Browse Course Material
Course info.
- Dr. Peter Shanahan
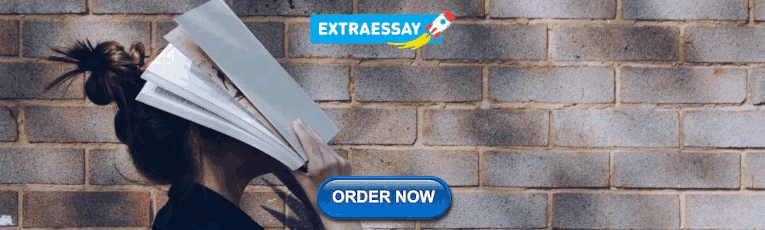
Departments
- Civil and Environmental Engineering
As Taught In
- Aquatic Sciences and Water Quality Control
- Hydrology and Water Resource Systems
Learning Resource Types
Water and wastewater treatment engineering, l02_water_qualit.pdf.
This resource includes lecture handouts of the Instructor on the topic: Why Treat Water and Wastewater? Water Quality Parameters and Standards.

You are leaving MIT OpenCourseWare
Advertisement
Assessment of Drinking Water Quality Using Water Quality Index: A Review
- Review Paper
- Published: 30 January 2023
- Volume 8 , article number 6 , ( 2023 )
Cite this article
- Atanu Manna 1 &
- Debasish Biswas ORCID: orcid.org/0000-0001-8747-0934 2
829 Accesses
9 Citations
Explore all metrics
Nowadays, declining water quality is a significant concern for the world because of rapid population growth, agricultural and industrial activity enhancement, global warming, and climate change influencing hydrological cycles. Assessing water quality becomes necessary by using a suitable method to reduce the risk of geochemical contaminants. Water’s physical and chemical properties are compared to a standard guideline to determine its quality. The water quality index (WQI) model is a commonly helpful technique for evaluating surface and groundwater quality. The model mainly employs aggregation techniques to diminish large amounts of data to a sole value. The WQI model has been used across the globe to assess ground and surface water using regional standards. The model has become popular for its ease of use and general structure. Typically, WQI models include five stages: (1) choosing water quality indicators, (2) generating sub-parameters for each variable, (3) calculating variable weighting numbers, (4) aggregating sub-parameters to finding the total WQI value, and (5) classification of WQI value to highlight the category of water quality. In addition, the model creates ambiguity when converting vast volumes of data into a single value. The study considered 2011–2021 blinded peer-reviewed articles and book chapters to assess WQI models and their application in evaluating drinking water quality. This study mainly concentrated on the comparison of WQI models and their applications. The study also focused on the selection of parameters and problems associated with the accuracy of the models.
This is a preview of subscription content, log in via an institution to check access.
Access this article
Price excludes VAT (USA) Tax calculation will be finalised during checkout.
Instant access to the full article PDF.
Rent this article via DeepDyve
Institutional subscriptions
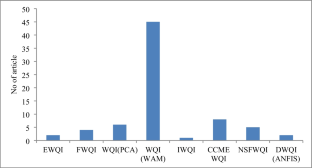
Source: The Author
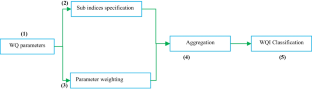
Similar content being viewed by others
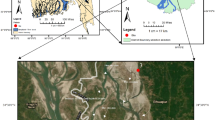
Evaluation of the surface water quality using global water quality index (WQI) models: perspective of river water pollution
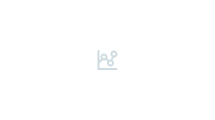
Water quality indices: challenges and applications—an overview
Water Indices: Specification, Criteria, and Applications—A Case Study
Data availability.
It is a review-based article and the gathered secondary information is highlighted in the supplementary file.
Damo R, Icka P (2013) Evaluation of water quality index for drinking water. Pol J Environ Stud 22(4):1045–1051. https://www.researchgate.net/profile/Pirro-Icka/publication/287957321_Evaluation_of_Water_Quality_Index_for_Drinking_Water/links/5923fd63aca27295a8aad7c1/Evaluation-of-Water-Quality-Index-for-Drinking-Water.pdf . Accessed 6 Nov 2021
Uddin MG, Nash S, Olbert AI (2021) A review of water quality index models and their use for assessing surface water quality. Ecol Indic 122:107218. https://doi.org/10.1016/j.ecolind.2020.107218
Article CAS Google Scholar
Uddin MG, Moniruzzaman M, Quader MA, Hasan MA (2018) Spatial variability in the distribution of trace metals in groundwater around the Rooppur nuclear power plant in Ishwardi, Bangladesh. Groundw Sustain Dev 7:220–231. https://doi.org/10.1016/j.gsd.2018.06.002
Article Google Scholar
Egbueri JC, Ameh PD, Unigwe CO (2020) Integrating entropy-weighted water quality index and multiple pollution indices towards a better understanding of drinking water quality in Ojoto area, SE Nigeria. Sci African 10:e00644. https://doi.org/10.1016/j.sciaf.2020.e00644
Ortega DJP, Pérez DA, Américo JHP, de Carvalho SL, Segovia JA (2016) Development of index of resilience for surface water in watersheds. J Urban Environ Eng 10(1):72–82. https://doi.org/10.4090/juee.2016.v10n1.007282
Alcamo J (2019) Water quality and its interlinkages with the sustainable development goals. Curr Opin Environ Sustain 36:126–140. https://doi.org/10.1016/j.cosust.2018.11.005
Li P, Wu J (2019) Drinking water quality and public health. Exposure and Health 11(2):73–79. https://doi.org/10.1007/s12403-019-00299-8
Guo X, Zhang XX, Yue HC (2018) Evaluation of hierarchically weighted principal component analysis for water quality management at Jiaozuo mine. Int Biodeterior Biodegradation 128:182–185. https://doi.org/10.1016/j.ibiod.2017.11.012
Ighalo JO, Adeniyi AG (2020) A comprehensive review of water quality monitoring and assessment in Nigeria. Chemosphere, 127569. https://doi.org/10.30564/jees.v3i1.2900
Motlagh AM, Yang Z, Saba H (2020) Groundwater quality. Water Environ Res 92(10):1649–1658. https://doi.org/10.1002/wer.1412
Zhang Q, Xu P, Qian H (2020) Groundwater quality assessment using improved water quality index (WQI) and human health risk (HHR) evaluation in a semi-arid region of northwest China. Exposure and Health 12(3):487–500. https://doi.org/10.1007/s12403-020-00345-w
Egbueri JC, Ezugwu CK, Ameh PD, Unigwe CO, Ayejoto DA (2020) Appraising drinking water quality in Ikem rural area (Nigeria) based on chemometrics and multiple indexical methods. Environ Monit Assess 192(5). https://doi.org/10.1007/s10661-020-08277-3
Fu B, Merritt WS, Croke BFW, Weber TR, Jakeman AJ (2019) A review of catchment-scale water quality and erosion models and a synthesis of future prospects. Environ Model Softw 114:75–97. https://doi.org/10.1016/j.envsoft.2018.12.008
Hui T, Xiujuan L, Qifa S, Qiang L, Zhuang K, Yan G (2020) Evaluation of drinking water quality using the water quality index (WQI), the synthetic pollution index (SPI) and geospatial tools in Lianhuashan District, China. Pol J Environ Stud 30(1):141–153. https://doi.org/10.15244/pjoes/120765
Lumb A, Sharma TC, Bibeault J-F (2011) A review of genesis and evolution of water quality index (WQI) and some future directions. Water Qual Expo Health 3(1):11–24. https://doi.org/10.1007/s12403-011-0040-0
Solangi GS, Siyal AA, Babar MM, Siyal P (2019a) Application of water quality index, synthetic pollution index, and geospatial tools for the assessment of drinking water quality in the Indus Delta, Pakistan. Environ Monit Assess, 191(12). https://doi.org/10.1007/s10661-019-7861-x
Abba SI, Hadi SJ, Sammen SS, Salih SQ, Abdulkadir RA, Pham QB, Yaseen ZM (2020) Evolutionary computational intelligence algorithm coupled with self-tuning predictive model for water quality index determination. J Hydrol 587:124974. https://doi.org/10.1016/j.jhydrol.2020.124974
Singh B, Sihag P, Singh VP, Sepahvand A, Singh K (2021) Soft computing technique-based prediction of water quality index. Water Supply. https://doi.org/10.2166/ws.2021.157
Tung TM, Yaseen ZM (2021) Deep learning for prediction of water quality index classification: tropical catchment environmental assessment. Nat Resour Res 30:4235–4254. https://doi.org/10.1007/s11053-021-09922-5
Banda TD, Kumarasamy M (2020) Development of a universal water quality index (UWQI) for South African river catchments. Water 12(6):1534. https://doi.org/10.3390/W12061534
Mamun M, An KG (2021) Application of multivariate statistical techniques and water quality index for the assessment of water quality and apportionment of pollution sources in the Yeongsan river, South Korea. Int J Environ Res Public Health 18(16):8268. https://doi.org/10.3390/ijerph18168268
Tripathi M, Singal SK (2019) Use of principal component analysis for parameter selection for development of a novel water quality index: a case study of river Ganga India. Ecol Ind 96:430–436. https://doi.org/10.1016/j.ecolind.2018.09.025
Abbasnia A, Yousefi N, Mahvi AH, Nabizadeh R, Radfard M, Yousefi M, Alimohammadi M (2019) Evaluation of groundwater quality using water quality index and its suitability for assessing water for drinking and irrigation purposes: case study of Sistan and Baluchistan province (Iran). Hum Ecol Risk Assess Int J 25(4):988–1005. https://doi.org/10.1080/10807039.2018.1458596
Jha MK, Shekhar A, Jenifer MA (2020) Assessing groundwater quality for drinking water supply using hybrid fuzzy-GIS-based water quality index. Water Res 179:115867. https://doi.org/10.1016/j.watres.2020.115867
Mukate S, Wagh V, Panaskar D, Jacobs JA, Sawant A (2019) Development of new integrated water quality index (IWQI) model to evaluate the drinking suitability of water. Ecol Ind 101:348–354. https://doi.org/10.1016/j.ecolind.2019.01.034
Al-Hamdany NAS, Al-Shaker YMS, Al-Saffawi AYT (2020) Water quality assessment using the NSFWQI model for drinking and domestic purposes: a case study of groundwater on the left side of Mosul city, Iraq. Plant Archives 20(1):3079–3085. http://www.plantarchives.org/20-1/3079-3085(6183).pdf . Accessed 6 Nov 2021
Bouteraa O, Mebarki A, Bouaicha F, Nouaceur Z, Laignel B (2019) Groundwater quality assessment using multivariate analysis, geostatistical modeling, and water quality index (WQI): a case of study in the Boumerzoug-El Khroub valley of Northeast Algeria. Acta Geochimica 38(6):796–814. https://doi.org/10.1007/s11631-019-00329-x
RadFard M, Seif M, GhazizadehHashemi AH, Zarei A, Saghi MH, Shalyari N, Morovati R, Heidarinejad Z, Samaei MR (2019) Protocol for the estimation of drinking water quality index (DWQI)in water resources: artificial neural network (ANFIS)and Arc-Gis. MethodsX 6:1021–1029. https://doi.org/10.1016/j.mex.2019.04.027
Solangi GS, Siyal AA, Babar MM, Siyal P (2019) Evaluation of drinking water quality using the water quality index (WQI), the synthetic pollution index (SPI) and geospatial tools in Thatta district, Pakistan. Desalination Water Treat 160:202–213. https://doi.org/10.5004/dwt.2019.24241
Solangi GS, Siyal AA, Babar MM, Siyal P (2020) Groundwater quality evaluation using the water quality index (WQI), the synthetic pollution index (SPI), and geospatial tools: a case study of Sujawal district, Pakistan. Human Ecol Risk Assess 26(6):1529–1549. https://doi.org/10.1080/10807039.2019.1588099
Tiwari AK, Singh AK, Mahato MK (2018) Assessment of groundwater quality of Pratapgarh district in India for suitability of drinking purpose using water quality index (WQI) and GIS technique. Sustain Water Resour Manag 4(3):601–616. https://doi.org/10.1007/s40899-017-0144-1
Tiwari S, Babbar R, Kaur G (2018). Performance evaluation of two ANFIS models for predicting water quality Index of River Satluj (India). Adv Civil Eng 2018. https://doi.org/10.1155/2018/8971079
Seifi A, Dehghani M, Singh VP (2020) Uncertainty analysis of water quality index (WQI) for groundwater quality evaluation: application of Monte-Carlo method for weight allocation. Ecol Indic 117:106653. https://doi.org/10.1016/j.ecolind.2020.106653
Dutta N, Thakur BK, Nurujjaman M, Debnath K, Bal DP (2022) An assessment of the water quality index (WQI) of drinking water in the Eastern Himalayas of South Sikkim India. Groundw Sustain Dev 17:100735. https://doi.org/10.1016/j.gsd.2022.100735
Verma P, Singh PK, Sinha RR, Tiwari AK (2020) Assessment of groundwater quality status by using water quality index (WQI) and geographic information system (GIS) approaches: a case study of the Bokaro district, India. Appl Water Sci 10(1):1–16. https://doi.org/10.1007/s13201-019-1088-4
Gorai AK, Hasni SA, Iqbal J (2016) Prediction of ground water quality index to assess suitability for drinking purposes using fuzzy rule-based approach. Appl Water Sci 6(4):393–405. https://doi.org/10.1007/s13201-014-0241-3
Akhtar N, Ishak MIS, Ahmad MI, Umar K, MdYusuff MS, Anees MT, Qadir A, Ali Almanasir YK (2021) Modification of the water quality index (WQI) process for simple calculation using the multi-criteria decision-making (MCDM) method: a review. Water 13(7):905. https://doi.org/10.3390/w13070905
Aljanabi ZZ, Al-Obaidy A-HMJ, Hassan FM (2021) A brief review of water quality indices and their applications. IOP Conf Ser: Earth Environ Sci 779(1):12088. https://doi.org/10.1088/1755-1315/779/1/012088
Soumaila KI, Niandou AS, Naimi M, Mohamed C, Schimmel K, Luster-Teasley S, Sheick NN (2019) A systematic review and meta-analysis of water quality indices. J Agric Sci Technol B B(9):1–14. https://doi.org/10.17265/2161-6264/2019.01.001
Abed BS, Farhan A-R, Ismail AH, Al Aani S (2021) Water quality index toward a reliable assessment for water supply uses: a novel approach. Int J Environ Sci Technol, 1–14. https://doi.org/10.1007/s13762-021-03338-7
Wagh VM, Mukate SV, Panaskar DB, Muley AA, Sahu UL (2019) Study of groundwater hydrochemistry and drinking suitability through Water Quality Index (WQI) modelling in Kadava river basin, India. SN Applied Sciences 1(10):1–16. https://doi.org/10.1007/s42452-019-1268-8
Gupta S, Gupta SK (2021) A critical review on water quality index tool: genesis, evolution and future directions. Eco Inform 63:101–299. https://doi.org/10.1016/j.ecoinf.2021.101299
Hui T, Jizhong D, Qifa S, Yan G, Zhuang K, Hongtao J (2021) Evaluation of shallow groundwater for drinking purpose based on water quality index and synthetic pollution index in Changchun New District, China. Environ Forensic 22(1–2):189–204. https://doi.org/10.1080/15275922.2020.1834024
Ewaid SH, Abed SA, Al-Ansari N, Salih RM (2020) Development and evaluation of a water quality index for the Iraqi rivers. Hydrology 7(3):67. https://doi.org/10.3390/HYDROLOGY7030067
Ukah BU, Ameh PD, Egbueri JC, Unigwe CO, Ubido OE (2020) Impact of effluent-derived heavy metals on the groundwater quality in Ajao industrial area, Nigeria: an assessment using entropy water quality index (EWQI). Int J Energy Water Resour, 1–14. https://doi.org/10.1007/s42108-020-00058-5
Tiri A, Belkhiri L, Mouni L (2018) Evaluation of surface water quality for drinking purposes using fuzzy inference system. Groundw Sustain Dev 6:235–244. https://doi.org/10.1016/j.gsd.2018.01.006
Alver A (2019) Evaluation of conventional drinking water treatment plant efficiency according to water quality index and health risk assessment. Environ Sci Pollut Res 26(26):27225–27238. https://doi.org/10.1007/s11356-019-05801-y
Saw S, Mahato JK, Singh PK (2021). Suitability Evaluation of CCME-WQI and GWQI for the modeling of groundwater and human health risk assessment of heavy metals - Eastern India. Res Square, 0–24. https://doi.org/10.21203/rs.3.rs-1000020/v1
Patil VBB, Pinto SM, Govindaraju T, Hebbalu VS, Bhat V, Kannanur LN (2020) Multivariate statistics and water quality index (WQI) approach for geochemical assessment of groundwater quality—a case study of Kanavi Halla Sub-Basin, Belagavi, India. Environ Geochem Health 42(9):2667–2684. https://doi.org/10.1007/s10653-019-00500-6
Nath BK, Chaliha C, Bhuyan B, Kalita E, Baruah DC, Bhagabati AK (2018) GIS mapping-based impact assessment of groundwater contamination by arsenic and other heavy metal contaminants in the Brahmaputra River valley: a water quality assessment study. J Clean Prod 201:1001–1011. https://doi.org/10.1007/s10462-021-10007-1
Sutadian AD, Muttil N, Yilmaz AG, Perera BJC (2016) Development of river water quality indices—a review. Environ Monit Assess 188(1):58. https://doi.org/10.1007/s10661-015-5050-0
Swamee PK, Tyagi A (2007) Improved method for aggregation of water quality subindices. J Environ Eng 133(2):220–225. https://doi.org/10.1061/(ASCE)0733-9372(2007)133:2(220)
Uddin G, Nash S, Olbert AI (2022) Optimisation of parameters in a water quality index model using principal component analysis. Proc 39th IAHR World Congr 19:5739–5744. https://doi.org/10.3850/IAHR-39WC2521711920221326
Uddin MG, Nash S, Rahman A, Olbert AI (2022) A comprehensive method for improvement of water quality index (WQI) models for coastal water quality assessment. Water Research 219:118532. https://doi.org/10.1016/j.watres.2022.118532
Uddin MG, Nash S, Rahman A, Olbert AI (2023) Assessing optimisation techniques for improving water quality model. J Clean Prod 385:135671. https://doi.org/10.1016/j.jclepro.2022.135671
Uddin MG, Nash S, Rahman A, Olbert AI (2023) Performance analysis of the water quality index model for predicting water state using machine learning techniques. Process Saf Environ Prot 169:808–828. https://doi.org/10.1016/j.psep.2022.11.073
Uddin MG, Nash S, Rahman A, Olbert AI (2023) A novel approach for estimating and predicting uncertainty in water quality index model using machine learning approaches. Water Res 229:119422. https://doi.org/10.1016/j.watres.2022.119422
Islam M, Mostafa MG (2021) Development of an integrated irrigation water quality index (IIWQIndex) model. Water Supply 22(2):2322–2337. https://doi.org/10.2166/ws.2021.378
Wagh VM, Panaskar DB, Muley AA, Mukate SV (2017) Groundwater suitability evaluation by CCME WQI model for Kadava River Basin, Nashik, Maharashtra, India. Model Earth Syst Environ 3(2):557–565. https://doi.org/10.1007/s40808-017-0316-x
Elbeltagi A, Pande CB, Kouadri S, Islam ARM (2022) Applications of various data-driven models for the prediction of groundwater quality index in the Akot basin, Maharashtra India. Environ Sci Pollut Res 29(12):17591–17605. https://doi.org/10.1007/s11356-021-17064-7
Hossain M, Patra PK (2020) Water pollution index–a new integrated approach to rank water quality. Ecol Indic 117:106668. https://doi.org/10.1016/j.ecolind.2020.106668
Barbosa Filho J, de Oliveira IB (2021) Development of a groundwater quality index: GWQI, for the aquifers of the state of Bahia, Brazil using multivariable analyses. Sci Rep 11(1):1–22. https://doi.org/10.1038/s41598-021-95912-9
Gradilla-Hernández MS, de Anda J, Garcia-Gonzalez A, Montes CY, Barrios-Piña H, Ruiz-Palomino P, Díaz-Vázquez D (2020) Assessment of the water quality of a subtropical lake using the NSF-WQI and a newly proposed ecosystem specific water quality index. Environ Monit Assess 192(5):1–19. https://doi.org/10.1007/s10661-020-08265-7
Najafzadeh M, Homaei F, Farhadi H (2021) Reliability assessment of water quality index based on guidelines of national sanitation foundation in natural streams: integration of remote sensing and data-driven models. Artif Intell Rev 56(4):4619–4651. https://doi.org/10.1007/s10462-021-10007-1
Zotou I, Tsihrintzis VA, Gikas GD (2019) Performance of seven water quality indices (WQIs) in a Mediterranean River. Environ Monit Assess 191(8):1–14
Banda, Kumarasamy MA (2020) Review of the existing water quality indices (WQIs). J Phys Opt 39(2):1–19. https://www.researchgate.net/profile/Talent-Banda/publication/343430598_A_Review_of_the_Existing_Water_Quality_Indices_WQIs/links/5f299e76299bf13404a22edc/A-Review-of-the-Existing-Water-Quality-Indices-WQIs.pdf . Accessed 11 Nov 2021
Kamboj V, Kamboj N, Bisht A (2020) An overview of water quality indices as promising tools for assessing the quality of water resources. Agro Environ Media - Agric Environ Sci Acad pp. 188–214. https://doi.org/10.26832/aesa-2020-aepm-013
Haider H, Ghumman AR, Al-Salamah IS, Thabit H (2020) Assessment framework for natural groundwater contamination in arid regions: development of indices and wells ranking system using fuzzy VIKOR method. Water 12(2):423. https://doi.org/10.3390/w12020423
Pak HY, Chuah CJ, Tan ML, Yong EL, Snyder SA (2021) A framework for assessing the adequacy of water quality index–quantifying parameter sensitivity and uncertainties in missing values distribution. Sci Total Environ 751(10):141982. https://doi.org/10.1016/j.scitotenv.2020.141982
Smith DG (1990) A better water quality indexing system for rivers and streams. Water Res 24(10):1237–1244. https://doi.org/10.1016/0043-1354(90)90047-A
Shah KA, Joshi GS (2017) Evaluation of water quality index for River Sabarmati, Gujarat, India. Appl Water Sci 7(3):1349–1358. https://doi.org/10.1007/s13201-015-0318-7
Said A, Stevens DK, Sehlke G (2004) An innovative index for evaluating water quality in streams. Environ Manage 34(3):406–414. https://doi.org/10.1007/s00267-004-0210-y
Adimalla N, Qian H, Li P (2019) Entropy water quality index and probabilistic health risk assessment from geochemistry of groundwaters in hard rock terrain of Nanganur County, South India. Geochemistry 80(4):125544. https://doi.org/10.1016/j.chemer.2019.125544
Long Y, Yang Y, Lei X, Tian Y, Li Y (2019) Integrated assessment method of emergency plan for sudden water pollution accidents based on improved TOPSIS, Shannon entropy and a coordinated development degree model. Sustainability 11(2):510. https://doi.org/10.1007/s12403-019-00299-8
Yang Z, Wang Y (2020) The cloud model based stochastic multi-criteria decision making technology for river health assessment under multiple uncertainties. J Hydrol 581:124437. https://doi.org/10.1016/j.jhydrol.2019.124437
Hanoon MS, Ahmed AN, Fai CM, Birima AH, Razzaq A, Sherif M, Sefelnasr A, El-Shafie A (2021) Application of artificial intelligence models for modeling water quality in groundwater: comprehensive review, evaluation and future trends. Water Air Soil Pollut 232(10):1–41. https://doi.org/10.1007/s11270-021-05311-z
Chanapathi T, Thatikonda S (2019) Fuzzy-based regional water quality index for surface water quality assessment. J Hazard Toxic Radioact Waste 23(4):4019010. https://doi.org/10.1061/(asce)hz.2153-5515.0000443
Hamdan ANA, Al Saad ZAA, Abu-Alhail S (2021) Fuzzy system modelling to assess water quality for irrigation purposes. J Water Land Dev 50:98–107. https://doi.org/10.24425/jwld.2021.138165
Hue NH, Thanh NH (2020) Surface water quality analysis using Fuzzy logic approach: a case of inter-provincial irrigation network in Vietnam. IOP Conf Ser: Earth Environ Sci 527(1):12017. https://doi.org/10.1088/1755-1315/527/1/012017
Lindang HU, Tarmudi ZH, Jawan A (2017) Assessing water quality index in river basin: Fuzzy inference system approach. Malays J Geosci 1(1):27–31. https://doi.org/10.1007/s12403-019-00299-8
Sharifi H, Roozbahani A, Shahdany SMH (2021) Evaluating the performance of agricultural water distribution systems using FIS, ANN and ANFIS intelligent models. Water Resour Manage 35(6):1797–1816
Selvaraj A, Saravanan S, Jennifer JJ (2020) Mamdani fuzzy based decision support system for prediction of groundwater quality: an application of soft computing in water resources. Environ Sci Pollut Res 27(20):25535–25552. https://doi.org/10.1007/s11356-020-08803-3
Hajji S, Yahyaoui N, Bousnina S, Ben Brahim F, Allouche N, Faiedh H, Bouri S, Hachicha W, Aljuaid AM (2021) Using a Mamdani Fuzzy Inference System Model (MFISM) for ranking groundwater quality in an agri-environmental context: case of the Hammamet-Nabeul shallow aquifer (Tunisia). Water 13(18):2507. https://doi.org/10.3390/w13182507
Kambalimath S, Deka PC (2020) A basic review of fuzzy logic applications in hydrology and water resources. Appl Water Sci 10(8):191. https://doi.org/10.1007/s13201-020-01276-2
Islam AR, Al Mamun A, Rahman MM, Zahid A (2020) Simultaneous comparison of modified-integrated water quality and entropy weighted indices: implication for safe drinking water in the coastal region of Bangladesh. Ecol Indic 113:106229. https://doi.org/10.1016/j.ecolind.2020.106229
Rabeiy RES (2018) Assessment and modeling of groundwater quality using WQI and GIS in Upper Egypt area. Environ Sci Pollut Res 25(31):30808–30817. https://doi.org/10.1007/s11356-017-8617-1
Uddin MG, Olbert AI, Nash S (2020) Assessment of water quality using water quality index (WQI). CERI 2020 Proc 85:966–982
Google Scholar
Talat RA, Al-Assaf AYR, Al-Saffawi AYT (2019) Valuation of water quality for drinking and domestic purposes using WQI : a case study for groundwater of Al-Gameaa and Al-Zeraee quarters in Mosul city/Iraq. J Phys: Conf Ser 1294(7). https://doi.org/10.1088/1742-6596/1294/7/072011
Kachroud M, Trolard F, Kefi M, Jebari S, Bourrié G (2019) Water quality indices: challenges and application limits in the literature. Water 11(2):361. https://doi.org/10.3390/w11020361
Fathi E, Zamani-Ahmadmahmoodi R, Zare-Bidaki R (2018) Water quality evaluation using water quality index and multivariate methods, Beheshtabad River, Iran. Appl Water Sci 8(7):1–6. https://doi.org/10.1007/s13201-018-0859-7
Feng Y, Bao Q, Chenglin L, Bowen W, Zhang Y (2018) Introducing biological indicators into CCME WQI using variable fuzzy set method. Water Resour Manage 32(8):2901–2915. https://doi.org/10.1007/s13201-018-0859-7
Gikas GD, Sylaios GK, Tsihrintzis VA, Konstantinou IK, Albanis T, Boskidis I (2020) Comparative evaluation of river chemical status based on WFD methodology and CCME water quality index. Sci Total Environ 745:140849. https://doi.org/10.1016/j.scitotenv.2020.140849
Ott WR (1978) Environmental indices: theory and practice. Ann Arbor Science Publishers, Inc., Ann Arbor, MI. https://www.osti.gov/biblio/6681348 . Accessed 13 Nov 2021
Ismail AH, Robescu D (2019) Assessment of water quality of the Danube river using water quality indices technique. Environ Eng Manag J 18(8):1727–1737. https://doi.org/10.30638/eemj.2019.163
Khan Y, Chai SS (2017) Ensemble of ANN and ANFIS for water quality prediction and analysis-a data driven approach. J Telecommun Electron Comput Eng (JTEC) 9(29):117–122
CAS Google Scholar
Patki VK, Jahagirdar S, Patil YM, Karale R, Nadagouda A (2021). Prediction of water quality in municipal distribution system. Materials Today: Proceedings. https://doi.org/10.1016/j.matpr.2021.02.826
Volodina V, Challenor P (2021) The importance of uncertainty quantification in model reproducibility. Phil Trans R Soc A 379(2197):20200071. https://doi.org/10.1098/rsta.2020.0071
Rodrigues C, Cunha MÂ (2017) Assessment of the microbiological quality of recreational waters: indicators and methods. Euro-Mediterr J Environ Integr 2(1):1–18. https://doi.org/10.1007/s41207-017-0035-8
Heiß L, Bouchaou L, Tadoumant S, Reichert B (2020) Index-based groundwater vulnerability and water quality assessment in the arid region of Tata city (Morocco). Groundw Sustain Dev 10:100344. https://doi.org/10.1016/j.gsd.2020.100344
Download references
Acknowledgements
The authors are thankful to Vidyasagar University for providing good research environment.
The authors state that they did not receive any funding, grants, or other forms of support in the development of this paper.
Author information
Authors and affiliations.
Centre for Environmental Studies, Vidyasagar University, Midnapore, 721102, India
Atanu Manna
Department of Business Administration, Vidyasagar University, Midnapore, West Bengal, 721102, India
Debasish Biswas
You can also search for this author in PubMed Google Scholar
Contributions
The study’s conception and design were aided by all of the authors. The first author [Atanu Manna] conducted the literature search and data presentation in this review article, which was drafted and critically revised by the corresponding author [Dr. Debasish Biswas].
Corresponding author
Correspondence to Debasish Biswas .
Ethics declarations
Competing interests.
The authors declare no competing interests.
Ethical Approval and Consent to Participate
The authors strictly adhere to all ethical considerations during the literature search and data presentation to conduct this study.
Consent for Publication
Not applicable.
Competing Interest
Additional information, publisher's note.
Springer Nature remains neutral with regard to jurisdictional claims in published maps and institutional affiliations.
Rights and permissions
Springer Nature or its licensor (e.g. a society or other partner) holds exclusive rights to this article under a publishing agreement with the author(s) or other rightsholder(s); author self-archiving of the accepted manuscript version of this article is solely governed by the terms of such publishing agreement and applicable law.
Reprints and permissions
About this article
Manna, A., Biswas, D. Assessment of Drinking Water Quality Using Water Quality Index: A Review. Water Conserv Sci Eng 8 , 6 (2023). https://doi.org/10.1007/s41101-023-00185-0
Download citation
Received : 22 September 2022
Revised : 08 January 2023
Accepted : 22 January 2023
Published : 30 January 2023
DOI : https://doi.org/10.1007/s41101-023-00185-0
Share this article
Anyone you share the following link with will be able to read this content:
Sorry, a shareable link is not currently available for this article.
Provided by the Springer Nature SharedIt content-sharing initiative
- Water quality index
- Water quality parameters
- Model accuracy
- Aggregation method
- Find a journal
- Publish with us
- Track your research

An official website of the United States government
Here’s how you know
Official websites use .gov A .gov website belongs to an official government organization in the United States.
Secure .gov websites use HTTPS A lock ( Lock A locked padlock ) or https:// means you’ve safely connected to the .gov website. Share sensitive information only on official, secure websites.
JavaScript appears to be disabled on this computer. Please click here to see any active alerts .
Factsheets on Water Quality Parameters
The water quality parameter factsheets were developed to provide an introduction to monitoring common parameters; Temperature, Dissolved Oxygen, pH, Turbidity, Macroinvertebrates, E. coli, Nutrients, Habitat Assessment and Metals. They are particularly useful for training new water quality monitoring staff and explaining water quality sampling to outside partners. References can be found in the last factsheet for more detailed information on each parameter.
- Temperature Parameter Factsheet
- Dissolved Oxygen Parameter Factsheet
- pH Parameter Factsheet
- Turbidity Parameter Factsheet
- Macroinvertebrates Parameter Factsheet
- E. coli Parameter Factsheet
- Nutrients Parameter Factsheet
- Habitat Assessment Parameter Factsheet
- Metals Parameter Factsheet
- References for Parameter Factsheets
- Ambient Water Monitoring and Assessment Home
- Tribal Section 106
- Clean Water Act Section 303(d): Impaired Waters and Total Maximum Daily Loads (TMDLs)
- Consolidated Assessment and Listing Methodology and Appendices
- Information on Bioassessment and Biocriteria Programs for Streams and Wadeable Rivers
- Tribal Assessment Modules
- Water Quality Criteria
- Water Quality Standards
- How's My Waterway
- Integrated Reporting Guidance
- National Water Quality Inventory Report to Congress
- Report on the Environment
- Design Tool
- Lake Context Tool
- Water Quality Parameter Factsheets
- Quality Assurance Project Plan Development Tool
- Template for Developing Quality Assurance Project Plans for Participatory Science Projects
- National Coastal Condition Assessment Dashboard
- National Lakes Assessment Dashboard
- National Rivers and Streams Assessment Dashboard
- National Wetlands Condition Assessment Dashboard
- Justice, Equity, Diversity and Inclusion Committee
- Methods and Data Comparability Board
- National Environmental Methods Index
- Volunteer Monitoring Committee
- Water Quality Portal
- Participatory Science
Thank you for visiting nature.com. You are using a browser version with limited support for CSS. To obtain the best experience, we recommend you use a more up to date browser (or turn off compatibility mode in Internet Explorer). In the meantime, to ensure continued support, we are displaying the site without styles and JavaScript.
- View all journals
- My Account Login
- Explore content
- About the journal
- Publish with us
- Sign up for alerts
- Open access
- Published: 05 March 2024
Groundwater quality assessment using water quality index and principal component analysis in the Achnera block, Agra district, Uttar Pradesh, Northern India
- Shahjad Ali 1 ,
- Sitaram Verma 2 ,
- Manish Baboo Agarwal 1 ,
- Raisul Islam 3 ,
- Manu Mehrotra 1 ,
- Rajesh Kumar Deolia 4 ,
- Jitendra Kumar 5 ,
- Shailendra Singh 6 ,
- Ali Akbar Mohammadi 7 ,
- Deep Raj 8 ,
- Manoj Kumar Gupta 9 ,
- Phuyen Dang 10 , 11 &
- Mehdi Fattahi 10 , 11
Scientific Reports volume 14 , Article number: 5381 ( 2024 ) Cite this article
2659 Accesses
1 Citations
Metrics details
- Environmental chemistry
- Environmental monitoring
The qualitative and quantitative assessment of groundwater is one of the important aspects for determining the suitability of potable water. Therefore, the present study has been performed to evaluate the groundwater quality for Achhnera block in the city of Taj, Agra, India, where groundwater is an important water resource. The groundwater samples, 50 in number were collected and analyzed for major ions along with some important trace element. This study has further investigated for the applicability of groundwater quality index (GWQI), and the principal component analysis (PCA) to mark out the major geochemical solutes responsible for origin and release of geochemical solutes into the groundwater. The results confirm that, majority of the collected groundwater samples were alkaline in nature. The variation of concentration of anions in collected groundwater samples were varied in the sequence as, HCO 3− > Cl − > SO4 2− > F − while in contrast the sequence of cations in the groundwater as Na > Ca > Mg > K. The Piper diagram demonstrated the major hydro chemical facies which were found in groundwater (sodium bicarbonate or calcium chloride type). The plot of Schoellar diagram reconfirmed that the major cations were Na + and Ca 2+ ions, while in contrast; major anions were bicarbonates and chloride. The results showed water quality index mostly ranged between 105 and 185, hence, the study area fell in the category of unsuitable for drinking purpose category. The PCA showed pH, Na + , Ca 2+ , HCO 3− and fluoride with strong loading, which pointed out geogenic source of fluoride contamination. Therefore, it was inferred that the groundwater of the contaminated areas must be treated and made potable before consumption. The outcomes of the present study will be helpful for the regulatory boards and policymaker for defining the actual impact and remediation goal.
Similar content being viewed by others
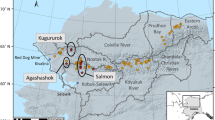
Metal mobilization from thawing permafrost to aquatic ecosystems is driving rusting of Arctic streams
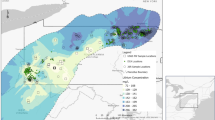
Estimates of lithium mass yields from produced water sourced from the Devonian-aged Marcellus Shale

Globally elevated greenhouse gas emissions from polluted urban rivers
Introduction.
Quality of life is associated with quality of water we consume. Out of all water resource, groundwater is one of the important drinking water resources 1 , 2 . In the arid and semi-arid regions, especially for the developing countries like India and Bangladesh, the rapid population growth associated with intensive developmental activities results in a severe increase in water demand 1 , 2 , 3 . The day-to-day degradation of groundwater quality has now become one of the serious challenges in the world. Billions of people across the globe are compelled to consume the polluted water due to the scarcity of potable water, and therefore the scarcity of groundwater is an alarming threat to the humans. It has now been well established that groundwater is at higher risk, in terms of its purity 3 , 4 , 5 , 6 . In remote areas, the situation of groundwater is even more miserable, due to over withdrawal of groundwater. The residents of urban areas have to walk several kilometers to fetch potable water 5 , 6 . The government and various non-governmental organizations (NGOs) are working hard enough to provide contaminants free potable water to every individual 7 . It has been reported in previous literatures that, contaminants like heavy metals, pesticides, organic and inorganic pollutant are causing the serious human health disease such as hypertension, hypocalcaemia, kidney stones, gastro-renal discomfort, arterial calcification, thrombosis 8 , 9 , 10 , 11 , 12 . Apart from the availability of heavy metals in drinking water, the presence of nitrogen has also been proven as the strong potential threat to the quality of drinking water 13 , 14 , 15 . With the increasing groundwater pollution, it is essential to analyze groundwater chemical characteristics and evaluate groundwater quality for water supply purpose. In this regard, methods like groundwater quality index (GWQI), the fuzzy comprehensive method and the health risk weight method (HRWM) have been widely used by researchers. Among these methods, the water quality index (WQI) has been more commonly used by international researcher due to its simple calculation, practicality, and versatile applications 16 , 17 , 18 .
GWQI is a mathematical expression that can be used to determine the quality of groundwater in different locations globally. The idea of GWQI has been kept to assess the water quality throughout diverse world-wide areas 19 , 20 . It is an important tool for the decision-makers to choose the best method for pre-remediation goal 21 , 22 , 23 , 24 . As a result, it has become a crucial component in the evaluation of water quality. In India, multiples research in different areas indicated that the sources of drinking water contain heavy metals like cadmium, lead, mercury, arsenic, and manganese. Also, research findings have shown elevated levels of fluoride, which exceed World Health Organization (WHO), limits of 1.5 ppm 25 , 26 , 27 , 28 , 29 , 30 , 31 . In a study on the Ramganga aquifer of Bareilly District in Uttar Pradesh (India), it was found that the groundwater which were extracted from shallow aquifer contain high percentage of zinc and nickel, whereas the samples collected from deep aquifer consists of heavy metals like copper, cobalt, nickel, manganese, cadmium, and zinc 32 . Previous research revealed that the quality of drinkable water in several regions of northern India is unfit for drinking. About 35 districts are reported to have been found variously affected with arsenic toxicity 31 , 32 , 33 .
Considering the above mention highlights, this study was undertaken to achieve the following objectives: (a) the primary aim of the present research work was to explore the level of contamination in one of the unexplored parts of northern India, which has not been marked before in the previous studies, i.e., the city of Taj Mahal, Agra, India, (b) Further, qualitative of groundwater has been estimated by hydro-chemical analysis and GWQI estimation respectively, (c) to use PCA for the determination of the components that influence the discharge of hydro chemical solutes into the groundwater, (d) to investigate the correlation between hydro chemical parameters and their common source of origin.
Materials and methods
Study area and its geology.
The present study was focused on one of the blocks of city of Taj-Mahal, Agra, India. This city is located on the bank of river Yamuna, Uttar Pradesh, India, between 27°11′ N and 78°02’ E (Fig. 1 ). With a rapid pace of population increase, it is one of biggest city in northern India. There are 15 administrative blocks, 904 villages, and six tehsils in the Agra district. With reference to the 2011 India’s Census, the Agra district has over 7 million households, with a population of 44, 18,797 of which 53.52% are males and 46.48% are females. The weather of the sampled area was semi-arid to sub-tropic type, with an average annual precipitation of 687 mm and evaporation of 1466 mm/year. The average temperature range varies from21.9 to 45 °C in hot days and 3.9–32.2 °C in cold days. Annually, the rainfall averages to about 687.2 mm due to the southwest monsoon, and consequently the daily relative humidity ranges from 30 to 100% 34 .
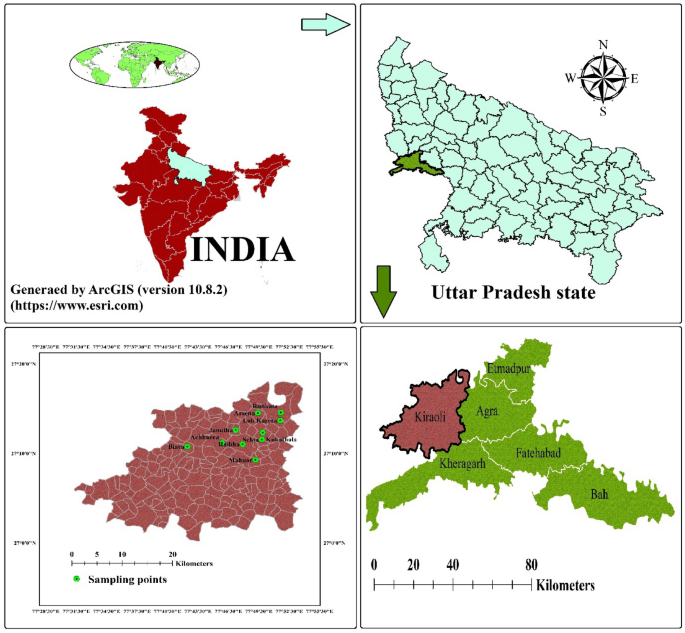
Locations of Achhnera block in Agra, North India.
The study area occupies a part of Indo-Gangetic plain and its major part is underlined by alluvial sediments of quaternary age encompasses primarily a sequence of clay, silt, sand of different grades, gravels and kankar in different magnitude. In this study region, over 90% of the population use groundwater for drinking purposes thus, investigations of quality of groundwater are among the highest priorities. In this study, ArcGIS (version 10.8.2) was used for geographical data processing and visualization. ArcGIS is a product of Esri and more details about the software can be found on their official website ( https://www.esri.com ).
Sample collection and hydro-geochemical analysis
The samples were collected from the selected area via tube wells, hand pumps and wells. All samples were collected in a time interval of one year from February 2022 to January 2023. A total of 50 groundwater samples from 10 villages (5 × 10) were collected and preserved in polypropylene bottles at 4ºC. The sampling locations have been plotted through ArcGIS 10.2 (Fig. 1 ). To stabilize the pH, conductivity and temperature of the sampling area, the hand pumps were used for some time before collection of samples. All chemical used were of analytical grade (Merck Darmstadt, Germany). During the analysis of samples standard methods were used as given in APHA 2012. Concentration of fluoride (F − ), sulphate (SO 4 2− ) and nitrate (NO 3− ) ions were determined by using spectrophotometer. Mohr’s method (AgNO 3 ) was used to determine chloride (Cl − ) content in the samples. Titration and flame photometry method was used to determine hardness, alkalinity, Mg 2+ , Ca 2+ , Na + , and K + ions in the water samples. Total dissolved solids (TDS) and pH were analyzed by multi-parameter kit 35 . The results were counter checked by the calculation of cation and anion balance. The estimated error was less than ± 5% for all the collected samples.
Calculation of the WQI of the samples
The WQI model is an interested tool for assessing groundwater and surface water quality. It uses aggregation techniques that allow conversion of extensive water quality data into a single value or index. Globally, the WQI model has been applied to evaluate water quality according to local criteria. The guidelines laid by WHO for drinking water are illustrated in (Table 1 ).
Calculation of the unit weight (Wn)
The following equations refer to the calculation Wn (Eq. 1 ).
where \(K = \frac{1}{{\sum {Xs} }}\) , Wn: unit weight parameter 36 , 37 ; Xs: suggested standard for parameter, K: Proportionality Constant; n = number of different water quality parameters.
Calculation of groundwater quality rating
Quality rating scale Qn was computed according to WHO guidelines using the relation of Eq. ( 2 ).
Xn—actual concentration of water quality parameters; Xi—ideal value of different water quality parameters (0 for all parameters except pH 7 ppm).
Estimation of Water Quality Index (WQI)
Various researchers have followed the above method to calculate WQI. Generally, the water quality index (WQI) differentiates potable water into different classes, as shown below in Table 2 36 , 38 , 39 , 40 .
Principal component analysis (PCA)
A well-reported statistical approach in ground water research, the principal component analysis. The data clarification is obtained along the hidden factor created by original factors such as water quality indicators by regard at the key source of variance in the data. The hidden parameters from a matrix composed of factor loading (weight of principal variable) and factor score (prediction of sampling location on the principal component axis). The PCA has been executed in this study to determine homologous behavior and common origin of different physicochemical properties of groundwater. Further PCA was carried out to identify the various factors responsible for release of contaminants into groundwater 41 , 42 .
Statistics used and Data analysis
The data was analyzed using SPSS 16.0 (SPSS Inc. Chicago, USA) and Microsoft Excel 2013. Through SPSS 16.0 spearman correlation was calculated to know the inter-relationship between various hydro-chemical solutes 25 . Further Spatial distribution map has been drawn using arc GIS-10.2 by ESR to evaluate the spatial distribution of fluoride from samples collected from different parts of villages.
Result and discussion
Hydrochemistry of groundwater of achhnera block, agra.
The physiochemical properties of groundwater samples have been presented in Table 3 . The alkalinity of the groundwater sample has been found in the range from 187 to 493.8 ppm, with an average value of 343 ppm, which is within the permissible limit of 600 ppm 39 . TDS of the samples was very high from 801 to 2065 ppm with the average value of 1327 ppm, which is higher than prescribed limit 39 . The concentration of chloride ranged from 226 to 814 ppm with the average value of 470 ppm. The concentration of sodium, potassium, sulphate, and nitrate ions were found in the range of 165–680 ppm, 12–67 ppm, 37–114 ppm, and 4.6–11 ppm, respectively. The hardness was observed between the ranges of 155 to 485 ppm with an average value of 320 ppm, and correspondingly, the concentration of calcium and magnesium ions was ranged from 64–160 ppm to 6.8–32 ppm, respectively. The most prominent anion found in underground water samples was HCO 3− , but some samples had Cl − ions as the most prominent anion. Out of all the samples collected, about 50% of them have pH values above the permissible limit of WHO and BIS standards (IS: 10500, 2012) i.e., 6.5–8.5 38 , 39 . The concentration of fluoride in the sampled water was found in the range of 0.910 to 2.46 ppm, with the average value of 1.628 ppm as shown as in Fig. 2 . The result demonstrated that the concentration of fluoride ion was on elevated side, crossing the permissible limit of WHO (1.5 ppm) 38 .
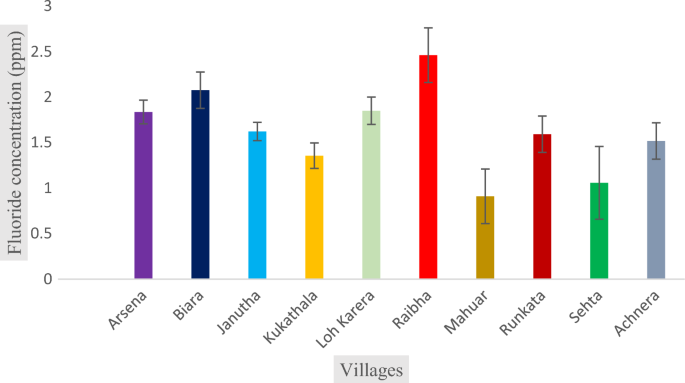
Fluoride concentration in Achhnera block.
In Korea area, Kim et al. studied the co-contamination of arsenic and fluoride in the groundwater of an alluvial aquifer and reported that the concentrations of fluoride ions among the total 50 samples collected, 35 samples have increased level, which indicated that the soil and the rock of that region contain fluoride-rich minerals 43 . In a similar kind of study carried out by Ali et al.2021, also showed the elevated groundwater fluoride in some blocks of the Agra district and it was observed that the concentration of fluoride in the range of0.14 to 4.88 mg/L 3 . Another study carried out by Ansari and Umar (2019), found very much similar results in Unnao, Uttar Pradesh (India), and the concentration of fluoride was reported in the range between 0.06 to 1.83 44 . A very similar study performed by Chaurisiaya et al. (2018) observed the concentration of fluoridebetween0.28 to 2.01 in Varanasi, Uttar Pradesh, India. Similarly, in some other previous research, the concentration of fluoride ions was ranged from 0.32 to 3.5 in Banda, Uttar Pradesh 45 . Tiwari et al. (2016) reported the elevated range of fluoride concentration i.e., between 0.41 and 3.99 in Pratapgarh, Uttar Pradesh, India 46 . Dev and Raju (2014) found the fluoride concentration between0.08 to 6.7 in Sonbadra, Uttar Pradesh 47 . Hence, it may be inferred that the major portion of northern India is endemic to elevated fluoride concentration (Table 4 ).
Geochemical characterization of Achhnera block
For all groundwater samples, the primary dissolved ions were shown through Piper trilinear diagrams and Schoellar diagrams to comprehend the geochemical progression of groundwater. AqQa v1.X, a Rock ware program, was used to plot the diagrams. Separate ternary plots revealed the cations and anions in the piper diagram. Magnesium, calcium, and sodium, potassium was the apex of cation plot while chloride, sulphate, and carbonate, and bicarbonate ions were the apexes of anions plot (Fig. 3 ). The predominant cation present in the samples was sodium. As a result, the water quality of Achhnera region was classified as either Na + /HCO 3− or Na + /Cl − type, and Ca 2+/ HCO 3− type. When fluorite get dissolves in water containing sodium bicarbonate, there is often a moderate correlation between increased fluoride levels due to the presence of bicarbonates 48 . Ionic components of groundwater samples have been displayed in Schoellar diagram (Fig. 4 ). The primary ionic components of groundwater are SO 4 2− , HCO 3− , Cl − , Mg 2+ , Ca 2+ , Na + , and K + , and their concentrations are shown in the semi-logarithmic Schoellar diagram as equivalents per million per kilogram of solution (meq/kg). Each ion's concentration in each sample was shown by points on six evenly spaced lines, and those points were linked by a line.
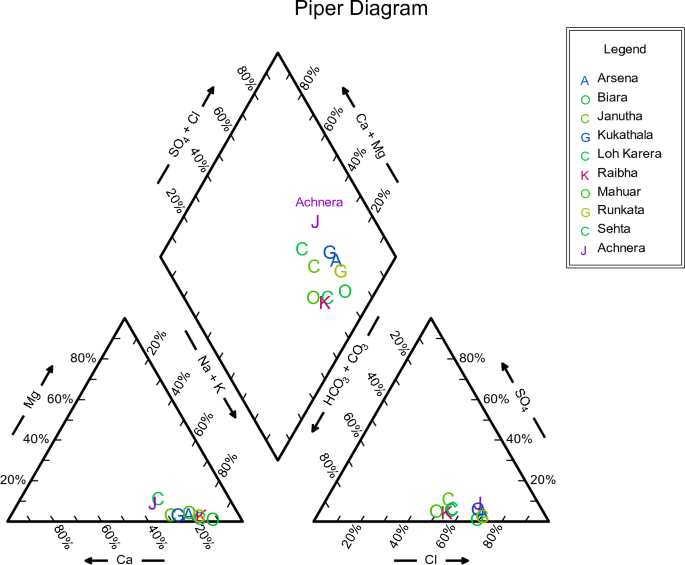
Piper plots for groundwater samples at Achhnera block, Agra, North India.
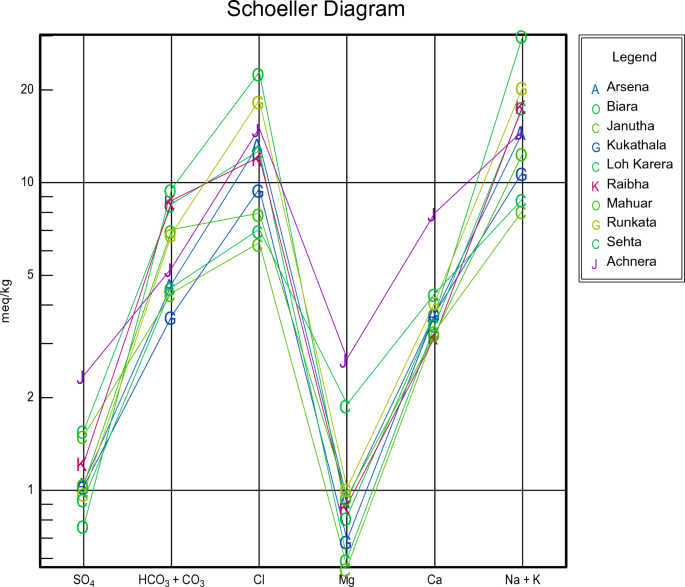
Schoeller diagram Achhnera block, Agra, North India.
In one of the studies on Poyang Lake, China, the presence of nitrogen-nitrate was reported as major threat to the lake with the extensive ongoing agricultural practices 49 , 50 . In the study, the multi-methods which include grey correlation analysis, Pearson correlation, mathematical statistics, and human health risk assessment were used for the investigation of spatiotemporal variations and potential risks of nitrogen.
The PCA has been executed in this study to determine homologous behavior and common origin of different physicochemical properties of groundwater. The values of different Principal Components (PCs) can be considered under strong, moderate, and weak loadings, if their value ranges from 1–0.75, 0.75–0.50 to 0.50–0.30, respectively. The application of PCA in the present study is to obtain correlations between the hydro-chemical components of the groundwater samples.
The PCA of the groundwater samples revealed that the variables are inter-correlated with 38.29% of the total variance. As per Kaiser Criterion, the PCs values, whose eigen values were found more than one, can be considered in factor analysis 51 . After varimax rotation, only three PCs values were found more than one, as shown in the scree plot Fig. 5 , and the rest can be ignored as their eigen values have been found less than one. Hence, three principal components have been extracted for the consideration. Table 5 showed the variance in the three PC values which is 38%, 32% and 10.85% reasonable correspondingly; hence, the rest of the components can be ignored. Principal component one (PC-1) comprised TDS, NO 3− , HCO 3− , Na + , TA, and fluoride with moderate to strong loading. Fluoride ion in PCA-1 showing the moderate to strong loading with TDS, NO 3− , HCO 3− , Na + and Ca 2+ , which appeared to be linked with geological origin fluoride in the present block, and their origin has been significantly correlated. The changes in the concentration of fluoride were directly related with the TDS, NO 3− , HCO 3− , Na + , Total Alkalinity, which can be explained due to the evolution of the fluoride from the fluoride bearing minerals present in host rocks and their interaction with groundwater. Therefore, it is concluded that there are no human sources of fluoride in groundwater, indicating that it is obtained geologically. Principal component two (PC-2) includes TH, Ca 2+ and Mg 2+ showing high positive factor loadings while in case of principal component three (PC-3) includes pH, Cl − , moderate to weak loading. Thus, it can be predicted from the PCA that the component one represents the controlling factors, which is responsible for rerelease of fluoride ions, as all sensitive parameters (TDS, NO 3− , HCO 3− , Na + and Ca 2+ ) of groundwater have moderate to strong loading with respect to all other principal components (Fig. 6 ). While other sensitive parameters (TDS, NO 3− , HCO 3− , Na + and Ca 2+ ) of groundwater show moderate to strong loading with respect to all other principal components.
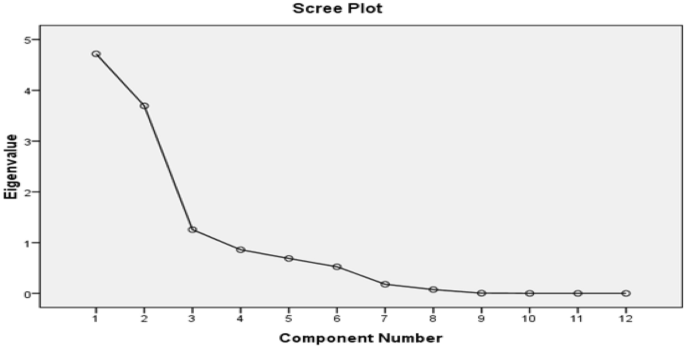
Scree plot of PCA of Achhnera block, Agra, Northern India.
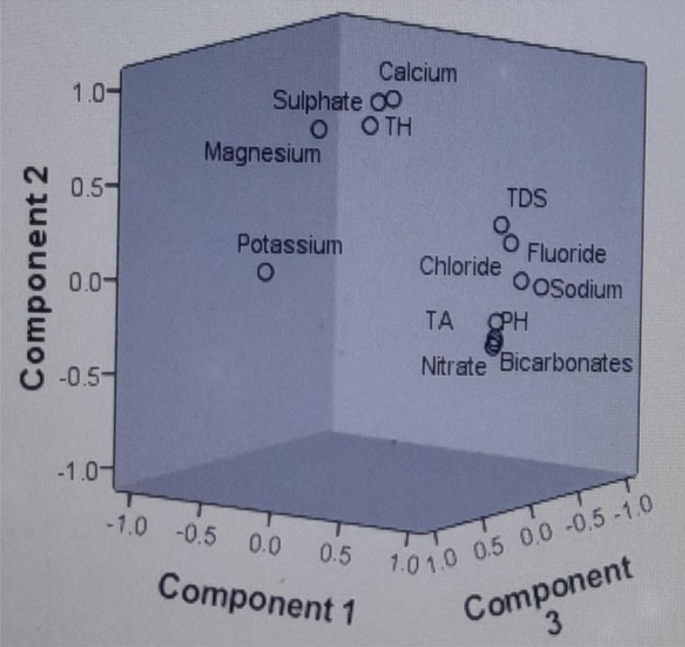
Component plot in rotated space of physico-chemical components of Achhnera block, Agra, North India.
Correlation analysis of Achhnera block
The correlation coefficient data of rural parts of Achhnera block, Agra region, North India are tabulated in Table 6 . Alkalinity was found to increase due to the replacement of fluoride with hydroxide ions. A positive correlation of concentration of hydrogen ion and sodium ion is observed with fluoride ion. The region may be due to high pH. Many researchers have found in their experimental work that there is a strong correlation ship between F − and H + ions as they have a strong tendency of combining and forming HF 52 , 53 , 54 . The concentration of sodium and bicarbonates have shown positive correlation with fluoride, which can be explained due to the high alkalinity in the sampled water, resulting in the dissolution of fluoride in groundwater 51 , 52 , 53 , 54 .
A strong correlation coefficient between different water quality parameters is seen at those places where the climatic conditions are humid like Assam (India). The fluoride content in these water samples are increasing in these arid and semi-arid climate regions because of the slow rate of water percolation through the ground 43 , 55 . Increase in concentration of OH − , HCO 3− , and CO 3 2− results in increase in alkalinity of water sample. Various studies carried out in different regions of the world show that desorption of as ion and F − from metallic oxide surface causes higher pH of the sampled water which is also confirmed by various experimental studies 30 , 43 , 65 . Table 7 provides a result of the impact of these variables on release of two pollutants separately and on the conductive environment for co-occurrence.
WQI and spatial distribution
In the area under investigation, it has been observed that water quality index ranges from 105 to 185, delineated as per the Table 8 . Therefore, ‘special treatment’ is needed in the study area, to qualify in ‘fit water’ category. It was found that the ions like F − , Cl − , Na + and alkalinity were above the permissible limit, resulting high total dissolved solids (TDS) value, which might be the cause of geogenic activities.
Through (Inverse Distance Weighted) IDW methods, the spatial distribution of factor scores was interpolated (Fig. 7 ). The graphical presentation of the WQI of the Achhnera block is illustrated in Fig. 7 a. Based on the measurement of physiochemical aspects of different samples, taken from different locations and the WQI range of the sampled region is shown in the pie chart (Fig. 7 b). From the analysis of the different water quality parameters, it was found that all the calculated values surpass the permissible limits suggested by WHO & BIS 38 , 39 , which results in a high level of TDS values. It is concluded from the experimental results that the high percentage of fluoride in the samples of Achhnera block, Agra district may be due to its geological conditions and the water of this region is unfit for drinking and cannot be used for various other purposes.
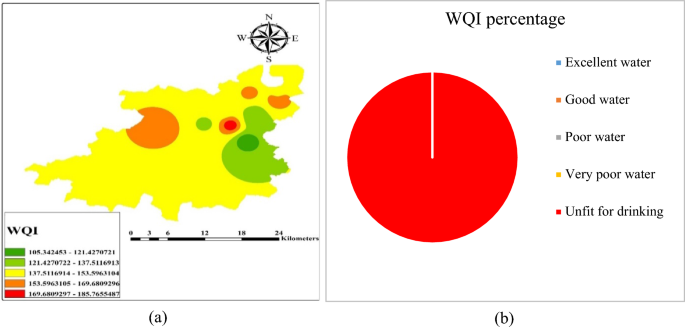
( a ) Spatial distribution of WQI in the study area and ( b ) graphical data representation of WQI classifications of Achhnera block, Agra region, Uttar Pradesh, North India.
Comparative study of the rural and urban areas using water quality parameters
It showed the comparative study done on the extent of pollution in drinking water between urban areas and the rural areas of Agra district of northern India. Study on GWQI of urban areas was previously carried out by Ali et al. 56 and current study is based on rural areas of Agra district of northern India (Fig. 8 ). Twelve important water quality parameters were compared in groundwater quality analysis (GWQI). The GWQI of urban area were ranged from 50.01 to 130.62, which reveals that more than half of the urban region was found in the category unfit for drinking (64%), nearly one fourth of the region lies in the poor category range (21.42%) and the remaining region lies in the very poor category range (14.28%). Figure 8 a, showed that no samples lie in the category of good or excellent. It was inferred that the large value of water quality index at urban regions was due to the geogenic as well as with some anthropogenic source (outlet of fertilizer industry).
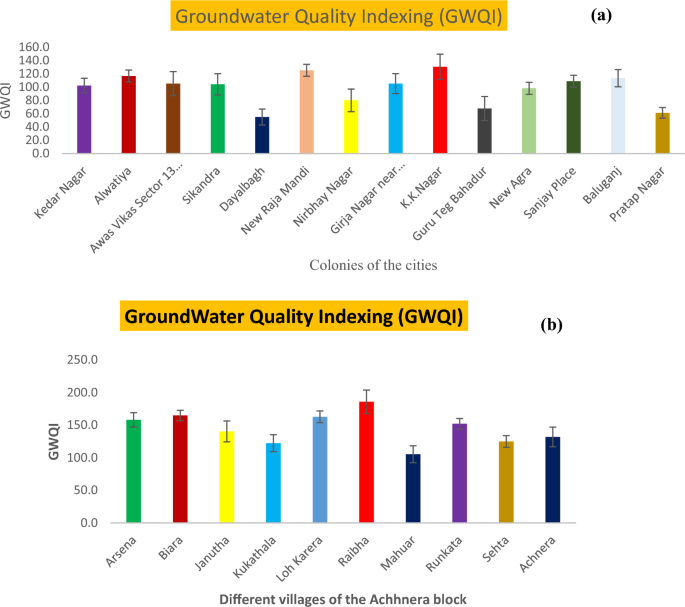
Bar chart of WQI comparative study with standard deviation of urban ( a ) and rural ( b ) areas of Agra region, Uttar Pradesh, Northern India.
Present study in, Achhnera block, shows that the WQI ranges from 185 to 105, delineated in Fig. 8 b. The analysis of WQI revealed that the sampled area lies in the unfit category for the drinking purpose. Therefore, it is advised that the drinking water should be treated before making it suitable for drinking in Achhnera block of Agra region, Northern India. It can be concluded from the comparative analysis that potable water of the rural areas is comparatively more polluted than that of the urban areas, which may be due to geogenic as well as anthropogenic activities (use of fluoride laden fertilizer in the field, leeches into the groundwater) 13 , 14 , 15 .
Conclusions
The present study has been performed to evaluate the groundwater quality for Achhnera block in the city of Taj, Agra, India, where groundwater is an important water resource. Therefore, this study was designed to the applicability of GWQI, and the PCA to mark out the major geochemical solutes responsible for origin and release of geochemical solutes into the groundwater. This study confirms that, majority of the groundwater samples in the study areas were mostly alkaline in nature. Elevated values of electrical conductivity, total dissolved solid, total hardness, fluoride and chloride in groundwater samples were mainly due to rock water interaction and high rate of evaporation. The results conclude that the water quality index belongs to unfit category for potable use in the study area, hence, almost all sampling tube-wells of the study area fell in the category of unsuitable for drinking purpose. Further, hydrochemistry of groundwater confirms that, most of the collected groundwater samples in the study area were comparatively saltier than freshwater. The Piper diagram concludes that, the major hydro chemical facies found in groundwater were sodium bicarbonate type or calcium chloride type. Finally, the PCA shows the pH, Na + , Ca 2+ and fluoride with high loading, suggests geogenic source of fluoride contamination. Therefore, it is recommended that the water of Achhnera block of Agra region Northern India, should be treated properly before use as potable water. It can be concluded from the comparative analysis that regions of the rural areas are comparatively more polluted than that of urban areas, which may be due to geogenic as well as anthropogenic activities (use of fluoride laden fertilizer in the field, leeches into the groundwater). Complete distribution of physico-chemical characteristics of water is shown in this study which can be used as a tool to improve the water quality for drinking purposes.
Data availability
The datasets generated and analyzed during the current study were available from the corresponding author on reasonable request.
Ali, S. et al. Qualitative assessment of ground water using the water quality index from a part of Western Uttar Pradesh, North India. Desalin. Water Treat. 252 , 332–338 (2022).
Article CAS Google Scholar
Rao, Q., Qiu, Y. & Li, J. Water quality assessment and variation trends analysis of the min river sea-entry section, China. Water Air Soil Pollut. 230 , 1–11 (2019).
Ali, S. et al. Health risk assessment due to fluoride contamination in groundwater of Bichpuri, Agra, India: A case study. Model. Earth Syst. Environ. 8 , 299–307 (2022).
Article Google Scholar
Takdastan, A. et al. Neuro-fuzzy inference system Prediction of stability indices and Sodium absorption ratio in Lordegan rural drinking water resources in west Iran. Data Brief. 18 , 255–261 (2018).
Article PubMed Central PubMed Google Scholar
Shams, M., Mohammadi, A. & Sajadi, S. A. Evaluation of corrosion and scaling potential of water in rural water supply distribution networks of Tabas, Iran. World Appl. Sci. J. 17 , 1484–1489 (2012).
CAS Google Scholar
Faraji, H. et al. Correlation between fluoride in drinking Water and its levels in breast milk in Golestan Province, Northern Iran. Iran. J. Public Health 43 , 1664–1668 (2014).
PubMed Central PubMed Google Scholar
Ramakrishnaiah, C. R. et al. Assessment of water quality index for the groundwater in Tumkur Taluk, Karnataka State, India. J. Chem. 6 , 523–530 (2009).
Berman, J. WHO: Waterborne disease is world’s leading killer. VOA News 29 , 66 (2009).
Google Scholar
Malik, A. et al. Water-borne diseases, cost of illness and willingness to pay for diseases interventions in rural communities of developing countries. Iran. J. Public Health 41 , 39–49 (2012).
PubMed Central CAS PubMed Google Scholar
Khan, S. U. et al. Optimizing fluoride removal and energy consumption in a batch reactor using electrocoagulation: A smart treatment technology. In Smart Cities—Opportunities and Challenges: Select Proceedings of ICSC 2019 vol. 58, 767–778 (2020).
Rokni, L. et al. Effect of persistent organic pollutants on human health in South Korea: A review of the reported diseases. Sustainable 15 , 10851 (2023).
Ali, S. et al. Spatial analysis and probabilistic risk assessment of exposure to fluoride in drinking water using GIS and Monte Carlo simulation. Environ. Sci. Pollut. Res. 29 , 5881–5890 (2022).
Wang, X. et al. Watershed scale spatiotemporal nitrogen transport and source tracing using dual isotopes among surface water, sediments and groundwater in the Yiluo River Watershed, Middle of China. Sci. Total Environ. 833 , 155180 (2022).
Article ADS CAS PubMed Google Scholar
Gibert, O. et al. Removal of nitrate from groundwater by nano-scale zero-valent iron injection pulses in continuous-flow packed soil columns. Sci. Total Environ. 810 , 152300 (2022).
Wang, X. et al. Spatiotemporal changes of nitrate retention at the interface between surface water and groundwater: Insight from watershed scale in an elevated nitrate region. Hydrol. Process. 37 , 14856 (2023).
Article ADS CAS Google Scholar
Jafarzade, N. et al. Viability of two adaptive fuzzy systems based on fuzzy c means and subtractive clustering methods for modeling Cadmium in groundwater resources. Heliyon 9 (8), 66 (2023).
Singh, P. K. et al. Qualitative assessment of surface water of West Bokaro Coalfield, Jharkhand by using water quality index method. Int. J. Chem. Technol. Res. 5 , 2351–2356 (2013).
Zhang, Q. et al. Groundwater quality assessment using a new integrated-weight water quality index (IWQI) and driver analysis in the Jiaokou Irrigation District, China. Ecotoxicol. Environ. Saf. 212 , 111992 (2021).
Article CAS PubMed Google Scholar
Dash, S. & Kalamdhad, A. S. Hydrochemical dynamics of water quality for irrigation use and introducing a new water quality index incorporating multivariate statistics. Environ. Earth Sci. 80 , 1–21 (2021).
Abbasnia, A. et al. Evaluation of groundwater quality using water quality index and its suitability for assessing water for drinking and irrigation purposes: Case study of Sistan and Baluchistan province (Iran). Hum. Ecol. Risk Assess. 25 , 988–1005 (2019).
Rad Fard, M. et al. Protocol for the estimation of drinking water quality index (DWQI) in water resources: Artificial neural network (ANFIS) and Arc-Gis. Methods X 6 , 1021–1029 (2019).
Thanh Giao, N. et al. Spatiotemporal analysis of surface water quality in Dong Thap province, Vietnam using water quality index and statistical approaches. Water 13 , 336 (2021).
Radfard, M. et al. Drinking water quality and arsenic health risk assessment in Sistan and Baluchestan, Southeastern Province, Iran. Hum. Ecol. Risk Assess. 25 , 949–965 (2018).
Abbasnia, A. et al. Groundwater quality assessment for irrigation purposes based on irrigation water quality index and its zoning with GIS in the villages of Chabahar, Sistan and Baluchistan, Iran. Data Brief. 19 , 623–631 (2018).
Taloor, A. K. et al. Spring water quality and discharge assessment in the Basantar watershed of Jammu Himalaya using geographic information system (GIS) and water quality Index (WQI). Groundw Sustain Dev. 10 , 100364 (2020).
Badeenezhad, A. et al. Estimation of the groundwater quality index and investigation of the affecting factors their changes in Shiraz drinking groundwater, Iran. Groundw. Sustain. Dev. 11 , 100435 (2020).
Uddin, M. G. et al. A review of water quality index models and their use for assessing surface water quality. Ecol. Indic. 122 , 107218 (2021).
Verma, P. et al. Assessment of groundwater quality status by using water quality index (WQI) and geographic information system (GIS) approaches: A case study of the Bokaro district, India. Appl. Water. Sci. 10 , 1–16 (2020).
Chakraborty, B. et al. Geospatial assessment of groundwater quality for drinking through water quality index and human health risk index in an upland area of Chota Nagpur Plateau of West Bengal, India. In Spatial Modeling and Assessment of Environmental Contaminants: Risk Assessment and Remediation 327–358 (2021).
Verma, S. & Sinha, A. Appraisal of groundwater arsenic on opposite banks of River Ganges, West Bengal, India, and quantification of cancer risk using Monte Carlo simulations. Environ. Sci. Pollut. Res. 30 , 25205–25225 (2023).
Chakrabarty, S. & Sarma, H. P. Heavy metal contamination of drinking water in Kamrup district, Assam, India. Environ. Monit. Assess. 179 , 479–486 (2011).
Mazhar, S. N. & Ahmad, S. Assessment of water quality pollution indices and distribution of heavy metals in drinking water in Ramganga aquifer, Bareilly District Uttar Pradesh, India. Groundw. Sustain. Dev. 10 , 100304 (2020).
Singh, K. K. et al. Understanding urban groundwater pollution in the Upper Gangetic Alluvial Plains of northern India with multiple industries and their impact on drinking water quality and associated health risks. Groundw. Sustain. Dev. 21 , 100902 (2023).
Gopal, B. & Chauhan, M. River Yamuna from source to Delhi: Human impacts and approaches to conservation. Restor. River Yamuna 66 , 45–69 (2007).
Rice, E. W., Bridgewater, L. & Association, A. P. H. Standard Methods for the Examination of Water and Wastewater Vol. 10 (American Public Health Association Washington, 2012).
Balan, I. et al. An assessment of groundwater quality using water quality index in Chennai, Tamil Nadu, India. Chron. Young Sci. 3 , 146–146 (2012).
Brown, R. M. et al. A water quality index—Crashing the psychological barrier. In Indicators of Environmental Quality: Proceedings of a symposium held during the AAAS Meeting in Philadelphia, Pennsylvania, December 26–31 , 1971 173–182 (Springer, 1972).
WHO, G. Guidelines for drinking-water quality. World Health Organ. 216 , 303–304 (2011).
BIS, I., 10500: Indian Standard Drinking Water-Specification (Second Revision) , (Bureau of Indian Standards, 2012).
Tyagi, S. et al. Water quality assessment in terms of water quality index. J. Am. Water Resour. Assoc. 1 , 34–38 (2013).
Benkov, I. et al. Principal component analysis and the water quality index—A powerful tool for surface water quality assessment: A case study on Struma River Catchment, Bulgaria. Water 15 , 1961 (2023).
Shokry, A. et al. Groundwater quality index based on PCA: Wadi El-Natrun, Egypt. J. Afr. Earth Sci. 172 , 103964 (2020).
Edmunds, W. M. & Smedley, P. L. Groundwater geochemistry and health: An overview. Geol. Soc. Lond. Spec. Publ. 113 , 91–105 (1996).
Ansari, J. A. & Umar, R. Evaluation of hydrogeochemical characteristics and groundwater quality in the quaternary aquifers of Unnao District, Uttar Pradesh, India. Hydro Res. 1 , 36–47 (2019).
Chaurasia, A. K. et al. A groundwater quality assessment using water quality index (WQI) in parts of Varanasi District, Uttar Pradesh, India. J. Geol. Soc. India 92 , 76–82 (2018).
Singh, S. Fluoride contamination in groundwater in some villages of Banda District, Uttar Pradesh, India. Int. J. Innov. Sci. Res. Technol. 3 , 66 (2016).
Dey, S., & Raju, N. J. Hydrogeochemical assessment to explore the extent, nature and source of fluoride contamination within the groundwaters of the Panda River Basin, Sonbhadra District, Uttar Pradesh, India. In Geostatistical and Geospatial Approaches for the Characterization of Natural Resources in the Environment: Challenges, Processes and Strategies 221–229 (Springer, 2016).
Reddy, A. G. S. Geochemical evaluation of nitrate and fluoride contamination in varied hydrogeological environs of Prakasam district, southern India. Environ. Earth Sci. 71 , 4473–4495 (2014).
Liu, Z. et al. Eutrophication causes analysis under the influencing of anthropogenic activities in China’s largest fresh water lake (Poyang Lake): Evidence from hydrogeochemistry and reverse simulation methods. J. Hydrol. 625 , 130020 (2023).
Liu, Z. et al. Multi-methods to investigate spatiotemporal variations of nitrogen-nitrate and its risks to human health in China’s largest fresh water lake (Poyang Lake). Sci. Total. Environ. 863 , 160975 (2023).
Kaiser, H. F. The application of electronic computers to factor analysis. Educ. Psychol. Meas. 20 , 141–151 (1960).
Khan, S. M. M. N. & Ravikumar, A. Role of alkalinity for the release of fluoride in the groundwater of Tiruchengode Taluk, Namakkal District, Tamilnadu, India. Chem. Sci. Trans. 2 , S302–S308 (2013).
Patolia, P. & Sinha, A. Fluoride contamination in Gharbar Village of Dhanbad District, Jharkhand, India: source identification and management. Arab. J. Geosci. 10 , 1–10 (2017).
Jha, P. K. & Tripathi, P. Arsenic and fluoride contamination in groundwater: A review of global scenarios with special reference to India. Groundw. Sustain. Dev. 13 , 100576 (2021).
Kumar, M. et al. Scenario, perspectives and mechanism of arsenic and fluoride co-occurrence in the groundwater: A review. Chemosphere 249 , 126126 (2020).
Ali, S. et al. Physico-chemical characterization of groundwater in terms of Water Quality Index (WQI) for urban areas of Agra, North India. Appl. Ecol. Environ. Sci. 10 , 409–416 (2022).
Ali, S. et al. Investigation and mapping of fluoride-endemic areas and associated health risk—A case study of Agra, Uttar Pradesh, India. Hum. Ecol. Risk Assess. 23 , 590–604 (2017).
Kim, S. et al. Co-contamination of arsenic and fluoride in the groundwater of unconsolidated aquifers under reducing environments. Chemosphere 87 , 851–856 (2012).
Hossain, S. et al. Geochemical processes controlling fluoride enrichment in groundwater at the western part of Kumamoto area, Japan. Water Air Soil Pollut. 227 , 1–14 (2016).
Currell, M. et al. Controls on elevated fluoride and arsenic concentrations in groundwater from the Yuncheng Basin, China. Appl. Geochem. 26 , 540–552 (2011).
Bhattacharya, P. et al. Distribution and mobility of arsenic in the Rio Dulce alluvial aquifers in Santiago del Estero Province, Argentina. Sci. Total Environ. 358 , 97–120 (2006).
Deng, Y. et al. Isotope and minor element geochemistry of high arsenic groundwater from Hangjinhouqi, the Hetao Plain, Inner Mongolia. Appl. Geochem. 24 , 587–599 (2009).
Kim, K. & Jeong, G. Y. Factors influencing natural occurrence of fluoride-rich groundwaters: A case study in the southeastern part of the Korean Peninsula. Chemosphere 58 , 1399–1408 (2005).
Amini, M. et al. Statistical modeling of global geogenic fluoride contamination in groundwaters. Environ. Sci. Technol. 42 , 3662–3668 (2008).
Gomez, M. L. et al. Arsenic and fluoride in a loess aquifer in the central area of Argentina. Environ. Geol. 57 , 143–155 (2009).
Download references
Acknowledgements
The authors would like to acknowledge (Anand Engineering College, Agra, Northern India) for granting permission to perform research work.
Author information
Authors and affiliations.
Department of Applied Sciences, Anand Engineering College, Agra, Uttar Pradesh, India
Shahjad Ali, Manish Baboo Agarwal & Manu Mehrotra
Department of Environmental Science and Engineering, IIT(ISM), Dhanbad, Jharkhand, India
Sitaram Verma
Department of Civil Engineering, GLA University, Mathura, India
Raisul Islam
Department of Applied Science (Mathematics), G.L. Bajaj Group of Institutions, Mathura, India
Rajesh Kumar Deolia
Department of Mathematics and Computing, Madhav Institute of Technology and Science, Gwalior, India
Jitendra Kumar
Department of Mechanical Engineering, Anand Engineering College, Agra, India
Shailendra Singh
Department of Environmental Health Engineering, Neyshabur University of Medical Sciences, Neyshabur, Iran
Ali Akbar Mohammadi
Department of Environment Science and Engineering, SRM University-AP, Amaravati, Andhra Pradesh, India
Department of Applied Science, Bundelkhand Institute of Engineering and Technology (BIET), Jhansi, India
Manoj Kumar Gupta
Institute of Research and Development, Duy Tan University, Da Nang, Vietnam
Phuyen Dang & Mehdi Fattahi
School of Engineering and Technology, Duy Tan University, Da Nang, Vietnam
You can also search for this author in PubMed Google Scholar
Contributions
Conceived and designed the experiments: S.A. and A.A.M. performed the experiments: S.V., R.K.D., R.I., M.B.A., M.M., analyzed and interpreted the data; D.R., J.K., contributed reagents, materials, analysis tools or data: M.K.G. and M.F. wrote the paper: R.I., M.B.A., M.M., M.K.G., P.D. and M.F.
Corresponding authors
Correspondence to Ali Akbar Mohammadi or Mehdi Fattahi .
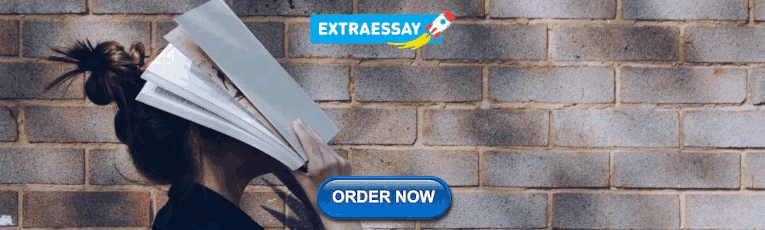
Ethics declarations
Competing interests.
The authors declare no competing interests.
Additional information
Publisher's note.
Springer Nature remains neutral with regard to jurisdictional claims in published maps and institutional affiliations.
Rights and permissions
Open Access This article is licensed under a Creative Commons Attribution 4.0 International License, which permits use, sharing, adaptation, distribution and reproduction in any medium or format, as long as you give appropriate credit to the original author(s) and the source, provide a link to the Creative Commons licence, and indicate if changes were made. The images or other third party material in this article are included in the article's Creative Commons licence, unless indicated otherwise in a credit line to the material. If material is not included in the article's Creative Commons licence and your intended use is not permitted by statutory regulation or exceeds the permitted use, you will need to obtain permission directly from the copyright holder. To view a copy of this licence, visit http://creativecommons.org/licenses/by/4.0/ .
Reprints and permissions
About this article
Cite this article.
Ali, S., Verma, S., Agarwal, M.B. et al. Groundwater quality assessment using water quality index and principal component analysis in the Achnera block, Agra district, Uttar Pradesh, Northern India. Sci Rep 14 , 5381 (2024). https://doi.org/10.1038/s41598-024-56056-8
Download citation
Received : 12 October 2023
Accepted : 01 March 2024
Published : 05 March 2024
DOI : https://doi.org/10.1038/s41598-024-56056-8
Share this article
Anyone you share the following link with will be able to read this content:
Sorry, a shareable link is not currently available for this article.
Provided by the Springer Nature SharedIt content-sharing initiative
- Water quality index
- Schollar diagram
- Hydrochemistry
- Principal component analysis
By submitting a comment you agree to abide by our Terms and Community Guidelines . If you find something abusive or that does not comply with our terms or guidelines please flag it as inappropriate.
Quick links
- Explore articles by subject
- Guide to authors
- Editorial policies
Sign up for the Nature Briefing newsletter — what matters in science, free to your inbox daily.

Open Access is an initiative that aims to make scientific research freely available to all. To date our community has made over 100 million downloads. It’s based on principles of collaboration, unobstructed discovery, and, most importantly, scientific progression. As PhD students, we found it difficult to access the research we needed, so we decided to create a new Open Access publisher that levels the playing field for scientists across the world. How? By making research easy to access, and puts the academic needs of the researchers before the business interests of publishers.
We are a community of more than 103,000 authors and editors from 3,291 institutions spanning 160 countries, including Nobel Prize winners and some of the world’s most-cited researchers. Publishing on IntechOpen allows authors to earn citations and find new collaborators, meaning more people see your work not only from your own field of study, but from other related fields too.
Brief introduction to this section that descibes Open Access especially from an IntechOpen perspective
Want to get in touch? Contact our London head office or media team here
Our team is growing all the time, so we’re always on the lookout for smart people who want to help us reshape the world of scientific publishing.
Home > Books > Water Quality - Science, Assessments and Policy
Water Quality Parameters
Submitted: 15 August 2019 Reviewed: 10 September 2019 Published: 16 October 2019
DOI: 10.5772/intechopen.89657
Cite this chapter
There are two ways to cite this chapter:
From the Edited Volume
Water Quality - Science, Assessments and Policy
Edited by Kevin Summers
To purchase hard copies of this book, please contact the representative in India: CBS Publishers & Distributors Pvt. Ltd. www.cbspd.com | [email protected]
Chapter metrics overview
15,333 Chapter Downloads
Impact of this chapter
Total Chapter Downloads on intechopen.com

Total Chapter Views on intechopen.com
Overall attention for this chapters
Since the industrial revolution in the late eighteenth century, the world has discovered new sources of pollution nearly every day. So, air and water can potentially become polluted everywhere. Little is known about changes in pollution rates. The increase in water-related diseases provides a real assessment of the degree of pollution in the environment. This chapter summarizes water quality parameters from an ecological perspective not only for humans but also for other living things. According to its quality, water can be classified into four types. Those four water quality types are discussed through an extensive review of their important common attributes including physical, chemical, and biological parameters. These water quality parameters are reviewed in terms of definition, sources, impacts, effects, and measuring methods.
- water quality
- physical parameters
- chemical parameters
- biological parameters
- radioactive substances
- toxic substances
- indicator organisms
Author Information
Nayla hassan omer *.
- Department of Environmental Engineering, College of Water and Environmental Engineering, Sudan University for Science and Technology, Khartoum, Sudan
*Address all correspondence to: [email protected]
1. Introduction
Water is the second most important need for life to exist after air. As a result, water quality has been described extensively in the scientific literature. The most popular definition of water quality is “it is the physical, chemical, and biological characteristics of water” [ 1 , 2 ]. Water quality is a measure of the condition of water relative to the requirements of one or more biotic species and/or to any human need or purpose [ 3 , 4 ].
2. Classification of water
Based on its source, water can be divided into ground water and surface water [ 5 ]. Both types of water can be exposed to contamination risks from agricultural, industrial, and domestic activities, which may include many types of pollutants such as heavy metals, pesticides, fertilizers, hazardous chemicals, and oils [ 6 ].
Potable water: It is safe to drink, pleasant to taste, and usable for domestic purposes [ 1 , 7 ].
Palatable water: It is esthetically pleasing; it considers the presence of chemicals that do not cause a threat to human health [ 7 ].
Contaminated ( polluted) water: It is that water containing unwanted physical, chemical, biological, or radiological substances, and it is unfit for drinking or domestic use [ 7 ].
Infected water: It is contaminated with pathogenic organism [ 7 ].
3. Parameters of water quality
There are three types of water quality parameters physical, chemical, and biological [ 8 , 9 ]. They are summarized in Table 1 .
3.1 Physical parameters of water quality
3.1.1 turbidity.
Turbidity is the cloudiness of water [ 10 ]. It is a measure of the ability of light to pass through water. It is caused by suspended material such as clay, silt, organic material, plankton, and other particulate materials in water [ 2 ].
It can increase the cost of water treatment for various uses [ 11 ].
The particulates can provide hiding places for harmful microorganisms and thereby shield them from the disinfection process [ 12 ].
Suspended materials can clog or damage fish gills, decreasing its resistance to diseases, reducing its growth rates, affecting egg and larval maturing, and affecting the efficiency of fish catching method [ 13 , 14 ].
Suspended particles provide adsorption media for heavy metals such as mercury, chromium, lead, cadmium, and many hazardous organic pollutants such as polychlorinated biphenyls (PCBs), polycyclic aromatic hydrocarbons (PAHs), and many pesticides [ 15 ].
The amount of available food is reduced [ 15 ] because higher turbidity raises water temperatures in light of the fact that suspended particles absorb more sun heat. Consequently, the concentration of the dissolved oxygen (DO) can be decreased since warm water carries less dissolved oxygen than cold water.
Turbidity is measured by an instrument called nephelometric turbidimeter, which expresses turbidity in terms of NTU or TU. A TU is equivalent to 1 mg/L of silica in suspension [ 10 ].
Turbidity more than 5 NTU can be visible to the average person while turbidity in muddy water, it exceeds 100 NTU [ 10 ]. Groundwater normally has very low turbidity because of the natural filtration that occurs as the water penetrates through the soil [ 9 , 16 ].
3.1.2 Temperature
Palatability, viscosity, solubility, odors, and chemical reactions are influenced by temperature [ 10 ]. Thereby, the sedimentation and chlorination processes and biological oxygen demand (BOD) are temperature dependent [ 11 ]. It also affects the biosorption process of the dissolved heavy metals in water [ 17 , 18 ]. Most people find water at temperatures of 10–15°C most palatable [ 10 , 19 ].
3.1.3 Color
Materials decayed from organic matter, namely, vegetation and inorganic matter such as soil, stones, and rocks impart color to water, which is objectionable for esthetic reasons, not for health reasons [ 10 , 20 ].
Color is measured by comparing the water sample with standard color solutions or colored glass disks [ 10 ]. One color unit is equivalent to the color produced by a 1 mg/L solution of platinum (potassium chloroplatinate (K 2 PtCl 6 )) [ 10 ].
Apparent color is the entire water sample color and consists of both dissolved and suspended components color [ 10 ].
True color is measured after filtering the water sample to remove all suspended material [ 19 ].
Color is graded on scale of 0 (clear) to 70 color units. Pure water is colorless, which is equivalent to 0 color units [ 10 ].
3.1.4 Taste and odor
Taste and odor in water can be caused by foreign matter such as organic materials, inorganic compounds, or dissolved gasses [ 19 ]. These materials may come from natural, domestic, or agricultural sources [ 21 ].
The numerical value of odor or taste is determined quantitatively by measuring a volume of sample A and diluting it with a volume of sample B of an odor-free distilled water so that the odor of the resulting mixture is just detectable at a total mixture volume of 200 ml [ 19 , 22 ]. The unit of odor or taste is expressed in terms of a threshold number as follows:
where TON is the threshold odor number and TTN is the threshold taste number.
3.1.5 Solids
Solids occur in water either in solution or in suspension [ 22 ]. These two types of solids can be identified by using a glass fiber filter that the water sample passes through [ 22 ]. By definition, the suspended solids are retained on the top of the filter and the dissolved solids pass through the filter with the water [ 10 ].
If the filtered portion of the water sample is placed in a small dish and then evaporated, the solids as a residue. This material is usually called total dissolved solids or TDS [ 10 ].
freshwater: <1500 mg/L TDS;
brackish water: 1500–5000 mg/L TDS;
saline water: >5000 mg/L TDS.
The residue of TSS and TDS after heating to dryness for a defined period of time and at a specific temperature is defined as fixed solids. Volatile solids are those solids lost on ignition (heating to 550°C) [ 10 ].
Total solids:

Interrelationship of solids found in water [ 22 ].
Total dissolved solids:
Total suspended solids:
Fixed and volatile suspended solids:
where VSSA = weight of residue + dish and filter before ignition, mg and VSSB = weight of residue + dish and filter after ignition, mg.
3.1.6 Electrical conductivity (EC)
The electrical conductivity (EC) of water is a measure of the ability of a solution to carry or conduct an electrical current [ 22 ]. Since the electrical current is carried by ions in solution, the conductivity increases as the concentration [ 10 ] of ions increases. Therefore, it is one of the main parameters used to determine the suitability of water for irrigation and firefighting.
U.S. units = micromhos/cm
S.I. units = milliSiemens/m (mS/m) or dS/m (deciSiemens/m)
Ultra-pure water: 5.5 × 10 −6 S/m;
Drinking water: 0.005–0.05 S/m;
Seawater: 5 S/m.
The electrical conductivity can be used to estimate the TDS value of water as follows [ 10 , 22 ]:
TDS can be used to estimate the ionic strength of water in the applications of groundwater recharging by treated wastewater [ 22 ]. The normal method of measurement is electrometric method [ 10 ].
3.2 Chemical parameters of water quality
pH is one of the most important parameters of water quality. It is defined as the negative logarithm of the hydrogen ion concentration [ 9 , 12 ]. It is a dimensionless number indicating the strength of an acidic or a basic solution [ 23 ]. Actually, pH of water is a measure of how acidic/basic water is [ 19 , 20 ]. Acidic water contains extra hydrogen ions (H + ) and basic water contains extra hydroxyl (OH − ) ions [ 2 ].
As shown in Figure 2 , pH ranges from 0 to 14, with 7 being neutral. pH of less than 7 indicates acidity, whereas a pH of greater than 7 indicates a base solution [ 2 , 24 ]. Pure water is neutral, with a pH close to 7.0 at 25°C. Normal rainfall has a pH of approximately 5.6 (slightly acidic) owing to atmospheric carbon dioxide gas [ 10 ]. Safe ranges of pH for drinking water are from 6.5 to 8.5 for domestic use and living organisms need [ 24 ].

pH of water.
A change of 1 unit on a pH scale represents a 10-fold change in the pH [ 10 ], so that water with pH of 7 is 10 times more acidic than water with a pH of 8, and water with a pH of 5 is 100 times more acidic than water with a pH of 7. There are two methods available for the determination of pH: electrometric and colorimetric methods [ 10 ].
Excessively high and low pHs can be detrimental for the use of water. A high pH makes the taste bitter and decreases the effectiveness of the chlorine disinfection, thereby causing the need for additional chlorine [ 21 ]. The amount of oxygen in water increases as pH rises. Low-pH water will corrode or dissolve metals and other substances [ 10 ].
Pollution can modify the pH of water, which can damage animals and plants that live in the water [ 10 ].
Most aquatic animals and plants have adapted to life in water with a specific pH and may suffer from even a slight change [ 15 ].
Even moderately acidic water (low pH) can decrease the number of hatched fish eggs, irritate fish and aquatic insect gills, and damage membranes [ 14 ].
Water with very low or high pH is fatal. A pH below 4 or above 10 will kill most fish, and very few animals can endure water with a pH below 3 or above 11 [ 15 ].
Amphibians are extremely endangered by low pH because their skin is very sensitive to contaminants [ 15 ]. Some scientists believe that the current decrease in amphibian population throughout the globe may be due to low pH levels induced by acid rain.
Heavy metals such as cadmium, lead, and chromium dissolve more easily in highly acidic water (lower pH). This is important because many heavy metals become much more toxic when dissolved in water [ 21 ].
A change in the pH can change the forms of some chemicals in the water. Therefore, it may affect aquatic plants and animals [ 21 ]. For instance, ammonia is relatively harmless to fish in neutral or acidic water. However, as the water becomes more alkaline (the pH increases), ammonia becomes progressively more poisonous to these same organisms.
3.2.2 Acidity
Acidity is the measure of acids in a solution. The acidity of water is its quantitative capacity to neutralize a strong base to a selected pH level [ 10 ]. Acidity in water is usually due to carbon dioxide, mineral acids, and hydrolyzed salts such as ferric and aluminum sulfates [ 10 ]. Acids can influence many processes such as corrosion, chemical reactions and biological activities [ 10 ].
Carbon dioxide from the atmosphere or from the respiration of aquatic organisms causes acidity when dissolved in water by forming carbonic acid (H 2 CO 3 ). The level of acidity is determined by titration with standard sodium hydroxide (0.02 N) using phenolphthalein as an indicator [ 10 , 20 ].
3.2.3 Alkalinity
The alkalinity of water is its acid-neutralizing capacity comprised of the total of all titratable bases [ 10 ]. The measurement of alkalinity of water is necessary to determine the amount of lime and soda needed for water softening (e.g., for corrosion control in conditioning the boiler feed water) [ 22 ]. Alkalinity of water is mainly caused by the presence of hydroxide ions (OH − ), bicarbonate ions (HCO 3− ), and carbonate ions (CO 3 2− ), or a mixture of two of these ions in water. As stated in the following equation, the possibility of OH − and HCO 3 − ions together are not possible because they react together to produce CO 3 2− ions:
Alkalinity is determined by titration with a standard acid solution (H 2 SO 4 of 0.02 N) using selective indicators (methyl orange or phenolphthalein).
The high levels of either acidity or alkalinity in water may be an indication of industrial or chemical pollution. Alkalinity or acidity can also occur from natural sources such as volcanoes. The acidity and alkalinity in natural waters provide a buffering action that protects fish and other aquatic organisms from sudden changes in pH. For instance, if an acidic chemical has somehow contaminated a lake that had natural alkalinity, a neutralization reaction occurs between the acid and alkaline substances; the pH of the lake water remains unchanged. For the protection of aquatic life, the buffering capacity should be at least 20 mg/L as calcium carbonate.
3.2.4 Chloride
Chloride occurs naturally in groundwater, streams, and lakes, but the presence of relatively high chloride concentration in freshwater (about 250 mg/L or more) may indicate wastewater pollution [ 7 ]. Chlorides may enter surface water from several sources including chloride-containing rock, agricultural runoff, and wastewater.
Chloride ions Cl − in drinking water do not cause any harmful effects on public health, but high concentrations can cause an unpleasant salty taste for most people. Chlorides are not usually harmful to people; however, the sodium part of table salt has been connected to kidney and heart diseases [ 25 ]. Small amounts of chlorides are essential for ordinary cell functions in animal and plant life.
Sodium chloride may impart a salty taste at 250 mg/L; however, magnesium or calcium chloride are generally not detected by taste until reaching levels of 1000 mg/L [ 10 ]. Standards for public drinking water require chloride levels that do not exceed 250 mg/L. There are many methods to measure the chloride concentration in water, but the normal one is the titration method by silver nitrate [ 10 ].
3.2.5 Chlorine residual
Chlorine (Cl 2 ) does not occur naturally in water but is added to water and wastewater for disinfection [ 10 ]. While chlorine itself is a toxic gas, in dilute aqueous solution, it is not harmful to human health. In drinking water, a residual of about 0.2 mg/L is optimal. The residual concentration which is maintained in the water distribution system ensures good sanitary quality of water [ 11 ].
Chlorine can react with organics in water forming toxic compounds called trihalomethanes or THMs, which are carcinogens such as chloroform CHCl 3 [ 11 , 22 ]. Chlorine residual is normally measured by a color comparator test kit or spectrophotometer [ 10 ].
3.2.6 Sulfate
Sulfate ions (SO 4 2− ) occur in natural water and in wastewater. The high concentration of sulfate in natural water is usually caused by leaching of natural deposits of sodium sulfate (Glauber’s salt) or magnesium sulfate (Epson salt) [ 11 , 26 ]. If high concentrations are consumed in drinking water, there may be objectionable tastes or unwanted laxative effects [ 26 ], but there is no significant danger to public health.
3.2.7 Nitrogen
There are four forms of nitrogen in water and wastewater: organic nitrogen, ammonia nitrogen, nitrite nitrogen, and nitrate nitrogen [ 10 ]. If water is contaminated with sewage, most of the nitrogen is in the forms of organic and ammonia, which are transformed by microbes to form nitrites and nitrates [ 22 ]. Nitrogen in the nitrate form is a basic nutrient to the growth of plants and can be a growth-limiting nutrient factor [ 10 ].
A high concentration of nitrate in surface water can stimulate the rapid growth of the algae which degrades the water quality [ 22 ]. Nitrates can enter the groundwater from chemical fertilizers used in the agricultural areas [ 22 ]. Excessive nitrate concentration (more than 10 mg/L) in drinking water causes an immediate and severe health threat to infants [ 19 ]. The nitrate ions react with blood hemoglobin, thereby reducing the blood’s ability to hold oxygen which leads to a disease called blue baby or methemoglobinemia [ 10 , 19 ].
3.2.8 Fluoride
A moderate amount of fluoride ions (F − ) in drinking water contributes to good dental health [ 10 , 19 ]. About 1.0 mg/L is effective in preventing tooth decay, particularly in children [ 10 ].
Excessive amounts of fluoride cause discolored teeth, a condition known as dental fluorosis [ 11 , 19 , 26 ]. The maximum allowable levels of fluoride in public water supplies depend on local climate [ 26 ]. In the warmer regions of the country, the maximum allowable concentration of fluoride for potable water is 1.4 mg/L; in colder climates, up to 2.4 mg/L is allowed.
There are four methods to determine ion fluoride in water; the selection of the used method depends on the type of water sample [ 10 ].
3.2.9 Iron and manganese
Although iron (Fe) and manganese (Mn) do not cause health problems, they impart a noticeable bitter taste to drinking water even at very low concentration [ 10 , 11 ].
These metals usually occur in groundwater in solution as ferrous (Fe 2+ ) and manganous (Mn 2+ ) ions. When these ions are exposed to air, they form the insoluble ferric (Fe 3+ ) and manganic (Mn 3+) forms making the water turbid and unacceptable to most people [ 10 ].
These ions can also cause black or brown stains on laundry and plumbing fixtures [ 7 ]. They are measured by many instrumental methods such as atomic absorption spectrometry, flame atomic absorption spectrometry, cold vapor atomic absorption spectrometry, electrothermal atomic absorption spectrometry, and inductively coupled plasma (ICP) [ 10 ].
3.2.10 Copper and zinc
Copper (Cu) and zinc (Zn) are nontoxic if found in small concentrations [ 10 ]. Actually, they are both essential and beneficial for human health and growth of plants and animals [ 25 ]. They can cause undesirable tastes in drinking water. At high concentrations, zinc imparts a milky appearance to the water [ 10 ]. They are measured by the same methods used for iron and manganese measurements [ 10 ].
3.2.11 Hardness
Hardness is a term used to express the properties of highly mineralized waters [ 10 ]. The dissolved minerals in water cause problems such as scale deposits in hot water pipes and difficulty in producing lather with soap [ 11 ].
Calcium (Ca 2+ ) and magnesium (Mg 2+ ) ions cause the greatest portion of hardness in naturally occurring waters [ 9 ]. They enter water mainly from contact with soil and rock, particularly limestone deposits [ 10 , 27 ].
Temporary hardness which is due to carbonates and bicarbonates can be removed by boiling, and
Permanent hardness which is remaining after boiling is caused mainly by sulfates and chlorides [ 10 , 21 , 22 ]
Water with more than 300 mg/L of hardness is generally considered to be hard, and more than 150 mg/L of hardness is noticed by most people, and water with less than 75 mg/L is considered to be soft.
From health viewpoint, hardness up to 500 mg/L is safe, but more than that may cause a laxative effect [ 10 ]. Hardness is normally determined by titration with ethylene diamine tetra acidic acid or (EDTA) and Eriochrome Black and Blue indicators. It is usually expressed in terms of mg/L of CaCO 3 [ 10 , 19 ].
An accepted water classification according to its hardness is as in Table 2 [ 19 ].
Parameters of water quality.
Classification of water according to its hardness.
3.2.12 Dissolved oxygen
Dissolved oxygen (DO) is considered to be one of the most important parameters of water quality in streams, rivers, and lakes. It is a key test of water pollution [ 10 ]. The higher the concentration of dissolved oxygen, the better the water quality.
Oxygen is slightly soluble in water and very sensitive to temperature. For example, the saturation concentration at 20°C is about 9 mg/L and at 0°C is 14.6 mg/L [ 22 ].
The actual amount of dissolved oxygen varies depending on pressure, temperature, and salinity of the water. Dissolved oxygen has no direct effect on public health, but drinking water with very little or no oxygen tastes unpalatable to some people.
There are three main methods used for measuring dissolved oxygen concentrations: the colorimetric method—quick and inexpensive, the Winkler titration method—traditional method, and the electrometric method [ 10 ].
3.2.13 Biochemical oxygen demand (BOD)
Bacteria and other microorganisms use organic substances for food. As they metabolize organic material, they consume oxygen [ 10 , 22 ]. The organics are broken down into simpler compounds, such as CO 2 and H 2 O, and the microbes use the energy released for growth and reproduction [ 22 ].
When this process occurs in water, the oxygen consumed is the DO in the water. If oxygen is not continuously replaced by natural or artificial means in the water, the DO concentration will reduce as the microbes decompose the organic materials. This need for oxygen is called the biochemical oxygen demand (BOD). The more organic material there is in the water, the higher the BOD used by the microbes will be. BOD is used as a measure of the power of sewage; strong sewage has a high BOD and weak sewage has low BOD [ 22 ].
The complete decomposition of organic material by microorganisms takes time, usually 20 d or more under ordinary circumstances [ 22 ]. The quantity of oxygen used in a specified volume of water to fully decompose or stabilize all biodegradable organic substances is called the ultimate BOD or BOD L .
BOD is a function of time. At time = 0, no oxygen will have been consumed and the BOD = 0. As each day goes by, oxygen is used by the microbes and the BOD increases. Ultimately, the BOD L is reached and the organic materials are completely decomposed.
A graph of the BOD versus time is illustrated as in Figure 3 . This is called the BOD curve, which can be expressed mathematically by the following equation:

BOD curve [ 22 ].
where BOD t = BOD at any time t, mg/L; BOD L = ultimate BOD, mg/L; k = a constant representing the rate of the BOD reaction; t = time, d.
The value of the constant rate k depends on the temperature, the type of organic materials, and the type of microbes exerting the BOD [ 22 ].
3.2.14 Chemical oxygen demand (COD)
The chemical oxygen demand (COD) is a parameter that measures all organics: the biodegradable and the non-biodegradable substances [ 22 ]. It is a chemical test using strong oxidizing chemicals (potassium dichromate), sulfuric acid, and heat, and the result can be available in just 2 h [ 10 ]. COD values are always higher than BOD values for the same sample [ 22 ].
3.2.15 Toxic inorganic substances
Metallic compounds: This group includes some heavy metals that are toxic, namely, cadmium (Cd), chromium (Cr), lead (Pb), mercury (Hg), silver (Ag), arsenic (As), barium (Ba), thallium (Tl), and selenium (Se) [ 22 , 28 ]. They have a wide range of dangerous effects that differ from one metal to another. They may be acute fatal poisons such as (As) and (Cr 6+ ) or may produce chronic diseases such as (Cd, Hg, Pb, and Tl) [ 21 , 29 , 30 , 31 , 32 ]. The heavy metals concentration can be determined by atomic absorption photometers, spectrophotometer, or inductively coupled plasma (ICP) for very low concentration [ 10 ].
Nonmetallic compounds: This group includes nitrates (NO 3 − ) and cyanides (CN − ), nitrate has been discussed with the nitrogen in the previous section. Regarding cyanide, as Mackenzie stated [ 11 ] it causes oxygen deprivation by binding the hemoglobin sites and prevents the red blood cell from carrying the oxygen [ 11 ]. This causes a blue skin color syndrome, which is called cyanosis [ 33 ]. It also causes chronic effects on the central nervous system and thyroid [ 33 ]. Cyanide is normally measured by colorimetric, titrimetric, or electrometric methods [ 10 ].
3.2.16 Toxic organic substances
There are more than 100 compounds in water that have been listed in the literature as toxic organic compounds [ 11 , 22 ]. They will not be found naturally in water; they are usually man-made pollutants. These compounds include insecticides, pesticides, solvents, detergents, and disinfectants [ 11 , 21 , 22 ]. They are measured by highly sophisticated instrumental methods, namely, gas chromatographic (GC), high-performance liquid chromatographic (HPLC), and mass spectrophotometric [ 10 ].
3.2.17 Radioactive substances
Potential sources of radioactive substances in water include wastes from nuclear power plants, industries, or medical research using radioactive chemicals and mining of uranium ores or other radioactive materials [ 11 , 21 ]. When radioactive substances decay, they release beta, alpha, and gamma radiation [ 34 ]. Exposure of humans and other living things to radiation can cause genetic and somatic damage to the living tissues [ 34 , 35 ].
Radon gas is of a great health concern because it occurs naturally in groundwater and is a highly volatile gas, which can be inhaled during the showering process [ 35 ]. For drinking water, there are established standards commonly used for alpha particles, beta particles, photons emitters, radium-226 and -228, and uranium [ 34 , 35 ].
The unit of radioactivity used in water quality applications is the picocurie per liter (pCi/L); 1 pCi is equivalent to about two atoms disintegrating per minute. There are many sophisticated instrumental methods to measure it [ 35 ].
3.3 Biological parameters of water quality
One of the most helpful indicators of water quality may be the presence or lack of living organisms [ 10 , 15 ]. Biologists can survey fish and insect life of natural waters and assess the water quality on the basis of a computed species diversity index (SDI) [ 15 , 19 , 36 , 37 ]; hence, a water body with a large number of well-balanced species is regarded as a healthy system [ 17 ]. Some organisms can be used as an indication for the existence of pollutants based on their known tolerance for a specified pollutant [ 17 ].
Microorganisms exist everywhere in nature [ 38 ]. Human bodies maintain a normal population of microbes in the intestinal tract; a big portion of which is made up of coliform bacteria [ 38 ]. Although there are millions of microbes per milliliter in wastewater, most of them are harmless [ 37 ]. It is only harmful when wastewater contains wastes from people infected with diseases that the presence of harmful microorganisms in wastewater is likely to occur [ 38 ].
3.3.1 Bacteria
Bacteria are considered to be single-celled plants because of their cell structure and the way they ingest food [ 10 , 37 ]. Bacteria occur in three basic cell shapes: rod-shaped or bacillus, sphere-shaped or coccus, and spiral-shaped or spirellus [ 19 ]. In less than 30 min, a single bacterial cell can mature and divide into two new cells [ 39 ].
Under favorable conditions of food supply, temperature, and pH, bacteria can reproduce so rapidly that a bacterial culture may contain 20 million cells per milliliter after just 1 day [ 22 , 37 ]. This rapid growth of visible colonies of bacteria on a suitable nutrient medium makes it possible to detect and count the number of bacteria in water [ 39 ].
There are several distinctions among the various species of bacteria. One distinction depends on how they metabolize their food [ 38 ]. Bacteria that require oxygen for their metabolism are called aerobic bacteria, while those live only in an oxygen-free environment are called anaerobic bacteria. Some species called facultative bacteria can live in either the absence or the presence of oxygen [ 37 , 38 , 39 ].
At low temperatures, bacteria grow and reproduce slowly. As the temperature increases, the rate of growth and reproduction doubles in every additional 10°C (up to the optimum temperature for the species) [ 38 ]. The majority of the species of bacteria having an optimal temperature of about 35°C [ 39 ].
A lot of dangerous waterborne diseases are caused by bacteria, namely, typhoid and paratyphoid fever, leptospirosis, tularemia, shigellosis, and cholera [ 19 ]. Sometimes, the absence of good sanitary practices results in gastroenteritis outbreaks of one or more of those diseases [ 19 ].
3.3.2 Algae
Algae are microscopic plants, which contain photosynthetic pigments, such as chlorophyll [ 37 , 39 ]. They are autotrophic organisms and support themselves by converting inorganic materials into organic matter by using energy from the sun, during this process they take in carbon dioxide and give off oxygen [ 38 , 39 ]. They are also important for wastewater treatment in stabilization ponds [ 22 ]. Algae are primarily nuisance organisms in the water supply because of the taste and odor problems they create [ 2 , 16 ]. Certain species of algae cause serious environmental and public health problems; for example, blue-green algae can kill cattle and other domestic animals if the animals drink water containing those species [ 37 , 39 ].
3.3.3 Viruses
Viruses are the smallest biological structures known to contain all genetic information necessary for their own reproduction [ 19 ]. They can only be seen by a powerful electronic microscope [ 39 ]. Viruses are parasites that need a host to live [ 39 ]. They can pass through filters that do not permit the passage of bacteria [ 37 ]. Waterborne viral pathogens are known to cause infectious hepatitis and poliomyelitis [ 19 , 25 , 37 ]. Most of the waterborne viruses can be deactivated by the disinfection process conducted in the water treatment plant [ 19 ].
3.3.4 Protozoa
Protozoa are single-celled microscopic animal [ 19 ], consume solid organic particles, bacteria, and algae for food, and they are in turn ingested as food by higher level multicellular animals [ 37 ]. Aquatic protozoa are floating freely in water and sometimes called zooplankton [ 37 ]. They form cysts that are difficult to inactivate by disinfection [ 19 ].
3.3.5 Indicator organisms
A very important biological indicator of water and pollution is the group of bacteria called coliforms [ 20 ]. Pathogenic coliforms always exist in the intestinal system of humans, and millions are excreted with body wastes [ 37 ]. Consequently, water that has been recently contaminated with sewage will always contain coliforms [ 19 ].
A particular species of coliforms found in domestic sewage is Escherichia coli or E. coli [ 22 ]. Even if the water is only slightly polluted, they are very likely to be found. There are roughly 3 million of E. coli bacteria in 100 mL volume of untreated sewage [ 10 ]. Coliform bacteria are aggressive organisms and survive in the water longer than most pathogens. There are normally two methods to test the coliform bacteria—the membrane filter method and multiple-tube fermentation method [ 10 , 37 ]. Since the test of coliform bacteria is very important for public health, the first method will be described in details in the coming section.
3.3.5.1 Testing for coliforms: membrane filter method
A measured volume of sample is filtered through a special membrane filter by applying a partial vacuum [ 10 , 39 ].
The filter, a flat paper-like disk, has uniform microscopic pores small enough to retain the bacteria on its surface while allowing the water to pass through. The filter paper is then placed in a sterile container called a petri dish, which contains a special culture medium that the bacteria use as a food source [ 39 ].
Then, the petri dish is usually placed in an incubator, which keeps the temperature at 35°C, for 24 h. After incubation, colonies of coliform bacteria each containing millions of organisms will be visible [ 10 ]. The coliform concentration is obtained by counting the number of colonies on the filter; each colony counted represents only one coliform in the original sample [ 10 , 39 ].
Coliform concentrations are expressed in terms of the number of organisms per 100 mL of water as follows:
4. Water quality requirements
Water quality requirements differ depending on the proposed used of water [ 19 ]. As reported by Tchobanoglous et al. [ 19 ], “water unsuitable for one use may be quite satisfactory for another and water may be considered acceptable for a particular use if water of better quality is not available.”
Water quality requirements should be agreed with the water quality standards, which are put down by the governmental agency and represent the legislation requirements. In general, there are three types of standards: in-stream, potable water, and wastewater effluent [ 19 ], each type has its own criteria by using the same methods of measurement. The World Health Organization (WHO) has established minimum standards for drinking water that all countries are recommended to meet [ 25 ].
5. Conclusion
The physical, chemical, and biological parameters of water quality are reviewed in terms of definition, sources, impacts, effects, and measuring methods. The classification of water according to its quality is also covered with a specific definition for each type.
- 1. Spellman FR. Handbook of Water and Wastewater Treatment Plant Operations. 3rd ed. Boca Raton: CRC Press; 2013
- 2. Alley ER. Water Quality Control Handbook. Vol. 2. New York: McGraw-Hill; 2007
- 3. Shah C. Which Physical, Chemical and Biological Parameters of Water Determine Its Quality?; 2017
- 4. Tchobanoglous G, Schroeder E. Water Quality: Characteristics, Modeling, Modification. 1985
- 5. Gray N. Water Technology. 3rd ed. London: CRC Press; 2017
- 6. Davis ML, Masten SJ. Principles of Environmental Engineering and Science. New York: McGraw-Hill; 2004
- 7. Chatterjee A. Water Supply Waste Disposal and Environmental Pollution Engineering (Including Odour, Noise and Air Pollution and its Control). 7th ed. Delhi: Khanna Publishers; 2001
- 8. Gray NF. Drinking Water Quality: Problems and Solutions. 2nd ed. Cambridge: Cambridge University Press; 2008
- 9. Spellman FR. The Drinking Water Handbook. 3rd ed. Boca Raton: CRC Press; 2017
- 10. APHA. Standard Methods for the Examination of Water and Wastewater. 21st ed. Washington, DC: American Public Health Association; 2005
- 11. Davis ML. Water and Wastewater Engineering—Design Principles and Practice. New York: McGraw-Hill; 2010
- 12. Edzwald JK. Water Quality and Treatment a Handbook on Drinking Water. New York: McGraw-Hill; 2010
- 13. Tarras-Wahlberg H, Harper D, Tarras-Wahlberg N. A first limnological description of Lake Kichiritith, Kenya: A possible reference site for the freshwater lakes of the Gregory Rift valley. South African Journal of Science. 2003; 99 :494-496
- 14. Kiprono SW. Fish Parasites and Fisheries Productivity in Relation to Exreme Flooding of Lake Baringo, Kenya [PhD]. Nairobi: Kenyatta University; 2017
- 15. Cole S, Codling I, Parr W, Zabel T, Nature E, Heritage SN. Guidelines for Managing Water Quality Impacts within UK European Marine Sites; 1999
- 16. Viessman W, Hammer MJ. Water Supply and Pollution Control. 7th ed. Upper Saddle River: New Jersey Pearson Prentice Hall; 2004
- 17. Abbas SH, Ismail IM, Mostafa TM, Sulaymon AH. Biosorption of heavy metals: A review. Journal of Chemical Science and Technology. 2014; 3 :74-102
- 18. White C, Sayer J, Gadd G. Microbial solubilization and immobilization of toxic metals: Key biogeochemical processes for treatment of contamination. FEMS Microbiology Reviews. 1997; 20 :503-516
- 19. Tchobanoglous G, Peavy HS, Rowe DR. Environmental Engineering. New York: McGraw-Hill Interamericana; 1985
- 20. Tomar M. Quality Assessment of Water and Wastewater. Boca Raton: CRC Press; 1999
- 21. DeZuane J. Handbook of Drinking Water Quality. 2nd ed. New York: John Wiley & Sons; 1997
- 22. Tchobanoglous G, Burton FL, Stensel HD. Metcalf & Eddy Wastewater Engineering: Treatment and Reuse. 4th ed. New Delhi: Tata McGraw-Hill Limited; 2003
- 23. Hammer MJ. Water and Wastewater Technology. 7th ed. Upper Saddle River: Pearson education; 2011
- 24. World Health Organization Guidelines for drinking-water quality. 4th ed. Geneva: WHO; 2011
- 25. World Health Organization. Guidelines for Drinking-Water Q , Vol. 2, Health criteria and other supporting information. 1996
- 26. Davis ML, David A. Introduction to Environmental Engineering. 4th ed. New York: McGraw-Hill Companies; 2008
- 27. McGhee TJ, Steel EW. Water Supply and Sewerage. New York: McGraw-Hill; 1991
- 28. Järup L. Hazards of heavy metal contamination. British Medical Bulletin. 2003; 68 :167-182
- 29. Campanella B, Onor M, D’Ulivo A, Giannecchini R, D’Orazio M, Petrini R, et al. Human exposure to thallium through tap water: A study from Valdicastello Carducci and Pietrasanta (northern Tuscany, Italy). Science of the Total Environment. 2016; 548 :33-42
- 30. Das AK, Dutta M, Cervera ML, de la Guardia M. Determination of thallium in water samples. Microchemical Journal. 2007; 86 :2-8
- 31. Lasheen MR, Shehata SA, Ali GH. Effect of cadmium, copper and chromium (VI) on the growth of Nile water algae. Water, Air, & Soil Pollution. 1990; 50 :19-30
- 32. World Health Organization. Chromium in Drinking-Water. Geneva: World Health Organization (WHO); 2003
- 33. Dojlido J, Best GA. Chemistry of Water and Water Pollution. Chichester: Ellis Horwood Limited; 1993
- 34. Skeppström K, Olofsson B. Uranium and radon in groundwater. European Water. 2007; 17 :51-62
- 35. Cothern CR. Radon, Radium, and Uranium in Drinking Water. Boca Raton: CRC Press; 2014
- 36. Alabaster JS, Lloyd RS. Water Quality Criteria for Freshwater Fish. 2nd ed. Cambridge: Butterworths; 1984
- 37. Nathanson JA. Basic Environmental Technology: Water Supply. New Delhi: Printice-Hall of India; 2004
- 38. Wiesmann U, Choi IS, Dombrowski E-M. Fundamentals of Biological Wastewater Treatment. Darmstadt: John Wiley & Sons; 2007
- 39. Mara D, Horan NJ. Handbook of Water and Wastewater Microbiology. London: Elsevier; 2003
© 2019 The Author(s). Licensee IntechOpen. This chapter is distributed under the terms of the Creative Commons Attribution 3.0 License , which permits unrestricted use, distribution, and reproduction in any medium, provided the original work is properly cited.
Continue reading from the same book
Water quality.
Edited by J. Kevin Summers
Published: 29 July 2020
By Kate K. Mulvaney, Nathaniel H. Merrill and Marisa ...
1398 downloads
By Abdallah Zacharia, Anne H. Outwater and Rob Van De...
1727 downloads
By Hongbo Du, Subhani Bandara, Laura E. Carson and Ra...
1288 downloads
County project aims to control flooding, improve water quality in North Canton, Plain area

- The basin is located on property owned by the county next to the Redwood Apartments.
Continued development upstream in the years since has exacerbated the need for an expanded basin.
- The contract was awarded to United Earthworks of Deerfield.
PLAIN TWP. − A major expansion of the detention basin on Mount Pleasant Street NW is the latest effort to ease flooding in the Zimber Ditch area near North Canton.
The basin, located on property owned by the county next to the Redwood Apartments, was built in 2006. Its construction was first in a series of moves to mitigate downstream flooding.
The county took another step in 2015, when the Stark County Park District bought and demolished five homes in the Zimber Ditch flood area and turned it to greenspace.
"There have been quite a few major rain events," County Administrator Brant Luther said. "It seemed to everyone that if the basin was just a little bit longer and larger, and held that rainwater back a little bit longer to allow it to drain out slower, it would really help downstream flooding."
The project, which will begin soon, will expand the basin by one-third and convert it into a water quality basin. The basin has capacity to hold 14,388,592 gallons of water. The expansion will add an additional 878,900 gallons.
Mount Pleasant Street Detention Basin expansion project | PDF
The county has been looking to expand the detention basin for several years, ever since the county bought additional nearby land in 2017.
"It's taken us eight years, but we're finally getting it done," Stark County Commissioner Richard Regula said at a recent county commissioners meeting.
The upcoming expansion will include deepening the existing basin an additional 3 to 4 feet and expanding it to the south.
'A small additional cost to make a big impact'
Stark County is designated as an area that has impaired streams with high pollution. Because of this, the EPA has required the county to convert a water quantity basin into a water quality basin.
Since the county was already interested in expanding the Mount Pleasant one, Subdivision Engineer Curtis Bungard approached commissioners about doing the conversion at the same time.
"[The EPA] said we only have to do this for a one-acre site, but we're choosing this large site that treats over 300 acres," he said. "When I learned that the commissioners were doing this big expansion project anyhow, so there's already a big investment ... It is a small additional cost to make a big impact, so it was just good timing."
Crews will excavate, allowing storm water sediments to settle to the bottom before the water drains from the basin, improving water quality downstream. The areas will also have plants to help with water filtration.
The county plans to adjust how fast water leaves the basin, ensuring there is enough drainage to improve flooding but also enough time for sediments to settle.
In the past, heavy rains and debris caused the basin to overflow, Bungard said.
"There was one event a year or so ago where these [openings] got clogged up with some construction debris, and the whole basin filled up and went over the road," he said.
The contract was awarded to United Earthworks of Deerfield. Bungard said the county was able to get a favorable bid significantly under the estimate.
"We knew that there was going to be a wide range of bids depending on how close the bidder could take the soil, and this bidder happened to have a site that was within, he says, one minute of driving," he said at a recent commissioners meeting.
The county engineer estimated the project to cost $2,194,951. United Earthworks was awarded the contract at $1,585,860.
The project will be funded through a tax increment financing agreement (TIF). The TIF was established during a 2016 renovation for the Venue at Belden commercial plaza at Everhard Road and Whipple Avenue NW. The county TIF allowed the commissioners to collect 75% of the taxes from the improved value of the property for 10 years. The money was set aside for road improvements or drainage improvements in the area.
The site is downstream from the basin, so the commissioners are able to use the TIF funding on the project.
Reach Grace at 330-580-8364 or [email protected]. Follow her on X @GraceSpringer16.
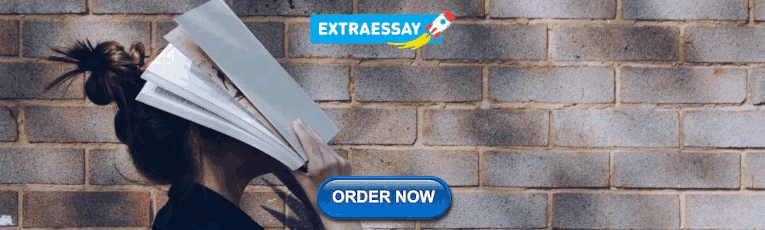
IMAGES
VIDEO
COMMENTS
Water quality is expressed in several parameters, including physical parameters such as turbidity, temperature, and dissolved solids, chemical parameters such as metal levels, pH, and dissolved ...
There are three types of water quality parameters physical, chemical, and biological [8, 9]. They are summarized in Table 1. 3.1 Physical parameters of water quality 3.1.1 Turbidity Turbidity is the cloudiness of water [10]. It is a measure of the ability of light to pass through water. It is caused by suspended material such as clay, silt, organic
water quality assessment. It then goes on to describe the different approaches and methods that can be used to monitor water quality in rivers, lakes and reservoirs and gives some examples of typical water quality assessments in these environments. It is strongly recommended that this quidebook is read
Learn how to monitor and assess groundwater quality from this UNEP guidebook, covering its features, availability and chemical aspects.
Water Quality Parameters and Standards. Browse Course Material Syllabus Calendar Readings Lecture Notes Assignments ... l02_water_qualit.pdf Download File DOWNLOAD. Course Info Instructor ... assignment_turned_in Problem Sets with Solutions. grading Exams with Solutions.
Assessment of Water Quality Parameters: A Review. S. Gorde, M. Jadhav. Published 2013. Environmental Science. Water is the most important in shaping the land and regulating the climate. It is one of the most important compounds that profoundly influence life. The quality of water usually described according to its physical, chemical and ...
PDF-1.6 %âãÏÓ 13702 0 obj >stream 1 0 [`
The main water quality parameters that are measured directly in natural water are pH, dissolved oxygen (DO) concentration, redox potential, turbidity, salinity, temperature, and stream flow. Laser diffractometry is based on the in situ analysis of the diffraction pattern, which is obtained from a laser beam passing through the particles ...
Definition (40 CFR 131.3(b)): "Elements of State water quality standards, expressed as constituent concentrations, levels or narrative statements, representing water quality that supports a particular designated use. When criteria are met, water quality will generally protect the designated use.".
Keywords Water quality index · Water quality parameters · Model accuracy · Aggregation method Introduction Drinking water deterioration is becoming a global concern due to widespread human activities []. However, humans 1 and nature are responsible for the decline of surface and ground water quality (WQ) [2 ]. Natural factors like lithologi-
Introduction. Water quality refers to the chemical, physical, biological, and radiological characteristics of water. It is a measure of the condition of water relative to the requirements of one or more biotic species, or to any human need or purpose. Human activities that involve urbanization, agricultural development, over use of fertilizers ...
The water quality parameter factsheets were developed to provide an introduction to monitoring common parameters; Temperature, Dissolved Oxygen, pH, Turbidity, Macroinvertebrates, E. coli, Nutrients, Habitat Assessment and Metals. They are particularly useful for training new water quality monitoring staff and explaining water quality sampling ...
Water is a vital natural resource for human survival as well as an efficient tool of economic development. Drinking water quality is a global issue, with contaminated unimproved water sources and inadequate sanitation practices causing human diseases (Gorchev & Ozolins, 1984; Prüss-Ustün et al., 2019).Approximately 2 billion people consume water that has been tainted with feces ().
The water quality index (WQI) is a crucial tool in environmental monitoring, offering a comprehensive evaluation of water quality. This index transforms a variety of parameters into a single numerical value, thereby facilitating the classification of water samples into distinct safety levels ( Tasneem and Abbasi, 2012, Sutadian et al., 2016 ...
3.3 Water quality parameters included in water quality assessment 5 3.3.1 pH 5 3.3.2 Conductivity 5 3.3.3 Alkalinity 6 3.3.4 Phosphorus 6 3.3.5 Nitrogen 6 ... to project local and lake wide water quality conditions over the next twenty years. A set of management tools, including a near shore water ...
variations of selected water parameters and identify the pollution sources dam. The physical and chemical parameters were analyzed as per APHA revealed that there were fewer variations in the physicochemical, ionic, heavy metals analysis of the present water quality parameters undertaken and results received through
Download PDF. Article; ... From the analysis of the different water quality parameters, it was found that all the calculated values surpass the permissible limits suggested by WHO & BIS 38,39 ...
pH is one of the most important parameters of water quality. It is defined as the negative logarithm of the hydrogen ion concentration [ 9, 12 ]. It is a dimensionless number indicating the strength of an acidic or a basic solution [ 23 ]. Actually, pH of water is a measure of how acidic/basic water is [ 19, 20 ].
involved in this project as an international student and inspired me greatly to work and study on my topic. His willingness to motivate me and my colleagues contributed to our projects. ... Table 3.5, Water quality test parameters for produced water reusing ..... 52 Table 3.6, Costs of certified labs in CO..... 53 Table 3.7, Water parameters ...
Guidance Laboratories - UNECE
The project, which will begin soon, will expand the basin by one-third and convert it into a water quality basin. The basin has capacity to hold 14,388,592 gallons of water. The expansion will add ...