Scientific Research and Methodology
5.2 precision and accuracy.
Two issues concerning sampling, raised in Sect. 5.1 , were: which individuals should be in the sample, and how many individuals should be in the sample be. These two issues address two different aspects of sampling: precision and accuracy (Fig. 5.1 ).
Accuracy refers to how close a sample estimate is to the population value (on average ). Precision refers to how close all the possible sample estimates are likely to be (that is, how much variation is likely in the sample estimates).
Using this language:
- The type of sampling (i.e., the way in which the samples in selected) impacts the accuracy of the sample estimate. In other words, the type of sampling impacts the external validity of the study.
- The size of the sample impacts the precision of the sample estimate.
For example, large samples are more likely to be precise estimates because each possible sample value will produced similar estimates, but they may or may not be accurate estimates. Similarly, random samples are likely to produce accurate estimates (and hence the study is more likely to be externally valid), but they may not be precise unless the sample is also large.

FIGURE 5.1: Precision and accuracy: Each coloured dot is like a sample estimate of the population value (shown by the black central dot)

Example 5.2 (Precision and accuracy) To estimate the average age of all Queenslanders , we could ask 9000 Queensland school children (a large sample indeed!).
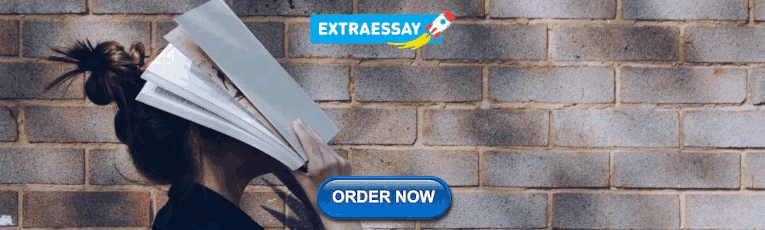
What Is the Difference Between Accuracy and Precision?
Accuracy is close to a known value; precision measures repeatability
boonchai wedmakawand / Getty Images
- Chemical Laws
- Periodic Table
- Projects & Experiments
- Scientific Method
- Biochemistry
- Physical Chemistry
- Medical Chemistry
- Chemistry In Everyday Life
- Famous Chemists
- Activities for Kids
- Abbreviations & Acronyms
- Weather & Climate
- Ph.D., Biomedical Sciences, University of Tennessee at Knoxville
- B.A., Physics and Mathematics, Hastings College
Accuracy and precision are two important factors to consider when taking data measurements . Both accuracy and precision reflect how close a measurement is to an actual value, but accuracy reflects how close a measurement is to a known or accepted value, while precision reflects how reproducible measurements are, even if they are far from the accepted value.
Key Takeaways: Accuracy Versus Precision
- Accuracy is how close a value is to its true value. An example is how close an arrow gets to the bull's-eye center.
- Precision is how repeatable a measurement is. An example is how close a second arrow is to the first one (regardless of whether either is near the mark).
- Percent error is used to assess whether a measurement is sufficiently accurate and precise.
You can think of accuracy and precision in terms of hitting a bull's-eye. Accurately hitting the target means you are close to the center of the target, even if all the marks are on different sides of the center. Precisely hitting a target means all the hits are closely spaced, even if they are very far from the center of the target. Measurements that are both precise and accurate are repeatable and very near true values.
There are two common definitions of accuracy . In math, science, and engineering, accuracy refers to how close a measurement is to the true value.
The ISO ( International Organization for Standardization ) applies a more rigid definition, where accuracy refers to a measurement with both true and consistent results. The ISO definition means an accurate measurement has no systematic error and no random error. Essentially, the ISO advises that accurate be used when a measurement is both accurate and precise.
Precision is how consistent results are when measurements are repeated. Precise values differ from each other because of random error, which is a form of observational error.
You can think of accuracy and precision in terms of a basketball player. If the player always makes a basket, even though he strikes different portions of the rim, he has a high degree of accuracy. If he doesn't make many baskets but always strikes the same portion of the rim, he has a high degree of precision. A player whose free throws always make the basket the exact same way has a high degree of both accuracy and precision.
Take experimental measurements for another example of precision and accuracy. You can tell how close a set of measurements are to a true value by averaging them . If you take measurements of the mass of a 50.0-gram standard sample and get values of 47.5, 47.6, 47.5, and 47.7 grams, your scale is precise, but not very accurate. The average of your measurements is 47.6, which is lower than the true value. Yet, your measurements were consistent. If your scale gives you values of 49.8, 50.5, 51.0, and 49.6, it is more accurate than the first balance but not as precise. The average of the measurements is 50.2, but there is a much larger range between them. The more precise scale would be better to use in the lab, providing you made an adjustment for its error. In other words, it's better to calibrate a precise instrument than to use an imprecise, yet accurate one.
Mnemonic to Remember the Difference
An easy way to remember the difference between accuracy and precision is:
- A C curate is C orrect (or C lose to real value)
- P R ecise is R epeating (or R epeatable)
Accuracy, Precision, and Calibration
Do you think it's better to use an instrument that records accurate measurements or one that records precise measurements? If you weigh yourself on a scale three times and each time the number is different, yet it's close to your true weight, the scale is accurate. Yet it might be better to use a scale that is precise, even if it is not accurate. In this case, all the measurements would be very close to each other and "off" from the true value by about the same amount. This is a common issue with scales, which often have a "tare" button to zero them.
While scales and balances might allow you to tare or make an adjustment to make measurements both accurate and precise, many instruments require calibration. A good example is a thermometer . Thermometers often read more reliably within a certain range and give increasingly inaccurate (but not necessarily imprecise) values outside that range. To calibrate an instrument, record how far off its measurements are from known or true values. Keep a record of the calibration to ensure proper readings. Many pieces of equipment require periodic calibration to ensure accurate and precise readings.
Accuracy and precision are only two important concepts used in scientific measurements. Two other important skills to master are significant figures and scientific notation . Scientists use percent error as one method of describing how accurate and precise a value is. It's a simple and useful calculation.
- Accuracy Definition in Science
- Tips and Rules for Determining Significant Figures
- Random Error vs. Systematic Error
- How to Measure Mass Using a Balance
- Measurement Definition in Science
- How to Calculate Percent Error
- Absolute and Relative Error Calculation
- The Difference Between Celsius and Centigrade
- How to Calculate Experimental Error in Chemistry
- Make Your Own Biltmore Cruiser Stick
- How to Measure Distances on a Map
- The Relative Uncertainty Formula and How to Calculate It
- Accurately Measure Elapsed Time Using Delphi Performance Counter
- The History of the Thermometer
- Potassium-Argon Dating Methods
- Using Significant Figures in Precise Measurement

- Science Notes Posts
- Contact Science Notes
- Todd Helmenstine Biography
- Anne Helmenstine Biography
- Free Printable Periodic Tables (PDF and PNG)
- Periodic Table Wallpapers
- Interactive Periodic Table
- Periodic Table Posters
- How to Grow Crystals
- Chemistry Projects
- Fire and Flames Projects
- Holiday Science
- Chemistry Problems With Answers
- Physics Problems
- Unit Conversion Example Problems
- Chemistry Worksheets
- Biology Worksheets
- Periodic Table Worksheets
- Physical Science Worksheets
- Science Lab Worksheets
- My Amazon Books
What Is the Difference Between Accuracy and Precision?

Accuracy and precision are two important concepts in science. Both terms apply to any experimental measurement you make. But what do they actually mean? What is the difference between them and which is more important?
What Is Accuracy?

Accuracy is a measure of how closely your experimental measurements agree with known values. The closer your measurements are to the known value, the more accurate the measurement.
If you think of the known value as the bullseye on a target, accurate shots (or measurements) mean the average of your shots is at or near the bullseye. The shots in this picture are accurate, but they are all over the target. This brings up the concept of precision.
What Is Precision?

Precision is a measure of how close your experimental measurements agree with each other. The closer each measurement is to the other measurements, the more precise your measurement.
If the measurements were shots in a target, the closer the holes are to each other, the more precise the shots. Here, the shots are all clustered together. They would be considered a precise collection of shots. The only problem is they are not very accurate.
How to Control Accuracy and Precision

Both accuracy and precision are the goal of any measurement. Variations in accuracy and precision are largely controllable.
In target shooting, you improve accuracy by moving closer to the target, or using an aiming aid like a scope or laser pointer. You improve precision by mounting your gun to a table or bench or shooting indoors out of the wind.
In a lab, improve accuracy and precision by using the same procedure taking each measurement and using tools designed for measurements. For example, you get a more accurate and precise volume measurement using a graduated cylinder than you do using a water glass.
In general, use good tools designed for the measurement you are trying to make and pay close attention to what you are doing when you use them. This helps any measurement you make.
Which Is More Important? Accuracy or Precision?

Accuracy is more important when trying to hit a target. You either hit your target or you don’t. It does no good to be precise if you miss all your shots. You can improve accuracy in future measurements by factoring in a correction factor. If your shots are all going to the left, alter your aim to the right. Accuracy is something you can fix in future measurements.
Precision is more important in calculations. When using a measured value in a calculation, you can only be as precise as your least precise measurement. This is the main idea behind the topic of significant figures in calculations. You can improve precision by using a better measuring tool and/or improving your skill at using the tool.
Accuracy and precision are both important to good measurements in science. Try to make the best of both in all your measurements. But, no matter how hard you try, measurements always deviate slightly from true values. This is because of error .
Related Posts
Precision and Accuracy
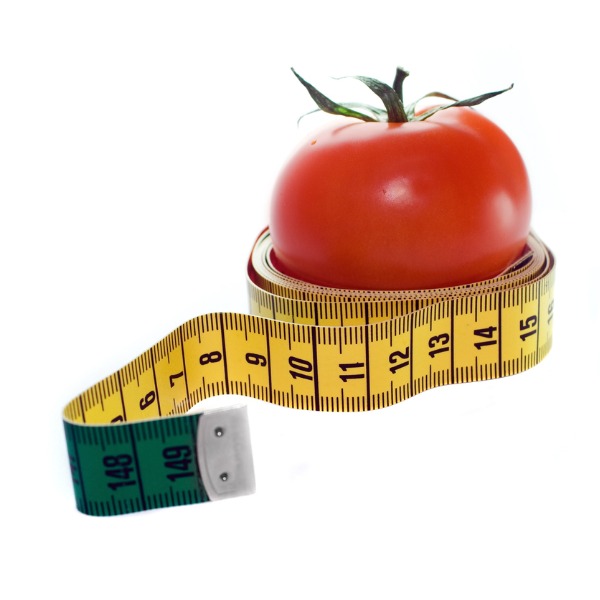
A measuring tape wrapped around a tomato (Michael Bodmann, iStockphoto)
How does this align with my curriculum?
Share on: facebook x/twitter linkedin pinterest.
Learn about precision and accuracy and why they are important in science.
In order to get the most reliable results in a scientific inquiry, it is important to minimize bias and error , as well as to be precise and accurate in the collection of data. Both accuracy and precision have to do with how close a measurement is to its actual or true value.
What is the difference between “Precision” and “Accuracy”?
In everyday life, students may use the terms “precision” and “accuracy” interchangeably, but they have different meanings in math, science and experimental inquiry.
Accuracy refers to how close a measured value is to the actual (‘true’) value. For example, if you were to weigh a standard 100g weight on a scale, an accurate reading for that weight would be as close as possible to 100g. If you got a reading of 105g, then you would say that you were not getting an accurate reading (and your scale would need calibrating).
But what if you don’t know what the “true” value for something is? You still want to be able to take measurements that are as true as possible. Certain devices and tools allow you to be more accurate than others. For example, let’s say that you had two groups of students measuring the width of a tomato plant stem. One group used plastic rulers that only measured to the millimeter (mm). The students had to determine whether they thought the reading was closer to 3 or 4 mm (see below). Another group of students used Vernier calipers to take the measurements. They were able to measure to a tenth of a millimeter. If several measurements are made with a more precise instrument, such as calipers, then the average value can be considered a more accurate value than the one taken with a ruler.
Vernier Caliper
Animated vernier calipers (Source: Joaquim Alves Gaspar [CC BY 2.5] via Wikimedia Commons ).
Shown is an animated GIF of vernier calipers measuring a nut. The animation starts with the jaws of the yellow calipers opening. Then a grey, hexagonal nut appears between the jaws. The jaws close against the right and left edges of the nut. Then we zoom in to see the measurements on the calipers. There are two different rulers that measure the opening of the jaws - the top one in centimetres and the bottom one in 10ths of a millimetre. The nut measures 2.4 mm on the top ruler, and 0.07 mm on the bottom ruler, for a total of 2.47 cm. These measurements are labelled on the animation with red arrows.
Plastic Ruler - Less Accurate
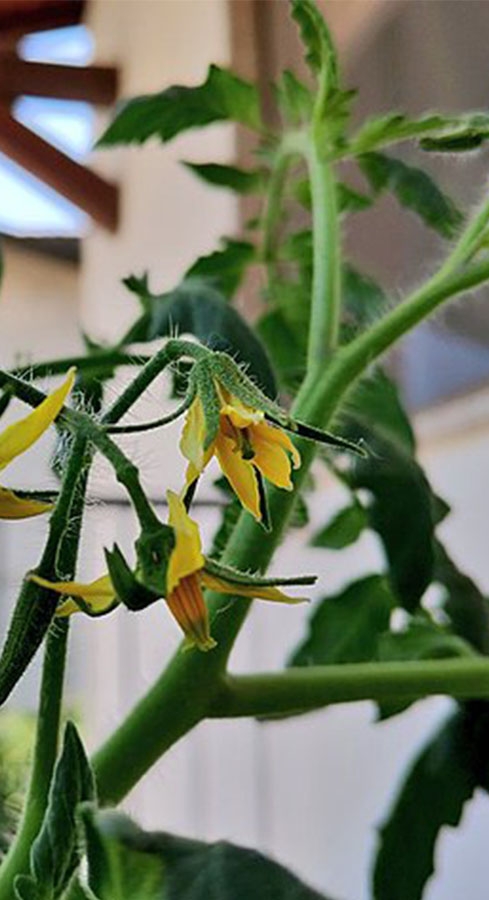
Shown is a colour photograph of a tomato plant with flowers. The green, fuzzy stem of a tomato plant stretches from the bottom left corner of the photograph, almost to the top right corner. The stem is thicker at the bottom than the top. There are smaller branches growing off of it, and these have long, dark green leaves with scalloped edges. In the foreground, another stem with three yellow flowers, is in focus.
Student responses: 3mm, 4mm, 3mm, 4mm
Vernier Calipers - More Accurate
Shown is a colour photograph of a tomato plant with flowers. The green, fuzzy stem of a tomato plant stretches from the bottom left corner of the photograph, almost to the top right corner. The stem is thicker at the bottom than the top. There are smaller branches growing off of it, and these have long, dark green leaves with scalloped edges. In the foreground, another stem with three yellow flowers, is in focus.
Student responses: 3.5mm, 3.3mm, 3.4mm, 3.5mm
Precision refers to how close measured values are to each other. For example, one group of students (Group A) counted the number of tomatoes on their tomato plant, while another group of students (Group B) did the same for the tomatoes on their tomato plant.
How many tomatoes are on the plant?
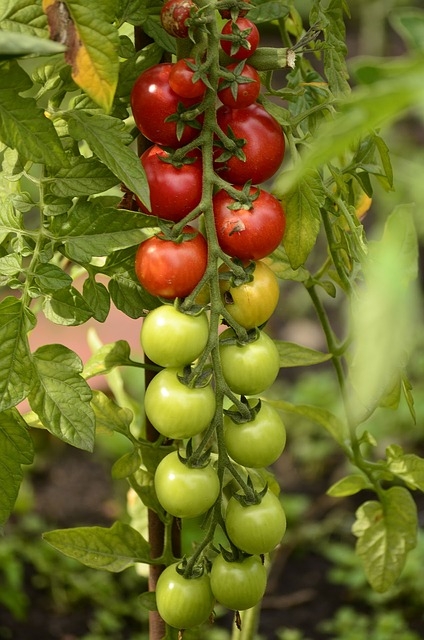
Shown is a colour photograph of a tomato plant with fruit.
The photograph is filled with stems and leaves of tomato plants in various shades of green. In the centre, a stem full of tomato fruit stretches from the top of the image to the bottom. The tomatoes at the top are bright red and the ones at the bottom are pale green.
Student responses: 37, 36, 37, 36, 37, 36
Shown is a colour photograph of a tomato plant with fruit. The photograph is filled with stems and leaves of tomato plants in various shades of green. In the centre, a stem full of tomato fruit stretches from the top of the image to the bottom. The tomatoes at the top are bright red and the ones at the bottom are pale green.
Student responses: 35, 38, 31, 40, 32, 37
Notice that all of the values for Group A are very similar (either 36 or 37). The data would be considered to be very precise. On the other hand, the values for Group B show a much larger range (between 31 and 40). These measurements are not precise.
Precise and Accurate
It is possible to be accurate without being precise and precise without being accurate.
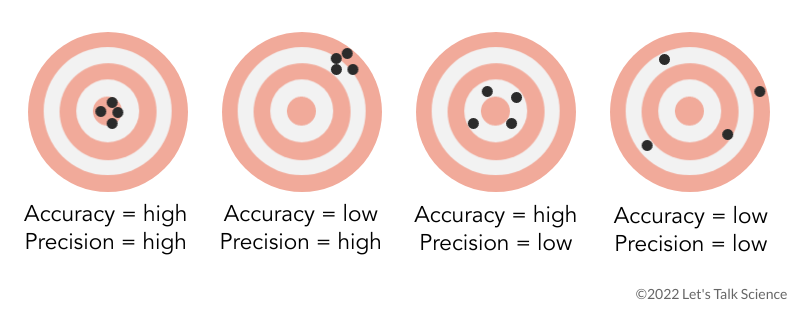
Shown is a colour illustration of four bullseye targets with black dots representing shots aimed at each target. Each bullseye consists of red and white concentric rings, marked with four black dots. The first bullseye, on the left, has all four dots in the smallest ring, in the centre of the target. This is labelled Accuracy = high, Precision = high. The second bullseye has all four dots in a cluster near the top right edge of the target, on the two outer rings. The is labelled Accuracy = low, Precision = high. The third bullseye has four dots spaced around the second ring, just outside the centre of the target. This is labelled Accuracy = high, Precision = low. The last bullseye, on the right, has four dots scattered around the outside of the target, with one in the third ring, two in the fourth ring, and one in the fifth ring. This is labelled Accuracy = low, Precision = low.
Students should always strive to make measurements that are BOTH accurate and precise.
For example, two sets of data were collected about the surface area of tomato plant leaves.
Accurate AND Precise
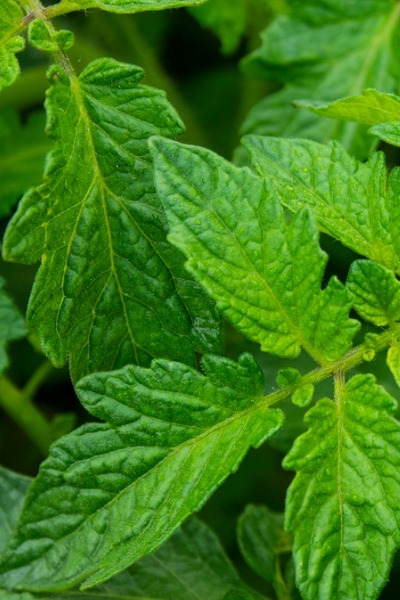
Shown is a colour photograph of a tomato plant, with a focus on three leaves in the foreground. The photograph is filled with bright green leaves. In the foreground, three leaves are in sharp, close focus, so their texture is visible. Each leaf is teardrop-shaped, with scalloped edges and a central stem that makes a deep valley from top to bottom. Many smaller valleys branch off from the central one, and even smaller valleys branch off from those. This creates a leathery appearance.
Student responses: 53 cm 2 , 52 cm 2 , 53 cm 2 , 53 cm 2
Values are close to each other and close to the average
NOT Accurate and NOT Precise
Shown is a colour photograph of a tomato plant, with a focus on three leaves in the foreground. The photograph is filled with bright green leaves. In the foreground, three leaves are in sharp, close focus, so their texture is visible. Each leaf is teardrop-shaped, with scalloped edges and a central stem that makes a deep valley from top to bottom. Many smaller valleys branch off from the central one, and even smaller valleys branch off from those. This creates a leathery appearance.
Student responses: 47 cm 2 , 52 cm 2 , 59 cm 2 , 56 cm 2
Values are not close to each other and some not close to the average
Precision and Accuracy in Tomatosphere™
Accuracy & precision hint.
To remember which is which, think:
Accuracy = Close to Correct
Precision = Repeatable
In the Seed Investigation, students are encouraged to make both precise and accurate measurements of seed germination. This primarily includes recording precise and accurate data for when the seeds germinate (both seed leaves are up and open). For example, if multiple students count the number of seeds they see germinated on a given day, they should each count the same number of seedlings. In further inquiries with the growing tomato plants, students may measure tomato plants’ height, dry mass, surface area of leaves, width of stems, etc.
Guided Practice
Have students read and answer the following questions.
- Look at the measurements from the following two groups of students. Which group has the more accurate measurements for the height of the 50 cm plant? Group A : 49.7 cm, 50.0 cm, 49.8 cm, 50.1 cm, 50.2 cm Group B : 52.1 cm, 52.0 cm, 52.1 cm, 51.9 cm, 52.0 cm
- Look at the measurements from the following two groups of students. Which group has the more precise measurements for the height of the 50 cm plant? Group A : 49.9 cm, 50.0 cm, 49.8 cm, 50.1 cm, 50.2 cm Group B : 52.1 cm, 52.0 cm, 52.1 cm, 51.9 cm, 52.0 cm
- Look at the following measurements of soil pH from one pot. Are they precise? Explain. 7.0, 5.2, 8.1, 6.3, 7.8
Exercise 2
In their own words, have students define the terms “precision” and “accuracy.”
Have students identify a real-life example of a situation when precision is important. Have them also identify a situation when accuracy is important.
Have the students create their own analogy, such as the bullseye analogy used on many websites, to explain the difference between accuracy and precision.
- Calculate the averages for each group. Group A has an average of 49.82 and Group B has an average of 52. Since the average of Group A is closer to 50 cm than the average of Group B, the values for Group A are more accurate.
- The values for Group B are closer to each other (smaller range) than the values for Group A; therefore Group B has more precise measurements, even though they are not as accurate.
- No, they are not. The range is from 5.2 to 8.1, which is a big difference on the pH scale for soil taken from one pot.
What's the difference between accuracy and precision? This video (4:52 min.) by TED-Ed Animations, explains the importance of both accuracy and precision to scientific inquiry, using William Tell and NASA’s Mars missions.
What Is the Difference Between Accuracy and Precision? - Science Notes This page, from Science Notes, demonstrates accuracy and precision using target graphics, and explains when each one is most important.
Accuracy and Precision (2012) This video from Region 10 ESC (2:34 min.) explains how you can have a precise measurement that is not accurate, and an accurate measurement that is not precise. Or worse, a measurement that is both inaccurate and imprecise!
Related Topics
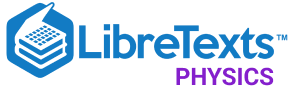
- school Campus Bookshelves
- menu_book Bookshelves
- perm_media Learning Objects
- login Login
- how_to_reg Request Instructor Account
- hub Instructor Commons
Margin Size
- Download Page (PDF)
- Download Full Book (PDF)
- Periodic Table
- Physics Constants
- Scientific Calculator
- Reference & Cite
- Tools expand_more
- Readability
selected template will load here
This action is not available.
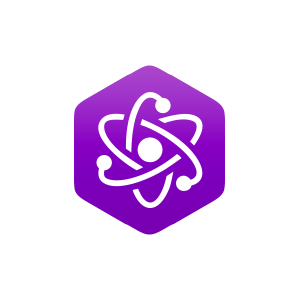
1.3: Accuracy, Precision, and Significant Figures
- Last updated
- Save as PDF
- Page ID 1479
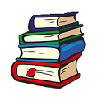
\( \newcommand{\vecs}[1]{\overset { \scriptstyle \rightharpoonup} {\mathbf{#1}} } \)
\( \newcommand{\vecd}[1]{\overset{-\!-\!\rightharpoonup}{\vphantom{a}\smash {#1}}} \)
\( \newcommand{\id}{\mathrm{id}}\) \( \newcommand{\Span}{\mathrm{span}}\)
( \newcommand{\kernel}{\mathrm{null}\,}\) \( \newcommand{\range}{\mathrm{range}\,}\)
\( \newcommand{\RealPart}{\mathrm{Re}}\) \( \newcommand{\ImaginaryPart}{\mathrm{Im}}\)
\( \newcommand{\Argument}{\mathrm{Arg}}\) \( \newcommand{\norm}[1]{\| #1 \|}\)
\( \newcommand{\inner}[2]{\langle #1, #2 \rangle}\)
\( \newcommand{\Span}{\mathrm{span}}\)
\( \newcommand{\id}{\mathrm{id}}\)
\( \newcommand{\kernel}{\mathrm{null}\,}\)
\( \newcommand{\range}{\mathrm{range}\,}\)
\( \newcommand{\RealPart}{\mathrm{Re}}\)
\( \newcommand{\ImaginaryPart}{\mathrm{Im}}\)
\( \newcommand{\Argument}{\mathrm{Arg}}\)
\( \newcommand{\norm}[1]{\| #1 \|}\)
\( \newcommand{\Span}{\mathrm{span}}\) \( \newcommand{\AA}{\unicode[.8,0]{x212B}}\)
\( \newcommand{\vectorA}[1]{\vec{#1}} % arrow\)
\( \newcommand{\vectorAt}[1]{\vec{\text{#1}}} % arrow\)
\( \newcommand{\vectorB}[1]{\overset { \scriptstyle \rightharpoonup} {\mathbf{#1}} } \)
\( \newcommand{\vectorC}[1]{\textbf{#1}} \)
\( \newcommand{\vectorD}[1]{\overrightarrow{#1}} \)
\( \newcommand{\vectorDt}[1]{\overrightarrow{\text{#1}}} \)
\( \newcommand{\vectE}[1]{\overset{-\!-\!\rightharpoonup}{\vphantom{a}\smash{\mathbf {#1}}}} \)
Learning Objectives
By the end of this section, you will be able to:
- Determine the appropriate number of significant figures in both addition and subtraction, as well as multiplication and division calculations.
- Calculate the percent uncertainty of a measurement.
Accuracy and Precision of a Measurement
Science is based on observation and experiment—that is, on measurements. Accuracy is how close a measurement is to the correct value for that measurement. For example, let us say that you are measuring the length of standard computer paper. The packaging in which you purchased the paper states that it is 11.0 inches long. You measure the length of the paper three times and obtain the following measurements: 11.1 in., 11.2 in., and 10.9 in. These measurements are quite accurate because they are very close to the correct value of 11.0 inches. In contrast, if you had obtained a measurement of 12 inches, your measurement would not be very accurate.
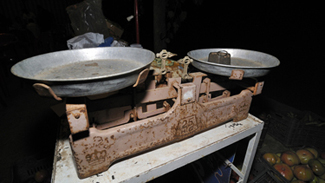
The precision of a measurement system refers to how close the agreement is between repeated measurements (which are repeated under the same conditions). Consider the example of the paper measurements. The precision of the measurements refers to the spread of the measured values. One way to analyze the precision of the measurements would be to determine the range, or difference, between the lowest and the highest measured values. In that case, the lowest value was 10.9 in. and the highest value was 11.2 in. Thus, the measured values deviated from each other by at most 0.3 in. These measurements were relatively precise because they did not vary too much in value. However, if the measured values had been 10.9, 11.1, and 11.9, then the measurements would not be very precise because there would be significant variation from one measurement to another.
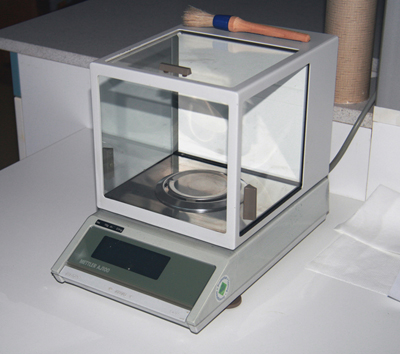
The measurements in the paper example are both accurate and precise, but in some cases, measurements are accurate but not precise, or they are precise but not accurate. Let us consider an example of a GPS system that is attempting to locate the position of a restaurant in a city. Think of the restaurant location as existing at the center of a bull’s-eye target, and think of each GPS attempt to locate the restaurant as a black dot. In Figure \(\PageIndex{3}\), you can see that the GPS measurements are spread out far apart from each other, but they are all relatively close to the actual location of the restaurant at the center of the target. This indicates a low precision, high accuracy measuring system. However, in Figure 4, the GPS measurements are concentrated quite closely to one another, but they are far away from the target location. This indicates a high precision, low accuracy measuring system.
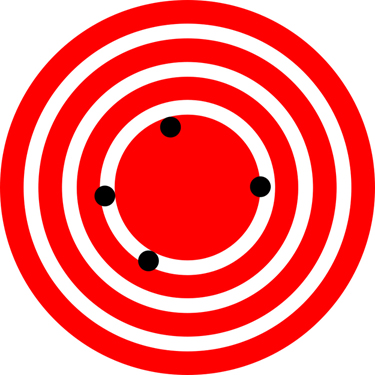
Accuracy, Precision, and Uncertainty
The degree of accuracy and precision of a measuring system are related to the uncertainty in the measurements. Uncertainty is a quantitative measure of how much your measured values deviate from a standard or expected value. If your measurements are not very accurate or precise, then the uncertainty of your values will be very high. In more general terms, uncertainty can be thought of as a disclaimer for your measured values. For example, if someone asked you to provide the mileage on your car, you might say that it is 45,000 miles, plus or minus 500 miles. The plus or minus amount is the uncertainty in your value. That is, you are indicating that the actual mileage of your car might be as low as 44,500 miles or as high as 45,500 miles, or anywhere in between. All measurements contain some amount of uncertainty. In our example of measuring the length of the paper, we might say that the length of the paper is 11 in., plus or minus 0.2 in. The uncertainty in a measurement, A, is often denoted as δA (“delta A”), so the measurement result would be recorded as A ± δA. In our paper example, the length of the paper could be expressed as 11 in.± 0.2.
The factors contributing to uncertainty in a measurement include:
- Limitations of the measuring device,
- The skill of the person making the measurement,
- Irregularities in the object being measured,
- Any other factors that affect the outcome (highly dependent on the situation).
In our example, such factors contributing to the uncertainty could be the following: the smallest division on the ruler is 0.1 in., the person using the ruler has bad eyesight, or one side of the paper is slightly longer than the other. At any rate, the uncertainty in a measurement must be based on a careful consideration of all the factors that might contribute and their possible effects.
MAKING CONNECTIONS: REAL-WORLD CONNECTIONS – FEVERS OR CHILLS?
Uncertainty is a critical piece of information, both in physics and in many other real-world applications. Imagine you are caring for a sick child. You suspect the child has a fever, so you check his or her temperature with a thermometer. What if the uncertainty of the thermometer were 3.0ºC? If the child’s temperature reading was 37.0ºC (which is normal body temperature), the “true” temperature could be anywhere from a hypothermic 34.0ºC to a dangerously high 40.0ºC. A thermometer with an uncertainty of 3.0ºC would be useless.
Percent Uncertainty
One method of expressing uncertainty is as a percent of the measured value. If a measurement A is expressed with uncertainty, \(δA\), the percent uncertainty (%uncertainty) is defined to be
\[\% \,\text{unc} =\dfrac {δA}{A} \times 100\%\]
Example \(\PageIndex{1}\): Calculating Percent Uncertainty: A Bag of Apples
A grocery store sells 5-lb bags of apples. You purchase four bags over the course of a month and weigh the apples each time. You obtain the following measurements:
Week 1 weight: 4.8 lb Week 2 weight: 5.3 lb Week 3 weight: 4.9 lb Week 4 weight: 5.4 lb
You determine that the weight of the 5-lb bag has an uncertainty of ±0.4lb. What is the percent uncertainty of the bag’s weight?
First, observe that the expected value of the bag’s weight, \(A\), is 5 lb. The uncertainty in this value, \(δA\), is 0.4 lb. We can use the following equation to determine the percent uncertainty of the weight:
\(\text{% unc} =\frac{δA}{A}×100%\).
Plug the known values into the equation:
\(\text{% unc} =\frac{0.4 lb}{5 lb}×100%=8%\).
We can conclude that the weight of the apple bag is \(5lb±8%\). Consider how this percent uncertainty would change if the bag of apples were half as heavy, but the uncertainty in the weight remained the same. Hint for future calculations: when calculating percent uncertainty, always remember that you must multiply the fraction by 100%. If you do not do this, you will have a decimal quantity, not a percent value.
Uncertainties in Calculations
There is an uncertainty in anything calculated from measured quantities. For example, the area of a floor calculated from measurements of its length and width has an uncertainty because the length and width have uncertainties. How big is the uncertainty in something you calculate by multiplication or division? If the measurements going into the calculation have small uncertainties (a few percent or less), then the method of adding percents can be used for multiplication or division. This method says that the percent uncertainty in a quantity calculated by multiplication or division is the sum of the percent uncertainties in the items used to make the calculation . For example, if a floor has a length of 4.00m and a width of 3.00m, with uncertainties of 2% and 1%, respectively, then the area of the floor is 12.0m2 and has an uncertainty of 3%. (Expressed as an area this is 0.36m2, which we round to \(0.4\,m^2\) since the area of the floor is given to a tenth of a square meter.)
Exercise \(\PageIndex{1}\)
A high school track coach has just purchased a new stopwatch. The stopwatch manual states that the stopwatch has an uncertainty of ±0.05s. Runners on the track coach’s team regularly clock 100-m sprints of 11.49 s to 15.01 s. At the school’s last track meet, the first-place sprinter came in at 12.04 s and the second-place sprinter came in at 12.07 s. Will the coach’s new stopwatch be helpful in timing the sprint team? Why or why not?
No, the uncertainty in the stopwatch is too great to effectively differentiate between the sprint times.
Precision of Measuring Tools and Significant Figures
An important factor in the accuracy and precision of measurements involves the precision of the measuring tool. In general, a precise measuring tool is one that can measure values in very small increments. For example, a standard ruler can measure length to the nearest millimeter, while a caliper can measure length to the nearest 0.01 millimeter. The caliper is a more precise measuring tool because it can measure extremely small differences in length. The more precise the measuring tool, the more precise and accurate the measurements can be.
When we express measured values, we can only list as many digits as we initially measured with our measuring tool. For example, if you use a standard ruler to measure the length of a stick, you may measure it to be 36.7cm. You could not express this value as 36.71cm because your measuring tool was not precise enough to measure a hundredth of a centimeter. It should be noted that the last digit in a measured value has been estimated in some way by the person performing the measurement. For example, the person measuring the length of a stick with a ruler notices that the stick length seems to be somewhere in between 36.6cm and 36.7cm, and he or she must estimate the value of the last digit. Using the method of significant figures, the rule is that the last digit written down in a measurement is the first digit with some uncertainty. In order to determine the number of significant digits in a value, start with the first measured value at the left and count the number of digits through the last digit written on the right. For example, the measured value 36.7cm has three digits, or significant figures. Significant figures indicate the precision of a measuring tool that was used to measure a value.
Special consideration is given to zeros when counting significant figures. The zeros in 0.053 are not significant, because they are only placekeepers that locate the decimal point. There are two significant figures in 0.053. The zeros in 10.053 are not placekeepers but are significant—this number has five significant figures. The zeros in 1300 may or may not be significant depending on the style of writing numbers. They could mean the number is known to the last digit, or they could be placekeepers. So 1300 could have two, three, or four significant figures. (To avoid this ambiguity, write 1300 in scientific notation.) Zeros are significant except when they serve only as placekeepers .
Exercise \(\PageIndex{2}\)
Determine the number of significant figures in the following measurements:
- × 10 3
- 1; the zeros in this number are placekeepers that indicate the decimal point
- 6; here, the zeros indicate that a measurement was made to the 0.1 decimal point, so the zeros are significant
- 1; the value 3 signifies the decimal place, not the number of measured values
- 5; the final zero indicates that a measurement was made to the 0.001 decimal point, so it is significant
- 4; any zeros located in between significant figures in a number are also significant
Significant Figures in Calculations
When combining measurements with different degrees of accuracy and precision, the number of significant digits in the final answer can be no greater than the number of significant digits in the least precise measured value . There are two different rules, one for multiplication and division and the other for addition and subtraction, as discussed below.
1. For multiplication and division: The result should have the same number of significant figures as the quantity having the least significant figures entering into the calculation. For example, the area of a circle can be calculated from its radius using A=πr2. Let us see how many significant figures the area has if the radius has only two—say, r=1.2m. Then,
\[A=πr2=(3.1415927...)×(1.2m)^2=4.5238934\,m^2\]
is what you would get using a calculator that has an eight-digit output. But because the radius has only two significant figures, it limits the calculated quantity to two significant figures or
\[A=4.5\,m^2,\]
even though \( is good to at least eight digits.
2. For addition and subtraction: The answer can contain no more decimal places than the least precise measurement. Suppose that you buy 7.56-kg of potatoes in a grocery store as measured with a scale with precision 0.01 kg. Then you drop off 6.052-kg of potatoes at your laboratory as measured by a scale with precision 0.001 kg. Finally, you go home and add 13.7 kg of potatoes as measured by a bathroom scale with precision 0.1 kg. How many kilograms of potatoes do you now have, and how many significant figures are appropriate in the answer? The mass is found by simple addition and subtraction:
kg − 6.052\, kg + 13.7\, kg \, 15.208\, kg = 15.2\, kg .\]
Next, we identify the least precise measurement: 13.7 kg. This measurement is expressed to the 0.1 decimal place, so our final answer must also be expressed to the 0.1 decimal place. Thus, the answer is rounded to the tenths place, giving us 15.2 kg.
Significant Figures in this Text
In this text, most numbers are assumed to have three significant figures. Furthermore, consistent numbers of significant figures are used in all worked examples. You will note that an answer given to three digits is based on input good to at least three digits, for example. If the input has fewer significant figures, the answer will also have fewer significant figures. Care is also taken that the number of significant figures is reasonable for the situation posed. In some topics, particularly in optics, more accurate numbers are needed and more than three significant figures will be used. Finally, if a number is exact , such as the two in the formula for the circumference of a circle, \( = 2 π r ,\) it does not affect the number of significant figures in a calculation.
Exercise \(\PageIndex{3}\)
Perform the following calculations and express your answer using the correct number of significant digits.
- A woman has two bags weighing 13.5 pounds and one bag with a weight of 10.2 pounds. What is the total weight of the bags?
- The force \(F\) on an object is equal to its mass m multiplied by its acceleration \(a\). If a wagon with mass 55 kg accelerates at a rate of \(0.0255 m/s^2\), what is the force on the wagon? (The unit of force is called the newton, and it is expressed with the symbol N.)
(a) 37.2 pounds; Because the number of bags is an exact value, it is not considered in the significant figures.
(b) 1.4 N; Because the value 55 kg has only two significant figures, the final value must also contain two significant figures.
PHET EXPLORATIONS: ESTIMATION
Explore size estimation in one, two, and three dimensions! Multiple levels of difficulty allow for progressive skill improvement.

- Accuracy of a measured value refers to how close a measurement is to the correct value. The uncertainty in a measurement is an estimate of the amount by which the measurement result may differ from this value.
- Precision of measured values refers to how close the agreement is between repeated measurements.
- The precision of a measuring tool is related to the size of its measurement increments. The smaller the measurement increment, the more precise the tool.
- Significant figures express the precision of a measuring tool.
- When multiplying or dividing measured values, the final answer can contain only as many significant figures as the least precise value.
- When adding or subtracting measured values, the final answer cannot contain more decimal places than the least precise value.
Contributors and Attributions
Paul Peter Urone (Professor Emeritus at California State University, Sacramento) and Roger Hinrichs (State University of New York, College at Oswego) with Contributing Authors: Kim Dirks (University of Auckland) and Manjula Sharma (University of Sydney). This work is licensed by OpenStax University Physics under a Creative Commons Attribution License (by 4.0) .
Accuracy vs. Precision
Accuracy and precision are used in context of measurement. Accuracy refers to the degree of conformity and correctness of something when compared to a true or absolute value, while precision refers to a state of strict exactness — how consistently something is strictly exact.
In other words, the precision of an experiment, object, or value is a measure of the reliability and consistency. The accuracy of an experiment, object, or value is a measurement of how closely results agree with the true or accepted value.
Both accuracy and precision are terms used in the fields of science , engineering, and statistics.
Comparison chart
Defining accuracy and precision.
In his review of Simon Winchester's The Perfectionists: How Precision Engineers Created the Modern World , James Gleick writes for the New York Review of Books
... precision involves an ideal of meticulousness and consistency, while accuracy implies real-world truth. When a sharpshooter fires at a target, if the bullets strike close together—clustered, rather than spread out—that is precise shooting. But the shots are only accurate if they hit the bull’s eye. A clock is precise when it marks the seconds exactly and unvaryingly but may still be inaccurate if it shows the wrong time.
A measurement may be accurate but not precise, precise but not accurate, or neither, or both.
An example of bad precision with good accuracy might be a lab refrigerator that holds a constant temperature of 38.0F. A temperature sensor is tested 10 times in the refrigerator. The temperatures from the test yield the temperatures of: 37.8, 38.3, 38.1, 38.0, 37.6, 38.2, 38.0, 38.0, 37.4, 38.3. This distribution shows no impressive tendency toward a particular value (lack of precision) but each value does come close to the actual temperature (high accuracy).
Another example is the Antikythera mechanism , an ancient Greek analog computer that was used to predict astronomical positions and eclipses. It had 37 gear wheels and could follow the movements of the moon, including the irregular orbit of the moon where the moon’s velocity is higher in its perigee than in its apogee. The device was reasonably precise but not very accurate .
Target Comparison
Accuracy is the degree of correctness, while precision is how strict that correctness is (or isn't) — how reproducible results are. For this example, consider results from a round of target practice.
Arrows are fired at a target, and measurements are taken in relation to the bull's eye at the center of the target. Accuracy describes how close the arrows are to the bull's-eye. The closer an arrow is to the bull's eye, the more accurate the shot.
How precise the shots are depends on how often the arrows land near each other on the target. When all or most arrows are grouped tightly together, the shots fired can be considered precise since they all landed near the same spot, if not necessarily near the bull's-eye. This is how results can indicate precision but not necessarily accuracy. However, it is important to note that it is not possible to reliably achieve accuracy without precision.

Number of Measurements
Accuracy can be improved by taking repeat measurements and taking an average. (This assumes that errors are randomly above and below the true value to the same degree). Therefore an experiment with a low degree of precision can provide accurate values where appropriate statics are applied.
Conversely, precision cannot be improved by taking repeated measurements but it is impossible to quantify precision without experimental repeats.
The danger when evaluating an experiment is that some errors are not random. In this case, an experiment could yield inaccurate results yet be highly precise.
While a precise measurement may speak highly of an instrument's quality, an accurate reading will not reflect on the quality. Accuracy is an agreement of a measured value with an expected value. For example, a stopped clock will be accurate twice in day, but it will not be precise — i.e., able to consistently and accurately keep time throughout the day. In the case of a clock, how precisely it measures time matters a great deal and determines quality .
- Wikipedia: Accuracy
Related Comparisons

Share this comparison via:
If you read this far, you should follow us:
"Accuracy vs Precision." Diffen.com. Diffen LLC, n.d. Web. 12 May 2024. < >
Comments: Accuracy vs Precision
- Hypothesis vs Theory
- Standard Deviation vs Variance
- Mean vs Median
- Data vs Information
- Principal vs Principle
- Accountability vs Responsibility
- Ensure vs Insure
- Then vs Than
Edit or create new comparisons in your area of expertise.
Stay connected
© All rights reserved.
Exploring Our Fluid Earth
Teaching science as inquiry.
- Create new account
- Reset your password
For more option use Advanced Search
Practices of Science: Precision vs. Accuracy
- If the darts are neither close to the bulls-eye, nor close to each other, there is neither accuracy, nor precision (SF Fig. 1.5 A).
- If all of the darts land very close together, but far from the bulls-eye, there is precision, but not accuracy (SF Fig. 1.5 B).
- If the darts are all about an equal distance from and spaced equally around the bulls-eye there is mathematical accuracy because the average of the darts is in the bulls-eye. This represents data that is accurate, but not precise (SF Fig. 1.5 C). However, if you were actually playing darts this would not count as a bulls-eye!
- If the darts land close to the bulls-eye and close together, there is both accuracy and precision (SF Fig. 1.5 D).
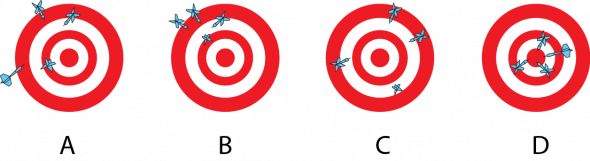
SF Fig. 1.5. Dartboards showing different accuracy and precision scenarios.
Image by Byron Inouye
- The oceanographer checks the weather forecast the night before her trip so she knows what to wear on the boat. The TV forecaster says it will be between 26 and 31 degrees (°) Celsius (C) at noon the next day. The actual temperature reading the next day on the boat at noon is 28° C.
- When the oceanographer’s Global Positioning System (GPS) indicates that she is at the location of the underwater buoy, she anchors the boat and jumps in the water to collect the data logger. However, she can’t see the buoy. The other GPS units belonging to her colleagues on the boat also indicate that they are at the correct location. After an extensive search, the oceanographer finds the buoy 50 meters (m) from the boat.
- While on the way back to shore, the oceanographer throws in a fishing line to see if she can catch anything for dinner. She is lucky enough to catch a mahi-mahi. When she pulls it out of the water, her colleagues estimate the weight of the fish. Their estimates are 16.1 kilograms (kg), 16.8 kg, and 15.9 kg. When they weigh the fish upon returning to shore, the actual weight is 18.2 kg.
- Write your own scenario illustrating the difference between accuracy and precision. Swap your scenario with a classmate. Identify your classmate’s scenario measurements as accurate or inaccurate and precise or imprecise.
- How is this model different from scientists who are measuring a natural phenomenon?
- Is there a way for scientists to determine how accurate their measurements are? Explain your answer

Authors & Partners
Partner Organizations

Exploring Our Fluid Earth, a product of the Curriculum Research & Development Group (CRDG), College of Education. © University of Hawai‘i, . This document may be freely reproduced and distributed for non-profit educational purposes.

Recent Posts

12/12/2023 Generic Medications: The Effective and Safe Alternative

12/12/2023 Clinical Studies in children's: A necessity in Ecuador

13/11/2023 Mind over Matter: Exploring the Link between Stress and Immunity

17/10/2023 Post-Traumatic Stress Disorder (PTSD): Let’s learn about the Trauma and its Recovery!

07/09/2023 Navigating the Seas of Clinical Research: Accuracy vs. Precision vs. Reliability
- December 2023 (2)
- November 2023 (1)
- October 2023 (1)
- September 2023 (1)
- August 2023 (1)
- July 2023 (1)
- June 2023 (3)
- May 2023 (2)
- March 2023 (1)
- February 2023 (2)
- January 2023 (1)
- July 2022 (1)
- November 2021 (1)
5 Reasons why Precision is Important in Research
Precision and accuracy are two ways that scientists think about error. Accuracy refers to how close a measurement is to the true or accepted value. Precision refers to how close measurements of the same item are to each other. Precision is independent of accuracy . That means it is possible to be very precise but not very accurate, and it is also possible to be accurate without being precise. The best-quality scientific observations are both accurate and precise.
In the field of clinical research , precision is not just a buzzword; it is an essential element that drives the accuracy and reliability of study outcomes. Precision encompasses various aspects, from study design and data collection to analysis and interpretation. In this blog post, we will explore why precision holds immense significance in clinical research and how it impacts the credibility and applicability of research findings.

Enhancing Validity and Reliability:
Precision plays a pivotal role in ensuring the validity and reliability of clinical research . Rigorous study designs, well-defined protocols, and standardized data collection methods are key components of precision. By adhering to these principles, researchers can minimize bias, confounding factors, and errors that may compromise the integrity of their findings. Precise methodologies increase the chances of obtaining consistent and accurate results, enhancing the overall trustworthiness of the study.
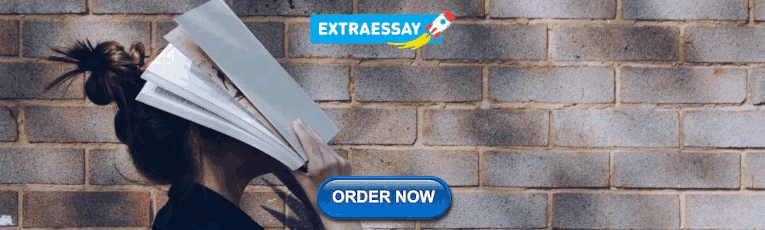
Enabling Accurate Treatment Assessments:
Precision in clinical research is crucial for evaluating the effectiveness and safety of treatments. Precisely designed studies allow researchers to measure treatment outcomes with accuracy, enabling them to determine whether a particular intervention truly makes a difference. Precision in outcome measures, statistical analyses, and data interpretation helps identify the magnitude and statistical significance of treatment effects, enabling informed decision-making regarding patient care and public health interventions.
Facilitating Personalized Medicine:
Precision medicine aims to deliver tailored healthcare interventions based on individual characteristics, such as genetics, lifestyle, and environmental factors. Clinical research with a high degree of precision is vital for identifying biomarkers, genetic variants, and other factors that influence disease progression and treatment response. By uncovering these nuances, researchers can develop personalized approaches that maximize treatment efficacy and minimize adverse effects, ultimately improving patient outcomes.

Supporting Regulatory Approval and Clinical Guidelines:
Precision in clinical research is closely tied to regulatory approval and the development of clinical guidelines. Regulatory agencies rely on robust, well-designed studies with precise outcomes to evaluate the safety and effectiveness of new interventions. Precise research findings are critical for establishing evidence-based guidelines that inform clinical practice, helping healthcare professionals make informed decisions in patient care.
Enhancing Reproducibility and External Validity:
Precise research protocols and transparent reporting facilitate reproducibility, allowing other researchers to validate study findings and build upon them. External validity, or the generalizability of research findings to broader populations, is also influenced by precision. By ensuring precise methodologies and diverse participant representation, researchers increase the likelihood that their results can be extrapolated to wider populations, improving the relevance and impact of their work.

Accuracy Research has a multidisciplinary team of highly qualified and trained personnel committed to carrying out fully compliant clinical research.
- Our Services
- Respiratory
- Rheumatology
- Tropical Diseases
- Osteoporosis
- Osteoarthritis
- Ophthalmology
- Gastroenterology
- Central Nervous System
- Cardiovascular
© 2024 ACCURACY RESEARCH. HEALTH CONSULTING & CONTRACT RESEARCH ORGANIZATION. Investigación en Salud. Cía. Ltda

- Key Differences
Know the Differences & Comparisons
Difference Between Accuracy and Precision
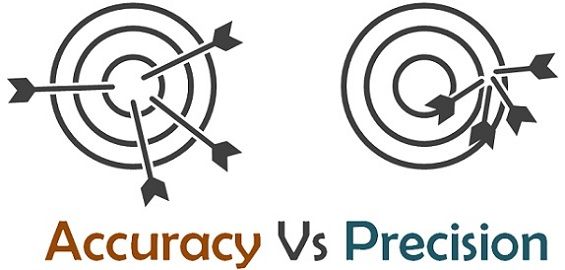
Accuracy symbolizes the extent of conformity, whereas Precision indicates the extent of reproducibility.
At the time of taking measurements, these two are always taken into account, due to their utmost importance in the various field, such as science, statistics, research and engineering. So, let’s take a glance at the difference between accuracy and precision.
Content: Accuracy Vs Precision
Comparison chart, definition of accuracy.
By the term ‘accuracy’, we mean the degree of compliance with the standard measurement, i.e. to which extent the actual measurement is close to the standard one, i.e. bulls-eye. It measures the correctness and closeness of the result at the same time by comparing it to the absolute value.
Therefore, the closer the measurement, the higher is the level of accuracy. It mainly depends on the way; data is collected.
Definition of Precision
Precision represents the uniformity or repeatability in the measurements. It is the degree of excellence, in the performance of an operation or the techniques used to obtain the results. It measures the extent to which the results are close to each other, i.e. when the measurements are clustered together.
Therefore, the higher the level of precision the less is the variation between measurements. For instance: Precision is when the same spot is hit, again and again, which is not necessarily the correct spot.
Key Differences Between Accuracy and Precision
The difference between accuracy and precision can be drawn clearly on the following grounds:
- The level of agreement between the actual measurement and the absolute measurement is called accuracy. The level of variation that lies in the values of several measurements of the same factor is called as precision
- Accuracy represents the nearness of the measurement with the actual measurement. On the other hand, precision shows the nearness of an individual measurement with those of the others.
- Accuracy is the degree if conformity, i.e. the extent to which measurement is correct when compared to the absolute value. On the other hand, precision is the degree of reproducibility, which explains the consistency of the measurements.
- Accuracy is based on a single factor, whereas precision is based on more than one factor.
- Accuracy is a measure of statistical bias while precision is the measure of statistical variability.
- Accuracy focuses on systematic errors, i.e. the errors caused by the problem in the instrument. As against this, precision is concerned with random error, which occurs periodically with no recognisable pattern.
So, if the actual measurement is high in accuracy and precision, the result would be free from errors. If the actual measurement is precise but inaccurate, then the result is in disagreement with the expected one. If the actual result is accurate but imprecise, then there are huge variations in the measurements. And finally, if the actual measurement is neither accurate nor precise, then the result would lack correctness and exactness at the same time.
You Might Also Like:
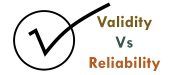
Ayesha says
October 30, 2018 at 10:11 am
Excellent explanation, I would never understand the difference in such a clear and easy way. Today my concepts are crystal clear about this difference. Thanks
December 9, 2019 at 7:52 am
If you were my physical sciences educator back in high school, I wouldn’t be here now
Leave a Reply Cancel reply
Your email address will not be published. Required fields are marked *
Save my name, email, and website in this browser for the next time I comment.

An official website of the United States government
The .gov means it’s official. Federal government websites often end in .gov or .mil. Before sharing sensitive information, make sure you’re on a federal government site.
The site is secure. The https:// ensures that you are connecting to the official website and that any information you provide is encrypted and transmitted securely.
- Publications
- Account settings
Preview improvements coming to the PMC website in October 2024. Learn More or Try it out now .
- Advanced Search
- Journal List
- HHS Author Manuscripts

Accuracy, Precision, and Reliability of Chemical Measurements in Natural Products Research
Joseph m. betz.
a Office of Dietary Supplements, U.S. National Institutes of Health, Bethesda, MD 20892, USA
Paula N. Brown
b Centre for Applied Research & Innovation, British Columbia Institute of Technology, Burnaby, BC, V5G 3H2, Canada
Mark C. Roman
c Tampa Bay Analytical Research, Inc., Largo, FL 33777
Associated Data
Natural products chemistry is the discipline that lies at the heart of modern pharmacognosy. The field encompasses qualitative and quantitative analytical tools that range from spectroscopy and spectrometry to chromatography. Among other things, modern research on crude botanicals is engaged in the discovery of the phytochemical constituents necessary for therapeutic efficacy, including the synergistic effects of components of complex mixtures in the botanical matrix. In the phytomedicine field, these botanicals and their contained mixtures are considered the active pharmaceutical ingredient (API), and pharmacognosists are increasingly called upon to supplement their molecular discovery work by assisting in the development and utilization of analytical tools for assessing the quality and safety of these products. Unlike single-chemical entity APIs, botanical raw materials and their derived products are highly variable because their chemistry and morphology depend on the genotypic and phenotypic variation, geographical origin and weather exposure, harvesting practices, and processing conditions of the source material. Unless controlled, this inherent variability in the raw material stream can result in inconsistent finished products that are under-potent, over-potent, and/or contaminated. Over the decades, natural products chemists have routinely developed quantitative analytical methods for phytochemicals of interest. Quantitative methods for the determination of product quality bear the weight of regulatory scrutiny. These methods must be accurate, precise, and reproducible. Accordingly, this review discusses the principles of accuracy (relationship between experimental and true value), precision (distribution of data values), and reliability in the quantitation of phytochemicals in natural products.
1. INTRODUCTION
The word “pharmacognosy” was coined in the early 19th century to designate the discipline related to the study of medicinal plants [ 1 ]. The science of pharmacognosy became aligned with botany and plant chemistry, and until the early 20 th century, dealt mostly with physical description and identification of whole and powdered plant drugs including their history, commerce, collection, preparation, and storage. Advances in organic chemistry added a new dimension to the description and quality control of these drugs, and the discipline has since expanded to include discovery of novel chemical therapeutic agents from the natural world.
While discovery of new chemical entities has become the modern focus of much natural products work, identification and quality control remain important for pharmacopoeial identification and quality control of goods traded as crude botanicals or extracts [ 2 ]. Books and courses on analytical chemistry often do not fully describe the overall process of analytical method design, development, optimization, and validation [ 3 ]. As a result, the chemical literature is rich in procedures that have been developed with variable rigor and conclusions that imply, rather than prove, correctness and validity of reported results. Peer-review of publications that report quantitative results but are not primarily analytical papers may not address method validity and the methods may not be useful for actual samples. The role of reliable measurements in regulatory settings has obvious public health implications; tight control over active ingredients, nutrients and other constituents of foods and supplements (including deleterious substances such as pesticides and toxic elements) are necessary for safety and efficacy.
While this review cannot capture the breadth of all existing rules surrounding measurements made on commercial goods, two excerpts from U.S. Good Manufacturing Practice (GMP) regulations for drugs and dietary supplements shall highlight the importance that the U.S. government places on the integrity of data. For drugs, 21 CFR Part 211.194 (a)(2) requires a “statement of each method used. … statement shall indicate the location of data that establish that the methods used in the testing… meet proper standards of accuracy and reliability…” [ 4 ]. For dietary supplements, 21 CFR Part 111.75 requires manufacturers to “ensure that the tests and examinations that you use to determine whether the specifications are met are appropriate, scientifically valid methods”, and notes that “a scientifically valid method is one that is accurate, precise, and specific for its intended purpose” [ 5 ]. The International Conference on Harmonisation of Technical Requirements for Registration of Pharmaceuticals for Human Use (ICH) [ 6 ] defines fitness for purpose as the “degree to which data produced by a measurement process enables a user to make technically and administratively correct decisions for a stated purpose.” This relates to scope and applicability. In order for a method to be of use, it needs to be tailored to specific analytes, matrices and expected concentration ranges.
However, method development and validation can be challenging when dealing with poorly defined analytes, such as antioxidants, flavonoids and phenolics, as well as the complex matrices of botanical raw materials and finished products. Defining analytes and matrices in the fitness for purpose statement is important for developing a successful method.
2. PARAMETERS OF VALIDATION
Various organizations are involved with analytical method validation: (a) the International Union of Pure and Applied Chemistry (IUPAC) publishes chemical data and standard methods for analytical, clinical, quality control and research laboratories, while ICH has developed validation guidelines [ 6 ]; (b) FDA s “Guidance for Industry: Analytical Procedures and Methods Validation” provides recommendations on submitting analytical procedures, validation data and samples to support the documentation of the identity, strength, quality, purity and potency of drug substances and drug products [ 7 ]. A more specific guidance document focuses on the “what” and “how” of chromatographic method validation [ 8 ]; (c) AOAC International (AOACI) produces rigorous, well recognized validation guidelines that range from single laboratory validation (SLV) guidelines [ 9 ] complete with acceptance criteria [ 10 ] and sample protocol [ 11 ] to guidelines for the conduct of interlaboratory collaborative studies [ 12 ].
While there are numerous approaches to quantitative chemical analysis of natural products, space is limited and this review will focus on validation of chromatographic methods since they are the most widely used for determination of phytochemicals in raw materials and finished products. Analytical methods are not universal; characteristics, techniques, scope and applicability can differ substantially. Thus, it is impossible to have a single set of instructions that can be used to validate all methods. However they do share basic commonalities that can be addressed to ensure confidence in their use and the measurements obtained. Beyond the health implications of inaccurate measurements made on commercial products, practitioners should be aware that inaccurate quantitative measurements can cause significant bias when they are published.
2.1 ACCURACY AND PRECISION
A good starting point for basic definitions and descriptions of the key terms and concepts pertaining to the assurance of the quality of quantitative chemical measurements is the U.S. Food and Drug Administration s (FDA) Reviewer Guidance [ 8 ]. The two most important elements of a chromatographic test method are accuracy and precision. Accuracy is a measure of the closeness of the experimental value to the actual amount of the substance in the matrix. Precision measures of how close individual measurements are to each other.
2.1.1 Accuracy and Recovery
The purpose of analysis of botanicals and other natural products is quantitation of target compounds in the matrix in which the compounds occur. The most common technique for determining accuracy in natural product studies is the spike recovery method, in which the amount of a target compound is determined as a percentage of the theoretical amount present in the matrix. In a spike recovery experiment, a measured amount of the constituent of interest is added to a matrix (spiked) and then the analysis is performed on the spiked material, from the sample preparation through chromatographic determination. A comparison of the amount found versus the amount added provides the recovery of the method, which is an estimate of the accuracy of the method. In an ideal situation, such as the determination of a synthetic pesticide in food, the matrix will be devoid of the target analyte(s). However, this is seldom the case in phytochemical studies where the target analyte occurs naturally in the matrix. Therefore, analysts will frequently perform parallel analyses of spiked and un-spiked materials. The theoretical recovery of the target analyte from the spiked material is the sum of the amount of added analyte plus the amount of naturally occurring analyte (as determined in the parallel analysis of unspiked material). The difference between the theoretical amount and the amount analytically determined in the spiked matrix provides an estimate of accuracy. Other approaches to spike recovery studies include adding the target analyte to a similar matrix that does not contain the target and spiking the target analyte into natural matrix from which the target has been exhaustively extracted and then dried. Recovery is frequently concentration dependent; the FDA guidance for drugs [ 8 ] suggests that matrices be spiked at 80, 100, and 120% of the expected value, and that the experiment be performed in triplicate. For botanical materials and dietary supplements; where the analyte may be present over a large concentration range, recovery should be determined over the entire analytical range of interest for the method.
While analyte addition has both pros and cons, it is one commonly practiced in the natural products community. Other techniques such as exhaustive extraction can be used to help verify the accuracy of the method. In some cases a certified reference material may be available that contains the substance(s) of interest. These materials contain a known amount of the analyte with a given uncertainty and can be used in lieu of and/or in addition to analyte spiking. If available, certified reference materials can be obtained from national metrological laboratories such as the U.S. National Institute for Standards and Technology (NIST), the Environmental Protection Agency (EPA), or commercial suppliers.
Various factors affect the accuracy of an analytical method. These range from extraction efficiency to stability of the analyte to adequacy of the chromatographic separation and can generally be optimized during the method development and optimization phase of a study.
Important but frequently overlooked factors that affect accuracy are assumptions made in setting up and performing the assays. The first assumption involves the purity of the reference materials used to establish the identity of the analyte, create the calibration curve, and arrive at a quantitative analytical result. Available in milligram to gram quantities, these materials are usually accompanied by a label declaration of purity and/or a certificate of analysis that includes a purity declaration. Depending on their stability and the technique(s) used to determine their purity, the actual purity of these materials may differ from the claimed value, and investigators should take steps to assure identity and purity before using them.
The second assumption also involves calibration standards. There are many compounds that are not commercially available or that are prohibitively expensive. As a result, some analyses are designed to use a single compound that is nominally similar to all of the analytical targets, and quantitative results for the other compounds are expressed in terms of the one compound at hand (normalization). In UV detection, this may be appropriate if the specific extinction coefficients of the target compounds are similar; the less similar they are, the more inaccurate are the results.
2.1.2 Accuracy Case Study
An HPLC investigation [ 13 ] of cranberry ( Vaccinium macrocarpon Aiton) was performed using two different means of constructing the calibration curve for the major cranberry anthocyanins. The first set of experiments was modeled after previous approaches [ 14 ] and compared results of the quantitation of individual anthocyanins in cranberry fruit using cyanidin-3-glucoside as calibrant for all compounds. The underlying assumption was that detector response at a wavelentgth of 520 nm would be the same for all of the anthocyanins. In the second experiment, the major anthocyanins were obtained and used to construct individual calibration curves for each. When individual calibration curves were used, the amounts of individual compounds were found to be different from those reported using normalization ( Figure 1 , Table 1 ).

Graphical comparison of anthocyanin content in cranberry fruit when determined by normalization using cyanidin 3-O-glucoside as the external calibrant as compared to quantitation using calibration curves generated for each individual anthocyanin [ 7 ]. C3Ga=Cyanidin-3- O -galactoside, C3Gl=Cyanidin-3- O -glucoside, C3Ar= Cyanidin-3- O -Arabinoside, P3Ga=Peonidin-3- O -galactoside, P3Ar=Peonidin-3- O -galactoside.
Anthocyanin content of cranberries determined by HPLC using normalization to cyanidin 3-O-glucoside [ 13 , 14 ] vs. anthocyanin content determined using individual anthocyanins as calibrants [ 13 ]
Purity of reference materials can also affect accuracy. An illustration of the importance of verifying the purity of chemicals used as calibrants is provided in Table 2 . In the HPLC investigation of cranberry anthocyanins described above [ 13 ], calibration standards for the five major cranberry anthocyanins were purchased from a commercial supplier. In preparation for the analysis, the investigator determined the purity of the purchased standards using a standard approach [ 15 ]. While the manufacturer’s certificates of analysis declared that all five compounds were > 97% pure (as determined by HPLC), the investigators found that their actual purity ranged from 66–97%. Calculation of individual anthocyanin content of cranberry using the declared purity of the calibration standards would have resulted in inaccurate results for several of the compounds. In addition, actual purities were different for different lots of the same material.
Claimed and actual purity of commercial cranberry anthocyanins [ 13 ]
2.1.3 Precision
The FDA guidance document on validation of chromatographic methods [ 8 ] breaks the overall concept of precision into three components: repeatability , intermediate precision , and reproducibility. Repeatability is a measure of the within-laboratory uncertainty. It takes into account the reproducibility of injections and other aspects of the analysis such as weighing, fluid dispensing and handling, serial dilution, and adequacy of extraction. Among other factors, calibration of balances and glassware can increase repeatability. The guidance recommends that a validation package include data from a minimum of 10 injections that show a relative standard deviation of less than one percent. Intermediate precision is a measure of the ruggedness of the method, i.e., reliability when performed in different environments. Demonstration of intermediate precision requires that the method be run on multiple days by different analysts and on different instruments. At a minimum, such studies should be run on at least two separate occasions. Reproducibility is an indication of the precision that can be achieved between different laboratories and is evaluated using multi-laboratory collaborative studies.
As with accuracy, precision can be affected by a number of factors. Use of inappropriate or uncalibrated equipment such as pipets or analytical balances, failure to control light or moisture when required, or inadequately trained analysts can all reduce precision. Inadequate chromatographic resolution, tailing peaks, and attempts to measure different analytes across an excessive dynamic range can also decrease precision as data handling systems struggle to perform integrations against unstable baselines. The problem is especially acute when simultaneously determining low and high levels of analytes in complex natural products. Finally, the lack of homogeneity between test portions in multi-laboratory studies can result in apparent imprecision.
2.1.4 Precision-Case Study
Decoctions of Má Huáng or ephedra ( Ephedra sinica Stapf., E. equisetina Bunge, E. intermedia var. tibetica Stapf., or E. distachya L.) are used in Traditional Chinese Medicine to expel cold wind. In western allopathic medicine, ephedrine and pseudoephedrine, first isolated from Ephedra spp. [ 16 ], are used for treatment of asthma and as a decongestant. Until banned from use as a dietary supplement ingredient by FDA in 2004 [ 17 ], ephedra plants and their extracts were used as ingredients in dietary supplements intended for weight loss and to “increase energy” [ 16 ]. Early FDA attempts to analyze ephedra-containing products for alkaloid content met with mixed success as the available published analytical methods were designed primarily for ephedrine and/or pseudoephedrine in finished pharmaceutical dosage forms or for a single plant species. Ephedra products marketed in the US as dietary supplements were almost always sold as mixtures of several plant species and often included caffeine and other alkaloids. Figure 2A is typical a HPLC chromatogram [ 18 ] of a multi-botanical ephedra product using a published method for separation of ephedrine alkaloids in ephedra herb [ 19 ]. The sample was run as part of an FDA investigation [ 18 ], and sample preparation involved a solvent extraction without additional cleanup. Note the complexity of the chromatogram and the incomplete resolution of the pseudoephedrine (P) and N-methylephedrine (N-ME) peaks from non-ephedra botanical constituents. The separation was sufficient to allow identification of the major alkaloids, but repeat injections of the same sample yielded different area under the curve values due to difficulties in integration.

Comparison LC-UV chromatograms of dietary supplement products containing ephedra, caffeine, and other botanical ingredients using three different analytical methods. A: Extraction with no cleanup [ 18 , 19 ]. B: Solid-phase extraction [ 20 , 21 ]. C: AOAC Official Method of Analysis [ 22 – 24 ]. E=Ephedrine, P=Pseudoephedrine, N-ME=N-methylephedrine, NE=Norephedrine, NPE=Norpseudoephedrine, N-MPE=N-methylpseudoephedrine, Ph=Phentermine.
Figure 2B shows a chromatogram of a multi-herb ephedra product obtained [ 20 ] using a method [ 21 ] that included a solid-phase extraction cleanup step and phentermine (Ph) as internal standard. It provides for near-baseline separation of the six ephedra alkaloids in the complex multi-botanical product because the sample cleanup has removed most of the interfering substances. This method gave good precision for ephedrine (E) and pseudoephedrine (P) measurements, but norpseudoephedrine (NPE) was present in small quantities relative to E and was not well resolved from a small inflection in the baseline at about the same retention time. Thus, unreliable integration of the peak reduced precision for NPE. In addition, column performance and mobile-phase composition had to be carefully monitored for this separation. The peak eluting at 11.219 minutes in Figure 2B (just after pseudoephedrine) was identified by LC/MS as a phthalate that was leached from the solid-phase extraction (SPE) column used for cleanup. Consequently, small deviations in the organic content of the mobile-phase or column aging caused loss of resolution and imprecise integration of the pseudoephedrine peak.
Finally, Figure 2C shows a typical HPLC chromatogram of a multi-botanical ephedra product [ 22 ] obtained using the AOAC Official Method of Analysis [ 23 ]. This method yields much improved resolution and lack of interference for NPE, E, PE, and N-ME. A small interference with an unknown constituent remains with the NE peak. In the validation study that led to the approval of the official method, overall precision was deemed adequate only for E and PE [ 24 ]. Quantitative determination of the other four compounds was not sufficiently precise due to a lack of homogeneity in the blind duplicate test articles sent to the individual investigators in the collaborative study rather than to any fault of the method itself [ 24 ].
3. ADDITIONAL VALIDATION PARAMETERS
Additional parameters to be evaluated when demonstrating accuracy and precision are part of the method development and optimization process, or are performed during the validation process when demonstrating acceptable method performance. These parameters include limits of detection and quantification, linearity of the method, range, recovery, robustness and selectivity.
3.1 LIMITS OF DETECTION AND QUANTIFICATION
The Limit of Detection (LOD) is defined as the smallest amount or concentration of an analyte that can be reliably detected in a given type of sample or medium by a specific measurement process [ 25 ]. The United States Pharmacopeia defines the LOD as 2 or 3 times the baseline noise [ 26 ]. This is derived from the assumption that 3 times the noise will contain approximately 100% of the data from a normal distribution. Alternatively, the AOAC [ 9 ] and IUPAC [ 27 ] calculate limits from the variability of a blank matrix. With this methodology, the LOD is based on a minimum of 6 independent determinations of a matrix blank, where the LOD will equal the sum of the mean of blank measures and the product of the standard deviation of the blank measures and a numerical factor chosen according to the confidence level desired. The confidence level should be the Student t statistic with α = 0.05 [ 28 ], Alternatively, a value of 3 can also be used according to AOAC [ 9 ] and IUPAC [ 27 ].
The FDA chromatography guidance document notes that simply using instrument noise to estimate the limits is not adequate [ 8 ]. According to FDA, the value obtained from the chromatogram can be considered as an instrument detection limit rather than a method detection limit because the baseline noise technique does not take into consideration errors that occur during sample preparation. Although a blank that has gone through the entire sample preparation procedure may account for some of these errors, it is important to consider analyte specific effects, such as the UV extinction coefficient, which may contribute to the detection limit. Therefore, it is recommended that the LODs be calculated from the analysis of samples containing the analyte of interest [ 8 , 27 , 28 ]. The U.S. Environment Protection Agency (EPA) defines the Method Detection Limit (MDL) to be product of the standard deviation and Student t value calculated from the analysis of at least seven samples containing a low level of analyte that is near the actual detection limit [ 29 ]. All of the described methods are statistical estimates of the limit of detection and the levels should be verified under actual conditions of use.
Another limit to consider for an analytical method is the Limit of Quantification (LOQ). The LOQ is the amount of substance that can reliably be assigned a quantitative value. This limit is usually defined as 10% RSD [ 27 ] or as a fixed multiple (typically 10) of the noise [ 26 ] or standard deviation [ 29 ] used to calculate the detection limit.
3.2 LINEARITY & RANGE
In a validated method, the detector response should be linear over the anticipated range of analyte concentrations. Linearity is determined by creating a minimum 5 level calibration curve using the analyte(s) of interest. The resulting plot of detector response versus analyte concentration should have a regression coefficient of at least 0.999, and should be visually inspected for areas of non-linearity. Figures 3A and 3B [ 8 ] show plots of area under the curve versus concentration for two different analytes. Figure 3A shows an acceptable linearity over the entire range of concentrations evaluated, while Figure 3B does not. Figure 3C is a gas chromatogram of an extract of an ephedra product [ 16 ] obtained using a nitrogen/phosphorous detector. The chromatogram is enlarged to allow visualization of the minor alkaloid peaks (N-MPE, PE, N-ME, NE), and the ephedrine peak was truncated in this view. Truncation can result in integration errors, and in fact the calibration curve across the entire range of analytes was not linear. In this case, the sample had to be analyzed twice: the first analysis was performed on an undiluted sample, and the second on a diluted sample in order to bring the detector response for the ephedrine peak into the linear portion of the calibration curve. Both analyses were necessary, because the dilution step dropped the minor alkaloid concentrations below their limits of detection. Knowing the working range, (i.e., the interval between the high and low levels of analytes to be determined) of a method prevents erroneous interpretation of results.

Linearity and dynamic range. A, B: Calibration curves plotting generic detector response versus concentration [ 8 ]. C: Gas chromatogram of an ephedra product extract using a Nitrogen/Phosphorous Detector [ 16 ]. E=Ephedrine, P=Pseudoephedrine, N-ME=N-methylephedrine, NPE=Norephedrine, N-MPE=N-methylpseudoephedrine, Ph=Phentermine, ?=Unknown.
3.3 ROBUSTNESS
Robustness is typically evaluated during method development/optimization, but can have a pronounced effect on the validation of a method. Robustness experiments measure a method s ability to remain unaffected by small but deliberate variations in method parameters. Examples of potentially sensitive processes include extraction time, extraction temperature, and extraction process (soxhlet, wrist shaker, orbital shaker). Column oven temperature, the percent organic phase, pH, or buffer concentration of mobile phase may also be important for chromatographic separations. Figure 4 provides a graphic comparison between chromatography outcomes in LC/MS analyses of ephedrine alkaloids and shows the differences in baseline noise, chromatographic resolution, peak shape, and analysis time achieved when HPLC columns with different carbon loading (4A) or ion-pairing reagents (4B) were used [ 30 ]. Impact of ion-pairing reagents and other factors on detector response is not addressed, but may be important to overall method performance.

Representative total ion chromatogram of mixtures of six ephedra alkaloid standards plus ephedrine-d 5 . A: Separation using 3 different ion-pairing reagents. B: Separation on LC columns with different amounts of carbon loading. 1=norephedrine, 2=norpseudoephedrine, 3=ephedrine-d 5 , 4=ephedrine, 5=pseudoephedrine, 6=N-methylephedrine, and 7=methylpseudoephedrine [ 23 ].
Although the parameters affecting the method can be explored using an approach that tests one variable at a time, the use of factorial studies can be much more efficient when facing a large number of factors [ 9 , 31 ]. For instance, the AOAC International recommends the use of a Youden Ruggedness Trial that permits the examination of up to 7 factors in a single experiment requiring only 8 determinations [ 9 ].
3.4 SPECIFICITY (SELECTIVITY)
It is vital to ensure the identity of the chromatographic peak that will be measured. When evaluating the previously mentioned HPLC method for determination of ephedrine-alkaloids in botanical supplements [ 21 ], a matrix blank was run using Ephedra nevadensis as the test article. This North American species was once thought to contain pseudoephedrine [ 32 ], but this claim has been controversial. Analysis using the method shown in Figure 2B produced a chromatogram (not shown) that had a flat baseline except for a small, unexpected peak that the HPLC/UV data system erroneously identified as pseudoephedrine. As noted previously, LC/MS analysis found this peak to be a phthalate from the solid-phase extraction column. Instead of an confirming the presence of pseudoephedrine in E. nevadensis , this only showed that certain solvents are incompatible with certain brands of SPE columns. The claim that E. nevadensis contains ephedrine-types alkaloids was subsequently dismissed [ 33 ]. A classical technique for verifying, but not proving, analyte identity is standard addition to a natural matrix that contains the compound of interest. Other techniques for analyte verification include the use of a photodiode array detector or a mass spectrometer. An earlier technique collects the eluted peak and performs subsequent mass spectrometry or another identity analysis.
3.5 REFERENCE MATERIALS
Finally, identity, purity, and stability of reference compounds must be confirmed. While the case for reference material purity was already made above, the authors have experienced instances in which commercial chemicals intended for use as reference materials have been incorrectly identified. In one case, proton NMR was used to confirm the identity of purchased hydrastine when received from the supplier. The experiment demonstrated that the alkaloid dimer (hydrastine) had decomposed into hydrastinine, its constituent monomers. In a second case, the detergent, 3-[(3-cholamidopropyl)dimethylammonio]-1-propanesulfonate (CHAPS), had been shipped labeled as caffeine. These incidents typically do not make it into the peer-reviewed literature, but do occur.
In the age of reliable autosamplers, it is also important to assure the stability of analytical standards and target analytes in solution for the duration of the test-run. In the gas chromatogram seen in Figure 3C , the small peak eluting a few minutes before the N-MPE peak was not present when the extract was first made. As the solution aged, it turned from clear and colorless to yellow. As the color of the solution increased, so did the size of the unidentified peak. Solutions of the pure compound NE also turned yellow with time, even at refrigerator temperatures, and the size of the unknown peak increased as the intensity of the yellow color increased. More important, the size of the NE peak decreased as the size of the unknown peak increased.
In practice, it is often difficult or impossible to confirm the purity of reference materials due to their limited availability and cost. In these situations, certificates of analyses should be examined for accuracy and completeness. Determination of moisture, residual solvents, residue on ignition (inorganics), and chromatographic purity (preferably by two independent methods) are all needed to obtain an accurate assessment of material suitability. Moisture in particular can be problematic, and it is important to equilibrate the standards before use under the same conditions used prior to the moisture determination.
3.6 CHROMATOGRAPHIC PERFORMANCE
While extraction efficiency, analyte stability and purity, linearity, recovery, and selectivity are important to the final result, they must all lead to a viable separation. This is evaluated by determining system suitability. A typical approach involves development of an optimized method with adequate system suitability, prior to performing validation studies. The FDA reviewer guidance [ 8 ] suggests that the peak of interest should have a capacity factor (k′) greater than or equal to 2 and a resolution (R S ) greater than 2. Additional desirable characteristics are provided in detail in the FDA guidance [ 6 ] and in numerous other sources [ 3 , 9 , 12 , 26 , 27 , 34 – 37 ].
4. CONCLUSIONS
Systematic evaluation of analytical method performance is critical to the utility of analytical methods and to the integrity of scientific research. While accuracy, precision, and fitness for purpose are often assumed in published methods, this assumption does not bear close scrutiny in many cases. Accurate measurements are as important in clinical- and pre-clinical studies as they are in regulatory or manufacturing environments. While demonstration of performance should be a pre-requisite for any quantitative method used in a laboratory, the burden of proving that any measurements made are correct and reproducible depends on the intended use and pedigree of the method being evaluated.
There are a number of validation study designs available, and each is intended to accomplish certain pre-defined goals. In-house or single laboratory validation (SLV) studies can demonstrate applicability of the method to the analysis at hand, evaluate intra-laboratory performance, ruggedness, accuracy, and repeatability while identifying interferences and critical control points [ 9 ]. Inter-laboratory collaborative studies, including but not limited to studies for the purpose of creating AOAC Official Methods of Analysis, provide information on inter-laboratory reproducibility [ 12 ].
Finally, performing validation experiments is often viewed as “technician s work”. However, designing an appropriate validation protocol that will demonstrate the functional qualities required of the method, performing the appropriate statistics on the results, and drawing the correct conclusions from those statistics requires considerable knowledge and intellectual input. Knowledgeable senior scientists should be involved in assuring integrity of published quantitative chemical data of natural product analysis.
Supplementary Material
Publisher's Disclaimer: This is a PDF file of an unedited manuscript that has been accepted for publication. As a service to our customers we are providing this early version of the manuscript. The manuscript will undergo copyediting, typesetting, and review of the resulting proof before it is published in its final citable form. Please note that during the production process errors may be discovered which could affect the content, and all legal disclaimers that apply to the journal pertain.
Research on High-Precision and Low-Power Quartz MEMS Gyroscope Signal Demodulation Circuit Based on AFE and FPGA Fusion
Ieee account.
- Change Username/Password
- Update Address
Purchase Details
- Payment Options
- Order History
- View Purchased Documents
Profile Information
- Communications Preferences
- Profession and Education
- Technical Interests
- US & Canada: +1 800 678 4333
- Worldwide: +1 732 981 0060
- Contact & Support
- About IEEE Xplore
- Accessibility
- Terms of Use
- Nondiscrimination Policy
- Privacy & Opting Out of Cookies
A not-for-profit organization, IEEE is the world's largest technical professional organization dedicated to advancing technology for the benefit of humanity. © Copyright 2024 IEEE - All rights reserved. Use of this web site signifies your agreement to the terms and conditions.

Title: Research on high-precision time synchronisation technology for sea mobile platforms
Authors : Dao Peng Dong; Hong Shuo Wu; Qing Feng Guo; Jin Wei Yang; Xi Li
Addresses : Cheng Du Spaceon Electronics Co., Ltd., Cheng Du 610036, China; Laboratory of Science and Technology on Marine Navigation and Control, China State Shipbuilding Corporation, 300131, China ' Tianjin Institute of Navigation Instruments, 300131, China ' Cheng Du Spaceon Electronics Co., Ltd., Cheng Du 610036, China ' Cheng Du Spaceon Electronics Co., Ltd., Cheng Du 610036, China ' Cheng Du Spaceon Electronics Co., Ltd., Cheng Du 610036, China
Abstract : Under static conditions, at present, mature technologies for long-distance and high-precision time synchronisation include satellite common view (CV) and two-way satellite time and frequency transfer (TWSTFT) and so on. However, under dynamic conditions, research on high-precision time synchronisation technology is relatively lacking such as mobile platforms on the sea. Considering the dynamic conditions of mobile platforms on the sea, a method of position's smooth filtering with velocity is proposed to improve the accuracy of position measurement, reducing the time measurement error introduced by position error. The proposed method ultimately improves the CV comparison accuracy between sea surface mobile platforms. The simulation and actual test results show that by using the method of position's smooth filtering with velocity, the CV comparison accuracy between mobile platforms on the sea can reach 10 ns.
Keywords : mobile platform; time and frequency synchronisation; spaceon electronics; common view; CV.
DOI : 10.1504/IJICT.2024.138561
International Journal of Information and Communication Technology, 2024 Vol.24 No.6, pp.57 - 70
Received: 17 Jan 2024 Accepted: 24 Feb 2024 Published online: 12 May 2024 *
Keep up-to-date
- Our Newsletter ( subscribe for free )
- New issue alerts
- Inderscience is a member of publishing organisations including:
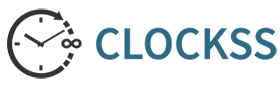
Image-Recognition-Based Embedded System for Excavator Bucket Tracking in Construction Sites
- Regular Paper
- Published: 16 May 2024
Cite this article
- Jaemin Shin 1 ,
- Hyunbin Park 1 ,
- Hyeonjae Jeong 1 ,
- Hyeongyeong Jeong 1 &
- Beaksuk Chu ORCID: orcid.org/0000-0002-3253-1506 2
Construction sites involving excavators often generate substantial amounts of fine dust at the operating position of the excavator bucket. To address the problem, this research proposes a system that tracks the excavator’s bucket using only a single camera and an artificial intelligence-based image recognition algorithm, aiming to improve accuracy and efficiency compared to conventional methods that utilize multiple sensors. To enhance the accuracy of image recognition, a bucket dataset containing background images was utilized. Real-time object tracking performance exceeding 30 FPS was achieved by applying a graphics processing unit optimizer. Moreover, a function was implemented to track a specific object when multiple objects with similar characteristics are detected. The system also features a control system that utilizes these functions to apply a pan-tilt motion mechanism to the camera, enabling the tracking of the identified bucket position. Extensive experiments, including image recognition for tracking objects exhibiting various motion trajectories and estimating the position of invisible objects, have been conducted to validate the performance of the system.
This is a preview of subscription content, log in via an institution to check access.
Access this article
Price includes VAT (Russian Federation)
Instant access to the full article PDF.
Rent this article via DeepDyve
Institutional subscriptions
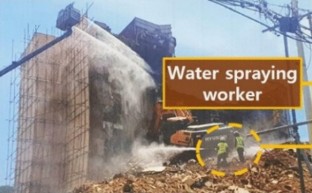
Karkhanis, V., & Joshi, J. M. (2011). Cement dust exposure-related emphysema in a construction worker. Lung India: Official Organ of Indian Chest Society, 28 (4), 294–294. https://doi.org/10.4103/0970-2113.85694
Article Google Scholar
Tong, R., Cheng, M., Zhang, L., Liu, M., Yang, X., Li, X., & Yin, W. (2018). The construction dust-induced occupational health risk using Monte-Carlo simulation. Journal of Cleaner Production, 184 , 598–608. https://doi.org/10.1016/j.jclepro.2018.02.286
Haynes, R., & Savage, A. (2007). Assessment of the health impacts of particulates from the redevelopment of kings cross. Environmental Monitoring and Assessment, 130 , 47–56. https://doi.org/10.1007/s10661-006-9449-5
Mondal, A. K., Mondal, S., Devalla, V., Sharma, P., & Gupta, M. K. (2016). Advances in floating aerogenerators: Present status and future. International Journal of Precision Engineering and Manufacturing, 17 , 1555–1568. https://doi.org/10.1007/s12541-016-0182-x
Sharma, A., Mondal, S., Mondal, A. K., Baksi, S., Patel, R. K., Chu, W. S., & Pandey, J. K. (2017). 3D printing: It’s microfluidic functions and environmental impacts. International Journal of Precision Engineering and Manufacturing-Green Technology, 4 , 323–334. https://doi.org/10.1007/s40684-017-0038-6
Yu, H., Cheng, W., Peng, H., & Xie, Y. (2018). An investigation of the nozzle’s atomization dust suppression rules in a fully-mechanized excavation face based on the airflow-droplet-dust three-phase coupling model. Advanced Powder Technology, 29 (4), 941–956. https://doi.org/10.1016/j.apt.2018.01.012
Zhang, Y., Tang, W., Li, H., Guo, J., Wu, J., & Guo, Y. (2022). The evaluation of construction dust diffusion and sedimentation using wind tunnel experiment. Toxics, 10 (8), 412. https://doi.org/10.3390/toxics10080412
Gambatese, J. A., & James, D. E. (2001). Dust suppression using truck-mounted water spray system. Journal of Construction Engineering and Management, 127 (1), 53–59. https://doi.org/10.1061/(ASCE)0733-9364(2001)127:1(53)
Lee, S., Park, H., & Chu, B. (2022). Excavator posture estimation and position tracking system based on kinematics and sensor network to control mist-spraying robot. IEEE Access, 10 , 107949–107960. https://doi.org/10.1109/ACCESS.2022.3212732
Arshi, O., & Mondal, S. (2023). Advancements in sensors and actuators technologies for smart cities: A comprehensive review. Smart Constr. Sustain. Cities, 1 , 18. https://doi.org/10.1007/s44268-023-00022-2
Sharma, N., Pandey, J. K., & Mondal, S. (2023). A review of mobile robots: applications and future prospect. International Journal of Precision Engineering and Manufacturing, 24 , 1695–1706. https://doi.org/10.1007/s12541-023-00876-7
Pandey, U., Pathak, A., Kumar, A., & Mondal, S. (2023). Applications of artificial intelligence in power system operation, control, and planning: A review. Clean Energy, 7 (6), 1199–1218. https://doi.org/10.1093/ce/zkad061
Ghosh, K., Pandey, U., Pathak, A., & Mondal, S. (2023). Simulation of density based traffic control system using proteus 7.1 professional. In G. Mathur, M. Bundele, A. Tripathi, & M. Paprzycki (Eds.), Proceedings of 3rd international conference on artificial intelligence: advances and applications: ICAIAA 2022 (pp. 493–504). Singapore: Springer Nature Singapore. https://doi.org/10.1007/978-981-19-7041-2_41
Chapter Google Scholar
Soltani, M. M., Zhu, Z., & Hammad, A. (2017). Skeleton estimation of excavator by detecting its parts. Automation in Construction, 82 , 1–15. https://doi.org/10.1016/j.autcon.2017.06.023
Lee, S., & Lee, S. (2022). Recent advances of artificial intelligence in manufacturing industrial sectors: A review. International Journal of Precision Engineering and Manufacturing (IJPEM), 23 , 111–129. https://doi.org/10.1007/s12541-021-00600-3
Park, S. (2023). Improving image monitoring performance for underwater laser cutting using a deep neural network. International Journal of Precision Engineering and Manufacturing (IJPEM), 24 , 671–682. https://doi.org/10.1007/s12541-023-00771-1
Chiddarwar, S. (2023). Integrating virtual twin and deep neural networks for efficient and energy-aware robotic deburring in industry 4.0. International Journal of Precision Engineering and Manufacturing (IJPEM), 24 , 1517–1534. https://doi.org/10.1007/s12541-023-00875-8
Ren, Z., Fang, F., & Yan, N. (2022). State of the Art in Defect Detection Based on Machine Vision. Int. J. of Precis. Eng. and Manuf.-Green Tech. 9, 661–691. https://doi.org/10.1007/s40684-021-00343-6
Kong, J. H., & Lee, S. W. (2023). Development of melt-pool monitoring system based on degree of irregularity for defect diagnosis of directed energy deposition process. International Journal of Precision Engineering and Manufacturing-Smart Technology, 1 (2), 137–143. https://doi.org/10.57062/ijpem-st.2023.0045
Wojke, N., Bewley, A., & Paulus, D. (2017). Simple online and real-time tracking with a deep association metric. IEEE International Conference on Image Processing (ICIP), 2017 , 3645–3649. https://doi.org/10.1109/ICIP.2017.8296962
Bochkovskiy, A., Wang, C. Y., & Liao, H. Y. (2020). YOLOv4: Optimal speed and accuracy of object detection. arXiv preprint arXiv:2004.10934 . https://doi.org/10.48550/arXiv.2004.10934
Choi, E., & Kim, J. (2020). Deep learning based defect inspection using the intersection over minimum between search and abnormal regions. International Journal of Precision Engineering and Manufacturing, 21 , 747–758. https://doi.org/10.1007/s12541-019-00269-9
Kim, S. W., Kong, J. H., & Lee, S. W. (2022). Recent advances of artificial intelligence in manufacturing industrial sectors: A review. International Journal of Precision Engineering and Manufacturing, 23 , 111–129. https://doi.org/10.1007/s12541-021-00600-3
Vanholder, H. (2016). Efficient Inference with TensorRT. GPU Technology Conference, 1 , 2.
Google Scholar
Kwak, J., Lee, S., & Baek, J. (2022). Autonomous UAV target tracking and safe landing on a leveling mobile platform. International Journal of Precision Engineering and Manufacturing, 23 , 305–317. https://doi.org/10.1007/s12541-021-00617-8
Liu, P., Chi, H. L., Li, X., & Guo, J. (2021). Effects of dataset characteristics on the performance of fatigue detection for crane operators using hybrid deep neural networks. Automation in Construction, 132 , 103901. https://doi.org/10.1016/j.autcon.202
Shahinfar, S., Meek, P., & Falzon, G. (2020). “How many images do I need?” Understanding how sample size per class affects deep learning model performance metrics for balanced designs in autonomous wildlife monitoring. Ecological Informatics, 57 , 101085. https://doi.org/10.1016/j.ecoinf.2020.101085
Download references
Acknowledgements
This research was supported by the MSIT (Ministry of Science and ICT), Korea, under the ICAN (ICT Challenge and Advanced Network of HRD) program (IITP-2024-RS-2022-00156394), supervised by the IITP (Institute of Information & Communications Technology Planning & Evaluation).
Author information
Authors and affiliations.
Department of Aeronautics, Mechanical, and Electronic Convergence Engineering, Kumoh National Institute of Technology, 61, Daehak-Ro, Gumi-Si, Gyeongsangbuk-Do, 391777, South Korea
Jaemin Shin, Hyunbin Park, Hyeonjae Jeong & Hyeongyeong Jeong
Department of Mechanical System Engineering, Kumoh National Institute of Technology, 61, Daehak-Ro, Gumi-Si, Gyeongsangbuk-Do, 3917, South Korea
Beaksuk Chu
You can also search for this author in PubMed Google Scholar
Corresponding author
Correspondence to Beaksuk Chu .
Additional information
Publisher's note.
Springer Nature remains neutral with regard to jurisdictional claims in published maps and institutional affiliations.
Rights and permissions
Springer Nature or its licensor (e.g. a society or other partner) holds exclusive rights to this article under a publishing agreement with the author(s) or other rightsholder(s); author self-archiving of the accepted manuscript version of this article is solely governed by the terms of such publishing agreement and applicable law.
Reprints and permissions
About this article
Shin, J., Park, H., Jeong, H. et al. Image-Recognition-Based Embedded System for Excavator Bucket Tracking in Construction Sites. Int. J. Precis. Eng. Manuf. (2024). https://doi.org/10.1007/s12541-024-01025-4
Download citation
Received : 16 August 2023
Revised : 12 April 2024
Accepted : 17 April 2024
Published : 16 May 2024
DOI : https://doi.org/10.1007/s12541-024-01025-4
Share this article
Anyone you share the following link with will be able to read this content:
Sorry, a shareable link is not currently available for this article.
Provided by the Springer Nature SharedIt content-sharing initiative
- Vision recognition
- Deep learning
- Excavator bucket tracking
- Multi-object tracking
- Find a journal
- Publish with us
- Track your research
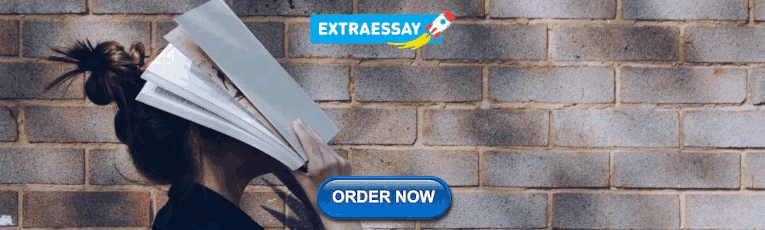
IMAGES
VIDEO
COMMENTS
Accuracy assesses whether a series of measurements are correct on average. For example, if a part has an accepted length of 5mm, a series of accurate data will have an average right around 5mm. In statistical terms, accuracy is an absence of bias. In other words, measurements are not systematically too high or too low.
Common technical definition. Accuracy is the proximity of measurement results to the accepted value; precision is the degree to which repeated (or reproducible) measurements under unchanged conditions show the same results. In the fields of science and engineering, the accuracy of a measurement system is the degree of closeness of measurements ...
5.2 Precision and accuracy. Two issues concerning sampling, raised in Sect. 5.1, were: which individuals should be in the sample, and how many individuals should be in the sample be. These two issues address two different aspects of sampling: precision and accuracy (Fig. 5.1). Accuracy refers to how close a sample estimate is to the population value (on average).
Accuracy and precision are two important factors to consider when taking data measurements. Both accuracy and precision reflect how close a measurement is to an actual value, but accuracy reflects how close a measurement is to a known or accepted value, while precision reflects how reproducible measurements are, even if they are far from the ...
This entry was posted on April 14, 2014 by Todd Helmenstine (updated on August 16, 2021) Accuracy is how close a measurement is to the true value while precision is close measurements are to each other. Accuracy and precision are two important concepts in science. Both terms apply to any experimental measurement you make.
Accuracy and Precision (2012) This video from Region 10 ESC (2:34 min.) explains how you can have a precise measurement that is not accurate, and an accurate measurement that is not precise. Or worse, a measurement that is both inaccurate and imprecise! Learn about precision and accuracy and why they are important in science.
The research goal was to estimate the reliability and validity of the questionnaire on children's health status. The questionnaire is a parent-report instrument, and it consists of 30 questions that parents answered about their children's health status. ... Measurement: Accuracy and Precision, Reliability and Validity . In: Kirch, W. (eds ...
An important factor in the accuracy and precision of measurements involves the precision of the measuring tool. In general, a precise measuring tool is one that can measure values in very small increments. For example, a standard ruler can measure length to the nearest millimeter, while a caliper can measure length to the nearest 0.01 ...
We support that accuracy cannot be intended as composed by trueness and precision (as declared by various standards of international—as ISO—or national organisms), since trueness and precision require large number (infinite number, according to VIM 2012) of replicate measured quantity values to be assessed while accuracy refers to a single ...
Acquire supplementary data that provide indicators of the quality of experimental data. These indicators include precision (i.e., repeatability, with statistics such as standard deviation and variance), accuracy (which can be assessed by applying alternative [orthogonal] methods or by comparison to a reference material), sensitivity to environmental or experimental perturbants (by testing for ...
Accuracy and precision are used in context of measurement. Accuracy refers to the degree of conformity and correctness of something when compared to a true or absolute value, while precision refers to a state of strict exactness — how consistently something is strictly exact.. In other words, the precision of an experiment, object, or value is a measure of the reliability and consistency.
reflect the precision of these measurements (Kotz and Johnson 1982 /1988). Accuracy Bias and precision combine to define the performance of an estimator. The more biased and the less precise an estimator is, the worse its overall ability to make an accurate point estimation. Accuracy is thus defined as the overall distance between estimated (or ...
In middle and high school, science teachers emphasized the importance of precision, accuracy, and validity in measurement. It may have seemed redundant in years past, but these principles have become even more important in academic research. Whether you're an interested hobbyist or a science lecturer, every higher ed professional connected to ...
The terms 'accuracy' and 'precision' are commonly used in the scientific literature to describe the quality of, for example, measurements or methods, where traditional definitions of the terms often associate 'accuracy' with systematic errors and 'precision' with random errors.
The goal of this article is to define, examine, and discuss the validity of research problems in primary psychological research. The psychological-research process starts with (0) an idea about the phenomenon of interest, followed by (1) a research-problem statement that includes a literature review of past research on the phenomenon and the research question the studies seek to answer (ideas ...
The importance of precision in the history of science is difficult to overestimate. As a famous example in chemistry, Lavoisier's advances in the precision of measurement helped overturn the phlogiston theory that had dominated for approximately two centuries (see Asimov, 1965, and Trafimow & Rice, 2009, for accessible descriptions).Advances in measurement precision have also enabled ...
A classic way of demonstrating the difference between precision and accuracy is with a dartboard. Think of the bulls-eye (center) of a dartboard as the true value. ... Exploring Our Fluid Earth, a product of the Curriculum Research & Development Group (CRDG), College of Education. University of Hawaii, 2011. This document may be freely ...
Enhancing Validity and Reliability: Precision plays a pivotal role in ensuring the validity and reliability of clinical research. Rigorous study designs, well-defined protocols, and standardized data collection methods are key components of precision. By adhering to these principles, researchers can minimize bias, confounding factors, and ...
Accuracy symbolizes the extent of conformity, whereas Precision indicates the extent of reproducibility. At the time of taking measurements, these two are always taken into account, due to their utmost importance in the various field, such as science, statistics, research and engineering.
2.1 ACCURACY AND PRECISION. A good starting point for basic definitions and descriptions of the key terms and concepts pertaining to the assurance of the quality of quantitative chemical measurements is the U.S. Food and Drug Administration s (FDA) Reviewer Guidance [].The two most important elements of a chromatographic test method are accuracy and precision.
Accuracy. Accurate measurements are those close to what they should be. We know the temperature of the human body is 37°C. An accurate thermometer will give us this value (unless we are poorly ...
2.1.3. Precision. The FDA guidance document on validation of chromatographic methods [8] breaks the overall concept of precision into three components: repeatability, intermediate precision, and reproducibility. Repeatability is a measure of the within-laboratory uncertainty. It takes into account the reproducibility of injections and other aspects of the analysis such as weighing, fluid ...
Feature papers represent the most advanced research with significant potential for high impact in the field. A Feature Paper should be a substantial original Article that involves several techniques or approaches, provides an outlook for future research directions and describes possible research applications.
Mainstream quartz MEMS gyroscope signal demodulation methods are coherent demodulation methods, and the sensitivity of phase limits their accuracy improvement. In order to improve the accuracy of quartz MEMS gyroscope signal demodulation and achieve low-power gyroscope signal demodulation, a high-precision and low-power quartz MEMS gyroscope signal demodulation circuit based on AFE and FPGA ...
The Agro-Ecological (AE) zone plays a vital role in determining which crops are suitable for cultivation in specific areas of land. However, changing environmental and climate conditions have made it increasingly difficult for farmers to choose the right crops, resulting in both time and financial losses. Traditional research in this field has struggled with issues like inaccurate predictions ...
However, under dynamic conditions, research on high-precision time synchronisation technology is relatively lacking such as mobile platforms on the sea. Considering the dynamic conditions of mobile platforms on the sea, a method of position's smooth filtering with velocity is proposed to improve the accuracy of position measurement, reducing ...
Construction sites involving excavators often generate substantial amounts of fine dust at the operating position of the excavator bucket. To address the problem, this research proposes a system that tracks the excavator's bucket using only a single camera and an artificial intelligence-based image recognition algorithm, aiming to improve accuracy and efficiency compared to conventional ...
The Zestimate® home valuation model is Zillow's estimate of a home's market value. A Zestimate incorporates public, MLS and user-submitted data into Zillow's proprietary formula, also taking into account home facts, location and market trends. It is not an appraisal and can't be used in place of an appraisal.