Contribution of Amedeo Avogadro to Chemistry Essay (Article)
- To find inspiration for your paper and overcome writer’s block
- As a source of information (ensure proper referencing)
- As a template for you assignment
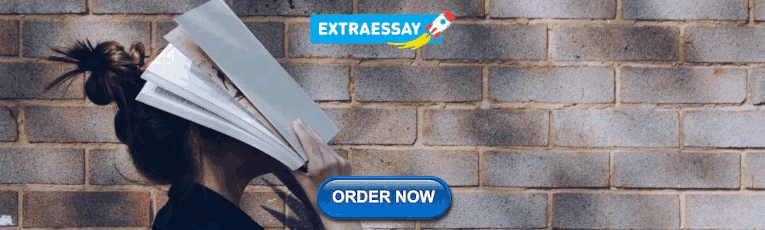
Introduction
Avogadro’s interest in natural philosophy, avogadro’s contribution to chemistry, the avogadro’s number.
Lorenzo Amedeo Avogadro was born in 1776 in Turin city of Italy and died in 1856 at the age of 81 years after making a great positive contribution in the field of science especially chemistry. He schooled in Turin and having come from a family background of lawyers, his career was oriented towards law whereby at the age of 16 years he had already become a bachelor of jurisprudence and four years down this profession he had managed to get a doctorate in ecclesiastical law which he began to practice and latter in 1801 he received an appointment in the department of Eridano as the secretary to the prefecture.
Along with his successful career in legal matters, Avogadro had a lot of interest in the field of natural philosophy which influenced him to pursue private studies in both physics and mathematics in the year 1800 whereby three years down studies he carried out scientific research on electricity together with his brother. In 1803 he was appointed to be a demonstrator at Turin academy which was followed by him becoming a professor of natural philosophy at Vercelli University in 1809.
Avogadro received another appointment to head the chair in mathematical physic when it was first established in Italy in1820 at the university of Turin an appointment which he did not hold for long because the political changes at that time suppressed the chair making him lose the job in1822 until 1832 when he was reinstated. (Mario, 2003)
In his article, journal de physique, which was published in 1811, Avogadro clearly described the distinction between an atom and a molecule which Dalton had failed to differentiate in his work in1808 and was confused between the two. Dalton assumed that in common compounds with two elements, each element contributed one atom. Dalton had considered particles to be the same as atoms and failed to admit that one oxygen particle could yield two particles of water.
It was Avogadro who made it clear that ‘’atoms’’ of hydrogen and oxygen are ”molecules” made up of two atoms each and therefore when two hydrogen molecules combine with one oxygen molecule, two water molecules are produced. Avogadro suggested that at the same conditions of pressure and temperature all gases with equal volumes contained an equal number of molecules which is the modern definition of Avogadro’s principle. This principle was the basis of determining the molar masses as well as atomic masses.
Avogadro’s number which is also known as Avogadro’s constant is the number of particles in one mole of any substance and its value is 6.0221367 x 1023. This number was not actually determined by Amedeo himself because he did not know about moles at this time or of any number that would later bear his name. It was through the work of Cannizzaro in 1860 that used the ideas of Avogadro to come up with the number and obtained sets of atomic weights which were based on oxygen with an atomic mass of 16.
Today our definition of mole determines the size of Avogadro’s number and this number demonstrates the small size of both atoms and molecules in comparison with the number of substances we are aware of in our daily life. For example, we define a mole of a substance as the amount of the substance which contains as many particles as there are carbon atoms in12 grams of the carbon-12 isotope. (Mario, 2003)
Mario M. (2003): Amedeo Avogadro: Hardcover publishers.
- Design of Rome and Milan in the 20th Century
- Crowdfunding Project to Help Homeless People
- Apple Inc.'s Logo and Name Origin
- Conceptual Chemistry. Wind Turbine vs. Coal Energy
- The Fluorescence and Ultraviolet/Visible Light
- Earth's Atmosphere and Natural Pollution
- Oxidation and Reduction Process
- Activation Energy Barrier Definition
- Chicago (A-D)
- Chicago (N-B)
IvyPanda. (2021, September 30). Contribution of Amedeo Avogadro to Chemistry. https://ivypanda.com/essays/contribution-of-amedeo-avogadro-to-chemistry/
"Contribution of Amedeo Avogadro to Chemistry." IvyPanda , 30 Sept. 2021, ivypanda.com/essays/contribution-of-amedeo-avogadro-to-chemistry/.
IvyPanda . (2021) 'Contribution of Amedeo Avogadro to Chemistry'. 30 September.
IvyPanda . 2021. "Contribution of Amedeo Avogadro to Chemistry." September 30, 2021. https://ivypanda.com/essays/contribution-of-amedeo-avogadro-to-chemistry/.
1. IvyPanda . "Contribution of Amedeo Avogadro to Chemistry." September 30, 2021. https://ivypanda.com/essays/contribution-of-amedeo-avogadro-to-chemistry/.
Bibliography
IvyPanda . "Contribution of Amedeo Avogadro to Chemistry." September 30, 2021. https://ivypanda.com/essays/contribution-of-amedeo-avogadro-to-chemistry/.
Academia.edu no longer supports Internet Explorer.
To browse Academia.edu and the wider internet faster and more securely, please take a few seconds to upgrade your browser .
Enter the email address you signed up with and we'll email you a reset link.
- We're Hiring!
- Help Center
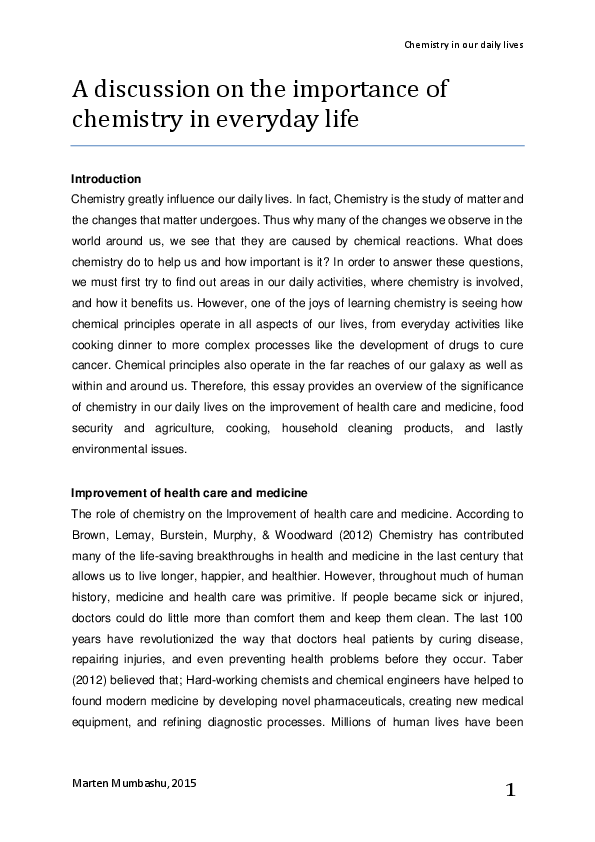
Chemistry in our daily lives A discussion on the importance of chemistry in everyday life

Related Papers
Jeremy Ramsden
The ways science and technology can contribute to health is reviewed.
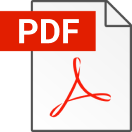
Ijcas Journal
The chemist's most impressive contribution to medicine has been his expansion of the physician's selection of drugs. Drugs and medicines are Chemicals used medically for treating diseases and injuries. Drugs carry the added connotation of narcotics and addiction. The most lucrative field in organic chemical product is that of pharmaceutical products. Vitamins hormones antibiotics, anaesthetics, antiseptics and germicides are all products of organic chemistry. Most of the rather substantial increase in human life expectancy in recent years has been attributed to the development of new medicinal agents. Medicinal chemicals are available to treat various human diseases. There are antibacterial, antiviral, antifungal, antidepressants and anticancer and anti-AIDS drugs. There are medicines to treat stroke and heart attack, ulcers, parasites, and various hormone deficiencies.
Hilal Kılıç
Technology is a huge part of our lives. Developing technology directs the lives of people and living things in every field. Thanks to the technology, medicine continues to develop everyday too. In this way, the new treatments play a major role in the recovery of people and other living creatures. With the advencement of technology and medicine, the health of people and other living things become better. The technological inventions found can be adjusted to the medical field. Technology helps to find and develop treatments. Some diseases are fatal, but patients can be saved through treatments. Diseases such as cancer is example of them. The new diagnostic and treatment methods which are found with technology, play a major role in the fight against cancer. Radiation theraphy is one of those treatments. This treatment is the use of high-energy rays, such as X-rays, for the treatment of cancer. Although, there are concerns about the dangers of radiation, successful cancer treatment with radiotheraphy and radiosurgery is achieved. Diseases such as injuries and skin diseases are also treated with areas that benefit from technology in medicine. At the beginning of the diagnosis and treatment process, patients are examined in clinical way. After the examination methods varying, according to the type of disease, samples taken from the patients are sent to the laboratories. As a result of the examination of the samples of the samples taken from the patients, data is collected in the laboratory by using "laboratory management modules" supported by artifical intelligence. After that, medical imaging techniques are used to diagnose the patients. X-ray, tomography, ultrasonography are some of them. The X-ray is a pioneer in the treatment of diseaeses. Today, the use of drugs in the treatment of many diseases is very important. Medicines or drugs are used to treat, vaccinate or relieve symptoms. Firstly, the pharmaceutical industry discovers, develops, produces and markets drugs. With proper and regular drug use, the treatment of diseases accelerated. Nanotechnology is used in many areas of drug treatment. The use of carbon nanotubes for the development of new bones and nanoparticle drugs for the treatment of cancer are examples of it. Secondly, it is possible to make more detailed analyzes about existing diseases or living anatomy by using nanotechnology in the medical field. The examination of many of the current diseases on the basis of cellular or smaller molecules is a revolution in medicine. Thirdly, nanotechnology can stop bleeding. A liquid containing synthetic nanomaterials can heal wounds in the skin. In this way, the treatment of injuries accelerated.
Vijay Kothari
Samuel Vebgyor
Clinical Chemistry
Laurence Demers
International Journal of Advanced Research
Subhas Chandra Bhat
Fernando A G Alcoforado
Article describes advancements of medicine from 18th century to the contemporary era
Kalpana Singh
Jonas S U N S H I N E Callewaert
Every factor in human well-being is also an element of nutrition. All needs are really nutritive needs. Deprivation of any single need may mean our demise or impairment of our growth, development or health. A single factor insufficiently or incorrectly supplied can lead to disease and suffering. Most people are aware of the essentials of life. But they lose sight of these funda- mentals as being factors and influences that are necessary to well-being within the con- text of society. Therefore, they’re likely to violate the very laws of their existence and contribute to their own sickness and suffering. When in a state of disease, most people do not realize they have brought it upon themselves. They are aided in placing blame outside themselves by a profession that takes the stance that they’ve had an unfortunate bit of bad luck or they have been invaded by some microbial enemy. Though the needs of the ill differ from those of well peo- ple only in that their conditions must be made favorable to recuperation, both ill people and the medical professionals undertake a course of treatment that compounds sickness. Both the physician and the sufferer enter into an attempt to poison the ailing body back into health. The fact is that drugging only makes a body worse. The causes of health are very simple. Our needs do not change substantially when we become ill. Even illness itself won’t occur if the needs of our bodies and minds are properly met. The nineteen factor elements for optimal well-being are listed as follows: 1. Pure air 2. Pure water 3. Cleanliness—both internal and external 4. Sleep 5. Temperature maintenance 6. Pure wholesome food to which we are biologically adapted 7. Exercise and activity 8. Sunshine upon our bodies 9. Rest and relaxation 10. Play and recreation 11. Emotional poise 12. Security of life and its means 13. Pleasant environment 14. Creative, useful work 15. Self-Mastery 16. Belonging 17. Motivation 18. Expression of the natural instincts 19. Indulgence of aesthetic senses. Let us explore the first two of these needs in detail.
RELATED TOPICS
- We're Hiring!
- Help Center
- Find new research papers in:
- Health Sciences
- Earth Sciences
- Cognitive Science
- Mathematics
- Computer Science
- Academia ©2024

The Importance of Chemical Research to the U.S. Economy (2022)
Chapter: 2 understanding the economic impacts of chemistry, 2 understanding the economic impacts of chemistry.
Chemical research has contributed to economic prosperity and financial security in the United States. Products from chemical companies, such as polymers, coatings, pharmaceuticals, and pesticides, play an important role in everyday life. These chemicals are all derived from products and processes that started in some way as fundamental chemical research. Of course, all products have a unique trajectory from fundamental research to final product, but this movement frequently requires many iterations, steps, and decades of complementary research discoveries. These research and development (R&D) efforts, when successful, are converted into products or processes that are used by the chemical industry to increase quality of life. The outcome can produce a novel chemical or technique, or can improve upon an entity that already exists by, for example, making a better product or decreasing the environmental impact of a process.
As the committee discussed the size and impact of chemical research on the chemical economy, it took a very broad approach to defining what is included in the chemical economy. As noted in Chapter 1 , the chemical economy includes all parts of any value chain that rely on chemical knowledge and transformation processes for advancement and growth. This broad approach is important when considering fundamental research that has impacted the chemical economy, and the breadth will be reflected in any subsequent analyses and examples of success.
To evaluate the impact of chemical research on the chemical economy, as well as the U.S. economy more broadly, the committee used several approaches. Two lines of evidence used were economic output of and employment from chemical companies and the chemical sector. The committee relied on previous assessments of the chemical economy and the impacts of chemical research on the economy, as well as an economic analysis that was produced by an independent consulting firm. To try to evaluate the size of the chemical economy, as well as the impact of R&D on the chemical economy, Vertex Evaluation and Research, LLC (Vertex) assembled a team led by Lee Fleming of the University of California, Berkeley’s Haas School of Business and Daniel Basco from Vertex, and included a group of collaborators from IP Checkups, an organization that specializes in patent analysis, to address these questions. In response to a Request for Proposal from the study committee ( Appendix B ), Vertex put together an analysis plan with three phases, which included plans to “assess the economic value of the chemical economy, assess industries where chemical research is driving employment opportunities and value creation, and assess how chemical research is contributing to sustainable economic progress” ( Fleming and Basco, 2021 ). Most of the material related to the size of the chemical economy, and importance and impact of chemical research in the chemical economy came in their first phase of work. Most of their analysis relied on chemical patents and their valuation, which served as a proxy for chemical research since there are economic methods for valuating patents. Throughout this chapter, there are discussions of various aspects of the report from Vertex, including the interesting findings and important caveats.
The use of this report and the other collected data helped to paint a picture of the impact of the chemical industry but frequently fell short of helping the committee gain an understanding of the economic impact of fundamental research. To better understand the economic impacts of chemical research, individual case studies were selected that highlight chemical discoveries that led to a product or process with widespread implications for society.
2.1 BRIEF HISTORY OF THE U.S. CHEMICAL INDUSTRY
The chemical industry in the United States has been a prominent player on the world’s stage since the early 20th century. While it is notable that the United States emerged later than many European countries, the U.S. domestic market, transportation system, and R&D infrastructure propelled it to become one of the largest chemical producers in the world. With the boost in chemical production from World War I and World War II, the United States established and maintained
a strong presence in the chemical marketplace from 1920 to 1960, sometimes referred to as the “golden age of innovation in the chemical industry” ( Arora and Gambardella, 2010 ).
While it is impossible to name all of the products and processes that were responsible for the thriving industry, it is important to note that these inventions included chemicals and materials that improved and saved lives, as well as those that wrought tremendous harm. Many of the prominent examples include chemical weapons that were researched and used during this period of invention, and include, but are not limited to, mustard gas in World War I and, later, the herbicide Agent Orange during the Vietnam War ( Everts, 2015 ). Half a century to more than a century later, we continue to struggle with the use of chemical agents in conflicts despite widespread international support limiting their production and use ( OPCW, 2020 ). A prominent example of a product that improved quality of life is the discovery of nylon by Wallace Carothers of DuPont. This discovery was a major breakthrough in polymer chemistry and led to the production of the first fully synthetic fibers. It was noted by Hounshell and Smith (1988) that the success of this innovation encouraged a research-based approach at DuPont. In addition to the production of nylon, basic research by industrial chemists led to the discovery and production of a large number of polymers such as polyester, various acrylics, neoprene, and many more. Underpinning the massive commercial success of these products was the research and innovation that led to them. This fundamental research also laid important groundwork in the chemical understanding of materials and their interactions.
The U.S. chemical industry was also particularly good at developing processes for the production of chemical goods. Scaling up chemical production, like in the case of sulfuric acid or fertilizers, was a fruitful collaboration between chemical researchers and engineers ( Arora and Gambardella, 2010 ). The growing popularity of the automobile and the resulting need for refined petroleum were partially responsible for the development of chemical engineering in America ( NASEM, 2022a ). The ability of the United States to produce large quantities of usable feedstock, especially the refining of crude oil, and to synthesize commodity chemicals was an additional boon to the economic success of the U.S. chemical industry. These industrial capabilities were also important for laying the groundwork for developing and understanding industrial chemical processes that are critical now, and will be used and refined even as commodity chemicals need to be increasingly produced from new feedstocks.
Developing successful large-scale production processes was also important to improving human health. In 1939, the first large-scale production of the antibiotic penicillin was accomplished in Brooklyn, New York ( Aldridge et al., 1999 ). While the synthesis of this life-saving molecule was done by biological fermentation, it was chemists and chemical engineers who developed new fermentation conditions that allowed for the extraction, purification, and stabilization of the final molecule. The design and commercial-scale production of this antibiotic led the way for the large-scale production of other specialty chemicals and pharmaceuticals.
The U.S. chemical industry was also responsible for technological advances that moved computation forward, including revolutionizing the semiconductor. In 1979, researchers at IBM developed chemically amplified photoresists, the result of R&D of new polymers capable of a photoactivated chemical “chain reaction.” This new chemical amplification process gave a 30-fold improvement in light sensitivity over its predecessor ( Brock, 2007 ). The increased sensitivity made it ideal for a growing electronics industry that was constantly searching to perform computational tasks at faster speeds and smaller scales. Chemically amplified resists are now integrated into most electronic devices and are important to billions of people around the world.
As the U.S. chemical industry continued to innovate throughout the late 20th century, chemical production grew and adversely affected the environment. This had a disproportionate impact on local and indigenous communities ( Borunda, 2021 ; Langston, 2010 ). With heavy influence from these communities, a growing environmental movement sought accountability for the environmental damages that the chemical industry played a prominent role in accelerating. The interplay
between environmental sustainability and the chemical sciences is covered in more detail in Chapter 3 but remains an important consideration as we look further at the history and impact of the chemical economy.
2.2 ESTIMATING CURRENT SIZE AND IMPACT OF THE CHEMICAL ECONOMY
The chemical industry has a significant direct impact on the economy through its contribution to gross domestic product (GDP), employment, and national competitiveness ( Figure 2-1 ). An expanded analysis shows that there is also a significant indirect impact, noting that chemical knowledge is used in a wide variety of areas, and chemical products are used and consumed by almost every sector of the U.S. economy. This section highlights the direct economic impacts of the chemical economy by showing the value and employment added by the chemical sector. Several methods of indirect impact are also shown, primarily focusing on measurable impacts such as the use of chemical knowledge in other economic sectors or the reliance that patents have on chemical knowledge. Another aspect of indirect impacts is considered later in the chapter where the report notes the wide social, health, environmental, and economic impacts that specific chemical products can have on the entire national population. It can be difficult to measure the widespread impacts of every chemical product in every economic sector, but this can be displayed through specific examples as shown later in this chapter (see Section 2.3.3 ). Some of these examples include the widespread use and environmental potential of batteries, the economic and societal improvements afforded by oral contraception, and the immediate helpfulness and economic revival afforded by treatments for COVID-19.
2.2.1 Measuring Direct Contribution to the U.S. Economy
There are many measures of the size of the chemical economy, both domestically and internationally, and it is important to provide a short digest of some of the most prominent resources in order to get an idea of the size and impact of the chemical industry. To accomplish this, the
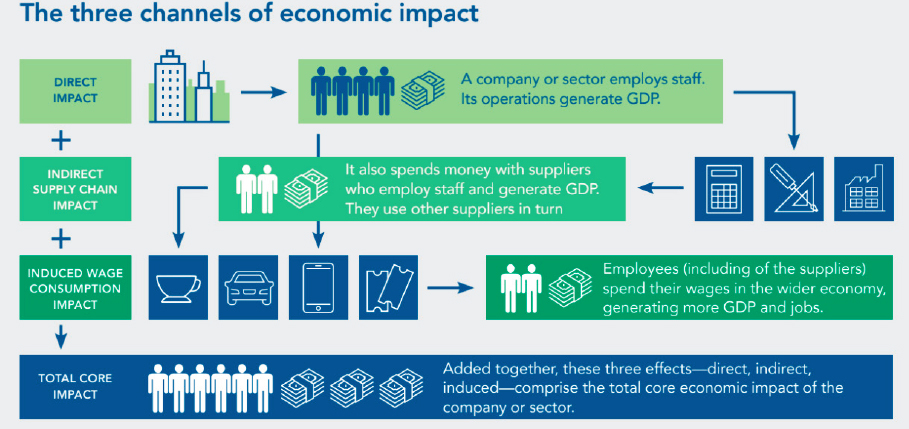
committee looked to two prominent resources: a report from the International Council of Chemical Associations (ICCA) and Oxford Economics (OE) published in 2019, and the 2021 Guide to the Business of Chemistry , produced by the American Chemistry Council ( ACC, 2021 ). Here the chapter includes ICCA/OE and ACC reports of sales, imports, exports, employment, and value added from the chemical industry. Both reports measured the size of the chemical industry and the impact that it has on the overall economy. An important caveat is that these analyses exclude pharmaceuticals and medical manufacturing, and only include equipment when specified. Based on the definition of the chemical economy established by this report, pharmaceuticals would be included, and their impacts will be addressed throughout the report.
2.2.1.1 Monetary Impact of the Chemical Economy
It is first important to look at the direct value added and sales from the chemical economy, and the chemical economy’s impact on the overall economy. The ICCA and OE (2019) report estimated that the chemical industry contributed $5.7 trillion to the global GDP in 2017, or approximately 7% of the world’s GDP that year. The ACC reports numbers that are specific to the United States and notes that, in 2020, the “final sales” of the industry were $457 billion, of which $123 billion were intraindustry sales to other chemical producers ( ACC, 2021 ). Of the remaining $334 billion, the leading buying sectors were rubber and plastic, pharmaceuticals, health care, other manufacturing, paper, and agriculture ( Figure 2-2 ). Note that these were direct sales and do not include indirect uses, for example, if car manufacturers buy from tire manufacturers.
Additionally, in 2020, the value added in the U.S. economy from the chemical industry was $225 billion. The report from ICCA and OE estimated the chemical industry’s contribution to North American GDP to be $866 billion in 2017, of which $235 billion is the direct impact of the chemical industry, a number comparable to what was reported by the ACC. Importantly, the ICCA and OE report did not assess individual contributions of different countries in North America.

The ACC report also noted that exports and imports have grown roughly threefold over the past two decades, and the United States has maintained a positive trade balance since 2016, which has been around $20 billion to $30 billion ( Figure 2-3 ). Despite this growth, the output of the chemical industry has grown more slowly in recent years, with only a 3% increase in output between 2012 and 2019. In some ways, this slow growth indicates that the chemical industry as a whole is mature. This is especially true since innovation is mostly incremental, and changes in how products are customized and delivered to the customer are growing in importance, relative to fundamental advances.
As mentioned previously, the committee defined the chemical economy to include all products and parts of a value chain that rely on chemical knowledge, meaning that pharmaceuticals, but not biopharmaceuticals (see Section 1.2.1 for further explanation), should be included in any analysis of the chemical economy. Although most analyses do not include pharmaceutical impact, some data do describe the value of the pharmaceutical industry in the United States. When considering pharmaceutical manufacturing, which falls under the North American Industry Classification System (NAICS) code 3254, the committee was careful to exclude “Biological Product Manufacturing,” which is a subsection of this sector, designated as NAICS 325414. The value of production for the pharmaceutical industry that contributed to the chemical economy, excluding biological products, was around $160 billion in 2020 ( Figure 2-4 ). The value of production has also been steadily increasing since 2000. While it is challenging to consider these numbers within the same analysis as the rest of the chemical economy, it is important to note that the value added from the pharmaceutical industry to the chemical economy in the United States is on a scale relatively similar to the value added from the rest of the chemical industry ( BLS, 2022 ).
2.2.1.2 Employment
Another important aspect of the chemical economy’s impact is through employment numbers and employment potential. The ICCA and OE (2019) report noted that the chemical industry in 2017 supported 120 million jobs related to “all aspects of the global chemical industry.” The report also notes that the North American chemical industry supported 6.1 million jobs in 2017, of which 600,000 jobs were supported directly in the chemistry industry itself. The ACC annual report documents similar employment numbers that are specific for the United States, showing that in 2020, in addition to directly employing 529,000 workers, the entire chemical enterprise supported 4.1 million individuals who were interacting with the chemical economy ( ACC, 2021 ). Employment in the industry within the United States has remained relatively steady with direct employment numbers staying between 525,000 and 550,000 since 2016.

The North American chemical industry holds the top global position in terms of GDP/employees, when compared to other global regions ( Figure 2-5 ). This is one of the reasons that the U.S. chemical industry pays its employees so well. The ACC (2021) report showed that employees are paid 23% more than those in other U.S. manufacturing jobs. Additionally, the job outlook for chemists and materials scientists is fairly positive, and employment is expected to grow approximately 6% over the next decade, which is similar to the average growth of all occupations ( BLS, 2021 ).
2.2.2 Indirect Contributions of Chemistry to the U.S. Economy
To better understand the impact of chemistry on the U.S. economy, we must also consider its indirect impacts, such as the chemical knowledge that is used in a much wider range of industries. We touched on the indirect impacts in relation to economic output and employment that are supported by, but not directly within, the chemical economy. Some of the industries that are dependent on the spillover of knowledge, products, and processes from the chemical economy include strategically important industries such as semiconductors, computers, aerospace, medical equipment, and electrical equipment, in addition to other economically significant industries such as construction, food agriculture, and vehicles ( ACC, 2021 ). Overall, the ACC report estimates that, based on dependent industries, the “business of chemistry” was responsible for adding approximately $5.2 trillion to the U.S. economy in 2020, which was about 25% of the U.S. GDP.
A report from the consulting group Vertex, which was prepared for this study (see Section 1.4 for more details), similarly noted that chemical knowledge and chemical inventions spill over into other industries ( Fleming and Basco, 2021 ). The report first notes that chemistry patents accounted for approximately 18% of all patents filed by the U.S. Patent and Trademark Office from 2000 to 2020, with a much higher share of patents in years before 2006 ( Figure 2-6 ). One publication
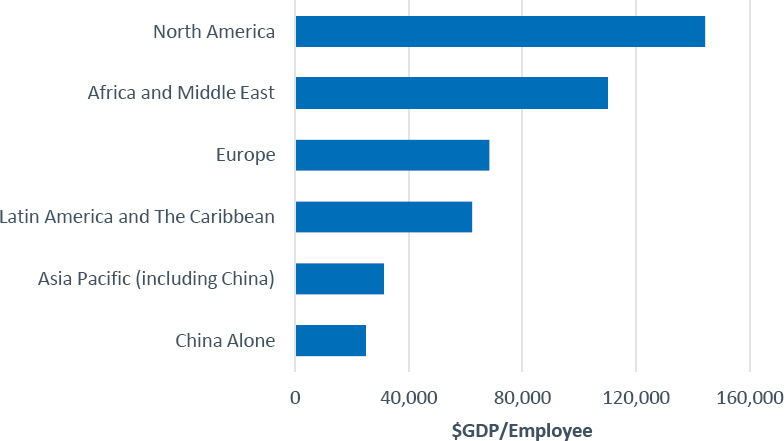
explained this decrease by showing that, following a trend of increasing numbers of chemical patents between 1975 and 1991, the number of chemistry patents began to stagnate between 1991 and 2007 ( Figure 2-7 ) ( Autor et al., 2019 ). During this entire time period (1975–2007), computer patents had a boom, partially explaining the stagnation of chemical patenting as the new tech industry started to have major breakthroughs. This also explains why we see a decreasing trend in the percentage of chemistry patents in the Vertex analysis, which runs from 2000 to 2020.
In looking at chemistry-related patents, Vertex noted that a spillover of knowledge from chemical patents occurs when these patents are cited by patents from other areas such as human necessities, transport, materials, and new technology. Vertex data further suggest that 8.5% of patents rely on chemical research, as measured by citations, found within patents, to scientific publications
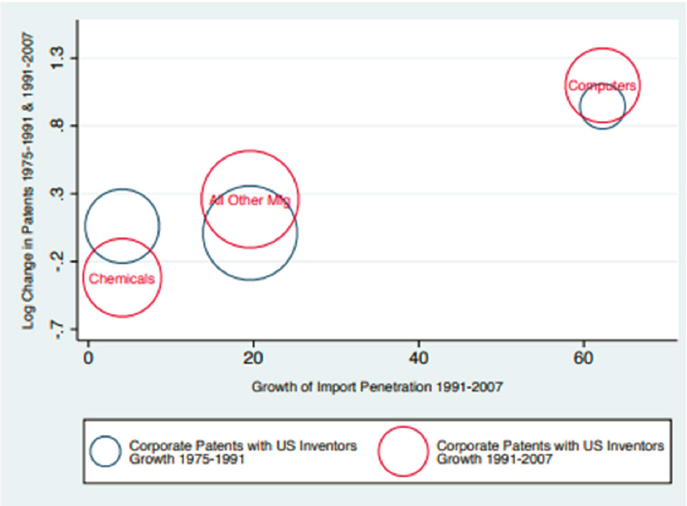
in chemistry-related journals ( Table 2-1 ). In addition, of the patents that cite chemical research issued between 2000 and 2020, 73% are chemistry patents, and the rest (approximately 27%) are nonchemistry patents.
The Vertex report also presented the proportion of patents that cite chemical research in relation to patents that cite any scientific research. Figure 2-8 shows the time trend of number of patents by publicly traded U.S. corporations that cite chemistry research versus patents by the same set of corporations that cite nonchemistry scientific research. All of the cited research is designated as nonpatent literature (NPL). Among patents that cite any research, 20% of them specifically cited chemistry research over the period from 2000 to 2020, but this number has declined from 25% in 2000 to 14.4% in 2020.
To better understand the indirect impacts related to the spillover of chemical knowledge into other areas of R&D, Vertex analyzed the value of all patents that use chemical knowledge. To
TABLE 2-1 Number of Chemistry and Nonchemistry Patents That Cite Chemistry Research from Scientific Publications in Chemistry-Related Journals
SOURCE: Data from Fleming and Basco, 2021 .
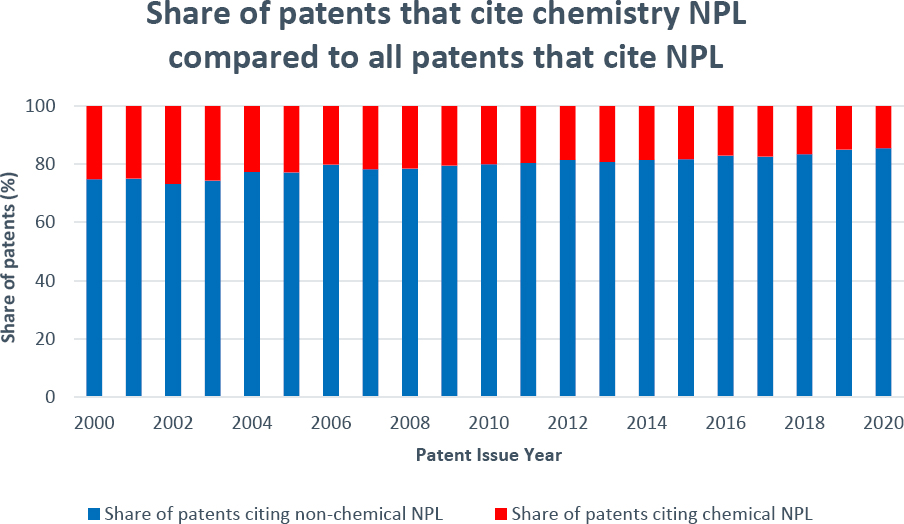
perform this valuation, they matched each patent to estimates of patent values for corporate patents from Kogan et al. (2017) to calculate the value (known as the KPSS value). Importantly, the Kogan et al. estimates rely on the change in the stock market value of a company 3 days following the filing of a patent. Although this is an imperfect measurement, it is considered state of the art for global patent valuation. When considering the value of each patent, the average KPSS value of patents citing chemistry research versus patents citing other NPL can be graphed ( Figure 2-9 ). Patents citing chemical research are more valuable on average than other patents citing nonchemical research.
These data show that the use of chemical knowledge produces products and processes with a higher valuation than the average use of other types of scientific knowledge. The ICCA and OE (2019) report points out the importance of chemical patents, and notes that “chemical products also fuel innovations and patents in other industries, such as photovoltaic cells for electricity production, lightweight vehicle parts, germ-resistant coating for medical instruments, etc.” If we consider this, together with the information that chemistry supports approximately 25% of U.S. GDP, it shows that chemical knowledge and innovation have a high impact on a large portion of the U.S. economy.
2.3 RESEARCH AND INNOVATION IN THE CHEMICAL INDUSTRY
The chemical industry has historically been a hub of innovation and discovery. ICCA and OE (2019) reported that in 2017, the global chemical industry spent $51 billion on R&D, with the United States being the second-largest contributor ($12 billion) after China ($14 billion). That
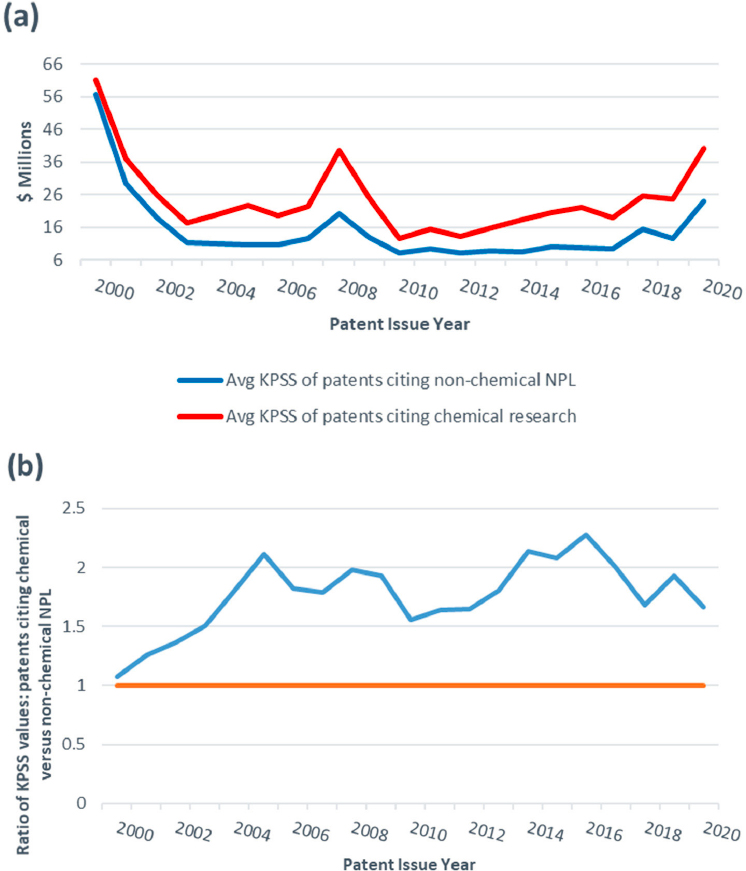
report also notes that the patent intensity 1 for the United States is 50% higher for chemistry than for the economy as a whole ( Hu and Png, 2013 ). Greater patent intensity means that individual patents have a higher impact on a particular sector of the economy. These values reveal the impact of innovation in the chemical industry.
Universities spent about $4 billion in 2019 on chemistry-related basic and applied R&D ( Figure 2-9 ) ( Fleming and Basco, 2021 ). Funding sources of this $4 billion include about $2.1 billion from federal sources and about $250 million from “business sources” ( Figure 2-10 ). Including the money they spent on university R&D, industry spent about $10 billion on R&D in 2019: 46% on
___________________
1 According to the ICCA and OE (2019) report, “patent intensity is measured as the number of U.S. patents awarded to an industry relative to total industry sales in the United States.”
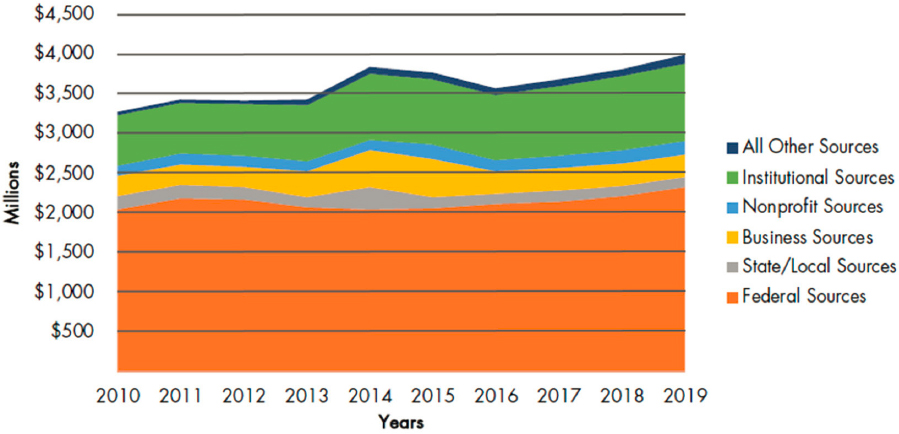
basic and applied research (defined as “fundamental research” in this report), and the remainder on development ( ACC, 2020 ). This money does not include funding from the pharmaceutical sector, which spent about $72.8 billion in the United States on R&D in 2020 ( ACC, 2020 ; Mikulic, 2021 ). 2 Further details on funding and R&D spending is covered in Chapter 6 .
2.3.1 Measuring the Value of Chemical Research
The large expenditure on R&D clearly establishes the importance of chemical research and innovation to the chemical industry. To better address the Statement of Task, the committee sought to understand the connection between fundamental chemical research and the chemical economy. It is important to note that there are substantial challenges associated with this task. Understanding the impact of chemical research is nontrivial because chemical discoveries can take years or even decades before their economic impacts are measurable. In addition, most chemical discoveries that are considered “breakthroughs” are based on a large body of knowledge that took decades, or even centuries, to build. Continuing to build this expansive knowledge base that researchers are able to pull from is one of the most important arguments for funding chemical research. But, it makes assessing the economic impact of chemical research very challenging, because it is difficult to make a global assessment of how research dollars that build a pool of chemical knowledge translate into economic output.
Using the best information and methods available, Vertex looked at the valuation of all chemistry-related patents, using patents as a proxy for chemical research in their analysis. This is a valuation similar to what was described in Section 2.2.2 , except here, Vertex considered all chemistry-related patents, not just those that rely on chemical research. Vertex estimated the value of chemical patents in 2021 dollars to be between $132 billion and $555 billion per year from 2000 to 2020 ( Figure 2-11 ). Acknowledging the caveats of these measurements and assuming that these may be
2 Research at pharmaceutical companies ranges from small-molecule drug discovery and synthesis all the way to clinical trials, and therefore does not neatly fall under the umbrella of chemical research. Additionally, pharmaceutical companies spent money in 2019 and 2020 to research vaccines, prophylactics, tests, antibody therapies, and small-molecule drugs to combat SARS-CoV-2.
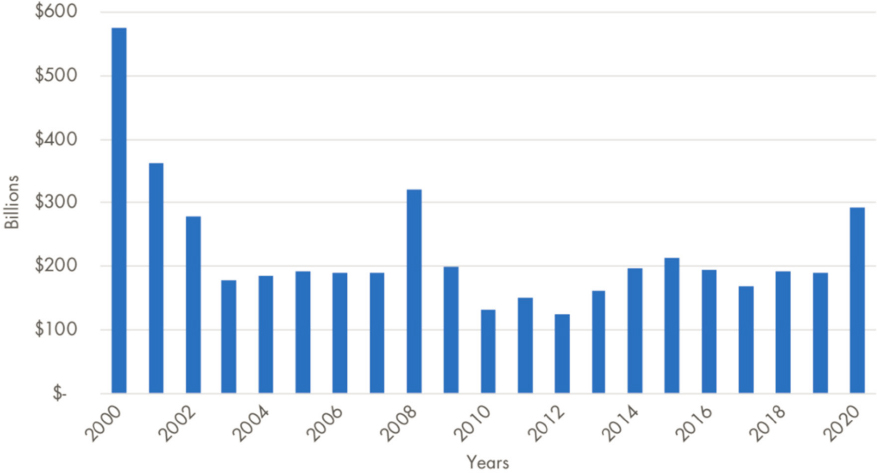
overestimates, Vertex deflated the values by 33% 3 and arrived at a conservative estimate of $221 billion/year to $370 billion/year, in 2021 dollars. Vertex also showed that chemical patents tend to be more valuable than the average patent ( Figure 2-8 ). This indicates that chemical research has a higher private payoff than the average product or process that is patented after research. To quantify this, Vertex showed that while chemical patents accounted for 14% of all corporate patents between 2000 and 2020, they accounted for 23% of all value in the same time period. There are some other important caveats to this patent valuation, including
- The calculation only values corporate patents and is mute on the value of patents assigned to start-ups, universities, and other organizations not listed on the stock market.
- Based on the way the values were calculated, patents issued to a firm on the same day take the same value, even if one patent is more valuable to the firm than the others.
- These values are sensitive to stock market volatility.
- When chemical knowledge is used in the chemical industry, it is sometimes not patented and instead remains as a trade secret, so that the chemistries can only be used by the company who discovered or developed the process or product.
During their analysis, Vertex had many challenges drawing connections between R&D and economic impact. Importantly, they noted that “data limitations inhibit a comprehensive analysis.” Specifically, they noted data limitations related to “patent value estimation, wide-spread availability of licensing terms data, and wide-spread availability of government grant data.” To lessen the gaps, and assist future economic evaluations, the Vertex report noted several possible changes that could make an analysis more comprehensive. The first is the continued support of social science and business research by government and philanthropic organizations, especially in the pursuit of new and improved patent valuation methods. Vertex noted that the state of the art for global patent
3 Based on an alternative measure that estimates value of chemical patents using a Tobin’s q regression ( Lindenberg and Ross, 1981 ).
valuation is likely a significant overestimate and improvements are needed. Second, they suggest supporting “efforts to collect data on how government research funding benefits the economy.” One example they point to is Michigan’s Institute for Research on Innovation and Science, 4 which is aggregating data on grants and personnel. Last, they note that if the U.S. Patent and Trade Office were to “strengthen data collection and publication,” this would help any analyses on intellectual property and its connection to the economy.
2.3.2 Future Analyses to Help Understand the Impact of Chemical Research on the Economy
To continue building a full picture of how chemical research impacts the chemical economy, there are a number of other areas and analyses that could help the community gain a quantitative assessment of this relationship. Although the use of global patent valuation gives some insights, it falls short of a full understanding. Some ideas that might produce valuable results include the following:
- An in-depth patent analysis that focuses on one or more top companies in the United States . The committee heard from a Dutch patent attorney who put together tools for in-depth patent analyses at the companies where she was employed as a patent attorney ( van Tol-Koutstaal, 2021 ). These analyses require a large amount of concerted time and effort for each company, but help to minimize the complications around global patent valuation metrics and the unknown sources of some patents. A valuation such as this could give a precise overall picture of how the products of research are converted into economic output but with the caveat of being specific to the sectors occupied by the company.
- An analysis of licensing revenues generated from patents . Although an analysis like this would require a special effort because the data are not easily available, it would provide useful data around what types of research produce patents that interest external groups. It would be most useful if this was done for a particular company or a specific patent category, and the data would likely show interesting trends on what technologies are most desired.
- Drawing correlations between the number of patents and economic growth . While the current study looked at direct evidence of economic performance, a concerted effort to correlate the number of patents with economic growth for a particular area or company would provide further useful information. This is a very challenging analysis, and some efforts have shown that the number of patents filed by a particular country do not necessarily relate to the impact or economic growth of that country, due to the large number of confounding factors ( Elsevier Analytical Services, 2021 ). To figure out if a correlation exists, researchers would have to develop a way to link data from patents to the firms where they are generated, and then link that information to economic growth. These data would be helpful for understanding how research that generates patented technologies helps to directly impact economic growth.
All of these ideas provide avenues for future analysis and research as we continue to understand the impact of chemical discovery on the U.S. economy. Many, though, come with the caveat that they are analyzing a small sector or a single company. This report took a global and comprehensive view of chemical research and the chemical enterprise. Both types of information are needed for a complete picture.
4 See https://iris.isr.umich.edu/ .
2.3.3 Examples of Chemistry Research That Have Benefited the Economy
While we have noted the challenges in assessing the scale of the chemical economy, as well as the impact of R&D on the economy, one of the best ways to understand both concepts is through specific examples. There are many cases where chemical research has led to widespread economic and societal improvements. Many of these examples make it clear that fundamental chemical research has brought about pivotal discoveries that have led to new products or processes. Some of these examples also show the sprawling impact that such inventions have had. Six examples spanning from the 1960s to today are highlighted: rechargeable batteries, therapeutics, silicon chips, oral contraceptives, catalytic converters, and treatments for COVID-19.
2.3.3.1 Secondary (Rechargeable) Batteries
Modern secondary (rechargeable) batteries have contributed significantly to important economic, energy, and sustainability objectives. Lithium-ion and nickel-metal hydride batteries began widescale commercial production in the 1990s and were essential to the introduction of electric and hybrid electric vehicles that are competitive with their internal combustion counterparts in terms of performance and cost ( Sepulveda et al., 2021 ). They have made possible ubiquitous mobile technologies, including telephones and portable computers, and are particularly beneficial in establishing reliable telecommunication and financial infrastructures in developing countries ( NASEM, 2021b ). The cost and performance characteristics of nickel-metal hydride batteries allow them to directly replace cylindrical primary (nonrechargeable) alkaline and Leclanché (carbon-zinc) batteries in virtually all applications ( Revankar, 2019 ). The significance of lithium-ion battery research was recognized with the award of the 2019 Nobel Prize in Chemistry ( Smithsonian Magazine , 2019 ).
Complementary breakthroughs in industry and academia led to the development of lithium-ion batteries. Stanley Whittingham, who worked for ExxonMobil, started working on a fast-charging battery, but the lithium and titanium combination he used was unstable. John B. Goodenough, an engineer at the University of Texas at Austin, made a cathode of lithium cobalt oxide and discovered that the battery power and capacity doubled while making it safer to use. Akira Yoshino at Meijo University in Nagoya improved the battery’s capacity and safety ( Smithsonian Magazine , 2019 ). Philips Research Laboratories demonstrated the first prototype nickel-metal hydride battery in 1984, building on fundamental research on metal hydrides that began in the 1800s.
The development and improvement of battery technology has important implications for environmental sustainability and plays a large role in the U.S. economy. Batteries are an important method of energy storage that enable the introduction of environmentally sustainable technologies such as electric vehicles and renewable energy sources that include solar and wind power. Additionally, a report produced for Battery Council International estimated the economic impact of the U.S. lead battery industry, and noted that the entire enterprise annually supports $10.9 billion in U.S. GDP and 92,200 jobs ( EDR Group, 2019 ).
2.3.3.2 Biocatalysis in Synthetic Chemistry
The basic research from Frances Arnold and her lab has had a significant impact on the development of simpler and more sustainable synthetic processes to make new molecules ( Turner, 2003 ). She uses “directed evolution” to develop new enzymes with new functionality. Directed evolution of enzymes for biocatalysis is built on a foundation of chemical knowledge from biophysical chemists and analytical chemists working together to understand the structural interactions and reaction mechanisms of different enzymes. The first protein structures of myoglobin and hemoglobin were published in 1958 and 1960, respectively, with a subsequent Nobel Prize in Chemistry awarded in
1962 ( Dauter and Wlodawer, 2016 ). The ability to visualize protein structures allowed chemists to model and understand enzymatic reactions, leading eventually to work on directed evolution that has been widely adopted by the chemical industry. This technology is the basis of the company Codexis, which develops highly specific and efficient enzymes used in DNA and RNA synthesis and in biopharma manufacturing. Begun in 2002, Codexis had 165 employees and revenues of $64 million by 2020.
An enzyme engineered using this technology was recognized in the 2010 Presidential Green Chemistry Challenge. Merck and Codexis were given the Greener Reaction Conditions Award for their development of a second-generation green synthesis of sitagliptin, the active ingredient in Januvia, a treatment for type 2 diabetes ( Codexis, 2021 ). Merck notes that “this collaboration has led to an enzymatic process that reduces waste, improves yield and safety and eliminates the need for a metal catalyst” ( EPA, 2010 ). Before the use of this greener synthesis, the initial manufacturing process filed in 2005 and approved in 2006 had some inherent liabilities including inadequate stereoselectivity, which required a crystallization step; high-pressure hydrogenation (at 250 psi), which required expensive, specialized manufacturing equipment; and the need for a rhodium catalyst ( EPA, 2010 ). Using directed evolution, a transaminase catalyst was developed by Codexis which enabled a new manufacturing process to supplant many of these concerns. The evolved transaminase improved the catalytic activity of the original enzyme by more than 25,000-fold. The challenge award website from the U.S. Environmental Protection Agency notes that
[t]he streamlined, enzymatic process eliminates the high-pressure hydrogenation, all metals (rhodium and iron), and the wasteful chiral purification step. The benefits of the new process include a 56 percent improvement in productivity with the existing equipment, a 10–13 percent overall increase in yield, and a 19 percent reduction in overall waste generation. ( EPA, 2010 )
This new process has been used in manufacturing since 2012 for this critical drug ( EPA, 2010 ).
2.3.3.3 Chemistry of Silicon Computer Chips
Modern computation is a product of many different fields of research, but chemical research has played a big part in increasing the computing speed and miniaturization of the computational technology that we enjoy today. The social, economic, and health impacts of portable computers and mobile phones are enormous.
In the mid-20th century, fundamental research at the intersection of physics, engineering, and chemistry produced innovations that led directly to modern computer architectures and the present computing ecosystem. The first patents for semiconductor devices appeared in the early 1900s ( Ward, 2014 ), and the 1956 Nobel Prize in Physics was awarded to William Shockley, John Bardeen, and Walter Brattain for their research creating the first semiconductor-based transistor ( Nobel Prize Outreach, 2022d ). One of the next major advances was research in photolithography in the 1950s, which enabled printing of miniaturized integrated circuits ( Computer History Museum, 2022 ). In particular, fundamental research in photoresists and catalysts at different length scales facilitated technology development and drove Moore’s Law for computer chip manufacturing at companies such as IBM ( Figure 2-12 ). As an example, research on photoacid generators (small molecules that react with specific wavelengths of light to form a superacid in the solid state) led to the development of material combinations needed for sub-10-nm technology. At the time of this report, a remarkable 2-nm feature size had been reached, made possible with technology based on these early discoveries derived from fundamental chemistry, polymer chemistry, and materials science. Because of these advances in chemistry, the size of computer chips has decreased steadily over the years, leading to the incorporation of more and more transistors on computer chips and concomitantly faster computation speeds ( Shalf, 2020 ).
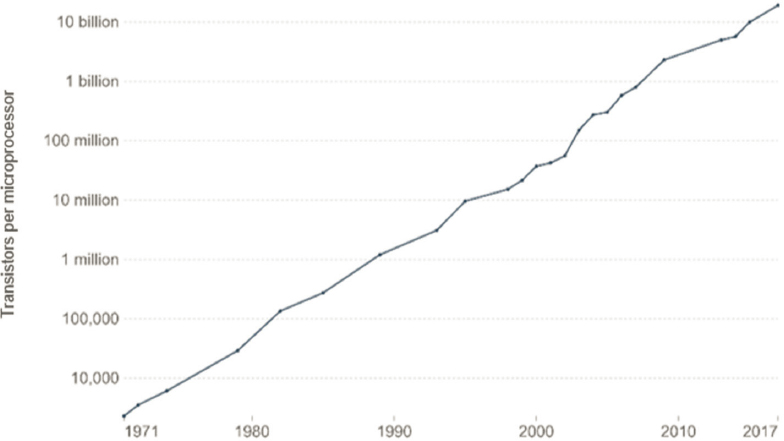
The rapidly growing need for computational technologies has caused silicon chips to be more critical to the U.S. economy than ever before. As chemistry, engineering, and physics have allowed for increased performance of semiconductors, the semiconductor industry is now directly responsible for a large amount of the U.S. GDP. According to a report from the Semiconductor Industry Association (SIA) and OE, “the U.S. semiconductor industry is substantial, directly contributing $246.4 billion to U.S. GDP and directly employing over 277,000 workers in 2020” ( SIA and OE, 2021 ). Similar to the chemical industry as a whole, the semiconductor industry has a wide range of indirect economic impacts due to the use of semiconductors in such a wide variety of technologies. The report notes that “300 downstream economic sectors accounting for over 26 million U.S. workers are consumers of and are therefore enabled by semiconductors” ( SIA and OE, 2021 ).
2.3.3.4 Oral Contraceptives
Oral contraception for women has had an undeniably large effect on the economy and on the role of women in society. Norethindrone (also known as norethisterone), the original compound that was used in birth control pills in the 1950s, was still the 143rd most prescribed drug in the United States in 2019, while a combination drug of norethisterone and ethinyl estradiol was the 42nd most prescribed drug ( ClinCal, 2019 ). Oral contraceptives provided women a level of control over their own reproductive health and the ability to make decisions about family planning that they never had before.
The adoption of the “Pill” for use in contraception took a long time. When it was introduced in the 1960s, it was only used for “cycle control” in married women. But, in the 1980s, the Pill became more widely accepted as a method of family planning, especially with the increasing number of women who were in medicine and other professional careers ( Liao and Dollin, 2012 ). Oral contraception has also empowered women around the world, providing them with reproductive autonomy and helping to balance the power dynamic in reproductive decision making. According to the United States Agency for International Development, family planning provides a large number of benefits, including protecting women and children’s health, reducing HIV and AIDS, decreasing
abortions, improving educational and employment opportunities for women, and reducing poverty ( USAID, 2021 ).
The original synthesis of norethindrone, a steroid derivative, was completed on October 15, 1951, by Luis Miramontes, a young Mexican chemist working under the supervision of Carl Djerassi at the company Syntex ( Figure 2-13 ) ( Djerassi, 2006 ). Syntex, located in Mexico City, was a unique company at the time, because it invested heavily in basic research around steroid hormone synthesis and was responsible for approximately 30% of the industrial publications on the topic prior to 1960 ( Olofson and Gortler, 1999 ). Much of the reason for this heavy investment in steroid chemistry was related to the discovery that a native yam plant, Dioscorea mexicana , contained a substantial amount of the natural product diosgenin. In the 1930s, a chemist named Russell Marker, from Pennsylvania State University, discovered a simple synthetic route, termed the “marker degradation,” to convert diosgenin to the steroid hormone progesterone ( Olofson and Gortler, 1999 ). Progesterone was the main focus of the steroid hormone industry at the time because “of its value in treating various menstrual disorders and preventing certain types of miscarriages” ( Olofson and Gortler, 1999 ).
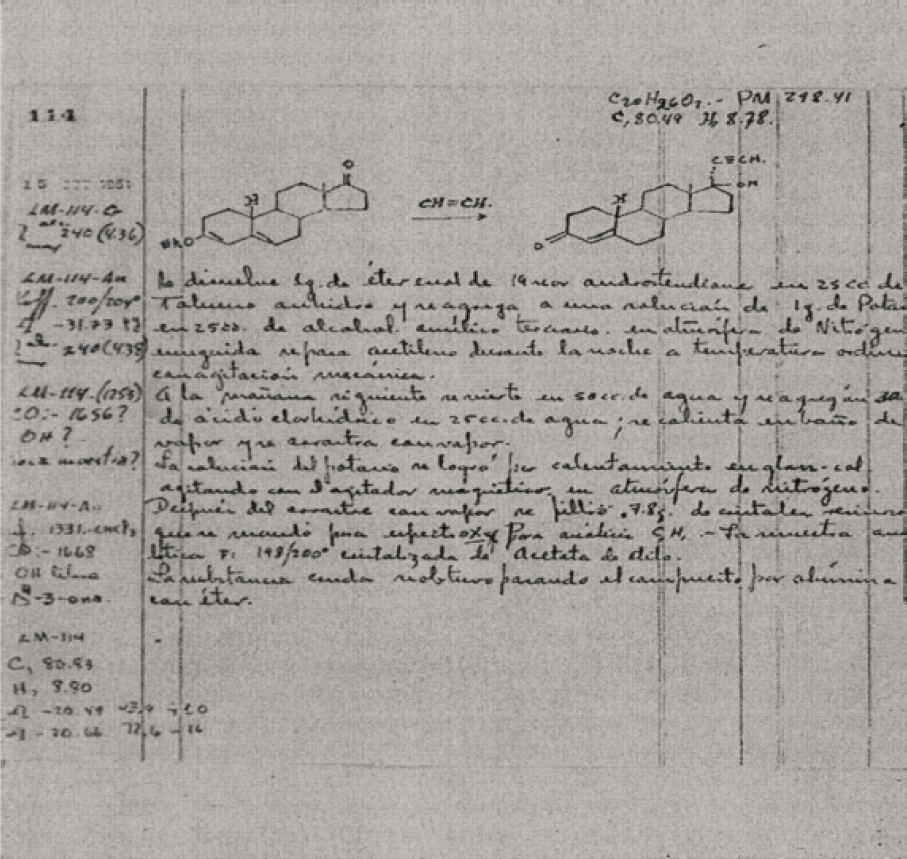
By the time Djerassi came to Syntex in 1949, his research program was less interested in the synthesis of progesterone and instead focused on cortisone, recently discovered as a treatment for rheumatoid arthritis. While working on the cortisone synthesis from the diosgenin precursor, Djerassi was also interested in another possible line of research around progesterone derivatives. At the time, modifying progesterone was widely considered to be useless because any modifications seemed to cause the molecule to lose biological potency. However, a researcher at the University of Pennsylvania performed a really messy and impure synthesis to create a derivative of progesterone that removed a methyl group. This “19-norprogesterone” seemed to display hints of activity that were higher than progesterone and opened the door for progesterone modifications that might lead to higher potencies. After researching and trying out a large number of different progesterone derivatives, a final formulation was synthesized and then sent out for biological evaluation.
The final formulation of the “Pill” produced by Djerassi and Miramontes provided a simple synthesis derived from an abundant natural product. Norethindrone also had higher potency than progesterone, a characteristic that was critical for oral administration. Progesterone, while still effective, cannot be taken as an oral medication. The flood of research in natural products synthesis and steroid hormone development was a critical scientific endeavor that led to the final formulation of oral contraceptives and led to a simpler synthesis of many other important compounds, including progesterone, cortisone, and estrogen.
2.3.3.5 Catalytic Converters
As noted later in this report, in Section 3.4.4.1 , the invention of the catalytic converter led to a significant decrease in air pollution from internal combustion engines. The introduction of the catalytic converter in the 1970s produced huge economic and environmental benefits by reducing airborne particulates, acid rain, smog, and these pollutants’ concomitant health effects.
While there are several chemical strategies used inside a catalytic converter, all of them deploy some combination of platinum group metals (PGMs) platinum, palladium, and rhodium with other components to provide functions such as oxygen and hydrocarbon storage ( Farrauto et al., 2019 ). Two separate National Medals of Technology and Innovation were awarded in 2002 related to the invention and development of catalytic converters. One went to John J. Mooney and Carl D. Keith at Engelhard (now BASF) for the invention and development of the three-way catalyst system that allowed the simultaneous control of Carbon Monoxide, hydrocarbons, and NO x . A second award went to Haren S. Gandhi of Ford Motor Company both for his contributions toward developing these systems as well as for driving the focus on recycling of spent converters, which improved the sustainability of PGMs in their deployment ( USPTO, 2002 ). Despite the current maturity of these catalytic systems, their performance continues to improve thanks to the application of advances from fundamental studies ( Paolucci et al., 2017 ).
Chemical and materials sciences were critical in the development of other catalytic converter components beyond the catalysts. The bulk structure is a ceramic honeycomb typically made of cordierite, which has been extruded into a form factor that allows high gas flow while minimizing backpressure ( Farrauto et al., 2019 ). The science needed to produce these structures was based on years of fundamental studies in rheology, surfactants, and oxide chemistries ( Govender and Friedrich, 2017 ). Rodney D. Bagley, Irwin W. Lachman, and Ronald M. Lewis of Owens Corning (now Corning) were awarded a National Medal of Technology and Innovation in 2003 in recognition of the impact that their development of ceramic substrates for catalytic converters has had ( USPTO, 2003 ). Chemical exploration of improved honeycomb designs remains an opportunity for fundamental research. One exciting development is the use of 3-D printing combined with advanced computational methods to design and manufacture the honeycombs ( Kovacev et al., 2021 ). There
are also great opportunities to expand the use of these materials and related substrates into other environmental research areas ( Hosseini et al., 2020 ).
2.3.3.6 Chemical Research Toward the COVID-19 Response
A timely example of the large-scale benefits of fundamental chemical research is its impact on the response to COVID-19, the pandemic that cost nearly 1,000,000 American lives as of February 2022 ( NCHS, 2019 ). The Congressional Budget Office estimates $7.6 trillion in lost output for a decade from COVID-19 ( Cutler and Summers, 2020 ). Estimates that account for mortality, morbidity, mental health conditions, and direct economic losses place the economic cost at $19 trillion, assuming the pandemic had been largely controlled by fall 2021 ( CBO, 2020 ). Response to a pandemic of this magnitude requires a multipronged approach including public health measures, vaccines that can reduce the incidence and severity of disease, and therapeutics that target multiple parts of the viral life cycle. This multipronged approach requires a multidisciplinary effort, with inputs from scientific researchers, health care workers, public health professionals, and so many more. Chemical research continues to play a significant role in this effort and has contributed to a number of areas, including the development of nonnatural nucleotides ( Nance and Meier, 2021 ) and lipid nanoparticles ( Eygeris et al., 2022 ) that are crucial for the stability and delivery of mRNA vaccines. Additionally, fundamental chemical research from the late 1980s onward continues to be pivotal for the discovery of oral medications that have shown efficacy in clinical trials for COVID-19, including remdesivir ( Gottlieb et al., 2022 ), molnupiravir ( Bernal et al., 2022 ), and nirmatrelvir (Paxlovid) ( Hammond et al., 2022 ).
Remdesivir and molnupiravir are two in a large class of drugs that are chemically modified variations of nucleotides, the molecules that are building blocks for both RNA and DNA. Early examples of this class of therapeutics were created in the 1960s as possible anticancer drugs and in the 1980s were discovered to be promising therapies for HIV/AIDS. Modified nucleotides have subsequently been used as therapies for hepatitis, Ebola, and other viral diseases ( NIAID, 2018 ). Fundamental research in the chemical synthesis of modified nucleotides directly enabled applied research in the use and manufacture of these molecules as drugs. As a concrete example, fundamental research in the 1980s and early 1990s discovered novel strategies to control the geometric arrangement of atoms in the modified nucleotides and therefore synthesize the specific enantiomer that is effective for safely treating disease ( Wilson and Liotta, 1990 ).
This entire body of work enabled the discovery of molnupiravir, which was first investigated as a potential therapy for Venezuelan equine encephalitis virus (VEEV) but was later shown to have broad-antiviral activity against other diseases, including Ebola ( Painter et al., 2021 ). Finding drugs for VEEV was a priority for the Department of Defense (DoD), and DoD, along with other government agencies, provided $35 million between 2013 and 2020 to Emory University researchers for the development of molnupiravir. This included 6 years of nonclinical testing for the drug, and the testing of its use to fight MERS-CoV ( Abinader, 2021 ). Emory has five published U.S. patent applications directed to derivatives of N 4 -hydroxycytidine, the molnupiravir parent compound, which acknowledges U.S. government funding for the development of these patents ( Abinader, 2021 ). Emory University subsequently licensed molnupiravir to Ridgeback Biotherapeutics, which in turn collaborated with Merck for clinical trials of molnupiravir for COVID-19 and for molnupiravir manufacturing ( Abinader, 2021 ).
In the case of molnupiravir, the U.S. Food and Drug Administration issued an emergency use authorization (EUA) in March 2022 ( FDA, 2022 ). The drug’s use is restricted to high-risk individuals who contract COVID-19, but Merck is already projecting between $5 billion and $5.5 billion worth of sales in 2022 ( Dunleavy, 2022 ). Paxlovid was similarly issued an EUA in December 2021 ( Katella, 2022 ), and analysts are predicting $22 billion in sales after reporting $1.5 billion in the
first quarter of 2022 ( Kimball, 2022 ). Both of these pharmacueticals are having an immediate and noticeable economic and public health impact.
2.4 UNDERSTANDING U.S. COMPETITIVENESS IN THE CHEMICAL ECONOMY
To fully understand the economic impact of the U.S. chemical economy, it is important to evaluate its role on the global economic stage and understand changes or shifts that have occurred over time. The U.S. chemical economy is also part of a complex ecosystem that, while competitive with chemical industries in other countries, is also heavily reliant on them for collaboration, innovation, and workforce needs. These factors must be considered as a part of the same ecosystem and kept in mind in creating policies for improving the chemical economy.
2.4.1 Comparative Global Research Output in Chemistry
To analyze global competitiveness, we can compare research outputs of different countries in the form of publications, economic outputs, and several other points of analysis. It is important first to look at the research portfolio of individual countries for the purposes of understanding where the research interests are in different nations. In 2020, more than 50% of the publications from the United States were in the life sciences (37% health sciences and 14% biological and biomedical sciences) ( Figure 2-14 ) ( NSB, 2021 ). In contrast, only 4% of U.S. publications focused on chemistry and materials science. Inevitably, chemical knowledge informed some of the publications in the life sciences, but it is important to note that chemistry-specific publications are a low percentage of U.S. research output. China, India, and Japan each had 8% of their publication output in chemistry-specific research, with 5%, 7%, and 3% in materials science, respectively. These differing portfolio shares in chemistry are one indicator of a relative advantage of China in the area of chemistry publication ( Figure 2-14 ).
In addition to the data showing research portfolios, it is helpful to understand the relative abundance of publications and patents in particular areas. The metrics for scientific productivity are imperfect because of the many caveats associated with using quantitation such as number of publications or number of patents in a particular area. However, these numbers, when considered as a part of a holistic picture, can help to understand trends and intensity of inventions in a scientific field during specific time periods. The National Science Foundation’s (NSF’s) National Science Board measures publication numbers using fractional article counts, which means that “each country receives fractional credit on the basis of the proportion of its participating authors” ( NSB, 2021 ). The U.S. fractional share in all science and engineering (S&E) articles has declined by about half (from 31.6% to 15.5%) in the period between 1996 and 2020, and the U.S. share in chemistry-specific articles has also declined by about half over the same period (from 19.8% to 8.8%) ( Figure 2-15c and d ) ( NSB, 2021 ). Interestingly, while the U.S. percentages have declined, the total fractional count of articles published by U.S. authors has increased when looking at all S&E fields (313,000 in 1996 to 456,000 in 2020) and remained stagnant when considering chemistry-specific S&E articles (between 10,000 and 20,000 over the same time period) ( Figure 2-15a and b ). The decrease in percentage of fractional S&E publications is therefore largely because China’s total numbers of all S&E articles and chemistry-specific S&E articles increased dramatically. China’s fractional publication count of all S&E articles was 34,000 in 1996 and increased 20-fold by 2020 to 670,000. Similarly, the number of chemistry articles saw an 11-fold increase, going from 4,700 in 1996 to 55,600 in 2020. This increase in total fractional counts led to China’s share in overall S&E articles increasing more than sixfold (from 3% to 23%) in the period between 1996 and 2020. Additionally, China’s share in chemistry has also increased more than fourfold over the same period
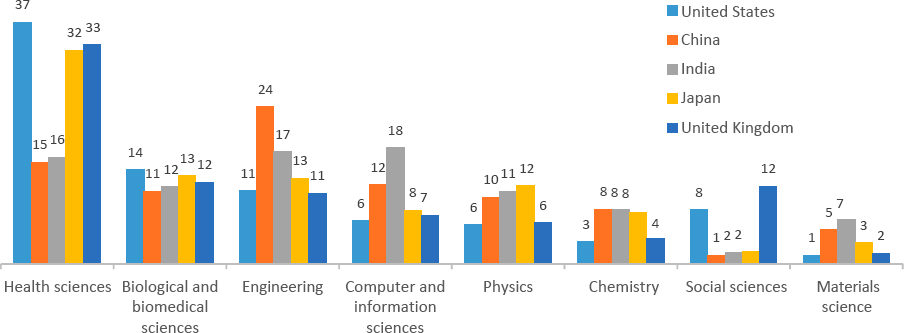
(from 7% to 31%). No other country experienced such a drastic increase in publication output over this time period, although notably, India doubled its percentage of all S&E articles published. Most other countries have remained relatively static ( Figure 2-15d ).
Although the number of S&E articles from the United States increased from 1996 to 2020, chemistry-specific articles remained at around 10,000–20,000 articles per year ( Figure 2-15a and b ). There are several possible explanations for this trend. The first is that the chemical industry and its related chemical research and publication output has stagnated. Another possibility is that chemistry research in the United States has become a science that is now used to support and inform other topic areas such as the life sciences, and publications in these areas, while they might be based on chemical discovery, are not counted toward chemistry publications. Likely, it is some combination of these and other factors. As shown in Figure 2-15 , China’s publication rate has been steadily increasing over the past 20 years, likely due to its strong history of seeing the value in investing in the chemical sciences ( Jia, 2018 ).
Another way to look at publications is to consider the share of S&E articles that are the most cited (e.g., top 1% cited), with citations normalized by subfield and year. Articles published in the United States in 2018 were about 60% above the world average for all S&E articles and 55% above the world average in chemistry ( Figure 2-15 ). The only other countries to have an above-average number of highly cited papers are the United Kingdom and, in later years, Germany. This correlates well with countries that have well-established scientific research programs. When looking at China’s share of the top 1% of cited S&E articles, the numbers in 2018 seem to be on par with the world average, while the share in the top 1% of cited articles in chemistry is 39% above the world average.
Looking at the trends over time for the share of highly cited articles per country, it is notable that the U.S. share in all S&E articles had been stagnant from 1996 to 2015 and then declined in more recent years, from 72% above the world average to 60% above the world average. Compared to this overall trend, the United States has had a steeper decline in chemistry, dipping from 93% above the world average to 55% above the world average ( Figure 2-16 ).
Aside from publications, another possible metric of research productivity is to look at the share of patents published by different countries in the area of chemistry. In several different subfields of chemistry, the United States was the dominant publisher in 2017–2019 ( WIPO, 2021 ). For example, the United States filed the most overall patent applications in biotechnology and pharmaceuticals ( Table 2-2 ). China was more active than all other countries in basic materials chemistry, chemical
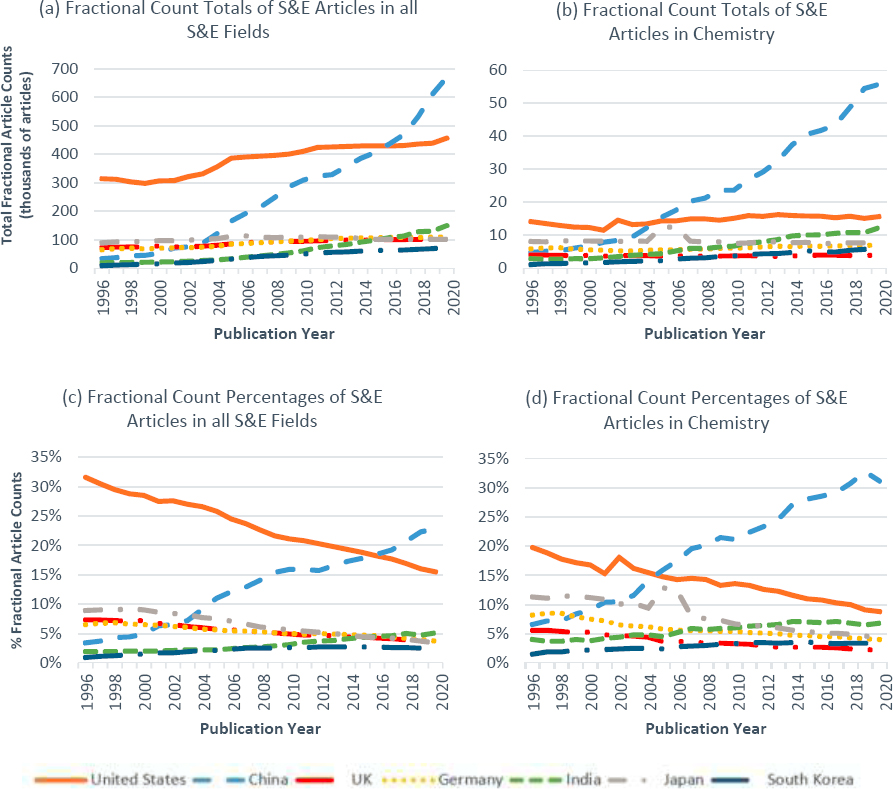
engineering, and environmental technology ( Table 2-2 ). Several other countries, such as Russia, Germany, and Japan, were leaders in other areas of chemistry, but there are no particularly strong indicators that one country holds an advantage in patenting over another. Additionally, there are many caveats associated with patent data that were laid out in detail earlier in the chapter.
2.4.2 Comparative Global Economic Output
We can further consider the value added of chemicals and chemical products by industry in order to assess the competitiveness of the chemical economy. Between 2002 and 2010, the United States was the unparalleled leader in the share of value added by chemical industry (IHS Markit, 2022 ). However, throughout that period, the U.S. chemical industry saw a steady decline. In 2011, the value-added chemical and chemical products industry in China surpassed that of the United
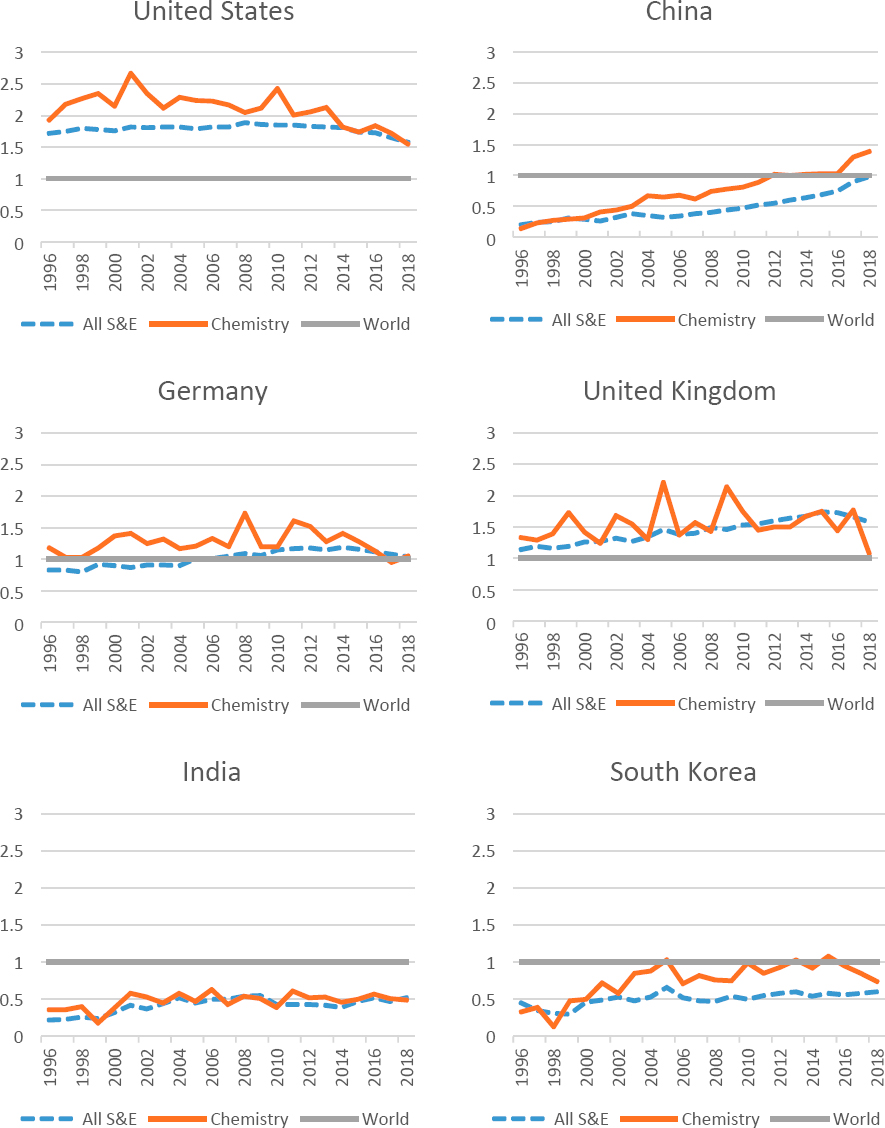
TABLE 2-2 Share of Total Published Patent Applications by Country and Subfield, 2017–2019
NOTE: Numbers in bold indicate top number of patent applications for a particular field. SOURCE: Data from WIPO, 2021 .
States, following a steady increase in China’s output over the entire period. Importantly, the U.S. share in the international chemical economy held steady and even saw a slight increase between 2010 and 2018, indicating that, despite China’s continual increase, the United States was also experiencing growth. This was in large part due to the extraction and increased supply of natural gas (see Box 2-1 ). By 2018, the U.S. chemical industry value added was 0.216 trillion current U.S. dollars, second to China’s value added in the chemical industry at 0.298 trillion current U.S. dollars. That year, China and the United States accounted for 50% of the world chemical output ( IHS Markit, 2022 ).
Another metric of the economic competitiveness of chemistry in the United States and in the world is sales of the leading chemical companies globally. In 2020, as reported by Chemical & Engineering News , the top 50 chemical companies, headquartered in 19 countries around the world, had total combined sales of nearly $796 billion. Ten of those companies are headquartered in the United States ( Table 2-3 ) and had combined total sales in 2020 of $154 billion ( Tullo, 2021 ). Among the U.S. chemical companies in the top 50, only two, Dow Chemical (#3) and LyondellBasell (#10), are in the top 10. Nonetheless, the 10 U.S. companies claim the largest percentage of total sales (19.3%) among the 19 countries on the list.
All of these factors taken together paint a picture of several countries who are dominant in the chemical sciences enterprise, with leadership in various facets from the United States, China, Japan, Germany, and the UK. Before making decisions about international competitiveness and leadership, it is important to consider a wide swath of data and a number of different metrics. The United States continues to be an important player in chemical research and the chemical industry. To continue being a leader in this space, the United States has to consider making data-driven and aspirational investment decisions relating to the chemical sciences enterprise.
TABLE 2-3 Top 50 Chemical Companies by Sales
SOURCE: Data from Tullo, 2021 .
2.4.3 Impact of International Researchers on the U.S. Chemical Economy
The U.S. chemical economy is part of a complex ecosystem that is heavily reliant on other countries for collaboration, innovation, and workforce needs. The Science and Engineering Indicators produced by NSF’s National Science Board noted that in 2017, 30% of workers in S&E occupations were foreign-born. When looking specifically at the workforce in the physical sciences, approximately 34% of Ph.D.-level scientists were foreign-born ( Figure 2-17 ). Additionally, approximately 27% and 23% of physical sciences workforce bachelors and masters recipients, respectively, were foreign-born ( Figure 2-17 ) ( NSB, 2019 ). Importantly, these data do not specify what sector these individuals are working in, and they do not specify chemists or chemical engineers, but the number of foreign-born workers in the United States is still large. The highest percentage of this workforce is from Asia, with China, India, and South Korea in the top three places ( NSB, 2019 ). If you also look at those receiving degrees, 36.2% of doctoral-degree chemists and 47.3% of doctoral-degree chemical engineers in the United States between 2010 and 2020 were foreign-born citizens ( NCSES, 2020b ). Based on the 2020 NCSES data, approximately 73% of these graduates say they plan to stay in the United States, and these numbers held true with doctoral recipients from 2011 to 2013 staying in the United States at 71% and those recipients from 2006 to 2008 staying at 72%, when the data were collected in 2017 ( NCSES, 2020b ).
As an additional example, while the Nobel Prizes are an imperfect measure of impact, it is notable that the number of researchers that make up the category of “foreign-born U.S. laureate” is greater than the number of prizes won by most countries. Including the 2021 Nobel Prize, 46% of all prizes have been given to researchers in the United States, and of that, 16% of all prize recipients are foreign-born U.S. laureates ( Figure 2-18 ). It becomes fairly clear that in a highly competitive international environment, the United States remains a desirable place to perform groundbreaking research.

2.5 CONCLUSIONS
This chapter outlines the scope and impact of the U.S. chemical economy and identifies linkages between chemical research and the chemical economy using various measurable parameters and case studies. The data show that the chemical economy in the United States is still strong but could be stronger if we want to experience leadership growth. Some of the quantitative data from this chapter show that in 2020, the U.S. chemical economy, excluding pharmaceuticals, had $457 billion in sales, directly added $225 billion to the overall economy, and employed 529,000 individuals. These numbers have been relatively steady for the past 5 years. The chemical economy additionally has indirect impacts on the U.S. economy, and in 2020 the “business of chemistry” was estimated to be responsible for $5.2 trillion, or 25% of U.S. GDP, and the employment of 4.1 million individuals who in some way interact with the chemical economy.
This chapter also highlights the great difficulty in quantifying the true impact of fundamental chemical research. Despite this, the importance of chemical discovery is quite evident in specific technologies that have a huge impact on the economy and society, such as batteries, biocatalysis in synthesis, silicon chips, oral contraceptives, catalytic converters, and pharmaceuticals to fight SARS-CoV-2. In each of these cases, a large body of chemical knowledge that was built over decades or centuries contributed to a chemical discovery that enabled important advances. From the information gathered about the size and impact of fundamental chemical research and the U.S. chemical economy, the committee highlighted several conclusions.
Conclusion 2-1: Chemical research has an outsized economic value based on the spillover of chemical knowledge and products into other areas and the fact that chemical patents, as well as patents that rely on chemical knowledge, have a higher average value than other patents. Chemical patents accounted for 14% of all corporate patents between 2000 and 2020, but they accounted for 23% of all value in the same time period.
Conclusion 2-2: It is challenging to directly link chemical research to economic impact because each chemical product or process relies on a broad body of chemical knowledge and discovery that is built over decades or centuries, and chemical knowledge is also deeply integrated into other disciplines, making the specific impacts of chemistry in the broad scientific enterprise difficult to deconvolute. Additionally, analyzing the economic impact of chemical research suffers from a lack of data, including patent value estimations, widely available licensing terms data, and government grant data.
Conclusion 2-3: Chemistry is a foundational and central scientific discipline, and sustained investment in fundamental chemical research provides the chemical knowledge for technology development, generating unexpected discoveries that are the basis for innovation. These innovations directly influence the chemical economy, environment, and quality of life and also advance knowledge and discovery in many other scientific and technological disciplines, such as the life sciences, information technology, earth sciences, and engineering.
Conclusion 2-4: The chemical economy is critically important for our national economy and our leadership in the international chemical enterprise. This leadership relies heavily on advances in fundamental chemistry that drive the creation of new tools, technologies, processes, and products and enables environmental considerations. However, our nation’s
leadership in the chemical industry cannot be taken for granted, and this leadership needs continued and sustained nurturing and support.
Conclusion 2-5: The success of the chemical economy relies on a large group of employees who work both within and proximal to the chemical enterprise. Continuing to attract and retain diverse talent in the chemical sciences, both internationally and domestically, is critically important to a thriving chemical economy.
This page intentionally left blank.
Chemistry plays a pivotal role in the strength of the U.S. economy and the advancement of humankind. Chemists' achievements include life-saving pharmaceuticals, advanced energy solutions, improved agricultural productivity, and novel materials used in products from clothing to electronic devices. The many sectors reliant on the U.S. chemical economy account for about 25% of the U.S. GDP and support 4.1 million U.S. jobs. However, a new and evolving chemistry landscape requires changes with regard to funding, training, and a focus on integrating sustainability into manufacturing, product usage, and product disposal.
This report identifies strategies and options for research investments that will support U.S. leadership while considering environmental sustainability and developing a diverse chemical economy workforce with equitable opportunities for all chemistry talent. The report recommends that funding agencies and philanthropic organizations who support the chemical sciences fund as large a breadth of fundamental research projects as possible. Chemical industry and their partners at universities, scientific research institutions, and national laboratories should align the objectives of fundamental research to directly assist with new practices toward environmental stewardship, sustainability, and clean energy. Additionally, the report recommends that funding agencies make substantial investment toward education research to enable innovative ways of teaching about emerging concepts, tools and technologies.
READ FREE ONLINE
Welcome to OpenBook!
You're looking at OpenBook, NAP.edu's online reading room since 1999. Based on feedback from you, our users, we've made some improvements that make it easier than ever to read thousands of publications on our website.
Do you want to take a quick tour of the OpenBook's features?
Show this book's table of contents , where you can jump to any chapter by name.
...or use these buttons to go back to the previous chapter or skip to the next one.
Jump up to the previous page or down to the next one. Also, you can type in a page number and press Enter to go directly to that page in the book.
Switch between the Original Pages , where you can read the report as it appeared in print, and Text Pages for the web version, where you can highlight and search the text.
To search the entire text of this book, type in your search term here and press Enter .
Share a link to this book page on your preferred social network or via email.
View our suggested citation for this chapter.
Ready to take your reading offline? Click here to buy this book in print or download it as a free PDF, if available.
Get Email Updates
Do you enjoy reading reports from the Academies online for free ? Sign up for email notifications and we'll let you know about new publications in your areas of interest when they're released.
- Search Menu
Sign in through your institution
- Browse content in Arts and Humanities
- Browse content in Archaeology
- Anglo-Saxon and Medieval Archaeology
- Archaeological Methodology and Techniques
- Archaeology by Region
- Archaeology of Religion
- Archaeology of Trade and Exchange
- Biblical Archaeology
- Contemporary and Public Archaeology
- Environmental Archaeology
- Historical Archaeology
- History and Theory of Archaeology
- Industrial Archaeology
- Landscape Archaeology
- Mortuary Archaeology
- Prehistoric Archaeology
- Underwater Archaeology
- Zooarchaeology
- Browse content in Architecture
- Architectural Structure and Design
- History of Architecture
- Residential and Domestic Buildings
- Theory of Architecture
- Browse content in Art
- Art Subjects and Themes
- History of Art
- Industrial and Commercial Art
- Theory of Art
- Biographical Studies
- Byzantine Studies
- Browse content in Classical Studies
- Classical Literature
- Classical Reception
- Classical History
- Classical Philosophy
- Classical Mythology
- Classical Art and Architecture
- Classical Oratory and Rhetoric
- Greek and Roman Archaeology
- Greek and Roman Papyrology
- Greek and Roman Epigraphy
- Greek and Roman Law
- Late Antiquity
- Religion in the Ancient World
- Digital Humanities
- Browse content in History
- Colonialism and Imperialism
- Diplomatic History
- Environmental History
- Genealogy, Heraldry, Names, and Honours
- Genocide and Ethnic Cleansing
- Historical Geography
- History by Period
- History of Agriculture
- History of Education
- History of Emotions
- History of Gender and Sexuality
- Industrial History
- Intellectual History
- International History
- Labour History
- Legal and Constitutional History
- Local and Family History
- Maritime History
- Military History
- National Liberation and Post-Colonialism
- Oral History
- Political History
- Public History
- Regional and National History
- Revolutions and Rebellions
- Slavery and Abolition of Slavery
- Social and Cultural History
- Theory, Methods, and Historiography
- Urban History
- World History
- Browse content in Language Teaching and Learning
- Language Learning (Specific Skills)
- Language Teaching Theory and Methods
- Browse content in Linguistics
- Applied Linguistics
- Cognitive Linguistics
- Computational Linguistics
- Forensic Linguistics
- Grammar, Syntax and Morphology
- Historical and Diachronic Linguistics
- History of English
- Language Variation
- Language Families
- Language Evolution
- Language Reference
- Language Acquisition
- Lexicography
- Linguistic Theories
- Linguistic Typology
- Linguistic Anthropology
- Phonetics and Phonology
- Psycholinguistics
- Sociolinguistics
- Translation and Interpretation
- Writing Systems
- Browse content in Literature
- Bibliography
- Children's Literature Studies
- Literary Studies (Modernism)
- Literary Studies (Romanticism)
- Literary Studies (American)
- Literary Studies (Asian)
- Literary Studies (European)
- Literary Studies (Eco-criticism)
- Literary Studies - World
- Literary Studies (1500 to 1800)
- Literary Studies (19th Century)
- Literary Studies (20th Century onwards)
- Literary Studies (African American Literature)
- Literary Studies (British and Irish)
- Literary Studies (Early and Medieval)
- Literary Studies (Fiction, Novelists, and Prose Writers)
- Literary Studies (Gender Studies)
- Literary Studies (Graphic Novels)
- Literary Studies (History of the Book)
- Literary Studies (Plays and Playwrights)
- Literary Studies (Poetry and Poets)
- Literary Studies (Postcolonial Literature)
- Literary Studies (Queer Studies)
- Literary Studies (Science Fiction)
- Literary Studies (Travel Literature)
- Literary Studies (War Literature)
- Literary Studies (Women's Writing)
- Literary Theory and Cultural Studies
- Mythology and Folklore
- Shakespeare Studies and Criticism
- Browse content in Media Studies
- Browse content in Music
- Applied Music
- Dance and Music
- Ethics in Music
- Ethnomusicology
- Gender and Sexuality in Music
- Medicine and Music
- Music Cultures
- Music and Culture
- Music and Media
- Music and Religion
- Music Education and Pedagogy
- Music Theory and Analysis
- Musical Scores, Lyrics, and Libretti
- Musical Structures, Styles, and Techniques
- Musicology and Music History
- Performance Practice and Studies
- Race and Ethnicity in Music
- Sound Studies
- Browse content in Performing Arts
- Browse content in Philosophy
- Aesthetics and Philosophy of Art
- Epistemology
- Feminist Philosophy
- History of Western Philosophy
- Metaphysics
- Moral Philosophy
- Non-Western Philosophy
- Philosophy of Action
- Philosophy of Law
- Philosophy of Religion
- Philosophy of Language
- Philosophy of Mind
- Philosophy of Perception
- Philosophy of Science
- Philosophy of Mathematics and Logic
- Practical Ethics
- Social and Political Philosophy
- Browse content in Religion
- Biblical Studies
- Christianity
- East Asian Religions
- History of Religion
- Judaism and Jewish Studies
- Qumran Studies
- Religion and Education
- Religion and Health
- Religion and Politics
- Religion and Science
- Religion and Law
- Religion and Art, Literature, and Music
- Religious Studies
- Browse content in Society and Culture
- Cookery, Food, and Drink
- Cultural Studies
- Customs and Traditions
- Ethical Issues and Debates
- Hobbies, Games, Arts and Crafts
- Natural world, Country Life, and Pets
- Popular Beliefs and Controversial Knowledge
- Sports and Outdoor Recreation
- Technology and Society
- Travel and Holiday
- Visual Culture
- Browse content in Law
- Arbitration
- Browse content in Company and Commercial Law
- Commercial Law
- Company Law
- Browse content in Comparative Law
- Systems of Law
- Competition Law
- Browse content in Constitutional and Administrative Law
- Government Powers
- Judicial Review
- Local Government Law
- Military and Defence Law
- Parliamentary and Legislative Practice
- Construction Law
- Contract Law
- Browse content in Criminal Law
- Criminal Procedure
- Criminal Evidence Law
- Sentencing and Punishment
- Employment and Labour Law
- Environment and Energy Law
- Browse content in Financial Law
- Banking Law
- Insolvency Law
- History of Law
- Human Rights and Immigration
- Intellectual Property Law
- Browse content in International Law
- Private International Law and Conflict of Laws
- Public International Law
- IT and Communications Law
- Jurisprudence and Philosophy of Law
- Law and Society
- Law and Politics
- Browse content in Legal System and Practice
- Courts and Procedure
- Legal Skills and Practice
- Primary Sources of Law
- Regulation of Legal Profession
- Medical and Healthcare Law
- Browse content in Policing
- Criminal Investigation and Detection
- Police and Security Services
- Police Procedure and Law
- Police Regional Planning
- Browse content in Property Law
- Personal Property Law
- Study and Revision
- Terrorism and National Security Law
- Browse content in Trusts Law
- Wills and Probate or Succession
- Browse content in Medicine and Health
- Browse content in Allied Health Professions
- Arts Therapies
- Clinical Science
- Dietetics and Nutrition
- Occupational Therapy
- Operating Department Practice
- Physiotherapy
- Radiography
- Speech and Language Therapy
- Browse content in Anaesthetics
- General Anaesthesia
- Neuroanaesthesia
- Clinical Neuroscience
- Browse content in Clinical Medicine
- Acute Medicine
- Cardiovascular Medicine
- Clinical Genetics
- Clinical Pharmacology and Therapeutics
- Dermatology
- Endocrinology and Diabetes
- Gastroenterology
- Genito-urinary Medicine
- Geriatric Medicine
- Infectious Diseases
- Medical Oncology
- Medical Toxicology
- Pain Medicine
- Palliative Medicine
- Rehabilitation Medicine
- Respiratory Medicine and Pulmonology
- Rheumatology
- Sleep Medicine
- Sports and Exercise Medicine
- Community Medical Services
- Critical Care
- Emergency Medicine
- Forensic Medicine
- Haematology
- History of Medicine
- Medical Ethics
- Browse content in Medical Skills
- Clinical Skills
- Communication Skills
- Nursing Skills
- Surgical Skills
- Browse content in Medical Dentistry
- Oral and Maxillofacial Surgery
- Paediatric Dentistry
- Restorative Dentistry and Orthodontics
- Surgical Dentistry
- Medical Statistics and Methodology
- Browse content in Neurology
- Clinical Neurophysiology
- Neuropathology
- Nursing Studies
- Browse content in Obstetrics and Gynaecology
- Gynaecology
- Occupational Medicine
- Ophthalmology
- Otolaryngology (ENT)
- Browse content in Paediatrics
- Neonatology
- Browse content in Pathology
- Chemical Pathology
- Clinical Cytogenetics and Molecular Genetics
- Histopathology
- Medical Microbiology and Virology
- Patient Education and Information
- Browse content in Pharmacology
- Psychopharmacology
- Browse content in Popular Health
- Caring for Others
- Complementary and Alternative Medicine
- Self-help and Personal Development
- Browse content in Preclinical Medicine
- Cell Biology
- Molecular Biology and Genetics
- Reproduction, Growth and Development
- Primary Care
- Professional Development in Medicine
- Browse content in Psychiatry
- Addiction Medicine
- Child and Adolescent Psychiatry
- Forensic Psychiatry
- Learning Disabilities
- Old Age Psychiatry
- Psychotherapy
- Browse content in Public Health and Epidemiology
- Epidemiology
- Public Health
- Browse content in Radiology
- Clinical Radiology
- Interventional Radiology
- Nuclear Medicine
- Radiation Oncology
- Reproductive Medicine
- Browse content in Surgery
- Cardiothoracic Surgery
- Gastro-intestinal and Colorectal Surgery
- General Surgery
- Neurosurgery
- Paediatric Surgery
- Peri-operative Care
- Plastic and Reconstructive Surgery
- Surgical Oncology
- Transplant Surgery
- Trauma and Orthopaedic Surgery
- Vascular Surgery
- Browse content in Science and Mathematics
- Browse content in Biological Sciences
- Aquatic Biology
- Biochemistry
- Bioinformatics and Computational Biology
- Developmental Biology
- Ecology and Conservation
- Evolutionary Biology
- Genetics and Genomics
- Microbiology
- Molecular and Cell Biology
- Natural History
- Plant Sciences and Forestry
- Research Methods in Life Sciences
- Structural Biology
- Systems Biology
- Zoology and Animal Sciences
- Browse content in Chemistry
- Analytical Chemistry
- Computational Chemistry
- Crystallography
- Environmental Chemistry
- Industrial Chemistry
- Inorganic Chemistry
- Materials Chemistry
- Medicinal Chemistry
- Mineralogy and Gems
- Organic Chemistry
- Physical Chemistry
- Polymer Chemistry
- Study and Communication Skills in Chemistry
- Theoretical Chemistry
- Browse content in Computer Science
- Artificial Intelligence
- Computer Architecture and Logic Design
- Game Studies
- Human-Computer Interaction
- Mathematical Theory of Computation
- Programming Languages
- Software Engineering
- Systems Analysis and Design
- Virtual Reality
- Browse content in Computing
- Business Applications
- Computer Games
- Computer Security
- Computer Networking and Communications
- Digital Lifestyle
- Graphical and Digital Media Applications
- Operating Systems
- Browse content in Earth Sciences and Geography
- Atmospheric Sciences
- Environmental Geography
- Geology and the Lithosphere
- Maps and Map-making
- Meteorology and Climatology
- Oceanography and Hydrology
- Palaeontology
- Physical Geography and Topography
- Regional Geography
- Soil Science
- Urban Geography
- Browse content in Engineering and Technology
- Agriculture and Farming
- Biological Engineering
- Civil Engineering, Surveying, and Building
- Electronics and Communications Engineering
- Energy Technology
- Engineering (General)
- Environmental Science, Engineering, and Technology
- History of Engineering and Technology
- Mechanical Engineering and Materials
- Technology of Industrial Chemistry
- Transport Technology and Trades
- Browse content in Environmental Science
- Applied Ecology (Environmental Science)
- Conservation of the Environment (Environmental Science)
- Environmental Sustainability
- Environmentalist Thought and Ideology (Environmental Science)
- Management of Land and Natural Resources (Environmental Science)
- Natural Disasters (Environmental Science)
- Nuclear Issues (Environmental Science)
- Pollution and Threats to the Environment (Environmental Science)
- Social Impact of Environmental Issues (Environmental Science)
- History of Science and Technology
- Browse content in Materials Science
- Ceramics and Glasses
- Composite Materials
- Metals, Alloying, and Corrosion
- Nanotechnology
- Browse content in Mathematics
- Applied Mathematics
- Biomathematics and Statistics
- History of Mathematics
- Mathematical Education
- Mathematical Finance
- Mathematical Analysis
- Numerical and Computational Mathematics
- Probability and Statistics
- Pure Mathematics
- Browse content in Neuroscience
- Cognition and Behavioural Neuroscience
- Development of the Nervous System
- Disorders of the Nervous System
- History of Neuroscience
- Invertebrate Neurobiology
- Molecular and Cellular Systems
- Neuroendocrinology and Autonomic Nervous System
- Neuroscientific Techniques
- Sensory and Motor Systems
- Browse content in Physics
- Astronomy and Astrophysics
- Atomic, Molecular, and Optical Physics
- Biological and Medical Physics
- Classical Mechanics
- Computational Physics
- Condensed Matter Physics
- Electromagnetism, Optics, and Acoustics
- History of Physics
- Mathematical and Statistical Physics
- Measurement Science
- Nuclear Physics
- Particles and Fields
- Plasma Physics
- Quantum Physics
- Relativity and Gravitation
- Semiconductor and Mesoscopic Physics
- Browse content in Psychology
- Affective Sciences
- Clinical Psychology
- Cognitive Neuroscience
- Cognitive Psychology
- Criminal and Forensic Psychology
- Developmental Psychology
- Educational Psychology
- Evolutionary Psychology
- Health Psychology
- History and Systems in Psychology
- Music Psychology
- Neuropsychology
- Organizational Psychology
- Psychological Assessment and Testing
- Psychology of Human-Technology Interaction
- Psychology Professional Development and Training
- Research Methods in Psychology
- Social Psychology
- Browse content in Social Sciences
- Browse content in Anthropology
- Anthropology of Religion
- Human Evolution
- Medical Anthropology
- Physical Anthropology
- Regional Anthropology
- Social and Cultural Anthropology
- Theory and Practice of Anthropology
- Browse content in Business and Management
- Business History
- Business Ethics
- Business Strategy
- Business and Technology
- Business and Government
- Business and the Environment
- Comparative Management
- Corporate Governance
- Corporate Social Responsibility
- Entrepreneurship
- Health Management
- Human Resource Management
- Industrial and Employment Relations
- Industry Studies
- Information and Communication Technologies
- International Business
- Knowledge Management
- Management and Management Techniques
- Operations Management
- Organizational Theory and Behaviour
- Pensions and Pension Management
- Public and Nonprofit Management
- Strategic Management
- Supply Chain Management
- Browse content in Criminology and Criminal Justice
- Criminal Justice
- Criminology
- Forms of Crime
- International and Comparative Criminology
- Youth Violence and Juvenile Justice
- Development Studies
- Browse content in Economics
- Agricultural, Environmental, and Natural Resource Economics
- Asian Economics
- Behavioural Finance
- Behavioural Economics and Neuroeconomics
- Econometrics and Mathematical Economics
- Economic Methodology
- Economic History
- Economic Systems
- Economic Development and Growth
- Financial Markets
- Financial Institutions and Services
- General Economics and Teaching
- Health, Education, and Welfare
- History of Economic Thought
- International Economics
- Labour and Demographic Economics
- Law and Economics
- Macroeconomics and Monetary Economics
- Microeconomics
- Public Economics
- Urban, Rural, and Regional Economics
- Welfare Economics
- Browse content in Education
- Adult Education and Continuous Learning
- Care and Counselling of Students
- Early Childhood and Elementary Education
- Educational Equipment and Technology
- Educational Strategies and Policy
- Higher and Further Education
- Organization and Management of Education
- Philosophy and Theory of Education
- Schools Studies
- Secondary Education
- Teaching of a Specific Subject
- Teaching of Specific Groups and Special Educational Needs
- Teaching Skills and Techniques
- Browse content in Environment
- Applied Ecology (Social Science)
- Climate Change
- Conservation of the Environment (Social Science)
- Environmentalist Thought and Ideology (Social Science)
- Natural Disasters (Environment)
- Social Impact of Environmental Issues (Social Science)
- Browse content in Human Geography
- Cultural Geography
- Economic Geography
- Political Geography
- Browse content in Interdisciplinary Studies
- Communication Studies
- Museums, Libraries, and Information Sciences
- Browse content in Politics
- African Politics
- Asian Politics
- Chinese Politics
- Comparative Politics
- Conflict Politics
- Elections and Electoral Studies
- Environmental Politics
- European Union
- Foreign Policy
- Gender and Politics
- Human Rights and Politics
- Indian Politics
- International Relations
- International Organization (Politics)
- International Political Economy
- Irish Politics
- Latin American Politics
- Middle Eastern Politics
- Political Theory
- Political Behaviour
- Political Economy
- Political Institutions
- Political Methodology
- Political Communication
- Political Philosophy
- Political Sociology
- Politics and Law
- Politics of Development
- Public Policy
- Public Administration
- Quantitative Political Methodology
- Regional Political Studies
- Russian Politics
- Security Studies
- State and Local Government
- UK Politics
- US Politics
- Browse content in Regional and Area Studies
- African Studies
- Asian Studies
- East Asian Studies
- Japanese Studies
- Latin American Studies
- Middle Eastern Studies
- Native American Studies
- Scottish Studies
- Browse content in Research and Information
- Research Methods
- Browse content in Social Work
- Addictions and Substance Misuse
- Adoption and Fostering
- Care of the Elderly
- Child and Adolescent Social Work
- Couple and Family Social Work
- Direct Practice and Clinical Social Work
- Emergency Services
- Human Behaviour and the Social Environment
- International and Global Issues in Social Work
- Mental and Behavioural Health
- Social Justice and Human Rights
- Social Policy and Advocacy
- Social Work and Crime and Justice
- Social Work Macro Practice
- Social Work Practice Settings
- Social Work Research and Evidence-based Practice
- Welfare and Benefit Systems
- Browse content in Sociology
- Childhood Studies
- Community Development
- Comparative and Historical Sociology
- Economic Sociology
- Gender and Sexuality
- Gerontology and Ageing
- Health, Illness, and Medicine
- Marriage and the Family
- Migration Studies
- Occupations, Professions, and Work
- Organizations
- Population and Demography
- Race and Ethnicity
- Social Theory
- Social Movements and Social Change
- Social Research and Statistics
- Social Stratification, Inequality, and Mobility
- Sociology of Religion
- Sociology of Education
- Sport and Leisure
- Urban and Rural Studies
- Browse content in Warfare and Defence
- Defence Strategy, Planning, and Research
- Land Forces and Warfare
- Military Administration
- Military Life and Institutions
- Naval Forces and Warfare
- Other Warfare and Defence Issues
- Peace Studies and Conflict Resolution
- Weapons and Equipment

- < Previous chapter
- Next chapter >
37CHAPTER 2 What Was Revolutionary about the Chemical Revolution?
- Published: July 2016
- Cite Icon Cite
- Permissions Icon Permissions
THE CHANGES TO CHEMICAL theory and practice that took place in late eighteenth-century France were truly revolutionary because of the radical nature of the theoretical and methodological changes that occurred, because they were deliberately so, and because that was the start of a tradition in the philosophy of chemistry. What makes the Chemical Revolution unique among scientific revolutions is that it was anticipated by both philosophers and scientists before it occurred. This meant that the chemists who effected those changes were aware of the subversive nature of their reforms and carried out the revolution in a deliberate fashion. Three major shifts in the science of chemistry coincided in late eighteenth-century France to make the Chemical Revolution the turning point in the history of chemistry: Oxygen chemistry overthrew the reigning phlogiston theory; a cadre of prominently political chemists reformed chemical terminology, providing a new system of names based on oxygen theory; and an empiricopragmatic conception of elements as simple substances replaced a waning belief in hypostatical chemical principles. This last shift (although itself gradual) ensured that the revolutionary changes in theory and nomenclature would be the last truly radical reforms chemistry would ever need. Furthermore, the Chemical Revolution was itself a revolution in the philosophy of chemistry as it forced a change in tacit assumptions about the nature of both matter and scientific knowledge. Moreover, studies of this revolution have long shaped general philosophy of science and continue to do so. Cherry-picking the history of science for examples to fit an a priori philosophical theory should be even less acceptable in philosophy of the special sciences than in other branches of philosophy. If philosophers of science are to learn from history, it should be by analyzing changes within periods that historians recognize as revolutionary and giving a philosophical account. Hence the Chemical Revolution is a crucial point for even the most minimally naturalistic philosophy of chemistry. For some time now, historians of science have understood that the chemistry practiced before the 1770s cannot be dismissed as prescientific mysticism, as was once supposed.
Signed in as
Institutional accounts.
- GoogleCrawler [DO NOT DELETE]
- Google Scholar Indexing
Personal account
- Sign in with email/username & password
- Get email alerts
- Save searches
- Purchase content
- Activate your purchase/trial code
- Add your ORCID iD
Institutional access
Sign in with a library card.
- Sign in with username/password
- Recommend to your librarian
- Institutional account management
- Get help with access
Access to content on Oxford Academic is often provided through institutional subscriptions and purchases. If you are a member of an institution with an active account, you may be able to access content in one of the following ways:
IP based access
Typically, access is provided across an institutional network to a range of IP addresses. This authentication occurs automatically, and it is not possible to sign out of an IP authenticated account.
Choose this option to get remote access when outside your institution. Shibboleth/Open Athens technology is used to provide single sign-on between your institution’s website and Oxford Academic.
- Click Sign in through your institution.
- Select your institution from the list provided, which will take you to your institution's website to sign in.
- When on the institution site, please use the credentials provided by your institution. Do not use an Oxford Academic personal account.
- Following successful sign in, you will be returned to Oxford Academic.
If your institution is not listed or you cannot sign in to your institution’s website, please contact your librarian or administrator.
Enter your library card number to sign in. If you cannot sign in, please contact your librarian.
Society Members
Society member access to a journal is achieved in one of the following ways:
Sign in through society site
Many societies offer single sign-on between the society website and Oxford Academic. If you see ‘Sign in through society site’ in the sign in pane within a journal:
- Click Sign in through society site.
- When on the society site, please use the credentials provided by that society. Do not use an Oxford Academic personal account.
If you do not have a society account or have forgotten your username or password, please contact your society.
Sign in using a personal account
Some societies use Oxford Academic personal accounts to provide access to their members. See below.
A personal account can be used to get email alerts, save searches, purchase content, and activate subscriptions.
Some societies use Oxford Academic personal accounts to provide access to their members.
Viewing your signed in accounts
Click the account icon in the top right to:
- View your signed in personal account and access account management features.
- View the institutional accounts that are providing access.
Signed in but can't access content
Oxford Academic is home to a wide variety of products. The institutional subscription may not cover the content that you are trying to access. If you believe you should have access to that content, please contact your librarian.
For librarians and administrators, your personal account also provides access to institutional account management. Here you will find options to view and activate subscriptions, manage institutional settings and access options, access usage statistics, and more.
Our books are available by subscription or purchase to libraries and institutions.
- About Oxford Academic
- Publish journals with us
- University press partners
- What we publish
- New features
- Open access
- Rights and permissions
- Accessibility
- Advertising
- Media enquiries
- Oxford University Press
- Oxford Languages
- University of Oxford
Oxford University Press is a department of the University of Oxford. It furthers the University's objective of excellence in research, scholarship, and education by publishing worldwide
- Copyright © 2024 Oxford University Press
- Cookie settings
- Cookie policy
- Privacy policy
- Legal notice
This Feature Is Available To Subscribers Only
Sign In or Create an Account
This PDF is available to Subscribers Only
For full access to this pdf, sign in to an existing account, or purchase an annual subscription.
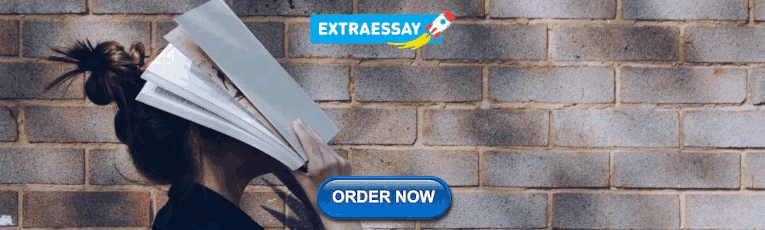
Why Is Chemistry Important?
Real Answers About Chemistry From Real People
Hero Images / Getty Images
- Chemical Laws
- Periodic Table
- Projects & Experiments
- Scientific Method
- Biochemistry
- Physical Chemistry
- Medical Chemistry
- Chemistry In Everyday Life
- Famous Chemists
- Activities for Kids
- Abbreviations & Acronyms
- Weather & Climate
- Ph.D., Biomedical Sciences, University of Tennessee at Knoxville
- B.A., Physics and Mathematics, Hastings College
Why is chemistry important? If you take chemistry or teach chemistry, you'll be asked to answer this question pretty often. It's easy to say chemistry is important because everything is made from chemicals but there are a lot of other reasons why chemistry is a big part of daily life and why everyone should understand basic chemistry. If you've ever wondered about the question yourself, this selection of answers from real chemists , teachers, students, and readers just like you will give you an idea of the many reasons why chemistry is so vital to our lives.
We Are Chemical Beings: Many biology and anatomy and physiology courses begin with chemistry. More than just nutrients, medicines and poisons, everything we do is chemical. Geology too: why do we wear diamonds and not calcium carbonate on our fingers? —foxkin
Importance of Chemistry to Life: (1) Many thing that are our in environment are made of chemicals. (2) Many things that we observe in the world are made of chemical effects. —Shola
Well, now you have asked something. My first days of chemistry started at the age of about 9 not long after WWII. Since then, I have received from the study a vast interest in everything and still I am learning at 70 years old—but in my mind I know it is chemistry that has made me what I am and what I believe in, to myself it is the most powerful mind mover of all...making one's mind explore and discover and understand what it's all about. I am still looking, experimenting, and wondering. Yes, to [me] chemistry is the all-powerful mover and doer of the whole mystery of life and the meanings. But sadly I can no longer explore the underground I so loved to in search for the Philosopher's stone .—David Bradbury
Prevents Poisoning or Worse: Water or sulfuric acid ? Propylene glycol or ethylene glycol? It's good to be able to tell them apart. Chemistry is important because it helps you identify toxic or dangerous substances. Of course, labeling your chemicals helps a lot too. —Gemdragon
Chemistry [has] great importance in our daily life... In our [bodies,] chemical reactions are going on. With the help of chemistry, we are able to cure most deadly or dangerous diseases. By the study of chemistry, we can learn the biochemical changes going on in our body. —Sneha Jadhao
Chemistry is a path of creativity, at least to me. It's a subject of logic and it creates a new way of thinking... Organic is like a puzzle which is very interesting to solve and bonding is just awesome. Chemistry is a study of life. Life is made of a string of particulate matter. —Dr. C. W. Huey
Because chemistry is all over in world & girls are impressed by this subject. —yog
Chemistry Means a Lot of Dollars: If you want a lot of dollars you must learn chemistry. —emad
Witchcraft: In Africa, we believe chemistry explains witchcraft [and that which is] responsible for the manufacture of the concoctions used in the art. —Patrick Chege
Chemistry is important as it is interrelated with many sciences such as physics biology, etc. —ANAS
Life is Made up of Chemistry: For me, chemistry is very interesting because I feel by learning it we can understand the other sciences too. My specialization is in analytical [chemistry.] This tells us about nutritional values, specimen analysis, toxicity, sampling, and so many valuable things. So chem is around us and inside us. Moreover, with today's instrumentation and with the help of a large variety of chemical measurements available, we can get the results of clinical, environmental, occupational health, safety applications, and industrial analysis. —Irfana Aamir
It is very important. Chemistry applies in every field of life. Education in chemistry is not only the source of getting a good job but also a fun and practical way to make life interesting. —sony
It's in Everything: ELECTRONS RULE!! Chemistry pervades all processes from air particulate to cellular specialized functions to the engineering materials for space exploration. We are Chemistry! —MJ
Paint Pigments: If it weren't for chemists, we wouldn't have all the modern pigments for paints we have today (including my long-time favorite Prussian blue, although the color maker was trying to make a red)! —Marion BE
Chemistry is important because everything around us is made of chemicals. —ntosh
Everything is chemistry so nothing can exist without chemistry. —Guest superchem
Answer: Everything in the world is basically now made up of chemistry. —Madelyn
Interactions Are Fun to Learn: Studying chemistry is not all about observing any reactions and recording the result. It's about knowing why they are able to react like that. It is really fascinating and an exercise for our brain. —Kate Williams
Why is Chemistry Important? As the earth originated, chemistry also began to play an important role... Life... began because of chemicals. Chemistry is everywhere. It is important to know it and to sustain life on Earth peacefully. Because of all these reasons humans [have become] more interested and given more importance to it. The mystery of chemistry is always taunting man to reveal its mystery. —Megha
Why is Chemistry Important in Our Society? Chemistry is important because it helps to build our body system. It helps us in our daily activities... and is also important because it helps us to know how to take good care of our health. —Ani Samuel
Chemistry plays a central role in science and is often entwined with other branches of science like physics, biology, geology etc. —Radhi R.
Chemistry = Daily Life: Chemistry is a branch of science which deals with the study of everything in our daily life. Chemistry is nonstop because it is spread in our daily life. —a7h
Chem is Life: Chemistry deals with the composition of things, from the food we eat, the rocks and the minerals, the mattresses we sleep on, etc. —Saha Aboo
Chemistry is Life Science: Chemistry is a science that is very close to human, non-human life and nonliving matters. It is essential to learn chemistry because of man's desire to improve medical solutions to the challenges of newly discovered ailments. —Peter Chiti
[When you add one chemical to another chemical, can have a violent reaction. For example, take water and add it to acid and see what a violent reaction you get as the two mix, resulting in the release of thermal energy and vapor. For this reason, it is important to know chemical properties and compounds.] —Kallie
[Chemistry helps our industry produce more materials—such as paints, plastics, iron or steel, cement, kerosene, and also motor oil. Chemistry also helps farmers to enrich the soil with chemicals ... to grow fresh vegetables.] —~gRatItUdEgIrL25~
Chemistry is important, especially in household things like condoms, cleaning, and cooking. —Cougar
Chemistry is Essential! In just one line we can say that the importance of chemistry is unparalleled and the scope of chemistry is unlimited. The importance of chemistry can't be pinned down with [a few] examples! We can lead a better life with chemistry. —Swathi P.S.
No Life Without Chemistry: Without chemistry no life for human beings... Chemistry is the God for all other subjects. —Sarandeva
Chemistry is important because everything around us is made up of chemicals and we use it in our day-to-day activities—in our house, industry, companies, etc. —Immanuel Abiola
Chemistry is the Universe: It is said that chemistry is the knowledge of observing this universe. And in our holy Quran, Allah Almighty said that "intelligent is that person who observes this universe." That's all about chemistry. —amin_malik
About Chemistry: [Chemistry is important as it makes us aware of the small secrets of our environment around us. By studying chemistry, we are able to know the basic mechanisms in our body in our daily life.] —Mrinal Mukesh
Learning of chemistry is important so as to get [good grades] in the examination. —nishant
Fish in Water: [Talking of chemistry in human life is like "a fish, deep inside the River Ganga, speaking of what Water is." Since the beginning of a body, until it vanishes in fire or soil, it's chemistry and chemistry. Think about it and understand.] —Bira Madhab
What we use in our everyday life that is made by different chemicals, so chemistry is very important for us. —jiten
Importance of Chemistry: Environmental chemistry describes various chemical elements present in the environment their reactions and effects on the environment. It illustrates the major environmental segments and their interrelationship and significances. —Aminul
Chemistry in Use 24 X 7: When we wake up we brush our teeth with toothpaste which is chemistry, then we bathe with soap ( alkaline ), we eat our food (vitamins, minerals, water, folic acid), we go to work by vehicles which feed on petrol... We ward off mosquitoes with repellents which is chemistry! —Prandeep Borthakur
Chemistry: It is important because it helps us to be more productive and develop our country. —Encarnacion
It's a Blessing: [I think chemistry is very important for our lives and for our existence. If there were no chemical reactions , then there would be no air—no air means no life, no life means no existence, and no existence means nothing living.] —Summa
Question: What is a chemical element ? Answer: A chemical element, or an element, is a material which cannot be broken down or changed into another substance using chemical means. Elements may be thought of as the basic chemical building blocks of matter. Depending on how much evidence you require to prove a new element has been created, there are 117 or 118 known elements. —Guest
The importance of chemistry won't be diminished over time, so it will remain a promising career path. —Important
[I think chemistry is important to our life. Look around us—the drugs, weedkiller, and food come from chemistry.] —Osei Stephen
Why is Chemistry Important in Life? I think without chemistry, one can not imagine his life. Chemistry is as important as food. —Dimple Sharma
Health: [If not for chemistry, by now, the world will not exist. Chemists all around the world through rigorous research have saved us, in term of health.] —Ajileye
Importance of Chemistry: Besides considering ‘what chemistry is and what one has in mind when he/she thinks of chemistry,’ the essence of importance of chemistry is hidden in the quintessence that it is not only the central science but also the mother of sciences and it is the mother that matters the most in every aspect and all respects. —Dr. Badruddin Khan
Why is Chemistry Important? The food we eat, air we breathe, water we drink—everything is made up of chemicals. Life can't exist without chemistry. —nag
What is Chemistry? [According to me, we can define chemistry as follows: C -creates H -hell or heaven on E -earth M -mysteriously I -investingly and S -surprisingly T -through R -reactions and its Y -yields.] —Sridevi
Though chemistry is difficult to learn, it is very important to learn it. The major advantage is in the medicinal field. —Shefali
It's Important: It doesn't take a chemistry major to know that some chemicals are dangerous. Having a basic knowledge chemistry can help you avoid materials that you would rather not come in contact with. That's why they put a list of ingredients on everything at the supermarket. —Blake
From morning to evening anything and everything we use is the product of chemistry. —Chandini Anand
Importance of Chemistry: Chemistry aids in the improvement of healthcare, the conservation of natural resources, and the protection of the environment. Chemistry is the central science, central to the understanding of other sciences and technology. —OhHowThisGenerationHasFallen
[Learning chemistry is important if you want to pass your chemistry exams .] —Keerthy
Definition of Chemistry: [In Hindi the word for chemistry is rasayan so chemistry gives us the ras of a subject. When we wake up, when we look at anything, that thing is made by chemicals and when we go to sleep, the bedsheet also is made by use of chemistry. Around us everywhere is chemistry so chemistry is an important subject. It carries us to success. I like chemistry very much.] —Aditya Dwivedi
Chemistry is so important because it has to do with everything in our daily lives. Chemistry just makes us understand how everything works a little better. For example, why a certain pain reliever works more than the other, or why you need oil to fry chicken. All this—believe it or not—is possible because of the study of chemistry. —Joselitop
Chemistry in Our Life: Chemistry is the most important thing in our lives. Everything that we use—from the toothbrush in the morning to the food we eat to the road we travel by and the books we read—are all there due to chemistry and that is why it is very important in our day-to-day life. —Priya
Science Student: [Chemistry is important to study because, in our day-to-day activities, chemistry shows us how we can manage things. For example, take the food we eat—chemistry explains how we can eat to a time table in such a way that it will suit our bodies. If not for the knowledge of chemistry, there would be no medicines. Chemistry also provides knowledge about how to produce many things for commercial purposes as well.] —Wuese Daniel
Why is Chemistry Important? Because everything is made of the chemicals which are needed in our daily life. We can not live without chemistry. —Liton
Kitchen Chemistry: Everything in the kitchen is chemistry. Mixing of substances is chemistry. —Abby Sams
Importance of Chemistry: Chemistry creates an atmosphere of understanding how and what our most precious world is made of. Everything is made up of multiples of infinitesimal atoms closely packed together to give us one whole product. Moreover, it elaborates on how different chemicals react with each other. Therefore, it is clear that chemistry is everywhere at any time! —Manqoba Mthabela
Uses of Chemistry: Chemistry is useful in all areas of life. You need chemistry to know how your cooking gas is been produced and even the name. You still need it to know the chemical process that is occurring in your cooking and even in your environment. Chemistry is essential to life. —Bimbim
Chemistry is important because it is the source of human activities. —Gift.21
- Examples of Chemical Reactions in Everyday Life
- Chemistry Pick Up Lines to Try on Your Crush
- Why Is Chemistry Important in Everyday Life?
- What Is the Importance of Chemistry?
- 10 Things You Need To Know About Chemistry
- Why Study Chemistry?
- 8 Reasons Why Chemical Reactions Are Important
- What Is Chemistry? Definition and Description
- Organic Chemistry Introduction
- Teach Yourself Chemistry Today
- Chemistry in Daily Life
- What Chemistry Is and What Chemists Do
- College Chemistry Topics
- What Is a Chemical and What Isn't a Chemical?
- What Is a Word Equation in Chemistry?
- Chemistry 101 - Introduction & Index of Topics
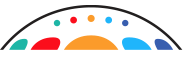
Image source: Geoff Hutchison / Flickr
- Technology & the future
What has chemistry ever done for you?
From batteries to painkillers, chemistry is essential to our modern lives.
Expert reviewers
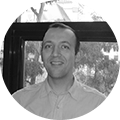
Dr Oliver Jones
Senior Lecturer in Analytical Chemistry
RMIT University
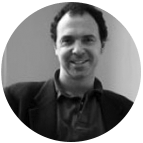
Professor Paul Mulvaney
Professor of Chemistry
School of Chemistry and Bio21 Institute, University of Melbourne
- Chemistry is a fundamental and enabling science that investigates molecules—the building blocks of all matter—and how they interact to affect the composition, structure and properties of substances.
- The industrial applications of chemistry directly affect our daily lives—what we eat, what we wear, our transport, the technology we use, how we treat illnesses and how we get electricity—to name just a few.
- Research is constantly deepening our understanding of chemistry, and leading to new discoveries.
- Chemistry will help us solve many future problems, including sustainable energy and food production, managing our environment, providing safe drinking water and promoting human and environmental health.
To many, chemistry is a foreign concept, belonging to the world of academia and textbooks with little relevance to our everyday life. In fact, you would be hard-pressed to find an aspect of your daily routine that isn’t directly impacted by chemistry research.
Chemistry is the study of molecules: the building blocks of matter. It is central to our existence, and leads our investigations into the human body, Earth, food, materials, energy, and anywhere and everywhere in between. The chemical industry, supported by chemistry research, underpins much of our economic progress, and provides wealth and prosperity for society. In Australia, 60,000 people are employed by the chemical industry and it contributes around $11.6 billion annually to our GDP.
What follows is a snapshot—just a small sample—of the major discoveries in chemistry that have helped to shape the way we live. From the first instances of metal work in the Copper Age in 5000 BC, to the digital era and the new cutting-edge technologies of today, such as nanoscience and biotechnology, chemists have more often than not been the driving force behind the progress in our standard of living.
Chemical theory has been developing since well before ‘chemist’ became a possible career choice. The phenomenon of fire was one of the first marvels that humankind sought to understand and the use of fire led to the study and manipulation of metals. This dates back to 5000 BC, when copper was first discovered and replaced stone as a material for making tools. It was obtained by a process called smelting GLOSSARY smelting extracting metal from ore (a mixture of rock and metal) by heating and melting , and was thought to also have produced the first glass as a side product.
The Bronze Age arose when it was discovered that copper could be combined with tin to produce a harder metal—you guessed it, bronze. This was the first alloy GLOSSARY alloy a mixture of metal with other metals or non-metals. Common alloys include steel, brass and pewter. They are often cheaper than elemental metals, with many similar or enhanced properties. ever made and resulted in stronger weapons and tools. The trading of these tools facilitated sharing of technology and knowledge between early civilisations. The Iron Age, following at around 1200 BC, saw the prevalence of iron increase as the major metal of choice for cutting tools and weapons. Iron was slower to evolve as a material because it required higher temperatures to work the metal. This shift saw a change in smelting practices, improved furnace technologies, and also the development of forging GLOSSARY forging shaping of metal using compressive forces, such as hammering , as opposed to the casting GLOSSARY casting Hot liquid metal is poured into a hollow cavity, and cooled in order to solidify it into a desired shape. techniques used in the Bronze Age.
.png)
Materials and manufacturing
The Iron Age also saw the development of many of the basic elements of urban development we are familiar with today such as cements, mortars and bitumen. During this period, populations became increasingly urbanised in large cities leading to construction of the first proper roads.
Around 500 years ago, chemistry became an earnest occupation. Elements other than naturally occurring metals were being identified and their properties were being explored—though they were still not entirely understood. People didn’t yet have much of an appreciation of the underlying science that determined material properties and it was unclear how many different basic or elemental building blocks there were.
Another important development was that of vulcanised rubber GLOSSARY vulcanised rubber hardened, more durable rubber, obtained by adding sulfur at high temperatures , in 1843 by Charles Goodyear. This led to pneumatic GLOSSARY pneumatic containing a cavity of air; operated by gas or air under pressure tyres and kicked off the polymer and plastics industries, which would later revolutionise the manufacture of household goods. The discovery by Alfred Nobel of dynamite in 1867 and more refined explosives later on led to rapid expansion of mining as a means to extract ores and minerals.
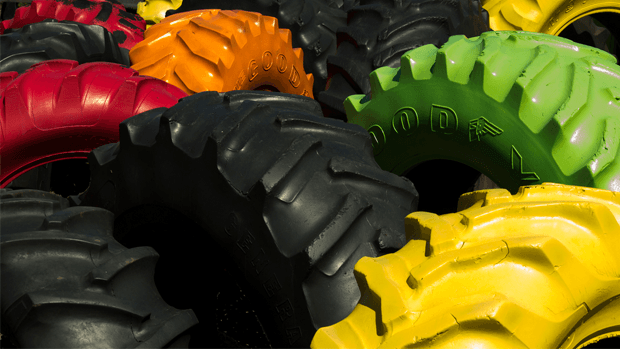
The synthesis of the first artificial dye, a purple colour later named mauveine, occurred in 1856. It was an accidental discovery made by William Perkin, an 18-year-old who was actually trying to make artificial quinine. Blue and purple pigments were historically incredibly rare, and mauveine was met with high demand. Its development drove further investigation into organic chemistry , and the production of related dyes and pigments. Some of the world’s biggest organic chemistry companies today were founded around this time due to demand for dye production.
Despite the increasing use of chemical compounds, it wasn’t until 1870 that Dimitri Mendeleev came up with a systematic way to arrange all the known chemical elements into the periodic table . The table is based on common chemical properties and trends in their behaviour. It is a concise, information-dense catalogue of all of the known different types of atoms, and it is still an essential tool for chemistry study today.
More recently, Harold Kroto, Richard Smalley, James Heath, Sean O'Brien and Robert Curl at Rice University made a new discovery regarding something we thought we thoroughly understood—they discovered a new form of carbon. Kroto, Curl and Smalley were later awarded the 1996 Nobel Prize in Chemistry for discovering fullerenes , a completely new arrangement of carbon atoms, forming ball-shaped cage-like structures. These have been useful in materials design and could have a number of biomedical applications.
This field of research has also led to the development of carbon nanotubes. Carbon nanotubes are used to create ultra-strong, lightweight materials, for use in aircraft for example.
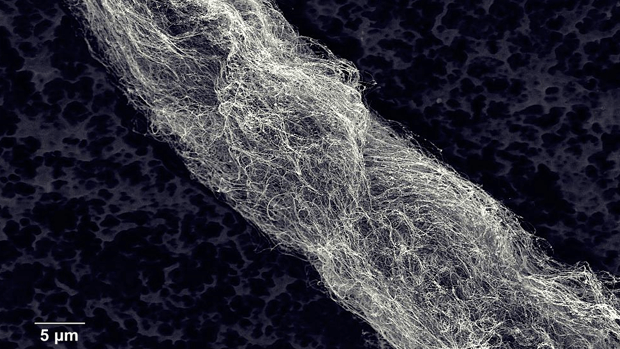
Another form of carbon that has unique properties is graphene. Graphene is a sheet made of a single layer of carbon atoms, and although a single layer of atoms may sound extremely flimsy, it’s actually extremely tough, 200 times stronger than steel, ultra-light, flexible, and an excellent conductor. Although scientists knew for quite a while that graphite was made of sheets of carbon atoms, it was only in 2004 that Professors Andre Geim and Kostya Novoselov were able to isolate a single layer on its own to make graphene. Graphene isn’t yet as ubiquitous in our everyday lives as some other great chemical discoveries—with this one, it’s more of a case of ‘What will graphene do for you in the future?’ With its unique properties, it has the potential to have a huge impact in several areas , including electronics, materials, energy technology and biomedical applications.
One of the key contributions the field of chemistry has provided to our burgeoning society is the ability to harness and store electrical energy—electricity. Electricity had long been an intellectual curiosity, and the phenomena became more understood through experiments by chemists and physicists.
Traditional energy production, via combustion and thermodynamics of fossil fuels, led to the industrial revolution. This boom in industry from the mid-1700s to the 1800s was an era of growth, with chemical engineers coming to the fore to scale up and industrialise processes of manufacture. It was during this time that many of the practical uses of chemistry we rely on today were developed.
The batteries that so many of our devices depend upon are underpinned by a chemical reaction that produces electricity. The first electrical battery was created by Alessandro Volta, who proved that electricity flowed through wires attached to different metals and the types of metal used affected the voltage. The term ‘volt’ as a measure of electric potential is named in honour of him. Although current batteries are far more sophisticated than in Volta’s day, there is renewed interest in pushing this vital chemical technology further , so that sustainable energy produced by solar cells or wind energy can be stored.
Food and agriculture
Complex technology goes into modern food production. From soil science to nutrition analysis, and from safety testing to food packaging and preservation, the chemical processes involved are extensive, and often not given much consideration. For example, if it wasn’t for refrigeration, our food distribution systems would be limited and storage would be short lived. The first cooling systems were developed in 1874. They used dimethyl ether, but it wasn’t long before ammonia-based systems were introduced, which are still in use today in industrial refrigerators.
Ammonia is also an integral chemical innovation for food production, chiefly due to its use in fertiliser production. Indeed it is estimated that around 1 per cent of the world’s energy is used to make ammonia. Increasing the productivity of our food-growing systems has become necessary due to the combined pressures of population increase, climate change and water shortages. If it wasn't for the Haber-Bosch process, our current agricultural output would be unsustainable. It was first developed in 1909, and allows for the efficient, large-scale production of ammonia (NH 3 ) by reacting atmospheric nitrogen (N 2 ) with hydrogen (H 2 ) at high temperature and pressure. This resulted in an easily accessible route to fertiliser production, and was responsible for quadrupling agricultural productivity. The discovery of pesticides and herbicides further increased crop yields, with DDT and glyphosate being key compounds. Today, around 40–60 per cent of global agriculture yields rely on artificial fertilisers.
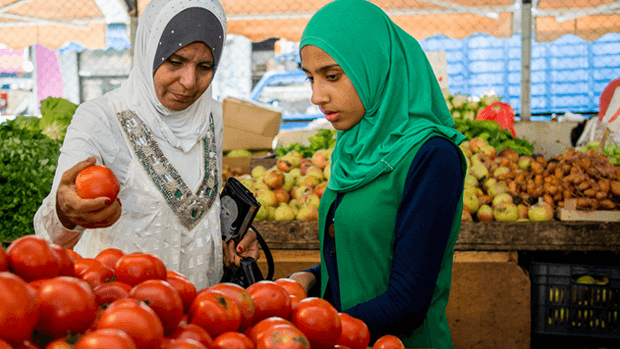
Human populations the world over rely on chemistry to maintain safe clean water supplies. Recycling will be essential for sustaining this resource into the future. Here in Australia, droughts forced us over the past decade to reduce our water use and reconsider our reliance on dams and reservoirs, and think about alternative water sources. There are already three large water desalination plants, in Sydney, Melbourne and Perth. Without this development in chemical engineering, nations including Saudi Arabia, Kuwait, the United Arab Emirates, Bahrain and Libya would most likely not have enough usable water to sustain their current populations. Effective resource management is becoming more important as we face environmental uncertainty , with chemistry playing a crucial role in potential solutions.
Modern health care is founded on many life-saving breakthroughs the field of chemistry has provided. These include developing new pharmaceuticals, diagnostic tools and better diagnostic equipment such as X-ray machines, MRI imaging, cancer tests and pregnancy kits. Analytical chemistry and forensic science are crucial for identifying poisons or toxins in food, plants and animals, and in tracing and identifying unknown chemicals and materials.
Medical practices have also drastically changed as chemical knowledge has advanced. The discovery of painkillers and anaesthetics opened up a whole new scope of opportunity for medical practitioners. Advanced surgery (rather than simple amputation) became possible. Compounds such as nitrous oxide GLOSSARY nitrous oxide a sedative agent also known as laughing gas, nitrous, nitro or NOS (N 2 O), or laughing gas, became popular and minor surgical procedures and dental work became slightly less risky, although infection was still a major challenge. Here, chemistry came to the rescue (again!) with the first antiseptics. In 1867, Joseph Lister introduced carbolic acid as an antiseptic to clean surgery wounds . Death rates in his surgery dropped from 45.7 per cent to 15 per cent.
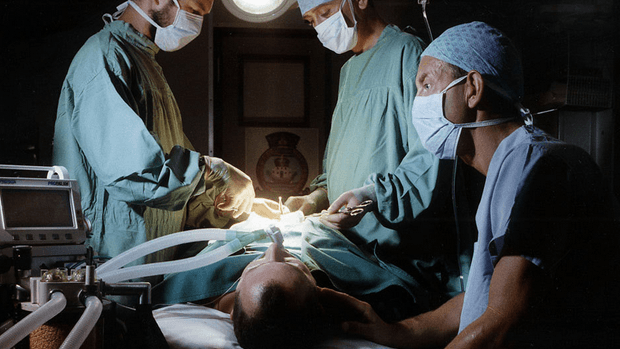
Still on this front, but somewhat later, Alexander Fleming discovered the first antibiotic, penicillin, in 1928. This discovery ushered in a whole new era in fighting bacteria-borne illness. It wasn’t until the 1940s however, when Howard Florey, a scientist from Adelaide, manufactured penicillin on a large scale that it was adopted for widespread use. His work resulted in the easy treatment of infection, and has also saved millions of lives. But the microbes have begun to fight back, meaning our days of simply solved infections may soon be behind us. Due to the ever-increasing prevalence of antimicrobial resistance , further work in this field of chemistry is more important than ever.
Marie Curie was the first woman to receive a Nobel Prize, and the first person to receive two Nobel Prizes and to this day is one of only two people to hold two in two different, scientific fields (physics and chemistry). She is an important icon for science and in particular for chemistry, as her work in the discovery of radioactive elements GLOSSARY radioactive elements unstable elements that decay with time. They have no stable naturally occurring form. provided the base work for innovations in X-ray imaging, nuclear power and radiotherapy.
In 1953, Francis Crick and James Watson published the structure of and mechanisms of DNA, which built largely on the work of Rosalind Franklin and Maurice Wilkins. Crick, Watson and Wilkins were awarded the 1962 Nobel Prize in Medicine for this discovery, by which time Rosalind Franklin had unfortunately died from cancer. This work has since helped to explain how diseases are passed down through generations, and explains other mysteries such as why we look like our parents, how cells function on a micro level and how life evolves. It was a pivotal moment for academic research, and has shaped the direction of research into medicines and health, with a push towards personalised medicine .
One aspect of chemical innovation that's largely taken for granted but is integral to the day-to-day life of many people now are the display screens in smart phones, televisions and computers. These devices use molecules known as liquid crystals to control light and images, which gives them their name—LCD (liquid crystal display) screens. Liquid crystals occur when a substance has an intermediary state between a solid and a liquid. Instead of having one melting point, describing the change from solid to liquid, a liquid crystal has two—an initial temperature at which a substance melts to form a turbid GLOSSARY turbid cloudy, opaque, or thick with suspended matter fluid, and a clear secondary melting point at which that turbid fluid becomes clear. Between these two points is the liquid crystalline state.
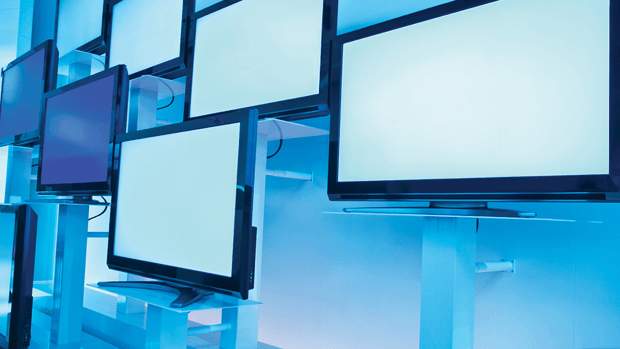
Liquid crystals have light modulating properties, and as such are useful in screens. They were first observed in 1888 by botanist and chemist Friedrich Reinitzer , who observed the effect in cholesterol extracted from carrots. LCD technology is likely to be superseded by light emitting diodes (LEDs) in the next decade. LED displays are more durable and consume less electricity. Their impact will depend on further materials breakthroughs in the fields of conducting polymers and nanomaterials such as quantum dots, which provide the bright, vivid colours needed for display screens.
The consequences of chemistry are far reaching. Chemistry has been largely responsible for shaping society as we know it; from developing stronger materials for large scale construction, down to what kind of cosmetic products you use every day. Society has benefited hugely from advances in the field, with the few key discoveries outlined here just a small cross-section of the chemical innovations that have driven society’s development. While discoveries in chemistry have made a huge impact, and continue to have emormous potential, we also need to ensure that we use them responsibly to ensure sustainability into the future.
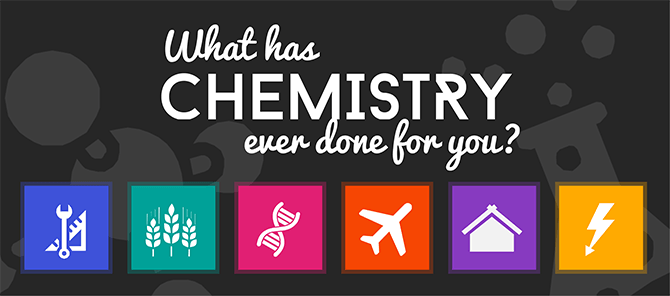
Batteries of the future
Feeding a hot, hungry world, fighting back: antimicrobial resistance.
- nanotechnology
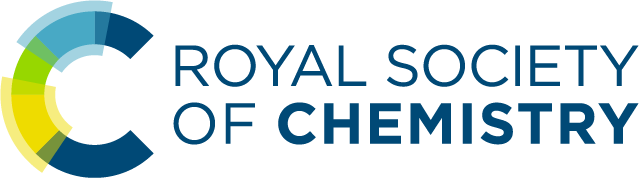
Policy, evidence and campaigns
- Environmental sustainability
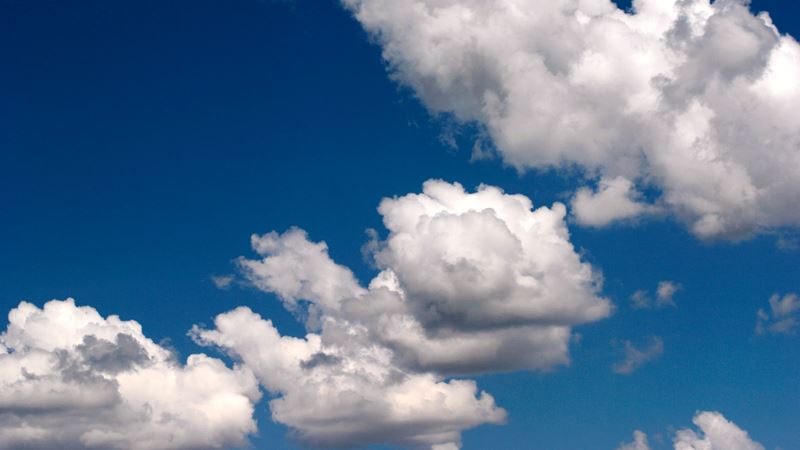
Environment
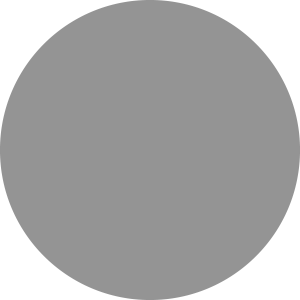
Over the years, we have made significant developments in agriculture, energy and health that have contributed to human well-being. However, some of these improvements in our lives have resulted in changes to the environment around us.
Our environment is a hugely complex system that includes the air we breathe, the land we live on, the water we drink and the climate around us. We must work to ensure that our developments in some areas do not adversely affect our environment whilst also ensuring that we mitigate any damage that has occurred. Work by some researchers has shown that we are already at a tipping point that might lead to “non-linear, abrupt environmental change within continental- to planetary-scale systems”.
The good news is that politicians globally are looking at how to solve this. The UN’s Sustainable Development Goals include universal calls to action to protect life on land and in water, producing clean water and tackling climate change. Meanwhile, the EU’s Environmental Action Plan includes nine priority objectives that aim to ensure “we live well, within the planet’s ecological limits”.
As we strive towards a better world, we work to ensure chemistry’s contributions are realised. Chemistry can help us to understand, monitor, protect and improve the environment around us. Chemists are developing tools and techniques to make sure that we can see and measure air and water pollution. They have helped to build the evidence that shows how our climate has changed over time. And they can be part of the effort to understand and address new problems that we face like microplastics and the potential effects of the different chemicals that we are exposed to.
On this page
Air Climate change
Chemical scientists have an important role to play in reducing air pollution (sometimes in unexpected ways), as well as in helping us to understand and monitor it.
The World Health Organisation reported that around 7 million people died as a result of air pollution in 2012. According to the Organisation for Economic Co-operation and Development, poor ambient air quality is set to be the world’s leading environmental cause of premature death by 2050 if we maintain current policies. By improving air quality, we can save millions of lives and improve our quality of living.
Cleaner air will also help us to protect the environment and preserve cultural heritage sites. Pollutants such as sulfur dioxide and nitrogen oxides can form acid rain, which pollutes soil and water and damages buildings such as the Acropolis and the Taj Mahal.
Other pollutants, such as ground level ozone, affect vegetation and so reduce agricultural yields.
Air pollution is a concern for the whole world as pollutants can be carried long distances, even across countries. However, scientists and engineers worldwide are tackling this issue, and we aim to support chemistry’s vital role in understanding air pollution and developing solutions to reduce it.
How chemistry helps
Chemistry of air pollution.
Air is a mixture of gases and particles, some of which are reactive and undergo complex chemical reactions in the atmosphere to form air pollutants such as ozone. Other air pollutants are emitted directly - for example, sulfur dioxide. Air pollutants can be solid, liquid or gas and come from natural and man-made sources; the biggest contributors to air pollution today are power stations, road transport, industry and residential burning of fuels.
A detailed understanding of pollutants and their chemistry is important for interpreting health effects, regulating emissions, and developing pollution-reducing technologies. For example, chemists have created a " master chemical mechanism " that describes the chemical reactions involved in degradation of volatile organic compounds in the lower atmosphere. This helps policy makers to “test” how effective a piece of proposed regulation or legislation would be. In another example, chemists identified trees as the source of high levels of organic pollutants during heatwaves. This unexpected result has improved the air quality forecasts provided to the public in the UK by taking into account natural emissions.
Monitoring air pollution
Accurate measurements of pollutants are vital for checking that we comply with national and international air quality directives. In addition, measurements can help us to understand correlations between health problems and air pollution - for example, the relationship between different types of particulate matter and cardiovascular problems.
The UK has around 300 air quality monitoring sites measuring a variety of pollutants, including ozone, nitrogen oxides, sulfur dioxide, carbon monoxide and particulates. One such site is next to a busy road in central Cambridge and measures the concentration of nitrogen oxides from the traffic in real time using chemiluminescence.
The graph below shows the output from one such measurement. The UK and EU air quality target for nitrogen dioxide is an annual mean of 40 micrograms per cubic metre, and the hourly concentration should not exceed 200 micrograms per cubic metre more than 18 times in a year.

Tackling air pollution
Tackling air pollution takes a combination of approaches, including regulation, land use planning, technological solutions (such as vehicle engine design), and consumer behaviour. Chemistry plays a role in developing technological solutions.
Chemists help to decrease emissions from transport in a variety of ways, ranging from developing cleaner fuels (such as low sulfur fuels) to increasing the efficiency of engines. Chemists are also working to enable new transport technologies – for example, batteries for electric vehicles and fuel cells for hydrogen vehicles, as well as systems to produce fuels from renewable energy sources rather than from fossil fuels.
Another way to reduce pollutant emissions is by fitting pollution control devices to the vehicle exhaust. For example, most petrol engines have three-way catalytic converters to reduce carbon monoxide, unburnt hydrocarbons and nitrogen oxides from the exhaust.
Platinum or palladium catalysts oxidise carbon monoxide and hydrocarbons to produce carbon dioxide and water, while rhodium catalysts reduce nitrogen oxides to produce nitrogen and oxygen. Chemists, materials scientists, and engineers develop and improve the catalysts, absorbers, and particulate filters that reduce pollutant emissions.
In the future, even the clothes you wear and our buildings could purify the air. Photocatalytic clothing can break down nitrogen oxides and volatile organic compounds using just oxygen and light. The same technology has been used in paint and cement, allowing buildings to clean the air around them.
In 2013 we brought together atmospheric chemists to discuss the role of aerosols in the atmosphere at our Faraday Discussion: Tropospheric Aerosol - Formation, Transformation, Fate and Impacts . You can read the papers published alongside the discussion in the Faraday Discussions journal.
Many of our members are actively engaged in understanding, monitoring and tackling air pollution. Our Automation and Analytical Management Interest Group hold an annual meeting on monitoring ambient air.
We awarded a blue plaque to Johnson Matthey PLC in recognition of the 40th anniversary of the world’s first commercial catalytic converters being manufactured on this site, and the subsequent development of catalysts and filters for gasoline and diesel vehicles that have cleaned billions of tonnes of pollutants from the environment worldwide. You can read more about the evolution of catalytic converters in Education in Chemistry , our magazine for teachers.
You can also see our public lecture given by Professor Alastair Lewis of the University of York, entitled 'Air pollution past, present and future'.
Climate change
In December 2015, nations around the world gathered in Paris to develop a new international climate change agreement.
In the lead-up to this important conference, we supported the chemical sciences community to discuss and contribute to our understanding of climate change (for example, through the Faraday Discussions series of conferences and publications) as well as its causes and impacts.
You can read more about causes and impacts of climate change in our special collection of research papers, review articles and themed collections . You can also read more about the role of atmospheric chemists in climate change research in Education in Chemistry.
We have also held scientific meetings and wider community engagement activities focused on the many ways in which chemistry will contribute to mitigating and adapting to climate change (for example, see our work on energy , food and water ).
You can read more about how chemistry contributes to tackling global challenges in RSC News .
We have joined with the Institution of Chemical Engineers to reaffirm our own position on climate change. Read our joint statement below.
UK science communiqué on climate change
We are one of 24 of the UK’s professional and learned societies that have endorsed a communiqué on climate change calling for government action. The organisations involved represent a diverse range of expertise from across the sciences, social sciences, arts, humanities, medicine and engineering.
Together, we launched this statement in July 2015, which was covered in the Guardian and Telegraph newspapers.
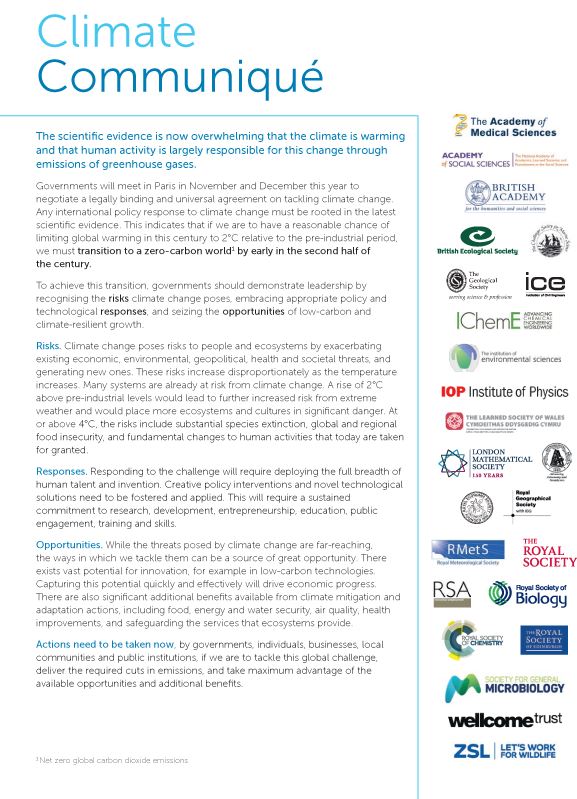
Our statement on climate change
The overwhelming weight of scientific evidence indicates that human activity is the predominant cause of recent climate change. It is clear that the increase in carbon dioxide and other greenhouse gas emissions since the industrial revolution is the chief cause of observed global warming. Regional and year-on-year variations are expected within climate systems, but the evidence shows warming over the last half-century that cannot be explained by natural causes.
Carbon dioxide is already at levels much higher than at any time in the last 800,000 years, and continued emissions are expected to lead to significant further warming. Moreover, the speed of warming will be faster than during past natural climate change events, making adaptation more difficult. This change in climate is expected to bring changes in regional temperature and precipitation and to increase the frequency of heat waves, heavy rainfall, and some other types of extreme weather events. These will have a serious adverse effect on human wellbeing and the natural world.
The choices we make now will have far-reaching consequences. We need to develop mitigation and adaptation strategies to address the challenges that climate change poses. These strategies include developing and deploying low carbon technologies, improving energy efficiency, and changing behaviours to enable sustainable development.
The Royal Society of Chemistry and the Institution of Chemical Engineers are committed to supporting the chemical sciences community in their contributions to tackling climate change. The chemical sciences help us to understand, mitigate, and adapt to climate change. The best evidence based on the best science is essential to inform the right policy decisions on all three fronts. Already, chemists and chemical engineers contribute in a variety of ways, such as improving our understanding of atmospheric and ocean chemistry, investigating the consequences of climate change, developing new energy and carbon mitigation solutions, and helping crops to tolerate the changing conditions.
For more information read the Royal Society and National Academy of Sciences report Climate Change: Evidence and Causes .
All sustainability policy summaries
All our environmental sustainability work, see all of our policies, reports and campaigns.

Environmental Science: Processes & Impacts blog
Chemistry in climate change.
Here we present a collection of research papers, review articles, and themed collections published by the Royal Society of Chemistry, designed to answer a variety of questions related to the causes and impacts of climate change. From atmospheric chemistry to geochemical cycling and analytical techniques, this collection contains the latest research at the cutting edge.
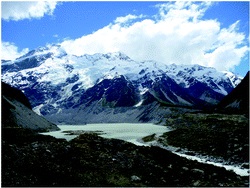
“In the lead up to the UN climate change conference in Paris in late 2015, it is timely to consider the importance of chemistry in climate science,” comments Susan Solomon , advisory board member of Environ. Sci.: Processes Impacts , “This collection showcases the essential contributions of chemical science to understanding climate change. As the world weighs mitigation and adaptation options, chemists will be part of the search for solutions.”
“The chemical sciences play a pivotal role in a sustainable and prosperous future” says Dominic Tildesley , President of the Royal Society of Chemistry “whether it’s developing new antibiotics to combat infection, converting waste to energy, or developing efficient solar energy cells, chemists are designing and applying tomorrow’s technologies.”
You can read all of these articles for free until 20 December 2014! We truly hope you enjoy this collection.
Learn more about our work to support the chemical sciences community working on solutions in climate change, energy, food, health and water .
Nina Notman meets some of the atmospheric chemists fitting the pieces of the climate change jigsaw together – find out more in Education in Chemistry .
Relevant Themed Issues:
Analytical Methods themed collection on Emerging analytical methods for global energy and climate issues .
ChemSocRev themed issued on Atmospheric chemistry .
ChemComm web collection on CO2 separation, capture and reuse .
Photochemical & Photobiological Sciences collection on the Environmental effects of ozone depletion and its interactions with climate change: 2014 Assessment .
Faraday Discussions on Tropospheric Aerosol – Formation, Transformation and Impacts .
Reviews and Perspectives:
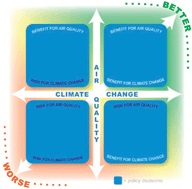
Air quality and climate – synergies and trade-offs Erika von Schneidemesser and Paul S. Monks Environ. Sci.: Processes Impacts , 2013, 15 , 1315-1325 DOI: 10.1039/C3EM00178D, Frontier From themed collection Environmental Science: Processes & Impacts : 2013 Review Articles
Chemical signals of past climate and environment from polar ice cores and firn air Eric W. Wolff Chem. Soc. Rev. , 2012, 41 , 6247-6258 DOI: 10.1039/C2CS35227C, Tutorial Review From themed collection Atmospheric chemistry
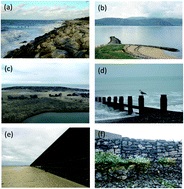
Particles, air quality, policy and health Mathew R. Heal, Prashant Kumar and Roy M. Harrison Chem. Soc. Rev. , 2012, 41 , 6606-6630 DOI: 10.1039/C2CS35076A, Critical Review From themed collection Atmospheric chemistry
Impact of a possible future global hydrogen economy on Arctic stratospheric ozone loss Bärbel Vogel, Thomas Feck, Jens-Uwe Grooß and Martin Riese Energy Environ. Sci. , 2012, 5 , 6445-6452 DOI: 10.1039/C2EE03181G, Minireview
Productivity of aquatic primary producers under global climate change Donat-P. Häder, Virginia E. Villafañe and E. Walter Helbling Photochem. Photobiol. Sci. , 2014, 13 , 1370-1392 DOI: 10.1039/C3PP50418B, Perspective
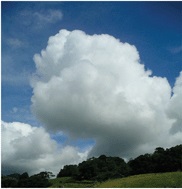
Ocean-atmosphere trace gas exchange Lucy J. Carpenter, Stephen D. Archer and Rachael Beale Chem. Soc. Rev. , 2012, 41 , 6473-6506 DOI: 10.1039/C2CS35121H, Critical Review From themed collection Atmospheric chemistry
The influence of glacial meltwater on alpine aquatic ecosystems: a review Krista E. H. Slemmons, Jasmine E. Saros and Kevin Simon Environ. Sci.: Processes Impacts , 2013, 15 , 1794-1806 DOI: 10.1039/C3EM00243H, Critical Review
Multiphase chemistry of atmospheric amines Chong Qiu and Renyi Zhang Phys. Chem. Chem. Phys. , 2013, 15 , 5738-5752 DOI: 10.1039/C3CP43446J, Perspective
Original research articles:
Exploring the potential influence of climate change and particulate organic carbon scenarios on the fate of neutral organic contaminants in the Arctic environment James M. Armitage and Frank Wania Environ. Sci.: Processes Impacts , 2013, 15 , 2263-2272 DOI: 10.1039/C3EM00315A, Paper From themed collection Open access articles from Environmental Science: Processes & Impacts
A reproducible method for the extraction, identification and quantification of the Arctic sea ice proxy IP 25 from marine sediments Simon T. Belt, Thomas A. Brown, Alba Navarro Rodriguez, Patricia Cabedo Sanz, Andrew Tonkin and Rebecca Ingle Anal. Methods , 2012, 4 , 705-713 DOI: 10.1039/C2AY05728J, Paper
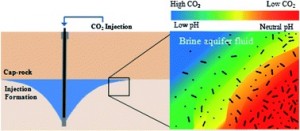
Changes in metal mobility associated with bark beetle-induced tree mortality Kristin M. Mikkelson, Lindsay A. Bearup, Alexis K. Navarre-Sitchler, John E. McCray and Jonathan O. Sharp Environ. Sci.: Processes Impacts , 2014, 16 , 1318-1327 DOI: 10.1039/C3EM00632H, Paper From themed collection 2014 Emerging Investigators
Determination of spatial and temporal variability of pH and dissolved oxygen concentrations in a seasonally hypoxic semi-enclosed marine basin using continuous monitoring Timothy Sullivan, Ciara Byrne, Luke Harman, John Davenport, Rob McAllen and Fiona Regan Anal. Methods , 2014, 6 , 5489-5497 DOI: 10.1039/C3AY42162G, Paper From themed collection Emerging analytical methods for global energy and climate issues
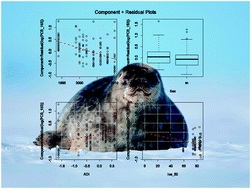
Heterogeneous and multiphase formation pathways of gypsum in the atmosphere Qingxin Ma, Hong He, Yongchun Liu, Chang Liu and Vicki H. Grassian Phys. Chem. Chem. Phys. , 2013, 15 , 19196-19204 DOI: 10.1039/C3CP53424C, Paper
Volatile organic compounds in Arctic snow: concentrations and implications for atmospheric processes Gregor Kos, Visahini Kanthasami, Nafissa Adechina and Parisa A. Ariya Environ. Sci.: Processes Impacts , 2014, Advance Article DOI: 10.1039/C4EM00410H, Paper
A simplified coulometric method for multi-sample measurements of total dissolved inorganic carbon concentration in marine waters Natchanon Amornthammarong, Peter B. Ortner, James Hendee and Ryan Woosley Analyst , 2014, 139 , 5263-5270 DOI: 10.1039/C4AN01049C, Paper
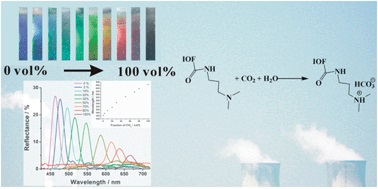
Analysis of secondary organic aerosols in air using extractive electrospray ionization mass spectrometry (EESI-MS) Lambert A. Doezema, Teresa Longin, William Cody, Véronique Perraud, Matthew L. Dawson, Michael J. Ezell, John Greaves, Kathleen R. Johnson and Barbara J. Finlayson-Pitts RSC Adv. , 2012, 2 , 2930-2938 DOI: 10.1039/C2RA00961G, Paper
The use of climatologies and Bayesian models to link observations to outcomes; an example from the Torres Strait Scott Bainbridge and Ray Berkelmans Environ. Sci.: Processes Impacts , 2014, 16 , 1041-1049 DOI: 10.1039/C3EM00675A, Paper
Gas–particle partitioning of atmospheric aerosols: interplay of physical state, non-ideal mixingand morphology Manabu Shiraiwa, Andreas Zuend, Allan K. Bertram and John H. Seinfeld Phys. Chem. Chem. Phys. , 2013, 15 , 11441-11453 DOI: 10.1039/C3CP51595H, Paper
Organic matrix effects on the formation of light-absorbing compounds from α-dicarbonyls in aqueous salt solution Greg T. Drozd and V. Faye McNeill Environ. Sci.: Processes Impacts , 2014, 16 , 741-747 DOI: 10.1039/C3EM00579H, Paper From themed collection Aquatic photochemistry
Three years (2008–2010) of measurements of atmospheric concentrations of organochlorine pesticides (OCPs) at Station Nord, North-East Greenland Rossana Bossi, Carsten Ambelas Skjøthb and Henrik Skovac Environ. Sci.: Processes Impacts , 2013, 15 , 2213-2219 DOI: 10.1039/C3EM00304C, Paper
Optical classification of algae species with a glass lab-on-a-chip Allison Schaap, Thomas Rohrlack and Yves Bellouard Lab Chip , 2012, 12 , 1527-1532 DOI: 10.1039/C2LC21091F, Paper
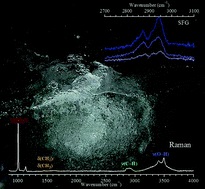
Raman microspectroscopy and vibrational sum frequency generation spectroscopy as probes of the bulk and surface compositions of size-resolved sea spray aerosol particles Andrew P. Ault, Defeng Zhao, Carlena J. Ebben, Michael J. Tauber, Franz M. Geiger, Kimberly A. Prather and Vicki H. Grassian Phys. Chem. Chem. Phys. , 2013, 15 , 6206-6214 DOI: 10.1039/C3CP43899F, Paper
Modelling phosphorus loading and algal blooms in a Nordic agricultural catchment-lake system under changing land-use and climate Raoul-Marie Couture, Koji Tominaga, Jostein Starrfelt, S. Jannicke Moe, Øyvind Kaste and Richard F. Wright Environ. Sci.: Processes Impacts , 2014, 16 , 1588-1599 DOI: 10.1039/C3EM00630A, Paper From themed collection Environmental Science: Processes & Impact s 2014 HOT Articles
Organic aerosols and inorganic species from post-harvest agricultural-waste burning emissions over northern India: impact on mass absorption efficiency of elemental carbon Prashant Rajput, M. M. Sarin, Deepti Sharma and Darshan Singh Environ. Sci.: Processes Impacts , 2014, 16 , 2371-2379 DOI: 10.1039/C4EM00307A, Paper
Theoretical investigation of vibrational relaxation of highly excited O 3 in collisions with HO 2 Lei Zhang, Pingya Luo, Ke Guo, Rong Zeng, Pedro J. S. B. Caridade and António J. C. Varandas RSC Adv. , 2014, 4 , 9866-9874 DOI: 10.1039/C3RA45634J, Paper
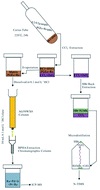
A method for determination of PGE–Re concentrations and Os isotopic compositions in environmental materials Peipei Zhao, Jie Li, Lifeng Zhong, Shengling Sun and Jifeng Xu Anal. Methods , 2014, 6 , 5537-5545 DOI: 10.1039/C3AY42064G From themed collection Emerging analytical methods for global energy and climate issues
A compact comprehensive two-dimensional gas chromatography (GC×GC) approach for the analysis of biogenic VOCs Samuel J. Edwards, Alastair C. Lewis, Stephen J. Andrews, Richard T. Lidster, Jacqueline F. Hamilton and Christopher N. Rhodes Anal. Methods , 2013, 5 , 141-150 DOI: 10.1039/C2AY25710F
Brown carbon formation from ketoaldehydes of biogenic monoterpenes Tran B. Nguyen, Alexander Laskin, Julia Laskin and Sergey A. Nizkorodov Faraday Discuss. , 2013, 165 , 473-494 DOI: 10.1039/C3FD00036B, Paper From themed collection Tropospheric Aerosol – Formation, Transformation and Impacts
Carbon footprint of geopolymeric mortar: study of the contribution of the alkaline activating solution and assessment of an alternative route A. Mellado, C. Catalán, N. Bouzón, M. V. Borrachero, J. M. Monzó and J. Payá RSC Adv. , 2014, 4 , 23846-23852 DOI: 10.1039/C4RA03375B, Paper
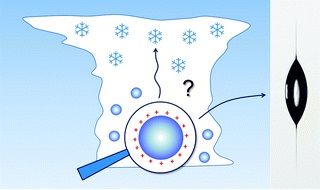
On the role of surface charges for homogeneous freezing of supercooled water microdroplets Daniel Rzesanke, Jens Nadolny, Denis Duft, René Müller, Alexei Kiselev and Thomas Leisner Phys. Chem. Chem. Phys. , 2012, 14 , 9359-9363 DOI: 10.1039/C2CP23653B, Paper From themed collection Structure and reactivity of small particles: from clusters to aerosols

Analyst , AnalyticalMethods , ChemComm , CSR , EES , ESNano , ESPI , Faraday , LOC , PCCP , PPS Posted in News , Themed Collections -->
- Search this blog Search for:
A little something about you, the author. Nothing lengthy, just an overview.
- About the journal
- Environmental Science journals
- Follow us on Twitter
- HOT Article Collection
- Journal Homepage
- Meet the Editorial Board
- Open Access Articles
- Recent Review Articles
- Board News (28)
- Chemistry World Highlights (20)
- Conferences (2)
- Cover articles (23)
- Editors Choice Collections (1)
- Emerging Investigators (38)
- Hot Articles (156)
- Peer Review (3)
- Reviews (4)
- Symposia (2)
- Themed Collections (41)
- Top Ten (30)
- Uncategorized (11)
- February 2024
- February 2023
- October 2022
- September 2022
- January 2022
- November 2021
- October 2021
- September 2021
- February 2021
- December 2020
- September 2020
- February 2020
- January 2020
- December 2019
- October 2019
- September 2019
- August 2019
- February 2019
- January 2019
- December 2018
- November 2018
- October 2018
- September 2018
- February 2018
- November 2017
- October 2017
- September 2017
- February 2017
- January 2017
- December 2016
- November 2016
- September 2016
- August 2016
- February 2016
- January 2016
- November 2015
- October 2015
- September 2015
- August 2015
- February 2015
- January 2015
- December 2014
- November 2014
- October 2014
- September 2014
- August 2014
- February 2014
- January 2014
- December 2013
- November 2013
- October 2013
- September 2013
- August 2013
- February 2013
- January 2013
- December 2012
- November 2012
- September 2012
- August 2012
- February 2012
- January 2012
- December 2011
- November 2011
- October 2011
- September 2011
- August 2011
- February 2011
- January 2011
- December 2010
- November 2010
- October 2010
- September 2010
- Terms of use
- Working for us
- Privacy & cookies
- Press office
- Accessibility
General Studies
All Programmes
Study Material
Famous Chemistry Scientists of India and their Contributions
Sub-Categories:
Science and Technology
Prelims: General Science.
Mains: Achievements of Indians in science and technology; indigenization of technology and developing new technology.
From ancient to modern times, the contributions of Indian scientists to chemistry have been significant. In ancient India , chemistry was known as Rasayan Shastra or Rasatantra or Rasakriya. In the early years after independence , Indian chemists contributed immensely to what came to be called 'reverse engineering' and they perfected the market for the manufacture of cheap generic drugs so much so that India has now come to be known as a 'pharmacy of the world', ranking 3rd in pharmaceutical production.
Development of Chemistry in India
Science and Technology in ancient and medieval India covered all the major branches of human knowledge and activities, including mathematics, astronomy, physics, chemistry, medical science and surgery.
- Ancient India's contribution to Science and Technology included principles of chemistry that did not remain abstract but also found expression in practical activities like the distillation of perfumes, and aromatic liquids, manufacturing of dyes and pigments and extraction of sugar.
- Residents of the IVC had access to gold and copper mining , and the area was possibly a source of semi-precious stones. This is seen in the jewellery recovered in the vicinity.
- For example: The Ramayana and the Mahabharata mention weapons with arrowheads coated with a variety of chemicals, indicating their knowledge of Alchemy .
- Vaiseshika Darsana of Kanada propounded the concept of the atom and its indivisibility and the law of cause and effect.
- ‘Artha Sastra’ also describes the qualities of Gold and silver.
- Gupta Empire: Nagarjuna, the famous alchemist lived during this period. The use of mercury and iron in medicines indicates a deep knowledge and understanding of Chemistry.
- Due to the smiths' knowledge of advanced metal technology , bronze images of the Buddha began to be produced on a large scale. The Mehrauli Iron Pillar stands free of rust and corrosion for centuries.
- The Rasvidya (Indian Alchemy texts) reveal the use of a variety of organic and inorganic substances.
Famous Chemistry Scientists of India
The achievements of Indian scientists have been recognized at the global level. These achievements will play a decisive role in India joining the list of developed countries.
Some prominent Indians who excelled in the field of chemistry are given below:
Prafulla Chandra Ray (1861-1944)

- Prafulla Chandra Ray was a chemist and industrialist who founded Bengal Chemicals & Pharmaceuticals (India’s first pharmaceutical company).
- He is regarded as the ‘Father of Chemical Science in India’ and he established the first Indian research school in chemistry.
- He contributed to inorganic and organic nitrites.
- In 1895 he reported the first synthesis of the hitherto unknown mercurous nitrite, Hg2(NO2)2.
- Around 1914, he initiated work on organic thio compounds like thiols, thioethers, and related species and their binding to metals.
- Books: A History of Hindu Chemistry from the Earliest Times to the Middle of Sixteenth Century (1902), ‘Life and Experience of a Bengali Chemist’ (autobiography).
Chintamani Nagesa Ramachandra Rao (1934)
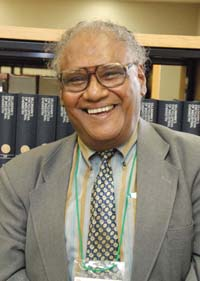
- Professor C.N.R. Rao is a well-known expert in structural and solid-state chemistry.
- He has contributed significantly to chemical spectroscopy, molecular structure, surface chemistry, and high-temperature superconductivity.
- His early research focussed on the application of spectroscopic techniques to study molecular structure.
He studied many chemically important issues using electronic and vibrational spectroscopy, including correlations between spectra and structural features, the effects of the environment, vibration analysis, etc.
- His research has paved the way for the creation of comprehensive models that explain the behaviour of numerous metal oxides and sulfides.
- Awards: He is the winner of the first India Science Award, (2006).
Padma Shri, Padma Vibhushan, and Bharat Ratna (2014).
Har Gobind Khorana (1922-2011)
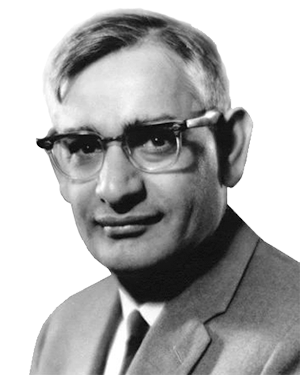
- Har Gobind Khorana was a founder of chemical biology and a pioneer in molecular biology.
- He was one of the first researchers to show the function of nucleotides in protein synthesis and helped to crack the genetic code.
- He had synthesised a more complex molecule, Coenzyme A, which is involved in digestion.
This success marked him as one of the most important biological chemists of his time.
- In 1972, he completed the total synthesis of a functional gene outside a living organism.
He also showed it could function appropriately in a bacterium.
- Awards: Nobel Prize for Physiology or Medicine in 1968 for contributions toward elucidating the genetic code.
Shanti Swarup Bhatnagar (1894-1955)

- Shanti Swarup Bhatnagar played a significant part in the building of post-independent S&T infrastructure and in the formulation of India’s S&T policies.
- He was the Founder-Director of the Council of Scientific and Industrial Research (CSIR), and the first Chairman of the University Grants Commission (UGC). He did considerable work in applied and industrial chemistry.
- The first industrial problem undertaken by him was the development of a process to convert bagasse (peelings of sugarcane) into food cake for cattle.
- Best known for making wax odourless, he also discovered how to make the kerosene flame taller and larger, thus helping multitudes of Indian households.
- Book: ‘Physical Principles and Applications of Magneto Chemistry (Bhatnagar jointly with K.N. Mathur)
- Order of the British Empire: Based on his significant contributions to both pure and applied chemistry, the British government awarded Bhatnagar the Order of the British Empire in 1936.
- Padma Vibhusan.
- The "Shanti Swarup Bhatnagar (SSB) Prize for Science & Technology" was instituted in his honour by the Council of Scientific and Industrial Research (CSIR) for notable and outstanding research in various fields of science.
Dr. Raghunath Anant Mashelkar (1943)
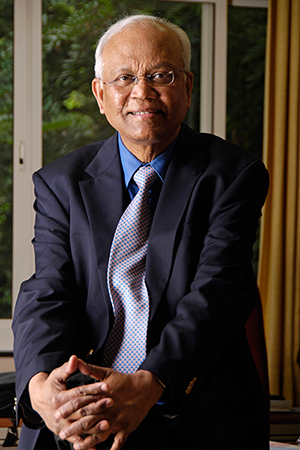
- Dr. Raghunath Anant Mashelkar is one of India’s most eminent scientists known for his contributions to India’s National Chemical Laboratory and CSIR.
- Dr Mashelkar’s pioneering research in polymer science and engineering is covered in several research journals.
- He is best known for his crusade against the US patents on turmeric, neem and basmati rice.
- Arguing that the healing properties of turmeric are well known as traditional knowledge in India, he fought a successful legal battle to revoke the patent. This victory was so significant that it changed the way patents were classified, and earned him the moniker of ‘The Warrior of Haldighati’.
- Padmashri, Padma Bhushan and Padma Vibhushan.
- First Indian to be elected a Fellow
Venkataraman Ramakrishnan (1952)

- Venkataraman Ramakrishnan is a Nobel Prize-winning biochemist. His contributions include work on the atomic structure of the ribosome.
- He studied the structure of chromatin and histone, which aids in the understanding of how DNA is arranged in cells.
- He determined the atomic structure of the 30S ribosomal subunit and then the structures of the whole ribosome in a variety of states and in complexes with various antibiotics.
- He explained through the 3D technique how ribosomes react with different chemicals.
- Nobel Prize in Chemistry for his work on ribosomal structure and was knighted in 2012.
FAQs on Famous Chemistry Scientists of India
Who were the indian scientists who contributed to chemistry.
Prafulla Chandra Ray, C N R Rao, Venkataraman Ramakrishnan, and Dr. Raghunath Anant Mashelkar are some of the Indian scientists who contributed to chemistry.
Who was the first Indian to get the Nobel Prize in Chemistry?
Venkatraman Ramakrishnan is an Indian-born physicist and molecular biologist who was awarded the 2009 Nobel Prize for Chemistry for his work on ribosomal structure.
Who is the father of Indian chemistry?
Sir Acharya Prafulla Chandra Ray was a remarkable scientist, industrialist, and philanthropist, widely known as "the father of Indian chemistry," Ray was a pioneer and figurehead of modern chemical research in India.
© 2024 Vajiram & Ravi. All rights reserved
This website uses cookies to improve your user experience. By continuing to use the site, you are accepting our use of cookies. Read the ACS privacy policy.
- ACS Publications
Call for Papers: Polymers for the Clean Energy Transition
- Mar 22, 2024
This Virtual Special Issue will showcase the crucial role of polymers in enabling new technologies and applications for clean energy. Submit your manuscript by June 30, 2024.
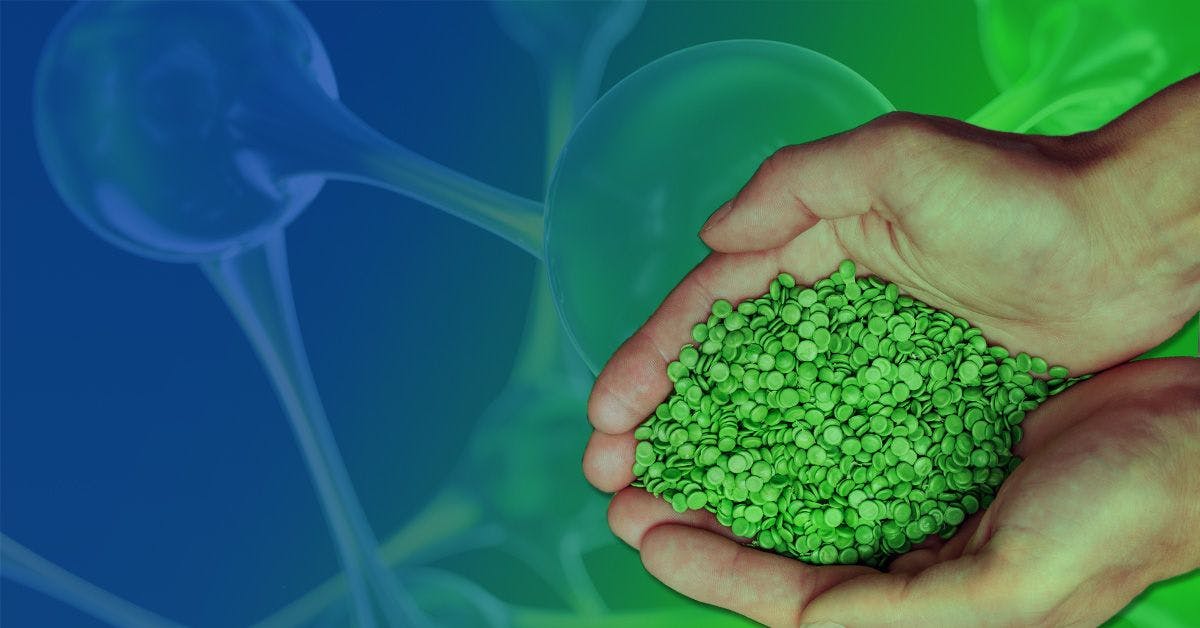
The continued transition to clean energy will be enabled by technologies ranging from solar-power generation to energy storage and transport to energy-efficient water and food production. In these applications and more, polymers will feature prominently as a functional material capable of meeting the stringent demands required for large-scale technology implementation, including supply chain flexibility and cost. While advances in polymer science and technology have already contributed to the clean energy transition, new insights and discoveries are warranted as the demand for clean energy increases and the climate continues to warm.
Given the importance of polymers in enabling new technologies for clean energy, broadly defined, this Virtual Special Issue in JACS Au aims to highlight work in the following areas, including but not limited to:
- Solar energy harvesting
- Energy storage
- Polymer Electrolytes
- Supercapacitors
- Conducting Polymers
- Polymeric membranes
- Carbon capture polymers
Organizing Editors
Professor Rodney D. Priestley , Associate Editor, JACS Au Princeton University, United States
Professor Emily Davidson , Guest Editor Princeton University, United States
Professor He Tian , Guest Editor East China University of Science and Technology, China
Submission Information
We welcome submissions for this Virtual Special Issue through June 30, 2024 . Submissions must comply with our Author Guidelines and will be subject to the usual rigorous peer review to ensure they fit with the scope and conform to the high-quality and significance of JACS Au before acceptance.
Papers accepted for publication for this Virtual Special Issue will be first published as they are accepted in a regular issue of the journal with a note indicating that they are part of the Virtual Special Issue. After all submissions have been published, they are then compiled online on a dedicated landing page to form the Virtual Special Issue.
How to Submit
- Log in to the ACS Paragon Plus submission site.
- Choose JACS Au as your journal.
- Select your manuscript type.
- Under ‘Special Issue Selection,’ choose ‘ Polymers for Clean Energy .'
If you have any general questions regarding submission to this Virtual Special Issue, please contact Professor Rodney Priestley ( [email protected] ).
Open Access
JACS Au is a gold open access journal, which means your work will be immediately freely available for anyone to read in perpetuity. Article Publication Charges (APC) apply for publication in this fully open access journal. Please see our website for more information on Open Access Pricing , our ACS Country Discount & Waiver Policy , and ACS Read and Publish Agreements .
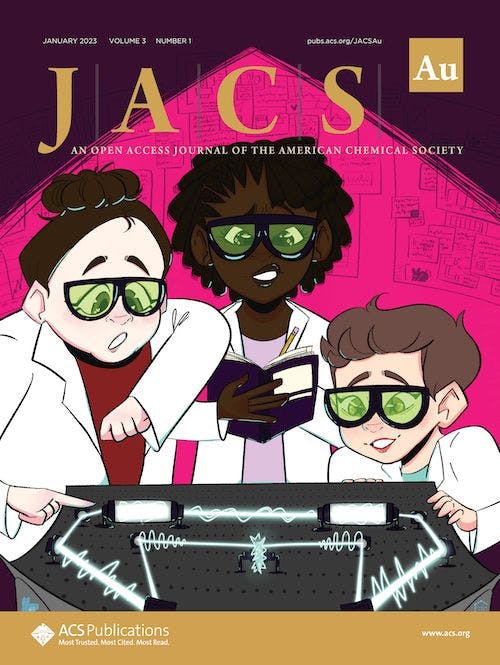
Stay Connected
Want the latest stories delivered to your inbox each month.
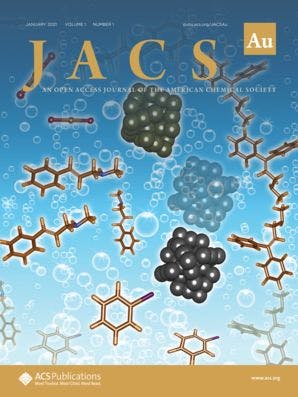
- OU Homepage
- The University of Oklahoma
OU Researcher Highlights Importance of Mentorship in Chemistry Journal

Kat Gebauer
Media Contact
Kat Gebauer (405) 325-1860 [email protected]
NORMAN, OKLA. – University of Oklahoma assistant professor Yuanning Feng, Ph.D. authored an article published in a special edition of Trends in Chemistry , “From mentee to mentor: advice for new principal investigators.” In the article, Feng describes lessons he has learned as a new mentor in his field and from his time as a mentee while pursuing his doctoral degree and working as a postdoctoral fellow.
The article describes three key pairs of factors that make for a strong relationship between mentors and mentees: kindness and helpfulness, communication and collaboration, and patience and tolerance.
Feng received a doctorate in chemistry from Northwestern University where he studied under the supervision of Sir Fraser Stoddart, Ph.D., who won the Nobel Prize in Chemistry in 2016, among other significant accolades. During his long tenure as a chemist and academic, Stoddart mentored hundreds of students and postdoctoral researchers. From the moment he first met Stoddart, Feng was struck by how gracious Stoddart was, even with students he did not know. Within the Stoddart Group, says Feng, members work ‘with,’ not ‘for’ Stoddart.
At a conference in 2022, the editor of Trends in Chemistry noticed the strength of mentorship between Stoddart and Feng. When she learned Feng had taken on his own leadership role at OU, she asked Feng to author the paper for the “Emerging Leaders in Chemistry” special issue for the 5th anniversary of Trends in Chemistry and the 50th anniversary of publisher Cell Press.
After joining the faculty at OU in August 2023, Feng spent the last year contemplating the mentorship that was modeled to him. “I learned a lot about how to deal with mentorship from Stoddart, and during the past two semesters, I’ve thought about advice I could offer to the other new faculty members.” Currently, Feng supervises one postdoctoral fellow, two graduate research assistants, and over ten undergraduate students, taking the lessons he learned from Stoddart and applying them in his own research group .
“The high esteem that principal investigators are often held in by members of their laboratories or working groups can create a power imbalance that leads to unhealthy mentorship,” said Feng.
For Feng, mentorship is important for all mentees he works with. He prioritizes their involvement in conferences and helps his students prepare for them and teaches them how to present their research. From helping students improve their writing skills through thorough yet constructive feedback to fostering an environment of positivity and patience, Feng’s dedication to the principles outlined in his article helps him nurture the next generation of scientists being educated at OU.

About the publication
“From mentee to mentor: advice for new principal investigators.” is published at https://doi.org/10.1016/j.trechm.2024.04.008
About the University of Oklahoma
Founded in 1890, the University of Oklahoma is a public research university located in Norman, Oklahoma. As the state’s flagship university, OU serves the educational, cultural, economic and health care needs of the state, region and nation. OU was named the state’s highest-ranking university in U.S. News & World Report’s most recent Best Colleges list . For more information about the university, visit ou.edu .
Recent News
University of Oklahoma assistant professor Yuanning Feng, Ph.D. authored an article published in a special edition of Trends in Chemistry describing lessons he has learned as a new mentor in his field and from his time as a mentee while pursuing his doctoral degree and working as a postdoctoral fellow.

App Created to Support Black Prostate Cancer Patients Post-Diagnosis
A University of Oklahoma researcher recently received a $1.3 million grant from the U.S. Department of Defense to test a newly created mobile app designed to address health disparities among Black men diagnosed with prostate cancer.

University of Oklahoma to Evaluate $36M Federal Grant to Strengthen State’s Early Childhood Systems
An interdisciplinary team of researchers from the University of Oklahoma has received a portion of a $36 million grant from the U.S. Department of Health and Human Services to evaluate strategies designed to strengthen educational support for Oklahoma’s children, ages birth through five years.

More OU News

- Accessibility
- Sustainability
- OU Job Search
- Legal Notices
- Resources and Offices
- OU Report It!
The Remarkable Contributions of Mae Jemison at Every Age
This essay is about the remarkable life and contributions of Mae Jemison, the first African American woman to travel in space. It highlights her early interest in science, her education at Stanford and Cornell, and her work with the Peace Corps. The essay details her historic space mission in 1992 and her subsequent efforts to promote science education and literacy through the Jemison Group and the Dorothy Jemison Foundation for Excellence. It underscores her ongoing influence as an advocate for diversity and inclusion in STEM fields and her continued impact on science, education, and technology. Jemison’s legacy is presented as a testament to perseverance, dedication, and the pursuit of excellence.
How it works
Mae Jemison’s story is profoundly exceptional. Born on October 17, 1956, she has emerged as an emblem of excellence and persistence across various domains, spanning from celestial voyages to medical and educational realms. Being the inaugural African American female voyager into the cosmos, she not only breached barriers but also ignited inspiration among innumerable individuals worldwide. However, her accomplishments transcend the mere epoch-making event, delineating a life teeming with pioneering contributions at every phase.
Raised in the milieu of Chicago, Jemison manifested an inquisitive and astute disposition, exhibiting an inherent proclivity towards both scientific inquiry and artistic expression since her formative years.
Charlie and Dorothy Jemison, her progenitors, nurtured her intellectual inclinations, fostering an ambiance where inquisitiveness found solace and nourishment. It was this nurturing environment that laid the groundwork for her subsequent triumphs. By the age of 16, Jemison harbored lofty aspirations, envisioning a trajectory steeped in scientific pursuits. This ambitious resolve led her to Stanford University, where she attained a Bachelor of Science degree in Chemical Engineering while concurrently fulfilling the requisites for a Bachelor of Arts in African and Afro-American Studies. The dual trajectory of her scholastic endeavors epitomized a broader vision—one that amalgamated technical prowess with a profound comprehension of cultural and societal dynamics.
Post her graduation from Stanford, Jemison embarked on a trajectory towards a medical vocation at Cornell University Medical College, culminating in the attainment of her M.D. in 1981. Her medical odyssey commenced earnestly within a general practice milieu, yet her impetus to effectuate a substantial difference soon steered her towards the Peace Corps. From 1983 to 1985, Jemison discharged her duties as a medical officer in Liberia and Sierra Leone. This experiential juncture was transformative, broadening her vista on healthcare and cementing her dedication towards global health and humanitarian endeavors. The adversities encountered and lives touched during this phase served to fortify her resolve to harness her skills for the collective welfare.
In 1987, a historic juncture transpired in Jemison’s career when NASA designated her as an astronaut candidate, heralding the commencement of a demanding training regimen that scrutinized her endurance and intellect. On September 12, 1992, Jemison embarked on her celestial sojourn aboard the Space Shuttle Endeavour as part of mission STS-47. This expedition etched her name in history as the pioneer African American woman to voyage into space—a milestone reverberating globally. Over the course of the eight-day mission, Jemison conducted experiments encompassing life sciences and materials processing, thereby furnishing invaluable data to NASA’s research endeavors. Her tenure in space not only underscored her scientific acumen but also underscored her capacity to thrive in one of the most arduous environments conceivable.
Post her triumphant mission, Jemison bid adieu to NASA in 1993 to embark on fresh challenges and prospects. She spearheaded the Jemison Group, a technology consultancy entity aimed at integrating cutting-edge technology into quotidian existence. This venture constituted merely one facet of her overarching commitment to fomenting innovation and ameliorating lives through scientific and technological avenues. In a similar vein, she established the Dorothy Jemison Foundation for Excellence, christened after her mother. The foundation’s mandate revolves around promoting science education and literacy, particularly among youth hailing from marginalized communities. Through initiatives like The Earth We Share (TEWS), an international science camp, Jemison has both inspired and enlightened the succeeding generation of scientists, engineers, and innovators.
Jemison’s impact as an educator and proponent of STEM (Science, Technology, Engineering, and Mathematics) disciplines is profound. She has delivered myriad speeches and lectures, accentuating the significance of diversity and inclusivity within these domains. Her endeavors have galvanized youngsters from all strata of society to pursue vocations in science and technology, thereby challenging the preconceived notions and barriers that have traditionally impeded participation in these spheres. Her message resonates unequivocally: scientific progress and innovation flourish upon diverse perspectives and inclusive practices.
As the sands of time have trickled, Jemison’s contributions have only deepened and expanded. She assumes diverse roles on various boards and advisory panels, incessantly advocating for progressions in science, education, and technology. Her entrenchment in these domains underscores her sustained dedication towards effectuating a meaningful difference, thereby underscoring the truism that age constitutes no impediment to impactful endeavors. Jemison’s life narrative stands as a potent testimony to the enduring value of curiosity, perseverance, and an unyielding pursuit of one’s objectives.
Mae Jemison’s odyssey serves as a poignant exemplification of shattering barriers and confronting conventions. From her nascent fascination with celestial bodies to her trailblazing exploits in space and her relentless endeavors to propel education and technology forward, she has epitomized that determination and diligence can surmount even the most daunting hurdles. Her legacy transcends her personal triumphs, serving as a guiding light for myriad individuals who aspire to effectuate positive change on a global scale.
Jemison’s influence extends beyond the precincts of space exploration, permeating into the broader vista of societal advancement. Her narrative underscores the quintessence of representation and the transformative potency of education and mentorship. By championing scientific literacy and inclusivity, she has laid a bedrock for a more equitable and innovative future. Her endeavors serve as a poignant reminder that advancements in any realm necessitate not just technical prowess but also a commitment to societal equity and communal engagement.
In the latter phase of her life, Jemison persists as a dynamic force, championing causes of import and channeling her expertise into various initiatives. Whether through her foundation, her oratory engagements, or her involvement in advisory capacities, she endures as a pivotal figure in the ongoing discourse concerning the role of science and technology in societal fabric. Her life’s work epitomizes the interconnectedness of erudition, empathy, and action.
Mae Jemison’s achievements stand as a testament to the boundless possibilities that unfurl with ardor, persistence, and a dedication to excellence. From her epochal voyage into the cosmos to her seminal endeavors in education and technology, she exemplifies that one individual’s ardor can ignite transformative change on a global panorama. As she persists in contributing across myriad domains, Jemison’s saga serves as a poignant reminder that the quest for knowledge and the impetus to ameliorate our world are endeavors unbound by temporal constraints.
Her odyssey is far from its denouement, and her contributions persist in shaping the vista of tomorrow. Mae Jemison transcends the realm of an astronaut; she embodies the quintessence of a scientist, a medic, an educator, and an advocate. Her life’s saga bridges the chasm between aspiration and fruition, underscoring that with the requisite support and determination, extraordinary feats become attainable. Her legacy is one of hope, progression, and an unwavering conviction that each of us can aspire to reach for the stars.

Cite this page
The Remarkable Contributions of Mae Jemison at Every Age. (2024, Jun 01). Retrieved from https://papersowl.com/examples/the-remarkable-contributions-of-mae-jemison-at-every-age/
"The Remarkable Contributions of Mae Jemison at Every Age." PapersOwl.com , 1 Jun 2024, https://papersowl.com/examples/the-remarkable-contributions-of-mae-jemison-at-every-age/
PapersOwl.com. (2024). The Remarkable Contributions of Mae Jemison at Every Age . [Online]. Available at: https://papersowl.com/examples/the-remarkable-contributions-of-mae-jemison-at-every-age/ [Accessed: 3 Jun. 2024]
"The Remarkable Contributions of Mae Jemison at Every Age." PapersOwl.com, Jun 01, 2024. Accessed June 3, 2024. https://papersowl.com/examples/the-remarkable-contributions-of-mae-jemison-at-every-age/
"The Remarkable Contributions of Mae Jemison at Every Age," PapersOwl.com , 01-Jun-2024. [Online]. Available: https://papersowl.com/examples/the-remarkable-contributions-of-mae-jemison-at-every-age/. [Accessed: 3-Jun-2024]
PapersOwl.com. (2024). The Remarkable Contributions of Mae Jemison at Every Age . [Online]. Available at: https://papersowl.com/examples/the-remarkable-contributions-of-mae-jemison-at-every-age/ [Accessed: 3-Jun-2024]
Don't let plagiarism ruin your grade
Hire a writer to get a unique paper crafted to your needs.
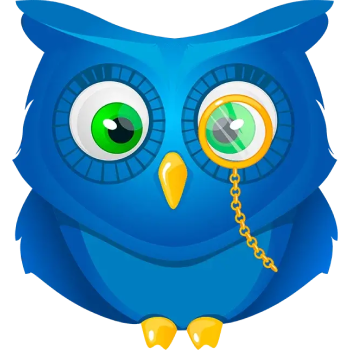
Our writers will help you fix any mistakes and get an A+!
Please check your inbox.
You can order an original essay written according to your instructions.
Trusted by over 1 million students worldwide
1. Tell Us Your Requirements
2. Pick your perfect writer
3. Get Your Paper and Pay
Hi! I'm Amy, your personal assistant!
Don't know where to start? Give me your paper requirements and I connect you to an academic expert.
short deadlines
100% Plagiarism-Free
Certified writers
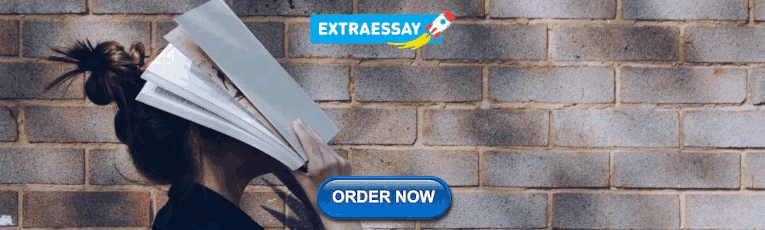
IMAGES
VIDEO
COMMENTS
Chemistry - Applications, Benefits, Impacts: For the first two-thirds of the 20th century, chemistry was seen by many as the science of the future. The potential of chemical products for enriching society appeared to be unlimited. Increasingly, however, and especially in the public mind, the negative aspects of chemistry have come to the fore. Disposal of chemical by-products at waste-disposal ...
Introduction. Lorenzo Amedeo Avogadro was born in 1776 in Turin city of Italy and died in 1856 at the age of 81 years after making a great positive contribution in the field of science especially chemistry. He schooled in Turin and having come from a family background of lawyers, his career was oriented towards law whereby at the age of 16 ...
Improvement of health care and medicine The role of chemistry on the Improvement of health care and medicine. According to Brown, Lemay, Burstein, Murphy, & Woodward (2012) Chemistry has contributed many of the life-saving breakthroughs in health and medicine in the last century that allows us to live longer, happier, and healthier.
BACKGROUND. A. Role of Chemistry in Society. Applications of chemical science have contributed significantly to the advancement of human civilization (1, 2, 3). With a growing understanding and ability to manipulate chemical molecules, the post-World War II chemist was con- sidered a societal problem solver.
chemistry, the science that deals with the properties, composition, and structure of substances (defined as elements and compounds), the transformations they undergo, and the energy that is released or absorbed during these processes. Every substance, whether naturally occurring or artificially produced, consists of one or more of the hundred-odd species of atoms that have been identified as ...
Chemistry is important in everyday life because: Everything is made of chemicals. You are made of chemicals. So is your dog. So is your desk. So is the sun. Drugs are chemicals. Food is made from chemicals. Many of the changes you observe in the world around you are caused by chemical reactions.
Chemistry can make pivotal contributions to help realize these ambitious goals, but first it must undergo major changes in its priorities, approaches and practices. View. Show abstract.
Both the intellectual contribution of chemistry-related enactors to the reflection of ethical aspects of chemical activity and the recurrent impact on its conduct and methodology is insignificant. ... media ethics, and political ethics. A huge amount of books, journal articles, and essays has been published in this field that is understood as a ...
2.2.2 Indirect Contributions of Chemistry to the U.S. Economy. ... The only other countries to have an above-average number of highly cited papers are the United Kingdom and, in later years, Germany. This correlates well with countries that have well-established scientific research programs. When looking at China's share of the top 1% of ...
This essay about Louis Pasteur highlights his groundbreaking discoveries in microbiology, immunology, and chemistry, which revolutionized our understanding of disease. From disproving spontaneous generation to developing the process of pasteurization, Pasteur's contributions reshaped scientific paradigms and saved countless lives.
ACS journals offer readers an extraordinary breadth of research and cutting-edge science from around the world. Every day, articles published in the journals influence and inspire scientists, policymakers, industry and the general public. We have compiled some of the latest fascinating research that shows how relevant chemistry is to our everyday lives. The world of […]
Ursula Klein, Wolfgang Lefèvre, and Mi Gyung Kim have challenged the novelty of Lavoisier's theoretical contributions by demonstrating the extent to which he was indebted to the affinity chemists of the early eighteenth century, especially Étienne-François Geoffroy. 6 Conversely, Maurice Crosland has claimed that the changes in chemistry ...
It's important to understand chemistry if you are studying any of the sciences because all of the sciences involve matter and the interactions between types of matter. Students wanting to become doctors, nurses, physicists, nutritionists, geologists, pharmacists, and (of course) chemists all study chemistry. You might want to make a career out ...
Chemistry is important because everything around us is made up of chemicals and we use it in our day-to-day activities—in our house, industry, companies, etc. —Immanuel Abiola. Chemistry is the Universe: It is said that chemistry is the knowledge of observing this universe.
Contributions of Famous Scientists to Chemistry Essay example. Chemistry is a branch of science that has been around for a long time. Chemistry is dated back as far as prehistoric times. Chemistry has been around for so long that scientist have put it in 4 general chronological categories. These four categories are prehistoric, beginning of the ...
Lavoisier made many fundamental contributions to the science of chemistry. Following his work, chemistry acquired a strict, quantitative nature, allowing reliable predictions to be made. ... In those papers he described his partial differential equation that is the basic equation of quantum mechanics and bears the same relation to the mechanics ...
The chemical industry, supported by chemistry research, underpins much of our economic progress, and provides wealth and prosperity for society. In Australia, 60,000 people are employed by the chemical industry and it contributes around $11.6 billion annually to our GDP. What follows is a snapshot—just a small sample—of the major ...
As we strive towards a better world, we work to ensure chemistry's contributions are realised. Chemistry can help us to understand, monitor, protect and improve the environment around us. Chemists are developing tools and techniques to make sure that we can see and measure air and water pollution. They have helped to build the evidence that ...
The essence of the role of chemistry in climate is captured on the cover of this issue. The Earth system is highly coupled. The coupling means that the different environmental issues noted earlier are often connected. ... He has published more than 250 peer-reviewed papers. He played a major role in building an EU stratospheric research program ...
Chemistry in Climate Change. 18 Nov 2014. By Liz Bowley. Here we present a collection of research papers, review articles, and themed collections published by the Royal Society of Chemistry, designed to answer a variety of questions related to the causes and impacts of climate change. From atmospheric chemistry to geochemical cycling and ...
Before understanding the importance of chemistry in general it is essential to know the importance of chemistry in everyday life. 1. Everything on earth is made up of chemicals: you, your food, and the products you use in daily life such as soaps, perfumes etc. all are made up of chemicals. Life without chemicals is impossible. ADVERTISEMENTS: 2.
Chemistry is defined as the science of the composition, structure, properties, and reactions of matter. It has played a major role in pharmaceutical advances, forensic science, and modern agriculture. The simple fact is that chemistry plays an important role in human's life from the moment we're born. It usually begins from the first thing ...
The Role of Chemistry in the Development of Sustainable Energy Sources - Essay; The Chemistry of Tea Brewing - Essay; Biomimicry and Its Applications in Chemistry - Essay; The Impact of p H on the Environment - Essay; The Role of Nanoparticles in Cancer Treatment - Essay; The Chemistry of Ice Cream - Essay
From ancient to modern times, the contributions of Indian scientists to chemistry have been significant. In ancient India, chemistry was known as Rasayan Shastra or Rasatantra or Rasakriya. In the early years after independence, Indian chemists contributed immensely to what came to be called 'reverse engineering' and they perfected the market for the manufacture of cheap generic drugs so much ...
Essay Example: Rosalind Franklin's role in uncovering DNA's structural intricacies represents a compelling and indispensable chapter in scientific history. Despite often being overshadowed by her male counterparts, her endeavors laid the groundwork for comprehending the double helix architecture
This Virtual Special Issue will showcase the crucial role of polymers in enabling new technologies and applications for clean energy. Submit your manuscript by June 30, 2024. The continued transition to clean energy will be enabled by technologies ranging from solar-power generation to energy storage and transport to energy-efficient water and ...
Quantum Bioinorganic Chemistry (QBIC) ... Chemistry - A European Journal. Accepted Articles e202401768. Research Article. Unravelling the Role of Structural Factors in the Luminescence Properties of Persulfurated Benzenes ... Search for more papers by this author. Simone D'Agostino, Simone D'Agostino. Universita di Bologna, Dipartimento di ...
At a conference in 2022, the editor of Trends in Chemistry noticed the strength of mentorship between Stoddart and Feng. When she learned Feng had taken on his own leadership role at OU, she asked Feng to author the paper for the "Emerging Leaders in Chemistry" special issue for the 5th anniversary of Trends in Chemistry and the 50th ...
The essay details her historic space mission in 1992 and her subsequent efforts to promote science education and literacy through the Jemison Group and the Dorothy Jemison Foundation for Excellence. It underscores her ongoing influence as an advocate for diversity and inclusion in STEM fields and her continued impact on science, education, and ...
Angewandte Chemie International Edition is one of the prime chemistry journals in the world, publishing research articles, highlights, communications and reviews across all areas of chemistry. Reactive oxygen species (ROS) play a crucial role in determining photocatalytic reaction pathways, intermediate species, and product selectivity.