial translocation selectively targets tumors that have rich vascular networks and chemotactic magnetism. Anaerobic and/or facultative bacteria, specifically, vigorously survive in hypoxic tumor microenvironments. 86 , 87 Tumoral bacteria are metabolically active, leading to alterations in the chemical structure of some chemotherapeutic agents and affecting the response to therapy. 88 , 89 Gammaproteobacteria located in pancreatic tumors confer resistance to gemcitabine, a commonly used drug in gastrointestinal cancers. 89 , 90 , 91 Fusobacterium nucleatum also promotes resistance in colorectal cancer by initiating autophagy and activating Toll-like receptors on cancer cells. 92
Intratumoral bacteria further modulate the immune system. Although some bacteria stimulate antitumoral immunity, others promote immunosuppression, affecting the response to immunotherapy. 86 , 93 , 94 , 95 , 96 , 97 , 98 The Fap2 protein of Fusobacterium prevents the activation of natural killer (NK) cells, protecting adenocarcinoma cell lines from NK cell antitumor activity. 99
Does the circadian cycle play a role in tumorigenesis?
The circadian clock controls a wide spectrum of processes in cellular physiology through metabolic and gene expression pathways. 100 In the past decade, epidemiological studies on night-shift workers, meal timing, and exposure to light have linked alterations in circadian patterns to tumorigenesis, 101 , 102 , 103 , 104 , 105 , 106 , 107 indicating that an active epigenetic mechanism may be responsible for wide-genome alterations.
Circadian clock disruptions have been correlated with cancer initiation and progression. Further alterations in transcription complexes and cellular metabolism drive cancer progression by influencing cancer cell interactions with the microenvironment. 100 The MYC oncogene plays a role in cyclical metabolism in osteosarcoma cells, leading to increased consumption of glucose and glutamine. 108 Moreover, a number of circadian regulating genes have been linked to MYC expression. Cryptochrome circadian regulator 2, a circadian repressor, promotes MYC degradation. 109 Furthermore, zinc finger and BTB domain-containing protein 17 (MIZ1), a MYC-binding protein, downregulates core clock gene expression. 110 In addition, brain and muscle ARNT-like 1 expression is inversely correlated with MYC. 110 However, further research is needed to elucidate the mechanism through which other circadian inputs, such as nutrition, affect circadian metabolism and metastasis. CD36 + metastasis-initiating cells rely on palmitic acid, a dietary lipid, to promote metastasis. Blocking CD36 inhibits metastatic ability, suggesting that a high-fat diet specifically boosts the metastatic potential of metastasis-initiating cells. 111
Invasive cancer cells: remodeling the extracellular matrix
The ECM is a scaffold of interconnected macromolecules forming networks that encompass cells present in tissues and organs. 112 This specialized niche alters the phenotypic properties of cells and affects their propensity to proliferate, migrate, and survive. 113 , 114 Upon physiological and pathological triggers, ECM-degrading enzymes, called matrikines, are released to remodel the ECM, to re-establish an appropriate functional meshwork and maintain tissue homeostasis. 114 , 115 In cancer metastasis, ECM remodeling is hijacked, leading to stromal tumorigenesis. 116 , 117 , 118 , 119 , 120 A variety of major ECM components, such as proteoglycans, collagen, laminins, fibronectin, elastin, other glycoprotein, and proteinases, are involved in the invasive and metastatic processes of cancer cells.
One important step in invasion is the disassembly of the ECM and its constituents through enzymes such as matrix metalloproteinases (MMPs). 121 MMPs play a major role in cell proliferation, survival, immune response, and angiogenesis, in addition to invasion. 122 , 123 MMPs are elevated in most cancer types and are continuously associated with poor prognosis. 124 , 125 Cancer cells adjust the metastatic niche to drive growth by remodeling the ECM. The changes in nutrient accessibility and metabolic reactions in tissues determine the likelihood of cancer cells to metastasize. For example, metastatic breast cancer cells metabolize pyruvate, which is plentiful in the lungs, to drive collagen-based ECM remodeling in the lung metastatic niche. 126
Versican, a hyalectan that is present in interstitial ECM, activates EGFR signaling via its EGF-like repeats, which leads to cancer cell growth and invasion. 127 , 128 Chondroitin sulfate proteoglycan 4 (CSPG4) is another ECM component that plays an integral role in stabilizing the interactions between cells in the ECM matrix. CSPG4 interacts with integrin α2β1 upon collagen type VI binding to activate the phosphatidylinositol 3-kinase (PI3K) pathway in sarcoma cells. 129 In addition, CSPG4 forms complexes with MMP-2 and membrane type 3 MMP on the surface of melanoma cells to facilitate MMP-2 activation and eventual degradation of the ECM. 130
Lumican is an ECM protein that organizes fibril organization and circumferential growth. It plays a major role in corneal transparency, epithelial cell migration, and tissue repair. In cancer, lumican attenuates the proliferation, migration, and invasion of breast cancer cells. It modifies cellular junctions and promotes MET 131 through direct interactions with other ECM molecules or by the modulation of membrane receptors 132 , 133 and MMP-14. 134 , 135 , 136
Glypicans are proteoglycans that participate in developmental morphogenesis. They play a dual role in fostering or suppressing tumorigenesis. 114 , 137 Glypican-3 exhibits a tumor suppressor phenotype. Decreased glypican-3 expression leads to the progression of malignancies, whereas its loss is associated with poor overall survival. 138 However, elevated expression of glypican-3 correlates with reduced cancer cell differentiation and the presence of lymph node metastases in lung cancer. 139 , 140 Glypican-5 overexpression also promotes tumor progression and metastasis in salivary adenoid cystic carcinoma and in rhabdosarcoma. 141 , 142 , 143
Serglycin is an intracellular proteoglycan that is expressed by hematopoietic cells. Its expression drives cancer growth and metastasis. 144 Serglycin induces EMT and chemoresistance, as well as enhances the biosynthesis of proteolytic enzymes that aid in ECM remodeling. 145 In breast cancer, serglycin activates CD44/CREB1 signaling to enhance the secretion of transforming growth factor-β (TGF-β2) and EMT. 146 In non-SCLC and head-and-neck cancers, serglycin activates CD44/NF-κB/claudin-1 cells and mitogen-activated protein kinase (MAPK)/β-catenin signaling to drive EMT and chemoresistance. 147 , 148 Serglycin inhibition restricts the development of metastasis through decreased expression of chemokines such as CCL2. 149 Serglycin overexpression controls the secretion of tumor-derived exosomes and their ability to trigger cancer cell invasion and metastasis. 150
Hyaluronic acid (HA) is a glycosaminoglycan that is a principal constituent of the tumor stroma and cancer cell surfaces. It is an important EMT mediator and metastatic cancers express increased levels of HA, its CD44 receptor, and its synthase in the tumor cell microenvironment, 151 particularly in breast, oral, prostate, and ovarian cancers. 152 , 153
HA-mediated EMT enhancement is driven by the expression of zinc finger E-box-binding homeobox 1 (ZEB1) and its interaction with CD44, which in turn activates HA synthase 2 ( HAS2 ) expression. 154 HAS2 expression regulates TGFβ-induced EMT 155 through the expression of fibronectin, snail 1, and ZEB1 . HAS2 has also been shown to be vital for the communication between cancer stem cells and tumor-associated macrophages (TAMs). 156 This interaction leads to enhanced secretion of platelet-derived growth factor-BB from TAMs, which activates stromal cells and rejuvenates cancer stem cells. 156 Inhibiting HAS2 activity via 4-methylumbelliferone limits HA synthesis and prevents metastasis in several cancer models. 156 , 157 , 158 , 159
The striking effect of HA on tumor progression is highly associated with its molecular weight and interactions with other proteins in the ECM. 160 , 161 Low molecular weight (LMW) HA has well‐established tumorigenic proprieties. 161 , 162 In breast cancer, decreasing LMW‐HA production significantly inhibits cancer cell migration and invasion. 163 Moreover, excess LMW‐HA in the tumor microenvironment facilitates lymphatic metastasis via disruption of intercellular adhesion among lymphatic endothelial cells. 164 In addition, in the tumor interstitial fluid of colorectal cancer patients, LMW‐HA concentrations are increased and associated with lymphatic vessel invasion by cancer cells, and the development of lymph node metastases. 160
Altogether, the ECM is a complex and dynamic system that is composed of a wide spectrum of cells and matrikines that participate in invasion and metastasis.
How does autophagy contribute to cancer cell invasion?
Autophagy, the autophagosomal–autolysosomal process, is initiated by the advancement of various human cancers to metastasis. In vivo studies show that autophagy is involved in modulating tumor cell motility and invasion, cancer stem cell viability and differentiation, resistance to anoikis, EMT, metastatic cell dormancy, and escape from immune surveillance, with developing functions in forming the PMN and other metastatic facets. 165 Autophagy inhibition does not affect cell viability, proliferation, or migration but significantly reduces cellular invasion. 166 It was suggested that membrane-trafficking may play a critical role in the benign-to-malignant transition that is also central to the initiation of metastasis. 166
Can neurons initiate metastasis?
It has always been puzzling how nerves emerge in the tumor microenvironment and what their role might be. Neural progenitors from the central nervous system that express doublecortin infiltrate prostate tumors and metastases. 167 These progenitors initiate neurogenesis, which is the process by which neurons are produced from neural stem cells. 168 These nerve fibers in the tumor microenvironment regulate cancer initiation and dissemination, providing insights into how doublecortin-expressing neurons can be targeted for therapy.
Does the immune environment at the primary site play a role in metastasis?
The immune microenvironment around the tumor plays a major role in dictating the metastatic potential of the disseminating cells. A study analyzed tumors from more than 800 people with colorectal cancer, comparing people whose tumors were metastatic with those who were not. 35 The primary tumors from both groups had analogous mutation patterns in cancer genes; however, tumors that had metastasized had fewer cytotoxic T cells. 35 In addition, the invasive ends of the spread tumor cells had reduced densities of lymphatic vessels that carry immune cells. 35 Such changes contribute to metastasis and suggest that immunotherapies that enhance T-cell responses can stop metastasis in people with early-stage cancer. 169 Moreover, silencing the IFN regulatory factor (Irf)-7 pathway helps metastatic cells to escape immune surveillance. 170 In fact, a substantial number of genes that are suppressed in bone metastases are targets of Irf7 and restoration of Irf7 in tumor cells or administration of IFN led to decreased bone metastases and longer survival time. 170 In mice that are deficient in the IFN receptor or in NK and CD8(+) T-cell responses, metastasis was faster, indicating that Irf7-driven suppression of metastasis depends on IFN signaling to host immune cells. 170 , 171
Can surgical intervention contribute to metastatic dissemination?
Sometimes, disseminated cancer cells survive and retain the ability to invade even after the removal of the primary tumor. Often, patients with pancreatic ductal adenocarcinoma develop liver metastases following surgical excision of the primary tumor. 172 Metastasis possibly arises from dormant disseminated cancer cells that evade elimination by the immune system and are present at the time of surgery. 172 Analyzing mouse models and tissue samples from patients with pancreatic ductal adenocarcinoma showed that dormant disseminated cancer cells do not express a cell surface molecule that elicits T-cell-mediated attacks. 172 This phenotype is related to their inability to relieve endoplasmic reticulum stress. 172 When this stress is lifted, disseminated cells start multiplying and invading to form metastases. 172
Anesthetics during surgery also have an underlying mechanism in promoting metastatic dissemination. In murine models of breast cancer, sevoflurane led to significantly increased lung metastasis compared with that of propofol. 173 Interestingly, sevoflurane increased interleukin (IL)-6 levels, which in turn led to signal transducer and activator of transcription (STAT)-3 activation and the subsequent infiltration of myeloid cells into the lung. 173 By altering the tumor microenvironment through cytokines, anesthetics can promote cancer metastasis.
Intravasation
Intravasation, the dissemination of cancer cells to organs through the lumen of the vasculature, is mediated actively or passively. 12 , 174 This depends on the tumor type, microenvironment, and vasculature. 175 A three-dimensional microfluidic model shows that the endothelium poses a barrier to tumor cell intravasation and is regulated by factors that are present in the tumor microenvironment. 176 Using live-cell fluorescence microscopy and a tissue-engineered tumor-microvessel platform, a mitosis-mediated mechanism whereby tumor cells located along the vessel periphery disrupt the vessel endothelium through cell division and detach into circulation was elucidated. 177 Furthermore, the architectural constraints of tissue impose some mechanical pressures on invading tumor cells during intravasation. 178 Nuclear squeezing is particularly challenging on the integrity of the nucleus of the invaded cell. This causes genomic rearrangement to occur, which increases the metastatic potential. 178
Integrins are the key cellular adhesion receptors that are involved in nearly every step of cancer progression from primary tumor development to metastasis. 179 Altered integrin expression is frequently detected in tumors, where integrins have roles in supporting oncogenic growth factor receptor (GFR) signaling and GFR-dependent cancer cell migration and invasion. 179 Furthermore, integrins regulate the colonization process in metastatic locations by easing anchorage-independent survival of circulating tumor cells (CTCs). Metastatic cells use E-cadherin in metastatic sites to detach, disseminate, and seed. 180 This promotes metastatic cell survival and blocks reactive oxygen-mediated apoptosis. 180 As such, inhibiting E-cadherin in metastatic breast cancer cells may hold therapeutic potential against breast cancer. 180
Circulation
How do tumor cells survive in circulation.
The circulatory journey is harsh for most intravasating cancer cells. Interactions between CTCs and the microenvironmental components of circulation determine survival and the ability of CTCs to eventually extravasate in distant sites. 181 , 182 , 183
Most CTCs circulate as single cells, whereas others travel in clusters (Fig. 4 ). However, circulating clusters are much more likely to form metastases. 184 In addition to the invading cancer cells, clusters contain stromal cells and immune components from the original microenvironment that contribute to the heterogeneity of the cluster and enhance its survival. 184 , 185 , 186 , 187 , 188 Neutrophils participate in cluster formation and suppress leukocyte activation, which increases the chances of CTC survival. 189 Moreover, the interaction of CTCs with platelets leads to the formation of a coating shield of platelets around cancer cells that prevents CTC detection by immune cells and provides the structure needed to bear the physical stresses of circulation. 190 , 191 , 192
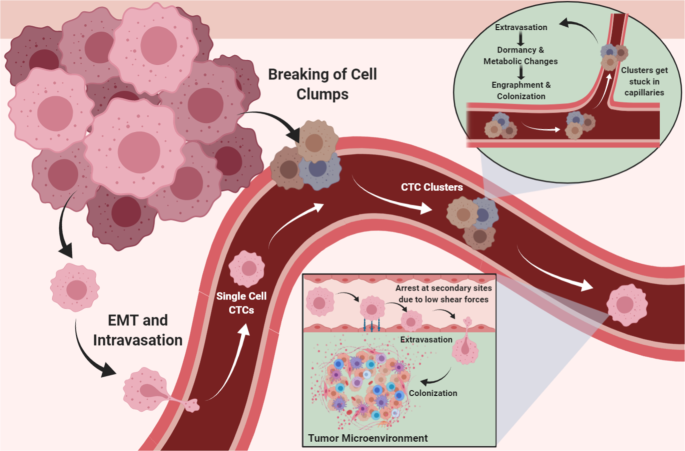
Cancer cells circulate as single units or in clusters. After arresting at secondary sites or becoming stuck in capillaries, circulating tumor cells (CTCs) extravasate and colonize their new niches. Some cells undergo dormancy as an adaptation mechanism to the new stressful environment
An important factor in the metastatic process is the ability of CTCs to adhere and extravasate through endothelial cells and colonize the PMN. 193 As soon as CTCs arrest in capillaries, they either extravasate by transendothelial migration or grow within the vessel before eventual extravasation and colonization of the PMN. 194 , 195 , 196 , 197
Do circulating tumor cells interact with immune cells?
CTCs must adapt to the strict selective environment present in the lumen of the vasculature. The dissemination of CTCs is supported by close association with activated platelets and macrophages. 198 Therefore, CTCs form heteroaggregates that sustain adhesion to the endothelium and thus contribute to metastasis. 190 However, this belief has been challenged by showing that an increase in megakaryocytes confers some measure of protection against metastasis. 199 In addition, neutrophils in circulation have been found to inhibit metastasis. 6 , 200 Blood sampling in 70 women with advanced-stage breast cancer showed a CTC–immune cell association. 201 The white blood cells that showed the greatest interaction were neutrophils, suggesting that neutrophil clustering with CTCs increases the metastatic potential of CTCs. 201 The advancement of the disease in people with advanced breast cancer was faster among individuals who had CTC–neutrophil clusters when compared with that of people who lacked such clusters. 201 Furthermore, CTCs from both CTC–neutrophil clusters and others that had not been part of a neutrophil cluster were injected into the bloodstream of tumor-free mice. A substantially increased number of metastases were found in the mice that received CTCs from CTC–neutrophil clusters. In addition, upon the eradication of neutrophils in mice with breast tumors, the number of CTC–neutrophil clusters was markedly decreased. 201 These mice had delayed metastases in the lungs when compared with those of mice bearing breast tumors that did not have their neutrophils depleted. 201 Moreover, the complex interchange between cancer cells and white blood cells facilitates metastasis, because metastatic cells possess sugar on their cell surface that binds to galectin-3. 202 This enhances the ability of cells to colonize by interacting with mobilized white blood cells. 202
Resisting vascular forces and mechanical pressure
The journey of CTCs in the blood vessels is not easy. CTCs sense and respond to tissue mechanics and instigate brief or lasting tissue alterations, including ECM stiffening, compression and deformation, protein unfolding, proteolytic remodeling, and jamming transitions. 203 Mechanical pressures are likely to be found during arrest of CTCs at distant sites, when exiting vessels (extravasation), and during metastatic growth. Permissive flow regimens in vascular regions, in addition to the location and efficiency of CTC lodging at distant sites, play large roles in the distant metastasis process. 204 The passage of CTCs through the bloodstream is halted when their adhesive capacity becomes greater than the shear forces imposed on them by the blood flow. 204 Therefore, regions with low hemodynamic flow are the regions where most CTCs stabilize and engage with endothelial cells. It is in such regions that single CTCs might sequentially form intravascular clusters. 205 Once CTCs are fixed in the microvasculature, they are fragmented by the flow of blood. This generates immune-interacting intermediate molecules that promote extravasation and develop metastases from the surviving CTCs. 206 , 207 This hypothesis was further tested in a cohort of 100 patients with brain metastases and found that these metastases formed in regions with low cerebral blood flow. 204 Therefore, shear forces play an important role in hematogenous metastasis and in determining the location of the final arrest.
How does the release of chemokines and cytokines help circulating tumor cells?
The migration of metastatic cells in circulation often relies on a spectrum of chemokines and complement components that direct tumor cells through the vasculature 208 , 209 and metabolic factors that result in an antioxidant effect. 210 Granulocyte macrophage colony-stimulating factor and cytokines such as IL-5, which are induced in obesity, lead to lung neutrophilia in obese mice and aid in breast cancer metastasis. 211 In addition, when crowded, cancer cells boost the production of IL-6 and IL-8, two immune molecules that stimulate biochemical pathways and facilitate tumor migration. 212 In mouse breast cancer models, blocking IL-6 and IL-8 receptors through experimental treatments minimized metastasis at lymph nodes, lungs, and liver compared with those of the control groups. 212 Further data suggest that metastatic tumors induce the release of IL-1β, which induces gamma delta (γδ) T cells to release IL-17, suppressing cytotoxic CD8 + T lymphocytes and promoting metastasis. 213 In addition, the loss of TP53 in cancer cells induces the secretion of WNT ligands that stimulate the production of IL-1β, thus driving prometastatic neutrophilic inflammation. 214
Is tumor cell circulation contingent on the route of the bloodstream?
It is now accepted that CTCs can exploit and survive in the bloodstream during tumor metastasis. 204 , 207 However, CTCs have also been found to cause distant metastases through the lymphatic circulation. 215 , 216 , 217 The “sequential progression model” is the basis for excision of tumor-draining lymph nodes during surgery. 215 Metastatic cancer cells can travel from a primary tumor to a distant site via two courses: directly through the bloodstream or through a lymph node near the primary cancer site. 218
The biological mechanisms by which tumor cells survive and grow within lymph nodes are not yet clear. In murine models, cancer cells acclimatize to the lymph node microenvironment by shifting their metabolism to fatty acid oxidation. 218 The signaling pathway on which the adaptation process is based is driven and activated by the yes-associated protein (YAP) transcription factor. 218 Notably, inhibition of fatty acid oxidation or YAP signaling blocked lymph node metastasis in mice. 218
Diagnostics in circulation: where are we now?
Enrichment of CTCs allowed their classification and subsequent tumor analysis. 219 CTC characterization helps reflect on the molecular foundations of metastatic tumors, whereas cell-free DNA (cfDNA) offers new genetic material for further exploration in trials. 220 cfDNA reflects the heterogeneity of CTCs in patients with high counts of CTCs and thus enables monitoring of the metastatic burden for clinical decision-making. 221 In addition, cfDNA profiling tracks the subclonal nature of cancer metastasis. 222 As such, liquid biopsy of CTCs and/or cfDNA in the peripheral blood might have the potential to further the current understanding of metastasis biology. 219 However, it is worthwhile to ponder whether currently used techniques for enrichment and detection of CTCs allow us to identify actual metastasis‐initiating cells and whether liquid biopsy can be used to investigate the effectiveness of cancer treatment.
Targeting circulating tumor cells: can it be done?
For a long time, the low sensitivity of CTC detection assays has halted CTC elimination. In addition, the exclusion of patients with metastasis from clinical trials prevented faster progress. 223 However, advancements in the field have changed the reigning paradigm and offered hope for future success. A photoacoustic method for direct use in patients with melanoma has been developed, allowing for the detection of very low numbers of CTCs in vivo and their subsequent destruction with laser pulses. 224 This reflects the therapeutic potential of such approaches. In addition, distinct DNA methylation profiles are present among CTC clusters from patients and murine models with breast cancer when compared with that of single CTCs. 225 This, along with the phenotypic differences, can be targeted in future therapeutic options.
Extravasation
How do circulating tumor cells extravasate.
When CTCs pass through small capillaries, they become entrapped. This either leads to microvascular rupture or forces the cell to undergo extravasation. 3 As organs such as the liver and bone have highly permeable sinusoidal vessels, CTCs exhibit a high rate of metastasis in these organs. 12 In other organs, extravasating cells are faced with tight barriers and basement membranes that require genetic and molecular mediation to be able to transmigrate.
Extravasation is a complex process that involves ligand–receptor interactions, chemokines, and circulating nontumor cells. 174 , 226 , 227 Integrins, again, play a vital role in determining the sites at which extravasation and colonization occur by facilitating anchorage-independent survival of CTCs. 179
Many have reported that cancer cell extravasation occurs in a similar fashion to leukocyte transendothelial migration. 174 , 228 , 229 In recent years, it has been shown that cancer cells induce programmed necrosis of endothelial cells, driving metastatic cells to extravasate. Treatment with the receptor-interacting serine/threonine-protein kinase (RIPK)-1-inhibitor necrostatin-1 or endothelial-cell-specific deletion of RIPK3 reduced endothelial necroptosis and metastatic extravasation. 230
Are circulating tumor cells target-specific?
Organotropism was first touched upon by Paget as part of the “seed and soil” hypothesis. 231 Breast cancer research has supported this hypothesis, 232 , 233 with researchers elucidating the genetic basis for cancer colonization in distant organs. 234 Moreover, the host microenvironment and the adaptive process that invading cancer cells undergo play a role in extravasation and colonization of cancer cells at specific sites. 235 For example, breast cancer most frequently metastasizes to the bone, often after long latency, suggesting that metastatic seeds are resistant to therapy and can regrow (Fig. 5 ). Calcium flux, for instance, has been identified as a mechanism of crosstalk between the osteogenic niche and cancer cells, which promotes the progression of bone metastasis. 236 Another example involves patients with postpartum breast cancer, who are at elevated risk for liver metastasis. 237 The identification of the “weaning-induced liver involution,” which establishes a metastatic microenvironment, may account, in part, for the poor prognosis of patients with postpartum breast cancer. 237
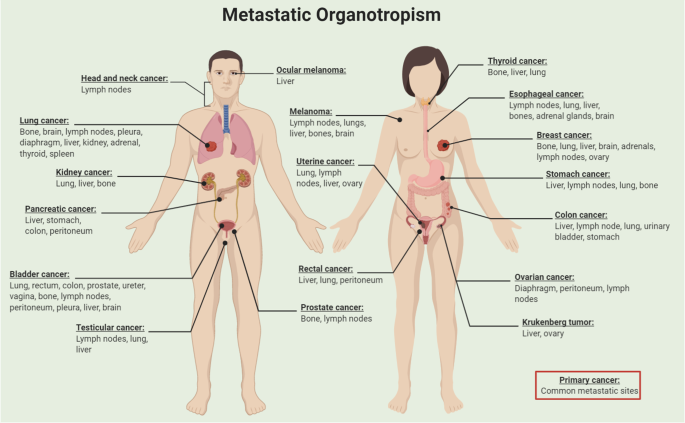
Metastastatic Organotropism: Clinical observations suggest that most cancers metastasize to specific target organs, a process known as “metastatic organotropism”
Colonization
How does the colonizing cell overcome stromal challenges.
Circulating cells that extravasate at the target site are challenged with harsh conditions that make survival difficult. 22 A number of secreted tumor-derived factors and bone marrow-derived cells signal the formation of the PMN, in which the tumor cells colonize and grow. 12 , 238 , 239 , 240 In addition to tumor-derived factors, exosomes play a major role (discussed earlier). Exosomes have a role in educating bone marrow progenitor cells to become metastatic. 240 Further research showed similar results in pancreatic cancer, whereby exosomes initiated PMN formation in the liver. 73 However, cancer cell–host cell interactions are also important for proper colonization. Hepatocytes control myeloid cell accumulation and fibrosis within the liver and thus increase the susceptibility of the liver to metastatic colonization. In murine models of pancreatic cancer, hepatocytes induce IL-6-mediated STAT3 signaling and increase secretion of serum amyloid A1 and A2 (SAA). Inhibition of IL-6-STAT3-SAA signaling prevents the establishment of a PMN and inhibits liver metastasis. 241
Establishing a vascular network is crucial for proper metastatic colonization. Vascular mimicry drives the ability of some breast cancer cells to contribute to distant metastases through the overexpression of SERPINE2 and SLPI. 242 These two genes are overexpressed preferentially in human patients with breast cancer lung metastases, suggesting their potential for metastatic progression. 242
Colonizing cancer cells are also capable of utilizing neuronal signaling pathways for growth and adaptation. The proximity of breast cancer cells to neuronal synapses allows cancer cells to hijack N -methyl- d -aspartate receptor signaling to promote brain metastasis. 243 Protocadherin 7 is a protein that promotes the assembly of cancer cell–astrocyte gap junctions composed of connexin 43. Metastatic cancer cells use these junctions to transfer the second messenger cGAMP to astrocytes, activating the stimulator of interferon genes pathway and producing inflammatory cytokines such as IFNα and tumor necrosis factor. In turn, these factors activate STAT1 and NF-κB pathways in brain metastatic cells, thereby supporting tumor growth and chemoresistance. 244
Dormancy: why do cancer cells go to sleep?
By definition, cancer dormancy is an arrest phase in cancer progression that occurs during the primary tumor formation phase or after invasion into secondary sites. 245 Metastatic dormancy specifically occurs due to the delayed acclimatization of disseminating cancer cells to their secondary niches 246 and affects single invading cells or cancer clusters after circulation.
In many cancer survivors, dormant cancer cells are present long after radical removal of the primary tumor and are thought to be responsible for late relapses. 246 Dormancy constitutes quiescence, angiogenic dormancy in which an equilibrium is realized between dividing and dying (vascular-lacking) cancer cells, and immune-mediated dormancy in which the tumor mass is preserved by immune cell cytotoxicity. 12 , 247 Some believe that the target organ microenvironment instructs CTCs to enter dormancy, whereas others think that primary tumors pre-encode a dormancy signature on CTCs that only becomes evident when CTCs enter the host microenvironment. Another potential explanation is that early dissemination spawns CTCs that respond to dormancy-inducing signals and enter dormancy in target organs. 248
What are the mechanisms that govern dormancy?
Regulation of tumor cell dormancy involves reciprocal crosstalk between the environment and mechanisms that control transcriptional programs. 249 Single-cell dormancy describes the reversible state of quiescence that the metastatic cell enters in response to stressful stimuli, while expressing the Ki67 proliferation marker. 245 Metabolic homeostasis is maintained in the dormant state through the downregulation of two of the most well-studied pathways that are activated during oncogenesis, the RAS–MEK–ERK/MAPK and PI3K-AKT signaling cascades, which play critical roles in governing cancer cell dormancy. 250
Factors secreted by the PMN, such as mesenchymal cell‐derived bone morphogenetic proteins (BMPs) and growth arrest‐specific 6 produced by osteoblasts, also shift cancer cells towards dormancy. 251 , 252 BMP7 activates the metastatic suppressor gene N‐myc downstream‐regulated gene 1 ( NDGR1 ), leading to an increase in p38 MAPK activation, cell cycle inhibitor p21 expression, and cell cycle arrest. 251
Molecular interactions between mitogen‐ and stress‐induced signaling are vital in regulating the dormancy/activation state of metastatic cancer cells. The ratio of extracellular signal‐regulated kinase (ERK1/2) to p38 MAPK regulates the cell cycle. High levels of ERK1/2 activity favor proliferation, whereas high levels of p38 favor dormancy. Increased p38 MAPK activity triggers the activation of the unfolded protein response, which upregulates activating transcription factor 6, thus promoting cell arrest and survival. 253 , 254 These observations support the notion that the activation of stress signaling pathways induces a sustained state of quiescence that is linked to dormancy (Fig. 6 ).
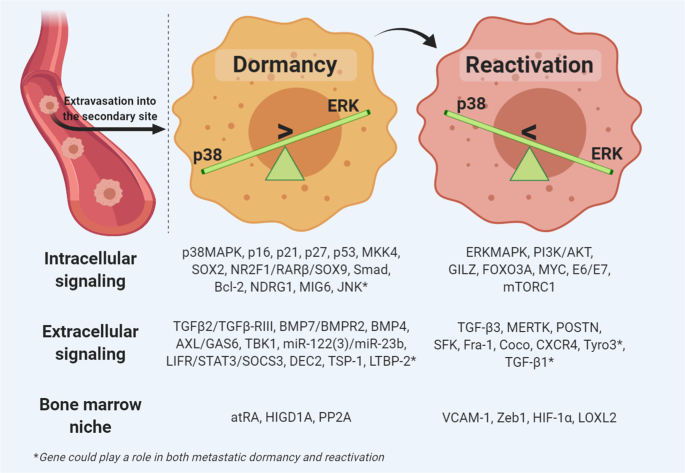
Dormancy and reactivation of cancer cells: The genetic and signaling pathways that govern cancer cell dormancy and subsequent reactivation involve intracellular signaling, extracellular signaling, and induction signals originating from the bone marrow niche
How does dormancy occur in metastatic clusters?
Dormancy in metastatic cancer clusters occurs when the rate of cellular proliferation within the cluster is equal to the rate of apoptosis. As such, the tumor cluster does not expand into micrometastasis. This balance is achieved through suppressive gene signaling, restricted angiogenesis, and/or an active immune microenvironment. 245 Suppressive gene signaling can be achieved through the induction of differentially expressed in chondrocytes 2 ( DEC2 ), a tumor suppressor gene. TGFβ induces DEC2, which inhibits cyclin-dependent kinase 4 and activates p27, forcing the cell to enter a state of quiescence. 255 Blocking blood vessel formation through activation of thrombospondin-1 256 or through the inhibition of chaperones, such as heat shock 27 kDa protein, pushes metastatic clusters into a dormant state. 257 The immune system is also a major factor in cancer antiproliferation. T cells and NK cells, in addition to macrophages, clear metastatic cells through cytolysis. 258 Dormant tumor cells express weak antigens to escape the immune system, which could be the reason behind relapse following immunotherapy. 249
From dormancy to activation: how does the dormant cell wake up?
Researchers have begun to understand the process that allows certain cancer cells to become dormant for periods of time and emerge later with recurring disease. These cancer cells enter a state of latency and slow division by inhibiting a WNT protein-driven signaling pathway. 246 In addition, these cells exhibit increased levels of the stem cell genes SRY-box (SOX)-2 and SOX9, which allow for the growth of new tumors if certain conditions exist. To reduce the ability of the immune system to identify them, these dormant cancer cells downregulate the expression of immune cell-recognizable molecules. 246 This allows tumor cells to evade an immune response until conditions allow the development of metastasis. Persistent host organ inflammation and the complementary establishment of neutrophilic extracellular traps may transform dormant cancer cells into aggressive metastases. 259 Others believe that the shift from dormancy to activation occurs with respect to organotropism, 3 indicating that the host microenvironment plays a role in waking the cells from their dormant state. 260 More importantly, it has been established that high levels of ERK1/2 with respect to p38 MAPK promote reactivation.
Cancer cell plasticity and tumor progression
Cancer cell plasticity facilitates the development of therapy resistance and malignant progression. Plasticity bestows upon cancer cells the ability to dynamically switch between a differentiated state, with limited tumorigenic potential, and an undifferentiated or cancer stem-like cell state, which is responsible for long-term tumor growth. However, researchers remain hopeful that cancer cell plasticity can be exploited therapeutically. Some have forced the transdifferentiation of EMT-derived breast cancer cells into post-mitotic and functional adipocytes by using a combination therapy of MEK inhibitors and the antidiabetic drug rosiglitazone, thereby inhibiting the metastatic process. 261 Genome-wide in vivo screens can identify novel host regulators of metastatic colonization. In vivo studies have identified multiple genes that, when disrupted, modify the ability of tumor cells to establish metastases. 262 Often, endovascular progenitor cells function as precursors of endothelial cells. 263 These progenitor cells express the transcription factor SOX18 and are thus unaffected by therapies that target vascular endothelial growth factor. By ablating Notch signaling, SOX18 is inhibited, which subsequently halts melanoma metastasis in murine models. 263
In many instances, glucocorticoids are used to treat patients with cancer-related complications. The progression of breast cancer is initiated by increasing stress hormone and glucocorticoid levels, which subsequently activates secondary site glucocorticoid receptors, enhances cancer colonization, and decreases survival rates. 202 This suggests the use of caution when treating cancer patients with glucocorticoid therapy.
Despite the displayed effectiveness of cytotoxic chemotherapy in treating invasive breast cancer, it has been shown that the treatment displays prometastatic effects. 264 Paclitaxel and doxorubicin trigger the production of tumor-derived extracellular vesicles in models of chemoresistant breast cancer in mice. 264 These vesicles facilitate the colonization of tumors at metastatic sites in the lungs. 264
Suppressing the suppressor
Metastasis suppressors inhibit cancer growth and proliferation at the metastatic site without affecting the primary tumor. 265 , 266 They target oncogenic pathways and proteins that are involved in invasion and eventual metastatic colonization. For example, A-kinase anchor protein 8 is a splicing regulatory factor that suppresses EMT and breast cancer metastasis. 267 In highly metastatic cells, metastasis suppressors are usually downregulated in comparison with primary tumor cells. 265 , 268 , 269 In the past decade, a significant number of metastasis suppressors have been identified (Fig. 7 ). Most notably, miRNAs that suppress oncogenes and inhibit tumorigenic signaling have been recognized and explored as potential biomarkers and targets of metastasis. 265 , 270 , 271 , 272 , 273 , 274 , 275 , 276 , 277 , 278 , 279 , 280 , 281 , 282 , 283 , 284 , 285 , 286 , 287 , 288 , 289 , 290 , 291 , 292 , 293
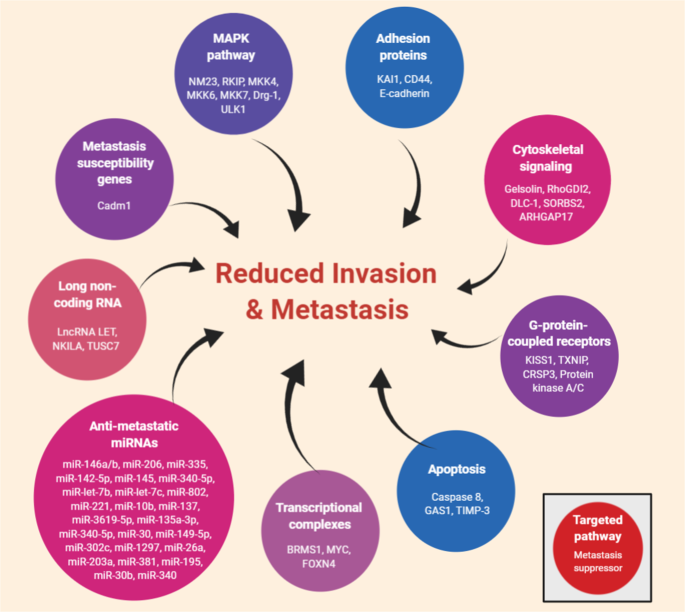
Metastasis suppressor genes that have been identified in the literature: Metastasis suppressors halt metastatic proliferation at the secondary site without changing the primary cancer. They work through oncogenic signaling pathways to suppress invasion and eventual colonization
Therapeutic strategies to target the pathways of metastasis
The field of metastasis research is more than 100 years old. However, metastasis remains the primary cause of cancer-related deaths. Major obstacles lie in the lack of clinical trials that target metastasis and the lack of knowledge of the biological underpinnings that govern the metastatic process. 169 , 171 , 220 , 223 Designing targeted therapies for metastatic cancer cells should take into account the genetic and phenotypic differences between parental and metastatic/circulating cells. 294
Today, the diagnosis of metastatic cancer continues to be associated with a terminal label. Although prevention of metastasis has been demonstrated preclinically, drug development has been hindered due to poor trial design and therapeutic strategies. Advancements in immunotherapy have improved survival and patient outcomes in metastatic melanoma. 295 , 296 In addition, the development of novel androgen receptor inhibitors extended the survival of metastatic prostate cancer. 297 However, long-term follow-ups have failed to demonstrate consistency in the survival benefits of patients with metastatic breast cancer. 298
Strategies that target pathways in the metastatic cascade have been studied and explored. 294 The seeding of cancer cells can be targeted by inhibiting intratumoral interactions, intercellular crosstalk through ECM adhesion molecules, the release of proteases, EMT, and intravasation. However, at the time of metastasis diagnosis, cancer cells may have already seeded in the circulatory system or colonized a distant site. 220 Therefore, targeting metastatic colonization seems to be the most plausible therapeutic strategy, as it correlates mostly with the clinical scene. Dormancy has also been studied as a potential target of metastatic colonization. Some have proposed therapies that help sustain the dormant state. 299 Others have designed combination treatments that target G0 tumor cells. Moreover, monoclonal antibodies have been developed to target single cancer cells at this stage. 300
In addition to CTCs (discussed earlier), the diagnostic and predictive potential of exosomes renders them key for liquid biopsies. 220 , 301 In terms of targeting tumor-secreted factors, exosome affinity plasmapheresis has been developed to trap exosomes with immunosuppressive or tumorigenic material (NCT02439008); however, the trial has been terminated due to a lack of patient accrual.
The brain continues to be a special site for metastasis, as colonizing cells are offered a safe haven through the existence of the blood–brain barrier (BBB). The BBB allows the crossing of tumor cells and prevents the passage of therapeutic agents. 302 Therefore, agents that are known to cross the BBB must be tested in brain metastasis settings and novel agents with the ability to cross the BBB must be designed. 223
Overall, metastasis is a complex challenge that requires more than one therapeutic agent for effective inhibition. Therefore, embracing the combination therapy model and targeting multiple pathways simultaneously seems to be key to countering the significant genomic and phenotypic alterations presented by metastatic cancer cells. 303
Concluding remarks
Metastasis is the final frontier in cancer for which more efficacious therapies are needed. However, the development of effective treatments is contingent upon understanding the underpinnings that govern the metastatic process from start to finish. As such, exploring metastatic evolution is necessary to be able to design better therapeutics in the future.
Luzzi, K. J. et al. Multistep nature of metastatic inefficiency: dormancy of solitary cells after successful extravasation and limited survival of early micrometastases. Am. J. Pathol. 153 , 865–873 (1998).
Article CAS PubMed PubMed Central Google Scholar
Maitra, A. Molecular envoys pave the way for pancreatic cancer to invade the liver. Nature 567 , 181–182 (2019).
Article CAS PubMed Google Scholar
Massague, J. & Obenauf, A. C. Metastatic colonization by circulating tumour cells. Nature 529 , 298–306 (2016).
Hanahan, D. & Weinberg, R. A. Hallmarks of cancer: the next generation. Cell 144 , 646–674 (2011).
Steeg, P. S. Tumor metastasis: mechanistic insights and clinical challenges. Nat. Med. 12 , 895–904 (2006).
Lambert, A. W., Pattabiraman, D. R. & Weinberg, R. A. Emerging biological principles of metastasis. Cell 168 , 670–691 (2017).
Bakhoum, S. F. et al. Chromosomal instability drives metastasis through a cytosolic DNA response. Nature 553 , 467 (2018).
Tabassum, D. P. & Polyak, K. Tumorigenesis: it takes a village. Nat. Rev. Cancer 15 , 473–483 (2015).
Gundem, G. et al. The evolutionary history of lethal metastatic prostate cancer. Nature 520 , 353 (2015).
Clark, A. G. & Vignjevic, D. M. Modes of cancer cell invasion and the role of the microenvironment. Curr. Opin. Cell Biol. 36 , 13–22 (2015).
Cheung, K. J. & Ewald, A. J. A collective route to metastasis: seeding by tumor cell clusters. Science 352 , 167–169 (2016).
Fouad, Y. A. & Aanei, C. Revisiting the hallmarks of cancer. Am. J. Cancer Res. 7 , 1016–1036 (2017).
CAS PubMed PubMed Central Google Scholar
Ye, X. & Weinberg, R. A. Epithelial-mesenchymal plasticity: a central regulator of cancer progression. Trends Cell Biol. 25 , 675–686 (2015).
Knott, S. R. V. et al. Asparagine bioavailability governs metastasis in a model of breast cancer. Nature . 554 , 378–381 (2018).
Nieto, M. A., Huang, R. Y. J., Jackson, R. A. & Thiery, J. P. Emt: 2016. Cell 166 , 21–45 (2016).
Katsuno, Y., Lamouille, S. & Derynck, R. TGF-beta signaling and epithelial-mesenchymal transition in cancer progression. Curr. Opin. Oncol. 25 , 76–84 (2013).
De Craene, B. & Berx, G. Regulatory networks defining EMT during cancer initiation and progression. Nat. Rev. Cancer 13 , 97–110 (2013).
Article PubMed CAS Google Scholar
Pastushenko, I. et al. Identification of the tumour transition states occurring during EMT. Nature 556 , 463 (2018).
Erdogan, B. et al. Cancer-associated fibroblasts promote directional cancer cell migration by aligning fibronectin. J. Cell Biol. 216 , 3799–3816 (2017).
Rankin, E. B. & Giaccia, A. J. Hypoxic control of metastasis. Science 352 , 175–180 (2016).
Lamouille, S., Xu, J. & Derynck, R. Molecular mechanisms of epithelial-mesenchymal transition. Nat. Rev. Mol. Cell Biol. 15 , 178–196 (2014).
Valastyan, S. & Weinberg, R. A. Tumor metastasis: molecular insights and evolving paradigms. Cell 147 , 275–292 (2011).
Fischer, K. R. et al. Epithelial-to-mesenchymal transition is not required for lung metastasis but contributes to chemoresistance. Nature 527 , 472–476 (2015).
Zheng, X. F. et al. Epithelial-to-mesenchymal transition is dispensable for metastasis but induces chemoresistance in pancreatic cancer. Nature 527 , 525 (2015).
Diepenbruck, M. & Christofori, G. Epithelial-mesenchymal transition (EMT) and metastasis: yes, no, maybe? Curr. Opin. Cell Biol. 43 , 7–13 (2016).
Esposito, M. et al. Bone vascular niche E-selectin induces mesenchymal-epithelial transition and Wnt activation in cancer cells to promote bone metastasis. Nat. Cell Biol. 21 , 627–639 (2019).
Lawson, D. A. et al. Tumour heterogeneity and metastasis at single-cell resolution. Nat. Cell Biol. 20 , 1349–1360 (2018).
Van’t Veer, L. J. et al. Gene expression profiling predicts clinical outcome of breast cancer. Nature 415 , 530 (2002).
Article Google Scholar
Ramaswamy, S., Ross, K. N., Lander, E. S. & Golub, T. R. A molecular signature of metastasis in primary solid tumors. Nat. Genet. 33 , 49–54 (2003).
Hunter, K., Welch, D. R. & Liu, E. T. Genetic background is an important determinant of metastatic potential. Nat. Genet. 34 , 23 (2003).
Robinson, D. R. et al. Integrative clinical genomics of metastatic cancer. Nature 548 , 297–303 (2017).
Birkbak, N. J. & McGranahan, N. Cancer genome evolutionary trajectories in metastasis. Cancer Cell. 37 , 8–19 (2020).
Article PubMed Google Scholar
Yang, D. et al. Intertumoral heterogeneity in SCLC is influenced by the cell type of origin. Cancer Discov. 8 , 1316–1331 (2018).
Ganesh, K. et al. L1CAM defines the regenerative origin of metastasis-initiating cells in colorectal cancer. Nat. Cancer 1 , 28–45 (2020).
Article PubMed PubMed Central Google Scholar
Mlecnik, B. et al. The tumor microenvironment and Immunoscore are critical determinants of dissemination to distant metastasis. Sci Transl Med . 8 , 327ra26 (2016).
Carnero, A. & Lleonart, M. The hypoxic microenvironment: a determinant of cancer stem cell evolution. Bioessays 38 (Suppl 1), S65–S74 (2016).
Ratcliffe, P. J. Oxygen sensing and hypoxia signalling pathways in animals: the implications of physiology for cancer. J. Physiol. 591 , 2027–2042 (2013).
Semenza, G. L. Oxygen sensing, hypoxia-inducible factors, and disease pathophysiology. Annu. Rev. Pathol. 9 , 47–71 (2014).
Harris, A. L. Hypoxia–a key regulatory factor in tumour growth. Nat. Rev. Cancer 2 , 38–47 (2002).
Garcia-Heredia, J. M., Felipe-Abrio, B., Cano, D. A. & Carnero, A. Genetic modification of hypoxia signaling in animal models and its effect on cancer. Clin. Transl. Oncol. 17 , 90–102 (2015).
Semenza, G. L. VHL and p53: tumor suppressors team up to prevent cancer. Mol. Cell. 22 , 437–439 (2006).
Semenza, G. L. Cancer-stromal cell interactions mediated by hypoxia-inducible factors promote angiogenesis, lymphangiogenesis, and metastasis. Oncogene 32 , 4057–4063 (2013).
Hockel, M. et al. Tumor oxygenation: a new predictive parameter in locally advanced cancer of the uterine cervix. Gynecol. Oncol. 51 , 141–149 (1993).
Brizel, D. M. et al. Tumor oxygenation predicts for the likelihood of distant metastases in human soft tissue sarcoma. Cancer Res. 56 , 941–943 (1996).
CAS PubMed Google Scholar
Noman, M. Z. et al. Crosstalk between CTC, immune system and hypoxic tumor microenvironment. Cancer Microenviron. 7 , 153–160 (2014).
Tasdogan, A. et al. Metabolic heterogeneity confers differences in melanoma metastatic potential. Nature 577 , 115–120 (2020).
Papalazarou, V. et al. The creatine–phosphagen system is mechanoresponsive in pancreatic adenocarcinoma and fuels invasion and metastasis. Nat. Metab. 2 , 62–80 (2020).
Peinado, H. et al. Pre-metastatic niches: organ-specific homes for metastases. Nat. Rev. Cancer 17 , 302–317 (2017).
Zomer, A. et al. In vivo imaging reveals extracellular vesicle-mediated phenocopying of metastatic behavior. Cell 161 , 1046–1057 (2015).
Cancer-cell transfer filmed. Nature 521 , 396–396 (2015). https://www.nature.com/articles/521396d .
Weidle, U. H., Birzele, F., Kollmorgen, G. & Ruger, R. The multiple roles of exosomes in metastasis. Cancer Genomics Proteom. 14 , 1–15 (2017).
Article CAS Google Scholar
Tickner, J. A. et al. Functions and therapeutic roles of exosomes in cancer. Front Oncol. 4 , 127 (2014).
Harris, D. A. et al. Exosomes released from breast cancer carcinomas stimulate cell movement. PLoS ONE 10 , e0117495 (2015).
Article PubMed PubMed Central CAS Google Scholar
Singh, R. et al. Exosome-mediated transfer of miR-10b promotes cell invasion in breast cancer. Mol. Cancer 13 , 256 (2014).
Higginbotham, J. N. et al. Amphiregulin exosomes increase cancer cell invasion. Curr. Biol. 21 , 779–786 (2011).
McCready, J., Sims, J. D., Chan, D. & Jay, D. G. Secretion of extracellular hsp90alpha via exosomes increases cancer cell motility: a role for plasminogen activation. BMC Cancer 10 , 294 (2010).
Fu, H., Yang, H., Zhang, X. & Xu, W. The emerging roles of exosomes in tumor-stroma interaction. J. Cancer Res Clin. Oncol. 142 , 1897–1907 (2016).
Soung, Y. H. et al. Emerging roles of exosomes in cancer invasion and metastasis. BMB Rep. 49 , 18–25 (2016).
Goubran, H. A. et al. Regulation of tumor growth and metastasis: the role of tumor microenvironment. Cancer Growth Metastasis. 7 , 9–18 (2014).
Syn, N. et al. Exosome-mediated metastasis: from epithelial-mesenchymal transition to escape from immunosurveillance. Trends Pharm. Sci. 37 , 606–617 (2016).
Aga, M. et al. Exosomal HIF1alpha supports invasive potential of nasopharyngeal carcinoma-associated LMP1-positive exosomes. Oncogene 33 , 4613–4622 (2014).
You, Y. et al. Matrix metalloproteinase 13-containing exosomes promote nasopharyngeal carcinoma metastasis. Cancer Sci. 106 , 1669–1677 (2015).
Franzen, C. A. et al. Urothelial cells undergo epithelial-to-mesenchymal transition after exposure to muscle invasive bladder cancer exosomes. Oncogenesis 4 , e163 (2015).
Jeppesen, D. K. et al. Quantitative proteomics of fractionated membrane and lumen exosome proteins from isogenic metastatic and nonmetastatic bladder cancer cells reveal differential expression of EMT factors. Proteomics 14 , 699–712 (2014).
Escrevente, C., Keller, S., Altevogt, P. & Costa, J. Interaction and uptake of exosomes by ovarian cancer cells. BMC Cancer 11 , 108 (2011).
Tarbe, N. et al. Identification of rat pancreatic carcinoma genes associated with lymphogenous metastasis. Anticancer Res. 22 , 2015–2027 (2002).
Mu, W., Rana, S. & Zoller, M. Host matrix modulation by tumor exosomes promotes motility and invasiveness. Neoplasia 15 , 875–887 (2013).
Fabbri, M. et al. MicroRNAs bind to Toll-like receptors to induce prometastatic inflammatory response. Proc. Natl Acad. Sci. USA 109 , E2110–E2116 (2012).
Ye, S.-b et al. Tumor-derived exosomes promote tumor progression and T-cell dysfunction through the regulation of enriched exosomal microRNAs in human nasopharyngeal carcinoma. Oncotarget 5 , 5439 (2014).
Clayton, A. et al. Cancer exosomes express CD39 and CD73, which suppress T cells through adenosine production. J. Immunol. 187 , 676–683 (2011).
Peinado, H. et al. Melanoma exosomes educate bone marrow progenitor cells toward a pro-metastatic phenotype through MET. Nat. Med. 18 , 883 (2012).
Hood, J. L., San, R. S. & Wickline, S. A. Exosomes released by melanoma cells prepare sentinel lymph nodes for tumor metastasis. Cancer Res. 71 , 3792–3801 (2011).
Costa-Silva, B. et al. Pancreatic cancer exosomes initiate pre-metastatic niche formation in the liver. Nat. Cell Biol. 17 , 816–826 (2015).
Hoshino, A. et al. Tumour exosome integrins determine organotropic metastasis. Nature 527 , 329–335 (2015).
Yue, S., Mu, W., Erb, U. & Zöller, M. The tetraspanins CD151 and Tspan8 are essential exosome components for the crosstalk between cancer initiating cells and their surrounding. Oncotarget 6 , 2366 (2015).
PubMed Google Scholar
Luga, V. et al. Exosomes mediate stromal mobilization of autocrine Wnt-PCP signaling in breast cancer cell migration. Cell 151 , 1542–1556 (2012).
Smyth, T. J., Redzic, J. S., Graner, M. W. & Anchordoquy, T. J. Examination of the specificity of tumor cell derived exosomes with tumor cells in vitro. Biochim. Biophys. Acta 1838 , 2954–2965 (2014).
Zoller, M. Tetraspanins: push and pull in suppressing and promoting metastasis. Nat. Rev. Cancer 9 , 40–55 (2009).
Nanbo, A., Kawanishi, E., Yoshida, R. & Yoshiyama, H. Exosomes derived from Epstein-Barr virus-infected cells are internalized via caveola-dependent endocytosis and promote phenotypic modulation in target cells. J. Virol. 87 , 10334–10347 (2013).
Mulcahy, L. A., Pink, R. C. & Carter, D. R. Routes and mechanisms of extracellular vesicle uptake. J. Extracell. Vesicles . 3 (2014).
Zhang, L. et al. Microenvironment-induced PTEN loss by exosomal microRNA primes brain metastasis outgrowth. Nature 527 , 100–104 (2015).
Kaur, A. et al. Remodeling of the collagen matrix in aging skin promotes melanoma metastasis and affects immune cell motility. Cancer Discov. 9 , 64–81 (2019).
Ecker, B. L. et al. Age-related changes in HAPLN1 increase lymphatic permeability and affect routes of melanoma metastasis. Cancer Discov. 9 , 82–95 (2019).
Flavahan, W. A., Gaskell, E. & Bernstein, B. E. Epigenetic plasticity and the hallmarks of cancer. Science . 357 , pii: eaal2380 (2017).
Helmink, B. A. et al. The microbiome, cancer, and cancer therapy. Nat. Med. 25 , 377–388 (2019).
Pushalkar, S. et al. The pancreatic cancer microbiome promotes oncogenesis by induction of innate and adaptive immune suppression. Cancer Discov. 8 , 403–416 (2018).
Cronin, M. et al. Orally administered bifidobacteria as vehicles for delivery of agents to systemic tumors. Mol. Ther. 18 , 1397–1407 (2010).
Panebianco, C., Andriulli, A. & Pazienza, V. Pharmacomicrobiomics: exploiting the drug-microbiota interactions in anticancer therapies. Microbiome 6 , 92 (2018).
Lehouritis, P. et al. Local bacteria affect the efficacy of chemotherapeutic drugs. Sci. Rep. 5 , 14554 (2015).
Geller, L. T. et al. Potential role of intratumor bacteria in mediating tumor resistance to the chemotherapeutic drug gemcitabine. Science 357 , 1156–1160 (2017).
Alexander, J. L. et al. Gut microbiota modulation of chemotherapy efficacy and toxicity. Nat. Rev. Gastroenterol. Hepatol. 14 , 356–365 (2017).
Yu, T. et al. Fusobacterium nucleatum promotes chemoresistance to colorectal cancer by modulating autophagy. Cell 170 , 548–563 e516 (2017).
Zheng, J. H. et al. Two-step enhanced cancer immunotherapy with engineered Salmonella typhimurium secreting heterologous flagellin. Sci. Transl. Med . 9 , pii: eaak9537 (2017).
Kim, O. Y. et al. Bacterial outer membrane vesicles suppress tumor by interferon-gamma-mediated antitumor response. Nat. Commun. 8 , 626 (2017).
Panda, A. et al. Immune activation and benefit from Avelumab in EBV-positive gastric cancer. J. Natl Cancer Inst. 110 , 316–320 (2018).
Host, K. M. et al. Kaposi’s sarcoma-associated herpesvirus increases PD-L1 and proinflammatory cytokine expression in human monocytes. mBio 8 , e00917–e00917 (2017).
Smola, S. Immunopathogenesis of HPV-associated cancers and prospects for immunotherapy. Viruses . 9 , pii: E254 (2017).
Thiele Orberg, E. et al. The myeloid immune signature of enterotoxigenic Bacteroides fragilis-induced murine colon tumorigenesis. Mucosal Immunol. 10 , 421–433 (2017).
Gur, C. et al. Binding of the Fap2 protein of Fusobacterium nucleatum to human inhibitory receptor TIGIT protects tumors from immune cell attack. Immunity 42 , 344–355 (2015).
Masri, S. & Sassone-Corsi, P. The emerging link between cancer, metabolism, and circadian rhythms. Nat. Med. 24 , 1795–1803 (2018).
Lie, J.-A. S., Roessink, J. & Kjaerheim, K. Breast cancer and night work among Norwegian nurses. Cancer Causes Control. 17 , 39–44 (2006).
Papantoniou, K. et al. Night shift work, chronotype and prostate cancer risk in the MCC-Spain case-control study. Int J. Cancer 137 , 1147–1157 (2015).
Schernhammer, E. S. et al. Rotating night shifts and risk of breast cancer in women participating in the nurses’ health study. J. Natl Cancer Inst. 93 , 1563–1568 (2001).
Knutsson, A. et al. Breast cancer among shift workers: results of the WOLF longitudinal cohort study. Scand. J. Work Environ. Health 39 , 170–177 (2013).
Straif, K. et al. Carcinogenicity of shift-work, painting, and fire-fighting. Lancet Oncol. 8 , 1065–1066 (2007).
Kakizaki, M. et al. Sleep duration and the risk of prostate cancer: the Ohsaki Cohort Study. Br. J. Cancer 99 , 176–178 (2008).
Srour, B. et al. Circadian nutritional behaviours and cancer risk: New insights from the NutriNet-sante prospective cohort study: disclaimers. Int. J. Cancer 143 , 2369–2379 (2018).
Altman, B. J. et al. MYC disrupts the circadian clock and metabolism in cancer cells. Cell Metab. 22 , 1009–1019 (2015).
Huber, A. L. et al. CRY2 and FBXL3 cooperatively degrade c-MYC. Mol. Cell. 64 , 774–789 (2016).
Shostak, A. et al. MYC/MIZ1-dependent gene repression inversely coordinates the circadian clock with cell cycle and proliferation. Nat. Commun. 7 , 11807 (2016).
Pascual, G. et al. Targeting metastasis-initiating cells through the fatty acid receptor CD36. Nature 541 , 41–45 (2017).
Karamanos, N. K. et al. Proteoglycan chemical diversity drives multifunctional cell regulation and therapeutics. Chem. Rev. 118 , 9152–9232 (2018).
Frantz, C., Stewart, K. M. & Weaver, V. M. The extracellular matrix at a glance. J. Cell Sci. 123 , 4195–4200 (2010).
Theocharis, A. D., Skandalis, S. S., Gialeli, C. & Karamanos, N. K. Extracellular matrix structure. Adv. Drug Deliv. Rev. 97 , 4–27 (2016).
Maquart, F.-X. et al. An introduction to matrikines: extracellular matrix-derived peptides which regulate cell activity: implication in tumor invasion. Crit. Rev. Oncol. Hematol. 49 , 199–202 (2004).
Sonnenschein, C. & Soto, A. M. Carcinogenesis explained within the context of a theory of organisms. Prog. Biophys. Mol. Biol. 122 , 70–76 (2016).
Maffini, M. V. et al. The stroma as a crucial target in rat mammary gland carcinogenesis. J. Cell Sci. 117 , 1495–1502 (2004).
Barcellos-Hoff, M. H. & Ravani, S. A. Irradiated mammary gland stroma promotes the expression of tumorigenic potential by unirradiated epithelial cells. Cancer Res. 60 , 1254–1260 (2000).
Guerra, L., Odorisio, T., Zambruno, G. & Castiglia, D. Stromal microenvironment in type VII collagen-deficient skin: The ground for squamous cell carcinoma development. Matrix Biol. 63 , 1–10 (2017).
Iozzo, R. V. & Gubbiotti, M. A. Extracellular matrix: the driving force of mammalian diseases. Matrix Biol. 71 , 1–9 (2018).
Curran, S. & Murray, G. I. Matrix metalloproteinases: molecular aspects of their roles in tumour invasion and metastasis. Eur. J. Cancer 36 , 1621–1630 (2000).
Kessenbrock, K., Plaks, V. & Werb, Z. Matrix metalloproteinases: regulators of the tumor microenvironment. Cell 141 , 52–67 (2010).
Moss, L. A. S., Jensen-Taubman, S. & Stetler-Stevenson, W. G. Matrix metalloproteinases changing roles in tumor progression and metastasis. Am. J. Pathol. 181 , 1895–1899 (2012).
Egeblad, M. & Werb, Z. New functions for the matrix metalloproteinases in cancer progression. Nat. Rev. Cancer 2 , 161–174 (2002).
Hadler-Olsen, E., Winberg, J. O. & Uhlin-Hansen, L. Matrix metalloproteinases in cancer: their value as diagnostic and prognostic markers and therapeutic targets. Tumor Biol. 34 , 2041–2051 (2013).
Elia, I. et al. Breast cancer cells rely on environmental pyruvate to shape the metastatic niche. Nature 568 , 117 (2019).
Du, W. W. et al. The role of versican G3 domain in regulating breast cancer cell motility including effects on osteoblast cell growth and differentiation in vitro - evaluation towards understanding breast cancer cell bone metastasis. BMC Cancer 12 , 341 (2012).
Du, W. W. et al. Versican G3 promotes mouse mammary tumor cell growth, migration, and metastasis by influencing EGF receptor signaling. PLoS ONE 5 , e13828 (2010).
Cattaruzza, S. et al. NG2/CSPG4-collagen type VI interplays putatively involved in the microenvironmental control of tumour engraftment and local expansion. J. Mol. Cell Biol. 5 , 176–193 (2013).
Iida, J. et al. Cell surface chondroitin sulfate glycosaminoglycan in melanoma: role in the activation of pro-MMP-2 (pro-gelatinase A). Biochem J. 403 , 553–563 (2007).
Karamanou, K. et al. Lumican effectively regulates the estrogen receptors-associated functional properties of breast cancer cells, expression of matrix effectors and epithelial-to-mesenchymal transition. Sci. Rep. 7 , 45138 (2017).
Nikitovic, D. et al. Lumican, a small leucine-rich proteoglycan. IUBMB Life. 60 , 818–823 (2008).
Brézillon, S., Pietraszek, K., Maquart, F. X. & Wegrowski, Y. Lumican effects in the control of tumour progression and their links with metalloproteinases and integrins. FEBS J. 280 , 2369–2381 (2013).
Pietraszek, K. et al. Lumican: a new inhibitor of matrix metalloproteinase-14 activity. FEBS Lett. 588 , 4319–4324 (2014).
Stasiak, M. et al. Lumican inhibits SNAIL-induced melanoma cell migration specifically by blocking MMP-14 activity. PLoS ONE 11 , e0150226 (2016).
Pietraszek-Gremplewicz, K. et al. Small leucine-rich proteoglycans and matrix metalloproteinase-14: Key partners? Matrix Biol. 75-76 , 271–285 (2019).
Filmus, J. & Capurro, M. The role of glypicans in Hedgehog signaling. Matrix Biol. 35 , 248–252 (2014).
Han, S. et al. Identification of Glypican-3 as a potential metastasis suppressor gene in gastric cancer. Oncotarget 7 , 44406–44416 (2016).
PubMed PubMed Central Google Scholar
Lin, Q. et al. Expression of GPC3 protein and its significance in lung squamous cell carcinoma. Med. Oncol. 29 , 663–669 (2012).
Yu, X. et al. Differential expression of glypican-3 (GPC3) in lung squamous cell carcinoma and lung adenocarcinoma and its clinical significance. Genet Mol. Res. 14 , 10185–10192 (2015).
Zhang, Y. et al. The role of GPC5 in lung metastasis of salivary adenoid cystic carcinoma. Arch. Oral. Biol. 59 , 1172–1182 (2014).
Williamson, D. et al. Role for amplification and expression of glypican-5 in rhabdomyosarcoma. Cancer Res. 67 , 57–65 (2007).
Li, F., Shi, W., Capurro, M. & Filmus, J. Glypican-5 stimulates rhabdomyosarcoma cell proliferation by activating Hedgehog signaling. J. Cell Biol. 192 , 691–704 (2011).
Korpetinou, A. et al. Serglycin is implicated in the promotion of aggressive phenotype of breast cancer cells. PLoS ONE 8 , e78157 (2013).
Bouris, P. et al. Serglycin promotes breast cancer cell aggressiveness: Induction of epithelial to mesenchymal transition, proteolytic activity and IL-8 signaling. Matrix Biol. 74 , 35–51 (2018).
Liu, T., Zhang, L., Joo, D. & Sun, S. C. NF-kappaB signaling in inflammation. Signal Transduct. Target. Ther . 2 , pii: 17023 (2017).
Guo, J. Y. et al. Serglycin in tumor microenvironment promotes non-small cell lung cancer aggressiveness in a CD44-dependent manner. Oncogene 36 , 2457–2471 (2017).
Chu, Q. et al. Extracellular serglycin upregulates the CD44 receptor in an autocrine manner to maintain self-renewal in nasopharyngeal carcinoma cells by reciprocally activating the MAPK/beta-catenin axis. Cell Death Dis. 7 , e2456 (2016).
Roy, A. et al. Targeting serglycin prevents metastasis in murine mammary carcinoma. PLoS ONE 11 , e0156151 (2016).
Purushothaman, A. et al. Chondroitin sulfate proteoglycan serglycin influences protein cargo loading and functions of tumor-derived exosomes. Oncotarget 8 , 73723–73732 (2017).
Passi, A., Vigetti, D., Buraschi, S. & Iozzo, R. V. Dissecting the role of hyaluronan synthases in the tumor microenvironment. FEBS J. 286 , 2937–2949 (2019).
Chanmee, T., Ontong, P. & Itano, N. Hyaluronan: a modulator of the tumor microenvironment. Cancer Lett. 375 , 20–30 (2016).
Zhang, Z. et al. Hyaluronan synthase 2 expressed by cancer-associated fibroblasts promotes oral cancer invasion. J. Exp. Clin. Cancer Res. 35 , 181 (2016).
Preca, B. T. et al. A novel ZEB1/HAS2 positive feedback loop promotes EMT in breast cancer. Oncotarget 8 , 11530–11543 (2017).
Porsch, H. et al. Efficient TGFbeta-induced epithelial-mesenchymal transition depends on hyaluronan synthase HAS2. Oncogene 32 , 4355–4365 (2013).
Okuda, H. et al. Hyaluronan synthase HAS2 promotes tumor progression in bone by stimulating the interaction of breast cancer stem-like cells with macrophages and stromal cells. Cancer Res. 72 , 537–547 (2012).
Nagy, N. et al. Hyaluronan in immune dysregulation and autoimmune diseases. Matrix Biol. 78-79 , 292–313 (2019).
Piccioni, F. et al. 4-methylumbelliferone inhibits hepatocellular carcinoma growth by decreasing IL-6 production and angiogenesis. Glycobiology 25 , 825–835 (2015).
Yates, T. J. et al. Dietary supplement 4-methylumbelliferone: an effective chemopreventive and therapeutic agent for prostate cancer. J. Natl Cancer Inst . 107 , pii: djv085 (2015).
Schmaus, A. et al. Accumulation of small hyaluronan oligosaccharides in tumour interstitial fluid correlates with lymphatic invasion and lymph node metastasis. Br. J. Cancer 111 , 559–567 (2014).
Caon, I. et al. Revisiting the hallmarks of cancer: The role of hyaluronan. Semin. Cancer Biol . pii: S1044-579X(19)30042–2 (2019).
Misra, S., Hascall, V. C., Markwald, R. R. & Ghatak, S. Interactions between hyaluronan and its receptors (CD44, RHAMM) regulate the activities of inflammation and cancer. Front. Immunol. 6 , 201 (2015).
Wu, M. et al. A novel role of low molecular weight hyaluronan in breast cancer metastasis. FASEB J. 29 , 1290–1298 (2015).
Du, Y. et al. Low-molecular-weight hyaluronan (LMW-HA) accelerates lymph node metastasis of melanoma cells by inducing disruption of lymphatic intercellular adhesion. Oncoimmunology 5 , e1232235 (2016).
Mowers, E. E., Sharifi, M. N. & Macleod, K. F. Autophagy in cancer metastasis. Oncogene 36 , 1619–1630 (2017).
Macintosh, R. L. et al. Inhibition of autophagy impairs tumor cell invasion in an organotypic model. Cell Cycle 11 , 2022–2029 (2012).
Mauffrey, P. et al. Progenitors from the central nervous system drive neurogenesis in cancer. Nature 569 , 672–678 (2019).
Fares, J., Bou Diab, Z., Nabha, S. & Fares, Y. Neurogenesis in the adult hippocampus: history, regulation, and prospective roles. Int J. Neurosci. 129 , 598–611 (2019).
Fares, J., Fares, M. Y. & Fares, Y. Immune checkpoint inhibitors: Advances and impact in neuro-oncology. Surg. Neurol. Int. 10 , 9 (2019).
Bidwell, B. N. et al. Silencing of Irf7 pathways in breast cancer cells promotes bone metastasis through immune escape. Nat. Med. 18 , 1224–1231 (2012).
Fares, J., Fares, M. Y. & Fares, Y. Natural killer cells in the brain tumor microenvironment: defining a new era in neuro-oncology. Surg. Neurol. Int. 10 , 43 (2019).
Pommier, A. et al. Unresolved endoplasmic reticulum stress engenders immune-resistant, latent pancreatic cancer metastases. Science 360 , 1202 (2018).
Li, R., Huang, Y. & Lin, J. Distinct effects of general anesthetics on lung metastasis mediated by IL-6/JAK/STAT3 pathway in mouse models. Nat. Commun. 11 , 642 (2020).
Reymond, N., d’Agua, B. B. & Ridley, A. J. Crossing the endothelial barrier during metastasis. Nat. Rev. Cancer 13 , 858–870 (2013).
Bockhorn, M., Jain, R. K. & Munn, L. L. Active versus passive mechanisms in metastasis: do cancer cells crawl into vessels, or are they pushed? Lancet Oncol. 8 , 444–448 (2007).
Zervantonakis, I. K. et al. Three-dimensional microfluidic model for tumor cell intravasation and endothelial barrier function. Proc. Natl Acad. Sci. USA 109 , 13515–13520 (2012).
Wong, A. D. & Searson, P. C. Mitosis-mediated intravasation in a tissue-engineered tumor-microvessel platform. Cancer Res. 77 , 6453–6461 (2017).
Denais, C. M. et al. Nuclear envelope rupture and repair during cancer cell migration. Science 352 , 353–358 (2016).
Hamidi, H. & Ivaska, J. Every step of the way: integrins in cancer progression and metastasis. Nat. Rev. Cancer 18 , 532–547 (2018).
Padmanaban, V. et al. E-cadherin is required for metastasis in multiple models of breast cancer. Nature 573 , 439–444 (2019).
Pantel, K. & Speicher, M. R. The biology of circulating tumor cells. Oncogene 35 , 1216–1224 (2016).
Gao, H. et al. The BMP inhibitor Coco reactivates breast cancer cells at lung metastatic sites. Cell 150 , 764–779 (2012).
Lu, X. et al. VCAM-1 promotes osteolytic expansion of indolent bone micrometastasis of breast cancer by engaging alpha4beta1-positive osteoclast progenitors. Cancer Cell. 20 , 701–714 (2011).
Aceto, N. et al. Circulating tumor cell clusters are oligoclonal precursors of breast cancer metastasis. Cell 158 , 1110–1122 (2014).
Zhang, Y. et al. VN-NDP: a neighbor discovery protocol based on virtual nodes in mobile WSNs. Sensors . 19 , pii: E4739 (2019).
Yu, M. et al. Circulating breast tumor cells exhibit dynamic changes in epithelial and mesenchymal composition. Science 339 , 580–584 (2013).
Ankrum, J. A., Ong, J. F. & Karp, J. M. Mesenchymal stem cells: immune evasive, not immune privileged. Nat. Biotechnol. 32 , 252 (2014).
Duda, D. G. et al. Malignant cells facilitate lung metastasis by bringing their own soil. Proc. Natl Acad. Sci. USA 107 , 21677–21682 (2010).
Leach, J., Morton, J. P. & Sansom, O. J. Neutrophils: Homing in on the myeloid mechanisms of metastasis. Mol. Immunol. 110 , 69–76 (2019).
Gay, L. J. & Felding-Habermann, B. Contribution of platelets to tumour metastasis. Nat. Rev. Cancer 11 , 123–134 (2011).
Placke, T. et al. Platelet-derived MHC class I confers a pseudonormal phenotype to cancer cells that subverts the antitumor reactivity of natural killer immune cells. Cancer Res. 72 , 440–448 (2012).
Hu, C. et al. Thrombocytosis is a significant indictor of hypercoagulability, prognosis and recurrence in gastric cancer. Exp. Ther. Med. 8 , 125–132 (2014).
Strilic, B. & Offermanns, S. Intravascular survival and extravasation of tumor cells. Cancer Cell 32 , 282–293 (2017).
Leong, H. S. et al. Invadopodia are required for cancer cell extravasation and are a therapeutic target for metastasis. Cell Rep. 8 , 1558–1570 (2014).
Stoletov, K. et al. Visualizing extravasation dynamics of metastatic tumor cells. J. Cell Sci. 123 , 2332–2341 (2010).
Schumacher, D. et al. Platelet-derived nucleotides promote tumor-cell transendothelial migration and metastasis via P2Y2 receptor. Cancer Cell. 24 , 130–137 (2013).
Raskov, H., Orhan, A., Salanti, A. & Gögenur, I. Premetastatic niches, exosomes and circulating tumor cells: Early mechanisms of tumor dissemination and the relation to surgery. Int. J. Cancer (2019). https://doi.org/10.1002/ijc.32820 (in press).
Smith, H. A. & Kang, Y. The metastasis-promoting roles of tumor-associated immune cells. J. Mol. Med (Berl.). 91 , 411–429 (2013).
Jackson, W. et al. Role of megakaryocytes in breast cancer metastasis to bone. Cancer Res. 77 , 1942–1954 (2017).
Granot, Z. et al. Tumor entrained neutrophils inhibit seeding in the premetastatic lung. Cancer Cell 20 , 300–314 (2011).
Szczerba, B. M. et al. Neutrophils escort circulating tumour cells to enable cell cycle progression. Nature 566 , 553 (2019).
Obradovic, M. M. S. et al. Glucocorticoids promote breast cancer metastasis. Nature 567 , 540–544 (2019).
van Helvert, S., Storm, C. & Friedl, P. Mechanoreciprocity in cell migration. Nat. Cell Biol. 20 , 8–20 (2018).
Follain, G. et al. Hemodynamic forces tune the arrest, adhesion, and extravasation of circulating tumor cells. Dev. Cell. 45 , 33–52 e12 (2018).
Entenberg, D. et al. A permanent window for the murine lung enables high-resolution imaging of cancer metastasis. Nat. Methods 15 , 73 (2018).
Goetz, J. G. Metastases go with the flow. Science 362 , 999–1000 (2018).
Headley, M. B. et al. Visualization of immediate immune responses to pioneer metastatic cells in the lung. Nature 531 , 513–517 (2016).
Boire, A. et al. Complement component 3 adapts the cerebrospinal fluid for leptomeningeal metastasis. Cell 168 , 1101 (2017).
Bonecchi, R. et al. Chemokines and chemokine receptors: an overview. Front Biosci. Landmrk. 14 , 540–551 (2009).
Le Gal, K. et al. Antioxidants can increase melanoma metastasis in mice. Sci. Transl. Med . 7 , 308re8 (2015).
Quail, D. F. et al. Obesity alters the lung myeloid cell landscape to enhance breast cancer metastasis through IL5 and GM-CSF. Nat. Cell Biol. 19 , 974 (2017).
Jayatilaka, H. et al. Synergistic IL-6 and IL-8 paracrine signalling pathway infers a strategy to inhibit tumour cell migration. Nat. Commun . 8 , 15584 (2017).
Coffelt, S. B. et al. IL-17-producing gammadelta T cells and neutrophils conspire to promote breast cancer metastasis. Nature 522 , 345–348 (2015).
Wellenstein, M. D. et al. Loss of p53 triggers WNT-dependent systemic inflammation to drive breast cancer metastasis. Nature 572 , 538–542, (2019).
Naxerova, K. et al. Origins of lymphatic and distant metastases in human colorectal cancer. Science 357 , 55–60 (2017).
Brown, M. et al. Lymph node blood vessels provide exit routes for metastatic tumor cell dissemination in mice. Science 359 , 1408–1411 (2018).
Pereira, E. R. et al. Lymph node metastases can invade local blood vessels, exit the node, and colonize distant organs in mice. Science 359 , 1403–1407 (2018).
Lee, C. K. et al. Tumor metastasis to lymph nodes requires YAP-dependent metabolic adaptation. Science 363 , 644 (2019).
Joosse, S. A., Gorges, T. M. & Pantel, K. Biology, detection, and clinical implications of circulating tumor cells. EMBO Mol. Med. 7 , 1–11 (2015).
Fares, J. et al. Diagnostic clinical trials in breast cancer brain metastases: barriers and innovations. Clin. Breast Cancer 19, 383–391 (2019).
Shaw, J. A. et al. Mutation analysis of cell-free DNA and single circulating tumor cells in metastatic breast cancer patients with high circulating tumor cell counts. Clin. Cancer Res. 23 , 88–96 (2017).
Abbosh, C. et al. Phylogenetic ctDNA analysis depicts early-stage lung cancer evolution. Nature 545 , 446 (2017).
Fares, J. et al. Current state of clinical trials in breast cancer brain metastases. Neurooncol. Pract. 6 , 392–401 (2019).
Galanzha, E. I. et al. In vivo liquid biopsy using Cytophone platform for photoacoustic detection of circulating tumor cells in patients with melanoma. Sci. Transl. Med. 11 , eaat5857 (2019).
Gkountela, S. et al. Circulating tumor cell clustering shapes DNA methylation to enable metastasis seeding. Cell 176 , 98 (2019).
Alix-Panabieres, C. & Pantel, K. Clinical applications of circulating tumor cells and circulating tumor DNA as liquid biopsy. Cancer Discov. 6 , 479–491 (2016).
Stegner, D., Dutting, S. & Nieswandt, B. Mechanistic explanation for platelet contribution to cancer metastasis. Thromb. Res. 133 (Suppl 2), S149–S157 (2014).
Labelle, M. & Hynes, R. O. The initial hours of metastasis: the importance of cooperative host-tumor cell interactions during hematogenous dissemination. Cancer Discov. 2 , 1091–1099 (2012).
Joyce, J. A. & Pollard, J. W. Microenvironmental regulation of metastasis. Nat. Rev. Cancer 9 , 239–252 (2009).
Strilic, B. et al. Tumour-cell-induced endothelial cell necroptosis via death receptor 6 promotes metastasis. Nature 536 , 215–218 (2016).
Langley, R. R. & Fidler, I. J. The seed and soil hypothesis revisited–the role of tumor-stroma interactions in metastasis to different organs. Int. J. Cancer 128 , 2527–2535 (2011).
Minn, A. J. et al. Distinct organ-specific metastatic potential of individual breast cancer cells and primary tumors. J. Clin. Invest. 115 , 44–55 (2005).
Lorusso, G. & Ruegg, C. New insights into the mechanisms of organ-specific breast cancer metastasis. Semin. Cancer Biol. 22 , 226–233 (2012).
Nguyen, D. X. & Massague, J. Genetic determinants of cancer metastasis. Nat. Rev. Genet. 8 , 341–352 (2007).
Croucher, P. I., McDonald, M. M. & Martin, T. J. Bone metastasis: the importance of the neighbourhood. Nat. Rev. Cancer 16 , 373–386 (2016).
Wang, H. et al. The osteogenic niche is a calcium reservoir of bone micrometastases and confers unexpected therapeutic vulnerability. Cancer Cell. 34 , 823 (2018).
Goddard, E. T. et al. The rodent liver undergoes weaning-induced involution and supports breast cancer metastasis. Cancer Discov. 7 , 177–187 (2017).
Salvador, F. et al. Lysyl oxidase-like protein LOXL2 promotes lung metastasis of breast cancer. Cancer Res. 77 , 5846–5859 (2017).
Psaila, B., Kaplan, R. N., Port, E. R. & Lyden, D. Priming the ‘soil’ for breast cancer metastasis: the pre-metastatic niche. Breast Dis. 26 , 65–74 (2006).
Peinado, H., Lavotshkin, S. & Lyden, D. The secreted factors responsible for pre-metastatic niche formation: Old sayings and new thoughts. Semin. Cancer Biol. 21 , 139–146 (2011).
Lee, J. W. et al. Hepatocytes direct the formation of a pro-metastatic niche in the liver. Nature 567 , 249–252 (2019).
Wagenblast, E. et al. A model of breast cancer heterogeneity reveals vascular mimicry as a driver of metastasis. Nature 520 , 358–362 (2015).
Zeng, Q. et al. Synaptic proximity enables NMDAR signalling to promote brain metastasis. Nature 573 , 526–531 (2019).
Chen, Q. et al. Carcinoma-astrocyte gap junctions promote brain metastasis by cGAMP transfer. Nature 533 , 493–498 (2016).
Gomis, R. R. & Gawrzak, S. Tumor cell dormancy. Mol. Oncol. 11 , 62–78 (2017).
Giancotti, F. G. Mechanisms governing metastatic dormancy and Reactivation. Cell 155 , 750–764 (2013).
Sosa, M. S., Bragado, P. & Aguirre-Ghiso, J. A. Mechanisms of disseminated cancer cell dormancy: an awakening field. Nat. Rev. Cancer 14 , 611–622 (2014).
Aguirre-Ghiso, J. A. & Sosa, M. S. Emerging topics on disseminated cancer cell dormancy and the paradigm of metastasis. Annu, Rev. Cancer Biol. 2 , 377–393 (2018).
Sosa, M. S., Bernstein, E. & Aguirre-Ghiso, J. A. in Tumor Dormancy and Recurrence (eds Yuzhuo Wang & Francesco Crea) 1–16 (Springer International Publishing, 2017).
Yeh, A. C. & Ramaswamy, S. Mechanisms of cancer cell dormancy—another hallmark of cancer? Cancer Res. 75 , 5014–5022 (2015).
Kobayashi, A. et al. Bone morphogenetic protein 7 in dormancy and metastasis of prostate cancer stem-like cells in bone. J. Exp. Med. 208 , 2641–2655 (2011).
Shiozawa, Y. et al. GAS6/AXL axis regulates prostate cancer invasion, proliferation, and survival in the bone marrow niche. Neoplasia 12 , 116–127 (2010).
Aguirre-Ghiso, J. A. et al. Urokinase receptor and fibronectin regulate the ERKMAPK to p38MAPK activity ratios that determine carcinoma cell proliferation or dormancy in vivo. Mol. Biol. Cell. 12 , 863–879 (2001).
Aguirre-Ghiso, J. A., Estrada, Y., Liu, D. & Ossowski, L. ERKMAPK activity as a determinant of tumor growth and dormancy; regulation by p38SAPK. Cancer Res. 63 , 1684–1695 (2003).
Bragado, P. et al. TGF-β2 dictates disseminated tumour cell fate in target organs through TGF-β-RIII and p38α/β signalling. Nat. Cell Biol. 15 , 1351–1361 (2013).
Ghajar, C. M. et al. The perivascular niche regulates breast tumour dormancy. Nat. Cell Biol. 15 , 807–817 (2013).
Straume, O. et al. Suppression of heat shock protein 27 induces long-term dormancy in human breast cancer. Proc. Natl Acad. Sci. USA 109 , 8699–8704 (2012).
Endo, H. & Inoue, M. Dormancy in cancer. Cancer Sci. 110 , 474–480 (2019).
Albrengues, J. et al. Neutrophil extracellular traps produced during inflammation awaken dormant cancer cells in mice. Science 361 , 1353 (2018).
Gay, L. J. & Malanchi, I. The sleeping ugly: tumour microenvironment’s act to make or break the spell of dormancy. BBA Rev. Cancer 1868 , 231–238 (2017).
CAS Google Scholar
Ishay-Ronen, D. et al. Gain fat-lose metastasis: converting invasive breast cancer cells into adipocytes inhibits cancer metastasis. Cancer Cell. 35 , 17 (2019).
van der Weyden, L. et al. Genome-wide in vivo screen identifies novel host regulators of metastatic colonization. Nature 541 , 233–236 (2017).
Donovan, P. et al. Endovascular progenitors infiltrate melanomas and differentiate towards a variety of vascular beds promoting tumor metastasis. Nat. Commun . 10 , 18 (2019).
Keklikoglou, I. et al. Chemotherapy elicits pro-metastatic extracellular vesicles in breast cancer models. Nat. Cell Biol. 21 , 190 (2019).
Khan, I. & Steeg, P. S. Metastasis suppressors: functional pathways. Lab Invest. 98 , 198–210 (2018).
Shevde, L. A. & Welch, D. R. Metastasis suppressor pathways–an evolving paradigm. Cancer Lett. 198 , 1–20 (2003).
Hu, X. et al. The RNA-binding protein AKAP8 suppresses tumor metastasis by antagonizing EMT-associated alternative splicing. Nat. Commun. 11 , 486 (2020).
Steeg, P. S. Metastasis suppressors alter the signal transduction of cancer cells. Nat. Rev. Cancer 3 , 55–63 (2003).
Mao, L. et al. ULK1 phosphorylates Exo70 to suppress breast cancer metastasis. Nat. Commun. 11 , 117 (2020).
Li, M., Cai, O. & Tan, S. LOXL1-AS1 drives the progression of gastric cancer via regulating miR-142-5p/PIK3CA axis. Onco Targets Ther. 12 , 11345–11357 (2019).
Kong, J., Shen, S., Yang, X. & Wang, W. Transhepatic-intrahepatic branches of the portal vein catheterization for ex vivo liver resection and autotransplantation: two case reports of novel approach to perfuse the liver. Medicine 98 , e14706 (2019).
Wang, X., Su, D., Qin, Z. & Chen, Z. Identification of FOXN4 as a tumor suppressor of breast carcinogenesis via the activation of TP53 and deactivation of Notch signaling. Gene 722 , 144057 (2020).
Lu, G. & Zhang, Y. MicroRNA-340-5p suppresses non-small cell lung cancer cell growth and metastasis by targeting ZNF503. Cell Mol. Biol. Lett. 24 , 34 (2019).
Ablikim, M. et al. Determination of the pseudoscalar decay constant f (Ds+) via D s + → μ + ν μ. Phys. Rev. Lett. 122 , 071802 (2019).
Liao, X. et al. Partial nephrectomy vs cryoablation for T1a renal cell carcinoma: a comparison of survival benefit stratified by tumour size. Cancer Epidemiol. 59 , 221–226 (2019).
Xu, J. et al. Surufatinib in advanced well-differentiated neuroendocrine tumors: a multicenter, single-arm, open-label, phase Ib/II trial. Clin. Cancer Res. 25 , 3486–3494 (2019).
Yu, M. et al. miR-10b downregulated by DNA methylation acts as a tumor suppressor in HPV-positive cervical cancer via targeting Tiam1. Cell. Physiol. Biochem. 51 , 1763–1777 (2018).
Huang, B., Huang, M. & Li, Q. MiR-137 suppresses migration and invasion by targeting EZH2-STAT3 signaling in human hepatocellular carcinoma. Pathol. Res. Pract. 214 , 1980–1986 (2018).
Zhang, Q. et al. MicroRNA-3619-5p suppresses bladder carcinoma progression by directly targeting beta-catenin and CDK2 and activating p21. Cell Death Dis. 9 , 960 (2018).
Duan, S. et al. MicroRNA-135a-3p is downregulated and serves as a tumour suppressor in ovarian cancer by targeting CCR2. Biomedicine Pharmacother. 107 , 712–720 (2018).
Yang, L., Km, Y. & Tie, J. miR-340-5p is a potential prognostic indicator of colorectal cancer and modulates ANXA3. Eur. Rev. Med Pharm. Sci. 22 , 4837–4845 (2018).
Croset, M. et al. miRNA-30 family members inhibit breast cancer invasion, osteomimicry, and bone destruction by directly targeting multiple bone metastasis-associated genes. Cancer Res. 78 , 5259–5273 (2018).
Xu, R. D. et al. miR-149-5p inhibits cell growth by regulating TWEAK/Fn14/PI3K/AKT pathway and predicts favorable survival in human osteosarcoma. Int J. Immunopathol. Pharmacol. 32 , 2058738418786656 (2018).
Ablikim, M. et al. Observation of the semileptonic decay D0→a0(980)−e+νe and evidence for D+→a0(980)0e+νe. Phys. Rev. Lett. 121 , 081802 (2018).
Gao, W. et al. Downregulation of MiR-1297 predicts poor prognosis and enhances gastric cancer cell growth by targeting CREB1. Biomed. Pharmacother. 105 , 413–419 (2018).
Pan, S. et al. Tumor suppressive role of ARHGAP17 in colon cancer through Wnt/β-catenin signaling. Cell. Physiol. Biochem. 46 , 2138–2148 (2018).
Zhang, X. et al. MicroRNA-26a is a key regulon that inhibits progression and metastasis of c-Myc/EZH2 double high advanced hepatocellular carcinoma. Cancer Lett. 426 , 98–108 (2018).
Zhao, L. et al. The RNA binding protein SORBS2 suppresses metastatic colonization of ovarian cancer by stabilizing tumor-suppressive immunomodulatory transcripts. Genome Biol. 19 , 35 (2018).
Gergely, J. E., Dorsey, A. E., Dimri, G. P. & Dimri, M. Timosaponin A-III inhibits oncogenic phenotype via regulation of PcG protein BMI1 in breast cancer cells. Mol. Carcinog. 57 , 831–841 (2018).
Wang, L. et al. HOXD3 targeted by miR-203a suppresses cell metastasis and angiogenesis through VEGFR in human hepatocellular carcinoma cells. Sci. Rep. 8 , 2431 (2018).
Tu, C., Wang, F. & Wan, J. MicroRNA-381 inhibits cell proliferation and invasion in endometrial carcinoma by targeting the IGF-1R. Mol. Med. Rep. 17 , 4090–4098 (2018).
Zhu, S. et al. LINC00473 antagonizes the tumour suppressor miR-195 to mediate the pathogenesis of Wilms tumour via IKKα. Cell Prolif. 51 , e12416 (2018).
Wang, N. et al. Targeting of NT5E by miR-30b and miR-340 attenuates proliferation, invasion and migration of gallbladder carcinoma. Biochimie 146 , 56–67 (2018).
Steeg, P. S. Targeting metastasis. Nat. Rev. Cancer 16 , 201–218 (2016).
Hodi, F. S. et al. Improved survival with ipilimumab in patients with metastatic melanoma. N. Engl. J. Med. 363 , 711–723 (2010).
Tawbi, H. A. et al. Combined Nivolumab and Ipilimumab in melanoma metastatic to the brain. N. Engl. J. Med. 379 , 722–730 (2018).
Scher, H. I. et al. Increased survival with enzalutamide in prostate cancer after chemotherapy. N. Engl. J. Med. 367 , 1187–1197 (2012).
Tevaarwerk, A. J. et al. Survival in patients with metastatic recurrent breast cancer after adjuvant chemotherapy: little evidence of improvement over the past 30 years. Cancer 119 , 1140–1148 (2013).
Marshall, J. C. et al. Effect of inhibition of the lysophosphatidic acid receptor 1 on metastasis and metastatic dormancy in breast cancer. J. Natl Cancer Inst. 104 , 1306–1319 (2012).
Goss, P. E. & Chambers, A. F. Does tumour dormancy offer a therapeutic target? Nat. Rev. Cancer 10 , 871 (2010).
Wee, I. et al. Role of tumor-derived exosomes in cancer metastasis. Biochim Biophys. Acta Rev. Cancer 1871 , 12–19 (2019).
Ulasov, I., Fares, J., Timashev, P. & Lesniak, M. S. Editing cytoprotective autophagy in glioma: an unfulfilled potential for therapy. Trends Mol. Med. 26 , P252–262 (2019).
Fares, J. et al. Landscape of combination therapy trials in breast cancer brain metastasis. Int. J. Cancer (2020). https://doi.org/10.1002/ijc.32937 (in press).
Download references
Author information
Authors and affiliations.
Department of Neurological Surgery, Feinberg School of Medicine, Northwestern University, Chicago, IL, 60611, USA
Jawad Fares
High-Impact Cancer Research Program, Harvard Medical School, Boston, MA, 02115, USA
Faculty of Medicine, American University of Beirut, Beirut, Lebanon
Mohamad Y. Fares, Hussein H. Khachfe & Hamza A. Salhab
Neuroscience Research Center, Faculty of Medical Sciences, Lebanese University, Beirut, Lebanon
Mohamad Y. Fares, Hussein H. Khachfe, Hamza A. Salhab & Youssef Fares
You can also search for this author in PubMed Google Scholar
Corresponding author
Correspondence to Jawad Fares .
Ethics declarations
Competing interests.
The authors declare no competing interests.
Rights and permissions
Open Access This article is licensed under a Creative Commons Attribution 4.0 International License, which permits use, sharing, adaptation, distribution and reproduction in any medium or format, as long as you give appropriate credit to the original author(s) and the source, provide a link to the Creative Commons license, and indicate if changes were made. The images or other third party material in this article are included in the article’s Creative Commons license, unless indicated otherwise in a credit line to the material. If material is not included in the article’s Creative Commons license and your intended use is not permitted by statutory regulation or exceeds the permitted use, you will need to obtain permission directly from the copyright holder. To view a copy of this license, visit http://creativecommons.org/licenses/by/4.0/ .
Reprints and permissions
About this article
Cite this article.
Fares, J., Fares, M.Y., Khachfe, H.H. et al. Molecular principles of metastasis: a hallmark of cancer revisited. Sig Transduct Target Ther 5 , 28 (2020). https://doi.org/10.1038/s41392-020-0134-x
Download citation
Received : 30 October 2019
Revised : 05 February 2020
Accepted : 11 February 2020
Published : 12 March 2020
DOI : https://doi.org/10.1038/s41392-020-0134-x
Share this article
Anyone you share the following link with will be able to read this content:
Sorry, a shareable link is not currently available for this article.
Provided by the Springer Nature SharedIt content-sharing initiative
This article is cited by
Trna-derived small rnas in human cancers: roles, mechanisms, and clinical application.
Molecular Cancer (2024)
Targeting interleukin-6 as a treatment approach for peritoneal carcinomatosis
- Neda Dadgar
- Christopher Sherry
- Patrick L. Wagner
Journal of Translational Medicine (2024)
Chemotherapy-induced PTEN-L secretion promotes the selection of PTEN-deficient tumor cells
- Zhenzhen Pan
Journal of Experimental & Clinical Cancer Research (2024)
Ubiquitin ligase subunit FBXO9 inhibits V-ATPase assembly and impedes lung cancer metastasis
- Xiaodong Chen
Experimental Hematology & Oncology (2024)
Beyond the barrier: the immune-inspired pathways of tumor extravasation
- Sara Di Russo
- Francesca Romana Liberati
- Alessio Paone
Cell Communication and Signaling (2024)
Quick links
- Explore articles by subject
- Guide to authors
- Editorial policies

- Alzheimer's disease & dementia
- Arthritis & Rheumatism
- Attention deficit disorders
- Autism spectrum disorders
- Biomedical technology
- Diseases, Conditions, Syndromes
- Endocrinology & Metabolism
- Gastroenterology
- Gerontology & Geriatrics
- Health informatics
- Inflammatory disorders
- Medical economics
- Medical research
- Medications
- Neuroscience
- Obstetrics & gynaecology
- Oncology & Cancer
- Ophthalmology
- Overweight & Obesity
- Parkinson's & Movement disorders
- Psychology & Psychiatry
- Radiology & Imaging
- Sleep disorders
- Sports medicine & Kinesiology
- Vaccination
- Breast cancer
- Cardiovascular disease
- Chronic obstructive pulmonary disease
- Colon cancer
- Coronary artery disease
- Heart attack
- Heart disease
- High blood pressure
- Kidney disease
- Lung cancer
- Multiple sclerosis
- Myocardial infarction
- Ovarian cancer
- Post traumatic stress disorder
- Rheumatoid arthritis
- Schizophrenia
- Skin cancer
- Type 2 diabetes
- Full List »
share this!
June 6, 2024
This article has been reviewed according to Science X's editorial process and policies . Editors have highlighted the following attributes while ensuring the content's credibility:
fact-checked
peer-reviewed publication
trusted source
Study uncovers how cancer stem cells spread and resist treatment
by University of Oxford
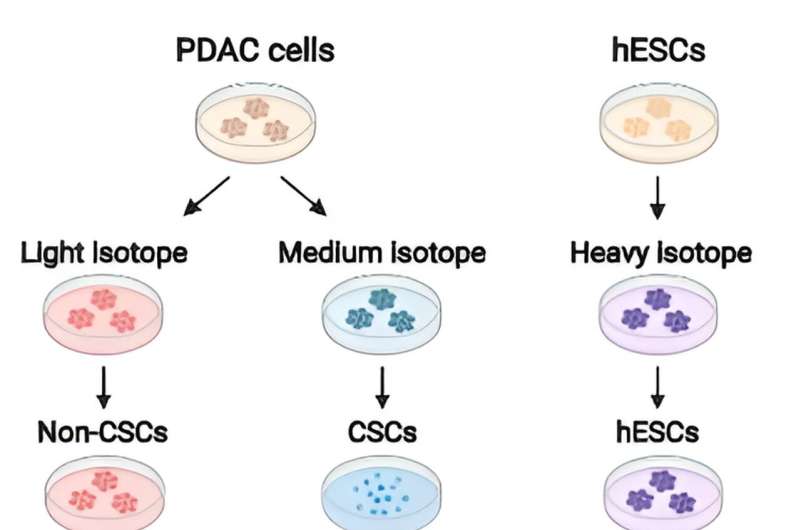
NDORMS researchers have identified a critical axis that controls the formation and behavior of cancer stem cells (CSCs), a subpopulation of cells that influence how lethal the cancer can be, its resistance to chemotherapy, and its ability to spread to other parts of the body.
The findings , published in Nature Communications , could pave the way for more effective cancer treatments.
The research, led by Associate Professor and CRUK career development fellow Siim Pauklin from NDORMS, focused on understanding the intricate interplay between cell-autonomous (mechanisms inside cells that function independently of signals coming from outside the cells) and non-cell-autonomous (external signals from outside cells) pathways that govern the stem cell-like characteristics of cancer stem cells (CSCs) in pancreatic cancer .
CSCs have also been discovered in the brain, breast, colon, esophagus, liver, lung, ovarian, prostate, stomach and thyroid cancers, among others.
Using a quantitative proteomic approach to analyze the set of proteins in the extracellular space (the secretome) of pancreatic CSCs, the research reveals how a complex axis involving the retinoblastoma (RB) proteins and the E2F transcription factors controls the production of signaling molecules that promote CSC formation and chemoresistance.
"We were surprised to find that the well-known cell cycle regulators pRb and E2F, which were previously thought to only have cell-autonomous effects, actually have a non-cell-autonomous role in controlling the secretion of key WNT ligands that drive the stem cell-like properties of CSCs," said Siim. "This work provides important insights into the non-cell autonomous mechanisms that allow cancer stem cells to thrive and evade therapy."
The researchers discovered that the E2F1 and E2F4 transcription factors induce the expression of WNT ligands, such as WNT7A, WNT7B, WNT10A, and WNT4, while the pRb and RBL2 proteins act to reduce their expression. This delicate balance is disrupted in cancer cells harboring KRAS mutations, leading to the aberrant secretion of these WNT ligands and the activation of the WNT/β-catenin signaling pathway in CSCs.
"The interplay between cell-autonomous transcriptional regulators and non-cell-autonomous signaling pathways is a crucial, yet often overlooked, aspect of cancer stem cell biology," Siim said. "Our findings highlight the importance of targeting these extracellular communication networks to effectively eliminate this resilient population of cells."
The study's insights could pave the way for the development of more effective cancer therapies, as targeting the identified pathway could potentially disrupt the self-renewal, chemoresistance, and metastatic potential of CSCs, which are often responsible for treatment failure and disease recurrence.
Explore further
Feedback to editors

Building a blueprint of metabolic health—from mouse to human
5 minutes ago

High levels of toxic gas detected in Louisiana, threatening children's health
33 minutes ago

The heart grows more in pregnant rats with high blood pressure
41 minutes ago

Social determinants of health linked with youth-onset prediabetes
51 minutes ago

Depressive symptoms may hasten memory decline in older people

Cognitive test is poor predictor of athletes' concussion, research suggests

Research on the visual rabbit illusion takes a leap forward

Possible mechanism for fold formation in the cerebral cortex discovered

A milestone in epigenetics of reproduction: Researchers crack the male fertility code

New insights on polymicrobial infections in chronic lung diseases
Related stories.

New target identified for pancreatic cancer treatment
Sep 26, 2023

Therapeutically harnessing cancer stem cell-derived exosomes
Jan 3, 2024

Mechanical constraints guide spatial pattern of glioblastoma cancer stem cells
Apr 2, 2024

Musashi 1 in breast cancer: Implications for dormancy and survival in bone marrow
May 16, 2023

New way to kill breast cancer stem cells that have ancestral features and resist chemotherapy
Nov 24, 2023

Study links protein secreted by blood vessels to drug-resistant cancer
May 15, 2024
Recommended for you

Researchers develop new tool to aid processing of spatial transcriptomic data
2 hours ago

Genetic mechanisms may reveal retinal vascular disease therapeutic targets

'Self-taught' AI tool helps to diagnose and predict severity of common lung cancer
6 hours ago

New AI tool finds rare variants linked to heart disease in 17 genes
Let us know if there is a problem with our content.
Use this form if you have come across a typo, inaccuracy or would like to send an edit request for the content on this page. For general inquiries, please use our contact form . For general feedback, use the public comments section below (please adhere to guidelines ).
Please select the most appropriate category to facilitate processing of your request
Thank you for taking time to provide your feedback to the editors.
Your feedback is important to us. However, we do not guarantee individual replies due to the high volume of messages.
E-mail the story
Your email address is used only to let the recipient know who sent the email. Neither your address nor the recipient's address will be used for any other purpose. The information you enter will appear in your e-mail message and is not retained by Medical Xpress in any form.
Newsletter sign up
Get weekly and/or daily updates delivered to your inbox. You can unsubscribe at any time and we'll never share your details to third parties.
More information Privacy policy
Donate and enjoy an ad-free experience
We keep our content available to everyone. Consider supporting Science X's mission by getting a premium account.
E-mail newsletter
- BiologyDiscussion.com
- Follow Us On:
- Google Plus
- Publish Now
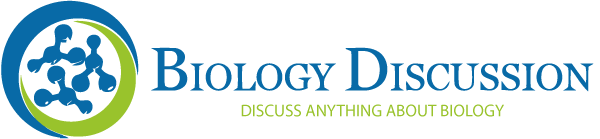
Essay on Cancer Cell Proliferation: Top 7 Essays | Diseases | Biology
ADVERTISEMENTS:
Here is an essay on ‘Cancer Cell Proliferation’ for class 11 and 12. Find paragraphs, long and short essays on ‘Cancer Cell Proliferation’ especially written for college and medical students.
Essay on Cancer Cell Proliferation
Essay Contents:
- Essay on Molecular Changes in Cancer Cell Proliferation
1. Essay on the Introduction to Cancer Cell Proliferation:
Cancer cells exhibit a number of unusual properties that distinguish them from normal cells. One key property is the ability of cancer cells to proliferate in an uncontrolled fashion, thereby leading to a progressive accumulation of dividing cells without regard to the needs of the body as a whole.
In reality, uncontrolled proliferation is not a single discrete property but rather a collection of traits that together allow cancer cells to escape from the usual restraints on cell proliferation. The net result is that cancer cells circumvent the mechanisms designed to ensure that cells divide only when (and where) new cells are needed.
The normal mechanisms for controlling cell proliferation and see how they behave in cancer cells. Through such a discussion it will become apparent that cancer cells exhibit a distinctive collection of abnormal properties, and while no single property is necessarily seen in every cancer cell, as a group these traits contribute to the “profile” of a typical cancer cell.
Although cancer can result from abnormal proliferation and development of tumours, the key issue is, whether the tumour is benign or malignant. The malignant tumour is capable of invading normal tissues, spreading in the body via circulatory or lymphatic systems and establishing secondary tumours (metastasis).
Most cancers fall into one of three main groups: carcinomas which arise from epithelial cells; sarcomas are solid tumours of connective tissues such as muscle, bone, cartilage and fibrous tissue; leukemia’s and lymphomas arise from blood forming cells and from cells of the immune systems, respectively. Further classification of tumours is based on tissue of origin and type of cell involved in malignancy.
A key feature of tumours is their development from single cells that begin to proliferate abnormally, hence clones of cells are present in tumours. The development of malignancy in cell clones is a multistep process accompanied by a series of changes in the cells. In general, cancer is considered as a multistep process involving mutation and selection of cells with progressively increasing capacity for proliferation, invasion and metastasis.
The first step, tumour initiation involves genetic alteration leading to abnormal proliferation of a single cell. Tumour progression continues as additional changes take place in tumour cell population. Metastasis occurs when tumour cells invade other organs and establish secondary sites of malignancy.
Substances that can cause cancer are called carcinogens, and include many agents such as radiation, chemicals, viruses and many more. Radiation and chemicals can initiate cancer by damaging DNA and inducing mutations in key target genes.
Some carcinogens contribute to cancer development by stimulating cell proliferation, rather than by inducing mutations. Such compounds are designated tumour promoters because by inducing increased cell division they produce a proliferative cell population during early stages of tumour development.
Classic examples are the phorbol esters that stimulate cell proliferation by activating protein kinase C. Hormones, particularly estrogens, are important as tumour promoters in the development of some human cancers. For example, the uterine epithelium responds to excess estrogen and increases the likelihood of development of endometrial cancer. Some viruses also induce cancer in experimental animals and humans, such as liver cancer and cervical carcinoma in humans.
Cancer cells display features that distinguish them from their normal counterparts. Cancer cells have abnormalities in the mechanisms that regulate normal cell proliferation, differentiation and survival. In culture, cancer cells can be distinguished from normal cells in displaying density- dependent inhibition of cell proliferation. Normal cells continue to divide until they reach a finite cell density.
They then stop dividing and become quiescent, arrested in the G0 stage of the cell cycle. The proliferation of cancer cells is independent of density-dependent inhibition. Such cells do not respond to signals that cause normal cells to cease proliferation.
Instead, tumour cells continue to grow to high cell densities in culture, this behaviour corresponds with their uncontrolled proliferation in vivo. The proliferation of many normal cells is controlled in part, by polypeptide growth factors. Cancer cells have reduced requirement for extracellular growth factors.
Both in vitro and in vivo, growth factor requirements of cancer cells are reduced contributing to unregulated proliferation of tumour cells. In some cases cancer cells produce growth factors that stimulate their own proliferation. Such an abnormal production of a growth factor leads to continuous stimulation of cell division, called autocrine growth stimulation, and makes cancer cells less dependent on growth factors from normal sources.
2. Essay on the Features of Cancer Cell Proliferation :
The concept of uncontrolled proliferation is unique to multicellular organisms. In most single-celled organisms, such as bacteria or yeast, the presence of sufficient nutrients in the surrounding environment is the main factor that determines whether cells will grow and divide.
The situation is reversed in multicellular organisms; cells are usually surrounded by nutrient-rich extracellular fluids, but the organism as a whole would be quickly destroyed if each cell were to continually grow and divide just because it had access to adequate nutrients. Cancer is a potentially lethal reminder of what happens when cell proliferation continues unabated without being coordinated with the needs of the organism as a whole.
i. Cancer Cells Produce Tumors when Injected into Laboratory Animals :
It is the loss of normal growth control that causes cancer cells to produce a continually growing mass of tissue—in other words, a tumor—but uncontrolled growth does not mean that tumor cells always divide more rapidly than normal cells. Tumors can grow slowly, quickly, or somewhere in between. The distinctive feature of tumor growth is not the speed of cell division but its uncontrolled nature.
In contrast to the proliferation of normal cells, where cell division and cell differentiation are kept in proper balance, this finely tuned arrangement is disrupted in tumors and cell division is uncoupled from cell differentiation, thereby leading to a progressive increase in the number of dividing cells.
To determine experimentally whether a particular cell behaves in this way, the cell must be injected into an appropriate host organism to see if a tumor will develop. Experiments involving animal cells are fairly straightforward because the cells can simply be injected into animals of the same genetic type.
The situation with human cells is more complicated. Injecting human cancer cells into other humans for testing purposes would be unethical, and using standard laboratory animals is not reliable: An animal’s immune system is likely to reject human cells because they are of foreign origin.
One way around this obstacle is to inject human cells into mutant strains of mice whose immune systems are unable to attack and destroy foreign cells. When human cancer cells are injected into such immunologically deficient animals, the cells will usually grow into tumors without being rejected.
ii. Cancer Cells Exhibit Decreased Density-Dependent Inhibition of Growth :
Although studying cancer cells in intact organisms is useful for investigating some of the properties of malignant tumors, issues related to the control of cell proliferation are often easier to investigate in cells grown under artificial laboratory conditions. In such cell culture studies, cancer cells are isolated from a tumor and placed in a defined growth medium containing nutrients, salts, and other molecules required for cell growth.
The main reason for studying cells in culture is that this method allows the cells to be observed under carefully controlled conditions where their behavior can be assessed without the complicating effects of secondary factors present in an intact organism.
When most types of normal cells are placed in a culture vessel (test tube, bottle, flask, or dish) and then covered with an appropriate growth medium, they divide until the surface of the container is covered by a single layer of cells. When this monolayer stage is reached, cell movements and cell division both tend to stop.
In the early 1950s, Michael Abercrombie and Joan Heaysman introduced the term “contact inhibition” to refer to the decrease in cell motility that occurs when cells make contact with one another in culture. The same term has also been used to refer to the inhibition of cell division that takes place when culture conditions become crowded.
Because of the confusion that can result from the double meaning of this term, the phrase density-dependent inhibition of growth is now routinely used when referring to the inhibition of cell division that occurs in crowded cultures.
In contrast to normal cells, cancer cells do not stop dividing when they reach the monolayer stage. Instead, they continue to divide and gradually pile up on top of one another, forming multilayered aggregates (Figure 1). In other words, cancer cells are less susceptible to density- dependent inhibition of growth than are their normal counterparts.
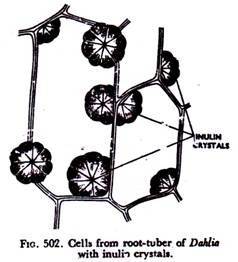
The relationship between the tendency of cancer cells to grow to high population densities in culture and their ability to form tumors has been investigated using cancer cells that differ in their susceptibility to density- dependent inhibition of growth.
Cells that are very sensitive to density-dependent inhibition can be produced by growing cells under un-crowded conditions; every time the population density increases and crowding is imminent, the cells are simply diluted and transferred to a new culture flask.
Cells obtained in this way will not grow to high population densities in culture. Alternatively, cell populations that are insensitive to density-dependent inhibition of growth can be produced by consistently growing cells in overcrowded conditions. Such cell populations become less susceptible to density-dependent inhibition of growth, reaching much higher population densities before cell division stops.
When these different cell populations are tested for their ability to produce tumors in mice, tumor-forming ability is found to be directly related to the loss of density- dependent growth control; in other words, cells capable of growing to the highest population densities in culture are most effective at forming tumors in animals (Figure 2).
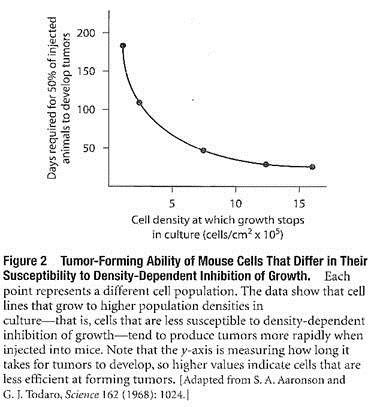
iii. Cancer Cell Proliferation is Anchorage- Independent :
Another way in which the proliferation of cancer cells differs from that of normal cells involves the requirement for anchorage. Most normal cells will not proliferate if they are put in a liquid growth medium and shaken or stirred to keep them in suspension, nor will they proliferate if they are placed in a semisolid medium such as soft agar. When they are provided with an appropriate solid surface to which they can adhere, however, the cells will attach to the surface, spread out, and begin to proliferate (Figure 3).
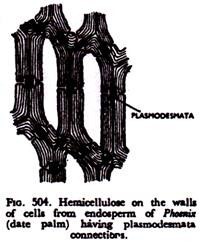
The growth of normal cells is therefore said to be anchorage-dependent. In contrast, most cancer cells grow well not just when they are anchored to a solid surface, but also when they are suspended in a liquid or semisolid medium. The growth of cancer cells is therefore said to be anchorage-independent.
In intact organisms, the requirement that cells be anchored before they can reproduce is met by binding cells to the extracellular matrix, an insoluble meshwork of protein and polysaccharide fibers that fills the spaces between neighboring cells. Cells attach themselves to the extracellular matrix through cell surface proteins called integrins, which bind to molecules present in the matrix.
Apoptotic cell death triggered by lack of contact with the extracellular matrix is called anoikis (from the Greek word for “homelessness”). Anoikis is an important safeguard for maintaining tissue integrity because it prevents normal cells from floating away and setting up housekeeping in another tissue.
The lack of anchorage simply causes cells to commit suicide along the way. Cancer cells are not subject to this normal safeguard because they are anchorage-independent and so can spread to distant sites without self-destructing.
Considerable evidence suggests that anchorage- independent growth exhibited by cells grown in culture is related to their ability to form tumors. One set of studies involved cells with many of the traits of cancer cells, including decreased density-dependent inhibition of growth, low requirements for external growth factors, and anchorage-independent growth.
Single cells were isolated from the original population and allowed to proliferate separately, thereby creating a series of clones, which are individual cell populations each derived from the proliferation of a single cell.
Careful analysis of the clones revealed that some of them had lost one or more of the initial properties. When the ability of these clones to produce tumors in animals was compared, anchorage- independent growth was the only property consistently retained by all the clones that could produce tumors. In other words, the ability to form tumors appeared to require cells whose growth in culture is anchorage-independent.
This connection between anchorage-independent growth and tumor formation is not without its exceptions, however. Some cells exposed to cancer-causing chemicals have been found to exhibit anchorage-independent growth in culture but do not form tumors when injected into animals.
In addition, studies involving a long-term culture of mouse cells, which were anchorage-dependent and unable to form tumors in animals, showed that the cells could acquire the capacity to form tumors if they were attached to glass beads prior to being implanted in mice. Such observations indicate that despite its general association with the ability to form tumors, anchorage- independent growth in culture is not an absolute prerequisite for tumor formation.
iv. Mechanisms for Replenishing Telomeres make Cancer Cells Immortal :
One of the most striking differences between normal cells and cancer cells involves their reproductive lifespans. When normal cells are grown in culture, they usually divide for only a limited number of times. For example, human fibroblasts—a cell type whose behavior has been extensively studied—divide about 50 to 60 times when placed in culture and then stop dividing, undergo a variety of degenerative changes, and may even die (Figure 4).

Cancer cells exhibit no such limit and continue dividing indefinitely, behaving as if they were immortal. A striking example is provided by HeLa cells, which were obtained from a malignant tumor of the uterus arising in a woman named Henrietta Lacks (hence the name “HeLa” cells).
After removing the tumor in a cancer operation performed in 1951, doctors placed some of its cells in culture. The cultured cells began to grow and divide and have continued to do so for more than 50 years, dividing more than 18,000 times with no signs of stopping.
Why are cancer cells capable of reproducing indefinitely in culture, whereas most normal human cells divide no more than 50 or 60 times? The answer is related to the mechanism by which cells replicate their DNA. Each time a cell divides, its chromosomal DNA molecules must be duplicated so that a complete set of genetic instructions can be distributed to each of the two cells produced by cell division.
However, the biochemical mechanism responsible for DNA replication has an inherent limitation- The enzymes that replicate DNA are unable to copy the very end of a linear DNA molecule, perhaps the final 50 to 100 nucleotides or so.
As a result, each time a DNA molecule is replicated; it is in danger of losing a small amount of DNA at each of its two ends. If this trend were to continue indefinitely, DNA molecules would become shorter and shorter until there was nothing left, and we would not be here today!
To solve this so-called end-replication problem, cells place a special type of DNA sequence at the two ends, or telomeres, of each chromosomal DNA molecule. The special DNA consists of multiple copies of a short base sequence repeated over and over again. For example, in humans the six-base sequence TTAGGG is repeated about 2500 times in a row at the ends of each chromosomal DNA molecule at birth.
These telomere sequences are protected by telomere capping proteins, and the DNA also loops back upon itself to protect the end of the chromosome even further (Figure 5). Unlike genes, whose DNA base sequences code for useful products, telomeric DNA does not code for anything but simply consists of the same six-base sequence repeated again and again.
Placing such noncoding telomere DNA at the ends of each chromosome ensures that a cell will not lose any important genetic information when DNA molecules are shortened slightly during replication.

Since telomeres get shorter with each cell division, they provide a counting device for tracking how many times a cell has divided. If a cell divides too many times, the telomeres become extremely short and are in danger of disappearing entirely. When this happens, the telomeric DNA becomes too short to bind telomeric capping proteins or generate a loop, exposing a bare end of double-stranded DNA.
Such unprotected DNA ends are very unstable and often fuse with each other, creating joined chromosomes that tend to become fragmented and separate improperly at the time of cell division.
In normal cells, such a hazardous outcome is prevented by a mechanism in which the unprotected DNA at the end of a chromosome triggers a pathway that halts cell division or triggers cell death. This pathway helps protect organisms from any inappropriate, excessive proliferation of adult cells.
But what happens with cells that must divide for prolonged periods of time, such as the germ cells that give rise to sperm and eggs or the bone marrow cells that continually produce new blood cells? Such cells prevent excessive telomere shortening by producing an enzyme called telomerase, which adds new copies of the telomeric repeat sequence to the ends of existing DNA molecules.
The telomerase-catalyzed addition of new telomere repeat sequences prevents the gradual decline in telomere length that would otherwise occur at both ends of a chromosome during DNA replication. The presence of telomerase therefore allows cells to divide indefinitely without telomere shortening.
How do the preceding considerations apply to cancer cells? If cancer cells behaved like most normal cells, which do not produce telomerase, repeated cell divisions would cause the telomeres to become unusually short and the cells would eventually be destroyed. Most cancer cells circumvent this problem by activating the gene that produces telomerase, thereby causing new copies of the telomeric repeat sequence to be continually added to the ends of their DNA molecules.
A few cancer cells activate an alternative mechanism for maintaining telomere sequences that involves the exchange of sequence information between chromosomes. By one mechanism or the other, cancer cells maintain telomere length above a critical threshold and can therefore divide indefinitely.
3. Essay on Growth Factors and the Cancer Cell Cycle :
We have now seen that cancer cells differ from most normal cells in that they grow to high population densities in culture, exhibit anchorage-independent proliferation, and divide indefinitely because they possess mechanisms for maintaining telomere length.
These traits play an important permissive role in allowing cancer cells to continue dividing, but they do not actually cause cells to divide. The driving force for ongoing proliferation can be traced to abnormalities in the signalling systems that control cell division, the topic to which we now turn.
i. Cancer Cells Exhibit a Decreased Dependence on External Growth Factors :
The cells of multicellular animals do not normally divide unless they are stimulated to do so by an appropriate signalling protein known as a growth factor. For example, if cells are isolated from an organism and placed in a culture medium containing nutrients and vitamins, they will not proliferate unless an appropriate growth factor is also provided.
Growth media are therefore commonly supplemented with blood serum, which contains several growth factors that stimulate cell proliferation. One is platelet-derived growth factor (PDGF), a protein produced by blood platelets that stimulates the proliferation of connective tissue cells and smooth muscle cells. Another growth factor in blood serum, called epidermal growth factor (EGF), is also widely distributed in tissues.
Some growth factors, such as EGF, stimulate the growth of a wide variety of cell types, whereas others act more selectively on particular target cells. Growth factors play important roles in stimulating tissue growth during embryonic and early childhood development, and during wound repair and cell replacement in adults.
For example, release of the growth factor PDGF from blood platelets at wound sites is instrumental in stimulating the growth of tissue required for wound healing.
Growth factors exert their effects by binding to receptor proteins located in the plasma membrane that forms the outer boundary of all cells. Different cell types have different plasma membrane receptors and hence differ in the growth factors to which they respond (Figure 6).

The binding of a growth factor to its corresponding receptor triggers a multistep cascade in which a series of signal transduction proteins relay the signal throughout the cell, triggering molecular changes that stimulate (or occasionally inhibit) cell growth and division.
Cells will not normally divide unless they are stimulated by an appropriate growth factor, but this restraint is circumvented in cancer cells by various mechanisms that create a constant signal to divide, even in the absence of growth factors.
Some cancer cells achieve this autonomy by producing their own growth factors, thereby causing cell proliferation to be stimulated without the need for growth factors produced by other cells. Similarly, other cancer cells possess abnormal receptors that are permanently activated, causing cell division to occur whether growth factors are present or not.
Cancer cells may also produce excessive quantities or hyperactive versions of other proteins involved in relaying signals from cell surface receptors to the cell division machinery in the cell’s interior. The net effect of the preceding types of alterations is to cause the pathways that signal cell proliferation to become hyperactive or even autonomous, functioning in the absence of growth factors.
ii. The Cell Cycle is Composed of G1, S, G2, and M Phases :
To understand how pathways activated by growth factors ultimately cause a cell to divide, it is first necessary to review the events associated with cell division. In cells that are dividing, the nuclear DNA molecules must be duplicated and then distributed in a way that ensures that the two new cells each receive a complete set of genetic instructions. In preparing for and accomplishing these tasks, cells pass through a series of discrete stages called G1 phase, S phase, G2 phase, and M phase.
The four phases are collectively referred to as the cell cycle (Figure 7).
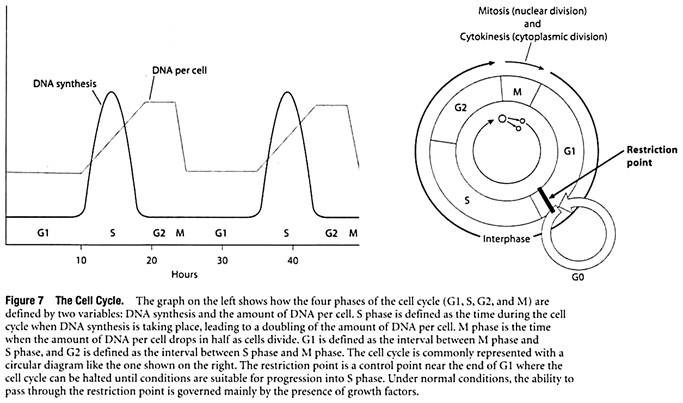
G1—the first phase to occur after a cell has just divided—vanes the most in length. A typical G1 phase lasts about 8 to 10 hours in human cells, but rapidly dividing cells may spend only a few minutes or hours in Gl. Conversely, cells that divide very slowly may become arrested in G1 and spend weeks, months, or even years in the offshoot of G1 called the G0 phase (G zero). After completing G1, the cell enters S phase, a period of roughly 6 to 8 hours when the chromosomal DNA molecules are replicated.
Next comes G2 phase, where 3 to 4 hours are spent making final preparations for cell division. The cell then enters M phase, which takes about an hour to physically divide the original cell into two new cells. The main events of M phase include division of the nucleus, or mitosis, followed by division of the cytoplasm, or cytokinesis. The two newly formed cells then enter again into G1 phase and begin preparations for another round of cell division.
Taken together, the G1, S, and G2 phases are collectively referred to as interphase. Besides providing the time needed for a cell to make copies of its DNA molecules, interphase is also a period of cell growth. Interphase occupies about 95% of a typical cell cycle; whereas the actual process of cell division (M phase) only takes about 5%.
Overall, the time occupied by the various stages of the cycle allows a typical human cell to divide as often as once every 18 to 24 hours. However, the various cell types that make up the body differ greatly in cycle time, ranging from cells that divide very rapidly and continuously to differentiated cells that do not divide at all.
The variability observed in rates of cell division means that mechanisms must exist for regulating progression through the cell cycle. A key control point has been identified during late G1, where the cell cycle is usually halted in cells that stop dividing. For example, the division of cultured cells can be slowed down or stopped by allowing the cells to run out of either nutrients or growth factors, or by adding inhibitors of vital processes such as protein synthesis.
In such cases, the cell cycle is halted in late GI at a point referred to as the restriction point. Under normal conditions, the ability to pass through the restriction point is governed mainly by the presence of growth factors.
Cells that successfully move through the restriction point are committed to S phase and the remainder of the cell cycle, whereas those that do not pass the restriction point enter into GO and reside there for variable periods of time, awaiting a signal that will allow them to re-enter G1 and pass through the restriction point.
iii. Progression through the Cell Cycle is Driven by Cyclin-Dependent Kinases :
At the molecular level, passage through the restriction point and other key points in the cell cycle is controlled by proteins known as cyclin-dependent kinases (Cdks). Cdks are protein kinases, a term referring to a class of enzymes that regulate the activity of targeted protein molecules by catalyzing their phosphorylation (attachment of phosphate groups to the targeted proteins).
During protein phosphorylation reactions, the phosphate group is donated to the targeted protein by the high-energy compound ATP (adenosine triphosphate), which is converted to ADP (adenosine diphosphate) during the reaction. Cells contain dozens of different protein kinases, each designed to regulate the activity of a specific group of proteins by catalyzing their phosphorylation.
As the name implies, a cyclin-dependent kinase (Cdk) only exhibits protein kinase activity when it is bound to another type of protein called a cyclin. Progression through the cell cycle is controlled by several Cdks that bind to different cyclins, thereby creating a variety of Cdk- cyclin complexes.
Cyclins involved in regulating the progression from G1 to S phase are called G1 cyclins, and the Cdk molecules to which they bind are known as G1 Cdks. Likewise, cyclins involved in regulating passage from G2 into M phase are called mitotic cyclins, and the Cdk molecules to which they bind are known as mitotic Cdks. Cdk-cyclin complexes act by phosphorylating specific target proteins whose actions are required for various stages of the cell cycle.
How do Cdk-cyclin complexes ensure that passage through key points in the cell cycle only occurs at the appropriate time? In addressing this question, we will briefly consider the behavior of the mitotic Cdk-cyclin complex (mitotic Cdk bound to mitotic cyclin), which regulates passage from G2 to M phase.
Mitotic cyclin is continuously synthesized throughout interphase and gradually increases in concentration during G1, S, and G2, eventually reaching a concentration that is high enough to bind to mitotic Cdk (Figure 8).
The resulting mitotic Cdk- cyclin triggers passage from G2 into M phase by phosphorylating key proteins involved in the early stages of mitosis. For example, proteins phosphorylated by mitotic Cdk-cyclin trigger nuclear envelope breakdown, chromosome condensation, and mitotic spindle formation.

Shortly thereafter, mitotic cyclin is targeted for degradation by an enzyme called the anaphase-promoting complex and mitotic Cdk becomes inactive, triggering the exit from mitosis. During the next cell cycle, mitosis cannot be triggered until the concentration of mitotic cyclin builds up again.
Besides being regulated by the availability of cyclins, the activity of the various Cdk-cyclin complexes is controlled by reactions in which Cdk molecules are altered by phosphorylation (addition of phosphate groups) and dephosphorylation (removal of phosphate groups).
Figure 9 illustrates how the mitotic Cdk-cyclin complex is regulated in this way:

In step ①, the binding of mitotic cyclin to mitotic Cdk creates a complex that is initially inactive. Before it can trigger passage from G2 into M phase, the complex requires the addition of an activating phosphate group to a particular amino acid of the Cdk molecule. Prior to adding this phosphate, however, inhibitory phosphate groups are first attached to the Cdk molecule at two other locations, preventing the Cdk from functioning (step ②). The activating phosphate group, highlighted with yellow in step ③, is then added. The last step in the activation sequence is the removal of the inhibiting phosphates by a specific enzyme called a protein phosphatase (step ④).
Once the phosphatase begins removing the inhibiting phosphates, a positive feedback loop is set up: The activated Cdk-cyclin complex generated by this reaction stimulates the phosphatase, thereby causing the activation process to proceed more rapidly. After being activated, the mitotic Cdk-cyclin complex triggers passage from G2 into M phase by catalyzing the phosphorylation of proteins required for the onset of mitosis.
Growth Factor Signaling Pathways Act on the Restriction Point by Stimulating Phosphorylation of the Rb Protein :
Now that Cdk-cyclins have been introduced, we can explain how growth factors exert their control over cell proliferation. If normal cells are placed in a culture medium containing nutrients and vitamins but no growth factors, the cells become arrested at the restriction point. Subsequent addition of growth factors is sufficient to cause the cells to start dividing again.
How do growth factors cause G1-arrested cells to resume progression through the cell cycle? The binding of a growth factor to its corresponding cell surface receptor causes the receptor to become activated, and the activated receptor then triggers a complex pathway of reactions involving dozens of different cytoplasmic and nuclear molecules that relay the signal throughout the cell. Here we are concerned only with the question- How do these pathways impinge on the cell cycle and cause cells to pass through the restriction point and into S phase?
The answer to this question is that growth factor signaling pathways trigger the production of Cdk-cyclins that in turn catalyze the phosphorylation of target proteins required for the transition into S phase.
A key target is the Rb protein, a molecule that normally restrains cell proliferation by preventing passage through the restriction point (Figure 10). After Cdk-cyclin phosphorylates Rb, it can no longer exert this inhibitory influence and cells are free to pass through the restriction point and into S phase.
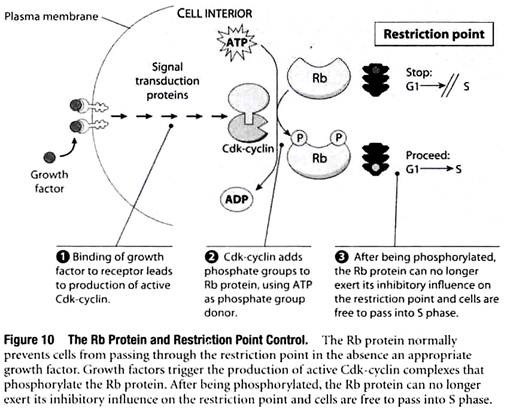
Checkpoint Pathways Monitor for DNA Replication, Chromosome-to-Spindle Attachments, and DNA Damage :
The ability of growth factors to promote passage through the restriction point by stimulating the production of Cdk-cyclins that phosphorylate Rb is just one example of how the cell cycle is controlled by external and internal factors that determine whether or not a cell should divide.
Another type of cell cycle control involves a series of checkpoint pathways that prevent cells from proceeding from one phase to the next before the preceding phase has been properly completed. These checkpoint pathways monitor conditions within the cell and transiently halt the cell cycle at various points if conditions are not suitable for continuing (Figure 11).

One such mechanism, called the DNA replication checkpoint, monitors the state of DNA replication to ensure that DNA synthesis has been completed prior to proceeding with cell division. If DNA replication is not complete, the cell cycle is halted to allow DNA replication to be finished prior to entering M phase. The existence of the DNA replication checkpoint has been demonstrated by treating cells with inhibitors of DNA synthesis.
Under such conditions the final dephosphorylation step involved in activating mitotic Cdk-cyclin (step 4 in Figure 9) is blocked through a series of events triggered by proteins associated with replicating DNA. The resulting lack of active mitotic Cdk-cyclin halts the cell cycle at the end of G2 until DNA replication is completed.
A second checkpoint mechanism, called the spindle checkpoint, acts between the metaphase and anaphase stages of mitosis, the point where the two duplicate sets of chromosomes are about to be parceled out to the two new cells being formed by the process of cell division.
At the end of metaphase, the two sets of chromosomes are normally lined up at the center of the mitotic spindle, a structure composed of microtubules that attach to the chromosomes and eventually pull them into the two newly forming cells. Before chromosome movement begins (the event that marks the beginning of anaphase), the spindle checkpoint mechanism is invoked to make certain that the chromosomes are all properly attached to the spindle.
If the chromosomes are not completely attached, the cell cycle is temporarily halted at this point to allow the process to be completed. In the absence of such a control mechanism for monitoring chromosome- to-spindle attachments, there would be no guarantee that each of the newly forming cells would receive a complete set of chromosomes.
A third type of checkpoint is used to prevent cells with damaged DNA from proceeding through the cell cycle. In this case, a series of DNA damage checkpoints monitor for DNA damage and halt the cell cycle at various points—including late G1, S, and late G2—by inhibiting different Cdk-cyclin complexes. A molecule called the p53 protein plays a central role in these checkpoint pathways.
In the presence of damaged DNA, the p53 protein accumulates and triggers cell cycle arrest to provide time for the DNA damage to be repaired. If the damage cannot be repaired , p53 may also trigger cell death by apoptosis. The ability of p53 to trigger cell cycle arrest or cell death prevents cells with damaged DNA from proliferating and passing the damage on to succeeding generations of cells.
iv. Cell Cycle Control Mechanisms are Defective in Cancer Cells :
The preceding discussion of cell cycle control mechanisms has focused largely on the behavior of normal cells. How do these principles apply to the behavior of cancer cells, which grow and divide in an uncontrolled fashion? We have already seen that cancer cells often produce excessive amounts (or hyperactive versions) of growth factors, receptors, or other components of growth factor signaling pathways.
Such alterations cause an excessive production of the Cdk-cyclins that phosphorylate the Rb protein, thereby providing an ongoing stimulus for cells to pass through the restriction point and divide.
The situation is made even worse by the fact that the restriction point often fails to function properly in cancer cells. When cancer cells are grown under suboptimal conditions—for example, insufficient growth factors, high cell density, lack of anchorage, or inadequate nutrients— that would cause normal cells to become arrested at the restriction point, cancer cells continue to grow and divide without halting at the restriction point.
In other words, cancer cells exhibit a loss of restriction point control. Under extremely adverse conditions, such as severe nutritional deprivation, cancer cells die at random points in the cell cycle rather than arresting at the restriction point.
In addition to the loss of restriction point control, cancer cells frequently exhibit defects in the checkpoint pathways that would otherwise respond to internal problems, such as DNA damage, by halting the cell cycle. Failures in checkpoint pathways, along with the loss of restriction point control, allow cancer cells to continue proliferating under conditions in which the cell cycle of normal cells would stop.
The difference between cell cycle regulation in cancer cells and normal cells can be exploited experimentally using drugs that act at different points in the cycle. For example, staurosporine is a drug that halts the cell cycle at the restriction point, and camptothecin is a drug that kills cells in S phase by disrupting DNA synthesis.
As shown in Figure 12, when cultures of normal cells are exposed to staurosporine followed by camptothecin, the staurosporine halts cells at the restriction point and thus prevents them from entering S phase and being killed by camptothecin. If the two drugs are later removed, the cells again pass through the restriction point and resume dividing.
With cancer cells, the results are quite different. Staurosporine does not stop cancer cells in G1 because of the loss of restriction point control, so the cells proceed into S phase and are killed by camptothecin. This discovery that cancer cells can be killed by drug combinations that do not harm normal cells raises the possibility that similar strategies might eventually be devised for treating cancer patients.
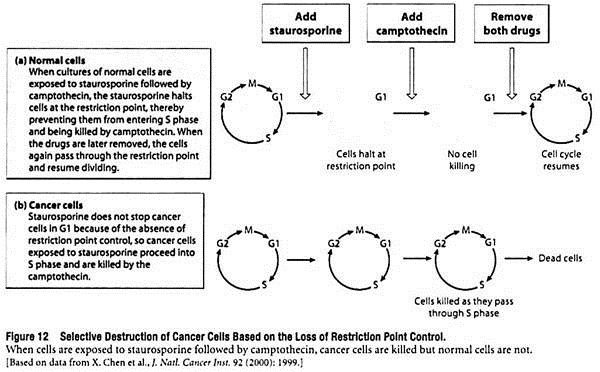
4. Essay on Traits Affecting Cancer Cell Proliferation:
An unrestrained cell cycle, however, is not the only factor that contributes to the uncontrolled production of tumor cells. The number of cells that accumulate in a growing tumor is determined not just by the rate at which cells divide, but also by the rate at which they die. As in the case of the cell cycle, cell death is controlled by pathways that fail to function properly in cancer cells, thereby permitting the survival of cells that would otherwise be destroyed.
Traits that affect cancer cell proliferation by affecting the cell cycle and cell division:
i. Apoptosis is a Mechanism for Eliminating Un-Needed or Defective Cells :
Cell death seems like it would be a random, uncontrolled, undesirable event. In reality, organisms possess a precisely regulated genetic program for inducing individual cells to kill themselves when appropriate. This suicide program, called apoptosis, is designed to prevent the accumulation of unneeded or defective cells that arise during embryonic development as well as later in life.
For example, you might think that embryos would produce only the exact number of cells they need, but that is hardly the case. Embryos produce many extra cells that will not form part of the final organ or tissue in which they arise. A case in point is the human hand, which starts off as a solid mass of tissue.
The fingers are then carved out of the tissue by a process in which apoptosis is invoked to destroy the cells that would otherwise form a webbing between the fingers. Apoptosis is also important in the newly forming brain, where extra nerve cells created during embryonic development are destroyed by apoptosis during early infancy as the final network of nerve connections is established.
Another function of apoptosis is to rid the body of defective cells. For example, cells infected with viruses often invoke apoptosis to trigger their own destruction, thereby limiting reproduction and spread of the virus. Cells with damaged DNA may also trigger apoptosis, especially if the damage cannot be repaired. This ability to destroy genetically damaged cells is especially useful in helping avert the development of cancer.
ii. Apoptosis is Carried Out by a Caspase Cascade :
Apoptosis is a unique type of cell death, quite different from what happens when cells are destroyed by physical injury or exposure to certain poisons. In response to such nonspecific damage, cells undergo necrosis, a slow type of death in which cells swell and eventually burst, spewing their contents into the surrounding tissue. Necrosis often results in an inflammatory reaction that can cause further cell destruction, which makes it potentially dangerous.
In contrast, apoptosis kills cells in a quick and neat fashion without causing damage to surrounding tissue. The process involves a carefully orchestrated sequence of intracellular events that systematically dismantle the cell (Figure 13). The first observable change in a cell undergoing apoptosis is cell shrinkage. Next, small bubble-like protrusions of cytoplasm (“blebs”) start forming at the cell surface as the nucleus and other cellular structures begin to disintegrate.
The chromosomal DNA is then degraded into small pieces and the entire cell breaks apart, forming small fragments known as apoptotic bodies. Finally, the apoptotic bodies are swallowed up by neighboring cells called phagocytes, which are specialized for ingesting foreign matter and breaking it down into molecules that can be recycled for other purposes.

Apoptosis is carried out by a series of protein- degrading enzymes known as caspases. Normally, caspases reside in cells in the form of inactive precursors called procaspases. When a cell receives a signal to commit suicide, an initiating member of the procaspase family is converted into an active caspase.
The activated caspase catalyzes the conversion of another procaspase into an active caspase, which activates yet another procaspase, and so forth. Some members of this caspase cascade destroy key cellular proteins.
For example, one caspase degrades a protein involved in maintaining the structural integrity of the nucleus, and another caspase degrades a protein whose destruction releases an enzyme that causes fragmentation of chromosomal DNA. Hence, the net effect of the caspase cascade is the activation of a series of enzymes that degrade the cell’s main components, thereby leading to an orderly disassembly of the dying cell.
iii. Cancer Cells are able to Evade Apoptosis :
The presence of procaspases within a cell means that the cell is programmed with the seeds of its own destruction, ready to commit suicide quickly if so required. It is therefore crucial that the mechanisms employed to control caspase activation are precisely and carefully regulated and are called into play only when there is a legitimate need to destroy an unneeded or defective cell. There are two main routes for activating the caspase cascade, an external pathway and internal pathway (see Figure 13, bottom).
The external pathway is employed when a cell has been targeted for destruction by other cells in the surrounding tissue. In such cases, neighboring cells produce molecules that transmit a “death signal” by binding to death receptors present on the outer surface of the targeted cell.
The activated death receptors then interact with, and trigger activation of, initiator procaspase molecules located inside the cell, thereby starting the caspase cascade.
The internal pathway—a pathway that is particularly relevant to the field of cancer biology—functions mainly in the destruction of cells that have sustained extensive DNA damage. Although cells possess several mechanisms for repairing DNA damage, in many cases it is safer to destroy cells in which there is any question about the integrity of their DNA.
In this way, the potential danger posed by the proliferation of mutant cells is minimized. The protein plays a pivotal role in the mechanism by which, apoptosis is induced in cells that have sustained extensive DNA damage. The presence of damaged DNA triggers the accumulation of the p53 protein, which in turn stimulates the production of proteins that alter the permeability of mitochondrial membranes. The altered mitochondria then release a group of proteins, especially cytochrome c, that activate the caspase cascade and thereby cause the cell to be destroyed by apoptosis.
Given that killing defective cells is one of the main functions of apoptosis, why aren’t cancer cells destroyed?
After all, cancer cells fit the definition of defective cells- They grow in an uncontrolled fashion and, as you will learn shortly, possess DNA mutations and other chromosomal abnormalities. The reason cancer cells are still able to survive is that they have developed ways of avoiding apoptosis. One common mechanism is that many cancer cells have mutations that disable the gene coding for thereby disrupting the main internal pathway for triggering apoptosis.
Mutations the gene are the most common genetic defect observed in human cancers. Other genes involved in apoptosis may also be altered in cancer cells. For example, the gene coding for the Bcl2 protein, a naturally occurring inhibitor of apoptosis, is altered in some cancers in a way that causes too much Bcl2 to be produced, thereby blocking apoptosis.
5. Essay on the Role of DNA in Cancer Cell Proliferation:
i. Cancer Cells are Genetically Unstable and often Exhibit Gross Chromosomal Abnormalities :
The number of mutations accumulated by cancer cells is generally greater than would be expected in comparable populations of normal cells. This tendency to accumulate an excessive number of mutations and other kinds of DNA damage is called genetic instability.
As we have just seen, one reason for genetic instability is that cancer cells may exhibit defects in DNA repair that diminish their ability to correct DNA mutations. The net result is elevated mutation rates that can be hundreds or even thousands of times higher than normal.
Another factor is that cancer cells often exhibit defects in the pathways that trigger apoptosis in response to DNA damage. As a result, cells that have incurred extensive DNA damage that is beyond repair do not self-destruct by apoptosis as would normally be expected.
Mistakes in the handling of chromosomes during cell division also contribute to genetic instability by creating gross abnormalities in chromosome structure and number. Normally, human cells other than sperm and eggs possess 23 pairs of chromosomes, or a total of 46 chromosomes per cell.
Such cells are said to be diploid (from the Greek word diplous, meaning “double”) because two copies of each type of chromosome are present, one derived from each parent. In contrast, cancer cells are often aneuploid, which means that they possess an abnormal number of chromosomes. Aneuploidy usually involves both the loss of some chromosomes and extra copies of others.
In addition to an abnormal number of chromosomes, cancer cells often possess chromosomes whose structure has been altered by deletions (loss of long stretches of DNA) and translocations (exchange of long stretches of DNA between different chromosomes).
One of the first chromosomal abnormalities to be consistently observed in any type of cancer was the Philadelphia chromosome, an oddly shaped chromosome present in the cancer cells of nearly 90% of all individuals with chronic myelogenous leukemia (Figure 19).
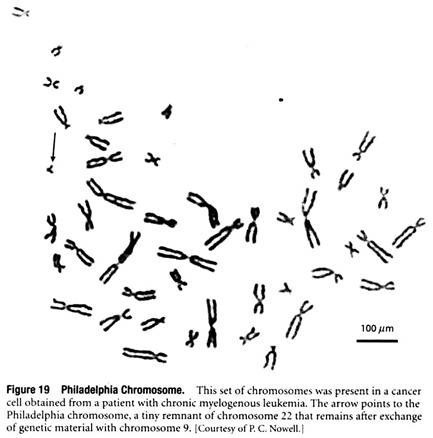
The Philadelphia chromosome is produced by DNA breakage near the ends of chromosomes 9 and 22, followed by reciprocal exchange of DNA between the two chromosomes. A similar phenomenon is observed in Burkitt’s lymphoma, a cancer of human lymphocytes, in which segments derived from chromosomes 8 and 14 are exchanged. In both of these situations, scientists have identified the specific gene whose alteration by chromosomal translocation leads to cancer.
Abnormalities in chromosome number and structure contribute to cancer development in various ways. Some crucial genes may be lost, other genes may become overactive, and yet other genes may become structurally altered and produce abnormal products. In contrast to mutations involving short stretches of DNA, gross chromosomal defects are extremely difficult, if not impossible, to repair.
The best solution for cells exhibiting such chromosomal problems is suicide by apoptosis. However, if cells have genetic defects that disrupt the pathways responsible for carrying out apoptosis, self- inflicted suicide is not an option and the genetically damaged cells will continue to proliferate.
ii. DNA Mutations can Lead to Cancer :
The presence of gross chromosomal defects in cancer cells was first reported almost a hundred years ago, and the smaller changes in base sequence that occur in the DNA of cancer cells have also been recognized for many years.
For a long time, it was difficult to distinguish whether such DNA abnormalities are responsible for causing cancer or whether they simply represent secondary changes that arise because cancer cells are dividing in a rapid, uncontrolled fashion. In other words, the question was much like the classic problem of “which came first, the chicken or the egg?” Do DNA abnormalities cause cancer to arise, or does cancer cause DNA abnormalities to arise?
This issue was finally resolved in the early 1980s, when DNAs isolated from several different human cancers were shown to cause cancer under laboratory conditions. In the first studies of this type (Figure 20), DNA was extracted from human bladder cancer tissue and applied to a culture of normal mouse cells under experimental conditions that favor incorporation of the foreign DNA into the cells’ chromosomes.

The uptake of foreign DNA by cells under such artificial laboratory conditions is called transfection. In response, some of the cultured mouse cells began to proliferate excessively. When these proliferating cells were injected back into mice, the animals developed cancer.
Similar experiments using DNA extracted from normal human tissues did not produce mouse cells capable of forming tumors. It was therefore concluded that DNA obtained from human bladder cancer contains gene sequences, not present in normal DNA, that are capable of causing cancer.
Similar results were subsequently obtained using DNA isolated from other human cancers. Such studies have facilitated the identification of a number of specific genes that contribute to cancer development.
6. Essay on Immune System and Cancer Cell Proliferation:
The ability of cancer cells to proliferate in an uncontrolled fashion, combined with their capacity to spread through the body, makes them a potentially lethal hazard. Are any of the body’s normal defense mechanisms capable of protecting against such a threat? The immune system is designed to defend against infection by potentially harmful agents, such as bacteria, viruses, fungi, parasites, and it also attacks foreign tissues and cells.
Is the immune system also capable of recognizing cancer cells, and if so, how does cancer cells frequently manage to thrive despite the immune system? In addressing these questions, let us start by reviewing the basic mechanisms involved in an immune response.
i. Immune Responses are Carried Out by B Lymphocytes, T Lymphocytes, and NK Cells:
Molecules capable of provoking an immune response are referred to as antigens. To function as an antigen, a substance must be recognized as being “foreign”—that is, different from molecules normally found in a person’s body. The more a molecule differs in structure from normal tissue constituents, the greater the intensity of the immune response mounted against that substance.
Antigens also need to be susceptible to degradation and processing by cells. This requirement explains why non-degradable foreign materials, such as stainless steel pins and plastic valves, can be surgically implanted into humans without eliciting an immune response. To trigger an efficient immune response, an antigen must be degraded and processed by specialized antigen-presenting cells that “present” antigens to cells of the immune system in a way designed to activate an immune response.
Macrophages and dendritic cells are among the most commonly encountered antigen-presenting cells. As shown in Figure 21, antigens engulfed by these cells are degraded into small fragments that eventually become bound to cell surface proteins called major histocompatibility complex (MHC) molecules. When an antigen fragment bound to an MHC molecule is present at the surface of an antigen- presenting cell, the MHC-antigen complex stimulates cells called lymphocytes to mount an attack against that particular antigen.
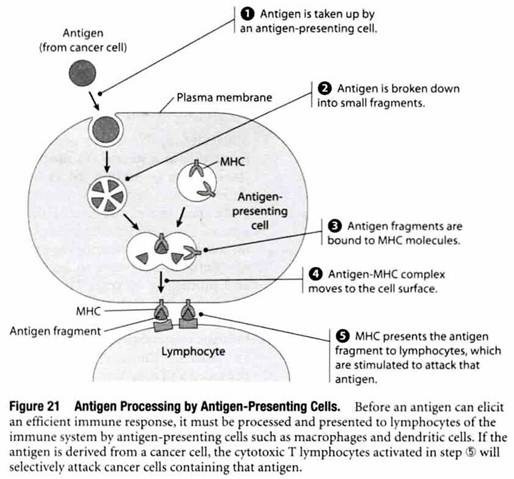
The stimulated lymphocytes attack foreign antigens in two different ways. One group of lymphocytes, called B lymphocytes, produce proteins called antibodies, which circulate in the bloodstream and penetrate into extracellular fluids, where they bind to the foreign antigen that induced the immune response. Another group of lymphocytes, called cytotoxic T lymphocytes (CTLs), bind to cells exhibiting foreign antigens on their surface and kill the targeted cells by causing them to burst.
In addition to B and T lymphocytes, a small fraction of the total lymphocyte population consists of natural killer (NK) cells that possess the intrinsic ability to recognize and kill certain kinds of tumor cells (as well as virus-infected cells).
In contrast to a typical immune response involving antibody formation or cytotoxic T lymphocytes, NK cells do not need to recognize a specific antigen before attacking a target cell. Instead, they are programmed to attack a broad spectrum of abnormal cells in a relatively indiscriminate fashion, while leaving normal cells unharmed.
ii. Some Cancer Cells Possess Antigens that Trigger an Immune Response :
Because so many people develop cancer, it is clear that the ability of NK cells to attack tumors is often overwhelmed.
A more powerful and efficient immune response requires the participation of cytotoxic T lymphocytes, whose killing activity is selectively directed at cells containing a specific antigen. For cytotoxic T lymphocytes to become involved, however, they need to recognize an antigen as being foreign or abnormal.
The question of whether cancer cells exhibit unique antigens that can elicit such an immune response has had a long and controversial history. Part of the difficulty in reaching a consensus arises because cancers exhibit a variety of antigenic changes. In human melanomas, for example, at least three classes of antigens have been detected.
Antigens of the first type are specific both for melanomas and for the person from whom a particular melanoma is obtained. Antigens of the second type are specific for melanomas but not for the particular person who has the melanoma. Antigens of the two preceding types cannot be detected in normal cells and are therefore examples of tumor-specific antigens.
Antigens of the third type are present in both normal and melanoma cells, although their concentration in melanoma cells is greater. Such antigens which are present in higher concentration in a tumor but are not unique to tumors are more accurately referred to as tumor-associated antigens.
The distinction between tumor-specific and tumor- associated antigens is sometimes difficult to make. For example, a group of molecules called MAGE antigens are expressed in melanomas and several other cancers but not in most normal tissues. The MAGE antigens are therefore close to being “tumor-specific”, and an immune response directed against them would be expected to be reasonably selective, causing minimum damage to normal tissues.
Other tumor-associated antigens, such as the prostate-specific antigen (PSA) produced by prostate cancer cells, are unique to a specific tissue. Although antigens of this type are produced by normal cells as well, an immune response directed against them would be relatively selective in that it is only directed against a single tissue.
Antigens that are genuinely tumor-specific occur in cancers that produce structurally abnormal proteins. Such proteins do not appear in normal cells and thus can be recognized by the immune system as being “foreign”. There are several examples of mutant cancer cell genes that produce abnormal proteins.
These molecules can act as antigens that elicit a highly selective, cytotoxic T cell response against the tumor cells in which they are found, provided that the mutant proteins are processed and presented to the immune system in the appropriate fashion.
iii. The Immune Surveillance Theory Postulates that the Immune System is able to Protect against Cancer :
The existence of tumor-specific antigens raises an interesting question- Why don’t people with cancer reject their own tumors? The immune surveillance theory postulates that immune destruction of newly forming cancer cells is in fact a routine event in healthy individuals, and cancer simply reflects the occasional failure of an adequate immune response to be mounted against aberrant cells.
The validity of this theory has been debated for many years, with various kinds of evidence being cited both for and against it.
Some of the evidence involves organ transplant patients who take immunosuppressive drugs, which depress immune function and thereby decrease the risk of immune rejection of the transplanted organ. As would be predicted by the immune surveillance theory, individuals treated with immunosuppressive drugs develop many cancers at higher rates than normal (Figure 22).

Although this finding appears to support the idea that the immune system normally helps prevent cancers from developing, it is also possible that the immunosuppressive drugs are acting directly to trigger the development of cancer. For example, cyclosporin—one of the most effective and commonly used immunosuppressive drugs—has been shown to stimulate the proliferation and motility of isolated cancer cells growing in culture.
These results indicate that direct effects of immunosuppressive drugs on newly forming cancer cells may contribute to the increased tumor growth observed in individuals taking such drugs.
A more direct approach for evaluating the immune surveillance theory involves the use of animals that have been genetically altered to introduce specific defects in the immune system. One study of this type employed mutant mice containing disruptions in Rag2, a gene expressed only in lymphocytes.
The mutant mice, which produce no functional lymphocytes, were found to develop cancer more frequently than do normal mice. An increased cancer risk was observed both for cancers that arise spontaneously and for cancers that were induced by injecting animals with a cancer-causing chemical. Such results indicate that a normally functioning immune system helps protect mice against the development of cancer.
Nonetheless, the question still exists as to the relevance of these findings to human cancers. If the immune system plays a significant role in protecting humans from common cancers, you would expect to see a dramatic increase in overall cancer rates in AIDS patients with severely depressed immune function.
While people with AIDS do exhibit higher rates for a few types of cancer, especially Kaposi’s sarcoma and lymphomas, increased rates for the more common forms of cancer have not been observed. Most of the cancers that do occur in higher rates in AIDS patients are known to be caused by viruses.
Such observations suggest that immune surveillance may play an important role in protecting humans from virally induced cancers but that it is less effective in preventing the more common forms of cancer.
iv. Cancer Cells have Various Ways of Evading the Immune System :
Based on the large number of people who develop cancer each year, it is clear that tumors routinely find ways of evading destruction by the immune system. One mechanism is based on tumor progression, which refers to the gradual changes in the makeup of cancer cell populations that occur over time as natural selection favors the survival of cells that are more aggressive and aberrant.
During tumor progression, cells containing antigens that elicit a strong immune response are most likely to be attacked and destroyed. Conversely, cells that either lack or produce smaller quantities of antigens marking them for destruction are more likely to survive and proliferate. So as tumor progression proceeds, there is a continual selection for cells that invoke less of an immune response.
Cancer cells have also devised ways of actively confronting and overcoming the immune system. For example, some cancer cells produce molecules that kill T lymphocytes or disrupt their ability to function.
Tumors may also surround themselves with a dense layer of supporting tissue that shields them from immune attack. And some cancer cells simply divide so quickly that the immune system cannot destroy them fast enough to keep tumor growth in check. Consequently, the larger a tumor grows, the easier it becomes to overwhelm the immune system.
Although tumors are often successful at evading immune attack, immune rejection is not necessarily an unattainable objective. Experiments in mice have shown that immunizing an animal with tumor antigens can trigger an effective immune response under conditions in which the tumor growing in the animal had not elicited any response on its own. Observations like this one suggest that it may be possible to stop or even prevent the development of cancer by stimulating a person’s immune system to attack cancer cells.
7. Essay on Molecular Changes in Cancer Cell Proliferation:
Some of these alterations involve unique or abnormal proteins that are not ordinarily encountered in adult tissues, but most of the molecular changes seen in cancer cells proliferation are increases or decreases in the quantity or activity of normal proteins. We will examine a few such changes that are especially noteworthy.
i. Cancer Cells Exhibit Cell Surface Alterations that Affect Adhesiveness and Cell-Cell Communication :
Alterations in the makeup of the outer cell membrane (plasma membrane) are almost universally observed in cancer cells, yet it is often difficult to assess the significance of such changes because similar alterations occur in normal cells when they begin to proliferate.
For example, membrane transport proteins responsible for the uptake of sugars, amino acids, and other nutrients are frequently activated in tumor cells, but similar increases are observed in normal cells that have been stimulated to divide by adding nutrients or growth factors. It is therefore important to distinguish between membrane changes that play unique roles in cancer cells and membrane changes that are characteristic of dividing cells in general.
Among the cell surface changes that are particularly distinctive and important for the behavior of cancer cells are those that influence adhesiveness. In normal tissues, cell-cell adhesion helps keep cells in their place; in cancers, this adhesiveness is diminished or missing entirely. The reduced adhesiveness of cancer cells can often be traced to defects in E-cadherin, a cell-cell adhesion protein that is located at the cell surface.
A second cell surface property altered in cancer cells is their enhanced tendency to clump together when exposed in the laboratory to proteins called lectins. A lectin is a carbohydrate-binding protein possessing two or more carbohydrate-binding sites, which means that a single lectin molecule can link two cells together by binding to carbohydrate groups exposed on the surface of each cell.
As a result, when lectins are added to a suspension of isolated cells, the lectin molecules link the cells together to form large clumps (Figure 23). It was initially thought that the increased susceptibility to lectin-induced clumping meant that cancer cells possess more cell surface carbohydrate groups to which lectins can bind.
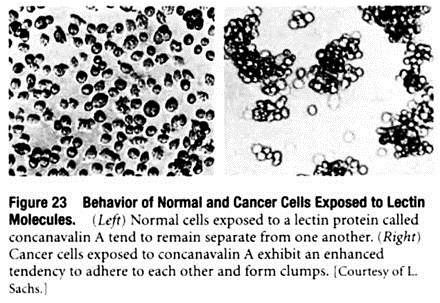
Careful measurements, however, have led to the conclusion that the total number of these carbohydrate groups tends to be similar in normal and cancer cells. What does appear to differ is the mobility of carbohydrate groups within the plasma membrane, which tends to be greater in cancer cells than in normal cells. This ability of cell surface carbohydrate groups to move more readily in cancer cells apparently increases the rate at which they can bind to added lectins.
Another cell surface alteration commonly observed in cancer cells is a decrease in the number of gap junctions, which are specialized cell surface structures composed of a protein called connexin. Gap junctions play a role in cell- cell communication by joining adjacent cells together in a way that allows small molecules to pass directly from one cell to another.
The idea that gap junctions are deficient in cancer cells has come from experiments showing that small fluorescent molecules injected into normal cells move rapidly into surrounding cells that are normal but not into cells that are malignant (Figure 24). To investigate whether this deficiency plays any role in the loss of growth control, studies have been performed in which normal cells were fused with cancer cells that had lost the ability to form gap junctions.

Initially, the resulting hybrid cells produced gap junctions and exhibited normal growth control, but the gap junctions eventually disappeared from some of the hybrid cells. At that point the cells reverted to uncontrolled growth; raising the interesting possibility that normal growth control is influenced by the ability of cells to communicate through gap junctions.
ii. Cancer Cells Produce Embryonic Proteins, Proteases, and Stimulators of Blood Vessel Growth :
A great deal of effort has been expended in searching for molecules that are produced only by cancer cells and that might therefore serve as “markers” for detecting the presence of cancer. Unfortunately, few of the molecules identified thus far are broadly useful as unique identifiers of cancer cells, although a number of them can be employed for detecting the presence of specific kinds of cancer. For example, some cancer cells manufacture and secrete proteins that are usually found only in embryos.
One such protein, alpha-fetoprotein, is produced by embryonic liver cells but is detectable in only trace amounts in normal adults. In people with liver cancer, the concentration of alpha-fetoprotein in the blood increases dramatically. Carcinoembryonic antigen (CEA), a protein produced in the embryonic digestive tract, and fetal hormones such as chorionic gonadotropin and placental lactogen, are also secreted by some cancers.
Blood tests for embryonic markers such as alpha-fetoprotein and carcinoembryonic antigen can therefore be used to monitor the presence of certain kinds of cancer, but the fact that these substances are made by only a few tumor types limits the applicability of this approach.
Other proteins produced by cancer cells are not unique to such cells but have provided some important insights into the behavior of malignant tumors. For example, cancer cells tend to produce proteases (protein- degrading enzymes) that facilitate the breakdown of structures that would otherwise represent barriers to cancer cell movement and invasion.
Although proteases are also secreted by certain kinds of normal cells, their enhanced production by cancer cells facilitates the ability of malignant tumors to invade surrounding tissues and enter the circulatory system. Cancer cells also produce proteins that stimulate the growth of blood vessels, thereby helping ensure that tumors have a sufficient blood supply.
Related Articles:
- Essay on the Causes of Cancer (962 Words) | Cancer
- Essay on the Biology of Cancer | Types | Diseases | Biology
Biology , cancer , Cancer Cell Proliferation , Diseases , Essay , Essay on Cancer Cell Proliferation
- Anybody can ask a question
- Anybody can answer
- The best answers are voted up and rise to the top
Forum Categories
- Animal Kingdom
- Biodiversity
- Biological Classification
- Biology An Introduction 11
- Biology An Introduction
- Biology in Human Welfare 175
- Biomolecules
- Biotechnology 43
- Body Fluids and Circulation
- Breathing and Exchange of Gases
- Cell- Structure and Function
- Chemical Coordination
- Digestion and Absorption
- Diversity in the Living World 125
- Environmental Issues
- Excretory System
- Flowering Plants
- Food Production
- Genetics and Evolution 110
- Human Health and Diseases
- Human Physiology 242
- Human Reproduction
- Immune System
- Living World
- Locomotion and Movement
- Microbes in Human Welfare
- Mineral Nutrition
- Molecualr Basis of Inheritance
- Neural Coordination
- Organisms and Population
- Photosynthesis
- Plant Growth and Development
- Plant Kingdom
- Plant Physiology 261
- Principles and Processes
- Principles of Inheritance and Variation
- Reproduction 245
- Reproduction in Animals
- Reproduction in Flowering Plants
- Reproduction in Organisms
- Reproductive Health
- Respiration
- Structural Organisation in Animals
- Transport in Plants
- Trending 14
Privacy Overview
Cookie | Duration | Description |
---|---|---|
cookielawinfo-checkbox-analytics | 11 months | This cookie is set by GDPR Cookie Consent plugin. The cookie is used to store the user consent for the cookies in the category "Analytics". |
cookielawinfo-checkbox-functional | 11 months | The cookie is set by GDPR cookie consent to record the user consent for the cookies in the category "Functional". |
cookielawinfo-checkbox-necessary | 11 months | This cookie is set by GDPR Cookie Consent plugin. The cookies is used to store the user consent for the cookies in the category "Necessary". |
cookielawinfo-checkbox-others | 11 months | This cookie is set by GDPR Cookie Consent plugin. The cookie is used to store the user consent for the cookies in the category "Other. |
cookielawinfo-checkbox-performance | 11 months | This cookie is set by GDPR Cookie Consent plugin. The cookie is used to store the user consent for the cookies in the category "Performance". |
viewed_cookie_policy | 11 months | The cookie is set by the GDPR Cookie Consent plugin and is used to store whether or not user has consented to the use of cookies. It does not store any personal data. |
- DOI: 10.1016/j.bcp.2024.116319
- Corpus ID: 270049024
Ubiquitin-specific peptidase 15 regulates the TFAP4/PCGF1 axis facilitating liver metastasis of colorectal cancer and cell stemness.
- Hongzhuan Yin , Di Wu , +2 authors Lianrong Zhao
- Published in Biochemical Pharmacology 1 May 2024
- Medicine, Biology
45 References
Carcinoma-associated fibroblasts promote the stemness and chemoresistance of colorectal cancer by transferring exosomal lncrna h19, s-adenosylmethionine inhibits colorectal cancer cell migration through mirna-mediated targeting of notch signaling pathway, ubiquitin-specific protease 15 contributes to gastric cancer progression by regulating the wnt/β-catenin signaling pathway, usp47 maintains the stemness of colorectal cancer cells and is inhibited by parthenolide., tm4sf1 promotes emt and cancer stemness via the wnt/β-catenin/sox2 pathway in colorectal cancer, usp15 negatively regulates lung cancer progression through the traf6-becn1 signaling axis for autophagy induction, tfap4 promotes the growth of prostate cancer cells by upregulating foxk1, elevated tfap4 regulates lncrna trerna1 to promote cell migration and invasion in gastric cancer., microrna‑135b regulates the stability of pten and promotes glycolysis by targeting usp13 in human colorectal cancers., usp47-mediated deubiquitination and stabilization of tcea3 attenuates pyroptosis and apoptosis of colorectal cancer cells induced by chemotherapeutic doxorubicin, related papers.
Showing 1 through 3 of 0 Related Papers
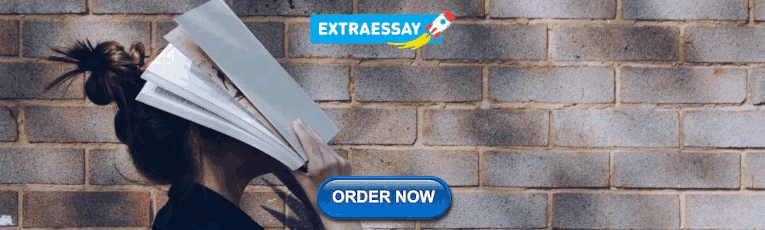
IMAGES
VIDEO
COMMENTS
The Biology of Cancer. Cancer is a disease that begins with genetic and epigenetic alterations occurring in specific cells, some of which can spread and migrate to other tissues. 4 Although the biological processes affected in carcinogenesis and the evolution of neoplasms are many and widely different, we will focus on 4 aspects that are particularly relevant in tumor biology: genomic and ...
The Definition of Cancer. Cancer is a disease in which some of the body's cells grow uncontrollably and spread to other parts of the body. Cancer can start almost anywhere in the human body, which is made up of trillions of cells. Normally, human cells grow and multiply (through a process called cell division) to form new cells as the body ...
Cancer is basically a disease of uncontrolled cell division. Its development and progression are usually linked to a series of changes in the activity of cell cycle regulators. For example, inhibitors of the cell cycle keep cells from dividing when conditions aren't right, so too little activity of these inhibitors can promote cancer.
Find out which papers captured our readers' attention in 2023. All papers published in Cancer Cell a year ago are available for free. Celebrating 20 years and beyond. Latest cancer news and research. Cancer Cell provides a high-profile forum to promote major translational and clinical advances in cancer research and oncology.
Nature Cell Biology aims to be a forum for papers that employ robust models and provide mechanistic insight into fundamental questions in cancer cell biology. Nearly all our cancer biology papers ...
T cells play a central role in immune responses to cancer. In this guide to cancer immunotherapy, the authors provide a comprehensive historical and biological perspective on cancer immunotherapy ...
Takeaway. Cancer causes cells to divide uncontrollably. This can result in tumors, damage to the immune system, and other impairment that can be fatal. Cancer can affect various parts of the body ...
The number of new cell therapy trials has decreased in the past two years. Our analysis of changes in the cell therapy landscape over the past five years shows that the number of new trials peaked ...
Characteristics of Cancer Cells. Cancer cells grow and divide at an abnormally rapid rate, are poorly differentiated, and have abnormal membranes, cytoskeletal proteins, and morphology. The abnormality in cells can be progressive with a slow transition from normal cells to benign tumors to malignant tumors. In 2000 cancer biologists Robert ...
Cancer is a leading cause of death worldwide, accounting for nearly 10 million deaths in 2020, or nearly one in six deaths. The most common cancers are breast, lung, colon and rectum and prostate cancers. Around one-third of deaths from cancer are due to tobacco use, high body mass index, alcohol consumption, low fruit and vegetable intake, and ...
Hematologic (blood) cancers are cancers of the blood cells, including leukemia, lymphoma, and multiple myeloma. Solid tumor cancers are cancers of any of the other body organs or tissues. The most common solid tumors are breast, prostate, lung, and colorectal cancers. These cancers are alike in some ways, but can be different in the ways they ...
1. Excessive smoking has been found to be associated with lung cancer, cancer in urinary bladder, mouth, throat, larynx etc. 2. Chewing of tobacco, betel nuts etc. are believed to be the cause of cancer in mouth, throat, oesophagus etc. 3. A large number of dyes, chemicals, heavy metals can cause cancer. 4.
In 1996, European cancer researchers investigating a protein that's unusually abundant in drug-resistant cancer cells cloned the gene and discovered that it resembled the gene for MVP in rats. Working with Valerie Kickhoefer in Rome's lab, the group then found that the drug-resistant cancer cells generated many more vaults than nonresistant ...
In the recent few decades, the number of cancer has been increasingly on the rise. So let us take a look at the meaning, causes, and types of cancer in this essay on cancer. Cancer comes in many forms and types. Cancer is the collective name given to the disease where certain cells of the person's body start dividing continuously, refusing to ...
There are many differences between cancer cells and normal cells in noncancerous (benign) or cancerous (malignant) tumors. The major differences between normal cells and cancer cells relate to growth, communication, cell repair and death, "stickiness" and spread, appearance, maturation, evasion of the immune system, function and blood supply ...
Genetic, cell, and tissue engineering. There are broadly two ways to target cancer cells to optimize the effectiveness of treatment: enhance cancer cell susceptibility to drug treatment or the immune response, and empower the immune response to locate and destroy cancer cells more effectively. Genetic and cell engineering address both approaches.
Chromosomal instability: the initial trigger. Dissemination of cancer cells precedes the initial steps of the invasion-metastasis cascade. 6 The cascade is the consequence of chromosomal ...
Nov. 29, 2022 — Understanding how cancer cells evolve from healthy brain cells and evade treatment could open up potential new drug therapies for glioblastomas, one of the most common and lethal ...
DOI: 10.1038/s41467-024-47680-z. NDORMS researchers have identified a critical axis that controls the formation and behavior of cancer stem cells (CSCs), a subpopulation of cells that influence ...
That's the conclusion of a recent study investigating eight cell lines that are consistently misspelled across 420 papers published from 2004 to 2023, including in highly ranked journals in cancer research. Some of the misspellings may have been inadvertent errors, but a subset of 235 papers provided details about seven of the eight lines ...
1. Essay on Cancer Treatment: (Around 200 Words) Success of Cancer Treatments: The success rates for current cancer treatments are strongly influenced by the stage at which the disease is diagnosed. When cancer is detected early and tumor cells are still localized to their initial site of origin, cure rates tend to be very high, even for ...
1. Essay on the Introduction to Cancer Cell Proliferation: Cancer cells exhibit a number of unusual properties that distinguish them from normal cells. One key property is the ability of cancer cells to proliferate in an uncontrolled fashion, thereby leading to a progressive accumulation of dividing cells without regard to the needs of the body ...
Basic areas of interest to a broad readership of Cancer Letters include the molecular genetics and cell biology of cancer, radiation biology, molecular pathology, hormones and ... View all calls for papers for special issues. More opportunities to publish your research: Browse open Calls for Papers beta. Special issues and article collections. ...
The present study was planned to explore the anticancer effects of pelargonidin against the lung cancer A549 cells via analyzing oxidative stress-mediated apoptosis. The viability of both control and pelargonidin-treated A549 cells was analyzed using the MTT cytotoxicity assay at different time periods.
The transcription factor, activating enhancer‑binding protein 4 (TFAP4), acts as a key modulator of translation regulatory long non‑coding RNA 1(TRERNA1), which has been proven to promote the invasion and metastasis of gastric cancer (GC) cells.