
An official website of the United States government
The .gov means it’s official. Federal government websites often end in .gov or .mil. Before sharing sensitive information, make sure you’re on a federal government site.
The site is secure. The https:// ensures that you are connecting to the official website and that any information you provide is encrypted and transmitted securely.
- Publications
- Account settings
Preview improvements coming to the PMC website in October 2024. Learn More or Try it out now .
- Advanced Search
- Journal List
- Ther Adv Ophthalmol
- v.13; Jan-Dec 2021

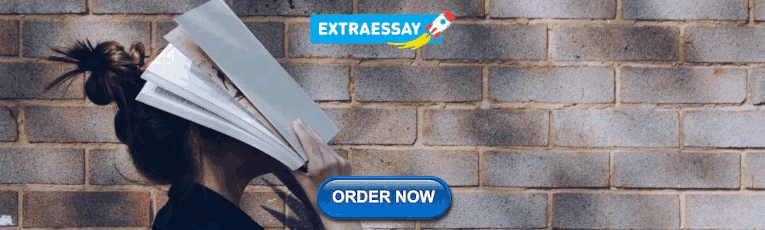
Advances in the diagnosis and treatment of keratoconus
Eray atalay.
Department of Ophthalmology, Medical School, Eskişehir Osmangazi University, Meşelik Kampüsü, Odunpazarı, Eskişehir 26040, Turkey
Onur Özalp
Department of Ophthalmology, Medical School, Eskişehir Osmangazi University, Eskişehir, Turkey
Nilgün Yıldırım
Keratoconus had traditionally been considered a rare disease at a time when the imaging technology was inept in detecting subtle manifestations, resulting in more severe disease at presentation. The increased demand for refractive surgery in recent years also made it essential to more effectively detect keratoconus before attempting any ablative procedure. Consequently, the armamentarium of tools that can be used to diagnose and treat keratoconus has significantly expanded. The advances in imaging technology have allowed clinicians and researchers alike to visualize the cornea layer by layer looking for any early changes that might be indicative of keratoconus. In addition to the conventional geometrical evaluation, efforts are also underway to enable spatially resolved corneal biomechanical evaluation. Artificial intelligence has been exploited in a multitude of ways to enhance diagnostic efficiency and to guide treatment. As for treatment, corneal cross-linking treatment remains the mainstay preventive approach, yet the current main focus of research is on increasing oxygen availability and developing new strategies to improve riboflavin permeability during the procedure. Some new combined protocols are being proposed to simultaneously halt keratoconus progression and correct refractive error. Bowman layer transplantation and additive keratoplasty are newly emerging alternatives to conventional keratoplasty techniques that are used in keratoconus surgery. Advances in tissue engineering and regenerative therapy might bring new perspectives for treatment at the cellular level and hence obviate the need for invasive surgeries. In this review, we describe the advances in the diagnosis and treatment of keratoconus primarily focusing on newly emerging approaches and strategies.
Introduction
The armamentarium of tools that can be used to diagnose keratoconus has significantly expanded in recent years ( Figure 1 ). Advances in ocular imaging technology not only allowed significant improvements in the geometrical evaluation of the cornea but also has opened new avenues for characterizing other corneal features such as the biomechanics. With these developments, a more in-depth understanding of disease pathology became possible along with the realization that the actual prevalence of keratoconus may be much higher than what was reported over three decades ago using less-sophisticated diagnostic modalities. 1 Advances in treatment followed suit, providing the opportunity to avoid full-thickness keratoplasty and its associated complications with newer preventive and refractive approaches. This review will focus on the latest developments in the diagnosis and treatment of keratoconus with a primary emphasis on newly emerging approaches and strategies. Hence, approaches that are already established and in widespread clinical use will not be covered. Publications that relate to advances in diagnosis and treatment of keratoconus (indexed in PubMed) between the years 2017 and 2020 were used in this review.

A chart of advances in the diagnosis of keratoconus.
Advances in diagnosis
Corneal epithelial and bowman’s layer thickness mapping.
The corneal epithelium is a dynamic layer that adapts to the changes in the underlying stroma either by thickening or thinning. This property has been used to diagnose keratoconus particularly in instances where epithelial remodeling masks slight changes in the corneal curvature, hampering corneal topographical evaluation. Anterior segment optical coherence tomography (AS-OCT) has shown excellent repeatability for measuring corneal epithelial thickness, and its clinical use for this purpose has become more widespread. Initially designed to characterize epithelial thickness in a 6-mm zone, a recent upgrade allows expansion up to 9 mm, equivalent to an area that is approximately two and a quarter times larger than the former. 2 Using a custom-designed polarization-sensitive OCT, Pircher and colleagues 3 were able to map the thickness of the Bowman’s layer in addition to the epithelium from limbus-to-limbus and noted a highly irregular “moth-like” damage pattern in the Bowman’s layer in keratoconic eyes. Another group employed a commercially available swept-source OCT and by postprocessing, delineation of the air–epithelium edge and epithelium–Bowman’s layer interface was made possible. 4 This approach enabled them to quantify the curvature and aberrations in these locations, which in a subsequent study was shown to outperform conventional Scheimpflug imaging in diagnosing forme fruste keratoconus. 5
Corneal biomechanics
Analysis of corneal biomechanical properties brings with it the prospects of revealing the cause (biomechanical weakening) possibly much earlier than the effect (corneal tomographical changes), therefore allowing a timelier diagnosis. Moreover, a spatially resolved evaluation of corneal biomechanics has the potential to aid in localizing relatively weaker corneal areas in an individual thereby allowing a more personalized treatment. Although still in its infancy, Brillouin spectroscopy is one candidate that we will soon see transition from the research phase to widespread clinical use. In brief, this noncontact technique relies on the detection of a Brillouin frequency shift in a laser light that occurs during an interaction with the phonons in a matter. The bulk elastic modulus of the cornea is derived using the mathematical relationship between the frequency shift and the velocity of the interacting phonons, and hence the elastic modulus. 6 Although with great future potential, its reported in vivo accuracy in distinguishing eyes with keratoconus from normal eyes was relatively weak. 6 , 7 As opposed to Brillouin spectroscopy, optical coherence elastography provides a depth-dependent analysis of the cornea using the ultrasound elastography principle. 8 A pilot study has shown that there was selective anterior stromal weakening in eyes with keratoconus when compared with normal eyes. 9 Other recently reported techniques that have been used in the biomechanical characterization of the cornea include digital image correlation, 10 high-resolution shear wave imaging, 11 and phase-decorrelation OCT. 12 As the cornea is a multilayered tissue with different anatomical and mechanical characteristics in the x, y, and z-directions, approaches that aim for a three-dimensional (3D) biomechanical evaluation would be beneficial. Furthermore, it must be kept in mind that the cornea is an anisotropic viscoelastic material exhibiting a nonlinear stress–strain relationship; hence, it does not have a constant elastic modulus. This makes it difficult to accurately characterize the biomechanical properties of the cornea in an in vivo setting.
The widely held belief that keratoconus is a noninflammatory condition that has been challenged in recent years by findings that point to the contributory role of inflammation in ectasia development. Earlier studies have reported an increase in tear inflammatory cytokine and matrix metalloproteinase levels along with significant reductions in tear IgA, total tear protein production, and lactoferrin. 13 – 15 Parallel to these findings, serum analysis of patients with keratoconus showed altered levels of immunoglobulins suggesting a link between atopy and keratoconus. 16 Although the mechanism is still unclear, it has been found that keratoconus is associated with atopic diseases, in which serum immunoglobulin levels are detected to be high, such as asthma, allergic rhinitis, combination of allergic conjunctivitis, chronic blepharitis, and vernal keratoconjunctivitis. 17 A recent comparative study by McKay and colleagues 18 revealed that the distribution of tear immunoglobulin heavy and light chains is different between keratoconus and healthy controls, suggesting that a disturbance in B-cell function may also play a role in keratoconus pathogenesis. Another study found higher levels of innate biomarkers, namely toll-like receptors 2 and 4, on the ocular surface of subclinical keratoconus patients, and drawing from this finding, the authors advocated their use as a biomarker in identifying keratoconus cases. 19 Interestingly, Fodor and colleagues 20 demonstrated that a concomitant increase in tear nerve growth factor and interleukin (IL)-13 predicts keratoconus progression with a sensitivity and specificity of 80% and 100%, respectively.
Several studies have shown altered hormone levels in the saliva, plasma, tear, hair follicles, and aqueous humor of patients with keratoconus providing evidence to hormonal regulation of cornea. 21 – 25 In a study by Sharif and colleagues, 22 the diagnostic accuracy of tear, plasma, and saliva prolactin–induced protein levels ranged from 92.8% to 93.7% and was found to be independent of the severity of keratoconus. The authors postulated that this protein could serve as a low-cost biomarker in keratoconus screening.
Artificial Intelligence
Artificial intelligence (AI) has been exploited in a myriad of ways in keratoconus diagnosis. Two recent studies showed that deep learning can effectively distinguish keratoconus from healthy eyes and determine the stage of the disease. 26 , 27 Combining features from AS-OCT and Scheimpflug imaging was shown to enhance the discriminant ability of a neural network algorithm for subclinical keratoconus compared to single instrument–derived parameters. 28 Integrating data derived from biomechanical evaluation of the cornea in such models may allow a more multifaceted approach further increasing diagnostic precision. 29 Deep learning has also been employed to aid in faster and more efficient segmentation of corneal OCT images (CorneaNet) aiming to create a model that could be used for early keratoconus detection. 30 With newer AI models that are based on longitudinal data sets, disease monitoring, and AI-guided therapies may also be possible. 31
Advances in treatment
Corneal cross-linking.
Corneal cross-linking (C-CXL), first described by Wollensak and colleagues 32 in 2003, is the mainstay preventive approach to halt keratoconus progression. A systematic review has shown the efficacy and safety of CXL in keratoconus along with its biomechanical principles. 33 Although still widely adopted and used, the initial Dresden protocol has its setbacks owing to the unique physicochemical characteristics of the C-CXL reaction. A detailed account of the specific steps of the C-CXL reaction is out of the scope of this review, yet the mostly recognized and reported challenges with C-CXL, in general, include the rapid oxygen depletion particularly with higher irradiances, 34 limited riboflavin penetration through an intact epithelium, 35 the depth-dependent concentration gradient of riboflavin within the cornea, 36 prolonged treatment duration, and issues with potential endothelial toxicity in thin corneas. Some of the novel approaches to overcome these challenges and other emerging protocols along with alternative cross-linking methods are described below ( Figure 2 ).

A chart of advances in the treatment of keratoconus.
Approaches to increase oxygen availability
Oxygen plays a pivotal role in C-CXL largely because, in a low-oxygen-concentration environment, the reaction (type I reaction) not only is much less efficient with fewer cross-links being formed but also results in the generation of toxic hydrogen peroxide as the final byproduct. 37 , 38 In the presence of sufficient oxygen, type-II reaction ensues allowing a safer, more effective and controlled C-CXL. Rapid depletion of oxygen and hence related problems are even more pronounced at high irradiances in accelerated C-CXL protocols. A pulsed C-CXL approach with on and off cycles was recently conceptualized to allow recovery time for oxygen replenishment and hence increase its stromal availability. In a recent large prospective uncontrolled study conducted at the Moorfields Eye Hospital, Gore and colleagues 39 have reported keratometric stabilization in 98.3% of the 870 patients with an epi-off pulsed high-fluence protocol (30 mW/cm 2 for 4 minutes with 1.5 seconds on/off cycles) after 2 years of follow-up. In keeping with this finding, studies have shown deeper demarcation lines (a contested indicator of successful cross-linking) and more resistance to enzymatic digestion with pulsed protocols compared to continuous accelerated protocols. 40 , 41 However, it should be kept in mind that oxygen consumption occurs at a much faster pace than replenishment rendering short pulse intervals ineffective at complete oxygen restoration. 42
In addition to acting as a barrier for oxygen penetration, the corneal epithelium consumes much higher oxygen than the stroma further compounding oxygen availability issues in transepithelial approaches. This effect has been exemplified in a study by Sun and colleagues 43 who have shown rather limited efficacy with an epi-on pulsed light protocol (45 mW/cm 2 for 5 minutes and 20 seconds with a 1 second on/off cycle). In an ex vivo model of porcine eyes, Hill and colleagues 44 were able to demonstrate that aerobic conditions could be obtained by providing supplemental oxygen in an epi-on pulsed high-irradiance protocol, suggesting that optimization of oxygen may be beneficial in accelerated epi-on protocols. To test this clinically, Mazzotta and colleagues 45 have used oxygen delivery goggles to provide supplemental oxygen during an accelerated epi-on protocol and have reported meaningful improvements in visual acuity and corneal curvature with no significant adverse effects after 6 months of follow-up.
Approaches to increase riboflavin delivery
Riboflavin’s hydrophilicity and large molecular size make its passage difficult through an intact epithelium. Furthermore, although monophosphate isomers of riboflavin found in riboflavin formulations increase the solubility and require less energy to be photoactivated than riboflavin alone, they also increase the electronegativity acting as a repellant against the negatively charged proteoglycans in the corneal stroma. 35 This further decreases the penetration into the corneal stroma. To overcome this limitation, methods involving the transport of riboflavin by the use of iontophoresis had been tried over the recent years with comparable success to standard C-CXL. 46 , 47 However, these approaches were still hindered by the fact that longer exposures and hence longer treatment times were needed. Mazzotta and colleagues 48 have combined the pulsed high-fluence irradiance protocol with iontophoresis and have recently published the 3-year results of a shortened iontophoresis-assisted C-CXL technique, coined as the enhanced fluence pulsed light iontophoresis (EF I-CXL). This study showed satisfactory outcomes and similar demarcation line characteristics as with standard C-CXL. Iontophoresis procedure was split into two cycles by another group allowing time for riboflavin to penetrate the deeper stromal layers. 49 Iontophoresis has also been found useful in an ex vivo rabbit eye model in increasing stromal penetration depth of rose bengal, another cross-linking agent that can only diffuse through the 100 to 125 µm of the corneal stroma with conventional approaches.
Other approaches to increase riboflavin delivery have primarily aimed at chemically disrupting or loosening epithelial tight junctions, thereby increasing riboflavin permeability. Some of the tried molecules include diluted ethanol, benzalkonium chloride, trometamol, ethylenediaminetetraacetic acid, and vitamin E. 37 Sodium iodide is a novel excipient in the riboflavin formulation which safeguards riboflavin against UVA photodegradation into its inactive derivatives, theoretically avoiding the need for high stromal concentrations and frequent instillation. 50 – 52 It also stimulates the formation of oxygen from the byproduct of the type-I reaction (hydrogen peroxide) favoring the occurrence of type-II reaction over the former. 37 More recently, Aytekin and colleagues 53 harnessed nanostructured lipid carriers in designing a novel riboflavin delivery system coupled with additional molecules that either confer a positive charge (Stearylamine) or act as a permeation enhancer (Trancutol P). Another novel drug delivery method involved loading of riboflavin into a microemulsion system which showed favorable outcomes in terms of stromal diffusion and C-CXL efficiency in a rabbit eye model. 54 These systems however have not passed the scrutiny of a clinical trial and hence are only experimental.
Assessment of intrastromal riboflavin has also been evaluated as a means to personalize riboflavin delivery and optimize treatment outcomes. Techniques that were used in a laboratory environment included spectrophotometry, high-performance liquid chromatography, confocal fluorescence microscopy, and two-photon optical microscopy, all of which involve some degree of invasiveness and are not readily translatable into a clinical setting. 55 – 58 To fill in this lacuna, Lombardo and colleagues 59 have recently developed a noninvasive optical method for real-time assessment of intrastromal riboflavin concentration which has the potential to be useful in delivering personalized C-CXL treatments.
Thin corneas
Thin corneas pose a significant challenge whereby endothelial damage becomes inevitable with decreasing corneal thickness. Several solutions have been postulated to address this issue which includes administering hypoosmolar riboflavin, customized pachymetry-guided epithelial debridement, contact lens–assisted cross-linking, and accelerated high-fluence C-CXL. A more recent approach involves placing a stromal lenticule harvested with femtosecond laser during small incision lenticule extraction (SMILE) surgery for myopic correction or from a donor cornea onto the apex of the cone, thereby augmenting corneal thickness in this area. 60 , 61
Customized C-CXL
The co-utilization of imaging modalities with C-CXL has allowed the delivery of targeted cross-linking to specific areas of the cornea. Customized cross-linking (PiXL) has paved the way to tailor the treatment according to the individual patient’s needs by offering a dualistic action to effectively halt keratoconus progression and provide a refractive correction at the same time. This approach aims at delivering the maximum treatment at the apex of the cone, supposedly the weakest area, therefore allowing a more controlled flattening/steepening inside and outside the treatment area. This selective treatment induces refractive changes favoring normalization of corneal curvature. The precise location to treat can be determined using tomography-driven finite-element models, 62 maximum tomographic posterior float, 63 – 65 and thinnest pachymetry. 45 , 66 Such approaches, however, rely on the geometric surrogates of biomechanical weakening and hence not the cause but the effect. With the advances in spatially resolved in vivo biomechanical imaging of the cornea either with Brillouin spectroscopy 7 or optical coherence elastography, 67 biomechanics-guided C-CXL may be possible soon.
Emerging combined protocols
C-CXL addresses the progression of keratoconus and even with customized C-CXL, refractive correction that can be achieved is only limited. The impetus to simultaneously improve vision and halt disease progression has driven clinicians to find ways to safely combine refractive procedures with C-CXL. This approach has been coined the term CXL Plus, denoting that C-CXL is complemented with a refractive procedure, either by photorefractive keratectomy (PRK) or intracorneal ring segment implantation (ICRS). 68 The results of a recently published large-scale prospective nonrandomized study that compared C-CXL alone with simultaneous C-CXL + ICRS and simultaneous C-CXL + PRK suggests that C-CXL + ICRS may be more suited for patients with higher irregular astigmatism and worse visual acuity, whereas C-CXL + PRK may be more effective in patients who require correction of irregular astigmatism but have better visual acuity. 69
As is known, performing refractive surgery in an ectatic cornea comes with a cost of further deteriorating biomechanical instability. To counter this, strategies have been developed that minimize the volume of the tissue to be ablated without compromising the refractive outcome. In a pilot study, Yang and colleagues 70 have studied the effect of “minimized-volume ablation” with accelerated C-CXL on the Schwind AMARIS 750 excimer laser platform (Schwind eye-tech solutions GmbH, Kleinostheim, Germany) in patients with grade I–III keratoconus and have found favorable results both in terms of safety and efficacy in correcting mild refractive errors. Similarly, a protocol in the name of “Central Corneal Regularization (CCR)” on the iVis Suite customized excimer laser ablation treatment platform (iVis Technologies S. r. l., Taranto, Italy) was tried with keratoconus patients (Amsler-Krumeich stage I–II) and was shown to improve both uncorrected and corrected visual acuity. 71 , 72 A subsequent comparative study also showed that the combination of the CCR/PRK approach with C-CXL results in a more effective reduction in higher-order aberrations and Kmax than what can be achieved with C-CXL alone. 73 Kanellopoulos AJ modified the Athens protocol by incorporating topographically guided varied pattern C-CXL into the protocol thereby reducing the amount of tissue that has to be removed by PRK. 74 The main obstacle in combining PRK with C-CXL however is that the minimum corneal thickness after ablation must still fulfill the 400 µm criterion, making this approach less feasible for more advanced keratoconus cases. The Tel Aviv protocol is another protocol in which PRK and CXL are combined. 75 , 76 During this procedure, 50-µm laser ablation of the epithelium and anterior stroma is performed using the excimer laser. 75 , 76 While astigmatic correction is planned as 50% of manifest refractive astigmatism (on the same axis), spherical ablation is applied subsequently in the epithelium and anterior stroma, not exceeding a total ablation of 50 µm. 75 , 76 The Tel Aviv protocol was reportedly to halt progression of keratoconus without excessive thinning in the corneas, and improved visual acuity and astigmatism. 75 , 76 The combination of transepithelial PTK and CXL, defined as the Cretan protocol by Kymionis and colleagues, 77 , 78 yielded better visual and refractive results in keratoconus patients compared to mechanical epithelial debridement. This method is an alternative option for patients who cannot undergo PRK due to thin cornea.
ICRS becomes a better option in more irregular corneas and its combination with C-CXL has been well described; however, it is still debated as to which procedure should be performed first in combined protocols. In a recent meta-analysis, Hashemi and colleagues 79 reported that simultaneous ICRS with C-CXL provides the best outcomes compared to staged approaches. Triple procedures combining PRK + C-CXL and ICRS have also been described. 80
New molecules and strategies
Various photoactivated cross-linkers other than riboflavin include rose bengal, Eosin Y, and WST-D, all of which are excited by different spectra of light. 81 Among the previously reported chemical cross-linkers genipin, 82 glyceraldehyde, 83 glutaraldhyde, 84 Açaí Extract, 85 formaldehyde releasers, 86 decoron, 87 and nitroalcohols 88 have been explored for use in corneal or scleral cross-linking. These agents do not require exposure to irradiance to be activated, but none of them has been translated into clinical practice. More recently, newer molecules have been described one of which is the transglutaminase, an enzyme that was first isolated from Streptoverticillium sp and had found its use in the food and manufacturing industries. 89 This agent does not need photoactivation and was shown to effectively increase the stiffness of the cornea without causing any damage to the endothelium or keratocytes. 89
A new photoactivator system for riboflavin has been recently proposed under the name of nonlinear optical cross-linking (NLO CXL) that exploits femtosecond laser. 90 – 92 This technique has several hypothetical advantages over UVA cross-linking. In contrast to UVA cross-linking that uses a single photon, NLO CXL requires two-photons, statistically increasing the likelihood of riboflavin photoactivation and subsequent radical formation. 90 Furthermore, NLO CXL offers precise x–y–z dimensional control over the volume and depth of tissue that is to be subjected to femtosecond laser and hence cross-linking. 90 Femtosecond laser may also be used to micromachine channels on the epithelium which could improve stromal penetration of riboflavin. 90
While there have been significant modifications and updates in the CXL procedure with newer protocols, the Dresden CXL protocol remains the standard procedure for stabilizing keratoconus. As such, it is more accepted and preferred all over the world as being a safer and more effective method in the long term. 93 , 94
New keratoplasty procedures
The last two decades have seen a paradigm shift in keratoplasty moving from the insertion of full-thickness grafts to lamellar grafts. A lamellar approach not only decreases the likelihood of immune rejection but also offers less-induced astigmatism and better visual outcomes particularly in the case of endothelial transplants. Some of the novel corneal transplantation approaches in keratoconus have been detailed below.
Bowman layer transplantation
Keratoconus has been classically linked with Bowman membrane fragmentation early in the course of the disease. 95 Owing to Bowman membrane’s essential role in providing biomechanical support to maintain corneal shape, it was theorized that replacement of this tissue could halt further deterioration and maintain vision. 96 , 97 Bowman layer transplantation (BLT) has recently been gaining interest particularly in patients with advanced keratoconus where C-CXL and ICRS may not be possible either due to corneal thickness constraints or significant steepening ( Figure 3 ). Studies have shown that not only does it halt keratoconus progression, it also provides flattening with a 5-year progression/complication-free estimated survival rate of 84%. 98 No study to date has reported any immune rejection episode partly owing to the accellularity of the Bowman membrane. 97 To reduce the likelihood of microperforation during manual dissection, a femtosecond laser has been used for the creation of the stromal pocket. More recently, visualization of the dissection plane could be improved with the use of an intraoperative optical coherence tomography. 99 The graft localization in the classic technique is at the mid-stromal level; however, a modification that has recently been described involves the insertion of the graft as an onlay in the subepithelial area. 100 , 101 This procedure does not require pocket creation and its preliminary results are promising.

Bowman layer transplantation: (a) Bowman layer inlay: a graft is implanted intrastromally and (b) Bowman layer onlay: a graft is localized onto the Bowman layer or anterior stroma.
Additive keratoplasty
This newly defined approach comprises of femtosecond laser-assisted insertion of a corneal lamella (prepared from a donor cornea or harvested during a lenticule extraction procedure) into an ectatic cornea. The procedure aims to increase corneal thickness, provide flattening in the conic area, and increasing biomechanical stability. Advantages include reduced immune reaction, minimal invasiveness, and shorter surgery as opposed to DALK or full-thickness grafting. 102 In a preliminary study, Mastropasqua and colleagues 103 demonstrated the effect of lenticule addition keratoplasty in nonprogressive advanced stage keratoconus eyes with improvements in visual acuity, corneal curvature, and corneal thickness. Using a slightly different lenticule shape, Jin and colleagues 104 compared lenticule addition keratoplasty and penetrating keratoplasty in progressing eyes and have found that lenticule addition keratoplasty enabled better visual and biomechanical outcomes. The next step in this lacuna of research is to use tissue engineering methods to decellularize stromal lenticules so as to reduce or completely eliminate the likelihood of immune rejection. Results of a phase-1 study that evaluated the safety and efficacy of decellularized stromal lenticule implantation with or without autologous adipose-tissue-derived stem cells have been recently published. Although only modest improvements were noted within the time-frame of the study, the procedure was considered generally safe with a significant potential to be explored in further clinical trials. 105
Cellular therapies
Keratoconus is typically associated with keratocyte apoptosis partly due to rubbing associated repeated epithelial trauma, inflammation, and upregulation of degradative enzymes. 106 Restoration of the keratocyte population by means of cellular therapy is an interesting approach that has the potential to re-establish the anatomy and physiology of a keratoconic cornea. Preclinical studies have shown that human-derived mesenchymal stem cells have the potential to differentiate into adult keratocytes and also synthesize collagen when injected into the host rabbit cornea. 107 , 108 In a preliminary study, Alio and colleagues injected autologous adipose-derived adult stem cells into femtosecond laser formed corneal pockets of five patients with advanced keratoconus. Remarkably, the injected stem cells remained viable within the tissue after 6 months and acquired the ability to synthesize new collagen. 109 Although cellular/regenerative therapy for corneal diseases is an exciting field of research, caution should be exercised in generalizing these findings particularly in keratoconus, a corneal disease that may require rectifying degraded mechanical properties by volume replacement in addition to cellular restoration. 110
Future directions
Advances in diagnosis and management of keratoconus are evolving at an unprecedented pace. We owe this rapid development primarily to the realization that keratoconus is not a rare disease as it was once thought. From the diagnostics perspective, we will soon witness the implementation of spatially resolved in vivo corneal biomechanical evaluation that will complement conventional geometrical evaluation and pave the way for personalized therapies. As with all diseases, the focus should be on preventing rather than treating before visual deterioration becomes evident. An interesting single-arm clinical trial is currently ongoing testing the efficacy of oral riboflavin followed by sunlight exposure on keratometric stabilization and visual acuity after a follow-up of 6 months. 111 If this simple treatment proves effective, dietary modifications may become the first-line treatment in keratoconus. Tissue engineering and regenerative therapy in keratoconus is also an exciting field of research that offers the opportunity to address disease pathology at the cellular level. Last, but by no means least, efforts expended in patient education and in increasing public awareness is an integral part of the collective combat against this visually debilitating corneal disease. 112
Conflict of interest statement: The authors declared no potential conflicts of interest with respect to the research, authorship, and/or publication of this article.
Funding: The authors received no financial support for the research, authorship, and/or publication of this article.
Ethical statement: This article does not contain any studies with human participants or animals performed by any of the authors. The manuscript is limited to a review of literature about the advances in the diagnosis and treatment of keratoconus. Therefore, no approval from ethical review board is required.

Contributor Information
Eray Atalay, Department of Ophthalmology, Medical School, Eskişehir Osmangazi University, Meşelik Kampüsü, Odunpazarı, Eskişehir 26040, Turkey.
Onur Özalp, Department of Ophthalmology, Medical School, Eskişehir Osmangazi University, Eskişehir, Turkey.
Nilgün Yıldırım, Department of Ophthalmology, Medical School, Eskişehir Osmangazi University, Eskişehir, Turkey.
- Open access
- Published: 06 November 2023
New dawn for keratoconus treatment: potential strategies for corneal stromal regeneration
- Shengqian Dou 1 , 2 ,
- Xiaoxue Liu 1 , 3 , 4 ,
- Weiyun Shi 1 , 3 , 4 &
- Hua Gao ORCID: orcid.org/0000-0002-1326-6946 1 , 3 , 4
Stem Cell Research & Therapy volume 14 , Article number: 317 ( 2023 ) Cite this article
4537 Accesses
Metrics details
Keratoconus is a progressive, ectatic and blinding disorder of the cornea, characterized by thinning of corneal stroma. As a highly prevalent among adolescents, keratoconus has been a leading indication for corneal transplantation worldwide. However, the severe shortage of donor corneas is a global issue, and the traditional corneal transplantation surgeries may superinduce multiple complications, necessitating efforts to develop more effective strategies for keratoconus treatment. In this review, we summarized several strategies to promote corneal stromal regeneration or improve corneal stromal thickness, including cell-based therapies, biosynthetic alternatives for inducing corneal regeneration, minimally invasive intrastromal implantation and bioengineered tissues for implantation. These strategies provided more accessible but safer alternatives from various perspectives for keratoconus treatment, paving the way for arresting the keratoconus progression in its earlier stage. For the treatments of corneal ectatic diseases beyond keratoconus, these approaches will provide important references and widen the therapy options in a donor tissue-independent manner.
Keratoconus is a progressive corneal ectatic disorder characterized by thinning of corneal stroma and asymmetrical conical protrusion of the cornea, which can lead to visual impairment or even blindness [ 1 , 2 , 3 ]. Keratoconus is one of the leading indications for corneal transplantation surgery worldwide [ 4 , 5 ], with an incidence of 1/2000 in the general population and even higher among young adults [ 2 , 6 ]. Keratoconus is the result of complex genetic and environmental interactions [ 7 , 8 , 9 ]. The most severe stage of keratoconus manifests with excessive ectasia, scarring and thinning stroma, which significantly impairs the vision, and the only option left for patients is corneal transplantation [ 1 ]. However, the severe shortage of the donor corneas available for transplant represents a global burden of blindness, with one cornea available for every 70 recipients in waiting [ 10 ]. Besides, traditional corneal transplantation surgeries can cause various complications, such as the severed corneal nerve plexus, dry eye, glaucoma and tissue rejection. Due to the immune rejection and chronic corneal allograft dysfunction, the poor long-term graft survival rate after keratoplasty usually brings a huge burden on patients. For these reasons, intense research effort has focused on corneal stromal regeneration to increase the corneal thickness of patients with keratoconus, and multiple therapy paradigms have been explored as alternative treatment modalities to preserve and improve the vision [ 11 , 12 , 13 , 14 ]. In this review, the strategies for corneal stromal regeneration are summarized, highlighting potential approaches for keratoconus treatment.
Strategies for corneal stromal regeneration
Cell therapy for keratoconus treatment.
Currently, corneal collagen cross-linking and corneal transplant remain the most preferred or even the only option for keratoconus treatment. However, neither of these approaches can fundamentally solve the underlying issue of the disease. Approximately 80–85% of the corneal thickness is composed of the corneal stroma, in which collagen fibrils and extracellular matrix are tightly arranged [ 15 , 16 ]. Keratocyte loss and excessive degradation of collagen fiber by matrix metalloproteinases are the culprit of keratoconus pathogenesis [ 17 , 18 ]. Hence, replacing or reviving the corneal stromal cells might be an ideal and direct approach; therefore, cell-based therapies for corneal stromal regeneration during keratoconus treatment have emerged and gained great concern.
To date, various ideas and choices for cell therapy of keratoconus were developed (Fig. 1 , Table 1 ). Keratocytes in the cornea are derived from neural crest cells. The number of keratocytes are limited in vivo, but they can be cultured in vitro and supplied as reliable cell source for intrastromal injection [ 19 , 20 ]. Besides, keratocyte progenitor cells, the committed stem cell populations that maintain capacity to self-renewal and differentiation, are thought to be a potential option for keratoconus treatment. The transplantation of healthy keratocyte progenitor cells into keratoconus corneas would provide a novel treatment modality that may slow the progression of keratoconus [ 21 ]. Moreover, the corneal stromal stem cells, a rare cell population resident in the peripheral cornea and limbus, can be isolated by specific surface markers from limbal stromal tissues [ 22 , 23 , 24 , 25 ]. Du et al. injected the human corneal stromal stem cells into mice corneas and did not observe elicit immune rejection over an extended period of time, suggesting an opportunity to develop cell-based therapies for corneal stromal diseases [ 26 ].
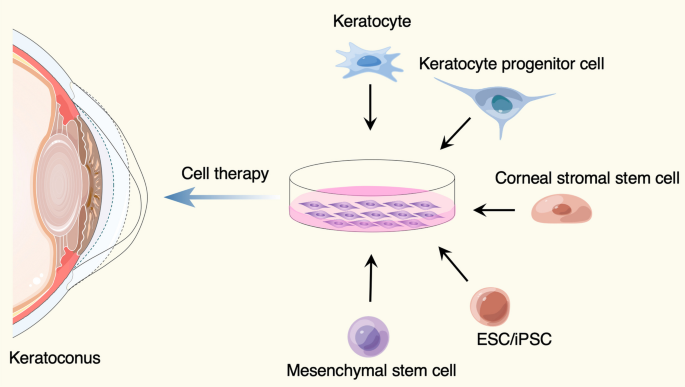
Cell sources used for keratoconus treatment. The figure was prepared by our group, and some of the elements in the diagram were provided by Figdraw ( http://www.figdraw.com )
However, several cell types mentioned above are still dependent on the corneal tissues, and the shortage of donor corneal tissues and the limited numbers of the particular cell populations is a significant challenge. The corneal stromal cells were found to have properties similar to other mesenchymal stem cells from various tissues [ 24 , 27 , 28 ], including adipose tissue [ 29 , 30 , 31 ], hematopoietic stem cells [ 32 ], dental pulp [ 33 , 34 ] and umbilical cord blood [ 35 ], which have been demonstrated to be used for keratoconus cell therapy [ 11 ]. For example, implantation of autologous adipose tissue-derived stem cells (ADSCs) into corneal stroma has been successfully tested for the treatment of keratoconus [ 30 , 31 , 36 , 37 ]. In addition, embryonic stem cells (ESCs) and induced pluripotent stem cells (iPSCs) also provide sufficient cell sources that could be differentiated to keratocytes required for keratoconus therapy [ 38 ].
Biosynthetic alternatives for inducing corneal regeneration
Replacement of the damaged tissue with corneal transplants is widely accepted treatment for corneal blindness. Over ten years ago, Per Fagerholm et al. developed a kind of recombinant human collagen type III (RHCIII), which has undergone synthesized in yeast, chemically cross-linked, and molded into a biosynthetic cornea mimic [ 39 ]. They conducted a phase 1 clinical study in which the biosynthetic cornea mimics were implanted to replace the distorted corneas of 10 patients with keratoconus or central scar. Strikingly, corneal re-epithelialization occurred in all patients, and nerve regeneration and touch sensitivity were also restored, demonstrating the property of the biosynthetic mimics in facilitating endogenous tissue regeneration. After then, further optimization of the biosynthetic corneal implants was done [ 40 , 41 ]. More significantly, Christopher D. McTiernan et al. developed a regeneration-stimulating liquid corneal replacement in a syringe that gels in situ, LiQD cornea, that comprises short collagen-like peptides, polyethylene glycol and fibrinogen [ 42 ]. The self-assembling synthetic collagen analog, as a low-cost and immune-compatible alternative, offering a safe and effective option to help address the current donor cornea shortage. Detailed information of these approaches is listed in Table 1 .
Mechanical methods to improve corneal stomal thickness
- Minimally invasive intrastromal implantation
Beside the strategy of corneal stromal cell replacement, restoring the physical properties of the corneal stroma cannot be ignored during keratoconus treatment [ 14 ]. Substantial biomechanical imbalance and weakening of the cornea can distinctly deteriorate the ocular surface homeostasis [ 43 , 44 ]. As we know, eye-rubbing is one of the major risk factors for keratoconus progressive, which can induce distinct alterations in corneal biomechanics [ 45 , 46 , 47 ]. And mechanical stretch was a trigger for keratoconus development and biomechanics-enzymes axis played a pathogenic role in keratoconus, as identified in our study [ 48 ]. Therefore, strengthening the biomechanical properties of the cornea should be considered during keratoconus treatment.
In recent years, corneal collagen cross-linking therapy, as a primary operative correction for progressive keratoconus, are used routinely to increase the biomechanical stability of the cornea. However, for keratoconus that has progressed to the most severe stage, corneal transplantation is the only option [ 1 ] (Table 2 ). Penetrating keratoplasty (PK) is a transplant procedure with full-thickness resection of the cornea, followed by grafting it with a full-thickness donor cornea, which was the treatment of choice for keratoconus until the late twentieth century [ 49 , 50 , 51 ]. When indicated, refinements in surgical approaches, like the deep anterior lamellar keratoplasty (DALK) [ 49 , 50 , 51 ] and anterior lamellar keratoplasty (ALK) [ 52 ], that were surgical procedures for removing part of the cornea. For instance, DALK involves replacement of the pathological corneal stroma down to the Descemet’s membrane but with the functional corneal endothelium retained, offers an effective alternative procedure that may lessen the risks including graft rejection and irregular astigmatism in PK. Despite DALK's success in restoring keratoconus patients' vision, there is still room for improvement regarding the operational complexity, restoring the physical properties of corneal stroma, preservation of the anterior corneal structure and nerve plexus, and suture-related complications. Therefore, suture-free implementation with smaller access cuts may be a preferred surgical option to arrest the progress of keratoconus, such as epikeratophakia (EP) [ 53 , 54 ], Bowman layer (BL) transplantation [ 55 , 56 ] and allogenic lenticule implantation [ 57 , 58 , 59 , 60 ]. Besides, our group have introduced a new effective procedure for the treatment of advanced keratoconus, named “femtosecond laser-assisted minimally invasive lamellar keratoplasty” (FL-MILK), in which partial thickness corneal stroma (stromal button) was implanted to the allogeneic corneal stroma through a small incision created by femtosecond laser (intrastromal pocket) [ 61 ] (as illustrated schematically in Fig. 2 ). Our study also indicated that FL-MILK can stabilize progressive KC in mild-to-moderate cases and advanced cases at 24-month follow-up with sustainable flattening effect of the anterior cornea curvature [ 62 ]. Indeed, while improving the stromal thickness, this minimally invasive surgical methods can maximally maintain the structural integrity and physical properties of the cornea, providing a feasible option for keratoconus treatment that should be put on the agenda.
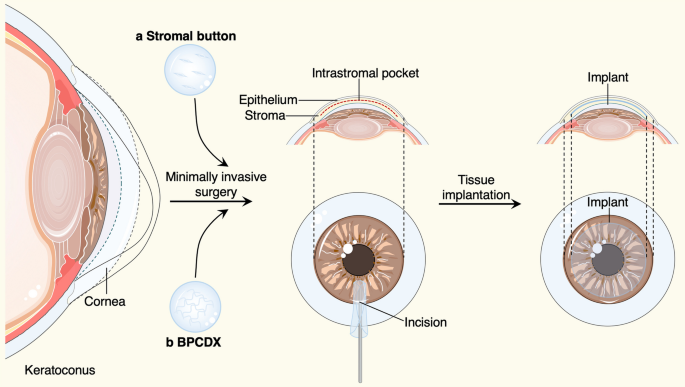
Minimally invasive surgical methods and bioengineered grafts for keratoconus treatment. An intrastromal pocket with a small incision were created by femtosecond laser, the human stromal button ( a ) or bioengineered BPCDX graft ( b ) were gently inserted into the intrastromal pocket to increase the corneal thickness. The figure was prepared by our group
Bioengineered corneal tissues for implantation
For the keratoplasty to treat keratoconus, several materials can be used as biomedical implants. The natural cornea has particular advantage in mechanical properties and structures, while the severe shortage of donor corneas presents a global concern. Hence, intense research efforts have focused on effective alternatives to conventional corneal grafts. May Grifith et al . successfully multilayered corneal equivalents constructed from immortalized cell lines [ 63 ]. Per Fagerholm et al . conducted a phase 1 clinical study in which biosynthetic mimics of corneal extracellular matrix were implanted to induce corneal regeneration [ 39 ]. Our group have developed a protective decellularization strategy for the preparation of decellularized porcine cornea (DPC), which achieved equivalent levels in numerous properties compared with that of human cornea grafts [ 64 ]. All these studies offered prospects for visual rehabilitation of corneal blindness. Even more to the point, Mehrdad Rafat and colleagues have described a cell-free engineered corneal tissue, which was derived from purified type I porcine collagen with dual chemical and photochemical cross-linking applied, termed the bioengineered porcine construct, double cross-linked (BPCDX) [ 65 ] (Fig. 2 ). The authors extracted and purified collagen from a by-product of the food industry, the porcine skin, providing an abundant yet sustainable and cost-effective supply of raw materials for implants. At the same time, likewise, the authors insert the implant within the corneal stroma through a minimally invasive procedure. Notably, after 2 years of follow-up, no adverse event was reported, all participants' vision improved to the same degree as with a standard donor tissue transplant. The strategy proposed by this work in which accessible bioengineered corneal tissues and minimally invasive surgical methods were elaborately combined, would be an attractive option for treatment of advanced keratoconus, especially in resource-limited settings. Details of the approaches mentioned in this part are listed in Table 2 .
In this review, we summarized several approaches to promote corneal stromal regeneration or improve corneal stromal thickness, including cell-based therapies, biosynthetic alternatives for inducing corneal regeneration, minimally invasive intrastromal implantation and bioengineered tissues for implantation. Among these, a series of mechanical methods to improve corneal stomal thickness have been applied in clinical treatment of keratoconus. For instance, historically, PK has been the gold standard approach for the surgical treatment of advanced keratoconus with its good visual outcomes [ 50 , 51 ]. However, DALK is increasingly becoming the preferred primary surgical option in contemporary practice owing to its reduced rejection and astigmatism in PK complications. But the complexity of operation and risks of suture-related complications in DALK complications cannot be ignored, which prompted the occurrence of minimally invasive surgical methods [ 49 , 50 , 51 ]. For example, FL-MILK can maximally maintain the structural integrity and physical properties of the cornea while improving the stromal thickness, and its more precise and quick recovery might make it an effective alternative for the treatment of advanced keratoconus [ 61 ]. Besides, combined more accessible bioengineered corneal tissues and minimally invasive methods would be an attractive option for keratoconus treatment. Indeed, longer follow-up period and more cases are needed for several new improving approaches.
In addition, the severe shortage of donor tissue impeded the treatment of keratoconus through corneal transplant surgery, especially in resource-limited settings. Therefore, explorations in developing strategies to promote corneal stromal regeneration has never stopped. The ideal cell-based therapy is expected to replace or revive the diseased keratocyte cells by inducing regeneration or by exogenous transplantation of keratocyte-committed cells. Here we listed the cell sources, stage of research, advantages and limitations for various cell-based therapeutic methods. Among these, implantation of autologous ADSCs into corneal stroma has been successfully tested for the treatment of keratoconus in clinical trials [ 30 , 31 , 36 , 37 ], with its abundant and easily accessible cell source. Besides, biosynthetic alternatives for inducing corneal regeneration, including RHCIII [ 39 ] and LiQD cornea [ 42 ], providing low-cost and immune-compatible alternatives to help address the donor cornea shortage.
Conclusions
Collectively, this review highlighted the advances in therapeutic strategies that can promote corneal stromal regeneration or improve corneal stromal thickness for keratoconus treatment, providing important reference and foundations for developing potential interventions. These approaches have brought hopes for keratoconus therapy with more safe and accessible alternative options, reducing the surgical complication and burden of limited donor corneas globally. Generally, DALK has become an alternative to PK, while minimally invasive surgery will become a major trend in the future treatment of keratoconus. And keratocyte regeneration therapies will also usher in a new era, especially for the ADSCs-based treatment, though the potential of several novel therapies for achieving effective stromal regeneration need further explorations. Certainly, further studies should be conducted to confirm the optimal therapeutic methods and conditions for keratoconus intervention, and novel approaches would be developed to control and arrest the progression of keratoconus in its much earlier stage, which might hopefully postpone or prevent an invasive corneal surgery. For keratoconus treatment, the light is shining brighter on its way.
Availability of data and materials
All datasets used in this study are available from the corresponding author on reasonable request.
Abbreviations
Adipose tissue-derived stem cells
Embryonic stem cells
Induced pluripotent stem cells
Penetrating keratoplasty
Deep anterior lamellar keratoplasty
Anterior lamellar keratoplasty
Epikeratophakia
Bowman layer
Femtosecond laser-assisted minimally invasive lamellar keratoplasty
Bioengineered porcine construct, double cross-linked
Recombinant human collagen type III
Gomes JA, Tan D, Rapuano CJ, Belin MW, Ambrosio R Jr, Guell JL, Malecaze F, Nishida K, Sangwan VS, Group of Panelists for the Global Delphi Panel of K, et al. Global consensus on keratoconus and ectatic diseases. Cornea. 2015;34(4):359–69.
Article PubMed Google Scholar
Kymes SM, Walline JJ, Zadnik K, Sterling J, Gordon MO. Collaborative longitudinal evaluation of keratoconus study g: changes in the quality-of-life of people with keratoconus. Am J Ophthalmol. 2008;145(4):611–7.
Article PubMed PubMed Central Google Scholar
Nichols JJ, Steger-May K, Edrington TB, Zadnik K. Group Cs: the relation between disease asymmetry and severity in keratoconus. Br J Ophthalmol. 2004;88(6):788–91.
Article CAS PubMed Google Scholar
Park CY, Lee JK, Gore PK, Lim CY, Chuck RS. Keratoplasty in the United States: a 10-year review from 2005 through 2014. Ophthalmology. 2015;122(12):2432–42.
Article Google Scholar
Gao H, Huang T, Pan Z, Wu J, Xu J, Hong J, Chen W, Wu H, Kang Q, Zhu L, et al. Survey report on keratoplasty in China: a 5-year review from 2014 to 2018. PLoS ONE. 2020;15(10): e0239939.
Article CAS PubMed PubMed Central Google Scholar
Jian W, Shen Y, Chen Y, Tian M, Zhou X. Ocular dimensions of the Chinese adolescents with keratoconus. BMC Ophthalmol. 2018;18(1):43.
Rabinowitz YS. Keratoconus. Surv Ophthalmol. 1998;42(4):297–319.
Davidson AE, Hayes S, Hardcastle AJ, Tuft SJ. The pathogenesis of keratoconus. Eye (Lond). 2014;28(2):189–95.
Hao XD, Chen XN, Zhang YY, Chen P, Wei C, Shi WY, Gao H. Multi-level consistent changes of the ECM pathway identified in a typical keratoconus twin’s family by multi-omics analysis. Orphanet J Rare Dis. 2020;15(1):227.
Gain P, Jullienne R, He Z, Aldossary M, Acquart S, Cognasse F, Thuret G. Global survey of corneal transplantation and eye banking. JAMA Ophthalmol. 2016;134(2):167–73.
Shetty R, Mahendran K, Joshi PD, Jeyabalan N, Jayadev C, Das D. Corneal stromal regeneration-keratoconus cell therapy: a review. Graefes Arch Clin Exp Ophthalmol. 2023.
Lagali N. Corneal stromal regeneration: current status and future therapeutic potential. Curr Eye Res. 2020;45(3):278–90.
Article CAS Google Scholar
El Zarif M, Alio JL, Alio Del Barrio JL, De Miguel MP, Abdul Jawad K, Makdissy N. Corneal stromal regeneration: a review of human clinical studies in keratoconus treatment. Front Med (Lausanne). 2021;8: 650724.
Brunette I, Roberts CJ, Vidal F, Harissi-Dagher M, Lachaine J, Sheardown H, Durr GM, Proulx S, Griffith M. Alternatives to eye bank native tissue for corneal stromal replacement. Prog Retin Eye Res. 2017;59:97–130.
Matthyssen S, Van den Bogerd B, Dhubhghaill SN, Koppen C, Zakaria N. Corneal regeneration: a review of stromal replacements. Acta Biomater. 2018;69:31–41.
Song P, Wang S, Zhang P, Sui W, Zhang Y, Liu T, Gao H. The superficial stromal scar formation mechanism in keratoconus: a study using laser scanning in vivo confocal microscopy. Biomed Res Int. 2016;2016:7092938.
Fini ME, Yue BY, Sugar J. Collagenolytic/gelatinolytic metalloproteinases in normal and keratoconus corneas. Curr Eye Res. 1992;11(9):849–62.
Kao WW, Vergnes JP, Ebert J, Sundar-Raj CV, Brown SI. Increased collagenase and gelatinase activities in keratoconus. Biochem Biophys Res Commun. 1982;107(3):929–36.
Jester JV, Jin HC. Modulation of cultured corneal keratocyte phenotype by growth factors/cytokines control in vitro contractility and extracellular matrix contraction. Exp Eye Res. 2003;77(5):581–92.
Yam GHF, Fuest M, Yusoff NZBM, Goh TW, Bandeira F, Setiawan M, Seah XY, Lwin NC, Stanzel TP, Ong HS, et al. Safety and feasibility of intrastromal injection of cultivated human corneal stromal keratocytes as cell-based therapy for corneal opacities. Invest Ophth Vis Sci. 2018;59(8):3340–54.
Patel DV, McKelvie J, Sherwin T, McGhee C. Keratocyte progenitor cell transplantation: a novel therapeutic strategy for corneal disease. Med Hypotheses. 2013;80(2):122–4.
Nagymihaly RM, Moe MC, Petrovski G. Isolation and culture of corneal stromal stem cells. Methods Mol Biol. 2020;2145:1–15.
Kureshi AK, Funderburgh JL, Daniels JT. Human corneal stromal stem cells exhibit survival capacity following isolation from stored organ-culture corneas. Invest Ophthalmol Vis Sci. 2014;55(11):7583–8.
Article CAS PubMed Central Google Scholar
Pinnamaneni N, Funderburgh JL. Concise review: Stem cells in the corneal stroma. Stem Cells. 2012;30(6):1059–63.
Basu S, Hertsenberg AJ, Funderburgh ML, Burrow MK, Mann MM, Du Y, Lathrop KL, Syed-Picard FN, Adams SM, Birk DE, et al. Human limbal biopsy-derived stromal stem cells prevent corneal scarring. Sci Transl Med. 2014;6(266):266–172.
Du Y, Carlson EC, Funderburgh ML, Birk DE, Pearlman E, Guo N, Kao WW, Funderburgh JL. Stem cell therapy restores transparency to defective murine corneas. Stem Cells. 2009;27(7):1635–42.
Vereb Z, Poliska S, Albert R, Olstad OK, Boratko A, Csortos C, Moe MC, Facsko A, Petrovski G. Role of human corneal stroma-derived mesenchymal-like stem cells in corneal immunity and wound healing. Sci Rep. 2016;6:26227.
Branch MJ, Hashmani K, Dhillon P, Jones DR, Dua HS, Hopkinson A. Mesenchymal stem cells in the human corneal limbal stroma. Invest Ophthalmol Vis Sci. 2012;53(9):5109–16.
El Zarif M, Alio JL, Alio Del Barrio JL, Abdul Jawad K, Palazon-Bru A, Abdul Jawad Z, De Miguel MP, Makdissy N. Corneal stromal regeneration therapy for advanced keratoconus: long-term outcomes at 3 years. Cornea. 2021;40(6):741–54.
Alio Del Barrio JL, El Zarif M, de Miguel MP, Azaar A, Makdissy N, Harb W, El Achkar I, Arnalich-Montiel F, Alio JL. Cellular therapy with human autologous adipose-derived adult stem cells for advanced keratoconus. Cornea. 2017;36(8):952–60.
Donthineni PR, Bagga B, Singh V, Shukla S. Cellular therapy with human autologous adipose-derived adult stem cells for advanced keratoconus. Cornea. 2017;36(12):e36–7.
Liu H, Zhang J, Liu CY, Hayashi Y, Kao WW. Bone marrow mesenchymal stem cells can differentiate and assume corneal keratocyte phenotype. J Cell Mol Med. 2012;16(5):1114–24.
Mansoor H, Ong HS, Riau AK, Stanzel TP, Mehta JS, Yam GH. Current trends and future perspective of mesenchymal stem cells and exosomes in corneal diseases. Int J Mol Sci 2019;20(12).
Syed-Picard FN, Du Y, Lathrop KL, Mann MM, Funderburgh ML, Funderburgh JL. Dental pulp stem cells: a new cellular resource for corneal stromal regeneration. Stem Cells Transl Med. 2015;4(3):276–85.
Liu H, Zhang J, Liu CY, Wang IJ, Sieber M, Chang J, Jester JV, Kao WW. Cell therapy of congenital corneal diseases with umbilical mesenchymal stem cells: lumican null mice. PLoS ONE. 2010;5(5): e10707.
Article PubMed Central Google Scholar
Arnalich-Montiel F, Pastor S, Blazquez-Martinez A, Fernandez-Delgado J, Nistal M, Alio JL, De Miguel MP. Adipose-derived stem cells are a source for cell therapy of the corneal stroma. Stem Cells. 2008;26(2):570–9.
Ramin S, Abbasi A, Ahadi M, Moallemi Rad L, Kobarfad F. Assessment of the effects of intrastromal injection of adipose-derived stem cells in keratoconus patients. Int J Ophthalmol. 2023;16(6):863–70.
Joseph R, Srivastava OP, Pfister RR. Modeling keratoconus using induced pluripotent stem cells. Invest Ophthalmol Vis Sci. 2016;57(8):3685–97.
Fagerholm P, Lagali NS, Merrett K, Jackson WB, Munger R, Liu Y, Polarek JW, Soderqvist M, Griffith M. A biosynthetic alternative to human donor tissue for inducing corneal regeneration: 24-month follow-up of a phase 1 clinical study. Sci Transl Med. 2010;2(46):46–61.
Fagerholm P, Lagali NS, Ong JA, Merrett K, Jackson WB, Polarek JW, Suuronen EJ, Liu Y, Brunette I, Griffith M. Stable corneal regeneration four years after implantation of a cell-free recombinant human collagen scaffold. Biomaterials. 2014;35(8):2420–7.
Jangamreddy JR, Haagdorens MKC, Islam MM, Lewis P, Samanta A, Fagerholm P, Liszka A, Ljunggren MK, Buznyk O, Alarcon EI, et al. Short peptide analogs as alternatives to collagen in pro-regenerative corneal implants. Acta Biomater. 2018;69:120–30.
McTiernan CD, Simpson FC, Haagdorens M, Samarawickrama C, Hunter D, Buznyk O, Fagerholm P, Ljunggren MK, Lewis P, Pintelon I et al. LiQD cornea: pro-regeneration collagen mimetics as patches and alternatives to corneal transplantation. Sci Adv. 2020;6(25).
Vinciguerra R, Herber R, Wang Y, Zhang F, Zhou X, Bai J, Yu K, Chen S, Fang X, Raiskup F, et al. Corneal biomechanics differences between Chinese and Caucasian healthy subjects. Front Med (Lausanne). 2022;9: 834663.
Zhang X, Ding L, Sun L, Huang Y, Han T, Qian Y, Zhou X. Prognostic nomograms predicting risk of keratoconus in very asymmetric ectasia: combined corneal tomographic and biomechanical assessments. Front Bioeng Biotechnol. 2022;10: 839545.
Sahebjada S, Al-Mahrouqi HH, Moshegov S, Panchatcharam SM, Chan E, Daniell M, Baird PN. Eye rubbing in the aetiology of keratoconus: a systematic review and meta-analysis. Graefes Arch Clin Exp Ophthalmol. 2021;259(8):2057–67.
Bawazeer AM, Hodge WG, Lorimer B. Atopy and keratoconus: a multivariate analysis. Br J Ophthalmol. 2000;84(8):834–6.
Yang KL, Xu LY, Fan Q, Ren SW: Association between corneal stiffness parameter at the first applanation and keratoconus severity. J Ophthalmol. 2020;2020.
Dou S, Wang Q, Zhang B, Wei C, Wang H, Liu T, Duan H, Jiang H, Liu M, Qi X, et al. Single-cell atlas of keratoconus corneas revealed aberrant transcriptional signatures and implicated mechanical stretch as a trigger for keratoconus pathogenesis. Cell Discov. 2022;8(1):66.
Borderie VM, Sandali O, Bullet J, Gaujoux T, Touzeau O, Laroche L. Long-term results of deep anterior lamellar versus penetrating keratoplasty. Ophthalmology. 2012;119(2):249–55.
Yoshida J, Toyono T, Shirakawa R, Miyai T, Usui T. Risk factors and evaluation of keratoconus progression after penetrating keratoplasty with anterior segment optical coherence tomography. Sci Rep. 2020;10(1):18594.
Feizi S, Javadi MA, Karimian F, Bayat K, Bineshfar N, Esfandiari H. Penetrating keratoplasty versus deep anterior lamellar keratoplasty for advanced stage of keratoconus. Am J Ophthalmol. 2023;248:107–15.
Patil M, Mehta JS. Lamellar keratoplasty for advanced keratoconus. Asia Pac J Ophthalmol (Phila). 2020;9(6):580–8.
Krumeich JH, Daniel J, Knulle A. Live-epikeratophakia for keratoconus. J Cataract Refract Surg. 1998;24(4):456–63.
Krumeich JH, Daniel J. Live epikeratophakia and deep lamellar keratoplasty for I-III stage-specific surgical treatment of keratoconus. Klin Monbl Augenheilkd. 1997;211(2):94–100.
Dragnea DC, Birbal RS, Ham L, Dapena I, Oellerich S, van Dijk K, Melles GRJ. Bowman layer transplantation in the treatment of keratoconus. Eye Vis (Lond). 2018;5:24.
van der Star L, van Dijk K, Vasiliauskaite I, Dapena I, Oellerich S, Melles GRJ. Long-term outcomes of bowman layer inlay transplantation for the treatment of progressive keratoconus. Cornea. 2022;41(9):1150–7.
Li M, Zhao F, Li M, Knorz MC, Zhou X. Treatment of corneal ectasia by implantation of an allogenic corneal lenticule. J Refract Surg. 2018;34(5):347–50.
Ganesh S, Brar S. Femtosecond intrastromal lenticular implantation combined with accelerated collagen cross-linking for the treatment of keratoconus-initial clinical result in 6 eyes. Cornea. 2015;34(10):1331–9.
Jin H, He M, Liu H, Zhong X, Wu J, Liu L, Ding H, Zhang C, Zhong X. Small-incision femtosecond laser-assisted intracorneal concave lenticule implantation in patients with keratoconus. Cornea. 2019;38(4):446–53.
Wei Q, Ding H, Nie K, Jin H, Zhong T, Yu H, Yang Z, Hu S, He L, Zhong X. Long-term clinical outcomes of small-incision femtosecond laser-assisted intracorneal concave lenticule implantation in patients with keratoconus. J Ophthalmol. 2022;2022:9774448.
Gao H, Liu M, Li N, Chen T, Qi X, Xie L, Shi W. Femtosecond laser-assisted minimally invasive lamellar keratoplasty for the treatment of advanced keratoconus. Clin Exp Ophthalmol. 2022;50(3):294–302.
Li N, Liu M, Tian G, Chen T, Lin Y, Qi X, Shi W, Gao H. Effects of femtosecond laser-assisted minimally invasive lamellar keratoplasty (FL-MILK) on mild-to-moderate and advanced keratoconus. Graefes Arch Clin Exp Ophthalmol. 2023;
Griffith M, Osborne R, Munger R, Xiong X, Doillon CJ, Laycock NL, Hakim M, Song Y, Watsky MA. Functional human corneal equivalents constructed from cell lines. Science. 1999;286(5447):2169–72.
Shi W, Zhou Q, Gao H, Li S, Dong M, Wang T, Jia Y, Dong C, Wang X, Guo Z, et al. Protectively decellularized porcine cornea versus human donor cornea for lamellar transplantation. Adv Funct Mater. 2019;29(37).
Rafat M, Jabbarvand M, Sharma N, Xeroudaki M, Tabe S, Omrani R, Thangavelu M, Mukwaya A, Fagerholm P, Lennikov A, et al. Bioengineered corneal tissue for minimally invasive vision restoration in advanced keratoconus in two clinical cohorts. Nat Biotechnol. 2022;
Download references
Acknowledgements
We thank Tong Liu for constructive suggestions on this review. Some of the elements in the diagram were provided by Figdraw ( http://www.figdraw.com )
This work was supported by the National Natural Science Foundation of China (82070923, 82101092, 82371032), Taishan Scholar Program (201812150, 202306390), Major Basic Research Project of Natural Science Foundation of Shandong Province (ZR2023ZD60) and the Academic Promotion Program and Innovation Project of Shandong First Medical University (2019RC009).
Author information
Authors and affiliations.
State Key Laboratory Cultivation Base, Shandong Provincial Key Laboratory of Ophthalmology, Eye Institute of Shandong First Medical University, Qingdao, China
Shengqian Dou, Xiaoxue Liu, Weiyun Shi & Hua Gao
Qingdao Eye Hospital of Shandong First Medical University, Qingdao, China
Shengqian Dou
Eye Hospital of Shandong First Medical University, Jinan, China
Xiaoxue Liu, Weiyun Shi & Hua Gao
School of Ophthalmology, Shandong First Medical University, Jinan, China
You can also search for this author in PubMed Google Scholar
Contributions
H.G. conceptualized, acquired funding and supervised this study. S.D. drafted the manuscript. X.L. performed literature search and collection. W.S. provided advice and discussed the manuscript.
Corresponding author
Correspondence to Hua Gao .
Ethics declarations
Ethical approval and consent to participate.
Not applicable.
Consent for publication
Competing interests.
The authors declare no competing interests.
Additional information
Publisher's note.
Springer Nature remains neutral with regard to jurisdictional claims in published maps and institutional affiliations.
Rights and permissions
Open Access This article is licensed under a Creative Commons Attribution 4.0 International License, which permits use, sharing, adaptation, distribution and reproduction in any medium or format, as long as you give appropriate credit to the original author(s) and the source, provide a link to the Creative Commons licence, and indicate if changes were made. The images or other third party material in this article are included in the article's Creative Commons licence, unless indicated otherwise in a credit line to the material. If material is not included in the article's Creative Commons licence and your intended use is not permitted by statutory regulation or exceeds the permitted use, you will need to obtain permission directly from the copyright holder. To view a copy of this licence, visit http://creativecommons.org/licenses/by/4.0/ . The Creative Commons Public Domain Dedication waiver ( http://creativecommons.org/publicdomain/zero/1.0/ ) applies to the data made available in this article, unless otherwise stated in a credit line to the data.
Reprints and permissions
About this article
Cite this article.
Dou, S., Liu, X., Shi, W. et al. New dawn for keratoconus treatment: potential strategies for corneal stromal regeneration. Stem Cell Res Ther 14 , 317 (2023). https://doi.org/10.1186/s13287-023-03548-5
Download citation
Received : 02 August 2023
Accepted : 27 October 2023
Published : 06 November 2023
DOI : https://doi.org/10.1186/s13287-023-03548-5
Share this article
Anyone you share the following link with will be able to read this content:
Sorry, a shareable link is not currently available for this article.
Provided by the Springer Nature SharedIt content-sharing initiative
- Keratoconus treatment
- Corneal stromal regeneration
- Cell therapy
- Biosynthetic alternatives
- Bioengineered tissues
Stem Cell Research & Therapy
ISSN: 1757-6512
- Submission enquiries: Access here and click Contact Us
- General enquiries: [email protected]
Thank you for visiting nature.com. You are using a browser version with limited support for CSS. To obtain the best experience, we recommend you use a more up to date browser (or turn off compatibility mode in Internet Explorer). In the meantime, to ensure continued support, we are displaying the site without styles and JavaScript.
- View all journals
- My Account Login
- Explore content
- About the journal
- Publish with us
- Sign up for alerts
- Open access
- Published: 01 March 2021
A multi-ethnic genome-wide association study implicates collagen matrix integrity and cell differentiation pathways in keratoconus
- Alison J. Hardcastle ORCID: orcid.org/0000-0002-0038-6770 1 , 2 na1 ,
- Petra Liskova ORCID: orcid.org/0000-0001-7834-8486 1 , 3 , 4 na2 ,
- Yelena Bykhovskaya 5 , 6 na2 ,
- Bennet J. McComish ORCID: orcid.org/0000-0003-1940-0007 7 na2 ,
- Alice E. Davidson ORCID: orcid.org/0000-0002-1816-6151 1 na2 ,
- Chris F. Inglehearn ORCID: orcid.org/0000-0002-5143-2562 8 na2 ,
- Xiaohui Li ORCID: orcid.org/0000-0002-5037-3572 9 ,
- Hélène Choquet ORCID: orcid.org/0000-0001-9839-8667 10 ,
- Mahmoud Habeeb 11 , 12 ,
- Sionne E. M. Lucas 7 ,
- Srujana Sahebjada ORCID: orcid.org/0000-0002-1945-7209 13 , 14 ,
- Nikolas Pontikos ORCID: orcid.org/0000-0003-1782-4711 1 ,
- Karla E. Rojas Lopez 1 ,
- Anthony P. Khawaja ORCID: orcid.org/0000-0001-6802-8585 1 , 2 , 15 ,
- Manir Ali ORCID: orcid.org/0000-0003-3204-3788 8 ,
- Lubica Dudakova 3 ,
- Pavlina Skalicka 4 ,
- Bart T. H. Van Dooren 11 , 16 ,
- Annette J. M. Geerards 17 ,
- Christoph W. Haudum ORCID: orcid.org/0000-0002-3872-5643 18 ,
- Valeria Lo Faro ORCID: orcid.org/0000-0003-4931-7327 19 , 20 ,
- Abi Tenen 21 , 22 , 23 ,
- Mark J. Simcoe ORCID: orcid.org/0000-0003-2432-0810 24 , 25 ,
- Karina Patasova 24 , 25 ,
- Darioush Yarrand ORCID: orcid.org/0000-0003-3570-3921 25 ,
- Jie Yin 10 ,
- Salina Siddiqui 8 , 26 ,
- Aine Rice 8 ,
- Layal Abi Farraj ORCID: orcid.org/0000-0003-1484-192X 8 ,
- Yii-Der Ida Chen 9 ,
- Jugnoo S. Rahi 27 ,
- Ronald M. Krauss 28 ,
- Elisabeth Theusch 28 ,
- Jac C. Charlesworth 7 ,
- Loretta Szczotka-Flynn 29 ,
- Carmel Toomes 8 ,
- Magda A. Meester-Smoor 11 , 12 ,
- Andrea J. Richardson 13 ,
- Paul A. Mitchell 30 ,
- Kent D. Taylor ORCID: orcid.org/0000-0002-2756-4370 9 ,
- Ronald B. Melles 10 ,
- Anthony J. Aldave 31 ,
- Richard A. Mills 32 ,
- Ke Cao 13 , 14 ,
- Elsie Chan 13 , 14 ,
- Mark D. Daniell 13 , 14 ,
- Jie Jin Wang ORCID: orcid.org/0000-0001-9491-4898 33 ,
- Jerome I. Rotter ORCID: orcid.org/0000-0001-7191-1723 9 ,
- Alex W. Hewitt ORCID: orcid.org/0000-0002-5123-5999 7 , 21 , 22 , 23 ,
- Stuart MacGregor ORCID: orcid.org/0000-0001-6731-8142 34 ,
- Caroline C. W. Klaver ORCID: orcid.org/0000-0002-2355-5258 11 , 12 ,
- Wishal D. Ramdas ORCID: orcid.org/0000-0002-1780-5281 11 ,
- Jamie E. Craig 7 , 32 ,
- Sudha K. Iyengar 29 ,
- David O’Brart 24 , 35 ,
- Eric Jorgenson ORCID: orcid.org/0000-0002-5829-8191 10 na1 ,
- Paul N. Baird ORCID: orcid.org/0000-0002-1305-3502 14 na1 ,
- Yaron S. Rabinowitz 5 , 6 na1 ,
- Kathryn P. Burdon ORCID: orcid.org/0000-0001-8217-1249 7 , 32 na1 ,
- Chris J. Hammond ORCID: orcid.org/0000-0002-3227-2620 24 , 25 , 35 na1 ,
- Stephen J. Tuft ORCID: orcid.org/0000-0001-8192-5192 1 , 2 na1 &
- Pirro G. Hysi ORCID: orcid.org/0000-0001-5752-2510 24 , 25 , 27 na1
Communications Biology volume 4 , Article number: 266 ( 2021 ) Cite this article
7543 Accesses
39 Citations
62 Altmetric
Metrics details
- Corneal diseases
Genome-wide association studies
Keratoconus is characterised by reduced rigidity of the cornea with distortion and focal thinning that causes blurred vision, however, the pathogenetic mechanisms are unknown. It can lead to severe visual morbidity in children and young adults and is a common indication for corneal transplantation worldwide. Here we report the first large scale genome-wide association study of keratoconus including 4,669 cases and 116,547 controls. We have identified significant association with 36 genomic loci that, for the first time, implicate both dysregulation of corneal collagen matrix integrity and cell differentiation pathways as primary disease-causing mechanisms. The results also suggest pleiotropy, with some disease mechanisms shared with other corneal diseases, such as Fuchs endothelial corneal dystrophy. The common variants associated with keratoconus explain 12.5% of the genetic variance, which shows potential for the future development of a diagnostic test to detect susceptibility to disease.
Similar content being viewed by others
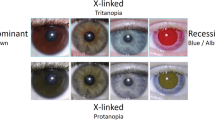
What colour are your eyes? Teaching the genetics of eye colour & colour vision. Edridge Green Lecture RCOphth Annual Congress Glasgow May 2019
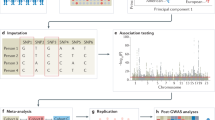
Identifying proteomic risk factors for cancer using prospective and exome analyses of 1463 circulating proteins and risk of 19 cancers in the UK Biobank
Introduction.
Keratoconus is a leading cause for visual impairment in adolescents and young adults which, untreated, can lead to legal blindness 1 , 2 , 3 , 4 , 5 , 6 , 7 . The prevalence of keratoconus varies between ethnic groups, with figures as high as 1.2% reported in some predominantly European populations 8 , to 2.3–3.3% in Maori or Iranian populations 9 , 10 . A high occurrence rate in first degree relatives, and concordance in twins, suggest that keratoconus has a strong genetic component 11 , 12 . Keratoconus can also be a comorbidity of other genetically determined conditions such as Down syndrome 13 . Several loci and variants for keratoconus have been identified through linkage studies and genome-wide association studies (GWASs) for central corneal thickness (CCT) 14 , 15 , 16 , 17 , 18 , 19 , 20 . However, although CCT is highly heritable, it is a stable characteristic, in contrast to the acquired and progressive corneal thinning that is a feature of keratoconus. Previous studies have also implicated single nucleotide polymorphism (SNP) alleles upstream of the ZNF469 locus that is associated with a higher CCT but an increased risk for keratoconus 16 , 20 , 21 . Therefore, alternative mechanisms, in addition to those influencing CCT, are likely to be involved. This incomplete knowledge of the genetic predisposition for keratoconus limits our understanding of the mechanisms that drive this disease. In this study we present the largest GWAS for keratoconus performed to date for 4669 cases and 116,547 controls.
Meta-analyses of genome-wide associations with keratoconus
We performed the analyses in three stages (Fig. 1 ). First, a discovery analysis was conducted in 2116 cases and 24,626 controls of European ancestry. For the second stage, we compared and replicated the discovery results in a meta-analysis of three independent European cohorts (1389 cases and 79,727 controls), and in a separate meta-analysis of two non-European cohorts (759 South Asian cases and 8009 controls, and 405 African cases and 4185 controls). Finally, we combined the discovery and replication cohorts in an overall meta-analysis. Genomic control factors 22 were consistent with polygenicity expectations and the absence of uncontrolled population structure in any of the components of this study (Supplementary Data 1 ).
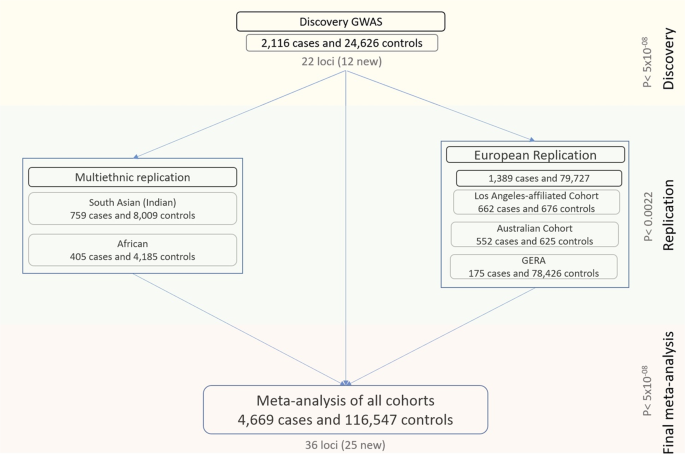
There were three main phases: a discovery in a European cohort of 2116 cases and 24,626 controls, a replication in a combined meta-analysis of three other European cohorts as well as in two smaller non-European cohorts, and a final meta-analysis involving all the multi-ethnic cases and controls from the previous stages.
The discovery analysis identified 22 GWAS-significant associations (Supplementary Data 2 ), including six loci previously associated with keratoconus ( FOXO1 17 , COL5A1 17 , FNDC3B 17 , ZNF469 17 , LOX 23 and near PNPLA2 24 ), four that were previously associated with CCT 16 , 18 but not keratoconus, and 12 entirely novel loci. Among the novel keratoconus loci, the most significant association was found at a gene-poor region on chr21q2 ( p = 1.34 × 10 −13 for rs76747345).
At the replication stage, we carried forward the most significant SNPs within each of their regions of association, or other GWAS-significant proxy SNPs if necessary, whenever the index SNPs were missing in the replication data. Nine of the 22 regions (of which 2 are novel) replicated after Bonferroni multiple testing correction ( p < 0.05/22 = 0.0022) and another four (of which 2 are novel) at FDR < 0.05 (Supplementary Data 2 ). All SNPs except three, for which the replication meta-analysis had insufficient power, showed directional consistency (Supplementary Fig. 1 ). We also observed associations that were highly directionally concordant in non-European samples (Fig. 2 ). Despite the lower statistical power, there was good replication in the South Asian samples (12 SNPs nominally significant, of which 8 remained significant after correction for multiple testing), but slightly less so in Africans (Supplementary Data 3 ).
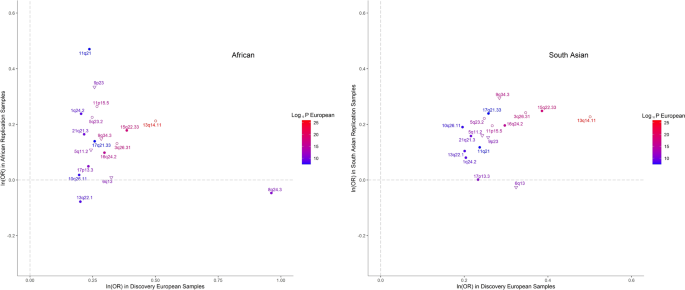
Each dot represents SNPs shown in Supplementary Data 3 and their labels are the respective chromosomal band on which they are located. The shapes of each data point refer to a previous GWAS association with keratoconus (empty circles), with CCT only (empty triangles) or novel associations (solidly filled circular shapes). The colors of both the points and their labels represent the significance (−log10( p -value) of association observed in the European discovery cohort. Polymorphisms identified in the discovery cohort but not shown here were not available for analysis in the replication cohorts.
The final meta-analysis combined data for all 4669 cases and 116,547 controls. Although the genomic inflation factor was nominally large (λ = 1.29), a further LD score regression analysis 25 (on European samples only) suggests that these results were in line with expectations of polygenicity (ldsc intercept = 1.09, SE = 0.009). We continue to observe homogeneous effect sizes across all populations (Supplementary Data 4 ). This meta-analysis yielded significant associations clustering around 36 independent regions (Fig. 3 , Table 1 ), of which 31 are reported for the first time, including six previously associated with CCT but not specifically with keratoconus at GWAS significance.
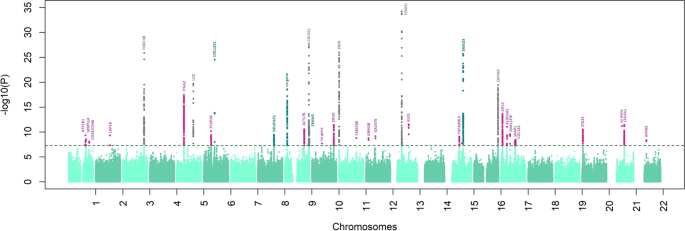
Analyses were conducted in 4669 cases and 116,547 controls. The log10( p -value) from the final meta-analysis is shown on the y-axis for all the SNPs along the different autosomes (x-axis). Novel associations for keratoconus are in pink. The names of the coding genes nearest to the most significantly associated SNPs are shown, or “no gene” when the association was >250 kb from a coding gene. Different colors are used for genetic loci and genes that to our knowledge, were previously associated with CCT (dark green) keratoconus (dark gray).
Strong associations were found near or within genes that code for fibrillar collagens (types I and V), microfibrillar (VI) and peri-fibrillar (XII) structures 26 , implicating impaired cohesion of the collagen matrix in the pathogenesis of keratoconus. Association was also found for rs35523808 (p.Glu2160Val, p = 2.90 × 10 −25 ), a missense and potentially deleterious variant within COL12A1 (Table 1 , Supplementary Data 5 ). Collagen XII is localized in Bowman layer and the interfibrillar matrix of the corneal stroma, where it regulates the organization and mechanical properties of collagen fibrils 27 . We found significant association within the COL6A1 gene (rs142493024, p = 9.07 × 10 −12 ). The collagen VI protein is localized to corneal stroma filaments where it contributes to the integrity of the extracellular matrix 28 . Other associations were for COL1A1 (rs2075556, p = 3.35 × 10 −09 ) and COL5A1 (rs3118518, p = 1.83 × 10 −28 ). Collagen V is a regulator of collagen fibril formation, matrix assembly and tissue function in the corneal stroma 29 . Pathogenic variants in COL12A1 , COL6A1, COL1A1, and COL5A1 have been described in individuals with different subtypes of the connective tissue disorders Ehlers-Danlos Syndrome and osteogenesis imperfecta 30 , 31 , 32 , 33 .
We observed a strong association at the previously described 23 LOX locus (rs840464 p = 1.72 × 10 −20 , Table 1 ). LOX encodes lysyl oxidase, an enzyme that initiates the cross-linking of collagens and elastin 34 . One of the associated SNPs at this locus, rs840462 ( p = 2.08 × 10 −17 ), displayed the most significant expression quantitative trait locus (eQTL) effects over the transcription of the LOX gene ( p = 1.61 × 10 −13 ) in a GTEx dataset for sun-exposed skin (Supplementary Data 6 ). A significant association was found near the integrin gene ITGA2 (rs12515400, p = 3.16 × 10 −18 ). The protein encoded by this gene participates in complexes of integrin α2β1, which are collagen receptors 35 and which enhance type I collagen polymerization 36 .
We found association near the ALDH3A1 gene (rs4646785, p = 9.01 × 10 −12 ) for the same SNP that is also a significant eQTL, controlling transcription (eQTL p = 6.92 × 10 −10 ) in sun-exposed skin (Supplementary Data 6 ) and other tissues. ALDH3A1 encodes a corneal crystallin, a major component of the corneal stroma and epithelium that is upregulated in keratoconus corneas compared to controls 37 . It modulates corneal epithelial differentiation and homeostasis and may protect the eye from UV light-induced oxidative stress 38 , 39 , 40 , 41 , 42 .
For the first time, we report novel genetic associations implicating corneal cell differentiation and homeostasis in the pathogenesis of keratoconus. A significant association was found for rs17285550 ( p = 2.84 × 10 −12 ) near KLF5 , a transcription factor that regulates corneal epithelial cell idenitity 43 , 44 , 45 , 46 . KLF5 knockout mice have an abnormal collagen matrix 47 and suppressed levels of FNDC3B , another gene associated with keratoconus in our study (rs4894414, p = 1.21 × 10 −26 ). We found association for SNPs located near genes involved in fibroblast-keratocyte differentiation, such as rs761276 in the intergenic region between CD34 and CD46 ( p = 8.02 × 10 −09 ). CD34 is a negative marker of keratocyte differentiation in the cornea, expressed in stromal fibroblasts but not mature keratocytes 48 . Association was also found for SNPs located at the enhancer region of SMAD3 (rs12912010, p = 1.99 × 10 −26 ), a member of a family of genes involved in fibroblast differentiation 49 .
Association with several transcription factors involved in cell differentiation was revealed. The NANOS1 and EIF3A genes are located in the same associated region (near rs658352, p = 3.71 × 10 −12 ). NANOS1 controls cell cycle progression and is involved in the SMAD3 /TGFβ fibroblast maturation pathway 50 , 51 , while EIF3A is a cell differentiation suppressor 52 . FOXO1 (rs2721051, p = 5.71 × 10 −35 ) is implicated in the maintenance of keratinocyte stem cell identity 53 , and one associated region (rs12948086, p = 5.33 × 10 −09 ) overlaps a cluster of HOX genes, that participate in early embryonic differentiation and morphogenesis 54 .
Our results show that two loci previously implicated in Fuchs endothelial corneal dystrophy (FECD) 55 are also associated with keratoconus; the PIDD1 / SLC25A22 locus (rs7117921, p = 1.09 × 10 −26 ) and ATP1B1 (rs1200108, p = 4.52 × 10 −10 ). The allele increasing the keratoconus risk at both loci also conferred susceptibility to FECD (Supplementary Data 7 ).
Functional exploration of variants associated with keratoconus
Genes located in regions associated with keratoconus are broadly expressed across all GTEx 56 and available eye tissues 57 (Supplementary Fig. 2 ), more so in fetal corneas than in other eye tissues (Supplementary Data 8 , Supplementary Fig. 3 ). In addition, certain genes near the association peaks were shown previously to be differentially expressed in keratoconus compared to control corneas 58 . Expression of STK35 , that is associated in our analyses (rs6106210, p = 2.85 × 10 −11 ), was increased two-fold in keratoconus corneas (FDR = 3.24 × 10 −03 ) and RAB11FIP4 (rs56161228, p = 2.70 × 10 −10 ) found in our study, was increased by 2.4 fold (FDR = 3.24 × 10 −03 ).
Gene-set enrichment analyses found associations with Gene Ontology annotations (Supplementary Data 9 ), including transcriptional regulation ( p = 1.0 × 10 −06 ), embryonic and primary germ layer development ( p = 7.0 × 10 −06 ), and embryonic morphogenesis ( p = 3.0 × 10 −06 ).
Because of limited availability of corneal eQTL datasets, we conducted a heritability-partitioning analysis to test for enrichment of genes in available eQTLs from other tissues 59 . The strongest enrichment, albeit not significant after multiple testing, were mainly collagen and fibroblast-rich tissues, such as aortic valve, myometrium, and skin (Supplementary Data 10 ).
We subsequently investigated the mechanisms through which the DNA variants associated with keratoconus in our meta-analyses alter the susceptibility to disease. We found that many of these variants contribute to epigenetic changes of the genomic regions in which they are located, alter the efficiency of transcription of nearby genes, or both (Supplementary Fig. 4 ). Mendelian randomization-based (SMR) tests 60 which included all the SNPs for which we had summary statistics, regardless of the degree of genetic association with the trait, suggest that methylation is a widespread mechanism mediating the effect of disease-associated SNPs. We found evidence that SNPs associated with keratoconus often alter methylation of many genes, including LOX, PDDC1, SMAD3, HOXB1, KLF5, and BANP (Supplementary Data 11 ). Interestingly, methylation of KLF4 was significantly (SMR p = 5.10 × 10 −08 ) influenced by SNPs associated with keratoconus. KLF4 is a transcription factor involved in tissue differentiation and development. It induces stem cell pluripotency in fibroblasts 61 and regulates corneal epithelial cell cycle progression by suppressing canonical TGFβ signaling 62 and is functionally related to KLF5 , which specifies corneal epithelial cell identity 46 . We also found several eQTL mediated effects (Supplementary Data 12 ), which were less significant than the methylation-mediated effects.
Inter-trait correlation and pleiotropy of keratoconus-associated loci
The strongest genetic correlations between keratoconus and other ocular traits (Supplementary Data 13 ) were with spherical equivalent ( p g = 2.07 × 10 −08 ) and CCT ( p g = 2.5 × 10 −07 ). However, as previously noted 16 , correlation of effect sizes with CCT was not always uniform. SNPs at the ZNF469/BANP locus, but also several loci that, to the best of our knowledge, are newly identified, such as the ATP1B1 locus, diverged considerably from the linear correlation with CCT (Fig. 4 , Supplementary Data 14 ). This supports the view that other mechanisms of loss of corneal integrity and corneal fragility, independent of corneal thickness, contribute to the pathogenesis of keratoconus. There were also nominally significant genetic correlations with asthma and ulcerative colitis (Supplementary Data 13 ), possibly because of the coincidental high collagen content in tissues involved in these diseases, or reflecting shared inflammatory components 63 .
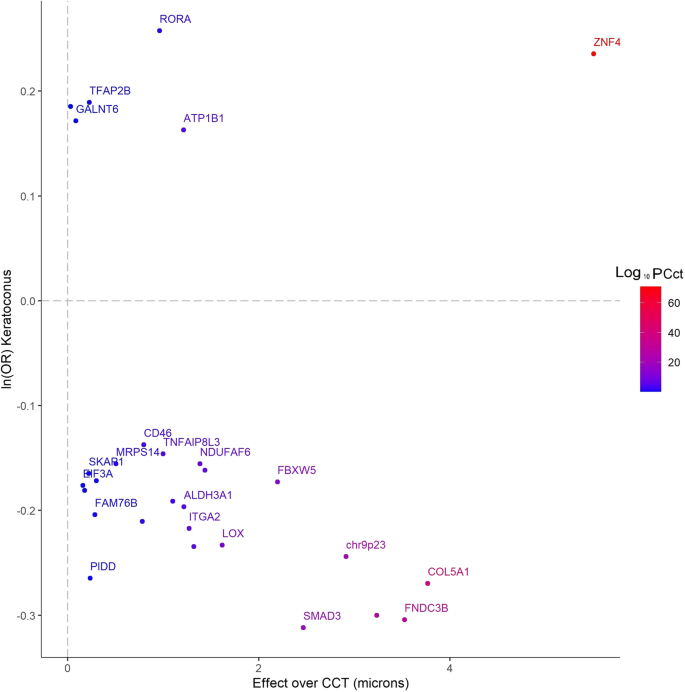
Data reported by Choquet et al. 20 (also in Supplementary Data 14 ), with those over keratoconus (4669 cases and 116,547 controls, natural logarithm of the ORs, the y -axis). The alleles increasing susceptibility to keratoconus were selected as reference alleles. The genes located nearest to the most significant SNPs are shown in the labels and their color-codes denote the significance (−log10( p -value) of the association with CCT 20 . For the sake of clarity, only some of the genes are labeled. Unlabeled points denote SNPs in intragenic regions (distance from the nearest known protein-coding transcript greater than 250kbp). The variants are annotated to the nearest known protein-coding transcript. Because of their proximity with other SNPs, some loci in this figure are not labeled. Polymorphisms identified in the discovery cohort, but not shown in this figure were not available for analysis in the replication cohorts.
Predictive value of common genetic markers associated with keratoconus
An LD score regression analysis revealed that genetic markers associated with keratoconus in our meta-analysis help explain 12.5% of overall keratoconus heritability among the same populations of European ancestry. Next, we assessed the predictive value of the markers we identified. A predictive model tested in a small, but independent panel of mixed British, Dutch and Austrian keratoconus patients and controls of European descent, found that the markers were moderately predictive (AUC = 0.737, SE = 0.017, Fig. 5 ) of keratoconus. The addition of rare variants, in future studies, is likely to further increase the predictive value of genetic testing.
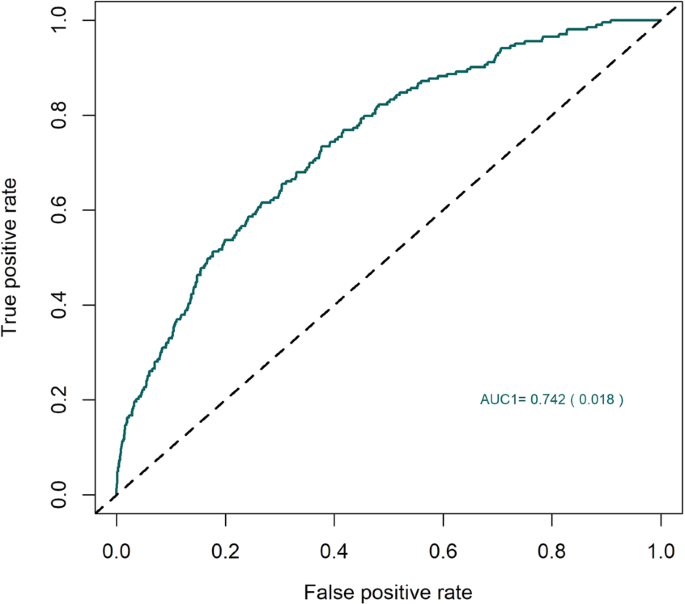
The area under the receiver operating characteristic curve for the performance of a keratoconus predictive model that used the SNPs identified through the multi-ethnic analysis. The model was trained in the discovery populations of European ancestry and was replicated in a completely independent European panel of 222 keratoconus patients and 2208 controls.
In conclusion, we report 36 genetic loci strongly associated with keratoconus, 31 of which we identify for the first time. Their effect sizes are remarkably consistent across different ethnic groups (Supplementary Fig. 5 ). Larger studies are required to identify the remaining genetic risk for this condition (Supplementary Fig. 6 ). Our data highlight the importance of the integrity of the corneal collagen matrix in keratoconus. For the first time, we have identified a substantial role for cell differentiation pathways and stem cell regulators such as KLF4 and KLF5 in the pathogenesis of keratoconus, and a role for genes influencing connective tissue maturity.
Our study has several strengths. It is currently the largest of its kind which has allowed the identification of several loci, mostly novel, that predispose to keratoconus. In addition, although dominated by cases and control of European ancestry, the multi-ethnic component of our study allowed an evaluation of the strength of these associations among individuals of African and Asian descent. A limitation of this study is the lack of reliable corneal-specific eQTL and methylation level assessment. This has impeded the functional annotation of the discovered genetic loci, which are currently annotated to the nearest transcript-coding gene. Assuming that the effect of many SNPs is mediated through eQTL or methylation changes, cornea-specific analyses would improve these annotations. Lack of available tissue-specific expression and methylation data also currently limits our ability to further characterize these loci functionally. Our current approach would allow the identification of relationships that transcend tissue specificity and are observed in different cell lines. Also, the genomic inflation factor was moderately high, especially in our non-European cohorts, for which we are not able to fully evaluate the degree to which this is driven by polygenicity, a consequence of the presence of high-effect common variants in a low prevalence disease, or any other potential explanation.
Identification of genetic risk factors and novel disease mechanisms represents a substantial advance of our understanding of the corneal disease and, more broadly, connective tissue homeostasis, highlighting targets for the development of novel therapies. Two patient groups could directly benefit from an improved estimate of their risk of progressive corneal thinning. Firstly, individuals with subclinical keratoconus, in whom corneal collagen cross-linking would be an option to stop disease progression to reduce the need for contact lens wear for visual correction or eventual corneal transplantation. Secondly, genomic screening could be performed before laser refractive surgery for the correction of short sight (laser vision correction), to identify individuals at risk of severe visual loss from secondary progressive corneal thinning. Apart from refractive error, these individuals have normal eyes before surgery and there is currently no reliable mechanism to identify individuals at risk of developing this secondary corneal change, similar to keratoconus. For both groups, genomic data to estimate risk could be incorporated into models based on clinical parameters such as refractive error, corneal thickness, corneal shape, and corneal biomechanics 64 , 65 .
Study overview
This study was approved by the Institutional Review Board (IRB) or equivalent at all participating institutions, and participants provided written informed consent for the use of their genetic information. The study was conducted in concordance with the provisions of the Declaration of Helsinki.
Controls were extracted from a pool of 80,000 randomly selected participants in the UK Biobank cohort. Exclusions included any individual with any ICD9 or ICD10 code for any corneal disease. The cases and controls were ethnically matched.
Multi-ethnic discovery cohort
Phenotyping.
The majority of participants were recruited from specialist clinics at Moorfields Eye Hospital, London, UK. The study was approved by the Moorfields Eye Hospital Research Ethics Committee (09/H0721/19). The diagnosis of keratoconus was established based on clinical signs of corneal thinning and corneal distortion, with confirmation by corneal imaging (Orbscan, Bausch & Lomb, Rochester, USA, or Pentacam, Oculus, Wetzlar, Germany). Previous bilateral keratoplasties for keratoconus were also accepted as confirmation of disease status. Patients with keratoconus who had syndromic disease (e.g. Down syndrome, Leber congenital amaurosis, Ehlers Danlos syndrome) were excluded, as were patients with corneal dystrophy, of cornea guttata suggestive of coexisting Fuchs endothelial corneal dystrophy.
Recruitment from specialist corneal clinics at St. James’s University Hospital, Leeds, UK, was approved by Leeds East Research Ethics Committee (reference 10/H1306/63). The diagnosis of keratoconus was determined clinically and confirmed with corneal imaging (Orbscan, Bausch & Lomb, Rochester, USA, or Pentacam, Oculus, Wetzlar, Germany). Patients who had keratoconus associated with syndromes and those without the capacity to consent were excluded.
Participants were recruited from the Corneal and External Eye Disease clinics at Guy’s and St. Thomas’ National Health Service Foundation Trust, London, UK. The diagnosis of keratoconus was established based on a history of uni- or binocular progressive visual disturbance and the presence of some of all of a number of clinical signs seen on slit-lamp biomicroscopic examination including corneal stroma thinning, sub-epithelial iron deposition (Fleischer ring), stromal scarring, deep vertical stromal stress lines (Vogt’s striae) and corneal ectasia, with confirmation by three-dimensional corneal imaging (Pentacam, Oculus, Wetzlar, Germany). A history of previous bilateral keratoplasty for keratoconus was also accepted as confirmation of disease status. Patients with keratoconus who had syndromic disease (e.g. Down syndrome, Leber congenital amaurosis) were excluded.
Czech participants were selected based on the same criteria as used by Moorfields Eye Hospital London. The study protocol for Czech participants was approved by the Ethics Committee of the General Teaching Hospital and Charles University, Prague. Patients were recruited at the Department of Ophthalmology in Prague between 2011 and 2017. The diagnosis of keratoconus was based on the detection of localized steepening on corneal topography maps, together with localized corneal thinning in at least one eye. Only patients with KC grade 1 or higher according to the Pentacam (Oculus Optikgeräte GmbH, Wetzlar, Germany) build in software classification were included. Some eyes had advanced disease with typical signs such as Vogt striae, Fleischer ring, and stromal scarring, but this was not an inclusion requirement for the purposes of this study. Patients with bilateral keratoplasties for KC were also considered as cases. Patients with a known monogenic disorder were excluded.
The Melbourne patients were individuals with keratoconus and European background who were recruited from public clinics at the Royal Victorian Eye and Ear Hospital (RVEEH), private and optometry clinics in Melbourne, Australia. They were required to complete a study questionnaire, clinical examination, and details of family history of disease. A patient information sheet, consent form, privacy statement, and patient rights were provided to all individuals participating in the study. The study protocol was approved by the Royal Victorian Eye and Ear Hospital Human Research and Ethics Committee (Project#10/954H). Written informed consent was obtained from each participant and all protocols followed the tenets of the Declaration of Helsinki. A blood/saliva sample was also collected. DNA was extracted from the blood or saliva sample using NucleoSpin® QuickPure kits 66 . Keratoconus was diagnosed on the basis of the presence of one or more of the following: (1) an irregular corneal shape, as determined by distortion of keratometric mires and/or corneal tomographic images, (2) scissoring of the retinoscopic reflex; and (3) demonstration of at least one biomicroscopic sign, including Vogt’s striae, Fleischer’s ring or corneal thinning and scarring typical of Keratoconus 67 . Potential subjects with non-keratoconus ocular disease in both eyes such as keratectasia, corneal degenerations, macular disease, and optic nerve disease (e.g., optic neuritis, optic atrophy) were excluded from the study.
Both cases and controls were genotyped using the Affymetrix UK Biobank Axiom Array. For the discovery cohort cases, DNA was extracted from peripheral blood samples using standard methods (unless otherwise stated). DNA samples ( n = 4,032) were quantified and normalized prior to genotyping using the Axiom 2.0 assay for GeneTitan on UK Biobank Axiom arrays (High-Throughput Genomics Centre at the Wellcome Trust Centre for Human Genetics, University of Oxford). 99% of samples reached the SNP QC call rate threshold of ≥97%. The procedures that produced the genotyping information in the UK Biobank participants that were used as controls for our analyses are described elsewhere 68 .
Intensity (CEL) files from cases and controls were called together using the “apt-probeset-genotype” program from Affymetrix ( http://www.affymetrix.com/support/developer/powertools/changelog/apt-probeset-genotype.html , Thermo Fisher Scientific, Waltham, MA). Libraries were downloaded from the UK Biobank Axiom Array product support pages ( https://www.thermofisher.com/order/catalog/product/902502 ). Controls genotyped using the UKBiLEVE SNP chip were removed from the calling process. Genotyping was done in batches of 4,000-5,000 samples each. We subsequently used SNP Polisher functionalities (probeset metrics, classification, OTV caller) following the Affymetrix recommendations ( https://www.affymetrix.com/support/developer/powertools/changelog/VIGNETTE-snp-polisher-apt.html#otvcaller ).
Post genotyping, UK Biobank sample genotypes were compared (via chi-square association testing) with the genotypes released from the UK Biobank. Only unrelated individuals (PI_HAT < 0.05) were included in the analyses. Subsequently, analyses were run in separate ethnic groups (African, South Asian, and Afro-Caribbean). SNPs with excessive (>0.05) missing genotypes and large ( p < 0.01) differences in allele frequency compared with the official UK Biobank genotypes were removed from further analyses. This threshold of significance was adopted because even random missing samples that failed genotyping, either at the UK Biobank processing centre or in our labs, could cause minor and non-significant differences in allele frequencies.
Ancestry information and case-control matching
Cases and controls were subsequently matched for ancestry. First, a Principal Component Analysis (PCA) was run on independent (r2 < 0.3) directly genotyped SNP, then the samples were clustered in either one of the three major ancestral clusters: European, South Asian, or African. Samples that were not part of any of the clusters were removed from further consideration.
To avoid subtler population structure, arising either from ethnic heterogeneity or batch effects, we matched cases and controls based on the information from the first 10 principal components. We sought to match one case with up to 10 controls, if possible.
Only cases and controls that were matched in such a way were taken forward and imputed together.
Imputation was run separately for the European and non-European ancestral groups (African and South Asian). The Europeans were split into two groups with an equal number of cases and controls and were imputed up to the HRC r1.1 2016 haplotypes using Minimac4 after Eagle 2.4 pre-phasing. Non-European ancestral groups were imputed up to the 1000 G Phase 3 v5.
Association analyses
Association analyses were conducted based on Firth’s regression models, given that our case-controls were imbalanced. Regression models were built with the keratoconus status as the dependent variable and the allele dosage at each SNP locus as a predictor. Analyses were adjusted for sex and the first 20 principal components and were conducted for each ancestral group separately. The largest case-control samples were of European ancestry and were used for discovery purposes. Samples of African and South Asian ancestries were used for validation purposes.
Replication cohorts
The los angeles-affiliated cohort, enrollment and phenotyping.
Clinically affected keratoconus patients were enrolled into the study at three major sites: the longitudinal videokeratography and genetic study at the Cornea Genetic Eye Institute at Cedars-Sinai Medical Center, Los Angeles, CA, USA; the Jules Stein Eye Institute at UCLA, Los Angeles, CA, USA; and the University Hospitals Eye Institute at University Hospitals Case Medical Center and Case Western Reserve University, Cleveland, OH, USA. All patients diagnosed with keratoconus (see below) were offered recruitment into the study.
In addition to keratoconus patients, 126 local controls were recruited at Cedars-Sinai Medical Center. Convenience Caucasian controls from a Cholesterol and Atherosclerosis Pharmacogenetics (CAP) study were also included to make the sample size of controls equivalent to that of cases. CAP sample involved 944 unaffected volunteers, 609 of whom were self-reported white. Participants were aged 30 or above and were recruited from two clinical sites located in Los Angeles and San Francisco, CA, respectively. Additional details of CAP samples are described elsewhere 69 .
The diagnosis of keratoconus was performed by a corneal specialist ophthalmologist or an experienced research optometrist and based on clinical examination and videokeratography pattern analysis. Clinical examination included slit-lamp biomicroscopy, cycloplegic retinoscopy, and fundus evaluations. Slit-lamp biomicroscopy was used to identify stromal corneal thinning, Vogt’s striae, or a Fleischer ring. Retinoscopy examination was performed with a fully dilated pupil 20 min after phenylephrine 2.5% and cyclopentolate 1% drops had been instilled in the eye to determine the presence or absence of retro-illumination signs of keratoconus, such as the oil droplet sign and scissoring of the red reflex. Videokeratography evaluation was performed on each eye using the Topographic Modeling System (Computed Anatomy, New York, NY, USA), Orbscan II (Bausch & Lomb, Rochester, NY, USA), Oculus Pentacam (Oculus, Inc, Lynnwood, WA, USA) or Keratron (Optikon, Rome, Italy). Subjects were considered to have keratoconus if they had at least one clinical sign of keratoconus and a confirmatory videokeratography map with an asymmetric bowtie with a skewed radial axis above and below the horizontal meridian (AB/SRAX) pattern 70 . Importantly, topography was screened for mimicking disease such as pellucid marginal degeneration, which was excluded. Subjects that had bilateral keratoplasty for keratoconus were included if the surgical pathology report confirmed the presence of the disease.
DNA was extracted from EBV transformed lymphoblastoid cell lines established from peripheral whole blood of each study participant using NucleoSpin Tissue kit (MACHEREY-NAGEL Inc., Bethlehem, PA, USA) and from saliva samples using QIAsymphony DNA Kit (Qiagen, Germantown, MD).
Genotyping was performed using the Illumina HumanOmni2.5 Beadchip for all keratoconus patients, 126 local controls and 50 quality controls from CAP study. Genome-wide genotyping of half of CAP study subjects was performed using Illumina HumanHap300 BeadChip and of the remaining half of the samples with Illumina HumanCNV610-Quad Beadchip. Additional genotyping with iSelect Beadchip and Metabo-Chip was also available for some CAP samples. Samples with sex mismatches, relatedness (pi-hat>0.1875), low call rate (<95%), and SNPs with low minor allele frequency (MAF < 1%), low genotyping rate (<95%), and Hardy–Weinberg equilibrium p values less than 10 −6 were excluded from the analysis. Both cases and controls datasets were imputed using the Michigan Imputation Server 71 with the Haplotype Reference Consortium (HRC) 72 . Post-imputation QC removed SNPs with low imputation quality (rsq < 0.1) and a concordance rate less than 95% among 50 quality control samples. QC was performed using PLINK v1.9 73 . Principal components analysis (PCA) was performed with EIGENSTRAT 74 Self-identified Hispanic samples were checked by PCA, and outliers significantly different from self-reported European individuals were excluded. After verifying the participants’ ancestry through a principal component analysis, 662 cases and 676 controls of full European ancestry were included in the analyses.
In total, 662 keratoconus cases and 676 controls (123 local controls and 553 controls from CAP study) with both phenotyping and genotyping data were available for analysis after QC. Under an additive genetic model, we conducted association tests between keratoconus and all autosome SNPs with MAF greater than 5%. The logistic regression model was performed adjusting for gender and three principal components (PCs) with RVTESTS 75 .
Australian cohort
Genome-wide association analysis of this cohort has been described previously 24 . In brief, participants with keratoconus were ascertained through the eye clinic of Flinders Medical Centre, Adelaide; optometry and ophthalmology clinics in Adelaide and Melbourne; or an Australia-wide invitation to members of Keratoconus Australia, a community-based support group for patients. Approval was given by the Southern Adelaide Clinical Human Research Ethics Committee (HREC), the HREC of the Royal Victorian Eye and Ear Hospital and the Health and Medical HREC of the University of Tasmania. All participants gave informed consent and the study conformed to the tenets of the Declaration of Helsinki.
Patients were classified as having keratoconus if they had at least one clinical sign of keratoconus and a confirmatory videokeratography or a penetrating keratoplasty performed because of keratoconus, as described previously. 76 The controls included 465 unaffected individuals from the Blue Mountains Eye Study 77 and an additional 211 unaffected individuals 78 from the Australian cohort previously described as controls in a GWAS for age-related macular degeneration (AMD) from the International AMD Genomics Consortium 79 .
DNA for cases and controls was extracted from whole blood using the QiaAMP DNA Maxi kit (Qiagen, Hilden, Germany).
Cases were genotyped for 551,839 variants using the HumanCoreExome array (HumanCoreExome-24v1-1_A, Illumina, San Diego, CA, USA) while for the controls, genotypes of 569,645 variants were generated with a customized Illumina HumanCoreExome array (“HumanCoreExome_Goncalo_15038949_A”) as described previously 79 . Only SNPs common to both arrays were included.
Quality control was carried out according to the protocol described by Anderson et al. 80 , modified as follows. Reverse and ambiguous strand SNPs were detected using snpflip ( https://github.com/biocore-ntnu/snpflip , accessed March 24, 2017) and flipped or excluded. Ancestry outliers identified by principal component analysis (PCA) using EIGENSTRAT 74 , as well as individuals missing genotype rate > 0.05, heterozygosity more than three standard deviations from the mean, or discordant sex information, were excluded. Related individuals were detected by calculating pairwise identity by descent (IBD), and the individual with the lower genotyping rate in any pair with IBD > 0.185 was removed. Markers were excluded if they had significantly different missing data rates between cases and controls, total missing genotype rate > 3%, minor allele frequency (MAF) < 0.01, or deviated significantly ( P < 10 −5 ) from Hardy–Weinberg equilibrium. Following all exclusions, there were 522 cases (mean age 45) and 655 controls (mean age 65) genotyped for 264,115 common platform SNPs.
Genotypes of autosomal SNPs were phased with Eagle (version 2.3.5) 81 and imputed to the EUR subset of the 1000 Genomes Project reference panel (Phase III, version 5) 82 using Minimac3 (version 2.0.1) 71 . Indels, SNPs within 5 bp of an indel, rare variants (MAF < 0.01), and variants with poor imputation quality ( R 2 < 0.8) were excluded. A total of 6,252,612 including 250,964 genotyped variants, passed quality control. Association analysis was performed on most-likely genotypes under a logistic regression model using PLINK (version 1.90) 83 using the first three principal components as covariates.
Genetic epidemiology research in adult health and aging cohort (GERA)
The Genetic Epidemiology Research in Adult Health and Aging (GERA) cohort is part of the Kaiser Permanente Research Program on Genes, Environment, and Health (RPGEH) and has been previously described in detail 84 , 85 . The GERA cohort comprises 110,266 adult men and women who are consented participants in the RPGEH, an unselected cohort of adult participants who are members of Kaiser Permanente Northern California (KPNC), an integrated health care delivery system, with ongoing longitudinal records from vision examinations. For this analysis, 78,583 adults, who self-reported as non-Hispanic white, were included. Of which 72 cases were males and 85 females, with an average age of 61.9 years (S.D. 12.3). All study procedures were approved by the Institutional Review Board of the Kaiser Foundation Research Institute.
Keratoconus cases were identified in the KPNC electronic health record system based on the following International Classification of Diseases, Ninth Revision (ICD-9) diagnosis codes: 371.60, 371.61, and 371.62. All selected keratoconus cases ( N = 157) had at least one diagnosis of keratoconus made by a Kaiser Permanente ophthalmologist. Our keratoconus control group ( N = 78,426) included all the non-cases.
DNA samples from GERA individuals were extracted from Oragene kits (DNA Genotek Inc., Ottawa, ON, Canada) at KPNC and genotyped at the Genomics Core Facility of the University of California, San Francisco (UCSF). DNA samples were genotyped at over 665,000 single nucleotide polymorphisms (SNPs) on Affymetrix Axiom arrays (Affymetrix, Santa Clara, CA, USA) 86 , 87 . SNPs with initial genotyping call rate ≥97%, allele frequency difference ≤0.15 between males and females for autosomal markers, and genotype concordance rate >0.75 across duplicate samples were included 85 . Around 94% of samples and more than 98% of genetic markers assayed passed quality control (QC) procedures. In addition to those QC criteria, SNPs with genotype call rates <90% were removed, as well as SNPs with a minor allele frequency (MAF) < 1%.
Following genotyping QC, we conducted statistical imputation of additional genetic variants. Following the pre-phasing of genotypes with Shape-IT v2.r72719 88 , variants were imputed from the cosmopolitan 1000 Genomes Project reference panel (phase I integrated release; http://1000genomes.org ) using IMPUTE2 v2.3.0 89 , 90 , 91 . As a QC metric, we used the info r 2 from IMPUTE2, which is an estimate of the correlation of the imputed genotype to the true genotype 92 . Variants with an imputation r 2 < 0.3 were excluded, and we restricted to SNPs that had a minor allele count (MAC) ≥ 20.
We ran a logistic regression of keratoconus and each SNP using PLINK 83 v1.9 ( www.cog-genomics.org/plink/1.9/ ) with the following covariates: age, sex, and genetic ancestry principal components (PCs). We modeled data from each genetic marker using additive dosages to account for the uncertainty of imputation 93 . Eigenstrat 74 v4.2 was used to calculate the PCs 84 . The top 10 ancestry PCs were included as covariates, as well as the percentage of Ashkenazi ancestry to adjust for genetic ancestry, as described previously 84 .
UK samples of non-European ancestry
Among the subjects recruited at the Moorfield Eye Hospital, there were 759 cases of South Asian ancestry and 405 of African descent. Phenotypes were obtained as described before. These samples were matched from a separate pool of ethnic minorities from the UK Biobank and analyzed using Firth’s regression models, adjusting for sex and the first 20 principal components that were calculated within the ethnically matched case-control group. These samples were primarily used for replication and validation purposes, but also contributed to the final meta-analysis.
Cohorts used for prediction
Prediction models were trained in cases and controls of European descent described above and tested in 222 keratoconus patients and 2208 keratoconus-free controls, pooled from the Erasmus Medical Centre cohort and the UK Biobank.
The Erasmus Medical Centre (EMC) cohort
The Erasmus Medical Centre (EMC) cohort consisted of Caucasian keratoconus patients ( n = 156) and controls ( n = 1476). Keratoconus samples were collected from both the Erasmus MC and the Rotterdam Eye Hospital. Control samples were collected from 1) the Rotterdam study ( n = 448), a population-based study described previously 94 ; 2) the Biomarkers of personalized Medicine (BioPersMed) study ( n = 891) which included healthy subjects with one or more risk factors for cardiovascular diseases (Graz, Austria); 3) controls from the Amsterdam Glaucoma Study ( n = 137), previously described 95 . All patients and controls underwent a full ophthalmic examination at the correspondent medical centers as part of the inclusion protocol of each study. The studies were approved by the Institutional Review Board at each institute and informed consents were collected from all participants.
DNA from cases and controls was genotyped using the Illumina bead chip (Infinium Global Screening Array-24 V2; Illumina Inc) at the Human Genetics facility at EMC. Quality control was performed using Genome studio (Illumina-designed software) and PLINK on 683880 genotyped variants from 1632 individuals. In summary, samples with more than 10% missing variants and variants with minor allele frequency less than 5% were excluded. Moreover, a Hardy–Weinberg equilibrium cut-off of 10 −7 was used for control samples. The genomic inflation factor (lambda) of 1.08 (based on median chi-square), showing no significant dispersion of test statistics from the expected distribution. A total of 454925 variants (genotyping rate of 0.998) from 1629 subjects (155 cases and 1474 controls) were imputed using Michigan imputation server. Post-imputation quality checks were performed and a total of 57 variants from 1629 subjects were included in the final analysis.
The UK Biobank subset
A subset of 66 cases and 733 controls were extracted from the UK Biobank cohort. In brief cases were individuals who reported keratoconus (ICD code H18.6) and controls were UK Biobank participants who did not report keratoconus but also were negative for other significant corneal disease (ICD10 codes H18.7, H18.8 and H18.9) and without any prior history of eye surgery. UK Biobank participants that were included as controls in the previously described analyses were specifically excluded from this step. All methods, genotyping, imputation, and basic QC were similar to what is described elsewhere before 96 .
Meta-analyses
We conducted three meta-analyses. For the initial meta-analysis (discovery), we used summary statistic results from the discovery cohorts. These cohorts were genotyped on the same chip and recruited using consistent methodologies. The second meta-analysis aggregated data from summary statistics of three independent cohorts of European ancestry, recruited in addition to the discovery cohort. For the final meta-analysis, we used all available information from all available cohorts. Very rare variants (MAF < 0.01) and rare ones (0.01 < MAF < 0.05) with low imputation quality scores (<0.8) or high meta-analysis heterogeneity I 2 > 0.75 were excluded.
For all meta-analyses we applied a fixed-effect inverse variance method as implemented in the software METAL 97 and GWAMA 98 . No genomic control adjustment was applied during the meta-analysis.
Effective population size and power calculations
Power calculations were conducted using the Stata 15 “ power” ’ package (StataCorp LLC, College Station, TX).
The effective population size was calculated per each locus, aggregating the effective population sizes of each cohort participating in the meta-analyses, using this equation:
as recommended elsewhere 97 , where N.eff is the effective sample size, N.cases is the number of cases, and N.controls is the number of subjects without keratoconus.
Only SNPs with minor allele frequency of at least 1%, which were available from at least 70% of the maximum number of participants across all studies, and that were not missing in more than one stratum (cohorts), were considered.
Multiple testing correction
Two methods of correcting for multiple testing were used. The first was a classic Bonferroni correction, in which the threshold of significance (0.05) was divided by the number of tests ( n ):
Given the large number of loci for which replication was needed, we additionally calculated the False Discovery Rates, using the Benjamini–Hochberg method 99 .
Genomic inflation
To assess the potential inflation of association probabilities, genomic inflation factors 22 were calculated and Q-Q plots were drawn using the package “gap” in R ( https://cran.r-project.org/ ).
LDscore regression-based methods
Calculation of genomic inflation attributable to polygenicity vs. stratification.
We used LD score regression-based methods to calculate the genomic inflation factors, and measures of stratification within the samples of European ancestry. We followed the LDSC authors’ recommendations ( https://groups.google.com/g/ldsc_users/c/yJT-_qSh_44/m/MmKKJYsBAwAJ ) to normalize the effective sample sizes and account for the different numbers of cases and controls in the different populations, thereafter using a sample prevalence estimate of 0.5, and a disease prevalence in the population of 0.001.
Calculation of genetic correlation
Bivariate genetic correlations between keratoconus and other complex traits whose summary statistics are publicly available were assessed following previously described methodologies 100 , using the program LD Score ( https://github.com/bulik/ldsc ).
We used a maximum-likelihood model to estimate the distribution of effect sizes, based on summary statistics of observations and linkage disequilibrium patterns to predict the likely number of SNPs that explain keratoconus heritability as well as explore the relationship between future sample sizes and the number of SNPs identified and variance or heritability explained as described elsewhere 101 and implemented in the GENESIS R package ( https://github.com/yandorazhang/GENESIS ).
SNPs and gene annotations
Polymorphisms associated at a GWAS level ( P < 5 × 10 −08 ) were clustered within an “associated genomic region”, defined as a contiguous genomic region where GWAS-significant markers were within 1 million base pairs from each other, as suggested elsewhere 102 . Significant polymorphisms were annotated with the gene inside whose transcript-coding region they are located, or alternatively, if located between two genes, with the gene nearest to it. The associated genomic regions were collectively annotated with the gene overlapping, or nearest the most significantly associated variant within that region. In addition, the polymorphic sites were functionally annotated using SNPnexus 103 . CADD scores were generated using Ensembl variant effect predictor ( http://grch37.ensembl.org/Homo_sapiens/Tools/VEP ).
Previous association with keratoconus and CCT
We collected evidence of previous associations with keratoconus and CCT by querying the GWAS catalog 104 and looking up reports in peer-reviewed articles 16 , 17 , 20 , 24 , 105 for genomic markers and regions associated with either. We specifically looked for genome-wide significance level of association.
SMR (Summary data-based Mendelian randomization) uses GWAS variants as instrumental variables and gene expression levels or methylation levels as mediating traits, in order to test whether the causal effect of a specific variant on the phenotype-of-interest acts via a specific gene 60 (but note, in practice, SMR is unable to differentiate between causation and horizontal pleiotropy). SMR incorporates the Heterogeneity in Dependent Instrument (HEIDI) test, which is designed to detect variants with horizontally pleiotropic effects (via their heterogeneity in the SNP-outcome vs. SNP-intermediate trait relationship, in comparison to nearby variants). SMR analyses were repeated after excluding ‘outlier’ variants detected using HEIDI.
Test description
The SMR software helps perform two tests. The first is an SMR test, which correlates GWAS effects with eQTL or methylation effects (or any other intermediate trait) 60 . This test suggests causation, although it is unable to fully differentiate between it and pleiotropy. The second test is that of Heterogeneity in Dependent Instrument (HEIDI). This test against the null hypothesis that changes in both eQTL (or other intermediary traits) and the phenotype of interest are caused by one single SNP, which is therefore considered as the candidate for the putative causal effect.
Datasets for the SMR analyses: eQTL, cis-mQTL
To perform the above-mentioned tests of causation/pleiotropy, we used three different datasets of association between genetic variants and intermediate traits. The eQTL associations were obtained from the untransformed peripheral blood samples of 5311 subjects 106 and methylation data from the analyses of LBC methylation of 1980 subjects described elsewhere 107 . They were the largest eQTL datasets available.
Gene-set enrichment
To identify pathways or other gene sets that were over or under-represented among our results, we used a Gene-Set Enrichment Analysis (GSEA) as implemented in the Meta-Analysis Gene Set Enrichment of Variant (MAGENTA) software 108 . This program assigns scores to each gene based on the strength of association with keratoconus, adjusting for potential confounders such as gene length and linkage disequilibrium. Enrichment for any gene set was assessed within genes above the cut-off of the highest 75th centile of significant gene scores. For the current study, the most recent versions of Gene Ontology (GO), Panther, KGG, Biocarta, and MSigDB databases were used. We also carried out a similar enrichment analysis for the presence of transcription factor binding sites. A permutational procedure and false-discovery rates were used to calculate the significance of enrichment and control for multiple testing.
GSEA definitions
For the enrichment analyses, we used updated versions of the GSEA gene sets as described before 109 . We used the versions from November 2018 which were downloaded from http://software.broadinstitute.org/gsea/login.jsp
Gene expression, GTEx, and other transcription data
We obtained data on tissue expression from several sources for genes located within associated loci. Information about the expression of the genes of interest in systemic (i.e. non-ocular) tissues was obtained from the GTEx Portal for GTEx release v7 ( https://gtexportal.org/home/datasets ). RNA sequencing data were obtained for both fetal and adult corneal, trabecular meshwork, and ciliary body, as described elsewhere 57 , which we downloaded from the authors’ supplementary information. In addition, we extracted data from the subset of subjects with presumed healthy adult retinas (AMD=1), described elsewhere 110 that obtained from the GTEx Portal ( https://gtexportal.org/home/datasets ).
Transcription data were processed using different platforms and were available in different units (Transcripts per Million bases, TPM, for the retina and GTEx tissues, and Fragments per Kilobase, FPKM for the other tissues). For purposes of comparing expression across different tissues for which different methodologies may have been used, expression levels for all tissues were rank-transformed. Hierarchical clustering was used to help visualize similarities and differences of patterns of transcript expression across different tissues (“hclust” package in R).
LD score regression applied to specifically expressed genes (LDSC-SEG)
Disease-relevant tissues and cell types were identified by analyzing gene expression data together with summary statistics from the meta-analysis of keratoconus in all cohorts, as described elsewhere 59 . Briefly, genes were ranked based on the t-statistic of their expression in each tissue and the 10% most expressed genes for each tissue were considered “specifically expressed genes”. A stratified LD score regression was applied to the meta-analysis summary statistics to evaluate the contribution of the focal genome annotation to trait heritability.
Prediction analyses
We built a model which included sex, and the major genetic variants associated with keratoconus. The model included all SNPs from a conditional analysis from a European-only conditional analysis 111 , as implemented in the program GCTA 112 (v1.92.1beta6). The model was trained using the cases and controls of European descent only from the discovery cohort and tested in an independent panel of keratoconus cases and controls. Genotype missingness was < 1% in all cases and controls, and whenever a genotypic value was missing, it was replaced with the population average (calculated on both cases and controls) for each locus. A Receiver Operating Characteristic (ROC) curve was drawn for each case and an Area Under the Curve (AUC) was calculated. R programming language and software environment for statistical computing ( https://cran.r-project.org/ ) was used for both the logistic regression models (‘glm’) and to evaluate the performance of the model (‘ROCR’).
Reporting summary
Further information on research design is available in the Nature Research Reporting Summary linked to this article.
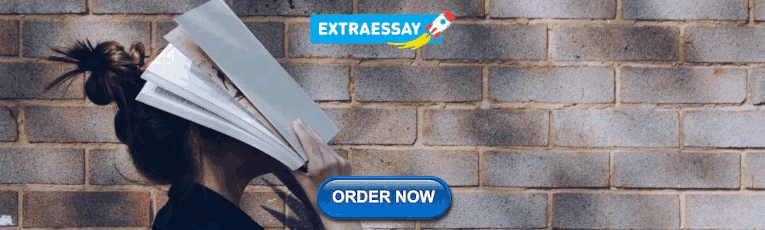
Data availability
The GWAS summary statistics are available in Supplementary Data 15 . The summary statistics are also available through the GWAS Catalogue. Source data for the main figures can be found in Supplementary Data 16 – 19 .
Godefrooij, D. A., de Wit, G. A., Uiterwaal, C. S., Imhof, S. M. & Wisse, R. P. Age-specific incidence and prevalence of keratoconus: a nationwide registration study. Am. J. Ophthalmol. 175 , 169–172 (2017).
Article PubMed Google Scholar
Nielsen, K., Hjortdal, J., Aagaard Nohr, E. & Ehlers, N. Incidence and prevalence of keratoconus in Denmark. Acta Ophthalmol. Scand. 85 , 890–892 (2007).
Kymes, S. M., Walline, J. J., Zadnik, K. & Gordon, M. O. Collaborative longitudinal evaluation of keratoconus study, g. Quality of life in keratoconus. Am. J. Ophthalmol. 138 , 527–535 (2004).
Bak-Nielsen, S., Ramlau-Hansen, C. H., Ivarsen, A., Plana-Ripoll, O. & Hjortdal, J. A nationwide population-based study of social demographic factors, associated diseases and mortality of keratoconus patients in Denmark from 1977 to 2015. Acta Ophthalmol . 97 , 497–504 (2018).
Mas Tur, V., MacGregor, C., Jayaswal, R., O’Brart, D. & Maycock, N. A review of keratoconus: diagnosis, pathophysiology, and genetics. Surv. Ophthalmol. 62 , 770–783 (2017).
Gain, P. et al. Global survey of corneal transplantation and eye banking. JAMA Ophthalmol. 134 , 167–173 (2016).
Matthaei, M. et al. Changing indications in penetrating keratoplasty: a systematic review of 34 years of global reporting. Transplantation 101 , 1387–1399 (2017).
Chan, E. et al. Prevalence of keratoconus based on Scheimpflug imaging: The Raine Study. Ophthalmology https://doi.org/10.1016/j.ophtha.2020.08.020 (2020).
Hashemi, H., Khabazkhoob, M. & Fotouhi, A. Topographic keratoconus is not rare in an Iranian population: the Tehran Eye Study. Ophthalmic Epidemiol. 20 , 385–391 (2013).
Papali’i-Curtin, A. T. et al. Keratoconus prevalence among high school students in New Zealand. Cornea 38 , 1382–1389 (2019).
Tuft, S. J. et al. Keratoconus in 18 pairs of twins. Acta Ophthalmol. 90 , e482–e486 (2012).
Wang, Y., Rabinowitz, Y. S., Rotter, J. I. & Yang, H. Genetic epidemiological study of keratoconus: evidence for major gene determination. Am. J. Med. Genet. 93 , 403–409 (2000).
Article CAS PubMed Google Scholar
Woodward, M. A., Blachley, T. S. & Stein, J. D. The association between sociodemographic factors, common systemic diseases, and keratoconus: an analysis of a Nationwide Heath Care Claims Database. Ophthalmology 123 , 457–65 e2 (2016).
Bisceglia, L. et al. Linkage analysis in keratoconus: replication of locus 5q21.2 and identification of other suggestive Loci. Invest. Ophthalmol. Vis. Sci. 50 , 1081–1086 (2009).
Burdon, K. P. et al. Association of polymorphisms in the hepatocyte growth factor gene promoter with keratoconus. Invest Ophthalmol. Vis. Sci. 52 , 8514–8519 (2011).
Article CAS PubMed PubMed Central Google Scholar
Iglesias, A. I. et al. Cross-ancestry genome-wide association analysis of corneal thickness strengthens link between complex and Mendelian eye diseases. Nat. Commun. 9 , 1864 (2018).
Article PubMed PubMed Central CAS Google Scholar
Lu, Y. et al. Genome-wide association analyses identify multiple loci associated with central corneal thickness and keratoconus. Nat. Genet. 45 , 155–163 (2013).
Dyrlund, T. et al. Human cornea proteome: identification and quantitation of the proteins of the three main layers including epithelium, stroma and endothelium. Investigative Ophthalmol. Visual Sci. 54 (2013).
Tang, Y. G. et al. Genomewide linkage scan in a multigeneration Caucasian pedigree identifies a novel locus for keratoconus on chromosome 5q14.3-q21.1. Genet. Med. 7 , 397–405 (2005).
Choquet, H. et al. A multiethnic genome-wide analysis of 44,039 individuals identifies 41 new loci associated with central corneal thickness. Commun. Biol. 3 , 301 (2020).
Article PubMed PubMed Central Google Scholar
Liskova, P., Dudakova, L., Krepelova, A., Klema, J. & Hysi, P. G. Replication of SNP associations with keratoconus in a Czech cohort. PLoS ONE 12 , e0172365 (2017).
Devlin, B. & Roeder, K. Genomic control for association studies. Biometrics 55 , 997–1004 (1999).
Li, X. et al. A genome-wide association study identifies a potential novel gene locus for keratoconus, one of the commonest causes for corneal transplantation in developed countries. Hum. Mol. Genet 21 , 421–429 (2012).
Article PubMed CAS Google Scholar
McComish, B. J. et al. Association of Genetic Variation With Keratoconus. JAMA Ophthalmol. 38 , 174–181 (2019).
Bulik-Sullivan, B. K. et al. LD Score regression distinguishes confounding from polygenicity in genome-wide association studies. Nat. Genet. 47 , 291–295 (2015).
Gelse, K., Poschl, E. & Aigner, T. Collagens-structure, function, and biosynthesis. Adv. Drug Deliv. Rev. 55 , 1531–1546 (2003).
Young, B. B., Zhang, G., Koch, M. & Birk, D. E. The roles of types XII and XIV collagen in fibrillogenesis and matrix assembly in the developing cornea. J. Cell Biochem. 87 , 208–220 (2002).
Feneck, E. M., Lewis, P. N., Ralphs, J. & Meek, K. M. A comparative study of the elastic fibre system within the mouse and human cornea. Exp. Eye Res. 177 , 35–44 (2018).
Sun, M. et al. Collagen V is a dominant regulator of collagen fibrillogenesis: dysfunctional regulation of structure and function in a corneal-stroma-specific Col5a1-null mouse model. J. Cell Sci. 124 , 4096–4105 (2011).
De Paepe, A., Nuytinck, L., Hausser, I., Anton-Lamprecht, I. & Naeyaert, J. M. Mutations in the COL5A1 gene are causal in the Ehlers-Danlos syndromes I and II. Am. J. Hum. Genet 60 , 547–554 (1997).
PubMed PubMed Central Google Scholar
Klaassens, M. et al. Ehlers-Danlos arthrochalasia type (VIIA-B)-expanding the phenotype: from prenatal life through adulthood. Clin. Genet. 82 , 121–130 (2012).
Zou, Y. et al. Recessive and dominant mutations in COL12A1 cause a novel EDS/myopathy overlap syndrome in humans and mice. Hum. Mol. Genet. 23 , 2339–2352 (2014).
Delbaere, S. et al. Novel defects in collagen XII and VI expand the mixed myopathy/Ehlers-Danlos syndrome spectrum and lead to variant-specific alterations in the extracellular matrix. Genet. Med. 22 , 112–123 (2019).
Lucero, H. A. & Kagan, H. M. Lysyl oxidase: an oxidative enzyme and effector of cell function. Cell Mol. Life Sci. 63 , 2304–2316 (2006).
Kern, A., Eble, J., Golbik, R. & Kuhn, K. Interaction of type IV collagen with the isolated integrins alpha 1 beta 1 and alpha 2 beta 1. Eur. J. Biochem. 215 , 151–159 (1993).
Velling, T., Risteli, J., Wennerberg, K., Mosher, D. F. & Johansson, S. Polymerization of type I and III collagens is dependent on fibronectin and enhanced by integrins alpha(11)beta(1) and alpha(2)beta(1). J. Biol. Chem. 277 , 37377–37381 (2002).
Joseph, R., Srivastava, O. P. & Pfister, R. R. Differential epithelial and stromal protein profiles in keratoconus and normal human corneas. Exp. Eye Res. 92 , 282–298 (2011).
Jester, J. V. Corneal crystallins and the development of cellular transparency. Semin Cell Dev. Biol. 19 , 82–93 (2008).
Pei, Y., Reins, R. Y. & McDermott, A. M. Aldehyde dehydrogenase (ALDH) 3A1 expression by the human keratocyte and its repair phenotypes. Exp. Eye Res. 83 , 1063–1073 (2006).
Stagos, D., Chen, Y., Cantore, M., Jester, J. V. & Vasiliou, V. Corneal aldehyde dehydrogenases: multiple functions and novel nuclear localization. Brain Res. Bull. 81 , 211–218 (2010).
Townsend, A. J. et al. Selective protection by stably transfected human ALDH3A1 (but not human ALDH1A1) against toxicity of aliphatic aldehydes in V79 cells. Chem. Biol. Interact. 130-132 , 261–273 (2001).
Koppaka, V. et al. ALDH3A1 plays a functional role in maintenance of corneal epithelial homeostasis. PLoS One 11 , e0146433 (2016).
Kenchegowda, D., Harvey, S. A., Swamynathan, S., Lathrop, K. L. & Swamynathan, S. K. Critical role of Klf5 in regulating gene expression during post-eyelid opening maturation of mouse corneas. PLoS ONE 7 , e44771 (2012).
Diakiw, S. M., D’Andrea, R. J. & Brown, A. L. The double life of KLF5: Opposing roles in regulation of gene-expression, cellular function, and transformation. IUBMB Life 65 , 999–1011 (2013).
Dong, J. T. & Chen, C. Essential role of KLF5 transcription factor in cell proliferation and differentiation and its implications for human diseases. Cell Mol. Life Sci. 66 , 2691–2706 (2009).
Stephens, D. N. et al. The Ets transcription factor EHF as a regulator of cornea epithelial cell identity. J. Biol. Chem. 288 , 34304–34324 (2013).
Swamynathan, S. K. et al. Conditional deletion of the mouse Klf4 gene results in corneal epithelial fragility, stromal edema, and loss of conjunctival goblet cells. Mol. Cell Biol. 27 , 182–194 (2007).
Joseph, A. et al. Expression of CD34 and L-selectin on human corneal keratocytes. Invest Ophthalmol. Vis. Sci. 44 , 4689–4692 (2003).
Evans, R. A., Tian, Y. C., Steadman, R. & Phillips, A. O. TGF-beta1-mediated fibroblast-myofibroblast terminal differentiation-the role of Smad proteins. Exp. Cell Res. 282 , 90–100 (2003).
Li, B. et al. Knockdown of eIF3a ameliorates cardiac fibrosis by inhibiting the TGF-beta1/Smad3 signaling pathway. Cell Mol. Biol. (Noisy-le.-Gd.) 62 , 97–101 (2016).
CAS Google Scholar
Dong, Z. et al. Role of eIF3a in regulating cell cycle progression. Exp. Cell Res. 315 , 1889–1894 (2009).
De Keuckelaere, E., Hulpiau, P., Saeys, Y., Berx, G. & van Roy, F. Nanos genes and their role in development and beyond. Cell Mol. Life Sci. 75 , 1929–1946 (2018).
Saoncella, S. et al. Nuclear Akt2 opposes limbal keratinocyte stem cell self-renewal by repressing a FOXO-mTORC1 signaling pathway. Stem Cells 32 , 754–769 (2014).
Noordermeer, D. et al. The dynamic architecture of Hox gene clusters. Science 334 , 222–225 (2011).
Afshari, N. A. et al. Genome-wide association study identifies three novel loci in Fuchs endothelial corneal dystrophy. Nat. Commun. 8 , 14898 (2017).
Consortium, G. T. Human genomics. The Genotype-Tissue Expression (GTEx) pilot analysis: multitissue gene regulation in humans. Science 348 , 648–660 (2015).
Article CAS Google Scholar
Carnes, M. U., Allingham, R. R., Ashley-Koch, A. & Hauser, M. A. Transcriptome analysis of adult and fetal trabecular meshwork, cornea, and ciliary body tissues by RNA sequencing. Exp. Eye Res. 167 , 91–99 (2018).
Khaled, M. L. et al. Differential expression of coding and long noncoding RNAs in keratoconus-affected corneas. Invest Ophthalmol. Vis. Sci. 59 , 2717–2728 (2018).
Finucane, H. K. et al. Heritability enrichment of specifically expressed genes identifies disease-relevant tissues and cell types. Nat. Genet. 50 , 621–629 (2018).
Zhu, Z. et al. Integration of summary data from GWAS and eQTL studies predicts complex trait gene targets. Nat. Genet. 48 , 481–487 (2016).
Takahashi, K. & Yamanaka, S. Induction of pluripotent stem cells from mouse embryonic and adult fibroblast cultures by defined factors. Cell 126 , 663–676 (2006).
Tiwari, A. et al. KLF4 regulates corneal epithelial cell cycle progression by suppressing canonical TGF-beta signaling and upregulating CDK inhibitors P16 and P27. Invest Ophthalmol. Vis. Sci. 60 , 731–740 (2019).
Galvis, V. et al. Keratoconus: an inflammatory disorder? Eye (Lond.) 29 , 843–859 (2015).
Bohac, M. et al. Incidence and clinical characteristics of post LASIK ectasia: a review of over 30,000 LASIK cases. Semin. Ophthalmol. 33 , 869–877 (2018).
Ferdi, A. C. et al. Keratoconus natural progression: a systematic review and meta-analysis of 11 529 Eyes. Ophthalmology 126 , 935–945 (2019).
Richardson, A. J., Narendran, N., Guymer, R. H., Vu, H. & Baird, P. N. Blood storage at 4 degrees C-factors involved in DNA yield and quality. J. Lab Clin. Med. 147 , 290–294 (2006).
Sahebjada, S. et al. Association of the hepatocyte growth factor gene with keratoconus in an Australian population. PLoS ONE 9 , e84067 (2014).
Bycroft, C. et al. The UK Biobank resource with deep phenotyping and genomic data. Nature 562 , 203–209 (2018).
Simon, J. A. et al. Phenotypic predictors of response to simvastatin therapy among African-Americans and Caucasians: the Cholesterol and Pharmacogenetics (CAP) Study. Am. J. Cardiol. 97 , 843–850 (2006).
Rabinowitz, Y. S. Videokeratographic indices to aid in screening for keratoconus. J. Refract. Surg. 11 , 371–379 (1995).
Das, S. et al. Next-generation genotype imputation service and methods. Nat. Genet. 48 , 1284–1287 (2016).
McCarthy, S. et al. A reference panel of 64,976 haplotypes for genotype imputation. Nat. Genet. 48 , 1279–1283 (2016).
Purcell, S. et al. PLINK: a tool set for whole-genome association and population-based linkage analyses. Am. J. Hum. Genet. 81 , 559–575 (2007).
Price, A. L. et al. Principal components analysis corrects for stratification in genome-wide association studies. Nat. Genet. 38 , 904–909 (2006).
Zhan, X., Hu, Y., Li, B., Abecasis, G. R. & Liu, D. J. RVTESTS: an efficient and comprehensive tool for rare variant association analysis using sequence data. Bioinformatics 32 , 1423–1426 (2016).
Burdon, K. P. et al. Apparent autosomal dominant keratoconus in a large Australian pedigree accounted for by digenic inheritance of two novel loci. Hum. Genet. 124 , 379–386 (2008).
Burdon, K. P. et al. Association of open-angle glaucoma loci with incident glaucoma in the Blue Mountains Eye Study. Am. J. Ophthalmol. 159 , 31–36.e1 (2015).
Dimasi, D. P. et al. Genetic investigation into the endophenotypic status of central corneal thickness and optic disc parameters in relation to open-angle glaucoma. Am. J. Ophthalmol. 154 , 833–842.e2 (2012).
Fritsche, L. G. et al. A large genome-wide association study of age-related macular degeneration highlights contributions of rare and common variants. Nat. Genet. 48 , 134–143 (2016).
Anderson, C. A. et al. Data quality control in genetic case-control association studies. Nat. Protoc. 5 , 1564–1573 (2010).
Loh, P.-R. et al. Reference-based phasing using the Haplotype Reference Consortium panel. Nat. Genet. 48 , 1443–1448 (2016).
CAS PubMed PubMed Central Google Scholar
Auton, A. et al. A global reference for human genetic variation. Nature 526 , 68–74 (2015).
Chang, C. C. et al. Second-generation PLINK: rising to the challenge of larger and richer datasets. Gigascience 4 , 7 (2015).
Banda, Y. et al. Characterizing race/ethnicity and genetic ancestry for 100,000 subjects in the genetic epidemiology research on adult health and aging (GERA) cohort. Genetics 200 , 1285–1295 (2015).
Kvale, M. N. et al. Genotyping informatics and quality control for 100,000 subjects in the genetic epidemiology research on adult health and aging (GERA) cohort. Genetics 200 , 1051–1060 (2015).
Hoffmann, T. J. et al. Next generation genome-wide association tool: design and coverage of a high-throughput European-optimized SNP array. Genomics 98 , 79–89 (2011).
Hoffmann, T. J. et al. Design and coverage of high throughput genotyping arrays optimized for individuals of East Asian, African American, and Latino race/ethnicity using imputation and a novel hybrid SNP selection algorithm. Genomics 98 , 422–430 (2011).
Delaneau, O., Marchini, J. & Zagury, J. F. A linear complexity phasing method for thousands of genomes. Nat. Methods 9 , 179–181 (2012).
Howie, B., Fuchsberger, C., Stephens, M., Marchini, J. & Abecasis, G. R. Fast and accurate genotype imputation in genome-wide association studies through pre-phasing. Nat. Genet. 44 , 955–959 (2012).
Howie, B., Marchini, J. & Stephens, M. Genotype imputation with thousands of genomes. G3 1 , 457–470 (2011).
Howie, B. N., Donnelly, P. & Marchini, J. A flexible and accurate genotype imputation method for the next generation of genome-wide association studies. PLoS Genet. 5 , e1000529 (2009).
Marchini, J. & Howie, B. Genotype imputation for genome-wide association studies. Nat. Rev. Genet. 11 , 499–511 (2010).
Huang, L., Wang, C. & Rosenberg, N. A. The relationship between imputation error and statistical power in genetic association studies in diverse populations. Am. J. Hum. Genet. 85 , 692–698 (2009).
Ikram, M. A. et al. The Rotterdam Study: 2018 update on objectives, design and main results. Eur. J. Epidemiol. 32 , 807–850 (2017).
Ramdas, W. D. et al. Common genetic variants associated with open-angle glaucoma. Hum. Mol. Genet. 20 , 2464–2471 (2011).
Hysi, P. G. et al. Meta-analysis of 542,934 subjects of European ancestry identifies new genes and mechanisms predisposing to refractive error and myopia. Nat. Genet. 52 , 401–407 (2020).
Willer, C. J., Li, Y. & Abecasis, G. R. METAL: fast and efficient meta-analysis of genomewide association scans. Bioinformatics 26 , 2190–2191 (2010).
Magi, R. & Morris, A. P. GWAMA: software for genome-wide association meta-analysis. BMC Bioinform. 11 , 288 (2010).
Article Google Scholar
Benjamini, Y. & Hochberg, Y. Controlling the false discovery rate: a practical and powerful approach to multiple testing. J. R. Statistical Soci. Ser. B (Methodological) 57 , 289–300 (1995).
Bulik-Sullivan, B. et al. An atlas of genetic correlations across human diseases and traits. Nat. Genet. 47 , 1236–1241 (2015).
Zhang, Y., Qi, G., Park, J. H. & Chatterjee, N. Estimation of complex effect-size distributions using summary-level statistics from genome-wide association studies across 32 complex traits. Nat. Genet. 50 , 1318–1326 (2018).
Wood, A. R. et al. Defining the role of common variation in the genomic and biological architecture of adult human height. Nat. Genet. 46 , 1173–1186 (2014).
Dayem Ullah, A. Z., Lemoine, N. R. & Chelala, C. SNPnexus: a web server for functional annotation of novel and publicly known genetic variants (2012 update). Nucleic Acids Res. 40 , W65–W70 (2012).
MacArthur, J. et al. The new NHGRI-EBI Catalog of published genome-wide association studies (GWAS Catalog). Nucleic Acids Res. 45 , D896–D901 (2017).
Hao, X. D., Chen, P., Chen, Z. L., Li, S. X. & Wang, Y. Evaluating the association between keratoconus and reported genetic loci in a Han chinese population. Ophthalmic Genet. 36 , 132–136 (2015).
Westra, H. J. et al. Systematic identification of trans eQTLs as putative drivers of known disease associations. Nat. Genet. 45 , 1238–1243 (2013).
McRae, A. F. et al. Identification of 55,000 Replicated DNA Methylation QTL. Sci. Rep. 8 , 17605 (2018).
Segre, A. V. et al. Common inherited variation in mitochondrial genes is not enriched for associations with type 2 diabetes or related glycemic traits. PLoS Genet 6 , e1001058 (2010).
Subramanian, A. et al. Gene set enrichment analysis: a knowledge-based approach for interpreting genome-wide expression profiles. Proc. Natl Acad. Sci. USA 102 , 15545–15550 (2005).
Ratnapriya, R. et al. Retinal transcriptome and eQTL analyses identify genes associated with age-related macular degeneration. Nat. Genet. 51 , 606–610 (2019).
Zhu, Z. et al. Causal associations between risk factors and common diseases inferred from GWAS summary data. Nat. Commun. 9 , 224 (2018).
Yang, J., Lee, S. H., Goddard, M. E. & Visscher, P. M. GCTA: a tool for genome-wide complex trait analysis. Am. J. Hum. Genet. 88 , 76–82 (2011).
Download references
Acknowledgements
The publication of this article has been enabled by a grant from Moorfields Eye Charity (GR001317). UK Biobank: This research has been conducted using the UK Biobank Resource under Application Number 17615. UK Biobank was established by the Wellcome Trust medical charity, Medical Research Council, Department of Health, Scottish Government, and Northwest Regional Development Agency. It also had funding from the Welsh Assembly Government, British Heart Foundation, and Diabetes UK. UCL and Moorfields, London, UK: We are grateful to all the patients at Moorfields Eye Hospital who participated in this study. This study was funded by Moorfields Eye Charity and the Rosetrees Trust. We were supported by infrastructure and funding from the National Institute for Health Research Biomedical Research Centre at Moorfields Eye Hospital and UCL Institute of Ophthalmology. AED is supported by AED is supported by a UK Research and Innovation Future Leadership Fellowship, Moorfields Eye Charity, and Academy of Medical Sciences Springboard award. We thank Beverley Scott and Naushin Waseem for assistance with DNA extraction and DNA banking, and the High-Throughput Genomics Group at the Wellcome Trust Centre for Human Genetics (funded by Wellcome Trust grant reference 203141/Z/16/Z) for performing the genotyping on the discovery cohort. APK and NP are supported by Moorfields Eye Charity Career Development Fellowships. GERA, USA: We are grateful to the Kaiser Permanente Northern California members who have generously agreed to participate in the Kaiser Permanente Research Program on Genes, Environment, and Health. Support for participant enrollment, survey completion, and biospecimen collection for the RPGEH was provided by the Robert Wood Johnson Foundation, the Wayne and Gladys Valley Foundation, the Ellison Medical Foundation, and Kaiser Permanente Community Benefit Programs. Genotyping of the GERA cohort was funded by a grant from the National Institute on Aging, National Institute of Mental Health, and National Institute of Health Common Fund (RC2 AG036607). Data analyses were facilitated by National Eye Institute (NEI) grant R01 EY027004 (E.J.), National Institute of Diabetes and Digestive and Kidney Diseases grant R01 DK116738 (E.J.). The Los Angeles-affiliated cohort, USA: The authors gratefully acknowledge the support of The Eye Defects Research Foundation Inc. and NEI R01-09052. CAP study (control cohort) was supported by the National Institutes of Health grant U19 HL069757 from the National Heart, Lung, and Blood Institute; and grant UL1TR000124 from the National Center for Advancing Translational Sciences. In addition, this work was supported in part by the National Institutes of Health, National Eye Institute contract R01 EY009052, and by the National Center for Advancing Translational Sciences, CTSI grant UL1TR001881. Australia: The Australian replication case cohort was funded by a National Health and Medical Research Council (NHMRC) project grant 1104700 and Centre for Research Excellence 1023911. Control genotype data for this cohort were provided by the international AMD Genetics Consortium genotyped under The Center for Inherited Diseases Research (CIDR) Program contract number HHSN268201200008I. SM is supported by an NHMRC Fellowship and NHMRC grants 1150144, 1116360. Dr Burdon was supported by an NHMRC Senior Research Fellowship (1059954). King’s College London and St. Thomas Hospital, UK: M.J. Simcoe is a recipient of a Fight for Sight PhD studentship. K. Patasova is a recipient of a Fight for Sight PhD studentship. P.G. Hysi the recipient of a Fight for Sight ECI fellowship. P.G. Hysi and C.J. Hammond acknowledge the TFC Frost Charitable Trust Support for the KCL Department of Ophthalmology. The statistical analyses were run in King’s College London Rosalind HPC and Department of Twin Research and Genetic Epidemiology Linux clusters and server. The UK Biobank data was accessed as part of the UK Biobank projects 669 and 17615. Leeds University and Leeds Teaching Hospitals NHS Trust, UK: We thank the patients and relatives who participated in this project. This study was supported by grants SAC007 and SAC033 from the National Eye Research Centre, and LAF was supported by a PhD grant award from Fight For Sight. S.Siddiqui was supported by the MRC grant 408757. Thanks to Erica Di Martino for laboratory support. University of Melbourne, Royal Victorian Eye and Ear Hospital, Australia: This study was supported by the Australian National Health and Medical Research Council (NHMRC) project grant 1104700 and Senior Research Fellowship (1138585 to PNB), the Angior Family Foundation and a Lions Eye Foundation Fellowship (SS). The Centre for Eye Research Australia (CERA) receives Operational Infrastructure Support from the Victorian Government. The authors wish to thank all participants who took part in this Keratoconus study and made this work possible. The authors would also like to thank Eye Surgery Associates, Lindsay and Associates and Keratoconus Australia, as well as Mr Tony Ngo for assistance with subject recruitment. Charles University and General University Hospital in Prague, Czech Republic: PL, LD and PS were supported by UNCE/MED/007 and PROGRES1086, Q26/LF1 research programs of the Charles University and by grant GACR 20-19278S. We thank the participating patients and staff of the Cornea service from the Department of Ophthalmology, General University Hospital in Prague for assistance with recruitment. The Erasmus Medical Center: The authors are grateful to Barbara Obermayer-Pietsch, Arthur A Bergen, and Andre G. Uitterlinden for their tremendous help throughout this project. The study was supported by the Combined Ophthalmic Research Rotterdam (CORR) grant.
Author information
These authors jointly supervised this work: Alison J. Hardcastle, Eric Jorgenson, Paul N. Baird, Yaron S. Rabinowitz, Kathryn P. Burdon, Chris J. Hammond, Stephen J. Tuft, Pirro G. Hysi.
These authors contributed equally: Petra Liskova, Yelena Bykhovskaya, Bennet J. McComish, Alice E. Davidson, Chris F. Inglehearn.
Authors and Affiliations
UCL Institute of Ophthalmology, London, UK
Alison J. Hardcastle, Petra Liskova, Alice E. Davidson, Nikolas Pontikos, Karla E. Rojas Lopez, Anthony P. Khawaja & Stephen J. Tuft
Moorfields Eye Hospital, NHS Foundation Trust, London, UK
Alison J. Hardcastle, Anthony P. Khawaja & Stephen J. Tuft
Department of Paediatrics and Inherited Metabolic Disorders, First Faculty of Medicine, Charles University and General University Hospital in Prague, Prague, Czech Republic
Petra Liskova & Lubica Dudakova
Department of Ophthalmology, First Faculty of Medicine, Charles University and General University Hospital in Prague, Prague, Czech Republic
Petra Liskova & Pavlina Skalicka
The Cornea Eye Institute, Beverly Hills, CA, USA
Yelena Bykhovskaya & Yaron S. Rabinowitz
Department of Surgery and Board of Governors Regenerative Medicine Institute, Cedars-Sinai Medical Center, Los Angeles, CA, USA
Menzies Institute for Medical Research, University of Tasmania, Hobart, TAS, Australia
Bennet J. McComish, Sionne E. M. Lucas, Jac C. Charlesworth, Alex W. Hewitt, Jamie E. Craig & Kathryn P. Burdon
Division of Molecular Medicine, Leeds Institute of Medical Research, University of Leeds, Leeds, UK
Chris F. Inglehearn, Manir Ali, Salina Siddiqui, Aine Rice, Layal Abi Farraj & Carmel Toomes
Institute for Translational Genomics and Population Sciences, The Lundquist Institute for Biomedical Innovation (formerly Los Angeles Biomedical Research Institute) at Harbor-UCLA Medical Center; Department of Pediatrics, Harbor-UCLA Medical Center, Torrance, CA, USA
Xiaohui Li, Yii-Der Ida Chen, Kent D. Taylor & Jerome I. Rotter
Division of Research, Kaiser Permanente Northern California, Oakland, CA, USA
Hélène Choquet, Jie Yin, Ronald B. Melles & Eric Jorgenson
Department of Ophthalmology, Erasmus Medical Center GD, Rotterdam, The Netherlands
Mahmoud Habeeb, Bart T. H. Van Dooren, Magda A. Meester-Smoor, Caroline C. W. Klaver & Wishal D. Ramdas
Department of Epidemiology, Erasmus Medical Center GD, Rotterdam, The Netherlands
Mahmoud Habeeb, Magda A. Meester-Smoor & Caroline C. W. Klaver
Centre for Eye Research Australia, Royal Victorian Eye and Ear Hospital, East Melbourne, VIC, Australia
Srujana Sahebjada, Andrea J. Richardson, Ke Cao, Elsie Chan & Mark D. Daniell
Department of Surgery, Ophthalmology, University of Melbourne, Royal Victorian Eye and Ear Hospital, East Melbourne, VIC, Australia
Srujana Sahebjada, Ke Cao, Elsie Chan, Mark D. Daniell & Paul N. Baird
NIHR Biomedical Research Centre, Moorfields Eye Hospital, London, UK
Anthony P. Khawaja
Amphia Hospital, Breda, The Netherlands
Bart T. H. Van Dooren
The Rotterdam Eye Hospital, Rotterdam, The Netherlands
Annette J. M. Geerards
Division of Endocrinology and Diabetology, Endocrinology Lab Platform, Department of Internal Medicine, Medical University of Graz, Graz, Austria
Christoph W. Haudum
Department of Ophthalmology, University Medical Center Groningen (UMCG), Groningen, the Netherlands
Valeria Lo Faro
Department of Ophthalmology, Academic Medical Center, Amsterdam, The Netherlands
Vision Eye Institute, Melbourne, VIC, Australia
Abi Tenen & Alex W. Hewitt
School of Primary and Allied Health Care, Monash University, Melbourne, VIC, Australia
Melbourne Stem Cell Centre, Melbourne, VIC, 3800, Australia
Section of Ophthalmology, School of Life Course Sciences, King’s College London, London, UK
Mark J. Simcoe, Karina Patasova, David O’Brart, Chris J. Hammond & Pirro G. Hysi
Department of Twin Research and Genetic Epidemiology, King’s College London, London, UK
Mark J. Simcoe, Karina Patasova, Darioush Yarrand, Chris J. Hammond & Pirro G. Hysi
Department of Ophthalmology, St James’s University Hospital, Leeds, UK
Salina Siddiqui
UCL Great Ormond Street Hospital Institute of Child Health, London, UK
Jugnoo S. Rahi & Pirro G. Hysi
Oakland Research Institute, Oakland, CA, USA
Ronald M. Krauss & Elisabeth Theusch
Department of Ophthalmology, Case Western Reserve University, Cleveland, OH, USA
Loretta Szczotka-Flynn & Sudha K. Iyengar
Centre for Vision Research, Department of Ophthalmology, Westmead Institute for Medical Research, University of Sydney, Westmead, NSW, Australia
Paul A. Mitchell
The Jules Stein Institute, University of California Los Angeles, Los Angeles, CA, USA
Anthony J. Aldave
Department of Ophthalmology, Flinders University, Adelaide, SA, Australia
Richard A. Mills, Jamie E. Craig & Kathryn P. Burdon
Health Services and Systems Research, Duke-NUS Medical School, Singapore, Singapore
Jie Jin Wang
QIMR Berghofer Medical Research Institute, Brisbane, QLD, Australia
Stuart MacGregor
St Thomas Hospital, Guy’s and St. Thomas NHS Trust, London, London, UK
David O’Brart & Chris J. Hammond
You can also search for this author in PubMed Google Scholar
Contributions
A.J.H., P.L., S.J.T., and P.G.H. conceived the project; A.J.H., Y.B., B.J.M., A.E.D., C.F.I., X.L., H.C., M.H., S.E.M.L., S.Sahebjada, N.P., K.E.R.L., M.A., L.D., P.S., B.T.H.V.D., A.J.M.G., C.W.H., V.L.F., A.T., M.J.S., K.P., D.Y., J.Y., S.Sidiqui, A.R., L.A.F., Y-D.I.C.,J.S.R., R.M.K., E.T., J.C.C., L.S-F., C.T., M.A.M-S., A.J.R., P.A.M., K.D.T., R.B.M., A.J.A, R.A.M., K.C., E.C., M.D.D., J.J.W., J.I.R., A.W.H., S.M., C.C.W.K., W.D.R., J.E.C., S.K.I., D.O., E.J., P.N.B., Y.S.R., and K.P.B. were responsible for collecting clinical data and performing genotyping in each study; A.J.H., C.J.H., P.G.H. drafted the paper; A.J.H., Y.B., C.J.H., A.P.K., C.J.H., S.J.T., and P.G.H. critically reviewed the manuscript.
Corresponding authors
Correspondence to Alison J. Hardcastle , Stephen J. Tuft or Pirro G. Hysi .
Ethics declarations
Competing interests.
H.C. is an Editorial Board Member for Communications Biology, but was not involved in the editorial review of, nor the decision to publish, this article. The authors declare the following competing interests: D.O. holds non-commercial research grants with Rayner Inc and Avedro Inc, holds stock in Sparca Inc. All other authors declare no competing interests.
Additional information
Publisher’s note Springer Nature remains neutral with regard to jurisdictional claims in published maps and institutional affiliations.
Supplementary information
Supplementary information, description of additional supplementary files, supplementary data 1-14, supplementary data 15, supplementary data 16, supplementary data 17, supplementary data 18, supplementary data 19, reporting summary, rights and permissions.
Open Access This article is licensed under a Creative Commons Attribution 4.0 International License, which permits use, sharing, adaptation, distribution and reproduction in any medium or format, as long as you give appropriate credit to the original author(s) and the source, provide a link to the Creative Commons license, and indicate if changes were made. The images or other third party material in this article are included in the article’s Creative Commons license, unless indicated otherwise in a credit line to the material. If material is not included in the article’s Creative Commons license and your intended use is not permitted by statutory regulation or exceeds the permitted use, you will need to obtain permission directly from the copyright holder. To view a copy of this license, visit http://creativecommons.org/licenses/by/4.0/ .
Reprints and permissions
About this article
Cite this article.
Hardcastle, A.J., Liskova, P., Bykhovskaya, Y. et al. A multi-ethnic genome-wide association study implicates collagen matrix integrity and cell differentiation pathways in keratoconus. Commun Biol 4 , 266 (2021). https://doi.org/10.1038/s42003-021-01784-0
Download citation
Received : 06 July 2020
Accepted : 02 February 2021
Published : 01 March 2021
DOI : https://doi.org/10.1038/s42003-021-01784-0
Share this article
Anyone you share the following link with will be able to read this content:
Sorry, a shareable link is not currently available for this article.
Provided by the Springer Nature SharedIt content-sharing initiative
This article is cited by
Genetic variants in the foxo1 and znf469 genes are associated with keratoconus in sweden: a case-control study.
- Wolf Wonneberger
- Bertil Sterner
- Anna Zettergren
BMC Ophthalmology (2024)
Targeted next-generation sequencing analysis in Italian patients with keratoconus
- Marco Lombardo
- Umberto Camellin
- Domenico Schiano Lomoriello
A multi-ancestry GWAS of Fuchs corneal dystrophy highlights the contributions of laminins, collagen, and endothelial cell regulation
- Bryan R. Gorman
- Michael Francis
- Sudha K. Iyengar
Communications Biology (2024)
Keratoconus International Consortium (KIC)- advancing keratoconus research
- Srujana Sahebjada
- Paul N. Baird
BMC Ophthalmology (2023)
Contribution of common and rare variants to Asian neovascular age-related macular degeneration subtypes
- Hengtong Li
- Ching-Yu Cheng
Nature Communications (2023)
By submitting a comment you agree to abide by our Terms and Community Guidelines . If you find something abusive or that does not comply with our terms or guidelines please flag it as inappropriate.
Quick links
- Explore articles by subject
- Guide to authors
- Editorial policies
Sign up for the Nature Briefing newsletter — what matters in science, free to your inbox daily.

Advances in the diagnosis and treatment of keratoconus
Affiliations.
- 1 Department of Ophthalmology, Medical School, Eskişehir Osmangazi University, Meşelik Kampüsü, Odunpazarı, Eskişehir 26040, Turkey.
- 2 Department of Ophthalmology, Medical School, Eskişehir Osmangazi University, Eskişehir, Turkey.
- PMID: 34263132
- PMCID: PMC8246497
- DOI: 10.1177/25158414211012796
Keratoconus had traditionally been considered a rare disease at a time when the imaging technology was inept in detecting subtle manifestations, resulting in more severe disease at presentation. The increased demand for refractive surgery in recent years also made it essential to more effectively detect keratoconus before attempting any ablative procedure. Consequently, the armamentarium of tools that can be used to diagnose and treat keratoconus has significantly expanded. The advances in imaging technology have allowed clinicians and researchers alike to visualize the cornea layer by layer looking for any early changes that might be indicative of keratoconus. In addition to the conventional geometrical evaluation, efforts are also underway to enable spatially resolved corneal biomechanical evaluation. Artificial intelligence has been exploited in a multitude of ways to enhance diagnostic efficiency and to guide treatment. As for treatment, corneal cross-linking treatment remains the mainstay preventive approach, yet the current main focus of research is on increasing oxygen availability and developing new strategies to improve riboflavin permeability during the procedure. Some new combined protocols are being proposed to simultaneously halt keratoconus progression and correct refractive error. Bowman layer transplantation and additive keratoplasty are newly emerging alternatives to conventional keratoplasty techniques that are used in keratoconus surgery. Advances in tissue engineering and regenerative therapy might bring new perspectives for treatment at the cellular level and hence obviate the need for invasive surgeries. In this review, we describe the advances in the diagnosis and treatment of keratoconus primarily focusing on newly emerging approaches and strategies.
Keywords: advances; diagnosis; keratoconus; treatment.
© The Author(s), 2021.
Publication types
- Basic & Translational Research
- Comprehensive
- Cornea/Ocular Surface
- Imaging & Diagnostics
- Neuro-ophthalmology
- Oculoplastics
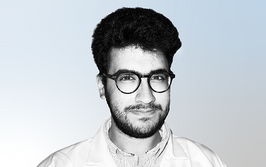
- AI: Enhancing the Human Touch
- Targeting the Supraciliary Space in Glaucoma Treatment
- Exploring the Interventional Glaucoma Mindset
- AI-Powered Glaucoma Risk Stratification
- The CorNeat EverPatch: Superior Alternative to Tissue Grafts
- Rehabilitating Vision After Keratoconus
- Professional Development
- Business and Innovation
- Health Economics and Policy
- Education and Training
- Practice Management

- Also in the News…
- Paying the LQpay Way
- The Year 2035 and the 30 Percent Deficit
- Rising Star: Sally L. Baxter
- Nobility’s True Badge
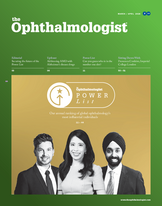
The Ophthalmologist Power List 2024 is Live!
Different Strokes
- February 2024
- December 2023
- October 2023
- August 2023
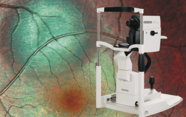
- Uncover the Unique DNA of SPECTRALIS®
- Less Steps, More Vision
- Sulcus-based enhancement of visual quality
- THE NEW FEMTO Z8 NEO
- New: Precizon Presbyopic IOL for the correction of presbyopia in astigmatism
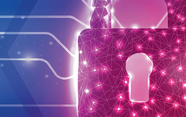
- AI that Empowers: Deeper Diagnostic Insights into Geographic Atrophy and Other Ophthalmic Diseases
- Seek (with AI) and You Shall Find: Case Study
- TrabEx Pro: The Next Level in MIGS
- Driving Forward Dry Eye Diagnosis
- Different Methods, Similar Outcomes: Case Study
- relevant and personalised updates about your field
- access to Case Studies/product profiles
- digital magazine subscription
Corneal Cross-Linking: What’s New in 2023?
A quick tour of the latest breakthroughs in keratoconus treatment
Farhad Hafezi | 06/28/2023 | 7 min read | News
One fascinating aspect of ophthalmology is its rapid pace of transformation. In the past decade, we have witnessed remarkable advancements in treating diseases that once had limited therapeutic options. Keratoconus (KC) is no exception, and I would like to share some of the newest developments in the field. Perhaps the biggest change is that we have moved beyond solely trying to address disease progression. For over two decades, ophthalmologists have had the rightly famous “Dresden protocol” method (1) for performing corneal cross-linking (CXL). Epithelium removal, saturation of the stroma with 0.1% riboflavin, followed by 30 minutes of 3 mW/cm² ultraviolet-A (UV-A) irradiation strengthens the cornea and slows, or, more often, halts the progression of the ectasia. But this is slow. In theory, increasing the UV intensity with modern LED UV light sources should decrease the time needed to deliver the total UV energy (fluence), and accelerate the cross-linking process. But for many years, in practice, we couldn’t accelerate CXL without losing a certain degree of strengthening efficacy (2). We discovered almost a decade ago that if you try to accelerate the delivery of the Dresden protocol fluence (5.4 J/cm²), you rapidly run out of oxygen, which turns out to be an essential component of the UV-riboflavin photochemical reaction (3). However, there is good news: our research group developed a new epithelium (epi)-off protocol that is fast and just as strong as Dresden. We now have a 10 J/cm² protocol that requires 9 minutes 15 seconds of UV irradiation; three times faster than Dresden and equally strong (4). This is the protocol I use most in children, in adolescents, or in cases where I need a strong cross-linking effect. This is undeniably good news – we have already published the early rounds of laboratory data and we’re preparing to publish 2-year follow-up clinical data to further validate our initial findings. Another area that has shown promising progress is the treatment of ultra-thin corneas. As you’re well aware, to protect the corneal endothelium Dresden protocol CXL isn’t performed on corneas thinner than 400 µm. So what do you do with an extremely thin, ectatic cornea? Let’s revisit the two protocols we’ve relied on in the past. Our group in Zurich introduced the use of hypo-osmolar riboflavin to cause corneal swelling (5), and a few years later, Soosan Jacob proposed the use of a riboflavin-soaked specialized contact lens for these thin corneas (6). Neither artificial thickening technique is ideal. Swelling the cornea might give good results from a biomechanical point of view, but some corneas swell lots and others don’t at all. In other words, you always risk facing a surprise in the OR. If there’s one thing ophthalmologists don’t enjoy, it’s that. Contact-lens assisted CXL resulted in a ~30% loss of the cross-linking strengthening effect. But something about artificially thickening the cornea doesn’t make sense. I feel like I’m telling the patient, “My technique can only do this, so please adapt you and your condition to my technique.” Why can’t I do the opposite and adapt the technique to the cornea? I think you’ll agree this makes much more sense… Asking this question played a pivotal role in the development of the sub400 individualized cross-linking approach. The algorithm underpinning this approach was published six years ago (7), and serves as the foundation for our protocol. It’s used to calculate the reduction in fluence required to safely cross-link thinner corneas. The clinical validation of this approach is published in the American Journal of Ophthalmology , and provides ophthalmologists with a simple, standardized and reliable framework to cross-link thin corneas (8). A critical step in this process occurs after the corneal abrasion and riboflavin introduction – the measurement of corneal thickness using an ultrasound pachymeter. By analyzing the resulting topographic map, the location of the thinnest point becomes evident. Suppose, for instance, the thinnest point measures 280 µm. All clinicians need to do is look up our publication, which we have made accessible to everyone via the Light for Sight Foundation’s website, and see how many minutes of UV irradiation are required for the measured corneal thickness. Using the 280 µm thickness example, the ophthalmologist needs to deliver 5 minutes of UV irradiation at 3 mW/cm². It works like a charm and is an integrated cross-linking protocol in the C-eye cross-linking device. Next, let's delve into epi-on cross-linking. The Dresden protocol requires epithelial debridement, as simple riboflavin solutions would not otherwise pass and enter the stroma. However, this brings significant downsides, including patient discomfort and an elevated risk of infection. This requires patients be diligently managed after the procedure until the epithelial cells have regrown and the roughly 8-mm hole made at the start of the procedure is closed. How can we achieve both an intact epithelium and a robust cross-linking effect? Can we eliminate the need for corneal abrasion? Others have used penetration enhancers (to weaken the epithelium intra-cell tight junctions) or iontophoresis (to electrostatically force riboflavin across the epithelial cells) to get the riboflavin to the cornea, and supplemental oxygen to compensate for the oxygen absorbed by the epithelium that remains present. We finally found a way without depending upon either oxygen goggles or iontophoresis to make epi-on work.

Figure 1. The multiphoton microscope with me and Emilio Torres-Netto
How? Throughout the entire COVID-19 pandemic, we were working away at the multiphoton microscope at the Swiss Federal Institute of Technology (Figure 1). We found a way to not only get rid of the supplemental oxygen requirement, but also the need for iontophoresis. So now we have no abrasion, no increased risk of infection, and no need for extra oxygen or iontophoresis. This breakthrough, after ten years of research, was like putting the pieces of a puzzle together. We are now at the point where we can use atmospheric air – no supplementary oxygen required. We use what we call “second-generation” penetration enhancers, which allow the riboflavin to penetrate the epithelium, and a special pulsed, slow irradiation, high-fluence cross-linking protocol. According to our published laboratory data, we are as efficient in strengthening the cornea as the 10 minute / 9 mW/cm² accelerated epi-off CXL protocol. The last topic to mention here is the evolution of second-generation customized keratoconus treatment. As I’m sure readers will know, seven years ago, the initial research on customized cross-linking was published by the group of Theo Seiler Sr. (9). The data was extremely interesting, with progressive flattening of up to nearly 3 D occurring over the months after the procedure. This was achieved by delivering UV irradiation with a device that incorporated an eye tracker and delivered different irradiation intensities to different zones of the cornea. This resulted in increased irradiation over the cone, and progressively less irradiation moving outward. Unfortunately, the technique requires a bulky, pricey and stationary device, and so our team wondered: can we do better? Enter PACE: phototherapeutic keratectomy (PTK)-Assisted Customized Epi-on cross-linking. We have achieved almost twice the flattening effect as first-generation customized CXL, and we do this by combining CXL with an epithelial map-guided PTK. And as you can see in the images in Figure 2, the results are extremely promising. The refraction is essentially stable, we have a flattening of almost 5 D, a coupling effect, steepening of 2 D. So 5 + 2 is a regularization of 7 D in just 2 weeks, and accordingly, visual acuity increases; note how symmetric the cornea topography is over the pupil area (Figure 2).

Figure 2. Before and after PACE; note the symmetrical corneal topography, which greatly increases visual acuity
How did we do this? We did not use an eye tracker. We used a mixture of epi-off and epi-on cross-linking (Figure 3). We performed a small epithelial map driven PTK just over the tip of the cone, and then we put riboflavin onto the cornea to enhance penetration through the epi-off area. This created both epi-off and epi-on areas, and this results in a riboflavin gradient between these areas. This gradient is very useful, because we can treat a single cornea and achieve different cross-linking efficacies in different areas, thanks to the riboflavin gradient and epithelium status. By flattening the cone so much, you drive patients towards hypermetropia, so we find that patients who are myopic – roughly in the range of 53–55 D – are excellent candidates for PACE technology. We are still finalizing and refining the technique, but I am happy to confirm that we see a rapid, mean flattening of approximately 5 D, without issue. With these results and this cornea strengthening technique, then this raises the possibility of performing a wavefront-guided photorefractive keratectomy (PRK) 6–12 months later, if needed. Currently, we are focused on determining the stabilization timeframe. How rapidly do the results manifest? When does stabilization commence? Once the corneal topography is stable, wavefront-guided PRK interventions become possible. As we continue to refine and perfect these new techniques (along with the technology to implement them correctly) we can expect one more change: even better outcomes for our patients.

Figure 3. Customized corneal cross-linking with PACE
When you click “Subscribe” we will email you a link, which you must click to verify the email address above and activate your subscription. If you do not receive this email, please contact us at [email protected] . If you wish to unsubscribe, you can update your preferences at any point.
- Wollensak G, Spoerl E, Seiler T. Riboflavin/ultraviolet-a-induced collagen crosslinking for the treatment of keratoconus. Am J Ophthalmol. 2003;135(5):620-627. PMID: 12719068
- Hammer A, Richoz O, Arba Mosquera S, Tabibian D, Hoogewoud F, Hafezi F. Corneal biomechanical properties at different corneal cross-linking (Cxl) irradiances. Invest Ophthalmol Vis Sci. 2014;55(5):2881-2884. PMID: 24677109
- Torres-Netto EA, Kling S, Hafezi N, Vinciguerra P, Randleman JB, Hafezi F. Oxygen diffusion may limit the biomechanical effectiveness of iontophoresis-assisted transepithelial corneal cross-linking. J Refract Surg. 2018;34(11):768-774. PMID: 30428097.
- Abrishamchi R, Abdshahzadeh H, Hillen M, et al. High-fluence accelerated epithelium-off corneal cross-linking protocol provides Dresden protocol-like corneal strengthening. Transl Vis Sci Technol. 2021;10(5):10. PMID: 34542574.
- Hafezi F, Mrochen M, Iseli HP, Seiler T. Collagen crosslinking with ultraviolet-A and hypoosmolar riboflavin solution in thin corneas. J Cataract Refract Surg. 2009;35(4):621-624. PMID: 19304080.
- Jacob S, Kumar DA, Agarwal A, Basu S, Sinha P, Agarwal A. Contact lens-assisted collagen cross-linking (Cacxl): A new technique for cross-linking thin corneas. J Refract Surg. 2014;30(6):366-372. PMID: 24972403.
- Kling S, Hafezi F. An algorithm to predict the biomechanical stiffening effect in corneal cross-linking. J Refract Surg. 2017;33(2):128-136. PMID: 28192592
- Hafezi F, Kling S, Gilardoni F, et al. Individualized corneal cross-linking with riboflavin and UV-A in ultrathin corneas: the sub400 protocol. Am J Ophthalmol. 2021;224:133-142. PMID: 33340508
- Seiler TG, Fischinger I, Koller T, Zapp D, Frueh BE, Seiler T. Customized corneal cross-linking: one-year results. Am J Ophthalmol. 2016;166:14-21. PMID: 26944278.
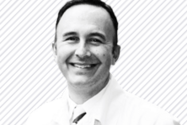
Farhad Hafezi is a Professor of Ophthalmology at the University of Geneva, Switzerland; Research Group Leader at the CABMM of the University of Zurich, Switzerland; Chief Medical Officer of the ELZA Institute, Zurich, Switzerland; Adjunct Clinical Professor of Ophthalmology at the USC Roski Eye Institute, Los Angeles, USA; Research Professor at the NYU Grossman School of Medicine, New York, and Visiting Professor at the Wenzhou Medical University, Wenzhou, China.
More articles by Farhad Hafezi
11/23/2023 | Contributed by OCULUS | 4 min read
07/26/2023 | Contributed by OCULUS | 4 min read
09/11/2020 | Contributed by Quidel
06/23/2021 | Contributed by Ziemer
11/16/2020 | Contributed by ESW Vision
11/02/2020 | Contributed by Rayner
01/16/2024 | Sponsored by Zeiss
10/18/2023 | Sponsored by Glaukos
06/07/2022 | Sponsored by Zepto
Access our product directory to see the latest products and services from our industry partners
05/07/2024 | Alun Evans | 5 min read
05/09/2024 | Sahil Syed | 3 min read
05/13/2024 | Alun Evans | 5 min read
05/06/2024 | Alice T. Epitropoulos, Darrell White | 4 min read
04/22/2024 | Sponsored by Espansione Group | 4 min read
Register to access our FREE online portfolio, request the magazine in print and manage your preferences.
- Unlimited access to ALL articles
- News, interviews & opinions from leading industry experts
- Receive print (and PDF) copies of The Ophthalmologist magazine
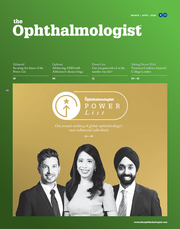
The Ophthalmologist website is intended solely for the eyes of healthcare professionals. Please confirm below:
Masks Strongly Recommended but Not Required in Maryland, Starting Immediately
Due to the downward trend in respiratory viruses in Maryland, masking is no longer required but remains strongly recommended in Johns Hopkins Medicine clinical locations in Maryland. Read more .
- Vaccines
- Masking Guidelines
- Visitor Guidelines
Wilmer Eye Institute
Keratoconus research.
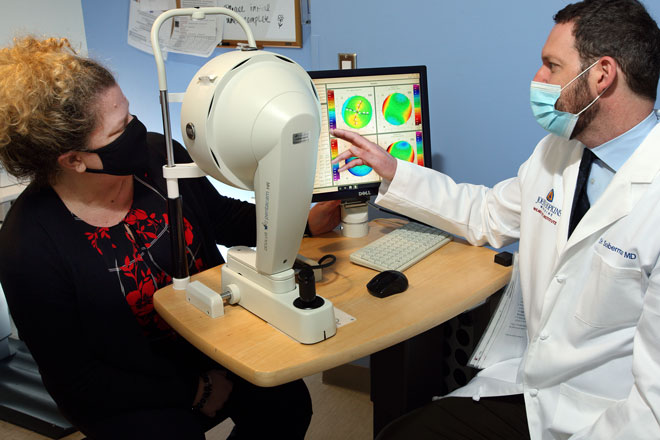
The causes of keratoconus and underlying pathogenic changes are poorly understood. The keratoconus research team, comprised of some of the most accomplished experts in research and clinical care, are trying to change that. Basic science discoveries are being translated into increased understanding of the origins and progression of the disease, and exploration of new treatments and therapies.
Areas of Focus
Our keratoconus researchers are currently focused on:
- Establishing a keratoconus patient database with clinical information including disease severity, associated medical problems, and family history
- Identifying novel therapies for keratoconus through the study of biological pathways that govern the outermost layer of the cornea (epithelium)
- Investigating the importance of mild, chronic inflammation as a cause of keratoconus
- Identifying genes and testing DNA associated with keratoconus using new methods of genetic analysis
- Developing and testing new treatments for keratoconus
- Developing cell culture models of keratoconus to specifically study candidate gene functions
Read more about our current research.
Our Faculty
Michelle hessen, o.d.,.
Assistant Professor of Ophthalmology
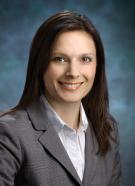
Uri Soiberman, M.D.
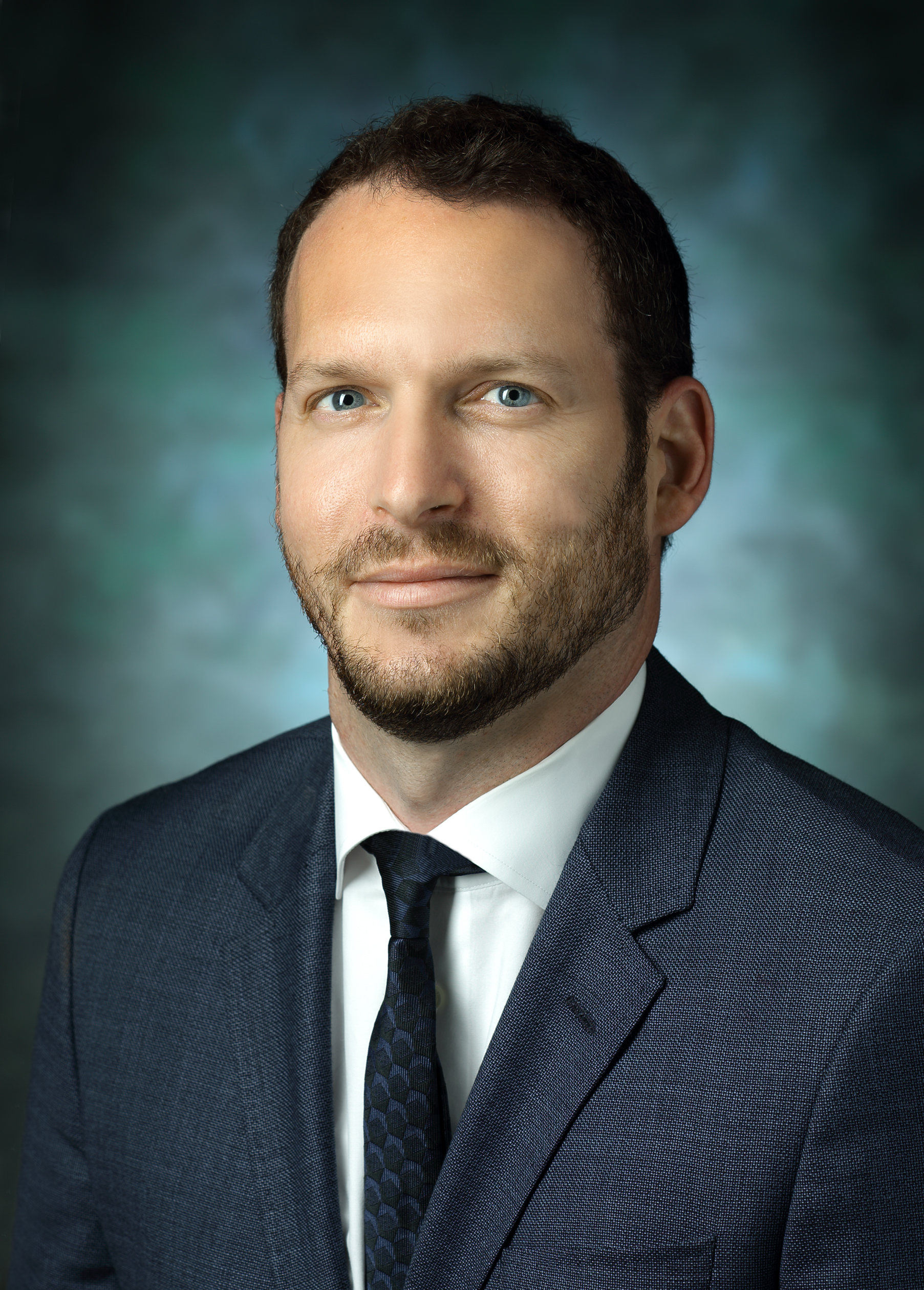
Keratoconus Treatments
Learn more about the current treatment and procedure options available for early and advanced stages of keratoconus.
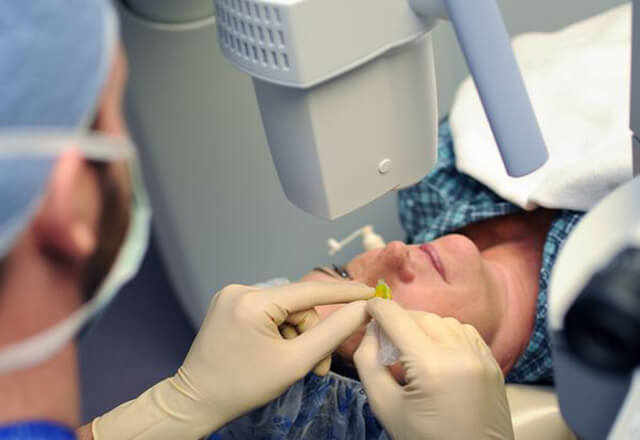
Support Wilmer Research
Donate online to partner with us on our quest to find the next treatments and cures.
Over the Phone
Speak with someone about making a charitable gift to Wilmer. Please call 410-955-2020 .
Download our giving form to mail in your generous donation.
SYSTEMATIC REVIEW article
Utility of artificial intelligence in the diagnosis and management of keratoconus: a systematic review.
- Bascom Palmer Eye Institute, Miller School of Medicine, University of Miami, Miami, FL, United States
Introduction: The application of artificial intelligence (AI) systems in ophthalmology is rapidly expanding. Early detection and management of keratoconus is important for preventing disease progression and the need for corneal transplant. We review studies regarding the utility of AI in the diagnosis and management of keratoconus and other corneal ectasias.
Methods: We conducted a systematic search for relevant original, English-language research studies in the PubMed, Web of Science, Embase, and Cochrane databases from inception to October 31, 2023, using a combination of the following keywords: artificial intelligence, deep learning, machine learning, keratoconus, and corneal ectasia. Case reports, literature reviews, conference proceedings, and editorials were excluded. We extracted the following data from each eligible study: type of AI, input used for training, output, ground truth or reference, dataset size, availability of algorithm/model, availability of dataset, and major study findings.
Results: Ninety-three original research studies were included in this review, with the date of publication ranging from 1994 to 2023. The majority of studies were regarding the use of AI in detecting keratoconus or subclinical keratoconus (n=61). Among studies regarding keratoconus diagnosis, the most common inputs were corneal topography, Scheimpflug-based corneal tomography, and anterior segment-optical coherence tomography. This review also summarized 16 original research studies regarding AI-based assessment of severity and clinical features, 7 studies regarding the prediction of disease progression, and 6 studies regarding the characterization of treatment response. There were only three studies regarding the use of AI in identifying susceptibility genes involved in the etiology and pathogenesis of keratoconus.
Discussion: Algorithms trained on Scheimpflug-based tomography seem promising tools for the early diagnosis of keratoconus that can be particularly applied in low-resource communities. Future studies could investigate the application of AI models trained on multimodal patient information for staging keratoconus severity and tracking disease progression.
1 Introduction
1.1 keratoconus.
Keratoconus is a progressive corneal ectasia characterized by stromal thinning and corneal steepening, ranging in severity from asymptomatic subclinical disease to severe corneal scarring requiring corneal transplantation for visual rehabilitation ( 1 ). As the condition progresses, patients may experience decreased visual acuity, photophobia, and image distortion ( 1 ). A meta-analysis study reported that younger patients and patients with a baseline maximum keratometry steeper than 55 D were more likely to experience disease progression ( 2 ). Patients with a medical history involving allergic eye disease or atopic conditions were also more likely to experience keratoconus progression ( 3 ). The pathophysiology of keratoconus involves reduced keratocyte density, loss of stromal lamellae with fibroblast degradation, redistribution of collagen, and increased proteolysis leading to a breakdown in structural integrity ( 4 ). This condition most commonly occurs between the second and third decade of life and has an estimated global prevalence of 1.38 per 1000 individuals ( 5 , 6 ). The global prevalence of keratoconus varies across populations but has been previously estimated to range between 0.2-4790 per 100,000 individuals, with the lowest reported in Russia ( 5 ). The highest prevalence rates have been reported in Asian and Middle Eastern communities ( 5 ).
Multiple ancillary testing modalities have been used in conjunction with clinical examination for the diagnosis of keratoconus. Placido-based videokeratoscopy and ultrasonic central pachymetry were previously used in the diagnosis and severity staging of keratoconus ( 7 ). Modern Placido disc-based corneal topography devices are still popular, as they provide information about corneal curvature, surface irregularities, and aberrations by generating color-coded maps ( 1 , 8 ). More recently, Scheimpflug-based corneal tomography imaging has allowed for greater analysis of the cornea by generating three-dimensional representations of the anterior segment to provide information about corneal thickness as well as the anterior and posterior cornea ( 7 , 9 ). Corneal tomography is now the gold standard method for corneal ectasia diagnosis, and the Pentacam® (OCULUS, Arlington, WA, USA) is one of the most common Scheimpflug-based corneal tomography devices ( 9 , 10 ). Another adjunct imaging technology used for evaluation of corneal ectasias is anterior-segment optical coherence tomography (AS-OCT), which generates cross-sectional corneal images that can be used to identify asymmetry in corneal thinning, posterior curvature, and epithelial/total corneal thickness via epithelial mapping ( 1 ). Corneal biomechanics, including corneal hysteresis and deformation amplitude, can also be used to diagnose early stages of keratoconus through an association with central corneal thickness ( 11 ).
Early keratoconus diagnosis is important for successful management and prevention of disease progression ( 12 ). Corneal cross-linking (CXL) is a procedure developed in 2003 that promotes bond formation between corneal collagen fibrils using riboflavin and ultraviolet-A light, which has demonstrated excellent long-term efficacy in reducing progression of keratoconus but has a threshold of procedural safety for the thinnest pachymetry value that can be treated ( 12 ). As CXL alone does not significantly improve visual outcomes, early detection and disease management can also allow for enhanced visual rehabilitation of patients without requiring keratoplasty, including options of hard contact lenses, intracorneal ring segment implantation, intraocular collamer lens implantation, and CXL combined with laser ablative procedures (e.g. photorefractive keratectomy) ( 12 ).
1.2 Models of artificial intelligence
The application of artificial intelligence (AI) in the diagnosis and management of ophthalmic diseases has been rapidly increasing since the 1970s ( 13 ). AI associates a particular outcome with variables of different weights, and trained models can be applied in establishing disease diagnosis, determining management, and predicting the prognosis of patients ( 14 ). Machine learning (ML) is a subset of AI that uses a training dataset for tasks but does not require programming ( 15 ). Features from the input data, which may include imaging and patient demographic information, are used to form a feature vector which then serves as the starting point for the ML model ( 16 ). Supervised ML is trained on a set of inputs with correct outcome labels available ( 17 ). In contrast, unsupervised ML models receive inputs without outcome labels ( 18 ). ML techniques include logistic regression, decision tree, random forest, support vector machine, and multilayer perceptron ( Table 1 ) ( 15 , 16 ).
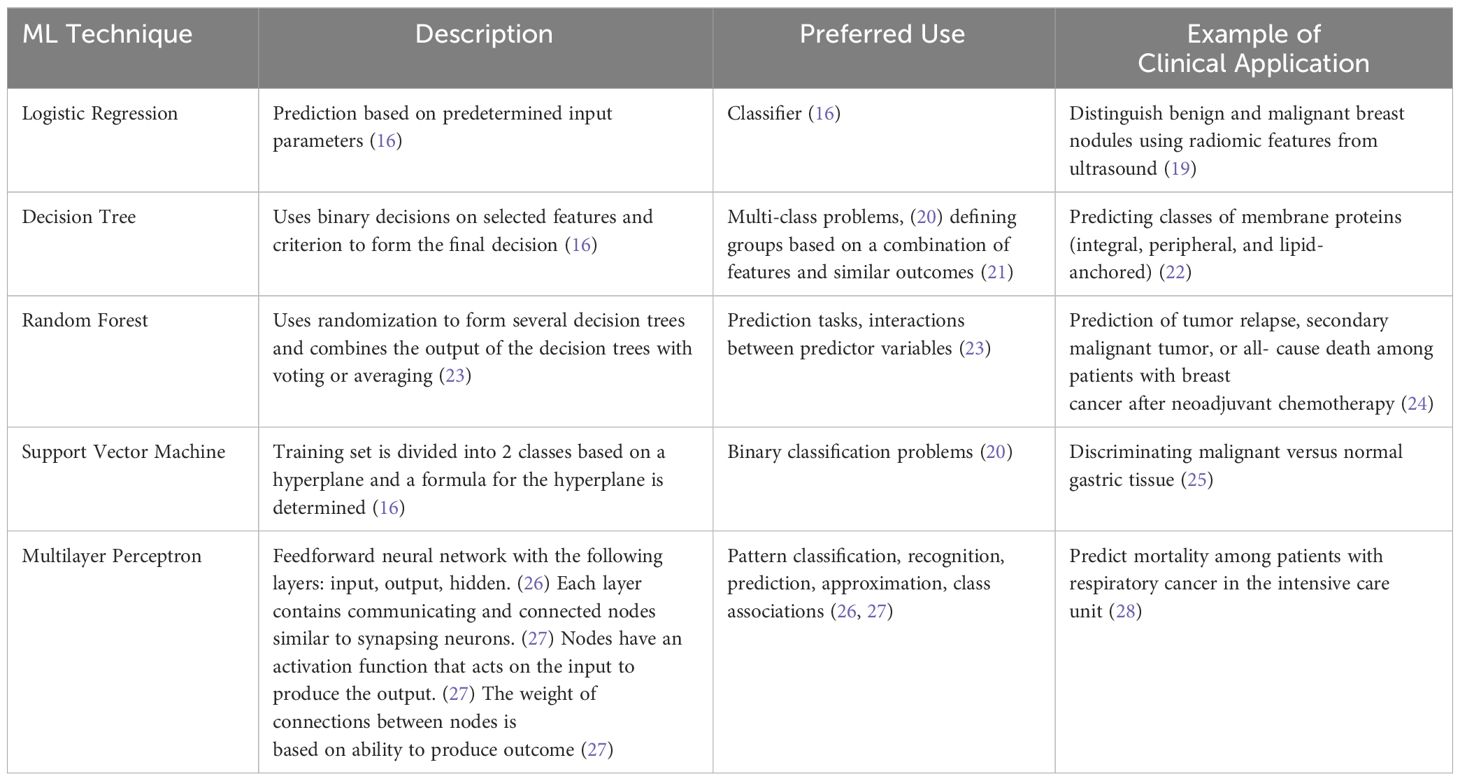
Table 1 Description, use, and examples of machine learning techniques in medical literature.
Deep learning (DL) is a subset of machine learning that includes convolutional neural networks and does not require manual feature extraction from the research team ( 15 , 29 ). DL models are composed of neural network layers that represent operations, and the output of one layer serves as the input of the next layer ( 30 ). Convolutional neural networks consist of convolution layers, pooling layers, and a fully connected network layer ( 16 ). Back propagation, normalization of input, dropout, and residual networks may be used to reduce error, reduce overfitting, and improve training ( 16 ).
The application of AI models is evaluated through several measures, which most commonly include accuracy, sensitivity, specificity, and area under the receiver operating curve (AUC or AUROC). The output of AI models in the majority of included studies is a class prediction (i.e. keratoconus versus healthy). Accuracy is the proportion of predictions that are true positives and negatives among all predictions. Sensitivity of a class is the proportion of true positives among all predictions of that class. Specificity describes the rate of true negatives ( 31 ). AUC ranges from 0 to 1 and is the area under the curve of the function modeled by sensitivity and 1-specificity. This provides a measure of diagnostic accuracy, with higher scores (closer to 1) representing greater accuracy ( 32 ).
1.3 Ophthalmic applications of artificial intelligence
The application of AI models in ophthalmology has been widely studied, particularly for posterior segment diseases including diabetic retinopathy, glaucoma, age-related macular degeneration, and retinopathy of prematurity (ROP) ( 33 , 34 ). Ng et al. reported that AI algorithms for diabetic retinopathy may be closest to application in clinical medicine ( 33 ). DL systems are able to detect diabetic retinopathy, predict disease progression, and predict diabetic macular edema using fundus images ( 33 ). There also exist DL algorithms to detect glaucoma and predict progression using fundus photographs, optical coherence tomography, and Humphrey visual fields ( 33 ). AI systems are particularly useful for detecting ROP from fundus imaging given that there is grading variation among experts, and some low-resource countries have heavy disease burden with limited access to specialist care ( 33 ). DL algorithms have demonstrated high AUC, sensitivity, and specificity for identifying retinopathy of prematurity requiring further management ( 33 ).
There has also been an increase in reports evaluating the application of AI systems in detecting anterior segment diseases, including keratoconus, infectious keratitis, cataract, and pterygium using anterior segment photographs and AS-OCT images ( 35 ). AI has also been used to screen patients for post-corneal transplant rejection as well as to grade cataracts ( 35 ). However, several studies regarding the application of AI in anterior segment disease diagnosis and management were limited by a small sample size with low heterogeneity ( 35 ).
As shown, clinical utilization of AI models in ophthalmology has rapidly increased in the past 7 years since the first reports of DL systems trained for screening of retinal pathologies ( 36 , 37 ). Due to the greater variety of anterior segment imaging and variable use in evaluating different conditions, widespread adoption of ML/DL algorithms for corneal pathology has not yet occurred. However, as imaging-based evaluation of keratoconus has now become standard of care due to improved technology, this study aims to provide a systematic review on the current state of utilizing AI and ML/DL platforms in the diagnosis, evaluation, management, and prognosis of keratoconus and corneal ectasias.
2 Methods of literature search
2.1 search strategy.
Using the Preferred Reporting Items for Systematic reviews and Meta-Analyses (PRISMA) framework as a guide, a systematic review technique was used to evaluate studies describing artificial intelligence and keratoconus. The search strategy was created based on the population, interventions, comparators, outcomes, and study design (PICOS) architecture, resulting in the study question: “Is artificial intelligence a sensitive and specific tool for the diagnosis and management of keratoconus or other corneal ectasias compared to clinical diagnosis and management led by ophthalmologists?” The systematic search was conducted using the PubMed, Web of Science, Embase, and Cochrane databases from inception to October 31, 2023, to select full-length, English articles in peer-reviewed journals. The MeSH keywords included in the search strategy were keratoconus, corneal ectasia, artificial intelligence, machine learning, and deep learning, with all combinations of these terms searched.
2.2 Inclusion and exclusion criteria
Original, English-language research articles published in peer-reviewed journals regarding the use of any AI, ML or DL model in the evaluation of the diagnosis, pathophysiology, severity, clinical progression, or evaluation of the response to management of keratoconus and other corneal ectasias were included in this study. Case reports, editorials, commentaries, conference abstracts, and literature reviews were excluded. Non-English-language articles were also excluded. Publications were not restricted by year. Figure 1 displays PRISMA diagram of the study selection methodology ( 38 ).
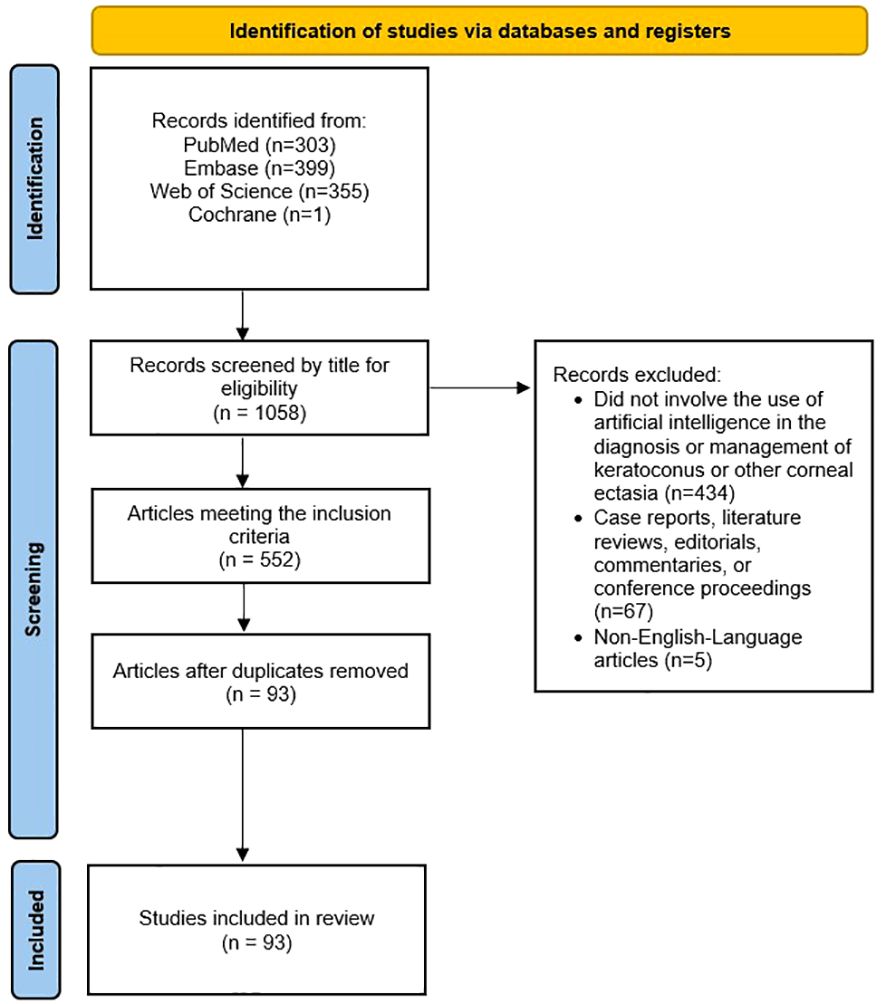
Figure 1 Preferred Reporting Items for Systematic Reviews and Meta-Analyses (PRISMA) diagram of the study selection methodology for this review.
2.3 Data extraction
The following variables were collected for each included study:
● Type of artificial intelligence
● Input used for training
● Output
● Ground truth or reference standard
● Dataset size (number of images, eyes, and patients, when applicable and available)
● Availability of algorithm/model
● Source and availability of dataset
● Major study findings
2.4 Quality assessment
The Quality Assessment of Diagnostic Accuracy Studies-2 (QUADAS-2) tool was used to assess the risk of bias and applicability concerns of studies regarding the diagnosis, severity, and clinical grading of keratoconus and other corneal ectasias ( 39 ). The Quality Assessment of Prognostic Accuracy Studies (QUAPAS) tool was used to assess the risk of bias and applicability concerns of studies regarding the prediction of disease progression and response to treatment/management ( 40 ). A quality assessment for studies regarding the etiology and pathogenesis was not completed due to the absence of a validated assessment tool for this type of study.
The search strategy yielded 93 original research studies. These studies are summarized in Table 2 , and we discuss some key studies in the following Results section. There were no concerns regarding the applicability of any studies given the broad nature of our review question and strict application of the inclusion and exclusion criteria.
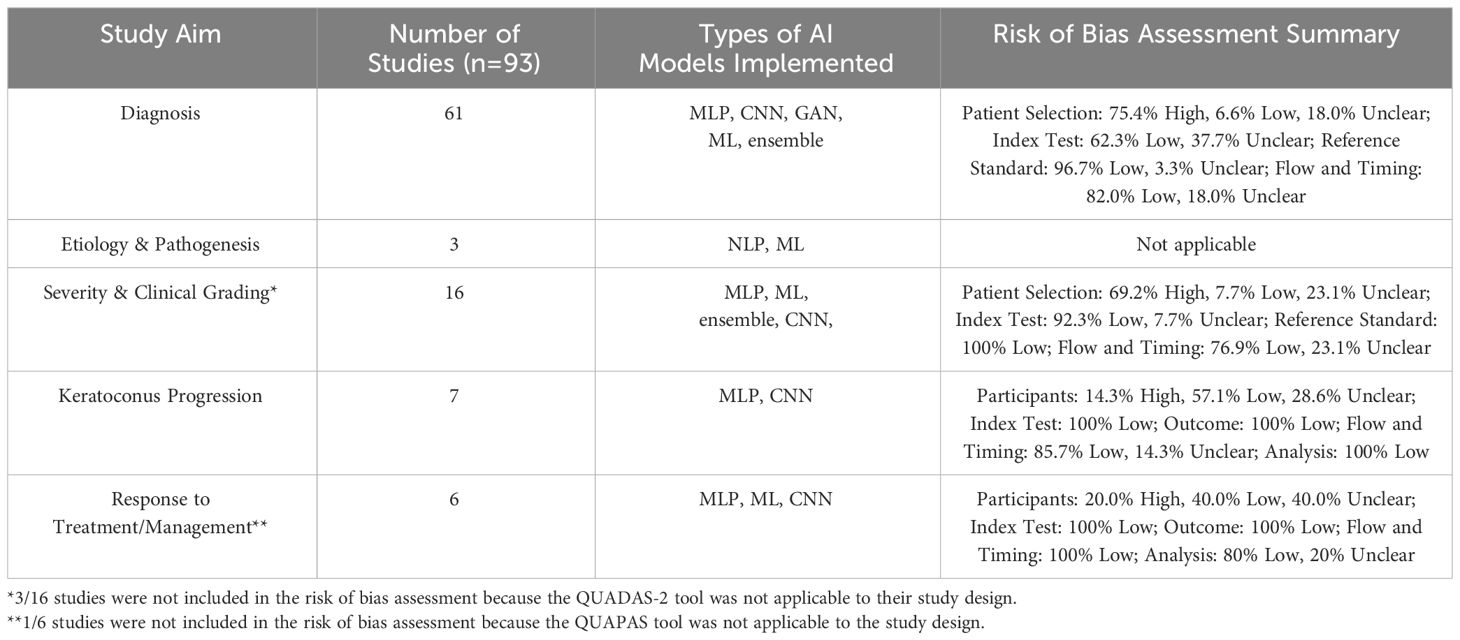
Table 2 Overview of the number of studies, types of AI models implemented for each study aim, and quality assessment of studies included in this review. The types of AI models include multilayer perceptron (MLP), convolutional neural network (CNN), generative adversarial network (GAN), traditional machine learning (ML), ensemble, and natural language processing (NLP).
3.1 Diagnosis of keratoconus
Among the included studies, AI was most frequently used for the diagnosis of keratoconus and other ectasias, particularly in subclinical cases (n = 61, Supplementary Table 1 ) ( 31 , 41 – 100 ). The earliest study using artificial intelligence to detect keratoconus was published in 1994 by Maeda et al. ( 65 ) This study used eight indices from TMS-1 videokeratoscope data (Computed Anatomy Inc., New York City, NY, USA) to detect keratoconus among a set of eyes with normal corneas and corneas with various diagnoses ( 65 ). The linear discriminant function generated a Keratoconus Prediction Index value that was used to classify eyes as “keratoconus” or “nonkeratoconus” based on a cutoff value ( 65 , 100 ). The model demonstrated sensitivities of 100% and 89% with three false-positives and one false-positive in the training and validation set, respectively ( 65 ). More recently, Silverman et al. evaluated the use of stepwise linear discriminant analysis and neural networks in detecting keratoconus using corneal epithelial and stromal thickness maps from Artemis 1® (StarFish Medical, Victoria, BC, Canada) very high frequency ultrasound arc-scans ( 96 ). Eyes with keratoconus were reported to have focal epithelial and stromal thinning with a surrounding ring of epithelial thickening ( 96 , 101 ).
The majority of the included studies regarding keratoconus or corneal ectasia diagnosis with AI used data from corneal topography ( 47 , 51 – 53 , 56 , 57 , 67 , 73 , 74 , 77 , 78 , 83 , 91 , 92 , 94 , 100 ), Scheimpflug-based tomography ( 43 , 45 , 49 , 54 , 55 , 59 , 60 , 62 , 64 , 66 , 70 , 76 , 79 , 80 , 84 , 86 – 88 , 90 , 95 , 99 ), or optical coherence tomography (OCT) ( 31 , 46 , 50 , 53 , 68 , 69 , 72 , 80 , 84 ) as the input. For example, de Almeida et al. utilized 52 parameters from Pentacam® (OCULUS, Arlington, WA, USA) tomography to generate the Corneal Tomography Multivariate Index (CTMVI) using paraconsistent feature engineering and a support vector machine classifier ( 90 ). CTMVI was then used to discriminate between very asymmetric ectasia with normal topography and healthy corneas. When using these 52 features, CTMVI demonstrated a sensitivity, specificity, and AUC of 0.844, 0.874, and 0.926, respectively ( 90 ). After combining CTMVI and the Pentacam Random Forest Index, the model demonstrated a sensitivity and specificity of 0.99 and 0.84, respectively. Other input for AI-based keratoconus or corneal ectasia diagnosis included data from corneal deformation videos ( 42 , 55 , 71 , 97 ), air-puff tonometry ( 50 ), corneal endothelial images from specular microscopy ( 81 ), and lateral segment photographs ( 93 ).
Beyond diagnosing subclinical keratoconus or keratoconus, Ahn et al. demonstrated that AI can be used to screen patients in a primary care setting that may need further evaluation with corneal topography for keratoconus. Using input parameters of subjective visual impairment (based on a patient survey), visual acuity, intraocular pressure, and autokeratometry parameters, they compared the performance of five previously-reported AI models ( 102 – 106 ). The ensemble model with soft voting method demonstrated superior performance with this task with a sensitivity of 90.5% and 96.4% in the internal and external test datasets, respectively ( 44 ). Intraocular pressure and mean corneal power were the most highly ranked in the feature importance analysis ( 44 ). A soft voting ensemble classifier has been previously used to predict major adverse cardiovascular events among patients with acute coronary system ( 107 ).
In the absence of a large dataset of eyes with keratoconus, Lavric et al. and Subramanian et al. used SyntEyes KTC to generate a sufficiently large set of corneal topography images for model training ( 57 , 97 ). The SyntEyes KTC model was developed by Rozema et al. using Scheimpflug tomography, ocular biometry, and wavefront data of 145 eyes with keratoconus ( 108 ). Abdelmotaal et al. used a conditional generative adversarial network (CGAN) called pix2pix to produce images of keratoconus eyes ( 95 ). The pix2pix CGAN is composed of a Generator that uses the input image to form the output image, and a Discriminator that determines the similarity of the generated image to an image from the original dataset or an image from the Generator ( 95 ). The authors reported that the model produced subjectively and objectively plausible images of keratoconus, early keratoconus, and normal eyes ( 95 ). They trained a VGG-16 deep convolutional neural network on combinations of original images and images synthesized by the pix2pix CGAN. The VGG-16 model trained with all original images and synthesized images demonstrated the highest accuracy of 99.56% in discriminating early keratoconus, keratoconus, and normal eyes ( 95 ).
3.2 Etiology & pathogenesis of keratoconus
Only three studies have investigated the utility of AI in exploring the genetic etiology and mechanical pathogenesis of keratoconus ( Supplementary Table 2 ) ( 109 – 111 ). Hosoda et al. conducted a genome-wide association study (GWAS) of central corneal thickness using IBM’s Watson for Drug Discovery AI technology. They found the STON2 rs2371597 and SMAD3 rs12913547 loci to be involved in keratoconus development. STON2 and SMAD3 have roles in extracellular matrix (ECM) remodeling, so these variants may contribute to the stromal ECM changes described in keratoconus ( 109 , 112 ). The authors noted that additional GWAS may be used to identify pathways driving keratoconus development ( 109 ). Wang et al. identified 8 differentially expressed genes (AREG, BBC3, DUSP2, MAP3K8, SMAD7, CDKN1A, JUN, and LIF) between patients with and without keratoconus using the random forest model, support vector machine model, and generalized linear model ( 110 ). These genes may affect cell mitosis (AREG), macrophage dysfunction (BBC3), cell cycle arrest (CDKN1A), apoptosis (DUSP2, CDKN1A), and proliferation, differentiation, and death (JUN) ( 110 ). The authors concluded that abnormal cell proliferation, differentiation, and autophagy pathways may be involved in keratoconus development ( 110 ).
Given the reported association between mechanical eye rubbing and pathogenesis of keratoconus, Nokas et al. built a wrist-mounted sensor that used an accelerometer, gyroscope, and machine learning algorithms to detect eye rubbing activity and remind the user to cease such activity ( 111 ). With limited AI-based studies on this topic, future research could further investigate susceptibility genes and utility of behavior modification in keratoconus pathogenesis.
3.3 Severity & clinical grading of keratoconus
Sixteen original research articles identified in this review assessed severity or other clinical features of patients with keratoconus ( Supplementary Table 3 ) ( 104 , 113 – 127 ). Among studies evaluating keratoconus severity, the ground truth included staging based on topography findings ( 104 , 113 , 118 , 120 , 125 , 127 ), tomography findings ( 116 ), the Ectasia Screening Index ( 114 ), Keratoconus Severity Index ( 119 ), and Amsler-Krumeich criteria ( 120 , 121 , 126 ). Chen et al. compared six convolutional neural network models trained with one or a combination of four color-coded corneal tomography maps (axial, anterior elevation, posterior elevation, and pachymetry) as well as a majority voting strategy model to predict the presence and stage of keratoconus ( 121 ). The model trained with all four maps demonstrated the best AUC in distinguishing healthy from keratoconus eyes ( 121 ). Interestingly, the majority voting model and the model using the back elevation map demonstrated the highest AUC for discriminating healthy and stage 1 keratoconus eyes, as well as stage 1 and stage 2 keratoconus eyes, respectively ( 121 ).
Dong et al. and Dos Santos et al. both used deep learning models to segment corneal OCT scans ( 122 , 123 ). Dong et al. used a corneal segmentation algorithm to measure the thickness of epithelial and stromal tissue with an error of less than 4 microns ( 123 ). The OCT images were taken by the Optovue RTVUE 100 device (Optovue, Inc., Fremont, CA, USA). They reported that as keratoconus progressed, total corneal thickness decreased, particularly temporal and inferior to the pupil center ( 123 ). Epithelial thickness also decreased as keratoconus severity increased ( 123 ). However, keratoconic eyes with stromal scarring demonstrated a larger epithelial thickness with irregular variations ( 123 ). Dos Santos et al. developed CorneaNet, a neural network to segment OCT images of eyes with and without keratoconus and produce thickness maps of the epithelium, Bowman layer, and stroma ( 122 ). The image scans were acquired on a custom-built, ultra-high resolution OCT system ( 128 ). CorneaNet achieved an accuracy of 99.56% with this task, but the authors noted that accuracy may be limited by network architecture, image noise, as well as insufficient and incorrect training data ( 122 ).
3.4 Keratoconus progression
Seven studies investigated AI-based prediction of disease progression in eyes with keratoconus ( Supplementary Table 4 ) ( 129 – 135 ). The majority of these studies only provided binary categorization of keratoconus eyes as progressive or nonprogressive. Kundu et al. developed two AI models, based on either tomographic changes or clinical risk factors, to identify risk factors underlying keratoconus progression and label patients with either likely disease “progression” or “no progression.” ( 129 ) They found that elevated serum immunoglobin E (IgE), systemic allergies, eye rubbing, and serum vitamin D level were important characteristics in the evaluation for the risk of keratoconus progression ( 129 ). Patients categorized into the progression group had a significantly higher serum IgE compared to those categorized into the no progression group ( 129 ). Eye rubbing may also be due to ocular irritation, fatigue, and stress ( 129 ). Eye rubbing reduces keratocyte density and modifies intraocular pressure, which can contribute to keratoconus development ( 129 ). The results of this study were limited in their generalizability given that the study was based only on an Asian Indian group of patients ( 129 ).
Alternatively, Kamiya et al. used a deep learning model to predict keratoconus progression with color-coded maps from AS-OCT ( 133 ). They reported that after adjusting for age, the accuracy of their algorithm improved from 0.794 to 0.849 ( 133 ). However, this study was limited by the absence of external validation and confirmation of repeatability, small sample size, possibly inaccurate keratoconus diagnosis, and the effect of contact lenses ( 133 ).
3.5 Response to treatment/management
Six studies featured the use of AI in characterizing the response to different treatment modalities, such as by predicting postoperative outcomes or the need for future intervention ( Supplementary Table 5 ) ( 136 – 141 ). Both Valdés-Mas et al. and Lyra et al. employed machine learning to predict postoperative refractive outcomes after intracorneal ring implantation, including corneal curvature, astigmatism, asphericity, and keratometry ( 138 , 140 ). Liu et al. utilized machine learning to predict postoperative visual acuity and keratometry two years after corneal crosslinking ( 139 ). While these studies demonstrate the potential of AI models to aid in disease prognostication after different treatments, validation studies are necessary prior to their widespread generalization and adoption.
4 Discussion
Keratoconus is a corneal ectatic disease that can be diagnosed through several imaging modalities, including corneal topography and tomography ( 1 ). Furthermore, early detection of keratoconus and other corneal ectasias with prompt management can help slow disease progression and reduce the risk for vision loss or need for a corneal transplant ( 12 ). Therefore, AI systems that can process images and generate predictions may be used to reduce the rate of missed or delayed diagnoses, thereby improving patient outcomes. We conducted a systematic review to evaluate the state of AI systems that have been applied in the diagnosis and management of keratoconus and other corneal ectasias among adult patients.
We found that the majority of original research studies involving the clinical application of AI algorithms among patients with keratoconus are based on disease diagnosis. The most current and highest impact studies in the field include Al-Timemy et al., Tan et al., Ambrosio et al., Kuo et al., Kamiya et al., and Chen et al. ( 42 , 52 , 72 , 82 , 88 , 121 ) The majority of these studies used a convolutional neural network AI model, which is a DL method that does not require manual feature extraction from the research team unlike ML algorithms ( 15 , 29 ). Most of these studies also used Scheimpflug-based corneal tomography scans or indices as their model’s input. This may be explained by the ability of Scheimpflug-based corneal tomography to provide additional information about the posterior cornea, which has been shown to exhibit ectatic changes earlier than the anterior cornea in keratoconus, allowing for earlier diagnosis ( 142 ). Additionally, the Belin ABCD classification system (A: anterior radius of curvature, B: back (posterior) radius of curvature, C: minimal corneal thickness, and D: best spectacle distance visual acuity) was developed to monitor disease progression evident on the posterior corneal surface in the absence of anterior corneal surface changes ( 142 ). Given that the aforementioned studies demonstrated relatively high diagnostic accuracy of DL algorithms trained on tomography input, further refinement and validation of AI systems evaluating information from Scheimpflug-based tomography may be adopted in clinical practice to streamline the identification of patients with subclinical keratoconus that may benefit from close monitoring for progression to clinical disease. Additionally, a mobile-based application with this technology can be used to develop a screening program for subclinical keratoconus and other corneal ectasia in low-resource countries.
AI models have been less commonly used in other contexts, such as identifying genetic susceptibility and predicting disease progression. Unlike disease diagnosis, these contexts may rely on model input that involves more subjective elements, such as demographic, environmental risk factors, and other clinical factors. For example, Kundu et al. recently developed an AI model using the random forest algorithm to predict progressive keratoconus using clinical and ocular surface risk factors determined from a patient questionnaire ( 129 ). Their system demonstrated an AUC of 0.81, and 76% of cases classified as progressive by an AI model trained on tomographic changes were also classified as progressive by the clinical risk factors AI model ( 129 ). Within each nondiagnostic context, the AI systems demonstrated relative variability in their performance in completing classification or prediction tasks, and further research is needed to draw conclusions regarding the application of AI models in nondiagnostic contexts for keratoconus. In the future, validated AI models trained on environmental and clinical risk factors could be particularly useful in predicting disease progression to identify patients at risk for severe disease. These identified patients may potentially benefit from prophylactic corneal crosslinking to strengthen corneal integrity and reduce risk of progression.
Some studies included in this review were limited by their design. For example, only one specialist determined the presence of keratoconus for the reference standard of some diagnostic accuracy studies, including Almeida Jr. et al., Lucena et al., Lopes et al., Chandapura et al., Cohen et al., Zéboulon et al., Mosa et al., and Zaki et al. ( 45 , 47 , 49 , 53 , 54 , 75 , 87 , 93 ) This could have resulted in biased classification of eyes. Additionally, some studies included a relatively low sample size of study groups, which reduced the power of the study. Ahn et al. included only 69, 39, and 43 patients in the subclinical keratoconus study group in the training, internal, and external datasets, respectively ( 44 ). Other studies, such as Cohen et al., do not include parameters for demographic information, such as age, sex, or race/ethnicity which can affect the baseline corneal curvature and thickness ( 54 , 143 – 145 ).
The strengths of this study lie in the comprehensiveness of clinical contexts included in this systematic review. While previously published reviews regarding the application of AI in keratoconus and other corneal ectasia focus on diagnostic accuracy ( 146 – 149 ), we also included studies regarding grading disease severity, predicting disease progression, understanding etiology and pathogenesis, and predicting response to treatment or management. However, our review has several limitations. To begin with, except for nine studies published prior to 2013, the majority of studies included in our review were published within the last decade. This may be explained by the fact that the application of ML/DL in medicine, including ophthalmology, has grown in popularity more recently ( 150 ). Furthermore, we searched a limited number of medical databases, including PubMed, Embase, Web of Science, and Cochrane. Studies meeting our eligibility criteria but not indexed in these databases may have been excluded from this review. While we completed a qualitative data extraction among included studies, we were unable to conduct a meta-analysis with statistical methodology given the contextual and methodological variation between studies and their AI systems. Lastly, some studies included in this review were based on specific patient populations with low diversity, which decreases the generalizability of this review’s findings.
With the incorporation of ML/DL AI algorithms and advancements in corneal imaging technology, the potential ability of clinicians to detect and treat keratoconus at earlier stages to prevent disease progression is promising. AI can also be used to determine ectasia severity, identify susceptibility genes, categorize the keratoconus as progressive or non-progressive, and predict response to surgical management. These applications are particularly important for low-resource nations which may have a scarcity of cornea specialists. In these underserved areas, the incorporation of validated AI models could help reduce the rate of missed or delayed corneal ectasia diagnoses. For example, validated smartphone applications could allow for longitudinal at-home screening of keratoconus among patients with risk factors for the condition. Looking ahead, some clinical applications of AI include using multimodal patient data (a combination of corneal images, demographic information, and environmental risk factors) as the input to determine the keratoconus stage and track disease progression.
Additionally, AI can be used in the surgical planning of some therapeutic options for keratoconus that are growing in popularity, such as ray-tracing-guided transepithelial photorefractive keratectomy with accelerated crosslinking, which may help reduce refractive overcorrection and stromal tissue ablation ( 151 ). Mazzotta et al. used a tissue-preservation algorithm involving ray tracing among 38 patients with stable keratoconus undergoing this procedure ( 151 ). They reported that the algorithm and surgical treatment significantly improved visual outcomes in their cohort ( 151 ). This demonstrates that AI-based algorithms could personalize surgical planning to improve postoperative outcomes, so the implementation of these algorithms for other surgical procedures for visual rehabilitation in keratoconus patients should be investigated.
Data availability statement
The original contributions presented in the study are included in the article/ Supplementary Material . Further inquiries can be directed to the corresponding author.
Author contributions
DG: Investigation, Writing – original draft, Writing – review & editing. AYZ: Conceptualization, Methodology, Supervision, Writing – original draft, Writing – review & editing.
The author(s) declare that no financial support was received for the research, authorship, and/or publication of this article.
Conflict of interest
The authors declare that the research was conducted in the absence of any commercial or financial relationships that could be construed as a potential conflict of interest.
Publisher’s note
All claims expressed in this article are solely those of the authors and do not necessarily represent those of their affiliated organizations, or those of the publisher, the editors and the reviewers. Any product that may be evaluated in this article, or claim that may be made by its manufacturer, is not guaranteed or endorsed by the publisher.
Supplementary material
The Supplementary Material for this article can be found online at: https://www.frontiersin.org/articles/10.3389/fopht.2024.1380701/full#supplementary-material
1. Asimellis G, Kaufman EJ. Keratoconus . Treasure Island (FL: StatPearls Publishing Copyright © 2023, StatPearls Publishing LLC (2023).
Google Scholar
2. Ferdi AC, Nguyen V, Gore DM, Allan BD, Rozema JJ, Watson SL. Keratoconus natural progression: A systematic review and meta-analysis of 11 529 eyes. Ophthalmology . (2019) 126:935–45. doi: 10.1016/j.ophtha.2019.02.029
PubMed Abstract | CrossRef Full Text | Google Scholar
3. Antunes-Foschini R, Doná H, de Mello PHSA, Pereira RB, Marqueis IM, Rocha EM, et al. Natural history and predictors for progression in pediatric keratoconus. Sci Rep . (2023) 13:4940. doi: 10.1038/s41598-023-32176-5
4. Mas Tur V, MacGregor C, Jayaswal R, O’Brart D, Maycock N. A review of keratoconus: Diagnosis, pathophysiology, and genetics. Survey Ophthalmol . (2017) 62:770–83. doi: 10.1016/j.survophthal.2017.06.009
CrossRef Full Text | Google Scholar
5. Santodomingo-Rubido J, Carracedo G, Suzaki A, Villa-Collar C, Vincent SJ, Wolffsohn JS. Keratoconus: An updated review. Contact Lens Anterior Eye . (2022) 45:101559. doi: 10.1016/j.clae.2021.101559
6. Hashemi H, Heydarian S, Hooshmand E, Saatchi M, Yekta A, Aghamirsalim M, et al. The prevalence and risk factors for keratoconus: A systematic review and meta-analysis. Cornea . (2020) 39:263–70. doi: 10.1097/ICO.0000000000002150
7. Belin MW, Ambrósio R. Scheimpflug imaging for keratoconus and ectatic disease. Indian J Ophthalmol . (2013) 61:401–6. doi: 10.4103/0301-4738.116059
8. Cavas-Martínez F, de la Cruz Sánchez E, Nieto Martínez J, Fernández Cañavate FJ, Fernández-Pacheco DG. Corneal topography in keratoconus: state of the art. Eye Vis (Lond) . (2016) 3:5. doi: 10.1186/s40662-016-0036-8
9. Hashemi H, Mehravaran S. Day to day clinically relevant corneal elevation, thickness, and curvature parameters using the orbscan II scanning slit topographer and the pentacam scheimpflug imaging device. Middle East Afr J Ophthalmol . (2010) 17:44–55. doi: 10.4103/0974-9233.61216
10. Alghamdi A, Khan MS, Dakhil TA. Understanding corneal epithelial thickness mapping. Middle East Afr J Ophthalmol . (2022) 29:147–55. doi: 10.4103/meajo.meajo_207_22
11. Masiwa LE, Moodley V. A review of corneal imaging methods for the early diagnosis of pre-clinical Keratoconus. J Optom . (2020) 13:269–75. doi: 10.1016/j.optom.2019.11.001
12. Bui AD, Truong A, Pasricha ND, Indaram M. Keratoconus diagnosis and treatment: recent advances and future directions. Clin Ophthalmol . (2023) 17:2705–18. doi: 10.2147/OPTH.S392665
13. Honavar SG. Artificial intelligence in ophthalmology - Machines think! Indian J Ophthalmol . (2022) 70:1075–9. doi: 10.4103/ijo.IJO_644_22
14. Siddiqui AA, Ladas JG, Lee JK. Artificial intelligence in cornea, refractive, and cataract surgery. Curr Opin Ophthalmol . (2020) 31:253–60. doi: 10.1097/ICU.0000000000000673
15. Rampat R, Deshmukh R, Chen X, Ting DSW, Said DG, Dua HS, et al. Artificial intelligence in cornea, refractive surgery, and cataract: basic principles, clinical applications, and future directions. Asia Pac J Ophthalmol (Phila) . (2021) 10:268–81. doi: 10.1097/APO.0000000000000394
16. Erickson BJ. Basic artificial intelligence techniques: machine learning and deep learning. Radiol Clin North Am . (2021) 59:933–40. doi: 10.1016/j.rcl.2021.06.004
17. Sidey-Gibbons JAM, Sidey-Gibbons CJ. Machine learning in medicine: a practical introduction. BMC Med Res Methodol . (2019) 19:64. doi: 10.1186/s12874-019-0681-4
18. Singh Pathania Y, Budania A. Artificial intelligence in dermatology: “unsupervised” versus “supervised” machine learning. Int J Dermatol . (2021) 60:e28–e9. doi: 10.1111/ijd.15288
19. Shi S, An X, Li Y. Ultrasound radiomics-based logistic regression model to differentiate between benign and Malignant breast nodules. J Ultrasound Med . (2023) 42:869–79. doi: 10.1002/jum.16078
20. Baştanlar Y, Ozuysal M. Introduction to machine learning. Methods Mol Biol . (2014) 1107:105–28. doi: 10.1007/978-1-62703-748-8_7
21. Venkatasubramaniam A, Wolfson J, Mitchell N, Barnes T, JaKa M, French S. Decision trees in epidemiological research. Emerg Themes Epidemiol . (2017) 14:11. doi: 10.1186/s12982-017-0064-4
22. Sankari ES, Manimegalai D. Predicting membrane protein types using various decision tree classifiers based on various modes of general PseAAC for imbalanced datasets. J Theor Biol . (2017) 435:208–17. doi: 10.1016/j.jtbi.2017.09.018
23. Rigatti SJ. Random forest. J Insur Med . (2017) 47:31–9. doi: 10.17849/insm-47-01-31-39.1
24. Jin Y, Lan A, Dai Y, Jiang L, Liu S. Development and testing of a random forest-based machine learning model for predicting events among breast cancer patients with a poor response to neoadjuvant chemotherapy. Eur J Med Res . (2023) 28:394. doi: 10.1186/s40001-023-01361-7
25. Zhang S, Li Z, Xin XG. [Support vector machine?assisted diagnosis of human Malignant gastric tissues based on dielectric properties]. Nan Fang Yi Ke Da Xue Xue Bao . (2017) 37:1637–42. doi: 10.3969/j.issn.1673-4254.2017.12.13
26. Abirami S, Chitra P. Chapter Fourteen - Energy-efficient edge based real-time healthcare support system. In: Raj P, Evangeline P, editors. Advances in computers , vol. 117 . Cambridge, Massachusetts: Elsevier (2020). p. 339–68.
27. Choi RY, Coyner AS, Kalpathy-Cramer J, Chiang MF, Campbell JP. Introduction to machine learning, neural networks, and deep learning. Transl Vis Sci Technol . (2020) 9:14. doi: 10.1167/tvst.9.2.14
28. Nistal-Nuño B. Outcome prediction for critical care patients with respiratory neoplasms using a multilayer perceptron neural network. Einstein (Sao Paulo) . (2023) 21:eAO0071. doi: 10.31744/einstein_journal/2023AO0071
29. Sarker IH. Deep learning: A comprehensive overview on techniques, taxonomy, applications and research directions. SN Comput Sci . (2021) 2:420. doi: 10.1007/s42979-021-00815-1
30. Heilbroner SP, Miotto R. Deep learning in medicine. Clin J Am Soc Nephrol . (2023) 18:397–9. doi: 10.2215/CJN.0000000000000080
31. Elsawy A, Abdel-Mottaleb M. A novel network with parallel resolution encoders for the diagnosis of corneal diseases. IEEE Trans BioMed Eng . (2021) 68:3671–80. doi: 10.1109/TBME.2021.3082152
32. Zou KH, O’Malley AJ, Mauri L. Receiver-operating characteristic analysis for evaluating diagnostic tests and predictive models. Circulation . (2007) 115:654–7. doi: 10.1161/CIRCULATIONAHA.105.594929
33. Ng Wei Y, Zhang S, Wang Z, Ong Charles Jit T, Gunasekeran Dinesh V, Lim Gilbert Yong S, et al. Updates in deep learning research in ophthalmology. Clin Sci . (2021) 135:2357–76. doi: 10.1042/CS20210207
34. Ting DSW, Pasquale LR, Peng L, Campbell JP, Lee AY, Raman R, et al. Artificial intelligence and deep learning in ophthalmology. Br J Ophthalmol . (2019) 103:167–75. doi: 10.1136/bjophthalmol-2018-313173
35. Ting DSJ, Foo VH, Yang LWY, Sia JT, Ang M, Lin H, et al. Artificial intelligence for anterior segment diseases: Emerging applications in ophthalmology. Br J Ophthalmol . (2021) 105:158–68. doi: 10.1136/bjophthalmol-2019-315651
36. Gulshan V, Peng L, Coram M, Stumpe MC, Wu D, Narayanaswamy A, et al. Development and validation of a deep learning algorithm for detection of diabetic retinopathy in retinal fundus photographs. Jama . (2016) 316:2402–10. doi: 10.1001/jama.2016.17216
37. Ting DSW, Cheung CY, Lim G, Tan GSW, Quang ND, Gan A, et al. Development and validation of a deep learning system for diabetic retinopathy and related eye diseases using retinal images from multiethnic populations with diabetes. Jama . (2017) 318:2211–23. doi: 10.1001/jama.2017.18152
38. Page MJ, Moher D, Bossuyt PM, Boutron I, Hoffmann TC, Mulrow CD, et al. PRISMA 2020 explanation and elaboration: updated guidance and exemplars for reporting systematic reviews. BMJ . (2021) 372:n160. doi: 10.1136/bmj.n160
39. Whiting PF, Rutjes AW, Westwood ME, Mallett S, Deeks JJ, Reitsma JB, et al. QUADAS-2: a revised tool for the quality assessment of diagnostic accuracy studies. Ann Intern Med . (2011) 155:529–36. doi: 10.7326/0003-4819-155-8-201110180-00009
40. Lee J, Mulder F, Leeflang M, Wolff R, Whiting P, Bossuyt PM. QUAPAS: an adaptation of the QUADAS-2 tool to assess prognostic accuracy studies. Ann Intern Med . (2022) 175:1010–8. doi: 10.7326/M22-0276
41. Mohammadpour M, Heidari Z, Hashemi H, Yaseri M, Fotouhi A. Comparison of artificial intelligence-based machine learning classifiers for early detection of keratoconus. Eur J Ophthalmol . (2022) 32:1352–60. doi: 10.1177/11206721211073442
42. Tan Z, Chen X, Li K, Liu Y, Cao H, Li J, et al. Artificial intelligence-based diagnostic model for detecting keratoconus using videos of corneal force deformation. Transl Vis Sci Technol . (2022) 11:32. doi: 10.1167/tvst.11.9.32
43. Xu Z, Feng R, Jin X, Hu H, Ni S, Xu W, et al. Evaluation of artificial intelligence models for the detection of asymmetric keratoconus eyes using Scheimpflug tomography. Clin Exp Ophthalmol . (2022) 50:714–23. doi: 10.1111/ceo.14126
44. Ahn H, Kim NE, Chung JL, Kim YJ, Jun I, Kim TI, et al. Patient selection for corneal topographic evaluation of keratoconus: A screening approach using artificial intelligence. Front Med (Lausanne) . (2022) 9:934865. doi: 10.3389/fmed.2022.934865
45. Almeida GC Jr., Guido RC, Balarin Silva HM, Brandão CC, de Mattos LC, Lopes BT, et al. New artificial intelligence index based on Scheimpflug corneal tomography to distinguish subclinical keratoconus from healthy corneas. J Cataract Refract Surg . (2022) 48:1168–74. doi: 10.1097/j.jcrs.0000000000000946
46. Kundu G, Shetty R, Khamar P, Mullick R, Gupta S, Nuijts R, et al. Universal architecture of corneal segmental tomography biomarkers for artificial intelligence-driven diagnosis of early keratoconus. Br J Ophthalmol . (2023) 107:635–43. doi: 10.1136/bjophthalmol-2021-319309
47. Lucena AR, Araújo MO, Carneiro RFL, Cavalcante TDS, Ribeiro ABN, Anselmo FJM. Development of an application for providing corneal topography reports based on artificial intelligence. Arq Bras Oftalmol . (2021) 85:351–8. doi: 10.5935/0004-2749.20220051
48. Issarti I, Consejo A, Jiménez-García M, Hershko S, Koppen C, Rozema JJ. Computer aided diagnosis for suspect keratoconus detection. Comput Biol Med . (2019) 109:33–42. doi: 10.1016/j.compbiomed.2019.04.024
49. Lopes BT, Ramos IC, Salomão MQ, Guerra FP, Schallhorn SC, Schallhorn JM, et al. Enhanced tomographic assessment to detect corneal ectasia based on artificial intelligence. Am J Ophthalmol . (2018) 195:223–32. doi: 10.1016/j.ajo.2018.08.005
50. Lu NJ, Elsheikh A, Rozema JJ, Hafezi N, Aslanides IM, Hillen M, et al. Combining spectral-domain OCT and air-puff tonometry analysis to diagnose keratoconus. J Refract Surg . (2022) 38:374–80. doi: 10.3928/1081597X-20220414-02
51. Lavric A, Valentin P. KeratoDetect: keratoconus detection algorithm using convolutional neural networks. Comput Intell Neurosci . (2019) 2019:8162567. doi: 10.1109/ECAI46879.2019
52. Kuo BI, Chang WY, Liao TS, Liu FY, Liu HY, Chu HS, et al. Keratoconus screening based on deep learning approach of corneal topography. Transl Vis Sci Technol . (2020) 9:53. doi: 10.1167/tvst.9.2.53
53. Chandapura R, Salomao MQ, Ambrosio R Jr., Swarup R, Shetty R, Sinha Roy A. Bowman’s topography for improved detection of early ectasia. J Biophotonics . (2019) 12:e201900126. doi: 10.1002/jbio.201900126
54. Cohen E, Bank D, Sorkin N, Giryes R, Varssano D. Use of machine learning to achieve keratoconus detection skills of a corneal expert. Int Ophthalmol . (2022) 42:3837–47. doi: 10.1007/s10792-022-02404-4
55. Ambrósio R Jr., Lopes BT, Faria-Correia F, Salomão MQ, Bühren J, Roberts CJ, et al. Integration of scheimpflug-based corneal tomography and biomechanical assessments for enhancing ectasia detection. J Refract Surg . (2017) 33:434–43. doi: 10.3928/1081597X-20170426-02
56. Gao HB, Pan ZG, Shen MX, Lu F, Li H, Zhang XQ. KeratoScreen: early keratoconus classification with zernike polynomial using deep learning. Cornea . (2022) 41:1158–65. doi: 10.1097/ICO.0000000000003038
57. Subramanian P, Ramesh GP. Keratoconus classification with convolutional neural networks using segmentation and index quantification of eye topography images by particle swarm optimisation. BioMed Res Int . (2022) 2022:8119685. doi: 10.1155/2022/8119685
58. Lu NJ, Koppen C, Hafezi F, Ni Dhubhghaill S, Aslanides IM, Wang QM, et al. Combinations of Scheimpflug tomography, ocular coherence tomography and air-puff tonometry improve the detection of keratoconus. Cont Lens Anterior Eye . (2023) 46:101840. doi: 10.1016/j.clae.2023.101840
59. Cao K, Verspoor K, Sahebjada S, Baird PN. Evaluating the performance of various machine learning algorithms to detect subclinical keratoconus. Transl Vis Sci Technol . (2020) 9:24. doi: 10.1167/tvst.9.2.24
60. Smadja D, Touboul D, Cohen A, Doveh E, Santhiago MR, Mello GR, et al. Detection of subclinical keratoconus using an automated decision tree classification. Am J Ophthalmol . (2013) 156:237–46 e1. doi: 10.1016/j.ajo.2013.03.034
61. Karimi A, Meimani N, Razaghi R, Rahmati SM, Jadidi K, Rostami M. Biomechanics of the healthy and keratoconic corneas: A combination of the clinical data, finite element analysis, and artificial neural network. Curr Pharm Des . (2018) 24:4474–83. doi: 10.2174/1381612825666181224123939
62. Ruiz Hidalgo I, Rozema JJ, Saad A, Gatinel D, Rodriguez P, Zakaria N, et al. Validation of an objective keratoconus detection system implemented in a scheimpflug tomographer and comparison with other methods. Cornea . (2017) 36:689–95. doi: 10.1097/ICO.0000000000001194
63. Maeda N, Klyce SD, Smolek MK. Comparison of methods for detecting keratoconus using videokeratography. Arch Ophthalmol . (1995) 113:870–4. doi: 10.1001/archopht.1995.01100070044023
64. Cao K, Verspoor K, Chan E, Daniell M, Sahebjada S, Baird PN. Machine learning with a reduced dimensionality representation of comprehensive Pentacam tomography parameters to identify subclinical keratoconus. Comput Biol Med . (2021) 138:104884. doi: 10.1016/j.compbiomed.2021.104884
65. Maeda N, Klyce SD, Smolek MK, Thompson HW. Automated keratoconus screening with corneal topography analysis. Invest Ophthalmol Vis Sci . (1994) 35:2749–57.
PubMed Abstract | Google Scholar
66. Feng R, Xu Z, Zheng X, Hu H, Jin X, Chen DZ, et al. KerNet: A novel deep learning approach for keratoconus and sub-clinical keratoconus detection based on raw data of the pentacam HR system. IEEE J BioMed Health Inform . (2021) 25:3898–910. doi: 10.1109/JBHI.2021.3079430
67. Al-Timemy AH, Mosa ZM, Alyasseri Z, Lavric A, Lui MM, Hazarbassanov RM, et al. A hybrid deep learning construct for detecting keratoconus from corneal maps. Transl Vis Sci Technol . (2021) 10:16. doi: 10.1167/tvst.10.14.16
68. Elsawy A, Eleiwa T, Chase C, Ozcan E, Tolba M, Feuer W, et al. Multidisease deep learning neural network for the diagnosis of corneal diseases. Am J Ophthalmol . (2021) 226:252–61. doi: 10.1016/j.ajo.2021.01.018
69. Fassbind B, Langenbucher A, Streich A. Automated cornea diagnosis using deep convolutional neural networks based on cornea topography maps. Sci Rep . (2023) 13:6566. doi: 10.1038/s41598-023-33793-w
70. Kovacs I, Mihaltz K, Kranitz K, Juhasz E, Takacs A, Dienes L, et al. Accuracy of machine learning classifiers using bilateral data from a Scheimpflug camera for identifying eyes with preclinical signs of keratoconus. J Cataract Refract Surg . (2016) 42:275–83. doi: 10.1016/j.jcrs.2015.09.020
71. Leao E, Ing Ren T, Lyra JM, MaChado A, Koprowski R, Lopes B, et al. Corneal deformation amplitude analysis for keratoconus detection through compensation for intraocular pressure and integration with horizontal thickness profile. Comput Biol Med . (2019) 109:263–71. doi: 10.1016/j.compbiomed.2019.04.019
72. Kamiya K, Ayatsuka Y, Kato Y, Fujimura F, Takahashi M, Shoji N, et al. Keratoconus detection using deep learning of colour-coded maps with anterior segment optical coherence tomography: a diagnostic accuracy study. BMJ Open . (2019) 9:e031313. doi: 10.1136/bmjopen-2019-031313
73. Maeda N, Klyce SD, Smolek MK. Neural network classification of corneal topography. Preliminary demonstration. Invest Ophthalmol Vis Sci . (1995) 36:1327–35.
74. Prakash G, Perera C, Jhanji V. Comparison of machine learning-based algorithms using corneal asymmetry vs. single-metric parameters for keratoconus detection. Graefes Arch Clin Exp Ophthalmol . (2023) 261:2335–42. doi: 10.1007/s00417-023-06049-6
75. Zeboulon P, Debellemaniere G, Bouvet M, Gatinel D. Corneal topography raw data classification using a convolutional neural network. Am J Ophthalmol . (2020) 219:33–9. doi: 10.1016/j.ajo.2020.06.005
76. Ruiz Hidalgo I, Rodriguez P, Rozema JJ, ND S, Zakaria N, Tassignon MJ, et al. Evaluation of a machine-learning classifier for keratoconus detection based on scheimpflug tomography. Cornea . (2016) 35:827–32. doi: 10.1097/ICO.0000000000000834
77. Souza MB, Medeiros FW, Souza DB, Garcia R, Alves MR. Evaluation of machine learning classifiers in keratoconus detection from orbscan II examinations. Clinics (Sao Paulo) . (2010) 65:1223–8. doi: 10.1590/S1807-59322010001200002
78. Accardo PA, Pensiero S. Neural network-based system for early keratoconus detection from corneal topography. J BioMed Inform . (2002) 35:151–9. doi: 10.1016/S1532-0464(02)00513-0
79. Castro-Luna G, Jimenez-Rodriguez D, Castano-Fernandez AB, Perez-Rueda A. Diagnosis of subclinical keratoconus based on machine learning techniques. J Clin Med . (2021) 10:4281. doi: 10.3390/jcm10184281
80. Shi C, Wang M, Zhu T, Zhang Y, Ye Y, Jiang J, et al. Machine learning helps improve diagnostic ability of subclinical keratoconus using Scheimpflug and OCT imaging modalities. Eye Vis (Lond) . (2020) 7:48. doi: 10.1186/s40662-020-00213-3
81. Wan Q, Wei R, Ma K, Yin H, Deng YP, Tang J. Deep learning-based automatic diagnosis of keratoconus with corneal endothelium image. Ophthalmol Ther . (2023) 12:3047–65. doi: 10.1007/s40123-023-00795-w
82. Al-Timemy AH, Alzubaidi L, Mosa ZM, Abdelmotaal H, Ghaeb NH, Lavric A, et al. A deep feature fusion of improved suspected keratoconus detection with deep learning. Diagnostics (Basel) . (2023) 13:1689. doi: 10.3390/diagnostics13101689
83. Castro-Luna GM, Martinez-Finkelshtein A, Ramos-Lopez D. Robust keratoconus detection with Bayesian network classifier for Placido-based corneal indices. Cont Lens Anterior Eye . (2020) 43:366–72. doi: 10.1016/j.clae.2019.12.006
84. Abdelmotaal H, Mostafa MM, Mostafa ANR, Mohamed AA, Abdelazeem K. Classification of color-coded scheimpflug camera corneal tomography images using deep learning. Transl Vis Sci Technol . (2020) 9:30. doi: 10.1167/tvst.9.13.30
85. Twa MD, Parthasarathy S, Roberts C, Mahmoud AM, Raasch TW, Bullimore MA. Automated decision tree classification of corneal shape. Optom Vis Sci . (2005) 82:1038–46. doi: 10.1097/01.opx.0000192350.01045.6f
86. Fırat M, Çankaya C, Çınar A, Tuncer T. Automatic detection of keratoconus on Pentacam images using feature selection based on deep learning. Int J Imaging Syst Technol . (2022) 32:1548–60. doi: 10.1002/ima.22717
87. Mosa ZM, Ghaeb NH, Ali AH. Detecting keratoconus by using SVM and decision tree classifiers with the aid of image processing. Baghdad Sci J . (2019) 16:1022–9. doi: 10.21123/bsj.2019.16.4(Suppl.)
88. Ambrosio R Jr., MaChado AP, Leao E, Lyra JMG, Salomao MQ, Esporcatte LGP, et al. Optimized artificial intelligence for enhanced ectasia detection using scheimpflug-based corneal tomography and biomechanical data. Am J Ophthalmol . (2023) 251:126–42. doi: 10.1016/j.ajo.2022.12.016
89. Lavric A, Popa V, Takahashi H, Yousefi S. Detecting keratoconus from corneal imaging data using machine learning. IEEE Access . (2020) 8:149113–21. doi: 10.1109/Access.6287639
90. de Almeida GC Jr., Guido RC, Neto JS, Rosa JM, Castiglioni L, de Mattos LC, et al. Corneal Tomography Multivariate Index (CTMVI) effectively distinguishes healthy corneas from those susceptible to ectasia. Biomed Signal Process Control . (2021) 70. doi: 10.1016/j.bspc.2021.102995
91. Zeboulon P, Debellemaniere G, Gatinel D. Unsupervised learning for large-scale corneal topography clustering. Sci Rep . (2020) 10:16973. doi: 10.1038/s41598-020-73902-7
92. Al-Timemy AH, Ghaeb NH, Mosa ZM, Escudero J. Deep transfer learning for improved detection of keratoconus using corneal topographic maps. Cogn Computation . (2021) 14:1627–42. doi: 10.1007/s12559-021-09880-3
93. Zaki WMD W, Mat Daud M, Saad AH, Hussain A. Abdul mutalib H. A mobile solution for lateral segment photographed images based deep keratoconus screening method. Int J Integrated Eng . (2021) 13:18–27. doi: 10.30880/ijie.2021.013.05.003
94. Alshammari G, Hamad AA, Abdullah ZM, Alshareef AM, Alhebaishi N, Alshammari A, et al. Applications of deep learning on topographic images to improve the diagnosis for dynamic systems and unconstrained optimization. Wireless Commun Mobile Computing . (2021) 2021:1–7. doi: 10.1155/2021/4672688
95. Abdelmotaal H, Abdou AA, Omar AF, El-Sebaity DM, Abdelazeem K. Pix2pix conditional generative adversarial networks for scheimpflug camera color-coded corneal tomography image generation. Transl Vis Sci Technol . (2021) 10:21. doi: 10.1167/tvst.10.7.21
96. Silverman RH, Urs R, Roychoudhury A, Archer TJ, Gobbe M, Reinstein DZ. Epithelial remodeling as basis for machine-based identification of keratoconus. Invest Ophthalmol Vis Sci . (2014) 55:1580–7. doi: 10.1167/iovs.13-12578
97. Abdelmotaal H, Hazarbassanov RM, Salouti R, Nowroozzadeh MH, Taneri S, Al-Timemy AH, et al. Keratoconus detection-based on dynamic corneal deformation videos using deep learning. Ophthalmol Sci . (2024) 4:100380. doi: 10.1016/j.xops.2023.100380
98. Francis M, Shetty R, Padmanabhan P, Vinciguerra R, Vinciguerra P, Lippera M, et al. New simulation software to predict postoperative corneal stiffness before laser vision correction. J Cataract Refract Surg . (2023) 49:620–7. doi: 10.1097/j.jcrs.0000000000001169
99. Velazquez-Blazquez JS, Bolarin JM, Cavas-Martinez F, Alio JL. EMKLAS: A new automatic scoring system for early and mild keratoconus detection. Transl Vis Sci Technol . (2020) 9:30. doi: 10.1167/tvst.9.2.30
100. Santhiago MR, Araujo DC, Stival LR, Smadja D, Veloso AA. Ectasia risk model: A novel method without cut-off point based on artificial intelligence improves detection of higher-risk eyes. J Refract Surg . (2022) 38:716–24. doi: 10.3928/1081597X-20221018-01
101. Reinstein DZ, Archer TJ, Gobbe M. Corneal epithelial thickness profile in the diagnosis of keratoconus. J Refract Surg . (2009) 25:604–10. doi: 10.3928/1081597X-20090610-06
102. Li Y, Li M, Li C, Liu Z. Forest aboveground biomass estimation using Landsat 8 and Sentinel-1A data with machine learning algorithms. Sci Rep . (2020) 10:9952. doi: 10.1038/s41598-020-67024-3
103. Arik SÖ, Pfister T. TabNet: attentive interpretable tabular learning. ArXiv . (2019). doi: 10.48550/arXiv.1908.07442
104. Ghaderi M, Sharifi A, Jafarzadeh Pour E. Proposing an ensemble learning model based on neural network and fuzzy system for keratoconus diagnosis based on Pentacam measurements. Int Ophthalmol . (2021) 41:3935–48. doi: 10.1007/s10792-021-01963-2
105. Saikia MJ, Kuanar S, Mahapatra D, Faghani S. Multi-modal ensemble deep learning in head and neck cancer HPV sub-typing. Bioengineering (Basel) . (2023) 11:13. doi: 10.3390/bioengineering11010013
106. Xie X, Xie M, Moshayedi AJ, Noori Skandari MH. A hybrid improved neural networks algorithm based on L2 and dropout regularization. Math Problems Engineering . (2022) 2022:8220453. doi: 10.1155/2022/8220453
107. Sherazi SWA, Bae J-W, Lee JY. A soft voting ensemble classifier for early prediction and diagnosis of occurrences of major adverse cardiovascular events for STEMI and NSTEMI during 2-year follow-up in patients with acute coronary syndrome. PloS One . (2021) 16:e0249338. doi: 10.1371/journal.pone.0249338
108. Rozema JJ, Rodriguez P, Ruiz Hidalgo I, Navarro R, Tassignon MJ, Koppen C. SyntEyes KTC: higher order statistical eye model for developing keratoconus. Ophthalmic Physiol Opt . (2017) 37:358–65. doi: 10.1111/opo.12369
109. Hosoda Y, Miyake M, Meguro A, Tabara Y, Iwai S, Ueda-Arakawa N, et al. Keratoconus-susceptibility gene identification by corneal thickness genome-wide association study and artificial intelligence IBM Watson. Commun Biol . (2020) 3:410. doi: 10.1038/s42003-020-01137-3
110. Wang L, Wang Y, Liu J, Zhao W. Identification of important genes of keratoconus and construction of the diagnostic model. Genet Res (Camb) . (2022) 2022:5878460. doi: 10.1155/2022/5878460
111. Nokas G, Kotsilieris T. Preventing keratoconus through eye rubbing activity detection: A machine learning approach. Electronics . (2023) 12:1028. doi: 10.3390/electronics12041028
112. Kenney MC, Nesburn AB, Burgeson RE, Butkowski RJ, Ljubimov AV. Abnormalities of the extracellular matrix in keratoconus corneas. Cornea . (1997) 16:345–51. doi: 10.1097/00003226-199705000-00016
113. Herber R, Pillunat LE, Raiskup F. Development of a classification system based on corneal biomechanical properties using artificial intelligence predicting keratoconus severity. Eye Vis (Lond) . (2021) 8:21. doi: 10.1186/s40662-021-00244-4
114. Yousefi S, Yousefi E, Takahashi H, Hayashi T, Tampo H, Inoda S, et al. Keratoconus severity identification using unsupervised machine learning. PloS One . (2018) 13:e0205998. doi: 10.1371/journal.pone.0205998
115. Mahmoud HAH, Mengash HA. Automated keratoconus detection by 3D corneal images reconstruction. Sensors (Basel) . (2021) 21:2326. doi: 10.3390/s21072326
116. Issarti I, Consejo A, Jiménez-García M, Kreps EO, Koppen C, Rozema JJ. Logistic index for keratoconus detection and severity scoring (Logik). Comput Biol Med . (2020) 122:103809. doi: 10.1016/j.compbiomed.2020.103809
117. Aatila M, Lachgar M, Hamid H, Kartit A. Keratoconus severity classification using features selection and machine learning algorithms. Comput Math Methods Med . (2021) 2021:9979560. doi: 10.1155/2021/9979560
118. Malyugin B, Sakhnov S, Izmailova S, Boiko E, Pozdeyeva N, Axenova L, et al. Keratoconus diagnostic and treatment algorithms based on machine-learning methods. Diagnostics (Basel) . (2021) 11:1933. doi: 10.3390/diagnostics11101933
119. Tai HY, Lin JJ, Huang YH, Shih PJ, Wang IJ, Yen JY. Correlation between corneal dynamic responses and keratoconus topographic parameters. J Int Med Res . (2022) 50:3000605221108100. doi: 10.1177/03000605221108100
120. Kamiya K, Ayatsuka Y, Kato Y, Shoji N, Mori Y, Miyata K. Diagnosability of keratoconus using deep learning with placido disk-based corneal topography. Front Med (Lausanne) . (2021) 8:724902. doi: 10.3389/fmed.2021.724902
121. Chen X, Zhao J, Iselin KC, Borroni D, Romano D, Gokul A, et al. Keratoconus detection of changes using deep learning of colour-coded maps. BMJ Open Ophthalmol . (2021) 6:e000824. doi: 10.1136/bmjophth-2021-000824
122. Dos Santos VA, Schmetterer L, Stegmann H, Pfister M, Messner A, Schmidinger G, et al. CorneaNet: fast segmentation of cornea OCT scans of healthy and keratoconic eyes using deep learning. BioMed Opt Express . (2019) 10:622–41. doi: 10.1364/BOE.10.000622
123. Dong Y, Li D, Guo Z, Liu Y, Lin P, Lv B, et al. Dissecting the profile of corneal thickness with keratoconus progression based on anterior segment optical coherence tomography. Front Neurosci . (2021) 15:804273. doi: 10.3389/fnins.2021.804273
124. Lavric A, Anchidin L, Popa V, Al-Timemy AH, Alyasseri Z, Takahashi H, et al. Keratoconus severity detection from elevation, topography and pachymetry raw data using a machine learning approach. IEEE Access . (2021) 9:84344–55. doi: 10.1109/ACCESS.2021.3086021
125. Bolarín JM, Cavas F, Velázquez JS, Alió JL. A machine-learning model based on morphogeometric parameters for RETICS disease classification and GUI development. Appl Sci . (2020) 10:1874. doi: 10.3390/app10051874
126. Zorto AD, Sharif MS, Wall J, Brahma A, Alzahrani AI, Alalwan N. An innovative approach based on machine learning to evaluate the risk factors importance in diagnosing keratoconus. Inf Med Unlocked . (2023) 38. doi: 10.1016/j.imu.2023.101208
127. Smolek MK, Klyce SD. Current keratoconus detection methods compared with a neural network approach. Invest Ophthalmol Vis Sci . (1997) 38:2290–9.
128. Werkmeister RM, Sapeta S, Schmidl D, Garhöfer G, Schmidinger G, Aranha Dos Santos V, et al. Ultrahigh-resolution OCT imaging of the human cornea. BioMed Opt Express . (2017) 8:1221–39. doi: 10.1364/BOE.8.001221
129. Kundu G, Shetty N, Shetty R, Khamar P, D’Souza S, Meda TR, et al. Artificial intelligence-based stratification of demographic, ocular surface high-risk factors in progression of keratoconus. Indian J Ophthalmol . (2023) 71:1882–8. doi: 10.4103/IJO.IJO_2651_22
130. Shetty R, Kundu G, Narasimhan R, Khamar P, Gupta K, Singh N, et al. Artificial intelligence efficiently identifies regional differences in the progression of tomographic parameters of keratoconic corneas. J Refract Surg . (2021) 37:240–8. doi: 10.3928/1081597X-20210120-01
131. Reddy JC, Bhamidipati P, Dwivedi S, Dhara KK, Joshi V, Hasnat Ali M, et al. KEDOP: Keratoconus early detection of progression using tomography images. Eur J Ophthalmol . (2022) 32:2554–64. doi: 10.1177/11206721221087566
132. Jiménez-García M, Issarti I, Kreps EO, ND S, Koppen C, Varssano D, et al. Forecasting progressive trends in keratoconus by means of a time delay neural network. J Clin Med . (2021) 10:3238. doi: 10.3390/jcm10153238
133. Kamiya K, Ayatsuka Y, Kato Y, Shoji N, Miyai T, Ishii H, et al. Prediction of keratoconus progression using deep learning of anterior segment optical coherence tomography maps. Ann Transl Med . (2021) 9:1287. doi: 10.21037/atm
134. Kato N, Masumoto H, Tanabe M, Sakai C, Negishi K, Torii H, et al. Predicting keratoconus progression and need for corneal crosslinking using deep learning. J Clin Med . (2021) 10:844. doi: 10.3390/jcm10040844
135. Cao K, Verspoor K, Chan E, Daniell M, Sahebjada S, Baird PN. Stratification of keratoconus progression using unsupervised machine learning analysis of tomographical parameters. Intelligence-Based Med . (2023) 7:100095. doi: 10.1016/j.ibmed.2023.100095
136. Fariselli C, Vega-Estrada A, Arnalich-Montiel F, Alio JL. Artificial neural network to guide intracorneal ring segments implantation for keratoconus treatment: a pilot study. Eye Vis (Lond) . (2020) 7:20. doi: 10.1186/s40662-020-00184-5
137. Yousefi S, Takahashi H, Hayashi T, Tampo H, Inoda S, Arai Y, et al. Predicting the likelihood of need for future keratoplasty intervention using artificial intelligence. Ocul Surf . (2020) 18:320–5. doi: 10.1016/j.jtos.2020.02.008
138. Valdés-Mas MA, Martín-Guerrero JD, Rupérez MJ, Pastor F, Dualde C, Monserrat C, et al. A new approach based on Machine Learning for predicting corneal curvature (K1) and astigmatism in patients with keratoconus after intracorneal ring implantation. Comput Methods Programs Biomed . (2014) 116:39–47. doi: 10.1016/j.cmpb.2014.04.003
139. Liu Y, Shen D, Wang HY, Qi MY, Zeng QY. Development and validation to predict visual acuity and keratometry two years after corneal crosslinking with progressive keratoconus by machine learning. Front Med (Lausanne) . (2023) 10:1146529. doi: 10.3389/fmed.2023.1146529
140. Lyra D, Ribeiro G, Torquetti L, Ferrara P, MaChado A, Lyra JM. Computational models for optimization of the intrastromal corneal ring choice in patients with keratoconus using corneal tomography data. J Refract Surg . (2018) 34:547–50. doi: 10.3928/1081597X-20180615-01
141. Firat M, Cinar A, Cankaya C, Firat IT, Tuncer T. Prediction of Pentacam image after corneal cross-linking by linear interpolation technique and U-NET based 2D regression model. Comput Biol Med . (2022) 146:105541. doi: 10.1016/j.compbiomed.2022.105541
142. Belin MW. Chapter 15 - scheimpflug imaging for keratoconus and ectatic disease. In: Izquierdo L, Henriquez M, Mannis M, editors. Keratoconus . Elsevier, New Delhi (2023). p. 203–20.
143. Hashemi H, Asgari S, Emamian MH, Mehravaran S, Fotouhi A. Age-related changes in corneal curvature and shape: the shahroud eye cohort study. Cornea . (2015) 34:1456–8. doi: 10.1097/ICO.0000000000000595
144. Baboolal SO, Smit DP. South African Eye Study (SAES): ethnic differences in central corneal thickness and intraocular pressure. Eye . (2018) 32:749–56. doi: 10.1038/eye.2017.291
145. Alsaqr AM, Fagehi R, Sharha AA, Alkhudair M, Alshabrami A, Muammar AB, et al. Ethnic differences of corneal parameters: A cross-sectional study. Open Ophthalmol J . (2021) 15:13–20. doi: 10.2174/1874364102115010013
146. Pagano L, Posarelli M, Giannaccare G, Coco G, Scorcia V, Romano V, et al. Artificial intelligence in cornea and ocular surface diseases. Saudi J Ophthalmol . (2023) 37:179–84. doi: 10.4103/sjopt.sjopt_52_23
147. Shanthi S, Aruljyothi L, Balasundaram MB, Janakiraman A, Nirmaladevi K, Pyingkodi M. Artificial intelligence applications in different imaging modalities for corneal topography. Surv Ophthalmol . (2022) 67:801–16. doi: 10.1016/j.survophthal.2021.08.004
148. Zhang Z, Wang Y, Zhang H, Samusak A, Rao H, Xiao C, et al. Artificial intelligence-assisted diagnosis of ocular surface diseases. Front Cell Dev Biol . (2023) 11. doi: 10.3389/fcell.2023.1133680
149. Hashemi H, Doroodgar F, Niazi S, Khabazkhoob M, Heidari Z. Comparison of different corneal imaging modalities using artificial intelligence for diagnosis of keratoconus: a systematic review and meta-analysis. Graefes Arch Clin Exp Ophthalmol . (2023) 11:1133680. doi: 10.1007/s00417-023-06154-6
150. Li Z, Wang L, Wu X, Jiang J, Qiang W, Xie H, et al. Artificial intelligence in ophthalmology: The path to the real-world clinic. Cell Rep Med . (2023) 4:101095. doi: 10.1016/j.xcrm.2023.101095
151. Mazzotta C, Stojanovic A, Romano V, Addabbo G, Borroni D, Balamoun AA, et al. Ray-tracing transepithelial excimer laser central corneal remodeling plus pachymetry-guided accelerated corneal crosslinking for keratoconus. Cornea . (2024) 43:285–94. doi: 10.1097/ICO.0000000000003380
Keywords: keratoconus, corneal ectasia, artificial intelligence, machine learning, deep learning
Citation: Goodman D and Zhu AY (2024) Utility of artificial intelligence in the diagnosis and management of keratoconus: a systematic review. Front. Ophthalmol. 4:1380701. doi: 10.3389/fopht.2024.1380701
Received: 02 February 2024; Accepted: 23 April 2024; Published: 17 May 2024.
Reviewed by:
Copyright © 2024 Goodman and Zhu. This is an open-access article distributed under the terms of the Creative Commons Attribution License (CC BY) . The use, distribution or reproduction in other forums is permitted, provided the original author(s) and the copyright owner(s) are credited and that the original publication in this journal is cited, in accordance with accepted academic practice. No use, distribution or reproduction is permitted which does not comply with these terms.
*Correspondence: Angela Y. Zhu, [email protected]
† These authors have contributed equally to this work
Disclaimer: All claims expressed in this article are solely those of the authors and do not necessarily represent those of their affiliated organizations, or those of the publisher, the editors and the reviewers. Any product that may be evaluated in this article or claim that may be made by its manufacturer is not guaranteed or endorsed by the publisher.
By Andrew Beers , Associate Editor
What’s on the Horizon for Keratoconus
The number of keratoconus treatment options is growing, with new techniques and devices in development..
V arious studies have suggested that the incidence rate of keratoconus is between 0.2 and 4,760 per 100,000 people and 1.5 and 25 per 100,000 people/year. 1 Previous treatment options for the masses included cornea transplant, penetrating keratoplasty, Intacs and various types of lenses-rigid gas permeable lenses, scleral lenses and soft contact lenses for milder cases. But what if every keratoconus patient had a host of novel treatment options available to them?
Corneal collagen cross-linking has been the cutting-edge treatment option for keratoconus, 2 and a number of ophthalmic medical technology and pharmaceutical companies have been pioneering the latest advancements in corneal cross-linking, and, in some cases, researchers have been developing management techniques in tandem with this technology. To appreciate the scope of the developments in keratoconus management, here’s a list of devices and techniques that may eventually come to your practice.
CXLens (TECLens)
TECLens announced its pilot trial results for its transepithelial corneal crosslinking contact lens device, CXLens, in 2021. Now, the company is gearing up for Phase II/III of U.S. Food and Drug Administration trials.
CXLens is an on-eye UV-A light-emitting contact lens device that operates using fiber optics for corneal cross-linking. Its main use is for the treatment of keratoconus, but Roy S. Chuck, MD, the Chairman of Ophthalmology at Albert Einstein College of Medicine and cofounder of TECLens, notes that the device is beginning to be applied for treating refractive errors.
“The original pilot trial publication of the CXLens described that the device was putting the UV light source on a controlled contact lens,” Dr. Chuck explains. “Since then, we’ve added a feedback element. This is an ultrasound biosensor in the lens. So, in real time, you can cross-link, and you can go ahead and monitor the cross-link and really monitor the cornea as it stiffens.” The idea for this additional technology was to provide surgeons with ample control while performing the cross-linking procedure. To further strengthen the control of the procedure, TECLens has developed more advanced features.
“We’re also starting to develop more computer modeling to go ahead and use in conjunction with the cross-linking and ultrasound biosensors,” Dr. Chuck continues. “The ultimate goal is control. If you can control shape change and stiffening change in the cornea using these three elements of cross-linking, feedback of ultrasound and computer modeling to help guide the whole thing, you should have something that’s quite precise and hopefully can control the cornea and it’s reshaping in all kinds of ways.”
In addition to these novel approaches to development, TECLens has partnered with SERVImed Industrial Spa to combine their product Ribocross (riboflavin 0.1%; dextran 10%; Vitamin E TPGS solution) with CXLens technology. Although Ribocross has received Orphan Drug Status from the FDA, Dr. Chuck explains that this solution won’t be introduced with CXLens until the device is fully developed and approved.
According to the pilot study, 3 nine corneal transplant candidates with advanced keratoconus received a scleral lens reservoir. The lenses were fixed with 0.007% benzalkonium chloride preserved with 0.25% riboflavin-monophosphate. After 30 minutes, the lens was removed, and subjects received treatment from the CXLens (375-nm UV-A light at 4mW/cm 2 ). After another 30 minutes, each subject had received a dose of 7.2 J/cm 2 . During the follow-up after six months, subjects who were treated with the CXLens had an average -1.0 ±1.6 D decrease in maximum keratometry.
“Our goal has always been to address the issue of precision medicine, patient specific medicine and customized control,” says Dr. Chuck. “As we go forward, it’s all about treating the patient using their own data rather than trying to guess, and we’re trying to take the guesswork out of what we do by doing everything with ultrasound monitoring and finite element modeling. Hopefully, this will give us the most precise correction possible.”
Glaukos was the first company to have an FDA-approved corneal cross-linking device. Their latest FDA approved epithelium-off riboflavin solution, Photrexa, is used along with Glaukos’ iLink Corneal Cross-Linking system. Currently, Glaukos is developing their epithelium-on platform, Epioxa, and a third-generation corneal cross-linking system.
• Epioxa . In 2023, Glaukos announced that the Phase III FDA trial for Epoxia had reached enrollment completion. This confirmatory pivotal trial has enrolled 312 eyes for epi-on corneal cross-linking treatment. The subjects will be randomly selected in a 2:1 ratio to receive Epioxa therapy or a placebo. This trial will evaluate whether the therapy is safe and effective when attempting to stop the progression of keratoconus and/or reduce the maximum corneal curvature (Kmax) for keratoconic eyes. In a press release, Glaukos stated that the primary endpoint that needed to be achieved was the mean change in Kmax from baseline through a 12-month follow-up period. If there’s a significant difference between the treated subjects and the control’s primary endpoint results and if there’s a ≥1 D difference, then the study would be considered a success by the FDA.
• NXL UV-A Device (Third Generation). Additionally, Glaukos reported its findings on the safety of its third-generation corneal cross-linking system with various riboflavin doses. 4 The company reported that three subjects received a high dose of UV-A while nine subjects received low, medium or high doses of UV-A in a dose-escalating manner. Every patient received epi-on therapy for this study. In 2023, only a total of 17 percent of subjects completed the six-month follow-up period and showed mild adverse events similar to those observed in conventional cross-linking treatments. At ASCRS 2024, Glaukos reported the 12-month data from the study, and they noted no change in adverse events from the six-month follow-up.
• IVMED-80. After Glaukos entered a licensing agreement with iVeena to develop and commercialize IVMED-80 (copper sulfate eye drops), the pharmacological treatment is currently undergoing Phase III FDA trials. In 2020, under the leadership of iVeena, a team of researchers evaluated the safety and preliminary efficacy of the eye drops in Phase I/IIa FDA trials. 5
IVMED-80 was developed to increase lysyl oxidase, a cross-linking enzyme that, when decreased, is linked to keratoconus. 6 During the 26-week trial, a total of 36 patients were randomly divided equally into three treatment groups: Group 1 received IVMED-80 treatment for six weeks, then stopped treatment for the remaining 20 weeks, Group 2 received IVMED-80 treatment for 16 weeks, then stopped treatment for the remaining 10 weeks, and Group 3 received placebo eye drops for 16 weeks, then stopped treatment for the remaining 10 weeks.
According to the study, no adverse events were reported during the 16 weeks of treatment. At 16 weeks, 19 IVMED-80 patients showed a 1-D reduction of Kmax, while the placebo group progressed by 0.46 D of Kmax. Subjects in Group 1 showed a 0.46 D reduction of Kmax at two months, but their eyes reverted back to baseline by 16 weeks. The researchers reported that there was no clinically significant effect on IOP or other ocular findings.
EpiSmart (Epion Therapeutics)
According to their website, Phase III FDA trials (Apricity A and Apricity B) for Epion’s minimally invasive keratoconus treatment, EpiSmart, commenced in 2023. They plan on enrolling 800 subjects from across the United States, including patients as young as 8 years old, as long as they’ve met the enrollment criteria.
This epi-on platform includes RiboStat, a high concentrated riboflavin/sodium iodide solution. This is administered using EpiPrep, a two-part system including a disposable wand to enhance epithelial permeability and a disposable loading sponge to sustain high drug concentration during stromal loading. This is when the EpiSmart UV-A device is introduced to perform epi-on corneal cross-linking.
During Phase II FDA trials, 7 Epion researchers enrolled 2,228 subjects who were assessed at six and 12 months postoperatively. The subjects were randomly divided into three different treatment groups, each receiving various doses of UV-A treatment. The doses administered were 2.4 J/cm 2 over 20 minutes, 3.6 J/cm 2 over 20 minutes and 3.6 J/cm 2 over 30 minutes. The primary endpoint observed by the researchers was logMAR CDVA, while secondary endpoints included logMAR UCVA, Kmax and minimum corneal thickness.
Researchers reported that 1,922 subjects were diagnosed with keratoconus and the other subjects had postsurgical or other ectasias. The primary endpoint was achieved and resulted in a significant improvement in CDVA for all groups. UCVA and Kmax secondary endpoints were significant as well, but minimum corneal thickness remained unchanged. Although no serious adverse events were reported, 195 cases (8.7 percent) had at least one adverse event. The most prevalent complication was a mild corneal epithelial defect, which was found in 31 cases (1.4 percent).
Omni CXL (Micro Medical Devices)
According to Micro Medical Devices, the Omni CXL is a transportable corneal cross-linking system awaiting market approval in the U.S. This device received it’s CE mark in 2006 for European distribution and now it’s in clinical use in over 100 countries.
Micro Medical Devices explains that the device can be tuned to output energy from 3 mW to 45 mW per cm 2 with automatic time adjustment. The system is built with an integrated infrared camera for eye tracking to ensure proper distance. Both the tracking area and the threshold can be individually set. Automatic riboflavin selection recommendation is available when using the integrated pachymeter. Additionally, patient data and treatment settings can be exported as a PDF and saved to the patient’s medical record.
Micro Medical Devices filed for FDA market approval in 2018.
“Corneal allogenic intrastromal ring segments, or the CAIRS technique, was a method that started in 2015 to treat keratoconus,” says Soosan Jacob, MD, the director and chief of Dr. Agarwal’s Refractive and Cornea Foundation and developer of CAIRS. “CAIRS represents the use of any kind of allogenic tissue which is inserted into the cornea as segments, which could be either uniform segments or customized segments.
“Since about 2018, we started customizing CAIRS,” continues Dr. Jacob. “This is true customization, not just based on the arc length or location, but actually based on the individual patient’s keratometric maps, thickness maps and other maps. What you have to realize is that no two keratoconic patients are similar. Even if they fall into broadly the same phenotypic classes. Because we customize for every zone of the patient’s map, we have variable thicknesses available with CAIRS, which gives you much better customization and therefore improved visual acuity results.
“We use a double-bladed design, which is patented, for cutting the CAIRS segments, and this gives us the ability to really exquisitely customize to each patient, or rather to each patient’s type of keratoconus,” says Dr. Jacob. “CAIRS is a minimally invasive surgery because the entire surgery is done through about a 1-mm incision or 1.5-mm incision and the channels are created using a femtosecond laser. So, we use the laser to create the CAIRS channel and then implant the CAIRS segments into the channels.”
When it was first introduced, surgeons would make a manual incision and implant the donor tissue with SK Intacs as a guidewire. 8 Now, many studies are observing the safety and efficacy of femtosecond-assisted CAIRS procedures. At ASCRS 2024, Bianca N. Susanna, MD, reported on the latest research findings for femtosecond laser assisted personalized CAIRS implantation in keratoconic eyes. 9 After three months, subjects’ UDVA improved from 0.01 ±0.06 preoperatively to 0.29 ±0.2 on the last follow-up day. Also, CDVA improved from 0.29 ±0.13 to 0.5 ±0.24. A total of 21 patients gained two or more Snellen lines of CDVA. Furthermore, spherical equivalent (-8.87 ±2.07 D to -2.90 ±4.45 D) and cylinder (-3.75 ±2.11 D to -2.35 ±0.91 D) improved for subjects. It was reported that topographic astigmatism increased from 4.20 ±2.75 to 5.03 ±2.46. During the follow-up period, no serious adverse events were observed.
After the allogenic tissues are implanted, the cornea undergoes cross-linking treatment. Dr. Jacob explains that both conventional accelerated cross-linking and accelerated contact lens-assisted cross-linking can be employed during the procedure, but each method is used for specific cases.
“When the minimum stromal thickness is less than 400 µm, we go in with CACXL, which is contact lens-assisted cross-linking,” explains Dr. Jacob. “If there’s more than 400 µm, we do conventional accelerated cross-linking. In both these situations, the power and the duration that we use is 10 mW/cm 2 for nine minutes.”
Published in 2014, the first study on contact lens-assisted cross-linking showed the safety and effectiveness of the treatment, proponents say. 10 This treatment uses an ultraviolet barrier-free soft contact lens that’s placed on the patient’s cornea after being soaked in iso-osmolar riboflavin 0.1% for 30 minutes. As Dr. Jacob stated previously, the necessary corneal thickness for CACXL is less than 400 µm, and this study shows that the minimum thickness requirement for CACXL treatment is 350 µm. This method thickens the cornea to more than 400 µm, which is why it’s applied when a patient is seen with a stromal thickness of less than 400 µm.
Dr. Jacob adds that CACXL has its advantages when combined with CAIRS. She explains that in some cases, the thickness of the cornea is less than the minimum thickness needed for CACXL. After implanting the allogenic tissue into the eye, the cornea thickens, allowing patients to be treated with CACXL.
“We have done this kind of approach in some cases of topographic-guided PRK where the patient progressed and topographic-guided PRK was done with CXL,” Dr. Jacob mentions. “TGPRK with CXL was done for patients with keratoconus and after a few years a patient’s keratoconus progressed and they came to us. The patient had extreme thinning and fortunately it was in the same area where they had CAIRS implanted. Now, since it was so thin, we wouldn’t have been able to do a CACXL or CXL directly without CAIRS, but because we already placed CAIRS within this patient, we were able to increase the thickness of the cornea immediately in the thin zone because that was where the CAIRS was implanted, and it could be followed up with cross-linking.”
Now, common transplantation adverse events may occur, but Dr. Jacob doesn’t view this as an issue. “CAIRS can be associated with complications, but you have to remember that this is a reversible and adjustable procedure. If for some reason the patient doesn’t like the visual quality, then you can just reverse it by removing the CAIRS. On the other hand, if you feel that you need to get more or less results, you can also adjust the CAIRS segment easily.”
There are many devices and techniques in the pipeline for keratoconus management, but there’s more work that needs to be done. Each device mentioned in this article is still undergoing FDA trials and the CAIRS technique is advancing as more researchers introduce novel ways to create incisions, customize ring segments and perform different corneal cross-linking methods. It seems like minimally invasive treatment options for keratoconus are going to continue to grow, and it’ll be exciting to see what’s next on the horizon.
Dr. Chuck is the cofounder of TECLens. Dr. Jacob has no financial interests to disclose.
1. Santodomingo-Rubido J, Carracedo G, Suzaki A, et al. Keratoconus: An updated review. Contact Lens and Anterior Eye 2022;45:3.
2. Feldman BH, Halfpenny C, Bernfeld E, et al. Corneal collagen cross-linking. https://eyewiki.aao.org/Corneal_Collagen_Cross-Linking. Accessed on March 28, 2024.
3. Dackowski EK, Logroño JB, Rivera C, et al. Tranepithelial corneal crosslinking using a novel ultraviolet light-emitting contact lens device: A pilot study. Translational Vision Science & Technology 2021;10:5.
4. Smith V, Heersink SB, Hersh P, et al. Riboflavin ophthalmic solution activated in situ with a novel scanning laser-based bioactivation system, the NXL UV-A device, demonstrates safety of corneal cross-linking for the treatment of keratoconus. Investigative Ophthalmology & Visual Science 2023;64:8:1675.
5. Molokhia S, Muddana SK, Hauritz H, et al. IVMED 80 eye drops for treatment of keratconus in patients -Phase 1/2a. Invest Ophthalmol Vis Sci 2020;60:2587.
6. Dudakova L, Jirsova K. The impairment of lysyl oxidase in keratoconus and in keratoconus-associated disorders. J Neural Transm (Vienna) 2013;120:6:977-82.
7. Epstein RJ, Belin MW, Gravemann D, et al. EpiSmart crosslinking for keratoconus: A Phase 2 study. Cornea 2023;42:7:858-866.
8. Susanna BN. Exclusively femtosecond-assisted personalized Cairs: Description of a novel technique for creation and insertion. https://ascrs.confex.com/ascrs/24am/meetingapp.cgi/Paper/102884. Accessed on April 1, 2024.
9. Susanna BN. Femtosecond laser assisted personalized Cairs implantation in keratoconic eyes. https://ascrs.confex.com/ascrs/24am/meetingapp.cgi/Paper/102832. Accessed on April 1, 2024.
10. Jacob S, Kumar DA, Agarwal A, et al. Contact lens-assisted collagen cross-linking (CACXL): A new technique for cross-linking thin corneas. J Refract Surg 2014;30:6:366-72.
Related Articles
Meet the Challenges of Vasculitic PUK
Outdoor activities and myopia development, fuchs’ dystrophy and femto cataract surgery.
Refractive Surgeons Await Cross-linking
How to manage dsaek complications, current issue.
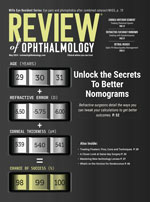
Table of Contents
Read digital edition, read pdf edition, subscriptions.

Copyright © 2024 Jobson Medical Information LLC unless otherwise noted.
All rights reserved. Reproduction in whole or in part without permission is prohibited.

- Biosimilars
- Cataract Therapeutics
- Gene Therapy
- Optic Relief
- Geographic Atrophy
- Ocular Surface Disease
- Practice Management
- Therapeutics
- Understanding Antibiotic Resistance
- Ocular Allergy
- Clinical Diagnosis
Changing landscape of keratoconus management
Alternate methods of screening may lead to earlier diagnosis of disease.

(Image Credit: AdobeStock/Eyematrix)
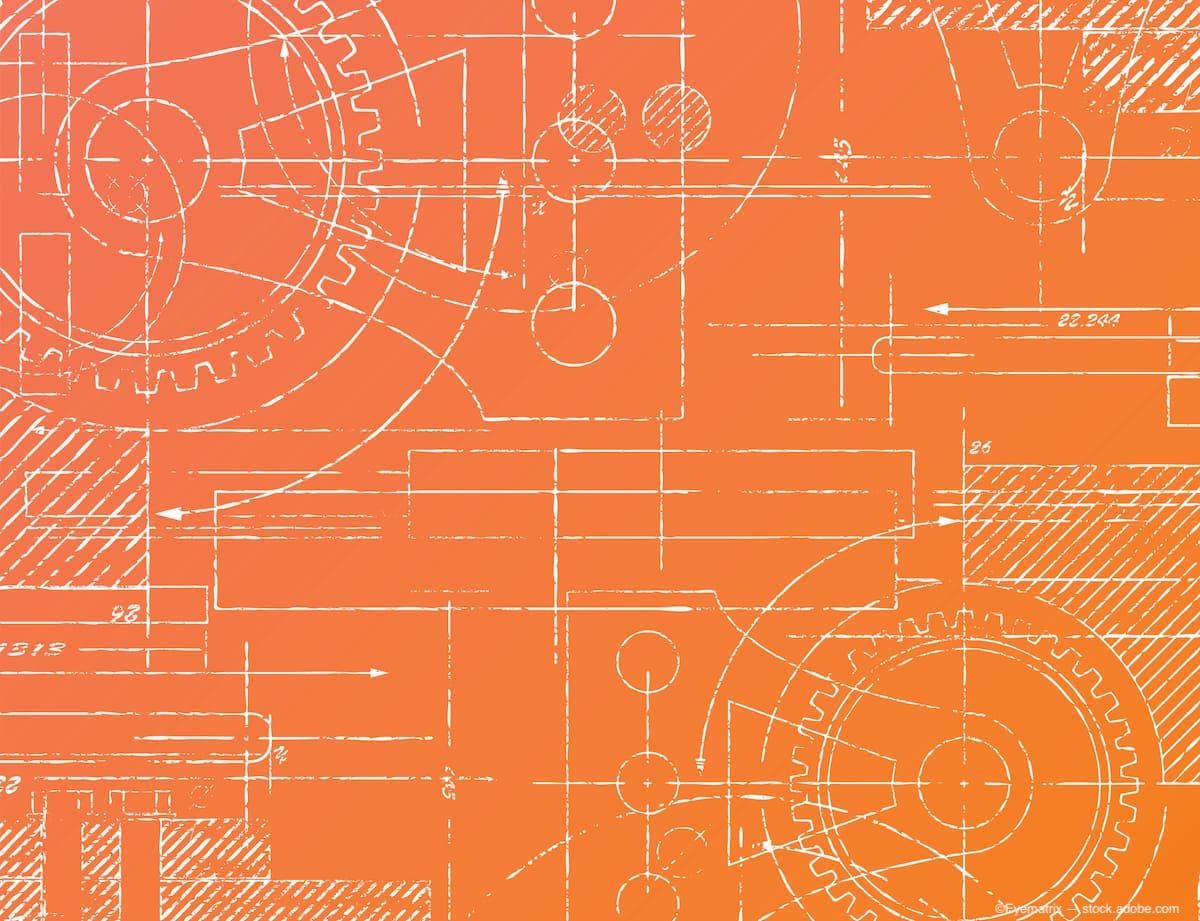
Reviewed by Owen Clinger, BS; and Gaurav Prakash, MD, FRCS
Patients are currently diagnosed with keratoconus based on the results of pachymetry, topography, or tomography. Researchers from the University of Pittsburgh in Pennsylvania propose that corneal wavefront analysis may be more advantageous because of the capability of diagnosing keratoconus earlier than with the other methods.
“This may represent a paradigm shift in the diagnosis of the disease,” according to the authors of the study, Owen Clinger, BS; Vishal Jhanji, MD; and Gaurav Prakash, MD, from the Department of Ophthalmology, University of Pittsburgh, who described their study at the American Society of Cataract and Refractive Surgery Annual Meeting in Boston, Massachusetts.
They explained that keratoconus is a chronic, progressive disease that accelerates during the early teenage years. It can stabilize when patients are in their fifth and sixth decades of life based on age, race, ethnicity, and genetic history; however, by that time the damage is done. Earlier diagnosis is ideal.
When keratoconus has not manifested clinically, clinicians using corneal wavefront analysis can examine he corneal biomechanics. Family history, genetics, and age are also useful preclinically.
Study design
The investigators conducted a study in which they evaluated the normative range and distribution patterns for the anterior, posterior, and total corneal wavefronts in patients with keratoconus and compared a subgroup analysis with other tomographic parameters. The study found some interesting early results, and the investigators described what to look for during corneal wavefront analysis to pinpoint keratoconus sooner in the disease process. Early diagnosis, the authors explained, is possible because wavefront can break down irregular corneas into their constituent aberrations.
Retrospective case series
One eye each of 1014 cases with keratoconus was included in the study. The investigators extracted corneal wavefront and tomography data from the OCULUS Pentacam. Patients with poor-quality scans and those who underwent a previous surgery were excluded. A cross-sectional subgroup analysis was performed based on severity of the keratoconus cases to create baseline data for the corneal wavefront in keratoconus.
Wavefront revelations
The investigators found that the magnitude of the root mean square of the higher-order aberrations was correlated with the stage of keratoconus, especially in the 8-mm corneal zone. “The 8-mm corneal wavefront showed stronger progression of keratoconus with good predictive fits,” they noted. They also found that third-order aberrations were the most prominent among the higher-order aberrations in all stages of keratoconus.
Other relevant findings were that coma was the most prominent aberration with all degrees of severity of keratoconus in the 6- and 8-mm corneal zones and that spherical aberrations became more prominent than trefoil aberrations in stage 3 keratoconus in the 8-mm zone and stages 3 and 4 keratoconus in the 6-mm zone. “Our study provides a large normative database of aberrations seen in keratoconus,” the researchers concluded. “We also provide a reference range for corneal wavefront in keratoconus at the 6- and 8-mm zones.”
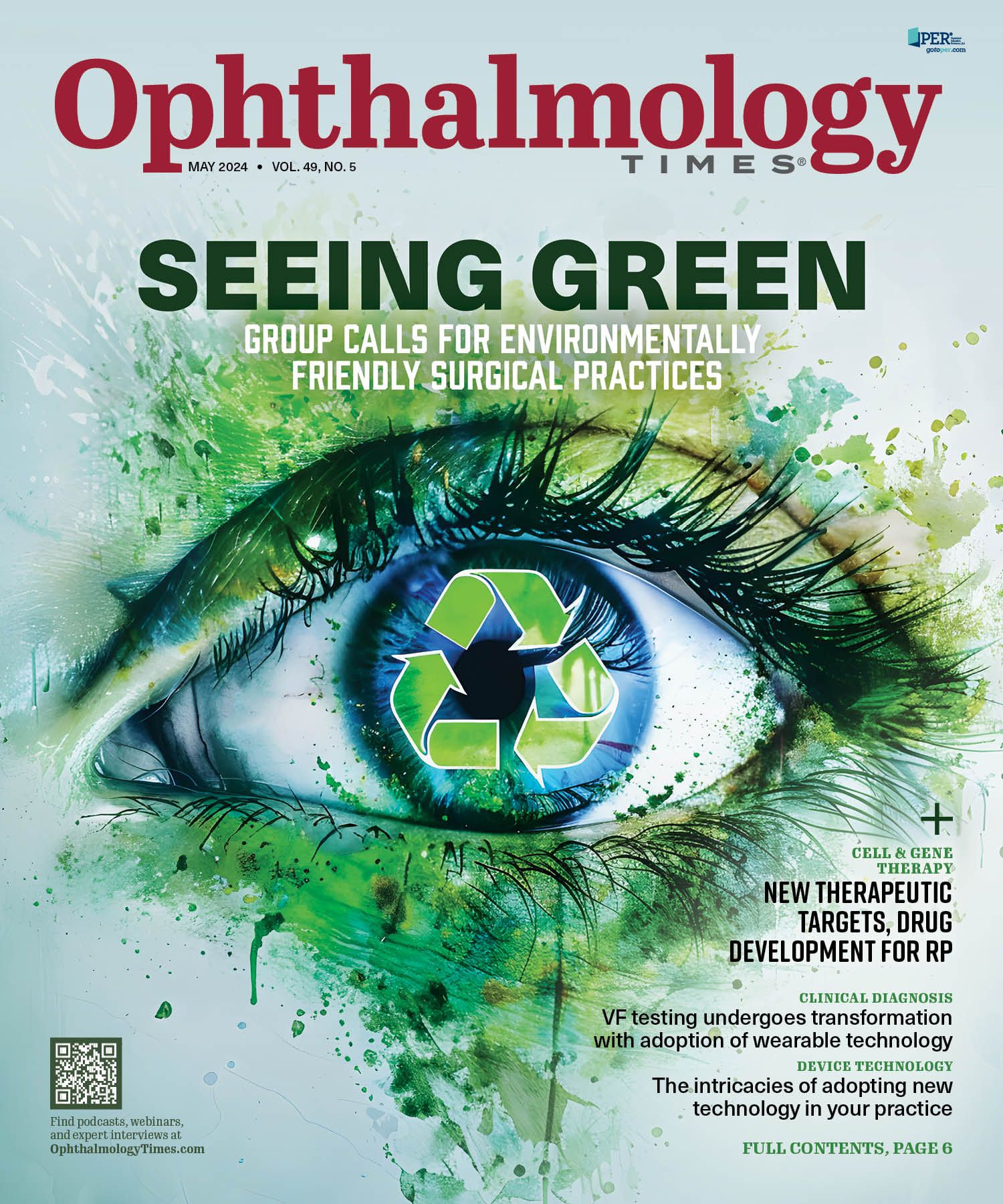
Researchers: ‘Smart’ contact lenses may one day enable wireless glaucoma detection
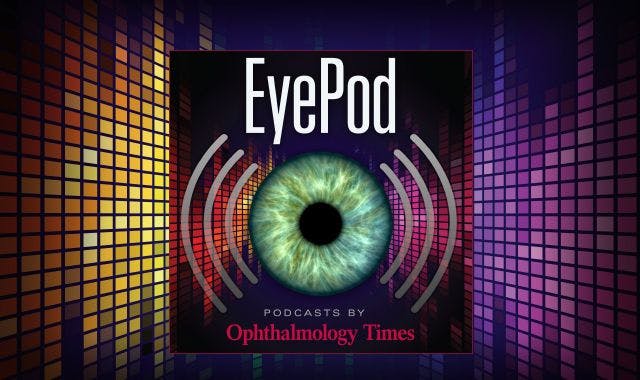
(Podcast) Confronting Demodex blepharitis: From rude awakening to FDA-approved solution
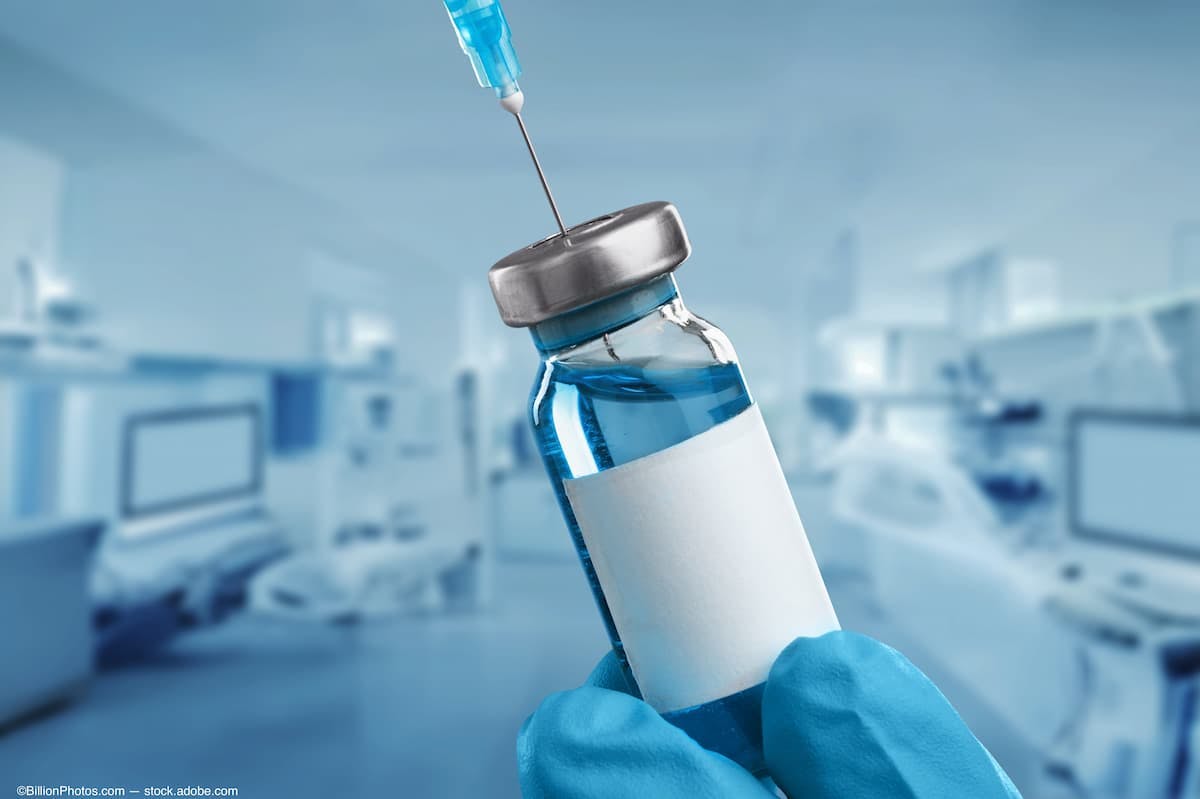
Aurion Biotech completes dosing of all subjects in Phase 1/2 CLARA trial
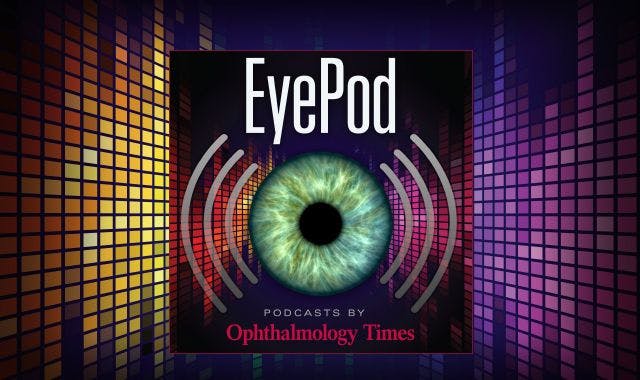
EyePod: The dry eye pipeline is more than a topical discussion
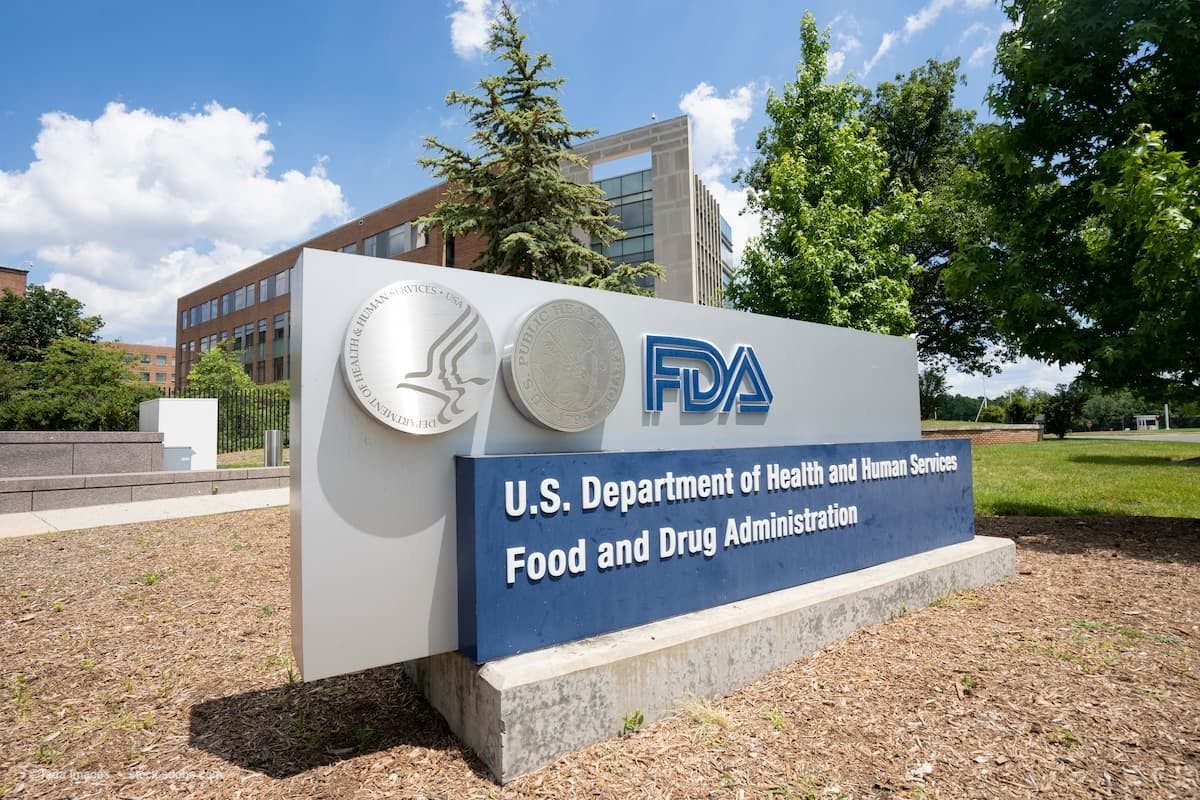
Pantheon Vision holds two pre-submission meetings with FDA
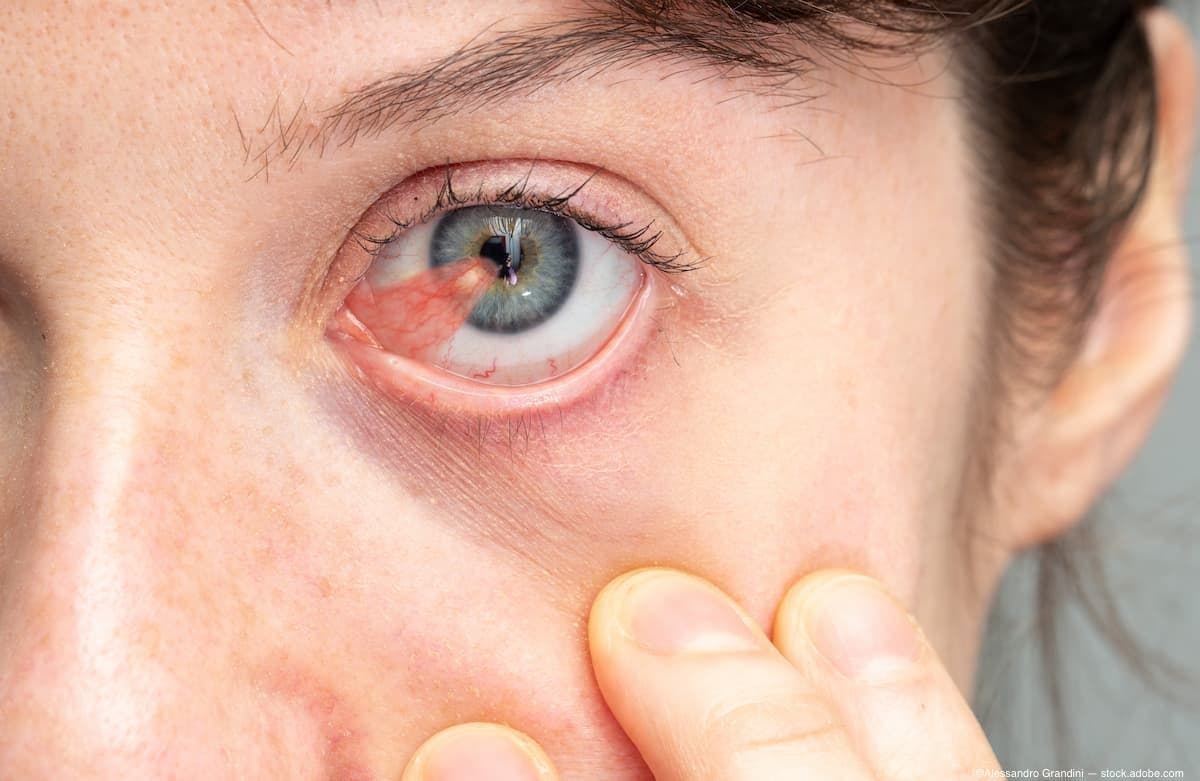
Survey by Cloudbreak Pharma shows patient frustration in treatment options for pterygium
2 Commerce Drive Cranbury, NJ 08512
609-716-7777

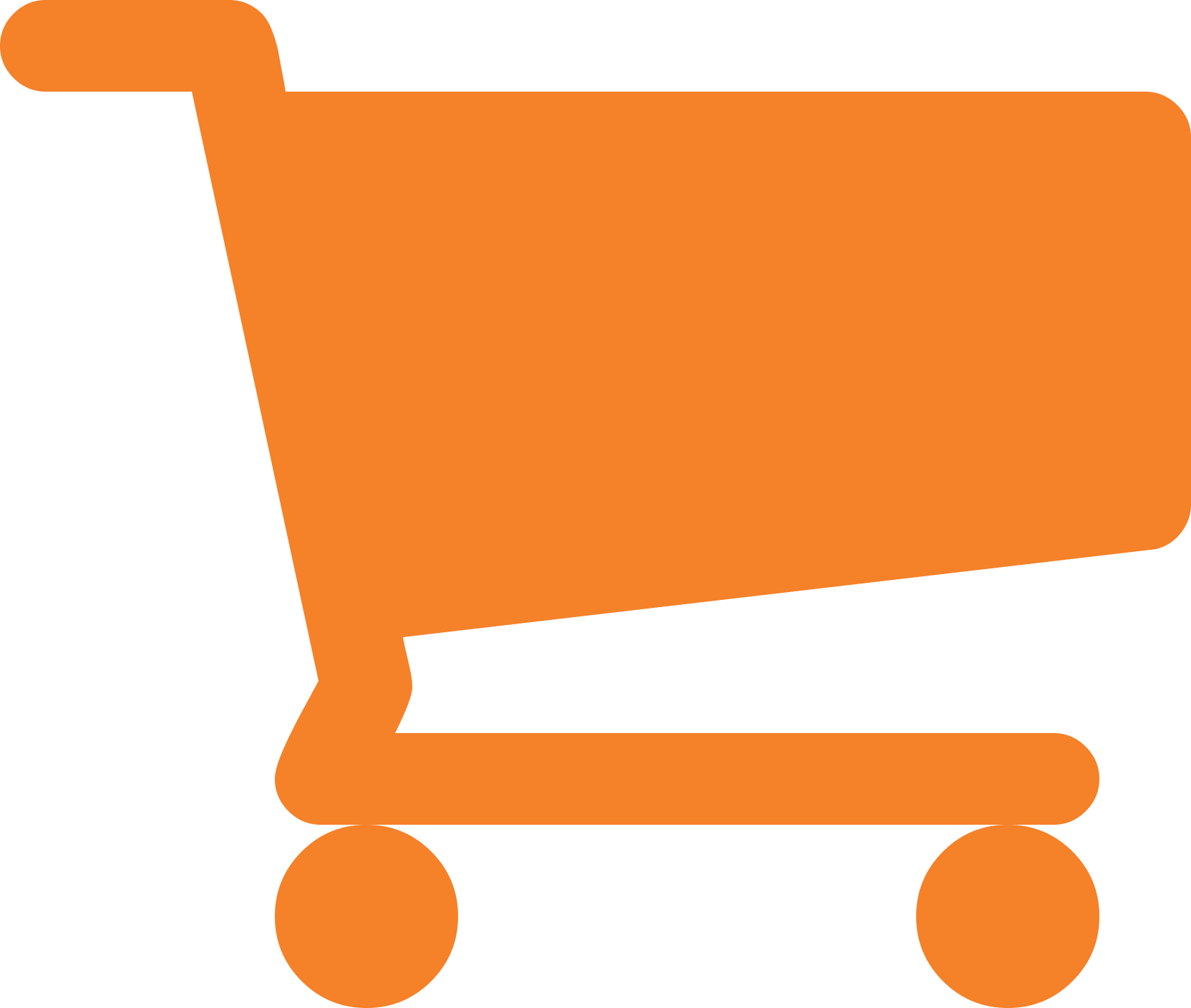
- For Ophthalmologists
- For Practice Management
- For Clinical Teams
- For Public & Patients
Museum of the Eye
- Latest Issue
- Back Issues (Nov. 2012 to present)
- Archive Highlights (pre Oct. 2012)
- Dr. Richard Mills' Opinions, 2002 to 2016
- Write For Us
- Corporate Webinars
- EyeNet Magazine
- / September 2020
- / Diagnosis and Management of Keratoconus
Diagnosis and Management of Keratoconus
- Mark Complete
NOTE: This article has been updated since print publication. Figure 3 shows a different image of Fleischer ring.
Download PDF
Keratoconus (KC) is a bilateral, progressive, noninflammatory ectatic condition in which there is conical protrusion of a thinned central cornea. Patients experience significant visual impairment from the resultant irregular astigmatism and high myopia. The worldwide prevalence of this condition is estimated to be 1.38 per 1,000. 1 KC has been found to affect all ethnicities, although the prevalence and incidence are higher among South Asians and Middle Easterners compared with those of European ancestry. 2 The condition affects both sexes, and there are contradictory studies on whether the prevalence differs significantly between the sexes. 3
Etiology and Pathogenesis
KC is a complex disease with a multifactorial etiology, likely encompassing both genetic and environmental factors. Although only 8% to 10% of KC patients have a family history of the disease, a genetic basis for the condition is supported by autosomal dominant and recessive patterns of inheritance, association with other genetic disorders, and twin concordance studies. 3 Numerous candidate genes have been identified through genomic studies.
Mechanical and other risk factors are also implicated in the development of KC. These include eye rubbing, trauma from poorly fitting contact lenses, and allergic eye disease. 3
The pathophysiology of KC is not completely understood. Biochemical instability leading to central or paracentral stromal thinning has been attributed to an imbalance between proteolytic enzymes and proteinase inhibitors. 3
Presentation and Course
Symptoms. Although KC is bilateral, it typically progresses asymmetrically. Patients commonly present with complaints of blurring, distorted vision, and frequent change in spectacle prescriptions. Other symptoms include glare, photophobia, and distorted night vision. In advanced KC, high myopia, irregular astigmatism, and stromal scars lead to significant visual impairment.
Onset and progression. The onset of KC typically occurs around the second decade of life, with the disease progressing slowly thereafter and ceasing in most patients by the fourth decade. Early in the disease, KC is asymptomatic, and many cases remain undiagnosed unless assessed by corneal tomography. Although several indices are available to monitor the progression of keratoconus, there is no consensus on which is most reliable. 3
Complications. Acute corneal hydrops, the development of stromal edema following a break in the Descemet membrane, is a potential complication of KC ( Fig. 1 ). It presents with a rapid onset of pain and loss of vision. Although corneal hydrops may resolve spontaneously within six to 10 weeks, many patients ultimately require keratoplasty because of corneal scarring. 3
Several important clinical features can aid in the diagnosis of KC.
Examination. Scissoring of the red reflex on retinoscopy is a reliable and sensitive method for detecting early-stage KC.
External indicators include the Munson sign (V-shaped deformation of the lower eyelid caused by the cone when the patient looks down; Fig. 2 ) and Rizzuti sign (conical illumination on the nasal sclera when light is directed on the cornea from the temporal side). However, these external signs are typically not observed in mild KC.
Slit-lamp evaluation. Examining the patient at the slit lamp may reveal several key diagnostic features of KC. Central and paracentral thinning of the cornea is a characteristic sign. The Fleischer ring, a yellow or brown ring encircling the cone, is caused by the deposition of hemosiderin; it is best appreciated with a cobalt blue light filter ( Fig. 3 ). Vogt striae, which are often seen in the deep stroma, are bright, parallel stress lines caused by the tension of corneal stretching. External pressure on the globe eliminates these lines on slit-lamp examination. In addition, corneal nerves can be visualized as fine white lines entering into the stroma from the limbus.
Topography and tomography. Corneal topography and tomography provide valuable information about the corneal curvature. Corneal topography allows noninvasive qualitative and quantitative characterization of corneal morphology. Topographic maps will show irregular astigmatism with steepening. The following maps are analyzed: anterior, sagittal, and tangential curvature maps; anterior and posterior elevation maps; and the thickness map. 4
Corneal tomography provides additional parameters for evaluating the anterior and posterior corneal surfaces. Early posterior corneal structural changes, including stromal thinning and elevation changes, are observed prior to anterior surface changes in KC. 4 This allows for reliable detection of early-stage KC even before a patient becomes symptomatic.
Other techniques. Other adjunctive technologies can aid in confirming the diagnosis of KC. One of these, the Ocular Response Analyzer (Reichert), evaluates corneal biomechanics by measuring corneal hysteresis, the difference in applanation pressure when the cornea bends inward in response to a jet of air and when it returns to its normal state. 4 Compared with normal corneas, keratoconic corneas typically exhibit lower corneal hysteresis values. 4
High-resolution optical coherence tomography (OCT) is a useful and rapid diagnostic adjunct modality that allows analysis and mapping of the thickness of the corneal epithelium. Epithelial mapping has shown increased overall peripheral epithelial thickness with thinner central epithelium in keratoconic eyes compared with normal eyes. 5 These changes may occur early in the disease process and are thought to be a compensatory mechanism. 5
Classification
Morphologically, KC is differentiated into three types of cones increasing in size: 1) small, isolated, round cones with steep curvature; 2) ellipsoid oval cones; and 3) large globus cones that cover the majority of the cornea.
Grading. The oldest and most commonly used grading system, the Amsler-Krumeich scale, is based on corneal thickness, anterior keratometric measurements, and refractive error.
The more recently developed classification known as the ABCD grading system incorporates average anterior radius of curvature (A) and posterior average radius of curvature (B), both measured in a 3-mm zone centered on the thinnest point of the cornea, along with thinnest pachymetry measurement (C), and best spectacle-corrected distance visual acuity (D). 6 This system integrates tomographic values and visual acuity to better characterize the anatomic and functional aspects of keratoconic corneas. 6
Differential Diagnosis
Several corneal ectatic disorders require careful differentiation. Forme fruste keratoconus (subclinical KC) is an early, asymptomatic form of the disease with no apparent clinical signs; it can be diagnosed only through analysis of corneal morphology. 3
Pellucid marginal degeneration (PMD) is a bilateral, noninflammatory ectatic disorder similar to KC. Clinically, PMD patients are typically asymptomatic, except for slow, progressive reduction in visual acuity refractory to spectacle correction. PMD is characterized by inferior corneal thinning, typically in a band-line area concentric to the limbus on slit-lamp evaluation and a “crab-claw” appearance on topography. 7
Keratoglobus is a corneal thinning disorder characterized by global thinning and protrusion. Unlike KC, it is typically nonprogressive and present from birth. While the thinning in KC is focal, keratoglobus demonstrates protrusion and thinning of the entire cornea and is more prominent in the periphery than is KC. 7
A number of approaches have been developed to improve the quality of vision in affected patients and, in some cases, to slow or stop disease progression. The choice of therapy depends on the severity of the disease and the age of the patient, as well as the contraindications and possible complications of these treatment modalities. Keratoconic patients in their third decade of life should be followed every six months. Patients with higher risk factors, including pregnancy or young age (under 20 years), require evaluation every three months. 8 Patients with severe KC often require combination therapy.
Spectacles and contact lenses. Spectacles can be used to correct astigmatism in early-stage, stable KC. When the astigmatism can no longer be managed with glasses, contact lenses are the next step. Soft contact lenses may be sufficient in mild KC, with rigid gas-permeable contact lenses becoming necessary in more advanced disease. However, although many designs are available, conventional contact lenses may be uncomfortable on a keratoconic eye, and patients may experience dryness, itching, and pain. 8
Scleral lenses. Unlike conventional contact lenses that rest directly on the cornea, scleral lenses have a larger diameter and rest on the sclera, vaulting over the cornea. With these lenses, there is a fluid layer between the lens and cornea. The PROSE (prosthetic replacement of the ocular surface ecosystem; BostonSight) treatment incorporates a scleral lens customized for each patient. Although scleral lenses have a higher cost and more challenging fitting process, they offer increased stability, improved visual outcomes, and better comfort compared with standard contact lenses. 9
Collagen cross-linking. The technique of collagen cross-linking (CXL) with ultraviolet A and riboflavin stabilizes corneal tissue, halting or arresting disease progression. 10 In addition, CXL has been found to improve BCVA by 1 to 2 lines and reduce maximum keratometry (Kmax) by 1 to 2 D. 10 Currently, CXL is recommended for patients with progressive KC who have a clear cornea and a minimum corneal thickness of 400 μm. The advent of this modality has reduced the need for keratoplasties. 11 Adverse effects include infectious keratitis, edema, and haze. 10
In early U.S. studies, custom topography-guided photorefractive keratectomy has been used as an adjunct to improve visual function and normalize remaining corneal surface abnormalities. This treatment should be deferred until the cornea has stabilized, at least three months after CXL. 12
Intracorneal ring segments. Intracorneal ring segments (ICRS) made of polymethyl methacrylate can be implanted into deep corneal stroma. Through an arc-shortening effect, ICRS flatten the corneal surface, reducing the refractive error. 13 The amount of refractive correction depends on the diameter and thickness of the rings. Shorter and thinner arcs are used to correct astigmatism, while longer and thicker arcs correct myopia. Complications of ICRS include fluctuating visual outcomes, infection, dysphotopsias, and corneal melting. 13
Keratoplasty. When less-invasive procedures are not effective, patients may require corneal transplantation. Penetrating keratoplasty (PK) for KC is an effective procedure with good visual outcomes. Recovery takes several weeks to months, with visual function stabilizing up to one year after surgery. Reported complications include allograft rejection, iatrogenic astigmatism, and recurrence of KC. Up to half of transplanted eyes will require contact lens correction for full visual rehabilitation. 14
To preserve unaffected native endothelial cells, surgeons may perform a deep anterior lamellar keratoplasty (DALK) if the Descemet membrane has not been previously ruptured, as in hydrops. While the visual outcomes are comparable to PK, DALK eliminates the risk of endothelial rejection and steroid-induced secondary glaucoma. However, Descemet membrane perforation is a potential intraoperative complication that may require conversion to PK, and interface haze may limit full visual recovery. 14
Over the past two decades, technological advancements have improved the early diagnosis and management of KC. The diagnostic workup should involve a detailed medical history, a thorough slit-lamp examination, and imaging analysis techniques such as tomography and OCT. Treatment plans remain patient specific and should be based on a collaborative discussion that appropriately addresses the individual’s concerns and expectations for visual outcome.
__________________________
1 Hashemi H et al. Cornea . 2020;39(2):263-270.
2 Bialasiewicz A, Edward DP. Middle East Afr J Ophthalmol. 2013;20(1):3-4.
3 Mas Tur V et al. Surv Ophthalmol. 2017;62(6):770-783.
4 Martinez-Abad A, Piñero DP. J Cataract Refract Surg. 2017;43(9):1213-1227.
5 Li Y et al. J Cataract Refract Surg . 2016;42(2):284-295.
6 Belin MW, Duncan JK. Klin Monbl Augenheilkd. 2016;233(6):701-707.
7 Martinez-Abad A, Piñero DP. Cont Lens Anterior Eye. 2019;42(4):341-349.
8 Gomes JA et al. Cornea . 2015;34(4):359-369.
9 Koppen C et al. Am J Ophthalmol. 2018;185:43-47.
10 Raiskup F et al. J Cataract Refract Surg. 2015;41(1):41-46.
11 Sandvik GF et al. Cornea . 2015;34(9):991-995.
12 Nattis A et al. J Cataract Refract Surg. 2018;44(8):1003-1011.
13 Park SE et al. Curr Opin Ophthalmol. 2019;30(4):220-228.
14 Mohammadpour M et al. J Curr Ophthalmol. 2017;30(2):110-124.
Mr. Nuzbrokh is a fourth-year medical student and Dr. Rosenberg is a cornea and refractive surgery fellow; both are in the Department of Ophthalmology at Weill Cornell Medical College, New York, N.Y. Dr. Nattis is a cornea specialist and director of research at SightMD, Babylon, N.Y. Relevant financial disclosures: Mr. Nuzbrokh and Dr. Rosenberg: None. Dr. Nattis: Alcon: C,L,S.
For full disclosures and the disclosure key, see below.
All content on the Academy’s website is protected by copyright law and the Terms of Service . This content may not be reproduced, copied, or put into any artificial intelligence program, including large language and generative AI models, without permission from the Academy.
- About the Academy
- Jobs at the Academy
- Financial Relationships with Industry
- Medical Disclaimer
- Privacy Policy
- Terms of Service
- Statement on Artificial Intelligence
- For Advertisers
- Ophthalmology Job Center
FOLLOW THE ACADEMY
Medical Professionals
Public & Patients
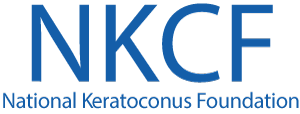
Keratoconus Research
Scientific research has answered many of our questions about keratoconus, but many more remain. Throughout the world, researchers are working to increase understanding of this disease. Each year, NKCF convenes and supports conferences to encourage scientific collaboration.
More than 150 years ago, Dr. John Nottingham, an English ophthalmologist, published a 270-page essay on the subject of the ‘conical cornea’. While many of the treatments he wrote about are no longer recommended, his descriptions of KC and vision distortions experienced by his patients could have been written by a doctor today.
Understanding the biochemical or biomechanical properties of the cornea will help researchers develop eye drops that could restore cornea thickness. Studying DNA samples will demonstrate how genetics influence disease progression. Equipment that can help diagnose KC before any vision changes are observed has been developed. Contact lenses are under constant redesign and redevelopment. In animal models, stem cells have been used successfully to help damaged corneas regenerate cells. Innovative surgical procedures using lasers and non-surgical treatments using techniques like crosslinking are being refined. Individualization of crosslinking treatments is another area being pursued by researchers.
Early research focused on trial and error disease management. Today, high-tech analysis of patient data, population studies, and corneal examination at a molecular level has led to an explosion of information. Proof of this increased attention to keratoconus is evident: there were more scientific articles published on the subject of keratoconus during 2016 that during the entire decade of the 1980s.
To learn more about keratoconus research as well as living with KC, visit nkcf.wpengine.com .
Visit the National Library of Medicine at https://www.ncbi.nlm.nih.gov/pubmed/ and use the keyword keratoconus to see the latest literature coming from the medical community.
Visit the website containing information about clinical trials hosted by the National Institutes of Health at https://clinicaltrials.gov/ .
Visit the US Department of Health and Human Services on-line database, RePORT, (Research Portfolio Online Reporting Tools) https://projectreporter.nih.gov/reporter.cfm to learn about government funding for medical research. Using search term ‘keratoconus’ will generate a list of currently funded research projects.
Want the Latest News & Research About Keratoconus?
Sign-up for our newsletter.


- Learning Center
Conditions & Research Areas
Bullous keratopathy, fuchs' dystrophy, keratoconus, refractive error, cornea transplants, endothelial keratoplasty (dsek & dmek), anterior lamellar keratoplasty (alk), penetrating keratoplasty (pk), artificial cornea, traveling to indianapolis for your transplant, descemet's stripping only (dso), other treatment options, artificial iris, cataract surgery with advanced lens options, corneal crosslinking, dry eye treatments, glaucoma surgery, lasik/refractive surgery, lens exchange, how the eye works, what is the cornea, how to care for your eyes, financial assistance/help, covid-19 and your transplant, read our latest annual report.

This detailed report includes a letter from our Chairman, Fiscal Year Financial Information, lists of studies, presentations and journal articles and recognition of our generous supporters.

Approximately 50 to 200 of every 100,000 people are afflicted with keratoconus. In the USA, a study found a prevalence of 54.5 per 100,000 people ( Kennedy et al, Am J Ophthalmol 1986; 100:267-73 ). Keratoconus occurs in all races and usually affects both eyes. Sensitive techniques, such as corneal topography, often detect keratoconus in both eyes in cases thought to be only in one eye based on physical examination and refraction. Keratoconus often starts in puberty and slowly progresses over decades and then stabilizes. In progressive cases, severe irregular astigmatism and scarring may require a corneal transplant in order to restore useful vision to the eye.
Heredity of keratoconus has not been clearly established. While there are families in which multiple persons are affected, factors such as allergic conditions and contact lens use make analysis difficult. As a general rule, chances of a blood relative developing clinical keratoconus are less than 10%.
Conditions associated with keratoconus include atopic disease (atopic or allergic dermatitis, allergic rhinitis, asthma), Down’s syndrome (5-8 % of Down’s patients), and connective tissue disorders (Ehlers-Danlos syndrome and osteogenesis imperfecta). Chronic eye rubbing is associated with keratoconus and may accelerate progression. Eye rubbing may be the common link between keratoconus and allergies, atopic disease and Down’s syndrome.
Findings in keratoconus include protrusion of the cornea, striae or wrinkles of the posterior cornea (Vogt’s striae), superficial scarring of the anterior cornea, and staining of the corneal surface epithelium with iron (Fleischer ring). Corneal hydrops, or marked swelling of the cornea in keratoconus, may occur when severe bulging of the cornea results in a tear in the deepest layer of the cornea (Descemet’s membrane), allowing fluid from the inside of the eye to permeate the cornea. Severe haziness, often accompanied by blister like lesions of the superficial cornea, results in impairment of vision and discomfort.
Keratoconus Treatments
Cross-linking is the only FDA-approved treatment for halting the progression of keratoconus. Once the condition progresses, Intacs or a cornea transplant are more advanced treatment options.
Crosslinking for Keratoconus
The Cornea Research Foundation of America contributed to the recent US Food and Drug Administration (FDA) approval of cross-linking for halting or slowing the progression of keratoconus and corneal extasia after prior refractive surgery. These studies were designed to assess the safety and effectiveness of CXL for halting or slowing the progression of these conditions. We are providing patients with continued access to cross-linking through an ongoing investigational study. View this informational brochure to learn about the procedure.
Treating visual focusing problems associated with keratoconus
Intacs for keratoconus.
If keratoconus progresses to the point where a contact lens cannot be fit or does not adequately correct vision, surgery may be indicated. Small plastic ring segments placed in the cornea can produce a more regular corneal surface in about 2 out of 3 keratoconus patients.
Cornea Transplants for Keratoconus
Historically, in about 1 out of 5 patients, keratoconus progresses to the point where a cornea transplant is needed to restore a more normal shape to the cornea. Data from the Cornea Transplant Database of corneal transplant shows that transplants performed for keratoconus are in the highest category for successful outcomes. In most cases, Dr. Price recommends the newer targeted transplant technique known as deep anterior lamellar keratoplasty (DALK) to help minimize the risk of graft rejection. Keratoconus Support Group
Historically, Keratoconus Group was established in 2007 and is the largest support community for patients with keratoconus with more than 15,000 members on their forums and discussion boards. Visit their website to connect with others experiencing keratoconus.
- Research & Findings
- Get Involved
- Medical Education
- Skip to main content
- Keyboard shortcuts for audio player
Influx of deadly street fentanyl reaching the U.S. continues to grow, research shows

Two new reports show the fentanyl crisis isn't easing, as drug cartels continue to flood U.S. communities with the deadly illicit opioid often disguised as fake prescription pills.
Copyright © 2024 NPR. All rights reserved. Visit our website terms of use and permissions pages at www.npr.org for further information.
NPR transcripts are created on a rush deadline by an NPR contractor. This text may not be in its final form and may be updated or revised in the future. Accuracy and availability may vary. The authoritative record of NPR’s programming is the audio record.
Cornell Chronicle
- Architecture & Design
- Arts & Humanities
- Business, Economics & Entrepreneurship
- Computing & Information Sciences
- Energy, Environment & Sustainability
- Food & Agriculture
- Global Reach
- Health, Nutrition & Medicine
- Law, Government & Public Policy
- Life Sciences & Veterinary Medicine
- Physical Sciences & Engineering
- Social & Behavioral Sciences
- Coronavirus
- News & Events
- Public Engagement
- New York City
- Photos of the Week
- Big Red Sports
- Freedom of Expression
- Student Life
- University Statements
Around Cornell
- All Stories
- In the News
- Expert Quotes
- Cornellians
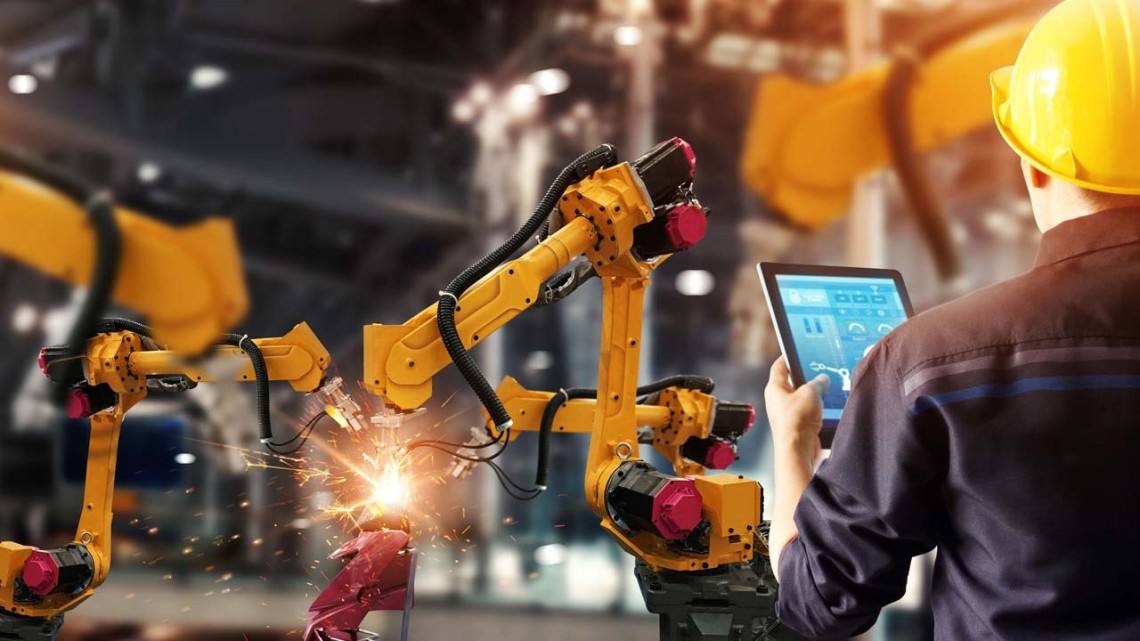
News directly from Cornell's colleges and centers
Research: Technology is changing how companies do business
By sarah mangus-sharpe.
A new study from the Cornell SC Johnson College of Business advances understanding of the U.S. production chain evolution amidst technological progress in information technology (IT), shedding light on the complex connections between business IT investments and organizational design. Advances in IT have sparked significant changes in how companies design their production processes. In the paper " Production Chain Organization in the Digital Age: Information Technology Use and Vertical Integration in U.S. Manufacturing ," which published April 30 in Management Science, Chris Forman , the Peter and Stephanie Nolan Professor in the Dyson School of Applied Economics and Management , and his co-author delved into what these changes mean for businesses and consumers.
Forman and Kristina McElheran, assistant professor of strategic management at University of Toronto, analyzed U.S. Census Bureau data of over 5,600 manufacturing plants to see how the production chains of businesses were affected by the internet revolution. Their use of census data allowed them to look inside the relationships among production units within and between companies and how transaction flows changed after companies invested in internet-enabled technology that facilitated coordination between them. The production units of many of the companies in their study concurrently sold to internal and external customers, a mix they refer to as plural selling. They found that the reduction in communication costs enabled by the internet shifted the mix toward more sales outside of the firm, or less vertical integration.
The research highlights the importance of staying ahead of the curve in technology. Companies that embrace digital technologies now are likely to be the ones that thrive in the future. And while there are still many unanswered questions about how these changes will play out, one thing is clear: The relationship between technology and business is only going to become more and more intertwined in the future.
Read the full story on the Cornell SC Johnson College of Business news site, BusinessFeed.
Media Contact
Media relations office.
Get Cornell news delivered right to your inbox.
You might also like
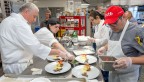
Gallery Heading
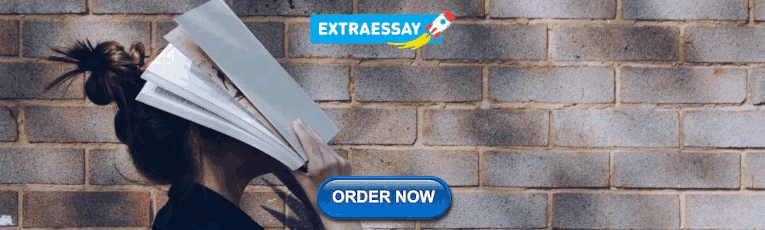
IMAGES
VIDEO
COMMENTS
Remarkably, the injected stem cells remained viable within the tissue after 6 months and acquired the ability to synthesize new collagen. 109 Although cellular/regenerative therapy for corneal diseases is an exciting field of research, caution should be exercised in generalizing these findings particularly in keratoconus, a corneal disease that ...
Keratoconus is a progressive corneal ectatic disorder characterized by thinning of corneal stroma and asymmetrical conical protrusion of the cornea, which can lead to visual impairment or even blindness [1,2,3].Keratoconus is one of the leading indications for corneal transplantation surgery worldwide [4, 5], with an incidence of 1/2000 in the general population and even higher among young ...
IVMED-80 is a relatively new treatment for keratoconus, and further research is needed to fully understand its long-term effects and safety. However, the current evidence suggests that it may be a promising non-surgical option for patients with keratoconus and an alternative to epi-off and epi-on light-induced CXL with better tolerance and ...
Current Keratoconus Research. Keratoconus is a progressive disease which causes the cornea to change shape, lose mechanical strength, and disturb the passage of light to the retina. This causes distorted vision, increased sensitivity to light and glare, frequently changing eyeglass prescription, and can lead to sudden clouding of vision.
Keratoconus: New Consensus, New Goals. In a first-ever collaboration, 4 supranational cornea societies joined forces in a complex, multistep process to develop the Global Consensus on Keratoconus and Ectatic Diseases. 1 A total of 45 keratoconus (KC) specialists—9 section coordinators and 36 expert panelists nominated by the societies ...
Keratoconus is a leading cause for visual impairment in adolescents and young adults which, untreated, can lead to legal blindness 1,2,3,4,5,6,7.The prevalence of keratoconus varies between ethnic ...
Keratoconus had traditionally been considered a rare disease at a time when the imaging technology was inept in detecting subtle manifestations, resulting in more severe disease at presentation. ... yet the current main focus of research is on increasing oxygen availability and developing new strategies to improve riboflavin permeability during ...
A quick tour of the latest breakthroughs in keratoconus treatment. Farhad Hafezi | 06/28/2023 | 7 min read | News. One fascinating aspect of ophthalmology is its rapid pace of transformation. In the past decade, we have witnessed remarkable advancements in treating diseases that once had limited therapeutic options.
Keratoconus is a bilateral and asymmetric disease which results in progressive thinning and steeping of the cornea leading to irregular astigmatism and decreased visual acuity. Traditionally, the condition has been described as a noninflammatory disease; however, more recently it has been associated with ocular inflammation. Keratoconus normally develops in the second and third decades of life ...
The keratoconus research team, comprised of some of the most accomplished experts in research and clinical care, are trying to change that. Basic science discoveries are being translated into increased understanding of the origins and progression of the disease, and exploration of new treatments and therapies. Areas of Focus
When several research papers reporting on similar findings appeared during the literature search, the most updated article (s) was typically used for review. 2. Definition. The word keratoconus derives from the Greek words 'kéras', meaning cornea, and 'cōnus', meaning cone, which together means 'cone-shaped' cornea.
January 7, 2022. Diagnosing patients early in their disease course is the best-case scenario in most conditions, and keratoconus is no exception. Finding keratoconic eyes before progression steals vision, so those eyes can be successfully treated with therapies such as corneal cross-linking (CXL), is the goal.
3.1 Diagnosis of keratoconus. Among the included studies, AI was most frequently used for the diagnosis of keratoconus and other ectasias, particularly in subclinical cases (n = 61, Supplementary Table 1) (31, 41-100).The earliest study using artificial intelligence to detect keratoconus was published in 1994 by Maeda et al. This study used eight indices from TMS-1 videokeratoscope data ...
V arious studies have suggested that the incidence rate of keratoconus is between 0.2 and 4,760 per 100,000 people and 1.5 and 25 per 100,000 people/year. 1 Previous treatment options for the masses included cornea transplant, penetrating keratoplasty, Intacs and various types of lenses-rigid gas permeable lenses, scleral lenses and soft contact lenses for milder cases.
Changing landscape of keratoconus management. Alternate methods of screening may lead to earlier diagnosis of disease. Reviewed by Owen Clinger, BS; and Gaurav Prakash, MD, FRCS. Patients are currently diagnosed with keratoconus based on the results of pachymetry, topography, or tomography. Researchers from the University of Pittsburgh in ...
Keratoconus (KC) is a bilateral, progressive, noninflammatory ectatic condition in which there is conical protrusion of a thinned central cornea. ... New York, N.Y. Dr. Nattis is a cornea specialist and director of research at SightMD, Babylon, N.Y. Relevant financial disclosures: Mr. Nuzbrokh and Dr. Rosenberg: None. Dr. Nattis: Alcon: ...
Proof of this increased attention to keratoconus is evident: there were more scientific articles published on the subject of keratoconus during 2016 that during the entire decade of the 1980s. To learn more about keratoconus research as well as living with KC, visit nkcf.wpengine.com. Visit the National Library of Medicine at https://www.ncbi ...
Keratoconus. Keratoconus (KCN) is a disease characterized by thinning and protrusion of the cornea, resulting in an irregular, conical shape (Figure 1 and 2). Irregular astigmatism occurs as the keratoconus progresses, and results in blurred vision, which can be impossible to correct with spectacles. Usually keratoconus occurs in both eyes, and ...
Insights into vitamin B2 therapy for keratoconus . Seattle, Wash. — Vitamin B2, riboflavin, is a water-soluble B vitamin that plays an important role in many bodily functions such as energy production, protecting cells from damage, and metabolizing fats, carbs and proteins. Riboflavin is found in certain foods and is sold as a dietary supplement. In 2017, a U.S. clinical trial studied ...
The researchers found an elevated risk for keratoconus (RR: 1.538, 95% CI: 1.131-2.092) in the atopic dermatitis population compared with matched controls; 103 patients (0.055%) were diagnosed with keratoconus in the study group, compared with 67 patients (0.036%) in the control group. "This retrospective study suggests a possible increased ...
Keratoconus (KC) is a disorder of the eye that results in progressive thinning of the cornea. This may result in blurry vision, double vision, nearsightedness, irregular astigmatism, and light sensitivity leading to poor quality-of-life. Usually both eyes are affected. In more severe cases a scarring or a circle may be seen within the cornea.. While the cause is unknown, it is believed to ...
In this study, the authors investigated the incidence and characteristics of suture-related complications in patients with keratoconus who underwent deep anterior lamellar keratoplasty. Overall, suture-related complications were observed in 69.2% of the eyes. Loose sutures or spontaneous suture ruptures were the most common complications.
Efficacy and Safety of Lotilaner Ophthalmic Solution 0.25% for the Treatment of Demodex Blepharitis: A Meta-Analysis of Randomized Controlled Trials. Syed Muhammad Muneeb Akhtar, Areeba Fareed, Muhammad Sohaib Asghar, Munazza Mumtaz, Sehajpreet Kaur. Article 102148.
MANN: Well, there are actually two new reports out showing the supply of illicit fentanyl smuggled into the U.S. from Mexico has surged beyond anyone's expectations. Research in the International ...
The US Department of Health and Human Services on Wednesday suspended funding to EcoHealth Alliance, a virus research organization tied to controversy about the origins of the virus that causes ...
A new study from the Cornell SC Johnson College of Business advances understanding of the U.S. production chain evolution amidst technological progress in information technology (IT), shedding light on the complex connections between business IT investments and organizational design. Advances in IT have sparked significant changes in how companies design their production processes.