
NurseStudy.Net
Nursing Education Site
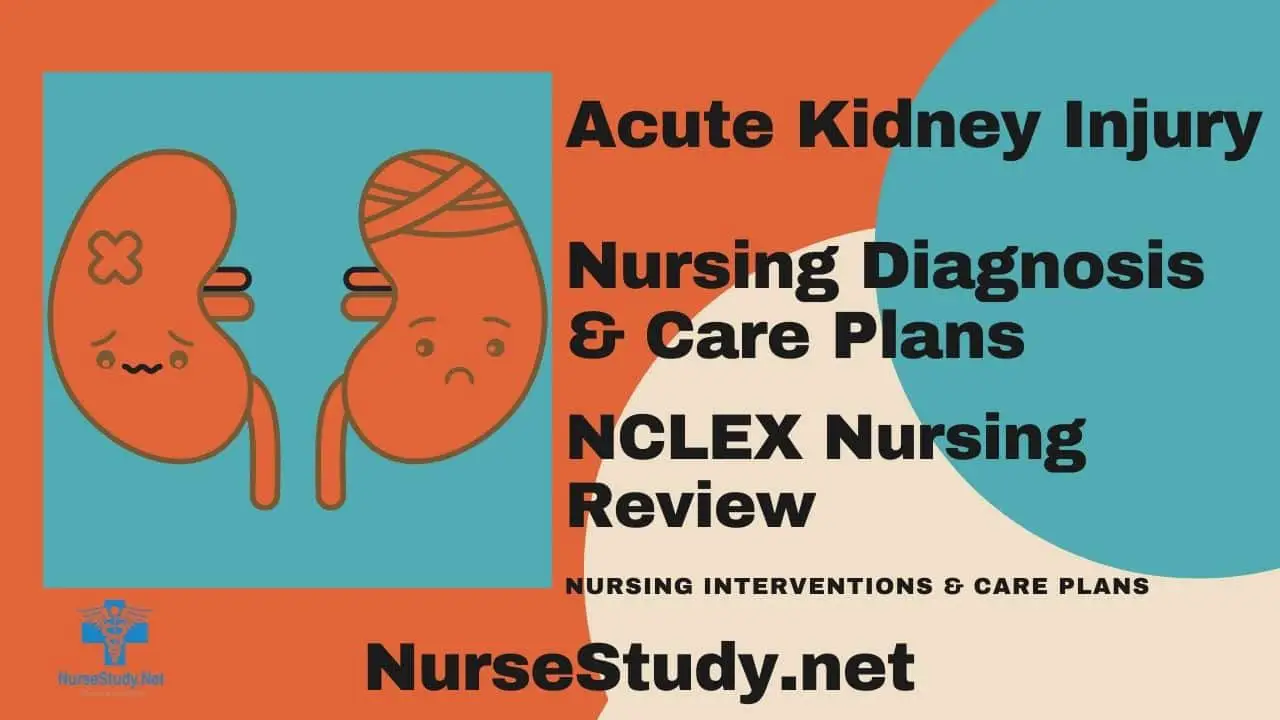
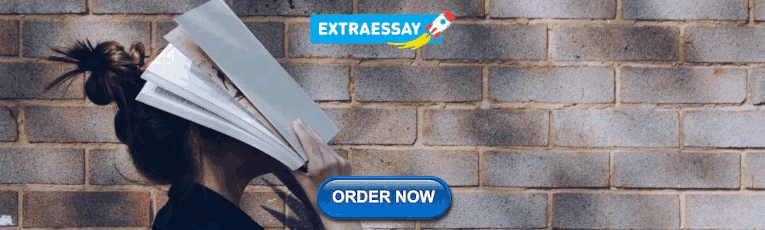
Acute Kidney Injury Nursing Diagnosis and Nursing Care Plan
Last updated on January 26th, 2024 at 05:13 pm
Acute Kidney Injury Nursing Care Plans Diagnosis and Interventions
Acute Kidney Injury NCLEX Review and Nursing Care Plans
The kidneys have a wide range of functions. Their primary role is to remove waste from the bloodstream. They also manage blood pressure by removing excess fluid from the blood.
Also, kidneys aid in the production of red blood cells, the regulation of electrolytes, and the activation of vitamin D.
Knowing that our kidneys serve a variety of roles in maintaining our bodies’ health, one can only imagine what would happen if they were damaged. One of the common kidney diseases that occur most of the time in conjunction with another medical condition or event is acute kidney injury (AKI).
Acute kidney injury is a sudden loss of kidney function in which the kidneys are unable to filter waste products from the blood. When the kidneys lose their ability to filter waste, the chemical makeup of the blood may go out of balance, and dangerous levels of waste may accumulate.
Acute renal failure is another name for acute kidney injury. It can damage other organs, including the brain, heart, and lungs , and develops fast, generally in a few hours or days.
Acute kidney injury is more likely to occur among individuals who have already been admitted to a hospital, particularly those who are severely ill and require intensive care.
Acute kidney injury affects one in every million persons in the United States each year, and it is identified in 1% among all hospital admissions.
If left untreated, acute kidney injury can lead to chronic renal failure , which is fatal and requires a lot of treatment. Acute kidney injury may also be reversible in patients who do not have other serious health problems. These patients may regain normal or near-normal kidney function if managed earlier.
The goal of nursing care for individuals with acute kidney injury is to address or eliminate any causes that can be reversed. Prompt diagnosis of AKI’s underlying causes, correcting fluid and electrolyte imbalances, acid-base balance stabilization, proper nutrition, and preventing complications are all part of patient care.
Signs and Symptoms of Acute Kidney Injury
Acute kidney injury can have diverse signs and symptoms, depending on the etiology, which includes the following:
- Scanty or insufficient urine output, but may also be normal to some patients on occasion
- Swelling of the legs, ankles, and around the eyes as a result of fluid retention
- Tiredness or fatigue
- Breathing difficulty
- Pressure or pain in the chest
- abnormal heart rhythm
- In severe cases, seizures or comas
In some circumstances, acute kidney injury has no signs and symptoms and is only discovered by coincidence when a healthcare provider orders various tests.
Causes of Acute Kidney Injury
Acute kidney injury can be caused by a variety of causes, including the following:
- Decreased blood flow to the kidneys. Acute kidney injury can be caused by a variety of diseases and conditions that impede or reduce blood flow to the kidneys, such as:
- Hypotension (low blood pressure) or shock
- Loss of blood or fluids (such as bleeding, severe diarrhea )
- Decreased heart function caused by cardiovascular disorders.
- Organ failure
- Over usage of non-steroidal anti-inflammatory drugs ( NSAIDs ), used to treat headaches, colds, flu, and other common illnesses.
- Anaphylaxis
- Severe burns
- Major operations
2. Direct Kidney Damage. Diseases, conditions, and substances that damage the kidneys and cause acute kidney injury include:
- Sepsis or severe response to an infection
- Inflammation of the small filters in the kidneys – known as glomerulonephritis
- Hemolytic uremic syndrome – a disorder in which red blood cells are destroyed prematurely
- Lupus , an autoimmune disease that can also cause glomerulonephritis
- Scleroderma , a rare skin and connective tissue disorders
- A rare blood condition known as thrombocytopenic purpura
- Toxins from muscle tissue disintegration ( rhabdomyolysis ) induce acute kidney injury
- Tumor lysis syndrome – occurs when tumor cells are broken down, resulting in the release of toxins that can damage the kidneys
- Infection from viruses
- Certain medications such as dyes used during imaging examinations, chemotherapy drugs, and some antibiotics
- Toxic substances similar as alcohol , heavy metals, and illegal drugs
3. Urine Obstruction. Conditions or disorders that obstruct the flow of urine out of the body can cause acute kidney injury in some individuals. The following circumstances can result in a blockage:
- Cancer of the prostate, cervix, or bladder
- Enlargement of the prostate or Benign prostatic hyperplasia (BPH) in men
- Nerve damage affecting bladder control and urination
- Stones such as renal calculi, nephrolithiasis or urolithiasis
- Bleeding from the urinary tract causing blood clots
Risk Factors of Acute Kidney Injury
Acute kidney injury typically often develops as a result of another medical illness or occurrence. The following are among the conditions that can increase the risks of getting acute kidney injury:
- Hospital confinement, especially for a serious medical condition that necessitates critical care
- Peripheral artery disease
- Diabetes Mellitus
- Elevated blood pressure
- Heart failure
- Other kidney problems
- Liver failure or other liver diseases
- Cancers and chemotherapies
Treatment for Acute Kidney Injury
Acute kidney injury frequently necessitates hospitalization for treatment, though the majority of individuals who have them have already been admitted to a hospital for various reasons. The length of stay in the hospital is determined by the cause of the acute kidney injury and the rate at which the kidneys recover.
The primary goal of the healthcare practitioner is to find out what is causing the acute kidney injury so that it can be treated. Until the kidneys recover, the healthcare provider will attempt to address all the symptoms and complications.
Dialysis may also be required in more critical situations to assist in the replacement of renal function until the kidneys are fully recovered.
After an acute kidney injury, there is an increased risk of developing additional health problems such as other kidney disease, stroke , or heart disease in the future. Recurrence of acute kidney injury is also possible. Every time an acute kidney injury occurs, the chances of developing kidney disease and kidney failure also increase.
It is vital to regularly get in touch with the physician to keep track of kidney function. Preventing acute kidney injury or finding and treating it as soon as feasible is the best method to reduce the risk of kidney damage and preserve renal function.
Prevention of Acute Kidney Injury
It can be difficult to anticipate or avoid acute kidney injury, there are a few ways to help kidneys stay in good condition:
- Read the labels carefully when taking over-the-counter (OTC) painkillers. To avoid acute kidney injury, strictly follow the directions for OTC painkillers, as taking too much can cause kidney damage. If an individual has a history of kidney disease, diabetes, or high blood pressure, this is even more essential.
- Manage kidney and other chronic illnesses with the support of a healthcare provider. Stay on track with the treatment plans and follow the physician’s instructions to manage health for kidney disease or another condition, such as diabetes or high blood pressure, that raises the risk of acute kidney injury.
- Take the action to live a healthy life. Be active, eat a well-balanced and healthy diet, and only drink alcohol in moderation as much as possible.
Acute Kidney Injury Nursing Diagnosis
Nursing care plan for acute kidney injury 1.
Fluid Volume Exc ess
Nursing Diagnosis: Fluid Volume Excess related to impaired regulatory mechanism of the kidneys secondary to acute kidney injury as evidenced by generalized edema, decreased urine output with low urine specific gravity, distended neck veins, elevated blood pressure, sudden weight gain, congested lungs in x-ray, electrolytes imbalance, and decreased hematocrit level.
Desired Outcome:
The patient will have adequate urine output, acceptable laboratory tests results, steady weight, normal range of vital signs, and absence of edema.
Nursing Care Plan for Acute Kidney Injury 2
Risk for Decreased Cardiac Output
Nursing Diagnosis: Risk for Decreased Cardiac Output related to fluid overload and electrolyte imbalance secondary to acute kidney injury
Desired Outcome: The patient will maintain cardiac output as evidenced by an acceptable range of blood pressure and heart rate, firm peripheral pulses, and good capillary refill time.
Nursing Care Plan for Acute Kidney Injury 3
Risk for Imbalanced Nutrition
Nursing Diagnosis: Risk for Imbalanced Nutrition: Less Than Body Requirements related to dietary restrictions to reduce nitrogenous waste products, increased metabolic needs, and nausea/vomiting secondary to acute kidney injury
Desired Outcome: The patient will maintain acceptable weight.
Nursing Care Plan for Acute Kidney Injury 4
Risk for Infection
Nursing Diagnosis: Risk for Infection secondary to weakened immune system, invasive interventions such as urinary catheterization, and changes in nutritional consumption secondary to acute kidney injury
Desired Outcome: The patient will not manifest any signs and symptoms of infection.
Nursing Care Plan for Acute Kidney Injury 5
Risk for Deficient Fluid Volume
Nursing Diagnosis: Risk for Deficient Fluid Volume related to excessive loss of fluid secondary to acute kidney injury
Desired Outcome: The patient will demonstrate good skin turgor, moist mucosal membranes, acceptable input and output balance, palpable peripheral pulses, electrolytes within normal range, and steady weight and vital signs.
Nursing References
Ackley, B. J., Ladwig, G. B., Makic, M. B., Martinez-Kratz, M. R., & Zanotti, M. (2020). Nursing diagnoses handbook: An evidence-based guide to planning care . St. Louis, MO: Elsevier. Buy on Amazon
Gulanick, M., & Myers, J. L. (2017). Nursing care plans: Diagnoses, interventions, & outcomes . St. Louis, MO: Elsevier. Buy on Amazon
Ignatavicius, D. D., Workman, M. L., Rebar, C. R., & Heimgartner, N. M. (2018). Medical-surgical nursing: Concepts for interprofessional collaborative care . St. Louis, MO: Elsevier. Buy on Amazon
Silvestri, L. A. (2020). Saunders comprehensive review for the NCLEX-RN examination . St. Louis, MO: Elsevier. Buy on Amazon
Disclaimer:
Please follow your facilities guidelines, policies, and procedures.
The medical information on this site is provided as an information resource only and is not to be used or relied on for any diagnostic or treatment purposes.
This information is intended to be nursing education and should not be used as a substitute for professional diagnosis and treatment.

Anna Curran. RN, BSN, PHN
Leave a Comment Cancel reply
This site uses Akismet to reduce spam. Learn how your comment data is processed .

Comprehensive case study: acute kidney injury
Affiliation.
- 1 Henrico Doctors' Hospital, Richmond, VA, USA.
- PMID: 24600939
Publication types
- Case Reports
- Acute Kidney Injury / complications
- Acute Kidney Injury / diagnosis
- Acute Kidney Injury / nursing*
- Acute Kidney Injury / physiopathology
- Diabetic Angiopathies* / complications
- Diabetic Angiopathies* / diagnosis
- Diabetic Angiopathies* / nursing
- Diabetic Angiopathies* / physiopathology
- Middle Aged
- Patient Education as Topic
- Renal Dialysis
- Risk Reduction Behavior
- Vital Signs
8 Acute Renal Failure Nursing Care Plans
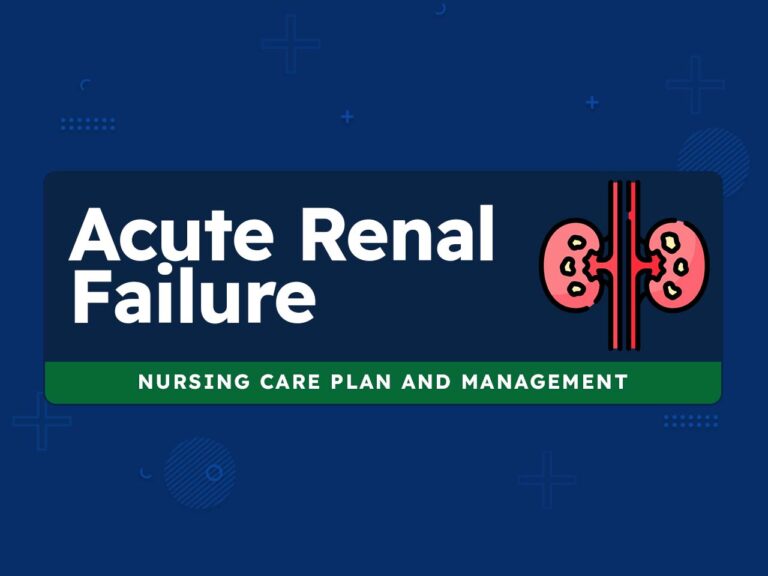
Use this nursing care plan and management guide to help care for patients with acute renal failure. Learn about the nursing assessment , nursing interventions , goals and nursing diagnosis for acute renal failure in this guide.
Table of Contents
What is acute renal failure, nursing problem priorities, nursing assessment, nursing diagnosis, nursing goals, 1. managing fluid volume and hypervolemia, 2. minimizing risk of cardiac complications, 3. promoting optimal nutritional balance, 4. promoting infection control and minimizing risk for infection, 5. minimizing risk for hypovolemia, 6. initiating health teachings and patient education, 7. administering medications and pharmacologic support, 8. monitoring laboratory and diagnostic procedures, recommended resources, references and sources.
Acute renal failure (ARF), also known as acute kidney failure or acute kidney injury (AKI) , is the abrupt loss of kidney function. The glomerular filtration rate (GFR) falls over a period of hours to a few days and is accompanied by a concomitant rise in serum creatinine and urea nitrogen. However, immediately after a kidney insult, BUN or serum creatinine levels may be normal. In the early phase, the only sign of a kidney injury may be decreased urine production (Workeneh & Batuman, 2022). If left untreated, acute renal failure may complicate chronic renal failure .
Traditionally, AKI may be classified into three general categories. Prerenal which occurs as an adaptive response to severe volume depletion and hypotension , with structurally intact nephrons. Intrinsic that occurs in response to cytotoxic, ischemic, or inflammatory insults to the kidney, with structural and functional damage. Lastly, Postrenal occurs from obstruction to the passage of urine.
The annual incidence of acute renal failure is 100 cases for every million people in the United States. It is diagnosed in 1% of hospital admissions. AKI develops within 30 days postoperatively in approximately 1% of general surgery cases and arises in more than 50% of intensive care unit clients (Workeneh & Batuman, 2022).
Most clients with AKI have no clinical symptoms related to AKI and are diagnosed on the basis of a routine laboratory blood test. Depending on the degree of kidney function impairment and duration, however, they might have hypertension , edema, decreased urine output, shortness of breath, anorexia , nausea , sleep disturbances, and altered mental status (Workeneh & Batuman, 2022).
The prognosis for clients with AKI is directly related to the cause of the injury and, to a great extent, to the presence or absence of preexisting kidney disease, as well as to the duration of kidney dysfunction prior to therapeutic intervention (Workeneh & Batuman, 2022).
Nursing Care Plans and Management
The nursing care plan and management for clients with acute renal failure are to promote renal function, correct or eliminate any reversible causes of kidney failure, and provide supportive care. Specific interventions include monitoring and managing fluid and electrolyte imbalances , optimizing nutrition , and ensuring medication safety . Nurses also work to support the patient’s emotional well-being and provide education on self-care and prevention of future kidney damage.
The following are the nursing priorities for patients with acute renal failure (ARF):
- Assessment and monitoring of renal function
- Fluid and electrolyte balance management
- Identification and treatment of the underlying cause
- Prevention and management of complications (e.g., electrolyte imbalances, metabolic acidosis)
- Monitoring and management of fluid overload or dehydration
- Hemodynamic stability and blood pressure control
- Education on self-care and compliance with treatment plans
Assess for the following subjective and objective data :
- Decreased urine output or oliguria (reduced urine volume)
- Swelling or edema in the hands, feet, or face
- Fatigue and weakness
- Shortness of breath or difficulty breathing
- Nausea, vomiting , or loss of appetite
- Confusion or altered mental status
- Abdominal pain or discomfort
- High blood pressure
- Irregular heart rhythm or palpitations
- Signs of fluid overload
Assess for factors related to the cause of acute renal fa ilure (ARF) :
- Compromised regulatory mechanism (renal failure)
- Fluid overload (kidney dysfunction/failure, overzealous fluid replacement)
- Fluid shifts, fluid deficit (excessive losses)
- Electrolyte imbalance ( potassium , calcium ); severe acidosis
- Uremic effects on cardiac muscle /oxygenation
- Protein catabolism; dietary restrictions to reduce nitrogenous waste products
- Increased metabolic needs
- Anorexia, nausea/ vomiting ; ulcerations of oral mucosa
- Depression of immunologic defenses (secondary to uremia)
- Invasive procedures/devices (e.g., urinary catheterization )
- Changes in dietary intake/ malnutrition
- Excessive loss of fluid ( diuretic phase of ARF, with rising urinary volume and delayed return of tubular reabsorption capabilities)
Following a thorough assessment , a nursing diagnosis is formulated to specifically address the challenges associated with acute renal failure based on the nurse ’s clinical judgement and understanding of the patient’s unique health condition. While nursing diagnoses serve as a framework for organizing care, their usefulness may vary in different clinical situations. In real-life clinical settings, it is important to note that the use of specific nursing diagnostic labels may not be as prominent or commonly utilized as other components of the care plan. It is ultimately the nurse’s clinical expertise and judgment that shape the care plan to meet the unique needs of each patient, prioritizing their health concerns and priorities.
Goals and expected outcomes may include:
- The client will display appropriate urinary output with specific gravity/laboratory studies near normal; stable weight, vital signs within the client’s normal range; and absence of edema.
- The client will maintain cardiac output as evidenced by BP and HR/rhythm within the client’s normal limits; peripheral pulses are strong and equal with adequate capillary refill time.
- The client will maintain/regain the weight as indicated by the individual situation, free of edema.
- The client will experience no signs/symptoms of infection .
- The client will display I&O near balance; good skin turgor , moist mucous membranes, palpable peripheral pulses, stable weight, vital signs, and electrolytes within normal range.
Nursing Interventions and Actions
Therapeutic interventions and nursing actions for patients with acute renal failure (ARF) may include:
In the case of prerenal acute kidney injury (AKI), fluid resuscitation is the gold standard, but if this resuscitation continues beyond the correction of hypovolemia , then it is associated with increased morbidity, mortality, and length of hospital stay as well as increased risk of AKI. Several observational studies have demonstrated a correlation between fluid overload and mortality in critically ill clients with acute respiratory distress syndrome , acute lung injury, sepsis , and AKI (Patil & Salunke, 2020).
Assess the level of consciousness. Investigate changes in mentation and the presence of restlessness. This may reflect fluid shifts, accumulation of toxins, acidosis, electrolyte imbalances, or developing hypoxia. Older adults with vague mental status change are commonly found to have prerenal or normotensive ischemic AKI. Insensible fluid losses can result in severe hypovolemia in clients with restricted fluid access and should be suspected in older adult clients and in comatose or sedated clients (Workeneh & Batuman, 2022).
Assess skin, face, and dependent areas for edema. Evaluate the degree of edema (on a scale of +1–+4). Edema occurs primarily in dependent tissues of the body, (hands, feet, lumbosacral area). The client can gain up to 10 lb (4.5 kg) of fluid before pitting edema is detected. Periorbital edema may be a presenting sign of this fluid shift because these fragile tissues are easily distended by even minimal fluid accumulation.
Monitor heart rate (HR), BP, and JVD/CVP. Tachycardia and hypertension can occur because of: (1) failure of the kidneys to excrete urine, (2) excess fluid resuscitation during efforts to treat hypovolemia and/or hypotension or convert oliguric phase of renal failure, (3) changes in the renin-angiotensin system. Invasive monitoring may be needed for assessing intravascular volume, especially in clients with poor cardiac function. Although clients with heart failure may have low blood pressure , volume expansion is present, and effective renal perfusion is poor, which can result in AKI (Workeneh & Batuman, 2022).
Auscultate lung and heart sounds. Fluid overload may lead to pulmonary edema and heart failure evidenced by the development of adventitious breath sounds, and extra heart sounds. The most important part of physical examination is the assessment of cardiovascular status and volume status. The cardiovascular examination may reveal murmurs, pericardial friction rub, and increased jugulovenous distention, rales, and S3 gallops (Workeneh & Batuman, 2022).
Accurately record intake and output (I&O) noting to include “hidden” fluids such as IV antibiotic additives, liquid medications, frozen treats, and ice chips. Religiously measure gastrointestinal losses and estimate insensible losses ( sweating ), including wound drainage, nasogastric outputs, and diarrhea . The widely accepted Kidney Disease: Improving Global Outcome (KDIGO) definition of AKI is based on the change of serum creatinine and urine output of < 0.5 mL/kg/hour for six hours. Accurate monitoring of I&O is necessary for determining renal function and fluid replacement needs and reducing the risk of fluid overload. Oliguria generally favors AKI. Abrupt anuria suggests acute urinary obstruction, acute severe glomerulonephritis , or embolic renal artery occlusion. A gradually diminishing urine output may indicate a urethral stricture or bladder outlet obstruction (Workeneh & Batuman, 2022).
Monitor urine-specific gravity. This measures the kidney’s ability to concentrate urine. In intrarenal failure, specific gravity is usually equal to or less than 1.010, indicating loss of ability to concentrate the urine. Aggressive fluid resuscitation is appropriate in prerenal AKI. However, overly aggressive volume resuscitation in a client with ATN who is unable to excrete the extra fluid can result in volume overload and respiratory embarrassment. To help with the differentiation of prerenal azotemia, analysis of urine may provide important clues (Sinert & Sugalski, 2019).
Weigh daily at the same time of day, on the same scale, with the same equipment and clothing. Daily body weight is the best monitor of fluid status. A weight gain of more than 0.5 kg/day suggests fluid retention. Over-aggressive volume resuscitation can cause tissue congestion and hypoxia and is associated with worse survival in general ICU populations and in clients with AKI (Fülöp et al., 2010).
Monitor diagnostic studies (Hgb, hct, sodium, potassium, bun, crea and urine sodium). See Laboratory and Diagnostic Procedures
Monitor Serial chest x-ray s. See Laboratory and Diagnostic Procedures
Monitor echocardiography results as indicated. See Laboratory and Diagnostic Procedure s
Scatter desired beverages throughout the 24-hour period and give various offerings (hot, cold, frozen). This helps avoid periods without fluids, minimizes boredom of limited choices, and reduces the sense of deprivation and thirst. Drinking black coffee, instead of coffee with high potassium and high phosphorus milk, or high-calorie sugar drinks is best. A cup of unsweetened green tea is full of compounds called “polyphenols”, which function as antioxidants. Carbonated, or sparkling, water hydrates, as well as water, does (Shusterman, 2021).
Maintain volume homeostasis and correct biochemical abnormalities. Kidneys may be able to return to normal functioning, preventing or limiting residual effects. Measures include correction of fluid overload, correction of severe acidosis, and correction of hematologic abnormalities such as transfusions and administration of medications (Workeneh & Batuman, 2022).
Use appropriate safety measures (raising side rails and restraints . Clients with CNS involvement may be dizzy and/or confused. The kidney and the brain are frequently injured during critical illness. Acute kidney injury, which affects up to half of the critically ill clients, is strongly associated with short and long-term morbidity and mortality. Similarly, acute brain dysfunction, which manifests as delirium or coma in the ICU, is common during critical illness and associated with adverse short and long-term outcomes (Siew et al., 2017).
Administer and/or restrict fluids as indicated. Fluid management is usually calculated to replace output from all sources plus estimated insensible losses (metabolism, diaphoresis). Prerenal failure (azotemia) is treated with volume expansion and/or vasopressors. The oliguric client with adequate circulating volume or fluid overload who is unresponsive to fluid restriction and diuretics requires dialysis. During the oliguric phase, “push/pull” therapy (push IV fluids and diuresis with diuretics ) may be tried to stimulate kidney function. However, a rapid fluid infusion can result in life-threatening fluid overload in clients with AKI (Sinert & Sugalski, 2019).
Assist the client in performing the passive leg-raising maneuver (PLR) or virtual fluid challenge as indicated. PLR is a test that predicts whether cardiac output will increase with volume expansion. By transferring a volume of around 300 mL of venous blood from the lower body toward the right heart, PLR mimics a fluid challenge (Effat et al., 2021).
Promote sodium and fluid restriction as indicated. Restriction of salt and fluid becomes crucial in the management of oliguric kidney failure, wherein the kidneys do not adequately excrete either toxins or fluids (Workeneh & Batuman, 2022). Oliguric clients should also have a fluid restriction of 400 ml plus the previous day’s urine output unless there are signs of volume depletion (Bindroo et al., 2022).
Insert indwelling catheter, as indicated. Catheterization excludes lower tract obstruction and provides means of accurate monitoring of urine output during the acute phase; however, indwelling catheterization may be contraindicated because of the increased risk of infection. Placement of a urinary catheter in AKI not only allows diagnosis and treatment of urethral and bladder outlet urinary obstruction but also allows for accurate measurement of urine output (Sinert & Sugalski, 2019).
Administer furosemide , vasodilators, and calcium channel blockers as indicated. See Pharmacologic Management
Prepare for dialysis as indicated: hemodialysis, peritoneal dialysis , or continuous renal replacement therapy (CRRT). Dialysis is done to correct volume overload, electrolyte, and acid-base imbalances, and to remove toxins. The type of dialysis chosen for ARF depends on the degree of hemodynamic compromise and the client’s ability to withstand the procedure. However, dialysis, especially hemodialysis, may delay the recovery of clients with AKI. Most authorities prefer using biocompatible membrane dialyzers for hemodialysis. Peritoneal dialysis is not frequently used in AKI. Nevertheless, it can technically be used in acute cases and probably is tolerated better hemodynamically than conventional hemodialysis (Workeneh & Batuman, 2022).
Assist in renal replacement therapy. The principal methods of renal replacement therapy (RRT) are intermittent hemodialysis, continuous venovenous hemodiafiltration, and peritoneal dialysis. Continuous RRT techniques are more expensive, associated with increased bleeding risk, and not universally available. However, in addition to avoiding hypotension, they are believed to achieve better control of uremia and clearance of solute from the extravascular compartment (Sinert & Sugalski, 2019).
In AKI, the kidneys are unable to remove excess fluid from the body, leading to fluid overload. This can increase the volume of blood that the heart has to pump leading to an increase in the workload of the heart and decreased cardiac output . AKI can also lead to electrolyte imbalances that can affect the heart’s electrical activity and reduce its ability to contract effectively.
Monitor BP and HR. Fluid volume excess, combined with hypertension (common in renal failure) and the effects of uremia, increases cardiac workload and can lead to cardiac failure. In ARF, cardiac failure is usually reversible. Vital signs can be considered indicators of kidney damage and therefore need to receive more attention from healthcare professionals. It was observed that temperature and breathing cycle increase as kidney damage increases, while blood pressure and pulse also undergo significant changes due to kidney damage (Sacomboio et al., 2021).
Observe E CG or telemetry for changes in rhythm. Changes in electromechanical function may become evident in response to progressing renal failure and accumulation of toxins and electrolyte imbalance . Peaked T wave, wide QRS, and prolonged PR interval are usually associated with hyperkalemia . The flat T wave, peaked P wave, and appearance of the U waves usually indicate hypokalemia . Prolonged QT interval may reflect calcium deficit .
Auscultate heart sounds. Development of S 3 /S 4 is indicative of failure. Pericardial friction rub may be the only manifestation of uremic pericarditis, requiring prompt intervention and possibly acute dialysis (Workeneh & Batuman, 2022).
Assess the color of skin, mucous membranes, and nail beds. Note capillary refill time. Pallor may reflect vasoconstriction or anemia . Cyanosis is a late sign and is related to pulmonary congestion and/or cardiac failure. AKI can cause a decrease in the production of RBCs, leading to anemia . Anemia can cause pallor, which is the paleness of the skin due to a decreased amount of oxygenated blood reaching the skin’s surface. AKI can also cause a build-up of fluid in the lungs , leading to respiratory distress and decreased oxygenation of the blood. This causes cyanosis .
Note the occurrence of slow pulse, hypotension, flushing, nausea and vomiting , and depressed level of consciousness. The use of drugs (like antacids) containing magnesium can result in hypermagnesemia , potentiating neuromuscular dysfunction, and risk of respiratory or cardiac arrest. Use aluminum-hydroxide-based antacid. Hypovolemia leads to hypotension; however, hypotension may not necessarily indicate hypovolemia (Workeneh & Batuman, 2022).
Monitor for GI bleeding by guaiac testing all stools for blood. See Laboratory and Diagnostic Procedure
Investigate reports of muscle cramps, numbness of fingers, muscle twitching, and hyperreflexia. Neuromuscular indicators of hypocalcemia can also affect cardiac contractility and function. Hypocalcemia causes muscle spasms and cramps, especially in the hands, feet, and face. This occurs because calcium is necessary for proper muscle function. Severe hypocalcemia can cause tetany, a condition in which the muscles in the hands and feet contract involuntarily, causing muscle spasms and twitching.
Monitor laboratory studies (potassium, calcium, and magnessium). See Laboratory and Diagnostic Procedure
Maintain bed rest or encourage adequate rest and provide assistance with care and desired activities. This reduces oxygen consumption and cardiac workload. During rest, the heart rate decreases, which reduces the workload on the heart and allows it to pump more efficiently. This can improve cardiac output and increase the amount of blood that is circulated throughout the body.
Encourage the client to perform relaxation techniques and improve sleep quality. Relaxation techniques reduce stress and lower the levels o stress hormones. These hormones can increase the heart rate and blood pressure, which decreases cardiac output. Improving sleep quality allows the body to repair and regenerate cells and tissues. This improves the overall health of the heart and improves cardiac output.
Administer and/or restrict fluids as indicated. Cardiac output depends on circulating volume (affected by both fluid excess and deficit) and myocardial muscle function. Clients with AKI represent challenging fluid management problems. Hypovolemia potentiates and exacerbates all forms of AKI. Reversal of hypovolemia by rapid fluid infusion is often sufficient to treat many forms of AKI. However, a rapid fluid infusion can result in life-threatening fluid overload (Sinert & Sugalski, 2019).
Provide supplemental oxygen if indicated. This maximizes available oxygen for myocardial uptake to reduce cardiac workload and cellular hypoxia. The heart requires a constant supply of oxygen to function properly. Supplemental oxygen allows the heart to function more efficiently and effectively.
Administer medications (inotropic agent, calcium gluconate, aluminum hydroxide gels, insulin , sodium bicarbonate , and sodium polystyrene sulfonate with or without sorbitol as indicated. See Pharmacologic Management
Prepare for/assist with dialysis as necessary. This may be indicated for persistent dysrhythmias, and progressive heart failure unresponsive to other therapies. The current recommendation by KDIGO is dialysis-dependent AKI clients is to deliver a kt/v of 3.9 per week when using intermittent or extended RRT and an effluent volume of 20 to 25 mg/kg/hour when using continuous renal replacement therapy (Workeneh & Batuman, 2022).
Prepare the client for echocardiography. Echocardiography is an essential tool for guiding resuscitation in critically ill clients. It is an evidence-based approach and ideally suited to address fluid responsiveness. Resuscitation often requires the infusion of IV fluids in an effort to reverse organ dysfunction (Effat et al., 2021).
Malnutrition is highly prevalent among AKI clients and is an important independent predictor of in-hospital mortality. Nutrient requirements for clients with AKI may differ widely between individual clients and nutrition support must be coordinated with the metabolic consequences associated with renal failure, the underlying disease process, as well as the derangements in nutrient balance induced by renal replacement therapy (RRT) (Hung et al., 2022).
Assess and document dietary intake. This aids in identifying deficiencies and dietary needs. General physical condition, uremic symptoms (nausea, anorexia), and multiple dietary restrictions affect food intake. Critically ill clients with AKI are often suffering from multiple organ failures that complicate nutritional support. The nutritional status of critically ill clients affects plans for nutritional support (Hung et al., 2022).
Weigh daily. The fasting or catabolic client normally loses 0.2 to 0.5 kg/day. Changes in excess of 0.5 kg may reflect shifts in fluid balance . Studies have shown that obesity was associated with an increased risk of developing AKI. Paradoxically, obese clients seem to have a survival benefit compared to underweight or normal-weight clients regardless of whether or not they required RRT. Clients with a BMI of ≥31 kg/m² had higher mortality, which was the same as those clients with a low body weight (BMI <18 kg/m²) (Hung et al., 2022).
Measure the client’s energy requirements. The energy requirements of clients with AKI are determined by the underlying disease and not by renal failure. Studies and clinical guidelines suggest that indirect calorimetry can be used to measure indirect energy requirements when available (Hung et al., 2022). Indirect calorimetry calculates heat production by measuring gas exchange . During resting conditions, food sources are broken down to produce energy (heat or kcal). The oxygen consumed and the carbon dioxide produced are measured to provide an indirect assessment of calorie requirement at rest per day (The Medical City, 2017).
Perform a general nutritional assessment and screen for malnutrition. Nutrition-related symptoms have been shown to have an important role in predicting malnutrition risk in kidney clients, and among those, appetite loss conveyed the highest prognostic power. Recently, a new renal inpatient nutritional screening tool (Renal iNUT) was specifically developed for hospitalized clients with AKI or CKD, showing good sensitivity, specificity, and positive predictive value. A general nutritional assessment should include client history, report of unintentional weight loss or decrease in physical performance before hospital or ICU admission, physical examination, and general assessment of body composition, muscle mass, and strength (Fiaccadori et al., 2021).
Assess lean body mass, muscle mass, and function. Body composition assessment should be preferred to anthropometry measurements when diagnosing and monitoring malnutrition in hospitalized clients with AKI. Because critically ill clients suffer an important and accelerated skeletal muscle loss already occurring in the first few days at the ICU, body composition monitoring is of major importance. Muscle function should be assessed by hand-grip strength (Fiaccadori et al., 2021).
Monitor laboratory studies such as BUN, albumin, transferrin, sodium, potassium, and phosphorus. These are indicators of nutritional needs, restrictions, and the necessity for and effectiveness of therapy. Renal function is critical to maintaining internal electrolyte balance. Severe electrolyte and acid-base disorders are considered important indicators for renal replacement treatment and cause of death . One national report in the UK stated that one-fifth of in-hospital AKI could be avoidable, if only they received better monitoring of electrolytes, recognition of risk factors, and prompt management (Chen et al., 2021).
Provide frequent, small feedings. This minimizes anorexia and nausea associated with a uremic state and/or diminished peristalsis . Two clinical trials compared trophic feeding with full-feeding nutrition for the first six days in acute lung injury clients on ventilator support. The trophic feeding group had less gastrointestinal intolerance and insulin use and lower plasma glucose levels. The definition of trophic feeding is to give enteral nutrition at 10 to 20 kcal/hour or up to 500 kcal/day. For critically ill clients with AKI, begin feeding with a low-energy diet or full-energy diet if the effect of mortality is still controversial. However, initial trophic feeding can reduce episodes of GI intolerance (Hung et al., 2022).
Give the client or family caregiver a list of permitted foods or fluids and encourage involvement in menu choices. This provides the client with a measure of control within dietary restrictions. Food from home may enhance appetite. Dietary changes are an important facet of AKI treatment. Restriction of salt and fluid becomes crucial in the management of oliguric kidney failure, wherein the kidneys do not adequately excrete either toxins or fluids (Workeneh & Batuman, 2022).
Offer frequent mouth care or rinse with 10% hydrogen peroxide if there is stomatitis. Give gums, hard candy, and breath mints between meals. Xerostomia denotes the subjective sensation of dry mouth and is related to the overall volume status of clients who are discouraged from drinking excess fluids. One-third of hemodialyzed clients present a characteristic halitosis called “uremic fetor” and a metallic taste due to high urea content in saliva and its breakdown in ammonia. An important problem is also represented by uremic stomatitis, which consists of painful lesions on oral mucosal surfaces. To promote the healing of the lesions, gargling with 10% hydrogen peroxide four times a day can be recommended (Costantinides, 2018).
Consult with the dietitian support team. This determines individual calorie and nutrient needs within the restrictions and identifies the most effective route and product (oral supplements, enteral or parenteral nutrition ). The client should have a dietary consult because salt and fluid restriction is vital when managing acute kidney injury. Similarly, the client should avoid a high-potassium diet when there is renal dysfunction (Goyal et al., 2022).
Include complex carbohydrates and fat sources to meet caloric needs and essential amino acids. Avoid concentrated sugar sources. Carbohydrates meet energy needs and limit tissue catabolism, preventing keto acid formation from protein and fat oxidation. Carbohydrate intolerance mimicking DM may occur in severe renal failure. Essential amino acids improve nitrogen balance and nutritional status, stimulate the repair of tubular epithelial cells, and enhance the client’s ability to fight systemic complications. Almost all of the standard enteral nutrition and parenteral nutrition formulas available today contain a high percentage of calories from carbohydrates, even in lipid-based all-in-one formulas. This non-protein macronutrient distribution may not be appropriate for hospitalized clients with kidney failure (Fiaccadori et al., 2021).
Provide a high-calorie, low to moderate-protein diet. The protein requirement of clients with AKI is determined by the underlying disease causing the AKI, the extent of catabolism, and the type of treatment. Since hypoalbuminemia is associated with effective hypovolemia and increases mortality in these clients, nutritional management should aim at supplying sufficient protein to maintain the serum albumin concentration and immune function. Nutritional protein administration should not be restricted to attenuating the rise in blood urea nitrogen or delaying the initiation of dialysis. The protein intake should be increased when clients undergo RRT (Hung et al., 2022).
Maintain proper electrolyte balance by strictly monitoring levels. Medications and a decrease in GFR can cause electrolyte imbalances and may further cause renal injury. Urine electrolyte findings can also serve as valuable indicators of functioning renal tubules. The fractional excretion of sodium (FENa) is the commonly used indicator. FENa can be a valuable test for helping to detect extreme renal avidity for sodium in conditions such as hepatorenal syndrome (Workeneh & Batuman, 2022).
Restrict potassium, sodium, and phosphorus intake as indicated. Restriction of these electrolytes may be needed to prevent further renal damage, especially if dialysis is not part of treatment, and during the recovery phase of ARF. Because potassium and phosphorus are not excreted optimally in clients with AKI, blood levels of these electrolytes tend to be high. Restriction of these elements in the diet may be necessary, with guidance from frequent measurements (Workeneh & Batuman, 2022).
Administer medications(iron preparations, calcium carbonate, selenium, vitamin C, and folic acid as indicated. See Pharmacologic Management
Provide enteral/parenteral nutrition as indicated. According to 2019 guidelines of the European Society for Clinical Nutrition and Metabolism (ESPEN) and 2016 guidelines of the ASPEN for critically ill clients, those who are at high nutritional risk should be started on enteral or parenteral nutrition within 24 to 48 hours of ICU admission. AKI clients at high nutritional risk who received early enteral nutrition had a significantly lower mortality rate compared to those who received late enteral nutrition. Parenteral nutrition should be given if, after 7 to 10 days, enteral nutrition is unable to meet 60% of energy requirements (Hung et al., 2022).
Maintain serum glucose levels between 140 t0 180 mg/dL. Clients are at increased risk of both hyper- and hypoglycemia . Insulin resistance is highly prevalent among clients with AKI and is associated with increased mortality risk. High blood glucose concentration can be considered one of the best independent predictors of mortality in this setting. Since insulin is metabolized by the kidney, renal function impairment may also act as a predisposing factor for hypoglycemia (Fiaccadori et al., 2021).
AKI also adversely affects immune function and is widely considered an immunosuppressed state. A wealth of data has accumulated that clients with AKI have a substantially increased risk of subsequent infection and sepsis. Based on early data and the fact that sepsis is the leading cause of death in clients with AKI, it is possible that the systemic inflammatory response syndrome (SIRS) and counter anti-inflammatory response syndrome responses in AKI result in a state of persistent or prolonged immunoparalysis that increases the risk for subsequent sepsis (Gist & Faubel, 2020).
Assess skin integrity . Excoriations from scratching may become secondarily infected. Livedo reticularis, digital ischemia , butterfly rash , and purpuras may suggest vasculitis . Some clients may have track marks to suggest endocarditis in an IV drug abuser (Workeneh & Batuman, 2022). Pruritus associated with calcium phosphate deposition and nail atrophy is common in clients with uremia (Alper, 2022).
Monitor vital signs. Fever (higher than 100.4°F) with increased pulse and respirations is typical of an increased metabolic rate resulting from an inflammatory process, although sepsis can occur without a febrile response. Clients with a worse prognosis of kidney disease presented febrile on admission. Temperature values dropped significantly after four days of hospitalization, indicating that temperature can be a good initial indicator of renal complications (Sacomboio et al., 2021).
Monitor WBC count with differential. See Laboratory and Diagnostic Procedure
Obtain specimen(s) for culture and sensitivity and administer appropriate antibiotics as indicated. See Laboratory and Diagnostic Procedure
Promote good hand washing by the client and staff. This reduces the risk of cross-contamination. One of the best preventive measures for protecting ourselves from the risk of getting infected is washing our hands properly and efficiently. Medical personnel that comes in direct contact with the clients have a high chance of spreading viruses and nosocomial infections; therefore, it is of utmost necessity that they know proper hand-washing technique and that they wash their hands regularly following standard hand-washing guidelines (Khadka & Dani, 2020).
Avoid invasive procedures, instrumentation, and manipulation of indwelling catheters whenever possible. Use an aseptic technique when caring for and manipulating IV and invasive lines. Change site dressings per protocol. Note edema, and purulent drainage. This limits the introduction of bacteria into the body. Early detection of developing infection may prevent sepsis. Specific risk factors for the development of infection post-AKI include at least 3 days of oliguria, a higher percentage of daily fluid overload, invasive procedures post-AKI, higher severity of illness score, and dialysis requirement (Gist & Faubel, 2020).
Provide routine catheter care and promote meticulous perineal care . Keep the urinary drainage system closed and remove the indwelling catheter as soon as possible. This reduces bacterial colonization and the risk of ascending UTIs. Placement of a urinary catheter may allow diagnosis and treatment of urethral and bladder outlet urinary obstruction and accurate measurement of urine output, but routine use must be tempered by consideration of the inherent risks of catheter-associated infections (Sinert & Sugalski, 2019).
Encourage deep breathing , coughing , and frequent position changes. This prevents atelectasis and mobilizes secretions to reduce the risk of pulmonary infections. Deep breathing followed by a forceful cough can help clear the lungs of any trapped mucus or particles that may be harboring infection. This can prevent the build-up of harmful bacteria or viruses in the lungs, reducing the risk of infection.
Administer intravenous fluids as indicated. Septic AKI clients tend to have lower urinary outputs, receive more fluid therapy and/or diuretics, and are more likely to develop fluid restriction than non-septic AKI clients. Crystalloids are the fluid of choice for resuscitation and hydroxyethyl starches (HES) or hyperchloremic solutions are not recommended (Godin et al., 2017).
Administer antimicrobial therapy as prescribed. See Pharmacologic Management
Administer vasopressors as prescribed. See Pharmacologic Management
Avoid nephrotoxic medications. Another key element in the management of septic clients in regard to AKI prevention is the avoidance of potentially nephrotoxic medication and contrast agents when possible, especially in high-risk clients (Godin et al., 2017).
Provide adequate nutritional support. Nutritional support is an important but often overlooked aspect of global client care. It is especially important in septic AKI, a hypercatabolic state that requires adapted protein and caloric intake. Numerous aspects need to be considered when calculating a septic AKI client’s nutritional requirements such as their baseline characteristics, underlying conditions, volume status, and possible protein loss through RRT. For this reason, each client requires an individual approach to nutritional support (Godin et al., 2017).
During the diuretic phase, daily urine output is approximately 1 to 3 liters but can reach as high as 5 liters or more. The kidneys recover their ability to excrete waste but cannot concentrate the urine. Hypovolemia and hypotension may occur due to massive volume loss. Due to the loss of large volumes of fluid and electrolytes, clients should be monitored for hyponatremia , hypokalemia, and dehydration . This phase may last 1 to 3 weeks (American Nephrology Nurses Association, 2019).
Measure I&O accurately. Weigh daily. Calculate insensible fluid losses. Assessment can help estimate fluid replacement needs. Fluid intake should approximate losses through urine, nasogastric or wound drainage, and insensible water losses (diaphoresis, metabolism). Insensible fluid losses can result in severe hypovolemia in clients with restricted fluid access and should be suspected in older adult clients and in comatose or sedated clients (Workeneh & Batuman, 2022).
Monitor BP (noting postural changes), HR, and mean arterial pressure (MAP) if possible. Orthostatic hypotension and tachycardia suggest hypovolemia. Hypotension below a particular threshold MAP was associated with stag 3 progression. Surviving Sepsis Campaign Guidelines recommend maintaining MAP ≥65 mmHg in clients with sepsis, while some earlier reports indicate that maintenance of MAP ≥70 mm Hg would prevent AKI and progression to severe stage AKI (Izawa et al., 2016).
Note signs and symptoms of dehydration : dry mucous membranes, thirst, dulled sensorium, and peripheral vasoconstriction. In the diuretic or post-obstructive phase of renal failure, urine output can exceed 3 liters/day. Extracellular fluid volume depletion activates the thirst center, and sodium depletion causes persistent thirst, unrelieved by drinking water. Continued fluid losses including inadequate replacement may lead to a hypovolemic state. Clients commonly present with symptoms related to hypovolemia, including thirst, decreased urine output, dizziness, and orthostatic hypotension. Older adults with vague mental status changes are commonly found to have prerenal or normotensive ischemic AKI (Workeneh & Batuman, 2022).
Measure and monitor urine output closely. In one study designed to determine the value of monitoring urine output in detecting AKI, researchers carefully measured output in critically ill clients; they found AKI incidence rose from 24% to 52% when urine output was added as a variable and monitored hourly. This study indicates urine output directly reflects glomerular filtration rate (GFR) in the kidney and therefore is a sensitive and early AKI biomarker (Dirkes, 2015).
Monitor laboratory studies In non-oliguric ARF or in the diuretic phase of ARF, large urine losses may result in sodium wasting while elevated urinary sodium acts osmotically to increase fluid losses. Restriction of sodium may be indicated to break the cycle. The kidneys play a role in sodium and potassium homeostasis, and their function decline leads to electrolyte disorder. Abnormal sodium levels may often be accompanied by hypokalemia or hyperkalemia (Gao et al., 2019).
Provide allowed fluids throughout the 24-hour period. The diuretic phase of ARF may revert to the oliguric phase if fluid intake is not maintained or nocturnal dehydration occurs. The longer the oliguric phase, the poorer the prognosis for the recovery of kidney function. Reversal of hypovolemia by rapid fluid infusion is often sufficient to treat many forms of AKI. However, a rapid fluid infusion can result in life-threatening fluid overload in clients with AKI (Sinert & Sugalski, 2019).
Control environmental temperature; limit bed linens as indicated. This may reduce diaphoresis, which contributes to overall fluid losses. The reduction of diaphoresis in response to a decrease in environmental temperature is due to several factors. One of the primary mechanisms is the constriction of blood vessels in the skin, which reduces the flow of blood to the sweat glands. This constriction of blood vessels is a part of the body’s natural response to cold temperatures, as it helps retain heat in the body’s core. This leads to a decrease in sweat production.
Administer intravenous fluids as indicated. Clients with AKI represent challenging fluid management problems. Hypovolemia potentiates and exacerbates all forms of AKI. Vigorous fluid administration in this setting aims to reverse renal ischemia and dilute nephrotoxins to either avert the onset of acute tubular necrosis or prevent recurrent injury that might compromise renal recovery (Effat et al., 2021).
Administer vasopressors as prescribed. In clients with vasomotor shock at risk for or with AKI, vasopressors are used along with fluids. For persistent hypotension not responsive to fluid resuscitation, vasopressors are started with the goal of a MAP of 65 or greater (American Nephrology Nurses Association, 2019). Catecholamines, vasopressin, and angiotensin II are the current therapeutic options available for use in increasing blood pressure in septic shock (Busse & Ostermann, 2019).
Insert a urinary catheter as indicated. Placement of a urinary catheter early in the workup of a client with AKI not only allows diagnosis and treatment of urethral and bladder outlet urinary obstruction but also allows for accurate measurement of urine output. Routine use of urinary catheters should be tempered by consideration of the inherent risks of catheter-associated infections (Sinert & Sugalski, 2019).
The importance of kidney care has become evident in studies showing that individuals who survive an episode of AKI are at increased risk of adverse outcomes that include both kidney and non-kidney sequelae. Unfortunately, under-recognition of the post-discharge implications of AKI may be common and compounded by fragmentation and poor coordination of care after hospital discharge (Silver & Siew, 2017).
Review disease process, prognosis, and precipitating factors if known. This provides a knowledge base from which the client can make informed choices. In low- and middle-income countries, although the majority of urban cases occur in the context of acute illness, usually in association with hypovolemia and sepsis, AKI occurring in the community is under-recognized. Hospital-acquired AKI develops in the intensive care unit, in the context of multiple organ failure, post-cardiovascular procedures, or sepsis as a complication of nephrotoxic medications (Lewington et al., 2013).
Identify symptoms requiring medical intervention: decreased urinary output, sudden weight gain, presence of edema, lethargy , bleeding, signs of infection, and altered mentation. Prompt evaluation and intervention may prevent serious complications or progression to chronic renal failure . A common factor across high-income and low- to middle-income countries is that most cases of AKI are managed by non-nephrologists, who may be unfamiliar with the risk factors and early manifestations of the disease. This contributes to delayed recognition and suboptimal management (Lewington et al., 2013).
Review dietary plans and restrictions. Include a fact sheet listing food restrictions. Adequate nutrition is necessary to promote tissue healing; adherence to restrictions may prevent complications. Because clients with AKI often have other serious diseases, nutritional support should be considered in a holistic manner. The nutritional requirements of clients with AKI are not determined by AKI per se but are related to the primary disease and any comorbidity. The severity of kidney injury affects the elimination of the daily production of metabolic wastes and nutrients lost due to dialysis treatment. Hypovolemia causing non-catabolic AKI is common in older and malnourished clients which may result from dehydration , upper gastrointestinal bleeding, congestive heart disease, and hypoalbuminemia (Hung et al., 2022).
Review the use of medication . Encourage the client to discuss all medications and herbal supplements with the healthcare provider. Medications that are concentrated in and/or excreted by the kidneys can cause toxic cumulative reactions and/or permanent damage to the kidneys. Some supplements may interact with prescribed medications and may electrolytes. Categorizations of drug-associated AKI are made based on the underlying mechanism of renal injury. Direct nephrotoxicity occurs from tubuloepithelial injury, interstitial nephritis, glomerular injury, or obstructive nephropathy whereas indirect nephrotoxicity develops from decreased renal blood flow. (Kellum & Hall, 2018)
Establish a regular schedule for weighing. A useful tool for monitoring fluid and dietary needs. Studies have shown that obesity was associated with an increased risk of developing AKI. Clients with a BMI of ≥ 31 kg/m² had higher mortality, which was the same as those clients with low body weight (BMI <18 kg/m² (Hung et al., 2022).
Explain the level of renal function after the acute episode is over. The client may experience residual defects in kidney function, which may or may not be permanent. Continued nephron loss causes more hyperfiltration until complete kidney failure results. This has been termed the hyperfiltration theory of kidney failure and explains the scenario in which progressive failure is frequently observed after apparent recovery from AKI (Workeneh & Batuman, 2022).
Encourage the client to observe the characteristics of urine and the amount, and frequency of output. Changes may reflect alterations in renal function and the need for dialysis. Findings of granular, muddy brown casts in urine sediment are highly suggestive of acute tubular necrosis (ATN). Reddish brown or cola-colored urine suggests the presence of myoglobin or hemoglobin (Workeneh & Batuman, 2022).
Provide social and emotional support to the client and family. This will reassure them of all the procedures that the client may undergo. Social support is defined as resources provided by a network of individuals and social groups. Members of one’s social network who provide support include peers, family, religious group members, professionals, etc. The members of social networks provide emotional, tangible, informational, and companionship support. Higher levels of social support can improve one’s ability to acquire and understand medical information, and to negotiate in the healthcare system, which would be particularly important for people to facilitate the establishment of healthful attitudes and behaviors (Chen et al., 2018).
Review fluid restriction. Remind the client to spread fluids over an entire day and to include all fluids (ice) in daily fluid counts. Depending on the cause and stage of ARF, the client may need to either restrict or increase the intake of fluids. Fluids should be considered drugs and should be used judiciously. Accurate assessment of intravascular fluid status, looking for fluid responsiveness, and planning individual targets should be followed in order to improve client outcomes (Patil & Salunke, 2020).
Discuss activity restriction and gradual resumption of desired activity. Encourage the use of energy-saving, relaxation , and diversional techniques. The client with severe ARF may need to restrict activity and/or may feel weak for an extended period during the lengthy recovery phase, requiring measures to conserve energy and reduce boredom. A study observed an association between higher levels of physical activity and improved renal function. It is known that those with recovery following AKI have better outcomes than those with persistent renal impairment (Asad et al., 2022).
Discuss the reality of the continued presence of fatigue . Decreased metabolic energy production, the presence of anemia, and states of discomfort commonly result in fatigue . In one study, survivors of severe AKI had a worse health-related quality of life than the general population, even after adjusting for their reduced kidney function. Both physical and mental components were affected. Increasing age and reduced kidney function were associated with poorer physical QOL (Workeneh & Batuman, 2022).
Discuss renal dialysis or transplantation if these are likely options for the future. Although these options would have been previously presented by the healthcare provider, the client may now be at a point when options need to be considered and may desire additional input. The current recommendation by KDIGO for dialysis-dependent AKI clients is to deliver a kt/v of 3.9 per week when using intermittent or extended RRT and an effluent volume of 20 to 25 mg/kg/hour when using continuous renal replacement therapy (CRRT) (Workeneh & Batuman, 2022).
Determine ADLs and personal responsibilities. Identify available resources and support systems. This helps the client manage lifestyle changes and meet personal needs. Several studies of social support for chronic diseases have found that social support can be instrumental in improving self-management behaviors and reducing factors affecting the progression of diseases. Social support has also been found to influence the client’s health behaviors and disease management outcomes (Chen et al., 2018).
Recommend scheduling activities with adequate rest periods. This prevents excessive fatigue and conserves energy for healing and tissue regeneration. Exercise also has the ability to impact many of the adverse outcomes associated with an episode of AKI. Moreover, the suitability of exercise programs for individuals following a cardiac event suggests that it may also be feasible in the AKI population. This is due to the assumed similarity in the functional status of both AKI and cardiac clients (Asad, 2020).
Stress the necessity of follow-up care and laboratory studies. Renal function may be slow to return following acute failure (up to 12 months), and deficits may persist, requiring changes in therapy to avoid recurrence. Renal recovery is usually observed within the first two weeks, and many nephrologists tend to diagnose clients with end-stage kidney failure 6 to 8 weeks after the onset of AKI. It is always better to check these clients periodically, because some clients may regain kidney function much later (Workeneh & Batuman, 2022).
Be well-informed about AKI before providing education to the client and the family. Nursing plays a key role as an integral part of the client care team. In high-income countries, nurses play a central role in the care of hospitalized clients where standards of care are well established. Credentialing is required and ongoing educational programs are mandated to maintain nursing competence. In contrast, in low- to middle-income countries, there is a wider variation in the level of nursing care. Care is often provided in small private clinics where standards for nursing care may be highly variable and educational activities may not be as well defined (Lewington et al., 2013).
Raise awareness about AKI in the community. There are opportunities to raise awareness of AKI as a complication of other disease processes in the developing world and mobilize local resources to manage it at an early phase. The WHO/UN UNDP Millenium Project and the Campaign to Eradicate Malaria deal with the main root causes of community disease in developing countries. The Millenium Project attempts to eradicate extreme poverty and hunger, achieve universal primary education, promote gender equality and empower women, improve maternal health and reduce child mortality, combat HIV / AIDS , malaria , and other diseases, ensure environmental sustainability, and promote a global partnership for development. All these issues are intimately related to AKI in developing countries (Lewington et al., 2013).
Arrange follow-up consultations with a nephrologist. Previous research has demonstrated that nephrologists are more skilled at recognizing and managing CKD complications according to evidence-based guidelines than primary care providers. Some centers have started to establish outpatient care models dedicated to clients who survive an episode of AKI. Clinic visits consist of a standardized assessment by a nephrologist that highlights blood pressure and urine albumin control, a review of core laboratory studies that are obtained quarterly, cardiovascular risk modification, management of CKD complications, medication reconciliation, and specialist referrals for co-morbidity management (Silver & Siew, 2017).
In the management of Acute Renal Failure (ARF), medications are primarily focused on addressing the underlying cause, managing complications, and promoting renal function recovery. Medications may include diuretics to increase urine output, electrolyte supplements to correct imbalances, medications to control blood pressure, renal vasodilators and antioxidant agents to minimize further renal damage and drugs to treat specific conditions contributing to ARF, such as antibiotics for sepsis.
Diuretics such as furosemide , bumetanide , torsemide, and mannitol These are given early in the oliguric phase of ARF in an effort to convert to a non-oliguric phase, flush the tubular lumen of debris, reduce hyperkalemia , and promote adequate urine volume. Furosemide can be used to correct volume overload when the kidneys are still responsive; this often requires high intravenous doses. Furosemide plays no role in converting an oliguric AKI to a non-oliguric AKI or in increasing urine output when a client is not hypervolemic (Workeneh & Batuman, 2022).
Vasodilators such as fenoldopam Fenoldopam is a selective dopamine -receptor agonist that acts as a rapid-acting vasodilator. It is six times more potent than dopamine in producing renal vasodilation. It increases renal blood flow to the cortex and medullary regions in the kidney, increases diuresis, and has minimal adrenergic effects. Fenoldopam is indicated for the treatment of severe hypertension, including for clients with renal compromise (Workeneh & Batuman, 2022).
Calcium channel blockers These are given early in nephrotoxic acute tubular necrosis (ATN) to reduce the influx of calcium into kidney cells, thereby helping to maintain cell integrity and improve GFR. Calcium channel blockers have been shown in animal models to be protective in AKI if given before renal insult. Their only benefit in humans is preventing AKI in renal transplant clients receiving cyclosporine (Sinert & Sugalski, 2019).
Inotropic agents such as dopamine Dopamine stimulates adrenergic and dopaminergic receptors. Its hemodynamic effect is dose-dependent. In small doses, dopamine predominantly stimulates dopaminergic receptors, which, in turn, produces vasodilation of the renal vasculature. Enhancing renal perfusion (Workeneh & Batuman, 2022).
Calcium gluconate Serum calcium is often low but does not require specific treatment in ARF. Calcium gluconate may be given to treat hypocalcemia and to offset the effects of hyperkalemia by modifying cardiac irritability. Calcium gluconate directly antagonizes the neuromuscular and cardiovascular effects of magnesium (Fulop & Batuman, 2020).
Aluminum hydroxide gels Increased phosphate levels may occur as a result of the failure of glomerular filtration and require the use of phosphate-binding antacids to limit phosphate absorption from the GI tract.
Glucose and/or insulin solution A temporary measure to lower serum potassium is by driving potassium into cells when the cardiac rhythm is endangered. Glucose and insulin may help promote magnesium entry into cells. Glucose should be administered with insulin to prevent hypoglycemia (Fulop & Batuman, 2020).
Sodium bicarbonate or sodium citrate This may be used to correct acidosis or hyperkalemia (by increasing serum pH) if the client is severely acidotic and not suffering from fluid overload. Raising bicarbonate concentration will increase the filtered bicarbonate load above the proximal tubule’s reduced absorptive capacity, resulting in a marked bicarbonate diuresis. (Najjar, 2022)
Sodium polystyrene sulfonate with or without sorbitol Exchange resin trades sodium for potassium in the GI tract to lower serum potassium levels. Sorbitol may be included to cause osmotic diarrhea to help excrete potassium. Sodium polystyrene sulfonate helps by removing extra potassium from the body. Due to its slow onset of action, it is a second-line agent in emergent situations (Rahman & Marathi, 2022).
Iron preparations Iron deficiency may occur if the protein is restricted, the client is anemic, or GI function is impaired. In randomized trials, 100% of clients received oral or parenteral iron over 29 days, and this might explain the higher increase in hemoglobin in their clients (Aoun et al., 2022).
Calcium carbonate This restores normal serum levels to improve cardiac and neuromuscular function, blood clotting , and bone metabolism. Low serum calcium is often corrected as phosphate absorption is decreased in the GI system. Calcium may be substituted as a phosphate binder (Fulop & Batuman, 2020).
Selenium, vitamin C, and folic acid These are vital as coenzymes in cell growth and actions. Intake is decreased because of protein restrictions. During critical illnesses, vitamins, and trace elements may impact immunomodulation, and wound healing , and may have antioxidant properties. The current recommendation is that the losses of selenium and other micronutrients in the effluent fluid should be replaced. The optimal dose remains unknown, but a dosage of 100 mg/dL has been suggested for vitamin C (Fiaccadori et al., 2021).
Antibiotics Early administration of appropriate antimicrobial therapy within six hours and ideally within one hour is the cornerstone treatment in sepsis and improves survival. In a multicenter retrospective study with hypotensive clients with septic shock , longer delays before administration of appropriate antimicrobial therapy were associated with early AKI development (Godin et al., 2017).
Vasopressors Vasopressor support is often needed in septic shock since fluid therapy alone does not correct sepsis-induced systemic vasodilation and endothelial dysfunction. Norepinephrine is the drug of choice for septic clients requiring vasopressors (Godin et al., 2017).
Laboratory and diagnostic procedures play a crucial role in the evaluation and management of Acute Renal Failure (ARF). Common tests include blood tests to assess kidney function (such as serum creatinine and blood urea nitrogen), electrolyte levels, and acid-base balance. Urine tests, such as urinalysis and urine electrolyte measurements, can provide valuable information on kidney function and help identify the underlying cause of ARF.
Blood urea nitrogen (BUN), and creatinine (cr) BUN assesses the management of renal dysfunction. Both values may increase but creatinine is a better indicator of renal function because it is not affected by hydration, diet, and tissue catabolism. Dialysis is usually indicated if the ratio is higher than 10:1 or if therapy fails to indicate fluid overload or metabolic acidosis. The ratio of BUN to creatinine is an important finding. The ratio can exceed 20:1 in conditions in which enhanced reabsorption of urea is favored (Workeneh & Batuman, 2022).
Urine sodium In acute tubular necrosis, tubular functional integrity is lost and sodium resorption is impaired, resulting in increased sodium excretion. Urine electrolyte findings can also serve as valuable indicators of functioning renal tubules. The fractional excretion of sodium (FENa) is the commonly used indicator. Calculating FENa is useful in AKI only in the presence of oliguria (Workeneh & Batuman, 2022).
Serum sodium Hyponatremia may result from fluid overload (dilutional) or the kidney’s inability to conserve sodium. Hypernatremia indicates total body water deficit. Clients suffering from AKI were associated with impaired sodium and potassium homeostasis. Hyponatremia and hypernatremia are common electrolyte abnormalities among critically ill clients. A study suggested that abnormal sodium is an important risk factor for mortality independent of potassium levels among clients with heart failure (Gao et al., 2019).
Serum potassium Lack of renal excretion and/or selective retention of potassium to excrete excess hydrogen ions leads to hyperkalemia, requiring prompt intervention. Hyperkalemia is a common and important complication of AKI (Sinert & Sugalski, 2019).
Hemoglobin and hematocrit Decreased values may indicate hemodilution ( hypervolemia ) however, during prolonged failure, anemia frequently develops as a result of RBC loss. Other possible causes (active or occult hemorrhage ) should also be evaluated. Postoperative anemia and a decrease in hemoglobin levels have been reported as predictive factors for AKI and in-hospital mortality in cardiac surgery . The results of the studies emphasize the importance of detecting and correcting anemia in clients with AKI (Lombardi et al., 2021).
Calcium In addition to its own cardiac effects, calcium deficit enhances the toxic effects of potassium. Hypocalcemia is common in AKI; marked hypocalcemia is more typical of chronic renal failure (Sinert & Sugalski, 2019).
Magnesium Dialysis or calcium administration may be necessary to combat the CNS- depressive effects of an elevated serum magnesium level. Decreasing renal function represents a risk factor for magnesium accumulation, in the setting of exogenous supplementation. In clients with AKI, hypermagnesemia usually presents during the oliguric phase; the serum magnesium level returns to normal during the polyuric phase (Fulop & Batuman, 2020).
Serial chest X-rays Increased cardiac size, prominent pulmonary vascular markings, pleural effusion, and congestion indicate acute responses to fluid overload or chronic changes associated with renal and heart failure. Obtain chest radiographs on a routine basis to look for evidence of volume overload (Sinert & Sugalski, 2019).
Echocardiography Echocardiography is an essential tool for guiding resuscitation in critically ill clients. Resuscitation often requires the infusion of intravenous fluids in an effort to reverse organ dysfunction. Echocardiography is an evidence-based approach and is ideally suited to address fluid overload (Effat et al., 2021).
Stool guaiac test Gastrointestinal bleeding is a known complication of renal failure; however, its pathogenesis remains uncertain. Some have attributed gastrointestinal bleeding to the effects of uremia on the gastrointestinal mucosa; others have suggested that uremia may affect platelet adhesiveness, which may explain the prolonged gastrointestinal bleeding seen in clients with renal failure. In addition, the role of heparinization and the widespread use of antiplatelet agents in clients on dialysis have been implicated in the etiology of gastrointestinal bleeding.
WBC count with differential. Although elevated WBCs may indicate generalized infection, leukocytosis is commonly seen in ARF and may reflect injury within the kidney. A shifting of the differential to the left is indicative of infection. It is known that alteration in WBC counts is associated with the risk of long-term kidney outcomes. Each WBC subtype are one of the immunologic factors that play a pivotal role in most processes of organ damage. This role can apply to the issue of kidney damage. (Han et al., 2014)
Culture and sensitivity Verification of infection and identification of specific organisms aids in the choice of the most effective treatment. Note: A number of anti-infective agents require adjustments of dose and/or time while renal clearance is impaired. The presence of eosinophils , as visualized with the Wright stain or Hansel stain, might suggest interstitial nephritis. However, it has poor sensitivity in AIN diagnosis and can also be seen in UTIs, glomerulonephritis, and atheroembolic disease (Workeneh & Batuman, 2022).
Recommended nursing diagnosis and nursing care plan books and resources.
Disclosure: Included below are affiliate links from Amazon at no additional cost from you. We may earn a small commission from your purchase. For more information, check out our privacy policy .
Ackley and Ladwig’s Nursing Diagnosis Handbook: An Evidence-Based Guide to Planning Care We love this book because of its evidence-based approach to nursing interventions. This care plan handbook uses an easy, three-step system to guide you through client assessment, nursing diagnosis, and care planning. Includes step-by-step instructions showing how to implement care and evaluate outcomes, and help you build skills in diagnostic reasoning and critical thinking.
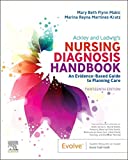
Nursing Care Plans – Nursing Diagnosis & Intervention (10th Edition) Includes over two hundred care plans that reflect the most recent evidence-based guidelines. New to this edition are ICNP diagnoses, care plans on LGBTQ health issues, and on electrolytes and acid-base balance.
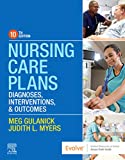
Nurse’s Pocket Guide: Diagnoses, Prioritized Interventions, and Rationales Quick-reference tool includes all you need to identify the correct diagnoses for efficient patient care planning. The sixteenth edition includes the most recent nursing diagnoses and interventions and an alphabetized listing of nursing diagnoses covering more than 400 disorders.
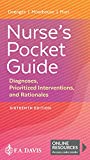
Nursing Diagnosis Manual: Planning, Individualizing, and Documenting Client Care Identify interventions to plan, individualize, and document care for more than 800 diseases and disorders. Only in the Nursing Diagnosis Manual will you find for each diagnosis subjectively and objectively – sample clinical applications, prioritized action/interventions with rationales – a documentation section, and much more!
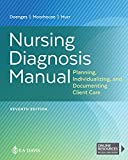
All-in-One Nursing Care Planning Resource – E-Book: Medical-Surgical, Pediatric, Maternity, and Psychiatric-Mental Health Includes over 100 care plans for medical-surgical, maternity/OB, pediatrics, and psychiatric and mental health. Interprofessional “patient problems” focus familiarizes you with how to speak to patients.
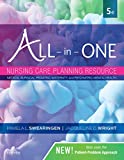
Other recommended site resources for this nursing care plan:
- Nursing Care Plans (NCP): Ultimate Guide and Database MUST READ! Over 150+ nursing care plans for different diseases and conditions. Includes our easy-to-follow guide on how to create nursing care plans from scratch.
- Nursing Diagnosis Guide and List: All You Need to Know to Master Diagnosing Our comprehensive guide on how to create and write diagnostic labels. Includes detailed nursing care plan guides for common nursing diagnostic labels.
Other care plans and nursing diagnoses related to reproductive and urinary system disorders:
- Acute Glomerulonephritis | 4 Care Plans
- Acute Renal Failure | 6 Care Plans
- Benign Prostatic Hyperplasia (BPH) | 5 Care Plans
- Chronic Renal Failure | 10 Care Plans
- Hemodialysis | 3 Care Plans
- Hysterectomy (TAHBSO) | 6 Care Plans
- Mastectomy | 15 Care Plans
- Menopause | 6 Care Plans
- Nephrotic Syndrome | 5 Care Plans
- Peritoneal Dialysis | 6 Care Plans
- Prostatectomy | 6 Care Plans
- Urolithiasis (Renal Calculi) | 4 Care Plans
- Urinary Tract Infection | 4 Care Plans
- Vesicoureteral Reflux (VUR) | 5 Care Plans
Resources you can use to further your research about acute kidney injury nursing care plan :
- Alper, A. B. (2022, January 6). Uremia: Practice Essentials, Pathophysiology, Etiology . Medscape Reference. Retrieved March 11, 2023.
- American Nephrology Nurses Association. (2019). Acute Kidney Injury Fact Sheet. American Nephrology Nurses Association .
- Aoun, M., Sleilaty, G., Boueri, C., Younes, E., Gabriel, K., Kahwaji, R.-M., Hilal, N., Hawi, J., Araman, R., Chelala, D., & Beaini, C. (2022). Erythropoietin in Acute Kidney Injury (EAKI): a pragmatic randomized clinical trial. BMC Nephrology , 23 (100).
- Asad, A. (2020, October 23). Exercise as a therapeutic option for acute kidney injury: mechanisms and considerations for the design of future clinical studies – BMC Nephrology . BMC Nephrology. Retrieved March 13, 2023.
- Asad, A., Thomas, A., Dungey, M., Hull, K. L., March, D. S., & Burton, J. O. (2022, April 11). Associations between physical activity levels and renal recovery following acute kidney injury stage 3: a feasibility study . NCBI. Retrieved March 13, 2023.
- Bindroo, S., Quintanilla Rodriguez, B. S., & Challa, H. J. (2022, August 8). Renal Failure – StatPearls . NCBI. Retrieved March 10, 2023.
- Busse, L. W., & Ostermann, M. (2019, September). Vasopressor Therapy and Blood Pressure Management in the Setting of Acute Kidney Injury. Seminars in Nephrology , 39 (5).
- Chen, X., Xu, J., Li, Y., Xu, X., Shen, B., Zou, Z., Ding, X., Teng, J., & Jiang, W. (2021, May 27). Risk Scoring Systems Including Electrolyte Disorders for Predicting the Incidence of Acute Kidney Injury in Hospitalized Patients . NCBI. Retrieved March 11, 2023.
- Chen, Y.-C., Chang, L.-C., Liu, C.-Y., Ho, Y.-F., Weng, S.-C., & Tsai, T.-I. (2018, March). The Roles of Social Support and Health Literacy in Self-Management Among Patients With Chronic Kidney Disease. Journal of Nursing Scholarship , 50 (3).
- Costantinides, F. (2018, November 13). Dental Care for Patients with End-Stage Renal Disease and Undergoing Hemodialysis . NCBI. Retrieved March 11, 2023.
- Dirkes, S. M. (2015, July 13). Acute kidney injury: Causes, phases, and early detection . American Nurse. Retrieved March 13, 2023.
- Effat, H., Alsayed, I. A., Alhusseiny, R., & Farouk, W. (2021, September). Fluid Management in Critically Ill Patients with Acute Kidney Injury: Role of Noninvasive Assessment of Stroke Volume. The Egyptian Journal of Critical Care Medicine , 8 (1).
- Fiaccadori, E., Sabatino, A., Barazzoni, R., Carrero, J. J., Cupisti, A., De Waele, E., Jonckheer, J., Singer, P., & Cuerda, C. (2021, April). ESPEN guideline on clinical nutrition in hospitalized patients with acute or chronic kidney disease. Clinical Nutrition , 40 (4).
- Fulop, T., & Batuman, V. (2020, May 4). Hypermagnesemia: Overview, Renal Failure, Other Causes . Medscape Reference. Retrieved March 10, 2023.
- Fülöp, T., Pathak, M. B., Schmidt, D. W., Lengvarszky, Z., Juncos, J. P., Lebrun, C. J., Brar, H., & Juncos, L. A. (2010, July). Volume-Related Weight Gain and Subsequent Mortality in Acute Renal Failure Patients Treated with Continuous Renal Replacement Therapy. American Society for Artificial Internal Organs Journal , 56 (4).
- Gao, X., Zheng, C., Liao, M., He, H., Liu, Y., Jing, C., Zeng, F., & Chen, Q. (2019). Admission serum sodium and potassium levels predict survival among critically ill patients with acute kidney injury: a cohort study. BMC Nephrology , 20 (311).
- Gist, K. M., & Faubel, S. (2020, June). Infection Post-AKI: Should We Worry? Nephron , 144 .
- Godin, M., Murray, P., & Mehta, R. L. (2017, September 27). Clinical Approach to the Patient with AKI and Sepsis . NCBI. Retrieved March 11, 2023.
- Goyal, A., Daneshpajouhnejad, P., Hashmi, M. F., & Bashir, K. (2022, August 18). Acute Kidney Injury – StatPearls . NCBI. Retrieved March 11, 2023.
- Han, S. S., Ahn, S. Y., Ryu, J., Baek, S. H., Kim, K., Chin, H. J., Na, K. Y., Chae, D.-W., & Kim, S. (2014). U-Shape Relationship of White Blood Cells with Acute Kidney Injury and Mortality in Critically Ill Patients. 232 , 3 .
- Hung, K.-Y., Chen, S.-T., Chu, Y.-Y., Ho, G., & Liu, W.-L. (2022, February). Nutrition support for acute kidney injury 2020-consensus of the Taiwan AKI task force. Journal of the Chinese Medical Association , 85 (2).
- Izawa, J., Kitamura, T., Iwami, T., Uchino, S., Takinami, M., Kellum, J. A., & Kawamura, T. (2016). Early-phase cumulative hypotension duration and severe-stage progression in oliguric acute kidney injury with and without sepsis: an observational study. Critical Care , 20 .
- Kellum, J. A., & Hall, S. (2018, February 26). Drug-Associated Acute Kidney Injury: Who’s at risk? NCBI. Retrieved March 13, 2023.
- Khadka, A., & Dani, S. (2020, December 31). Washing Hands according to the WHO Guideline since the COVID-19 Outbreak in the Context of Medical Undergraduates at a Tertiary Care Center: A Descriptive Cross-sectional Study . NCBI. Retrieved March 11, 2023.
- Lewington, A. J.P., Cerda, J., & Mehta, R. L. (2013, September). Raising awareness of acute kidney injury: a global perspective of a silent killer. Kidney International , 84 (3).
- Lombardi, Y., Ridel, C., & Touzot, M. (2021, February). Anaemia and acute kidney injury: the tip of the iceberg? Clinical Kidney Journal , 14 (2).
- The Medical City. (2017, January). News – Indirect Calorimetry . The Medical City. Retrieved March 11, 2023.
- Moorhouse, M. F., Doenges, M. E., & Murr, A. C. (2010). Nursing Care Plans : Guidelines for Individualizing Client Care Across the Life Span . F.A. Davis Company.
- Najjar, F. (2022, July 18). Renal Tubular Acidosis – StatPearls . NCBI. Retrieved March 10, 2023.
- Patil, V. P., & Salunke, B. G. (2020, April). Fluid Overload and Acute Kidney Injury. Indian Journal of Critical Care Medicine , 24 (3).
- Rahman, S., & Marathi, R. (2022, July 4). Sodium Polystyrene Sulfonate – StatPearls . NCBI. Retrieved March 10, 2023.
- Sacomboio, E. N. M., Campos, L. H., Daniel, F. N., & Ekundi-Valentin, E. (2021, November). Can vital signs indicate acute kidney injury in patients with malaria? Results of an observational study in Angola. Scientific African , 14 .
- Shusterman, B. (2021, May 19). Top 5 healthy drinks for people with kidney disease . American Kidney Fund. Retrieved March 10, 2023.
- Siew, E. D., Fissell, W. H., Tripp, C. M., Blume, J. D., Wilson, M. D., Clark, A. J., Vincz, A. J., Ely, E. W., Pandharipande, P. P., & Girard, T. D. (2017, June). Acute Kidney Injury as a Risk Factor for Delirium and Coma during Critical Illness. American Journal of Respiratory and Critical Care Medicine , 195 (12).
- Silver, S. A., & Siew, E. D. (2017, July). Follow-up Care in Acute Kidney Injury: Lost in Transition. Advances in Chronic Kidney Disease , 24 (4).
- Sinert, R. H., & Sugalski, G. (2019, November 20). Acute Kidney Injury (Renal Failure) in Emergency Medicine: Practice Essentials, Prognosis, Differential Diagnosis . Medscape Reference. Retrieved March 10, 2023.
- Workeneh, B. T., & Batuman, V. (2022, December 10). Acute Kidney Injury (AKI): Practice Essentials, Background, Pathophysiology . Medscape Reference. Retrieved March 10, 2023.
8 thoughts on “8 Acute Renal Failure Nursing Care Plans”
well its good i wish i can access this beautiful information updates
Thanks for your good service
Thanks for your good services and support towards our studies
Thanks so much for the struggle you are making for this group and may you be blessed
It’s educative…………i got what am expecting from it. Thank you so much, God bless you
hello. i am happy to get help from ur this page.i was assigned fr this acute renal failure toic.i have done my assignment by taking help this page.i am obliged.shabana hameed.RN,BSCN.
Thanks for the the information
Lots of good information; thanks.
Leave a Comment Cancel reply

Introduction
Case report, statement of ethics, disclosure statement, funding sources, author contributions, a case of acute kidney injury in a patient with renal hypouricemia without intense exercise.
- Split-Screen
- Article contents
- Figures & tables
- Supplementary Data
- Peer Review
- Open the PDF for in another window
- Get Permissions
- Cite Icon Cite
- Search Site
Daiki Aomura , Kosuke Sonoda , Makoto Harada , Koji Hashimoto , Yuji Kamijo; A Case of Acute Kidney Injury in a Patient with Renal Hypouricemia without Intense Exercise. Case Rep Nephrol Dial 12 May 2020; 10 (1): 26–34. https://doi.org/10.1159/000506673
Download citation file:
- Ris (Zotero)
- Reference Manager
Exercise-induced acute kidney injury (EIAKI) frequently develops in patients with renal hypouricemia (RHUC). However, several cases of RHUC with acute kidney injury (AKI) but without intense exercise have been reported. We encountered a 15-year-old male with RHUC who experienced AKI. He reported no episodes of intense exercise and displayed no other representative risk factors of EIAKI, although a vasopressor had been administered for orthostatic dysregulation before AKI onset. His kidney dysfunction improved with discontinuation of the vasopressor and conservative treatment. Thus, AKI can develop in patients with RHUC in the absence of intense exercise, for which vasopressors may be a risk factor.
Exercise-induced acute kidney injury (EIAKI) is a major complication in patients with renal hypouricemia (RHUC). EIAKI usually develops after intense exercise, such as anaerobic exertion, and is not accompanied by rhabdomyolysis [ 1 ]. However, there are several case reports of patients experiencing EIAKI without intense exercise [ 2-4 ]. Although the pathomechanism and risk factors of EIAKI remain unclear, many reports suggest that an oxidation-reduction imbalance is associated with EIAKI onset [ 5 ]. We herein report a case of acute kidney injury (AKI) in a patient with RHUC in the absence of intense exercise, which may have been caused by an oral vasopressor.
A 15-year-old male complained of strong fatigue after intense exercise since childhood. He had no remarkable medical history apart from allergic rhinitis. After entering high school, he often felt unwell, especially in the morning, and frequently missed classes. He was diagnosed as having orthostatic dysregulation and prescribed amezinium metilsulfate 10 mg/day, but his symptoms persisted. Eight days after the start of treatment he was switched to etilefrine 5 mg/day. However, his fatigue progressively worsened. He was found vomiting and unresponsive after collapsing in the bathroom on the eighth night following the prescription change and taken to the hospital by his family. In the emergency room he exhibited mild consciousness disturbance (Glasgow Coma Scale: E4V4M6) and complained of right lower abdominal pain. Laboratory tests (blood and urine), whole-body computed tomography, and head magnetic resonance imaging did not indicate any abnormalities (serum creatinine level 1.0 mg/dL, uric acid level 7.2 mg/dL). His conscious state and abdominal pain were improved on the next day, but his blood pressure gradually increased from 100/60 to 180/80 mm Hg and his serum creatinine level rose from 1.0 to 5.5 mg/dL during 5 days of admission. He was then transferred to our institution for the treatment of AKI and severe hypertension.
At the time of admission to our hospital the patient was fully conscious and alert. His body temperature was 37.2°C, blood pressure was 161/98 mm Hg, heart rate was 83 beats/min, and respiratory rate was 17 breaths/min. His height was 174 cm and his body weight was 54 kg. Physical examination detected no signs of dehydration, rash, or other abnormalities of the neck, chest, abdomen, or extremities. He had been taking loratadine 10 mg/day for his allergic rhinitis for several months. Both loratadine and etilefrine had been discontinued upon admission to the previous hospital. There was no family history of kidney dysfunction, and he reported no episodes of intense exercise other than daily commuting by bicycle to school. No alcohol consumption, smoking, or illegal drug use were reported. His laboratory data at the time of transfer to our hospital are summarized in Table 1 . Urinalysis showed mild proteinuria (0.66 g/gCr) and elevation of the tubulointerstitial injury marker β2 microglobulin (1,498 μg/L). Hematuria was not observed. His serum level of uric acid was low at 3.2 mg/dL and his fractional excretion of uric acid was high at 49.7%. Laboratory markers of rhabdomyolysis, diabetes mellitus, infection, and collagen diseases such as creatine phosphokinase, hemoglobin A1c, C-reactive protein, and autoimmune antibodies were all within normal range. An electrocardiogram disclosed left anterior hemiblock and nonspecific intraventricular conduction delay that had been detected when he was an elementary school student. A chest X-ray revealed no abnormalities. Ultrasound echography showed bilateral mild kidney swelling with increased renal cortical echogenicity (Fig. 1 ). No stenotic lesions were detected in the aorta or renal arteries, although the resistance index of the intrarenal arteries was slightly high (left 0.69, right 0.69), indicating a circulatory disturbance in the renal microvessels. Hydronephrosis and renal calcification were absent. An ultrasound-guided kidney biopsy performed 3 days after arrival at our hospital showed mild interstitial edema, vascular endothelial cell swelling in the renal interlobular arterioles, and no obvious signs of acute tubular necrosis (ATN) (Fig. 2 ). Treatment with continuous intravenous infusion of extracellular fluids and nicardipine gradually improved his kidney function and hypertension. His serum uric acid level decreased to 1.0 mg/dL (Fig. 3 ), and his fractional excretion of uric acid was at 55.9% at 10 days after admission. He was ultimately diagnosed as having AKI with RHUC and discharged 12 days after transfer to our hospital. Hypouricemia was found in his parents and a sister, indicating a hereditary condition. However, genetic screening did not detect any known causative RHUC mutations on URAT1/SLC22A12 or GLUT9/SLC2A9 .
Main laboratory data on admission to our hospital
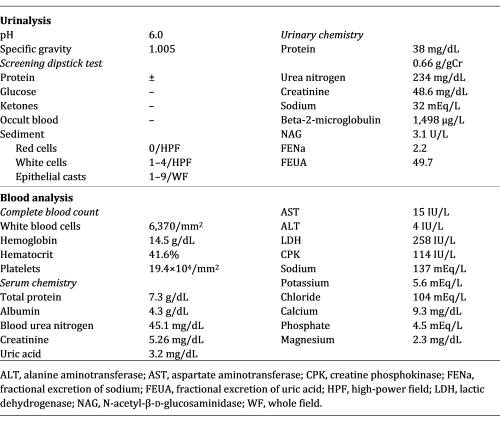
Renal ultrasound showed mild kidney swelling with increased renal cortical echogenicity. Hydronephrosis and renal calcification were not observed. Renal imaging findings were similar bilaterally (left 105 × 62 mm, right 115 × 63 mm).
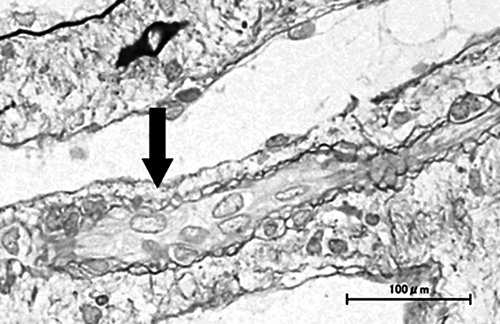
Kidney biopsy specimen findings. Mild interstitial edema and vascular lumen narrowing by endothelial cell swelling (arrow) were detected (periodic acid-methenamine silver stain). No other abnormalities were found, including signs of acute tubular necrosis.
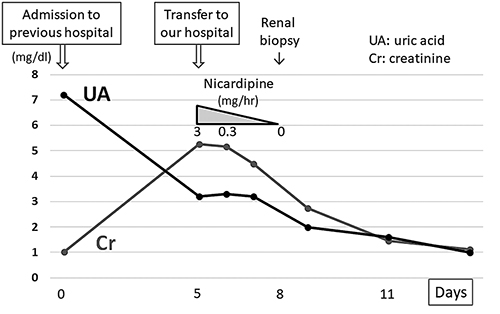
Clinical course of the present case. Vasopressors that had been administered for 15 days were discontinued on admission. After transfer to our hospital, his renal function improved gradually with continuous intravenous infusion of extracellular fluids and nicardipine. The serum uric acid level decreased steadily to 1.0 mg/dL during hospitalization.
Ishikawa et al. [ 6 ] first described EIAKI as AKI with accompanying abdominal or lower back pain after intense exercise, such as a 100-meter dash. EIAKI is differentiated from AKI with rhabdomyolysis by normal or slightly elevated serum myoglobin and creatine phosphokinase levels. EIAKI typically occurs in young males, with more than half having RHUC. Enhanced computed tomography often displays a wedge-shaped contrast defect in the kidneys. As for the clinical course of EIAKI, kidney dysfunction improves naturally without any special treatment [ 1, 7 ]. Although the reported patient had no intense episodes of exercise, EIAKI was diagnosed because he had RHUC, his kidney function recovered naturally, and he was young and male.
Blood pressure and serum creatinine level in our patient increased gradually following admission to the former hospital. As high blood pressure alone might cause AKI, we could not exclude the possible involvement of hypertension in AKI development. However, his serum creatinine level ultimately improved to 0.7 mg/dL after the final discharge despite having been 1.0 mg/dL on first admission, indicating that it had already been elevated by 0.3 mg/dL at the former hospital. Considering the fact that his blood pressure was normal on admission, AKI was thought to have developed before blood pressure elevation. Furthermore, his serum uric acid level was much higher on first admission (7.2 mg/dL) than at discharge (1.0 mg/dL), suggesting AKI onset prior to the former hospital visit. We suspected that AKI caused hypertension, which in turn worsened AKI. The elevation of blood pressure was assumed to be an exacerbation factor of EIAKI rather than its main cause.
The reported patient had no intense episodes of exercise. Lee et al. [ 3 ] described 17 AKI patients with abdominal or lower back pain who exhibited the characteristic patchy kidney sign on enhanced computed tomography. Among them, 5 patients reported no episodes of intense exercise. To the best of our knowledge, there have been 8 patients with EIAKI who did not have any episodes of intense exertion [ 2-4 ], with 5 experiencing infection or analgesic usage before EIAKI onset (Table 2 ), thought to be risk factors of EIAKI in addition to RHUC [ 3, 8, 9 ]. These reports support the notion that EIAKI can develop without intense exercise and the existence of risk factors other than strong exertion. However, to date no reports have focused on the relationship between lack of intense exercise and the etiology and development mechanism of EIAKI.
Clinical findings of current and previous reported cases of EIAKI without strenuous exercise
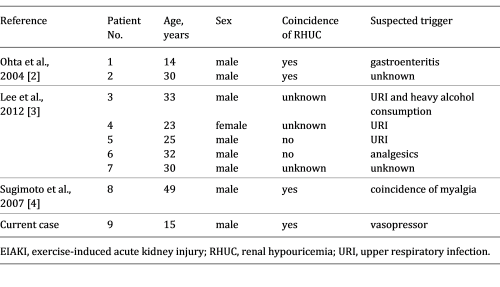
The pathomechanism of EIAKI is unclear, but renal circulatory disturbance by reactive oxygen species (ROS) is thought to be a main cause [ 5 ]. Intense exercise, such as anaerobic exertion, produces large amounts of ROS, which are rapidly removed by uric acid and other scavengers in the healthy population [ 8 ]. Patients with RHUC have insufficient scavengers, resulting in inadequate ROS removal and the subsequent activation of vasoconstrictive factors, vasoconstriction, and renal ischemia [ 2 ]. Since renal vasoconstriction is known to trigger further vasoconstriction and oxidative stress via activation of the renin-angiotensin system and blood pressure elevation [ 10 ], EIAKI patients are thought to show a vicious cycle between oxidative stress and vasoconstriction – oxidative stress causes stronger vasoconstriction and vasoconstriction causes more oxidative stress – culminating in acute and severe renal injury. In the present case, the patient had been taking vasopressors for orthostatic dysregulation for 15 days prior to the onset of AKI. Amezinium metilsulfate inhibits monoamine oxidase activity and suppresses the uptake of noradrenaline, while etilefrine activates type α1 and β1 adrenaline receptors. Thus, both vasopressors increased cardiac output and the constriction of peripheral vessels [ 11, 12 ]. Bellomo et al. [ 13 ] reported that activation of type α1 adrenaline receptors could cause excessive renal vasoconstriction and decreased renal blood flow in models of healthy renal hemodynamics. Radaković et al. [ 14 ] described that adrenaline induction increased ROS and caused a disruption in oxidant/antioxidant balance. Considering these results and the developmental mechanism of EIAKI (i.e., ROS and renal ischemia), we suspect that the vasopressors may have affected the onset or worsening of EIAKI by increasing ROS, exacerbating vasoconstriction, and forming a vicious cycle of diminished renal hemodynamics. Karasawa et al. [ 15 ] reported a case of EIAKI who was given midodrine, another vasopressor, before the onset of EIAKI, and Saito et al. [ 16 ] described that vasoexpansion by low-dose dopamine improved the resistance index of renal arterioles in 2 cases of EIAKI, implying the relation between vasopressors and EIAKI in clinical settings. Although no studies have directly addressed the relationship between vasopressors and EIAKI, past reports and our own results indicate an importance of catecholamine level homeostasis in the pathogenesis of EIAKI. We suspect that vasopressors may be associated with AKI onset in RHUC patients and may be a risk factor of EIAKI.
Renal biopsy showed no significant abnormalities in the present case. Although patients with EIAKI generally exhibit ATN, Ohta et al. [ 2 ] reported no abnormalities in 6 of 28 renal biopsies from EIAKI patients, which implied that EIAKI could develop without ATN. AKI with renal ischemia often causes ATN. However, tubular necrosis is sometimes absent without a sufficient degree or duration of ischemia, and early treatment for renal ischemia leads to a rapid improvement in renal function in such cases [ 17 ]. In the present patient, vasopressors, which might be a risk factor for EIAKI, were discontinued and intravenous antihypertensive medication was induced just after the first admission. The serum uric acid level was temporarily elevated on admission by AKI, and the patient’s scavenging ability with serum uric acid was thought to be temporarily improved. These factors could have mitigated the vicious cycle between renal vasoconstriction and oxidative stress, reduced the severity of renal ischemia, and prevented ATN development. However, as no studies have addressed the cause or meaning of a lack of ATN in some EIAKI patients, a greater number of studies are needed.
In conclusion, AKI can develop in patients with RHUC without intense exercise, possibly through the use of vasopressors. Further related case reports are needed to clarify the association between vasopressor use and AKI in patients with RHUC.
The present case report adhered to the Declaration of Helsinki. Informed consent for publication was obtained from the patient.
The authors declare no conflicts of interest.
The authors received no specific funding for this work.
D. Aomura drafted the article. K. Sonoda, M. Harada, and K. Hashimoto revised the article critically for important intellectual content. Y. Kamijo revised the article critically for important intellectual content and gave final approval of the version to be submitted.

Email alerts
Citing articles via, suggested reading.
- Online ISSN 2296-9705
INFORMATION
- Contact & Support
- Information & Downloads
- Rights & Permissions
- Terms & Conditions
- Catalogue & Pricing
- Policies & Information
- People & Organization
- Stay Up-to-Date
- Regional Offices
- Community Voice
SERVICES FOR
- Researchers
- Healthcare Professionals
- Patients & Supporters
- Health Sciences Industry
- Medical Societies
- Agents & Booksellers
Karger International
- S. Karger AG
- P.O Box, CH-4009 Basel (Switzerland)
- Allschwilerstrasse 10, CH-4055 Basel
- Tel: +41 61 306 11 11
- Fax: +41 61 306 12 34
- Contact: Front Office
- Experience Blog
- Privacy Policy
- Terms of Use
This Feature Is Available To Subscribers Only
Sign In or Create an Account
- Campus Directory
- Current Students
- Faculty & Staff

Acute Renal Failure Case Study
Our kidneys are incredible organs that get rid of toxins, retain substances needed by our bodies, and maintain the right balance of electrolytes, minerals, and water. Find out what happens to this 27-year-old when toxins accumulate in her kidneys leading to acute renal failure.
Module 11: Acute Tubular Necrosis
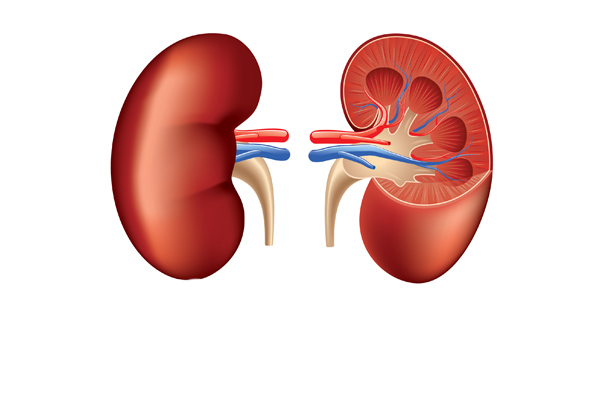
A 27 year old female was seen by medical personnel at an after...
Renal Failure - Page 1
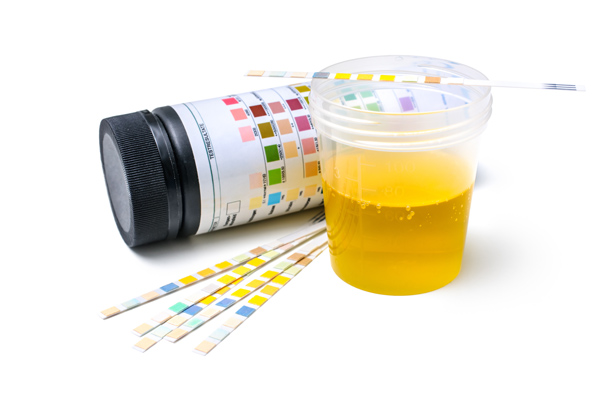
The patient was admitted to the hospital by the patient's...
Renal Failure - Page 2
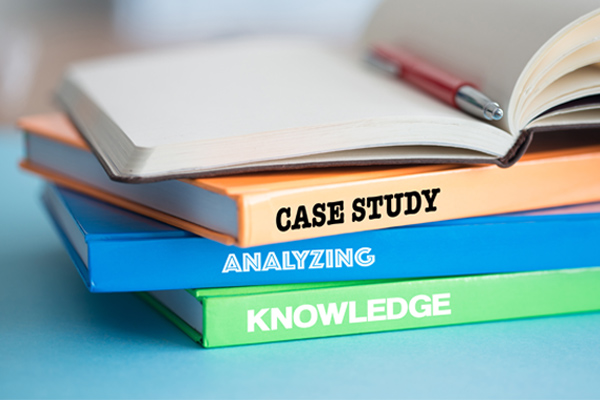
Case Summary
Summary of the Case
Renal Failure - Summary
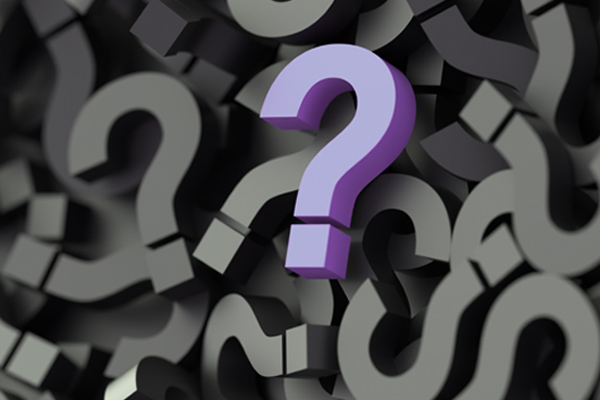
Answers to Case Questions
Renal Failure - Answers
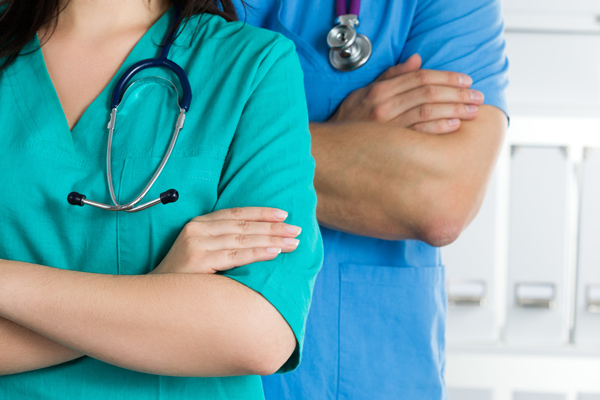
Professionals
Health Professionals Introduced in Case
Renal Failure - Professionals
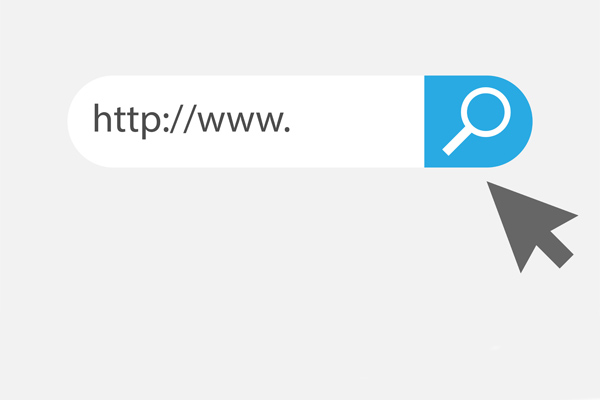
Additional Links
Opptional links to explore further
Renal Failure - Links
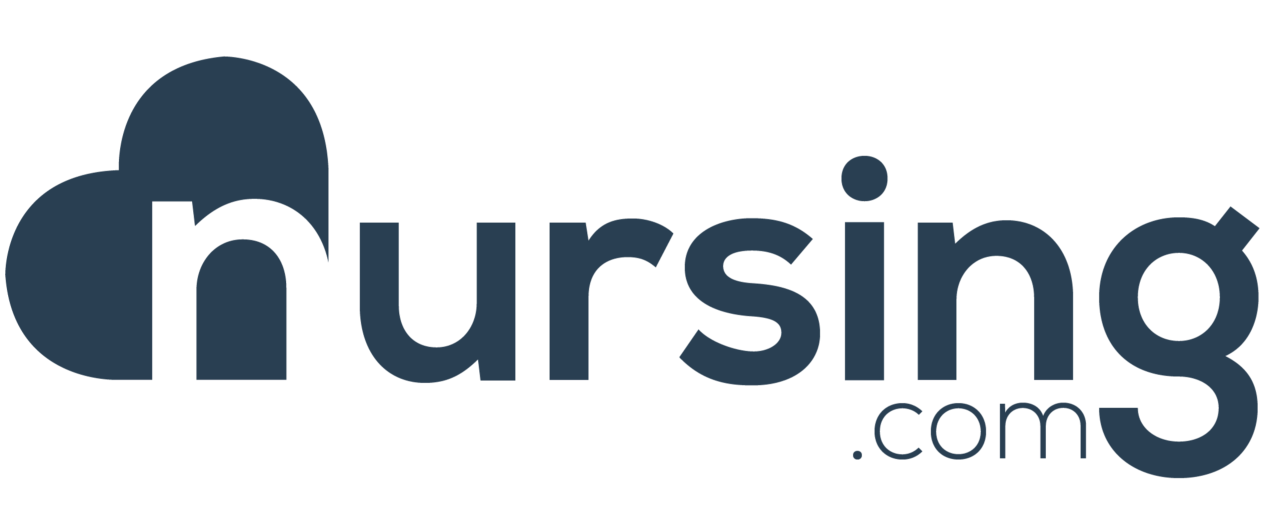
What Every Nursing Student Needs to Know About Acute Kidney Injury (AKI)

What are you struggling with in nursing school?
NURSING.com is the BEST place to learn nursing. With over 2,000+ clear, concise, and visual lessons, there is something for you!
Learning about Acute Kidney Injury
Every nursing student should understand that acute kidney injury (AKI) is a rapid decline in kidney function, potentially leading to life-threatening complications. Knowledge of its common causes, such as dehydration, medications, and sepsis, along with recognizing early signs and implementing prompt interventions, is crucial for improving patient outcomes.
.png?width=1200&height=630&name=nclex%20review%20for%20angina%20(2).png)
Acute kidney injury (AKI) represents a sudden and critical deterioration in kidney function, demanding immediate medical attention. This condition can arise from various factors such as severe infections, certain medications, dehydration, or underlying diseases.
When the kidneys are unable to effectively filter waste and excess fluids from the blood, harmful substances accumulate, disrupting the body's delicate balance. AKI may manifest as decreased urine output, fluid retention, fatigue, and nausea.
Timely intervention is essential, as untreated AKI can lead to serious complications like electrolyte imbalances, fluid overload, and even kidney failure. Healthcare professionals work to address the underlying cause while managing the AKI through careful monitoring, medication adjustments, and appropriate interventions to restore kidney function and mitigate potential long-term consequences.
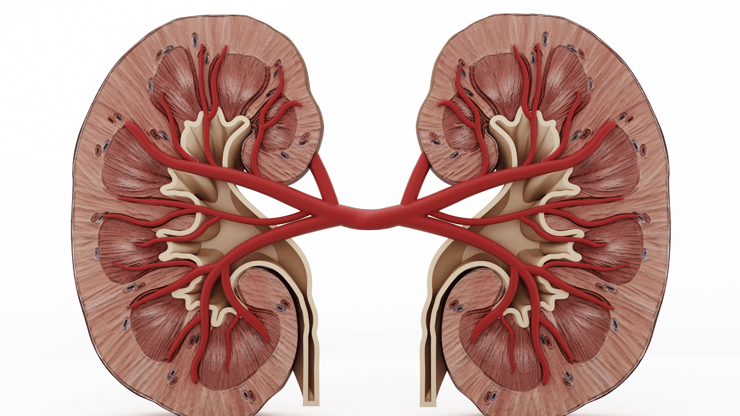
Overview of Acute Kidney Injury
1. Sudden onset of renal damage 2. Loss of renal function due to poor circulation or renal cell damage 3. Usually reversible may resolve on its own, but can lead to permanent damage if not reversed quickly
General Information on Acute Kidney Injury
a. Prerenal i. Decreased blood flow to the kidneys, accounts for a majority of cases→ Hypotension, Hypovolemia, ↓ Cardiac Output (i.e. Heart Failure, Shock) b. Intrarenal i. Damage within the kidney itself→ Tubular necrosis, infection, obstruction, contrast dye, nephrotoxic medications c. Postrenal i. Damage between the kidney and urethral meatus backs up, causing damage to kidneys→ due to infection, calculi, or obstruction 2. Phases a. Onset→ Note a decrease in baseline urine output b. Oliguric→ Decreased urine output <400 mL/ day. This is the sickest phase where there is a ↑ BUN/Creatinine and ↓ Glomerular Filtration Rate (GFR) c. Diuretic→ Beginning to recover, there is a gradual increase in urine output followed by diuresis d. Recovery→ has decreased edema, electrolytes normalize, and GFR increases
Nursing Assessment for Acute Kidney Injury
Therapeutic management for acute kidney injury.
1. Oliguric Phase a. Restrict fluid intake because there is volume overload, give diuretics for volume overload, and identify & treat the cause 2. Diuretic Phase a. Replace fluids and electrolytes and especially watch potassium & sodium levels 3. If not recovering, may need dialysis
Nursing Case Study for Acute Kidney Injury
Patient profile:.
- Decreased urine output
- Swelling in the legs and ankles
- Fatigue and weakness
- Elevated blood pressure
- Physical Examination: Mr. Smith displayed edema in the lower extremities and elevated blood pressure
- Laboratory Tests: Elevated serum creatinine and blood urea nitrogen (BUN) levels, indicating impaired kidney function
- Renal Ultrasound: Revealed acute kidney injury with reduced kidney perfusion
Medical History: Mr. Smith had a history of hypertension and diabetes, which may have contributed to his acute kidney injury
Diagnosis: Mr. Smith was diagnosed with acute kidney injury, a sudden decrease in kidney function, resulting in impaired filtration and waste-elimination Treatment Plan:
With diligent nursing care and medical management, Mr. Smith’s kidney function showed signs of improvement. His urine output increased, and his blood pressure was stabilized. Gradually, his serum creatinine and BUN levels began to decline.
Conclusion and Free Download
This Acute Kidney Injury (AKI) review provides essential knowledge for confidently approaching the NCLEX. Understanding its prevention, management, and interventions empowers nurses to provide effective care and save lives.
Looking for more must-know NCLEX review topics? Download our free eBook, "NCLEX Flash Notes: 77 Must-Know Nursing Topics for the NCLEX," by simply providing your email address below. I'll send you a complimentary copy straight to your inbox!
You CAN Do This
Happy Nursing!
You'll Need to Know This about Pelvic Inflammatory Disease (PID) for the NCLEX Exam
Urinary tract infection nclex review for nursing students, similar blog posts.
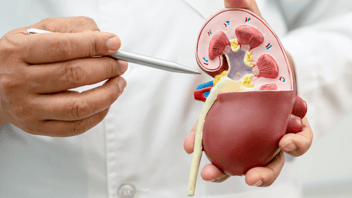
Chronic Kidney Disease (CKD) NCLEX Review for Nursing Students + Free Download
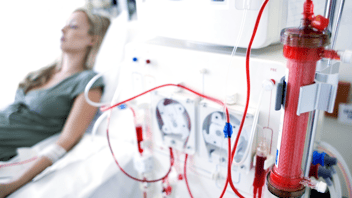
Dialysis: Overview and Nursing Case Study for Nursing School and NCLEX Prep
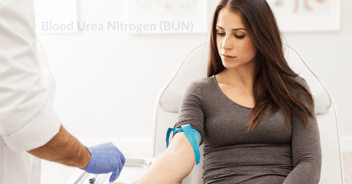
Complete Guide to Blood Urea Nitrogen (BUN) Lab Values: Nursing Lab Values
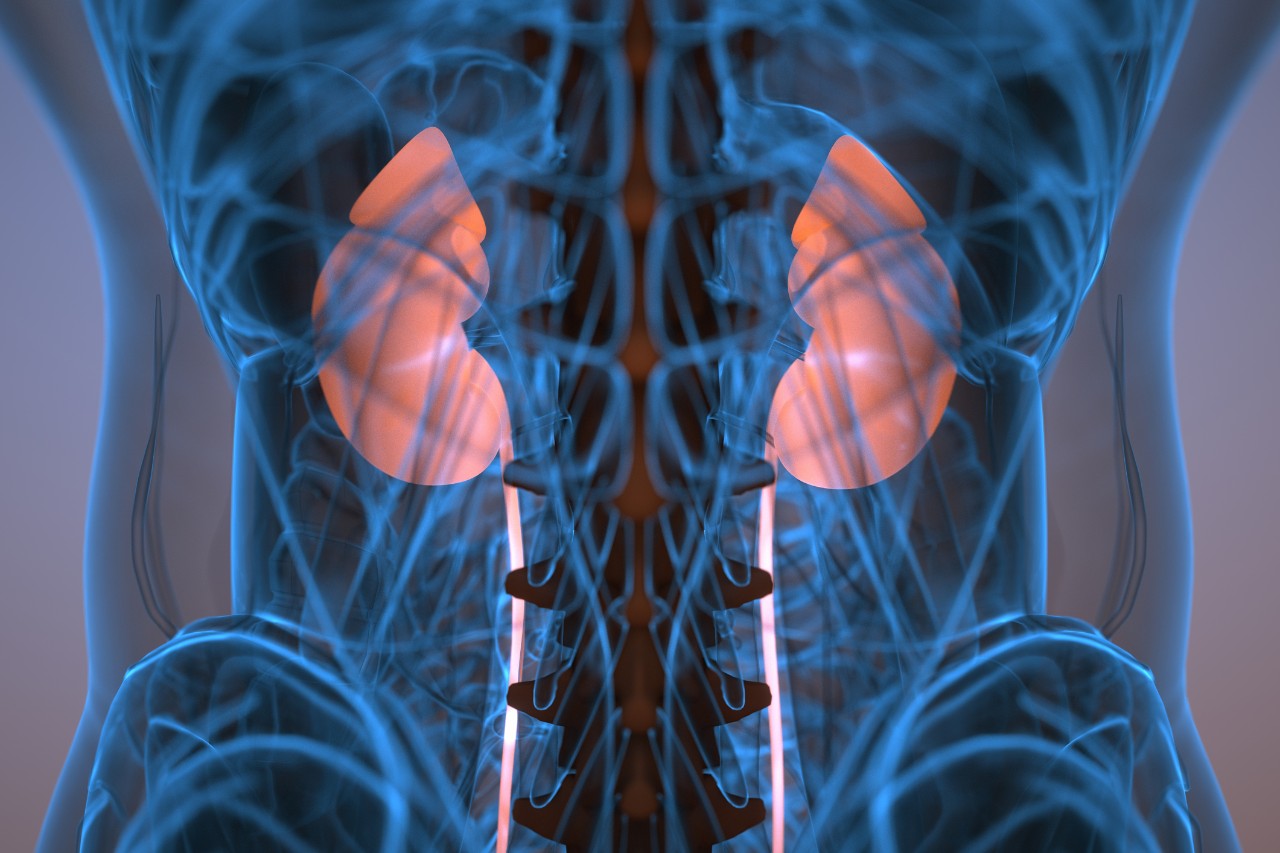
UC study uses health factors to predict kidney function recovery
Age, race, heart health, body mass among key factors in kidney care with dialysis-dependent aki.

Researchers at the University of Cincinnati College of Medicine have created a scoring model that uses key health indicators to accurately predict recovery for patients who experience kidney failure due to acute kidney injury (AKI), which occurs when kidneys stop working properly and can range from minor loss of kidney function to complete failure.
AKI is a major contributor to end-stage kidney disease (ESKD). About a third of patients with ESKD due to AKI recover kidney function.
Their findings, published in the Clinical Kidney Journal , predict kidney recovery within 90 days and 12 months after the start of dialysis for kidney failure patients due to AKI. The study looked at the health outcomes of 22,922 patients from the U.S. Renal Data System from 2005 to 2014 to offer predictions.
Lead author Silvi Shah, MD, associate professor in the Division of Nephrology at UC, explains that researchers examined several factors used in logistic regression models to analyze the effect of various covariates on a patient’s health outcome and found that 24% and 34% of patients with kidney failure due to AKI recovered kidney function within 90 days and 12 months, respectively.
Patient factors such as age, race or ethnicity, body mass index, congestive heart failure, cancer, amputation, functional status, hemoglobin and prior nephrology care are used in the regression model.
“One of the significant comorbidities was a history of heart failure,” Shah says. “If you had heart failure, you were at a lower chance of recovering as well. If you had a lower body mass index, you had a lower chance of recovery; if you had amputation or poor functional status, you had a low chance of recovery. These were some of the significant predictors of the score.”
Dr. Charuhas Thakar and Dr. Silvi Shah are shown in the UC College of Medicine. Photo by Colleen Kelley/UC Marketing + Brand.
Shah notes that younger people got more points in her prediction model because older age was also associated with a likelihood of lower recovery for kidney failure patients due to AKI.
The scoring model was developed as a way to help staff providing clinical care in a dialysis unit quickly triage patients based on characteristics that would be easily available in their medical history, explains Charuhas Thakar, MD, a senior author in the study, professor at Queen’s University Belfast in the United Kingdom and former division chief of nephrology at the University of Cincinnati.
“Patients who develop dialysis dependent AKI typically receive care in dialysis facilities amongst other patients with end stage kidney disease,” says Thakar. “Our goal was to quickly triage and determine who has the higher, medium or low prospect of recovery based on clinical characteristics the patient had in their medical history.”
“That was our broad approach. If your score is high, you have the best chance of recovery,” adds Thakar. “Patients who are more likely to recover can be monitored closely for that prospect, and we can focus our energies on preserving renal function. At the same time, those who are unlikely to recover should be allowed to undergo long term planning including transplantation. In summary, our study paves the way to individualize care as well as facilitate efficient use of treatment and resources.”
Shah says it’s important to understand that patients who have kidney failure due to AKI can recover.
“Around one-fourth of those patients will recover in 90 days, and around one-third of those patients will recover in 12 months,” says Shah. “There are several factors which can predict the recovery rate. If you have lower body mass index, if you are Black, have congestive heart failure or a history of amputation, you have lower chances of recovery.
“It helps us to do a risk prediction score and at the same time helps us to tell patients and healthcare providers what is the percentage recovery that may be expected,” adds Shah. “If you fall in the high score category, they have a 57% chance of recovery in 90 days. This is very encouraging for both patients and physicians."
She emphasizes that researchers included the largest data in the U.S. — using the United States Renal Data System — and captured all patients on dialysis in the country, including information for women, men and different races and ethnicities.
“One of the biggest strengths of the paper was that it was inclusive, and the score was individualized,” Shah says. “It can help in counseling and risk prediction and tailoring treatment specifically for patients that have dialysis-dependent AKI, and based on the score, we can tell patients what’s the chance of their recovery.”
Other co-authors of the research at the University of Cincinnati are Department of Environmental and Public Health Sciences professors Anthony Leonard, PhD; Karthikeyan Meganathan, PhD; and Annette Christianson; along with Kathleen Harrison from the Division of Nephrology. Jia Ng, MD, assistant professor of medicine at Hofstra University, is also a co-author of the study.
Both Silvi Shah and Jia Ng had career development awards from the National Institutes of Health. Ng also received support from the Breslin Family Foundation. Shah received intramural funds from the UC Division of Nephrology.
Disclosures: Jia Ng received consultancy fees from Vifor Pharmaceuticals. She is a founder of PublishedMD Consulting LLC.
Read the research study online .
Impact Lives Here
The University of Cincinnati is leading public urban universities into a new era of innovation and impact. Our faculty, staff and students are saving lives, changing outcomes and bending the future in our city's direction. Next Lives Here.
- College of Medicine
- Public Health
- Environmental Health
- Academic Health Center
Related Stories
May 21, 2024
Researchers at the University of Cincinnati College of Medicine have created a scoring model that uses key health indicators to accurately predict recovery for patients who suffer kidney failure due to acute kidney injury (AKI).
U.S. News & World Report: Metformin may help young patients with bipolar disorder avoid weight gain
October 31, 2023
U.S. News & World Report highlighted recent research led by the University of Cincinnati and Northwell Health that found the drug metformin can help prevent or reduce weight gain in youth taking medication to treat bipolar disorder.
UC research examines workplace stress in the aftermath of the COVID-19 pandemic
November 28, 2022
A new study from the University of Cincinnati examined the impact of workplace stress and provided insights for organizations to explore ways to reduce workplace stress for a better and healthier working environment. The study had two parts: a survey in which respondents described their experiences of workplace stress during the COVID-19 pandemic and a quantitative study using saliva cortisol as a biomarker of stress along with a stress diary to find out where and when workplace stress commonly occurred.
Heart failure subtype after acute kidney injury
- Open access
- Published: 17 May 2024
- Volume 25 , article number 167 , ( 2024 )
Cite this article
You have full access to this open access article
- Bethany C. Birkelo 1 ,
- Evan Brittain 2 ,
- Andrew Guide 3 ,
- Robert A. Greevy 3 , 4 , 5 ,
- Michael E. Matheny 4 , 5 , 6 , 7 ,
- Jeffrey Annis 8 ,
- Trey Richardson 4 ,
- Sarah Faubel 9 &
- Edward D. Siew 4 , 5 , 7
165 Accesses
Explore all metrics
Introduction
Acute kidney injury (AKI) is associated with increased risk of heart failure (HF). Determining the type of HF experienced by AKI survivors (heart failure with preserved or reduced ejection fraction, HFpEF or HFrEF) could suggest potential mechanisms underlying the association and opportunities for improving post-AKI care.
In this retrospective study of adults within the Vanderbilt University health system with a diagnosis of HF, we tested whether AKI events in the two years preceding incident HF associated more with HFpEF or HFrEF while controlling for known predictors. HF outcomes were defined by administrative codes and classified as HFpEF or HFrEF by echocardiogram data. We used multivariable logistic regression models to estimate the effects of AKI on the odds of incident HFpEF versus HFrEF.
AKI (all stages) trended towards a preferential association with HFpEF in adjusted analyses (adjusted OR 0.80, 95% CI 0.63 – 1.01). Stage 1 AKI was associated with higher odds of HFpEF that was statistically significant (adjusted OR 0.62, 95% CI 0.43 – 0.88), whereas stages 2–3 AKI showed a trend toward HFrEF that did not reach statistical significance (adjusted OR 1.11, 95% CI 0.76 – 1.63).
Conclusions
AKI as a binary outcome trended towards a preferential association with HFpEF. Stage 1 AKI was associated with higher odds of HFpEF, whereas stage 2–3 trended towards an association with HFrEF that did not meet statistical significance. Different mechanisms may predominate in incident HF following mild versus more severe AKI. Close follow-up with particular attention to volume status and cardiac function after discharge is warranted after even mild AKI.
Similar content being viewed by others
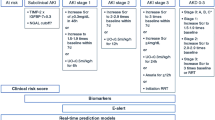
Acute kidney injury in the critically ill: an updated review on pathophysiology and management
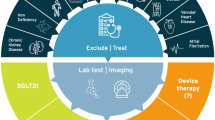
Heart failure with preserved ejection fraction: diagnosis, risk assessment, and treatment
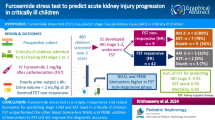
Furosemide stress test to predict acute kidney injury progression in critically ill children
Heart failure (HF) comprises an enormous burden on human health, afflicting over 64 million people globally [ 1 ]. Despite considerable overlap in risk factors, distinct pathophysiologic processes underlie HF with preserved ejection fraction (HFpEF) versus HF with reduced ejection fraction (HFrEF). While HFrEF results from a progressive loss of cardiomyocytes, often following cardiac ischemia, HFpEF is thought to be caused by multimorbidity-induced systemic inflammation that results in cardiomyocyte stiffness and fibrosis via coronary microvascular endothelial inflammation [ 2 ]. The high prevalence of comorbidities in patients with HFpEF support this paradigm [ 3 ].
Patients with HF commonly experience kidney dysfunction before and/or after they are diagnosed with HF. HF and kidney disease exist in a complex, bidirectional relationship in which dysfunction in one organ can cause or result from dysfunction in the other. Of the types of kidney disease leading to HF, chronic kidney disease (CKD) is the most well-described; up to one-fifth of patients with CKD go on to develop incident HF [ 4 ]. The association between CKD and HFpEF is particularly strong, with an independent association between the two conditions shown in observational data [ 5 ] and abnormalities in cardiac mechanics common to both conditions [ 6 , 7 ]. Acute kidney injury (AKI) is increasingly recognized as a risk factor for HF. Cardiac changes after AKI have been observed in preclinical studies [ 8 , 9 ], and observational studies have shown increased risk of HF hospitalizations [ 10 ] and incident HF [ 11 ] after AKI. The predominant type of HF that occurs after AKI (HFpEF versus HFrEF) is not known. Understanding HF type after AKI could suggest possible mechanisms of this aspect of cardiorenal syndrome and highlight areas of focus for post-AKI care. We hypothesized that AKI would be more strongly associated with incident HFpEF compared to HFrEF. To test this hypothesis, we used a population of HF patients to test whether AKI in the two years prior selectively associates with HFpEF or HFrEF.
Study setting and design
In this single center retrospective study, we compared the odds of incident HFpEF versus HFrEF after AKI among adults who obtained regular medical care (at least 3 visits within 5 years) through the Vanderbilt University health system between 2008 and 2022. We ascertained AKI events occurring in the two years prior to HF and adjusted for baseline comorbidities, vitals, and medications prior to the AKI time window (i.e., greater than two years prior to HF). Our choice of a two-year ascertainment period for AKI was informed by preclinical data demonstrating cardiac changes in the heart occurring within days of AKI, and we wanted to focus on the acute effect as opposed to a longer time period, which we would expect to result in a higher proportion of new onset CKD from unrecovered AKI events. The missing echocardiogram data presents a challenge in testing for a preferential association of AKI with HFpEF or HFrEF, so we focused on patients with available echocardiograms by utilizing a study design akin to a case–control design. A strength of the case–control design is the prevalence of the outcome in the sample does not need to reflect the prevalence in the general population in order to accurately estimate the odds ratios of interest. In this study design, patients with HFrEF are “cases” and those with HFpEF are “controls”, which allows the estimation of odds ratios to indicate whether each variable in a logistic model associates more strongly with HFrEF or HFpEF. For example, if AKI did not preferentially associate more with HFrEF or HFpEF, the odds ratio for AKI from the logistic model would be close to one. Conversely, an odds ratio significantly greater than one would indicate a preferential association with AKI and HFrEF; and significantly less than one would indicate a preferential association with AKI and HFpEF.
Data were obtained from the Synthetic Derivative (SD), a de-identified mirror image of the electronic health record (EHR) at Vanderbilt University Medical Center which contains date-shifted data from approximately 2.2 million unique patients, including demographics, diagnosis and procedure codes, medications, lab values, procedure reports, and clinical notes. Data in the SD is extracted from the prior homegrown EHR and from Epic since its go-live in 2017 at Vanderbilt University Medical Center (VUMC). This study was approved by the Institutional Review Board at VUMC. Informed consent was waived by the VUMC IRB due to the use of de-identified data which is considered non-human subjects research.
Eligibility criteria
See Fig. 1 . The source population consisted of 1,329,715 patients who obtained regular care (≥ 3 outpatient visits in 5 years) within the Vanderbilt University health system. Of those, we identified 89,391 with a HF diagnosis. Inclusion criteria included at least one outpatient serum creatinine value occurring between 2008 and 2020 and at least three years prior to HF diagnosis. Exclusion criteria included baseline eGFR < 15 ml/min/1.37m 2 , kidney transplantation, end-stage renal disease (ESRD), or receipt of dialysis prior to the AKI ascertainment window, and age < 18 years at HF diagnosis. To ensure all HF could be classified as HFpEF or HFrEF, patients without an echocardiogram within 31 days prior to or 365 days after HF diagnosis date were also excluded.

Consort diagram. Eligibility criteria applied to derive final study population
Data collection
Baseline comorbidity and vitals data were obtained from records occurring greater than two years prior to the outcome date (date of HF diagnosis, see Fig. 2 ). Identification of comorbidities and prevalent HF extended back to all available data. To capture active medication use, baseline medications were obtained from prescription information occurs between two and three years prior to outcome date. Exposure data (inpatient serum creatinine values) were obtained from hospitalization records 31 – 730 days prior to the outcome date. Exposure data was not captured in the 30 days prior to outcome date to exclude AKI that occurred concurrently with acute HF. HF outcomes were obtained from diagnosis codes and echocardiogram data (see Definitions for additional details). Diagnosis and procedure codes, which were used to define the HF outcome and baseline conditions, were defined using International Classification of Diseases (ICD) versions 9 (ICD-9) and 10 (ICD-10) and Current Procedural Terminology (CPT) Codes.
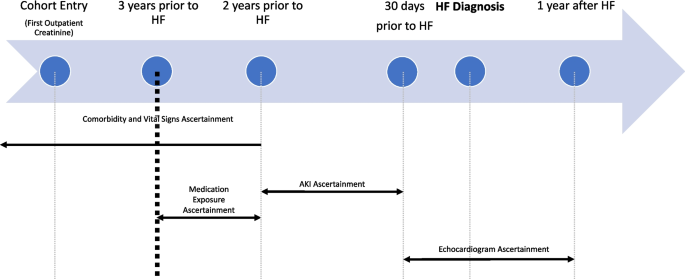
Ascertainment timeline. Time periods of ascertainment of baseline conditions, vital signs, medications, and exposure (AKI events) in relation to outcome (HF diagnosis date). Echocardiogram used to classify HF as HFpEF or HFrEF ascertained in 30 days before and up to 1 year following HF diagnosis date
Definitions
Cases and controls.
We defined cases as HFrEF and controls as HFpEF. A HF diagnosis was defined by either one inpatient diagnosis code or two outpatient diagnosis codes for HF or cardiomyopathy. The date of HF diagnosis was defined as the date of the first inpatient HF diagnosis code or the first of two outpatient diagnosis codes. HF was classified as HFpEF or HFrEF based on the ejection fraction on the echocardiogram closest in time to the HF diagnosis date (HFpEF > 45%, HFrEF ≤ 45%).
The exposure was hospitalized AKI, which was defined using inpatient serum creatinine values and staged using modified Kidney Disease Improving Global Outcomes creatinine-based criteria: stage 1, ≥ 0.3 mg/dl creatinine increase from baseline or creatinine 1.5 to 1.9 times baseline; stage 2, creatinine 2.0 to 2.9 times baseline; and stage 3, creatinine 3.0 times baseline or initiation of dialysis [ 12 ]. Baseline serum creatinine was defined as the mean outpatient serum creatinine value occurring 7–365 days prior to the AKI hospitalization. For those without an available baseline in the prior year, the lowest serum creatinine during the AKI hospitalization was used. Estimated glomerular filtration rate (eGFR) was calculated using the Chronic Kidney Disease Epidemiology Collaboration (CKD-EPI) Equation [ 13 ]. For patients with more than one AKI event during the ascertainment period, the most severe AKI was used in the analysis.
Baseline comorbidities, vital signs, and laboratory results were ascertained up to two years prior to outcome date. Preadmission medication use was ascertained from two to three years prior to outcome date.
Statistical approach
Descriptive statistics were obtained by reporting means and standard deviations for continuous covariates, and counts and percentages for categorical data; additionally, the descriptive data was split by HFpEF versus HFrEF. Multivariable logistic regression models were used to estimate the effects of AKI on incident (new onset) HFpEF versus HFrEF, with an odds ratio < 1 indicating greater odds of HFpEF and > 1 indicating greater odds of HFrEF. The primary exposure of interest was AKI. In the primary analysis, AKI was considered a binary variable. In secondary analyses, AKI was modelled as stage 1 and stages 2 or 3 (combined) to consider separately AKI events more likely to be prerenal azotemia (stage 1) versus those more likely to represent parenchymal damage (stages 2–3). To control for confounding, the models included 37 covariates which were ascertained prior to the AKI ascertainment window (see Fig. 2 ) and included demographics, baseline conditions, and medications. Continuous variables were modelled using three-knot restricted cubic splines to account for their non-linear effects in the models. To address missingness, multiple imputation utilizing predictive mean matching and 5–100 imputations-iterations was used. Confidence intervals were obtained using Wald tests. P values were obtained using chi-square tests. All analyses were conducted using R version 4.3.0.
We conducted several sensitivity analyses. First, to ensure that our results were not materially affected by misclassification of the outcome, we used a highly specific definition of HF that required an elevated BNP level (> 100 pg/ml) and a diuretic prescription occurring within 30 days of the HF diagnosis code date. Second, we conducted an analysis limited to patients with HF defined by an inpatient diagnosis code, which we expect to have a higher positive predictive value for HF compared to outpatient codes. Third, because of the high proportion of patients with a history of cancer and the lack of data on chemotherapy drug exposure, we conducted an analysis limited to patients without a history of cancer to ensure our results were not impacted by differential rates of exposure to cardiotoxic agents. Fourth, given the substantial population of adult congenital heart disease patients within the Vanderbilt Health system, we conducted an analysis limited to patients ages 30 years or older. Fifth, due to the degree of missingness of smoking status (missing in 67.5% of patients), we conducted an analysis using imputed smoking status. Sixth, we conducted an analysis limited to patients with EF < 40% or > 50% (thus excluding patients with heart failure with moderately reduced EF). Finally, to evaluate for differences in outcomes in men versus women, we replicated the primary analysis stratified by sex. The opposite directionality of the point estimates for stage 1 AKI versus stages 2–3 AKI in the secondary analyses motivated a post hoc analysis to evaluate for an effect of severe AKI in excess to the overall AKI effect using a model that included stages 1–3 AKI and stages 2–3 AKI as covariates. This model estimates an overall AKI association and a separate additional stage 2–3 AKI association.
Patient characteristics
We identified 6,996 patients with incident HF (5,149 with HFpEF and 1,847 with HFrEF) (See Table 1 ). At baseline, patients who developed HFpEF tended to be older, more often female, and more commonly had atrial fibrillation, anemia, COPD, hypertension, liver disease, and cancer, while those who developed HFrEF had higher prevalence of coronary artery disease and prior myocardial infarction (MI). Baseline eGFR was similar between both groups.
An AKI event occurred during the ascertainment period in 6.9% of those who developed HFpEF and 5.7% of those who developed HFrEF (see Table 2 ). The overall distribution of AKI severity was higher among those who developed HFrEF (61%, 20%, and 19% for stages 1, 2, and 3, respectively) versus HFpEF (72%, 16%, and 13% for stages 1, 2, and 3, respectively, P = 0.062) though the difference was not statistically significant.
See Fig. 3 . In the unadjusted analysis, AKI was not preferentially associated with HF subtype (OR 0.81, 95% CI 0.65 – 1.02). When examined by AKI severity, stage 1 AKI was associated with 31% higher odds of HFpEF (OR 0.69, 95% CI 0.52 – 0.92) whereas stage 2–3 had a non-significant trend towards HFrEF (OR 1.12, 95% CI 0.77 – 1.61).

Risk of HFpEF versus HFrEF. Results of primary analysis. Odds ratios for each covariate indicate higher odds of HFpEF (OR < 1) or HFrEF (OR > 1)
In the adjusted analysis, AKI trended toward an association with HFpEF (OR 0.80, 95% CI 0.63 – 1.01) but did not meet statistical significance. When examined by AKI severity, stage 1 AKI was associated with 32% higher odds of HFpEF (OR 0.68, 95% CI 0.51 – 0.91) whereas stage 2–3 had a non-significant trend towards HFrEF (OR 1.11, 95% CI 0.76 – 1.63). The covariates with statistically significant associations with HFpEF included older age, higher BMI, female sex, atrial fibrillation, and hypertension. The covariates with significant associations with HFrEF included coronary artery disease and black race.
Sensitivity analyses
Hf defined by diagnosis code + bnp + diuretic.
See Fig. 4 for sensitivity analyses results. Among patients for whom BNP and diuretic data were available, AKI (all stages) was associated with 13% higher odds of HFpEF (OR 0.87, 95% CI 0.56 – 1.33). Stage 1 AKI was associated with 38% higher odds of HFpEF (OR 0.62, 95% CI 0.36 – 1.07) and stage 2–3 AKI was associated with 51% higher odds of HFrEF (OR 1.51, 95% CI 0.77 – 2.98).

Risks of HFpEF versus HFrEF in primary analysis and sensitivity analyses. Odds ratios for each analysis indicate higher odds of HFpEF (OR < 1) or HFrEF (OR > 1)
HF defined by only inpatient diagnosis code
Among patients in whom HF was diagnosed by an inpatient diagnosis code, AKI (all stages) was associated with 14% higher odds of HFpEF (OR 0.86, 95% CI 0.64 – 1.14). Stage 1 AKI was associated with 25% higher odds of HFpEF (OR 0.75, 95% CI 0.53 – 1.08) and stage 2–3 AKI was associated with 8% higher odds of HFrEF (OR 1.08, 95% CI 0.69 – 1.70).
Patients without cancer
Among patients without a history of cancer, AKI (all stages) was associated with 24% higher odds of HFpEF (OR 0.76, 95% CI 0.57 – 1.01). Stage 1 AKI was associated with 39% higher odds of HFpEF (OR 0.61, 95% CI 0.42 – 0.87) and stage 2–3 AKI was associated with 15% higher odds of HFrEF (OR 1.15, 95% CI 0.73 – 1.80).
Patients > 30 years of age
Among patients aged 30 years or older, AKI (all stages) was associated with 19% higher odds of HFpEF (OR 0.81, 95% CI 0.63 – 1.03). Stage 1 AKI was associated with 32% higher odds of HFpEF (OR 0.68, 95% CI 0.50 – 0.92) and stage 2–3 AKI was associated with 16% higher odds of HFrEF (OR 1.16, 95% CI 0.78 – 1.72).
Including imputed smoking status
In this analysis that used imputed data for smoking status, AKI (all stages) was associated with 21% higher odds of HFpEF (OR 0.79, 95% CI 0.62 – 1.00). Stage 1 AKI was associated with 34% higher odds of HFpEF (OR 0.66, 95% CI 0.50 – 0.89) and stage 2–3 AKI was associated with 10% higher odds of HFrEF (OR 1.10, 95% CI 0.75 – 1.62).
Excluding patients with HF with mildly reduced EF (HFmrEF)
In this analysis that was limited to patients with EF < 40% or ≥ 50%, AKI (all stages) was associated with 22% higher odds of HFpEF (OR 0.78, 95% CI 0.60 – 1.03). Stage 1 AKI was associated with 39% higher odds of HFpEF (OR 0.61, 95% CI 0.43 – 0.87) and stage 2–3 AKI was associated with 25% higher odds of HFrEF (OR 1.25, 95% CI 0.81 – 1.92).
Analysis stratified by sex
To evaluate for differences in outcomes by sex, we first replicated the primary analysis in each sex. Among men, AKI (all stages) was associated with 27% higher odds of HFpEF (OR 0.73, 95% CI 0.53 – 0.99). Stage 1 AKI was associated with 45% higher odds of HFpEF (OR 0.55, 95% CI 0.38 – 0.81), while stage 2–3 AKI was associated with 30% higher odds of HFrEF (OR 1.30, 95% CI 0.78 – 2.18). Among women, AKI (all stages) was associated with 8% higher odds of HFpEF (OR 0.92, 95% CI 0.64 – 1.32). Stage 1 and stages 2–3 AKI were both associated with 8% higher odds of HFpEF (stage 1 OR 0.92, 95% CI 0.59 – 1.43; stage 2–3 OR 0.92, 95% CI 0.50 – 1.67.) We included a sex-by-AKI interaction term in the model to evaluate for effect modification by sex, which was not statistically significant.
Analysis of weighted cohort
As an additional sensitivity analysis, we used inverse probability of treatment weights (IPTW) to synthesize a cohort that could match the HFrEF patients to the HFpEF on all observed covariates except for AKI, as would be done in a case–control study with a sufficient number of controls to allow such matching. Characteristics of the weighted cohort are detailed in Table 3 . In this analysis, AKI (all stages) was associated with 20% higher odds of HFpEF (OR 0.80, 95% CI 0.60 – 1.05). Stage 1 AKI was associated with 33% higher odds of HFpEF (OR 0.67, 95% CI 0.48 – 0.93) and stage 2–3 AKI was associated with 14% higher odds of HFrEF (OR 1.14, 95% CI 0.72 – 1.82).
Post hoc analysis
Given the unexpected opposite directionality of the point estimates for stage 1 AKI compared to stages 2–3 AKI, we conducted a post hoc analysis to test for a unique additional effect of severe AKI in contrast to the overall AKI effect. In this analysis, the overall AKI effect (all AKI stages) was associated with 32% higher odds of HFpEF (OR 0.68, 95% CI 0.51 – 0.91), while the additional severe AKI effect (stage 2–3 AKI) was associated with 64% higher odds of HFrEF which was statistically significant (OR 1.64, 95% CI 1.03 – 2.63).
We also examined the number of MI events in each group to determine if a larger proportion of MIs during or after stage 2–3 AKI could explain the risk of HFrEF. Of the 460 patients with AKI, 39 had an MI (defined by presence of both a diagnosis code for myocardial infarction and elevated troponin level) during or within 5 days of the AKI hospitalization; of these, 31 developed HFpEF and 8 developed HFrEF. Similarly, 25% of those with stage 1 AKI and 20% of those with stage 2–3 AKI had an MI in the time between the AKI event and the date of HF.
AKI has been shown to be strongly associated with HF in prior studies [ 10 , 11 ]. The nature of this association and whether AKI is preferentially associated with the type of HF observed has not been previously studied. To our knowledge, this is the first study to examine the nature of HF experienced by AKI survivors. In this study, we found a statistically significant association between stage 1 AKI and HFpEF. Stages 2–3 AKI did not demonstrate a significant association with HF subtype, although point estimates trended towards HFrEF (ORs > 1). The association we found between stage 1 AKI and HFpEF is consistent with other known risk factors for HFpEF such as older age, female sex, obesity, and atrial fibrillation [ 5 , 14 , 15 , 16 ].
There are multiple potential explanations for our findings. As multimorbidity is a risk factor for HFpEF [ 17 ], the association between stage 1 AKI and HFpEF could reflect a comorbidity burden that both increases an individual’s risk of HFpEF and causes a decreased renal reserve that renders them more likely to have small fluctuations in serum creatinine during illness or hospitalization. Alternatively, rather than simply functioning as an indicator of multimorbidity and consequent risk of HFpEF, it is possible that mild AKI may mediate a direct effect on the heart. It is well established in preclinical studies that AKI adversely affects the heart and leads to echocardiographic evidence of cardiac dysfunction [ 18 , 19 , 20 ] including diastolic dysfunction [ 8 , 9 ]. In the ischemia reperfusion injury (IRI) model, a single episode of transient AKI leads to diastolic dysfunction by three days [ 8 ] which persists up to one year [ 8 , 9 ]. Preclinical data also provides insights on potential mechanisms of AKI-induced cardiac dysfunction, showing that AKI is associated with cardiomyocyte apoptosis, cardiac inflammation, mitochondrial dysfunction, and reduced ATP levels [ 21 ]. As both diastolic and systolic function are energy requiring processes, mitochondrial dysfunction leading to inadequate ATP levels is a plausible explanation of diastolic dysfunction in murine models (especially given the absence of traditional causes of diastolic dysfunction such as hypertension and severe acidosis) [ 8 , 9 ]. A specific circulating mediator of AKI-induced cardiac dysfunction has not yet been identified, although proinflammatory cytokines and uremic toxins may play a role [ 20 , 22 ]. Since AKI is known to induce systemic inflammation [ 23 ], which is a major driver of HFpEF pathogenesis [ 2 ], AKI-induced inflammation is a plausible potential mediator of the association with HFpEF.
Our findings related to more severe AKI were unexpected. While our small sample size may have precluded our ability to detect a statistically significant association between severe AKI and HFrEF, the opposite directionality of the point estimate for stage 2–3 AKI (compared to stage 1) could suggest a different or unique mechanism with more severe AKI. The post hoc analysis that modeled an overall AKI association (as could be caused by a multimorbidity effect) and an additional severe AKI association (as could be caused by a separate mechanism unique to stage 2–3 AKI) found a significant association unique to stage 2–3 AKI for HFrEF. Such an association could be explained by higher illness severity that results in a decreased ejection fraction (i.e., severe AKI occurring after a massive MI with cardiogenic shock). However, while administrative codes do not capture disease severity, the MI events that occurred during the AKI hospitalization overwhelmingly occurred in patients who developed HFpEF, so it seems unlikely that co-occurrence of MI and stage 2–3 AKI entirely explains the association. It is also possible that complications of or therapy for severe AKI may increase the risk of HFrEF, as both uremia and acidosis have been shown to affect cardiac function in preclinical studies [ 22 , 24 ], and hemodialysis can cause myocardial stunning [ 25 ]. Another potential explanation is AKI worsening preexisting cardiac injury. A recent study found that AKI dramatically worsened pre-existing cardiac injury in rats, resulting in global cardiac dysfunction including reduced left ventricular systolic function [ 26 ]. Future studies of HF risk after severe AKI events with larger sample sizes are needed to investigate this potential association further.
Strengths of this study include a creatinine-based definition of AKI, clinical adjudication of AKI cases classified as stage 3 based on dialysis (to ensure CKD progression was not misclassified as AKI), an outcome defined using echocardiogram data in addition to administrative codes, and a dataset enriched for patients obtaining longitudinal care within the health system. Limitations include a predominantly White population, which may limit generalizability to more diverse populations, and inclusion of only inpatient AKI, which may underestimate number of AKI events. While detailed phenotyping of AKI subtype (i.e., acute tubular injury, obstructive AKI, etc.) was not feasible, we modeled stage 1 versus stage 2–3 AKI, as stage 2–3 AKI is more likely to represent parenchymal damage. Future work that enables more granular AKI phenotyping in EHR data will facilitate investigations of the associations between different forms of AKI with HF. It is also possible that some patients may have developed HF that was diagnosed outside of the Vanderbilt system, however by requiring 3 outpatients visits within 5 years in our inclusion criteria, we enriched our dataset for patients receiving longitudinal care within our health system and thus limited the likelihood that patients would have been diagnosed with HF outside of the Vanderbilt health system. Additionally, as our objective in this study was to test for a preferential association of AKI with HFpEF or HFrEF, we did not seek to recapitulate the risk of HF after AKI, which has been previously demonstrated [ 10 , 11 ]. We also defined the HF outcome with an EF cutoff of ± 45%, rather than the current American College of Cardiology definitions (HFrEF < 40%, HFmrEF 40–49%, HFpEF ≥ 50%) [ 27 ], as the pathophysiology underpinning HFmrEF is less well-defined and use of a binary outcome allowed us to leverage the case–control study design to test for a preferential association between AKI and HF subtype. However, results of the sensitivity analysis excluding patients with HFmrEF were consistent with the primary analysis, which is reassuring that our choice of a 45% EF cutoff did not materially affect our findings. Finally, we cannot infer causation due to the observational nature of this study.
In summary, we found that while AKI overall did not have a preferential association with HFrEF or HFpEF, stage 1 AKI had a statistically significant association with HFpEF. Our findings underscore the importance of close follow-up and attention to volume status and cardiac function after a hospitalization complicated by even mild AKI.
Availability of data and materials
The data that support the findings of this study are not publicly available due to the inclusion of confidential electronic health record data. Data is available from the Vanderbilt University Medical Center Synthetic Derivative for researchers who meet the criteria for access, which requires a data use agreement. Contact [email protected] to request the data from this study.
Savarese G, Becher PM, Lund LH, Seferovic P, Rosano GMC, Coats AJS. Global burden of heart failure: a comprehensive and updated review of epidemiology. Cardiovasc Res. 2023;118(17):3272–87.
Article PubMed Google Scholar
Paulus WJ, Tschope C. A novel paradigm for heart failure with preserved ejection fraction: comorbidities drive myocardial dysfunction and remodeling through coronary microvascular endothelial inflammation. J Am Coll Cardiol. 2013;62(4):263–71.
Ather S, Chan W, Bozkurt B, Aguilar D, Ramasubbu K, Zachariah AA, et al. Impact of noncardiac comorbidities on morbidity and mortality in a predominantly male population with heart failure and preserved versus reduced ejection fraction. J Am Coll Cardiol. 2012;59(11):998–1005.
Article PubMed PubMed Central Google Scholar
Kottgen A, Russell SD, Loehr LR, Crainiceanu CM, Rosamond WD, Chang PP, et al. Reduced kidney function as a risk factor for incident heart failure: the atherosclerosis risk in communities (ARIC) study. J Am Soc Nephrol. 2007;18(4):1307–15.
Article CAS PubMed Google Scholar
Brouwers FP, de Boer RA, van der Harst P, Voors AA, Gansevoort RT, Bakker SJ, et al. Incidence and epidemiology of new onset heart failure with preserved vs. reduced ejection fraction in a community-based cohort: 11-year follow-up of PREVEND. Eur Heart J. 2013;34(19):1424–31.
Park M, Hsu CY, Li Y, Mishra RK, Keane M, Rosas SE, et al. Associations between kidney function and subclinical cardiac abnormalities in CKD. J Am Soc Nephrol. 2012;23(10):1725–34.
Article CAS PubMed PubMed Central Google Scholar
Unger ED, Dubin RF, Deo R, Daruwalla V, Friedman JL, Medina C, et al. Association of chronic kidney disease with abnormal cardiac mechanics and adverse outcomes in patients with heart failure and preserved ejection fraction. Eur J Heart Fail. 2016;18(1):103–12.
Fox BM, Gil HW, Kirkbride-Romeo L, Bagchi RA, Wennersten SA, Haefner KR, et al. Metabolomics assessment reveals oxidative stress and altered energy production in the heart after ischemic acute kidney injury in mice. Kidney Int. 2019;95(3):590–610.
Soranno DE, Kirkbride-Romeo L, Wennersten SA, Ding K, Cavasin MA, Baker P, et al. Acute Kidney Injury Results in Long-Term Diastolic Dysfunction That Is Prevented by Histone Deacetylase Inhibition. JACC Basic Transl Sci. 2021;6(2):119–33.
Ikizler TA, Parikh CR, Himmelfarb J, Chinchilli VM, Liu KD, Coca SG, et al. A prospective cohort study of acute kidney injury and kidney outcomes, cardiovascular events, and death. Kidney Int. 2021;99(2):456–65.
Bansal N, Matheny ME, Greevy RA Jr, Eden SK, Perkins AM, Parr SK, et al. Acute Kidney Injury and Risk of Incident Heart Failure Among US Veterans. Am J Kidney Dis. 2018;71(2):236–45.
Kellum JA, Lameire N, Group KAGW. Diagnosis, evaluation, and management of acute kidney injury: a KDIGO summary (Part 1). Crit Care. 2013;17(1):204.
Levey AS, Stevens LA, Schmid CH, Zhang YL, Castro AF 3rd, Feldman HI, et al. A new equation to estimate glomerular filtration rate. Ann Intern Med. 2009;150(9):604–12.
Owan TE, Hodge DO, Herges RM, Jacobsen SJ, Roger VL, Redfield MM. Trends in prevalence and outcome of heart failure with preserved ejection fraction. N Engl J Med. 2006;355(3):251–9.
Santhanakrishnan R, Wang N, Larson MG, Magnani JW, McManus DD, Lubitz SA, et al. Atrial Fibrillation Begets Heart Failure and Vice Versa: Temporal Associations and Differences in Preserved Versus Reduced Ejection Fraction. Circulation. 2016;133(5):484–92.
Lee MP, Glynn RJ, Schneeweiss S, Lin KJ, Patorno E, Barberio J, et al. Risk Factors for Heart Failure with Preserved or Reduced Ejection Fraction Among Medicare Beneficiaries: Application of Competing Risks Analysis and Gradient Boosted Model. Clin Epidemiol. 2020;12:607–16.
Jasinska-Piadlo A, Campbell P. Management of patients with heart failure and preserved ejection fraction. Heart. 2023;109(11):874–83.
Kelly KJ. Distant effects of experimental renal ischemia/reperfusion injury. J Am Soc Nephrol. 2003;14(6):1549–58.
Sumida M, Doi K, Ogasawara E, Yamashita T, Hamasaki Y, Kariya T, et al. Regulation of Mitochondrial Dynamics by Dynamin-Related Protein-1 in Acute Cardiorenal Syndrome. J Am Soc Nephrol. 2015;26(10):2378–87.
Prud’homme M, Coutrot M, Michel T, Boutin L, Genest M, Poirier F, et al. Acute Kidney Injury Induces Remote Cardiac Damage and Dysfunction Through the Galectin-3 Pathway. JACC Basic Transl Sci. 2019;4(6):717–32.
Faubel S. Acute kidney injury causes and exacerbates cardiac dysfunction. Am J Physiol Renal Physiol. 2023;324(6):F568–70.
Lekawanvijit S, Kompa AR, Krum H. Protein-bound uremic toxins: a long overlooked culprit in cardiorenal syndrome. Am J Physiol Renal Physiol. 2016;311(1):F52-62.
Rabb H, Griffin MD, McKay DB, Swaminathan S, Pickkers P, Rosner MH, et al. Inflammation in AKI: Current Understanding, Key Questions, and Knowledge Gaps. J Am Soc Nephrol. 2016;27(2):371–9.
Orchard CH, Kentish JC. Effects of changes of pH on the contractile function of cardiac muscle. Am J Physiol. 1990;258(6 Pt 1):C967–81.
Burton JO, Jefferies HJ, Selby NM, McIntyre CW. Hemodialysis-induced cardiac injury: determinants and associated outcomes. Clin J Am Soc Nephrol. 2009;4(5):914–20.
Dominguez JH, Xie D, Kelly KJ. Cardiac effects of renal ischemia. Am J Physiol Renal Physiol. 2023;324(1):F64–74.
Bozkurt B, Coats AJS, Tsutsui H, Abdehlhamid M, Adamopoulos S, Albert N. Universal Definition and Classification of Heart Failure: A Report of the Heart Failure Society of America, Heart Failure Assocation of the European Society of Cardiology, Japanese Heart Failure Society and Writing Committee of the Universal Definition of Heart Failure. J Card Fail. 2021. https://doi.org/10.1016/j.cardfail.2021.01.022 .
Download references
Acknowledgements
This study’s findings were presented as an oral abstract at the American Society of Nephrology Kidney Week in Philadelphia, Pennsylvania on November 3, 2023.
BCB is supported in this work by the KidneyCure Ben J. Lipps Research Fellowship Program. EDS is supported in this work by the Vanderbilt O’Brien Kidney Center grant P30-DK114809 Clinical and Translational Research Core. EB is supported in this work by the NHLBI grant R01 HL146588. RAG is supported in this work by the Vanderbilt O’Brien Kidney Center grant P30-DK114809 Clinical and Translational Research Core. Funders had no role in the study design, data collection, analysis, or interpretation, writing the manuscript, or the decision to submit the manuscript for publication.
Author information
Authors and affiliations.
Division of Nephrology and Hypertension, University of Minnesota, 717 Delaware St. SE, Minneapolis, MN, 55414, USA
Bethany C. Birkelo
Division of Cardiovascular Medicine, Vanderbilt University Medical Center, Nashville, TN, USA
Evan Brittain
Department of Biostatistics, Vanderbilt University Medical Center, Nashville, TN, USA
Andrew Guide & Robert A. Greevy
Division of Nephrology and Hypertension, Vanderbilt Center for Kidney Disease (VCKD) and Integrated Program for Acute Kidney Injury Research (VIP-AKI), Vanderbilt University Medical Center, Nashville, TN, USA
Robert A. Greevy, Michael E. Matheny, Trey Richardson & Edward D. Siew
VA Tennessee Valley, Health Services Research and Development, Nashville, USA
Robert A. Greevy, Michael E. Matheny & Edward D. Siew
Department of Biomedical Informatics, Vanderbilt University Medical Center, Nashville, TN, USA
Michael E. Matheny
VA Geriatrics Research Education and Clinical Center (GRECC), Tennessee Valley Health System (THVS), Veteran’s Health Administration, Nashville, TN, USA
Michael E. Matheny & Edward D. Siew
Vanderbilt Institute for Clinical and Translational Research, Vanderbilt University Medical Center, Nashville, TN, USA
Jeffrey Annis
Department of Medicine, University of Colorado School of Medicine, Aurora, CO, USA
Sarah Faubel
You can also search for this author in PubMed Google Scholar
Contributions
Research idea and study design: B.C.B., E.B., R.A.G., M.E.M., J.A., T.R., and E.D.S.; data acquisition: E.B. and J.A.; funding acquisition: E.B. and E.D.S.; resources: E.B. and E.D.S.; data analysis/interpretation: B.C.B., A.G., R.A.G., and E.D.S.; writing original draft: B.C.B., A.G., R.A.G., S.F., and E.D.S; supervision and mentorship: R.A.G., and E.D.S. All authors read and approved the final manuscript. Each author contributed significant intellectual input during the initial drafting and/or revision of the manuscript and all accept accountability for the accuracy and integrity of this work.
Corresponding author
Correspondence to Bethany C. Birkelo .
Ethics declarations
Ethics approval and consent to participate.
This study was approved by the Vanderbilt University Institutional Review Board. Informed consent was not required due to the use of de-identified data, which is considered non-human subjects research.
Competing interests
EDS reports receiving personal fees for serving on the editorial board for the Clinical Journal of the American Society of nephrology, royalties as an author for UpToDate, and an agreement to serve on a DSMB study for Novartis. MEM reports the following: Employer: Department of Veterans Affairs; Vanderbilt University Medical Center; Consultancy: NIH-VA-DoD Pain Management Grant Consortium (PMC3); Food and Drug Administration (FDA); and Advisory or Leadership Role: SMRB Study Section, VA HSR&D, Informatics & Methods Section; Steering Committee—Indianapolis VA HSR&D COIN Center; Steering Committee—VA HSR&D VIREC; Steering Committee—Salt Lake City VA HSR&D COIN Center; VA ORD Million Veterans Program Executive Steering Committee. SF reports receiving consulting fees from SeaStar Medical.
Additional information
Publisher’s note.
Springer Nature remains neutral with regard to jurisdictional claims in published maps and institutional affiliations.
Rights and permissions
Open Access This article is licensed under a Creative Commons Attribution 4.0 International License, which permits use, sharing, adaptation, distribution and reproduction in any medium or format, as long as you give appropriate credit to the original author(s) and the source, provide a link to the Creative Commons licence, and indicate if changes were made. The images or other third party material in this article are included in the article's Creative Commons licence, unless indicated otherwise in a credit line to the material. If material is not included in the article's Creative Commons licence and your intended use is not permitted by statutory regulation or exceeds the permitted use, you will need to obtain permission directly from the copyright holder. To view a copy of this licence, visit http://creativecommons.org/licenses/by/4.0/ . The Creative Commons Public Domain Dedication waiver ( http://creativecommons.org/publicdomain/zero/1.0/ ) applies to the data made available in this article, unless otherwise stated in a credit line to the data.
Reprints and permissions
About this article
Birkelo, B.C., Brittain, E., Guide, A. et al. Heart failure subtype after acute kidney injury. BMC Nephrol 25 , 167 (2024). https://doi.org/10.1186/s12882-024-03602-1
Download citation
Received : 02 February 2024
Accepted : 06 May 2024
Published : 17 May 2024
DOI : https://doi.org/10.1186/s12882-024-03602-1
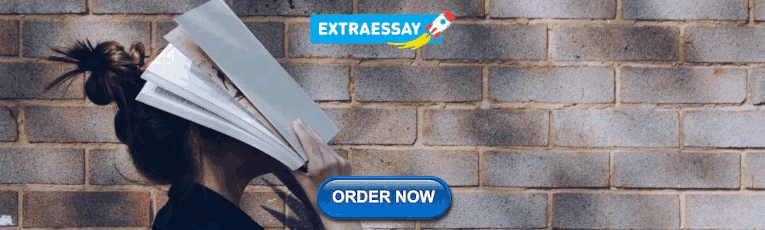
Share this article
Anyone you share the following link with will be able to read this content:
Sorry, a shareable link is not currently available for this article.
Provided by the Springer Nature SharedIt content-sharing initiative
- Acute kidney injury
- Cardiovascular outcomes
- Heart failure
- Find a journal
- Publish with us
- Track your research
Thank you for visiting nature.com. You are using a browser version with limited support for CSS. To obtain the best experience, we recommend you use a more up to date browser (or turn off compatibility mode in Internet Explorer). In the meantime, to ensure continued support, we are displaying the site without styles and JavaScript.
- View all journals
- My Account Login
- Explore content
- About the journal
- Publish with us
- Sign up for alerts
- Open access
- Published: 16 May 2024
Positive pathogens in stool could predict the clinical outcomes of sepsis-associated acute kidney injury in critical ill patient
- Yaoyuan Cao 1 &
- Fuxing Deng 2
Scientific Reports volume 14 , Article number: 11227 ( 2024 ) Cite this article
244 Accesses
1 Altmetric
Metrics details
- Acute kidney injury
- Bacterial infection
- Clostridium difficile
- Outcomes research
- Risk factors
In this study, we sought to evaluate the influence of positive pathogens in stool (PPS) on clinical outcomes in critical ill patients with Sepsis-associated acute kidney injury (S-AKI) from intensive care unit. Our sample consisted of 7338 patients, of whom 752 (10.25%) had PPS. We found that the presence of Clostridium difficile ( C. difficile ) and protists in stool samples was correlated with survival during hospitalization, as well as 30-day and 90-day survival. Interestingly, there was no significant difference in overall survival and 30-day in-hospital survival between the PPS group and the negative pathogens in stool (NPS) control group. However, the cumulative incidence of 90-day infection-related mortality was significantly higher in the PPS group (53 vs. 48%, P = 0.022), particularly in patients with C. difficile in their stool specimens. After adjusting for propensity scores, the results also have statistical significance. These findings suggest that PPS may affect the 90-days survival outcomes of S-AKI, particularly in patients with C. difficile and protists in their stool samples. Further research is warranted to further explore these associations.
Similar content being viewed by others

Significance of Clostridium difficile in community-acquired diarrhea in a tertiary care center in Lebanon
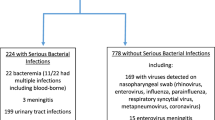
Febrile infants risk score at triage (FIRST) for the early identification of serious bacterial infections
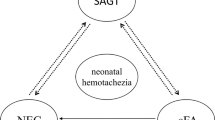
Clinical significance of positive fecal occult blood test in neonates
Introduction.
Sepsis association acute kidney injury (AKI) is a multifactorial clinical syndrome and a serious complication in critically ill patients 1 , 2 , 3 . It is associated with high mortality and increased healthcare costs. The mortality rate among AKI patients receiving renal replacement therapy (RRT) exceeds 50%, and the pathogenesis of this condition is not fully understood 4 , 5 . Sepsis is the leading cause of AKI in the intensive care unit (ICU). It can result from the destruction of the patient's mucosal barrier, an imbalance in the intestinal flora, the use of antibiotics and a lack of intestinal nutrition 6 , 7 . These factors can induce inflammatory responses that compromise host metabolism and immunity and promote the development of AKI. Several studies have demonstrated an association between gut microbiota and kidney injury, suggesting a close link between the kidneys and the gastrointestinal tract, known as the gut-kidney axis 8 , 9 , 10 . Dysregulated interactions between the intestinal epithelium, immune system, endogenous pathogens and kidneys may exacerbate systemic inflammation and AKI. Therefore, the relationship between stool pathogens and sepsis association AKI is of great importance. It can guide the subsequent treatment of patients with kidney disease and help reverse gastrointestinal dysfunction.
The gut-kidney axis has received considerable attention in recent years due to the intriguing interactions between these two organ systems. Acute kidney damage can be caused by pathogens in the intestines 11 . Several studies have investigated the impact of the stool pathogens on the progression of sepsis association AKI to chronic kidney disease (CKD) 12 , 13 , 14 , 15 . Given that most patients with sepsis are routinely treated with antibiotics, this could potentially lead to an imbalance in the intestinal flora and result in dynamic changes in the bacterial flora. A positive pathogen in stool (PPS) may reflect the dominant microorganisms in the gastrointestinal tract. The stool pathogens of patients with treated sepsis association AKI may be altered during transplantation due to intensive conditioning and administration of therapeutic antibiotics 16 .
However, the role of stool pathogens in sepsis-associated AKI has not been established by studies. The aim of this retrospective study was to determine the impact of stool pathogens on clinical outcomes in patients with sepsis-associated AKI. To control for confounders, we also performed propensity score matching (PSM) and causal inference for the analysis.
Results of stool cultures
A total of 27,234 stool samples were collected from 7338 patients. A PPS was observed in 752 patients (10.25%), whose age, weight, and sex were comparable to those of the persistently negative pathogens in stool (NPS) group (Table 1 ). The most common pathogen identified in stool samples was C. difficile , followed by Adenovirus , Enterococcus sp. , and Salmonella species (Table 2 ). One patient’s staphylococcal sample contained Methicillin-resistant Staphylococcus . The most common virus identified from stool samples was Adenovirus , while the most common Enterobacteriaceae was Salmonella . Multiple pathogens (≥ 2) were detected in 4 patients.
The impact of PPS on sepsis AKI
There was no statistical difference between the SOFA and GCS scores, indicating a similar level of critical illness in both groups of patients (Table 1 , P > 0.05). The median length of hospital stays for the NPS and PPS groups was 17 days (range: 10–29) and 22 days (range: 12–35), respectively (Table 1 , P < 0.001). In-hospital mortality was calculated using the chi-squared test and showed a statistically significant difference (Table 1 , P = 0.04). The mortality rate for patients with a positive stool test was 28% compared to 25% for patients with a negative stool test. There was no statistically significant difference observed between the white blood cells (WBC) and platelets (Table 1 , P > 0.05). There was also no statistical difference in the number of using RRT treatment between the two groups (Table 1 , P > 0.05). Regarding the sources of infectious pathogens, there are certain statistical differences between gram-negative bacteria and gram-positive bacteria. There were statistical differences in the source of infection, but most of the infections in the two groups occurred in the respiratory and blood systems (Table 1 , P < 0.05). There was a statistically significant difference in the time it took to test for fecal pathogens between the two groups, with the PPS group taking an average of 6 days and the NPS group taking an average of 8 days (Table 1 , P < 0.001).
Impact of PPS on 90-Day survival, hospital survival, and 30-day survival
The KM curves demonstrate that mortality in patients with sepsis-associated AKI was similar between the NPS (48.45%) and PPS (52.93%) groups ( P = 0.01; Fig. 1 A). The 30-day cumulative mortality rate in the PPS group was 30.85%, which was higher than the 28.35% in the NPS group ( P = 0.16; Fig. 1 B). The probability of death during hospitalization in the PPS group (42.25%) was slightly higher than in the NPS group (43.69%; P = 0.21; Fig. 1 C). The KM curves after PSM of hospital survival, 30-day survival, and 90-day survival are presented in Fig. 1 D–F. Differences between the two groups after PSM did not alter our results. Enterobacteriaceae and Enterococci in stool samples were not associated with patient survival during hospitalization or with 30-day and 90-day survival. However, the presence of C. difficile in stool samples was significantly associated with decreased 90 days survival (Fig. 2 A, B , Table 3 ). We performed causal inference of C. difficile positivity and 90-day prognosis, removed confounders, and drew a DAG diagram (Supplementary Fig. 1 ). After PSM analysis, the average treatment effect was 0.05 (95% CI 0.01–0.08, P < 0.05). This means that patients who develop C. difficile stool positivity have a 5% lower 90-day survival rate than those whose stool without C. difficile .
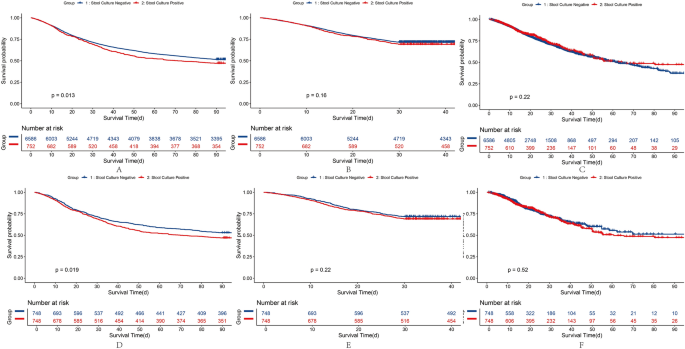
KM Curve and after-PSM KM Curve Plot of sepsis-associated AKI patients on the basis of the results of stool cultures. A, D, 90 days survival of raw data and after PSM; B, E,30 days survival of raw data and after PSM; C, F, Hospital Survival of raw data and after PSM.
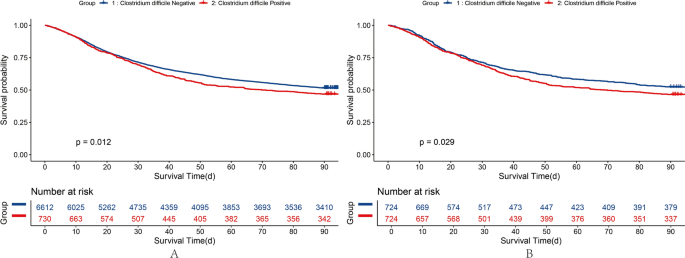
KM Curve of raw data and after PSM of 90 days Survival of sepsis-associated AKI patients on the basis of the results of C. difficile .
Variables that showed differences in 90-day patient survival were analyzed using single-factor Cox analysis with stool culture. Supplementary Table 1 demonstrates all variables initially considered in the forward stepwise model. The correlation between these factors and survival was statistically significant (Table 4 ).
Because treatment is already in using at the time of stool examination, antibiotics have an effect on fecal pathogens. The use of antibiotics was described in both groups (Table 5 ). In the group with positive pathogenic bacteria, vancomycin was used more frequently, with about 88% of patients using it, and there was a statistical difference between the two groups. Similarly, metronidazole was used more often in the PPS group and less often in the NPS group ( P < 0.001). Penicillin/cephalosporin and quinolones were used less in the PPS group than in the NPS group ( P < 0.05). There was no statistical significance in the antibiotic use of macrolides, sulfonamides, tetracyclines and aminoglycosides between the two groups ( P > 0.05).
In this study, we observed that the presence of C. difficile protists in stool samples was associated with a higher risk of in-hospital death, 30-day death, and 90-day death in patients with sepsis-associated AKI. PPS is associated with poor survival, particularly in those with C. difficile in their stool samples. To our knowledge, this study provides the first opportunity to investigate the exploratory role of PPS in patients with sepsis-associated AKI.
In this study, we observed that the presence of C. difficile and protists in stool samples significantly increased the risk of 90-day mortality. Protists were associated with worse in-hospital and 30-day survival. Multiple stool tests are necessary to avoid false negatives, so we did not include confounders in the number of tests 17 . Taking into account prolonged hospitalization with multiple fecal examinations, a worse 90-day survival was observed in PPS patients after propensity score matching to adjust for the possible influence of confounders.
C. difficile and protists in stool samples is associated with sepsis association AKI, and infections, and dysbiosis may negatively impact their survival 18 , 19 . This phenomenon also is due to increased levels of inflammatory cytokines and the fact that ischemia can lead to changes in the constituent organisms that make up the intestinal pathogens 20 , 21 . Sepsis can lead to a significant loss of microbial diversity in patients 22 . In our study, patients with elevated absolute neutrophil counts had lower survival rates than patients with decreased absolute neutrophil counts. Gastrointestinal colonization with C. difficile may be a potential risk factor for invasive fungal in sepsis-associated AKI patients. However, common dysbiosis pathogenic bacterial infections were not found in this study, like candidiasis. Therefore, patients with sepsis-associated AKI should be monitored for the presence of C. difficile in stool samples.
Patients whose stool samples contained C. difficile had worse survival outcomes than those with persistently NPS. In patients with sepsis-associated AKI, those whose intestines are colonized with antibiotic-resistant bacteria are likely to develop bacteremia. Inhibiting the intestinal flora can significantly reduce the incidence of bacteremia. High concentrations of C. difficile bacteria in feces can result in a high risk of bacterial translocation into the bloodstream. Microbial abundance and diversity in hemodialysis patients are on a downward trend. In addition, the mortality rate of sepsis-associated AKI patients undergoing further kidney replacement therapy is high, reaching up to 50% 23 . Broad-spectrum antibiotics increase the risk of Clostridium difficile infection. Therefore, reducing the use of high-risk antibacterial drugs associated with Clostridium difficile infection (such as second- and third-generation broad-spectrum cephalosporins, fluoroquinolones, clindamycin, etc.), as well as their frequency and duration, may improve patient clinical outcomes 24 , 25 .
Protists organisms constitute a heterogeneous category of eukaryotic microbes that bear a potential association with heightened mortality in individuals suffering from sepsis-related acute kidney injury 26 . The poor prognosis may be due to the severe immune stress caused by protists 27 . For instance, protozoan infections can lead to endothelial dysfunction, hinder perturb the integrity of the glycocalyx layer as well as foster platelet aggregation and the initiation of coagulation cascades culminating in fibrin deposition 28 . Our results resonate with prior studies, affirming the contributory role of protists in augmenting patient fatality rates.
In the ICU, patients with sepsis association AKI have lower long-term 90-day survival. Our analysis showed that patients who were positive pathogens in stool had lower 90-day survival rates. This is somewhat related to treatment. Vancomycin, a potent antibiotic that primarily targets Gram-positive bacteria, is particularly effective against methicillin-resistant Staphylococcus aureus (MRSA) and other drug-resistant staphylococci 29 . In the ICU, where patients with sepsis have PPS, the increased use of vancomycin may be indicative of efforts to control C. difficile infections rather than association with sepsis-associated acute kidney injury (AKI). In addition, our multivariate regression showed that it was associated with 90-day survival and also associated with quinolones. Quinolones are used less frequently in patients with positive stools, which may indicate the need for caution in clinical use. The source of the fungal infection also has a negative impact on the patient's prognosis for 90-day survival. Due to the patient's compromised immune function, it is difficult for the host to eliminate fungi, toxins, and metabolites 30 , 31 . The antibiotics used have a certain nephrotoxicity, resulting in a worse 90-day prognosis than in normal patients.
In the context of clinical implications, the detection of C. difficile and protists in stool samples, which are associated with adverse survival outcomes, indicates a recommendation for routine fecal microbiota screening in these patients, particularly during the early phase of hospitalization. Enhanced testing frequency minimizes false-negative results, enabling prompt identification and intervention in cases of potential microbial imbalance. In light of the observed association between antibiotic use and PPS, clinicians may wish to exercise caution when prescribing second- and third- generation cephalosporins, fluoroquinolones, clindamycin, and other high-risk antibiotics. It may be advisable to consider shorter courses where feasible. Treatment strategies should prioritize alternatives with reduced nephrotoxicity to safeguard both renal function and the integrity of the gut microbiome. Maintaining microbial diversity and gut microbiome stability is vital to prevent bacterial translocation and secondary infections. Moreover, it is imperative to conduct large-scale, prospective, multi-center studies to validate these observations and elucidate the correlations between gut microbiota and clinical outcomes across diverse populations. These endeavors will facilitate the development of more precise therapeutic guidelines, tailored to the unique microbial profiles of patients with sepsis-associated acute kidney injury.
A limitation of this study is its retrospective design coupled with a relatively small sample of bacterial species in the PPS group. Therefore, the role of microorganisms in fecal samples from patients with sepsis-associated AKI warrants further investigation. Second, this study was conducted with patients in the United States, where bacterial prevalence may vary. This variability could potentially influence the association between fecal microorganisms and clinical outcomes in other populations. Third, retrospective studies may have inherent flaws. For example, selection bias, included patients mainly collected stool after treatment, and treatment caused different causes of stool. Prospective studies are needed to investigate the correlation between the presence of stool pathogens at admission and prognosis.
In conclusion, our results suggest that PPS significantly influence the 90 days survival outcomes of patients with sepsis association AKI, particularly those with C. difficile in their stool samples. Depending on the differential detection of positive and negative antibiotics in stool samples, clinicians may find it beneficial to adjust their antimicrobial therapy regimens accordingly.
This study conducted a retrospective analysis of the MIMIC database, a comprehensive resource for intensive care patients 32 . The MIMIC database was established in 2003 through a collaborative effort involving the Beth Israel Deaconess Medical Center (BIDMC), MIT, the National Institutes of Health, Massachusetts General Hospital, emergency physicians, critical care physicians, computer scientists, and other critical care professionals. The current version, MIMIC-IV, includes patients admitted between 2008 and 2019. Prior to utilizing the database, our team completed the necessary training and obtained the appropriate certifications. As this project does not impact clinical care, all sensitive health information remains anonymous, thereby eliminating the need for individual patient consent. We followed the official MIMIC-IV tutorial to construct the research database using PostgreSQL (version 10.0, PostgreSQL Global Development Group). We extracted data from patients with sepsis-associated AKI who underwent comprehensive stool culture analysis for all bacteria and fungi in the gastrointestinal tract. The exclusion criteria for this study are as follows:1) Patients who are younger than 18 years old; 2) Patients with missing AKI diagnostic information, creatinine value, or urine volume. 3) There is a lack of information regarding the diagnosis of sepsis, and there is no record of the SOFA score or infection information. 4) There are no records of stool or digital rectal examinations for the patients. A total of 7338 patients were included (Table 1 ). Figure 3 a shows the patient screening flowchart, while Fig. 3 b illustrates various stool pathogen tests.
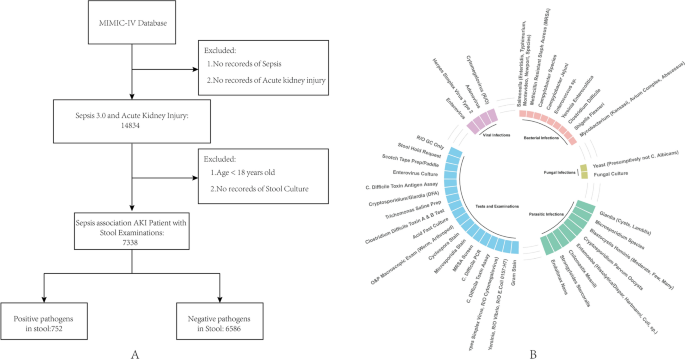
Cohort flow diagram. A, MIMIC-IV. B, Various stool pathogen tests.
Definitions and assessments
Patients with sepsis were selected and diagnosed according to the Third International Consensus Definitions for Sepsis and Septic Shock (Sepsis-3) 33 . In addition, we compiled additional codes as recommended by MIMIC-IV. Acute kidney injury (AKI) was defined according to the Kidney Disease: Improving Global Outcomes (KDIGO) criteria, which specify that AKI is either an increase of 0.3 mg/dl within 48 h or a 50% increase over baseline serum creatinine (sCr) 34 . The primary endpoint was 90-day survival. Secondary endpoints included in-hospital death and 30-day survival. The definition of positive pathogens in stool, during this ICU treatment, positive pathogens found in stool, digital rectal examination, and fecal swab were defined as positive pathogens in stool.
Statistical analysis
Missing values were imputed multiple times using the 'mice' package (version 3.15.0) in R. Baseline variables were compared using independent samples t-tests or Mann–Whitney U tests as appropriate. Survival probabilities were estimated using the Kaplan–Meier (KM) method. Potential prognostic factors were assessed by multivariate analysis using Cox proportional hazards regression and stepwise model selection methods. To illustrate the relationship between these three variables, we used a Directed Acyclic Graph (DAG). Because there were multiple stool tests for a long hospital stay and only one test for a short hospital stay, we considered length of treatment in hospital as a confounding factor and performed propensity score matching. Propensity scores were then used to control for confounding by R package ‘MatchIt’. The average treatment effect (ATE) was calculated using inverse probability weighting in this study. The multivariate Cox proportional hazards model included variables with statistically significant differences in individual factors. The ATE represents the causal effect of the variable of interest on patient 90 days causal inference radio in both the death and non-death study populations.
Independent variables with P > 0.05 were sequentially excluded from the model, while independent variables with P < 0.05 were considered statistically significant. The 'CBCgrp' package (version 2.8.2) in R (version 4.1.2) was used to calculate data 35 . All reported P -values are based on two-tailed hypothesis testing. Data analysis was primarily performed using competing risk analysis in the R package (version 4.1.2).
Data availability
The data is open source and freely available at https://www.physionet.org/content/mimiciv/2.2 .
Mandelbaum, T. et al. Outcome of critically ill patients with acute kidney injury using the acute kidney injury network criteria*. Crit. Care Med. 39 , 2659 (2011).
Article PubMed PubMed Central Google Scholar
Kaddourah, A., Basu, R. K., Bagshaw, S. M. & Goldstein, S. L. Epidemiology of acute kidney injury in critically Ill children and young adults. N. Engl. J. Med. 376 , 11–20. https://doi.org/10.1056/NEJMoa1611391 (2017).
Article PubMed Google Scholar
Vijayan, A. et al. Recovery after critical illness and acute kidney injury. Clin. J. Am. Soc. Nephrol. 16 , 1601–1609. https://doi.org/10.2215/cjn.19601220 (2021).
Article CAS PubMed PubMed Central Google Scholar
Fuhrman, D. Y., Kane-Gill, S., Goldstein, S. L., Priyanka, P. & Kellum, J. A. Acute kidney injury epidemiology, risk factors, and outcomes in critically ill patients 16–25 years of age treated in an adult intensive care unit. Ann. Intensive Care 8 , 26. https://doi.org/10.1186/s13613-018-0373-y (2018).
Barbar, S. D. et al. Timing of renal-replacement therapy in patients with acute kidney injury and sepsis. N. Engl. J. Med. 379 , 1431–1442. https://doi.org/10.1056/NEJMoa1803213 (2018).
Article CAS PubMed Google Scholar
Zhang, J. et al. Gut-kidney crosstalk in septic acute kidney injury. Crit. Care 22 , 117. https://doi.org/10.1186/s13054-018-2040-y (2018).
Sun, T., Wang, L. & Zhang, H. Intestinal microbiota in sepsis. Intensive Care Res. 2 , 1–7. https://doi.org/10.1007/s44231-022-00001-8 (2022).
Article CAS Google Scholar
Saranya, G. R. & Viswanathan, P. Gut microbiota dysbiosis in AKI to CKD transition. Biomed. Pharmacother. 161 , 114447. https://doi.org/10.1016/j.biopha.2023.114447 (2023).
Kobayashi, T., Iwata, Y., Nakade, Y. & Wada, T. Significance of the gut microbiota in acute kidney injury. Toxins https://doi.org/10.3390/toxins13060369 (2021).
Lei, J., Xie, Y., Sheng, J. & Song, J. Intestinal microbiota dysbiosis in acute kidney injury: novel insights into mechanisms and promising therapeutic strategies. Ren. Fail. 44 , 571–580. https://doi.org/10.1080/0886022X.2022.2056054 (2022).
Saithong, S. et al. Candida administration worsens neutrophil extracellular traps in renal ischemia reperfusion Injury mice: An impact of gut fungi on acute kidney injury. J. Innate Immun. 14 , 502–517. https://doi.org/10.1159/000521633 (2022).
Bäumler, A. J. & Sperandio, V. Interactions between the microbiota and pathogenic bacteria in the gut. Nature 535 , 85–93. https://doi.org/10.1038/nature18849 (2016).
Article ADS CAS PubMed PubMed Central Google Scholar
Rysz, J. et al. The impact of CKD on uremic toxins and gut microbiota. Toxins 13 , 252 (2021).
Hauser, A. B., Stinghen, A. E. M., Gonçalves, S. M., Bucharles, S. & Pecoits-Filho, R. A gut feeling on endotoxemia: Causes and consequences in chronic kidney disease. Nephron. Clin. Pract. 118 , c165–c172. https://doi.org/10.1159/000321438 (2010).
Chen, J.-H. et al. Early elimination of uremic toxin ameliorates AKI-to-CKD transition. Clin. Sci. 135 , 2643–2658. https://doi.org/10.1042/cs20210858 (2021).
Gharaie, S. et al. Microbiome modulation after severe acute kidney injury accelerates functional recovery and decreases kidney fibrosis. Kidney Int. 104 , 470–491. https://doi.org/10.1016/j.kint.2023.03.024 (2023).
Murad, Y. M. et al. False negative results in clostridium difficile testing. BMC Infect. Dis. 16 , 430. https://doi.org/10.1186/s12879-016-1741-6 (2016).
Peterson, C. T., Sharma, V., Elmén, L. & Peterson, S. N. Immune homeostasis, dysbiosis and therapeutic modulation of the gut microbiota. Clin. Exp. Immunol. 179 , 363–377. https://doi.org/10.1111/cei.12474 (2015).
Qin, X., Caputo, F. J., Xu, D. Z. & Deitch, E. A. Hydrophobicity of mucosal surface and its relationship to gut barrier function. Shock 29 , 372–376. https://doi.org/10.1097/shk.0b013e3181453f4e (2008).
Chung, S., Barnes, J. L. & Astroth, K. S. Gastrointestinal microbiota in patients with chronic kidney disease: A systematic review. Adv. Nutr. 10 , 888–901. https://doi.org/10.1093/advances/nmz028 (2019).
Mahmoodpoor, F., Rahbar Saadat, Y., Barzegari, A., Ardalan, M. & Zununi Vahed, S. The impact of gut microbiota on kidney function and pathogenesis. Biomed. Pharmacother. 93 , 412–419. https://doi.org/10.1016/j.biopha.2017.06.066 (2017).
Adelman, M. W. et al. The gut microbiome’s role in the development, maintenance, and outcomes of sepsis. Crit. Care 24 , 278. https://doi.org/10.1186/s13054-020-02989-1 (2020).
Luo, D. et al. The effects of hemodialysis and peritoneal dialysis on the gut microbiota of end-stage renal disease patients, and the relationship between gut microbiota and patient prognoses. Front. Cell Infect. Microbiol. 11 , 579386. https://doi.org/10.3389/fcimb.2021.579386 (2021).
LaPlante, K. L., Dhand, A., Wright, K. & Lauterio, M. Re-establishing the utility of tetracycline-class antibiotics for current challenges with antibiotic resistance. Ann. Med. 54 , 1686–1700. https://doi.org/10.1080/07853890.2022.2085881 (2022).
Talpaert, M. J., Gopal Rao, G., Cooper, B. S. & Wade, P. Impact of guidelines and enhanced antibiotic stewardship on reducing broad-spectrum antibiotic usage and its effect on incidence of Clostridium difficile infection. J. Antimicrob. Chemother. 66 , 2168–2174. https://doi.org/10.1093/jac/dkr253 (2011).
Liu, J., Xie, H., Ye, Z., Li, F. & Wang, L. Rates, predictors, and mortality of sepsis-associated acute kidney injury: A systematic review and meta-analysis. BMC Nephrol. 21 , 318. https://doi.org/10.1186/s12882-020-01974-8 (2020).
Ishikawa, K. Severe acute kidney injury associated with giardia lamblia infection. Am. J. Trop. Med. Hyg. 109 , 4–5. https://doi.org/10.4269/ajtmh.23-0075 (2023).
He, F.-F. et al. Sepsis-induced AKI: From pathogenesis to therapeutic approaches. Front. Pharmacol. https://doi.org/10.3389/fphar.2022.981578 (2022).
Stevens, D. L. The role of vancomycin in the treatment paradigm. Clin. Infect. Dis. 42 , S51–S57 (2006).
Delaloye, J. & Calandra, T. Invasive candidiasis as a cause of sepsis in the critically ill patient. Virulence 5 , 161–169. https://doi.org/10.4161/viru.26187 (2014).
Freitas, C. G. & Felipe, M. S. Candida albicans and antifungal peptides. Infect. Dis. Ther. 12 , 2631–2648. https://doi.org/10.1007/s40121-023-00889-9 (2023).
Johnson, A. E. W. et al. MIMIC-IV, a freely accessible electronic health record dataset. Sci. Data 10 , 1. https://doi.org/10.1038/s41597-022-01899-x (2023).
Shankar-Hari, M. et al. Developing a new definition and assessing new clinical criteria for septic shock: For the third international consensus definitions for sepsis and septic shock (Sepsis-3). Jama 315 , 775–787. https://doi.org/10.1001/jama.2016.0289 (2016).
Kellum, J. A. et al. Kidney disease: improving global outcomes (KDIGO) acute kidney injury work group. KDIGO clinical practice guideline for acute kidney injury. Kidney Int. Suppl. 2 , 1–138 (2012).
Google Scholar
Zhang, Z., Gayle, A. A., Wang, J., Zhang, H. & Cardinal-Fernandez, P. Comparing baseline characteristics between groups: An introduction to the CBCgrps package. Ann. Transl. Med. 5 , 484 (2017).
Download references
Acknowledgements
The patients participating in the study are acknowledged for their cooperation.
Author information
Authors and affiliations.
Department of Forensic Medicine, School of Basic Medical Sciences, Central South University, No 172. Tongzipo Road, Changsha, 410013, Hunan, People’s Republic of China
Yaoyuan Cao
National Clinical Research Center for Geriatric Disorders, Xiangya Hospital, Central South University, Changsha, 410008, China
Fuxing Deng
You can also search for this author in PubMed Google Scholar
Contributions
Yaoyuan Cao: Statistical analysis and writing the paper. Fuxing Deng: Study design, paper editing and overall study supervision.
Corresponding author
Correspondence to Fuxing Deng .
Ethics declarations
Competing interests.
The authors declare no competing interests.
Additional information
Publisher's note.
Springer Nature remains neutral with regard to jurisdictional claims in published maps and institutional affiliations.
Supplementary Information
Supplementary figure 1., supplementary table 1., rights and permissions.
Open Access This article is licensed under a Creative Commons Attribution 4.0 International License, which permits use, sharing, adaptation, distribution and reproduction in any medium or format, as long as you give appropriate credit to the original author(s) and the source, provide a link to the Creative Commons licence, and indicate if changes were made. The images or other third party material in this article are included in the article's Creative Commons licence, unless indicated otherwise in a credit line to the material. If material is not included in the article's Creative Commons licence and your intended use is not permitted by statutory regulation or exceeds the permitted use, you will need to obtain permission directly from the copyright holder. To view a copy of this licence, visit http://creativecommons.org/licenses/by/4.0/ .
Reprints and permissions
About this article
Cite this article.
Cao, Y., Deng, F. Positive pathogens in stool could predict the clinical outcomes of sepsis-associated acute kidney injury in critical ill patient. Sci Rep 14 , 11227 (2024). https://doi.org/10.1038/s41598-024-62136-6
Download citation
Received : 29 December 2023
Accepted : 14 May 2024
Published : 16 May 2024
DOI : https://doi.org/10.1038/s41598-024-62136-6
Share this article
Anyone you share the following link with will be able to read this content:
Sorry, a shareable link is not currently available for this article.
Provided by the Springer Nature SharedIt content-sharing initiative
- Stool pathogens
By submitting a comment you agree to abide by our Terms and Community Guidelines . If you find something abusive or that does not comply with our terms or guidelines please flag it as inappropriate.
Quick links
- Explore articles by subject
- Guide to authors
- Editorial policies
Sign up for the Nature Briefing newsletter — what matters in science, free to your inbox daily.


An official website of the United States government
The .gov means it’s official. Federal government websites often end in .gov or .mil. Before sharing sensitive information, make sure you’re on a federal government site.
The site is secure. The https:// ensures that you are connecting to the official website and that any information you provide is encrypted and transmitted securely.
- Publications
- Account settings
Preview improvements coming to the PMC website in October 2024. Learn More or Try it out now .
- Advanced Search
- Journal List

Acute Kidney Injury: From Diagnosis to Prevention and Treatment Strategies
Acute kidney injury (AKI) is characterized by an acute decrease in renal function that can be multifactorial in its origin and is associated with complex pathophysiological mechanisms. In the short term, AKI is associated with an increased length of hospital stay, health care costs, and in-hospital mortality, and its impact extends into the long term, with AKI being associated with increased risks of cardiovascular events, progression to chronic kidney disease (CKD), and long-term mortality. Given the impact of the prognosis of AKI, it is important to recognize at-risk patients and improve preventive, diagnostic, and therapy strategies. The authors provide a comprehensive review on available diagnostic, preventive, and treatment strategies for AKI.
1. Introduction
Acute kidney injury (AKI) is a frequent diagnosis with an incidence that varies from 5.0% to 7.5% in hospitalized patients and that reaches up to 50–60% in critically ill patients [ 1 , 2 , 3 , 4 , 5 , 6 ]. AKI is characterized by an acute decrease in renal function that can be multifactorial in its origin and is associated with complex pathophysiological mechanisms [ 1 , 7 ].
In the short term, AKI is associated with an increased length of hospital stay, health care costs, and in-hospital mortality, and its impact extends into the long term, with AKI being associated with increased risks of cardiovascular events, progression to chronic kidney disease (CKD), and long-term mortality [ 8 ].
The incidence of AKI has increased in the past few decades, which might reflect the impact of the increased recognition of this diagnosis and improvements in patient care, namely through improvements in dialytic care, the availability of less nephrotoxic drugs, and a decrease in the use of dopamine and diuretics [ 1 , 9 ]. Mortality rates have declined in critically ill patients with AKI, but mortality rates are still significantly high and increase with AKI severity, specifically in dialysis-requiring AKI [ 6 , 9 , 10 , 11 ].
AKI survivors are at increased risk of developing CKD, which defined by the persistence of kidney disease for a period of more than 90 days [ 8 ]. Additionally, investigators now consider that AKI and CKD are part of a disease continuum instead of separate entities [ 8 ]. Indeed, the term acute kidney disease (AKD) has been recently proposed to define the continuing pathological processes and adverse events developing after AKI [ 12 ].
AKD is defined as an acute or subacute damage and/or a loss of kidney function for a duration of between 7 and 90 days after exposure to an AKI-initiating event [ 12 ]. This highlights the importance of renal recovery, as recovery within 48 h is typically associated with the rapid reversal of AKI, as well as the impact of AKD on pre-existing CKD in increasing the risk for kidney disease progression [ 12 ].
Given the impact of the prognosis of AKI, it is important to enhance the recognition of at-risk patients and to improve preventive, diagnostic, and therapeutic strategies. The authors provide a comprehensive literature review on available preventive and therapeutic strategies for AKI.
2. Diagnosis of AKI
The currently widespread AKI classification was developed by the Kidney Disease Improving Global Outcomes (KDIGO) work group in 2012 and defines AKI as an increase in the serum creatinine (SCr) level to at least 0.3 mg/dL within 48 h, an increase in SCr to more than 1.5 times the baseline (which is known or presumed to have occurred within the prior 7 days), or a urine output (UO) decrease to less than 0.5 mL/kg/h for 6 h [ 13 ]. This classification also stratifies different stages of AKI severity and provides criteria that could be applied in clinical activity and investigation [ 14 ] ( Table 1 ).
Kidney Disease Improving Global Outcomes (KDIGO) classification.
SCr: serum creatinine; UO: urine output.
The current definition relies on SCr and UO, which are imperfect markers with significant limitations, namely that these do not account for the duration or cause of AKI [ 15 ]. SCr is an insensitive marker because it is altered by factors affecting its production (age, gender, diet, muscle mass, and sepsis), dilution (fluid administration), elimination (previous renal dysfunction), and secretion (medications). Thus, SCr cannot be used as an accurate estimate of glomerular filtration rate (GFR) in the non-steady state, and it underestimates the degree of dysfunction due to reduced muscle mass, increased catabolism, or positive fluid balance in critical patients. Additionally, it often takes two-to-three days before SCr is elevated after a renal insult when renal injury occurs in the setting of appropriate renal reserve, meaning that other nephrons increase function to compensate for injured nephrons, and so SCr may not change despite actual structural damage [ 16 , 17 ].
Though UO is an early marker for AKI, it also relies on patient’s volemic and hemodynamic status and the use of diuretics, and so it is difficult to assess without a urinary catheter and its usefulness relies on an hourly assessment that is time consuming [ 15 , 17 , 18 ].
Recently, potential urinary and serum biomarkers of AKI have been identified, namely cystatin-C, neutrophil gelatinase associated lipocalin (NGAL), N-acetyl-glucosaminidase (NAG), kidney injury molecule 1 (KIM-1), interleukin-6 (IL-6), interleukin-8 (IL-8), interleukin 18 (IL-18), liver-type fatty acid-binding protein (L-FABP), calprotectin, urine angiotensinogen (AGT), urine microRNAs, insulin-like growth factor-binding protein 7 (IGFBP7), and tissue inhibitor of metalloproteinases-2 (TIMP-2) [ 19 , 20 , 21 , 22 , 23 , 24 , 25 , 26 , 27 , 28 , 29 ]. Both NGAL and IGFBP7 with TIMP-2 are the most promising markers that have been validated in multiple settings. However, their increased cost and a lack of substantial evidence of improvement of outcomes are important limitations for their widespread clinical use [ 30 , 31 ].
These markers reflect different stages of the pathophysiology of AKI, so, the use of a panel of several biomarkers covering different phases of this syndrome might provide a better early diagnostic tool for AKI, as well as providing targets for future treatments [ 27 , 28 , 30 , 32 , 33 , 34 , 35 ].
3. Risk factors for AKI
Both patient susceptibilities and exposures are risk factors for AKI [ 11 , 36 , 37 ] ( Table 2 ).
Risk factors for acute kidney injury (AKI).
ACEi: angiotensin-converting enzyme inhibitor; ARB: angiotensin receptor blocker; NSAID: nonsteroidal anti-inflammatory drugs.
Patient age is an important non-modifiable risk factor, as the loss of renal reserve and the physiologic decline of GFR may place older patients at risk for AKI [ 38 , 39 , 40 , 41 , 42 ].
CKD patients possess a loss of autoregulation, abnormal vasodilation, susceptibility to antihypertensive agents, and nephrotoxins, and the side effects of medication contribute to the development of AKI [ 42 ]. Moreover, AKI and CKD have been described as interconnected syndromes because AKI leads to the worsening of CKD and CKD predisposes one to AKI [ 42 , 43 ]. CKD also limits renal recovery after AKI [ 44 ].
Patient comorbidities such as diabetes mellitus, hypertension, cardiovascular disease, chronic liver disease, and chronic obstructive pulmonary disease have also been identified as important AKI predictors [ 11 , 13 , 36 , 37 , 40 , 45 , 46 ]. Given the increasing incidence of HIV-infected patients in past few decades, HIV infection is also a risk factor that predisposes patients to AKI [ 47 , 48 ].
Exposure to sepsis, surgery, nephrotoxins, and shock are specific modifiable factors that contribute to AKI [ 1 , 13 ]. Indeed, large cohort studies focusing on critically ill patients have reported that the two most important causes of AKI are sepsis and surgery [ 6 , 49 ].
Additionally, recent research has reported that other factors like hyperuricemia, hypoalbuminemia, obesity, anemia, and hyperglycemia have been associated with an increased risk of AKI [ 49 , 50 , 51 , 52 , 53 , 54 , 55 , 56 , 57 , 58 , 59 , 60 , 61 , 62 , 63 , 64 , 65 , 66 , 67 , 68 , 69 ].
4. Causes and Assessment of AKI
AKI is a complex syndrome involving numerous pathophysiological processes that include pre-renal AKI, acute tubular necrosis, acute interstitial nephritis, acute glomerular diseases, and acute obstructive nephropathy [ 54 ].
These causes can be systematized into three groups, namely prerenal AKI, which accounts for up to 60% of cases and results from the functional adaptation to hypoperfusion of structurally normal kidneys; intrinsic renal AKI, which results from structural damage to any component of the renal parenchyma and accounts for up to 40% of cases; and, less frequently, postrenal AKI, which results from urinary tract obstruction [ 55 , 56 ]. The causes are summarized in Table 3 .
Causes of AKI.
IgA: Immunoglobulin A.
Essentially, the majority of causes of AKI are actually not renal-specific because the kidneys are highly sensitive to any systemic upset [ 55 ]. Indeed, the most common causes being septic shock, post major surgery, cardiogenic shock, and hypovolemia highlight this fact [ 57 ].
Most cases are multifactorial, and, following the inciting event causing kidney injury, numerous pathophysiologic pathways occur, including hemodynamic instability, microcirculatory dysfunction, tubular cell injury, tubular obstruction, renal congestion, microvascular thrombi, endothelial dysfunction, and inflammation [ 55 , 56 , 58 , 59 , 60 ].
The assessment of the cause of AKI must include a careful history, including medications and exposures, as well as a thorough physical examination. The assessment of fluid status and the presence of signs and symptoms of acute or chronic heart failure, infection, and urinary tract obstruction must be included in a first approach [ 54 , 61 ].
Laboratory evaluation should comprise SCr, urea, electrolytes, complete blood count, liver function tests, glucose level, bone profile, urine analysis, and microscopic examination, and a renal ultrasound must be performed to exclude obstruction. Urine output should be measured. A chest x-ray can provide evidence of a potential cause, such as pneumonia or vasculitis, but it can also prove useful in volume overload evaluation [ 54 , 61 ].
Less frequent causes of AKI such as vasculitis, glomerulopathy, and hemolytic uremic syndrome should be considered in the presence of fever, rash, joint pains, pulmonary infiltrates, abnormal urine analysis, thrombocytopenia, and hemolytic anemia when significant dehydration, hypotension, nephrotoxins, and obstruction have been excluded [ 54 , 61 ]. Thus, the determination of the cause of AKI must be completed with an assessment of antineutrophil cytoplasmic antibodies (ANCA), anti-glomerular basement membrane antibodies (anti-GBM), antinuclear antibodies (ANA), anti-double stranded DNA (anti-dsDNA) antibodies, complement factors, rheumatoid factor, antistreptolysin O titer (ASOT), cryoglobulins, serum electrophoresis, immunoglobulins, serum free light chains, hepatitis, and HIV serology [ 54 , 61 ] ( Table 4 ).
Preventive and treatment strategies for AKI.
ANA: antinuclear antibodies; ANCA: antineutrophil cytoplasmic antibodies; anti-GBM: anti-glomerular basement membrane; anti-dsDNA: anti-double stranded DNA; ARB: angiotensin receptor blocker; ASOT: antistreptolysin O titer; CKD: chronic kidney disease; RRT: renal replacement therapy.
5. Treatment of AKI
The therapeutic strategies for AKI based on the KDIGO guidelines and bundles of care are limited and mostly supportive [ 13 ].
The clinical approach should begin by hemodynamic stabilization, the early identification of complications of AKI, the identification of its cause, and its treatment [ 62 ]. Hemodynamic stabilization is of critical significance because autoregulation mechanisms are impaired in AKI [ 63 ].
Particular attention should be given to medications with renal toxicity, which should be discontinued, and dose adjustment according to renal function to avoid underdosing or adverse effects [ 62 , 64 ]. Additionally, in septic patients, prompt initiation of antibiotics is crucial [ 65 ].
It is important to rapidly identify and treat other complications in the therapeutic approach for the patient with AKI, such as hyperkalemia, metabolic acidosis, anemia, and fluid overload [ 13 ].
During the course of AKI, it is also recommended to prevent infection and start stress-ulcer prophylaxis [ 13 ].
5.1. Fluid Therapy
Fluid balance should be individualized, although the optimal fluid to this effect is undetermined. The titration of fluids is complex and requires the careful monitoring of patient’s volemia [ 63 ]. Hypovolemia reduces renal blood flow, but AKI patients are also at risk for volume overload [ 55 ]. Furthermore, a positive fluid balance is independently associated with increased mortality in AKI patients and contributes to worse outcomes in critically ill patients [ 66 , 67 , 68 ]. Goal-directed therapy guided by the assessment of fluid responsiveness appears to be associated with better outcomes [ 69 , 70 ].
Different types of fluids have different mechanisms of action. While colloids, such as albumin or starches, rely on oncotic gradients and selectively expand the extracellular space, crystalloids—namely saline, Ringer’s lactate or PlasmaLyte—equilibrate across intravascular and extravascular spaces [ 63 , 71 ].
Albumin appears to be relatively safe, but a consistent survival advantage compared with crystalloids has not been demonstrated [ 72 , 73 , 74 ]. The Saline Versus Albumin Fluid Evaluation (SAFE) trial reported that there was no renal or mortality benefit in patients who received albumin, but less total volume was required for resuscitation, which could be attractive in reducing positive fluid balance [ 72 ]. Furthermore, in the Colloids Versus Crystalloids for the Resuscitation of the Critically Ill (CRISTAL) trial, there was a decrease in need for mechanical ventilation, the need for vasopressors, and the mortality at 90-days in patients who received colloids [ 75 ].
Thus, albumin may prove important when large volumes of fluids are anticipated. Indeed, the recent Surviving Sepsis Campaign recommendations state that when patients require substantial amounts of crystalloids, albumin might be additionally used [ 76 ]. Still, there are no data supporting the routine use of colloids for volume resuscitation [ 77 ].
The administration of albumin in combination with terlipressin is also beneficial in specific conditions, such as cirrhotic patients and cardiac surgery patients with hypoalbuminemia in which the preoperative administration of albumin reduces the risk of postoperative AKI [ 78 , 79 ]. Nevertheless, caution must be taken in patients with traumatic brain injury, in which albumin has been associated with an increased mortality risk and should be avoided [ 72 ]. Some concerns have been raised on the use of hyperoncotic albumin in septic shock patients who are volume depleted and have increased vascular permeability, as it might increase AKI risk by promoting intracellular dehydration to expand volume [ 80 , 81 ].
Large randomized trials have reported higher incidences of AKI in patients treated with starches, which appears to be due to osmotic nephrosis with proximal tubule vacuolization and swelling. [ 63 , 82 , 83 ] The use of starches should thus be avoided [ 82 ].
There are less data concerning the use of gelatins and AKI, though observational data suggest these might contribute to AKI due to osmotic nephrosis [ 84 ]. Therefore, the use of gelatins is also not recommended in AKI [ 13 , 63 ].
Saline is the most frequently used crystalloid in critically ill patients [ 63 , 85 ]. Nevertheless, the administration of large volumes can cause hyperchloremia and metabolic acidosis. Hyperchloremia can lead to renal vasoconstriction and a consequent reduction in glomerular filtration [ 71 , 86 ].
Studies comparing different types of crystalloids in critically ill patients at risk of AKI have demonstrated conflicting results [ 85 , 86 , 87 , 88 , 89 ]. Yunos reported that a chloride-restrictive strategy was associated with a significant decrease in the incidence of AKI in critically ill patients [ 88 ]. The Saline versus PlasmaLyte for intensive care unit (ICU) Fluid Therapy (SPLIT) trial did not find differences in the rates of AKI or mortality in a population of mostly postoperative patients who received modest volumes for resuscitation [ 90 , 91 ]. In the Isotonic Solutions and Major Adverse Renal Events Trial (SMART) and in the Saline against Lactated Ringer’s or PlasmaLyte in the Emergency Department (SALT-ED), trial there were reductions in adverse kidney outcomes within 30 days in patients who received buffered solutions [ 85 , 92 ]. Though it is theorized that AKI occurs due to hyperchloremia only when large volumes are administered, a recent study of critically ill patients receiving large volumes of fluid did not demonstrate an association between chloride load and AKI risk after adjusting for disease severity [ 93 ]. Thus, saline remains the preferred solution for volume resuscitation, though chloride concentrations should be monitored [ 13 , 55 , 62 ].
5.2. Vasopressor Drugs
After volume resuscitation, vasopressor support should be considered to maintain renal perfusion in order to avoid positive fluid balance. In patients with AKI, the median blood pressure target should be higher than 65 mmHg to ensure accurate renal perfusion [ 13 , 94 , 95 , 96 ].
In vasodilatory states, noradrenaline is the recommended first-line vasopressor [ 76 , 97 ]. Noradrenaline improves microcirculatory flow by increasing perfusion pressure above the autoregulation threshold in hypotensive patients, but in high doses, it can cause a decrease in the flow by excessive vasoconstriction [ 98 ]. Thus, the current recommendations suggest to administer the lowest dose to achieve the blood pressure target and keep adequate perfusion parameters [ 98 ].
Vasopressin and terlipressin are effective alternatives for raising blood pressure, though their benefit on kidney function or mortality comparing to noradrenaline has not been demonstrated [ 99 , 100 , 101 , 102 ]. Angiotensin II has shown promising results on patient outcomes in recent studies, namely by improving survival and renal function recovery [ 103 ]. Nevertheless, further studies are still required to recommend the routine use of angiotensin II.
In contrary to previous beliefs, there is no evidence for a “renal dose” dopamine in AKI management, because there is no association between dopamine use in AKI and improvement in survival or renal function [ 104 , 105 , 106 , 107 ]. Fenoldopam has a similar hemodynamic renal effect to that oe low-dose dopamine and had been demonstrated to decrease systemic vascular resistance whilst increasing renal blood flow to the kidney [ 108 , 109 ]. Despite promising studies, there has been no conclusive evidence of the beneficial effect of fenoldopam in the management of AKI, and further investigation is warranted [ 110 , 111 ].
5.3. Diuretics
The use of diuretics is only recommended to manage fluid overload and electrolyte disturbances in AKI [ 13 , 112 ]. Based on pathophysiology studies, it was previously thought that loop diuretics might protect the loop of Henle from ischemia by decreasing its workload [ 112 ]. This has never been confirmed, and, on the contrary, it has been demonstrated that furosemide is not associated with clinical benefits in preventing AKI, decreasing the need for renal replacement therapy (RRT), renal recovery, or decreasing in-hospital mortality [ 113 , 114 , 115 ]. It is important to note that certain studies have associated the use of loop diuretics with an increased risk of mortality, which might be related to the delay in appropriate RRT start [ 112 , 115 , 116 ]. Additionally, a loop diuretic used in high doses may cause ototoxicity [ 112 ]. Therefore, the KDIGO guidelines do not recommend the use of diuretics to prevent AKI [ 13 ].
5.4. Drug Nephrotoxicity
Drug nephrotoxicity has been associated with 20–40% of AKI causes and can reach up to 60% in elderly patients [ 117 , 118 ]. Patients with underlying AKI or CKD, sepsis, acute or chronic liver failure, acute or chronic heart failure, pulmonary hypertension, malignancies, and exposure surgery are at increased risk for drug-induced nephrotoxicity [ 117 ].
The mechanisms of drug-induced nephrotoxicity are diverse and vary with different drug classes. ( Table 3 ). Drugs can induce not only direct toxicity due to tubular injury, interstitial nephritis, glomerular injury, or obstructive nephropathy but also indirect nephrotoxicity associated with a decrease in renal blood flow [ 119 ]. Furthermore, drug-induced nephrotoxicity results from the combination of innate drug toxicity, altered renal hemodynamics, previous renal disease, and altered drug pharmacokinetics in critical illness [ 119 , 120 ].
Due to the associated reduced glomerular pressure, patients exposed to nonsteroidal anti-inflammatory drugs (NSAIDs), renin–angiotensin–aldosterone system blockers, high-dose systemic vasoconstrictors, or calcineurin inhibitors are at high-risk to develop prerenal AKI in the setting of altered systemic and renal hemodynamics or fluid loss; these also increase the risk for acute tubular injury [ 117 ].
Acute tubular necrosis (ATN) is dose-dependent and is the most common form of drug-induced AKI in the hospital setting [ 121 ]. The most common drugs associated with ATN are aminoglycosides, vancomycin, radiocontrast media, cisplatin, amphotericin B, foscarnet, and osmotically active agents [ 117 , 121 ].
Acute interstitial nephritis (AIN) causes up to 10% of AKI cases. AIN is an idiosyncratic reaction that is not dose-dependent [ 117 , 120 ]. Antimicrobials such as b-lactams, sulfa-based drugs and quinolones, anti-ulcer agents, anti-convulsants, and diuretics are the most common drugs associated with AIN [ 117 , 118 , 122 ].
Post-renal AKI due to crystal-induced luminal obstruction can occur in patients exposed to acyclovir, sulfa-based medications, ciprofloxacin, and methotrexate [ 121 ]. Less frequently, drug-induced glomerular disease can result from the administration of hydralazine, propylthiouracil, allopurinol, and penicillamine, all of which have been associated with development of ANCA vasculitis, mitomycin C, oral contraceptive agents, calcineurin inhibitors, antineoplastic agents, ticlopidine, and quinine, all of which can cause thrombotic microangiopathy [ 121 ].
Therefore, the prescription of drugs must be carefully considered to minimize toxicity. The KDIGO guidelines recommend the early discontinuation of potential nephrotoxic drugs, the avoidance of radiocontrast and other nephrotoxic drugs, and drug dose monitoring [ 13 ].
5.5. Other Therapeutic Strategies
Remote ischemic preconditioning is a technique that induces multiple short cycles of ischemia and reperfusion by cuff inflation [ 123 ]. This has been tested as a possible method to protect the kidneys from ischemia reperfusion injury, although there is conflicting evidence regarding the results in reducing AKI or mortality, and it is not recommended in clinical practice [ 124 , 125 , 126 , 127 , 128 ].
Levosimendan has both vasodilatory and inotropic actions [ 129 ]. It might improve kidney function by improving cardiac function but also through afferent arteriolar vasodilatation, though this benefit remains debatable [ 130 ]. In a recent meta-analysis, the use of levosimendan was associated with a decrease in AKI incidence and mortality in cardiac surgery patients, proving its potential use [ 131 ].
Several new potential therapeutic targets for AKI are currently being investigated and entering clinical trials. These include pathways involved in inflammation, fibrosis, mitochondrial function, oxidative stress, and hemodynamics. Though promising, further clinical trials are still required.
Reltecimod is a peptide antagonist of CD28 (co-stimulatory receptor) that acts as an immunomodulator and has been demonstrated to attenuate the systemic inflammatory response and decrease organ dysfunction in necrotizing soft tissue infections [ 132 , 133 ].
Due to the fact that active vitamin D has anti-proliferative and pro-differentiation actions, it might be that lower levels of vitamin D contribute to AKI [ 134 , 135 ]. Indeed, critically ill patients with vitamin D deficiencies have been reported to have higher rates of AKI and further progression to CKD [ 135 , 136 , 137 ]. Calcifediol and calcitriol are still under investigation as possible treatments in early AKI [ 138 , 139 , 140 ].
Alkaline phosphatase is an enzyme expressed along the proximal tubule and has the ability to reduce renal inflammation. The use of human recombinant alkaline phosphatase has been investigated in clinical trials as a potential anti-inflammatory drug that could attenuate kidney injury or promote renal regeneration [ 141 , 142 , 143 ]. A recent trial reported that, despite not affecting short-term kidney function, there was a long-term benefit in kidney function [ 144 ].
Teprasiran is under investigation as an apoptosis inhibitor and has had promising results in patients undergoing cardiac surgery at risk for AKI by demonstrating a reduction in major adverse kidney events at 90 days [ 145 , 146 ].
Intensive investigation in this area reflects the fact that, to date, there are no established pharmacotherapies for AKI. The most important measures to be applied in clinical practice remain the hemodynamic monitoring and administration of fluids and vasopressors, the eviction and avoidance of nephrotoxins, and the treatment of AKI complications ( Table 4 ).
5.6. Renal Replacement Therapy
Conventional criteria for initiation of RRT in AKI are anuria, severe/refractory hyperkalemia, severe/refractory metabolic acidosis, refractory volume overload, severe azotemia, or clinical complications of uremia such as encephalopathy, pericarditis, or neuropathy [ 147 , 148 ].
There are different modalities of RRT that can be provided in cases of severe AKI, namely intermittent hemodialysis (HD), continuous RRT (CRRT), slow low-efficiency dialysis (SLED), or peritoneal dialysis (PD) [ 149 ]. Continuous RRT (CRRT) is the most common form of renal support provided to critically ill patients because it provides better volume control and acid–base and electrolyte correction while maintaining hemodynamic stability [ 147 , 148 ]. Though there is no sustained evidence reporting a difference in mortality between the use DH, SLED, or CRRT in AKI patients, there is a tendency for earlier renal recovery and decreased progression to CKD with CRRT use [ 150 , 151 ]. The KDIGO guidelines recommend the use of CRRT and HD as complementary therapies, although CRRT should be favored in hemodynamically unstable patients and in patients with increased intracranial pressure [ 13 ]. Ultimately, the choice between RRT modalities relies on patient clinical status, resource availability, and local expertise [ 149 ].
RRT is essential to maintain volume, electrolyte, acid-base, and uremic solute homeostasis in AKI patients. It is also theorized that RRT can modulate inflammation, which might prove crucial in septic patients, though this remains uncertain [ 147 , 152 ]. Nonetheless, RRT requires central venous dialysis catheter insertion, the exposure of blood to an extracorporeal circuit, and anticoagulation, and it can be associated with hemodynamic instability, which may contribute to delayed kidney recovery [ 147 , 152 ].
The timing to start RRT remains controversial. According to the KDIGO guidelines, RRT should be started when life-threatening changes in fluid, electrolyte, and acid-base balances exist, and it is recommended to consider the broader clinical context and trends of laboratory values when making the decision to start RRT [ 13 ]. It is also non-consensual if RRT can change patient outcomes or is merely a surrogate for the critical illness on patient outcomes [ 153 , 154 , 155 , 156 ].
Recent randomized clinical trials have evaluated the optimal timing to start RRT in critically ill AKI patients. The heterogeneity in definitions of timing and criteria has contributed to lack of a strong recommendation [ 153 , 154 ].
The Early Versus Late Initiation of RRT (ELAIN) was a single-center trial of 231 critically ill, mostly surgical AKI patients. In this study, Zarbock et al. defined the early group as starting RRT within 8 h of fulfilling KDIGO stage 2 AKI and elevated plasma NGAL levels, and they defined the delayed group as starting RRT within 12 h of developing KDIGO stage 3 AKI or in the presence of an absolute indication [ 157 ]. The early RRT group was associated with 15% less mortality, greater RRT independence, and less hospitalization days than the delayed RRT group. However, the delayed group included 9% of patients who did not start RRT due to the recovery of kidney function [ 157 ].
In contrast, in the Artificial Kidney Initiation in Kidney Injury (AKIKI) multicenter trial of 620 critically ill AKI patients, an early RRT start did not decrease mortality, with no differences noted in hospital stays and renal recovery, and the delayed group had greater RRT-free days and fewer incidences of catheter-related infections [ 158 ]. In this study, early RRT was defined as starting RRT within 6 h of fulfilling KDIGO stage 3 AKI, and delayed RRT was defined as RRT start only in response to the development of absolute indications. Additionally, only 51% in the delayed group started RRT [ 158 ].
Considering the contrasting results of these studies, another multicenter trial was conducted in 488 patients with septic shock and severe AKI; this was entitled Initiation of Dialysis Early versus Late in the Intensive Care Unit (IDEAL-ICU) [ 159 ]. In this study, Barbar et al. defined the early group as RRT start within 12 h of achieving the failure stage of the RIFLE (Risk, Injury, Failure, Loss of kidney function, and End-stage kidney disease) classification without life-threatening AKI complications, and they defined the delayed group as starting RRT after a delay of 48 h of achieving the failure stage of the RIFLE classification. The results of the IDEAL-ICU trial were consistent with AKIKI and also demonstrated no significant difference in mortality between groups [ 159 ].
Only the ELAIN trial provided evidence that suggested the benefit of an early RRT start, which has not been verified in most recent studies. Several factors might have contributed to this conflicting evidence.
Firstly, the population studied, the AKI diagnostic criteria, and the definition of timing in each study were different. The ELAIN trial included a mixed ICU population but was mostly surgical patients, whereas the AKIKI and IDEAL trials included medical ICU patients with a higher proportion of sepsis. The ELAIN trial also included a significantly higher proportion of CKD patients (with a glomerular filtration rate of higher than 30 mL/min/1.73 m 2 ) than the AKIKI and IDEAL trials (41% vs. 10% and 15%, respectively). Patients enrolled in the AKIKI and IDEAL trials were at least stage 3 KDIGO or failure on the RIFLE classification, while this was only the case for patients in the delayed group in the ELAIN trial. Thus, the inclusion of less severe patients in the ELAIN trial might have beneficially influenced the outcome. Interestingly, patients in the ELAIN trial received delayed RRT at a significantly earlier point than the delayed group in the AKIKI and IDEAL trials (25.5 vs. 57 and 51.5 h, respectively).
Secondly, RRT modalities were different in these trials. All patients in the ELAIN trial were started on continuous RRT, and a combination of continuous and intermittent RRT techniques were prescribed in the AKIKI and IDEAL trials. Therefore, the disparities between RRT modalities might have influenced the hemodynamic assessment, treatment, and outcome of these patients.
Finally, a lower proportion of patients in the delayed group of the AKIKI and IDEAL trials initiated RRT than the delayed group of the ELAIN trial (51% and 62% vs. 91%, respectively). This suggests that a conservative approach to RRT start in response to persistent AKI or complications might be acceptable, as several patients with KDIGO stage 3 recovered renal function and ultimately did not require RRT.
The results of the ongoing Standard Versus Accelerated Initiation of Dialysis in Acute Kidney Injury (STARRT-AKI) and AKIKI 2 multicenter trials are highly anticipated in order to provide a more definitive answer to the problem of optimal timing for RRT start [ 160 , 161 ].
The timing of initiation has only been assessed in critically ill and the post-surgical settings, [ 162 , 163 ] and the inconsistent results have led to a perpetuation of the traditional recommendations of RRT start.
6. Prevention of AKI
In the absence of effective therapeutic interventions on established AKI and due to its significant on morbidity and mortality, we can only rely on AKI prevention and early diagnosis to reduce its incidence and detrimental consequences.
On the other hand, it could be argued that risk assessment is futile because it is unclear which interventions for high-risk patients should be implemented and whether these interventions are actually effective.
However, recent studies have suggested that the risk stratification of patients for AKI can allow for the employment of effective intervention and reduce the incidence of AKI, although there has been no evidence of benefit for long-term renal outcomes [ 164 , 165 , 166 ].
The recent Acute Disease Quality Initiative (ADQI) conference on “Quality Improvement for AKI” proposed that the range of care in AKI should be a continuum from risk assessment and prevention in the community setting, to AKI prevention in the hospital, to optimizing AKI management, and finally to the surveillance of AKD and the prevention of recurrent AKI and progression to CKD [ 167 ].
At least 50% of AKI episodes are believed to begin in the community setting, so health care professionals should identify at-risk patients ( Table 2 ) and implement preventive interventions to decrease the incidence of AKI [ 168 , 169 , 170 ].
High-risk patients for AKI should have a kidney health assessment (KHA) every 12 months, at least 30 days before exposure and two-to-three days after exposure that carries AKI risk in order to define and modify their risk profile [ 167 , 171 ]. The KHA must include AKI and CKD history, blood pressure assessment, SCr level, urine dipstick, and medication list [ 167 ].
After an acute exposure to an AKI inciting event, namely nephrotoxic medications, radiocontrast, surgery, or acute disease, medications should be adjusted, further nephrotoxic exposures should be minimized, and AKI occurrence and its complications should be monitored [ 167 ].
At hospital admission, patients should also be screened for AKI risk [ 13 , 169 , 172 ]. In high-risk patients, the early correction of modifiable risk factors should be considered in order to prevent AKI occurrence [ 64 , 167 , 173 ].
The gold-standard of AKI prevention remains the optimization of hemodynamic and volume status, medication review such as the cessation of angiotensin-converting enzyme inhibitor/angiotensin receptor blocker (ACEi/ARB) and metformin, and the minimization of nephrotoxic exposure [ 13 , 174 ].
7. Follow-Up after AKI
For patients with AKI, the main goal should be the recovery to baseline kidney function in the shortest period of time in order to reduce duration and disease severity, thus highlighting the role of early diagnosis and prompt management [ 62 , 167 , 175 ].
To date, there remains no standardized AKI or AKD follow-up care, but several studies have reported low rates of nephrology follow-up after AKI across different settings. [ 176 , 177 ]. In the United States Renal Data System annual report of 2015, only 19% of patients had a nephrology follow-up at 12 months after an AKI hospitalization [ 178 ]. In another study, only 4% of patients were referred to a nephrologist at three months and only 9% at one year, though the mortality rate during this period was 22% [ 179 ].
The benefit of nephrology referral is uncertain, however, recent studies have suggested that in high-risk patients, an early nephrology referral may improve survival. Indeed, Harel et al. reported that only 41% of AKI patients had a nephrology follow-up and that this was associated with a 24% mortality reduction in two years of follow-up [ 180 ].
Determining which patients are at higher risk for CKD development after AKI is crucial. Risk factors for CKD after AKI include the severity, duration, and recurrence of AKI; the timing of renal recovery; advanced age; lower baseline renal function; diabetes mellitus; hypertension; chronic heart failure; hypoalbuminemia; proteinuria; chronic liver disease; and a higher Charlson comorbidity index [ 181 ].
The KDIGO guidelines and the ADQI consensus recommend that after an episode of AKI, patients should be followed by a nephrologist at least three months after the episode in order to assess kidney recovery and/or progression to CKD or progressive CKD [ 13 , 167 ]. The follow-up evaluation should include kidney function and proteinuria to assess prognosis and outcome, medication reconciliation, patient education to nephrotoxic avoidance, and the employment of strategies to prevent CKD progression [ 167 ].
Further research is warranted to identify high-risk patients, define timing for nephrology follow-up, and to develop strategies to improve patient outcomes.
8. Conclusions
Currently, there are no established pharmacotherapies for AKI. Treatment strategies for AKI comprise hemodynamic stabilization, the eviction of nephrotoxins, and the treatment of AKI complications. The gold-standard of AKI prevention includes identifying at-risk patients, optimizing hemodynamic and volume statuses, reviewing medication, and minimizing nephrotoxic exposure. Considering the significant prognostic impact of AKI, it is crucial to focus further research on AKI prevention and therapy.
Author Contributions
The authors participated as follows: J.G. drafted the article, J.A.F. participated in the literature review of data, C.O. revised the article, and J.A.L. revised the article and approved the final version to be submitted for publication. All authors have read and agreed to the published version of the manuscript.
There was no funding for this study.
Conflicts of Interest
The authors declare no conflict of interest.
- Open access
- Published: 21 May 2024
Development and validation of a nomogram for predicting in-hospital death in cirrhotic patients with acute kidney injury
- Xiang Li 1 , 2 na1 ,
- Xunliang Li 1 na1 ,
- Wenman Zhao 1 &
- Deguang Wang 1
BMC Nephrology volume 25 , Article number: 175 ( 2024 ) Cite this article
21 Accesses
Metrics details
The purpose of this study was to develop a nomogram for predicting in-hospital mortality in cirrhotic patients with acute kidney injury (AKI) in order to identify patients with a high risk of in-hospital death early.
This study collected data on cirrhotic patients with AKI from 2008 to 2019 using the Medical Information Mart for Intensive Care IV. Multivariate logistic regression was used to identify confounding factors related to in-hospital mortality, which were then integrated into the nomogram. The concordance index (C-Index) was used to evaluate the accuracy of the model predictions. The area under the curve (AUC) and decision curve analysis (DCA) was used to assess the predictive performance and clinical utility of the nomogram.
The final study population included 886 cirrhotic patients with AKI, and 264 (29.8%) died in the hospital. After multivariate logistic regression, age, gender, cerebrovascular disease, heart rate, respiration rate, temperature, oxygen saturation, hemoglobin, blood urea nitrogen, serum creatinine, international normalized ratio, bilirubin, urine volume, and sequential organ failure assessment score were predictive factors of in-hospital mortality. In addition, the nomogram showed good accuracy in estimating the in-hospital mortality of patients. The calibration plots showed the best agreement with the actual presence of in-hospital mortality in patients. In addition, the AUC and DCA curves showed that the nomogram has good prediction accuracy and clinical value.
Conclusions
We have created a prognostic nomogram for predicting in-hospital death in cirrhotic patients with AKI, which may facilitate timely intervention to improve prognosis in these patients.
Peer Review reports
Introduction
Acute kidney injury (AKI) is a common and severe complication of cirrhosis, characterized by a rise in serum creatinine (Scr) levels or a decrease in urine output [ 1 , 2 ]. In some investigations, the incidence of AKI in patients with cirrhosis was between 20% and 50% [ 2 , 3 ]. In addition, some studies have recorded mortality rates as high as 80% for cirrhotic patients with AKI [ 4 , 5 ]. Therefore, identifying patients with cirrhosis and AKI at a higher risk of death is essential and may help improve the prognosis of these patients through the timely implementation of medicinal therapies.
Nomograms are straightforward statistical visualization tools that can estimate the likelihood of a particular result. Nomograms are currently utilized extensively for the diagnosis of diseases and the estimation of mortality [ 6 , 7 , 8 , 9 ]. However, nomograms have been utilized seldom to predict in-hospital mortality in cirrhotic patients with AKI. Consequently, this research aimed to evaluate the risk factors for in-hospital death in cirrhotic patients with AKI and to develop a nomogram to predict the risk of in-hospital mortality in these patients, with the ultimate goal of giving possible therapeutic guidance for early diagnosis and care in high-risk patients.
Database introduction
The Medical Information Mart for Intensive Care IV (MIMIC IV) database is an extensive, anonymous, publicly available clinical database authorized by the Massachusetts Institute of Technology [ 10 ]. The database records clinical data on patients in the intensive care unit (ICU) at Beth Israel Deaconess Medical Center between 2008 and 2019. Individual patient consent and ethically informed consent declarations are unnecessary because the database contains no identifying information about the patients and has no bearing on clinical decision-making [ 11 ]. In order to apply for access to the database, we were granted eligibility after passing the needed assessment.
Study population
This study included all cirrhotic patients with AKI in the ICU. The International Classification of Diseases, Ninth Revision (ICD-9) codes 5712, 5715, and 5716 were used to identify people with cirrhosis. AKI was diagnosed according to the Kidney Disease: Improving Global Outcomes (KDIGO) 2012 criteria within 48 h of ICU admission [ 12 ]: (1) Scr increased to more than 1.5 times the baseline value within the previous 7 days; (2) past 48 h Scr increased ≥ 0.3 mg/dl; or (3) urine output < 0.5 ml/kg/h for 6 h or more. The lowest Scr result within the preceding seven days was utilized as the baseline Scr level [ 13 ]. If baseline Scr values were unavailable before admission, the first Scr value recorded after admission was utilized [ 14 ]. Only the initial admission was evaluated in analyzing patients with multiple ICU admissions. Patients under the age of 18 and those with ICU stays of less than 48 h were excluded.
Data collection
We extracted patient information from the MIMIC IV database, including etiology of cirrhosis, age, gender, weight, comorbidities, vital signs, laboratory indicators, urine output, mechanical ventilation, renal replacement therapy (RRT), vasopressors use, disease severity score and mortality. Comorbidities included congestive heart failure, peptic ulcer disease, myocardial infarction, peripheral vascular disease, diabetes, chronic pulmonary disease, rheumatic disease, cerebrovascular disease, chronic kidney disease, cancer, paraplegia, and acquired immune deficiency syndrome, ascites, and portal encephalopathy. The employed vital signs included heart rate, mean arterial pressure (MAP), respiratory rate, temperature, and oxygen saturation (SpO 2 ), with the mean values from the initial 24 h after ICU admission used. For laboratory indicators, the maximum values during the first 24 h of ICU admission were used and included hematocrit, hemoglobin, platelets, white blood cell (WBC), blood urea nitrogen (BUN), anion gap, international normalized ratio (INR), Scr, serum glucose, serum calcium, serum chloride, bicarbonate, serum potassium, serum sodium, partial thromboplastin time (PTT), and prothrombin time (PT), albumin, and bilirubin. The disease severity score included sequential organ failure assessment (SOFA) score and simplified acute physiology score II (SAPS II). In addition, we used the total urine output of the patient during the first 24 h after admission to the ICU.
Statistical analysis
In the MIMIC IV database, missing data are widespread, and to minimize severe bias, less than 20% of variables were missing in this analysis. In this work, various interpolations using the ‘mice’ package of the R software were utilized to fill in missing data.
Due to their non-normal distribution, continuous variables in this study were reported as the median and interquartile range (IQR), and the Mann-Whitney test was employed to evaluate differences between groups. Categorical variables were reported as numbers and percentages, and the chi-square test or Fisher’s exact test was used, as appropriate, to compare groups. In the multivariate logistic analysis, we considered both statistically significant factors in the univariate analysis ( p < 0.05) and those that had previously been shown to have clinical relevance. The final logistic regression model was chosen by using backward stepwise regression. The collinearity between the final model variables was evaluated using the variance inflation factor (VIF), with VIF ≤ 5 indicating the absence of collinearity. Based on the findings of multivariate logistic regression on the dependent variable, a nomogram was generated using the ‘rms’ package of the R software. Consequently, odds ratios (OR) with 95% confidence intervals (95% CI) were calculated. The concordance index (C-Index) was used to evaluate the discriminatory ability of the model and decrease overfitting bias. We also used the area under curve (AUC) and decision curve analysis (DCA) to assess the predictive performance and clinical utility of the model. R software (version 4.2.1) was used to conduct statistical analyses. P-values < 0.05 were considered statistically significant.
Mortality and characteristics of cirrhotic patients with acute kidney injury
A total of 1911 cirrhotic patients with AKI who were eligible to participate were identified. 573 patients were eliminated because of multiple ICU admissions, and 695 were excluded due to ICU stays of less than 48 h. The final study population included 886 cirrhotic patients with AKI, and 264 (29.8%) died in the hospital (Fig. 1 ).
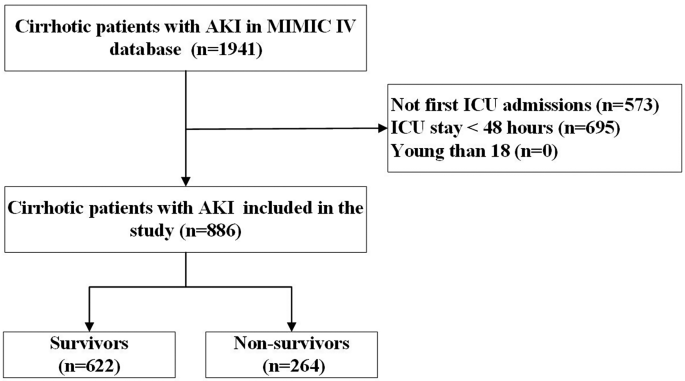
Study flowchart. Abbreviations MIMIC IV: Medical Information Mart for Intensive Care IV, ICU: intensive care unit, AKI: acute kidney injury
The incidence of cerebrovascular disease and sepsis was significantly higher in patients in the non-survivor group versus those in the survivor group ( p < 0.05). In addition, the non-survivor group had a higher heart rate, respiratory rate, vasopressors use, SOFA score, and SAPS II and a lower MAP, body temperature, SpO 2 , and urine output than the survivor group ( p < 0.05). For laboratory examinations, such as hemoglobin, platelets, WBC, anion gap, bicarbonate, BUN, serum calcium, serum chloride, Scr, INR, PT, and PTT were significantly different between the two groups ( P < 0.05). The essential aspects of the data collection are summarized in Table 1 .
Predictors for the death of cirrhotic patients with AKI
The univariate logistic regression analysis showed that cerebrovascular disease, sepsis, heart rate, MAP, respiratory rate, body temperature, SpO 2 , hemoglobin, platelets, WBC, anion gap, bicarbonate, BUN serum chloride, Scr, INR, PT, PTT, urine output, vasopressors use, SOFA score and SAPS II were associated with death in cirrhotic patients with AKI. To obtain the best predictors of mortality in patients with cirrhosis and AKI, we employed a multivariate logistic regression in which backward stepwise regression was used. The mean VIF of 1.58 for the multivariate logistic model indicates the direct absence of multicollinearity among variables. According to the OR in multivariate logistic regression, the high-risk factors of death were male (OR 1.728, CI 1.195-2.500; p < 0.05), age (OR 1.026, CI 1.010–1.043; p < 0.05), cerebrovascular disease (OR 5.662, CI 3.082–10.401; p < 0.05), heart rate (OR 1.023, CI 1.011–1.035; p < 0.05), respiratory rate (OR 1.082, CI 1.037–1.129; p < 0.05), BUN (OR 1.009, CI 1.001–1.017; p < 0.05), Scr (OR 1.197, CI 1.069–1.286; p < 0.05), INR (OR 1.297, CI 1.090–1.542; p < 0.05), bilirubin (OR 1.030, CI 1.010–1.049; p < 0.05) and SOFA score (OR 1.169, CI 1.113–1.228; p < 0.05). The OR for cerebrovascular disease in this study was 5.370, and patients with a history of cerebrovascular disease indicated were 5.370 times more likely to die in the hospital than those without a history of cerebrovascular disease. In addition, male patients were 1.982 times more likely to die in the hospital than female patients. On the other hand, body temperature (OR 0.507, CI 0.363–0.708; p < 0.05), SpO 2 (OR 0.862, CI 0.789–0.942; p < 0.05), hemoglobin (OR 0.868, CI 0.788–0.956; p < 0.05) and urine output (OR 1.000, CI 0.999-1.000; p < 0.05) were protective parameters against patient death (Table 2 ).
Nomogram construction and validation
A prognostic nomogram for early recognition of in-hospital mortality in cirrhotic patients with AKI was constructed using the multivariate logistic regression results, and points were assigned to the identified factors according to their regression coefficients (Fig. 2 ). As shown in the nomogram, patients with an elderly age; male; a history of cerebrovascular disease; higher heart rate, respiratory rate, BUN, Scr, INR and SOFA score were more likely to die. Nevertheless, patients with higher body temperature, SpO 2 , hemoglobin, and urine output had a lower risk of death. In addition, the nomogram illustrated higher SOFA score or lower urine output as the most significant contributors to death.

Nomogram for predicting in-hospital death of cirrhotic patients with acute kidney injury. Abbreviations INR: international normalized ratio, SOFA: sequential organ failure assessment
A bootstrapping technique with 1000 resamples as qualified by C-Index was used for internal validation to assess the model’s discriminatory power and reduce overfitting bias. In our investigation, the C-Index for the primary cohort was 0.811, the C-Index for the internal validation cohort was 0.796, and the calibration plot demonstrated excellent agreement between the nomogram prediction and the actual observations of mortality (Fig. 3 ). The AUC of the receiver operating characteristic curve was 0.811, 95% CI: 0.781–0.842, which indicates that the model has good predictive accuracy (Fig. 4 ). In addition, DCA curve showed that the nomogram model had high clinical value in the range of 1-96%, which further demonstrated that the nomogram model could accurately predict in-hospital mortality in cirrhotic patients with AKI (Fig. 5 ).
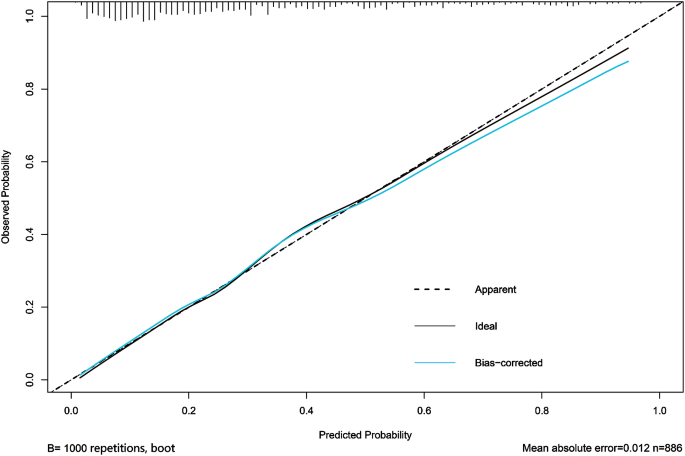
Calibration plots of internal validation
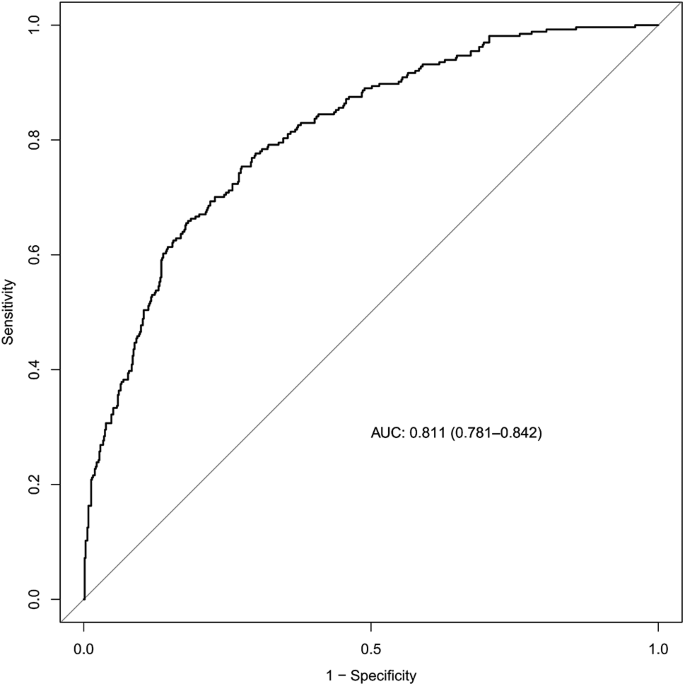
Receiver operating characteristic curve of nomogram. Abbreviations AUC: area under curve

Decision curve analysis curve of the nomogram
Cirrhosis is caused by numerous acute and chronic liver disorders. AKI is common and deadly in cirrhotic individuals, contributing to an unacceptable mortality rate [ 15 ]. The research also shows that the mortality rate of AKI is significantly higher in cirrhotic patients admitted to ICU than in those admitted to ordinary wards [ 16 , 17 ]. Despite discovering numerous treatment options, the prognosis for AKI in patients with cirrhosis remains poor. Therefore, it is critical to construct an effective model to identify cirrhotic patients with AKI at higher risk of in-hospital mortality, which may help guide treatment. In this investigation, we used logistic regression analysis to identify risk variables for in-hospital mortality in cirrhotic patients with AKI, including age, gender, cerebrovascular disease, heart rate, respiration rate, temperature, SpO 2 , hemoglobin, BUN, Scr, INR, bilirubin, urine volume, and the SOFA score. These risk factors were combined into a nomogram, which showed superior discrimination in predicting patient mortality.
Despite the abundance of research into the causes of AKI in cirrhotic patients, only a few studies have attempted to predict mortality in patients with cirrhosis and AKI [ 9 , 18 ]. The nomogram illustrated that the SOFA score, urine output, and body temperature were the three variables with the most predictive power for death in patients with cirrhosis and AKI. The SOFA score measures the burden of organ dysfunction to quantify organ impairment. Researchers discovered a strong link between the SOFA score and clinical outcomes, with higher scores often indicating a poor prognosis [ 19 ]. The results of this study show that the SOFA score is an independent predictor of mortality in patients with cirrhosis and AKI. In patients with cirrhosis and AKI, urine volume was also a risk factor for death. Oliguria is prevalent in intensive care unit patients and is the underlying cause of renal parenchymal damage [ 20 ]. Several studies have demonstrated a correlation between lower urine output and poor outcomes in critically ill individuals [ 21 ]. Temperature is a frequently assessed vital indicator for all ICU-admitted patients. Inconsistent reports exist about the effect of fever on mortality in intensive care unit (ICU) patients; some research implies that fever may contribute to mortality, while another meta-analysis suggests that the presence of fever per se may not increase mortality [ 22 ]. According to research by Laupland et al., who looked at data from 20,466 adult ICU patients, hypothermia is a significant risk factor for death in the medical ICU [ 23 ]. Our study also identified low body temperature as a risk factor for mortality among cirrhotic individuals with AKI. Therefore, hypothermia may be a significant and potentially controllable risk factor for death in patients with cirrhosis and AKI. In addition, age, gender, cerebrovascular disease, heart rate, respiration rate, SpO 2 , hemoglobin, BUN, Scr, INR, and bilirubin were also identified as possible risk factors for death in patients with cirrhosis and AKI.
Based on these probable risk factors of death, a quantitative and graphical nomogram was built for the purpose of predicting the in-hospital mortality of cirrhotic patients who were diagnosed with AKI in the current investigation. The nomogram could determine the scores relating to each potential risk factor. Nomogram, a single numerical estimate of the probability of an occurrence, has demonstrated superior performance than other methods of determining the chance of treatment success, complications, and mortality [ 24 ]. To our knowledge, our research is the first to develop a nomogram for predicting hospital mortality in cirrhotic patients with AKI, which can aid in the early identification and care of individuals at high risk.
In our study, we aimed to develop and validate a nomogram for the prediction of in-hospital mortality in patients with cirrhosis with AKI. Wan et al. evaluated overall survival in patients with cirrhosis with AKI using data from the MIMIC IV database, which provided valuable insights into long term prognostic factors [ 9 ]. Liao et al. developed nomograms to predict 15 and 30 day survival based on admission data from cirrhotic patients with AKI in the same dataset [ 25 ]. Although their study shares some similarities with ours, such as the use of MIMIC IV data and the focus on AKI in cirrhotic patients, there are several important differences. First, our nomogram was specifically designed to predict in-hospital mortality, enhancing the clinical utility of its immediate risk assessment. Second, we used rigorous methods, including multivariate logistic regression analysis and validation techniques, to develop and validate the nomogram. In addition, our study identified and incorporated other predictors not addressed in the studies by Wan et al. and Liao et al. such as cerebrovascular disease, body temperature, and SpO 2 , thereby improving the predictive accuracy and clinical applicability of the nomogram. In addition, Feng et al. developed a nomogram aimed at predicting AKI in cirrhotic patients upon ICU admission [ 26 ]. Their study provides valuable insights into early identification and risk stratification of AKI in this patient population, which is crucial for guiding clinical decision-making and interventions during the critical phase of ICU admission. In comparison to our study, which focuses on predicting in-hospital mortality in cirrhotic patients with AKI, Feng et al.‘s study addresses a different aspect of AKI management by targeting its early detection and prediction upon ICU admission. Our study complements the work of Feng et al. by providing a predictive model specifically tailored for predicting in-hospital mortality, which assists in identifying patients at imminent risk of death and guiding timely interventions and intensive monitoring during the hospital stay.
This study has some significant limitations. First, this was a retrospective modeling study conducted at a single location utilizing the MIMIC IV database, and we could not determine the causal relationship between features and outcomes. To confirm the efficacy of our method, we require additional prospective randomized clinical trials. Second, our study design’s retrospective and observational nature may lead to selection bias. Thirdly, we calculated specific missing data using padding, which may have resulted in disparities between the estimated and actual figures. Fourthly, even though our study employed bootstrapping technology for internal validation, future research will also require external validation. Fifthly, our study may not have captured all therapeutic measures that could influence outcomes in this patient population. The complexity of patient management in cirrhotic patients with AKI involves various therapeutic interventions, including medical treatments, invasive procedures, and supportive care measures, which may have an impact on patient outcomes. The absence of comprehensive data on all therapeutic interventions represents a limitation of our study and may have influenced the interpretation of the elaborated data. Finally, our study focused on the prognosis of cirrhotic patients with AKI in the ICU, and the findings may not apply to cirrhotic patients with AKI in non-ICUs and to AKI patients without cirrhosis in ICUs.
In conclusion, age, sex, cerebrovascular illness, heart rate, respiration rate, body temperature, SpO 2 , hemoglobin, BUN, Scr, INR, bilirubin, urine output, and SOFA score were possible mortality risk factors. In addition, a validated nomogram model based on possible risk variables was successfully developed to predict the in-hospital death of cirrhotic patients with AKI. The nomogram model may improve the identification of in-hospital deaths in patients with cirrhosis combined with AKI and contribute to timely intervention to improve patient prognosis.
Data availability
The datasets presented in the current study are available in the MIMIC-IV database ( https://physionet.org/content/mimiciv/1.0/ ).
Angeli P, Gines P, Wong F, Bernardi M, Boyer TD, Gerbes A, Moreau R, Jalan R, Sarin SK, Piano S, et al. Diagnosis and management of acute kidney injury in patients with cirrhosis: revised consensus recommendations of the International Club of ascites. Gut. 2015;64(4):531–7.
Article CAS PubMed Google Scholar
Gupta K, Bhurwal A, Law C, Ventre S, Minacapelli CD, Kabaria S, Li Y, Tait C, Catalano C, Rustgi VK. Acute kidney injury and hepatorenal syndrome in cirrhosis. World J Gastroenterol. 2021;27(26):3984–4003.
Article CAS PubMed PubMed Central Google Scholar
Garcia-Tsao G, Parikh CR, Viola A. Acute kidney injury in cirrhosis. Hepatology (Baltimore MD). 2008;48(6):2064–77.
Pan HC, Chien YS, Jenq CC, Tsai MH, Fan PC, Chang CH, Chang MY, Tian YC, Fang JT, Yang CW et al. Acute Kidney Injury Classification for Critically Ill Cirrhotic Patients: A Comparison of the KDIGO, AKIN, and RIFLE Classifications. Scientific reports 2016, 6:23022.
Xiong J, Pu L, Xiong H, Xiang P, Zhang M, Liu J, Li A. Evaluation of the criteria of hepatorenal syndrome type of acute kidney injury in patients with cirrhosis admitted to ICU. 2018, 53(12):1590–6.
Guan C, Li C, Xu L, Zhen L, Zhang Y, Zhao L, Zhou B, Che L, Wang Y, Xu Y. Risk factors of cardiac surgery-associated acute kidney injury: development and validation of a perioperative predictive nomogram. 2019, 32(6):937–45.
Zhang P, Guan C, Li C, Zhu Z, Zhang W, Luan H, Zhou B. A visual risk assessment tool for acute kidney injury after intracranial aneurysm clipping surgery. 2020, 42(1):1093–9.
Xu F, Zhang L, Wang Z, Han D, Li C, Zheng S, Yin H, Lyu J. A New Scoring System for Predicting In-hospital death in patients having liver cirrhosis with esophageal varices. Front Med. 2021;8:678646.
Article Google Scholar
Wan YP, Wang AJ, Zhang W, Zhang H, Peng GH, Zhu X. Development and validation of a nomogram for predicting overall survival in cirrhotic patients with acute kidney injury. World J Gastroenterol. 2022;28(30):4133–51.
Zhou S, Zeng Z, Wei H, Sha T, An S. Early combination of albumin with crystalloids administration might be beneficial for the survival of septic patients: a retrospective analysis from MIMIC-IV database. Ann Intensiv Care. 2021;11(1):42.
Article CAS Google Scholar
Johnson AE, Pollard TJ, Shen L, Lehman LW, Feng M, Ghassemi M, Moody B, Szolovits P. MIMIC-III, a freely accessible critical care database. 2016, 3:160035.
Andrassy KM. Comments on ‘KDIGO 2012 Clinical Practice Guideline for the evaluation and management of chronic kidney disease’. Kidney Int. 2013;84(3):622–3.
Huber M, Ozrazgat-Baslanti T, Thottakkara P, Scali S, Bihorac A, Hobson C. Cardiovascular-specific mortality and kidney disease in patients undergoing vascular surgery. JAMA Surg. 2016;151(5):441–50.
Article PubMed PubMed Central Google Scholar
Angeli P, Ginès P, Wong F, Bernardi M, Boyer TD, Gerbes A, Moreau R, Jalan R, Sarin SK, Piano S, et al. Diagnosis and management of acute kidney injury in patients with cirrhosis: revised consensus recommendations of the International Club of ascites. J Hepatol. 2015;62(4):968–74.
Article PubMed Google Scholar
Flamm SL, Brown K, Wadei HM. The current management of Hepatorenal Syndrome-Acute Kidney Injury in the United States and the potential of Terlipressin. 2021, 27(8):1191–202.
Xiong J, Zhang M, Guo X, Pu L, Xiong H, Xiang P, Liu J, Li A. Acute kidney injury in critically ill cirrhotic patients with spontaneous bacterial peritonitis: a comparison of KDIGO and ICA criteria. Archives Med Science: AMS. 2020;16(3):569–76.
Tariq R, Hadi Y, Chahal K, Reddy S, Salameh H, Singal AK. Incidence, mortality and predictors of Acute kidney Injury in patients with cirrhosis: a systematic review and Meta-analysis. J Clin Translational Hepatol. 2020;8(2):135–42.
Duah A, Duah F. Acute Kidney Injury in Patients with Liver Cirrhosis: Prevalence, Predictors, and In-Hospital Mortality at a District Hospital in Ghana. 2022, 2022:4589767.
Fan Z, Zhao Y, Wang S, Zhang F, Zhuang C. Clinical features and outcomes of tetanus: a retrospective study. Infect drug Resist. 2019;12:1289–93.
Macedo E, Malhotra R, Bouchard J, Wynn SK, Mehta RL. Oliguria is an early predictor of higher mortality in critically ill patients. Kidney Int. 2011;80(7):760–7.
Kellum JA, Sileanu FE, Murugan R, Lucko N, Shaw AD, Clermont G. Classifying AKI by urine output versus serum creatinine level. J Am Soc Nephrology: JASN. 2015;26(9):2231–8.
Egi M, Morita K. Fever in non-neurological critically ill patients: a systematic review of observational studies. J Crit Care. 2012;27(5):428–33.
Laupland KB, Zahar JR, Adrie C, Schwebel C, Goldgran-Toledano D, Azoulay E, Garrouste-Orgeas M, Cohen Y, Jamali S, Souweine B, et al. Determinants of temperature abnormalities and influence on outcome of critical illness. Crit Care Med. 2012;40(1):145–51.
Shariat SF, Capitanio U, Jeldres C, Karakiewicz PI. Can nomograms be superior to other prediction tools? BJU Int. 2009;103(4):492–5. discussion 495–497.
Liao T, Lu Y, Su T, Bei L, Li X, Lu Y, Ren S, Huang L, Feng LH. Development and validation of prognostic nomogram for cirrhotic patients with acute kidney injury upon ICU admission. 2024, 19(1):49–58.
Feng LH, Lu Y, Ren S, Liang H, Wei L, Jiang J. Development and validation of a dynamic online nomogram for predicting acute kidney injury in cirrhotic patients upon ICU admission. Front Med. 2023;10:1055137.
Download references
Acknowledgements
The authors thank all the participants for their contributions.
This work was supported by grants from the Natural Science Foundation of Anhui Province (2008085MH244), and Anhui Medical University 2021 Clinical and Pre-disciplinary Co-Construction (2021lcxk032). No funding bodies had any role in study design, data collection and analysis, decision to publish, or preparation of the manuscript.
Author information
Xiang Li and Xunliang Li contributed equally to this work and share first authorship.
Authors and Affiliations
Department of Nephrology, The Second Affiliated Hospital of Anhui Medical University, Hefei, China
Xiang Li, Xunliang Li, Wenman Zhao & Deguang Wang
Department of Nephrology, Affiliated Hospital of Jining Medical University, Jining, China
You can also search for this author in PubMed Google Scholar
Contributions
Xiang Li: Conceived and designed the experiments; Performed the experiments; Analyzed and interpreted the data; Contributed reagents, materials, analysis tools or data; Wrote the paper. Xunliang Li: Conceived and designed the experiments; Performed the experiments; Analyzed and interpreted the data. Wenman Zhao: Analyzed and interpreted the data. Deguang Wang: Conceived and designed the experiments.
Corresponding author
Correspondence to Deguang Wang .
Ethics declarations
Ethics approval and consent to participate.
Data for this study were obtained from the MIMIC IV database, the creation of which was approved by the Institutional Review Board of the Massachusetts Institute of Technology. All participant data were anonymized to safeguard their privacy. Ethical approval and informed consent were not required as we had obtained access to the MIMIC IV database and the data used in this study were anonymized.
Consent for publication
Not applicable.
Competing interests
The authors affirm that they do not have any known financial interests or personal relationships that could have potentially influenced the findings presented in this paper.
Additional information
Publisher’s note.
Springer Nature remains neutral with regard to jurisdictional claims in published maps and institutional affiliations.
Rights and permissions
Open Access This article is licensed under a Creative Commons Attribution 4.0 International License, which permits use, sharing, adaptation, distribution and reproduction in any medium or format, as long as you give appropriate credit to the original author(s) and the source, provide a link to the Creative Commons licence, and indicate if changes were made. The images or other third party material in this article are included in the article’s Creative Commons licence, unless indicated otherwise in a credit line to the material. If material is not included in the article’s Creative Commons licence and your intended use is not permitted by statutory regulation or exceeds the permitted use, you will need to obtain permission directly from the copyright holder. To view a copy of this licence, visit http://creativecommons.org/licenses/by/4.0/ . The Creative Commons Public Domain Dedication waiver ( http://creativecommons.org/publicdomain/zero/1.0/ ) applies to the data made available in this article, unless otherwise stated in a credit line to the data.
Reprints and permissions
About this article
Cite this article.
Li, X., Li, X., Zhao, W. et al. Development and validation of a nomogram for predicting in-hospital death in cirrhotic patients with acute kidney injury. BMC Nephrol 25 , 175 (2024). https://doi.org/10.1186/s12882-024-03609-8
Download citation
Received : 16 October 2023
Accepted : 13 May 2024
Published : 21 May 2024
DOI : https://doi.org/10.1186/s12882-024-03609-8
Share this article
Anyone you share the following link with will be able to read this content:
Sorry, a shareable link is not currently available for this article.
Provided by the Springer Nature SharedIt content-sharing initiative
- Acute kidney injury
BMC Nephrology
ISSN: 1471-2369
- Submission enquiries: [email protected]
- General enquiries: [email protected]
- Open access
- Published: 18 May 2024
Acute kidney injury after out-of-hospital cardiac arrest
- Karoline Korsholm Jeppesen 1 , 5 na1 ,
- Sebastian Buhl Rasmussen 2 , 5 na1 ,
- Jesper Kjaergaard 3 , 4 ,
- Henrik Schmidt 2 , 5 ,
- Simon Mølstrøm 2 ,
- Rasmus Paulin Beske 3 ,
- Johannes Grand 3 ,
- Hanne Berg Ravn 2 , 5 ,
- Matilde Winther-Jensen 3 ,
- Martin Abild Stengaard Meyer 3 ,
- Christian Hassager 3 , 4 &
- Jacob Eifer Møller 1 , 3 , 5
Critical Care volume 28 , Article number: 169 ( 2024 ) Cite this article
647 Accesses
1 Altmetric
Metrics details
Acute kidney injury (AKI) is a significant risk factor associated with reduced survival following out-of-hospital cardiac arrest (OHCA). Whether the severity of AKI simply serves as a surrogate measure of worse peri-arrest conditions, or represents an additional risk to long-term survival remains unclear.
This is a sub-study derived from a randomized trial in which 789 comatose adult OHCA patients with presumed cardiac cause and sustained return of spontaneous circulation (ROSC) were enrolled. Patients without prior dialysis dependent kidney disease and surviving at least 48 h were included (N = 759). AKI was defined by the kidney disease: improving global outcome (KDIGO) classification, and patients were divided into groups based on the development of AKI and the need for continuous kidney replacement therapy (CKRT), thus establishing three groups of patients—No AKI, AKI no CKRT, and AKI CKRT. Primary outcome was overall survival within 365 days after OHCA according to AKI group. Adjusted Cox proportional hazard models were used to assess overall survival within 365 days according to the three groups.
In the whole population, median age was 64 (54–73) years, 80% male, 90% of patients presented with shockable rhythm, and time to ROSC was median 18 (12–26) min. A total of 254 (33.5%) patients developed AKI according to the KDIGO definition, with 77 requiring CKRT and 177 without need for CKRT. AKI CKRT patients had longer time-to-ROSC and worse metabolic derangement at hospital admission. Overall survival within 365 days from OHCA decreased with the severity of kidney injury. Adjusted Cox regression analysis found that AKI, both with and without CKRT, was significantly associated with reduced overall survival up until 365 days, with comparable hazard ratios relative to no AKI (HR 1.75, 95% CI 1.13–2.70 vs. HR 1.76, 95% CI 1.30–2.39).
Conclusions
In comatose patients who had been resuscitated after OHCA, patients developing AKI, with or without initiation of CKRT, had a worse 1-year overall survival compared to non-AKI patients. This association remains statistically significant after adjusting for other peri-arrest risk factors.
Trial registration : The BOX trial is registered at ClinicalTrials.gov: NCT03141099.
Introduction
Acute kidney injury (AKI) is a serious complication occurring in around 40% of patients resuscitated after out-of-hospital cardiac arrest (OHCA) and is associated with worse short- and long-term survival [ 1 , 2 ]. Known risk factors for AKI includes prolonged time to return of spontaneous circulation (ROSC), initial non-shockable rhythm, and post-resuscitation shock [ 3 ]. AKI was previously regarded as primarily a consequence of unfavorable peri-arrest conditions [ 4 ]. However, several studies have demonstrated that AKI may have an impact on survival itself [ 1 , 2 , 5 , 6 , 7 , 8 , 9 , 10 ]. Additionally, patients presenting with preexisting kidney impairment prior to the OHCA event face an increased 90-day mortality [ 11 ]. Therefore, post-resuscitation care to attenuate further kidney impairment is important and may lead to a better outcome.
Patients resuscitated after cardiac arrest may develop post-cardiac arrest syndrome (PCAS)—which encompasses post-ischemic neurological dysfunction, myocardial stunning, systemic inflammatory response syndrome, and impaired vasoregulation [ 12 , 13 ]. The latter may result in low cardiac output syndrome with vasoplegic shock and ultimately poor end-organ perfusion [ 14 ]. Management consists of delicate balance of vasopressors, inotropes, fluid management, and diuretics. However, evidence on kidney protective targets is currently limited and the pathophysiology leading to AKI is not fully understood. Furthermore, it is crucial to acknowledge that the diagnosis of AKI encompasses various causes and conditions rather than representing a specific disease. Consequently, there may be prognostic differences when adjusting for individual risk factors and categorizing according to AKI severity.
The objective of the present study was to determine characteristics associated with AKI according to the Kidney Disease Improving Global Outcomes (KDIGO) classification [ 15 ] and the prognostic importance of AKI severity in a well-defined OHCA population enrolled in the Blood Pressure and Oxygenation Targets After OHCA (BOX) trial [ 16 , 17 ].
The BOX trial
The BOX trial (NCT03141099) was an investigator-initiated, randomized trial with a two-by-two factorial design where comatose OHCA patients were enrolled at 2 tertiary cardiac arrest centers in Denmark between March 10th, 2017, and December 11th, 2021. Trial methods and the primary outcome results are described elsewhere [ 16 , 17 ]. Briefly, adult patients (≥ 18 years) resuscitated after OHCA of presumed cardiac cause and that remained comatose after sustained ROSC were randomly assigned to a mean arterial pressure (MAP) treatment target of either 63 mmHg or 77 mmHg, and simultaneously randomized to open-label intervention of either restrictive (9–10 kPa) or liberal (13–14 kPa) oxygen treatment target [ 16 , 17 ]. In addition, patients were randomized to device-based temperature intervention targeting 36 °C for 24 h followed by either a target of 37 °C for 12 or 48 h [ 18 ]. The original two studies from the BOX trial evaluated AKI requiring CKRT as an adverse event and found no difference between targets of MAP or oxygen treatment [ 16 , 17 ]. Therefore, the data analysis in this sub-study was performed across the intervention groups.
Treatment protocol
All patients included in the trial underwent target temperature management (TTM) as described above. During TTM patients were sedated (propofol and fentanyl) and mechanically ventilated.
In the first 24 h, a positive volume balance of approximately 1000 ml and diuresis of 1–1.5 ml/kg/h was targeted. Furosemide was administered as a first-line drug to increase diuresis if needed. CKRT was initiated at the discretion of treating physicians based on common indications for CKRT including oliguria or anuria, refractory volume overload, severe metabolic acidosis, electrolyte disturbances, or manifest uremic symptoms.
Plasma creatinine was measured upon hospital admission, and henceforward daily in the intensive care unit. If multiple plasma creatinine measurements were available the highest value was used for AKI assessment. Plasma creatinine ratio was calculated as the ratio of plasma creatinine at 48 h and the value at admission. Estimated glomerular filtration rate (eGFR) was determined upon hospital admission using the CKD-EPI 2021 formula [ 19 ]. Collected urine output was recorded at 24- and 48 h. Urine creatinine clearance was calculated at 48 h based on 24-h urine creatinine and volume, and plasma-creatinine. Thus, only patients surviving beyond 48 h were included in the analysis to ensure the possibility to record kidney injury. Furthermore, patients receiving chronic dialysis before OHCA were excluded from analysis.
AKI was defined by the Kidney Disease: Improving Global Outcome (KDIGO) classification [ 15 ] (Table S1 , Supplemental Material), and patients were divided into groups based on development of AKI and need for CKRT, thus establishing three groups of patients—No AKI, AKI no CKRT and AKI CKRT.
The primary endpoint of this study was overall survival within 365 days after OHCA, stratified according to patients with no AKI, AKI no CKRT, and AKI CKRT. Furthermore, the characteristics associated with development of AKI after OHCA were assessed. Lastly, we assessed the need for intermittent or chronic dialysis at 3 months and 1 year post-discharge in patients who underwent CKRT within 5 days of their ICU stay.
Statistical analysis
The primary study’s sample size calculation determined the size of the study population in this pre-specified sub-study. Survival probabilities were calculated using the Kaplan–Meier estimator for No AKI, AKI no CKRT, and AKI CKRT, respectively. The Log-Rank test was used to test for statistically significant differences between groups.
Cox proportional hazard models were used to assess overall survival within 365 days from hospital admission for No AKI, AKI no CKRT, and AKI CKRT, respectively. The corresponding hazard ratios (HRs) and 95% confidence intervals (CIs) were listed with No AKI as the reference. The proportional hazards assumption and linearity on the log-hazard scale were assessed using cumulative martingale residuals. The Cox proportional hazard models were adjusted for the following confounders; age, sex, eGFR upon admission, diabetes mellitus, heart failure, witnessed arrest, bystander cardio-pulmonary resuscitation, shockable rhythm, time-to-ROSC, and acute percutaneous coronary intervention.
A two-sided p -value < 0.05 was considered statistically significant. All analyses were conducted in R Statistical Software version 6.1.524 (Posit team 2023).
The BOX trial enrolled 802 patients between March 2017 and December 2021. Consent was declined in 12 patients and 1 patient was randomized twice, hence, the intention-to-treat analysis included 789 patients [ 16 , 17 ].
In the present sub-study, we further excluded patients receiving chronic dialysis prior to admission and patients who died within 48 h of admission. Thus 759 patients underwent analysis (Figure S1 , Supplemental Material). Baseline characteristics for patients with No AKI, AKI no CKRT, and AKI CKRT are presented in Table 1 . The population consisted primarily of men around 60 years of age and cardiovascular comorbidities were common. Majority of patients had a witnessed cardiac arrest with bystander cardio-pulmonary resuscitation and an initial shockable rhythm.
- Acute kidney injury
A total of 254 (33.5%) patients developed AKI according to the KDIGO definition, 177 without the need for CKRT and 77 with the need for CKRT. Patients developing AKI were generally older and had longer time-to-ROSC and correspondingly more severe metabolic derangement at hospital admission (Table 1 ). Values of MAP and PaO 2 during the first 5 days of ICU are illustrated for AKI groups in Figs. 1 and 2 . Significantly lower weighted mean values of MAP, extending up to ICU day 5, were observed in AKI patients and demonstrated a declining trend with increasing AKI severity (Table 1 ). Conversely, mean PaO 2 and temperature exhibited comparable levels between groups (Table 1 ). Patients who developed AKI had a statistically significantly lower mean perfusion pressure during the period 6–12 h after admission to the ICU, but not at other time points (Figure S2 , Table S2 , Supplemental Material).
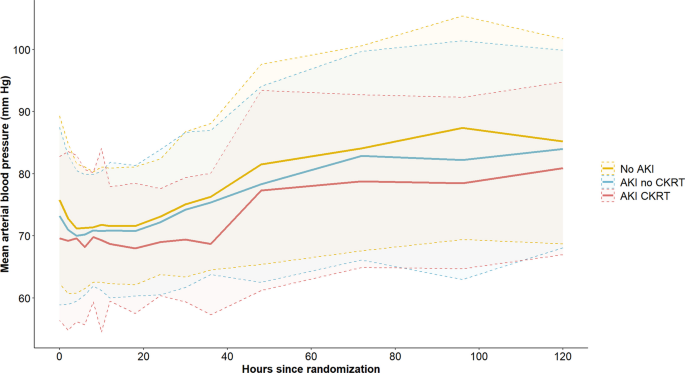
Mean arterial pressure. Mean arterial pressure (± standard deviation) over time according to group—No AKI, AKI no CKRT and AKI CKRT. AKI ; acute kidney injury, CKRT ; continuous kidney replacement therapy
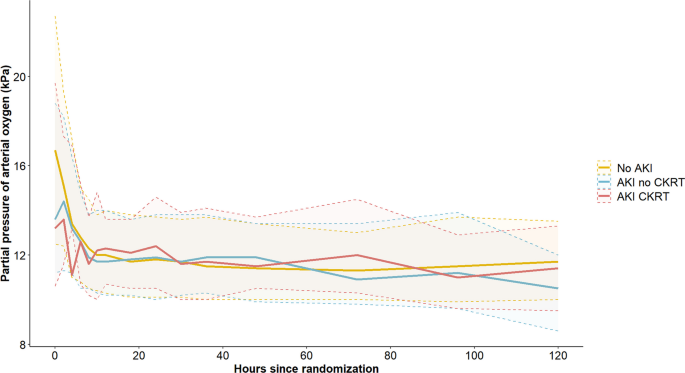
Partial pressure of arterial oxygen. Median partial pressure of arterial oxygen (25th–75th percentile) over time according to group—No AKI, AKI no CKRT, AKI CKRT. AKI ; acute kidney injury, CKRT ; continuous kidney replacement therapy
Blood samples and clinical markers of kidney function are listed in Table 2 . The eGFR at hospital admission was inversely related to the severity of AKI (Table 2 ). Furthermore, both a low urine output and increasing plasma creatinine were seen in the AKI CKRT group, while AKI diagnosis was primarily driven by an increase in creatinine in the AKI no CKRT group (Table 2 ). The levels of plasma creatinine within the first 7 days of hospitalization according to each group are presented in Fig. 3 .
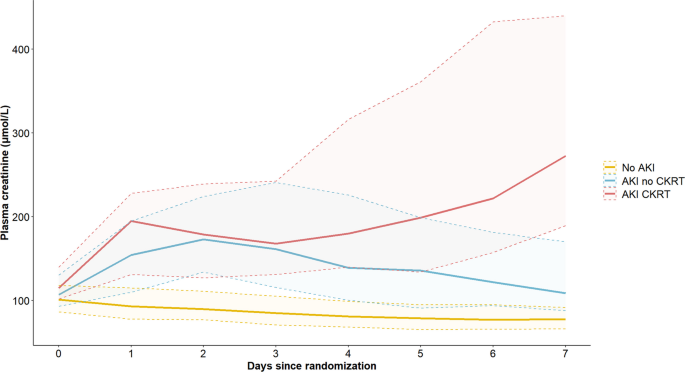
Plasma-creatinine. Median plasma-creatinine (25th–75th percentile) over time according to group—No AKI, AKI no CKRT, AKI CKRT. AKI ; acute kidney injury, CKRT ; continuous kidney replacement therapy
Among patients receiving CKRT within the first 5 ICU days, no survivors required intermittent or chronic dialysis at 3 months or 1 year after discharge.
Survival analysis
Kaplan–Meier survival estimates according to No AKI, AKI no CKRT, and AKI CKRT, respectively, are illustrated in Fig. 4 and demonstrate a statistically significant difference in survival between groups within 365 days after OHCA (Log-Rank p < 0.0001). Overall survival 365 days after hospital admission was 74% in patients with no AKI, 55% in patients with AKI and no CKRT, and 43% in the AKI CKRT group.
In unadjusted cox regression, AKI no CKRT (HR 1.99, 95% CI 1.51–2.63; p < 0.001) and AKI CKRT (HR 3.10, 95% CI 2.20–4.36; p < 0.001) were associated with increased hazard for death from all causes relative to patients without AKI (Table 3 ). After adjusting for age, sex, eGFR upon admission, diabetes mellitus, heart failure, witnessed arrest, bystander cardio-pulmonary resuscitation, shockable rhythm, time-to-ROSC, and acute percutaneous coronary intervention, both AKI with and without CKRT remained significantly associated with reduced overall survival after 365 days (Table 3 ). Notably, the hazard ratios for AKI with and without CKRT were comparable relative to no AKI (Table 3 ).
In the present study, including resuscitated comatose OHCA patients of presumed cardiac cause, patients developing AKI requiring CKRT had longer time-to-ROSC and correspondingly more severe metabolic derangement at hospital admission than AKI patients without CKRT or without AKI. Patients with AKI, with or without CKRT, had a worse 1-year overall survival compared with patients without AKI. Following adjustment for peri-arrest parameters, AKI remained a significant risk factor for reduced overall survival, irrespective of CKRT initiation.
Our results add to current knowledge regarding predictors of AKI in OHCA patients, and the long-term consequences of having an episode of AKI within the post-resuscitation period. Thirty percent of patients developing AKI received CKRT in our study. This is in line with the observed frequency in a recent systematic review on cardiac arrest survivors [ 2 ]. Despite the frequent use of CKRT, several large randomized trials have found no survival benefit from especially early and aggressive use of CKRT in critically ill patients [ 20 , 21 , 22 ]. Similarly, a recent study on cardiac arrest patients found no difference in in-hospital survival between patients with AKI requiring CKRT within 72 h of admission and patients without the need for CKRT [ 23 ]. Looking at the characteristics of CKRT patients, it seems that there is an gradual increase in plasma creatinine from day 3 to day 7 (Fig. 3 ). Considering that CKRT was initiated in 70% of patients by 48 h and that the duration of CKRT was less than 3 days in more than half of the patients, it is likely that the delayed increase in creatinine represents patients weaned off CKRT with re-established water excretion, but still lacking normal renal clearance (Table S3 and S4 , Supplemental Material). However, it seems that there are no long-term adverse clearance issues, as none of the patients who were on CKRT by day 5 required dialysis up to 1 year after discharge (Fig. 4 ).
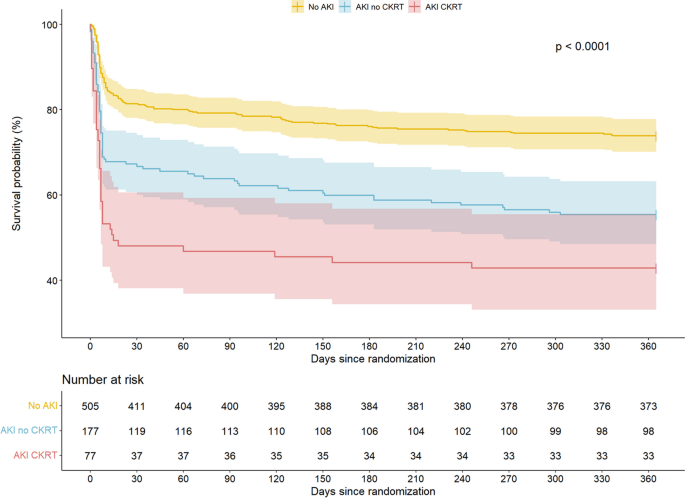
Kaplan Meier survival analysis. Kaplan Meier survival analysis displaying survival functions for No AKI, AKI no CKRT, and AKI CKRT. AKI ; acute kidney injury, CKRT ; continuous kidney replacement therapy
In this study, we found a 12.5 percentage point lower 1-year survival among AKI patients receiving CKRT compared to AKI patients without CKRT. This difference in survival was even more pronounced when comparing AKI CKRT patients with patients without AKI (31 percentage point difference). The main divergence in survival was related to differences in short-term mortality, particularly within the initial 10 days after hospital admission (Table S8 , Supplemental Material). Therefore, the main question remains whether severity of AKI is just a surrogate measure of worse peri-arrest conditions, or whether it is a significant risk factor for long-term survival in itself.
We identified increasing age, female sex, and particularly, time-to-ROSC as significantly associated with worse overall survival through adjusted Cox regression analysis. Conversely, having an initial shockable rhythm and receiving bystander cardiopulmonary resuscitation were significantly associated with a better outcome.
In alignment with the well-established cardiorenal syndrome, we observed a higher prevalence of cardiac pre-arrest comorbidities, such as ischemic heart disease and heart failure, within the AKI CKRT group (Table 1 ). Heart failure is known to trigger compensatory mechanisms, leading to fluid retention, venous congestion, and ultimately reduced kidney perfusion [ 24 ]. Furthermore, in OHCA patients with pre-existing cardiac dysfunction, a vicious cycle may be initiated due to a combination of hypoperfusion, ischemia–reperfusion injury, use of radiocontrast, positive pressure ventilation, etc. However, surprisingly, pre-existing heart failure did not emerge as a significant risk factor in the adjusted Cox analysis.
Patients with AKI requiring CKRT and AKI without CKRT were both associated with worse overall survival, but interestingly, following adjustment for known confounders, the hazards were similar between groups. Although the Cox regression analysis was adjusted for eGFR upon admission, some residual hazard may still be attributed to pre-admission kidney vulnerability in AKI patients, given the well-established association of chronic kidney impairment with cardiovascular death [ 25 ]. In this study, we found a gradually lower eGFR at baseline according to the severity of kidney injury (Table 2 ). This is in line with Tamura et al., who also found a graded association between lower eGFR at admission and a worse 3-month survival [ 11 ]. The variation in drug metabolism and clearance among AKI patients is recognized to influence treatment responses, potentially leading to an ominous outcome [ 26 ]. Given that preexisting kidney dysfunction is a risk factor for AKI, overall survival may be jeopardized due to a combination of cardiovascular dysfunction and altered pharmacokinetics [ 27 ].
Thus, regardless of severity, AKI occurrence is associated with worse overall survival, emphasizing the need for evidence-based post-resuscitation kidney-protective care. International post-resuscitation guidelines recommend a target of MAP > 65 mmHg, but based on sparse clinical evidence [ 28 ]. However, previous studies in OHCA have suggested a potential benefit of a further increase in MAP on kidney function [ 6 , 29 ]. In this present study, we observed a significant association between lower MAP during the initial 5 days of ICU admission (Table 1 ) and lower perfusions pressure during the 6–24 h of ICU admission (Table S2 , Supplemental Material), according to the severity of AKI. This association persisted, even though AKI CKRT patients had a significantly increased Vasoactive Inotropic Score (VIS) throughout the first 72 h (Table S5 , Supplemental Material). Other studies have also demonstrated higher use of vasopressors to be associated with AKI severity [ 30 , 31 ]. However, what at first glance may seem as a paradox or conflicting results, may be reconciled if certain phenotypes are more susceptible to lower perfusion pressure or if the association is the other way around, that patients with more severe organ injury require more inotropic support, increased filling pressures, and lower perfusion pressure. In this case, the lower MAP is not the cause but a consequence of the patient's condition.
Nevertheless, when evaluating observational data, it is important to cautiously assess the use of MAP as a surrogate measure for organ perfusion pressure. One study found a low MAP, but not a low cardiac output, to be associated with AKI development in OHCA patients [ 32 ].
To effectively control for potential confounders, it is essential to prioritize kidney protective endpoints in randomized clinical trials. We recently demonstrated that patients randomized to a combination of a low mean arterial blood-pressure (63 mmHg) and a liberal oxygen target (13–14 kPa) had a significantly increased risk of mild-stage AKI [ 33 ]. This is especially important in light of the results of the present study, which emphasize that even milder stages of AKI also are associated with a worse overall survival.
This study has some limitations. First, no specific sample size calculation was performed for this sub-study, however, due to the high event rate of AKI and death in this study, we were able to perform sufficient hypothesis testing on group level. Second, we used the plasma creatinine measured upon hospital admission to establish a baseline eGFR. Even though median transport to the hospital only was 22 (10–32) min, cardiopulmonary resuscitation may theoretically have resulted in creatinine release from the skeletal muscle and thereby influenced the baseline value. Third, since we do not have reliable estimates of the individual patient's fluid balance, this may confound the observed differences in relation to creatinine values and urine output. For instance, the fluid balance may be aimed negative due to volume overload in CKRT patients, and therefore “true creatinine” could potentially have been even higher in this group, which complicates head-to-head comparisons. Fourth, patients developing AKI in ICU have an increased risk of developing chronic kidney disease later on [ 34 ], but due to the limited follow-up duration of only 365 days, this may not yet have been established, and thus the longer-term consequences are not revealed within the observation time in the present study. Lastly, this study was conducted on a relatively homogenous population with favorable clinical characteristics. Therefore, it is speculative whether results can be generalized to OHCA populations without shockable rhythm or bystander cardiopulmonary resuscitation.
In conclusion, this study of OHCA patients, found that AKI patients requiring CKRT had longer time-to-ROSC and worse metabolic derangement at hospital admission. AKI patients, with or without initiation of CKRT, had a worse 1-year survival compared to non-AKI patients. This risk persisted after adjusting for other peri-arrest risk factors.
Availability of data and materials
The datasets used and/or analyzed during the current study are available from the corresponding author on reasonable request.
Abbreviations
- Continuous kidney replacement therapy
- Cardiopulmonary resuscitation
Glomerular filtration rate
Kidney disease: improving global outcomes-classification
Mean arterial pressure
Out-of-hospital cardiac arrest
Partial pressure of arterial oxygen
Post-cardiac arrest syndrome
Return of spontaneous circulation
Targeted temperature management
Storm C, Krannich A, Schachtner T, Engels M, Schindler R, Kahl A, et al. Impact of acute kidney injury on neurological outcome and long-term survival after cardiac arrest—a 10 year observational follow up. J Crit Care. 2018;47:254–9.
Article CAS PubMed Google Scholar
Prasitlumkum N, Cheungpasitporn W, Sato R, Chokesuwattanaskul R, Thongprayoon C, Patlolla SH, et al. Acute kidney injury and cardiac arrest in the modern era: an updated systematic review and meta-analysis. Hosp Pract. 1995;2021(49):280–91.
Google Scholar
Sandroni C, Dell’anna AM, Tujjar O, Geri G, Cariou A, Taccone FS. Acute kidney injury after cardiac arrest: a systematic review and meta-analysis of clinical studies. Minerva Anestesiol. 2016;82:989–99.
PubMed Google Scholar
Winther-Jensen M, Kjaergaard J, Lassen JF, Køber L, Torp-Pedersen C, Hansen SM, et al. Use of renal replacement therapy after out-of-hospital cardiac arrest in Denmark 2005–2013. Scand Cardiovasc J. 2018;52:238–43.
Jamme M, Ait Hamou Z, Ben Hadj Salem O, Guillemet L, Bougouin W, Pène F, et al. Long term renal recovery in survivors after OHCA. Resuscitation. 2019;141:144–50.
Article PubMed Google Scholar
Grand J, Hassager C, Winther-Jensen M, Rundgren M, Friberg H, Horn J, et al. Mean arterial pressure during targeted temperature management and renal function after out-of-hospital cardiac arrest. J Crit Care. 2019;50:234–41.
Beitland S, Nakstad ER, Staer-Jensen H, Draegni T, Andersen GØ, Jacobsen D, et al. Impact of acute kidney injury on patient outcome in out-of-hospital cardiac arrest: a prospective observational study. Acta Anaesthesiol Scand. 2016;60:1170–81.
Rundgren M, Ullén S, Morgan MPG, Glover G, Cranshaw J, Al-Subaie N, et al. Renal function after out-of-hospital cardiac arrest; the influence of temperature management and coronary angiography, a post hoc study of the target temperature management trial. Crit Care. 2019;23:163.
Article PubMed PubMed Central Google Scholar
Oh JH, Lee DH, Cho IS, Youn CS, Lee BK, Wee JH, et al. Association between acute kidney injury and neurological outcome or death at 6 months in out-of-hospital cardiac arrest: a prospective, multicenter, observational cohort study. J Crit Care. 2019;54:197–204.
Strand K, Søreide E, Kirkegaard H, Taccone FS, Grejs AM, Duez CHV, et al. The influence of prolonged temperature management on acute kidney injury after out-of-hospital cardiac arrest: a post hoc analysis of the TTH48 trial. Resuscitation. 2020;151:10–7.
Tamura T, Suzuki M, Hayashida K, Sasaki J, Yonemoto N, Sakurai A, et al. Renal function and outcome of out-of-hospital cardiac arrest—multicenter prospective study (SOS-KANTO 2012 Study). Circ J. 2018;83:139–46.
Nolan JP, Neumar RW, Adrie C, Aibiki M, Berg RA, Böttiger BW, et al. Post-cardiac arrest syndrome: epidemiology, pathophysiology, treatment, and prognostication. A Scientific Statement from the International Liaison Committee on Resuscitation; the American Heart Association Emergency Cardiovascular Care Committee; the Cou. Resuscitation. 2008;79:350–79.
Adrie C, Laurent I, Monchi M, Cariou A, Dhainaou J-F, Spaulding C. Postresuscitation disease after cardiac arrest: a sepsis-like syndrome? Curr Opin Crit Care. 2004;10:208–12.
Vallabhajosyula S, Dunlay SM, Prasad A, Sangaralingham LR, Kashani K, Shah ND, et al. Cardiogenic shock and cardiac arrest complicating ST-segment elevation myocardial infarction in the United States, 2000–2017. Resuscitation. 2020;155:55–64.
KDIGO AKI Work Group. KDIGO clinical practice guideline for acute kidney injury. Kidney Int Suppl. 2012;2:1–138.
Kjaergaard J, Møller JE, Schmidt H, Grand J, Mølstrøm S, Borregaard B, et al. Blood-pressure targets in comatose survivors of cardiac arrest. N Engl J Med. 2022;387:1456–66.
Schmidt H, Kjaergaard J, Hassager C, Mølstrøm S, Grand J, Borregaard B, et al. Oxygen targets in comatose survivors of cardiac arrest. N Engl J Med. 2022;387:1467–76.
Hassager C, Schmidt H, Møller JE, Grand J, Mølstrøm S, Beske RP, et al. Duration of device-based fever prevention after cardiac arrest. N Engl J Med. 2023;388:888–97.
Inker LA, Eneanya ND, Coresh J, Tighiouart H, Wang D, Sang Y, et al. New creatinine- and cystatin c-based equations to estimate GFR without race. N Engl J Med. 2021;385:1737–49.
Article CAS PubMed PubMed Central Google Scholar
Fayad AI, Buamscha DG, Ciapponi A. Timing of kidney replacement therapy initiation for acute kidney injury. Cochrane Database Syst Rev. 2022;11:CD010612.
Gaudry S, Hajage D, Benichou N, Chaïbi K, Barbar S, Zarbock A, et al. Delayed versus early initiation of renal replacement therapy for severe acute kidney injury: a systematic review and individual patient data meta-analysis of randomised clinical trials. Lancet. 2020;395:1506–15.
Bagshaw SM, Wald R, Adhikari NKJ, Bellomo R, da Costa BR, Dreyfuss D, et al. Timing of initiation of renal-replacement therapy in acute kidney injury. N Engl J Med. 2020;383:240–51.
Ghoshal S, Yang V, Brodie D, Radhakrishnan J, Roh DJ, Park S, et al. In-hospital survival and neurological recovery among patients requiring renal replacement therapy in post-cardiac arrest period. Kidney Int Rep. 2019;4:674–8.
Sriperumbuduri S, Clark E, Hiremath S. New insights into mechanisms of acute kidney injury in heart disease. Can J Cardiol. 2019;35:1158–69.
Jankowski J, Floege J, Fliser D, Böhm M, Marx N. Cardiovascular disease in chronic kidney disease: pathophysiological insights and therapeutic options. Circulation. 2021;143:1157–72.
Blanco VE, Hernandorena CV, Scibona P, Belloso W, Musso CG. Acute kidney injury pharmacokinetic changes and its impact on drug prescription. Healthcare (Basel). 2019;7:1.
Kellum JA, Romagnani P, Ashuntantang G, Ronco C, Zarbock A, Anders H-J. Acute kidney injury. Nat Rev Dis Primers. 2021;7:52.
Soar J, Berg KM, Andersen LW, Böttiger BW, Cacciola S, Callaway CW, et al. Adult advanced life support: 2020 international consensus on cardiopulmonary resuscitation and emergency cardiovascular care science with treatment recommendations. Resuscitation. 2020;156:A80-119.
Dupont V, Bonnet-Lebrun A-S, Boileve A, Charpentier J, Mira J-P, Geri G, et al. Impact of early mean arterial pressure level on severe acute kidney injury occurrence after out-of-hospital cardiac arrest. Ann Intensive Care. 2022;12:69.
Dutta A, Hari KJ, Azizian J, Masmoudi Y, Khalid F, Kowal JL, et al. Incidence, predictors, and prognosis of acute kidney injury among cardiac arrest survivors. J Intensive Care Med. 2021;36:550–6.
Park YS, Choi YH, Oh JH, Cho IS, Cha K-C, Choi B-S, et al. Recovery from acute kidney injury as a potent predictor of survival and good neurological outcome at discharge after out-of-hospital cardiac arrest. Crit Care. 2019;23:256.
Grand J, Bro-Jeppesen J, Hassager C, Rundgren M, Winther-Jensen M, Thomsen JH, et al. Cardiac output during targeted temperature management and renal function after out-of-hospital cardiac arrest. J Crit Care. 2019;54:65–73.
Rasmussen SB, Jeppesen KK, Kjaergaard J, Hassager C, Schmidt H, Mølstrøm S, et al. Blood pressure and oxygen targets on kidney injury after cardiac arrest. Circulation. 2023;148:1860–9.
Abdala PM, Swanson EA, Hutchens MP. Meta-analysis of AKI to CKD transition in perioperative patients. Perioper Med (Lond). 2021;10:24.
Download references
Acknowledgements
The authors would like to express our sincere gratitude to the patients, relatives, and the staff of the participating sites. In particular, the nursing staff of the Cardiac Intensive Care Units at both sites have committed themselves to diligent adherence to the trial protocol.
Open access funding provided by University of Southern Denmark. The Novo Nordisk Foundation funded the trial: NNF17OC0028706. A grant from the Lundbeck Foundation paid part of professor Hassagers salary (R186-2015-2132).
Author information
Karoline Korsholm Jeppesen and Sebastian Buhl Rasmussen have contributed equally to this work.
Authors and Affiliations
Department of Cardiology, Odense University Hospital, J. B. Winsloews Vej 4, 5000, Odense C, Denmark
Karoline Korsholm Jeppesen & Jacob Eifer Møller
Department of Anesthesiology and Intensive Care, Odense University Hospital, Odense, Denmark
Sebastian Buhl Rasmussen, Henrik Schmidt, Simon Mølstrøm & Hanne Berg Ravn
Department of Cardiology, The Heart Center, Copenhagen University Hospital Rigshospitalet, Copenhagen, Denmark
Jesper Kjaergaard, Rasmus Paulin Beske, Johannes Grand, Matilde Winther-Jensen, Martin Abild Stengaard Meyer, Christian Hassager & Jacob Eifer Møller
Department of Clinical Medicine, University of Copenhagen, Copenhagen, Denmark
Jesper Kjaergaard & Christian Hassager
Department of Clinical Research, University of Southern Denmark, Odense, Denmark
Karoline Korsholm Jeppesen, Sebastian Buhl Rasmussen, Henrik Schmidt, Hanne Berg Ravn & Jacob Eifer Møller
You can also search for this author in PubMed Google Scholar
Contributions
KKJ and SBR drafted the manuscript. KKJ, SBR and JEM analyzed the data. All authors (KKJ, SBR, JK, HS, SM, RPB, JG, HBR, MWJ, MASM, CH, JEM) contributed to conceptualization of the manuscript and interpretation of the data. The BOX trial was conceptualized by JK, JEM, HS and CH. All authors read and approved the final manuscript.
Corresponding author
Correspondence to Jacob Eifer Møller .
Ethics declarations
Ethics approval and consent to participate.
The Capital Region of Denmark Ethics Committee approved the trial (Approval id H-16033436). The Data Protection Authority approved the data handling agreement (RH-2016-373). A steering committee planned and oversaw the trial (Supplemental Material).
Consent for publication
Not applicable.
Competing interests
The authors declare that they have no competing interests.
Additional information
Publisher's note.
Springer Nature remains neutral with regard to jurisdictional claims in published maps and institutional affiliations.
Supplementary Information
Supplementary material 1., rights and permissions.
Open Access This article is licensed under a Creative Commons Attribution 4.0 International License, which permits use, sharing, adaptation, distribution and reproduction in any medium or format, as long as you give appropriate credit to the original author(s) and the source, provide a link to the Creative Commons licence, and indicate if changes were made. The images or other third party material in this article are included in the article's Creative Commons licence, unless indicated otherwise in a credit line to the material. If material is not included in the article's Creative Commons licence and your intended use is not permitted by statutory regulation or exceeds the permitted use, you will need to obtain permission directly from the copyright holder. To view a copy of this licence, visit http://creativecommons.org/licenses/by/4.0/ . The Creative Commons Public Domain Dedication waiver ( http://creativecommons.org/publicdomain/zero/1.0/ ) applies to the data made available in this article, unless otherwise stated in a credit line to the data.
Reprints and permissions
About this article
Cite this article.
Jeppesen, K.K., Rasmussen, S.B., Kjaergaard, J. et al. Acute kidney injury after out-of-hospital cardiac arrest. Crit Care 28 , 169 (2024). https://doi.org/10.1186/s13054-024-04936-w
Download citation
Received : 15 March 2024
Accepted : 29 April 2024
Published : 18 May 2024
DOI : https://doi.org/10.1186/s13054-024-04936-w
Share this article
Anyone you share the following link with will be able to read this content:
Sorry, a shareable link is not currently available for this article.
Provided by the Springer Nature SharedIt content-sharing initiative
- Post-cardiac arrest
Critical Care
ISSN: 1364-8535
- Submission enquiries: [email protected]
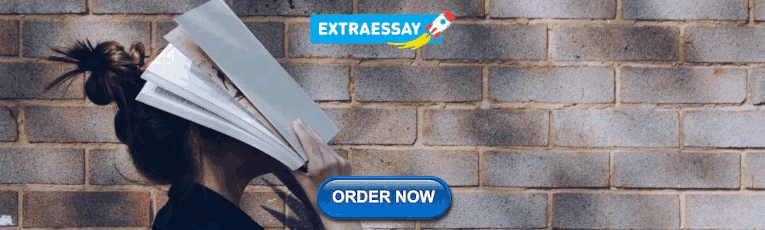
IMAGES
VIDEO
COMMENTS
Adult II Case Study-Acute Kidney Injury. Patient Profile A 78 year-old woman with a long history of hypertension, heart failure, and coronary artery surgery 12 years ago. She was admitted 2 days ago for heart failure exacerbation. Despite being treated with diuretics, her urinary output is decreased.
Case Study Objectives. Analyze and interpret clinical data and patient assessments to identify signs and symptoms of acute kidney injury (AKI) in a real-life patient scenario. Apply critical thinking skills to recognize the physiological mechanisms contributing to the development of AKI, considering factors such as dehydration, contrast dye ...
We're going to go through a case study for acute kidney injury together. Let's get started. In this scenario, our patient is Ms. Barkley. She's thin and frail, 64 years old and presents from a nursing home for acute abdominal pain as well as nausea and vomiting that she's been experiencing for the last two days.
A 15-year-old girl presented with acute kidney injury. Eight days earlier, bloody diarrhea and abdominal cramping had developed. On admission, the creatinine level was 7.71 mg per deciliter, and an...
Acute kidney injury can be caused by a variety of diseases and conditions that impede or reduce blood flow to the kidneys, such as: Hypotension (low blood pressure) or shock. Loss of blood or fluids (such as bleeding, severe diarrhea) Decreased heart function caused by cardiovascular disorders. Organ failure.
Here's the statistics and incidences for acute renal failure: ARF affects approximately 1% of patients on admission to the hospital, 2% to 5% during the hospital stay, 4% to 15% after cardiopulmonary bypass surgery, and 10% of cases acute renal failure occurs in isolation (i.e. single organ failure). In the United States, the annual incidence ...
Acute kidney injury (AKI), formerly known as acute renal failure (ARF), denotes a sudden and often reversible reduction in kidney function, as measured by glomerular filtration rate (GFR).[1][2][3] There is no clear definition of AKI. Several different criteria have been used in research studies, such as RIFLE, AKIN (Acute Kidney Injury Network), or KDIGO (Kidney Disease: Improving Global ...
PowerPoint Presentation. Case study. Patient David Smith. Situation. 68 year old gentleman admitted to Emergency Department with collapse. Background. Patient states feeling unwell for last 5 days and hasn't been eating and drinking as much as usual. Past medical history.
Acute kidney injury (AKI) is an abrupt and usually reversible decline in the glomerular filtration rate (GFR). Patients with AKI must be evaluated promptly to determine cause. Different disorders can BE associated with AKI, and biopsy is the most accurate instrument for diagnosis of different types of diseases. We report a case of 69-year-old ...
Acute kidney injury (AKI), also known as Acute Renal Failure (ARF) occurs when the kidneys lose their filtering ability resulting in the build-up of waste products in the blood.This condition develops rapidly, in hours or days, and is common in critically ill patients.. This condition affects other organs in the body if not treated promptly and can be life-threatening.
Acute kidney injury (AKI) is a heterogeneous disorder that is common in hospitalized patients and associated with short- and long-term morbidity and mortality. When AKI is present, prompt workup of the underlying cause should be pursued, with specific attention to reversible causes. Measures to prevent AKI include optimization of volume status and avoidance of nephrotoxic medications ...
A 68-year-old man was admitted to the hospital with fever, shortness of breath, and acute kidney injury. Testing of a nasopharyngeal swab for SARS-CoV-2 RNA was positive. Respiratory failure and hy...
Acute kidney injury (AKI), previously called acute renal failure (ARF), denotes a sudden and often reversible reduction in kidney function, as measured by glomerular filtration rate (GFR).[1][2][3] However, immediately after a renal insult, blood urea nitrogen (BUN) or creatinine (Cr) levels may be within the normal range, and the only sign of AKI may be a decline in urine output. AKI can lead ...
Comprehensive case study: acute kidney injury. Comprehensive case study: acute kidney injury Medsurg Nurs. 2013 Nov-Dec;22(6):400-2. Author Stephanie Gedeon 1 Affiliation 1 Henrico Doctors' Hospital ... Diabetic Angiopathies* / nursing Diabetic Angiopathies* / physiopathology ...
In the case of prerenal acute kidney injury (AKI), ... Stress the necessity of follow-up care and laboratory studies. Renal function may be slow to return following acute failure (up to 12 months), and deficits may persist, requiring changes in therapy to avoid recurrence. Renal recovery is usually observed within the first two weeks, and many ...
Exercise-induced acute kidney injury (EIAKI) is a major complication in patients with renal hypouricemia (RHUC). EIAKI usually develops after intense exercise, such as anaerobic exertion, and is not accompanied by rhabdomyolysis [].However, there are several case reports of patients experiencing EIAKI without intense exercise [].Although the pathomechanism and risk factors of EIAKI remain ...
Acute Renal Failure Case Study. Our kidneys are incredible organs that get rid of toxins, retain substances needed by our bodies, and maintain the right balance of electrolytes, minerals, and water. Find out what happens to this 27-year-old when toxins accumulate in her kidneys leading to acute renal failure.
Glomerular filtration rate (GFR) is the most-used kidney function index, with SCr and urine output changes serving as surro-gates for GFR changes. In young, healthy adults, normal GFR is approx-imately 120 mL/min/1.73m2; it decreases with age. Age, sex, and body size affect normal GFR readings.
Acute kidney injury (AKI) describes a sudden loss of excretory kidney function that can result in long-term kidney damage. ... which can also be reliably performed in the case of oliguria 132,133 ...
Nursing Assessment for Acute Kidney Injury. 1. Signs and symptoms result from the inability of the kidneys to regulate fluid and electrolytes. 2. Azotemia (retention of nitrogen wastes in the blood) → ↑ BUN/Creatinine. 4. Decreased urine output in the oliguric phase which should see an increase in diuretic phase. 5.
The nurse should monitor Mr. Brown for possible fluid retention related to acute kidney injury. Can you identify indicators of fluid volume excess? - Weight Gain. - Distended Neck Veins. Study with Quizlet and memorize flashcards containing terms like Prerenal Acute Kidney Injury, Intrarenal Acute Kidney Injury, Postrenal Acute Kidney Injury ...
Their findings, published in the Clinical Kidney Journal, predict kidney recovery within 90 days and 12 months after the start of dialysis for kidney failure patients due to AKI. The study looked ...
Researchers at the University of Cincinnati College of Medicine have created a scoring model that uses key health indicators to accurately predict recovery for patients who experience kidney failure due to acute kidney injury (AKI), which occurs when kidneys stop working properly and can range from minor loss of kidney function to complete failure.
Acute kidney injury (AKI) is associated with increased risk of heart failure (HF). Determining the type of HF experienced by AKI survivors (heart failure with preserved or reduced ejection fraction, HFpEF or HFrEF) could suggest potential mechanisms underlying the association and opportunities for improving post-AKI care. In this retrospective study of adults within the Vanderbilt University ...
In this study, we sought to evaluate the influence of positive pathogens in stool (PPS) on clinical outcomes in critical ill patients with Sepsis-associated acute kidney injury (S-AKI) from ...
1. Introduction. Acute kidney injury (AKI) is a frequent diagnosis with an incidence that varies from 5.0% to 7.5% in hospitalized patients and that reaches up to 50-60% in critically ill patients [1,2,3,4,5,6].AKI is characterized by an acute decrease in renal function that can be multifactorial in its origin and is associated with complex pathophysiological mechanisms [1,7].
Answer & Explanation. Case Study, Chapter 34: Acute Kidney Injury and Chronic Kidney Disease. Introduction: Will, a 68-year-old male with a history of hypertension, underwent regular dialysis therapy for End-Stage Renal Disease (ESRD) eight months ago. Prior to this, he experienced symptoms such as polyuria, nocturia, difficult hypertension ...
The purpose of this study was to develop a nomogram for predicting in-hospital mortality in cirrhotic patients with acute kidney injury (AKI) in order to identify patients with a high risk of in-hospital death early. This study collected data on cirrhotic patients with AKI from 2008 to 2019 using the Medical Information Mart for Intensive Care IV.
Acute kidney injury (AKI) is a significant risk factor associated with reduced survival following out-of-hospital cardiac arrest (OHCA). Whether the severity of AKI simply serves as a surrogate measure of worse peri-arrest conditions, or represents an additional risk to long-term survival remains unclear. This is a sub-study derived from a randomized trial in which 789 comatose adult OHCA ...
1 Urinary Function and Reproductive Function Case Studies Student's Name Title Professor's Name Date. 2 The attending physician is thinking that Mr. J.R. has developed an Acute Kidney Injury (AKI). Analyzing the case presented name the possible types of Acute Kidney Injury. Link the clinical manifestations described to the different types ...