Pokemon GO Starry Skies, Help Research Daytime or Nighttime Pokemon Choice
Home » Pokemon Go » Pokemon GO Starry Skies, Help Research Daytime or Nighttime Pokemon Choice
In our Pokemon GO Starry Skies, Help Research Daytime or Nighttime Pokemon Choice guide, we are going to give you the list of tasks in each of the two “branches” of the 2023 Solstice Horizons event. And, of course, we will also show you the rewards you are going to get depending on your Starry Skies choice. There’s a lot to get into, so let’s jump straight in.
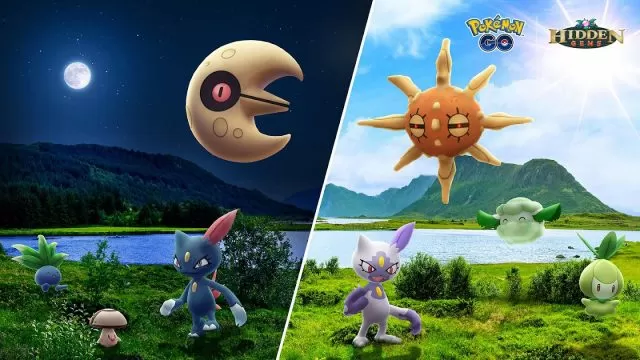
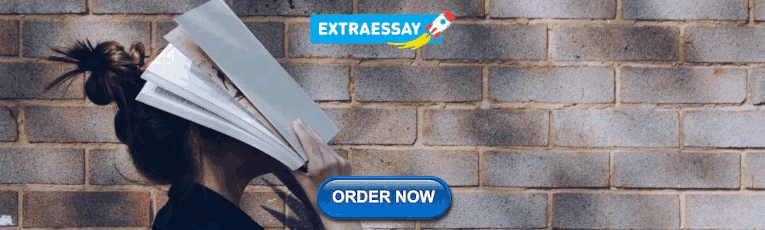
Pokemon GO Starry Skies Choice, Help Research Daytime or Nighttime Pokemon
The choice between helping research daytime or nighttime Pokemon in Pokemon GO Starry Skies really only affects the tasks that you’ll have to do. The rewards remain the same no matter which route you choose to follow. So, the best choice for you is whichever one has the tasks you prefer. First off, here’s the list of quests you’ll need to do if you go the daytime route, and what the rewards are.
- Catch 20 Grass- or Bug-type Pokemon – 10x Poke Balls
- Explore 10 km – Encounter with Inkay
- Spin 25 PokeStops or Gyms – 10x Pinap Berries
- Rewards for completing every step: Encounter with Galarian Slowpoke, 15x Poke Balls, 78x Stardust
- Make 10 Curveball Throws in a row – 3000x Stardust
- Catch 50 Pokemon with Weather Boost – Encounter with Metang
- Earn 10 Candies walking with your buddy – 15x Great Ball
- Rewards for completing every step: Encounter with Starmie, 1x Mossy Lure Module, 789x Stardust
- Hatch 5 Eggs – 5x Silver Pinap Berries
- Catch 50 Psychic-type Pokemon – Encounter with Alakazam
- Earn 7,890 Stardust – 1x Star Piece
- Rewards for completing every step: Encounter with Cosmog, 20x Ultra Balls, 7890x Stardust
So, those are the daytime tasks and rewards in the Pokemon GO Starry Skies Help Research Daytime or Nighttime choice. Now for the nighttime tasks. As we’ve said, the rewards are exactly the same; you’re not going to miss put on anything regardless of what you choose.
- Catch 20 Ghost- or Dark-type Pokemon – 10x Poke Balls
- Take snapshots of 20 different wild Pokemon – Encounter with Inkay
- Use 30 Berries to help catch Pokemon – 10x Pinap Berries
- Make 5 Excellent Throws – 3000x Stardust
- Catch 30 different species of Pokemon – Encounter with Metang
- Complete 15 Field Research tasks – 15x Great Ball
- Win 5 raids – 5x Silver Pinap Berries
YOU MAY ALSO READ
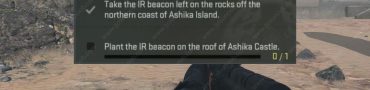
Leave a Reply Cancel reply
Your email address will not be published. Required fields are marked *
Save my name, email, and website in this browser for the next time I comment.
Follow Polygon online:
- Follow Polygon on Facebook
- Follow Polygon on Youtube
- Follow Polygon on Instagram
Site search
- Dragon’s Dogma 2
- Ghost of Tsushima
- Zelda: Tears of the Kingdom
- Baldur’s Gate 3
- GTA 5 cheats
- PlayStation
- Dungeons & Dragons
- Magic: The Gathering
- Board Games
- All Tabletop
- All Entertainment
- What to Watch
- What to Play
- Buyer’s Guides
- Really Bad Chess
- All Puzzles
Filed under:
- Pokémon Go guide
Pokémon Go ‘Solstice Horizons’ event, ‘Starry Skies’ Special Research guide
Shiny Fomantis makes its debut
Share this story
- Share this on Facebook
- Share this on Reddit
- Share All sharing options
Share All sharing options for: Pokémon Go ‘Solstice Horizons’ event, ‘Starry Skies’ Special Research guide
/cdn.vox-cdn.com/uploads/chorus_image/image/72376771/unnamed__1_.0.jpg)
The Pokémon Go “ Solstice Horizons ” event is a limited-time celebration of Pokémon that specifically love the day and night. It runs from June 16-25 .
The event features special Pokémon spawning at differing times of day, 2X Stardust for catching Pokémon, and Lunatone and Solrock spawning regardless of your location. (Both of these Pokémon are usually migratory and regional Pokémon .)
Notably, Fomantis is making its shiny debut, so lucky players will be able to find one of these critters.
:no_upscale()/cdn.vox-cdn.com/uploads/chorus_asset/file/24727249/jlee_230614_1001_pogo_shiny_fomantis_lurantis.jpg)
There will also be a Team Go Rocket portion of this event, but it doesn’t start until June 21.
Below, we list out all the event perks and bonuses, including the Special Research and Collection Challenge.
Pokémon Go ‘Starry Skies’ Special Research and rewards
This set of Special Research can be repeated if you pay $4.99 to get more Cosmog , if you want. It will initially be available free to any player who logs in and starts it by June 25.
Step 1 of 4
- Explore 2 km (Solosis)
- Catch 2 Psychic-type Pokémon (Abra)
- Catch 10 different species of Pokémon (Gothita)
Rewards : 5 Razz Berries, 7 Stardust, Wobbuffet encounter
After completing step 1 of the Starry Skies Special Research, you’ll have the option to choose between researching Daytime Pokémon or Nighttime Pokémon . Depending on which path you choose, you’ll have different research tasks but (mostly) the same rewards.
☼ Help Research Daytime Pokémon tasks
Step 2 of 4.
- Catch 20 Grass- or Bug-type Pokémon (10 Poké Balls)
- Explore 10 km (Inkay)
- Spin 25 PokéStops or Gyms (10 Pinap Berries)
Rewards : 15 Poké Balls, 78 Stardust, Galarian Slowpoke encounter
Step 3 of 4
- Make 10 Curveball Throws in a row (3,000 Stardust)
- Catch 50 Pokémon with weather boost (Metang)
- Earn 10 Candies walking with your buddy (15 Great Balls)
Rewards : 1 Mossy Lure Module, 789 Stardust, Starmie encounter
Step 4 of 4
- Hatch 5 eggs (3 Silver Pinap Berries)
- Catch 50 Psychic-type Pokémon (Alakazam)
- Earn 7,890 Stardust (1 Star Piece)
Rewards : 20 Ultra Balls, 7,890 Stardust, Cosmog encounter
☾ Help Research Nighttime Pokémon tasks
- Catch 20 Ghost- or Dark-type Pokémon (10 Poké Balls)
- Take snapshots of 20 different wild Pokémon (Inkay)
- Use 30 Berries to help catch Pokémon (10 Pinap Berries)
- Make 5 Excellent throws (3,000 Stardust)
- Catch 30 different species of Pokémon (Metang)
- Complete 15 Field Research tasks (15 Great Balls)
Rewards : 1 Glacial Lure Module, 789 Stardust, Starmie encounter
- Win 5 Raids (3 Silver Pinap Berries)
Pokémon Go ‘Solstice Horizons’ Collection Challenge: Daytime
Catch the following to get rewards:
Rewards: 2,000 Stardust, Fomantis encounter
Pokémon Go ‘Solstice Horizons’ Collection Challenge: Nighttime
Pokémon go ‘solstice horizons’ event field research and rewards, pokémon go ‘solstice horizons’ boosted spawns.
- Ledyba (daytime)
- Murkrow (daytime)
- Solrock (daytime)
- Drifloon (daytime)
- Purrloin (daytime)
- Sewaddle (daytime)
- Cottonee (daytime)
- Petilil (daytime)
- Hisuian Sneasel (daytime)
- Oddish (nighttime)
- Spinarak (nighttime)
- Misdreavus (nighttime)
- Lunatone (nighttime)
- Stunky (nighttime)
- Venipede (nighttime)
- Foongus (nighttime)
- Phantump (nighttime)
- Sneasel (nighttime)
- Fomantis (both daytime and nighttime)
Pokémon Go ‘Solstice Horizons’ raid targets
- Hisuian Sneasel
- Mega Sceptile

The next level of puzzles.
Take a break from your day by playing a puzzle or two! We’ve got SpellTower, Typeshift, crosswords, and more.
Sign up for the newsletter Patch Notes
A weekly roundup of the best things from Polygon
Just one more thing!
Please check your email to find a confirmation email, and follow the steps to confirm your humanity.
Oops. Something went wrong. Please enter a valid email and try again.
Loading comments...

Dune 2, The Fall Guy, Netflix’s Atlas, and every movie new to streaming this week

The best deals you should check out this Memorial Day weekend

Never mind Kojima’s Mad Max game — I want George Miller’s Metal Gear

The best echoes in Wuthering Waves and where to get them

Schedule a Gamer Night — it’s life-changing

Fortnite Chapter 5 Season 3 Medallion guide
Pokemon GO Starry Skies Best Path to Choose – Daytime or Nighttime Pokemon
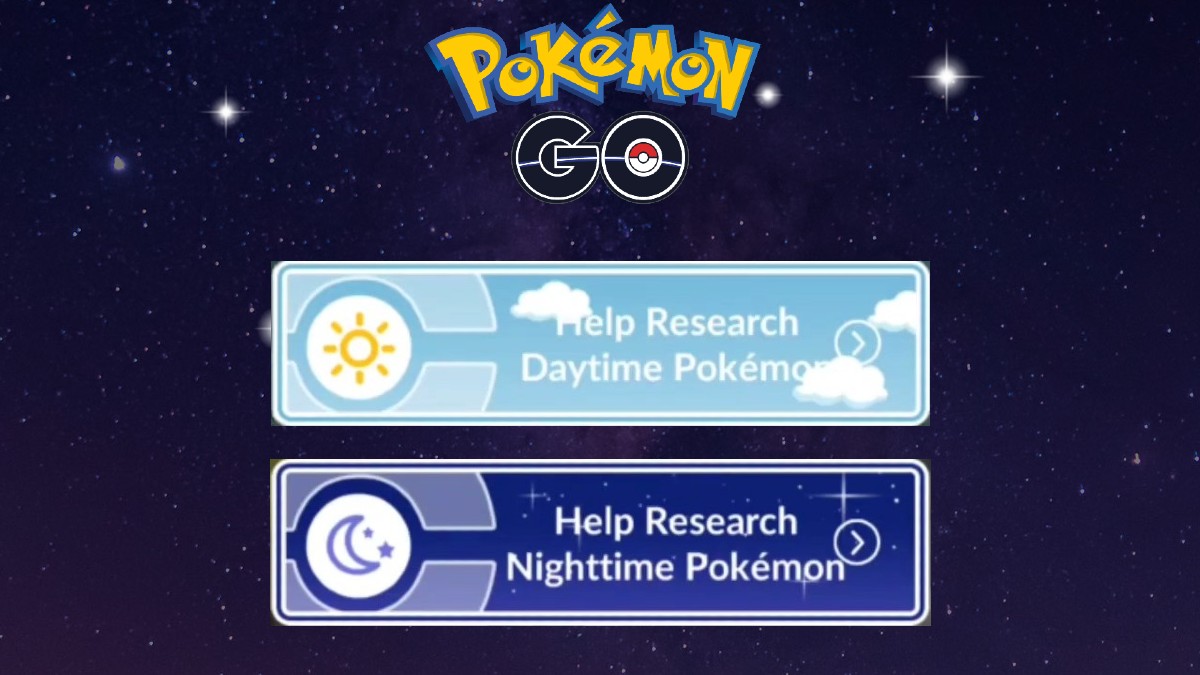
The Pokemon GO Starry Skies Special Research Story has two different paths to choose – Daytime Pokemon or Nighttime Pokemon. We’ve gone through the Research to tell you which of these paths is best to choose and why!
The best path in the Pokemon GO Starry Skies Research depends on when you play the game. If you play more during the day, choose Daytime; if you play more during the night, choose Nighttime.
This is because the rewards for both paths are exactly the same, including the much-requested second Cosmog encounter !
Which path you choose only changes the tasks and not the rewards you get. This means you can pick a path without any worry.

The tasks for the Daytime Path include catching Grass and Bug-type Pokemon, spinning PokeStops, and hatching Eggs. Meanwhile, the Nighttime Path requires catching Ghost or Dark-type Pokemon, completing Field Research, and winning Raids.
Of course, it is easier to find Grass and Bug-types during the day and Ghost and Dark-type Pokemon at night, but the rest of the tasks within this Special Research can be completed at any time.
If you still can’t decide which path to choose, here are all of the Tasks and rewards for Starry Skies Special Research !
Or, refer to the table below to see the differences in Tasks and choose whichever path you think will be easier.

All Pokemon GO Starry Skies Path Differences – Daytime vs. Nighttime
Step 2 and 3 of the Pokemon GO Starry Skies Research have completely different tasks depending on what path you take, while Step 4 has one different task:
Once you’ve completed the Starry Skies Special Research, you’ll encounter Cosmog. Here’s how to evolve Cosmog into Solgaleo and Lunala Pokemon GO .
Pokémon Go Starry Skies quest steps, best Choose Path and rewards
How to catch Cosmog through this special research quest.
Starry Skies is a special research quest which will allow you to catch a Cosmog in Pokémon Go .
Released on Friday 16th June as part of the Solstice Horizons event, this quest offers you the chance to catch a Cosmog, which you can eventually evolve into a Solgaleo or Lunala . This is perfect for players who wish to complete the Gen 7 Pokédex in Pokémon Go and currently only have one of these legendary Pokémon in their Pokédex.
Since Starry Skies is also a branching quest, it’s a good idea to know which Choose Path choice is best for you .
Below you’ll find the Starry Skies quest steps in Pokémon Go to help you complete this quest and add another Pokémon to your Alolan Pokédex.
On this page:
- 'Starry Skies' quest steps
- Daytime Path quest steps
- Nighttime Path quest steps
- Starry Skies best Choose Path
How does Starry Skies work in Pokémon Go
How to purchase starry skies in pokémon go, 'starry skies' quest steps in pokémon go.
Below you’ll find all of the Starry Skies quest steps in Pokémon Go. Keep in mind you must be above Level 5 to unlock this quest.
To access the Starry Skies quest, you must play Pokémon Go before Sunday 25th June at 8pm (local time) . You can also purchase an additional run through for the quest before this date, which you can learn more about in the sections below.
Since this is a special research quest, once it's been unlocked you can complete it at your own pace.
Starry Skies is also a branching quest, which means, after completing the first step, you’ll be asked to choose between the Daytime Path or the Nighttime Path. This choice will determine a number of the challenges you’ll face and one of the rewards you’ll receive. Both of these paths, however, result in a Cosmog encounter.
Be warned - this section does contain spoilers !
Thank you to Amiibofan101 from reddit for the help with this information!
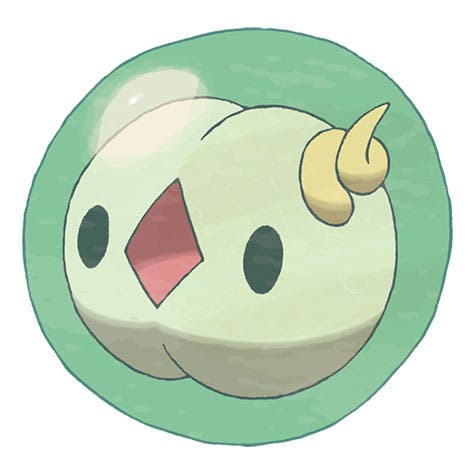
'Starry Skies' Step 1 of 4
- Explore 2km - Solosis encounter
- Catch 2 Psychic-type Pokémon - Abra encounter
- Catch 10 different species of Pokémon - Gothita encounter
Rewards: 7 Stardust, 5 Razz Berries and a Wobbuffet encounter.
You’ll be asked to choose between the Daytime Path or the Nighttime Path after completing this step. Choose carefully because you can’t change your choice after it's made and it will determine some of the challenges you face.
The following sections will outline the quest steps and rewards for both the Daytime and Nighttime Path.
Daytime Path quest steps in Pokémon Go
Below you’ll find the challenges and rewards for the Daytime Path of the Starry Skies special research quest in Pokémon Go:
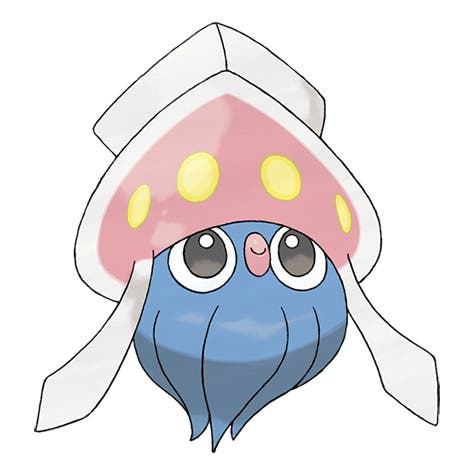
'Starry Skies' Step 2 of 4
- Catch 20 Grass or Bug-type Pokémon - 10 Poké Balls
- Explore 10km - Inkay encounter
- Spin 25 PokéStops or Gyms - 10 Pinap Berries
Rewards: 78 Stardust, 15 Poké Balls and a Galarian Slowpoke encounter.
'Starry Skies' Step 3 of 4
- Make 10 Curveball Throws in a row - 3000 Stardust
- Catch 50 Pokémon with Weather Boost - Metang encounter
- Earn 10 Candies walking with your buddy - 15 Great Ball
Rewards: 789 Stardust, 1 Mossy Lure Module and a Starmie encounter.
'Starry Skies' Step 4 of 4
- Hatch 5 Eggs - 3 Silver Pinap Berries
- Catch 50 Psychic- type Pokémon - Alakazam encounter
- Earn 7890 Stardust - 1 Star Piece
Rewards: 7890 Stardust, 20 Ultra Balls and a Cosmog encounter.
Nighttime Path quest steps in Pokémon Go
Here are the challenges and rewards for the Nighttime Path of the Starry Skies special research quest in Pokémon Go:
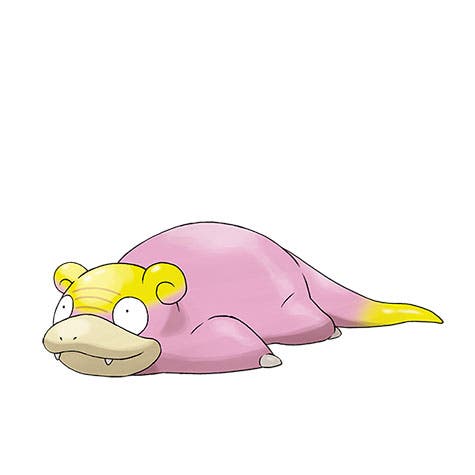
- Catch 20 Ghost or Dark-type Pokémon - 10 Poké Balls
- Take snapshot of 20 different wild Pokémon - Inkay encounter
- Use 30 Berries to help catch Pokémon - 10 Pinap Berries
- Make 5 Excellent Throws - 3000 Stardust
- Catch 30 different species of Pokémon - Metang encounter
- Complete 15 Field Research tasks - 15 Great Balls
Rewards: 789 Stardust, 1 Glacial Lure Module and a Starmie encounter.
- Win 5 raids - 3 Silver Pinap Berries
- Catch 50 Psychic-type Pokémon - Alakazam encounter
Starry Skies best Choose Path in Pokémon Go
After completing Step 1 of Starry Skies in Pokémon Go, you’ll be asked to pick between either the Daytime or Nighttime Path. Afterwards, a number of the challenges you face, along with one of the rewards, will depend on your choice path. Due to this, it’s important you choose your path carefully, especially since you can’t change paths .
Which path is best for you, however, depends on your Pokémon Go playstyle and what you like to do in the game.
Here’s a quick summary of the differences between the two paths:
- Daytime - Challenges relating to distance walked, buddy Pokémon , Curveball Throws and catching Pokémon, with a specific task focused on Grass and Bug-type Pokémon. Includes a Mossy Lure Module as a reward.
- Nighttime - Challenges relating to raids , field research tasks , Excellent Throws, taking snapshots and catching either Dark or Ghost-type Pokémon. Includes a Glacial Lure Module as a reward.
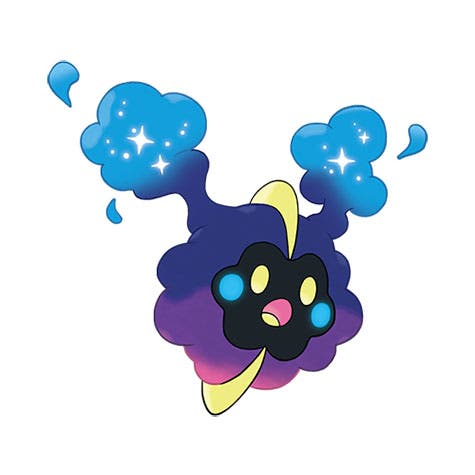
As you can see both from the summary and quest step sections above, both Starry Skies paths are designed to take you a good amount of time. For this reason, we recommend reading over the specific quest step sections for each Path before making your decision to ensure you pick the right one.
It can be argued that the Nighttime Path is slightly quicker to complete, since it doesn’t involve any walking related challenges, but you would need to use Premium or Remote Raid Passes to complete the raid-related challenge in one day. If you do choose this path, we recommend completing the first challenge in Step 2 - Catch 20 Ghost or Dark-type Pokémon - during the Solstice Horizons event, because Pokémon for both of these types will be spawning more frequently at night.
The Daytime Path may take you longer to complete - thanks to requiring you to walk 10km for one challenge, an egg-themed challenge and having two 'Catch 50 Pokémon' tasks. Yet, if you’re like me and are not very good at making Excellent Throws, you may find it easier to complete. It also doesn’t require you to spend any Raid Passes.
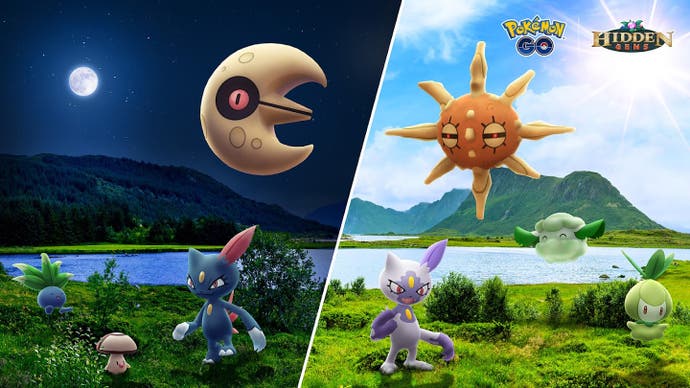
Keep in mind that the only reward change is the Lure you’ll receive. This means, if you really want either a Mossy or Glacial Lure Module but don’t want to pay for it, you should choose your path around that.
Personally, I will be choosing the Daytime Path because it better suits my playstyle of walking around, catching Pokémon and only partaking in one raid per week. (Just don’t feel like doing more than one a week at the moment…)
Starry Skies is a special research quest you can unlock by playing Pokémon Go at least once before Sunday 25th June at 8pm (local time) if you’re above Level 5. All you have to do is play Pokémon Go once to unlock the quest and then you’ll be able to complete it at your own pace.
You can also purchase an additional ticket for the quest for £4.99, $5 or the equivalent in your local currency until Sunday 25th June at 8pm (local time). This will allow you to play through the quest for a second time and, in doing so, catch an additional Cosmog.
If you do purchase this, make sure you log into Pokémon Go before Sunday 25th June at 8pm (local time) to ensure that it's been unlocked properly.
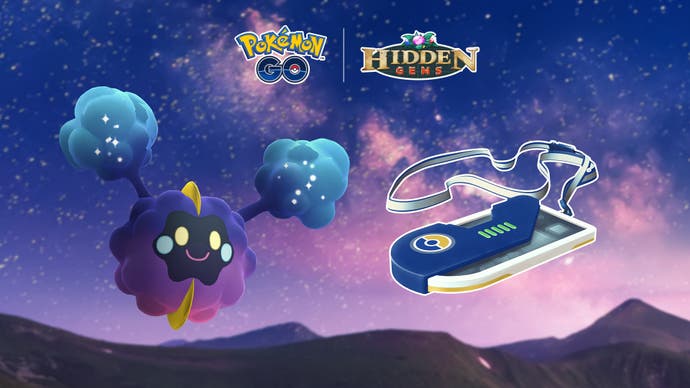
Starry Skies works mostly the same as any other special research quest in Pokémon Go - challenging you to complete a set of challenges to both progress the quest and earn rewards. Once a quest step is completed, you can earn a branch of rewards before moving onto the next until the quest is complete.
The only difference between Starry Skies and most other special research quests is that it’s a branching quest, which means, after Step 1, you will need to pick between one of two paths - Daytime or Nighttime. Your choice will determine some of the challenges you face and one the rewards. It’s important to remember that you can’t change your mind after selecting a path.
The World of Wonders Season is coming to an end in Pokémon Go. Enjoy the last event - Ultra Space Wonders - and complete the final stage of World of Wonders , so you can evolve Poipole into Naganadel! You can also work on the Masterwork Research: Catching Wonders quest for a Master Ball and Glitz and Glam quest for a Diancie. Don't forget to try out Routes , Gift Exchange and Party Play while you're hunting down rare Pokémon , fighting in the Go Battle League or competing in PokéStop Showcases .
You can purchase an additional ticket for the Starry Skies quest in Pokémon Go, which will allow you to replay the quest, until Sunday 25th June at 8pm (local time).
The quest costs £4.99, $5 or the equivalent in your local currency.
To purchase a ticket, visit the in-game Pokémon Go store, which is accessed via the game’s main menu. From there, you simply need to find the banner that advertises the quest.
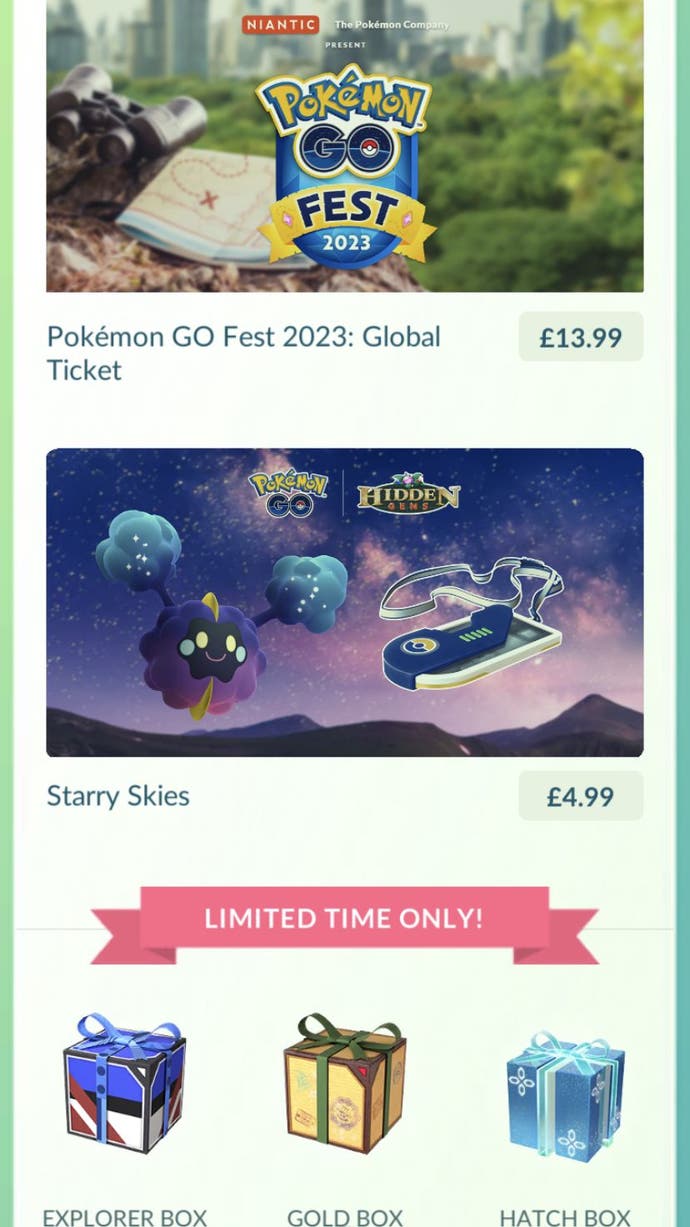
Selecting this banner will bring up more information about the quest and, to purchase it, select the 'Buy' option before following the purchase instructions specific for your device.
The quest should be available from the moment you purchase it, which means you can start working towards a third Cosmog straight away. Just make sure the quest is unlocked before Sunday 25th June at 8pm (local time).
Good luck completing the Starry Skies quest!
Read this next
- Pokémon Go plans to introduce item sharing within Parties
- Pokémon Go developer taking feedback after avatar update backlash
- Antarctic scientists get base added to Pokémon Go
Wuthering Waves Tempest Mephis location
Coin Master free spins May 2024
Grimoires Era codes
Today's Wordle hint and answer on Saturday 25th May for 1071
Today's Strands hints and answers for Saturday 25th May
Today’s Connections hint and answer on 25th May for 349
Genshin Impact 4.7 release date and 4.7 Banners and events
How to beat Ringmaster Scarr in Fortnite

'Pokémon GO' Starry Skies Questline Has Two Unique Paths — Should You Go Day or Night?
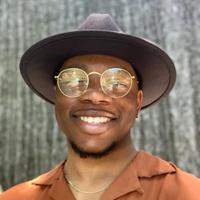
Jun. 22 2023, Published 3:12 p.m. ET
Kicking off on June 16, the ongoing Solstice Horizons event in Pokémon GO comes stacked with new challenges and rewards for Trainers to cut their teeth on until June 25 at 8 p.m. local time.
The event features boost encounter rates with day and night-specific Pokémon like Murkrow and Misdreavus and recently returned battles against Shadow Pokémon used by Team GO Rocket in the mobile AR game.
Another substantial addition that debuted with Solstice Horizons is the Starry Skies research questline starring Cosmog , a Psychic-type Legendary Pokémon introduced in Gen. VII.
Trainers must choose a day or night path to complete the quest during the event, each having different challenges respective to the time of day and focused on specific activities. Once you decide which quest path to take, you'll get locked into that side of Starry Skies.
Choosing the proper pathway for yourself based on what you can accomplish during the event timeline is critical. Here's a breakdown of the day and night path for Starry Skies in Pokémon GO and which one you should choose.
'Pokémon GO' Starry Skies quest day and night paths explained:
As long as you're above Level 5 in Pokémon GO , you can unlock the Starry Skies quest. After doing the first of four steps to complete the unique research quest, you can choose to branch into the Daytime or Nighttime Path.
Thankfully, both sides will ultimately lead to a battle encounter with Cosmog. The final quest rewards of 7890 Stardust and 20 Ultra Balls are also the same. Their key differences are the challenges and activities of steps 2-4 before the Cosmog encounter.
The Daytime Path features challenges related to distance walked, buddy Pokémon , curveball throws, and catching specific Grass and Bug-type Pokémon.
On the flip side, Nighttime focuses on raids, field research tasks, excellent throws, taking snapshots , and catching Dark and Ghost-type Pokémon.
Aside from the Glacial Lure and Mossy Lure Modules rewarded for step three on their respective paths, Daytime and Nighttime are distinguished by challenges and Pokémon encounters.
🇮🇳 🌟 Starry Skies (Day and Night Paths) - Cosmog Encounter 💫 Special Research 🗓️ Friday, June 16 2023 🕙 10:00 AM local time onwards #PokemonGO #Pokemon #Cosmog #SpecialResearch #Solgaleo #Lunala #infographics #Cosmoem pic.twitter.com/Mnju25lptW — Avinash Rayambaram (@rayambaram) June 16, 2023
So, should you go day or night for the 'Pokémon GO' Starry Skies quest?
Depending on the Lure you want, that may sway you to go either Daytime or Nighttime for the Starry Skies quest, but ultimately no one path is the best since the final quest prize is the same.
Daytime requires walking, has egg challenges, and doesn't spend your Raid Passes if you want to keep them. But if you're in the mood for tackling raids and scooping up Pokémon in the evening, Nighttime might be up your alley.
Choose the path that works best for you during your daily habits, but be careful and think about it before being restricted to one side.
Yes, You Can Play 'Diablo IV' on Mobile — Here’s What You Need to Know
Nintendo Revealed a New Princess Peach Game for Switch During Latest Nintendo Direct
Girlfriend Cries Over Partner Playing Video Games During Couple's Vacation
Latest Pokémon News and Updates
- ABOUT Distractify
- Privacy Policy
- Terms of Use
- CONNECT with Distractify
- Link to Facebook
- Link to Instagram
- Contact us by Email

Opt-out of personalized ads
© Copyright 2024 Distractify. Distractify is a registered trademark. All Rights Reserved. People may receive compensation for some links to products and services on this website. Offers may be subject to change without notice.
- World of Warcraft
- Baldur's Gate
- League of Legends
- Counter-Strike
- Ethics Policy
- Ownership Policy
- Fact Checking Policy
- Corrections Policy
- Affiliate Policy
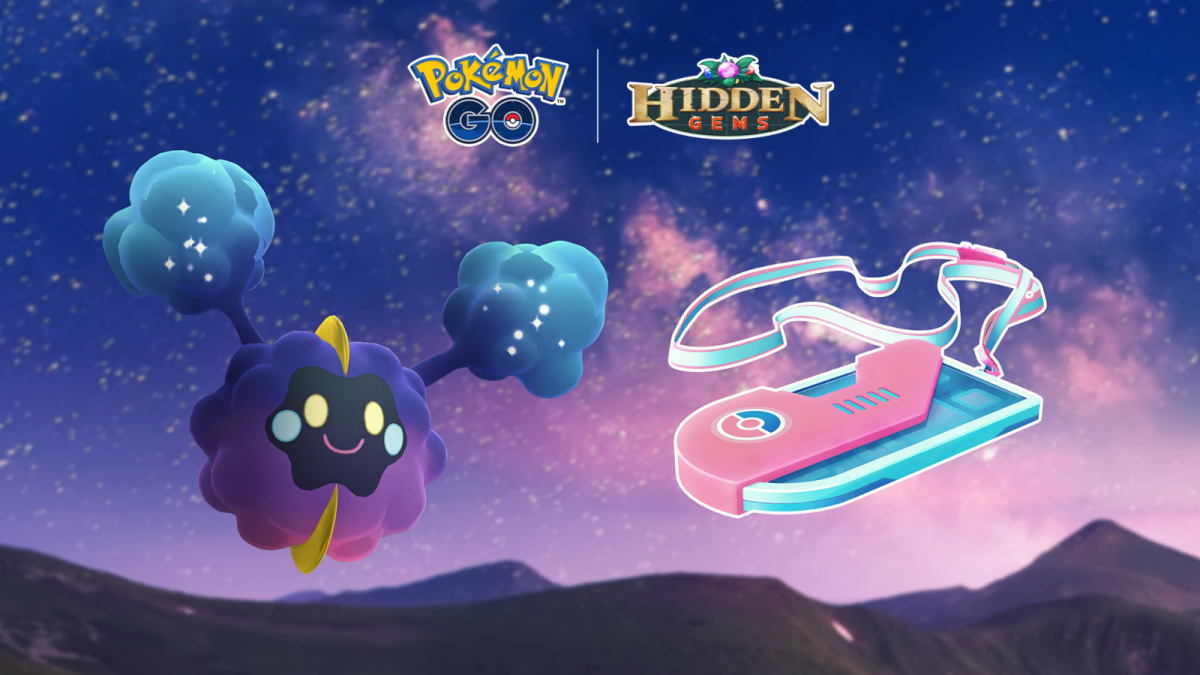
All Solstice Horizons Starry Skies Research tasks and rewards in Pokémon Go

Pokémon Go’s Solstice Horizons event is here, which means players around the world are going to see entirely different worlds in the game depending on when they play. And don’t forget there are new Research tasks and rewards to complete no matter the time of day.
OK, that might be a bit of an overstatement, but different Pokémon will spawn during the day than at night while this event is live from June 16 to 25. These rotating spawns include Hisuian Sneasel and Sewaddle during the day and Sneasel and Phantump at night. Certain Pokémon like Fomantis will appear during both periods of the day.
Players will get double Stardust for catching Pokémon and see Lunatone and Solrock spawning around the world throughout the event. This is also the first big event Niantic is tying into the new Pokémon Go Web Store, releasing a special Solstice Box early there before it is added to the in-game shop.
For anyone who wants a little bit extra out of Solistice Horizons, the Starry Skies Special Research story is available to purchase for $5.
This will give players a chance to catch another Cosmog, the first time this has happened since the Pokémon was introduced through Special Research last September. You can also complete it at any time if you choose to purchase it.
Here are all of the research tasks and rewards you will see along the way.
All Starry Skies Special Research tasks and rewards in Pokémon Go’s Solstice Horizons event
Starry skies page one.
- Solosis encounter
- Abra encounter
- Gothita encounter
Total reward: Wobbuffet encounter, five Razz Berries, and seven Stardust
Branched Choice: Daytime or Nighttime
Because this is a paid Special Research story for an event that focuses on two separate spawn cycles, players will get to pick their preferred time of day as a branched path once they complete the first page of Starry Skies research.
Related: Pokémon Go players can once more get their hands on a highly coveted Legendary during Solstice Horizons event
This choice really doesn’t impact much of anything, only altering a few of the research tasks you will have to complete. The daytime option focuses more on exploration while the nighttime path has catching and raid challenges.
Here are all the differences.
Starry Skies: Research Daytime Pokémon page two
- 10 Poké Balls
- Inkay encounter
- 10 Pinap Berries
Total reward: Galarian Slowpoke encounter, 15 Poké Balls, and 78 Stardust
Starry Skies: Research Daytime Pokémon page three
- 3,000 Stardust
- Metang encounter
- 15 Great Balls
Total reward: Starmie encounter, one Mossy Lure Module, and 789 Stardust
Starry Skies: Research Daytime Pokémon page four
- Three Silver Pinap Berries
- Alakazam encounter
- One Star Piece
Total reward: Cosmog encounter, 20 Ultra Balls, and 7,890 Stardust
Starry Skies: Research Nighttime Pokémon page two
Starry skies: research nighttime pokémon page three.
Total reward: Starmie encounter, one Glacial Lure Module, and 789 Stardust
Starry Skies: Research Nighttime Pokémon page four
All solstice horizons event field research tasks and rewards in pokémon go.
- 500 Stardust
- Fomantis encounter
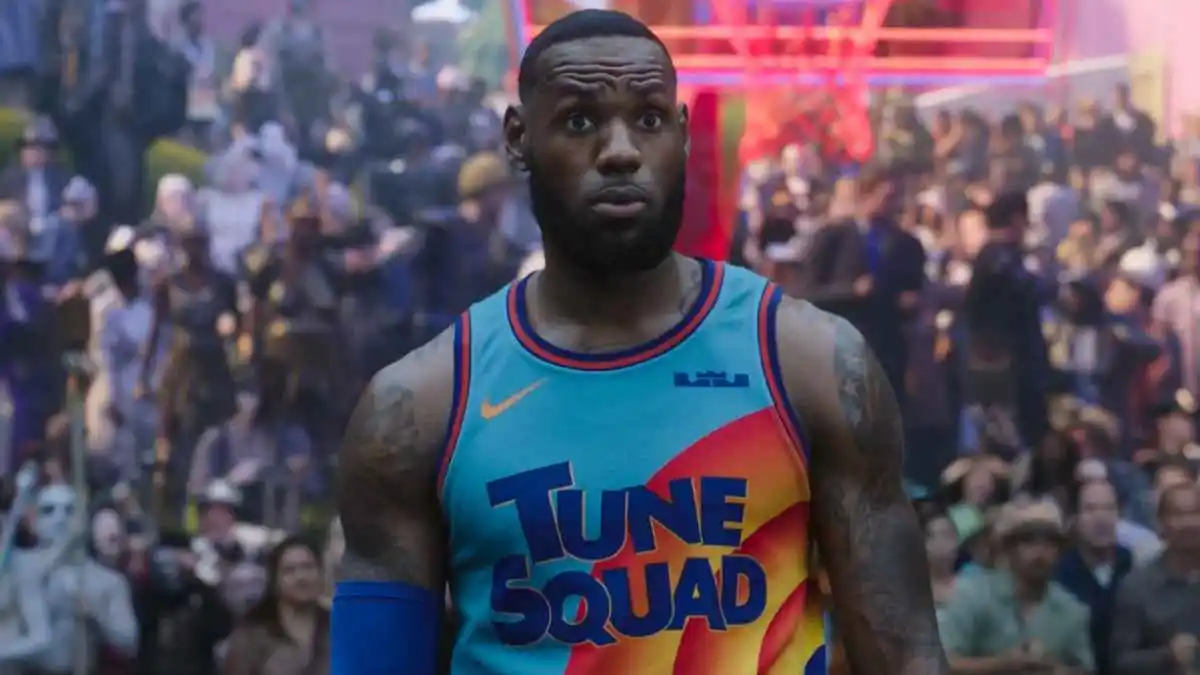

Research Tasks

An official website of the United States government
The .gov means it’s official. Federal government websites often end in .gov or .mil. Before sharing sensitive information, make sure you’re on a federal government site.
The site is secure. The https:// ensures that you are connecting to the official website and that any information you provide is encrypted and transmitted securely.
- Publications
- Account settings
Preview improvements coming to the PMC website in October 2024. Learn More or Try it out now .
- Advanced Search
- Journal List
- v.20(3); 2022 Mar

Recommendations for daytime, evening, and nighttime indoor light exposure to best support physiology, sleep, and wakefulness in healthy adults
Timothy m. brown.
1 Centre for Biological Timing, Faculty of Biology, Medicine and Health, University of Manchester, Manchester, United Kingdom
George C. Brainard
2 Department of Neurology, Thomas Jefferson University, Philadelphia, Pennsylvania, United States of America
Christian Cajochen
3 Centre for Chronobiology, University Psychiatric Clinics Basel, Transfaculty Research Platform Molecular and Cognitive Neurosciences, University of Basel, Basel, Switzerland
Charles A. Czeisler
4 Division of Sleep and Circadian Disorders, Departments of Medicine and Neurology, Brigham and Women’s Hospital, Boston, Massachusetts, United States of America
5 Division of Sleep Medicine, Harvard Medical School, Boston, Massachusetts, United States of America
John P. Hanifin
Steven w. lockley.
6 Surrey Sleep Research Centre, Faculty of Health and Medical Sciences, University of Surrey, Guildford, United Kingdom
Robert J. Lucas
Mirjam münch.
7 Research Centre for Hauora and Health, Massey University, Wellington, New Zealand
John B. O’Hagan
8 Centre for Radiation, Chemical and Environmental Hazards, Public Health England, Chilton, Didcot, United Kingdom
Stuart N. Peirson
9 Sleep and Circadian Neuroscience Institute, Nuffield Department of Clinical Neurosciences, University of Oxford, Oxford, United Kingdom
Luke L. A. Price
Till roenneberg.
10 Institutes for Medical Psychology and Occupational, Social and Environmental Medicine, Medical Faculty, Ludwig-Maximilians University (LMU), Munich, Germany
Luc J. M. Schlangen
11 Human Technology Interaction Group, Department of Industrial Engineering and Innovation Sciences, Eindhoven University of Technology, Eindhoven, the Netherlands
12 Intelligent Lighting Institute, Eindhoven University of Technology, Eindhoven, the Netherlands
Debra J. Skene
13 Chronobiology, Faculty of Health and Medical Sciences, University of Surrey, Guildford, United Kingdom
Manuel Spitschan
14 Translational Sensory & Circadian Neuroscience, Max Planck Institute for Biological Cybernetics, Tübingen, Germany
15 TUM Department of Sport and Health Sciences (TUM SG), Technical University of Munich, Munich, Germany
16 Department of Experimental Psychology, University of Oxford, Oxford, United Kingdom
Céline Vetter
17 Circadian and Sleep Epidemiology Laboratory, Department of Integrative Physiology, University of Colorado Boulder, Boulder, Colorado, United States of America
Phyllis C. Zee
18 Department of Neurology, Northwestern University, Chicago, Illinois, United States of America
19 Center for Circadian and Sleep Medicine, Northwestern University, Chicago, Illinois, United States of America
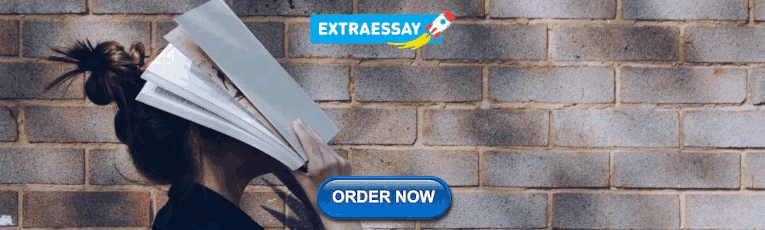
Kenneth P. Wright, Jr
20 Sleep and Chronobiology Laboratory, Department of Integrative Physiology, University of Colorado Boulder, Boulder, Colorado, United States of America
Ocular light exposure has important influences on human health and well-being through modulation of circadian rhythms and sleep, as well as neuroendocrine and cognitive functions. Prevailing patterns of light exposure do not optimally engage these actions for many individuals, but advances in our understanding of the underpinning mechanisms and emerging lighting technologies now present opportunities to adjust lighting to promote optimal physical and mental health and performance. A newly developed, international standard provides a SI-compliant way of quantifying the influence of light on the intrinsically photosensitive, melanopsin-expressing, retinal neurons that mediate these effects. The present report provides recommendations for lighting, based on an expert scientific consensus and expressed in an easily measured quantity (melanopic equivalent daylight illuminance (melaponic EDI)) defined within this standard. The recommendations are supported by detailed analysis of the sensitivity of human circadian, neuroendocrine, and alerting responses to ocular light and provide a straightforward framework to inform lighting design and practice.
Light exposure influences human health and wellbeing by modulating circadian rhythms and sleep. This Consensus View outlines the first expert scientific consensus recommendations for appropriate daily patterns of light exposure to promote health and wellbeing and inform lighting design and practice.
Introduction
Besides supporting visual perception, ocular light exposure influences many aspects of human physiology and behaviour, including circadian rhythms, sleep, and alertness (both via circadian system–dependent and circadian system–independent routes), mood, neuroendocrine, and cognitive functions (reviewed in [ 1 – 4 ]). This array of retinally driven responses to light (collectively termed “non–image-forming” or, as used here for brevity, “nonvisual”) are important determinants of health, well-being, and performance, and some are already clinically relevant, as evidenced by current light therapy for circadian rhythm sleep disorders and various forms of depression [ 5 – 7 ]. Industrialisation and urbanisation have progressively and dramatically altered individuals’ light exposures, resulting in less light, including natural light, during the daytime and less darkness during the night, due to spending more time indoors where electric lighting provides the dominant source of illumination. Substantial evidence indicates that such altered light exposure patterns (and associated circadian/sleep disruption) contribute to negative impacts on health, sleep, and productivity, ranging from acute increases in accident risk to increased incidence of cardiometabolic disorders and forms of cancer (reviewed in [ 8 – 14 ]). Therefore, there is an urgent need for evidence-led recommendations to help inform the design and application of light emission technologies and human light exposures.
To date, a key challenge when optimising light exposure for promoting human health, well-being, and performance has been the lack of an accepted scientific framework upon which to quantify the propensity for light to elicit the relevant responses and from which to base recommendations for lighting design and practice. Fortunately, as a result of several decades of scientific advances, research-based recommendations are now possible.
Building on initial observations that physiological responses to ocular light exposure can persist even in people who are totally visually blind [ 15 – 17 ], convergent evidence from studies of humans and animals has shown that such nonvisual responses (including effects on the circadian system, melatonin secretion, sleep/alertness, and pupil constriction) originate via a specialised class of retinal neurons, the intrinsically photosensitive retinal ganglion cells (ipRGCs) [ 18 – 26 ]. The light-sensing photopigment within the ipRGCs is melanopsin, which, in humans, is maximally sensitive to photons in a distinct portion of the visible spectrum to the cone photopigments ( λ max ≈ 480 nm before accounting for filtering through the lens and ocular media) [ 23 , 25 , 27 ]. As a result, the established photometric quantities used to describe brightness and luminous sensation as perceived by humans do not adequately reflect the spectral sensitivity of any melanopsin-dependent responses to light. Measures such as photopic (il)luminance, which primarily reflect the spectral sensitivity of long and medium wavelength sensitive cones, place substantially greater weight on longer wavelengths than those to which melanopsin is most sensitive. These measures therefore provide an inappropriate surrogate for quantifying the propensity of light to engage ipRGC-driven circadian, neuroendocrine, and neurobehavioural responses ( Fig 1A ).

Panel A illustrates the melanopic action spectrum ( s mel ( λ ) with peak sensitivity at 490 nm, following prereceptoral filtering appropriate for a 32-year-old observer) and the photopic (2° spectral luminous efficiency) function, V ( λ ), superimposed on the spectral power distribution of standard daylight (CIE illuminant D65 [ 142 ]). Spectral sensitivities are plotted in logarithmic coordinates. Panel B illustrates the weighted spectral power distribution for spectrum in A multiplied by the photopic and melanopic efficiency functions at 1,000 lux for illuminance ( E v ) and melanopic EDI ( E v , m e l D 65 ). Sensitivity curves in A are plotted from the tabulated values provided in the CIE S026 standard [ 34 ], with weighted irradiance (plots in B and associated calculations) derived using the procedures described in detail therein. CIE, Commission Internationale de l’Eclairage; ipRGC, intrinsically photosensitive retinal ganglion cell; melaponic EDI, melanopic equivalent daylight illuminance.
While the potential value of a melanopsin-based photometric quantity has been recognised for some time, there has also been uncertainty as to whether this provides a sufficiently detailed model of the spectral sensitivity of human ipRGC-driven responses to ocular light exposure [ 28 ]. Hence, while the spectral sensitivity of physiological responses to light in visually blind people and animals matches that expected for melanopsin [ 20 , 23 , 25 , 29 ], in the fully intact retina, ipRGCs can also receive signals originating from rods and/or cones [ 26 ]. Moreover, available data indicate that the relative contributions of melanopsin and rod/cone photoreception to nonvisual ocular light responses, and consequently their apparent sensitivity, may vary as a function of exposure duration, light intensity, and perhaps time of day and/or prior light exposure [ 25 , 28 , 30 – 33 ].
As an initial response to the absence of a suitable metric for quantifying ipRGC-dependent ocular light responses, in 2013, an expert working group proposed a system that weighted irradiance according to the effective in vivo spectral sensitivity of the 5 known human retinal opsin proteins (melanopsin, rhodopsin, S-, M-, and L-cone opsin) [ 28 ]. This framework has now been formalised into an international standard with a SI-compliant system of metrology for ipRGC-influenced responses to light (Commission Internationale de l’Eclairage (CIE) S 026 [ 34 ]). Within this system, the effective rates of photon capture for each of the human retinal opsins under a given light condition are equated to the photopic properties (e.g., illuminance) of a standard 6500 K (D65) daylight spectrum that would produce the same rate of photon capture. This approach defines, for each opsin class, the α-opic equivalent daylight illuminance (EDI; where α-opic denotes one of the 5 human opsin classes that can contribute to ipRGC-influenced responses, e.g., melanopic; Fig 1B ). Despite the significant advance provided by this new light measurement standard, to date, explicit scientific consensus guidance on the relationship between the 5 α-opic quantities and the magnitude of practically relevant ipRGC-dependent responses is lacking. For example, how should signals from melanopsin, cones, and rods be weighted? Do these weightings change with light exposure duration and history? What levels of α-opic EDI are appropriate in a given time of day and setting?
Importantly, as originally envisaged [ 28 ], adoption of the new measurement approach has facilitated a number of large-scale retrospective evaluations of historical data [ 35 – 39 ] and informed new hypothesis-driven investigations [ 40 – 43 ] on the photoreceptive physiology for circadian, neuroendocrine, and neurobehavioural responses in humans. In total, the evidence from such studies [ 35 – 43 ] supports the view that, under most practically relevant situations (extended exposures to polychromatic light in the absence of pharmacological pupil dilation), light sensitivity of human physiological responses can be reliably approximated by the α-opic irradiance for melanopsin or the corresponding EDI (melanopic EDI). Moreover, based on the consistency of melanopic irradiance–response relationships across studies [ 38 ], it is now possible to define realistic, evidence-based recommendations for light exposures that target nonvisual responses ( Fig 2 ). Alongside the emergence of freely available tools to calculate the relevant metrics [ 44 , 45 ], there now exists an easily measured and internationally accepted SI-compliant system of metrology [ 34 ] to inform lighting design and associated policy.

Data are derived from laboratory studies (in humans without the use of pupil dilators) investigating the impact of long exposures (>2 hours) to primarily broadband light sources on melatonin suppression [ 69 , 88 , 95 , 143 , 144 ] ( A ), circadian phase resetting [ 83 , 89 , 143 , 144 ] ( B ), and subjective alerting responses [ 86 ] ( C ), as analysed in [ 38 ]. Group data (round symbols) are presented as mean ± SD; otherwise, data for individual subjects are presented (square symbols). Shading represents the 95% confidence limits of an unconstrained 4-parameter sigmoid fit to the data. For comparison across different response types ( D ), data sets from A – C were normalised relative to the range of the curve fit for that response type. Shaded areas in D reflect the consensus recommendations of the Second International Workshop on Circadian and Neurophysiological Photometry for sleep, evening, and indoor daytime environments. Recommendations are intended to provide realistic targets that minimise inappropriate nonvisual responses in the sleep environment (melanopic EDI <1 lux) and reduce these so far as is practically possible presleep (3 hours before habitual sleep; melanopic EDI <10 lux) while maximising relevant effects across the intervening daytime hours (melanopic EDI >250 lux). The nonshaded region indicates the range of melanopic EDI that should, where possible, be avoided during evening and nighttime and are considered suboptimal for daytime environments. EDI, equivalent daylight illuminance.
Here, we describe expert consensus-based recommendations for daytime, evening, and nighttime light exposure, considerations associated with their applicability, the supporting scientific evidence, and any caveats associated with the recommendations as they stand.
Methodology
The Second International Workshop on Circadian and Neurophysiological Photometry in 2019 brought together experts in lighting, neurophysiological photometry and sleep, and circadian research (all workshop participants are included as authors of this manuscript). The workshop was chaired by Brown and Wright who invited participants based on professional and/or academic qualifications and on reputation of being a leading expert in the field, including being an author of key scientific publications and/or international standards on the topic. Workshop participants were provided goals and key questions to address prior to a structured face-to-face meeting. The primary focus of the meeting was to develop expert consensus recommendations for healthy daytime and evening/nighttime light environments tentatively based on the new SI-compliant measurement system (CIE S 026:2018) [ 34 ]. Initial questions for review and discussion were the following:
- What range of melanopic EDI can be reasonably considered to provide minimal and maximal impacts on nonvisual ocular light responses in humans?
- Do signals from rods and/or cones also play a major role, and, if so, what relevant guideline levels could be recommended to account for such actions?
- Do the answers to (1) and/or (2) vary across different nonvisual forming responses (e.g., circadian entrainment/resetting, sleep/arousal, effects on hormone secretion, and mood) and, if so, what is the most appropriate general recommendation that can be provided?
Participants were also asked to consider if recommended light exposures would vary depending on which specific biological effects one is trying to achieve and/or on the target population (e.g., shift workers, specific clinical applications, etc.) and to include empirical literature supporting their views. In the face-to-face meeting, the morning of the first day was devoted to detailed presentations and discussion of the relevant scientific literature, and the afternoon was devoted to breakout sessions for discussion of questions 1 to 3 noted above. The second day was devoted to further discussion with sufficient time to address all opinions, ideas, and concerns. Voting to determine the expert consensus recommendations occurred via an iterative process; voting was limited to workshop participants and, where consensus could not be initially reached, discussion and review of the relevant literature resumed until participants were in agreement. Following the establishment of the expert consensus recommendations, a writing plan was formulated to produce the current paper. Subgroups of workshop participants initially drafted sections of the manuscript most relevant to their specialist expertise, including providing accounts of the scientific evidence from laboratory and field studies, relevance to other existing visual standards, and other special considerations associated with application of the recommendations. The workshop chairs (Brown and Wright) then integrated the expert content into a complete draft manuscript, including the recommendations formalised during the meeting. Workshop participants reviewed, edited, and approved both the draft (available as a preprint [ 46 ]), and this final version, which provides additional rationale supporting the recommendations and their practical application. The recommendations and associated considerations described herein are therefore the product of a workshop involving the authors. We are aware that such a workshop can rarely be exhaustive with respect to expertise and/or views across all potential stakeholders.
Expert consensus-based recommendations
The recommendations, described below, are intended to provide realistic targets that will result in appropriate circadian, neuroendocrine, and neurobehavioural responses to ocular light exposure in humans.
Daytime light recommendations for indoor environments
Throughout the daytime, the recommended minimum melanopic EDI is 250 lux at the eye measured in the vertical plane at approximately 1.2 m height (i.e., vertical illuminance at eye level when seated). If available, daylight should be used in the first instance to meet these levels. If additional electric lighting is required, the polychromatic white light should ideally have a spectrum that, like natural daylight, is enriched in shorter wavelengths close to the peak of the melanopic action spectrum ( Fig 1A ).
Evening light recommendations for residential and other indoor environments
During the evening, starting at least 3 hours before bedtime, the recommended maximum melanopic EDI is 10 lux measured at the eye in the vertical plane approximately 1.2 m height. To help achieve this, where possible, the white light should have a spectrum depleted in short wavelengths close to the peak of the melanopic action spectrum.
Nighttime light recommendations for the sleep environment
The sleep environment should be as dark as possible. The recommended maximum ambient melanopic EDI is 1 lux measured at the eye.
In case certain activities during the nighttime require vision, the recommended maximum melanopic EDI is 10 lux measured at the eye in the vertical plane at approximately 1.2 m height.
Additional considerations
- Exposure to a stable and regular daily light–dark cycle is also likely to reinforce good alignment of circadian rhythms, which may further benefit sleep, cognition, and health. These recommendations should therefore be applied at the same time each day, so far as possible.
- These recommendations are not intended to supersede existing guidelines relating to visual function and safety. The nonvisual ocular light responses covered here should be an additional level of consideration provided that relevant visual standards can still be met.
- These recommendations are derived based on data from (and intended to apply to) healthy adults (aged 18 to 55) with regular daytime schedules. Special considerations may apply to specific populations (e.g., children, older people, shift workers, or other individuals whose light sensitivity deviates substantially from an “average” healthy adult) as discussed later in this publication (see “Special cases and exceptions”).
Relationship to existing standards
There are several national and international standards that are relevant to indoor light exposure in the built environment, which have been developed under rigorous due processes, consensus, and other criteria. In terms of biological safety, there is a recent recommended practice for photobiological safety that provides guidance on ocular and dermal health relative to light exposure from all varieties of indoor lamps and lamp systems (American National Standards Institute/Illuminating Engineering Society (ANSI/IES) RP-27-20) [ 47 ]. The International Commission on Non-Ionizing Radiation Protection (ICNIRP) has also released a recent statement concerning photobiological safety, specifically of light exposure from LEDs [ 48 ]. Other existing guidelines, codes, and specifications for lighting installations in indoor places primarily concentrate on visual function, including visual comfort, visual performance, and seeing safely for people with normal, or corrected to normal, vision.
Current specifications within lighting practice are based on illuminance and several additional qualitative and quantitative needs concerning glare, colour rendering, flicker and temporal light modulation, luminance distribution, and the directionality and variability (of both colour and level) of light. These specifications are crafted to enable people to perform their visual tasks accurately and efficiently, even for difficult circumstances or extended durations (e.g., Deutsches Institut für Normung (DIN) SPEC 67600 [ 49 ]; ANSI/IES RP-28-16 [ 50 ]; and EN 12464–1 [ 51 ]). Together with the focus on energy saving, the existing guidelines restrict the illuminance indoors to levels that are typically at least 1 order of magnitude below the natural light environment outdoors. Moreover, the electrical light sources in most common use, while optimised for their visual qualities, are typically substantially less efficient at stimulating melanopsin than natural daylight of equivalent illuminance, i.e., the light they provide has a low ratio of melanopic EDI to photopic illuminance (quantified by the melanopic daylight efficacy ratio (melanopic DER) [ 34 , 52 ]; see Fig 3 ). This leaves us with an indoor light environment that is potentially suboptimal for supporting human health, performance, and well-being [ 9 – 12 , 53 ]. For example, Comité Européen de Normalisation (CEN) guidelines specify a minimum task plane photopic illuminance of 500 lux for writing, typing, reading, and data processing tasks. When just meeting this illuminance threshold with regular lighting (i.e., melanopic DER well below 1; Fig 3A and 3B ), typical (vertical) melanopic EDIs encountered across the working day will fall below 200 lux (e.g., [ 54 – 56 ]). Moreover, specified illuminance levels for other settings, where visual demands are lower (e.g., corridors, rest rooms, etc.), will typically be substantially lower than the above (melanopic EDI <200 lux; [ 51 ]).

Panels A and B illustrate spectral power distributions (yellow) for commonly encountered fluorescent (A) and LED-based (B) white light sources. Panels C and D represent high melanopic content LED source of similar ( C ) and cooler (higher) correlated colour temperature ( D ) achievable with current technologies. Spectra in A and B represent CIE standard illuminants F11 and LED-B1, plotted from tabulated source data [ 142 ], spectra in C and D were modelled by combining weighted combinations of spectra from commercially available broad and narrowband LED sources. Melanopic (blue; V ( λ )) and photopic (green; V ( λ )) spectral efficiency functions are shown for reference. Photopic illuminance ( E v ) and melanopic equivalent daylight (D65) illuminance ( E v , m e l D 65 ) for each spectrum is provided above, along with the melanopic efficiency for that light source (melanopic daylight (D65) efficacy ratio; melanopic DER, defined as the ratio of melanopic irradiances for this source to that for a D65 light source at the same photopic illuminance [ 34 , 52 ]). Note, in this example, all sources provide a photopic illuminance of 300 lux, but vary in melanopic EDI, due to the relatively low melanopic DER of commonly used white light sources. CIE, Commission Internationale de l’Eclairage; melanopic DER, melanopic daylight efficacy ratio; melaponic EDI, melanopic equivalent daylight illuminance.
This publication is centrally based on an internationally balloted standard from the CIE [ 34 ], which now provides an accepted framework upon which to derive lighting specifications that optimise visual, circadian, neuroendocrine, and neurobehavioural responses to light. The corresponding expert-led consensus recommendations for biologically appropriate lighting are reflected in general melanopic EDI thresholds for various times of day/night. The recommendations presented here are intended to be widely achievable within the constraints of other relevant lighting guidelines (e.g., via lighting of appropriate spectral composition; Fig 3C and 3D ) and to provide a sound scientific basis for the formal development of recommended practices in light and lighting from national and international standards organisations (e.g., ANSI, CIE, DIN, IES, and the International Organization for Standardization; ISO).
In closing this section, we note that a number of other recommendations relevant to physiological and neurobehavioural effects of light have been proposed in recent years, including some guidelines and specifications by commercial (for-profit) entities (reviewed in [ 57 ]). Unlike these previous suggestions, the present recommendations are both built around an SI-complaint, internationally accepted and validated measurement system and are supported by expert scientific consensus, features recognised as critical by established industry regulatory and standardisation bodies [ 58 , 59 ].
Practical considerations
As noted above, while the recommendations detailed here are expected to be widely achievable, implementing these in any real-world setting necessitates care not to compromise other important regulations and/or considerations (e.g., visual appearance, glare, thermal comfort, safety, and energy efficiency). For example, an important consideration in achieving our recommendations for daytime settings is whether this would necessitate higher overall light levels and therefore increase energy expenditure and/or the risk of visual discomfort (e.g., glare). Notably, there is a range of approaches that (individually or in combination) could allow these recommendations to be met while avoiding such issues, including increasing the availability and accessibility of natural daylight (e.g., [ 56 ]), engineering the spectral content of electric lighting to increase melanopic DER, adjusting finishes and furnishings to optimise surface reflectances, and adjusting the placement, angular dispersion and size of accessible luminous surfaces to enhance vertical illuminances and/or minimise glare [ 60 – 64 ].
As an illustration of the above, a recent study modelling common office and educational settings found a combination of adjustments in surface finishes and spectral composition of lighting could readily produce 2- to 3-fold changes in vertical melanopsin-weighted light exposure [ 62 ]. For the conditions assessed there, where relevant national standards specified horizontal illuminances of 300 to 400 lux, achieving an average melanopic EDI of 250 lux in the vertical plane required an approximately 50% increase in horizontal illuminance and energy output when using an ‘off-the shelf’ tuneable white LED (6,500 K; melanopic DER = 0.83). With appropriate design, however, colour mixed LEDs can allow much higher melanopic DERs, even when maintaining warmer colour temperatures (e.g., up to 1.4 for 4,000 K sources [ 61 ]). Moreover, engineered LED luminaires that balance less extreme increases in melanopic DER with a good fidelity colour rendition index and slightly cooler white light [ 65 ] can facilitate meeting our daytime recommendations without significantly compromising energy expenditure or visual qualities (e.g., Fig 3D ). Further, the energy efficiency of colour-mixed LED sources is rapidly approaching that typical of standard phosphor-converted LEDs and is scheduled to exceed this over the next decade [ 66 ]. Thus, while optimising building and lighting design to maximise energy efficiency and minimise visual discomfort remain important goals, these should not ultimately prove impediments to implementing our recommendations in most settings.
By contrast, increased energy expenditure is not a concern with respect to our evening/nighttime recommendations, where the significant practical consideration is rather ensuring that there is sufficient light to comfortably and safely perform visually guided activities. For the sleep environment, it is already natural to greatly minimise light exposure (by turning off lights, covering windows, and the like). There is likely still a fair proportion of individuals for whom the sleep environment is currently slightly above a melanopic EDI of 1 lux (e.g., [ 67 68 ]), although we would not envisage any significant barriers to reducing this where required (e.g., via use of blackout blinds and the installation of orientation lighting where needed). Outside of the sleep environment, however, ensuring sufficient light is available for vision is of course essential.
From existing ambulatory field assessments, evening (photopic) illuminance is commonly reported in the order of 30 lux [ 69 – 73 ]. Although certain rooms (e.g., kitchens) may be more brightly lit, this value of 30 lux corresponds to vertical illuminances typically measured in most indoor domestic environments in the evening [ 74 ]. In such cases, meeting the threshold melanopic EDI of 10 lux need not require any significant change in overall illuminance. Hence, many commonly used domestic warm white (2,700 to 3,000 K) LEDs already have a melanopic DER sufficiently low (<0.35 [ 52 ]) to meet our target while maintaining an illuminance of approximately 30 lux. Consistent with this view, a recent study that assessed evening light exposure in home settings via wearable spectrophotometers found that in nearly 50% of occasions melanopic EDI was already at or below 10 lux [ 75 ]. Moreover, many cases where evening light exposure was above this level involved lighting enriched in shorter wavelengths and could, in principle, been brought in line with our recommendations simply by using lower melanopic DER light sources. Further, the use of appropriate task lighting and/or lighting specifically engineered to minimise melanopic output (e.g., [ 69 ]) may further support activities that benefit from illuminances above 30 lux while maintaining an overall environment where melanopic EDI at the eyes remains below 10 lux (although the latter ultra-low melanopic DER sources will likely come at the expense of reduced colour discrimination). A particular challenge, however, comes from indoor environments outside the home (e.g., spaces shared by individuals with radically different daily/work schedules), where existing visual standards will often specify illuminance levels (>100 lux) that cannot be achieved while maintaining a vertical melanopic EDI <10 lux and optimal colour discrimination. Nonetheless, while meeting our evening target may not be achievable in all instances, it should be broadly achievable in most domestic settings with currently available lighting technology and, therefore, for those with the regular daytime work schedules for which it is intended to apply.
A final point for consideration relates to the likely benefits of implementing our recommendations that may have to be weighed up to justify any associated costs (e.g., due to upgrading workplace lighting). As discussed in detail below, there is certainly evidence that increased daytime light can improve subjective or objective measures of performance, sleep, alertness, and/or mood and that decreased evening and nighttime exposures can reduce adverse effects of light on sleep, circadian rhythms, and long-term health (see “Evidence from real-world settings”). Directly quantifying the benefits that might be expected associated with implementing our recommendations is far more challenging. In the future, large-scale longitudinal studies that combine data on objectively measured performance (e.g., sick days, productivity, and incidence of accidents), health outcomes, and appropriately measured (personal) light exposure may provide such information. In the interim, it is worth noting that, even ignoring possible impacts on the incidence of common and costly health complications associated with circadian disruption (e.g., cardiovascular disease, diabetes, and cancer), benefits associated with improved sleep alone could potentially be substantial [ 76 ]. Indeed, insufficient sleep is estimated to cost the United States 2.4% GDP, due absenteeism, accidents, reduced productivity, etc. [ 76 , 77 ]. Moreover, even comparatively modest improvements for those with poor sleep (<6 hours sleep/night increased to 6 to 7 hours/night) are predicted to increase US GDP in the order 1.7% or approximately 300 billion USD/year [ 77 ].
Scientific rationale
Evidence from laboratory studies.
The rationale for basing these recommendations upon melanopic EDI is, in the first instance, provided by a comprehensive analysis of data aggregated from controlled laboratory studies (performed in healthy adults aged 18 to 55) that have evaluated the 2 best understood neuroendocrine and circadian light responses in humans: acute suppression of nocturnal pineal melatonin production and circadian phase resetting by evening or nighttime light exposure [ 36 – 39 ]. Those data indicate that, for a wide range of monochromatic, narrowband and broadband light sources and exposure durations, such ocular light responses are better predicted by melanopic irradiance than by photopic illuminance or other existing metrics. Additional contributions from photoreceptors other than melanopsin are expected based on known ipRGC biology [ 26 , 28 ], and evidence for such contributions has been observed under certain circumstances [ 30 , 78 , 79 ]. Importantly, however, the sum of empirical human data suggest that any such influences are sufficiently limited that, under most practically relevant circumstances, the spectral sensitivity of circadian and neuroendocrine and, by extension, other related nonvisual responses to ocular light exposure, can be well approximated by melanopic EDI.
The clearest evidence for contributions from photoreceptors other than melanopsin has so far come from evaluations of melatonin suppression in short (<1 hour) time windows following exposures to monochromatic light in participants with dilated pupils (to remove indirect effects of pupil constriction on apparent sensitivity). Data from 2 such studies are compatible with the possibilities that S-cones [ 78 ] or the photopic system [ 30 ] may contribute alongside melanopsin (see also reanalysis in [ 79 ]). Importantly, however, a large body of data with and without use of pupil dilation indicates that for exposures of an hour or more, melatonin suppression can be reliably predicted by melanopic EDI [ 37 , 38 , 80 , 81 ]. This conclusion is further strengthened by findings from recent studies that have employed photoreceptor isolating stimuli to confirm that melanopsin-selective changes in irradiance modulate melatonin production [ 40 , 41 ] but failed to find any effect of large variations in irradiance selectively targeting S-cones [ 42 ]. Further evidence consistent with a dominant role for melanopsin comes from earlier observations that totally blind humans (where remaining light responses match the spectral sensitivity expected for melanopsin) [ 23 , 25 ] can display near-full melatonin suppression [ 15 , 17 , 23 ], as do individuals with colour vision deficiencies [ 82 ].
In line with the data discussed above, totally blind individuals can also display circadian phase resetting responses to bright white light of comparable magnitude to sighted individuals [ 16 ]. Findings from one study in sighted individuals with pharmacologically dilated pupils are suggestive of cone contributions to circadian phase resetting following long (6.5 hours) exposures to dim monochromatic light [ 30 ]. However, an equivalent effect is not readily apparent across data from studies performed on participants with undilated pupils [ 38 , 83 , 84 ]. Thus, laboratory data collected under conditions that are more relevant to the real world, where pupils are freely light responsive, indicate that the influence of cones is sufficiently small that melanopic irradiance can provide a reliable approximation of the spectral sensitivity of circadian phase resetting.
By contrast to the circadian and neuroendocrine responses discussed above, other relevant effects of light that are of importance but mechanistically less well understood, such as acute light effects on alertness, have not yet received the same degree of analytic and parametric study. Nonetheless, light-dependent changes in subjective alertness have commonly been reported (reviewed in [ 2 , 85 ]) and, where performed, functional studies employing electroencephalogram (EEG) or magnetic resonance imaging approaches reveal clear neurophysiological correlates of such subjectively measured alertness changes (e.g., [ 43 , 86 , 87 ]).
With respect to the conditions under which such alerting effects occur, a recent comprehensive meta-analysis reveals that self-reported alerting responses to white light are commonly observable within a similar range of light intensities to those associated with effects on the circadian system (irrespective of time of day) [ 2 ]. Many of the original studies contributing to the latter analysis predate the discovery of melanopsin. It is possible, however, to obtain reasonable approximations of melanopic EDI from the photopic illuminance reported by earlier studies, by reference to the typical ratio of these 2 parameters expected for the relevant light sources (i.e., melanopic DER). For example, a recent meta-analysis [ 2 ] notes a significant subjective alerting effect of bright white light in almost 80% of studies (15 of 19) where the “dim” light condition was below 80 lux and the “bright” condition >500 lux (values that correspond to melanopic EDI of <50 lux and >250 lux, using a conservative melanopic DER of 0.6 and 0.5, respectively). Further, the published irradiance response data for subjective (and objective) alerting responses to nocturnal broadband white light exposure [ 86 ] align very well with the relationship between melanopic EDI and circadian-related responses determined from other studies that did not employ pupil dilation [ 38 , 88 , 89 ] ( Fig 2 ).
The recent meta-analysis discussed above [ 2 ], which could not reach definitive conclusions regarding spectral sensitivity of alerting responses, did not assess the extent to which the magnitude of alerting responses were predictable based on melanopic EDI. Nonetheless, the most informative studies included in that analysis [ 69 , 90 – 94 ] and other relevant studies and meta-analyses [ 36 , 38 , 39 , 69 , 95 ] indicate that alerting effects produced by light of varying spectral composition are certainly better predicted by melanopic irradiance than other available metrics. Moreover, recent studies provide evidence that selectively increasing melanopic irradiance, in the absence of changes in either illuminance or colour, can promote self-reported alertness during both day [ 43 ] and evening [ 40 ]. The former study also confirmed EEG correlates of enhanced daytime alertness via alpha attenuation test [ 43 ]. Collectively, these data do not exclude the possibility that cone signals might exert a greater influence over acute alerting responses to light than is apparent for circadian and neuroendocrine effects. Nonetheless, the bulk of available evidence supports the view that melanopic EDI is the best currently existing predictor of alerting responses to light and is relevant for both day and evening/nighttime scenarios. The currently available data do not provide any definitive evidence that the sensitivity of such alerting responses differs substantially relative to other melanopsin-driven responses to evening/nighttime light exposure ( Fig 2 ) or between night and day (reviewed in [ 2 , 85 ]). Accordingly, in the absence of new information, the sensitivity range defined for the more comprehensively studied circadian and neuroendocrine responses can be used as a sensible predictor of propensity of light to modulate alertness, regardless of time of day.
In sum, most of the available laboratory data suggest that melanopic EDI is a reliable index that provides a good approximation of the apparent spectral sensitivity of human circadian and acute nonvisual responses to ocular light exposure. In particular, for the extended exposures to polychromatic light that are relevant to everyday living environments, existing evidence indicates that any additional contributions from cones (or rods; whose spectral sensitivity is close to melanopsin [ 34 , 96 , 97 ]) do not compromise the predictive value of melanopic EDI.
As befitting a system evolved to optimise physiology and behaviour in anticipation of day–night transitions driven by the Earth’s rotation relative to the sun, the operating range of human circadian, neuroendocrine, and alerting responses to ocular light exposure spans the range of light intensities typically encountered between civil twilight and sunrise/sunset (i.e., melanopic EDI of approximately 1 to 1,000 lux; Fig 2 ). The recommendations indicated above are therefore intended to ensure that the sleeping environment is kept at a limit below which any appreciable nonvisual responses of this nature are elicited and to minimise negative effects of the light environment during sleep and presleep hours [ 98 ]. Similarly, recommendations for daytime and evening light exposure are intended, so far as practically possible, to respectively maximise and minimise any associated effects on sleep, alertness and the circadian system. By providing an appropriately marked day–night signal and reducing potential disruptive effects of evening light, collectively, these recommendations are expected to promote robust and appropriately timed circadian functions in most individuals [ 99 ], as well as to promote alertness throughout the day and support healthy sleep.
Also worthy of note here, a number of studies have provided evidence that undesirable effects of evening/nighttime light can be mitigated by brighter light exposure earlier in the day (e.g., [ 31 , 33 , 100 – 104 ]). While opposing actions of light exposure during morning/daytime and evening are a well-understood feature of circadian function [ 105 , 106 ], these modulatory effects also extend to more acute actions of evening light, such as its ability to suppress melatonin production. At present, the physiology responsible for such actions are not well understood, nor does currently available data enable a detailed assessment of the intensity and/or time range across which such effects operate. What is clear, however, is that modulatory effects of prior light exposure are certainly not limited to earlier parts of the day [ 33 , 104 ]. Accordingly, such observations suggest a further potential benefit of maintaining high melanopic light exposure throughout the day. The visual requirements necessary or desirable for some activities during later parts of the evening (e.g., relating to illuminance and/or colour) place a limit on the extent to which disruptive effects of white light can be entirely avoided simply by reducing melanopic EDI (e.g., using lighting with a lower melanopic DER). Higher levels of daytime light exposure may therefore help mitigate any disruptive effects associated with unavoidable light exposure in later parts of the evening.
Evidence from real-world settings
While our current understanding of the spectral sensitivity and dynamic range of circadian, neuroendocrine, and neurobehavioural light responses in humans is most directly informed by laboratory studies, our recommendations are also supported by field evaluations of the impact of environmental lighting.
Access to electric lighting is associated with reduced daytime and increased nighttime light exposure and altered sleep timing [ 107 – 110 ], with many individuals in modern society routinely experiencing melanopic EDI <250 lux during the day, especially those with delayed sleep schedules [ 72 , 73 ]. Accordingly, there have been a number of real-world studies implementing daytime high melanopic lighting interventions in workplaces, schools, and care homes that provide practical corroboration for the recommendation outlined above [ 111 ]. Such practically focused investigations were not designed to evaluate melanopsin contributions per se (with increases in melanopic EDI usually being accompanied by increases in colour temperature and/or illuminance), but such studies do provide valuable insight in likely real-world benefits associated with meeting our recommendations.
In offices, increasing the melanopic output of architectural lighting (approximately 2-fold) via short wavelength-enriched lamps (17,000 K; melanopic DER ≈1, versus 3,000 to 4,000 K; melanopic DER<0.6) had beneficial effects on self-reported alertness, performance, mood, and sleep quality [ 54 , 55 ]. Similarly, enhancing daytime melanopic exposure by increased access to natural daylight in the workplace improved sleep and objectively measured cognitive performance (higher-order decision-making) in office workers [ 56 ]. In these studies [ 54 – 56 ], the average melanopic EDI in the control working environment was <150 lux (standard 3,000 to 4,000 K fluorescent lighting; Fig 3A ), with the experimental “active” conditions increasing melanopic EDI to approximately 170 to 290 lux. Hence, modest and readily achievable adjustments to increase light exposure can be associated with measurable benefits, without any observable detrimental effects.
In schools, findings from a series of studies employing fluorescent lighting with various intensities and spectra indicate that settings with a higher melanopic output (melanopic EDI >500 lux) can improve measures of concentration and reading comprehension compared to current standard lighting (typically providing melanopic EDI <200 lux; [ 112 – 115 ]). Similar benefits of short wavelength-enriched (17,000 K) versus standard 4000 K fluorescent light on reducing sleepiness have also been shown in college-aged students during afternoon lectures [ 116 ]. Further, building on seminal work showing the benefits of increased daytime light levels for the elderly [ 117 ], several clinical trials have shown the benefits of enhanced melanopic light exposure during daytime hours on care home residents [ 118 – 120 ]. There is evidence of reduced circadian sensitivity/responsiveness to light in older adults (see “Special cases and exceptions”), including changes in lens transmission that could reduce effective retinal dose corresponding to a given melanopic EDI by approximately 50% relative to the (young) standard observer on which calculations are based [ 44 ]. Nonetheless, in these studies, compared to control conditions (typical daytime melanopic EDI <150 lux), implementation of higher melanopic, short-wavelength enriched, polychromatic lighting (5,500 to 17,000 K, providing melanopic EDI >250 lx) led to a range of improvements including reduced depression, agitation, and anxiety, better daytime activity and, in some studies, improved sleep quality.
Collectively, increasing melanopic light exposure during the day in line with our recommendations has been shown to benefit alertness, performance, and sleep in a wide range of real-world settings, even in the presence of daylight or stimulants such as caffeine or for younger or older age groups. Further, there is minimal evidence for negative effects of increased daytime melanopic light exposures. One care home study [ 120 ], where the brightest daytime light intervention was examined (bright 17,000 K lighting providing melanopic EDI approximately 900 lux), reported a reduction in sleep efficiency and quality when compared to standard 4000 K lighting (melanopic EDI approximately 100 lux). Further, in an office study of dayworkers where the melanopic EDI of control condition was already high (approximately 400 lux) further increases (melanopic EDI approximately 750 lux) associated with the use of an 8000 K lighting system appeared to prevent the normal seasonal advance in sleep timing [ 121 ]. While the latter could be considered beneficial, as it enhances circadian alignment to the working day, long-term effects of decoupling from seasonal environmental rhythms is, to date, unclear. Given these data, future research is warranted to identify the potential beneficial and adverse effects on human physiology, cognition, behaviour, and health of electric lighting that greatly exceeds our intensity recommendations.
In addition to reduced daytime light exposure, increased exposure to electric light in the evening and night is commonly considered to exert adverse effects on sleep, circadian rhythms, and health outcomes [ 8 – 11 , 67 , 68 , 122 ]. Indeed, even relatively low levels of light in the sleep environment (conservatively, melanopic EDI >3 lux) have been associated with impaired sleep and increased incidence of diabetes in large cohort studies [ 67 , 68 ]. Further, typical evening light levels often fall within the range where significant nonvisual responses would be predicted from laboratory studies [ 75 ]. For example, a significant source of evening light exposure is from visual displays, which in the absence of any other illumination, can provide melanopic EDI of >60 lux [ 52 , 123 , 124 ] (above the typical level of exposure required to produce half-maximal subjective alerting, melatonin suppressing, and circadian phase shifting responses in laboratory studies; Fig 2 ). Indeed, several studies have shown that light from modern visual displays is sufficient to reduce the evening rise in melatonin, impair sleepiness, and/or increase subjectively or objectively measured alertness [ 123 – 126 ]. Moreover, manipulations that reduce exposure to short wavelength light from such displays has, in some laboratory studies, been found to lessen these effects [ 125 , 126 ] as have selective reductions in melanopic output [ 40 ]. There have not yet been large-scale longitudinal field studies on how effective such manipulations might be, although it is noteworthy that the reductions in melanopic radiance achievable simply by adjusting the spectral content of current visual displays are modest (approximately 50% decrease). As such, we expect that such approaches will be most beneficial when combined with other strategies to minimise evening illumination (e.g., dimming of screens and low melanopic ambient lighting). In addition, the potential protective role of adequate daytime light exposure to attenuate adverse effects of evening and nighttime light exposure on circadian physiology requires future research.
Special cases and exceptions
While the current recommendations are intended to be widely applicable, the scientific underpinnings primarily derive from studies of neuroendocrine, circadian, sleep, and alerting responses to ocular light exposure in healthy young adults. Even among this group, findings from a recent laboratory study show significant (>10-fold) interindividual variations in sensitivity to white light–induced suppression of the evening rise in melatonin [ 88 ]. The physiology underlying this variability is currently unknown. Importantly, however, assuming such variability is indicative of that for the healthy adult populations contributing across the range of lab studies discussed above, it is inherently incorporated into our recommendations. Hence, targets for daytime and nighttime exposures are based on light intensities found to produce near maximal or minimal responses across the test population. With respect to the recommendation for evening settings, there may be more significant variability in the relative magnitude of circadian, neuroendocrine, and associated neurophysiological responses, based on the intraindividual differences noted above [ 88 ]. In the absence of any ready means for predicting individual differences in sensitivity, the present recommendation of a maximum melanopic EDI of 10 lux is intended to appropriately minimise undesirable effects of evening light for the “average” healthy adult while allowing for sufficient light for common evening activities (see “Practical considerations” for further discussion). As it stands, where the evening light environment currently results in a melanopic EDI above 10 lux, reducing exposure in line with our recommendations is certainly still expected to be beneficial, regardless of individual differences in sensitivity, although future developments may make it possible to refine recommendations for specific individuals.
The magnitude of circadian and neuroendocrine responses to light also depends on age, with those in young children being larger and those in older adults tending to be smaller when compared to young adults [ 127 – 131 ]. These observations may, in part, reflect age-related differences in the amount of light reaching the retina (due to changes in pupil size and lens transmittance), although more direct changes in sensitivity or amplitude may also be involved. Certainly, one previous study that investigated the impact of age-related changes in lens transmittance did not find that this was associated with the expected reduction in light induced melatonin suppression in older adults [ 132 ]. Changes in light exposure in line with the current recommendations are still expected to be of general benefit to both young [ 112 – 115 , 124 , 126 ] and older individuals [ 67 , 68 , 118 – 120 ] (where their current daytime light exposure falls below, or evening/nighttime exposure above, the relevant targets). Select groups, however, may further benefit from higher daytime (e.g., older people) and/or lower evening exposures (e.g., children) than indicated in the recommendations. Similarly, disruptions to sleep and circadian rhythms are commonly associated with many disorders and disease states [ 8 , 133 ]. While adjusting light exposure may be of benefit in some or all of these conditions, further research will be required to determine whether alterations to the recommended thresholds will be required for such individuals.
In addition to the points above, a particular challenge in optimising light exposure to benefit health and performance relates to shift workers. Current light exposure advice for night shift workers is still not mature [ 134 ], and we want to stress that the present recommendations are not intended for this purpose. There is certainly evidence that increasing melanopic light levels in the work environment can improve subjective and/or objectively measured alertness and performance in shift workers [ 135 – 138 ]. Important benefits such as these do, however, need to be weighed in the context of potential disruptions to circadian alignment and chronic effects on health [ 8 – 14 ]. Addressing these important questions remains a key area for future investigation and shift work–related consensus guidance on best practice.
As discussed above, it is also essential that any changes to light exposure intended to adjust melanopsin-dependent physiological responses do not compromise visual requirements. For example, the elderly may need brighter lighting than recommended above to move safely between the bedroom and bathroom at night [ 12 ]. In many cases, such issues may be addressed by using lighting with an appropriate spectral composition (i.e., by using lighting with a low melanopic DER) and/or lighting designs that avoid direct illumination of the eyes. Nonetheless, there may be some instances where meeting the requirements for visual performance, visual comfort, and safety are incompatible with our recommendations regarding nonvisual responses, in which case the former must take precedence. Finally, while it is possible to comply with the recommended melanopic EDI thresholds specified here solely via exposure to electric light, there are a number of known and suspected benefits of exposure to broad-spectrum, outdoor, daylight [ 53 , 139 – 141 ].
Future directions
The recommendations outlined here are derived from a synthesis of several decades of research into the biology regulating circadian, sleep, physiological and cognitive responses to light and their practical implications. There is, without question, evidence that the use of melanopic irradiance as a model for the spectral sensitivity of such responses represents a simplification of the underlying biology. Although, as an aside, we note that this is true also for the established and widely used, photometric quantities (luminance and illuminance) that are currently applied to quantify conventional “brightness.” Nevertheless, we leave open the possibility that a deeper understanding of rod and/or cone contributions to physiological responses will reveal multiphotoreceptor models of spectral sensitivity that may allow a more accurate prediction of circadian, sleep, neuroendocrine, and/or cognitive responses. The contribution of rods to such responses is an interesting topic for research in its own right. Nonetheless, including a rod component in any such future metrics is unlikely to substantially improve the accuracy with which they recreate the spectral sensitivity of the relevant response(s), since the very similar spectral sensitivity profiles of rods and melanopsin render effective irradiance for these 2 opsins highly correlated [ 97 ]. Conversely, cone spectral sensitivity is quite distinct from melanopsin and has the potential to substantially refine metrics for circadian and neurophysiological responses. In particular, future work may reveal specific lighting conditions that maximise cone influence to produce practically relevant modulations in nonvisual responses to light (e.g., on the circadian system, neuroendocrine function, sleep physiology, and/or and alerting responses). At present, however, existing evidence indicates that the use of melanopic irradiance/EDI would not lead one to substantially over- or underestimate biological and behavioural effects for the types of light exposure that are typically encountered across daily life [ 35 – 38 , 40 – 43 ].
Further research into the factors influencing individual differences in the sensitivity of melanopsin-mediated responses to light exposure may make it possible to tailor guidelines to specific groups or even individuals. For the time being, our recommendations are derived from group data that must incorporate much of this variability. As such, it is expected that the recommendations for daytime and the sleep environment should be broadly applicable and strongly engage relevant circadian and neurophysiological responses for the vast majority of healthy adults. Known, age-related sources of variability are already at least partly accounted for by the inclusion of corrections for changes in lens transmission described in the nonnormative appendices of the existing standard [ 34 ]. Recommendations may, however, be modified in the future for certain groups such as children, older adults, or patient groups whose sensitivity to light may differ from the healthy adult population on which the present recommendations are based.
The current recommendations are intended to inform lighting design considerations for typical, real-world environments such as offices and other workplaces, schools, and colleges, residences, care homes, and in- and outpatient settings. As noted above, application of our recommendations across such settings is facilitated by the free availability of tools for calculating melanopic EDI (and also estimating this given known illuminance and type of lighting) [ 44 , 45 ]. Nonetheless, the emergence of low-cost commercial sensors for direct measurement of melanopic EDI (akin to conventional “lux meters”) is expected to further increase the ease with which the recommendations can be adopted.
A final point for consideration relates to applications of light therapy for clinical conditions like affective and circadian rhythm sleep disorders or for purposes such as improving circadian regulation and alertness in night and shift workers or transmeridian travellers experiencing jet lag. The current recommendations are not directly formulated for such uses, but the existing applications of ocular light therapy likely involve the same or similar biological underpinnings as discussed above. Given existing evidence for benefits of bright light therapy [ 5 – 7 ], perhaps widespread adoption of the recommendations described here will contribute to a reduction in the prevalence of affective and sleep disorders. More significantly, however, we expect the scientific framework that informs these recommendations to provide a concrete basis upon which to generate hypotheses to test for the subsequent establishment of optimal light treatment recommendations for clinical and travel applications.
Abbreviations
Funding statement.
The 2nd International Workshop on Circadian and Neurophysiological Photometry (2019) was funded by the Biotechnology and Biological Sciences Research Council (UK) grant BB/N014901/1 to TMB and RJL, with additional contributions from the University of Manchester Centre for Biological Timing and the Neuroscience and Mental Health Domain. The funders had no role in study design, data collection and analysis, decision to publish, or preparation of the manuscript.
Loading metrics
Open Access
Consensus View
Consensus View articles present a comprehensive analysis by an independent and usually multidisciplinary panel of experts that makes specific recommendations on important scientific, publishing or policy issues.
See all article types »
Recommendations for daytime, evening, and nighttime indoor light exposure to best support physiology, sleep, and wakefulness in healthy adults
* E-mail: [email protected] (TMB); [email protected] (KPW)
Affiliation Centre for Biological Timing, Faculty of Biology, Medicine and Health, University of Manchester, Manchester, United Kingdom

Affiliation Department of Neurology, Thomas Jefferson University, Philadelphia, Pennsylvania, United States of America
Affiliation Centre for Chronobiology, University Psychiatric Clinics Basel, Transfaculty Research Platform Molecular and Cognitive Neurosciences, University of Basel, Basel, Switzerland
Affiliations Division of Sleep and Circadian Disorders, Departments of Medicine and Neurology, Brigham and Women’s Hospital, Boston, Massachusetts, United States of America, Division of Sleep Medicine, Harvard Medical School, Boston, Massachusetts, United States of America
Affiliations Division of Sleep and Circadian Disorders, Departments of Medicine and Neurology, Brigham and Women’s Hospital, Boston, Massachusetts, United States of America, Division of Sleep Medicine, Harvard Medical School, Boston, Massachusetts, United States of America, Surrey Sleep Research Centre, Faculty of Health and Medical Sciences, University of Surrey, Guildford, United Kingdom
Affiliations Centre for Chronobiology, University Psychiatric Clinics Basel, Transfaculty Research Platform Molecular and Cognitive Neurosciences, University of Basel, Basel, Switzerland, Research Centre for Hauora and Health, Massey University, Wellington, New Zealand
Affiliation Centre for Radiation, Chemical and Environmental Hazards, Public Health England, Chilton, Didcot, United Kingdom
Affiliation Sleep and Circadian Neuroscience Institute, Nuffield Department of Clinical Neurosciences, University of Oxford, Oxford, United Kingdom
Affiliation Institutes for Medical Psychology and Occupational, Social and Environmental Medicine, Medical Faculty, Ludwig-Maximilians University (LMU), Munich, Germany
Affiliations Human Technology Interaction Group, Department of Industrial Engineering and Innovation Sciences, Eindhoven University of Technology, Eindhoven, the Netherlands, Intelligent Lighting Institute, Eindhoven University of Technology, Eindhoven, the Netherlands
Affiliation Chronobiology, Faculty of Health and Medical Sciences, University of Surrey, Guildford, United Kingdom
Affiliations Translational Sensory & Circadian Neuroscience, Max Planck Institute for Biological Cybernetics, Tübingen, Germany, TUM Department of Sport and Health Sciences (TUM SG), Technical University of Munich, Munich, Germany, Department of Experimental Psychology, University of Oxford, Oxford, United Kingdom
Affiliation Circadian and Sleep Epidemiology Laboratory, Department of Integrative Physiology, University of Colorado Boulder, Boulder, Colorado, United States of America
Affiliations Department of Neurology, Northwestern University, Chicago, Illinois, United States of America, Center for Circadian and Sleep Medicine, Northwestern University, Chicago, Illinois, United States of America
- [ ... ],
Affiliation Sleep and Chronobiology Laboratory, Department of Integrative Physiology, University of Colorado Boulder, Boulder, Colorado, United States of America
- [ view all ]
- [ view less ]
- Timothy M. Brown,
- George C. Brainard,
- Christian Cajochen,
- Charles A. Czeisler,
- John P. Hanifin,
- Steven W. Lockley,
- Robert J. Lucas,
- Mirjam Münch,
- John B. O’Hagan,
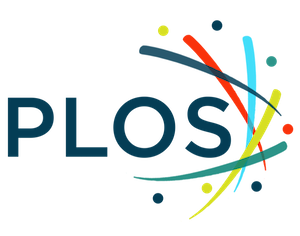
Published: March 17, 2022
- https://doi.org/10.1371/journal.pbio.3001571
- Reader Comments
Ocular light exposure has important influences on human health and well-being through modulation of circadian rhythms and sleep, as well as neuroendocrine and cognitive functions. Prevailing patterns of light exposure do not optimally engage these actions for many individuals, but advances in our understanding of the underpinning mechanisms and emerging lighting technologies now present opportunities to adjust lighting to promote optimal physical and mental health and performance. A newly developed, international standard provides a SI-compliant way of quantifying the influence of light on the intrinsically photosensitive, melanopsin-expressing, retinal neurons that mediate these effects. The present report provides recommendations for lighting, based on an expert scientific consensus and expressed in an easily measured quantity (melanopic equivalent daylight illuminance (melaponic EDI)) defined within this standard. The recommendations are supported by detailed analysis of the sensitivity of human circadian, neuroendocrine, and alerting responses to ocular light and provide a straightforward framework to inform lighting design and practice.
Citation: Brown TM, Brainard GC, Cajochen C, Czeisler CA, Hanifin JP, Lockley SW, et al. (2022) Recommendations for daytime, evening, and nighttime indoor light exposure to best support physiology, sleep, and wakefulness in healthy adults. PLoS Biol 20(3): e3001571. https://doi.org/10.1371/journal.pbio.3001571
Copyright: © 2022 Brown et al. This is an open access article distributed under the terms of the Creative Commons Attribution License , which permits unrestricted use, distribution, and reproduction in any medium, provided the original author and source are credited.
Funding: The 2nd International Workshop on Circadian and Neurophysiological Photometry (2019) was funded by the Biotechnology and Biological Sciences Research Council (UK) grant BB/N014901/1 to TMB and RJL, with additional contributions from the University of Manchester Centre for Biological Timing and the Neuroscience and Mental Health Domain. The funders had no role in study design, data collection and analysis, decision to publish, or preparation of the manuscript.
Competing interests: I have read the journal’s policy and the authors of this manuscript have the following competing interests: GCB was supported, in part, by DOE grant DE- EE0008207, NAS Award #HR 05-23 UNIT 905; NASA grant NNX15AC14G; the NSF ERC LESA Center; BIOS, Toshiba Materials Science, The Nova Institute (The Institute for Integrative Health) and the Philadelphia Section of the Illuminating Engineering Society. GCB reports having issued (USPTO 7,678,140 and 8,366,755) and pending (USPTO 16/252,300, 15/085,522 and 14/273,971) patents related to the photoreceptor system for melatonin regulation. That intellectual property has been licensed by Litebook Company Ltd. He has been a paid consultant by Lutron, Inc., and McCullough Hill LLC. In addition, he and his research program have received financial, material and travel support from a range of federal, industrial, legal and philanthropic organizations in the past and present. CC has received consulting, honoraria, travel, accommodation and/or subsistence fees for invited keynote lectures, conference presentations or teaching from Toshiba Materials, Velux, Firalux, Lighting Europe, Electrosuisse, Novartis, Roche, Elite, Servier, and WIR Bank. CC is a member of the Daylight Academy. CAC reports grants to BWH from FAA, NHLBI, NIA, NIOSH, NASA, and DOD; is/was a paid consultant to AARP (2018), American Academy of Dental Sleep Medicine (2017, 2018), Eisenhower Medical Center (2018), Emory University (2019), Ganésco, Inc. (2017), Inselspital Bern (2019), Institute of Digital Media and Child Development (2017, 2018, 2019), Klarman Family Foundation (2017, 2018, 2019), M. Davis and Co (2018), Physician’s Seal (2019), Samsung (2016), Sleep Research Society Foundation (2019), State of Washington Board of Pilotage Commissioners (2018), Tencent Holdings Ltd (2019), Teva Pharma Australia (2019, 2020), UC San Diego (2018), University of Michigan (2017), University of Washington (2017, 2018), and Vanda Pharmaceuticals Inc (2017, 2018, 2019, 2020), in which CAC also holds an equity interest; received travel support from Annenberg Center for Health Sciences at Eisenhower (2018), Aspen Brain Institute (2018), Bloomage International Investment Group, Inc. (2018, 2019), UK Biotechnology and Biological Sciences Research Council (2019), Bouley Botanical (2017, 2018, 2019), Dr. Stanley Ho Medical Development Foundation (2019), European Biological Rhythms Society (2017, 2019), German National Academy of Sciences (Leopoldina) (2019), Illuminating Engineering Society (2018), National Safety Council (2017, 2018, 2019), National Sleep Foundation (2017, 2018, 2019), Society for Research on Biological Rhythms (2018), Sleep Research Society Foundation (2018), Stanford Medical School Alumni Association (2019), Tencent Holdings Ltd (2019), University of Zurich (2018), and Vanda Pharmaceuticals Inc (2017, 2018, 2019), Ludwig-Maximilians-Universität München (2018), National Highway Transportation Safety Administration (2018), Office of Naval Research (2018), Salk Institute for Biological Studies/Fondation Ipsen (2018), The National Academy of Sciences, Engineering, and Medicine (2017), The Wonderful Company (2017), Department of Defense (2017); receives research/education support through BWH from Cephalon, Mary Ann & Stanley Snider via Combined Jewish Philanthropies, Harmony Biosciences LLC (2019, 2020), Jazz Pharmaceuticals PLC Inc (2017, 2018, 2019, 2020), Johnson & Johnson (2019), NeuroCare, Inc. (2019), Philips Respironics Inc/Philips Homecare Solutions (2017, 2 018, 2019, 2020), Regeneron Pharmaceuticals (2018, 2019, 2020), Regional Home Care (2019), Teva Pharmaceuticals Industries Ltd, Sanofi SA, Optum, ResMed, San Francisco Bar Pilots, Sanofi, Schneider, Simmons, Sysco, Philips, Vanda Pharmaceuticals; is/was an expert witness in legal cases, including those involving Advanced Power Technologies, Aegis Chemical Solutions LLC (2019), Amtrak (2019); Casper Sleep Inc (2019), C&J Energy Services (2019), Complete General Construction Co (2017), Dallas Police Association (2019), Enterprise Rent-A-Car (2019), Espinal Trucking/Eagle Transport Group LLC/Steel Warehouse Inc (2017, 2018, 2019), FedEx, Greyhound Lines Inc/Motor Coach Industries/FirstGroup America (2017, 2018, 2019), Pomerado Hospital/Palomar Health District (2017, 2018), PAR Electrical Contractors Inc (2019), Product & Logistics Services LLC/Schlumberger Technology Corp/Gelco Fleet Trust (2019), Puckett Emergency Medical Services LLC (2019), South Carolina Central Railroad Company LLC (2017, 2018), Union Pacific Railroad (2019), United Parcel Service/UPS Ground Freight Inc (2017, 2018), and Vanda Pharmaceuticals (2019, 2020); serves as the incumbent of an endowed professorship provided to Harvard University by Cephalon, Inc.; and receives royalties from McGraw Hill, and Philips Respironics (2017, 2018, 2019, 2020) for the Actiwatch-2 and Actiwatch Spectrum devices. CACs interests were reviewed and are managed by the Brigham and Women’s Hospital and Mass General Brigham in accordance with their conflict of interest policies. SWL has received consulting fees from Atlanta Falcons, Atlanta Hawks, BHP Billiton, Delos Living LLC, EyeJust Inc., McCullough Hill Leary PS, Noble Insights, OpTerra Energy Services Inc., Pegasus Capital Advisors, Phillips Lytle LLP, Plan LED, Rec Room, Serrado Capital, Slingshot Insights, Stantec and Team C Racing. He has current consulting contracts with Akili Interactive, Apex 2100 Ltd, Consumer Sleep Solutions, Headwaters Inc., Hintsa Performance AG, KBR Wyle Services, Light Cognitive, Lighting Science Group Corporation/HealthE, Look Optic, Mental Workout/Timeshifter, Paul Weiss Rifkind Wharton & Garrison LLP, Six Senses, and View Inc. SWL has received unrestricted equipment gifts from Bionetics Corporation and F.LUX Software LLC; a fellowship gift from Stockgrand Ltd; has equity in iSLEEP, Pty; royalties from Oxford University Press; honoraria plus travel, accommodation and/or meals for invited seminars, conference presentations or teaching from Estée Lauder, Ineos, Informa Exhibitions, MIT, Roxbury Latin School, and Teague; travel, accommodation and/or meals only (no honoraria) for invited seminars, conference presentations, teaching or editorial duties from DIN, Emory University, Lightfair, SLTBR, Solemma and Wiley. SWL has an ongoing investigator-initiated grant from F. Lux Software LLC and a Clinical Research Support Agreement from Vanda Pharmaceuticals Inc. SWL holds a process patent for ‘Systems and methods for determining and/or controlling sleep quality’, which is assigned to the Brigham and Women’s Hospital per Hospital policy, and ‘Method and system for generating and providing notifications for a circadian shift protocol’ held by Mental Workout Inc. SWL has served as a paid expert in legal proceedings related to light, health and work patterns. SWL was the Program Leader for ‘Safety and Productivity Improvements’ in the CRC for Alertness, Safety and Productivity from 2015-2019, through a part-time Adjunct Professor appointment at Monash University, Australia. SWL’s interests are reviewed and managed by Brigham and Women’s Hospital and Partners HealthCare in accordance with their conflict of interest policies. RJL and TMB have received research funding from Signify (formerly Philips Lighting). RJL has received speaker fees from Samsung. RJL, LP, LJMS and MS have served as members of the CIE Joint Technical Committee 9 on the definition of CIE S 026:2018. These were unpaid roles. JPH was supported, in part, by DOE grant DE- EE0008207, NAS Award #HR 05-23 UNIT 905; NASA grant NNX15AC14G; NSF Award #2037357; Rensselaer Polytechnic Institute; BIOS, Toshiba Materials Science, The Institute for Integrative Health; and the Philadelphia Section of the Illuminating Engineering Society. He has been a paid consultant by Lutron, Inc. and McCullough Hill LLC. MM is an unpaid founding member of the Daylight Academy (DLA). SNP reports no conflicting interests. JOH, In addition to paid employment with Public Health England, is Vice-President Standards of the CIE, a member of the Scientific Expert Group of the International Commission on Non-Ionizing Radiation Protection and a number of their Project Groups, Co-Convenor of an ISO committee on Integrative Lighting, a member of two committees of the Illuminating Engineering Society of North America, Convenor of an IEC committee and a member of a Core Group for the World Health Organization, all as unpaid roles. LP served as the CIE reporter to CIE TN 003:2015 on the first Manchester Workshop in 2013, is currently serving as Director and as Secretary of the CIE Division “Photobiology and Photochemistry”, as the CIE reporter on the CIE S 026 Toolbox ( doi.org/10.25039/S026.2018.TB ), as a member of CIE Joint Technical Committee 14 (working with ISO Joint Working Group 4) on Integrative Lighting, and as the CIE reporter on the second Manchester Workshop 2019 (attended by all the authors), all as unpaid roles LJMS’s full time position at Eindhoven University has been partially funded by Signify, he is also active in various unpaid roles within the International Commission on Illumination (CIE). DJS is a co-inventor on issued patents (EP1614441A1 and WO2015052207A1), a Founding member of the Daylight Academy and reports receiving research support from BBSRC and EU H2020-SC1-2020-Two-Stage-RTD, ENLIGHTENme, Innovative policies for improving citizens’ health and wellbeing addressing indoor and outdoor lighting (No. 945238). CV is an unpaid member of the Circadian Light Therapy (Inc.) and the Chronsulting Scientific Advisory Boards. In addition, CV’s research and scholarship is funded by the University of Colorado Boulder, the Colorado Clinical and Translational Sciences Institute, the National Institutes of Health, and the Department of Energy. MS is currently an unpaid member of CIE Technical Committee TC 1-98 ("A Roadmap Toward Basing CIE Colorimetry on Cone Fundamentals"). MS was an unpaid advisor to the Division Reportership DR 6-45 of Division 3 ("Publication and maintenance of the CIE S026 Toolbox"). Between 2017 and 2020, MS was elected Chair of the Color Technical Group within the Optical Society. Since 2020, MS is an elected member of the Daylight Academy, an unpaid member of the Board of Advisors of the Center for Environmental Therapeutics, and MS is a member of the Technical Advisory Board of Faurecia IRYStec Inc. Over the past two years (2019-2020), MS has received industrial research support from f.lux software LLC, Ocean Insight, and BIOS Lighting. MS reports funding from the Wellcome Trust, the Royal Society, EPSRC, Bioscientifica Trust, Fight for Sight, Freie Akademische Gesellschaft Basel, and the University of Oxford. PCZ reports funding from National Institutes of Health, Eisai, Philips, Jazz Pharmaceuticals, Technogel, Harmony Biosciences, Apnimed, X – a Division of Alphabet, Inc., Merck and Sanofi. In addition, PCZ has patent applications pending (62/038700; 15/517458). KPW reports during the conduct of the 2nd International Workshop and preparation of this manuscript being a board member of the Sleep Research Society; chair of the American Academy of Sleep Medicine Clinical Practice Guideline for the Treatment of Adults with Shift Work Disorder and Jet Lag Disorder Workgroup; receiving research support from the NIH, the Office of Naval Research, the PAC-12 conference, and consulting for Circadian Therapeutics, LTD., Circadian Biotherapies, Inc. Philips Respironics, U.S. Army Medical Research and Materiel Command-Walter Reed Army Institute of Research outside the submitted work.
Abbreviations: ANSI, American National Standards Institute; CEN, Comité Européen de Normalisation; CIE, Commission Internationale de l’Eclairage; DIN, Deutsches Institut für Normung; EEG, electroencephalogram; ICNIRP, International Commission on Non-Ionizing Radiation Protection; IES, Illuminating Engineering Society; ipRGC, intrinsically photosensitive retinal ganglion cell; ISO, International Organization for Standardization; melanopic DER, melanopic daylight efficacy ratio; melaponic EDI, melanopic equivalent daylight illuminance
Introduction
Besides supporting visual perception, ocular light exposure influences many aspects of human physiology and behaviour, including circadian rhythms, sleep, and alertness (both via circadian system–dependent and circadian system–independent routes), mood, neuroendocrine, and cognitive functions (reviewed in [ 1 – 4 ]). This array of retinally driven responses to light (collectively termed “non–image-forming” or, as used here for brevity, “nonvisual”) are important determinants of health, well-being, and performance, and some are already clinically relevant, as evidenced by current light therapy for circadian rhythm sleep disorders and various forms of depression [ 5 – 7 ]. Industrialisation and urbanisation have progressively and dramatically altered individuals’ light exposures, resulting in less light, including natural light, during the daytime and less darkness during the night, due to spending more time indoors where electric lighting provides the dominant source of illumination. Substantial evidence indicates that such altered light exposure patterns (and associated circadian/sleep disruption) contribute to negative impacts on health, sleep, and productivity, ranging from acute increases in accident risk to increased incidence of cardiometabolic disorders and forms of cancer (reviewed in [ 8 – 14 ]). Therefore, there is an urgent need for evidence-led recommendations to help inform the design and application of light emission technologies and human light exposures.
To date, a key challenge when optimising light exposure for promoting human health, well-being, and performance has been the lack of an accepted scientific framework upon which to quantify the propensity for light to elicit the relevant responses and from which to base recommendations for lighting design and practice. Fortunately, as a result of several decades of scientific advances, research-based recommendations are now possible.
Building on initial observations that physiological responses to ocular light exposure can persist even in people who are totally visually blind [ 15 – 17 ], convergent evidence from studies of humans and animals has shown that such nonvisual responses (including effects on the circadian system, melatonin secretion, sleep/alertness, and pupil constriction) originate via a specialised class of retinal neurons, the intrinsically photosensitive retinal ganglion cells (ipRGCs) [ 18 – 26 ]. The light-sensing photopigment within the ipRGCs is melanopsin, which, in humans, is maximally sensitive to photons in a distinct portion of the visible spectrum to the cone photopigments ( λ max ≈ 480 nm before accounting for filtering through the lens and ocular media) [ 23 , 25 , 27 ]. As a result, the established photometric quantities used to describe brightness and luminous sensation as perceived by humans do not adequately reflect the spectral sensitivity of any melanopsin-dependent responses to light. Measures such as photopic (il)luminance, which primarily reflect the spectral sensitivity of long and medium wavelength sensitive cones, place substantially greater weight on longer wavelengths than those to which melanopsin is most sensitive. These measures therefore provide an inappropriate surrogate for quantifying the propensity of light to engage ipRGC-driven circadian, neuroendocrine, and neurobehavioural responses ( Fig 1A ).
- PPT PowerPoint slide
- PNG larger image
- TIFF original image
https://doi.org/10.1371/journal.pbio.3001571.g001
While the potential value of a melanopsin-based photometric quantity has been recognised for some time, there has also been uncertainty as to whether this provides a sufficiently detailed model of the spectral sensitivity of human ipRGC-driven responses to ocular light exposure [ 28 ]. Hence, while the spectral sensitivity of physiological responses to light in visually blind people and animals matches that expected for melanopsin [ 20 , 23 , 25 , 29 ], in the fully intact retina, ipRGCs can also receive signals originating from rods and/or cones [ 26 ]. Moreover, available data indicate that the relative contributions of melanopsin and rod/cone photoreception to nonvisual ocular light responses, and consequently their apparent sensitivity, may vary as a function of exposure duration, light intensity, and perhaps time of day and/or prior light exposure [ 25 , 28 , 30 – 33 ].
As an initial response to the absence of a suitable metric for quantifying ipRGC-dependent ocular light responses, in 2013, an expert working group proposed a system that weighted irradiance according to the effective in vivo spectral sensitivity of the 5 known human retinal opsin proteins (melanopsin, rhodopsin, S-, M-, and L-cone opsin) [ 28 ]. This framework has now been formalised into an international standard with a SI-compliant system of metrology for ipRGC-influenced responses to light (Commission Internationale de l’Eclairage (CIE) S 026 [ 34 ]). Within this system, the effective rates of photon capture for each of the human retinal opsins under a given light condition are equated to the photopic properties (e.g., illuminance) of a standard 6500 K (D65) daylight spectrum that would produce the same rate of photon capture. This approach defines, for each opsin class, the α-opic equivalent daylight illuminance (EDI; where α-opic denotes one of the 5 human opsin classes that can contribute to ipRGC-influenced responses, e.g., melanopic; Fig 1B ). Despite the significant advance provided by this new light measurement standard, to date, explicit scientific consensus guidance on the relationship between the 5 α-opic quantities and the magnitude of practically relevant ipRGC-dependent responses is lacking. For example, how should signals from melanopsin, cones, and rods be weighted? Do these weightings change with light exposure duration and history? What levels of α-opic EDI are appropriate in a given time of day and setting?
Importantly, as originally envisaged [ 28 ], adoption of the new measurement approach has facilitated a number of large-scale retrospective evaluations of historical data [ 35 – 39 ] and informed new hypothesis-driven investigations [ 40 – 43 ] on the photoreceptive physiology for circadian, neuroendocrine, and neurobehavioural responses in humans. In total, the evidence from such studies [ 35 – 43 ] supports the view that, under most practically relevant situations (extended exposures to polychromatic light in the absence of pharmacological pupil dilation), light sensitivity of human physiological responses can be reliably approximated by the α-opic irradiance for melanopsin or the corresponding EDI (melanopic EDI). Moreover, based on the consistency of melanopic irradiance–response relationships across studies [ 38 ], it is now possible to define realistic, evidence-based recommendations for light exposures that target nonvisual responses ( Fig 2 ). Alongside the emergence of freely available tools to calculate the relevant metrics [ 44 , 45 ], there now exists an easily measured and internationally accepted SI-compliant system of metrology [ 34 ] to inform lighting design and associated policy.
Data are derived from laboratory studies (in humans without the use of pupil dilators) investigating the impact of long exposures (>2 hours) to primarily broadband light sources on melatonin suppression [ 69 , 88 , 95 , 143 , 144 ] ( A ), circadian phase resetting [ 83 , 89 , 143 , 144 ] ( B ), and subjective alerting responses [ 86 ] ( C ), as analysed in [ 38 ]. Group data (round symbols) are presented as mean ± SD; otherwise, data for individual subjects are presented (square symbols). Shading represents the 95% confidence limits of an unconstrained 4-parameter sigmoid fit to the data. For comparison across different response types ( D ), data sets from A – C were normalised relative to the range of the curve fit for that response type. Shaded areas in D reflect the consensus recommendations of the Second International Workshop on Circadian and Neurophysiological Photometry for sleep, evening, and indoor daytime environments. Recommendations are intended to provide realistic targets that minimise inappropriate nonvisual responses in the sleep environment (melanopic EDI <1 lux) and reduce these so far as is practically possible presleep (3 hours before habitual sleep; melanopic EDI <10 lux) while maximising relevant effects across the intervening daytime hours (melanopic EDI >250 lux). The nonshaded region indicates the range of melanopic EDI that should, where possible, be avoided during evening and nighttime and are considered suboptimal for daytime environments. EDI, equivalent daylight illuminance.
https://doi.org/10.1371/journal.pbio.3001571.g002
Here, we describe expert consensus-based recommendations for daytime, evening, and nighttime light exposure, considerations associated with their applicability, the supporting scientific evidence, and any caveats associated with the recommendations as they stand.
Methodology
The Second International Workshop on Circadian and Neurophysiological Photometry in 2019 brought together experts in lighting, neurophysiological photometry and sleep, and circadian research (all workshop participants are included as authors of this manuscript). The workshop was chaired by Brown and Wright who invited participants based on professional and/or academic qualifications and on reputation of being a leading expert in the field, including being an author of key scientific publications and/or international standards on the topic. Workshop participants were provided goals and key questions to address prior to a structured face-to-face meeting. The primary focus of the meeting was to develop expert consensus recommendations for healthy daytime and evening/nighttime light environments tentatively based on the new SI-compliant measurement system (CIE S 026:2018) [ 34 ]. Initial questions for review and discussion were the following:
- What range of melanopic EDI can be reasonably considered to provide minimal and maximal impacts on nonvisual ocular light responses in humans?
- Do signals from rods and/or cones also play a major role, and, if so, what relevant guideline levels could be recommended to account for such actions?
- Do the answers to (1) and/or (2) vary across different nonvisual forming responses (e.g., circadian entrainment/resetting, sleep/arousal, effects on hormone secretion, and mood) and, if so, what is the most appropriate general recommendation that can be provided?
Participants were also asked to consider if recommended light exposures would vary depending on which specific biological effects one is trying to achieve and/or on the target population (e.g., shift workers, specific clinical applications, etc.) and to include empirical literature supporting their views. In the face-to-face meeting, the morning of the first day was devoted to detailed presentations and discussion of the relevant scientific literature, and the afternoon was devoted to breakout sessions for discussion of questions 1 to 3 noted above. The second day was devoted to further discussion with sufficient time to address all opinions, ideas, and concerns. Voting to determine the expert consensus recommendations occurred via an iterative process; voting was limited to workshop participants and, where consensus could not be initially reached, discussion and review of the relevant literature resumed until participants were in agreement. Following the establishment of the expert consensus recommendations, a writing plan was formulated to produce the current paper. Subgroups of workshop participants initially drafted sections of the manuscript most relevant to their specialist expertise, including providing accounts of the scientific evidence from laboratory and field studies, relevance to other existing visual standards, and other special considerations associated with application of the recommendations. The workshop chairs (Brown and Wright) then integrated the expert content into a complete draft manuscript, including the recommendations formalised during the meeting. Workshop participants reviewed, edited, and approved both the draft (available as a preprint [ 46 ]), and this final version, which provides additional rationale supporting the recommendations and their practical application. The recommendations and associated considerations described herein are therefore the product of a workshop involving the authors. We are aware that such a workshop can rarely be exhaustive with respect to expertise and/or views across all potential stakeholders.
Expert consensus-based recommendations
The recommendations, described below, are intended to provide realistic targets that will result in appropriate circadian, neuroendocrine, and neurobehavioural responses to ocular light exposure in humans.
Daytime light recommendations for indoor environments
Throughout the daytime, the recommended minimum melanopic EDI is 250 lux at the eye measured in the vertical plane at approximately 1.2 m height (i.e., vertical illuminance at eye level when seated). If available, daylight should be used in the first instance to meet these levels. If additional electric lighting is required, the polychromatic white light should ideally have a spectrum that, like natural daylight, is enriched in shorter wavelengths close to the peak of the melanopic action spectrum ( Fig 1A ).
Evening light recommendations for residential and other indoor environments
During the evening, starting at least 3 hours before bedtime, the recommended maximum melanopic EDI is 10 lux measured at the eye in the vertical plane approximately 1.2 m height. To help achieve this, where possible, the white light should have a spectrum depleted in short wavelengths close to the peak of the melanopic action spectrum.
Nighttime light recommendations for the sleep environment
The sleep environment should be as dark as possible. The recommended maximum ambient melanopic EDI is 1 lux measured at the eye.
In case certain activities during the nighttime require vision, the recommended maximum melanopic EDI is 10 lux measured at the eye in the vertical plane at approximately 1.2 m height.
Additional considerations
- Exposure to a stable and regular daily light–dark cycle is also likely to reinforce good alignment of circadian rhythms, which may further benefit sleep, cognition, and health. These recommendations should therefore be applied at the same time each day, so far as possible.
- These recommendations are not intended to supersede existing guidelines relating to visual function and safety. The nonvisual ocular light responses covered here should be an additional level of consideration provided that relevant visual standards can still be met.
- These recommendations are derived based on data from (and intended to apply to) healthy adults (aged 18 to 55) with regular daytime schedules. Special considerations may apply to specific populations (e.g., children, older people, shift workers, or other individuals whose light sensitivity deviates substantially from an “average” healthy adult) as discussed later in this publication (see “Special cases and exceptions”).
Relationship to existing standards
There are several national and international standards that are relevant to indoor light exposure in the built environment, which have been developed under rigorous due processes, consensus, and other criteria. In terms of biological safety, there is a recent recommended practice for photobiological safety that provides guidance on ocular and dermal health relative to light exposure from all varieties of indoor lamps and lamp systems (American National Standards Institute/Illuminating Engineering Society (ANSI/IES) RP-27-20) [ 47 ]. The International Commission on Non-Ionizing Radiation Protection (ICNIRP) has also released a recent statement concerning photobiological safety, specifically of light exposure from LEDs [ 48 ]. Other existing guidelines, codes, and specifications for lighting installations in indoor places primarily concentrate on visual function, including visual comfort, visual performance, and seeing safely for people with normal, or corrected to normal, vision.
Current specifications within lighting practice are based on illuminance and several additional qualitative and quantitative needs concerning glare, colour rendering, flicker and temporal light modulation, luminance distribution, and the directionality and variability (of both colour and level) of light. These specifications are crafted to enable people to perform their visual tasks accurately and efficiently, even for difficult circumstances or extended durations (e.g., Deutsches Institut für Normung (DIN) SPEC 67600 [ 49 ]; ANSI/IES RP-28-16 [ 50 ]; and EN 12464–1 [ 51 ]). Together with the focus on energy saving, the existing guidelines restrict the illuminance indoors to levels that are typically at least 1 order of magnitude below the natural light environment outdoors. Moreover, the electrical light sources in most common use, while optimised for their visual qualities, are typically substantially less efficient at stimulating melanopsin than natural daylight of equivalent illuminance, i.e., the light they provide has a low ratio of melanopic EDI to photopic illuminance (quantified by the melanopic daylight efficacy ratio (melanopic DER) [ 34 , 52 ]; see Fig 3 ). This leaves us with an indoor light environment that is potentially suboptimal for supporting human health, performance, and well-being [ 9 – 12 , 53 ]. For example, Comité Européen de Normalisation (CEN) guidelines specify a minimum task plane photopic illuminance of 500 lux for writing, typing, reading, and data processing tasks. When just meeting this illuminance threshold with regular lighting (i.e., melanopic DER well below 1; Fig 3A and 3B ), typical (vertical) melanopic EDIs encountered across the working day will fall below 200 lux (e.g., [ 54 – 56 ]). Moreover, specified illuminance levels for other settings, where visual demands are lower (e.g., corridors, rest rooms, etc.), will typically be substantially lower than the above (melanopic EDI <200 lux; [ 51 ]).
https://doi.org/10.1371/journal.pbio.3001571.g003
This publication is centrally based on an internationally balloted standard from the CIE [ 34 ], which now provides an accepted framework upon which to derive lighting specifications that optimise visual, circadian, neuroendocrine, and neurobehavioural responses to light. The corresponding expert-led consensus recommendations for biologically appropriate lighting are reflected in general melanopic EDI thresholds for various times of day/night. The recommendations presented here are intended to be widely achievable within the constraints of other relevant lighting guidelines (e.g., via lighting of appropriate spectral composition; Fig 3C and 3D ) and to provide a sound scientific basis for the formal development of recommended practices in light and lighting from national and international standards organisations (e.g., ANSI, CIE, DIN, IES, and the International Organization for Standardization; ISO).
In closing this section, we note that a number of other recommendations relevant to physiological and neurobehavioural effects of light have been proposed in recent years, including some guidelines and specifications by commercial (for-profit) entities (reviewed in [ 57 ]). Unlike these previous suggestions, the present recommendations are both built around an SI-complaint, internationally accepted and validated measurement system and are supported by expert scientific consensus, features recognised as critical by established industry regulatory and standardisation bodies [ 58 , 59 ].
Practical considerations
As noted above, while the recommendations detailed here are expected to be widely achievable, implementing these in any real-world setting necessitates care not to compromise other important regulations and/or considerations (e.g., visual appearance, glare, thermal comfort, safety, and energy efficiency). For example, an important consideration in achieving our recommendations for daytime settings is whether this would necessitate higher overall light levels and therefore increase energy expenditure and/or the risk of visual discomfort (e.g., glare). Notably, there is a range of approaches that (individually or in combination) could allow these recommendations to be met while avoiding such issues, including increasing the availability and accessibility of natural daylight (e.g., [ 56 ]), engineering the spectral content of electric lighting to increase melanopic DER, adjusting finishes and furnishings to optimise surface reflectances, and adjusting the placement, angular dispersion and size of accessible luminous surfaces to enhance vertical illuminances and/or minimise glare [ 60 – 64 ].
As an illustration of the above, a recent study modelling common office and educational settings found a combination of adjustments in surface finishes and spectral composition of lighting could readily produce 2- to 3-fold changes in vertical melanopsin-weighted light exposure [ 62 ]. For the conditions assessed there, where relevant national standards specified horizontal illuminances of 300 to 400 lux, achieving an average melanopic EDI of 250 lux in the vertical plane required an approximately 50% increase in horizontal illuminance and energy output when using an ‘off-the shelf’ tuneable white LED (6,500 K; melanopic DER = 0.83). With appropriate design, however, colour mixed LEDs can allow much higher melanopic DERs, even when maintaining warmer colour temperatures (e.g., up to 1.4 for 4,000 K sources [ 61 ]). Moreover, engineered LED luminaires that balance less extreme increases in melanopic DER with a good fidelity colour rendition index and slightly cooler white light [ 65 ] can facilitate meeting our daytime recommendations without significantly compromising energy expenditure or visual qualities (e.g., Fig 3D ). Further, the energy efficiency of colour-mixed LED sources is rapidly approaching that typical of standard phosphor-converted LEDs and is scheduled to exceed this over the next decade [ 66 ]. Thus, while optimising building and lighting design to maximise energy efficiency and minimise visual discomfort remain important goals, these should not ultimately prove impediments to implementing our recommendations in most settings.
By contrast, increased energy expenditure is not a concern with respect to our evening/nighttime recommendations, where the significant practical consideration is rather ensuring that there is sufficient light to comfortably and safely perform visually guided activities. For the sleep environment, it is already natural to greatly minimise light exposure (by turning off lights, covering windows, and the like). There is likely still a fair proportion of individuals for whom the sleep environment is currently slightly above a melanopic EDI of 1 lux (e.g., [ 67 68 ]), although we would not envisage any significant barriers to reducing this where required (e.g., via use of blackout blinds and the installation of orientation lighting where needed). Outside of the sleep environment, however, ensuring sufficient light is available for vision is of course essential.
From existing ambulatory field assessments, evening (photopic) illuminance is commonly reported in the order of 30 lux [ 69 – 73 ]. Although certain rooms (e.g., kitchens) may be more brightly lit, this value of 30 lux corresponds to vertical illuminances typically measured in most indoor domestic environments in the evening [ 74 ]. In such cases, meeting the threshold melanopic EDI of 10 lux need not require any significant change in overall illuminance. Hence, many commonly used domestic warm white (2,700 to 3,000 K) LEDs already have a melanopic DER sufficiently low (<0.35 [ 52 ]) to meet our target while maintaining an illuminance of approximately 30 lux. Consistent with this view, a recent study that assessed evening light exposure in home settings via wearable spectrophotometers found that in nearly 50% of occasions melanopic EDI was already at or below 10 lux [ 75 ]. Moreover, many cases where evening light exposure was above this level involved lighting enriched in shorter wavelengths and could, in principle, been brought in line with our recommendations simply by using lower melanopic DER light sources. Further, the use of appropriate task lighting and/or lighting specifically engineered to minimise melanopic output (e.g., [ 69 ]) may further support activities that benefit from illuminances above 30 lux while maintaining an overall environment where melanopic EDI at the eyes remains below 10 lux (although the latter ultra-low melanopic DER sources will likely come at the expense of reduced colour discrimination). A particular challenge, however, comes from indoor environments outside the home (e.g., spaces shared by individuals with radically different daily/work schedules), where existing visual standards will often specify illuminance levels (>100 lux) that cannot be achieved while maintaining a vertical melanopic EDI <10 lux and optimal colour discrimination. Nonetheless, while meeting our evening target may not be achievable in all instances, it should be broadly achievable in most domestic settings with currently available lighting technology and, therefore, for those with the regular daytime work schedules for which it is intended to apply.
A final point for consideration relates to the likely benefits of implementing our recommendations that may have to be weighed up to justify any associated costs (e.g., due to upgrading workplace lighting). As discussed in detail below, there is certainly evidence that increased daytime light can improve subjective or objective measures of performance, sleep, alertness, and/or mood and that decreased evening and nighttime exposures can reduce adverse effects of light on sleep, circadian rhythms, and long-term health (see “Evidence from real-world settings”). Directly quantifying the benefits that might be expected associated with implementing our recommendations is far more challenging. In the future, large-scale longitudinal studies that combine data on objectively measured performance (e.g., sick days, productivity, and incidence of accidents), health outcomes, and appropriately measured (personal) light exposure may provide such information. In the interim, it is worth noting that, even ignoring possible impacts on the incidence of common and costly health complications associated with circadian disruption (e.g., cardiovascular disease, diabetes, and cancer), benefits associated with improved sleep alone could potentially be substantial [ 76 ]. Indeed, insufficient sleep is estimated to cost the United States 2.4% GDP, due absenteeism, accidents, reduced productivity, etc. [ 76 , 77 ]. Moreover, even comparatively modest improvements for those with poor sleep (<6 hours sleep/night increased to 6 to 7 hours/night) are predicted to increase US GDP in the order 1.7% or approximately 300 billion USD/year [ 77 ].
Scientific rationale
Evidence from laboratory studies.
The rationale for basing these recommendations upon melanopic EDI is, in the first instance, provided by a comprehensive analysis of data aggregated from controlled laboratory studies (performed in healthy adults aged 18 to 55) that have evaluated the 2 best understood neuroendocrine and circadian light responses in humans: acute suppression of nocturnal pineal melatonin production and circadian phase resetting by evening or nighttime light exposure [ 36 – 39 ]. Those data indicate that, for a wide range of monochromatic, narrowband and broadband light sources and exposure durations, such ocular light responses are better predicted by melanopic irradiance than by photopic illuminance or other existing metrics. Additional contributions from photoreceptors other than melanopsin are expected based on known ipRGC biology [ 26 , 28 ], and evidence for such contributions has been observed under certain circumstances [ 30 , 78 , 79 ]. Importantly, however, the sum of empirical human data suggest that any such influences are sufficiently limited that, under most practically relevant circumstances, the spectral sensitivity of circadian and neuroendocrine and, by extension, other related nonvisual responses to ocular light exposure, can be well approximated by melanopic EDI.
The clearest evidence for contributions from photoreceptors other than melanopsin has so far come from evaluations of melatonin suppression in short (<1 hour) time windows following exposures to monochromatic light in participants with dilated pupils (to remove indirect effects of pupil constriction on apparent sensitivity). Data from 2 such studies are compatible with the possibilities that S-cones [ 78 ] or the photopic system [ 30 ] may contribute alongside melanopsin (see also reanalysis in [ 79 ]). Importantly, however, a large body of data with and without use of pupil dilation indicates that for exposures of an hour or more, melatonin suppression can be reliably predicted by melanopic EDI [ 37 , 38 , 80 , 81 ]. This conclusion is further strengthened by findings from recent studies that have employed photoreceptor isolating stimuli to confirm that melanopsin-selective changes in irradiance modulate melatonin production [ 40 , 41 ] but failed to find any effect of large variations in irradiance selectively targeting S-cones [ 42 ]. Further evidence consistent with a dominant role for melanopsin comes from earlier observations that totally blind humans (where remaining light responses match the spectral sensitivity expected for melanopsin) [ 23 , 25 ] can display near-full melatonin suppression [ 15 , 17 , 23 ], as do individuals with colour vision deficiencies [ 82 ].
In line with the data discussed above, totally blind individuals can also display circadian phase resetting responses to bright white light of comparable magnitude to sighted individuals [ 16 ]. Findings from one study in sighted individuals with pharmacologically dilated pupils are suggestive of cone contributions to circadian phase resetting following long (6.5 hours) exposures to dim monochromatic light [ 30 ]. However, an equivalent effect is not readily apparent across data from studies performed on participants with undilated pupils [ 38 , 83 , 84 ]. Thus, laboratory data collected under conditions that are more relevant to the real world, where pupils are freely light responsive, indicate that the influence of cones is sufficiently small that melanopic irradiance can provide a reliable approximation of the spectral sensitivity of circadian phase resetting.
By contrast to the circadian and neuroendocrine responses discussed above, other relevant effects of light that are of importance but mechanistically less well understood, such as acute light effects on alertness, have not yet received the same degree of analytic and parametric study. Nonetheless, light-dependent changes in subjective alertness have commonly been reported (reviewed in [ 2 , 85 ]) and, where performed, functional studies employing electroencephalogram (EEG) or magnetic resonance imaging approaches reveal clear neurophysiological correlates of such subjectively measured alertness changes (e.g., [ 43 , 86 , 87 ]).
With respect to the conditions under which such alerting effects occur, a recent comprehensive meta-analysis reveals that self-reported alerting responses to white light are commonly observable within a similar range of light intensities to those associated with effects on the circadian system (irrespective of time of day) [ 2 ]. Many of the original studies contributing to the latter analysis predate the discovery of melanopsin. It is possible, however, to obtain reasonable approximations of melanopic EDI from the photopic illuminance reported by earlier studies, by reference to the typical ratio of these 2 parameters expected for the relevant light sources (i.e., melanopic DER). For example, a recent meta-analysis [ 2 ] notes a significant subjective alerting effect of bright white light in almost 80% of studies (15 of 19) where the “dim” light condition was below 80 lux and the “bright” condition >500 lux (values that correspond to melanopic EDI of <50 lux and >250 lux, using a conservative melanopic DER of 0.6 and 0.5, respectively). Further, the published irradiance response data for subjective (and objective) alerting responses to nocturnal broadband white light exposure [ 86 ] align very well with the relationship between melanopic EDI and circadian-related responses determined from other studies that did not employ pupil dilation [ 38 , 88 , 89 ] ( Fig 2 ).
The recent meta-analysis discussed above [ 2 ], which could not reach definitive conclusions regarding spectral sensitivity of alerting responses, did not assess the extent to which the magnitude of alerting responses were predictable based on melanopic EDI. Nonetheless, the most informative studies included in that analysis [ 69 , 90 – 94 ] and other relevant studies and meta-analyses [ 36 , 38 , 39 , 69 , 95 ] indicate that alerting effects produced by light of varying spectral composition are certainly better predicted by melanopic irradiance than other available metrics. Moreover, recent studies provide evidence that selectively increasing melanopic irradiance, in the absence of changes in either illuminance or colour, can promote self-reported alertness during both day [ 43 ] and evening [ 40 ]. The former study also confirmed EEG correlates of enhanced daytime alertness via alpha attenuation test [ 43 ]. Collectively, these data do not exclude the possibility that cone signals might exert a greater influence over acute alerting responses to light than is apparent for circadian and neuroendocrine effects. Nonetheless, the bulk of available evidence supports the view that melanopic EDI is the best currently existing predictor of alerting responses to light and is relevant for both day and evening/nighttime scenarios. The currently available data do not provide any definitive evidence that the sensitivity of such alerting responses differs substantially relative to other melanopsin-driven responses to evening/nighttime light exposure ( Fig 2 ) or between night and day (reviewed in [ 2 , 85 ]). Accordingly, in the absence of new information, the sensitivity range defined for the more comprehensively studied circadian and neuroendocrine responses can be used as a sensible predictor of propensity of light to modulate alertness, regardless of time of day.
In sum, most of the available laboratory data suggest that melanopic EDI is a reliable index that provides a good approximation of the apparent spectral sensitivity of human circadian and acute nonvisual responses to ocular light exposure. In particular, for the extended exposures to polychromatic light that are relevant to everyday living environments, existing evidence indicates that any additional contributions from cones (or rods; whose spectral sensitivity is close to melanopsin [ 34 , 96 , 97 ]) do not compromise the predictive value of melanopic EDI.
As befitting a system evolved to optimise physiology and behaviour in anticipation of day–night transitions driven by the Earth’s rotation relative to the sun, the operating range of human circadian, neuroendocrine, and alerting responses to ocular light exposure spans the range of light intensities typically encountered between civil twilight and sunrise/sunset (i.e., melanopic EDI of approximately 1 to 1,000 lux; Fig 2 ). The recommendations indicated above are therefore intended to ensure that the sleeping environment is kept at a limit below which any appreciable nonvisual responses of this nature are elicited and to minimise negative effects of the light environment during sleep and presleep hours [ 98 ]. Similarly, recommendations for daytime and evening light exposure are intended, so far as practically possible, to respectively maximise and minimise any associated effects on sleep, alertness and the circadian system. By providing an appropriately marked day–night signal and reducing potential disruptive effects of evening light, collectively, these recommendations are expected to promote robust and appropriately timed circadian functions in most individuals [ 99 ], as well as to promote alertness throughout the day and support healthy sleep.
Also worthy of note here, a number of studies have provided evidence that undesirable effects of evening/nighttime light can be mitigated by brighter light exposure earlier in the day (e.g., [ 31 , 33 , 100 – 104 ]). While opposing actions of light exposure during morning/daytime and evening are a well-understood feature of circadian function [ 105 , 106 ], these modulatory effects also extend to more acute actions of evening light, such as its ability to suppress melatonin production. At present, the physiology responsible for such actions are not well understood, nor does currently available data enable a detailed assessment of the intensity and/or time range across which such effects operate. What is clear, however, is that modulatory effects of prior light exposure are certainly not limited to earlier parts of the day [ 33 , 104 ]. Accordingly, such observations suggest a further potential benefit of maintaining high melanopic light exposure throughout the day. The visual requirements necessary or desirable for some activities during later parts of the evening (e.g., relating to illuminance and/or colour) place a limit on the extent to which disruptive effects of white light can be entirely avoided simply by reducing melanopic EDI (e.g., using lighting with a lower melanopic DER). Higher levels of daytime light exposure may therefore help mitigate any disruptive effects associated with unavoidable light exposure in later parts of the evening.
Evidence from real-world settings
While our current understanding of the spectral sensitivity and dynamic range of circadian, neuroendocrine, and neurobehavioural light responses in humans is most directly informed by laboratory studies, our recommendations are also supported by field evaluations of the impact of environmental lighting.
Access to electric lighting is associated with reduced daytime and increased nighttime light exposure and altered sleep timing [ 107 – 110 ], with many individuals in modern society routinely experiencing melanopic EDI <250 lux during the day, especially those with delayed sleep schedules [ 72 , 73 ]. Accordingly, there have been a number of real-world studies implementing daytime high melanopic lighting interventions in workplaces, schools, and care homes that provide practical corroboration for the recommendation outlined above [ 111 ]. Such practically focused investigations were not designed to evaluate melanopsin contributions per se (with increases in melanopic EDI usually being accompanied by increases in colour temperature and/or illuminance), but such studies do provide valuable insight in likely real-world benefits associated with meeting our recommendations.
In offices, increasing the melanopic output of architectural lighting (approximately 2-fold) via short wavelength-enriched lamps (17,000 K; melanopic DER ≈1, versus 3,000 to 4,000 K; melanopic DER<0.6) had beneficial effects on self-reported alertness, performance, mood, and sleep quality [ 54 , 55 ]. Similarly, enhancing daytime melanopic exposure by increased access to natural daylight in the workplace improved sleep and objectively measured cognitive performance (higher-order decision-making) in office workers [ 56 ]. In these studies [ 54 – 56 ], the average melanopic EDI in the control working environment was <150 lux (standard 3,000 to 4,000 K fluorescent lighting; Fig 3A ), with the experimental “active” conditions increasing melanopic EDI to approximately 170 to 290 lux. Hence, modest and readily achievable adjustments to increase light exposure can be associated with measurable benefits, without any observable detrimental effects.
In schools, findings from a series of studies employing fluorescent lighting with various intensities and spectra indicate that settings with a higher melanopic output (melanopic EDI >500 lux) can improve measures of concentration and reading comprehension compared to current standard lighting (typically providing melanopic EDI <200 lux; [ 112 – 115 ]). Similar benefits of short wavelength-enriched (17,000 K) versus standard 4000 K fluorescent light on reducing sleepiness have also been shown in college-aged students during afternoon lectures [ 116 ]. Further, building on seminal work showing the benefits of increased daytime light levels for the elderly [ 117 ], several clinical trials have shown the benefits of enhanced melanopic light exposure during daytime hours on care home residents [ 118 – 120 ]. There is evidence of reduced circadian sensitivity/responsiveness to light in older adults (see “Special cases and exceptions”), including changes in lens transmission that could reduce effective retinal dose corresponding to a given melanopic EDI by approximately 50% relative to the (young) standard observer on which calculations are based [ 44 ]. Nonetheless, in these studies, compared to control conditions (typical daytime melanopic EDI <150 lux), implementation of higher melanopic, short-wavelength enriched, polychromatic lighting (5,500 to 17,000 K, providing melanopic EDI >250 lx) led to a range of improvements including reduced depression, agitation, and anxiety, better daytime activity and, in some studies, improved sleep quality.
Collectively, increasing melanopic light exposure during the day in line with our recommendations has been shown to benefit alertness, performance, and sleep in a wide range of real-world settings, even in the presence of daylight or stimulants such as caffeine or for younger or older age groups. Further, there is minimal evidence for negative effects of increased daytime melanopic light exposures. One care home study [ 120 ], where the brightest daytime light intervention was examined (bright 17,000 K lighting providing melanopic EDI approximately 900 lux), reported a reduction in sleep efficiency and quality when compared to standard 4000 K lighting (melanopic EDI approximately 100 lux). Further, in an office study of dayworkers where the melanopic EDI of control condition was already high (approximately 400 lux) further increases (melanopic EDI approximately 750 lux) associated with the use of an 8000 K lighting system appeared to prevent the normal seasonal advance in sleep timing [ 121 ]. While the latter could be considered beneficial, as it enhances circadian alignment to the working day, long-term effects of decoupling from seasonal environmental rhythms is, to date, unclear. Given these data, future research is warranted to identify the potential beneficial and adverse effects on human physiology, cognition, behaviour, and health of electric lighting that greatly exceeds our intensity recommendations.
In addition to reduced daytime light exposure, increased exposure to electric light in the evening and night is commonly considered to exert adverse effects on sleep, circadian rhythms, and health outcomes [ 8 – 11 , 67 , 68 , 122 ]. Indeed, even relatively low levels of light in the sleep environment (conservatively, melanopic EDI >3 lux) have been associated with impaired sleep and increased incidence of diabetes in large cohort studies [ 67 , 68 ]. Further, typical evening light levels often fall within the range where significant nonvisual responses would be predicted from laboratory studies [ 75 ]. For example, a significant source of evening light exposure is from visual displays, which in the absence of any other illumination, can provide melanopic EDI of >60 lux [ 52 , 123 , 124 ] (above the typical level of exposure required to produce half-maximal subjective alerting, melatonin suppressing, and circadian phase shifting responses in laboratory studies; Fig 2 ). Indeed, several studies have shown that light from modern visual displays is sufficient to reduce the evening rise in melatonin, impair sleepiness, and/or increase subjectively or objectively measured alertness [ 123 – 126 ]. Moreover, manipulations that reduce exposure to short wavelength light from such displays has, in some laboratory studies, been found to lessen these effects [ 125 , 126 ] as have selective reductions in melanopic output [ 40 ]. There have not yet been large-scale longitudinal field studies on how effective such manipulations might be, although it is noteworthy that the reductions in melanopic radiance achievable simply by adjusting the spectral content of current visual displays are modest (approximately 50% decrease). As such, we expect that such approaches will be most beneficial when combined with other strategies to minimise evening illumination (e.g., dimming of screens and low melanopic ambient lighting). In addition, the potential protective role of adequate daytime light exposure to attenuate adverse effects of evening and nighttime light exposure on circadian physiology requires future research.
Special cases and exceptions
While the current recommendations are intended to be widely applicable, the scientific underpinnings primarily derive from studies of neuroendocrine, circadian, sleep, and alerting responses to ocular light exposure in healthy young adults. Even among this group, findings from a recent laboratory study show significant (>10-fold) interindividual variations in sensitivity to white light–induced suppression of the evening rise in melatonin [ 88 ]. The physiology underlying this variability is currently unknown. Importantly, however, assuming such variability is indicative of that for the healthy adult populations contributing across the range of lab studies discussed above, it is inherently incorporated into our recommendations. Hence, targets for daytime and nighttime exposures are based on light intensities found to produce near maximal or minimal responses across the test population. With respect to the recommendation for evening settings, there may be more significant variability in the relative magnitude of circadian, neuroendocrine, and associated neurophysiological responses, based on the intraindividual differences noted above [ 88 ]. In the absence of any ready means for predicting individual differences in sensitivity, the present recommendation of a maximum melanopic EDI of 10 lux is intended to appropriately minimise undesirable effects of evening light for the “average” healthy adult while allowing for sufficient light for common evening activities (see “Practical considerations” for further discussion). As it stands, where the evening light environment currently results in a melanopic EDI above 10 lux, reducing exposure in line with our recommendations is certainly still expected to be beneficial, regardless of individual differences in sensitivity, although future developments may make it possible to refine recommendations for specific individuals.
The magnitude of circadian and neuroendocrine responses to light also depends on age, with those in young children being larger and those in older adults tending to be smaller when compared to young adults [ 127 – 131 ]. These observations may, in part, reflect age-related differences in the amount of light reaching the retina (due to changes in pupil size and lens transmittance), although more direct changes in sensitivity or amplitude may also be involved. Certainly, one previous study that investigated the impact of age-related changes in lens transmittance did not find that this was associated with the expected reduction in light induced melatonin suppression in older adults [ 132 ]. Changes in light exposure in line with the current recommendations are still expected to be of general benefit to both young [ 112 – 115 , 124 , 126 ] and older individuals [ 67 , 68 , 118 – 120 ] (where their current daytime light exposure falls below, or evening/nighttime exposure above, the relevant targets). Select groups, however, may further benefit from higher daytime (e.g., older people) and/or lower evening exposures (e.g., children) than indicated in the recommendations. Similarly, disruptions to sleep and circadian rhythms are commonly associated with many disorders and disease states [ 8 , 133 ]. While adjusting light exposure may be of benefit in some or all of these conditions, further research will be required to determine whether alterations to the recommended thresholds will be required for such individuals.
In addition to the points above, a particular challenge in optimising light exposure to benefit health and performance relates to shift workers. Current light exposure advice for night shift workers is still not mature [ 134 ], and we want to stress that the present recommendations are not intended for this purpose. There is certainly evidence that increasing melanopic light levels in the work environment can improve subjective and/or objectively measured alertness and performance in shift workers [ 135 – 138 ]. Important benefits such as these do, however, need to be weighed in the context of potential disruptions to circadian alignment and chronic effects on health [ 8 – 14 ]. Addressing these important questions remains a key area for future investigation and shift work–related consensus guidance on best practice.
As discussed above, it is also essential that any changes to light exposure intended to adjust melanopsin-dependent physiological responses do not compromise visual requirements. For example, the elderly may need brighter lighting than recommended above to move safely between the bedroom and bathroom at night [ 12 ]. In many cases, such issues may be addressed by using lighting with an appropriate spectral composition (i.e., by using lighting with a low melanopic DER) and/or lighting designs that avoid direct illumination of the eyes. Nonetheless, there may be some instances where meeting the requirements for visual performance, visual comfort, and safety are incompatible with our recommendations regarding nonvisual responses, in which case the former must take precedence. Finally, while it is possible to comply with the recommended melanopic EDI thresholds specified here solely via exposure to electric light, there are a number of known and suspected benefits of exposure to broad-spectrum, outdoor, daylight [ 53 , 139 – 141 ].
Future directions
The recommendations outlined here are derived from a synthesis of several decades of research into the biology regulating circadian, sleep, physiological and cognitive responses to light and their practical implications. There is, without question, evidence that the use of melanopic irradiance as a model for the spectral sensitivity of such responses represents a simplification of the underlying biology. Although, as an aside, we note that this is true also for the established and widely used, photometric quantities (luminance and illuminance) that are currently applied to quantify conventional “brightness.” Nevertheless, we leave open the possibility that a deeper understanding of rod and/or cone contributions to physiological responses will reveal multiphotoreceptor models of spectral sensitivity that may allow a more accurate prediction of circadian, sleep, neuroendocrine, and/or cognitive responses. The contribution of rods to such responses is an interesting topic for research in its own right. Nonetheless, including a rod component in any such future metrics is unlikely to substantially improve the accuracy with which they recreate the spectral sensitivity of the relevant response(s), since the very similar spectral sensitivity profiles of rods and melanopsin render effective irradiance for these 2 opsins highly correlated [ 97 ]. Conversely, cone spectral sensitivity is quite distinct from melanopsin and has the potential to substantially refine metrics for circadian and neurophysiological responses. In particular, future work may reveal specific lighting conditions that maximise cone influence to produce practically relevant modulations in nonvisual responses to light (e.g., on the circadian system, neuroendocrine function, sleep physiology, and/or and alerting responses). At present, however, existing evidence indicates that the use of melanopic irradiance/EDI would not lead one to substantially over- or underestimate biological and behavioural effects for the types of light exposure that are typically encountered across daily life [ 35 – 38 , 40 – 43 ].
Further research into the factors influencing individual differences in the sensitivity of melanopsin-mediated responses to light exposure may make it possible to tailor guidelines to specific groups or even individuals. For the time being, our recommendations are derived from group data that must incorporate much of this variability. As such, it is expected that the recommendations for daytime and the sleep environment should be broadly applicable and strongly engage relevant circadian and neurophysiological responses for the vast majority of healthy adults. Known, age-related sources of variability are already at least partly accounted for by the inclusion of corrections for changes in lens transmission described in the nonnormative appendices of the existing standard [ 34 ]. Recommendations may, however, be modified in the future for certain groups such as children, older adults, or patient groups whose sensitivity to light may differ from the healthy adult population on which the present recommendations are based.
The current recommendations are intended to inform lighting design considerations for typical, real-world environments such as offices and other workplaces, schools, and colleges, residences, care homes, and in- and outpatient settings. As noted above, application of our recommendations across such settings is facilitated by the free availability of tools for calculating melanopic EDI (and also estimating this given known illuminance and type of lighting) [ 44 , 45 ]. Nonetheless, the emergence of low-cost commercial sensors for direct measurement of melanopic EDI (akin to conventional “lux meters”) is expected to further increase the ease with which the recommendations can be adopted.
A final point for consideration relates to applications of light therapy for clinical conditions like affective and circadian rhythm sleep disorders or for purposes such as improving circadian regulation and alertness in night and shift workers or transmeridian travellers experiencing jet lag. The current recommendations are not directly formulated for such uses, but the existing applications of ocular light therapy likely involve the same or similar biological underpinnings as discussed above. Given existing evidence for benefits of bright light therapy [ 5 – 7 ], perhaps widespread adoption of the recommendations described here will contribute to a reduction in the prevalence of affective and sleep disorders. More significantly, however, we expect the scientific framework that informs these recommendations to provide a concrete basis upon which to generate hypotheses to test for the subsequent establishment of optimal light treatment recommendations for clinical and travel applications.
- View Article
- PubMed/NCBI
- Google Scholar
- 34. CIE. S 026/E:2018. CIE System for Metrology of Optical Radiation for ipRGC-Influenced Responses to Light. Vienna: CIE Central Bureau; 2018. https://doi.org/10.25039/S026.2018
- 44. CIE. S 026 a-oic toolbox. Vienna: CIE Central Bureau, 2020. https://doi.org/10.25039/S026.2018
- 47. ANSI/IES. RP-27-20. Eecommended Practice: Photobiological safety for lighting systems: Illuminating Engineering Society; 2020.
- 49. DIN. SPEC 67600:2013–4 Biologically effective illumination—Design guidelines Deutsches Institut für Normung; 2013.
- 50. ANSI/IES. RP-28-16. Lighting And The Visual Environment For Seniors And The Low Vision Population: Illuminating Engineering Society; 2016.
- 51. CEN. EN 12464–1. Light and Lighting—Lighting of work places–Part1: Indoor work places: Comité Européen de Normalisation; 2021.
- 58. IES. PS-12-19: IES Position On UL RP 24480 Regarding Light and Circadian Entrainment. New York: Illuminating Engineering Society, 2020.
- 59. CIE. CIE Position Statement on Non-Visual Effects of Light: Reccommending Proper Light at the Proper Time, 2nd ed. Vienna: CIE Cenral Bureau, 2019.
- 142. CIE. 015:2018. Colorimetry 4th Edition. Vienna: CIE Central Bureau; 2018.
- Write to Us
- Corporate Training
- Water Cooler
Day Or Night: What Is The Optimal Time To Do Study?
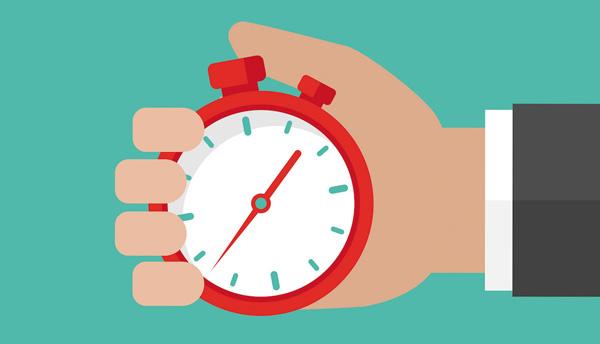
Is the daytime or nighttime better for studying? Here’s what the research says about studying the effectiveness at different times.
For students, settling down and focusing is key to a successful academic life. Yet with so many distractions around — smartphones, streaming, the internet — an engaging session of homework can be more elusive than expected.
Writing an essay, revising for a test, or committing essential facts to memory – these can take some discipline and planning. It’s stressful for those at school (and their parents) to realize suddenly the assignment hasn’t been touched and the weekend’s almost over.
Keeping to a regular schedule assists the kids in getting everything done and assimilating the information in a sustainable way. A good schedule and workstation facilitates effective learning and prevents the stress of last-minute rush. So when planning, is day or night better for studying?
Time and your brain
The time of day could have an impact on the type of brain activity or studying you should do. People’s brains might be sharper in the earlier hours, following a good night’s rest and after a healthful breakfast.
During the earlier hours, the human brain might be more receptive to grasping new things or revisiting notes. As you’re more alert then, you might be able to recall data and details more effectively.
Afternoons, on the other hand, could be better for integrating information, making connections, and getting the big-picture view. This can be a great period for enhancing the meaningfulness of their studies and deepen their understanding. In most cases , keeping to a predictable routine could be a great approach.
Night or day for studying?
While different times could be better for certain types of academic activity, whether nighttime or daytime is better will depend on the person. Everyone has their own natural rhythm and the research suggests you should align with it rather than against it.
During daylight larks, for example , will be efficient at analytical work that needs strong focus. More routine, repetitive work could be done later. However, night owls, in contrast, will find the reverse to be true. Some people might be a little bit of both a night owl and a morning lark.
Working out your learning style and identifying your energy levels can assist you in figuring out when is the best moment to do certain types of studies. Taking into account your favorite (and least favorite) subjects can also be important. For example, for someone who hates numbers, getting math homework out of the way earlier might be ideal.
Day studiers vs night studiers
The road to productivity lies in experimentation. Observe how you (or your child) does at different times. Consider when you assimilate information more effectively and when it’s easier to make connections between ideas. This can enable you to determine whether you’re a day studier or a nighttime studier — or perhaps somewhere in between.
Larks tend to have more energy in the early hours. They find it easier to concentrate and to absorb and retain facts in the morning. It lets you take advantage of natural light and helps you avoid disrupting your sleep routine. It’s also easier to connect with classmates for study groups.
Owls are those who have more energy and better focus later. They might find afternoons, evenings, or nighttime to be optimal periods for getting things done. You can take advantage of having fewer distractions and a quieter environment as people are winding down.
Furthermore, going to bed right after homework could help you consolidate facts more effectively, thereby enhancing your recall. Owls should be careful about getting enough sleep and avoid their schedule interrupting the quality of rest. It’s a good idea to start early if possible so you can finish and get to bed.
Finding the best time
So is it possible to find the optimal time for everyone? There isn’t one that applies to all. Each person will have their own preferences, learning style, and varying energy levels. Start by working out whether you’re an owl or lark and paying attention to your peak energy levels.
With a bit of experimentation, you and your child can find the best moment to undertake an effective review. This could change depending on the type of work the student is undertaking, along with their subject preferences.
Peak performance and productivity are never exact sciences, so parents and children will need to dedicate a little effort to find the prime time to study. Of course, identifying optimal periods is only one part of an effective study plan. To support those at school who are doing their best, empower students — especially older ones — in taking charge of their plans.
Reward them for achieving optimal outcomes. As parents, it’s also useful to guide your kids with a little context as to why certain topics might be important for their future. The opportunity to learn is a reward in itself, but children might appreciate an understanding of why subjects are useful.
Written By Luke Fitzpatrick
Luke Fitzpatrick has been published in Forbes, Yahoo! News and Influencive. He is also a guest lecturer at the University of Sydney, lecturing in Cross-Cultural Management and the Pre-MBA Program. You can connect with him on LinkedIn.
Recommended for you
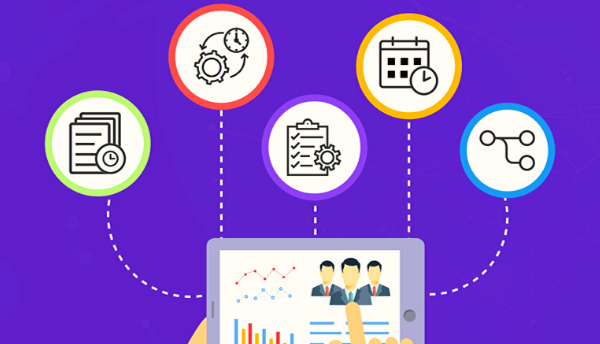
6 Efficient Ways to Improve the Commitment and Productivity of Contingent Workers
- Mike Johnston
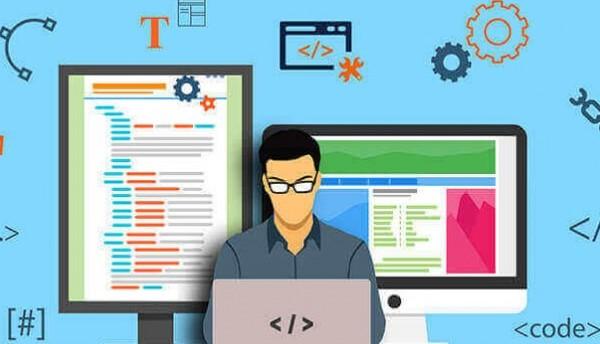
Why Should You Consider Using NodeJS For Web Development?
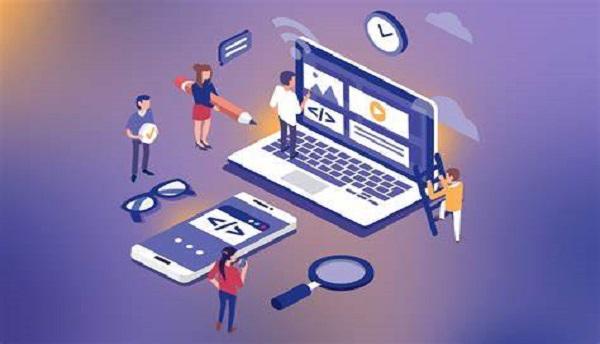
Best Web Development Certifications you need to know in 2022
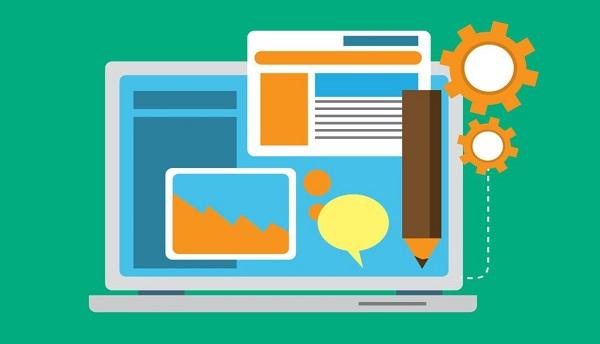
The Power Of Content Writing
- Sakshi Baid
Latest Blog
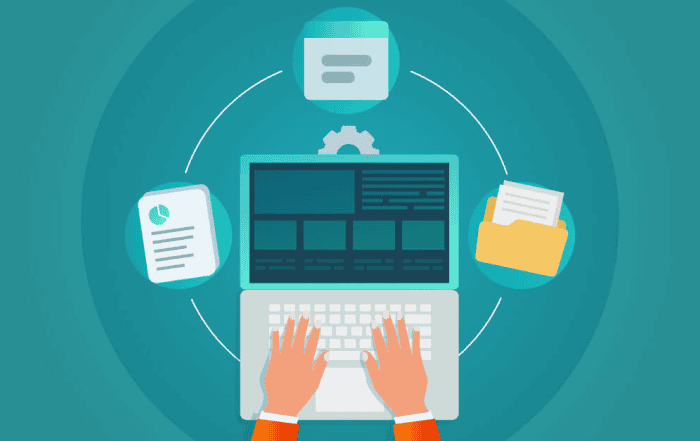
Streamline Document Management: Simplify Workflows with Microsoft Office 365
- Vijith Sivadasan
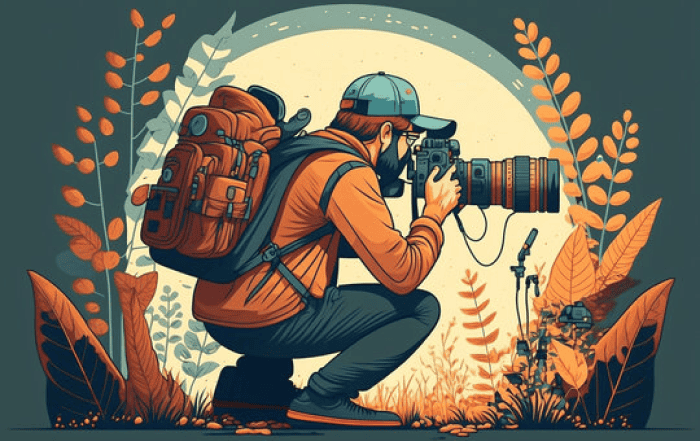
Boosting Productivity for Photographers with Microsoft Office 365
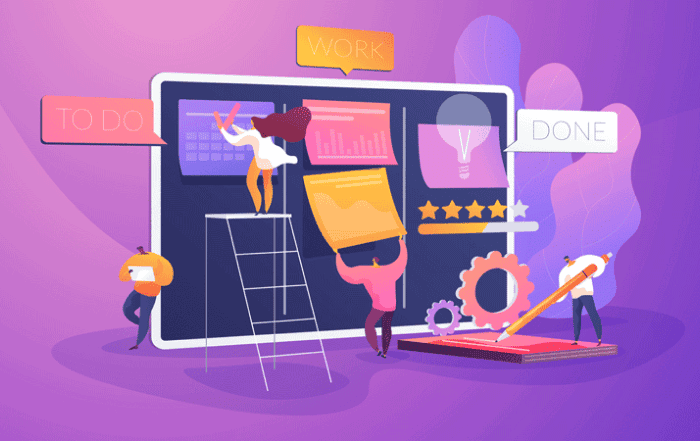
Efficient Project Management: Unleash the Power of Microsoft Office 365
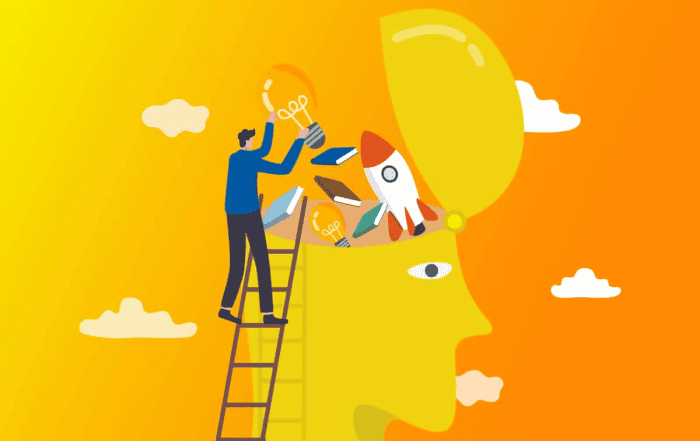
Unleash Organizational Wisdom: Harness Microsoft Office 365 for Knowledge Management
SAVE 35% on selected course fees. Ends 28th May 2024

Day vs night: when is the best time to study and why?
**This is an updated post**
You’re either an early bird or a night owl. But when it comes to learning, when is the best time to study? Is it better to study at night or in the morning? And what does science have to say about it?
Our bodies’ cycles of alertness and focus are governed by Circadian rhythms. They are what forms our 24-hour sleep/wake cycle that then drives when we are hungry, when we are tired and even when we undertake activities such as work and study.
But that’s just the beginning of the story. There are a whole bunch of lifestyle factors that influence when the best study time is for each individual person.
The truth is, there is no definitive “best” time to study, as every person is different. But there are benefits to studying during the day or night.
The benefits of studying during the day
It’s not hard to argue that studying during the day is a smart choice. After all, we’re usually refreshed in the morning after a good night’s sleep.
During the day you are less likely to require indoor lighting. Sunlight is actually good for your eyes. It provides just the right spectrum of light to maintain optimal eyesight and actually works with the pituitary gland to make you alert and awake. True fact!
Indoor lighting has actually been shown to interrupt the sleep cycle and most sleep doctors recommend that you expose yourself to as little artificial light at night as possible at night, including TV, computer and smartphone screens. This is why some devices now include a “blue light filter” which limits the amount of blue light displayed on the screen.
Positives of studying during the day:
- Natural light can help you focus, as opposed to artificial light.
- Your natural Circadian rhythms will be at work.
- You’ll be refreshed and alert, meaning you’ll be able to retain information better.
Tips for studying during the day:
- Find a space to study (inside or outside) that gives you plenty of natural light to see by.
- Eliminate possible distractions – does listening to music help you focus and block out distracting noises, like traffic sounds, or your loud neighbours?
- Breakfast is not the most important meal of the day, contrary to popular belief. But a healthy breakfast (think brain foods like avocados, blueberries and whole grains) will help improve your levels of concentration .
The benefits of studying at night
“But wait! I’m a night owl!” we hear you cry. Or perhaps you work a 9-to-5 job, or are looking after young children during the day and can only study after they’ve fallen asleep at night.
If night-time is when you feel like you are most productive, or when you have time to study, then don’t panic. Just as there are plenty of positives for studying during the day, there are various reasons why night-time is the best time to study for some.
The most obvious advantage of studying at night is the abundance of peace and quiet you have. Night-time is when people are more relaxed, quiet and contemplative. That environment can be exactly what some people need to get into the right headspace for study.
If you find that you can’t get a breakthrough on a project during the day, try taking a look after dark. You might find that you’re looking at things in a new way.
At night, the pressures of the daytime behind them, many feel that their mind is free to wander through more alternatives at night. And this can be useful for assessment-writing or working on creative pursuits.
Many authors, songwriters and scientists prefer to work at night because they feel that they tend to think more creatively after-hours. This is backed up by a study completed at the University of the Sacred Heart in Milan which showed that night owls were more likely to be creative than early birds.
Barack Obama himself is a self-confessed night owl.
Positives of studying during the evening:
- Peace and quiet – less distractions so you can knuckle down.
- Your daily tasks are done, so you can concentrate on your work.
- Night-time is when people tend to feel more creative.
Tips for studying at night:
- Limit possible distractions – turn your phone on silent and leave the TV off.
- Try to avoid caffeine, as it can take up to four hours to cycle through the body. It might give you a spike of energy, but it could prevent you from having a good night’s sleep.
- Create a routine that still gives you enough time for a good night’s rest.
What does the science say?
While Circadian rhythms are different for everyone, there are patterns that indicate that people really do fit into the “early bird” or the “night owl” categories.
The typical morning person will find that they are rearing to go as early as 7am. That burst of energy will last until around 10am when they need a little rest. Maybe this is when they take a coffee break and stretch their legs. Then they will get another burst of energy until around 3pm when they start to wind down.
The “night owl” tends to be the complete opposite. They will be sluggish until around midday when they have their first burst of energy. This will last until 3pm when they will have their second burst that will last until 7pm. From there, they will start winding down around 10pm and go quickly from activity to sleep.
So, when is it best to study?
It all depends on your lifestyle. Younger people with a routine that sees them more active at night will find that studying at night comes more naturally to them. If you’re an adult and re-entering study after a long time away, you might find that daytime is better suited to your established routine.
The important factor for everyone is making sure you are getting enough sleep. So whether you’re a morning person or a night owl, there’s nothing stopping you from taking on study in your own time and at your own pace right now.
Have you thought about taking up online study?
Whether you’re a morning or a night person, Open Colleges’ flexible online study means that you can study when you want, where you want. There are no deadlines and no classrooms, so you can fit study around your life. Learn more here.
Not quite ready to take the plunge and enrol? No problem. Why not dip your toe in the water with a 7-day free course trial? You can experience our world-class learning platform and get a feel for online study. With no obligations and no payment details required, it’s the perfect way to test the waters and maybe start a brand new, exciting career!
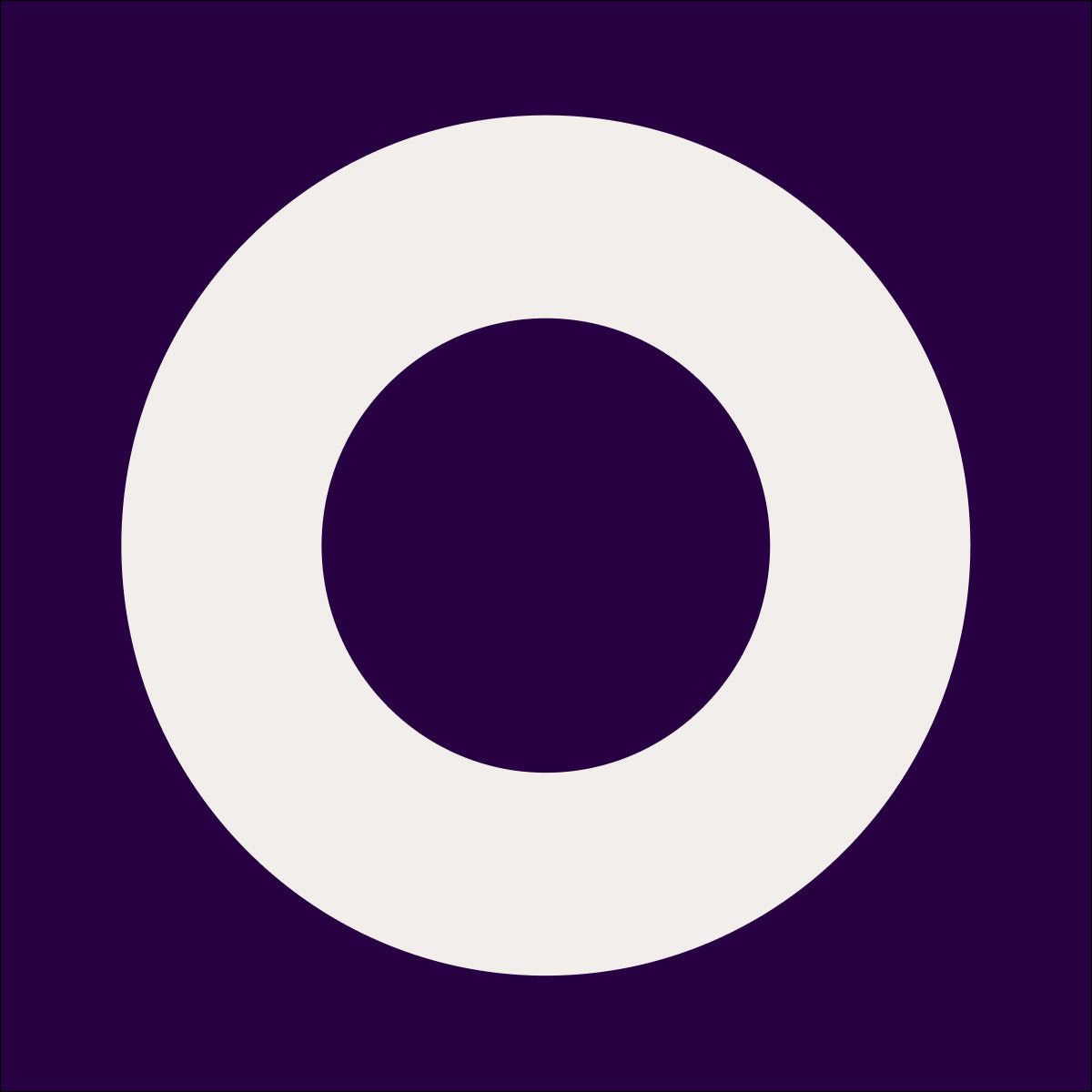
By Open Colleges
Related blogs.
Explore our collection of informative and educational blog posts to stay updated on the latest industry trends and expert advice.
Expert Tips to Succeed in Work Placement
How to become a youth worker in australia, understanding superseded courses: what it means for your education, terminology, top qualities of a successful healthcare aide, workers in need: a look at the aging care crisis, five quick tips for requesting a reference letter, the five best careers working with children.
- Choosing a selection results in a full page refresh.
- Opens in a new window.
SiOWfa16: Science in Our World: Certainty and Controversy
The course website and blog for the fall 2016 instance of penn state's sc200 course.

The Best Time of Studying: Daytime Vs. Nighttime
I do not know about other people, but I personality love studying at night when I am by myself. So what is actually the best time to study in a day? By the way, the time when I was writing this blog was 9 p.m. already.

It is really hard to say that one side is better than the other because there are people who love to studying during daytime, and there are people who like to study at night like me. I mean both sides have benefits, so there is actually no correct answer to this question in a scientific way.
The number one benefit for studying at night is an empty library! I can not express how much I appreciate the tranquility environments every time I walk into the library at night because it just puts me into that “ Studying mood ” where I tend to just focus on what I need to finish. By staying in a quiet library, it really helps me on my efficiency. For example, I would probably do twice more work in the library comparing with any other time.
Another benefit would be few distractions. It is really hard to be 100% concentrate during daytime without getting distracted by food, friends, the pool and video games like I always do. I especially can not say no to my friends when they ask me out for a lunch or playing the pool! So night time is definitely the best time for me to just sit down in my chair and do some real works without any distractions.
Studying at night also gives you a silence and quite environment which can makes you put more attention to whatever you need to do and get them done as soon as possible, so this is why I always give myself a three to four hours break just to do my homework or write an essay. Last but not least, studying at night also brings people some really creative ideas because when you are under a really silence environment, it is more easier to come up with generous ideas. So I prefer to study at night!
On the other hand, it also has so many good benefits for people who love to study during daytime. And the number one benefit I am going to talk about right here must be group studying or studying with your friends. It is so helpful and beneficial because you have the opportunity to get help and share your opinions with your friends right away.
Another good thing to study during daytime is that people tend to have more energy in the morning so that they feel motivational.
So there is no such thing as the best time to study during a day because everyone is different and has their own way of studying!
Website Source:
https://www.goconqr.com/en/examtime/blog/best-time-to-study/
5 thoughts on “ The Best Time of Studying: Daytime Vs. Nighttime ”
I prefer to study at night as well! I tend to nap for two hours everyday after class and let my work pile up so I’m up very late completing what I need to (hence why I’m posting this comment at 2 a.m.). Not only does my procrastination force me to do my work at night, but I would rather enjoy my day with my friends or sleeping and spend my nights cramming work. It’s an awful habit but I have come to the realization it is not going to break anytime soon.
This article caught my eye because I personally like to study better at night because I feel that I retain the information better the closer I review it before I go to bed. I feel if I review the information earlier in the day, everything I do after will drain out that information I learned.This article article I found changed my opinion a little.
I also like to study better at night. I think it is a lot easier to find a nice comfortable spot to study at night versus the daytime. I’m not sure why but I seem to remember stuff better when I study right before bed. I think it is because I think about the information that I just studied as I lay in bed. Studying at night fits into my schedule better too because it is hard for me to slow down and study in the middle of my busy day. At night I am more relaxed and in a better state for studying.
This article was very interesting and I have never thought about which is better: studying at nighttime or studying during the day. After hearing the fact that you go to the library at night when there are no people around, I thought about how much better that probably is. I always find myself getting distracted by people during the day, especially when I study at the library. I always wondered why I get so distracted but I think the fact that there are so many people and things to get distracted by during the day might be one of the main reasons. Here is another article on studying during the day versus studying at night. http://blog.opencolleges.edu.au/2015/08/14/st-day-vs-night-when-is-the-best-time-to-study-and-why/
I loved reading about your personal preferences; however, I completely disagree! For example, I could study just as efficiently in an empty library at night as studying in the arboretum during daytime. Another factor that could affect studying is your actual determination. You could reserve a study room at the library to go “do homework” with friends, and end up watching funny cat videos instead. In my opinion, it’s all about your willingness to actually get stuff done rather than what your environment is like. However, if your personal preference is finding a good study atmosphere, I think this blog post could really help you out! http://www.collegemagazine.com/15-best-places-study-penn-state/
Looking forward to reading more of your posts!
Leave a Reply Cancel reply
You must be logged in to post a comment.

Daytime eating may benefit mental health
A study on meal timing found that eating at night increased depression and anxiety-related mood levels among participants.
Beating the blues with food? A new study adds evidence that meal timing may affect mental health, including levels of depression- and anxiety-related mood. Investigators from Brigham and Women's Hospital, a founding member of the Mass General Brigham healthcare system, designed a study that simulated night work and then tested the effects of daytime and nighttime eating versus daytime eating only. The team found that, among participants in the daytime and nighttime eating group, depression-like mood levels increased by 26 percent and anxiety-like mood levels by 16 percent. Participants in the daytime-only eating group did not experience this increase, suggesting that meal timing may influence mood vulnerability. Results are published in the Proceedings of the National Academy of Sciences.
"Our findings provide evidence for the timing of food intake as a novel strategy to potentially minimize mood vulnerability in individuals experiencing circadian misalignment, such as people engaged in shift work, experiencing jet lag, or suffering from circadian rhythm disorders," said co-corresponding author Frank A. J. L. Scheer, PhD, Director of the Medical Chronobiology Program in the Brigham's Division of Sleep and Circadian Disorders. "Future studies in shift workers and clinical populations are required to firmly establish if changes in meal timing can prevent their increased mood vulnerability. Until then, our study brings a new 'player' to the table: the timing of food intake matters for our mood."
Shift workers account for up to 20 percent of the workforce in industrial societies and are directly responsible for many hospital services, factory work, and other essential services. Shift workers often experience a misalignment between their central circadian clock in the brain and daily behaviors, such as sleep/wake and fasting/eating cycles. Importantly, they also have a 25 to 40 percent higher risk of depression and anxiety.
"Shift workers -- as well as individuals experiencing circadian disruption, including jet lag -- may benefit from our meal timing intervention," said co-corresponding author Sarah L. Chellappa, MD, PhD, who completed work on this project while at the Brigham. Chellappa is now in the Department of Nuclear Medicine, University of Cologne, Germany. "Our findings open the door for a novel sleep/circadian behavioral strategy that might also benefit individuals experiencing mental health disorders. Our study adds to a growing body of evidence finding that strategies that optimize sleep and circadian rhythms may help promote mental health."
To conduct the study, Scheer, Chellappa, and colleagues enrolled 19 participants (12 men and 7 women) for a randomized controlled study. Participants underwent a Forced Desynchrony protocol in dim light for four 28-hour "days," such that by the fourth "day" their behavioral cycles were inverted by 12 hours, simulating night work and causing circadian misalignment. Participants were randomly assigned to one of two meal timing groups: the Daytime and Nighttime Meal Control Group, which had meals according to a 28-hour cycle (resulting in eating both during the night and day, which is typical among night workers), and the Daytime-Only Meal Intervention Group, which had meals on a 24-hour cycle (resulting in eating only during the day). The team assessed depression- and anxiety-like mood levels every hour.
The team found that meal timing significantly affected the participants' mood levels. During the simulated night shift (day 4), those in the Daytime and Nighttime Meal Control Group had increased depression-like mood levels and anxiety-like mood levels, compared to baseline (day 1). In contrast, there were no changes in mood in the Daytime Meal Intervention Group during the simulated night shift. Participants with a greater degree of circadian misalignment experienced more depression- and anxiety-like mood.
"Meal timing is emerging as an important aspect of nutrition that may influence physical health," said Chellappa. "But the causal role of the timing of food intake on mental health remains to be tested. Future studies are required to establish if changes in meal timing can help individuals experiencing depressive and anxiety/anxiety-related disorders."
- Sleep Disorder Research
- Workplace Health
- Insomnia Research
- Mental Health Research
- Mental Health
- Anger Management
- Narcolepsy (sleep disorder)
- Eating disorder
- Mental illness
- Seasonal affective disorder
- Bipolar disorder
- Bulimia nervosa
Story Source:
Materials provided by Brigham and Women's Hospital . Note: Content may be edited for style and length.
Journal Reference :
- Jingyi Qian, Nina Vujovic, Hoa Nguyen, Nishath Rahman, Su Wei Heng, Stephen Amira, Frank A. J. L. Scheer, Sarah L. Chellappa. Daytime eating prevents mood vulnerability in night work . Proceedings of the National Academy of Sciences , 2022; 119 (38) DOI: 10.1073/pnas.2206348119
Cite This Page :
Explore More
- Treating Cataracts and Other Eye Conditions
- Early Arrival of Palaeolithic People On Cyprus
- Networks Regulating Gene Function in Human Brain
- Birth of Universe's Earliest Galaxies
- Why the Brain Can Robustly Recognize B&W Images
- Birth Control Pill for Men?
- Intriguing World Sized Between Earth, Venus
- Billions of Orphan Stars Revealed
- Massive Catalog of Strange Worlds
- Mental Disorders May Spread Thru Social Networks
Trending Topics
Strange & offbeat.
What Is The Best Time To Study: Day Or Night?
When it comes to studying, finding the optimal time can greatly impact your productivity and learning outcomes. Determining the best time for studying is a common dilemma among students. Many wonder whether studying during the early hours of the day or late at night yields better results.
However, it is essential to understand that there is no universally applicable answer as each individual’s schedule, habits and preferences vary. The question of whether to study during the day or night is subjective and depends on individual preferences, habits, and circadian rhythms.
In this article, we will explore the merits and demerits of studying during both periods, helping you make an informed decision based on your unique circumstances.
Candidates for numerous Government Exams can learn more about the specifics of upcoming exams, on the linked page.
How To Know Which Is The Best Time To Study?
Determining the best time to study can be a personal decision based on individual preferences and circumstances. Some individuals are early birds who wake up early, complete their tasks, and sleep early, while others find it difficult to wake up and become more active as the day progresses. Finding the best time to study is a process of self-discovery. It may require some trial and error to identify the most productive and effective study periods for oneself. By being mindful of one’s energy levels, schedule and personal preferences, one can establish a study routine that optimises their learning potential.
Factors to Consider When Determining the Ideal Study Time:
Selecting the right study time is crucial for academic success, as it aligns with your biological clock and enhances your alertness and focus. Several important factors should be taken into account when determining the most effective time for studying:
- Consistency : Strive for consistency in your study routine, ideally setting aside dedicated study time several times a week. Regularity not only helps you establish a habit of studying but also enhances the quality of your work. By studying at the same time every day, your mind and body will adapt, leading to an optimal mindset for studying and increased concentration.
- Level of Alertness : Choose a time when your mind is at its most active and alert. This period of heightened brain activity enables better focus, concentration and improved retention of information.
- Minimising Distractions: Creating a study environment free from distractions is crucial. Select a time when interruptions are minimal, allowing you to dedicate extended periods of uninterrupted study. Some individuals prefer complete silence, while others find soft background music conducive to their concentration.
- Assess the Energy Levels: Observe your natural energy patterns throughout the day. Take note of when you feel most alert, focused, and energised. This can vary from person to person, but common periods of increased energy tend to be early morning, late morning or early evening. Identifying your peak energy times will help you determine when you are most likely to be productive and absorb information effectively.
- Listen to Your Body : Pay attention to how your body responds to studying at different times. If you feel excessively tired or struggle to concentrate during a particular time slot, it may not be the optimal time for you. Respect your body’s needs for rest and choose study periods that align with your natural rhythm.
- Adapt and Refine: As you gather data from your experiments and self-reflection, make adjustments to your study schedule. Fine-tune your routine based on the times that consistently yield the best results for your focus, productivity, and comprehension. Be flexible and open to adapting your study schedule as circumstances change.
- Recognising Focus and Motivation : Take note of the times when you feel most motivated and focused. These moments may differ for each individual. By studying during these periods, you can enhance your efficiency and retention of knowledge.
- Identifying Available Free Time : Recognise the window of free time within your schedule. Whether you are a student preparing for exams or a working individual seeking to enhance your skills, understanding when you have unallocated time allows you to plan and dedicate focused study sessions.
By considering these factors and experimenting with different study times, one can identify the optimal period that aligns with their personal preferences and maximises productivity and learning outcomes.
For important topics on English For Competitive exams , such as Precis and Letter writing format, rules and list of prepositions, articles, conjunctions, etc, candidates can check the link provided.

Benefits Of Studying In Day Vs Night
When it comes to studying, individuals have their unique inclinations and routines. While some prefer rising early in the morning to engage in study sessions, others opt to stay awake late at night to prepare for exams. However, it’s worth considering the advantages of studying during these distinct time periods. Here are the benefits associated with studying in the early morning and late at night.
Advantages of Studying During the Day:
- Availability of Resources: Studying during the day aligns with the typical school and work schedules, making it easier to access resources such as libraries, academic support, and interaction with peers or instructors. It allows for better coordination of group study sessions and collaborative learning opportunities.
- Increased Alertness: Many individuals tend to be more alert and mentally sharp during the daytime. This can lead to better concentration, improved information retention, and enhanced cognitive performance.
- Natural Lighting : Daytime studying allows you to benefit from natural light, which is not only easier on the eyes but also promotes a more positive mood. Natural light has been shown to enhance focus and productivity.
- Reduced Distractions: Daytime study sessions often provide a quieter environment with fewer distractions compared to studying at night. This can contribute to better focus and concentration on the study material.
Advantages of Studying at Night:
- Flexibility : Studying at night can provide flexibility for individuals with daytime commitments such as work or family responsibilities. It allows them to dedicate focused study time once their other obligations have been fulfilled.
- Personal Preference : Nighttime studying may align better with the preferences and habits of individuals who identify as night owls. They may naturally experience heightened alertness and find it easier to concentrate during the late hours.
- Personal Productivity : Some individuals find that they are more productive and focused during the late hours. With reduced external demands and distractions, they can allocate extended periods of uninterrupted time for studying, leading to increased productivity.
- Quiet and Fewer Distractions: Nighttime can offer a quieter environment with fewer external disturbances and interruptions. This can create an atmosphere conducive to deep focus and concentration, allowing for undisturbed study sessions.
For aspirants preparing for the government exams, English subject is an important one. Go through important questions and answers on English topics for practice and preparation.
- One-Word Substitution Questions & Answers
- Tenses Questions And Answers
- Direct & Indirect Speech Questions & Answers
More question-and-answer articles on important topics of the Verbal Ability section can be found on the linked page.
Key Considerations While Choosing the Optimal Study Time
When it comes to determining the best time to study, there are several factors to keep in mind to ensure one makes the most of his study hours. By considering these tips, one can create a study schedule that is tailored to his needs, boosts productivity and helps one achieve his academic goals.
Regardless of whether aspirants are an early riser or a night owl, these guidelines will assist them in optimising their study routine and maximising their learning experience.
Tips for Effective Early Morning Study Sessions:
- Prioritise Natural Lighting : Make sure your study area is well-lit with natural light, as it can improve your focus and overall mood.
- Minimise Distractions: Create a quiet and distraction-free environment to enhance your concentration. Keep away from noise, traffic and other potential disturbances.
- Fuel Your Body: Start your day with a nutritious breakfast to provide your brain with the necessary energy. However, avoid heavy meals that can lead to drowsiness.
Those finding it difficult to start with the English section of the Government exams, can refer to the links of relevant English language topics that are asked frequently in the examination:
- List of One Word Substitutions
- Adjective Degree Of Comparison Rules
- List of Homophones/Homonyms
- List of Synonyms and Antonyms
- Active And Passive Voice Rules
Tips for Productive Late-Night Study Sessions:
- Minimise Distractions : Disconnect from social media and other online platforms that may divert your attention. Create a study environment that is free from interruptions.
- Be Mindful of Caffeine Intake : While coffee or energy drinks may offer temporary bursts of energy, excessive caffeine consumption can disrupt your sleep. It’s important to strike a balance and avoid consuming stimulants close to bedtime.
- Establish a Balanced Schedule : Plan your study sessions in a way that allows for adequate restorative sleep. A well-rested mind is more receptive to learning.
Regardless of the time you choose to study, it is essential to maintain a healthy lifestyle, including a balanced diet and consistent sleep patterns. Tailor your study schedule to your personal habits and preferences, ensuring that you allocate focused time for learning while taking care of your overall well-being.
For the best preparation strategy for competitive exams , candidates can visit the linked article and get detailed study material and preparation tips to excel in the examination.
If candidates taking the exam for the first time require study materials and preparation advice for competitive exams, they can seek assistance from BYJU’S.

Connect with us for Free Preparation
Get access to free crash courses & video lectures for all government exams., register with byju's & download free pdfs, register with byju's & watch live videos.
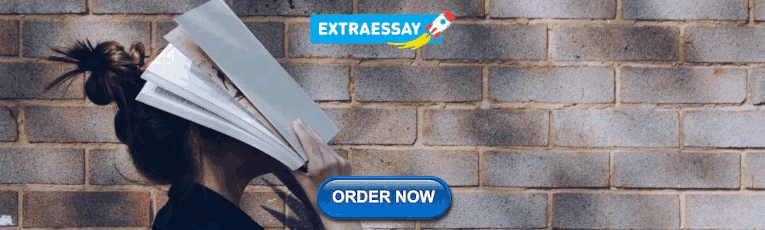
IMAGES
VIDEO
COMMENTS
So, those are the daytime tasks and rewards in the Pokemon GO Starry Skies Help Research Daytime or Nighttime choice. Now for the nighttime tasks. As we've said, the rewards are exactly the same; you're not going to miss put on anything regardless of what you choose. Step 2; Catch 20 Ghost- or Dark-type Pokemon - 10x Poke Balls
Before you stress about the choice between Help Research Daytime Pokémon and Help Research Nighttime Pokémon after clearing the first page of the Starry Skies Special Research, just know it all ...
☼ Help Research Daytime Pokémon tasks Step 2 of 4. Catch 20 Grass- or Bug-type Pokémon (10 Poké Balls) Explore 10 km (Inkay) ... ☾ Help Research Nighttime Pokémon tasks Step 2 of 4.
The best path in the Pokemon GO Starry Skies Research depends on when you play the game. If you play more during the day, choose Daytime; if you play more during the night, choose Nighttime. This is because the rewards for both paths are exactly the same, including the much-requested second Cosmog encounter! Which path you choose only changes ...
The 'Starry Skies' special research is a new special research that enables you to encounter a second Cosmog, released during the Solstice Horizons Event. It is available from Friday, June 16, 2023, at 10:00 a.m. until Sunday, June 25, 2023, at 8:00 p.m. local time.
Pokemon Go trainers will then be able to choose between Daytime and Nighttime branches. ... Help Research Nighttime Pokemon: Step 2 of 4. Catch 20 Ghost or Dark-type Pokemon - 10 Poke Balls ...
Ledyba - In the wild during the day and seasonal field research task (Use 5 Razz Berries to help catch Pokémon) Petilil - In the wild during the day Purrloin - In the wild during the day
Here are the challenges and rewards for the Nighttime Path of the Starry Skies special research quest in Pokémon Go: 'Starry Skies' Step 2 of 4. Rewards: 78 Stardust, 15 Poké Balls and a ...
The Daytime Path features challenges related to distance walked, buddy Pokémon, curveball throws, and catching specific Grass and Bug-type Pokémon. On the flip side, Nighttime focuses on raids, field research tasks, excellent throws, taking snapshots, and catching Dark and Ghost-type Pokémon.. Aside from the Glacial Lure and Mossy Lure Modules rewarded for step three on their respective ...
The daytime option focuses more on exploration while the nighttime path has catching and raid challenges. Here are all the differences. Starry Skies: Research Daytime Pokémon page two
Night Time Path Task List: x2 Tatch 20 Ghost or Dark-type Pokemon. x2 Take snapshots of 20 different wild Pokemon. x2 Use 30 berries to help catch Pokemon. x3 make 5 excellent throws. x3 Catch 30 different species of Pokemon. x3 Complete 15 Field Research Tasks. x4 Win 5 Raids. That's everything to know about the Pokemon Go Day or Night ...
Help Research Nighttime Pokémon: Help Research Daytime Pokémon 2 / 4: Catch 20 Grass or Bug type Pokémon: Poke Ball * 10: Slowpoke Encounter: Poke Ball * 15: Stardust * 78: Explore 10km: Inkay Encounter: Spin 25 PokéStops or Gyms: Pinap Berry * 10: Help Research Daytime Pokémon 3 / 4: Make 10 Curveball Throws in a row:
Daytime light recommendations for indoor environments. Throughout the daytime, the recommended minimum melanopic EDI is 250 lux at the eye measured in the vertical plane at approximately 1.2 m height (i.e., vertical illuminance at eye level when seated). If available, daylight should be used in the first instance to meet these levels.
Daytime light recommendations for indoor environments. Throughout the daytime, the recommended minimum melanopic EDI is 250 lux at the eye measured in the vertical plane at approximately 1.2 m height (i.e., vertical illuminance at eye level when seated). If available, daylight should be used in the first instance to meet these levels.
Time and your brain. The time of day could have an impact on the type of brain activity or studying you should do. People's brains might be sharper in the earlier hours, following a good night's rest and after a healthful breakfast. During the earlier hours, the human brain might be more receptive to grasping new things or revisiting notes.
This review discusses research that highlights key differences in the nighttime road environment compared to the day and how this affects visual function and driving performance, together with an overview of studies investigating how driver age and visual status affect nighttime driving performance. Research that has focused on the visibility ...
As far as I know, genesect was only ever in one research and it was a paid research like 3 years ago. It's been in raids somewhat recently but that's not the same as free research. 10. 543 votes, 230 comments. 859K subscribers in the TheSilphRoad community. Reddit's #1 spot for Pokémon GO™ discoveries and research. The Silph Road is….
The most obvious advantage of studying at night is the abundance of peace and quiet you have. Night-time is when people are more relaxed, quiet and contemplative. That environment can be exactly what some people need to get into the right headspace for study. If you find that you can't get a breakthrough on a project during the day, try ...
So what is actually the best time to study in a day? By the way, the time when I was writing this blog was 9 p.m. already. It is really hard to say that one side is better than the other because there are people who love to studying during daytime, and there are people who like to study at night like me.
The rules for using daytime and nighttime may also vary depending on the geographic location. For example, in some parts of the world, the sun may not set for months at a time during the summer, while in other parts, it may not rise for months during the winter. In these cases, the terms daytime and nighttime may not be as relevant or accurate. 4.
Daytime eating prevents mood vulnerability in night work. Proceedings of the National Academy of Sciences , 2022; 119 (38) DOI: 10.1073/pnas.2206348119 Cite This Page :
Pokemon GO Starry Skies, Help Research Daytime or Nighttime Pokemon Choice. Archived post. New comments cannot be posted and votes cannot be cast. 417 subscribers in the GameGuidesGN community. TLDR; Game guides and news.
Here are the benefits associated with studying in the early morning and late at night. Advantages of Studying During the Day: Availability of Resources: Studying during the day aligns with the typical school and work schedules, making it easier to access resources such as libraries, academic support, and interaction with peers or instructors ...
Altering the day/night sleep rhythm throws off the body's ability to manage blood sugar and inflammation, researchers found. Long-term, this could lead to the development of chronic diseases. TUESDAY, May 14, 2024 (HealthDay News) -- Night shift work can increase a person's risk of chronic disease, and a new study reveals one possible ...