- The Open University
- Accessibility hub
- Guest user / Sign out
- Study with The Open University
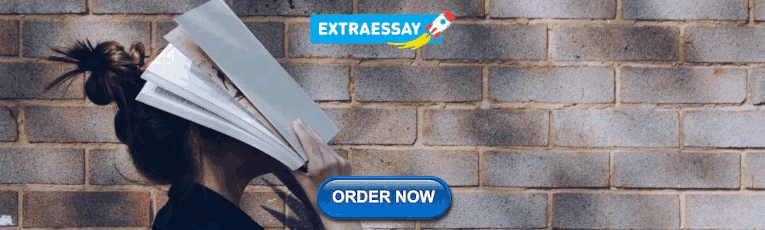
My OpenLearn Profile
Personalise your OpenLearn profile, save your favourite content and get recognition for your learning
About this free course
Become an ou student, download this course, share this free course.

Start this free course now. Just create an account and sign in. Enrol and complete the course for a free statement of participation or digital badge if available.
4 Graphical representation of waves
As you learn more about waves and the different situations in which they occur you will see that it is convenient to represent waves graphically. We can plot a wave as a graph in two ways: as a function of distance and as a function of time . It is important to appreciate the difference between them and the properties of the wave that are represented in both.
Taking the example of a stone splash in a pond, if we take a snapshot of the wave on the surface of a pond at a fixed point in time , such as a freeze frame of Figure 2, and plot the vertical position or displacement of a point at the surface as a function of distance from the point where the stone was dropped, we have a wave represented as a function of distance:

This figure shows a graph. The x-axis is labelled distance, but no tick marks or units are shown. The y-axis is unlabelled apart from an arrow at the top.
A red sine curve – that is, a smoothly curved line rising and falling regularly – starts at the origin, and rises first to a high point labelled ‘peak’. It then falls to an equal distance below the x-axis, labelled trough, and rises again to another peak, and falls to another trough and so on. There are three peaks and two troughs.
Amplitude is shown in three places on the graph. First, a dashed line has been drawn from the first peak back to the y-axis, horizontal to the x-axis, and the distance from the origin to the point where the dashed line crosses the y-axis is labelled ‘amplitude’. Second, the distance from the x-axis to the low point that represents the second trough is also labelled ‘amplitude’. Third, the distance from the x-axis to the top of the third peak is labelled ‘amplitude’. These measurements are all identical in length.
Wavelength is also illustrated on the graph in several places. The distance from the first peak to the second peak is labelled ‘wavelength’. The distance from the first trough to the second trough is labelled ‘wavelength’. Also, a number of dashed lines run horizontally, each joining a point the curve falling from the first peak to the first trough with an equivalent point on the curve joining the second peak to the second trough. These dashed lines are labelled ‘alternative measurements of wavelength’. These measurements are all identical in length.
In the case of the stone splash, the vertical axis represents the displacement of the water from the undisturbed water line, but as you encounter waves in other situations, you will come across different units on the vertical axis such as, for example, intensity or voltage. For this reason the vertical axis is unlabelled in Figure 5, as it represents the generic case of any wave that is plotted as a function of distance.
When a wave is plotted with distance on the horizontal axis, the distance from one peak to the next, or one trough to the next, marks one complete cycle, and this distance is known as the wavelength. Note that the peak to peak or trough to trough are merely the easiest places to measure the wavelength or the period. Any full cycle will do, as shown by the dashed lines in Figure 5. In other words, you can measure the wavelength or period as the distance between any pair of equivalent points, that is, points where the wave has the same 'height' and is changing in the same way (i.e. the gradient is either positive for both points or negative for both points).
The maximum vertical displacement of the wave from the undisturbed surface is the amplitude.
We can also plot a wave as a function of time. Using the same example of the stone splash in a pond, if we now focus on a point on the surface at a fixed distance from where the stone was dropped, such as one of the green points in Figure 3, and plot the vertical displacement as a function of time, we have a wave represented as a function of time:
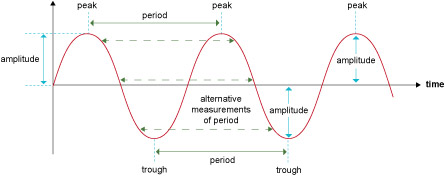
This figure shows a graph that is almost identical to that in Figure 5. The x-axis is labelled time, but no tick marks or units are shown. The y-axis is unlabelled apart from an arrow at the top.
A red sine curve – that is, a smoothly curved line rising and falling regularly – starts at the origin, and rises first to a high point labelled ‘peak’. It then falls to an equal distance below the x axis, labelled trough, and rises again to another peak, and falls to another trough and so on. There are three peaks and two troughs.
Amplitude is once again shown in three places on the graph. First, a dashed line has been drawn from the first peak back to the y-axis, horizontal to the x-axis, and the distance from the origin to the point where the dashed line crosses the y-axis is labelled ‘amplitude’. Second, the distance from the x-axis to the low point that represents the second trough is also labelled ‘amplitude’. Third, the distance from the x-axis to the top of the third peak is labelled ‘amplitude’. These measurements are all identical in length.
However, this graph does not show wavelength. Because the wave is now plotted as a function of time, this graph illustrates the measurement of the period of the wave.
Period is illustrated on the graph in several places. The distance from the first peak to the second peak is labelled ‘period. The distance from the first trough to the second trough is labelled ‘period. Also, a number of dashed lines run horizontally, each joining a point the curve falling from the first peak to the first trough with an equivalent point on the curve joining the second peak to the second trough. These dashed lines are labelled ‘alternative measurements of period. These measurements are all identical in length.
When a wave is plotted with time on the horizontal axis, the time taken from one peak to the next, or one trough to the next, marks one complete cycle, and this time is known as the period. As in the previous graph, the maximum vertical displacement of the wave from the undisturbed surface is the amplitude.
So the definitions of wavelength and period are rather similar. The wavelength is a distance and refers to points separated in space but measured at a fixed instant in time; the period is a time interval and refers to instances separated in time but measured at a fixed point in space.
13.2 Wave Properties: Speed, Amplitude, Frequency, and Period
Section learning objectives.
By the end of this section, you will be able to do the following:
- Define amplitude, frequency, period, wavelength, and velocity of a wave
- Relate wave frequency, period, wavelength, and velocity
- Solve problems involving wave properties
Teacher Support
The learning objectives in this section will help your students master the following standards:
- (B) investigate and analyze the characteristics of waves, including velocity, frequency, amplitude, and wavelength, and calculate using the relationship between wave speed, frequency, and wavelength;
- (D) investigate the behaviors of waves, including reflection, refraction, diffraction, interference, resonance, and the Doppler effect.
Section Key Terms
wavelength | wave velocity |
[BL] [OL] [AL] Review amplitude, period, and frequency for simple harmonic motion.
Wave Variables
In the chapter on motion in two dimensions, we defined the following variables to describe harmonic motion:
- Amplitude—maximum displacement from the equilibrium position of an object oscillating around such equilibrium position
- Frequency—number of events per unit of time
- Period—time it takes to complete one oscillation
For waves, these variables have the same basic meaning. However, it is helpful to word the definitions in a more specific way that applies directly to waves:
- Amplitude—distance between the resting position and the maximum displacement of the wave
- Frequency—number of waves passing by a specific point per second
- Period—time it takes for one wave cycle to complete
In addition to amplitude, frequency, and period, their wavelength and wave velocity also characterize waves. The wavelength λ λ is the distance between adjacent identical parts of a wave, parallel to the direction of propagation. The wave velocity v w v w is the speed at which the disturbance moves.
Tips For Success
Wave velocity is sometimes also called the propagation velocity or propagation speed because the disturbance propagates from one location to another.
Consider the periodic water wave in Figure 13.7 . Its wavelength is the distance from crest to crest or from trough to trough. The wavelength can also be thought of as the distance a wave has traveled after one complete cycle—or one period. The time for one complete up-and-down motion is the simple water wave’s period T . In the figure, the wave itself moves to the right with a wave velocity v w . Its amplitude X is the distance between the resting position and the maximum displacement—either the crest or the trough—of the wave. It is important to note that this movement of the wave is actually the disturbance moving to the right, not the water itself; otherwise, the bird would move to the right. Instead, the seagull bobs up and down in place as waves pass underneath, traveling a total distance of 2 X in one cycle. However, as mentioned in the text feature on surfing, actual ocean waves are more complex than this simplified example.
Watch Physics
Amplitude, period, frequency, and wavelength of periodic waves.
This video is a continuation of the video “Introduction to Waves” from the "Types of Waves" section. It discusses the properties of a periodic wave: amplitude, period, frequency, wavelength, and wave velocity.
The crest of a wave is sometimes also called the peak .
The Relationship between Wave Frequency, Period, Wavelength, and Velocity
Since wave frequency is the number of waves per second, and the period is essentially the number of seconds per wave, the relationship between frequency and period is
just as in the case of harmonic motion of an object. We can see from this relationship that a higher frequency means a shorter period. Recall that the unit for frequency is hertz (Hz), and that 1 Hz is one cycle—or one wave—per second.
The speed of propagation v w is the distance the wave travels in a given time, which is one wavelength in a time of one period. In equation form, it is written as
From this relationship, we see that in a medium where v w is constant, the higher the frequency, the smaller the wavelength. See Figure 13.8 .
[BL] For sound, a higher frequency corresponds to a higher pitch while a lower frequency corresponds to a lower pitch. Amplitude corresponds to the loudness of the sound.
[BL] [OL] Since sound at all frequencies has the same speed in air, a change in frequency means a change in wavelength.
[Figure Support] The same speaker is capable of reproducing both high- and low-frequency sounds. However, high frequencies have shorter wavelengths and are hence best reproduced by a speaker with a small, hard, and tight cone (tweeter), whereas lower frequencies are best reproduced by a large and soft cone (woofer).
These fundamental relationships hold true for all types of waves. As an example, for water waves, v w is the speed of a surface wave; for sound, v w is the speed of sound; and for visible light, v w is the speed of light. The amplitude X is completely independent of the speed of propagation v w and depends only on the amount of energy in the wave.
Waves in a Bowl
In this lab, you will take measurements to determine how the amplitude and the period of waves are affected by the transfer of energy from a cork dropped into the water. The cork initially has some potential energy when it is held above the water—the greater the height, the higher the potential energy. When it is dropped, such potential energy is converted to kinetic energy as the cork falls. When the cork hits the water, that energy travels through the water in waves.
- Large bowl or basin
- Cork (or ping pong ball)
- Measuring tape
Instructions
- Fill a large bowl or basin with water and wait for the water to settle so there are no ripples.
- Gently drop a cork into the middle of the bowl.
- Estimate the wavelength and the period of oscillation of the water wave that propagates away from the cork. You can estimate the period by counting the number of ripples from the center to the edge of the bowl while your partner times it. This information, combined with the bowl measurement, will give you the wavelength when the correct formula is used.
- Remove the cork from the bowl and wait for the water to settle again.
- Gently drop the cork at a height that is different from the first drop.
- Repeat Steps 3 to 5 to collect a second and third set of data, dropping the cork from different heights and recording the resulting wavelengths and periods.
- Interpret your results.
- No, only the amplitude is affected.
- Yes, the wavelength is affected.
Students can measure the bowl beforehand to help them make a better estimation of the wavelength.
Links To Physics
Geology: physics of seismic waves.
Geologists rely heavily on physics to study earthquakes since earthquakes involve several types of wave disturbances, including disturbance of Earth’s surface and pressure disturbances under the surface. Surface earthquake waves are similar to surface waves on water. The waves under Earth’s surface have both longitudinal and transverse components. The longitudinal waves in an earthquake are called pressure waves (P-waves) and the transverse waves are called shear waves (S-waves). These two types of waves propagate at different speeds, and the speed at which they travel depends on the rigidity of the medium through which they are traveling. During earthquakes, the speed of P-waves in granite is significantly higher than the speed of S-waves. Both components of earthquakes travel more slowly in less rigid materials, such as sediments. P-waves have speeds of 4 to 7 km/s, and S-waves have speeds of 2 to 5 km/s, but both are faster in more rigid materials. The P-wave gets progressively farther ahead of the S-wave as they travel through Earth’s crust. For that reason, the time difference between the P- and S-waves is used to determine the distance to their source, the epicenter of the earthquake.
We know from seismic waves produced by earthquakes that parts of the interior of Earth are liquid. Shear or transverse waves cannot travel through a liquid and are not transmitted through Earth’s core. In contrast, compression or longitudinal waves can pass through a liquid and they do go through the core.
All waves carry energy, and the energy of earthquake waves is easy to observe based on the amount of damage left behind after the ground has stopped moving. Earthquakes can shake whole cities to the ground, performing the work of thousands of wrecking balls. The amount of energy in a wave is related to its amplitude. Large-amplitude earthquakes produce large ground displacements and greater damage. As earthquake waves spread out, their amplitude decreases, so there is less damage the farther they get from the source.
Grasp Check
What is the relationship between the propagation speed, frequency, and wavelength of the S-waves in an earthquake?
- The relationship between the propagation speed, frequency, and wavelength is v w = f λ . v w = f λ .
- The relationship between the propagation speed, frequency, and wavelength is v w = λ f . v w = λ f .
Virtual Physics
Wave on a string.
In this animation, watch how a string vibrates in slow motion by choosing the Slow Motion setting. Select the No End and Manual options, and wiggle the end of the string to make waves yourself. Then switch to the Oscillate setting to generate waves automatically. Adjust the frequency and the amplitude of the oscillations to see what happens. Then experiment with adjusting the damping and the tension.
Which of the settings—amplitude, frequency, damping, or tension—changes the amplitude of the wave as it propagates? What does it do to the amplitude?
- Frequency; it decreases the amplitude of the wave as it propagates.
- Frequency; it increases the amplitude of the wave as it propagates.
- Damping; it decreases the amplitude of the wave as it propagates.
- Damping; it increases the amplitude of the wave as it propagates.
Solving Wave Problems
Worked example, calculate the velocity of wave propagation: gull in the ocean.
Calculate the wave velocity of the ocean wave in the previous figure if the distance between wave crests is 10.0 m and the time for a seagull to bob up and down is 5.00 s.
The values for the wavelength ( λ = 10.0 m ) ( λ = 10.0 m ) and the period ( T = 5.00 s) ( T = 5.00 s) are given and we are asked to find v w v w Therefore, we can use v w = λ T v w = λ T to find the wave velocity.
Enter the known values into v w = λ T v w = λ T
This slow speed seems reasonable for an ocean wave. Note that in the figure, the wave moves to the right at this speed, which is different from the varying speed at which the seagull bobs up and down.
Calculate the Period and the Wave Velocity of a Toy Spring
The woman in Figure 13.3 creates two waves every second by shaking the toy spring up and down. (a)What is the period of each wave? (b) If each wave travels 0.9 meters after one complete wave cycle, what is the velocity of wave propagation?
Strategy FOR (A)
To find the period, we solve for T = 1 f T = 1 f , given the value of the frequency ( f = 2 s − 1 ). ( f = 2 s − 1 ).
Enter the known value into T = 1 f T = 1 f
Strategy FOR (B)
Since one definition of wavelength is the distance a wave has traveled after one complete cycle—or one period—the values for the wavelength ( λ = 0.9 m ) ( λ = 0.9 m ) as well as the frequency are given. Therefore, we can use v w = f λ v w = f λ to find the wave velocity.
Enter the known values into v w = f λ v w = f λ
v w = f λ = ( 2 s −1 )(0 .9 m) = 1 .8 m/s . v w = f λ = ( 2 s −1 )(0 .9 m) = 1 .8 m/s .
We could have also used the equation v w = λ T v w = λ T to solve for the wave velocity since we already know the value of the period ( T = 0.5 s) ( T = 0.5 s) from our calculation in part (a), and we would come up with the same answer.
Practice Problems
The frequency of a wave is 10 Hz. What is its period?
- The period of the wave is 100 s.
- The period of the wave is 10 s.
- The period of the wave is 0.01 s.
- The period of the wave is 0.1 s.
What is the velocity of a wave whose wavelength is 2 m and whose frequency is 5 Hz?
Check Your Understanding
Use these questions to assess students’ achievement of the section’s Learning Objectives. If students are struggling with a specific objective, these questions will help identify such objective and direct them to the relevant content.
What is the amplitude of a wave?
- A quarter of the total height of the wave
- Half of the total height of the wave
- Two times the total height of the wave
- Four times the total height of the wave
- The wavelength is the distance between adjacent identical parts of a wave, parallel to the direction of propagation.
- The wavelength is the distance between adjacent identical parts of a wave, perpendicular to the direction of propagation.
- The wavelength is the distance between a crest and the adjacent trough of a wave, parallel to the direction of propagation.
- The wavelength is the distance between a crest and the adjacent trough of a wave, perpendicular to the direction of propagation.
- f = ( 1 T ) 2
- f = ( T ) 2
When is the wavelength directly proportional to the period of a wave?
- When the velocity of the wave is halved
- When the velocity of the wave is constant
- When the velocity of the wave is doubled
- When the velocity of the wave is tripled
This book may not be used in the training of large language models or otherwise be ingested into large language models or generative AI offerings without OpenStax's permission.
Want to cite, share, or modify this book? This book uses the Creative Commons Attribution License and you must attribute Texas Education Agency (TEA). The original material is available at: https://www.texasgateway.org/book/tea-physics . Changes were made to the original material, including updates to art, structure, and other content updates.
Access for free at https://openstax.org/books/physics/pages/1-introduction
- Authors: Paul Peter Urone, Roger Hinrichs
- Publisher/website: OpenStax
- Book title: Physics
- Publication date: Mar 26, 2020
- Location: Houston, Texas
- Book URL: https://openstax.org/books/physics/pages/1-introduction
- Section URL: https://openstax.org/books/physics/pages/13-2-wave-properties-speed-amplitude-frequency-and-period
© Jun 7, 2024 Texas Education Agency (TEA). The OpenStax name, OpenStax logo, OpenStax book covers, OpenStax CNX name, and OpenStax CNX logo are not subject to the Creative Commons license and may not be reproduced without the prior and express written consent of Rice University.

CIE A-Level Physics Notes
7.2.2 graphical wave analysis, understanding graphical representations of waves, transverse waves.
- Transverse waves are characterised by the motion of particles perpendicular to the wave's direction of travel.
- Crests signify the highest point, where particles experience the maximum positive displacement.
- Troughs represent the lowest point, indicating the maximum negative displacement.
- The amplitude of a wave, indicating its energy, is the vertical distance from the rest position to a crest or a trough.
- Wavelength , denoted as λ , is the horizontal distance between successive crests or troughs, crucial in determining wave properties.
- The frequency (f) , representing the number of waves passing a point per unit time, is inversely proportional to the wavelength.
Longitudinal Waves
- Longitudinal waves involve particle motion parallel to the wave's direction.
- Compressions are regions of high particle density.
- Rarefactions are areas of low particle density.
- The amplitude in longitudinal waves correlates with the density difference in compressions and rarefactions.
- The wavelength is the distance from one compression to the next.
- Frequency in longitudinal waves follows the same principles as in transverse waves.

Particle movement in Longitudinal wave and transverse wave
Image Courtesy Labster.com
Techniques for Analysing Wave Graphs
Wave speed calculation.
- Wave speed ( v ) is a critical property, calculated using v = f × λ .
- Accurate calculation of wave speed requires precise measurements of frequency and wavelength from the graph.

Frequency and Period Determination
- Frequency is calculated by counting the number of complete waves passing a point in a unit of time.
- The period (T) , the duration of one complete wave cycle, is calculated as T = 1/ f .
- Graphs allow for visual determination of these values, aiding in deeper wave analysis.

Period and Frequency of a Wave
Image Courtesy Geeksforgeeks
Phase Difference and Wave Interference
- Phase difference is essential in analysing wave interference and superposition.
- It is visually represented as the horizontal distance between similar points (like crest to crest) on different waves.
- Understanding phase differences helps in analysing phenomena like constructive and destructive interference.

Phase difference and interference
Image Courtesy Dina Zhabinskaya
Case Studies in Scientific Research and Industry
Seismology: earthquake wave analysis.
- Seismology heavily relies on understanding transverse (S-waves) and longitudinal waves (P-waves).

S-wave vs P-wave
Image Courtesy BYJU’s
- Seismographs capture these waves, enabling scientists to interpret and locate the epicentre of earthquakes.
- The distinct graphical representation of these waves aids in differentiating them and understanding the earth's internal structure.
Medical Imaging: Ultrasound Technology
- Ultrasound uses high-frequency longitudinal waves to create images of internal body structures.
- By analysing the time delay and intensity of the wave reflections, technicians can construct detailed internal images.
- Graphical analysis is crucial in determining the boundaries and densities of different tissues.
Communications Technology: Radio and Sound Waves
- Communication technologies depend on the graphical analysis of transverse electromagnetic waves and sound waves.
- Graphical representations help in adjusting frequencies and wavelengths for optimal signal transmission and reception.
Industrial Non-destructive Testing (NDT)
- NDT methods like ultrasonic testing utilise both transverse and longitudinal waves to inspect materials.
- Engineers analyse the reflected wave patterns graphically to detect internal flaws or discontinuities without causing damage.
Oceanography: Tsunami Wave Analysis
- In oceanography, particularly in tsunami monitoring, the analysis of wave patterns is vital.
- Graphical data from buoys and satellite measurements help in predicting tsunami formation and propagation.
Astronomy: Radio Wave Analysis
- Astronomers analyse radio waves emitted by celestial bodies to gather information about the universe.
- Graphical analysis of these waves provides insights into the composition, temperature, and movement of distant stars and galaxies.
Everyday Applications of Graphical Wave Analysis
- Graphical wave analysis extends beyond laboratories to everyday technologies like WiFi, smartphones, and television broadcasting.
- Analysing transverse electromagnetic waves is crucial for maintaining efficient communication networks.
Wave polarization is a feature of transverse waves and is represented graphically by the direction of the wave's oscillation. In a polarized wave, the oscillation occurs in a single plane, which can be depicted on a graph as consistent displacements along a specific axis. For example, in an electromagnetic wave, polarization could be represented by the electric field oscillating only in the vertical or horizontal direction. This concept does not apply to longitudinal waves, as their oscillations occur in the direction of wave propagation. Polarization is a key concept in many areas of physics, including optics, where it is crucial in understanding light behaviour, and in telecommunications, where it helps in the design of antennas and the analysis of signal transmission.
Damping effects in a wave are depicted graphically as a gradual decrease in the amplitude of the wave over time or distance. In a displacement-time graph, a damped wave will show peaks (crests) and valleys (troughs) that progressively become less pronounced. This reduction in amplitude is due to the energy of the wave being lost to the surrounding environment, commonly as heat. Damping is an important concept in real-world applications, as most waves will experience some form of damping. It is especially significant in mechanical systems where excessive vibrations can be harmful, and in acoustics, where damping affects the quality and duration of sound.
Harmonics in wave analysis refer to the integer multiples of a fundamental frequency of a wave. Graphically, harmonics are represented as waveforms with frequencies that are two times, three times, or higher multiples of the fundamental frequency. The first harmonic is the fundamental frequency, the second harmonic is twice the fundamental frequency, and so on. In a graph, these harmonics can be identified by their shorter wavelengths and increased frequencies as compared to the fundamental. Understanding harmonics is crucial in musical acoustics for the analysis of musical notes, in electrical engineering for analysing alternating current circuits, and in various other fields where wave behaviour is essential. Harmonics add complexity to a wave and can influence the overall shape and behaviour of the waveform observed in the graphical analysis.
The area under a wave graph does not have a direct physical significance in the same way it does in other types of graphs, like those representing motion in mechanics. In wave graphs, the key information is conveyed through the amplitude, wavelength, frequency, and phase of the wave, rather than the area under the curve. For instance, in a displacement-time graph for a wave, the area under the curve does not represent a physical quantity like energy or work, which is often the case in other areas of physics. Wave graphs are primarily used to understand and analyze wave properties such as speed, amplitude, wavelength, and frequency, rather than quantities that might be represented by the area under the graph.
The energy of a wave is directly related to its amplitude, which is clearly represented in its graphical depiction. In the graph of a wave, the amplitude is the maximum displacement of the wave from its rest position (either above or below). A higher amplitude indicates a wave carrying more energy. This is because the energy of a wave is proportional to the square of its amplitude. Therefore, a wave with twice the amplitude of another will have four times the energy. This relationship is crucial in applications such as sound waves, where louder sounds have larger amplitudes and thus more energy, and in electromagnetic waves, where the intensity of light is related to the amplitude of the wave.
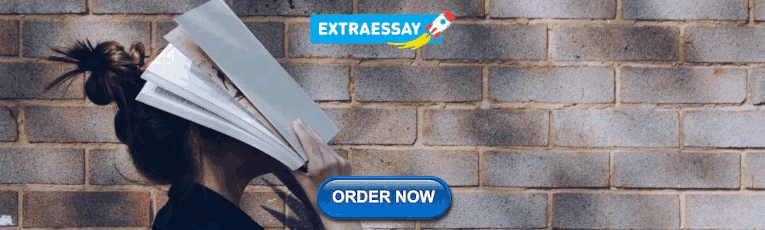
Practice Questions
The speed of a wave is calculated using the formula: speed = frequency x wavelength. For the longitudinal wave, the speed is 20 Hz×0.5 m=10 m/s. For the transverse wave, the speed is 25 Hz×0.4 m=10. Surprisingly, both waves travel at the same speed of 10 m/s. This result demonstrates that wave speed is independent of the wave type (longitudinal or transverse) and solely depends on the wave's frequency and wavelength.
The phase difference between Point A (crest) and Point B (trough) is half a wavelength, or 180 degrees. In wave analysis, this phase difference is significant because it represents a point of complete destructive interference if these points were to overlap with another wave. For example, if two identical waves were superimposed such that Point A of one wave aligns with Point B of another, they would cancel each other out at these points. Understanding phase differences is crucial for analysing phenomena like interference patterns, which are fundamental in various physics applications like noise cancellation and wave-based imaging techniques.

Shubhi is a seasoned educational specialist with a sharp focus on IB, A-level, GCSE, AP, and MCAT sciences. With 6+ years of expertise, she excels in advanced curriculum guidance and creating precise educational resources, ensuring expert instruction and deep student comprehension of complex science concepts.
Hire a tutor
Please fill out the form and we'll find a tutor for you.
Learning Introductory Physics with Activities
Paul J. Emigh, Rebecka Tumblin, Kathryn Hadley, Danielle Skinner
Search Results:
Section 7.2 sinusoidal waves.
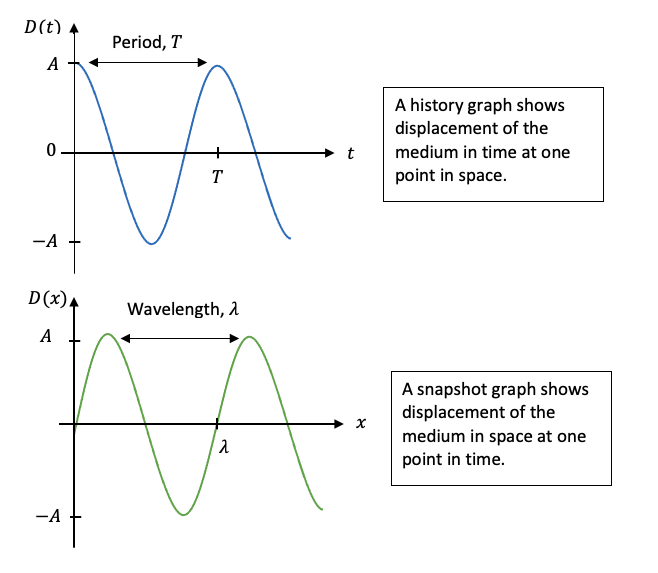
Exercises Activities
1 . exploring wavenumber., 2 . graphing phase..
- \(\displaystyle \sin{(kx)} \)
- \(\displaystyle \sin{(kx + \frac{\pi}{3})}\)
- \(\displaystyle \sin{(kx + \frac{\pi}{6})}\)
- \(\displaystyle \sin{(kx - \frac{\pi}{3})}\)
3 . Exploring Phase.
References references.
[1] Gif image in Figure 7.2.1 courtesy of Veritasium. https://www.youtube.com/watch?v=Iuv6hY6zsd0
[2] Figure 7.2.2 created by Rebecka Tumblin.
[3] Animations courtesy of Dr. Dan Russell, Grad. Prog. Acoustics, Penn State. licensed under a Creative Commons Attribution-NonCommercial-NoDerivatives 4.0 International License. For more information see https://www.acs.psu.edu/drussell/demos.html
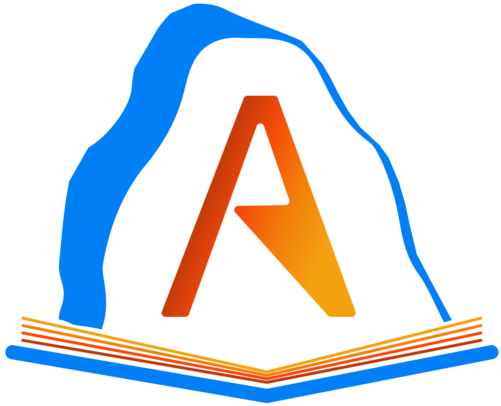
Graphs of Sine, Cosine and Tangent
A sine wave made by a circle:
A sine wave produced naturally by a bouncing spring:
Plot of Sine
The Sine Function has this beautiful up-down curve (which repeats every 2 π radians, or 360°).
It starts at 0 , heads up to 1 by π /2 radians (90°) and then heads down to −1 .
Plot of Cosine
Cosine is just like Sine, but it starts at 1 and heads down until π radians (180°) and then heads up again.
Plot of Sine and Cosine
In fact Sine and Cosine are like good friends : they follow each other, exactly π /2 radians (90°) apart.
Plot of the Tangent Function
The Tangent function has a completely different shape ... it goes between negative and positive Infinity , crossing through 0, and at every π radians (180°), as shown on this plot.
At π /2 radians (90°), and at − π /2 (−90°), 3 π /2 (270°), etc, the function is officially undefined , because it could be positive Infinity or negative Infinity .
Inverse Sine, Cosine and Tangent
The Inverse Sine, Cosine and Tangent graphs are:
Mirror Images
Here is Cosine and Inverse Cosine plotted on the same graph:
They are mirror images (about the diagonal)!
The same is true for Sine and Inverse Sine and for Tangent and Inverse Tangent . Can you see this in the graphs above?
Get in touch with us
Are you sure you want to logout?
Please select your grade.
- Earth and space

Graphical Representation of Sound Wave
Key concepts.
- Graphical representation of sound wave
- Oscillations
- Time period
- Relation between frequency and time period
Introduction:
Sound waves can be graphically represented by plotting the density or the pressure variation against the distance at a particular instant of time. The sound waves are characterized by various quantities such as wavelength, frequency, time-period etc., which are indicated via a graphical representation of the sound wave. We will be looking at these characteristics of sound wave in this section.
Explanation:
Graphical representation of sound wave: .
The density and/or pressure variations can be plotted against distance at a particular instant of time. The graph below shows the way in which the density or pressure of the medium vary when a sound wave propagates through it.
Compressions are the regions of high pressure and density and is represented by the upper portions of the curve.
Rarefactions are the regions of low pressure and density and is represented by the lower portions of the curve. i.e., the valleys.
The peaks represent the regions of maximum compression, i.e., they represent the highest density and pressure.

The bottom most points of the valleys represent the regions of minimum compression or maximum rarefaction, i.e., they represent the lowest density and pressure.
A peak is called a crest and a valley is called a trough of a wave.

Wavelength:
The distance between any two consecutive compressions or rarefactions is called the wavelength . Wavelength of a wave is denoted by a Greek letter “ λ ” called lambda . The SI unit of measurement of wavelength is meter (m) .
Frequency:
Frequency refers to how frequently an event takes place. The frequency of beating a drum refers to the number of times the drum is beaten in a unit time. The frequency of getting sixes when dice are thrown refers to the number of times the sixes are obtained on throwing the dice.
The propagation of a sound wave is characterized by the motion of alternate compressions and rarefactions, i.e., crests and troughs. The number of compressions or rarefactions that cross a particular point in a unit time is called the frequency of the wave. The frequency of a wave is denoted by a Greek letter “ ν ”called “nu” and is measured in the SI unit of Hertz (Hz) .
Oscillation:
When a sound wave propagates through a medium the density of a medium oscillates between a maximum and a minimum value.
This change in density or pressure from its maximum value to its minimum value and again to its maximum value makes one complete oscillation .
The parts of a wave which can be referred to as one complete oscillation, are shown by red dashed box in each picture below. Any of these can be referred to as a complete oscillation.

Time period:
The time taken by a wave to complete one oscillation is called its time period.
In other words, the time taken by two consecutive compressions or rarefactions to cross a fixed point is called time period .
It is denoted by a letter ‘ T’ and is measured in the SI units of ‘ seconds.’
Relation between frequency and time period:
The frequency and time period are related by the following formula.
This formula can be used to calculate frequency of a sound wave when its time period is given and vice versa.
Questions and Answers:
- Calculate the frequency of a sound wave whose time period is 0.001 s.
Time period = 0.001 s
The frequency is given by, ν = 1/T
Therefore, frequency = 1/0.001
frequency = 1000 Hz
- Calculate the time period of the sound wave whose frequency is 50 Hz.
Frequency = 50 Hz
Thus, the time period is given by, T = 1/ν
Therefore, time period = 1/50
time period = 0.02 s
- The propagation of sound waves can br graphically represented by plotting the pressure or the density variations against distance at a particular instant of time.
- The crests represent the region with maximum compression and the troughs represent the region of minimum compression.
- The distance between any two consecutive compressions or rarefactions is called the wavelength (X).
- The number of compressions or rarefactions that cross a particular point in a unit time is called the frequency (V) of the wave.
- The change in density or pressure from its maximum value to its minimum value and again to its maximum value makes one complete oscillation.
- The time taken by two consecutive compressions or rarefactions to cross a fixed point is called time period.
- The SI units of wavelength, frequency and time period are m, Hz and s respectively.
- The frequency and time period are related by the following formula. v=1/T
Related topics
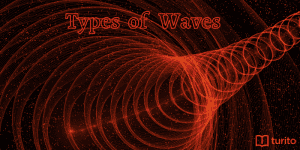
Different Types of Waves and Their Examples
Introduction: We can’t directly observe many waves like light waves and sound waves. The mechanical waves on a rope, waves on the surface of the water, and a slinky are visible to us. So, these mechanical waves can serve as a model to understand the wave phenomenon. Explanation: Types of Waves: Fig:1 Types of waves […]
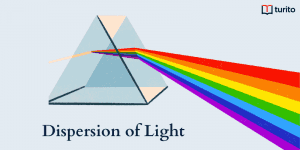
Dispersion of Light and the Formation of Rainbow
Introduction: Visible Light: Visible light from the Sun comes to Earth as white light traveling through space in the form of waves. Visible light contains a mixture of wavelengths that the human eye can detect. Visible light has wavelengths between 0.7 and 0.4 millionths of a meter. The different colors you see are electromagnetic waves […]
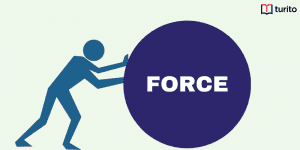
Force: Balanced and Unbalanced Forces
Introduction: In a tug of war, the one applying more force wins the game. In this session, we will calculate this force that makes one team win and one team lose. We will learn about it in terms of balanced force and unbalanced force. Explanation: Force Force is an external effort that may move a […]
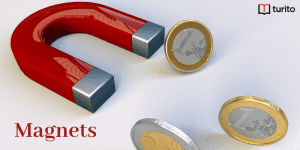
Magnets: Uses, Materials, and Their Interactions
Introduction: Nowadays magnets are widely used for many applications. In this session, we will discuss the basics of magnets and their properties, and the way they were and are used. Explanation: Magnets: Magnetic and Non-magnetic Materials: Poles of a Magnet: Fig No. 1.2: Poles of a magnet Compass: Interaction Between Magnets: The north pole of […]

Other topics

How to Find the Area of Rectangle?

How to Solve Right Triangles?

Ways to Simplify Algebraic Expressions
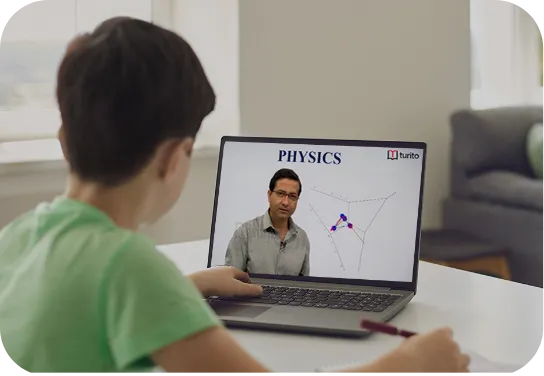
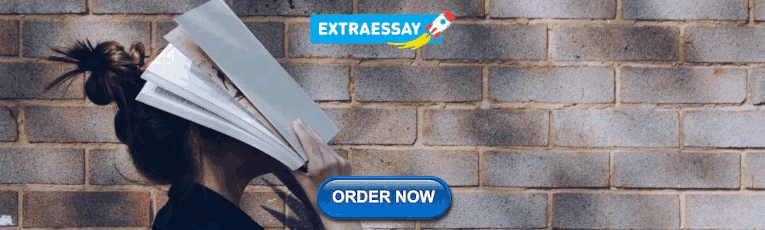
IMAGES
VIDEO
COMMENTS
4 Graphical representation of waves. As you learn more about waves and the different situations in which they occur you will see that it is convenient to represent waves graphically. We can plot a wave as a graph in two ways: as a function of distance and as a function of time. It is important to appreciate the difference between them and the ...
This video complements the lecture notes published at xmphysics.comA-level Physics Learning ResourcesCreated by Mr Chua Kah Hean (http://www.xmphysics.com)
Revision notes on 4.6.4 Graphical Representations of Transverse & Longitudinal Waves for the OCR A Level Physics syllabus, written by the Physics experts at Save My Exams.
Waves may be graphed as a function of time or distance. A single frequency wave will appear as a sine wave in either case. From the distance graph the wavele...
• The graphical representation of all the particle displacements at a given instant is shown in part (b). ... • Any wave can be expressed in terms of sinusoidally varying waves. (sine waves form a complete orthonormal set) • The figure shows a wave pulse obtained by adding together a
Waves: basic terms & graphical representation. Teaching Guidance for 14-16. Some basic ideas about waves can be demonstrated using mechanical waves along strings or springs, ripples on water, or special wave machines. The system carrying the waves is called the medium. A displacement against distance graph, or wave profile, shows the ...
Teacher Support [BL] For sound, a higher frequency corresponds to a higher pitch while a lower frequency corresponds to a lower pitch. Amplitude corresponds to the loudness of the sound. [BL] [OL] Since sound at all frequencies has the same speed in air, a change in frequency means a change in wavelength. [Figure Support] The same speaker is capable of reproducing both high- and low-frequency ...
This video complements the lecture notes published at xmphysics.comA-level Physics Learning ResourcesCreated by Mr Chua Kah Hean (http://www.xmphysics.com)
A waveform is a graphical representation of a signal in the form of a wave. It can be both sinusoidal and square-shaped, depending on the type of wave-generating input. There are two types of ...
Understanding Graphical Representations of Waves Transverse Waves. Transverse waves are characterised by the motion of particles perpendicular to the wave's direction of travel. In graphs, these waves display alternating crests and troughs. Crests signify the highest point, where particles experience the maximum positive displacement.
An important aspect of sinusoidal waves is that they are periodic in both space and time.The displacement \(D(x,t) \) of a particle in the medium depends on both the particle's position \(x \) and the time \(t\) when you observe it. In Figure 7.2.2, we show a graphical representation of a sinusoidal wave in space and time.The period of the wave, is related to the frequency of the wave
Graphical Representation y y vs t: Motion of a single point in the medium y vs x: Snap shot of the whole wave at one instant. Liu UCD Phy9B 07 16 y y =Acos(- ωt) = Acosωt x=0 ... Wave equation: Title: Microsoft PowerPoint - Ch15 Author: Kai Created Date: 10/2/2007 5:11:32 PM ...
Plot of the Tangent Function. The Tangent function has a completely different shape ... it goes between negative and positive Infinity, crossing through 0, and at every π radians (180°), as shown on this plot. At π /2 radians (90°), and at − π /2 (−90°), 3 π /2 (270°), etc, the function is officially undefined, because it could be ...
A sound wave can be represented by a variation of local pressure from atmospheric or a variation in the "displacement" of molecules. Consider this very simplistic "photograph" of a sound wave at an instant of time. At the top is representation of the state of the air without a sound wave being present and at the bottom with a sound wave present.
This video complements the lecture notes published at xmphysics.comA-level Physics Learning ResourcesCreated by Mr Chua Kah Hean (http://www.xmphysics.com)
Sound waves can be graphically represented by plotting the density or the pressure variation against the distance at a particular instant of time. The sound waves are characterized by various quantities such as wavelength, frequency, time-period etc., which are indicated via a graphical representation of the sound wave.