Jan | Feb | Mar | Apr | May | Jun | Jul | Aug | Sep | Oct | Nov | Dec |  DE-FO2-0003126: Wholesale Electricity Market Studies and EngagementsThe U.S. Department of Energy (DOE) is issuing, on behalf of the Grid Deployment Office (GDO), this Funding Opportunity Announcement (FOA). Awards made under this FOA will be funded, in whole or in part, with funds authorized under the Consolidated Appropriations Act, 2023 (Public Law 117-328). Creating more efficient and flexible wholesale markets that will support a more resilient and reliable grid will be critical as new load and generation come online. Functioning wholesale markets provide a platform for energy trading and the integration of electric resources into the grid. Efficient, fair, and transparent market constructs are thus foundational to transitioning to a clean, reliable, equitable electric grid. This FOA will assist applicantsâStates, ISOs/RTOs, and domestic entities that have partnered with States and/or ISOs/RTOs â that have formed partnerships with or otherwise include States, ISOs/RTOs to perform analytical studies on critical market issues or convene stakeholders to address issues facing developing or existing wholesale markets. GDO suggests five (5) broad priorities for proposed projects: 1) seams between markets, 2) regional resource adequacy, 3) market design and price formation, 4) regional footprint studies, and 5) integrated regional planning approaches. Please see the full FOA document in the Documents section below. Questions regarding the FOA must be submitted to [email protected]. The required Concept Paper due date for this FOA is 06/13/2024 at 5PM ET. The Full Application due date for this FOA is 08/22/2024 at 5PM ET. In the event that an Applicant experiences technical difficulties with a submission, the Applicant should contact the eXCHANGE helpdesk for assistance ([email protected]). Application Forms and TemplatesThe following forms and templates may be used as part of the application submission. Note that these forms and templates do not necessarily constitute all the documents required for a complete application. Please refer to the 'Application and Submission Information' of the published announcement to learn more about the required application content requirements. Full ApplicationThank you for visiting nature.com. You are using a browser version with limited support for CSS. To obtain the best experience, we recommend you use a more up to date browser (or turn off compatibility mode in Internet Explorer). In the meantime, to ensure continued support, we are displaying the site without styles and JavaScript. - View all journals
- Explore content
- About the journal
- Publish with us
- Sign up for alerts
- Perspective
- Open access
- Published: 28 May 2024
Alternative protein sources: science powered startups to fuel food innovationNature Communications volume 15 , Article number: 4425 ( 2024 ) Cite this article 2434 Accesses 24 Altmetric Metrics details - Agriculture
- Tissue engineering
Harnessing the potential of considerable food security efforts requires the ability to translate them into commercial applications. This is particularly true for alternative protein sources and startups being on the forefront of innovation represent the latest advancements in this field. Similar content being viewed by others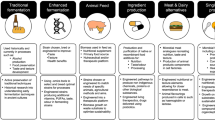 The microbial food revolution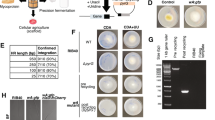 Edible mycelium bioengineered for enhanced nutritional value and sensory appeal using a modular synthetic biology toolkit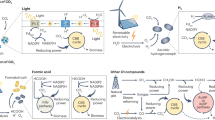 From sustainable feedstocks to microbial foodsIntroduction. In 2022 almost 735 million people, 9.2% of the global population, were undernourished 1 and as we are entering the second quarter of this century, the world faces a formidable task of feeding a growing population projected to reach 10 billion by 2050 2 . It is further aggravated by substantial food losses due to climate change and crop diseases 3 . To address this task requires a 50% increase in global food production in the next 25 years 2 and the significance of food innovation becoming increasingly evident in order to create solutions tackling the urgency and scale of this food security challenge. The World Resources Report ‘Creating a Sustainable Food Future’ 4 identified 22 solutions to address this need, which were grouped into five categories: (1) reduction of growth in demand for food and other agricultural products; (2) increase in food production without expanding agricultural land; (3) protection and restoration of natural ecosystems; (4) increase in fish supply; and (5) reduction of greenhouse gasses emissions from agricultural production. To implement these solutions, supporting the sustainable development goals 4 and also addressing the food security challenge, require new technologies and product innovations, i.e., it is essential to find innovative ways to produce and distribute food more efficiently and sustainably. Achieving this goal can involve developing novel farming techniques (precision agriculture), exploring alternative protein sources, or implementing smart technologies to reduce waste and optimise food supply chains. Considerable efforts have been put in place to address this challenge ranging from precision agriculture to food innovation (Fig. 1 ). This involves truly cross disciplinary innovation efforts for example, genetic modifications to increase crops’ resilience 5 , different antibacterial strategies to improve food security 6 , sensors 7 and big data advances for precision farming 8 , artificial intelligence (AI) and block chain technologies to manage food supply 9 , advancement in material science to develop functional packaging materials 10 , tissue engineering in the development of alternative protein sources 11 etc. 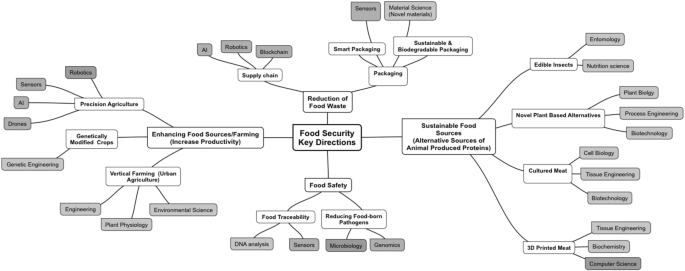 Multidisciplinary approaches are required to effectively address the Food Security Challenge which result in innovation technologies and approaches, e.g., advancement in genetic engineering to develop high-yield and disease resistant crops; using drones and big data analytics for precision farming; expanding use of 3D bioprinting to produce non-animal based meat alternatives. Note: the diagram aims to provide an overview of innovation examples across different scientific areas rather to list all scientific fields involved in the Food Security area . However, harnessing the potential of such technologies for food security requires the ability to translate them into commercial and scalable applications. In many cases, the first step of this translation is done by startups and/or university spins outs. This is particularly true for the development of alternative protein sources, which has been selected as a topic for this review. Alternative protein sources are used to substitute animal source protein-rich foods and form an integral part of sustainable food systems to meet protein demands that are projected to nearly double by 2050 12 . Two opposing protein transition trends are taking place: low-income populations shift from plant to animal protein sources while high income populations are looking to substitute animal protein sources 13 . Multiple factors are driving this transition including economic growth in lower income countries and having more animal products in their diet 13 , while the raising environmental and health concerns have led to dietary changes in higher income countries 13 . However, it is important to note that these are not conflicting trends, i.e., it is possible to reduce the consumption of meat in high income countries and at the same time increase animal-based protein consumption among the socio-demographic groups for which more protein in their diet would be beneficial 14 . The current review aims to bring together the latest advancements in the alternative protein sources research and insights into their translation into product applications, i.e., their impact outcomes to address the food security challenge. Startups eco-systemData source. Startups, which are positioned at the forefront of technological innovation and aim to turn groundbreaking research into product applications and creating new solutions, present a good indicator of the latest technology and research advancements and as such were used as a data source for this review. Due to their agile and lean structure, they can rapidly pivot and iterate to drive technological progress that in many cases are further strengthened by their collaborations and partnerships with academia, established companies and other organisations. Eco-system designMost of reviews dedicated to the development of alternative food sources are structured around the production method 15 , 16 , 17 , 18 , e.g. cell-cultured products, precision fermentation, insect proteins etc and/or a specific source of alternative proteins 19 , 20 , 21 , 22 . The current eco-system was designed based on an innovation strategy to address a problem – finding an alternative to animal/fish-based proteins. When it comes to finding an alternative, there are three main options to consider: (1) using a replacement, (2) modifying existing non-animal/non-fish sources of proteins, and (3) making an alternative source of proteins (Fig. 2 ). Replace: this option involves using a readily available substitute for the target compound, e.g., current vegetarian diet options. Modify: this option looks in modifying existing non-animal/non-fish sources of proteins to substitute the target compound, e.g., insect-based protein. Make: This option comes from a product innovation standpoint, providing the most potential, while holding the biggest challenges. It includes using novel technological processes to make proteins, e.g. 3D bioprinting, cell-cultured products, precision fermentation etc. 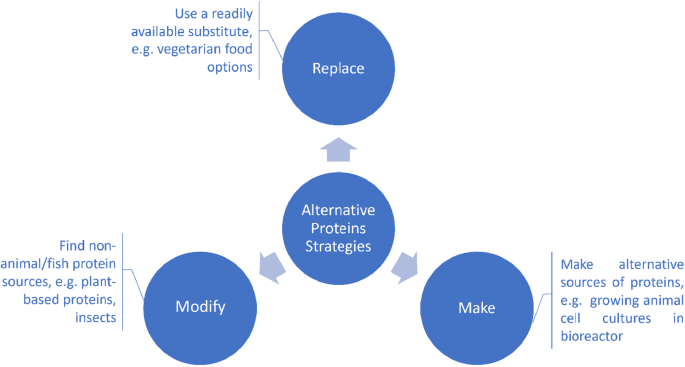 A problem-solving innovation strategy approach was used to design the startups eco-system. The problem to solve is to find an alternative to animal/fish-based proteins and when it comes to finding an alternative, there are three main options to consider: (1) using a replacement, (2) modifying existing non-animal/non-fish sources of proteins, and (3) making an alternative source of proteins. (1) Replace: this option involves using a readily available substitute for the target compound, e.g., current vegetarian diet options. (2) Modify: this option looks at modifying existing non-animal/non-fish sources of proteins to substitute the target compound, e.g., insect-based protein. (3) Make: This option comes from a product innovation standpoint, providing the most potential, while holding the biggest challenges. It includes using novel technological processes to make proteins, e.g. 3D bioprinting, cell-cultured products, precision fermentation etc. In terms of the market penetration, scalability and cost were main differentiating parameters between these three options (Fig. 3 ). 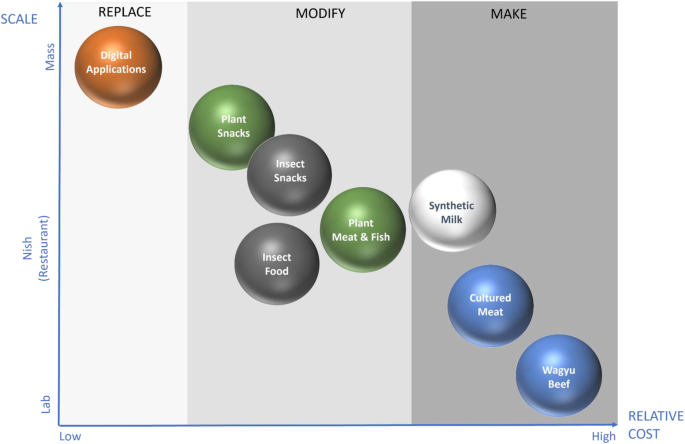 Assuming the same consumer perception of different alternative proteins products, their market penetration would primarily depend on their scalability (ability to move from niche to mass market without compromising quality) and cost (at least parity to the animal/fish-based protein products). These two parameters were used to map market the penetration potential of different alternative protein options using current market examples. Note: products examples are intended to illustrate a relative position and presented in a non-scale format. Eco-system compositionFor illustrative purposes, 33 startups have been selected to provide a representative sample of the alternative protein startups eco-system (Table 1 ). The selection process was based on (i) sources of alternative proteins: insects, plant and cells; (ii) technology approach used e.g., extrusion, precision fermentation, 3D bioprinting, cell culture and (iii) the a type of alternative product, e.g. alternative meat, fish, chicken, protein, milk etc. The startups selection was rendered based on them (i) having an alternative protein product offering; (ii) representing some of the main trends and (iii) being active, i.e. website news posting in 2023. (Fig. 4 ). 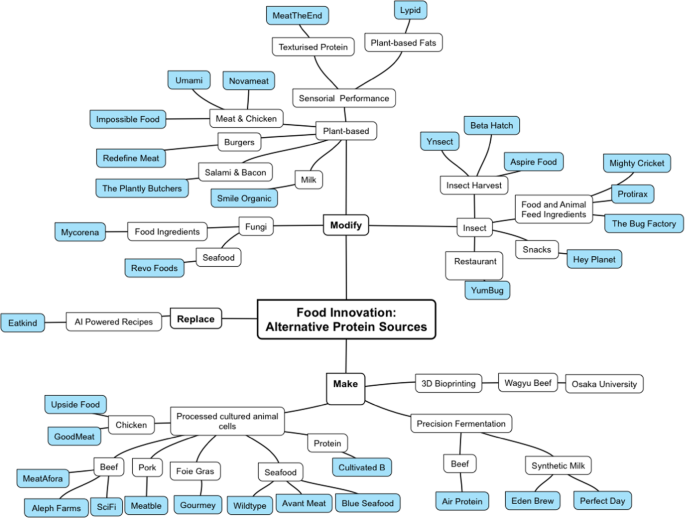 Startups eco-system is designed based on innovation strategy approach used to produce alternative proteins that forms three main pillars of the eco-system: (1) Replace, (2) Modify and (3) Make. Each pillar has 2–3 levels with one-to-two levels specific to each pillar. The specific levels were designed to represent specific features of each pillar, e.g. the first level in the make pillar is the type of manufacturing method/technology approach used to make alternative proteins while in the replacement pillar, it is based on different sources of the alternative proteins (insects, plants, fungi). The second level deals with product’s types, e.g. chicken, beef, salmon, snacks. The last level provides examples of startups selected based on them (i) having an alternative protein product offering; (ii) representing some of the main trends and (iii) being active, i.e. website news posting in 2023 and presented for illustrative purposes only. These companies have been reviewed across three main vectors: (1) scientific insight behind the technology; (2) product development stage and (3) key challenges. Alternative protein sourcesReplace (meat protein replacement). This option deals with using existing non-animal-derived ingredients to substitute proteins from animals and fish. While from a product innovation standpoint, it may not be considered significantly innovative, the process of considering replacement options utilises the latest developments in computer – digital databases and machine learning. A significant amount of data including food composition, nutritional composition and recipes is publicly available 23 , 24 , 25 . It provides a fruitful ground to utilise machine and deep learning approaches for food design 26 . Taking this approach, Eatkind Technologies Private Limited (India) has developed an AI-based tool (EatKind 27 ) to replace meat, egg and dairy ingredients in a recipe for a plant-based one. The EatKind site turns any recipe into a plant-based one by posting it in the site’s search box. Modify (non-meat source of proteins)The next option is using existing sources of proteins, such as insects and plants, as non-animal or/and fish protein s sources. Insect basedEntomophagy, the consumption of insects as food, has been a common practice in many cultures for centuries 28 . Insects have great potential as a sustainable animal protein source due to their low impact on resources, e.g. emitting less greenhouse gases, requiring less water and space 29 . The data from various comparative analyses 30 , 31 , 32 of the protein and other nutrients content in edible insects and animal-derived meat have demonstrated that both edible insects and animal-derived meat have varied nutritional content with more profound variations in edible insects 30 . The latter was considered to be due to the diversity of individual species 32 . Edible insects have a higher protein content than animal meat 31 , ranging from 23.1 g to 35.2 g per 100 g among edible insect species and 19.2 g to 21.5 per 100 g in different types of meat 31 . In regard to the nutrition value, it seems that it is not possible to explicitly state that edible insects would have a higher nutritional value than animal-derived meat, because of the differences in the content of individual nutrients in edible insects and animal-derived meat 30 , 31 . In addition to this, this analysis would be impacted by using different nutrient profiling models 30 . An estimated 2 billion people 33 across Africa, Asia, Central and South America, and Australia consume insects and there is an increased interest in Western countries in insects as a potential source of food. The Edible Insects Market size is estimated at USD 3.20 billion in 2023, and is expected to reach USD 7.60 billion by 2028, growing at a compound annual growth rate of 18.89% in 2023-2028 34 . Two main factors contribute to this trend: (1) the growing acceptance of insect-based food in Western societies 35 and (2) their lower environmental impact to address the food security challenge. This rapid growth is supported by increasing investments. While the edible insects’ market is highly fragmented 34 , it has attracted more than USD 1.3 billion in funding to date with more than half of it in the past couple of years 36 . Increasing investment in the startups’ research and development (R&D) also comes from partnerships with existing companies. For example, Protirax announced a strategic partnership with Tyson Foods, one of the world’s largest food companies 37 , Ÿnsect launched a dog feed brand in the US in collaboration with Pure Ultra Simple LLS, a dog food start-up (US) 38 . To help scale up the edible insect-based business globally, many government organisations are developing programmes and initiatives including a collaboration between the Australian Centre for International Agricultural Research (ACIAR), AgriFutures Australia and the International Centre for Insect Physiology and Ecology (ICIPE) resulting in the creation of the Emerging Insect Technology Hub (EIT-Hub) that aims to bring together industry stakeholders, scientists and investors to discuss issues linked to emerging insect technologies around insects as food, animal feed and fertiliser 39 . The other example is the ‘Insectrial Revolution’ project which received USD 7.5 million from the UK government’s Industrial Strategy Challenge Fund (ISCF). This project focusses on the construction of the country’s first large-scale industrial insect farm run on food waste. It is being led by led by the insect-farming company Entocycle (UK) and brings together a consortium of 15 partners providing their diverse expertise and ranging from academic partners and multinational companies, e.g. insects technology expertise (BetaBugs, Better Origin, Fera); science (Durham University, University of Stirling, University of Warwick, Scottish Aquaculture Innovation Centre); waste management (not-for-profit environmental organisation Zero Waste Scotland) and a multinational company (Tesco) 40 . Following the development of relevant regulatory frameworks and legislations covering edible insects, the companies (examples are given in Table 1 ) were able to place their product in the market, making them available to consumers. For example, in the United States, edible insects and insect-based food products must comply with the Federal Food, Drug, and Cosmetic Act (FD&C Act) 41 , in the European Union all insect-based products (whole insects, their parts or extracts) meant for human consumption have fallen under the novel food regulation EU 2015/2283 41 and in the United Kingdom the Food Standards Agency (FSA) is now requiring insect companies to submit dossiers of evidence of safety 42 . Insect-based food startups’ activities range from harvesting the insects to producing food products (examples are given in Table 1 ). These companies’ development is a result of a multi-disciplinary effort encompassing entomology (various rearing techniques), together with food and nutritional science (product formulation and processing methods). For example, Ÿnsect 43 , a French startup, has the largest vertical farm in the world and its recent innovation is a genotyping chip Axiom® YNS_Mol1 for insect breeding aid selection of larvae lines to produce insect-based proteins. This chip has been made available for companies and the scientific community. Big data genome analytics, RNAi and CRISPR are used by Beta 44 to customise their insects 44 . Other startups are working to integrate insect powders into the Western world diet by developing products palatable to the Western taste preferences, e.g., protein bars, chocolates, and beetle-based meats by Hey Planet 45 and/or YumBug 46 opening an insect food-based restaurant. The farming of insects for feed and the production of insect-based foods are relatively recent and bring both benefits and challenges. As with other foods, potential food hazards 41 , 42 of insects-based food could include biological agents (bacterial, viral, fungal, parasitic), chemical contaminants (pesticides, toxic metals, flame retardants), potential allergic reaction, in particular in individuals with crustaceans’ allergies to allergen cross-reactivity. The high nutritional content and the low carbon, water and ecological footprints associated with insect production, as compared to those of other livestock species, make them an attractive protein replacement option for a healthy diet both for animals and humans. However, further studies and monitoring will be required to determine their quality and safety 41 . From the companies’ perspectives more efforts will be required to increase broader consumers’ acceptability of insect-based food and address the current key barriers dealing with neophobia and repulsion (the yuck factor) with insect food 47 . The main focus areas to address these barriers include information dissemination about benefits and how to incorporate the insect-based food and improving sensorial experience by developing appealing products. Plant basedHumans have consumed plant-based protein food since ancient times. Records of using soybean in ancient kitchens to produce soybean milk as well as preparing tofu from coagulated soybean milk go back to the Han Dynasty in China 48 . Advancements in processing technology 19 , in particular, sheer cell, extrusion, structuring processes aiming to develop a fibrous structure, the development textured vegetable proteins, as well as ability to address environmental and food security challenges, have resulted in a significant increase in the consumption of plant-based foods. These methods also enable better mimicking of animal source foods by plant-based meat analogues/alternatives (PBMA) and plant-based dairy analogues/alternatives (PBDA). Currently, there are more than one thousand companies operating in this space with 40% of them focussing on PBMAs and PBDAs food production 49 . Over the last decade there has been a rapid rise in the number of PBMA and PBDA startups with 80% of the current companies in this sector being established during this period 49 . The same trend is also observed across different players ranging from academia research to large food companies. It is becoming a subject of numerous articles and review papers 19 that look at different aspects of producing PBMA and PBDA food including technological developments, life cycle impact assessments to evaluate the sustainability of plant-based meat products, the health benefits, consumers’ perceptions etc. Large food companies recognise the importance of alternative proteins and are increasing their investment as well launching plant-based version of their popular products including dairy-free Philadelphia cream cheese by Kraft Heinz and Mondelez International, Kellogg’s plant-based chicken waffle Eggo sandwich and Burger King’s Impossible burgers 50 . The presence of large food companies has a profound effect on the plant-based alternative market and is driving its consolidation, e.g. The Kellogg Company, Maple Leaf Foods and Conagra Brands taking nearly 70% of the plant-based meat sales in the US with the Kellogg Company accounting for almost 50% of the total sales 51 . Plant-based meat, seafood, eggs, and dairy companies attracted USD 1.2 billion investment in 2022 and the number of unique investors in plant-based companies grew by 17 percent and reached more than 1500 investors 50 . Similarly, to the edible insect category, the plant-based meat alternatives receive significant support from the public sector, e.g. the German government’s promise to invest USD 41 million in plant-based foods and alternative proteins 52 . Denmark, Sweden, and Switzerland committed to invest more than USD 150 million into plant-based protein R&D 50 . The newest versions of PBMA have similar textures, comparable smells, and appearance to help mimic animal meat. The Spanish startup, Novameat 53 uses 3D bioprinting to create fibres and microfibres that unlock the texture of meat and provide versatility to develop a range of PBMA products. To solve the texture challenge, the Israeli startup MeatTheEnd 54 has developed a proprietary technique to incorporate a unique pre-treatment step prior to extrusion to produce texturized protein ingredients. In combination with extrusion technology that is used in mass-scale production, this method results in a cost-effective solution for PBMA companies that seeks to improve the texture of their products. Lypid (US) is looking to address the sensorial and nutritional challenges of PBMA by providing plant-based fats. It uses encapsulation technique to produce emulsion of plant oils (‘alternative fat’) that behaves like animal fat 55 . Another source of alternative proteins are fungi that includes microorganisms such as yeasts and moulds with mushrooms being the most familiar form. A number of startup companies use filamentous fungi as a source of microproteins. For example, Revo Foods (Austria) has developed a proprietary extrusion process and fibrous protein matrix from filamentous fungi to produce 3D-printed salmon-like fillet on a commercial scale 56 while Mycorena (Sweden) is using a liquid fermentation process to produce fungi-based protein food ingredient and also fungi-stabilised fat that can be used to improve the sensorial performance of PBMA products 57 . A cross-sectional analysis 58 of more than 200 products in each product category, PBM and meat, demonstrated that PBM products had significantly lower protein content than meat products, for example, mean protein content per 100 g in meat sausages was 15 g and 12.1 g in PBM sausages; in meat burgers 19.9 g in PBM burgers and 23.3 g in plain chicken and 18.7 g in plant-based chicken. However, according to the UK’s Nutritional Profiling Model, more PBM products were classified as healthier than meat products, i.e. 14% of PBM and 40% of meat products were classified as ‘less healthy’ ( p < 0.001) 58 . Future studies are needed to better understand how the presence and absence of metabolites and nutrients in plant-based meat alternatives and meat impacts short- and long-term consumer health. Technological advances have enabled the field to address a range of critical issues; however, there are still a number of challenges including scalability and cost that remain. The main challenges around PBMAs include allergy concerns associated with soy and wheat; requiring additional flavouring ingredients to achieve the meaty flavour; ability to incorporate fat into the product and potentially a higher risk than meat products of microbial growth due to high-moisture environments with a neutral pH 59 . In terms of PBDAs, they have some performance issues dealing with stability and removal of off flavours, e.g. a beany flavour, bitter taste, and astringency. In addition to the above, there is limited scientific data related to the safety of PBMAs and PBDAs. Make (made/produced protein sources: lab grown meat)Creating a meat substitute is perhaps the most challenging option as it involves the production of meat in vitro. The foresight of growing meat outside an animal environment in 1931 when in his speech ‘Fifty Years Hence’, Winston Churchill said ‘We shall escape the absurdity of growing a whole chicken in order to eat the breast or wing, by growing these parts separately under a suitable medium.’ 60 The latest progress in cross disciplinary efforts including tissue engineering, stem cell biology, bioprocess engineering has made this feat possible. There are currently more than 150 companies 20 working to produce lab grown meat, which typically follow one of the following technologies routes: (1) processing cell cultures grown in bioreactors; (2) 3D-bioprinting or (3) precision fermentation (Fig. 4 ). Processed cell culturesHarrison’s pioneering work 20 in 1907 led to the development of the cell culture techniques. The progress made in more than 100 years of research in this field resulted into it becoming a widely used research tool 21 as well as a wide range of biotechnology applications including the tissue engineering, regeneration medicine, cells for vaccine and cell therapy. ‘Cultured meat’ presents one of the latest developments in the field of cell culture. The approach relies on growing and expanding animal stem cells inside a bioreactor and then using them to produce cultivated meat. In the early 2000s the first world’s research institute dedicated to cultured animal products was formed and it took just over 20 years from a cultured meat concept to a cultured meat product on the market. In recent years there has been a considerable rise of cultured meat startups with 156 companies operating in this space by in 2022 and USD 2.8 billion all-time investment in cultivated meat and seafood companies 12 . Their products range from common meat such as chicken (Upside Food 61 (US), GoodMeat/Eat Just 62 (US)), beef (Aleph Farms 63 (Israel)), pork (Meatable 64 (Netherlands)) to seafood (Wildtype 65 (US), Avant Meat 66 (China) and high-end food including foie gras (Gourmey 67 (France)). In the 2016-2022 period, the cultivated meat and seafood companies attracted USD 2.78 billion 12 . The funding streams include unique investors reaching 679 investors 12 , strategic partnerships between cultivated meat companies and major food companies with at least 35 major partnerships 12 including established companies like Nestlé, Merck KGaA, Mitsubishi, JBS, Kerry, and CP Kelco 12 and public funding. For example, Singapore’s government launched a number of programmes to support alternative protein startups and accelerate innovation including building the world’s first hybrid innovation centre dedicated to cultivated and plant-based meat products 68 . The development and manufacturing of alternative proteins, including cultivated meat, is a part of the UK Government £2 billion National Vision for Engineering Biology plan 69 . Cultured meat is still not widely available, and a number of hurdles 22 need to be overcome before it will become a product that is readily available to the masses. These challenges include the lack of regulatory guidelines: regulatory approvals are required to sell a product and many countries do not have established protocols for certifying cultured meat. The startups are navigating the current regulatory landscape, e.g., Singapore was the first country that approved cultivated chicken for public sale in 2020 70 , since then two startups, Upside Foods and Eat Just, received FDA’s approval 61 , 62 for their lab-grown chicken. Aleph Farms is seeking regulatory approval to sell its beefsteak in the UK and Switzerland 63 . To help facilitate the development of regulatory protocols, more understanding would be required to assess potential risks of microbial contamination, genetically engineered starting materials etc to address any food safety concerns. The other challenges include sensorial and visual acceptance and cost. For example, to develop a satisfying prototype version that could match the delicate flavour and creamy texture of foie gras, Gourmey tested 600 to 650 different compound interactions. Its product will be marketed as a “poultry delicacy as it cannot be called foie gras” in France. Wildtype is another startup that successfully managed the sensorial challenge and created sushi-grade salmon by cultivating cells extracted from salmon eggs and it has been reported that the resulting tastes like conventional sushi-grade salmon 65 . However, it comes at a cost - the 127 g (4.5 ounce) portion cost about USD 150 in food costs alone (USD 533 per 450 g (1 pound)). Limited information is available about the protein content in cultured meat 22 and according to morphological observations, there are indications that the current cultured meat with most of the cytoskeletal proteins is in the same range as traditional meat 71 . The cost represents another challenge of cultivated meat, which will decrease as technology improves to enable scale up. There is already evidence on making scalable beef cell lines using CRISPR by SciFi Foods 72 , a US based cultivated meat startup, which aims to eventually reach USD $1 per burger at a commercial scale. SCiFi noted that their technology allowed the cost reduction of cultivated meat by more than 1000 times compared to current production costs and less than USD 10 cost for its blended burger, part plant-based and part cultivated meat, that is 33,000 times less than the first cultivated burger developed by Mark Post and Peter Verstrate less than a decade ago that had a production cost of USD 330,000 73 . 3D bioprintingAdditive manufacturing, the process of joining materials to make objects from computer-aided design model data, such as 3D printing, have opened tremendous opportunities in a broad spectrum of applications in several industry sectors. The integration of 3D printing into tissue engineering provides opportunities for many innovation solutions including regeneration medicine, in-vitro models, pharmaceutical and food industries and healthcare challenges and heralds’ new frontiers in medicine, pharmaceutical, and food industries 74 . Scientists from Osaka University used this method to print Wagyu beef 75 that resembles the real pieces of meat and reproduces its complex structure formed by muscle fibres, fat, and blood vessels. The fibres fabricated from stem cells using bioprinting were then arranged in 3D to reproduce the structure of the real Wagyu meat and sliced perpendicularly, like the traditional Japanese candy Kintaro-ame. Tendon-gel-integrated bioprinting used for the fibre cells’ fabrication could expand a culture meat toolbox and provide a valuable approach for constructing engineered steak-like meat. Precision fermentationThe advancements in genome-based technologies enabled a transformation of the traditional fermentation process and development of precision fermentation that uses microbial hosts as ‘cell factories’ for producing specific functional ingredients 18 . This process has been used to produce animal-based proteins and can save water and land compared to traditional livestock farming with the added benefit of zero methane gas emission. For example, startups Perfect Day (US) 76 and Eden Brew (Australia) 77 use this approach to produce the same proteins found in cow milk and create synthetic milk that have similar taste, look, and feel to dairy milk. While the technology has significant potential to address environmental challenges, there is an on-going discussion of its downsides, for example, in the case of synthetic milk, its potential impact on to the dairy industry and conventional agriculture and the prospect of pushing out low-tech or small-scale dairy farms. However, to do so, the industry must grow exponentially and build new manufacturing infrastructure (e.g., fermentation tanks, bioreactors) that would require a considerable amount of investment. Another startup that uses precision fermentation is Air Protein (US) 78 . It uses microbes to transform carbon dioxide from air into meat that originates from the 1970s space programme where NASA scientists explored a way to feed astronauts on long space journeys by transforming elements in the air that the astronauts breathed into proteins 79 . The end fermentation product is a versatile protein-rich flour, which has a similar amino acid profile as meat protein and can be turned into any food using a combination of pressure, temperature, and other technologies. The manufacturing process has climate-saving potential: it is carbon-negative and compared to beef, uses 1.5 million times less land and reduces water usage 15,000 times. Like with other cultured meat challenges, the most crucial aspect is making the process cost competitive. Published techno-economic analysis indicated a production range in a hypothetical commercial-scale facility ranging from USD 17 to USD 65 per kilogram where the largest cost drivers include culture media, bioreactors, and labour 12 . New technologies and discoveries continue to fuel the field of alternative protein sources. Alternative protein sources used to fabricate meat, fish and diary food products can help to address food security challenges and mitigate environmental issues, by significantly reducing emissions and requiring far less land. For example, based on a life cycle assessment, plant-based meat can cut emissions by 90 percent, and use 99 percent less land and water compared to conventional meat 80 . The current alternative proteins sources include insect-, fungi- and plant-based proteins and cell-based proteins grown from animal cells in the form of cultivated meat and fish. Producing food from alternative proteins has made a big leap forward in the past decade with plant-, fungi- and insect-based food available in grocery shops and food made from meat substitutes being served in restaurants. However, there are still a number of challenges to overcome before these products will become a commodity accessible to everyone. The main challenges (Fig. 5 ) include consumer acceptance driven by their ability to mimic traditional food in terms of texture, taste and appearance; affordability due to high cost associated with a complex technological process and its scalability as well as raw materials cost and a development of regulatory framework to enable market accessibility that requires more research to demonstrate their long-term safety profile and potential health risks. 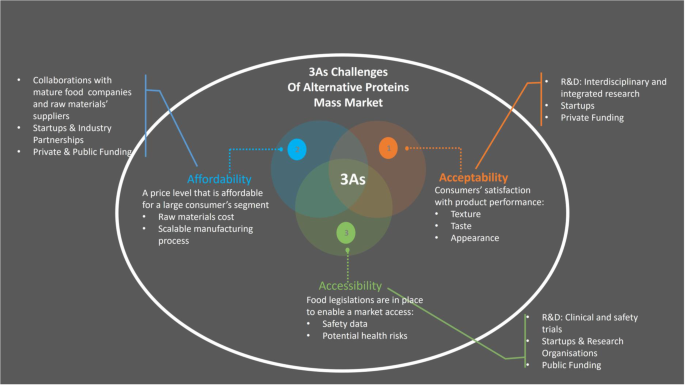 The main challenges for alternative proteins food to become a commodity could be divided into three groups: (1) Affordability, (2) Acceptability and (3) Accessibility that form 3As framework. Affordability challenge is driven by high cost associated with a complex technological process and its scalability as well as raw materials cost. Acceptability challenge deals with consumer acceptance of alternative protein proteins products and their ability to mimic traditional food in terms of texture, taste, and appearance. Accessibility challenge is ability to place these products on the market and rely on a regulatory framework that is in a development stage and requires more research to demonstrate their long-term safety profile and potential health risks. Multi-dimensional efforts are being put in place to address these challenges that include interdisciplinary and integrated research, new partnerships and global alliances and continuous investment from both private and public sources. Specific examples of these activities are presented for each of the 3As challenges. Interdisciplinary and integrated research, new partnerships and global alliances and continuous investment from both private and public sources 81 will enable these issues to be addressed. The Importance of alternative protein sources to achieve national economic growth, sustainability and food production objectives have been recognised by both government and non-government organisations including forming cross-stakeholders’ partnerships, e.g. the U.S.-based Alliance for Meat, Poultry, and Seafood Innovation, the APAC Society for Cellular Agriculture, and Cellular Agriculture Europe launched a new global alliance to collaborate on regulatory work, consumer research, and nomenclature 12 . The above creates fertile ground for startups in this sector to continue evolving and to deliver breakthrough innovation by bringing fresh perspectives, creativity, a willingness to take risks, targeting niche issues (in particularly, sensorial perception barriers) and their ability to bridge between the latest advancements in academic research and the go-to-market resources of large companies. Prevalence of undernourishment worldwide 2005-2022. Statista https://www.statista.com/statistics/264901/proportion-of-starving-people-in-the-world-population/#:~:text=Prevalence%20of%20undernourishment%20worldwide%202005%2D2022&text=After%20the%20share%20of%20people,were%20undernourished%20worldwide%20that%20year (2023). Ranganathan, J., Waite, R., Searchinger, T. & Hanson, C. World Resources Report: Creating a Sustainable Food Future https://www.wri.org/insights/how-sustainably-feed-10-billion-people-2050-21-charts (2018). Savary, S. et al. The global burden of pathogens and pests on major food crops. Nat. Ecol. Evol. 3 , 430–439 (2019). Article PubMed Google Scholar Creating a Sustainable Food Future: A Menu of Solutions. https://www.wri.org/research/creating-sustainable-food-future (2019). Kumar, K. et al. Genetically modified crops: current status and future prospects. Planta 251 , 91 (2020). Article CAS PubMed Google Scholar Dai, J., Bai, M., Li, C., Cui, H. & Lin, L. Advances in the mechanism of different antibacterial strategies based on ultrasound technique for controlling bacterial contamination in food industry. Trends Food Sci. Technol. 105 , 211–222 (2020). Article CAS Google Scholar Reyns, P. et al. A review of combine sensors for precision farming. Precis. Agric. 3 , 169–182 (2002). Article ADS Google Scholar Sourav, A. & Emanuel, A. W. R. Recent trends of big data in precision agriculture: a review. IOP Conf. Ser.: Mater. Sci. Eng. 1096 , 012081 (2021). Article Google Scholar Kamilaris, A., Fonts, A. & Prenafeta-Boldύ, F. X. The rise of blockchain technology in agriculture and food supply chains. Trends Food Sci. Technol. 91 , 640–652 (2019). Drago, E., Campardelli, R., Pettinato, M. & Perego, P. Innovations in smart packaging concepts for food: an extensive review. Foods 9 , 1628 (2020). Article CAS PubMed PubMed Central Google Scholar Ben-Ary, T., &. Levenber, S. Tissue engineering for clean meat production. Front. Sustain. Food https://doi.org/10.3389/fsufs.2019.00046 . (2019). Good Food Institute State of the Industry Report ‘Cultivated meat and seafood’ https://gfi.org/wp-content/uploads/2023/01/2022-Cultivated-Meat-State-of-the-Industry-Report-2-1.pdf . (2022). The GFI report provides a comprehensive overview of the science of cultivated meat and challenges that must be addressed for its commercialisation as well as its current development status . Andreoli, V., Bagliani, M., Corsi, A. & Frontuto, V. Drivers of protein consumption: a cross-country analysis. Sustainability 13 , 7399 (2021). The paper explores the relationship between per capita income and animal and vegetal protein consumption . Béné, C. & Lundy, M. Political economy of protein transition: battles of power, framings and narratives around a false wicked problem. Front. Sustain. 4 , 1098011 (2023). Wood, P. & Tavan, M. A review of the alternative protein industry. Curr. Opin. Food Sci. 47 , 100869 (2022). Lima, M., Costa, R., Rodrigues, I., Lameiras, J. & Botelho, G. A narrative review of alternative protein sources: highlights on meat, fish, egg and dairy analogues. Foods 11 , 2053 (2022). Munialo, C. D. A review of alternative plant protein sources, their extraction, functional characterisation, application, nutritional value and pinch points to being the solution to sustainable food production. Int J. Food Sci. Technol. https://doi.org/10.1111/ijfs.16467 (2023). Netsanet Shiferaw Terefe, N. S. Food Engineering Innovations Across the Food Supply Chain . 89-106 (ed. Juliano, P., et al.) (Academic Press, 2022). He, J., Evans, N. M., Liu, H. & Shao, S. A review of research on plant-based meat alternatives: driving forces, history, manufacturing, and consumer attitudes. Compr. Rev. Food Sci. Saf. 19 , 2639–2656 (2020). Jedrzejczak-Silicka, M. History of cell culture. new insights into cell culture technology. InTech https://doi.org/10.5772/66905 (2017). Edelman, P. D., McFarland, D. C., Mironov, V. A. & Matheny, J. G. Commentary: In vitro-cultured meat production. Tissue Eng. 11 , 659–662 (2005). PMID: 15998207. Broucke, K., Els Van Pamel, E., Van Coillie, E., Herman, L. & Van Royen, G. Cultured meat and challenges ahead: A review on nutritional, technofunctional and sensorial properties, safety and legislation. Meat Sci. 195 , 109006 (2023). FoodData Central (an integrated data system that provides expanded nutrient profile data and links to related agricultural and experimental research). https://fdc.nal.usda.gov/ (2023). FooDB (a web-based resource on food constituents, chemistry and biology and provides information on both macronutrients and micronutrients). https://foodb.ca/ (2022). Malaysian Food Composition Database (FCD): the database provides food composition and nutrients of food materials. https://myfcd.moh.gov.my/ (2015). Al-Sarayreh, A. Inverse design and AI/Deep generative networks in food design: a comprehensive review. Trends Food Sci. Technol. 138 , 215–228 (2023). Anything you eat, you can eat vegan! https://www.eatkind.co/GB (Woodhead Publishing, 2023). Meyer-Rochow, V. Can insects help to ease the problem of world food shortage. Search 6 , 261–262 (1975). Google Scholar Akhtar, Y., & Isman, M. B. In Woodhead Publishing Series in Food Science, Technology and Nutrition. Proteins in Food Processing (Second Edition). (ed. Rickey Y. Yada) 263-288. https://doi.org/10.1016/B978-0-08-100722-8.00011-5 (2018). Weru, J. et al. Comparison of healthfulness of conventional meats and edible insects in Sub-Saharan Africa using three nutrient profiling models. Bull. Natl Res Cent. 46 , 43 (2022). Orkusz, A. Edible Insects versus Meat-Nutritional Comparison: Knowledge of Their Composition Is the Key to Good Health. Nutrients 13 , 1207 (2021). This article compares the nutritional value of edible insects and meat and shows that the content of individual nutrients in both insects and meat varies significantly . Payne, C. L., Scarborough, P., Rayner, M. & Nonaka, K. Are edible insects more or less ‘healthy’ than commonly consumed meats? A comparison using two nutrient profiling models developed to combat over- and undernutrition. Eur. J. Clin. Nutr. 70 , 285–291 (2016). Kim, T. K., Yong, H. I., Kim, Y. B., Kim, H. W. & Choi, Y. S. Edible insects as a protein source: a review of public perception, processing technology, and research trends. Food Sci. Anim. Resour. 39 , 521–540 (2019). Article PubMed PubMed Central Google Scholar Edible Insects Market Size & Share Analysis - Growth Trends & Forecasts (2023 - 2028). Mordor Intelligence Report https://www.mordorintelligence.com/industry-reports/edible-insects-market (2023). Kröger, T., Dupont, J., Büsing, L. & Fiebelkorn, F. Acceptance of insect-based food products in western societies: a systematic review. Front. Nutr. (Lausanne) 8 , 759885–759885 (2022). Glasne, J. Surprisingly Large Sums Have Gone Into Bug Farming Startups. https://news.crunchbase.com/venture/foodtech-bug-farming-startups/ (2023). Tyson Foods Announces Partnership with Protix for More Sustainable Protein Production. https://protix.eu/wp-content/uploads/TysonFoodsAndProtixPartnership.pdf (2023). Ÿnsect launches its first product in the US with partner pure simple true LLC. https://www.ynsect.com/2021/11/09/ynsect-launches-its-first-product-in-the-us-with-partner-pure-simple-true-llc/ (2021). Insect industry abuzz with new knowledge sharing hub. https://www.aciar.gov.au/media-search/news/insect-industry-abuzz-new-knowledge-sharing-hub (2022). Insectrial Revolution: Shaping the future of sustainable protein. https://iuk.ktn-uk.org/casestudy/shaping-the-future-of-sustainable-protein/ (2021). Food and Agriculture Organisation of United Nation Report ‘Looking at edible insects from a food safety perspective. Challenges and opportunities for the sector’ https://doi.org/10.4060/cb4094en (2021). The report provides an overview of the various food safety issues associated with edible insects and the regulatory frameworks and highlights major challenges that would need to overcome to have a more global reach . Food Standard Agency Report ‘Technical Report Risk Profile on Edible Insects’ https://www.food.gov.uk/research/edible-insects-summary (2022). World-first: Ÿnsect reveals the first high-density genotyping chip for insect breeding. https://www.ynsect.com/2023/06/06/world-first-ynsect-reveals-the-first-high-density-genotyping-chip-for-insect-breeding/ (2023). From data farm to bug farm. https://betahatch.com/innovation/ (2023). Shop Hey Planet. https://www.hey-planet.com/collections/webshop (2024). We’ve opened a permanent bug-restaurant! https://www.yumbug.com/book-table (2024). Karin, M. E., Wendin, M., Nyberg, E. Factors influencing consumer perception and acceptability of insect-based foods. Current Opinion in Food Science, 40, 67-71 https://doi.org/10.1016/j.cofs.2021.01.007 (2021). This paper presents different factors influencing consumer perception and acceptability of insect-based foods and their complexity in influencing consumers’ perceptions and acceptability of insects as food . Bei-Zhong Han, B. Z., Rombouts, F. M. & Nout, M. J. R. A Chinese fermented soybean food. Int. J. Food Microbiol. 65 , 1–10 (2001). Alternative protein manufacturers and brands. https://gfi.org/resource/alternative-protein-company-database/#manufacturers-and-brands (2024). Good Food Institute State of the Industry Report ‘Pant-based meat, seafood, egg and dairy.’ https://gfi.org/wp-content/uploads/2023/01/2022-Plant-Based-State-of-the-Industry-Report.pdf (2022) The GFI report in-depth understanding of the plant-based protein market, issues, and opportunities and details some of the promising developments . FWW analysis of ‘Top Meat Substitute Makers (Refrigerated/ Frozen), 2019.’ (eds. Burton, Virgil L. and Robert S. Lazich). Market Share Reporter. 31st edn. Detroit: Gale (2021). Clark, D. Germany Unveils ‘Groundbreaking’ Investment In Plant-Based Food. https://plantbasednews.org/culture/law-and-politics/germany-investment-plant-based-future/ (2023). Biomimicry is our engine. https://www.novameat.com/our-technology (2023). Our Extrusion: Putting the Tech in Texture. https://www.meattheend.tech/our-technology (2021). Watson, E. Meet the founders: Lypid tackles fat, the final frontier for alt meat. https://agfundernews.com/meet-the-founders-lypid-tackles-fat-the-final-frontier-for-alt-meat (2023). Vegan Salmon Filet becomes first 3D printed product available in supermarkets. https://www.revo-foods_1.prowly.com/260307-vegan-salmon-filet-becomes-first-3d-printed-product-available-in-supermarkets (2023). Creating unique fungi solutions. https://mycorena.com/technology (2024). Alessandrini, R., Brown, M. K., Pombo-Rodrigues, S., Bhageerutty, S., He, F. J. & MacGregor, G. A. Nutritional quality of plant-based meat products available in the UK: a cross-sectional survey. Nutrients 13 , 4225 (2021). Elhalis, H., See, X. Y., Osen, R., Chin, X. H. & Chow, Y. Significance of fermentation in plant-based meat analogs: a critical review of nutrition, and safety-related aspects. Foods 12 , 3222 (2023). Fifty Years Hence. https://www.nationalchurchillmuseum.org/fifty-years-hence.html (1931). Cultivated meat. It’s science (but not rocket science). https://upsidefoods.com/innovation (2024). GOOD Meat Gets Full Approval in the U.S. for Cultivated Meat. https://www.goodmeat.co/all-news/good-meat-gets-full-approval-in-the-us-for-cultivated-meat (2023). Doenecke, P. Aleph Farms Seeks Swiss Regulatory Approval for Cultivated Meat. https://www.bloomberg.com/news/articles/2023-07-27/aleph-farms-seeks-swiss-regulatory-approval-for-cultivated-meat-lkleralp (2023). Meatable reveals its groundbreaking pork sausages product for the first time. https://meatable.com/news-room/ (2022). Kateman, B. Cell-Cultured Seafood Isn’t just An Idea; It’s A Reality. https://www.forbes.com/sites/briankateman/2022/06/06/cell-cultured-seafood-isnt-just-an-idea-its-a-reality/?sh=328115b3146d (2022). The Journey of Avant Meats. https://www.avantmeats.com/about-us (2024). Kahn, J. French startup promises cruelty-free foie gras, grown in a lab. https://fortune.com/2021/07/14/foie-gras-lab-grown-gourmey-startup-ethical-food/ (2021). World’s first hybrid meat innovation centre to open in Singapore in 2023. https://www.edb.gov.sg/en/business-insights/insights/world-s-first-hybrid-meat-innovation-centre-to-open-in-singapore-in-2023.html (2022). National Vision for Engineering Biology. https://assets.publishing.service.gov.uk/media/656de8030f12ef07a53e01ac/national_vision_for_engineering_biology.pdf (2023). Kessler, J. Singapore approves sale of lab-grown meat in world first. https://www.standard.co.uk/news/singapore-lab-grown-meat-chicken-nuggets-b141083.html (2020). Post, M. J.& Hocquette, J. F. Chapter 16 - New Sources of Animal Proteins: Cultured Meat, Editor(s): Peter P. Purslow, In Woodhead Publishing Series in Food Science, Technology and Nutrition, New Aspects of Meat Quality, Woodhead Publishing. Pages 425–441 (2017). Poinski, M. SCiFi Foods reduces the cost of cell-based beef 1000-fold. https://www.fooddive.com/news/scifi-foods-cell-based-cultivated-beef-1000-cost-reduction/627122/ (2022). Baker, A. The Cow That Could Feed the Planet. https://time.com/6109450/sustainable-lab-grown-mosa-meat/ (2021). Ramadan, Q. & Zourob, M. 3D bioprinting at the frontier of regenerative medicine, pharmaceutical, and food industries. Front. Med. Technol. 2 , 607648 (2021). Kang, D. H., Louis, F. & Liu, H. et al. Engineered whole cut meat-like tissue by the assembly of cell fibers using tendon-gel integrated bioprinting. Nat. Commun. 12 , 5059 (2021). Article ADS CAS PubMed PubMed Central Google Scholar Championing a more resilient future. https://perfectday.com/precision-fermentation-alliance/ (2023). Partnering with nature to meet dairy protein demand sustainably. https://www.edenbrew.com.au/product (2023). Lev-Ram, M. Meet Lisa Dyson, who looked to the space program for new ways to create nutritional food. https://fortune.com/2023/08/10/lisa-dyson-founders-forum-2023/ (2023). Carbon Capture Process Makes Sustainable Oil. https://spinoff.nasa.gov/Spinoff2019/ee_4.html (2019). Heller, M. C. & Keoleian, G. A. Beyond Meat’s Beyond Burger Life Cycle Assessment: A detailed comparison between a plant-based and an animal-based protein source. CSS Report, University of Michigan: Ann Arbor 1-38 (2018). https://hdl.handle.net/2027.42/192044 . A deeper dive into alternative protein investments in 2022: The case for optimism. https://gfi.org/blog/alternative-protein-investments-update-and-outlook/ (2023). Download references Author informationAuthors and affiliations. Department of Biosciences, Durham University, DH1 3LE, Durham, UK Elena Lurie-Luke You can also search for this author in PubMed Google Scholar ContributionsE.L.L. conceptualised the manuscript and was responsible for all aspects of its preparation. Corresponding authorCorrespondence to Elena Lurie-Luke . Ethics declarationsCompeting interests. The author declares no competing interests. Peer reviewPeer review information. Nature Communications thanks Federico Casanova and Marcelle Machluf for their contribution to the peer review of this work. Additional informationPublisher’s note Springer Nature remains neutral with regard to jurisdictional claims in published maps and institutional affiliations. Rights and permissionsOpen Access This article is licensed under a Creative Commons Attribution 4.0 International License, which permits use, sharing, adaptation, distribution and reproduction in any medium or format, as long as you give appropriate credit to the original author(s) and the source, provide a link to the Creative Commons licence, and indicate if changes were made. The images or other third party material in this article are included in the article’s Creative Commons licence, unless indicated otherwise in a credit line to the material. If material is not included in the article’s Creative Commons licence and your intended use is not permitted by statutory regulation or exceeds the permitted use, you will need to obtain permission directly from the copyright holder. To view a copy of this licence, visit http://creativecommons.org/licenses/by/4.0/ . Reprints and permissions About this articleCite this article. Lurie-Luke, E. Alternative protein sources: science powered startups to fuel food innovation. Nat Commun 15 , 4425 (2024). https://doi.org/10.1038/s41467-024-47091-0 Download citation Received : 18 December 2023 Accepted : 20 March 2024 Published : 28 May 2024 DOI : https://doi.org/10.1038/s41467-024-47091-0 Share this articleAnyone you share the following link with will be able to read this content: Sorry, a shareable link is not currently available for this article. Provided by the Springer Nature SharedIt content-sharing initiative By submitting a comment you agree to abide by our Terms and Community Guidelines . If you find something abusive or that does not comply with our terms or guidelines please flag it as inappropriate. Quick links- Explore articles by subject
- Guide to authors
- Editorial policies
Sign up for the Nature Briefing: Translational Research newsletter — top stories in biotechnology, drug discovery and pharma.  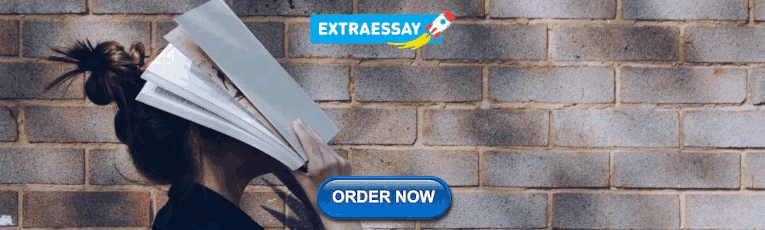 |
COMMENTS
The aim of the paper is to ascertain if renewable energy sources are sustainable and examine how a shift from fossil fuel-based energy sources to renewable energy sources would help reduce climate change and its impact. ... Research into alternate sources of energy dated back in the late 90s when the world started receiving shock from ...
The aim of this review paper is to understand and study further the current RE technologies such as solar energy, hydro energy, wind energy, bioenergy, geothermal energy, and hydrogen energy. ... Hydrogen energy sustainable since it can be generated from renewable and non-renewable energy sources [245], thus can be utilized in various sectors ...
The use of renewable energy resources, such as solar, wind, and biomass will not diminish their availability. Sunlight being a constant source of energy is used to meet the ever-increasing energy need. This review discusses the world's energy needs, renewable energy technologies for domestic use, and highlights public opinions on renewable energy. A systematic review of the literature was ...
The major sources of renewable. energy include solar, wind, biomass, geothermal, hydropower. and tidal energy. Non renewable energy, such as coal, natural. gas and oil, require costly explorations ...
More energy efficiency means less pollution, and energy efficiency has increased by around 2% annually in the past few years. But meeting the target for 2030 — to double the rate of the 1990 ...
The energy transition must reduce emissions substantially, while ensuring that sufficient energy is available for economic growth. The analysis shows that the CO 2 emissions intensity of global economic activity needs to be reduced by 85% between 2015 and 2050, and CO 2 emissions need to decline by more than 70% compared to the Reference Case in 2050. . The result is an annual decline of ...
fuel and two-third of the global energy demand is fulfilled by renewable energy sources. According to a report by Bloomberg New Energy Finance, b y 2050 about 50% of the. global energy demand is ...
Our study evaluated the effectiveness of using eight pathways in combination for a complete to transition from fossil fuels to renewable energy by 2050. These pathways included renewable energy development; improving energy efficiency; increasing energy conservation; carbon taxes; more equitable balancing of human wellbeing and per capita energy use; cap and trade systems; carbon capture ...
A large-scale analysis, comprising 1 million water bodies worldwide, shows that floating photovoltaics could contribute 16%, on average, of the electricity demands of some countries. R. Iestyn ...
About other renewable energy sources, Turkey had the largest share in generating energy from wind and solar energy, with an annual growth rate of (88.03%, 98.22%), respectively.
Energy security and sustainability are undeniably the main concerns in combatting climate change. While an immediate call for all-green and renewable energy seems to be impossible due to huge financial implications and inadequate supporting energy structure, an alternative to fossil fuels needs to be established. Natural gas, a naturally occurring fossil gas is a cleaner energy source option ...
Worldwide, energy is harnessed from fossil fuels as well as from alternative sources of energy. However, for energy generation, fossil fuels are exploited at a larger scale, and the role of alternative energy sources is not substantial. In future, the contribution of renewable energy sources will be crucial for energy sustainability.
This paper comprehensively reviews and explores renewable energy as an alternative energy source for efficient energy management in the agricultural sector. ... Renewable energy sources like wind and solar can be used to power farm vehicles in a way that is good for the economy ... Sardar Patel Renewable Energy Research Institute, Vallabh ...
Research published in this series may include views on policy, but the institute itself takes no institutional policy positions. ... accident was a turning point in the call for alternative energy sources. Renewable energy is ... In this paper, we discuss alternative technologies for enhancing renewable energy deployment and energy use efficiency.
Resources is an international peer-reviewed open access monthly journal published by MDPI. Please visit the Instructions for Authors page before submitting a manuscript. The Article Processing Charge (APC) for publication in this open access journal is 1600 CHF (Swiss Francs). Submitted papers should be well formatted and use good English.
Wind power, solar power and water power are technologies that can be used as the main sources of renewable energy so that the target of decarbonisation in the energy sector can be achieved. However, when compared with conventional power plants, they have a significant difference. The share of renewable energy has made a difference and posed various challenges, especially in the power ...
The increase in renewable energy use leads to a decline in fossil fuel and nuclear energy use in most of the regions, resulting in a 1-2% reduction in cumulative CO 2 emissions (2015-2100). In ...
Gross electricity generation from renewable energy—according to sources. Table 16 shows the gross electricity generation from renewable energy—source-wise. It can be concluded from the table that the wind-based energy generation as per 2017-2018 is most prominent with 51.71%, followed by solar energy (25.40%), Bagasse (11.63%), small ...
Introduction. In response to the advantages of renewable energy (Gullberg et al., 2014), many countries and regional organizations have entered into cooperative targeted renewable energy initiatives (Anand et al., 2021; Mohan, 2021; Sasmita and Sidhartha, 2021).Existing research on renewable cooperation (Feng et al., 2020) is mainly focused on a comprehensive analysis of the renewable energy ...
The Philippines is making a significant stride to become energy independent by developing more sustainable sources of energy. However, investment in renewable energy is challenged by competitive oil prices, very high investment cost for renewable energy, and high local electricity prices. This paper evaluates the attractiveness of investing in renewable energy sources over continue using oil ...
Electricity production from non-fossil-fuel sources has a significant coefficient of −0.079, very similar to what was found in model 1. This coefficient indicates that close to 13 kWh (1/0.079 ...
Instead of fossil fuels, the energy sector is based largely on renewable energy. Two-thirds of total energy supply in 2050 is from wind, solar, bioenergy, geothermal and hydro energy. Solar becomes the largest source, accounting for one-fifth of energy supplies. Solar PV capacity increases 20-fold between now and 2050, and wind power 11-fold.
The transition toward a 100% Renewable Energy System is a complex process with different technical and economic challenges. In order to achieve predetermined goals, several steps should be carried out simultaneously, including increment of energy efficiency, savings in primary energy consumption, and finally, deployment of variable renewable energy sources (VRES) [1].
A large share of non-renewable energy use in egg production is due to the operation of heating, ventilation, and air conditioning (HVAC) systems. ... this paper, along with the future research opportunities it provides, will be valuable to egg farmers, renewable energy industries, and HVAC engineers. ... Energy Sources Part Recovery Util ...
Globally, a bit more than a quarter of electricity comes from wind, solar and other renewable sources which, as opposed to fossil fuels, emit little to no greenhouse gases or pollutants into the ...
In 2018, we launched the China Clean Energy Fund to accelerate renewable progress in China. Together with our suppliers, we've invested in 1,000 megawatts of clean energy. Additionally, through the RE100 initiative, we partner with the world's most influential businesses that have committed to using 100% renewable electricity.
Renewable energy. Reducing transport emissions. Energy data. Energy efficiency. Energy markets. Energy programs. Energy security. Energy supply. Energy workforce. ... Science and research Undertaking research and collecting data to support informed decisions and policies. Climate change. Australia's biological resources ...
The required Concept Paper due date for this FOA is 06/13/2024 at 5PM ET. ... lowered energy burdens, increased access to renewable energy, improved air quality, increased public participation in energy decision-making processes, and improved quality of life for local residents. ... over a 5-year period from FY 2022 through 2026 to develop ...
The renewable energy sources is renewed and environmental friendly in nature. ... All authors of this research paper have directly participated in the planning, execution, or analysis of this study. ... International Journal of Renewable Energy Research, 9 (1) (2019), pp. 309-342. Google Scholar. Charles et al., 2019a.
When it comes to finding an alternative, there are three main options to consider: (1) using a replacement, (2) modifying existing non-animal/non-fish sources of proteins, and (3) making an ...