Advertisement
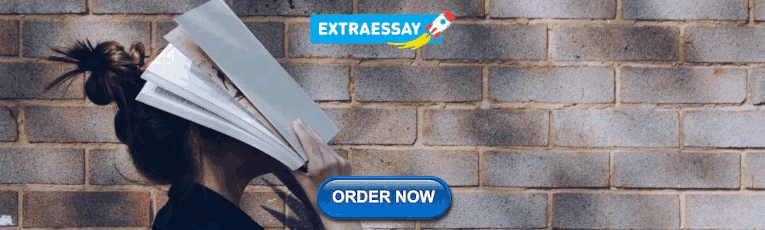
A review of natural fiber composites: properties, modification and processing techniques, characterization, applications
- Published: 16 September 2019
- Volume 55 , pages 829–892, ( 2020 )
Cite this article
- Aliakbar Gholampour ORCID: orcid.org/0000-0001-5069-2963 1 &
- Togay Ozbakkaloglu ORCID: orcid.org/0000-0003-3015-736X 2
18k Accesses
499 Citations
5 Altmetric
Explore all metrics
There has been much effort to provide eco-friendly and biodegradable materials for the next generation of composite products owing to global environmental concerns and increased awareness of renewable green resources. An increase in the use of natural materials in composites has led to a reduction in greenhouse gas emissions and carbon footprint of composites. In addition to the benefits obtained from green materials, there are some challenges in working with them, such as poor compatibility between the reinforcing natural fiber and matrix and the relatively high moisture absorption of natural fibers. Green composites can be a suitable alternative for petroleum-based materials. However, before this can be accomplished, there are a number of issues that need to be addressed, including poor interfacial adhesion between the matrix and natural fibers, moisture absorption, poor fire resistance, low impact strength, and low durability. Several researchers have studied the properties of natural fiber composites. These investigations have resulted in the development of several procedures for modifying natural fibers and resins. To address the increasing demand to use eco-friendly materials in different applications, an up-do-date review on natural fiber and resin types and sources, modification and processing techniques, physical and mechanical behaviors, applications, life-cycle assessment, and other properties of green composites is required to provide a better understanding of the behavior of green composites. This paper presents such a review based on 322 studies published since 1978.
This is a preview of subscription content, log in via an institution to check access.
Access this article
Price includes VAT (Russian Federation)
Instant access to the full article PDF.
Rent this article via DeepDyve
Institutional subscriptions
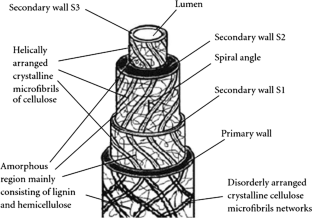
Similar content being viewed by others
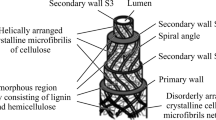
Advances in Natural-Fiber-Reinforced Composites: A Topical Review
Testing of Natural Fiber Composites
Different Natural Fiber Reinforced Composites and Its Potential Industrial and Domestic Applications: A Review
Campilho RDSG (2015) Natural fiber composites. CRC Press, Boca Raton
Google Scholar
Alix S, Colasse L, Morvan C, Lebrun L, Marais S (2014) Pressure impact of autoclave treatment on water sorption and pectin composition of flax cellulosic-fibres. Carbohydr Polym 102:21–29
CAS Google Scholar
Gurunathan T, Mohanty S, Nayak SK (2015) A review of the recent developments in biocomposites based on natural fibres and their application perspectives. Compos Part A Appl Sci Manuf 77:1–25
Faruk O, Bledzki AK, Fink H-P, Sain M (2012) Biocomposites reinforced with natural fibers: 2000–2010. Prog Poly Sci 37(11):1552–1596
Madsen B, Gamstedt EK (2013) Wood versus plant fibers: similarities and differences in composite applications. Adv Mater Sci Eng 2013:1–14
Rana AK, Mandal A, Bandyopadhyay S (2003) Short jute fiber reinforced polypropylene composites: effect of compatibiliser, impact modifier and fiber loading. Compos Sci Technol 63(6):801–806
Jawaid M, Khalil HPSA (2011) Cellulosic/synthetic fibre reinforced polymer hybrid composites: a review. Carbohydr Polym 86(1):1–18
Stamboulis A, Baillie CA, Peijs T (2001) Effects of environmental conditions on mechanical and physical properties of flax fibers. Compos Part A Appl Sci Manuf 32(8):1105–1115
Summerscales J (2018) Bast fibres and their composites. University of Plymouth, UK, https://www.fose1.plymouth.ac.uk/sme/mats347/bast_book.htm , Accessed 10 Aug 2019
George J, Sreekala MS, Thomas S (2001) A review on interface modification and characterization of natural fiber reinforced plastic composites. Polym Eng Sci 41(9):1471–1485
John MJ, Thomas S (2008) Biofibres and biocomposites. Carbohydr Polym 71(3):343–364
Zini E, Scandola M (2011) Green composites: an overview. Polym Compos 32(12):1905–1915
La Mantia FP, Morreale M (2011) Green composites: a brief review. Compos Part A Appl Sci Manuf 42(6):579–588
Ku H, Wang H, Pattarachaiyakoop N, Trada M (2011) A review on the tensile properties of natural fiber reinforced polymer composites. Compos Part B Eng 42(4):856–873
Dittenber DB, GangaRao HVS (2012) Critical review of recent publications on use of natural composites in infrastructure. Compos Part A Appl Sci Manuf 43(8):1419–1429
Khalil HPSA, Bhat AH, Yusra AFI (2012) Green composites from sustainable cellulose nanofibrils: a review. Carbohydr Polym 87(2):963–979
Koronis G, Silva A, Fontul M (2013) Green composites: a review of adequate materials for automotive applications. Compos Part B Eng 44(1):120–127
Nguong CW, Lee SNB, Debnath S (2013) A review on natural fibre reinforced polymer composites. World Acad Sci Eng Technol 7:1123–1130
Faruk O, Bledzki AK, Fink HP, Sain M (2014) Progress report on natural fiber reinforced composites. Macromol Mater Eng 299(1):9–26
Satyanarayana KG (2015) Recent developments in ‘green’ composites based on plant fibers-preparation, structure property studies. J Bioprocess Biotech 5(206):1–12
Mohammed L, Ansari MN, Pua G, Jawaid M, Islam MS (2015) A review on natural fiber reinforced polymer composite and its applications. Int J Polym Sci 2015:1–15
Pickering KL, Efendy MGA, Le TM (2016) A review of recent developments in natural fibre composites and their mechanical performance. Compos Part A Appl Sci Manuf 83:98–112
Sanjay MR, Madhu P, Jawaid M, Senthamaraikannan P, Senthil S, Pradeep S (2018) Characterization and properties of natural fiber polymer composites: a comprehensive review. J Clean Prod 172:566–581
Kalia S, Dufresne A, Cherian BM, Kaith BS, Avérous L, Njuguna J, Nassiopoulos E (2011) Cellulose-based bio-and nanocomposites: a review. Int J Polym Sci 2011:1–35
Mokhtar M (2007) Characterization and treatments of pineapple leaf fibre thermoplastic composite for construction application. Universiti Teknologi Malaysia, Johor Bahru
Alamri H, Low IM (2012) Mechanical properties and water absorption behaviour of recycled cellulose fibre reinforced epoxy composites. Polym Test 31(5):620–628
Masoodi R, Pillai KM (2012) A study on moisture absorption and swelling in bio-based jute-epoxy composites. J Reinf Plast Compos 31(5):285–294
Khalil HPSA, Bhat IUH, Jawaid M, Zaidon A, Hermawan D, Hadi YS (2012) Bamboo fibre reinforced biocomposites: a review. Mater Des 42:353–368
Chandramohan D, Marimuthu K (2011) A review on natural fibers. Int J Res Rev Appl Sci 8(2):194–206
Ramamoorthy SK, Skrifvars M, Persson A (2015) A review of natural fibers used in biocomposites: plant, animal and regenerated cellulose fibers. Polym Rev 55(1):107–162
Mohanty AK, Misra M, Drzal LT (2001) Surface modifications of natural fibers and performance of the resulting biocomposites: an overview. Compos Interfaces 8(5):313–343
Foulk JA, McAlister Iii DD (2002) Single cotton fiber properties of low, ideal, and high micronaire values. Text Res J 72(10):885–891
Mohanty AK, Misra M (1995) Studies on jute composites—a literature review. Polym Plast Technol Eng 34(5):729–792
Biswas S, Srikanth G, Nangia S (2001) Development of natural fibre composites in India. In: Proceedings of annual convention & trade show, Composites, Composites Fabricator’s Association at Tampa, Florida, USA, October 03–06
Yan L, Chouw N, Jayaraman K (2014) Flax fibre and its composites–a review. Compos Part B Eng 56:296–317
Shahzad A (2012) Hemp fiber and its composites—a review. J Compos Mater 46(8):973–986
Li Y, Mai Y-W, Ye L (2000) Sisal fibre and its composites: a review of recent developments. Compos Sci Technol 60(11):2037–2055
Akil H, Omar MF, Mazuki AAM, Safiee S, Ishak ZAM, Bakar AA (2011) Kenaf fiber reinforced composites: a review. Mater Des 32(8–9):4107–4121
Jayavani S, Deka H, Varghese TO, Nayak SK (2016) Recent development and future trends in coir fiber-reinforced green polymer composites: review and evaluation. Polym Compos 11(37):3296–3309
Pandey SN (2007) Ramie fibre: part I. Chemical composition and chemical properties. A critical review of recent developments. Text Prog 39(1):1–66
Balaji A, Karthikeyan B, Raj CS (2014) Bagasse fiber–the future biocomposite material: a review. Int J Cemtech Res 7(1):223–233
Sanadi AR, Caulfield DF, Jacobson RE, Rowell RM (1995) Renewable agricultural fibers as reinforcing fillers in plastics: mechanical properties of kenaf fiber-polypropylene composites. Ind Eng Chem Res 34(5):1889–1896
Mohanty AK, Misra M, Drzal LT (2005) Natural fibers, biopolymers, and biocomposites. CRC Press, Boca Raton
Hattalli S, Benaboura A, Ham-Pichavant F, Nourmamode A, Castellan A (2002) Adding value to Alfa grass ( Stipa tenacissima L.) soda lignin as phenolic resins 1. Lignin characterization. Polym Degrad Stab 76(2):259–264
Hoareau W, Trindade WG, Siegmund B, Castellan A, Frollini E (2004) Sugar cane bagasse and curaua lignins oxidized by chlorine dioxide and reacted with furfuryl alcohol: characterization and stability. Polym Degrad Stab 86(3):567–576
Malkapuram R, Kumar V, Negi YS (2009) Recent development in natural fiber reinforced polypropylene composites. J Reinf Plast Compos 28(10):1169–1189
Martí-Ferrer F, Vilaplana F, Ribes-Greus A, Benedito-Borrás A, Sanz-Box C (2006) Flour rice husk as filler in block copolymer polypropylene: effect of different coupling agents. J Appl Polym Sci 99(4):1823–1831
Rowell RM (2008) Natural fibres: types and properties. In: Pickering K (ed) Properties and performance of natural-fibre composites. Elsevier, Amsterdam, pp 3–66
Baeurle SA, Hotta A, Gusev AA (2006) On the glassy state of multiphase and pure polymer materials. Polymer 47(17):6243–6253
Lokensgard E (2016) Industrial plastics: theory and applications, 6th edn. Cengage Learning, p 544
Sinha R (2004) Outlines of polymer technology: manufacture of polymers. Prentice Hall of India Private Limited, India, New Delhi
Mohammad NAB (2007) Synthesis, characterization, and properties of the new unsaturated polyester resins for composite applications. MARA University of Technology, pp 45–56
Iijima T, Tochimoto T, Tomoi M (1991) Modification of epoxy resins with poly (aryl ether ketone) s. J Appl Polym Sci 43(9):1685–1692
Mukherjee RN, Pal SK, Sanyal SK, Phani KK (1984) Role of interface in fibre reinforced polymer composites with special reference to natural fibres. J Polym Mater 1:69–81
Pritchard G (2012) Developments in reinforced plastics—4. Elsevier Applied Science Publishers, New York
Sarkar BK, Roy R, Ray D (1997) Emerging dominance of composites as a structural material. J Inst Eng India Part MM Metall Mater Sci Div 78:31–37
Bastioli C (2001) Global status of the production of biobased packaging materials. Starch-Stärke 53(8):351–355
Edgar KJ, Buchanan CM, Debenham JS, Rundquist PA, Seiler BD, Shelton MC, Tindall D (2001) Advances in cellulose ester performance and application. Prog Polym Sci 26(9):1605–1688
TiaGe Volova (2004) Polyhydroxyalkanoates—plastic materials of the 21st century: production, properties, applications. Nova Publishers, New York
Sharma SK, Mudhoo A (2011) A handbook of applied biopolymer technology: synthesis, degradation and applications. Royal Society of Chemistry, London
PolymerOhio (2015) Bio-based resins: a growing opportunity for bioplastics packaging. Polymers PolymerOhio
Kuruppalil Z (2011) Green plastics: an emerging alternative for petroleum-based plastics. Int J Eng Res Innov 3(1):59–64
Lilholt H, Lawther JM (2000) Natural organic fibers. In: Kelly A, Zweben C (eds) Comprehensive composite materials, vol 1. Elsevier Science, Amsterdam
Gassan J, Gutowski VS (2000) Effects of corona discharge and UV treatment on the properties of jute-fibre epoxy composites. Compos Sci Technol 60(15):2857–2863
Bataille P, Dufourd M, Sapieha S (1994) Copolymerization of styrene on to cellulose activated by corona. Polym Int 34(4):387–391
Mukhopadhyay S, Fangueiro R (2009) Physical modification of natural fibers and thermoplastic films for composites—a review. J Thermoplast Compos Mater 22(2):135–162
Marais S, Gouanvé F, Bonnesoeur A, Grenet J, Poncin-Epaillard F, Morvan C, Métayer M (2005) Unsaturated polyester composites reinforced with flax fibers: effect of cold plasma and autoclave treatments on mechanical and permeation properties. Compos Part A Appl Sci Manuf 36(7):975–986
Martin AR, Manolache S, Mattoso LHC, Rowell RM, Denes F Plasma modification of sisal and high-density polyethylene composites: effect on mechanical properties. In, 2000 2000
di Benedetto RM, Gelfuso MV, Thomazini D (2015) Influence of UV radiation on the physical-chemical and mechanical properties of banana fiber. Mater Res 18:265–272
Beg MDH, Pickering KL (2008) Mechanical performance of Kraft fibre reinforced polypropylene composites: influence of fibre length, fibre beating and hygrothermal ageing. Compos Part A Appl Sci Manuf 39(11):1748–1755
Cao Y, Sakamoto S, Goda K (2007) Effects of heat and alkali treatments on mechanical properties of kenaf fibers. In: 16th international conference on composite materials, Kyoto, Japan, vol 1, pp 8–13
Rong MZ, Zhang MQ, Liu Y, Yang GC, Zeng HM (2001) The effect of fiber treatment on the mechanical properties of unidirectional sisal-reinforced epoxy composites. Compos Sci Technol 61(10):1437–1447
Singh B, Gupta M, Verma A (1996) Influence of fiber surface treatment on the properties of sisal-polyester composites. Polym Compos 17(6):910–918
Ray D, Sarkar BK, Rana AK, Bose NR (2001) The mechanical properties of vinylester resin matrix composites reinforced with alkali-treated jute fibres. Compos Part A Appl Sci Manuf 32(1):119–127
Iannace S, Ali R, Nicolais L (2001) Effect of processing conditions on dimensions of sisal fibers in thermoplastic biodegradable composites. J Appl Polym Sci 79(6):1084–1091
Walker JCF (2006) Primary wood processing: principles and practice. Springer, Berlin
Brígida AIS, Calado VMA, Gonçalves LRB, Coelho MAZ (2010) Effect of chemical treatments on properties of green coconut fiber. Carbohydr Polym 79(4):832–838
Aziz SH, Ansell MP (2004) The effect of alkalization and fibre alignment on the mechanical and thermal properties of kenaf and hemp bast fibre composites: part 1–polyester resin matrix. Compos Sci Technol 64(9):1219–1230
Suardana NPG, Piao Y, Lim JK (2011) Mechanical properties of hemp fibers and hemp/pp composites: effects of chemical surface treatment. Mater Phys Mech 11(1):1–8
Bledzki AK, Mamun AA, Lucka-Gabor M, Gutowski VS (2008) The effects of acetylation on properties of flax fibre and its polypropylene composites. Express Polym Lett 2(6):413–422
Li X, Tabil LG, Panigrahi S (2007) Chemical treatments of natural fiber for use in natural fiber-reinforced composites: a review. J Polym Environ 15(1):25–33
Tserki V, Zafeiropoulos NE, Simon F, Panayiotou C (2005) A study of the effect of acetylation and propionylation surface treatments on natural fibres. Compos Part A Appl Sci Manuf 36(8):1110–1118
Zafeiropoulos NE, Baillie CA (2007) A study of the effect of surface treatments on the tensile strength of flax fibres: part II. Application of Weibull statistics. Compos Part A Appl Sci Manuf 38(2):629–638
Manikandan Nair KC, Diwan SM, Thomas S (1996) Tensile properties of short sisal fiber reinforced polystyrene composites. J Appl Polym Sci 60(9):1483–1497
Wang B, Panigrahi S, Tabil L, Crerar W (2007) Pre-treatment of flax fibers for use in rotationally molded biocomposites. J Reinf Plast Compos 26(5):447–463
Joseph K, Mattoso LHC, Toledo RD, Thomas S, De Carvalho LH, Pothen L, Kala S, James B (2000) Natural fiber reinforced thermoplastic composites. Nat Polym Agrofibers Compos 159:159–201
Joseph PV, Joseph K, Thomas S, Pillai CKS, Prasad VS, Groeninckx G, Sarkissova M (2003) The thermal and crystallisation studies of short sisal fibre reinforced polypropylene composites. Compos Part A Appl Sci Manuf 34(3):253–266
Aranberri-Askargorta I, Lampke T, Bismarck A (2003) Wetting behavior of flax fibers as reinforcement for polypropylene. J Colloid Interface Sci 263(2):580–589
Bismarck A, Aranberri-Askargorta I, Springer J, Lampke T, Wielage B, Stamboulis A, Shenderovich I, Limbach HH (2002) Surface characterization of flax, hemp and cellulose fibers; surface properties and the water uptake behavior. Polym Compos 23(5):872–894
Fuqua MA, Ulven CA (2008) Characterization of polypropylene/corn fiber composites with maleic anhydride grafted polypropylene. J Biobased Mater Bioenergy 2(3):258–263
Hong CK, Kim N, Kang SL, Nah C, Lee YS, Cho BH, Ahn JH (2008) Mechanical properties of maleic anhydride treated jute fibre/polypropylene composites. Plast, Rubber Compos 37(7):325–330
Sun JX, Sun XF, Zhao H, Sun RC (2004) Isolation and characterization of cellulose from sugarcane bagasse. Polym Degrad Stab 84(2):331–339
Li X, Panigrahi S, Tabil LG (2009) A study on flax fiber-reinforced polyethylene biocomposites. Appl Eng Agric 25(4):525–531
Vilay V, Mariatti M, Taib RM, Todo M (2008) Effect of fiber surface treatment and fiber loading on the properties of bagasse fiber—reinforced unsaturated polyester composites. Compos Sci Technol 68(3–4):631–638
Sreekala MS, Kumaran MG, Joseph S, Jacob M, Thomas S (2000) Oil palm fibre reinforced phenol formaldehyde composites: influence of fibre surface modifications on the mechanical performance. Appl Compos Mater 7(5–6):295–329
George J, Janardhan R, Anand JS, Bhagawan SS, Thomas S (1996) Melt rheological behaviour of short pineapple fibre reinforced low density polyethylene composites. Polymer 37(24):5421–5431
Paul SA, Oommen C, Joseph K, Mathew G, Thomas S (2010) The role of interface modification on thermal degradation and crystallization behavior of composites from commingled polypropylene fiber and banana fiber. Polym Compos 31(6):1113–1123
Zafeiropoulos NE, Baillie CA, Hodgkinson JM (2002) Engineering and characterisation of the interface in flax fibre/polypropylene composite materials. Part II. The effect of surface treatments on the interface. Compos Part A Appl Sci Manuf 33(9):1185–1190
Corrales F, Vilaseca F, Llop M, Girones J, Mendez JA, Mutje P (2007) Chemical modification of jute fibers for the production of green-composites. J Hazard Mater 144(3):730–735
Li X, Panigrahi SA, Tabil LG, Crerar WJ (2004) Flax fiber-reinforced composites and the effect of chemical treatments on their properties. In: ASAE/CSAE North Central regional annual meeting, Manitoba, Canada, September 24–25 2004. ASAE, pp 1–10
Zadorecki P, Flodin P (1985) Surface modification of cellulose fibers. II. The effect of cellulose fiber treatment on the performance of cellulose–polyester composites. J Appl Polym Sci 30(10):3971–3983
Pickering KL, Li Y, Farrell RL, Lay M (2007) Interfacial modification of hemp fiber reinforced composites using fungal and alkali treatment. J Biobased Mater Bioenergy 1(1):109–117
Chabba S, Netravali AN (2004) ‘Green’composites using modified soy protein concentrate resin and flax fabrics and yarns. JSME Int J Ser A Solid Mech Mater Eng 47(4):556–560
Kumar R, Zhang L (2008) Water-induced hydrophobicity of soy protein materials containing 2, 2-diphenyl-2-hydroxyethanoic acid. Biomacromol 9(9):2430–2437
Kumar R, Zhang L (2009) Soy protein films with the hydrophobic surface created through non-covalent interactions. Ind Crops Prod 29(2–3):485–494
Newill JR, McKnight SH, Hoppel CP, Cooper GR (1999) Effects of coatings on moisture absorption in composite materials. Army Research Lab Aberdeen Proving Ground MD, US
Doherty W, Halley P, Edye L, Rogers D, Cardona F, Park Y, Woo T (2007) Studies on polymers and composites from lignin and fiber derived from sugar cane. Polym Adv Technol 18(8):673–678
Stark NM (1999) Wood fiber derived from scrap pallets used in polypropylene composites. For Prod J 49:39–46
Araujo JR, Waldman WR, De Paoli MA (2008) Thermal properties of high density polyethylene composites with natural fibres: coupling agent effect. Polym Degrad Stab 93(10):1770–1775
Yildiz S, Gezer ED, Yildiz UC (2006) Mechanical and chemical behavior of spruce wood modified by heat. Build Environ 41(12):1762–1766
Chand N, Dwivedi UK (2006) Effect of coupling agent on abrasive wear behaviour of chopped jute fibre-reinforced polypropylene composites. Wear 261(10):1057–1063
Corradini E, Ito EN, Marconcini JM, Rios CT, Agnelli JAM, Mattoso LHC (2009) Interfacial behavior of composites of recycled poly (ethyelene terephthalate) and sugarcane bagasse fiber. Polym Test 28(2):183–187
Elkington M, Bloom D, Ward C, Chatzimichali A, Potter K (2015) Hand layup: understanding the manual process. Adv Manuf Polym Compos Sci 1(3):138–151
Kumar AP, Singh RP, Sarwade BD (2005) Degradability of composites, prepared from ethylene–propylene copolymer and jute fiber under accelerated aging and biotic environments. Mater Chem Phys 92(2–3):458–469
Mulinari DR, Voorwald HJC, Cioffi MOH, Da Silva MLCP, da Cruz TG, Saron C (2009) Sugarcane bagasse cellulose/HDPE composites obtained by extrusion. Compos Sci Technol 69(2):214–219
Richardson MOW, Zhang ZY (2000) Experimental investigation and flow visualisation of the resin transfer mould filling process for non-woven hemp reinforced phenolic composites. Compos Part A Appl Sci Manuf 31(12):1303–1310
Rouison D, Sain M, Couturier M (2004) Resin transfer molding of natural fiber reinforced composites: cure simulation. Compos Sci Techno 64(5):629–644
Rouison D, Sain M, Couturier M (2006) Resin transfer molding of hemp fiber composites: optimization of the process and mechanical properties of the materials. Compos Sci Technol 66(7–8):895–906
Santos PA, Spinacé MAS, Fermoselli KKG, De Paoli M-A (2007) Polyamide-6/vegetal fiber composite prepared by extrusion and injection molding. Compos Part A Appl Sci Manuf 38(12):2404–2411
Scherübl B, Hintermann M (2005) Application of natural fibre reinforced plastics for automotive exterior parts, with a focus on underfloor systems. In: Proceedings of the 8th international AVK-TV conference, Baden, Germany, 2005. p D5
Van Voorn B, Smit HHG, Sinke RJ, De Klerk B (2001) Natural fibre reinforced sheet moulding compound. Compos Part A Appl Sci Manuf 32(9):1271–1279
Zou Y, Huda S, Yang Y (2010) Lightweight composites from long wheat straw and polypropylene web. Bioresour Technol 101(6):2026–2033
Dai D, Fan M (2014) Wood fibres as reinforcements in natural fibre composites: structure, properties, processing and applications. In: Hodzic A, Shanks R (Eds) Natural fibre composites. Elsevier, Amsterdam, pp 3–65
Sebe G, Cetin NS, Hill CAS, Hughes M (2000) RTM hemp fibre-reinforced polyester composites. Appl Compos Mater 7(5–6):341–349
Summerscales J, Searle TJ (2005) Low-pressure (vacuum infusion) techniques for moulding large composite structures. Proc Inst Mech Eng Part L J Mater Des Appl 219(1):45–58
Francucci G, Rodríguez ES, Vázquez A (2010) Study of saturated and unsaturated permeability in natural fiber fabrics. Compos Part A Appl Sci Manuf 41(1):16–21
Masoodi R, Pillai KM, Grahl N, Tan H (2012) Numerical simulation of LCM mold-filling during the manufacture of natural fiber composites. J Reinf Plast Compos 31(6):363–378
Goren A, Atas C (2008) Manufacturing of polymer matrix composites using vacuum assisted resin infusion molding. Arch Mater Sci Eng 34(2):117–120
Ashby MF (2012) Materials and the environment: eco-informed material choice. Elsevier, Amsterdam
Lystrup A, Løgostrup T, Knudsen H, Vestergaard T, Lilleheden L, Vestergaard J (1998) Hybrid yarn for thermoplastic fiber composites. Summary of technical results. Final report for MUP2 Framework Program, Denmark
Baley C, Lan M, Davies P, Cartié D (2015) Porosity in ocean racing yacht composites: a review. Appl Compos Mater 22(1):13–28
Madsen B, Lilholt H (2003) Physical and mechanical properties of unidirectional plant fibre composites—an evaluation of the influence of porosity. Compos Sci Technol 63(9):1265–1272
Vaxman A, Narkis M, Siegmann A, Kenig S (1989) Void formation in short-fiber thermoplastic composites. Polym Compos 10(6):449–453
Bowles KJ, Frimpong S (1992) Void effects on the interlaminar shear strength of unidirectional graphite-fiber-reinforced composites. J Compos Mater 26(10):1487–1509
Dong C, Takagi H (2014) Flexural properties of cellulose nanofibre reinforced green composites. Compos Part B Eng 58:418–421
Judd NCW, Wright WW (1978) Voids and their effects on the mechanical properties of composites—an appraisal. SAMPE J 14:10–14
Ghiorse SR (1993) Effect of void content on the mechanical properties of carbon/epoxy laminates. SAMPE Q 24:54–59
Zampaloni M, Pourboghrat F, Yankovich SA, Rodgers BN, Moore J, Drzal LT, Mohanty AK, Misra M (2007) Kenaf natural fiber reinforced polypropylene composites: a discussion on manufacturing problems and solutions. Compos Part A Appl Sci Manuf 38(6):1569–1580
Joseph S, Sreekala MS, Oommen Z, Koshy P, Thomas S (2002) A comparison of the mechanical properties of phenol formaldehyde composites reinforced with banana fibres and glass fibres. Compos Sci Technol 62(14):1857–1868
Biswas S, Kindo S, Patnaik A (2011) Effect of fiber length on mechanical behavior of coir fiber reinforced epoxy composites. Fibers Polym 12(1):73–78
Gao S-L, Mäder E (2006) Jute/polypropylene composites I. Effect of matrix modification. Compos Sci Technol 66(7–8):952–963
Karmaker AC, Shneider JP (1996) Mechanical performance of short jute fibre reinforced polypropylene. J Mater Sci Lett 15(3):201–202
Shibata S, Cao Y, Fukumoto I (2005) Press forming of short natural fiber-reinforced biodegradable resin: effects of fiber volume and length on flexural properties. Polym Test 24(8):1005–1011
Sharma NK, Kumar V (2013) Studies on properties of banana fiber reinforced green composite. J Reinf Plast Compos 32(8):525–532
Liu Q, Hughes M (2008) The fracture behaviour and toughness of woven flax fibre reinforced epoxy composites. Compos Part A Appl Sci Manuf 39(10):1644–1652
Zhang Y, Li Y, Ma H, Yu T (2013) Tensile and interfacial properties of unidirectional flax/glass fiber reinforced hybrid composites. Compos Sci Technol 88:172–177
Li Y, Chen C, Xu J, Zhang Z, Yuan B, Huang X (2015) Improved mechanical properties of carbon nanotubes-coated flax fiber reinforced composites. J Mater Sci 50(3):1117–1128. https://doi.org/10.1007/s10853-014-8668-3
Article CAS Google Scholar
Kafi AA, Magniez K, Fox BL (2011) Effect of manufacturing process on the flexural, fracture toughness, and thermo-mechanical properties of bio-composites. Compos Part A Appl Sci Manuf 42(8):993–999
Kinloch AJ, Taylor AC, Techapaitoon M, Teo WS, Sprenger S (2015) Tough, natural-fibre composites based upon epoxy matrices. J Mater Sci 50(21):6947–6960. https://doi.org/10.1007/s10853-015-9246-z
Hsieh TH, Kinloch AJ, Masania K, Lee JS, Taylor AC, Sprenger S (2010) The toughness of epoxy polymers and fibre composites modified with rubber microparticles and silica nanoparticles. J Mater Sci 45(5):1193–1210. https://doi.org/10.1007/s10853-009-4064-9
Agunsoye JO, Aigbodion VS (2013) Bagasse filled recycled polyethylene bio-composites: morphological and mechanical properties study. Results Phys 3:187–194
Wong KJ, Zahi S, Low KO, Lim CC (2010) Fracture characterisation of short bamboo fibre reinforced polyester composites. Mater Des 31(9):4147–4154
Muralidhar BA (2013) Tensile and compressive behaviour of multilayer flax-rib knitted preform reinforced epoxy composites. Mater Des 49:400–405
Bledzki AK, Gassan J, Zhang W (1999) Impact properties of natural fiber-reinforced epoxy foams. J Cell Plast 35(6):550–562
Cao Y, Shibata S, Fukumoto I (2006) Mechanical properties of biodegradable composites reinforced with bagasse fibre before and after alkali treatments. Compos Part A Appl Sci Manuf 37(3):423–429
Dhakal HN, Zhang ZY, Richardson MOW, Errajhi OAZ (2007) The low velocity impact response of non-woven hemp fibre reinforced unsaturated polyester composites. Compos Struct 81(4):559–567
Jandas PJ, Mohanty S, Nayak SK (2013) Mechanical properties of surface-treated banana fiber/polylactic acid biocomposites: a comparative study of theoretical and experimental values. J Appl Polym Sci 127(5):4027–4038
Liang S, Guillaumat L, Gning P-B (2015) Impact behaviour of flax/epoxy composite plates. Int J Impact Eng 80:56–64
Clarinval AM, Halleux J (2005) Classification of biodegradable polymers. In: Smith R (Ed) Biodegradable polymers for industrial applications. Elsevier, Amsterdam, pp 3–31
Bowen CR, Dent AC, Stevens R, Cain M, Stewart M (2005) Determination of critical and minimum volume fraction for composite sensors and actuators. In: First international conference on multi-material micro manufacture, Cardiff, UK, 2005. Elsevier, pp 1–4
Facca AG, Kortschot MT, Yan N (2007) Predicting the tensile strength of natural fibre reinforced thermoplastics. Compos Sci Technol 67(11–12):2454–2466
Ma X, Yu J, Kennedy JF (2005) Studies on the properties of natural fibers-reinforced thermoplastic starch composites. Carbohydr Polym 62(1):19–24
Węcławski BT, Fan M, Hui D (2014) Compressive behaviour of natural fibre composite. Compos Part B Eng 67:183–191
Afroughsabet V, Ozbakkaloglu T (2015) Mechanical and durability properties of high-strength concrete containing steel and polypropylene fibers. Constr Build Mater 94:73–82
Ismail MA (2007) Compressive and tensile strength of natural fibre-reinforced cement base composites. AL Rafdain Eng J 15(2):42–51
Gozde Ozerkan N, Ahsan B, Mansour S, Iyengar SR (2013) Mechanical performance and durability of treated palm fiber reinforced mortars. Int J Sustain Built Environ 2(2):131–142
Ragoubi M, Bienaimé D, Molina S, George B, Merlin A (2010) Impact of corona treated hemp fibres onto mechanical properties of polypropylene composites made thereof. Ind Crops Prod 31(2):344–349
Pizzi A, Kueny R, Lecoanet F, Massetau B, Carpentier D, Krebs A, Loiseau F, Molina S, Ragoubi M (2009) High resin content natural matrix–natural fibre biocomposites. Ind Crops Prod 30(2):235–240
Ragoubi M, George B, Molina S, Bienaimé D, Merlin A, Hiver JM, Dahoun A (2012) Effect of corona discharge treatment on mechanical and thermal properties of composites based on miscanthus fibres and polylactic acid or polypropylene matrix. Compos Part A Appl Sci Manuf 43(4):675–685
Seki Y, Sever K, Sarikanat M, Güleç HA, Tavman IH (2009) The influence of oxygen plasma treatment of jute fibers on mechanical properties of jute fiber reinforced thermoplastic composites. In: 5th international advanced technologies symposium (IATS’09), Karabuk, Turkey 2009. pp 1–4
Sinha E, Panigrahi S (2009) Effect of plasma treatment on structure, wettability of jute fiber and flexural strength of its composite. J Compos Mater 43(17):1791–1802
Seki Y, Sarikanat M, Sever K, Erden S, Gulec HA (2010) Effect of the low and radio frequency oxygen plasma treatment of jute fiber on mechanical properties of jute fiber/polyester composite. Fibers Polym 11(8):1159–1164
Jang JY, Jeong TK, Oh HJ, Youn JR, Song YS (2012) Thermal stability and flammability of coconut fiber reinforced poly (lactic acid) composites. Compos Part B Eng 43(5):2434–2438
Prasad SV, Pavithran C, Rohatgi PK (1983) Alkali treatment of coir fibres for coir-polyester composites. J Mater Sci 18(5):1443–1454. https://doi.org/10.1007/BF01111964
Ray D, Sarkar BK, Rana AK, Bose NR (2001) Effect of alkali treated jute fibres on composite properties. Bull Mater Sci 24(2):129–135
Onal L, Karaduman Y (2009) Mechanical characterization of carpet waste natural fiber-reinforced polymer composites. J Compos Mater 43(16):1751–1768
Huda MS, Drzal LT, Mohanty AK, Misra M (2008) Effect of chemical modifications of the pineapple leaf fiber surfaces on the interfacial and mechanical properties of laminated biocomposites. Compos Interfaces 15(2–3):169–191
Qin C, Soykeabkaew N, Xiuyuan N, Peijs T (2008) The effect of fibre volume fraction and mercerization on the properties of all-cellulose composites. Carbohydr Polym 71(3):458–467
Bledzki AK, Fink HP, Specht K (2004) Unidirectional hemp and flax EP-and PP-composites: influence of defined fiber treatments. J Appl Polym Sci 93(5):2150–2156
Venkateshwaran N, Perumal AE, Arunsundaranayagam D (2013) Fiber surface treatment and its effect on mechanical and visco-elastic behaviour of banana/epoxy composite. Mater Des 47:151–159
Xu Y, Kawata S, Hosoi K, Kawai T, Kuroda S (2009) Thermomechanical properties of the silanized-kenaf/polystyrene composites. Express Polym Lett 3(10):657–664
Pothan LA, Thomas S (2003) Polarity parameters and dynamic mechanical behaviour of chemically modified banana fiber reinforced polyester composites. Compos Sci Technol 63(9):1231–1240
Cantero G, Arbelaiz A, Llano-Ponte R, Mondragon I (2003) Effects of fibre treatment on wettability and mechanical behaviour of flax/polypropylene composites. Compos Sci Technol 63(9):1247–1254
Abdul Khalil HPS, Ismail H (2000) Effect of acetylation and coupling agent treatments upon biological degradation of plant fibre reinforced polyester composites. Polym Test 20(1):65–75
Ismail H, Khalil HPSA (2000) The effects of partial replacement of oil palm wood flour by silica and silane coupling agent on properties of natural rubber compounds. Polym Test 20(1):33–41
Sgriccia N, Hawley MC, Misra M (2008) Characterization of natural fiber surfaces and natural fiber composites. Compos Part A Appl Sci Manuf 39(10):1632–1637
Eng CC, Ibrahim NA, Zainuddin N, Ariffin H, Yunus WM, Wan Z (2014) Impact strength and flexural properties enhancement of methacrylate silane treated oil palm mesocarp fiber reinforced biodegradable hybrid composites. Sci World J 2014:1–8
Joseph S, Koshy P, Thomas S (2005) The role of interfacial interactions on the mechanical properties of banana fibre reinforced phenol formaldehyde composites. Compos Interfaces 12(6):581–600
Hill CAS, Abdul Khalil HPS (2000) Effect of fiber treatments on mechanical properties of coir or oil palm fiber reinforced polyester composites. J Appl Polym Sci 78(9):1685–1697
Zafeiropoulos NE, Williams DR, Baillie CA, Matthews FL (2002) Engineering and characterisation of the interface in flax fibre/polypropylene composite materials. Part I. Development and investigation of surface treatments. Compos Part A Appl Sci Manuf 33(8):1083–1093
Luz SM, Del Tio J, Rocha GJM, Gonçalves AR, Del’Arco AP Jr (2008) Cellulose and cellulignin from sugarcane bagasse reinforced polypropylene composites: effect of acetylation on mechanical and thermal properties. Compos Part A Appl Sci Manuf 39(9):1362–1369
Manikandan Nair KC, Thomas S, Groeninckx G (2001) Thermal and dynamic mechanical analysis of polystyrene composites reinforced with short sisal fibres. Compos Sci Technol 61(16):2519–2529
Sapieha S, Allard P, Zang YH (1990) Dicumyl peroxide-modified cellulose/LLDPE composites. J Appl Polym Sci 41(9–10):2039–2048
Abdul Razak N, Ibrahim N, Zainuddin N, Rayung M, Saad W (2014) The influence of chemical surface modification of kenaf fiber using hydrogen peroxide on the mechanical properties of biodegradable kenaf fiber/poly (lactic acid) composites. Molecules 19(3):2957–2968
Yang H-S, Kim H-J, Park H-J, Lee B-J, Hwang T-S (2007) Effect of compatibilizing agents on rice-husk flour reinforced polypropylene composites. Compos Struct 77(1):45–55
Li X, Zhang J, He J, Jeevan Prasad Reddy D, Varada Rajulu A (2010) Tensile properties of hildegardia fibers reinforced polypropylene biocomposites. J Compos Mater 44(14):1681–1687
Keener TJ, Stuart RK, Brown TK (2004) Maleated coupling agents for natural fibre composites. Compos Part A Appl Sci Manuf 35(3):357–362
Liu H, Wu Q, Zhang Q (2009) Preparation and properties of banana fiber-reinforced composites based on high density polyethylene (HDPE)/Nylon-6 blends. Bioresour Technol 100(23):6088–6097
Gassan J, Bledzki AK (2000) Possibilities to improve the properties of natural fiber reinforced plastics by fiber modification–Jute polypropylene composites–. Appl Compos Mater 7(5–6):373–385
Wielage B, Lampke T, Utschick H, Soergel F (2003) Processing of natural-fibre reinforced polymers and the resulting dynamic—mechanical properties. J Mater Process Technol 139(1–3):140–146
Misra S, Misra M, Tripathy SS, Nayak SK, Mohanty AK (2002) The influence of chemical surface modification on the performance of sisal-polyester biocomposites. Polym Compos 23(2):164–170
Sreekala MS, Kumaran MG, Thomas S (2002) Water sorption in oil palm fiber reinforced phenol formaldehyde composites. Compos Part A Appl Sci Manuf 33(6):763–777
Joseph K, Thomas S, Pavithran C (1996) Effect of chemical treatment on the tensile properties of short sisal fibre-reinforced polyethylene composites. Polymer 37(23):5139–5149
Paul SA, Boudenne A, Ibos L, Candau Y, Joseph K, Thomas S (2008) Effect of fiber loading and chemical treatments on thermophysical properties of banana fiber/polypropylene commingled composite materials. Compos Part A Appl Sci Manuf 39(9):1582–1588
Kalaprasad G, Francis B, Thomas S, Kumar CR, Pavithran C, Groeninckx G, Thomas S (2004) Effect of fibre length and chemical modifications on the tensile properties of intimately mixed short sisal/glass hybrid fibre reinforced low density polyethylene composites. Polym Int 53(11):1624–1638
Torres FG, Cubillas ML (2005) Study of the interfacial properties of natural fibre reinforced polyethylene. Polym Test 24(6):694–698
Mwaikambo LY, Ansell MP (2002) Chemical modification of hemp, sisal, jute, and kapok fibers by alkalization. J Appl Polym Sci 84(12):2222–2234
Guan J, Hanna MA (2006) Selected morphological and functional properties of extruded acetylated starch–cellulose foams. Bioresour Technol 97(14):1716–1726
Joonobi M, Harun J, Tahir PM, Zaini LH, SaifulAzry S, Makinejad MD (2010) Characteristic of nanofibers extracted from kenaf core. BioResources 5(4):2556–2566
Barone JR (2005) Polyethylene/keratin fiber composites with varying polyethylene crystallinity. Compos Part A Appl Sci Manuf 36(11):1518–1524
Zafeiropoulos NE, Baillie CA, Matthews FL (2001) An investigation of the effect of processing conditions on the interface of flax/polypropylene composites. Adv Compos Lett 10(6):293–297
Suryanegara L, Nakagaito AN, Yano H (2009) The effect of crystallization of PLA on the thermal and mechanical properties of microfibrillated cellulose-reinforced PLA composites. Compos Sci Technol 69(7–8):1187–1192
Quan H, Li Z-M, Yang M-B, Huang R (2005) On transcrystallinity in semi-crystalline polymer composites. Compos Sci Technol 65(7–8):999–1021
Zafeiropoulos NE, Baillie CA, Matthews FL (2001) A study of transcrystallinity and its effect on the interface in flax fibre reinforced composite materials. Compos Part A Appl Sci Manuf 32(3–4):525–543
Zafeiropoulos NE, Baillie CA, Matthews FL (2001) The effect of transcrystallinity on the interface of green flax/polypropylene composite materials. Adv Compos Lett 10(5):229–236
Sawpan MA, Pickering KL, Fernyhough A (2011) Effect of fibre treatments on interfacial shear strength of hemp fibre reinforced polylactide and unsaturated polyester composites. Compos Part A Appl Sci Manuf 42(9):1189–1196
Xiong X, Shen SZ, Hua L, Liu JZ, Li X, Wan X, Miao M (2018) Finite element models of natural fibers and their composites: a review. J Reinf Plast Compos 37(9):617–635
Ilczyszyn F, Cherouat A, Montay G Effect of hemp fibre morphology on the mechanical properties of vegetal fibre composite material. In, 2014 2014. Trans Tech Publ, pp 485-489
Virk AS, Hall W, Summerscales J (2012) Modulus and strength prediction for natural fibre composites. Mater Sci Technol 28(7):864–871
Kalaprasad G, Joseph K, Thomas S, Pavithran C (1997) Theoretical modelling of tensile properties of short sisal fibre-reinforced low-density polyethylene composites. J Mater Sci 32(16):4261–4267. https://doi.org/10.1023/A:1018651218515
Facca AG, Kortschot MT, Yan N (2006) Predicting the elastic modulus of natural fibre reinforced thermoplastics. Compos Part A Appl Sci Manuf 37(10):1660–1671
Beckermann GW, Pickering KL (2009) Engineering and evaluation of hemp fibre reinforced polypropylene composites: micro-mechanics and strength prediction modelling. Compos Part A Appl Sci Manuf 40(2):210–217
Migneault S, Koubaa A, Erchiqui F, Chaala A, Englund K, Wolcott MP (2011) Application of micromechanical models to tensile properties of wood–plastic composites. Wood Sci Technol 45(3):521–532
Munde YS, Ingle RB (2015) Theoretical modeling and experimental verification of mechanical properties of natural fiber reinforced thermoplastics. Proc Technol 19:320–326
Kern WT, Kim W, Argento A, Lee EC, Mielewski DF (2016) Finite element analysis and microscopy of natural fiber composites containing microcellular voids. Mater Des 106:285–294
Modniks J, Andersons J (2010) Modeling elastic properties of short flax fiber-reinforced composites by orientation averaging. Comput Mater Sci 50(2):595–599
Modniks J, Andersons J (2013) Modeling the non-linear deformation of a short-flax-fiber-reinforced polymer composite by orientation averaging. Compos Part B Eng 54:188–193
Sliseris J, Yan L, Kasal B (2016) Numerical modelling of flax short fibre reinforced and flax fibre fabric reinforced polymer composites. Compos Part B Eng 89:143–154
Stamboulis A, Baillie CA, Garkhail SK, Van Melick HGH, Peijs T (2000) Environmental durability of flax fibres and their composites based on polypropylene matrix. Appl Compos Mater 7(5–6):273–294
Apolinario G, Ienny P, Corn S, Léger R, Bergeret A, Haudin J-M (2016) Effects of water ageing on the mechanical properties of flax and glass fibre composites: degradation and reversibility. In: Natural fibres: advances in science and technology towards industrial applications. Springer, Berlin, pp 183–196
Assarar M, Scida D, El Mahi A, Poilâne C, Ayad R (2011) Influence of water ageing on mechanical properties and damage events of two reinforced composite materials: flax–fibres and glass–fibres. Mater Des 32(2):788–795
Lu MM, Van Vuure AW (2019) Improving moisture durability of flax fibre composites by using non-dry fibres. Compos Part A Appl Sci Manuf 123:301–309
Yousif BF, El-Tayeb NSM (2007) The effect of oil palm fibers as reinforcement on tribological performance of polyester composite. Surf Rev Lett 14(06):1095–1102
El-Tayeb NSM (2008) A study on the potential of sugarcane fibers/polyester composite for tribological applications. Wear 265(1–2):223–235
Yousif BF (2009) Frictional and wear performance of polyester composites based on coir fibres. Proc Inst Mech Eng Part J J Eng Tribol 223(1):51–59
Chin CW, Yousif BF (2009) Potential of kenaf fibres as reinforcement for tribological applications. Wear 267(9–10):1550–1557
Abu-Sharkh BF, Hamid H (2004) Degradation study of date palm fibre/polypropylene composites in natural and artificial weathering: mechanical and thermal analysis. Polym Degrad Stab 85(3):967–973
Singh B, Gupta M (2005) Performance of pultruded jute fibre reinforced phenolic composites as building materials for door frame. J Polym Environ 13(2):127–137
Dash BN, Rana AK, Mishra HK, Nayak SK, Tripathy SS (2000) Novel low‐cost jute–polyester composites. III. Weathering and thermal behavior. J Appl Polym Sci 78(9):1671–1679
Joseph S, Oommen Z, Thomas S (2006) Environmental durability of banana-fiber-reinforced phenol formaldehyde composites. J Appl Polym Sci 100(3):2521–2531
Bledzki AK, Gassan J (1999) Composites reinforced with cellulose based fibres. Prog Polym Sci 24(2):221–274
Sarani Z, Lee KP (2002) Polystyrene-benzoylated EFB reinforced composites. Polym Plast Technol Eng 41(5):951–962
Dicker MPM, Duckworth PF, Baker AB, Francois G, Hazzard MK, Weaver PM (2014) Green composites: a review of material attributes and complementary applications. Compos Part A Appl Sci Manuf 56:280–289
Symington MC, Banks WM, West OD, Pethrick RA (2009) Tensile testing of cellulose based natural fibers for structural composite applications. J Compos Mater 43(9):1083–1108
Raghavan J, Meshii M (1997) Creep rupture of polymer composites. Compos Sci Technol 57(4):375–388
Alvarez VA, Kenny JM, Vázquez A (2004) Creep behavior of biocomposites based on sisal fiber reinforced cellulose derivatives/starch blends. Polym Compos 25(3):280–288
Acha BA, Reboredo MM, Marcovich NE (2007) Creep and dynamic mechanical behavior of PP–jute composites: effect of the interfacial adhesion. Compos Part A Appl Sci Manuf 38(6):1507–1516
Xu Y, Wu Q, Lei Y, Yao F (2010) Creep behavior of bagasse fiber reinforced polymer composites. Bioresour Technol 101(9):3280–3286
Amiri A, Hosseini N, Ulven CA (2015) Long-term creep behavior of flax/vinyl ester composites using time-temperature superposition principle. J Renew Mater 3(3):224–233
Yang T-C, Wu T-L, Hung K-C, Chen Y-L, Wu J-H (2015) Mechanical properties and extended creep behavior of bamboo fiber reinforced recycled poly (lactic acid) composites using the time–temperature superposition principle. Constr Build Mater 93:558–563
Militký J, Jabbar A (2015) Comparative evaluation of fiber treatments on the creep behavior of jute/green epoxy composites. Compos Part B Eng 80:361–368
Annicchiarico D, Alcock JR (2014) Review of factors that affect shrinkage of molded part in injection molding. Mater Manuf Process 29(6):662–682
Hakimian E, Sulong AB (2012) Analysis of warpage and shrinkage properties of injection-molded micro gears polymer composites using numerical simulations assisted by the Taguchi method. Mater Des 42:62–71
Nian S-C, Wu C-Y, Huang M-S (2015) Warpage control of thin-walled injection molding using local mold temperatures. Int Commun Heat Mass Transf 61:102–110
Santos JD, Fajardo JI, Cuji AR, García JA, Garzón LE, López LM (2015) Experimental evaluation and simulation of volumetric shrinkage and warpage on polymeric composite reinforced with short natural fibers. Front Mech Eng 10(3):287–293
Tan H-S, Yu Y-Z, Xing L-X, Zhao L-Y, Sun H-q (2013) Density and shrinkage of injection molded impact polypropylene copolymer/coir fiber composites. Polym Plast Technol Eng 52(3):257–260
Kozłowski R, Władyka-Przybylak M (2008) Flammability and fire resistance of composites reinforced by natural fibers. Polym Adv Technol 19(6):446–453
John MJ, Anandjiwala RD (2008) Recent developments in chemical modification and characterization of natural fiber-reinforced composites. Polym Compos 29(2):187–207
Joseph PV (2001) Studies on short sisal fibre reinforced isotactic polypropylene composites. Ph.D. Thesis, Mahatma Gandhi University, India
Satyanarayana KG, Arizaga GGC, Wypych F (2009) Biodegradable composites based on lignocellulosic fibers—an overview. Prog Polym Sci 34(9):982–1021
Wielage B, Lampke T, Marx G, Nestler K, Starke D (1999) Thermogravimetric and differential scanning calorimetric analysis of natural fibres and polypropylene. Thermochim Acta 337(1–2):169–177
Yao F, Wu Q, Lei Y, Guo W, Xu Y (2008) Thermal decomposition kinetics of natural fibers: activation energy with dynamic thermogravimetric analysis. Polym Degrad Stab 93(1):90–98
Grand AF, Wilkie CA (2000) Fire retardancy of polymeric materials. CRC Press, New York
Pujari S, Ramakrishna A, Padal KTB (2017) Investigations on thermal conductivities of jute and banana fiber reinforced epoxy composites. J Inst Eng (India) Ser D 98(1):79–83
Li X, Tabil LG, Oguocha IN, Panigrahi S (2008) Thermal diffusivity, thermal conductivity, and specific heat of flax fiber—HDPE biocomposites at processing temperatures. Compos Sci Technol 68(7–8):1753–1758
Mangal R, Saxena NS, Sreekala MS, Thomas S, Singh K (2003) Thermal properties of pineapple leaf fiber reinforced composites. Mater Sci Eng, A 339(1–2):281–285
Osugi R, Takagi H, Liu K, Gennai Y (2009) Thermal conductivity behavior of natural fiber-reinforced composites. In: Proceedings of the Asian pacific conference for materials and mechanics, Yokohama, Japan, November 13–16 2009, pp 1–3
Mounika M, Ramaniah K, Prasad AVR, Rao KM, Reddy KHC (2012) Thermal conductivity characterization of bamboo fiber reinforced polyester composite. J Mater Environ Sci 3(6):1109–1116
Ramanaiah K, Prasad AVR, Chandra Reddy KH (2013) Mechanical and thermo-physical properties of fish tail palm tree natural fiber—reinforced polyester composites. Int J Polym Anal Charact 18(2):126–136
Agrawal R, Saxena NS, Sreekala MS, Thomas S (2000) Effect of treatment on the thermal conductivity and thermal diffusivity of oil-palm-fiber-reinforced phenolformaldehyde composites. J Polym Sci, Part B: Polym Phys 38(7):916–921
Feng D, Caulfield DF, Sanadi AR (2001) Effect of compatibilizer on the structure-property relationships of kenaf-fiber/polypropylene composites. Polym Compos 22(4):506–517
Mofokeng JP, Luyt AS, Tábi T, Kovács J (2012) Comparison of injection moulded, natural fibre-reinforced composites with PP and PLA as matrices. J Thermoplast Compos Mater 25(8):927–948
Azwa ZN, Yousif BF (2013) Characteristics of kenaf fibre/epoxy composites subjected to thermal degradation. Polym Degrad Stab 98(12):2752–2759
Costa C, Fonseca AC, Serra AC, Coelho JFJ (2016) Dynamic mechanical thermal analysis of polymer composites reinforced with natural fibers. Polym Rev 56(2):362–383
Pothan LA, Oommen Z, Thomas S (2003) Dynamic mechanical analysis of banana fiber reinforced polyester composites. Compos Sci Technol 63(2):283–293
Idicula M, Malhotra SK, Joseph K, Thomas S (2005) Dynamic mechanical analysis of randomly oriented intimately mixed short banana/sisal hybrid fibre reinforced polyester composites. Compos Sci Technol 65(7–8):1077–1087
Pan P, Zhu B, Kai W, Serizawa S, Iji M, Inoue Y (2007) Crystallization behavior and mechanical properties of bio-based green composites based on poly (l-lactide) and kenaf fiber. J Appl Polym Sci 105(3):1511–1520
Mylsamy K, Rajendran I (2011) The mechanical properties, deformation and thermomechanical properties of alkali treated and untreated Agave continuous fibre reinforced epoxy composites. Mater Des 32(5):3076–3084
Hebel DE, Javadian A, Heisel F, Schlesier K, Griebel D, Wielopolski M (2014) Process-controlled optimization of the tensile strength of bamboo fiber composites for structural applications. Compos Part B Eng 67:125–131
Lee JM, Heitmann JA, Pawlak JJ (2007) Local morphological and dimensional changes of enzyme-degraded cellulose materials measured by atomic force microscopy. Cellulose 14(6):643–653
George M, Mussone PG, Abboud Z, Bressler DC (2014) Characterization of chemically and enzymatically treated hemp fibres using atomic force microscopy and spectroscopy. Appl Surf Sci 314:1019–1025
Keshk S, Suwinarti W, Sameshima K (2006) Physicochemical characterization of different treatment sequences on kenaf bast fiber. Carbohydr Polym 65(2):202–206
Bilba K, Arsene M-A, Ouensanga A (2007) Study of banana and coconut fibers: botanical composition, thermal degradation and textural observations. Bioresour Technol 98(1):58–68
Spinacé MAS, Lambert CS, Fermoselli KKG, De Paoli M-A (2009) Characterization of lignocellulosic curaua fibres. Carbohydr Polym 77(1):47–53
Saha P, Manna S, Chowdhury SR, Sen R, Roy D, Adhikari B (2010) Enhancement of tensile strength of lignocellulosic jute fibers by alkali-steam treatment. Bioresour Technol 101(9):3182–3187
Sawpan MA, Pickering KL, Fernyhough A (2011) Effect of various chemical treatments on the fibre structure and tensile properties of industrial hemp fibres. Compos Part A Appl Sci Manuf 42(8):888–895
De Rosa IM, Kenny JM, Maniruzzaman M, Moniruzzaman M, Monti M, Puglia D, Santulli C, Sarasini F (2011) Effect of chemical treatments on the mechanical and thermal behaviour of okra ( Abelmoschus esculentus ) fibres. Compos Sci Technol 71(2):246–254
Fiore V, Valenza A, Di Bella G (2011) Artichoke (Cynaracardunculus L.) fibres as potential reinforcement of composite structures. Compos Sci Technol 71(8):1138–1144
Arrakhiz FZ, El Achaby M, Kakou AC, Vaudreuil S, Benmoussa K, Bouhfid R, Fassi-Fehri O, Qaiss A (2012) Mechanical properties of high density polyethylene reinforced with chemically modified coir fibers: impact of chemical treatments. Mater Des 37:379–383
Reddy KO, Maheswari CU, Shukla M, Song JI, Rajulu AV (2013) Tensile and structural characterization of alkali treated Borassus fruit fine fibers. Compos Part B Eng 44(1):433–438
Neto ARS, Araujo MAM, Souza FVD, Mattoso LHC, Marconcini JM (2013) Characterization and comparative evaluation of thermal, structural, chemical, mechanical and morphological properties of six pineapple leaf fiber varieties for use in composites. Ind Crops Prod 43:529–537
AlMaadeed MA, Kahraman R, Khanam PN, Al-Maadeed S (2013) Characterization of untreated and treated male and female date palm leaves. Mater Des 43:526–531
Lu X, Zhang MQ, Rong MZ, Shi G, Yang GC (2003) Self-reinforced melt processable composites of sisal. Compos Sci Technol 63(2):177–186
Alavudeen A, Rajini N, Karthikeyan S, Thiruchitrambalam M, Venkateshwaren N (2015) Mechanical properties of banana/kenaf fiber-reinforced hybrid polyester composites: effect of woven fabric and random orientation. Mater Des 1980–2015(66):246–257
Faruk Hossen M, Hamdan S, Rahman MR, Islam MS, Liew FK, hui Lai JC, Rahman MM (2016) Effect of clay content on the morphological, thermo-mechanical and chemical resistance properties of propionic anhydride treated jute fiber/polyethylene/nanoclay nanocomposites. Measurement 90:404–411
Saba N, Tahir P, Jawaid M (2014) A review on potentiality of nano filler/natural fiber filled polymer hybrid composites. Polymers 6(8):2247–2273
Denault J, Labrecque B (2004) Technology group on polymer nanocomposites–PNC-Tech. Industrial Materials Institute. National Research Council Canada, vol 75. Québec, Canada
Huang X, Netravali A (2007) Characterization of flax fiber reinforced soy protein resin based green composites modified with nano-clay particles. Compos Sci Technol 67(10):2005–2014
Sen A, Kumar S (2010) Coir-fiber-based fire retardant nano filler for epoxy composites. J Therm Anal Calorim 101(1):265–271
Alamri H, Low IM (2013) Effect of water absorption on the mechanical properties of nanoclay filled recycled cellulose fibre reinforced epoxy hybrid nanocomposites. Compos Part A Appl Sci Manuf 44:23–31
Lim KH, Majid MSA, Ridzuan MJM, Basaruddin KS, Afendi M (2017) Effect of nano-clay fillers on mechanical and morphological properties of Napier/epoxy Composites. In: International conference on applications and design in mechanical engineering (ICADME 2017), Shenzhen, China, 2017. IOP Publishing, pp 1–9
Ashik KP, Sharma RS, Raghavendra N (2017) Effect of filler on mechanical properties of natural fiber reinforced composites. Asian J Chem 29(8):1697–1701
Afroughsabet V, Biolzi L, Ozbakkaloglu T (2016) High-performance fiber-reinforced concrete: a review. J Mater Sci 51(14):6517–6551. https://doi.org/10.1023/A:1018651218515
Zimniewska M, Wladyka-Przybylak M (2016) Natural fibers for composite applications. In: Fibrous and textile materials for composite applications. Springer, Berlin, pp 171–204
Dweib MA, Hu B, Shenton Iii HW, Wool RP (2006) Bio-based composite roof structure: manufacturing and processing issues. Compos Struct 74(4):379–388
Ticoalu A, Aravinthan T, Cardona F (2010) A review of current development in natural fiber composites for structural and infrastructure applications. In: 2010. Engineers Australia, pp 113–117
Khondker OA, Ishiaku US, Nakai A, Hamada H (2005) Fabrication mechanical properties of unidirectional jute/PP composites using jute yarns by film stacking method. J Polym Environ 13(2):115–126
Yousif BF, Ku H (2012) Suitability of using coir fiber/polymeric composite for the design of liquid storage tanks. Mater Des 1980–2015(36):847–853
Bilisik K, Karaduman NS, Bilisik NE (2016) Fiber architectures for composite applications. In: Fibrous and textile materials for composite applications. Springer, Berlin, pp 75–134
MarketsandMarkets (2017) Biocomposites market by fiber (wood fiber and non-wood fiber), polymer (synthetic and natural), product (hybrid and green), end-use industry (transportation, building & construction and consumer goods), and region—global forecast to 2022, US
GrandViewResearch (2018) Biocomposites market size, share & trends analysis report by fiber type (wood, non-wood), by polymer type (natural, synthetic), by product type (green, hybrid), by end use, and segment forecasts, 2018–2025, US
Yang Y, Boom R, Irion B, van Heerden D-J, Kuiper P, de Wit H (2012) Recycling of composite materials. Chem Eng Process Process Intensif 51:53–68
TransparencyMarketResearch (2018) Global biocomposites market to benefit from increased demand in construction and automotive industry, to exhibit 9.46% CAGR by 2025 New York, US
Wötzel K, Wirth R, Flake M (1999) Life cycle studies on hemp fibre reinforced components and ABS for automotive parts. Die Angewandte Makromolekulare Chemie 272(1):121–127
Müssig J, Schmehl M, Von Buttlar HB, Schönfeld U, Arndt K (2006) Exterior components based on renewable resources produced with SMC technology—considering a bus component as example. Ind Crops Prod 24(2):132–145
Zah R, Hischier R, Leão AL, Braun I (2007) Curauá fibers in the automobile industry–a sustainability assessment. J Clean Prod 15(11–12):1032–1040
Schmehl M, Müssig J, Schönfeld U, Von Buttlar HB (2008) Life cycle assessment on a bus body component based on hemp fiber and PTP ® . J Polym Environ 16(1):51–60
Luz SM, Caldeira-Pires A, Ferrão PMC (2010) Environmental benefits of substituting talc by sugarcane bagasse fibers as reinforcement in polypropylene composites: ecodesign and LCA as strategy for automotive components. Resour Conserv Recycl 54(12):1135–1144
Wang J, Shi SQ, Liang K (2013) Comparative life-cycle assessment of sheet molding compound reinforced by natural fiber vs. glass fiber. J Agric Sci Technol B 3:493–502
La Rosa AD, Cozzo G, Latteri A, Recca A, Björklund A, Parrinello E, Cicala G (2013) Life cycle assessment of a novel hybrid glass-hemp/thermoset composite. J Clean Prod 44:69–76
Dissanayake NPJ, Summerscales J, Grove SM, Singh MM (2009) Life cycle impact assessment of flax fibre for the reinforcement of composites. J Biobased Mater Bioenergy 3(3):245–248
Dissanayake NPJ, Summerscales J, Grove SM, Singh MM (2009) Energy use in the production of flax fiber for the reinforcement of composites. J Nat Fibers 6(4):331–346
Download references
Author information
Authors and affiliations.
Department of Infrastructure Engineering, The University of Melbourne, Melbourne, VIC, Australia
Aliakbar Gholampour
Ingram School of Engineering, Texas State University, San Marcos, TX, USA
Togay Ozbakkaloglu
You can also search for this author in PubMed Google Scholar
Corresponding author
Correspondence to Togay Ozbakkaloglu .
Ethics declarations
Conflict of interest.
Both authors declare that they have no conflict of interest.
Additional information
Publisher's note.
Springer Nature remains neutral with regard to jurisdictional claims in published maps and institutional affiliations.
Rights and permissions
Reprints and permissions
About this article
Gholampour, A., Ozbakkaloglu, T. A review of natural fiber composites: properties, modification and processing techniques, characterization, applications. J Mater Sci 55 , 829–892 (2020). https://doi.org/10.1007/s10853-019-03990-y
Download citation
Received : 17 May 2019
Accepted : 04 September 2019
Published : 16 September 2019
Issue Date : January 2020
DOI : https://doi.org/10.1007/s10853-019-03990-y
Share this article
Anyone you share the following link with will be able to read this content:
Sorry, a shareable link is not currently available for this article.
Provided by the Springer Nature SharedIt content-sharing initiative
- Find a journal
- Publish with us
- Track your research
- Reference Manager
- Simple TEXT file
People also looked at
Review article, natural fibers as sustainable and renewable resource for development of eco-friendly composites: a comprehensive review.
- Department of Mechanical and Process Engineering, The Sirindhorn International Thai–German Graduate School of Engineering, King Mongkut's University of Technology North Bangkok, Bangkok, Thailand
The increase in awareness of the damage caused by synthetic materials on the environment has led to the development of eco-friendly materials. The researchers have shown a lot of interest in developing such materials which can replace the synthetic materials. As a result, there is an increase in demand for commercial use of the natural fiber-based composites in recent years for various industrial sectors. Natural fibers are sustainable materials which are easily available in nature and have advantages like low-cost, lightweight, renewability, biodegradability, and high specific properties. The sustainability of the natural fiber-based composite materials has led to upsurge its applications in various manufacturing sectors. In this paper, we have reviewed the different sources of natural fibers, their properties, modification of natural fibers, the effect of treatments on natural fibers, etc. We also summarize the major applications of natural fibers and their effective use as reinforcement for polymer composite materials.
Introduction
Material selection in design and manufacturing of a sustainable product plays a vital role in the field of engineering design. The materials are used to explore their physical properties along with their mechanical properties to make the product better one and reach its customer satisfaction levels. The polymer composite materials are one of such materials which provide the ease of processing, productivity, and cost reduction ( Faruk et al., 2012 ; Al-Oqla and Sapuan, 2014 ; Sanjay and Suchart, 2019 ). The composites are tailor-made materials which have a unique quality where the properties can be altered by varying the different reinforcement and matrix phase ( Bledzki and Gassan, 1999 ; Yogesha, 2017 ). Compared with the synthetic fibers, the natural fibers have many advantages due to their abundance, availability, and low cost ( Arpitha et al., 2017 ; Madhu et al., 2019b ). The natural fibers are introduced instead of synthetic fibers to make the composites lighter. The density of natural fibers (1.2–1.6 g/cm 3 ) is lower than glass fiber (2.4 g/cm 3 ), which leads to the making of the light-weight composites. As a result, there is an increase in the demand for the commercial use of natural fiber-based composites in various industrial sectors. Therefore, natural fibers such as hemp, jute, sisal, banana, coir, and kenaf are extensively used in the production of the lightweight composites ( Sreekala and Thomas, 2003 ; Thakur et al., 2014 ; Oksman et al., 2016 ). The natural fiber-based composites have been used in automotive interior linings (roof, rear wall, side panel lining), furniture, construction, packaging, and shipping pallets, etc. ( Oksman, 2001 ; Lau et al., 2018 ; Sood and Dwivedi, 2018 ; Santhosh Kumar and Hiremath, 2019 ). Natural fibers are extracted from different plants and animals (chicken feather, hair, etc.) ( Aziz and Ansell, 2004 ; Huda et al., 2006 ; Kicinska-Jakubowska et al., 2012 ). The plant fibers are made up of constituents like cellulose, lignin, hemicellulose, pectin, waxes, and water-soluble substances, which is represented in Figure 1 . The presence of cellulose which is hydrophilic in nature affects the interfacial bonding between the polymer matrix and the fibers because the matrix is hydrophobic. Chemical treatment of the natural fibers is one of the ways to optimize the interaction between the fibers and polymer matrix. As it reduces the OH functional groups present on the fiber surface and also it increases the surface roughness and hence enhances the interfacial interaction between the matrix and the fibers ( Liu et al., 2005 ; Mahjoub et al., 2014 ; Manimaran et al., 2017 ; Athith et al., 2018 ; Sanjay et al., 2019a ). The study of natural fibers is very essential to develop eco-friendly composites.
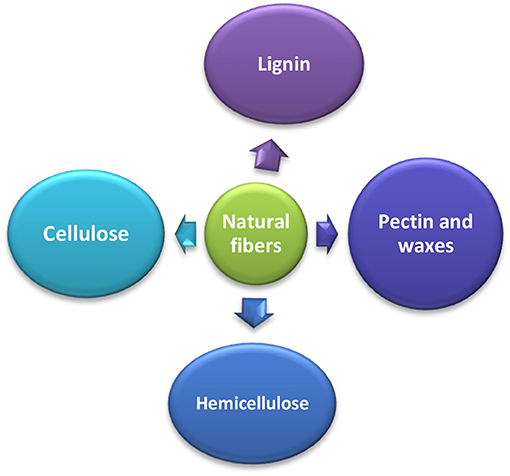
Figure 1 . Constituents of plant fibers ( Faruk et al., 2014 ).
Source, Properties, and Applications of Natural Fibers
Kenaf ( hibiscus cannabinus ).
The kenaf fibers are one of the important fibers belongs to bast fibers and it is mainly used for paper and rope production ( Hamidon et al., 2019 ; Omar et al., 2019 ). Kenaf is a fibrous plant. They are stiff, strong, and tough and have high resistance to insecticides. These plants are cultivated 4,000 years ago in Africa, Asia, America, and some parts of Europe ( Saba et al., 2015 ; Zamri et al., 2016 ; Shahinur and Hasan, 2019b ). The fibers are extracted from flowers, outer fiber, and inner core. The outer fiber is known as bast which makes 40% of the stalks dry weight and the inner core comprises of 60% of stalks dry weight. The kenaf plants upon harvesting are processed by using a mechanical fiber separator and the whole stalk is used in pulping. The extracted fibers must be treated chemically or bacterially to separate it from the non-fibrous substances like wax, pectin, and other substances ( Suharty et al., 2016 ; Arjmandi et al., 2017 ). These fibers can be converted into fine woven fabrics. Kenaf fibers are environmentally friendly as they are completely biodegradable. In the olden days, these fibers were used for textiles, cords, ropes, storage bags, and Egyptians used it for making boats. Nowadays these fibers are made as composites along with other materials and are used in automotive, construction, packaging, furniture, textiles, mats, paper pulp, etc. ( Nishino et al., 2003 ; Anuar and Zuraida, 2011 ; Atiqah et al., 2014 ; Kipriotis et al., 2015 ).
Hemp ( Cannabis sativa )
The Hemp is one of the kinds of plants species grown mainly in Europe and Asia. It grows up to 1.2–4.5 m and 2 cm in diameter ( Bhoopathi et al., 2014 ; Réquilé et al., 2018 ). The inner girth is surrounded by core, and the outer layer is the bast fiber and it is attached to the inner layer by glue-like substance or pectin. These fibers are used in rope, textiles, garden mulch, the assortment of building material and animal beddings. In recent developments, it is used to fabricate different composites ( Li et al., 2006 ; Martin et al., 2013 ; Väisänen et al., 2018 ). The hemp plants are harvested, and the woody core from bast fibers is separated by a sequence of mechanical process. The woody core is cleaned to obtain the required core content and sometimes they are cut to the desired size. While the separated bast fibers are further processed to form yarn or bundles ( Clarke, 2010 ; Duval et al., 2011 ; Fang et al., 2013 ; Raman Bharath et al., 2015 ; Sam-Brew and Smith, 2015 ).
Jute ( Corchorus capsularis )
The jute an important natural fiber grown in parts of Asia including India, Bangladesh, China, and Myanmar ( Khan and Khan, 2014 ; Das, 2017 ; Shahinur and Hasan, 2019a ). The jute plant grows up to 15–20 cm in 4 months, and the fibers are extracted after harvesting which is about 4 months from cultivation. The retting process is done either with the help of chemicals (N 2 H 8 C 2 O 4 , Na 2 SO 3 , etc.) or biologically ( Rahman, 2010 ). In biological retting, the stalks which are harvested are arranged in bundles and allowed to soak in water for about 20 days ( Banik et al., 2003 ; Behera et al., 2012 ). This removes the pectin between the bast and the wood core which helps in the separation of the fibers. Then these fibers are allowed to dry.
Flax ( Linum usitatissimum )
The flax fibers are produced from the prehistoric period. These fibers are separated from the stems of the plant Linum usitatissimum is mainly used to produce linen ( Ruan et al., 2015 ; De Prez et al., 2018 ; Bourmaud et al., 2019 ). These are cellulosic plants but they are more in crystalline form. These fibers measure up to 90 cm length and diameter of 12–16 μm. Netherlands, Belgium, and France are the leading manufacturers of these fibers. These fibers are used in furniture materials, textiles bed sheets, linen, interior decoration accessories, etc. ( Van de Weyenberg et al., 2003 ; Charlet et al., 2010 ; Angelini and Tavarini, 2013 ; Ramesh, 2019 ). The fiber extraction involves the retting, and scorching both this process will make some alterations in the properties of the fibers. The retting involves the enzymes which degrade the pectin around the flax fibers which results in separation of fibers. Canada is the largest flax producer and exporter in the world, produced about 872,000 tons ( Bos et al., 2006 ; Zafeiropoulos and Baillie, 2007 ; Martin et al., 2013 ; Zhu et al., 2013 ).
Ramie ( Boehmeria nivea )
Ramie is one of the herbaceous perennial plants cultivated extensively in the region native to China, Japan, and Malaysia where it has been used for over a century as one of the textile fabrics ( Nam and Netravali, 2006 ; Rehman et al., 2019 ; Yang et al., 2019 ). Ramie is a non-branching, fast-growing plant which grows up to 1–2 m height. The fibers extracted from the stem are the strongest and longest of the natural bast fibers. They are used to make sweaters in combination with cotton, also it is used in upholstery, gas mantle, fishing nets, and marine packings, etc. ( Cengiz and Babalik, 2009 ; Marsyahyo et al., 2009 ; Sen and Jagannatha Reddy, 2011b ). In addition to this attempt has been made for developing bio-based products by utilizing them in the field of automotive, furniture, construction, etc. The ramie fibers are extensively used for the production of a wide range of textiles, pulp, and paper, agrochemicals, composites, etc. The processing of the ramie fibers is similar to linen from flax ( Angelini and Tavarini, 2013 ; Bunsell, 2018 ).
Nettle ( Urtica dioica )
Nettle is the commonly grown herbaceous plant consists of 35–40 different species generally grown in Europe, Asia, Northern Africa, and North America ( Bacci et al., 2009 ; Akgül, 2013 ; Lanzilao et al., 2016 ). The plant usually grows up to 2 m in length, the leaves are soft and green which are 3–15 cm long. The leaves and stems are generally hairy and have stinging hairs on them ( Cummings and Olsen, 2011 ; Fang et al., 2013 ; Bourgeois et al., 2016 ). The fiber extraction is done by harvesting the plants during the flowering period. The fiber is extracted either by retting the stalks or by decorticating. The typical applications of nettle fibers are in the textile industry, bioenergy, animal housing, etc. Nowadays attempts have been made to use the nettle fibers on an industrial scale ( Bacci et al., 2009 ; Mortazavi and Moghaddam, 2010 ).
Pineapple Leaf ( Ananas comosus )
The pineapple plant is one of the abundantly cultivated plants which is easily available. The pineapple leaf fiber is crop waste after pineapple cultivation. It is a short tropical plant grows up to 1–2 m and the leaves are in cluster form consists of 20–30 leaves of about 6 cm wide. Approximately, 90–100 tons of pineapple leaves are grown per hectare. Among the different natural fibers, pineapple leaf fibers show good mechanical properties. Pineapple leaf fibers are multicellular and lingo-cellulosic. The fibers were extracted by hand using the scrapers ( Kengkhetkit and Amornsakchai, 2012 ; Laftah and Abdul Rahaman, 2015 ; Todkar and Patil, 2019 ). The various applications are in automobiles, textile, mats, construction, etc. The treated and surface-modified fibers are used for making conveyor belt cord, air-bag, advanced composites, etc. ( Paridah et al., 2004 ; Jawaid and Abdul Khalil, 2011 ; Reddy and Yang, 2015 ; Al-Maharma and Al-Huniti, 2019 ).
Sisal ( Agave sisalana )
The sisal is one of the most used natural fibers and Brazil is one of the largest producers of this fiber. It is a species native to south Mexico consists of the rosette of leaves grows up to 1.5–2 m tall ( Naveen et al., 2018 ; Sanjay et al., 2018 ; Senthilkumar et al., 2018 ; Devaraju and Harikumar, 2019 ). The sisal produces about 200–250 commercially usable leaves in the life span of 6–7 years. The sisal fibers are having good range of mechanical properties and are used in the automotive industry, shipping industry (for mooring small craft and handling cargo), civil constructions, used as fiber core of the steel wire cables of elevators, agricultural twine or baler twine, etc. ( Mihai, 2013 ; Ramesh et al., 2013 ; Nirmal et al., 2015 ; Aslan et al., 2018 ).
Date Palm ( Phoenix dactylifera )
The date palm is known as palm extensively grown for its fruit. The biodiversity of the date palm is all over the world comprising around 19 species with more than 5,000 cultivators all around the world ( Wales and Blackman, 2017 ; Alotaibi et al., 2019 ; Rivera et al., 2019 ). The date palm trees ( Phoenix dactylifera L.) are the tallest among the Phoenix species and can grow up to 23 m height ( Al-Oqla and Sapuan, 2014 ; Gheith et al., 2018 ; Masri et al., 2018 ). The date palm rachis and leaves are accumulated in large quantity after the harvesting of the date farm fruits every year in the farming lands of different countries. These fibers can be used as the potential cellulosic fiber sources. These fibers from leaves and rachis can be used as the reinforcement for thermoplastic and thermosetting polymers. Some researchers have found ways to use the date palm fibers in the automotive application ( Alawar et al., 2009 ; Arunachalam, 2012 ; Liu et al., 2018 ).
Cotton (Gossypium)
Cotton belongs to the sub-tribe Hibisceae and family of Malvaceae is an important agricultural crop ( Elmogahzy and Farag, 2018 ). It is the commonly used natural fiber for the production of cloths. The cotton is grown in tropical and subtropical regions, and China is the largest producer of cotton followed by India and the United States ( Mwaikambo et al., 2000 ; Colomban and Jauzein, 2018 ). Among the various species of cotton, upland cotton ( Gossypium hirsutum ) and pima cotton ( Gossypium barbadense ) are the most popular ( Zou et al., 2011 ; Al-Oqla et al., 2015 ; Sharma et al., 2017 ). The leaves of the cotton are removed and are collected and compressed into truckload-sized “modules.” Later the modules are transported to processing plant known as the cotton gin. The gin separates the seeds, sticks, burrs, etc. from the cotton fibers. The cotton fiber is used extensively in textile industries, and recently attempts have been made to develop the composites for industrial applications ( Cheung et al., 2009 ; Gupta and Srivastava, 2016 ; Balaji and Senthil Vadivu, 2017 ).
Coconut Fiber ( Cocos nucifera )
The coconut fiber is obtained from the husk of the coconut fruit. Among the different natural fibers, coconut fiber is the thickest. Coconut trees are mainly grown in tropical regions ( Nair, 2010 ; Arulandoo et al., 2016 ; Danso, 2017 ). The major share of the commercially produced coconut fiber comes from India, Sri Lanka, Indonesia, Philippines, and Malaysia ( Pham, 2016 ). Coir fiber, in particular, is a light and strong fiber that has been attracted scientific and commercial importance due to their specific characteristics and availability ( Sen and Jagannatha Reddy, 2011a ). Compared to other typical natural fibers, coconut fiber has higher lignin and lower cellulose and hemicellulose, together with its high microfibrillar angle, offers various valuable properties, such as resilience, strength, and damping, wear, resistance to weathering, and high elongation at break. The coir fiber is used for making ropes, mats, mattresses, brushes, in the upholstery industry, agriculture, construction, etc. ( Al-Oqla and Sapuan, 2014 ; Verma and Gope, 2014 ; Sengupta and Basu, 2016 ; dos Santos et al., 2018 ).
Kapok ( Ceiba pentandra )
Kapok belongs to the Bombacaceae family. It grows in tropical regions ( Arumugam, 2014 ; Zheng et al., 2015 ). Kapok fiber is silk cotton and the color of the fiber is yellowish or light brown. The fibers enclose the kapok seeds. Kapok fibers are cellulosic fibers, light-weight, and hydrophobic ( Prachayawarakorn et al., 2013 ; Wang et al., 2019 ). Conventionally, kapok fiber is used as buoyancy material, oil-absorbing material, reinforcement material, adsorption material, biofuel, etc. ( Tye et al., 2012 ; Dong et al., 2015 ; Zheng et al., 2015 ).
Bamboo (Bambusoideae)
Bamboo fiber is also known as natural glass fiber due to the alignment of fibers in the longitudinal directions ( Zakikhani et al., 2014 ; Wang and Chen, 2016 ). It is one of the extensively available trees in the dense forests especially in China, about 40 families, and 400 species are found ( Fan and Weclawski, 2016 ; Van Dam et al., 2018 ). Bamboo fiber is used as reinforcement in polymeric materials due to its light-weight, low cost, high strength, and stiffness. Bamboo has been traditionally used for making houses, bridges, traditional boats, etc. The fibers extracted from bamboo are used as reinforcement for making advanced composites in various industries ( Deshpande et al., 2000 ; Osorio et al., 2011 ; Zakikhani et al., 2014 ).
Silk ( Bombyx mori )
Silk fibers are extracted from silkworms for the clothing purpose since ancient times. Silk is produced largely in China, South Asia, and Europe ( Das and Natarajan, 2019 ; Shera et al., 2019 ). Fibers are extracted from the Cocoons which are the larvae of the insects undergoing complete metamorphosis. Silk fibers possess good mechanical properties such as high strength, extensibility, and compressibility ( Yuan et al., 2010 ; Murugesh Babu, 2016 ; Castrillón Martínez et al., 2017 ; McGregor, 2018 ).
Possibilities to Enhance the Properties of Natural Fibers
The disadvantage of natural fiber composites includes poor fiber-matrix interfacial bonding, poor wettability, water absorption, and moisture absorption. The hydrophilic nature of the natural fibers caused poor interfacial interaction between the polymer matrix and the fiber. Hence, it is required to optimize the fibers by chemical treatments and surface treatments ( Gassan and Bledzki, 1999 ; George et al., 2001 ; Li et al., 2007 ; Manimaran et al., 2018 ; Rangappa and Siengchin, 2018 ; Sanjay et al., 2018 ; Yashas Gowda et al., 2018 ).
Chemical Treatments
The recent trends in the development of the newer materials have led in replacing materials like glass and carbon reinforced composites with the natural fibers reinforced composites, for example in automobile interior, pedestrian bridge, shipping pallets, composite roof tiles, furniture, toys, etc. ( Senthamaraikannan et al., 2016 ; Senthamaraikannan and Kathiresan, 2018 ; Madhu et al., 2019a ; Sanjay et al., 2019b ). However, the main drawback of natural fibers as reinforcement is that they are incompatible with thermoplastics due to their hydrophilic nature which results in the poor interfacial interaction between the fibers and matrix. This result in the poor mechanical properties of the composites. Therefore, the modification of natural fibers is required to make them less hydrophilic. Here an attempt is made to brief about various chemical treatments on natural fibers ( Sepe et al., 2018 ).
Alkaline Treatment
The natural fiber consists of lignin, pectin, waxy materials, and natural oils which covers the outside layer of the fiber cell wall ( Liu et al., 2004 ; Edeerozey et al., 2007 ; Hamidon et al., 2019 ). The chemical treatment alters the structure of the natural fibers, and sodium hydroxide (NaOH) is one of the chemical reagent used for this process ( Rong et al., 2001 ; Baiardo et al., 2002 ; Sgriccia et al., 2008 ). The alkaline reagent is used to alter the structure of the cellulose in the plant fibers by cleaning the surface and the process called alkalization. Mwaikambo and Ansell treated hemp, jute, sisal, and kapok fibers with the NaOH at 20°C for about 48 h and washed using distilled water and acetic acid to neutralize the excess of NaOH. The thermal properties, surface morphology, and crystallinity index of the treated and untreated fibers were studied. The studies revealed that the chemically treated fibers showed the better fiber-resin adhesion lead to an increase in interfacial energy and thus enhancing the thermal and mechanical properties of the composites ( Mwaikambo and Ansell, 2002 ). Kenaf fiber mats were treated with the NaOH solution for 24 h at a temperature of 45°C. The mats were washed with tap water after the chemical treatment and were immersed in the distilled water containing 1% acetic acid to neutralize the excess of NaOH and the mats were dried for 12 h at 45°C in an oven. The mats were then treated with 5% aminopropyl triethoxysilane diluted with an aqueous solution of methanol. The authors observed a significant increase in mechanical properties for the treated kenaf fiber modified PP composites ( Asumani et al., 2012 ). In an interesting work, the retting process was used to extract the fibers from Napier grass and the aqueous sodium hydroxide solution, about 2–5% is used to treat the Napier grass fibers at room temperature for about 30 min to remove the hemicelluloses and to clean the fibers. The fibers then washed with distilled water repeatedly and dried at 100°C. The alkalization has reduced the amount of hemicellulose in fiber, thus resulting in better mechanical property than that of untreated fiber ( Reddy et al., 2012 ). The Carica papaya fibers were treated with the 5% concentration of NaOH by varying the soaking time from 15 to 90 min at the room temperature. The excess of NaOH from the surface was washed repeatedly using distilled water and was dried for about 56 h. The fibers treated at 60 min with 5% alkaline solution showed the optimum results which showed that complete elimination of hemicelluloses and lignin ( Saravanakumaar et al., 2018 ).
Silane Treatment
The sugar palm fibers are treated with 2% saline and 6% NaOH for 3 h. The authors observed an improved interfacial interaction between the fiber and thermoplastic polyurethane after the treatment ( Atiqah et al., 2018 ). Kabir et al. reviewed the treatment of silane on the surface of natural fibers. They stated that the silane groups act as a coupling agent between the fiber and the matrix and hence improvement's in mechanical properties are observed ( Kabir et al., 2012 ). In an interesting work, Bodur et al. studied the changes in tensile strength and Young's modulus of composites treated with silane for different soaking times. The results were compared with untreated fiber composites. The authors observed significant improvement in strength when compared with untreated fibers. The improvement in strength is due to the formation of silanol (Si-OH) groups that form strong bonds with the –OH groups of the fibers. The remaining Si-OH undergo condensation with adjacent Si-OH groups. The hydrophobic polymerized silane thus formed can attach to the polymer matrix via van der Waals forces. As a result, silane groups form an interface between the fiber and polymer and provides a good interfacial interaction. The high tensile strength of the low-density polyethylene composites is due to good interfacial interaction between the fiber and polymer matrix ( Bodur et al., 2016 ).
Acetylation Treatment
Acetylation of the natural fibers is the process of introducing an acetyl group on the surface of the fibers. This process was used to reduce the hydrophilic nature of fibers providing stability to the composites. The acetylation increases the fiber-matrix adhesion properties, hence the strong bond provides good properties to the natural fiber-based composites ( Hill et al., 1998 ; Rong et al., 2001 ; Sreekala and Thomas, 2003 ). The OH groups of the fibers react with the acetyl groups thus making the fibers more hydrophobic. Generally, lignin and hemicellulose which contain the hydroxyl group react with acetyl groups to become hydrophobic. Normally, before treatment with glacial acetic acid, the natural fiber is alkali-treated. The alkali-treated fibers were soaked in glacial acetic acid for 1 h and later soaked for 2–5 min in acetic anhydride containing two drops of concentrated H 2 SO 4 . The fibers were then washed and dried at 80°C using an oven for 6 h ( Paul et al., 1997 ; Manikandan Nair et al., 2001 ; Mishra et al., 2003 ).
Peroxide Treatment
The impact of peroxide treatment on the mechanical properties of the cellulose fibers reinforced polymer composites has been studied by various researchers. The peroxides decomposed to form free radicals. The generated free radicals react with the hydrogen group of the cellulose fibers and polymer matrix. The peroxide treatment of natural fibers is carried out after alkalization. The alkaline treated fibers were immersed in ca. 6% concentration of benzoyl peroxide or dicumyl peroxide in acetone for about 30 min ( Sreekala et al., 2000 , 2002 ; Li et al., 2007 ).
Benzoylation Treatment
Benzoylation is used to decrease the hydrophilic nature of the fibers ( Ali et al., 2016 ). The fiber-matrix bonding is improved by this treatment which increases the strength of the composites. For benzoylation, the fibers are first treated with NaOH followed by benzoyl chloride (C 6 H 5 COCl) treatment for 15 min. Later the fibers were isolated and treated with ethanol for 1 min and finally washed with distilled water and dried in an oven at 80°C for 24 h ( Manikandan Nair et al., 2001 ; Zhang et al., 2005 ). The thermal stability of the treated fibers was higher than that of the untreated fibers.
Potassium Permanganate (KMnO 4 ) Treatment
The potassium permanganate is used as the chemical reagent to modify the interfacial interaction between the fiber and matrix. Different treatment methodologies are introduced. In one of the studies, the alkaline treated fibers were treated with potassium permanganate for different concentration (0.005–0.205 %) for 1 min and dried using the oven ( Khan et al., 2006 ). Zaman et al. treated the jute fabrics with KMnO 4 along with acetone for different concentration (0.02, 0.03, 0.05, and 0.5%) and soaking times (1, 2, 3, and 5 min) and was dried in the oven ( Zaman et al., 2010 ).
Stearic Acid Treatment
The non-woven jute fibers were immersed in different concentration of stearic acid in anhydrous ethanol from 1 min to up to 4 h and dried at 100°C for 1 h ( Dolez et al., 2017 ). The 1% stearic acid mixed in ethyl alcohol and poured to a steel vessel containing alkali-treated short Sansevieria fibers along with stirring. Then the fibers were dried in woven at 80°C for 45 min ( Sreenivasan et al., 2012 ). Table 1 summarizes the different chemical treatments used for natural fibers.
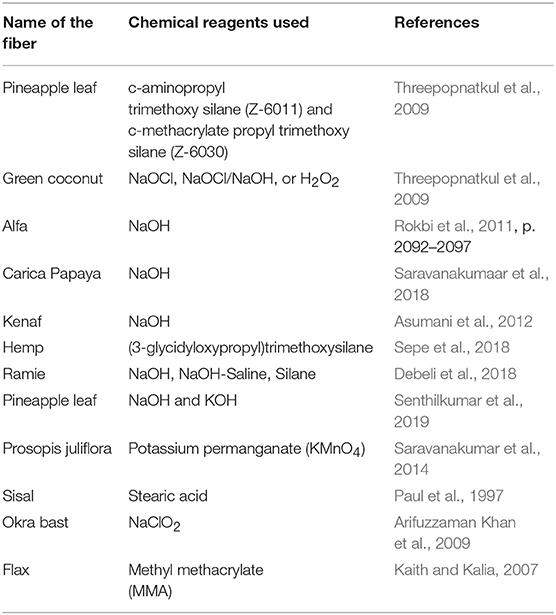
Table 1 . Chemical treatments for different natural fibers.
Effect of Treatments on Natural Fibers
The chemical treatments of the natural fibers mainly enhance the properties of the fiber by modifying their microstructure along with improvement in wettability, surface morphology, chemical groups and tensile strength of the fibers ( Saba et al., 2014 ; Dolez et al., 2017 ; Preet Singh et al., 2017 ; Halip et al., 2018 ; Yu et al., 2019 ). The chemical treatment of the fiber improved the interfacial adhesion between the fiber surface and polymer matrix thereby the thermomechanical properties of the composites. The chemical treatment on ramie fibers has shown that the treatment of fibers with alkaline or saline or the combined treatment results in the improvement of the tensile strength ( Gassan and Bledzki, 1997 ; Thakur and Thakur, 2014 ; Varghese and Mittal, 2017 ; Debeli et al., 2018 ; Sanjay et al., 2019a ). The chemical treatment is one of the important techniques used to reduce the hydrophilic nature of the natural fibers also it improves the adhesion with the matrix. The structural and morphological changes can be observed with the treatment of the fibers, and this is mainly due to the removal of non-cellulosic substances from the natural fibers. The significant improvements of the properties of the composites are reported after different chemical treatments along with the increase in the thermal stability of the composites reinforced with natural fibers ( Singh et al., 1996 ; Xie et al., 2010 ; Xu et al., 2013 ; Chen et al., 2018 ).
Natural Fibers as Reinforcement for Composites Materials
Over the past few decades, attempts have been made in developing the materials which replace the existing materials to have better mechanical and tribological properties for various applications ( Arpitha and Yogesha, 2017 ; Abdellaoui et al., 2019 ). In view of this the monolithic materials are replaced by the fibers and materials such as carbon, glass, aramid fibers which are extensively used in aerospace, automotive, construction, and sporting industries, etc. ( Balakrishnan et al., 2016 ; Pickering et al., 2016 ; Asim et al., 2018 ). However, these materials have some disadvantages like non-biodegradability, non-renewability, high-energy requirement for production, and also harmful to the environment as the production of these materials releases enormous amounts of carbon dioxide into the atmosphere. Therefore, to overcome all these drawbacks researchers has made an attempt to study on the different natural fiber-reinforced composites which have better properties so that they can replace synthetic fibers in various applications ( Wambua et al., 2003 ; Li et al., 2007 ; Sanjay et al., 2015 ; Mochane et al., 2019 ). As the demand for the newer materials which have better properties than the existing ones upsurges, the researchers have tried different types of natural materials with different natural fibers obtained from fruits, seeds, leaves, stem, animals, etc. ( Sanjay et al., 2019a ). The properties of a few important natural fibers are presented in Table 2 . As discussed above, the natural fibers are modified by using different chemical treatments thus modifying the properties and increasing the properties of natural fiber composites. Also, the polymers and other synthetic materials have been used along with the natural fibers to enhance the properties of the natural fibers and these ideas have led to the development of several hybrid composites reinforced with natural fibers, and filler materials ( Sawpan et al., 2011 ; Boopalan et al., 2013 ; Pickering et al., 2016 ; Sanjay et al., 2016 ; Madhu et al., 2018 ).
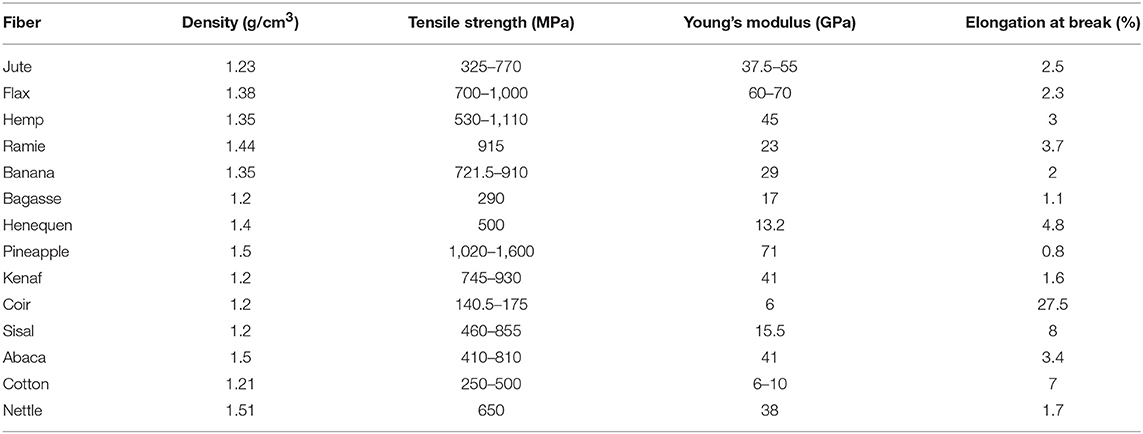
Table 2 . Properties of natural fibers ( Pandey et al., 2010 ; Ku et al., 2011 ; Komuraiah et al., 2014 ; Gurunathan et al., 2015 ).
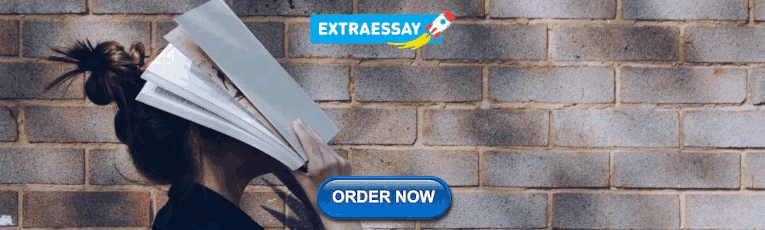
Properties of Natural Fiber Composites
Environmental awareness has attracted researchers to make new composites with more than one reinforcement of natural resources by hybridization. Hybridization involves a combination of fillers and natural fiber that results in increased mechanical properties of the composites ( Khan et al., 2005 ; Borba et al., 2013 ). Many numbers of literature are available which shows the mechanical properties of the natural fiber composites. The mechanical performance of fiber-reinforced composites can be affected by many factors including the volume or weight fraction of the reinforcement, the orientation of the fibers, the fiber aspect ratio, fiber-matrix adhesion, fiber alignment, distribution, use of additives, and chemical treatment of fibers. It is important to add that the moisture absorption of the composites also affects the mechanical behavior of the composites which leads to the poor interfacial bonding between fiber and hydrophobic matrix polymer ( Zakikhani et al., 2014 ; Biswas et al., 2015 ; Kinloch et al., 2015 ; Pickering et al., 2016 ; Dixit et al., 2017 ).
In the automotive industry, asbestos-based brake pads and lining couplings, etc., are not preferred due to its carcinogenic nature. The replacements to asbestos fiber include ceramic fiber, steel fiber, alumina fiber, glass fiber, carbon fiber, aramid fiber, and their combinations. However, the production cost of these fibers is very high and are not environmentally friendly. Xin et al. studied the friction and wear properties of treated sisal fiber reinforced composites as a substitute for asbestos-based brake pads. The treated sisal fiber reinforced composite exhibits properties equivalent to the commercial friction composite. The authors recommend treated sisal is an ideal substitute of asbestos for brake pads ( Xin et al., 2007 ).
The thermal stability is vital and at present is recognized to be one of the most important elements in the use of fibers as reinforcement for the composite. The chemical treatment of the natural fibers will improve the interfacial bonding between the matrix and fibers leads to improvement in thermal property of the composites ( Panaitescu et al., 2016 ; Balan et al., 2017 ; Zegaoui et al., 2018 ).
Joseph et al. studied the thermal stability and crystallization behavior of sisal/polypropylene composites. The sisal fibers were treated with a urethane derivative of polypropylene glycol (PPG/TDI), maleic anhydride-modified polypropylene (MAPP) and KMnO. The thermal properties of the composites were measured using thermogravimetric analysis and differential scanning calorimetry. The authors observed superior thermal properties for the treated fiber reinforced composites ( Joseph et al., 2003 ). The crystallinity also influences the thermal stability of the natural fiber composites. As the crystallinity of the material increased the thermal degradation temperature also increased ( Nasser et al., 2016 ). The thermogravimetry analysis of date palm trunk (DPTRF), leaf stalk (DPLST), sheath or leaf sheath (DPLSH), and fruit bunch stalk (DPFBS) fibers was carried out and analysis revealed that DPFBS and DPLST fibers have good thermal stability and might be applied in industrial manufacture of composites, which require high thermal resistance ( Alotaibi et al., 2019 ).
The pineapple reinforced polyethylene composites were studied for the electrical properties and found that due to the increased interfacial polarization and orientation with an increase in the number of fibers in composites the dielectric property increases ( Jayamol et al., 1997 ). Similarly, the composites prepared with using the sisal fiber showed electric anisotropic behavior ( Chand and Jain, 2005 ). It is observed that the chemical treatments like alkali, stearic acid, peroxide, acetylation, and permanganate decrease the dielectric property of composites due to the decrease in hydrophilicity of the composite ( Li et al., 2000 ). The electrical properties of phenol formaldehyde composites modified with banana fiber have been studied. The dielectric constant decreased with fiber loading and fiber treatment. For hybrid composites with glass fiber, the dielectric constant decreased with increasing glass fiber concentration ( Joseph and Thomas, 2008 ).
Applications
Automotive and aircraft industries have been actively manufacturing different kinds of natural fibers parts for their interior components ( Sanjay et al., 2016 ; Puttegowda et al., 2018 ). Insulation materials are also made from natural fibers for different application areas, such as blowing insulation, pouring insulation, impact sound insulation materials and ceiling panels for thermal insulation, and acoustic soundproofing ( Akin, 2010 ). Natural fibers show a sustainable future in architecture, with a vast variety of building materials, shapes, and even improving current commonly used materials. The use of synthetic fibers in the field of architecture could be substituted with natural fibers. It is used as material for sunscreens, cladding, walling, and flooring ( Steffens et al., 2017 ). The natural fibers such as flax, hemp, sisal, and wool are now used in Mercedes-Benz components ( Holbery and Houston, 2006 ). The coir/polyester-reinforced composites were used in the mirror casing, paperweights, voltage stabilizer cover, projector cover, helmet, and roof ( Khondker et al., 2005 ). The flax fibers were used in GreenBente24 boat ( Ticoalu et al., 2010 ). Rice husk fiber, cotton, ramie, jute fiber, kenaf are used in various applications like building materials, furniture industry, clothing, ropes, sewing thread, fishing nets, packing materials, and paper manufacture ( Sen and Jagannatha Reddy, 2011b ). Lots of efforts have been made to increase the use of natural fiber composites in the automotive industry, particularly in car interiors. Besides the use for car interior parts, it also used for manufacturing exterior auto body components ( Shuit et al., 2009 ; Monteiro et al., 2010 ; Shinoj et al., 2011 ; Mohammed et al., 2015 ).
Degradation of the Natural Fibers Reinforced Polymers
In the present scenario, there is an increase in awareness regarding the environmental pollutions due to industrial waste which has led to replacing the harmful synthetic materials with more eco-friendly materials. The use of plastics is increased especially for household and commercial use. The use of plastic products leads to the accumulation of non-biodegradable wastes and are a threat to the ecological system. Therefore, extensive research has been carried out over the last decade on the biodegradation of plastics. Natural fibers along with the synthetic biodegradable materials can be used to develop biocomposites which have benefits toward the environment like biodegradability, renewability of base material, and reduction in emission of greenhouse gasses. Degradation offers a lot of advantages such as the reduction of plastic waste and reduction in the cost of waste management ( Fakhrul and Islam, 2013 ; Gunti et al., 2018 ).
Degradation of the composite occurs with the breakdown of the composite materials, as well as with the loss of mechanical properties. In the outdoor environment, the degradation of natural fiber reinforced composites is influenced by atmospheric moisture, temperature, ultraviolet light and activities of microscopic organisms. The degradation occurs by the breakdown of hemicelluloses, lignin, and cellulose of the fiber. This can cause damage to the bonding between fibers and polymer matrix. Thus, leads to the lowering of the mechanical properties of the composites ( de Melo et al., 2017 ). The kenaf/POM composites were subjected to weathering by exposing to moisture, water spray, and UV light in an accelerated weathering chamber and the materials showed lower tensile strength and this result was attributed to the degradation of the cellulose, hemicelluloses, and lignin of kenaf fibers ( Abdullah et al., 2013 ). The effect of weathering on the degradation of jute/phenolic composites was investigated by Azwa et al. (2013) . It shows that 2 years of UV exposure on jute/phenolic composites has decreased the tensile strength by about 50%. The authors observed resin cracking, bulging, fibrillation, and black spots after exposure to weathering.
It is necessary to promote the use of natural fibers as reinforcement in the polymer so that the materials become biodegradable to some extent. Proper degradation of the plastics must be a better way to avoid the harmful effects on the environment. Therefore, one must always look for the plastics which are compostable or degradable. However, this cannot be implemented for every material but can be reduced with the use of biopolymers to some extent ( Chauhan and Chauhan, 2015 ; Thiagamani et al., 2019 ).
Future Market Trends
In current market trends, natural fibers reinforced polymers are experiencing comprehensive growth with good prospects in automotive and construction industries. Bast fiber such as hemp, kenaf, flax, etc., are preferred for automotive applications. On the other hand, wood plastic composite is the material of choice for construction industries. Looking at the developments of the current trends Europe is predicted to remain as the largest market for natural fiber-reinforced composites due to the high acceptance level of environmentally friendly composite materials by automotive industries, government agencies, and growth in small scale environmentally friendly industries. The improvement in materials performance will drive the growth of natural fiber reinforced polymer composites in new potential areas. Natural fiber composites are new in electrical, electronics and sporting segments, however, it has the potential to capture a good market share in the future.
Conclusions
Increased environmental awareness has resulted in the utilization of natural fiber as an effective reinforcement material in polymer matrix composites. Natural fibers are proficient materials which can replace the existing synthetic fibers. The fibers are usually extracted from plants and animals often offer poor resistance to moisture and incompatible nature of fibers become the main disadvantage. Therefore, modification of material properties has done through chemical treatments of natural fibers which improve the adhesion between the fibers and matrix and enhance the mechanical properties of the composites. In the near future, the natural fiber will become one of the sustainable and renewable resources in the composite field which can replace synthetic fibers in many applications.
Author Contributions
All authors listed have made a substantial, direct and intellectual contribution to the work, and approved it for publication.
This research was partly supported by the King Mongkut's University of Technology North Bangkok with Grant No. KMUTNB-63-KNOW-001.
Conflict of Interest Statement
The authors declare that the research was conducted in the absence of any commercial or financial relationships that could be construed as a potential conflict of interest.
Abdellaoui, H., Raji, M., Essabir, H., Bouhfid, R., and el kacem Qaiss, A. (2019). “Mechanical behavior of carbon/natural fiber-based hybrid composites,” in Mechanical and Physical Testing of Biocomposites, Fibre-Reinforced Composites and Hybrid Composites , eds M. Jawaid, M. Thariq, and N. Saba (Woodhead Publishing), 103–122. doi: 10.1016/B978-0-08-102292-4.00006-0
CrossRef Full Text | Google Scholar
Abdullah, M. Z., Dan-mallam, Y., Sri, P., and Megat, M. (2013). Effect of environmental degradation on mechanical properties of kenaf/polyethylene terephthalate fiber reinforced polyoxymethylene hybrid composite. Adv. Mater. Sci. Eng. 2013:671481. doi: 10.1155/2013/671481
Akgül, M. (2013). Suitability of stinging nettle ( Urtica dioica L.) stalks for medium density fiberboards production. Compos. Part B Eng. 45, 925–929. doi: 10.1016/j.compositesb.2012.09.048
Akin, D. E. (2010). “Chemistry of plant fibres,” in Industrial Applications of Natural Fibres: Structure, Properties and Technical Applications , ed J. Müssing (West Sussex: John Wiley & Sons Ltd.), 13–22.
Google Scholar
Alawar, A., Hamed, A. M., and Al-Kaabi, K. (2009). Characterization of treated date palm tree fiber as composite reinforcement. Compos. Part B Eng. 40, 601–606. doi: 10.1016/j.compositesb.2009.04.018
Ali, A., Shaker, K., Nawab, Y., Jabbar, M., Hussain, T., Militky, J., et al. (2016). Hydrophobic treatment of natural fibers and their composites — a review. J. Ind. Text. 47, 1–31. doi: 10.1177/1528083716654468
Al-Maharma, A., and Al-Huniti, N. (2019). Critical review of the parameters affecting the effectiveness of moisture absorption treatments used for natural composites. J. Compos. Sci. 3:27. doi: 10.3390/jcs3010027
Al-Oqla, F. M., and Sapuan, S. M. (2014). Natural fiber reinforced polymer composites in industrial applications: feasibility of date palm fibers for sustainable automotive industry. J. Clean. Prod. 66, 347–354. doi: 10.1016/j.jclepro.2013.10.050
Al-Oqla, F. M., Sapuan, S. M., Anwer, T., Jawaid, M., and Hoque, M. E. (2015). Natural fiber reinforced conductive polymer composites as functional materials: a review. Synth. Met. 206, 42–54. doi: 10.1016/j.synthmet.2015.04.014
Alotaibi, M. D., Alshammari, B. A., Saba, N., Alothman, O. Y., Sanjay, M. R., Almutairi, Z., et al. (2019). Characterization of natural fiber obtained from different parts of date palm tree ( Phoenix dactylifera L.). Int. J. Biol. Macromol. 135, 69–76. doi: 10.1016/j.ijbiomac.2019.05.102
PubMed Abstract | CrossRef Full Text | Google Scholar
Angelini, L. G., and Tavarini, S. (2013). Ramie [ Boehmeria nivea (L.) Gaud.] as a potential new fibre crop for the Mediterranean region: growth, crop yield and fibre quality in a long-term field experiment in Central Italy. Ind. Crops Prod. 51, 138–144. doi: 10.1016/j.indcrop.2013.09.009
Anuar, H., and Zuraida, A. (2011). Improvement in mechanical properties of reinforced thermoplastic elastomer composite with kenaf bast fibre. Compos. Part B Eng. 42, 462–465. doi: 10.1016/j.compositesb.2010.12.013
Arifuzzaman Khan, G. M., Shaheruzzaman, M., Rahman, M. H., Abdur Razzaque, S. M., Islam, M. S., and Alam, M. S. (2009). Surface modification of okra bast fiber and its physico-chemical characteristics. Fibers Polym. 10, 65–70. doi: 10.1007/s12221-009-0065-1
Arjmandi, R., Hassan, A., and Zakaria, Z. (2017). “Rice husk and kenaf fiber reinforced polypropylene biocomposites,” in Lignocellulosic Fibre and Biomass-Based Composite Materials , eds M. Jawaid, M. T. Paridah, and N. Saba (Woodhead Publishing), 77–94. doi: 10.1016/B978-0-08-100959-8.00005-6
Arpitha, G. R., Sanjay, M. R., Senthamaraikannan, P., Barile, C., and Yogesha, B. (2017). Hybridization effect of sisal/glass/epoxy/filler based woven fabric reinforced composites. Exp. Tech. 41, 577–584. doi: 10.1007/s40799-017-0203-4
Arpitha, G. R., and Yogesha, B. (2017). Science direct an overview on mechanical property evaluation of natural fiber reinforced polymers. Mater. Today Proc. 4, 2755–2760. doi: 10.1016/j.matpr.2017.02.153
Arulandoo, X., Sritharan, K., and Subramaniam, M. (2016). The Coconut Palm, 2nd Edn . Elsevier. doi: 10.1016/B978-0-12-394807-6.00237-9
Arumugam, V. (2014). A preliminary investigation on Kapok/polypropylene nonwoven composites for sound absorption. Ind. J. Fibre Text. Res. 37, 385–388.
Arunachalam, V. (2012). Date palm. Genomics Cultiv. Palms , 49–59. doi: 10.1016/B978-0-12-387736-9.00004-2
Asim, M., Saba, N., Jawaid, M., and Nasir, M. (2018). “Potential of natural fiber/biomass filler-reinforced polymer composites in aerospace applications,” in Sustainable Composites for Aerospace Applications , eds M. Jawaid and M. Thariq (Elsevier Ltd.), 253–268. doi: 10.1016/B978-0-08-102131-6.00012-8
Aslan, M., Tufan, M., and Küçükömeroglu, T. (2018). Tribological and mechanical performance of sisal-filled waste carbon and glass fibre hybrid composites. Compos. Part B Eng. 140, 241–249. doi: 10.1016/j.compositesb.2017.12.039
Asumani, O. M. L., Reid, R. G., and Paskaramoorthy, R. (2012). The effects of alkali–silane treatment on the tensile and flexural properties of short fibre non-woven kenaf reinforced polypropylene composites. Compos Part A Appl. Sci. Manufact. 43, 1431–1440. doi: 10.1016/j.compositesa.2012.04.007
Athith, D., Sanjay, M. R., Yashas Gowda, T. G., Madhu, P., Arpitha, G. R., Yogesha, B., et al. (2018). Effect of tungsten carbide on mechanical and tribological properties of jute/sisal/E-glass fabrics reinforced natural rubber/epoxy composites. J. Ind. Text. 48, 713–737. doi: 10.1177/1528083717740765
Atiqah, A., Jawaid, M., Ishak, M. R., and Sapuan, S. M. (2018). Effect of alkali and silane treatments on mechanical and interfacial bonding strength of sugar palm fibers with thermoplastic polyurethane. J. Nat. Fibers 15, 251–261. doi: 10.1080/15440478.2017.1325427
Atiqah, A., Maleque, M. A., Jawaid, M., and Iqbal, M. (2014). Development of kenaf-glass reinforced unsaturated polyester hybrid composite for structural applications. Compos. Part B 56, 68–73. doi: 10.1016/j.compositesb.2013.08.019
Aziz, S. H., and Ansell, M. P. (2004). The effect of alkalization and fibre alignment on the mechanical and thermal properties of kenaf and hemp bast fibre composites: part 1 - polyester resin matrix. Compos. Sci. Technol. 64, 1219–1230. doi: 10.1016/j.compscitech.2003.10.001
Azwa, Z. N., Yousif, B. F., Manalo, A. C., and Karunasena, W. (2013). A review on the degradability of polymeric composites based on natural fibres. Mater. Des. 47, 424–442. doi: 10.1016/j.matdes.2012.11.025
Bacci, L., Baronti, S., Predieri, S., and di Virgilio, N. (2009). Fiber yield and quality of fiber nettle ( Urtica dioica L.) cultivated in Italy. Ind. Crops Prod. 29, 480–484. doi: 10.1016/j.indcrop.2008.09.005
Baiardo, M., Frisoni, G., Scandola, M., and Licciardello, A. (2002). Surface chemical modification of natural cellulose fibers. J. Appl. Polym. Sci. 83, 38–45. doi: 10.1002/app.2229
Balaji, V., and Senthil Vadivu, K. (2017). Mechanical characterization of coir fiber and cotton fiber reinforced unsaturated polyester composites for packaging applications mechanical characterization of coir fiber and cotton fiber reinforced. J. Appl. Packag. Res. 9, 12–19.
Balakrishnan, P., John, M. J., Pothen, L., Sreekala, M. S., and Thomas, S. (2016). “Natural fibre and polymer matrix composites and their applications in aerospace engineering,” in Advanced Composite Materials for Aerospace Engineering , eds S. Rana and R. Fangueiro (Elsevier Ltd.), 365–383. doi: 10.1016/B978-0-08-100037-3.00012-2
Balan, A. K., Mottakkunnu Parambil, S., Vakyath, S., Thulissery Velayudhan, J., Naduparambath, S., and Etathil, P. (2017). Coconut shell powder reinforced thermoplastic polyurethane/natural rubber blend-composites: effect of silane coupling agents on the mechanical and thermal properties of the composites. J. Mater. Sci. 52, 6712–6725. doi: 10.1007/s10853-017-0907-y
Banik, S., Basak, M. K., Paul, D., Nayak, P., Sardar, D., Sil, S. C., et al. (2003). Ribbon retting of jute - a prospective and eco-friendly method for improvement of fibre quality. Ind. Crops Prod. 17, 183–190. doi: 10.1016/S0926-6690(02)00097-3
Behera, A. K., Avancha, S., Basak, R. K., Sen, R., and Adhikari, B. (2012). Fabrication and characterizations of biodegradable jute reinforced soy based green composites. Carbohydr. Polym. 88, 329–335. doi: 10.1016/j.carbpol.2011.12.023
Bhoopathi, R., Ramesh, M., and Deepa, C. (2014). Fabrication and property evaluation of banana-hemp-glass fiber reinforced composites. Proc. Eng. 97, 2032–2041. doi: 10.1016/j.proeng.2014.12.446
Biswas, S., Shahinur, S., Hasan, M., and Ahsan, Q. (2015). Physical, mechanical and thermal properties of jute and bamboo fiber reinforced unidirectional epoxy composites. Proc. Eng. 105, 933–939. doi: 10.1016/j.proeng.2015.05.118
Bledzki, A. K., and Gassan, J. (1999). Composites reinforced with cellulose based fibres. Prog. Polym. Sci. 24, 221–274. doi: 10.1016/S0079-6700(98)00018-5
Bodur, M. S., Bakkal, M., and Sonmez, H. E. (2016). The different chemical treatment methods. J. Compos. Mater. 50, 3817–3830. doi: 10.1177/0021998315626256
Boopalan, M., Niranjanaa, M., and Umapathy, M. J. (2013). Study on the mechanical properties and thermal properties of jute and banana fiber reinforced epoxy hybrid composites. Compos. Part B Eng. 51, 54–57. doi: 10.1016/j.compositesb.2013.02.033
Borba, P. M., Tedesco, A., and Lenz, D. M. (2013). Effect of reinforcement nanoparticles addition on mechanical properties of SBS/curauá fiber composites. Mater. Res. 17, 412–419. doi: 10.1590/S1516-14392013005000203
Bos, H. L., Müssig, J., and van den Oever, M. J. A. (2006). Mechanical properties of short-flax-fibre reinforced compounds. Compos. Part A Appl. Sci. Manuf. 37, 1591–1604. doi: 10.1016/j.compositesa.2005.10.011
Bourgeois, C., Leclerc, É. A., Corbin, C., Doussot, J., Serrano, V., Vanier, J. R., et al. (2016). L'ortie ( Urtica dioica L.), une source de produits antioxidants et phytochimiques anti-âge pour des applications en cosmétique. Comptes Rendus Chim. 19, 1090–1100. doi: 10.1016/j.crci.2016.03.019
Bourmaud, A., Siniscalco, D., Foucat, L., Goudenhooft, C., Falourd, X., Pontoire, B., et al. (2019). Evolution of flax cell wall ultrastructure and mechanical properties during the retting step. Carbohydr. Polym. 206, 48–56. doi: 10.1016/j.carbpol.2018.10.065
Bunsell, A. R. (ed.). (2018). “Introduction to the science of fibers,” in Handbook of Properties of Textile and Technical Fibres (Woodhead Publishing), 1–20. doi: 10.1016/B978-0-08-101272-7.00001-8
Castrillón Martínez, D. C., Zuluaga, C. L., Restrepo-Osorio, A., and Álvarez-López, C. (2017). Characterization of sericin obtained from cocoons and silk yarns. Proc. Eng. 200, 377–383. doi: 10.1016/j.proeng.2017.07.053
Cengiz, T. G., and Babalik, F. C. (2009). The effects of ramie blended car seat covers on thermal comfort during road trials. Int. J. Ind. Ergon. 39, 287–294. doi: 10.1016/j.ergon.2008.12.002
Chand, N., and Jain, D. (2005). Effect of sisal fibre orientation on electrical properties of sisal fibre reinforced epoxy composites. Compos. Part A Appl. Sci. Manuf. 36, 594–602. doi: 10.1016/j.compositesa.2004.08.002
Charlet, K., Jernot, J. P., Breard, J., and Gomina, M. (2010). Scattering of morphological and mechanical properties of flax fibres. Ind. Crops Prod. 32, 220–224. doi: 10.1016/j.indcrop.2010.04.015
Chauhan, A., and Chauhan, P. (2015). Natural fibers and biopolymer. J. Chem. Eng. Process Technol. 6, 1–4. doi: 10.4172/2157-7048.S6-001
Chen, Y., Su, N., Zhang, K., Zhu, S., Zhu, Z., Qin, W., et al. (2018). Effect of fiber surface treatment on structure, moisture absorption and mechanical properties of luffa sponge fiber bundles. Ind. Crops Prod. 123, 341–352. doi: 10.1016/j.indcrop.2018.06.079
Cheung, H., Ho, M., Lau, K., Cardona, F., and Hui, D. (2009). Natural fibre-reinforced composites for bioengineering and environmental engineering applications. Compos. Part B Eng. 40, 655–663. doi: 10.1016/j.compositesb.2009.04.014
Clarke, R. C. (2010). Traditional fiber hemp (Cannabis) production, processing, yarn making, and weaving strategies-functional constraints and regional responses. Part 2. J. Nat. Fibers 7, 229–250. doi: 10.1080/15440478.2010.504043
Colomban, P., and Jauzein, V. (2018). “Silk: fibers, films, and composites-types, processing, structure, and mechanics,” in Handbook of Properties of Textile and Technical Fibres , ed A. R. Bunsell (Woodhead Publishing), 137–183. doi: 10.1016/B978-0-08-101272-7.00005-5
Cummings, A. J., and Olsen, M. (2011). Mechanism of action of stinging nettles. Wilderness Environ. Med. 22, 136–139. doi: 10.1016/j.wem.2011.01.001
Danso, H. (2017). Properties of coconut, oil palm and bagasse fibres: as potential building materials. Proc. Eng. 200, 1–9. doi: 10.1016/j.proeng.2017.07.002
Das, S. (2017). Mechanical properties of waste paper/jute fabric reinforced polyester resin matrix hybrid composites. Carbohydr. Polym. 172, 60–67. doi: 10.1016/j.carbpol.2017.05.036
Das, S., and Natarajan, G. (2019). “Silk fiber composites in biomedical applications,” in Materials for Biomedical Engineering , eds V. Grumezescu and A. Mihai Grumezescu (Elsevier), 309–338. doi: 10.1016/B978-0-12-816872-1.00011-X
de Melo, R. P., Marques, M. F. V., Navard, P., and Duque, N. P. (2017). Degradation studies and mechanical properties of treated curauá fibers and microcrystalline cellulose in composites with polyamide 6. J. Compos. Mater. 51, 3481–3489. doi: 10.1177/0021998317690446
De Prez, J., Van Vuure, A. W., Ivens, J., Aerts, G., and Van de Voorde, I. (2018). Enzymatic treatment of flax for use in composites. Biotechnol. Rep. 20:e00294. doi: 10.1016/j.btre.2018.e00294
Debeli, D. K., Qin, Z., and Guo, J. (2018). Study on the pre-treatment, physical and chemical properties of ramie fibers reinforced poly (Lactic Acid) (PLA) biocomposite. J. Nat. Fibers 15, 596–610. doi: 10.1080/15440478.2017.1349711
Deshpande, A. P., Bhaskar Rao, M., and Lakshmana Rao, C. (2000). Extraction of bamboo fibers and their use as reinforcement in polymeric composites. J. Appl. Polym. Sci. 76, 83–92 doi: 10.1002/(SICI)1097-4628(20000404)76:1<83::AID-APP11>3.0.CO;2-L
Devaraju, A., and Harikumar, R. (2019). “Life cycle assessment of sisal fiber,” in Reference Module in Materials Science and Materials Engineering (Elsevier Ltd.). doi: 10.1016/B978-0-12-803581-8.10552-1
Dixit, S., Goel, R., Dubey, A., Shivhare, P. R., and Bhalavi, T. (2017). Natural fibre reinforced polymer composite materials - A review. Polym. Renew. Resour. 8, 71–78. doi: 10.1177/204124791700800203
Dolez, P. I., Arfaoui, M. A., Dub,é, M., and David, É. (2017). Hydrophobic treatments for natural fibers based on metal oxide nanoparticles and fatty acids. Proc. Eng. 200, 81–88. doi: 10.1016/j.proeng.2017.07.013
Dong, T., Xu, G., and Wang, F. (2015). Adsorption and adhesiveness of kapok fiber to different oils. J. Hazard. Mater. 296, 101–111. doi: 10.1016/j.jhazmat.2015.03.040
dos Santos, J. C., Siqueira, R. L., Vieira, L. M. G., Freire, R. T. S., Mano, V., and Panzera, T. H. (2018). Effects of sodium carbonate on the performance of epoxy and polyester coir-reinforced composites. Polym. Test. 67, 533–544. doi: 10.1016/j.polymertesting.2018.03.043
Duval, A., Bourmaud, A., Augier, L., and Baley, C. (2011). Influence of the sampling area of the stem on the mechanical properties of hemp fibers. Mater. Lett. 65, 797–800. doi: 10.1016/j.matlet.2010.11.053
Edeerozey, A. M. M., Akil, H. M., Azhar, A. B., and Ariffin, M. I. Z. (2007). Chemical modification of kenaf fibers. Mater. Lett. 61, 2023–2025. doi: 10.1016/j.matlet.2006.08.006
Elmogahzy, Y., and Farag, R. (2018). “Tensile properties of cotton fibers: importance, research, and limitations”, in Handbook of Properties of Textile and Technical Fibres , ed A. R. Bunsell (Woodhead Publishing), 223–273. doi: 10.1016/B978-0-08-101272-7.00007-9
Fakhrul, T., and Islam, M. A. (2013). Degradation behavior of natural fiber reinforced polymer matrix composites. Proc. Eng. 56, 795–800. doi: 10.1016/j.proeng.2013.03.198
Fan, M., and Weclawski, B. (2016). “Long natural fibre composites,” in Advanced High Strength Natural Fibre Composites in Construction , eds M. Fan and F. Fu (Woodhead Publishing), 141–177. doi: 10.1016/B978-0-08-100411-1.00006-6
Fang, H., Zhang, Y., Deng, J., and Rodrigue, D. (2013). Effect of fiber treatment on the water absorption and mechanical properties of hemp fiber/polyethylene composites. J. Appl. Polym. Sci. 127, 942–949. doi: 10.1002/app.37871
Faruk, O., Bledzki, A. K., Fink, H. P., and Sain, M. (2012). Biocomposites reinforced with natural fibers: 2000-2010. Prog. Polym. Sci. 37, 1552–1596. doi: 10.1016/j.progpolymsci.2012.04.003
Faruk, O., Bledzki, A. K., Fink, H. P., and Sain, M. (2014). Progress report on natural fiber reinforced composites. Macromol. Mater. Eng. 299, 9-26. doi: 10.1002/mame.201300008
Gassan, J., and Bledzki, A. K. (1997). The influence of fiber-surface treatment on the mechanical properties of jute-polypropylene composites. Compos. Part A Appl. Sci. Manuf. 28, 1001–1005. doi: 10.1016/S1359-835X(97)00042-0
Gassan, J., and Bledzki, A. K. (1999). Possibilities for improving the mechanical properties of jute/epoxy composites by alkali treatment of fibres. Compos. Sci. Technol. 59, 1303–1309. doi: 10.1016/S0266-3538(98)00169-9
George, J., Sreekala, M. S., and Thomas, S. (2001). A review on interface modification and characterization of natural fiber reinforced plastic composites. Polym. Eng. Sci. 41, 1471–1485. doi: 10.1002/pen.10846
Gheith, M. H., Aziz, M. A., Ghori, W., Saba, N., Asim, M., Jawaid, M., et al. (2018). Flexural, thermal and dynamic mechanical properties of date palm fibres reinforced epoxy composites. J. Mater. Res. Technol. 8, 853–860. doi: 10.1016/j.jmrt.2018.06.013
Gunti, R., Ratna Prasad, A. V., and Gupta, A. V. S. S. K. S. (2018). Mechanical and degradation properties of natural fiber-reinforced PLA composites: jute, sisal, and elephant grass. Polym. Compos. 39, 1125–1136. doi: 10.1002/pc.24041
Gupta, M. K., and Srivastava, R. K. (2016). Mechanical properties of hybrid fibers-reinforced polymer composite: a review. Polym. Plast. Technol. Eng. 55, 626–642. doi: 10.1080/03602559.2015.1098694
Gurunathan, T., Mohanty, S., and Nayak, S. K. (2015). A review of the recent developments in biocomposites based on natural fibres and their application perspectives. Compos Part Appl. Sci. Manuf. 77, 1–25. doi: 10.1016/j.compositesa.2015.06.007
Halip, J. A., Hua, L. S., Ashaari, Z., Tahir, P. M., Chen, L. W., and Uyup, M. K. A. (2018). “Effect of treatment on water absorption behavior of natural,” in Mechanical and Physical Testing of Biocomposites, Fibre-Reinforced Composites and Hybrid Composites , eds M. Jawaid, M. Thariq, and N. Saba (Woodhead Publishing), 141–156. doi: 10.1016/B978-0-08-102292-4.00008-4
Hamidon, M. H., Sultan, M. T. H., Ariffin, A. H., and Shah, A. U. M. (2019). Effects of fibre treatment on mechanical properties of kenaf fibre reinforced composites: a review. J. Mater. Res. Technol. 8, 3327–3337. doi: 10.1016/j.jmrt.2019.04.012
Hill, C. A. S., Khalil, H. P. S. A., and Hale, M. D. (1998). A study of the potential of acetylation to improve the properties of plant fibres. Ind. Crops Prod. 8, 53–63. doi: 10.1016/S0926-6690(97)10012-7
Holbery, J., and Houston, D. (2006). Natural-fiber-reinforced polymer composites in automotive applications. JOM 58, 80–86. doi: 10.1007/s11837-006-0234-2
Huda, M. S., Drzal, L. T., Mohanty, A. K., and Misra, M. (2006). Chopped glass and recycled newspaper as reinforcement fibers in injection molded poly(lactic acid) (PLA) composites: a comparative study. Compos. Sci. Technol. 66, 1813–1824. doi: 10.1016/j.compscitech.2005.10.015
Jawaid, M., and Abdul Khalil, H. P. S. (2011). Cellulosic/synthetic fibre reinforced polymer hybrid composites: a review. Carbohydr. Polym. 86, 1–18. doi: 10.1016/j.carbpol.2011.04.043
Jayamol, G., Bhagawan, S. S., and Thomas, S. (1997). Electrical properties of pineapple fibre reinforced polyethylene composites. J. Polym. Eng. 17, 383–404. doi: 10.1515/POLYENG.1997.17.5.383
Joseph, P. V., Joseph, K., Thomas, S., Pillai, C. K. S., Prasad, V. S., Groeninckx, G., et al. (2003). The thermal and crystallization studies of polypropylene composites. Compos. Part A Appl. Sci. Manufact. 34, 253–266. doi: 10.1016/S1359-835X(02)00185-9
Joseph, S., and Thomas, S. (2008). Electrical properties of banana fiber reinforced phenol formaldehyde composites. J. Appl. Polym. Sci. 109, 256–263. doi: 10.1002/app.27452
Kabir, M. M., Wang, H., Lau, K. T., and Cardona, F. (2012). Chemical treatments on plant-based natural fibre reinforced polymer composites: an overview. Compos. Part B Eng. 43, 2883–2892. doi: 10.1016/j.compositesb.2012.04.053
Kaith, B. S., and Kalia, S. (2007). Grafting of flax fiber ( Linum usitatissimum ) with vinyl monomers for enhancement of properties of flax-phenolic composites. Polym. J. 39, 1319–1327. doi: 10.1295/polymj.PJ2007073
Kengkhetkit, N., and Amornsakchai, T. (2012). Utilisation of pineapple leaf waste for plastic reinforcement: 1. A novel extraction method for short pineapple leaf fiber. Ind. Crops Prod. 40, 55–61. doi: 10.1016/j.indcrop.2012.02.037
Khan, J. A., and Khan, M. A. (2014). “The use of jute fibers as reinforcements in composites”, in Biofiber Reinforcements in Composite Materials , eds O. Faruk and M. Sain (Woodhead Publishing), 3–34. doi: 10.1533/9781782421276.1.3
Khan, M. A., Hassan, M. M., and Drzal, L. T. (2005). Effect of 2-hydroxyethyl methacrylate (HEMA) on the mechanical and thermal properties of jute-polycarbonate composite. Compos. Part A Appl. Sci. Manufact. 36, 71–81. doi: 10.1016/S1359-835X(04)00178-2
Khan, M. A., Hassan, M. M., Taslima, R., and Mustafa, A. I. (2006). Role of pretreatment with potassium permanganate and urea on mechanical and degradable properties of photocured coir (cocos nucifera) fiber with 1,6-hexanediol diacrylate. J. Appl. Polym. Sci. 100, 4361–4368. doi: 10.1002/app.23863
Khondker, O. A., Ishiaku, U. S., Nakai, A., and Hamada, H. (2005). Fabrication and mechanical properties of unidirectional jute/PP composites using jute yarns by film stacking method. J. Polym. Environ. 13, 115–126. doi: 10.1007/s10924-005-2943-y
Kicinska-Jakubowska, A., Bogacz, E., and Zimniewska, M. (2012). Review of natural fibers. Part I—vegetable fibers. J. Nat. Fibers 9, 150–167. doi: 10.1080/15440478.2012.703370
Kinloch, A. J., Taylor, A. C., Techapaitoon, M., Teo, W. S., and Sprenger, S. (2015). Tough, natural-fibre composites based upon epoxy matrices. J. Mater. Sci. 50, 6947–6960. doi: 10.1007/s10853-015-9246-z
Kipriotis, E., Heping, X., Vafeiadakis, T., Kiprioti, M., and Alexopoulou, E. (2015). Ramie and kenaf as feed crops. Ind. Crops Prod. 68, 126–130. doi: 10.1016/j.indcrop.2014.10.002
Komuraiah, A., Kumar, N. S., and Prasad, B. D. (2014). Chemical composition of natural fibers and its influence on their mechanical properties. Mech. Compos. Mat . 50, 359–376. doi: 10.1007/s11029-014-9422-2
Ku, H., Wang, H., Pattarachaiyakoop, N., and Trada, M. (2011). A review on the tensile properties of natural fiber reinforced polymer composites. Compos. Part B Eng. 42, 856–873. doi: 10.1016/j.compositesb.2011.01.010
Laftah, W. A., and Abdul Rahaman, W. A. W. (2015). Chemical pulping of waste pineapple leaves fiber for kraft paper production. J. Mater. Res. Technol. 4, 254–261. doi: 10.1016/j.jmrt.2014.12.006
Lanzilao, G., Goswami, P., and Blackburn, R. S. (2016). Study of the morphological characteristics and physical properties of Himalayan giant nettle ( Girardinia diversifolia L.) fibre in comparison with European nettle ( Urtica dioica L.) fibre. Mater. Lett. 181, 200–203. doi: 10.1016/j.matlet.2016.06.044
Lau, K., Hung, P., Zhu, M. H., and Hui, D. (2018). Properties of natural fibre composites for structural engineering applications. Compos. Part B Eng. 136, 222–233. doi: 10.1016/j.compositesb.2017.10.038
Li, X., Tabil, L. G., and Panigrahi, S. (2007). Chemical treatments of natural fiber for use in natural fiber-reinforced composites: a review. J. Polym. Environ. 15, 25–33. doi: 10.1007/s10924-006-0042-3
Li, Y., Mai, Y., and Ye, L. (2000). Sisal ® bre and its composites : a review of recent developments IM PA US AS DO ME US EX ON AS. Compos. Sci. Technol. 60, 2037–2055. doi: 10.1016/S0266-3538(00)00101-9
Li, Z., Wang, X., and Wang, L. (2006). Properties of hemp fibre reinforced concrete composites. Compos. Part A Appl. Sci. Manuf. 37, 497–505. doi: 10.1016/j.compositesa.2005.01.032
Liu, R., Lv, W., Wang, X., Zhai, M., Dong, P., and Wang, Z. (2018). Carbonization and combustion characteristics of palm fiber. Fuel 227, 21–26. doi: 10.1016/j.fuel.2018.02.088
Liu, W., Mohanty, A. K., Drzal, L. T., Askeland, P., and Misra, M. (2004). Effects of alkali treatment on the structure, morphology and thermal properties of native grass fibers as reinforcements for polymer matrix composites. J. Mater. Sci. 39:1051. doi: 10.1023/B:JMSC.0000012942.83614.75
Liu, W., Mohanty, A. K., Drzal, L. T., and Misra, M. (2005). Novel biocomposites from native grass and soy based bioplastic: processing and properties evaluation. Ind. Eng. Chem. Res. 44, 7105–7112. doi: 10.1021/ie050257b
Madhu, P., Sanjay, M. R., Pradeep, S., Subrahmanya Bhat, K., Yogesha, B., and Siengchin, S. (2019a). Characterization of cellulosic fibre from Phoenix pusilla leaves as potential reinforcement for polymeric composites. J. Mater. Res. Technol. 8, 2597–2604. doi: 10.1016/j.jmrt.2019.03.006
Madhu, P., Sanjay, M. R., Senthamaraikannan, P., Pradeep, S., Saravanakumar, S. S., and Yogesha, B. (2019b). A review on synthesis and characterization of commercially available natural fibers: Part II. J. Nat. Fibers 16, 25–36. doi: 10.1080/15440478.2017.1379045
Madhu, P., Sanjay, M. R., Senthamaraikannan, P., Pradeep, S., Siengchin, S., Jawaid, M., et al. (2018). Effect of various chemical treatments of prosopis juliflora fibers as composite reinforcement: physicochemical, thermal, mechanical, and morphological properties. J. Nat. Fibers 00, 1–12. doi: 10.1080/15440478.2018.1534191
Mahjoub, R., Yatim, J. M., Mohd Sam, A. R., and Hashemi, S. H. (2014). Tensile properties of kenaf fiber due to various conditions of chemical fiber surface modifications. Constr. Build. Mater. 55, 103–113. doi: 10.1016/j.conbuildmat.2014.01.036
Manikandan Nair, K. C., Thomas, S., and Groeninckx, G. (2001). Thermal and dynamic mechanical analysis of polystyrene composites reinforced with short sisal fibres. Compos. Sci. Technol. 61, 2519–2529. doi: 10.1016/S0266-3538(01)00170-1
Manimaran, P., Senthamaraikannan, P., Murugananthan, K., and Sanjay, M. R. (2018). Physicochemical properties of new cellulosic fibers from Azadirachta indica Plant. J. Nat. Fibers 15, 29–38. doi: 10.1080/15440478.2017.1302388
Manimaran, P., Senthamaraikannan, P., Sanjay, M. R., Marichelvam, M. K., and Jawaid, M. (2017). Study on characterization of furcraea foetida new natural fiber as composite reinforcement for lightweight applications. Carbohydr. Polym. 181, 650–658. doi: 10.1016/j.carbpol.2017.11.099
Marsyahyo, E., Jamasri, H. S. B., and Soekrisno (2009). Preliminary investigation on bulletproof panels made from ramie fiber reinforced composites for NIJ Level II, IIA, and IV. J. Ind. Text. 39, 13–26. doi: 10.1177/1528083708098913
Martin, N., Mouret, N., Davies, P., and Baley, C. (2013). Influence of the degree of retting of flax fibers on the tensile properties of single fibers and short fiber/polypropylene composites. Ind. Crops Prod. 49, 755–767. doi: 10.1016/j.indcrop.2013.06.012
Masri, T., Ounis, H., Sedira, L., Kaci, A., and Benchabane, A. (2018). Characterization of new composite material based on date palm leaflets and expanded polystyrene wastes. Constr. Build. Mater. 164, 410–418. doi: 10.1016/j.conbuildmat.2017.12.197
McGregor, B. A. (2018). “Physical, chemical, and tensile properties of cashmere, mohair, alpaca, and other rare animal fibers” in Handbook of Properties of Textile and Technical Fibres , ed A. R. Bunsell (Woodhead Publishing), 105–136. doi: 10.1016/B978-0-08-101272-7.00004-3
Mihai, M. (2013). Novel polylactide/triticale straw biocomposites : processing, formulation, and properties. Polym. Eng. Sci. 54. doi: 10.1002/pen.23575
Mishra, S., Mohanty, A. K., Drzal, L. T., Misra, M., Parija, S., Nayak, S. K., et al. (2003). Studies on mechanical performance of biofibre/glass reinforced polyester hybrid composites. Compos. Sci. Technol. 63, 1377-1385. doi: 10.1016/S0266-3538(03)00084-8
Mochane, M. J., Mokhena, T. C., Mokhothu, T. H., Mtibe, A., Sadiku, E. R., Ray, S. S., et al. (2019). Recent progress on natural fiber hybrid composites for advanced applications: a review. Express Polym. Lett. 13, 159–198. doi: 10.3144/expresspolymlett.2019.15
Mohammed, L., Ansari, M. N. M., Pua, G., Jawaid, M., and Islam, M. S. (2015). A review on natural fiber reinforced polymer composite and its applications. Int. J. Polym. Sci. 2015, 1–15. doi: 10.1155/2015/243947
Monteiro, S. N., Satyanarayana, K. G., Ferreira, A. S., Nascimento, D. C. O., Lopes, F. P. D., Silva, I. L. A., et al. (2010). Selection of high strength natural fibers. Rev. Mater. 15, 488–505. doi: 10.1590/S1517-70762010000400002
Mortazavi, S. M., and Moghaddam, M. K. (2010). An analysis of structure and properties of a natural cellulosic fiber (Leafiran). Fibers Polym. 11, 877–882. doi: 10.1007/s12221-010-0877-z
Murugesh Babu, K. (2016). “Silk from silkworms and spiders as high- performance fibers,” in Structure and Properties of High-Performance Fibers , ed G. Bhat (Woodhead Publishing), 27–366. doi: 10.1016/B978-0-08-100550-7.00013-9
Mwaikambo, L. Y., and Ansell, M. P. (2002). Chemical modification of hemp, sisal, jute, and kapok fibers by alkalization. J. Appl. Polym. Sci. 84, 2222–2234. doi: 10.1002/app.10460
Mwaikambo, L. Y., Martuscelli, E., and Avella, M. (2000). Kapok/cotton fabric-polypropylene composites. Polym. Test. 19, 905–918. doi: 10.1016/S0142-9418(99)00061-6
Nair, K. P. P. (ed.). (2010). “The coconut palm (Cocos nucifera L.),” in The Agronomy and Economy of Important Tree Crops of the Developing World (Elsevier Ltd.), 67–109. doi: 10.1016/B978-0-12-384677-8.00003-5
Nam, S., and Netravali, A. N. (2006). Green composites. I. Physical properties of ramie fibers for environment-friendly green composites. Fibers Polym. 7, 372–379. doi: 10.1007/BF02875769
Nasser, R. A., Salem, M. Z. M., Hiziroglu, S., Al-Mefarrej, H. A., Mohareb, A. S., Alam, M., et al. (2016). Chemical analysis of different parts of date palm ( Phoenix dactylifera L.) using ultimate, proximate and thermo-gravimetric techniques for energy production. Energies 9:374. doi: 10.3390/en9050374
Naveen, J., Jawaid, M., Amuthakkannan, P., and Chandrasekar, M. (2018). “Mechanical and physical properties of sisal and hybrid sisal fiber-reinforced polymer composites,” in Mechanical and Physical Testing of Biocomposites, Fibre-Reinforced Composites and Hybrid Composites , eds M. Jawaid, M. Thariq, and N. Saba (Woodhead Publishing), 427–440. doi: 10.1016/B978-0-08-102292-4.00021-7
Nirmal, U., Hashim, J., and Megat Ahmad, M. M. H. (2015). A review on tribological performance of natural fibre polymeric composites. Tribol. Int. 83, 77–104. doi: 10.1016/j.triboint.2014.11.003
Nishino, T., Hirao, K., Kotera, M., Nakamae, K., and Inagaki, H. (2003). Kenaf reinforced biodegradable composite. Compos. Sci. Technol. 63, 1281–1286. doi: 10.1016/S0266-3538(03)00099-X
Oksman, K. (2001). High quality flax fibre composites manufactured by the resin transfer moulding process. J. Reinf. Plast. Compos. 20, 621–627. doi: 10.1177/073168401772678634
Oksman, K., Aitomäki, Y., Mathew, A. P., Siqueira, G., Zhou, Q., Butylina, S., et al. (2016). Review of the recent developments in cellulose nanocomposite processing. Compos. Part A Appl. Sci. Manuf. 83, 2–18. doi: 10.1016/j.compositesa.2015.10.041
Omar, M. F., Jaya, H., and Zulkepli, N. N. (2019). “Kenaf fiber reinforced composite in the automotive industry,” in Module in Materials Science and Materials Engineering (Elsevier Ltd.). doi: 10.1016/B978-0-12-803581-8.11429-8
Osorio, L., Trujillo, E., Van Vuure, A. W., and Verpoest, I. (2011). Morphological aspects and mechanical properties of single bamboo fibers and flexural characterization of bamboo/ epoxy composites. J. Reinf. Plast. Compos. 30, 396–408. doi: 10.1177/0731684410397683
Panaitescu, D. M., Nicolae, C. A., Vuluga, Z., Vitelaru, C., Sanporean, C. G., Zaharia, C., et al. (2016). Influence of hemp fibers with modified surface on polypropylene composites. J. Ind. Eng. Chem. 37, 137–146. doi: 10.1016/j.jiec.2016.03.018
Pandey, J. K., Ahn, S. H., Lee, C. S., Mohanty, A. K., and Misra, M. (2010). Recent advances in the application of natural fiber based composites. Macro Mater. Eng. 295, 975–989. doi: 10.1002/mame.201000095
Paridah, M. T., Arib, R. M. N., Sapuan, S. M., Zaman, H. M. D. K., and Ahmad, M. M. H. M. (2004). Mechanical properties of pineapple leaf fibre reinforced polypropylene composites. Mater. Des. 27, 391–396. doi: 10.1016/j.matdes.2004.11.009
Paul, A., Joseph, K., and Thomas, S. (1997). Effect of surface treatments on the electrical properties of low-density polyethylene composites reinforced with short sisal fibers. Compos. Sci. Technol. 57, 67–79. doi: 10.1016/S0266-3538(96)00109-1
Pham, L. J. (2016). Coconut (Cocos nucifera). AOCS Press. doi: 10.1016/B978-1-893997-98-1.00009-9
Pickering, K. L., Efendy, M. G. A., and Le, T. M. (2016). A review of recent developments in natural fibre composites and their mechanical performance. Compos. Part A Appl. Sci. Manuf. 83, 98–112. doi: 10.1016/j.compositesa.2015.08.038
Prachayawarakorn, J., Chaiwatyothin, S., Mueangta, S., and Hanchana, A. (2013). Effect of jute and kapok fibers on properties of thermoplastic cassava starch composites. Mater. Des. 47, 309–315. doi: 10.1016/j.matdes.2012.12.012
Preet Singh, J. I., Dhawan, V., Singh, S., and Jangid, K. (2017). Study of effect of surface treatment on mechanical properties of natural fiber reinforced composites. Mater. Today Proc. 4, 2793–2799. doi: 10.1016/j.matpr.2017.02.158
Puttegowda, M., Rangappa, S. M., Jawaid, M., Shivanna, P., Basavegowda, Y., and Saba, N. (2018). “Potential of natural/synthetic hybrid composites for aerospace,in applications,” in Sustainable Composites for Aerospace Applications , eds M. Jawaid and M. Thariq (Woodhead Publishing), 315–351. doi: 10.1016/B978-0-08-102131-6.00021-9
Rahman, M. S. (2010). “Jute-a versatile natural fibre. Cultivation, extraction and processing,” in Industrial Applications of Natural Fibres Industrial Applications of Natural Fibres: Structure, Properties and Technical Applications , ed J. Müssig (Wiley), 135–161. doi: 10.1002/9780470660324.ch6
Raman Bharath, V. R., Vijaya Ramnath, B., and Manoharan, N. (2015). Kenaf fibre reinforced composites: a review. ARPN J. Eng. Appl. Sci. 10, 5483–5485.
Ramesh, M. (2019). Flax ( Linum usitatissimum L.) fibre reinforced polymer composite materials: a review on preparation, properties and prospects. Prog. Mater. Sci. 102, 109–166. doi: 10.1016/j.pmatsci.2018.12.004
Ramesh, M., Palanikumar, K., and Reddy, K. H. (2013). Mechanical property evaluation of sisal-jute-glass fiber reinforced polyester composites. Compos. Part B Eng. 48, 1-9. doi: 10.1016/j.compositesb.2012.12.004
Rangappa, S. M., and Siengchin, S. (2018). Natural fibers as perspective materials. KMUTNB Int. J. Appl. Sci. Technol. 11:2018. doi: 10.14416/j.ijast.2018.09.001
Reddy, K. O., Maheswari, C. U., Shukla, M., and Rajulu, A. V. (2012). Chemical composition and structural characterization of Napier grass fibers. Mater. Lett. 67, 35–38. doi: 10.1016/j.matlet.2011.09.027
Reddy, N., and Yang, Y. (2015) “Bacterial cellulose fibers,” in Innovative Biofibers from Renewable Resources (Berlin; Heidelberg: Springer), 307–329. doi: 10.1007/978-3-662-45136-6_61
Rehman, M., Gang, D., Liu, Q., Chen, Y., Wang, B., Peng, D., et al. (2019). Ramie, a multipurpose crop: potential applications, constraints and improvement strategies. Ind. Crops Prod. 137, 300–307. doi: 10.1016/j.indcrop.2019.05.029
Réquilé, S., Le Duigou, A., Bourmaud, A., and Baley, C. (2018). Peeling experiments for hemp retting characterization targeting biocomposites. Ind. Crops Prod. 123, 573–580. doi: 10.1016/j.indcrop.2018.07.012
Rivera, D., Obón, C., Alcaraz, F., Laguna, E., and Johnson, D. (2019). Date-palm (Phoenix, Arecaceae) iconography in coins from the Mediterranean and West Asia (485 BC−1189 AD). J. Cult. Herit. 37, 199–214. doi: 10.1016/j.culher.2018.10.010
Rokbi, M., Osmani, H., Imad, A., and Benseddiq, N. (2011). Effect of chemical treatment on flexure properties of natural fiber-reinforced polyester composite. Proc. Eng. 10, 2092–2097. doi: 10.1016/j.proeng.2011.04.346
Rong, M. Z., Zhang, M. Q., Liu, Y., Yang, G. C., and Zeng, H. M. (2001). The effect of fiber treatment on the mechanical properties of unidirectional sisal-reinforced epoxy composites. Compos. Sci. Technol. 61, 1437–1447. doi: 10.1016/S0266-3538(01)00046-X
Ruan, P., Du, J., Gariepy, Y., and Raghavan, V. (2015). Characterization of radio frequency assisted water retting and flax fibers obtained. Ind. Crops Prod. 69, 228–237. doi: 10.1016/j.indcrop.2015.02.009
Saba, N., Paridah, M. T., and Jawaid, M. (2015). Mechanical properties of kenaf fibre reinforced polymer composite: a review. Constr. Build. Mater. 76, 87–96. doi: 10.1016/j.conbuildmat.2014.11.043
Saba, N., Tahir, P. M., and Jawaid, M. (2014). A review on potentiality of nano filler/natural fiber filled polymer hybrid composites. Polymers (Basel). 6, 2247–2273. doi: 10.3390/polym6082247
Sam-Brew, S., and Smith, G. D. (2015). Flax and Hemp fiber-reinforced particleboard. Ind. Crops Prod. 77, 940–948. doi: 10.1016/j.indcrop.2015.09.079
Sanjay, M. R., Arpitha, G. R., Naik, L. L., Gopalakrishna, K., and Yogesha, B. (2016). Applications of natural fibers and its composites: an overview. Nat. Resour. 7, 108–114. doi: 10.4236/nr.2016.73011
Sanjay, M. R., Arpitha, G. R., Senthamaraikannan, P., Kathiresan, M., Saibalaji, M. A., and Yogesha, B. (2019a). The hybrid effect of jute/kenaf/e-glass woven fabric epoxy composites for medium load applications: impact, inter-laminar strength, and failure surface characterization. J. Nat. Fibers 16, 600–612. doi: 10.1080/15440478.2018.1431828
Sanjay, M. R., Arpitha, G. R., and Yogesha, B. (2015). Study on mechanical properties of natural - glass fibre reinforced polymer hybrid composites : a review. Mater. Today Proc. 2, 2959–2967. doi: 10.1016/j.matpr.2015.07.264
Sanjay, M. R., Madhu, P., Jawaid, M., Senthamaraikannan, P., Senthil, S., and Pradeep, S. (2018). Characterization and properties of natural fiber polymer composites: a comprehensive review. J. Clean. Prod. 172, 566-581. doi: 10.1016/j.jclepro.2017.10.101
Sanjay, M. R., Siengchin, S., Parameswaranpillai, J., Jawaid, M., Pruncu, C. I., and Khan, A. (2019b). A comprehensive review of techniques for natural fibers as reinforcement in composites: preparation, processing and characterization. Carbohydr. Polym. 207, 108–121. doi: 10.1016/j.carbpol.2018.11.083
Sanjay, M. R., and Suchart, S. (2019). Lightweight natural fiber composites. J. Appl. Agric. Sci. Technol. 3:178. doi: 10.32530/jaast.v3i2.108
Santhosh Kumar, S., and Hiremath, S. S. (2019). “Natural fiber reinforced composites in the context of biodegradability: a review,” in Reference Module in Materials Science and Materials Engineering (Elsevier Ltd.). doi: 10.1016/B978-0-12-803581-8.11418-3
Saravanakumaar, A., Senthilkumar, A., Saravanakumar, S. S., and Sanjay, M. R. (2018). Impact of alkali treatment on physico-chemical, thermal, structural and tensile properties of Carica papaya bark fibers. Int. J. Polym. Anal. Charact. 23, 529–536. doi: 10.1080/1023666X.2018.1501931
Saravanakumar, S. S., Kumaravel, A., Nagarajan, T., and Moorthy, I. G. (2014). Effect of chemical treatments on physicochemical properties of Prosopis juliflora fibers. Int. J. Polym. Anal. Charact. 19, 383–390. doi: 10.1080/1023666X.2014.903585
Sawpan, M. A., Pickering, K. L., and Fernyhough, A. (2011). Improvement of mechanical performance of industrial hemp fibre reinforced polylactide biocomposites. Compos. Part A Appl. Sci. Manuf. 42, 310–319. doi: 10.1016/j.compositesa.2010.12.004
Sen, T., and Jagannatha Reddy, H. N. (2011a). Application of sisal, bamboo, coir and jute natural composites in structural upgradation. Int. J. Innov. Manag. Technol. 2:186.
Sen, T., and Jagannatha Reddy, H. N. (2011b). Various industrial applications of hemp, kinaf, flax and ramie natural fibres. Int. J. Innov. Manag. Technol. 2.
Sengupta, S., and Basu, G. (2016). Properties of coconut fiber. Ref. Modul. Mater. Sci. Mater. Eng. doi: 10.1016/B978-0-12-803581-8.04122-9
Senthamaraikannan, P., and Kathiresan, M. (2018). Characterization of raw and alkali treated new natural cellulosic fiber from Coccinia grandis .L. Carbohydr. Polym. 186, 332–343. doi: 10.1016/j.carbpol.2018.01.072
Senthamaraikannan, P., Saravanakumar, S. S., Arthanarieswaran, V. P., and Sugumaran, P. (2016). Physico-chemical properties of new cellulosic fibers from the bark of Acacia planifrons. Int. J. Polym. Anal. Charact. 21, 207–213. doi: 10.1080/1023666X.2016.1133138
Senthilkumar, K., Saba, N., Rajini, N., Chandrasekar, M., Jawaid, M., Siengchin, S., et al. (2018). Mechanical properties evaluation of sisal fibre reinforced polymer composites: a review. Constr. Build. Mater. 174, 713–729. doi: 10.1016/j.conbuildmat.2018.04.143
Senthilkumar, K., Saba, N. R. N., Jawaid, M. C. M., and Siengchin, S. (2019). Effect of alkali treatment on mechanical and morphological properties of pineapple leaf fibre/polyester composites. J. Polym. Environ. 27, 1191–1201. doi: 10.1007/s10924-019-01418-x
Sepe, R., Bollino, F., Boccarusso, L., and Caputo, F. (2018). Influence of chemical treatments on mechanical properties of hemp fiber reinforced composites. Compos. Part B Eng. 133, 210–217. doi: 10.1016/j.compositesb.2017.09.030
Sgriccia, N., Hawley, M. C., and Misra, M. (2008). Characterization of natural fiber surfaces and natural fiber composites. Compos. Part A Appl. Sci. Manuf. 39, 1632–1637. doi: 10.1016/j.compositesa.2008.07.007
Shahinur, S., and Hasan, M. (2019a). “Jute/coir/banana fiber reinforced biocomposites: critical review of design, fabrication, properties and applications,” in Reference Module in Materials Science and Materials Engineering (Elsevier Ltd.). doi: 10.1016/B978-0-12-803581-8.10987-7
Shahinur, S., and Hasan, M. (2019b). Natural fiber and synthetic fiber composites: comparison of properties, performance, cost and environmental benefits,” in Reference Module in Materials Science and Materials Engineering (Elsevier Ltd.). doi: 10.1016/B978-0-12-803581-8.10994-4
Sharma, P., Pandey, A. K., and Srivastava, A. (2017). “Tensile and impact strength analysis of cotton fiber reinforced polymer composites,” in Arimpie. Available online at: https://www.elkjournals.com/microadmin/UploadFolder/3781paper%2042.pdf .
Shera, S. S., Kulhar, N., and Banik, R. M. (2019). “Silk and silk fibroin-based biopolymeric composites and their biomedical applications,” in Materials for Biomedical Engineering , eds V. Grumezescu and A. Mihai Grumezescu (Elsvier), 339–374. doi: 10.1016/B978-0-12-816872-1.00012-1
Shinoj, S., Visvanathan, R., Panigrahi, S., and Kochubabu, M. (2011). Oil palm fiber (OPF) and its composites: a review. Ind. Crops Prod. 33, 7–22. doi: 10.1016/j.indcrop.2010.09.009
Shuit, S. H., Tan, K. T., Lee, K. T., and Kamaruddin, A. H. (2009). Oil palm biomass as a sustainable energy source: a Malaysian case study. Energy 34, 1225–1235. doi: 10.1016/j.energy.2009.05.008
Singh, B., Gupta, M., and Verma, A. (1996). Influence of fiber surface treatment on the properties of sisal-polyester composites. Poly. Compos. 17, 910–918. doi: 10.1002/pc.10684
Sood, M., and Dwivedi, G. (2018). Effect of fiber treatment on flexural properties of natural fiber reinforced composites: a review. Egypt. J. Pet. 27, 775–783. doi: 10.1016/j.ejpe.2017.11.005
Sreekala, M. S., Kumaran, M. G., Joseph, S., Jacob, M., and Thomas, S. (2000). Oil palm fibre reinforced phenol formaldehyde composites: influence of fibre surface modifications on the mechanical performance. Appl. Compos. Mater. 7, 295–329. doi: 10.1023/A:1026534006291
Sreekala, M. S., Kumaran, M. G., and Thomas, S. (2002). Water sorption in oil palm fiber reinforced phenol formaldehyde composites. Compos. Part A Appl. Sci. Manuf. 33, 763–777. doi: 10.1016/S1359-835X(02)00032-5
Sreekala, M. S., and Thomas, S. (2003). Effect of fibre surface modification on water-sorption characteristics of oil palm fibres. Compos. Sci. Technol. 63, 861–869. doi: 10.1016/S0266-3538(02)00270-1
Sreenivasan, V. S., Ravindran, D., Manikandan, V., and Narayanasamy, R. (2012). Influence of fibre treatments on mechanical properties of short Sansevieria cylindrica/polyester composites. Mater. Des. 37, 111–121. doi: 10.1016/j.matdes.2012.01.004
Steffens, F., Steffens, H., and Oliveira, F. R. (2017). Applications of natural fibers on architecture. Proc. Eng. 200, 317–324. doi: 10.1016/j.proeng.2017.07.045
Suharty, N. S., Ismail, H., Diharjo, K., Handayani, D. S., and Firdaus, M. (2016). Effect of kenaf fiber as a reinforcement on the tensile, flexural strength and impact toughness properties of recycled polypropylene/halloysite composites. Proc. Chem. 19, 253–258. doi: 10.1016/j.proche.2016.03.102
Thakur, V. K., and Thakur, M. K. (2014). Processing and characterization of natural cellulose fibers/thermoset polymer composites. Carbohydr. Polym. 109, 102–117. doi: 10.1016/j.carbpol.2014.03.039
Thakur, V. K., Thakur, M. K., and Gupta, R. K. (2014). Review: raw natural fiber-based polymer composites. Int. J. Polym. Anal. Charact. 19, 256–271. doi: 10.1080/1023666X.2014.880016
Thiagamani, S. M. K., Krishnasamy, S., and Siengchin, S. (2019). Challenges of biodegradable polymers: an environmental perspective. Appl. Sci. Eng. Prog. 12, 2019. doi: 10.14416/j.asep.2019.03.002
Threepopnatkul, P., Kaerkitcha, N., and Athipongarporn, N. (2009). Composites: Part B Effect of surface treatment on performance of pineapple leaf fiber – polycarbonate composites. Compos. Part B 40, 628–632. doi: 10.1016/j.compositesb.2009.04.008
Ticoalu, A., Aravinthan, T., and Cardona, F. (2010). “A review of current development in natural fiber composites for structural and infrastructure applications,” in Southern Region Engineering Conference 11–12 November 2010, Toowoomba, Australia , 1–5.
Todkar, S. S., and Patil, S. A. (2019). Review on mechanical properties evaluation of pineapple leaf fibre (PALF) reinforced polymer composites. Compos. Part B Eng. 2019:106927. doi: 10.1016/j.compositesb.2019.106927
Tye, Y. Y., Lee, K. T., Wan Abdullah, W. N., and Leh, C. P. (2012). Potential of Ceiba pentandra (L.) Gaertn. (kapok fiber) as a resource for second generation bioethanol: effect of various simple pretreatment methods on sugar production. Bioresour. Technol . 116, 536–539. doi: 10.1016/j.biortech.2012.04.025
Väisänen, T., Batello, P., Lappalainen, R., and Tomppo, L. (2018). Modification of hemp fibers ( Cannabis sativa L.) for composite applications. Ind. Crops Prod. 111, 422–429. doi: 10.1016/j.indcrop.2017.10.049
Van Dam, J. E. G., Elbersen, H. W., and Daza Montaño, C. M. (2018). “Bamboo production for industrial utilization,” in Perennial Grassesfor Bioenergy and Bioproducts , ed E. Alexopoulou (Academic Press; Elsvier), 175–216. doi: 10.1016/B978-0-12-812900-5.00006-0
Van de Weyenberg, I., Ivens, J., De Coster, A., Kino, B., Baetens, E., and Verpoest, I. (2003). Influence of processing and chemical treatment of flax fibres on their composites. Compos. Sci. Technol. 63, 1241–1246. doi: 10.1016/S0266-3538(03)00093-9
Varghese, A. M., and Mittal, V. (2017). “Surface modification of natural fibers,” in Biodegradable and Biocompatible Polymer Composites , ed N. Gopal Shimpi (Woodhead Publishing), 115–155. doi: 10.1016/B978-0-08-100970-3.00005-5
Verma, D., and Gope, P. C. (2014). “The use of coir/coconut fibers as reinforcements in composites,” in Biofiber Reinforcements in Composite Materials , eds O. Faruk and M. Sain (Elsevier Ltd.), 285–319. doi: 10.1533/9781782421276.3.285
Wales, N., and Blackman, B. K. (2017). Plant domestication: wild date palms illuminate a crop's sticky origins. Curr. Biol. 27, R702–R704. doi: 10.1016/j.cub.2017.05.070
Wambua, P., Ivens, J., and Verpoest, I. (2003). Natural fibres: can they replace glass in fibre reinforced plastics? Compos. Sci. Technol. 63, 1259–1264. doi: 10.1016/S0266-3538(03)00096-4
Wang, G., and Chen, F. (2016). “Development of bamboo fiber-based composites,” in Advanced High Strength Natural Fibre Composites in Construction , ed M. Fan and F. Fu (Elsevier Ltd.), 235–255. doi: 10.1016/B978-0-08-100411-1.00010-8
Wang, H., Memon, H., Hassan, E. A. M., Miah, S., and Ali, A. (2019). Effect of jute fiber modification on mechanical properties of jute fiber composite. Materials (Basel) 12:E1226. doi: 10.3390/ma12081226
Xie, Y., Hill, C. A. S., Xiao, Z., Militz, H., and Mai, C. (2010). Silane coupling agents used for natural fiber/polymer composites: a review. Compos. Part A Appl. Sci. Manuf. 41, 806–819. doi: 10.1016/j.compositesa.2010.03.005
Xin, X., Xu, C. G., and Qing, L. F. (2007). Friction properties of sisal fibre reinforced resin brake composites. Wear 262, 736–741. doi: 10.1016/j.wear.2006.08.010
Xu, Y., Salmi, J., Kloser, E., Perrin, F., Grosse, S., Denault, J., et al. (2013). Feasibility of nanocrystalline cellulose production by endoglucanase treatment of natural bast fibers. Ind. Crops Prod. 51, 381–384. doi: 10.1016/j.indcrop.2013.09.029
Yang, G., Park, M., and Park, S.-J. (2019). Recent progresses of fabrication and characterization of fibers-reinforced composites: a review. Compos. Commun. 14, 34–42. doi: 10.1016/j.coco.2019.05.004
Yashas Gowda, T. G., Sanjay, M. R., Subrahmanya Bhat, K., Madhu, P., Senthamaraikannan, P., and Yogesha, B. (2018). Polymer matrix-natural fiber composites: an overview. Cogent Eng. 5, 1–13. doi: 10.1080/23311916.2018.1446667
Yogesha, B. (2017). ScienceDirect studies on natural / glass fiber reinforced polymer hybrid composites : an evolution. Mater. Today Proc. 4, 2739–2747. doi: 10.1016/j.matpr.2017.02.151
Yu, H., Wang, X., and Petru, M. (2019). The effect of surface treatment on the creep behavior of flax fiber reinforced composites under hygrothermal aging conditions. Constr. Build. Mater 208, 220–227. doi: 10.1016/j.conbuildmat.2019.03.001
Yuan, Q., Yao, J., Chen, X., Huang, L., and Shao, Z. (2010). The preparation of high performance silk fiber/fibroin composite. Polymer (Guildf). 51, 4843–4849. doi: 10.1016/j.polymer.2010.08.042
Zafeiropoulos, N. E., and Baillie, C. A. (2007). A study of the effect of surface treatments on the tensile strength of flax fibres: Part II. Application of Weibull statistics. Compos. Part A Appl. Sci. Manuf. 38, 629–638. doi: 10.1016/j.compositesa.2006.02.005
Zakikhani, P., Zahari, R., Sultan, M. T. H., and Majid, D. L. (2014). Extraction and preparation of bamboo fibre-reinforced composites. Mater. Des. 63, 820–828. doi: 10.1016/j.matdes.2014.06.058
Zaman, H. U., Khan, M. A., Khan, R. A., Rahman, M. A., Das, L. R., and Al-Mamun, M. (2010). Role of potassium permanganate and urea on the improvement of the mechanical properties of jute polypropylene composites. Fibers Polym. 11, 455–463. doi: 10.1007/s12221-010-0455-4
Zamri, M. H., Akil, H. M., and MohdIshak, Z. A. (2016). Pultruded kenaf fibre reinforced composites: effect of different kenaf fibre yarn tex. Proc. Chem. 19, 577–585. doi: 10.1016/j.proche.2016.03.056
Zegaoui, A., Ma, R., Dayo, A. Q., Derradji, M., Wang, J., Liu, W., et al. (2018). Morphological, mechanical and thermal properties of cyanate ester/benzoxazine resin composites reinforced by silane treated natural hemp fibers. Chinese J. Chem. Eng. 26, 1219–1228. doi: 10.1016/j.cjche.2018.01.008
Zhang, M. Q., Rong, M. Z., and Lu, X. (2005). Fully biodegradable natural fiber composites from renewable resources: all-plant fiber composites. Compos. Sci. Technol. 65, 2514–2525. doi: 10.1016/j.compscitech.2005.06.018
Zheng, Y., Wang, J., Zhu, Y., and Wang, A. (2015). Research and application of kapok fiber as an absorbing material: a mini review. J. Environ. Sci. (China) 27, 21–32. doi: 10.1016/j.jes.2014.09.026
Zhu, J., Zhu, H., Njuguna, J., and Abhyankar, H. (2013). Recent development of flax fibres and their reinforced composites based on different polymeric matrices. Materials 6, 5171–5198. doi: 10.3390/ma6115171
Zou, Y., Reddy, N., and Yang, Y. (2011). Reusing polyester/cotton blend fabrics for composites. Compos. Part B Eng. 42, 763–770. doi: 10.1016/j.compositesb.2011.01.022
Keywords: natural fiber, sustainable and renewable resource, eco-friendly composites, applications of natural fibers, reinforcement for composites materials, treatments on natural fibers
Citation: Thyavihalli Girijappa YG, Mavinkere Rangappa S, Parameswaranpillai J and Siengchin S (2019) Natural Fibers as Sustainable and Renewable Resource for Development of Eco-Friendly Composites: A Comprehensive Review. Front. Mater. 6:226. doi: 10.3389/fmats.2019.00226
Received: 26 June 2019; Accepted: 03 September 2019; Published: 27 September 2019.
Reviewed by:
Copyright © 2019 Thyavihalli Girijappa, Mavinkere Rangappa, Parameswaranpillai and Siengchin. This is an open-access article distributed under the terms of the Creative Commons Attribution License (CC BY) . The use, distribution or reproduction in other forums is permitted, provided the original author(s) and the copyright owner(s) are credited and that the original publication in this journal is cited, in accordance with accepted academic practice. No use, distribution or reproduction is permitted which does not comply with these terms.
*Correspondence: Suchart Siengchin, suchart.s.pe@tggs-bangkok.org
This article is part of the Research Topic
Biodegradable Matrices and Composites

An official website of the United States government
The .gov means it’s official. Federal government websites often end in .gov or .mil. Before sharing sensitive information, make sure you’re on a federal government site.
The site is secure. The https:// ensures that you are connecting to the official website and that any information you provide is encrypted and transmitted securely.
- Publications
- Account settings
Preview improvements coming to the PMC website in October 2024. Learn More or Try it out now .
- Advanced Search
- Journal List
- Materials (Basel)

Advances in Natural Fibers and Polymers
The use of natural fibers as reinforcement for polymer-based composites has been attracting the interest of the scientific community for a long time. Despite the maturity of natural fiber-reinforced polymers, such materials continue to arouse the interest of researchers.
On the one hand, the environmental concern of society has increased consistently during recent decades. This has caused growing attention to the impact of consumerism on the environment and the birth of laws and regulations promoted by national governments and international organizations. A clear example is the 2030 Agenda for Sustainable Development, promoted by the United Nations and signed by more than 190 countries. In this agenda, there are specific goals devoted to plastic recycling (goal 12) and to avoiding the use of plastic bags to keep the oceans clean (goal 14).
On the other hand, the use of natural fibers instead of mineral fibers has some advantages. Natural fibers are less abrasive and thus avoid the deterioration of equipment. Natural fibers are less dense than mineral fibers and their composites are lighter, providing better specific properties. This is of interest for automotive and aerospace industries, always seeking to reduce the weight of their vehicles. Additionally, natural fibers are safe to manipulate and, unlike mineral reinforcements, like glass fibers, are not harmful to human beings.
Lignocellulosic fibers can be obtained from wood, annual plants, as waste from agroforestry, or as a byproduct of some industrial processes, like textiles or papermaking. The possibility of using agroforestry waste as reinforcement for polymer-based composites can increase its value chain, creating richness by adding value to waste that is usually incinerated in the field. The use of byproducts can prevent disposal in landfills and the associated contamination. Other sources for natural fibers are biological, like air or feathers, which can be mixed with a matrix to obtain composite materials.
Nonetheless, there are some questions worth researching, to normalize the use of natural fibers instead of mineral ones. The use of hydrophilic natural fibers with hydrophobic matrices implies obtaining weak interphases that harm the potential mechanical properties of the composites. This is a very active field of research, which has achieved multiple solutions but must continue to be active, to obtain interphases that ensure a good combination between the tensile and impact properties of the materials. Moreover, natural fibers show a wider variation in their intrinsic properties than human-made reinforcements, decreasing the certainty of the composite material properties, which can vary from one batch to the other.
Another interesting field of research is the use of bio-based polymer as a matrix for natural fiber-reinforced composites. These matrices are oil independent and come from renewable resources. To date, its main drawback is that its cost is higher than that of oil-derived plastics, but bio-based polymers like poly(lactic acid) have a noticeably increased presence in the market. Bio-based plastics must show competitive properties in comparison to oil-derived polymers to be of interest to the industry. The use of natural fibers can support their use, by increasing their properties, decreasing the cost, and decreasing the environmental impact of the materials.
This Special Issue includes studies devoted to the study of the properties, uses, and impacts of natural fiber-reinforced composites.
The scientific topics addressed in this issue are summarized as follows:
- Annual plants
- Recycled strands
- Natural fiber composites
- Life cycle assessment
- Mechanical properties
- Micromechanics
- Biodegradable matrices
- Bio-based polymers
In brief, the scientific papers published in this thematic issue on advances in natural fibers and polymers are devoted to the following aspects.
In their study “Conductive Regenerated Cellulose Fibers for Multi-Functional Composites: Mechanical and Structural Investigation” [ 1 ], Zainab Al-Maqdasi et al. investigated the mechanical properties of regenerated cellulose fibers coated with copper via an electroless plating process. They also researched the molecular structure changes and suitability for use in sensing applications of the materials. The results indicated that regenerated cellulose fibers coated with copper show potential when combined with conventional composites of glass or carbon fibers as structure monitoring devices without significantly affecting their mechanical performance.
Xiaoxiao Zhang et al. devoted their paper “Reinforcing Mechanisms of Coir Fibers in Light-Weight Aggregate Concrete” [ 2 ] to the use of natural fibers from agricultural wastes, such as coir fibers as an alternative reinforcement in concrete composites. The goal of the research was to investigate the effects of coir fibers on the hydration reaction, microstructure, shrinkages, and mechanical properties of cement-based lightweight aggregate concrete (LWAC), concluding that treatments with coir fibers promote the hydration reaction of cement due to the accelerating effect of the different treating agents.
Riko Šafarič et al. published a paper titled “Preparation and Characterisation of Waste Poultry Feathers Composite Fibreboards” [ 3 ]. The growth of poultry meat production increases feather waste quantities every year. The researchers produced composite fiberboards with different percentages of wood and feathers, testing their mechanical properties. The obtained materials showed notable soundproofing properties and cost advantages.
Philipp Sauerbier et al. published a paper titled “Surface Activation of Polylactic Acid-Based Wood-Plastic Composite by Atmospheric Pressure Plasma Treatment” [ 4 ]. They produced composites based on polylactic acid (PLA) and investigated the influence of a dielectric barrier discharge (DBD) plasma treatment on the composites. The research revealed that after the treatment, there was a surface roughening, and contact angles decreased noticeably.
Ling Pan et al. wrote a paper titled “Preparation and Properties of Microcrystalline Cellulose/Fish Gelatin Composite Film” [ 5 ]. They used fish gelatin to prepare microcrystalline cellulose-based films. The researchers used a cross-linking agent to increase the interactions between microcrystalline cellulose and fish gelatin. The obtained results showed that the developed films were suitable for use in the biomedical field.
Dragan Kusić et al., in their research, titled “Thermal and Mechanical Characterization of Banana Fiber Reinforced Composites for Its Application in Injection Molding” [ 6 ], characterized composite materials based on high-impact polystyrene (HIPS), acrylonitrile butadiene styrene (ABS), and high-density polyethylene (HDPE) matrices, reinforced with short fibers of plantain. The results showed increases in the tensile and flexural properties when the natural fibers were added to the composites and the suitability of such waste fibers as polymer reinforcement.
Jerzy Korol et al. published “Comparative Analysis of Carbon, Ecological, and Water Footprints of Polypropylene-Based Composites Filled with Cotton, Jute and Kenaf Fibers” [ 7 ]. They studied the carbon, ecological, and water footprint assessment of polypropylene-based composites filled with cotton, jute, and kenaf fibers. The paper helped in understanding all the issues affecting the environmental impact of composite materials and avoiding unsubstantiated or preconceived conclusions.
Stefan Cichosz and Anna Masek contributed two papers to the Special Issue: “Superiority of Cellulose Non-Solvent Chemical Modification over Solvent-Involving Treatment: Solution for Green Chemistry (Part I)” [ 8 ], and “Superiority of Cellulose Non-Solvent Chemical Modification over Solvent-Involving Treatment: Application in Polymer Composite (part II)” [ 9 ]. In Part I, the researchers modified cellulose with a process that did not incorporate any solvent. The presented process fulfills green chemistry requirements and avoids using toxic solvents. Part II used cellulose fibers obtained by solvent and non-solvent processes as reinforcement. The analysis of the results showed the advantages of reinforcements obtained without solvents, in terms of mechanical properties and environmental impact.
Marc Delgado-Aguilar et al. published “Polylactic Acid/Polycaprolactone Blends: On the Path to Circular Economy, Substituting Single-Use Commodity Plastic Products” [ 10 ]. The authors studied the possibility of substituting oil-based polymers with bio-based plastics. The authors studied the micromechanics of the interphase between the matrix and the reinforcements and the influence of the percentage of fibers on the mechanical properties of the composites. The paper shows the suitability of bio-based plastic composites compared to oil-based composite commodities.
David Hernández Díaz et al. published “Impact Properties and Water Uptake Behavior of Old Newspaper Recycled Fibers-Reinforced Polypropylene Composites” [ 11 ]. The authors used fibers from recycled newspapers and researched the impact and water uptake properties of the composites. The composites showed impact strength and water uptake behaviors that compared positively with materials reinforced with raw lignocellulosic fibers.
In another paper, David Hernández Díaz et al. presented a graphic approach to the mechanical properties of composite materials. The article “Topography of the Interfacial Shear Strength and the Mean Intrinsic Tensile Strength of Hemp Fibers as a Reinforcement of Polypropylene” [ 12 ] features topography terrain display techniques to study the evolution of the mechanical properties of the composites against two factors. This graphical approach identified areas sensitive to the variation of one of the factors, especially the presence of coupling agents.
Elsadig Mahdi and Aamir Dean published “The Effect of Filler Content on the Tensile Behavior of Polypropylene/Cotton Fiber and poly(vinyl chloride)/Cotton Fiber Composites” [ 13 ]. The paper shows the impact of filler content on the mechanical properties of cotton fiber on PP- and PVC-based composites under quasi-static loading. The results showed the impact of the filler on the ability of the composites to absorb energy and the failure mechanism of the materials.
Mario D. Monzon et al. published “Experimental Analysis and Simulation of Novel Technical Textile Reinforced Composite of Banana Fibre” [ 14 ]. The authors characterized a technical textile made from enzyme-treated banana fibers. The paper presents a methodology to preview the behavior of the textiles when applied to real parts, with a 9% maximum error.
Maria Cristina Righetti et al. presented the paper “Thermal, Mechanical, Viscoelastic and Morphological Properties of Poly(lactic acid) based Biocomposites with Potato Pulp Powder Treated with Waxes” [ 15 ]. The treatment of potato pulp particles with oil-based waxes improved the strength of the interphase with the PLA matrix. This methodology expands the possible use of biocomposites, reducing the cost of the products and favoring a circular economy.
The contributions of the authors to this Special Issue on advances in natural fibers and polymers can be valuable for researchers, engineers, architects, designers, and other practitioners involved in the design and use of environmentally friendly materials. The papers show the interest in the natural fiber-reinforced polymer field of research and the opportunities for further research. There is much to research to show the environmental impact of natural fiber-reinforced composites compared commodity materials, and the development of materials able to be used for structural purposes.
I would like to express my gratitude to all the authors that have contributed to the Special Issue and all the reviewers involved in the process for their generous work.
I must thank the Materials Editorial Board for allowing me to edit this Special Issue, and for their support and patience.
Acknowledgments
As the Guest Editor, I would like to thank all the authors who submitted papers to this Special Issue. All the papers published were peer reviewed by experts in the field whose comments helped to improve the quality of the issue. I would also like to thank the Editorial Board of Materials for their assistance in managing this Special Issue. I especially want to acknowledge Emma Fang’s assistance and help during the editing process of the Special Issue.
This research received no external funding.
Conflicts of Interest
The author declares no conflict of interest.
Publisher’s Note: MDPI stays neutral with regard to jurisdictional claims in published maps and institutional affiliations.
Academia.edu no longer supports Internet Explorer.
To browse Academia.edu and the wider internet faster and more securely, please take a few seconds to upgrade your browser .
Enter the email address you signed up with and we'll email you a reset link.
- We're Hiring!
- Help Center
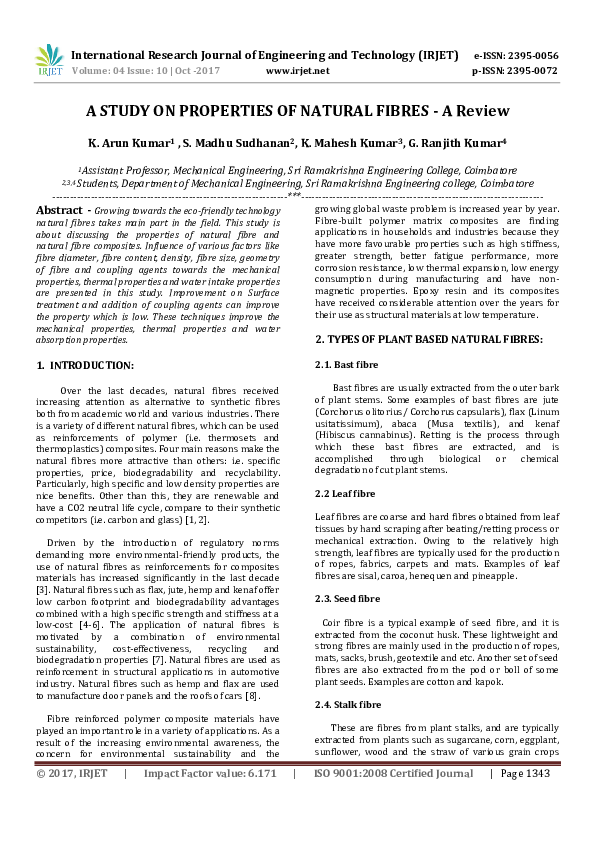
A STUDY ON PROPERTIES OF NATURAL FIBRES -A Review

https://irjet.net/archives/V4/i10/IRJET-V4I10244.pdf
Related Papers
International Journal for Research in Applied Science & Engineering Technology (IJRASET)
IJRASET Publication
Fibre is a long, thin strand or thread of material made by weaving or knitting threads together. Fibre is a hair like strand of material. A fibre is the smallest visible unit of any textile product. Fibres are flexible and may be spun into yarn and made into fabric. Natural fibres are taken from animals, vegetables or mineral sources. A few examples of widely used natural fibres include animal fibre such as wool and silk vegetables fibres, especially cotton and flax and asbestos, a mineral. Natural fibers are more important part in our human environment. Natural fibers are ecofriendly and inexpensive which are readily available in nature. In this chapter we discuss about the overview of natural fiber and their characteristic. this paper also deals with the impact of natural fibers over the synthetic fibers and also the application of natural fiber in various fields.
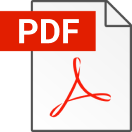
Conference Papers in Materials Science
Nesma Aboulkhair , Mike Clifford , Nicola Everitt
ARYAMAN SINGH
Various analysis has been completed every where the world for creating completely different reasonably natural fibres As a strengthening substance for the preparation of the varied varieties of composites. The material is typically composed of two elements, i.e. matrix and filler chemical therapies in the manner of fibre will clean Stop the absorption of wetness by the fiber surface, with chemicals Modify and raise the surface of the mechanical properties. These studies square measure targeted on developing composition and characterization of mechanical, rheological and structural analysis of obtained composites with completely different plant fillers. Variation of the natural part superimposed in fibre material and analyse its mechanical behaviour to get best style of fibre. These applications square measure principally their strong ratio-to-weight strength magnitude relation, high durability High temperatures, elevated creep resistance and high intensity. Testing of mechanical pro...
Jornual of AL-Farabi for Engineering Sciences
Noor Sabaah
Natural fibers are playing a major role in so many applications such as biomedical applications, aerospace Industry, structural applications, and automotive. This review aims to provide an overview of technological process (chemical treatment), availability, and the most prominent applications of natural fibers that made them preferable to be employed in these applications.
This paper is based on a study of natural fiber, its properties and its application in Today's world Natural fiber composite is the vastest field of research for engineers, professionals and scientists due to its countless properties like biodegradability, less-cost material, low weight, biodegradable, good mechanical properties, ease of availability and microstructural properties. A literature survey has been done on natural fibers (jute, sisal, kenaf, cotton, cotton straw, coir, abaca banana, hemp, neem, etc) and their utilization. This paper represents a review of various natural fibers (Sisal, Abaca and Hemp), their mechanical properties and their application.
Morphological and physicomechanical characterization of synthetic and natural fibers
Aleksey Blaznov , Zakhar S A K O S H E V Germanovich
Green and renewable materials are becoming promising worldwide. Here, we compared morphological and mechanical strength characteristics of natural plant-based bast fibers (flax, hemp and nettle) with those of synthesized fibers (glass, basalt, carbon, polyacrylonitrile (PAN), polycaproamide (PCA) and viscose). The industrial bast fibers from hemp and nettle were extracted by chemical treatment with a sodium carbonate solution. The natural fibers were comparable in size to the synthetic ones. The PCA fibers had the largest diameter of 23–28 µm. The carbon monofiber had the lowest diameter of 7–8 µm. The dimension of the natural elementary fibers was 10–25 µm. The natural fibers had a better interfacial bonding to an epoxy matrix than PCA. Moreover, the specific strength of the unimpregnated and epoxy-impregnated fibers was determined. The natural fibers were superior in strength performance to some of synthetic fibers (viscose), while the specific strength of the impregnated flax fiber was commensurate with that of the impregnated PAN and PCA fibers. The specific strength of the flax and hemp fibers once impregnated with the matrix increased four- and twofold, respectively. The impregnated flax fibers exhibited the best mechanical strength behavior among the hemp and nettle bast fibers. The natural fibers are biodegradable, have a low density, and are more eco-benign than the mineral fibers. The selected natural fibers can be used to fabricate composites therefrom.
Sandeep Bhardwaj
ankit manral
Natural fibers are being used as reinforcement in poly mer based composites to fabricate various high-end application components. Light weight, high strength to weight ratio and bio-degradability are key features of natural fibers. Mechanical propert ies, as well as thermal properties of the components developed using polymer co mposites depend on various microconstituent present in natural fiber. Every constituent present in natural fiber has individual characteristic fo r influencing the properties of the natural fiber reinforced co mposite. The present paper explores the effects of the different constituent present in natural fibers on thermal and mechanical properties of the fibers.
IOP Conference Series: Materials Science and Engineering
Timex Mascaranta
RELATED PAPERS
BMC Medical Informatics and Decision Making
Clinical Anatomy
KWANG WOO NAM
Jakariya sarkar
Nusantara Science and Technology Proceedings
Rachmi Saskia Husnika Putri
Journal of Bacteriology & Parasitology
DR Sheelendra M Bhatt
Hystrix-italian Journal of Mammalogy
Gaetano Aloise
Molecular Therapy - Oncolytics
Esther Wang
原版复刻阿卡迪亚大学毕业证 uvic毕业证文凭学位证书毕业证认证原版一模一样
DOAJ (DOAJ: Directory of Open Access Journals)
Legislación y Política de Ciencia y Género
Carolina Olvera
Acta Physica Hungarica A) Heavy Ion Physics
Maria Moura
Nanomaterials
Raymond Thomas
Engineering Fracture Mechanics
franck lauro
JURNAL SILOGISME : Kajian Ilmu Matematika dan Pembelajarannya
attin warmi
Revista Árvore
angelo santos
Thermochimica Acta
Aerotecnica Missili & Spazio
Renato Volpe
Journal of Heart and Lung Transplantation
Suresh Keshavamurthy
Journal of the International Child Neurology Association
Richard Idro
RELATED TOPICS
- We're Hiring!
- Help Center
- Find new research papers in:
- Health Sciences
- Earth Sciences
- Cognitive Science
- Mathematics
- Computer Science
- Academia ©2024
- Fibre to Fabric
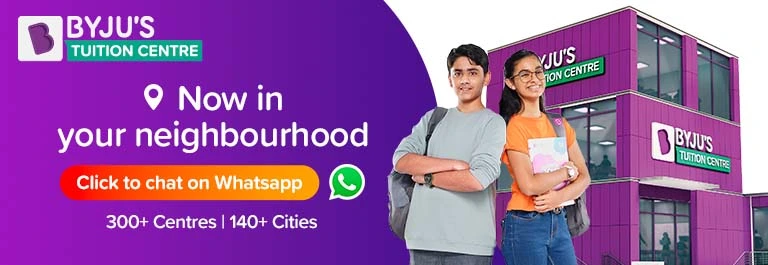
Synthetic Fibres And Natural Fibres
What are fibres.
Fibres are thread-like structures that are long, thin and flexible. These may be spun into yarns and then made into fabrics. There can be different types of fibres. On the basis of their origin, fibres are classified as natural fibres and synthetic fibres . Synthetic fibres can be produced in laboratory and can be cheaper compared to natural fibres but natural fibres are much more comfortable.
Table of Content
- Recommended videos on Fibres
- Natural fibres
- Examples of Natural fibres
- Synthetic fibres
- Advantages of Synthetic fibres
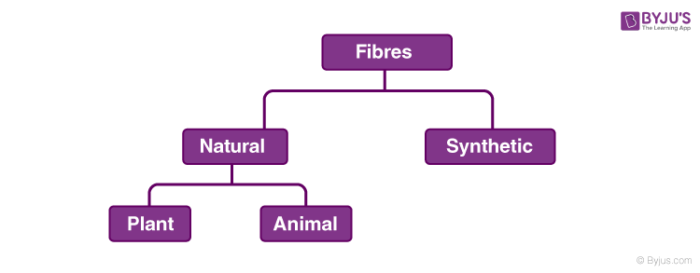
Recommended Videos on Fibres
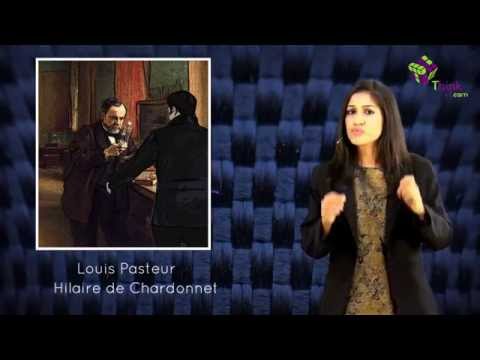
Natural Fibres
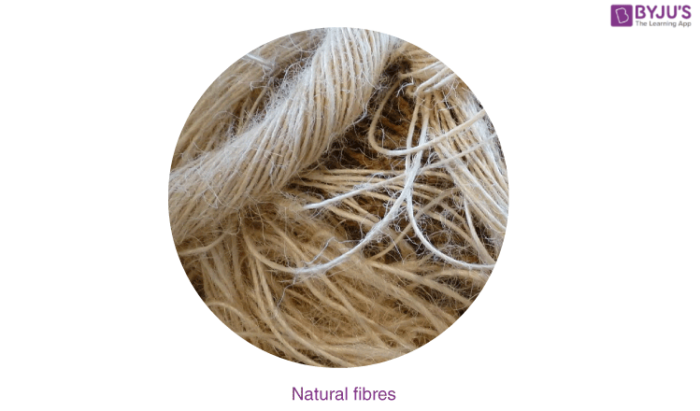
Natural fibres are the fibres that are obtained from plants, animals or mineral sources. Some examples are cotton, silk, wool etc. Natural fibres can again be divided into two types based on their source i.e. plants and animals.
Examples of Natural Fibres
1. Animal fibres: These are the fibres that are obtained from animals. For example Wool, silk etc.
- Wool: Wool is a natural textile fibre obtained from sheep, goats and camels. It traps a lot of air. Air is a bad conductor of heat. This makes clothes made from wool useful in winter.
- Silk: Silk is also a natural textile fibre which is obtained from silkworms. The rearing of silkworms to obtain silk is known as sericulture. Silk is mainly used for manufacturing clothes. Woven silk fibres are used for the construction of parachutes and bicycle tires
2. Plant fibres: These are the ones that are obtained from plants. These fibres are extracted from the plants to make fabrics.
- Cotton: It is one of the plant fibres that are used to make clothes. It is a soft staple fibre that is found as a balls around the seeds in a cotton plant. Cotton is used to make soft, breathable, and durable textile.
- Jute: It is a vegetable fibre that is soft, shiny and spun into coarse strong threads. Jute fibre is used for packaging a wide range of agricultural and industrial commodities that require bags, sacks, packs, and wrappings.
Synthetic Fibres
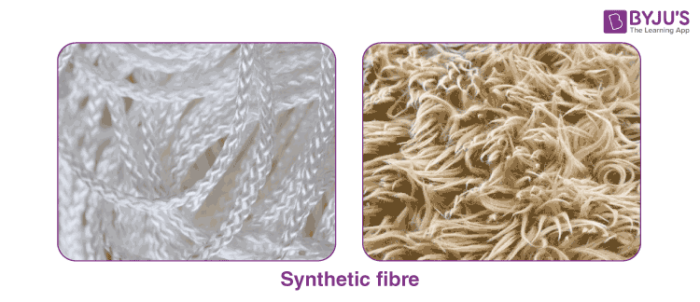
Synthetic fibres are man-made polymers designed to make a fabric. Polymers are obtained when many small units are joined together chemically.
Some of the examples of synthetic fibres are:
- Rayon: It is made from wood pulp. It is also known as artificial silk as it has characteristics resembling silk. Rayon is mainly used in clothing, carpets, medical dressings and for insulation.
- Nylon: It was the first synthetic fibre. It is used in the making of ropes, sleeping bags, parachutes, different types of clothes, etc. It is one of the strongest fibres known to us.
Advantages of Synthetic Fibres:
- They can be washed and dried quickly.
- They are easy to maintain.
- They are cheaper than natural fibres.
- Easily available.
- Do not wrinkle easily and are very durable.
Frequently Asked Questions – FAQs
What are examples of natural fibres.
Seed hairs, such as cotton, stems (or bast) fibres, such as flax and hemp, leaf fibres, such as sisal, and husk fibres, such as coconut, are all examples of plant fibres. Wool, hair, and secretions, such as silk, are examples of animal fibres.
What are natural fibres in short?
Plants, animals, and geological processes create natural fibres, often known as natural fibres. They can be utilized in composite materials where the orientation of the fibres affects the characteristics. Natural fibres can be flattened into sheets and used to produce paper or felt.
What are different natural fibres?
Seed hairs, such as cotton, stem (or bast) fibres, such as flax and hemp, leaf fibres, such as sisal, and husk fibres, such as coconut, are all examples of plant fibres. Wool, hair, and secretions, such as silk, are examples of animal fibres.
Which is man-made fibre?
Synthetic and cellulosic man-made fibres (MMF) are the two most common kinds. Cellulosic fibres are made from wood pulp while synthetic fibres are made from crude oil. Polyester, acrylic, and polypropylene are the most common synthetic staple fibres. Viscose, modal, and other cellulosic fibres are examples of cellulosic fibre.
What are the properties of fibre?
The kind of fibre influences essential qualities such as strength, durability, handling, elasticity, dyeability, lustre, friction properties, moisture absorption, heat isolation, and abrasion resistance, as well as all the physical and chemical properties of fibres and their end-products.
To know more about natural and synthetic fibres download BYJU’S – The Learning App.
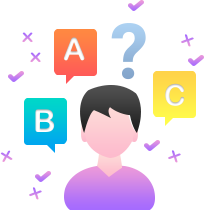
Put your understanding of this concept to test by answering a few MCQs. Click ‘Start Quiz’ to begin!
Select the correct answer and click on the “Finish” button Check your score and answers at the end of the quiz
Visit BYJU’S for all Chemistry related queries and study materials
Your result is as below
Request OTP on Voice Call
Leave a Comment Cancel reply
Your Mobile number and Email id will not be published. Required fields are marked *
Post My Comment

Register with BYJU'S & Download Free PDFs
Register with byju's & watch live videos.
- Switch skin

Advantages and Disadvantages of Natural and Manmade Fibres

A. Advantages and Disadvantages of Natural Fibre
I. advantages of natural fibre.
- Comfortable: Clothes made by natural fibres are more comfortable than those made of synthetic fibres.
- Environment: Producing materials from natural fibres are less harmful to our environment.
- Non allergic to skin
ii. Disadvantages of Natural Fibre
- Expensive: Materials produced by natural fibres are generally expensive as synthetic fibres can be made easily by manufacturing.
- Shrink: Natural fibres might shrink due to aggressive washing.
- Unlike manmade fibres, natural fibres are not available in high tenacity (HT) and medium tenacity (MT).
- Natural fibres do not possess a high degree of resiliency as compared to manmade fibres, hence the fabrics made out of them do get wrinkles from ‘baggy knees’, possess less crease recovery.
- Production of natural fibres cannot be completely controllable. Therefore, from year to year quantities of specific qualities vary and this tends to cause price fluctuations according to variations in demand which cannot be foreseen until the fibres have been produced.
- Variation in length, fineness, etc. of the natural fibre causes less regular and uniform yarn than that obtained from manmade fibres.
- The availability of natural fibres is affected by natural calamities and vagaries of nature.
- The production of natural fibres involves the use of land which is also required for growing the agriproducts. With the availability of limited amount of land on the surface of the earth, the demand for land for food and housing on one hand and that for the growth of natural fibres on the other is to be balanced.
B. Advantages and Disadvantages of Synthetic Fibre
I. advantages of synthetic fibre.
- Strong: Synthetic fibres are strong so they can take up heavy things easily.
- Retain their original shape: Synthetic fibres retain their original shape so it’s easy to wash and wear.
- Elastic: Can easily be stretched out.
- Soft: Synthetic fibres are generally soft so they are used in clothing materials.
- Colour: Varieties of colours are available as they are manufactured.
- Cost: Clothes made by synthetic fibres are generally cheaper than those made by natural fibres.
- Specific qualities of fibres can be produced deliberately and quickly in accordance with the demand.
- The filaments can be produced as fine or as coarse as required, staple lengths can be cut exactly to order. Fibres can be produced with a high degree of lustre, with reduced or completely dull lustre, as required.
- Unlike natural fibres, the final product of manmade fibres does not require cleaning.
- Most of the fibres are pure white or colourless when produced, but if necessary, colour can be incorporated during the production of the manmade fibres.
- The growth and utility of manmade fibres are mainly influenced by its positive qualities, viz. wrinkle resistance, crease recovery, easy care properties, etc. Manmade fibre fabrics bring out substantial saving on laundry costs; unlike cotton, it can be washed in a basin in the evening, hung up to dry and be worn without ironing the following morning.
- The light weight characteristic of manmade fibre fabrics gives more mobility because of less weight and quantity—a tourist can take along with, him a few clothes. Hence, these fibres are referred to as Easy-Care fibre fabrics.
- Most of the synthetic fibres possess high resistance to moth, mildew, insects, mould, which simplify the storage problems, the economy of little loss from these causes.
ii. Disadvantages of Synthetic Fibre
- Does not absorb moistures: Synthetic fibres do not absorb sweat, trapping heat in our body.
- Rough feel: Synthetic fibres may give the rough feel, making it unsuitable for pyjamas, underwear, etc.
- Some individuals are often prone to skin allergy, because of the dermatological action of manmade fibres. This puts a restriction on its use (such problems do not arise in the case of natural fibres).
- In general, the manmade fibres are generally hydrophobic in nature; this is necessarily a disadvantage when their products have to be worn next to the skin.
- These fabrics fail to absorb the perspiration ; thus the wearer feels discomfort in a hot climate.
- Manmade fibre fabrics are a little difficult to sew. Seams do not hold tight as in natural fibre fabrics. So, stitching charges were higher. But this is compensated for by durability and wash and wear properties.
Discover more from Online Textile Academy
Subscribe to get the latest posts to your email.
Type your email…
Definitions of Some Basic Terms| Textile Fibre Properties
Basics of textile fibres, related articles.

Characteristics/Requirements of Fibre Forming Polymers

Properties of Textile Fibres for Apparel/Domestic and Technical Grade Applications
Viscose rayon fibre manufacturing process: summary.
- Classification of Cotton Fibers: Botanical | Commercial | According to Length April 13, 2024
- Properties and Applications of Cotton Fibre July 15, 2017
Subscribe now to keep reading and get access to the full archive.
Continue reading
Adblock Detected
Scalable fabrication of collagen fiber-waterborne polyurethane foams with enhanced toughness and thermal insulation by constructing energy dissipation networks
- Li, Shuangyang
- Liang, Feng
- Zhou, Jianfei
Natural fiber-based foams demonstrate outstanding potential for energy-efficient building and personal protective equipment applications due to their lightweight, high porosity, and sustainability. However, the weak interfacial interaction among fibers, functional additives, and the matrix hampers effective stress dissipation, largely affecting the material performance. Herein, this paper demonstrates a facile strategy for fabricating high-performance collagen fiber-based foams by constructing efficient energy dissipation networks using tannin-modified leather collagen fibers (T-LCF) and waterborne polyurethane (WPU). Tannin acts as a coupling bridge, linking the fiber and polyurethane to create a hydrogen-bonded network, enhancing energy dissipation and imparting excellent mechanical properties. Compared to WPU foam (WPUF), the T-LCF-based foam (WPUF/T-LCF) showed 65%, 34 times, and 35% improvements in tensile strength, compressive strength, and ultimate oxygen index, respectively. This study provides new insights into the cleaner production of high-performance natural fiber-based foam composites and a new way for the high-value utilization of collagen solid waste.
- Collagen fiber;
- Waterborne polyurethane-based foams;
- Interfacial compatibility;
- Energy dissipation networks;
- Thermal properties
No Sources Found
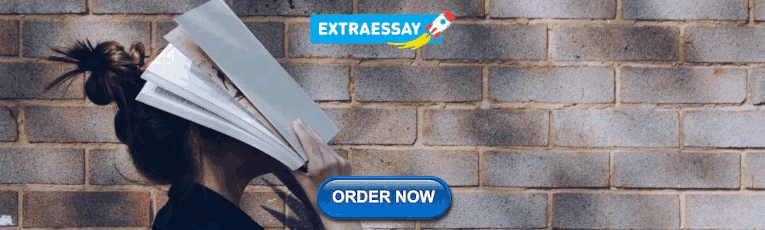
IMAGES
VIDEO
COMMENTS
1. Introduction. Scientists, researchers, and practitioners around the world have recently been working to maximize the potential of natural fibers to create the most sustainable, biodegradable, and high-quality natural fiber products [1,2,3].Natural fibers, which are renewable and ecologically acceptable sources of raw materials for producing environmentally friendly products, have played a ...
natural fibre, any hairlike raw material directly obtainable from an animal, vegetable, or mineral source and convertible into nonwoven fabrics such as felt or paper or, after spinning into yarns, into woven cloth. A natural fibre may be further defined as an agglomeration of cells in which the diameter is negligible in comparison with the length. . Although nature abounds in fibrous materials ...
Abaca fiber is commonly used in the production of ropes, twines, and mats [30, 35], and it is also used to produce floor panels in automotive manufacturing [39, 102, 103]. 2.1.11. Wood fiber. Wood is a natural fiber widely used in the manufactablturing of pulp and paper products [45]. There are two main types of wood fibers: hardwood and softwood.
In recent years, more renewable plant resources have been discovered and used because non-renewable resources are becoming scarce [].Figure 1 shows the structural organization of a natural fiber cell wall [].This type of fiber is strong, light, inexpensive, and renewable [].These inexpensive natural fibers can become a viable alternative for expensive and non-renewable synthetic fibers (e.g ...
World natural fibre production in 2018 was estimated to be 32 million metric tons. DNFI (Natural Fibres and the World Economy, July 2019), in cooperation with the Food and Agriculture Organization of the United Nations (FAO), collects statistics on 14 categories of natural fibres, ranging from abaca to wool and other animal fibres (Fig. 1.1).During the past decade, world natural fibre ...
Bamboo is w ood like tr opical grass w hile the sources f or stra w are. wheat stalks, grasses, sisal hem p and rice paper. The extensi ve utilization of natural fibers for a range of textile ...
Asian countries have abundant resources of natural fibers, but unfortunately, they have not been optimally utilized. The facts showed that from 2014 to 2020, there was a shortfall in meeting national demand of over USD 2.75 million per year. Therefore, in order to develop the utilization and improve the economic potential as well as the sustainability of natural fibers, a comprehensive review ...
Natural fibers or natural fibres (see spelling differences) are fibers that are produced by geological processes, or from the bodies of plants or animals. They can be used as a component of composite materials, where the orientation of fibers impacts the properties. Natural fibers can also be matted into sheets to make paper or felt.. The earliest evidence of humans using fibers is the ...
This comprehensive review explores the multifaceted world of natural fiber applications within the domain of composite materials. Natural fibers are meticulously examined in detail, considering their diverse origins, which encompass plant-derived fibers (cellulose-based), animal-derived fibers (protein-based), and even mineral-derived variations. This review conducts a profound analysis, not ...
In particular, composite materials are being developed and redesigned aiming to improve and to adapt traditional products and introduce new products in a sustainable and responsible way [].This paper examines and discusses the last published trends in the context of fibres-reinforced bio-composite materials while providing an insight about natural fibres for bio-composites, with the focus on ...
The increase in awareness of the damage caused by synthetic materials on the environment has led to the development of eco-friendly materials. The researchers have shown a lot of interest in developing such materials which can replace the synthetic materials. As a result, there is an increase in demand for commercial use of the natural fiber-based composites in recent years for various ...
ISSN:2581-4915. 1. A Review On Natural Fiber And Its Char acteristics. A Mani Kandan 1 and Prof. S Rajakumar 2. 1 PG Scholar, Department of Mechanical Engineering, Anna University Regional Campus ...
The papers show the interest in the natural fiber-reinforced polymer field of research and the opportunities for further research. There is much to research to show the environmental impact of natural fiber-reinforced composites compared commodity materials, and the development of materials able to be used for structural purposes. ...
Seed fibres - the fibres obtained from the seeds of different types of plants. Leaf fibres - the natural fibres that can be collected from the leaves of certain plants. Examples include pineapple and banana leaf fibres. Fruit fibres - the natural fibres that are obtained from the fruit of a plant (coconut fibre, for example).
In addition, the global market of natural fiber composites is expected to grow at an annual average rate of 9.59%, and will reach a USD 41 billion net worth by 2025 (Zwawi 2021 ). Table 1. shows the statistical analysis of the consumption of natural fiber composites in major regions of the world from 2018 to 2021, and.
Fig. 1 shows the potential application of banana pseudo-stem component which is divided into three parts, namely central core, banana fiber and waste materials after fiber extraction. While prospective utilization of pineapple leaf is presented in Fig. 2.Some utilizations of banana fibers have been reported including in papermaking [48, 49], fiber-cement composites [[50], [51], [52]], animal ...
Natural fiber is an emerging and environment friendly produc t, which is. widely applied in textile and other related sectors. The natura l fibers are. used for tex tiles application in fiber ...
Fibre is a long, thin strand or thread of material made by weaving or knitting threads together. Fibre is a hair like strand of material. A fibre is the smallest visible unit of any textile product. Fibres are flexible and may be spun into yarn and made into fabric. Natural fibres are taken from animals, vegetables or mineral sources.
In this context, the accepted contributions for this Special Issue include topics such as: New processes for natural fiber agriculture extraction and processing; Natural fiber reinforced biopolymers; Innovative natural fibers and structures; Functional natural fibers; Natural fiber modification techniques; Advanced fibrous structures based on ...
Separation and Identification of Terpenoids in Three Pineapple Fibers Using Ultra-High Performance Liquid Chromatography-Tandem Mass Spectrometry. Yijun Liu, Yuliang Chen, Jiameng Liu, Lin Zhu, Lijing Lin, Zhikai Zhuang, Jiangxiu He, Tao Li, Gang Chen & Siru Yao. Article: 2315596. Published online: 21 Feb 2024.
Synthetic and Natural Fibres - Fibres are thread-like structures that are long, thin and flexible. Fiber obtained from natural sources like plants and animals, for example, Cotton, silk, wool etc. Synthetic fibres is prepared from chemical substances in the industry. Visit BYJU'S to learn more about, Examples, Types, Advantage, Videos and FAQs of Synthetic and Natural Fibres.
Environment: Producing materials from natural fibres are less harmful to our environment. Non allergic to skin. ii. Disadvantages of Natural Fibre. Expensive: Materials produced by natural fibres are generally expensive as synthetic fibres can be made easily by manufacturing. Shrink: Natural fibres might shrink due to aggressive washing.
Natural fiber-based cement reduced the use of fossil resources and GHG compared to polyacrylonitrile fiber-based cement. The environmental impact of hemp and flax fiber concrete was approximately 10% lower than polyacrylonitrile fiber concrete. Hemp, flax, and sea grass-based concrete had a 6.6%, 6.6%, and 9.9% lower impact on ozone layer ...
Natural fiber-based foams demonstrate outstanding potential for energy-efficient building and personal protective equipment applications due to their lightweight, high porosity, and sustainability. However, the weak interfacial interaction among fibers, functional additives, and the matrix hampers effective stress dissipation, largely affecting the material performance.