Free Radicals
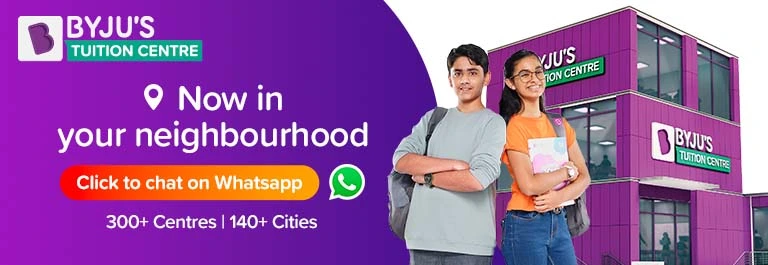
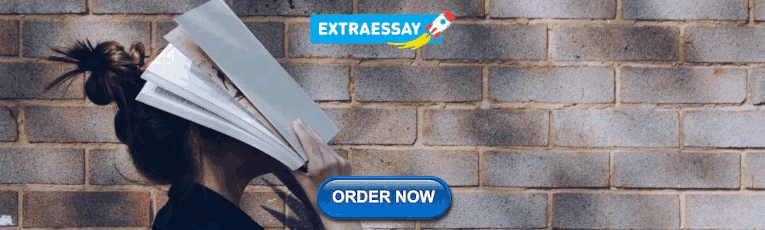
What are Free Radicals?
A free radical is termed as a molecular species which can contain an unpaired electron in its atomic orbital and can exist independently.
All the radicals share some common properties due to the unpaired electron .
Generally, molecules bear bonding electron pairs and lone pairs a nonbonding electron pair or un-shared electron pair. Each bonding or nonbonding electron pair has two electrons which are in opposite in spin orientation, +1/2 and -1/2 in one orbital based on Pauli’s exclusion principle , whereas an unpaired electron is a single electron, alone in one orbital. A molecule that has an unpaired electron is called a free radical and is a paramagnetic species.
Table of Contents
Properties of free radicals, examples of free radicals, types of free radicals, uses of free radicals, sources of free radicals.
- Frequently Asked Questions – FAQ
- Free radicals are unique and rare species and are present only under special and limited conditions. However, some free radicals are familiar to us in our lives.
- Molecular oxygen is a typical free radical, a bi-radical species. Standard and stable molecular oxygen is in triplet state and the two unpaired electrons have the same spin orientation in two orbitals, respectively, having the same orbital energy based on Hund’s rule.
- Nitrogen monoxide and nitrogen dioxide are also stable, free radical species. Moreover, the reactive species involved in immunity are oxygen free radicals, such as superoxide anion radical and singlet molecular oxygen.
- So free radicals are very familiar to us in our lives and are very important chemicals.
- Free radicals are highly reactive and very unstable. They can donate an electron or accept an electron from other molecules, therefore, can behave as oxidants or reductants.
Consider three reactive species: a methyl anion, methyl cation and methyl radical. These radicals are shown below.
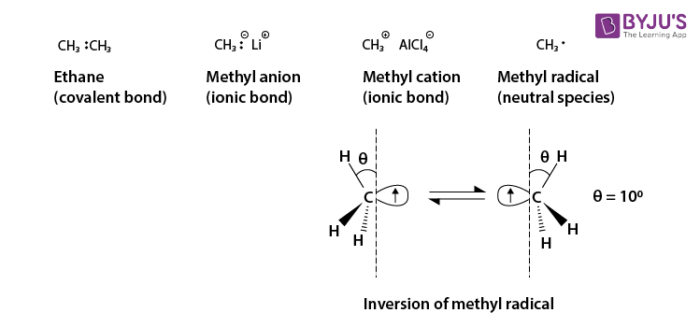
Ethane is composed of two methyl groups connected by a covalent bond and is a very stable compound. The methyl anion and methyl cation have an ionic bond mainly between carbons and counter ions respectively and are not particularly unstable though there are some rather moisture sensitive species.
However, the methyl radical is an extremely unstable and reactive species because its octet rule on the carbon is not satisfied. The carbon atom in the methyl cation adopts sp 2 hybridization and the structure is triangular and planar. The carbon atom in the methyl anion adopts sp 3 hybridization and the structure is tetrahedral, However the carbon atom in the methyl radical adopts a middle structure between the methyl cation and the methyl anion, and its pyramidal inversion rapidly occurs even at extremely low temperatures.
The most important free radical, which consists of oxygen are hydroxyl radicals
- Superoxide anion radical
- Hydrogen peroxide
- Hypochlorite
- Nitric oxide radical
- Peroxyntrite radical
Most organic radicals are quite unstable and very reactive. There are two kinds of radicals, neutral radicals and charged radicals as shown below.
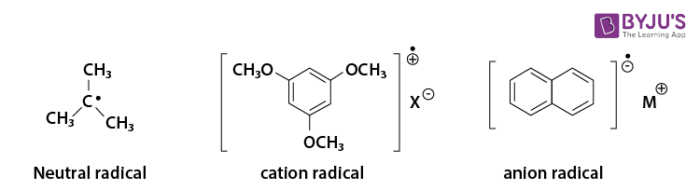
Moreover, there are two types of radicals: the sigma radicals and the pi radicals. An unpaired electron in the sigma-radical is in the sigma orbital and an unpaired electron in the pi radical is in the pi orbital respectively. Therefore, the radicals above are pi radicals, t-Butyl radical is also pi radical since this radical is stabilized by the hyperconjugation. However, the phenyl radical and the vinyl radical are typical sigma radicals.
Normally, pi radicals are stabilized by the hyperconjugation effect or the resonance effect. However, sigma radicals are very reactive because there is no such stabilizing effect.
- These highly reactive structures are present in the membranes of cells of damaging biologically relevant molecules such as the DNA, lipids, proteins, and carbohydrates etc.
- The free radicals attack important macromolecules which leads to cell damage and homeostatic disruption such as proteins, nucleic acids etc.
- Generally alkyl halides or aryl halides are used as radical precursors for R or Ar however halogenation of sugars and nucleosides which have many OH groups and other delicate functional groups is rather difficult.
- Barton Mccombie reaction is very useful for the radical reactions in sugars nucleosides and peptides.
- Other thiocarbonyl derivatives formed from alcohols with phenoxythiocarbonyl chloride, diimidazole etc can also be used instead of methyl xanthate.
Free radicals are generated internally through the following sources.
- Mitochondria
- Inflammation
- Phagocytosis
- Peroxisomes
Free radicals are found externally in the following sources.
- Environmental pollution
- Cigarette smoke
- Drugs and pesticides
- Ozone layer
Frequently Asked Questions
How do you get free radicals.
Oxidative stress arises when an oxygen molecule splits with unpaired electrons into single atoms which are considered free radicals. The body is continuously under attack due to oxidative stress. In the body, oxygen breaks up into single atoms of unpaired electrons.
What is radical in chemistry? Give example.
A radical is a chemical entity with an unpaired electron in it. A radical can be electrically neutral, radical cation charged positively, or radical anion charged negatively.
Example: In ultraviolet light, the chlorine molecule Cl 2 undergoes homolysis to form two radicals of Cl.
Is oxygen a free radical?
In the body, oxygen breaks up into single atoms of unpaired electrons. Electrons prefer to be in pairs, and these atoms, known as free radicals, scavenge the body to look for other electrons so that they can become a pair. This causes cells, proteins and DNA to become damaged.
What are the types of radicals?
Superoxide and hydroxyl radical are two essential oxygen dependent radicals. Under reducing conditions they originate from molecular oxygen. However, these same radicals can engage in unwanted side reactions resulting in cell damage due to their reactivity.
Where do free radicals come from?
Free radicals and other ROS are either derived from natural, essential metabolic processes in the human body or from external sources such as X-ray exposure, ozone, cigarette smoking, air pollution, and industrial chemical substances.
Why are free radicals so reactive?
Radicals contain unpaired electrons which makes them so reactive in that they need so much energy to shape. As we speak about radical reactivity, “more reactive” generally means a step towards more exothermic abstraction of the hydrogen atoms. That makes the reaction less prone to the carbon-centric radical’s stability.
How do free radicals affect the body?
Free radicals in the body cause similar degradation as they kill membranes of cells and make cells susceptible to decline and pathogens. Such free radicals destroy DNA and mitochondria, the fundamental building blocks of all tissues, and leave many health problems in their course.
What diseases do free radicals cause?
It is growing evidence that most of the degenerative diseases that afflict mankind derive from deleterious free radical reactions. Such disorders include atherosclerosis, cancer, inflammatory joint disease, asthma, diabetes, senile dementia and eye degeneration.
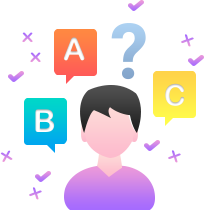
Put your understanding of this concept to test by answering a few MCQs. Click ‘Start Quiz’ to begin!
Select the correct answer and click on the “Finish” button Check your score and answers at the end of the quiz
Visit BYJU’S for all Chemistry related queries and study materials
Your result is as below
Request OTP on Voice Call
Leave a Comment Cancel reply
Your Mobile number and Email id will not be published. Required fields are marked *
Post My Comment

It’s a nice content on free radicals to give baseline information. Thanks to the author for such type of article.

Register with BYJU'S & Download Free PDFs
Register with byju's & watch live videos.
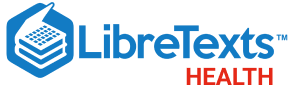
- school Campus Bookshelves
- menu_book Bookshelves
- perm_media Learning Objects
- login Login
- how_to_reg Request Instructor Account
- hub Instructor Commons
Margin Size
- Download Page (PDF)
- Download Full Book (PDF)
- Periodic Table
- Physics Constants
- Scientific Calculator
- Reference & Cite
- Tools expand_more
- Readability
selected template will load here
This action is not available.
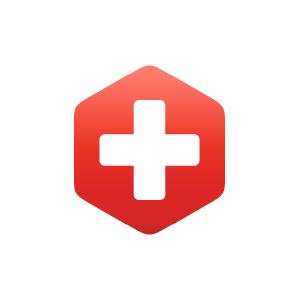
8.1: Introduction to Free Radicals and Antioxidants
- Last updated
- Save as PDF
- Page ID 21148
\( \newcommand{\vecs}[1]{\overset { \scriptstyle \rightharpoonup} {\mathbf{#1}} } \)
\( \newcommand{\vecd}[1]{\overset{-\!-\!\rightharpoonup}{\vphantom{a}\smash {#1}}} \)
\( \newcommand{\id}{\mathrm{id}}\) \( \newcommand{\Span}{\mathrm{span}}\)
( \newcommand{\kernel}{\mathrm{null}\,}\) \( \newcommand{\range}{\mathrm{range}\,}\)
\( \newcommand{\RealPart}{\mathrm{Re}}\) \( \newcommand{\ImaginaryPart}{\mathrm{Im}}\)
\( \newcommand{\Argument}{\mathrm{Arg}}\) \( \newcommand{\norm}[1]{\| #1 \|}\)
\( \newcommand{\inner}[2]{\langle #1, #2 \rangle}\)
\( \newcommand{\Span}{\mathrm{span}}\)
\( \newcommand{\id}{\mathrm{id}}\)
\( \newcommand{\kernel}{\mathrm{null}\,}\)
\( \newcommand{\range}{\mathrm{range}\,}\)
\( \newcommand{\RealPart}{\mathrm{Re}}\)
\( \newcommand{\ImaginaryPart}{\mathrm{Im}}\)
\( \newcommand{\Argument}{\mathrm{Arg}}\)
\( \newcommand{\norm}[1]{\| #1 \|}\)
\( \newcommand{\Span}{\mathrm{span}}\) \( \newcommand{\AA}{\unicode[.8,0]{x212B}}\)
\( \newcommand{\vectorA}[1]{\vec{#1}} % arrow\)
\( \newcommand{\vectorAt}[1]{\vec{\text{#1}}} % arrow\)
\( \newcommand{\vectorB}[1]{\overset { \scriptstyle \rightharpoonup} {\mathbf{#1}} } \)
\( \newcommand{\vectorC}[1]{\textbf{#1}} \)
\( \newcommand{\vectorD}[1]{\overrightarrow{#1}} \)
\( \newcommand{\vectorDt}[1]{\overrightarrow{\text{#1}}} \)
\( \newcommand{\vectE}[1]{\overset{-\!-\!\rightharpoonup}{\vphantom{a}\smash{\mathbf {#1}}}} \)
Learning Objectives
- Describe free radicals.
- Identify various free radical detoxifying enzymes and antioxidants.
- Explain oxidative stress and identify diseases associated with oxidative stress.
In mainstream advertising you may have heard that antioxidants can extend your life by preventing disease and slowing the aging process. But what are antioxidants? Where do you get them? And how do they work in the body? After reading this chapter you will be able to answer these questions, and your new knowledge will assist you in making dietary decisions to optimize your health.
Keep in mind as you read...there is no scientific evidence that antioxidants singularly provide bodily benefits, but there is evidence that certain benefits are achieved by ingesting antioxidants as part of a balanced, healthy, nutrient-rich diet. This is to say antioxidants may go a long way toward preventing damage, but other nutrients are necessary to repair damage and sustain health. No one nutrient acts alone!
Antioxidants are compounds that protect cells from damage caused by oxidation. But what is oxidation? Oxidation is a chemical reaction in which atoms lose electrons. Atoms have a nucleus (central core) which is positively charged. Orbiting around the nucleus are electrons which are negatively charged. The opposite attraction between the positively charged nucleus and negatively charged electrons keeps an atom stable. However, during metabolic reactions, atoms exchange electrons. Oxidation is the loss of electrons from an atom. Conversely, reduction is the gain of electrons by an atom. Oxidation and reduction usually occur together as an exchange reaction. One way to remember the difference between oxidation and reduction in the exchange reaction is to remember "OIL RIG":
- OIL = O xidation I s L oss of electrons
- RIG = R eduction I s G aining of electrons
Free Radicals
Oxidation sometimes results in the formation of free radicals. Remember those electrons that are orbiting the nucleus of an atom? Well those electrons contain energy; however, this energy is not always stable. The stability depends on the number of electrons that are within an atom. Atoms are more stable when their electrons orbit in pairs. An atom with an odd number of electrons must have an unpaired electron. When oxygen (a molecule with two atoms) has one unpaired electron it is known as superoxide (Figure \(\PageIndex{1}\)). Atoms and molecules such as superoxide that have unpaired electrons are called free radicals (Figure \(\PageIndex{2}\)). The unpaired electron in free radicals makes the atom or molecule unstable.
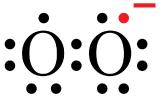
Electrons in atoms "hate" not existing in pairs. An atom with an unpaired electron (a free radical) wants to become stable again, so it quickly seeks out another electron to "steal" from another atom or molecule. The instability of free radicals is what poses a threat to macromolecules such as DNA, RNA, proteins, and fatty acids. Free radicals can cause chain reactions that ultimately damage cells. For example, a free radical may react with a fatty acid and steal one of its electrons. The fatty acid then becomes a free radical that can react with another fatty acid nearby. As this chain reaction continues, the permeability and fluidity of cell membranes changes, proteins in cell membranes experience decreased activity, and receptor proteins undergo changes in structure that either alter or stop their function. If receptor proteins designed to react to insulin levels undergo a structural change it can negatively effect glucose uptake.
Free radical reactions can continue unchecked unless stopped by a defense mechanism.
The Body’s Defense
Free radical development is unavoidable, but human bodies have adapted by setting up and maintaining defense mechanisms that reduce their impact. The body’s two major defense systems are free radical detoxifying enzymes and antioxidants. Free radical detoxifying enzyme systems are responsible for protecting the insides of cells from free radical damage. An antioxidant is any molecule that can block free radicals from stealing electrons; antioxidants act both inside and outside of cells.
Free Radical Detoxifying Enzymes
The three major enzyme systems and the chemical reactions they catalyze are:
- Superoxide Dismutases (SOD). These enzymes have either a manganese, copper, or zinc cofactor, which is essential for their free radical detoxifying activity. During SOD-mediated enzymatic catalysis, two superoxides are converted into hydrogen peroxide and oxygen. Hydrogen peroxide (H 2 O 2 ) is still considered a free radical, but it is less reactive than some other free radicals (e.g., superoxide). SOD enzymes are one of the fastest enzymes known, and they are also inducible, meaning that the higher their exposure to superoxides, the greater their number and detoxifying activity.
- Catalase. This enzyme contains iron as a cofactor and converts hydrogen peroxide to water and oxygen, thereby finishing the detoxification reaction started by SOD. In cells, catalase enzymes are found in high numbers and continuously patrol for hydrogen peroxide molecules. Catalase is highly efficient and is capable of destroying millions of hydrogen peroxide molecules per second.
- Glutathione Peroxidases. The majority of enzymes within this family are dependent on the micronutrient selenium. Similar to catalase, these enzymes convert hydrogen peroxide to water and oxygen.
Antioxidants
Antioxidants are compounds that protect cells from damage caused by oxidation. Antioxidants are broadly classified as either hydrophilic (water soluble) or hydrophobic (lipid soluble), and this classification determines where they act in the body. Hydrophilic antioxidants act in the cytosol of cells or in extracellular fluids such as blood; hydrophobic antioxidants are largely responsible for protecting cell membranes from free radical damage. The body can synthesize some antioxidants, but others must be obtained from the diet.
Antioxidants the Body Synthesizes
There are two antioxidants that the body synthesizes. They are:
- Glutathione. This molecule is composed of three amino acids and is found in high concentrations in cells. Glutathione contains a sulfur group that can donate an electron to a free radical, thereby stabilizing it. After glutathione has lost its electron, it is regenerated enzymatically so that it can perform its antioxidant function once again.
- Uric Acid. This molecule is a metabolic intermediate in the breakdown of nucleotides such as adenine, which is found in DNA and RNA, among other macromolecules. It circulates at high concentrations in the blood and disables circulating free radicals. However, too high of a concentration in the blood can cause gout, a painful joint disorder.
Antioxidants Obtained from the Diet
There are many different antioxidants in food (Table \(\PageIndex{1}\)). Antioxidant vitamins (e.g., Vitamin E, Vitamin C) donate their electrons to free radicals to stabilize them. Antioxidant phytochemicals (e.g., beta-carotene and other carotenoids) may inhibit the oxidation of lipids or donate electrons. Antioxidant minerals act as cofactors within complex antioxidant enzyme systems (e.g., superoxide dismutases, catalase, glutathione peroxidases described earlier) to convert free radicals to less damaging substances that can be excreted.
The Body’s Offense
While our bodies have acquired multiple defenses against free radicals, we also use free radicals to support its functions. For example, the immune system uses the cell-damaging properties of free radicals to kill pathogens. First, immune cells engulf an invader (such as a bacterium), then they expose it to free radicals such as hydrogen peroxide, which destroys its membrane. The invader is thus neutralized.
Free radicals are necessary for many other bodily functions as well. The thyroid gland synthesizes its own hydrogen peroxide, which is required for the production of thyroid hormone. Free radicals have been found to interact with proteins in cells to produce signaling molecules. The free radical nitric oxide has been found to help dilate blood vessels and act as a chemical messenger in the brain.
Sources of Free Radicals in the Environment
The body creates free radicals through the normal processes of metabolism (i.e., turning food into usable energy/ATP). When the amount of free radicals exceeds the body’s ability to eliminate or neutralize them, an oxidative imbalance results.
Substances and energy sources from the environment can add to or accelerate the production of free radicals within the body. Exposure to excessive sunlight, pollution, ozone, smoke, heavy metals, ionizing radiation, asbestos, and other toxic chemicals increase the amount of free radicals in the body. They do so by being free radicals themselves or by adding energy that provokes electrons to move between atoms. Excessive exposure to environmental sources of free radicals can contribute to disease by overwhelming the free radical detoxifying systems and those processes involved in repairing oxidative damage.
Oxidative Stress
Oxidative stress occurs when there is an imbalance between free radical production and their detoxification. Sustained oxidative tissue damage that can contribute to disease occurs only when free radical detoxification systems and repair systems are overwhelmed. Free radical-induced damage, when left unrepaired, destroys lipids, proteins, RNA, and DNA, and can contribute to disease. Oxidative stress has been implicated as a contributing factor to cancer, heart disease, arthritis, diabetes, kidney disease, Alzheimer’s disease, cataracts, Parkinson’s disease, and aging.
Key Takeaways
- Free radicals, unstable molecules with unpaired electrons, are an unavoidable byproduct of cellular metabolism.
- Free radicals can steal electrons from lipids, proteins, RNA, and DNA, causing them damage.
- The body has defenses against free radicals—free radical detoxifying enzymes and antioxidants.
- The body can synthesize some antioxidant molecules, but many are obtained from the diet.
- The body sometimes uses free radicals for beneficial functions such as killing pathogens.
- Oxidative stress is an imbalance between free radical production and detoxification and repair systems. It also plays an integral role in the development of many chronic diseases and in age-related decline of tissues.
- Excessive sunlight, pollution, ozone, smoke, heavy metals, radiation, asbestos, and other toxic chemicals increase the amount of free radicals in the body and can accelerate the progression of diseases in which oxidative stress is a contributing cause.

An official website of the United States government
The .gov means it’s official. Federal government websites often end in .gov or .mil. Before sharing sensitive information, make sure you’re on a federal government site.
The site is secure. The https:// ensures that you are connecting to the official website and that any information you provide is encrypted and transmitted securely.
- Publications
- Account settings
Preview improvements coming to the PMC website in October 2024. Learn More or Try it out now .
- Advanced Search
- Journal List
- Indian J Clin Biochem
- v.30(1); 2015 Jan

Free Radicals: Properties, Sources, Targets, and Their Implication in Various Diseases
Alugoju phaniendra.
Department of Biochemistry and Molecular Biology, Pondicherry University, Pondicherry, 605 014 India
Dinesh Babu Jestadi
Latha periyasamy.
Free radicals and other oxidants have gained importance in the field of biology due to their central role in various physiological conditions as well as their implication in a diverse range of diseases. The free radicals, both the reactive oxygen species (ROS) and reactive nitrogen species (RNS), are derived from both endogenous sources (mitochondria, peroxisomes, endoplasmic reticulum, phagocytic cells etc.) and exogenous sources (pollution, alcohol, tobacco smoke, heavy metals, transition metals, industrial solvents, pesticides, certain drugs like halothane, paracetamol, and radiation). Free radicals can adversely affect various important classes of biological molecules such as nucleic acids, lipids, and proteins, thereby altering the normal redox status leading to increased oxidative stress. The free radicals induced oxidative stress has been reported to be involved in several diseased conditions such as diabetes mellitus, neurodegenerative disorders (Parkinson’s disease-PD, Alzheimer’s disease-AD and Multiple sclerosis-MS), cardiovascular diseases (atherosclerosis and hypertension), respiratory diseases (asthma), cataract development, rheumatoid arthritis and in various cancers (colorectal, prostate, breast, lung, bladder cancers). This review deals with chemistry, formation and sources, and molecular targets of free radicals and it provides a brief overview on the pathogenesis of various diseased conditions caused by ROS/RNS.
In recent years there is an ever-increasing curiosity in studying the role of free radicals in biology, because of their pivotal role in various physiological conditions as well as their involvement in a diverse range of diseases. For the first time in 1900, Moses Gomberg, Professor of Chemistry at the University of Michigan, speculated the existence of an organic free radical, triphenyl methyl radical (Ph3C • ) in the living system [ 1 ]. Later in 1954, Gershman proposed “free radical theory of oxygen toxicity”, according to which, the toxicity of oxygen is due to its ability to form free radicals [ 2 ]. In the same year, the electron paramagnetic resonance (EPR) studies by Commoner et al. 1954 [ 3 ] confirmed the presence of free radicals in biological materials. Soon thereafter Denham Harman, in 1956, proposed the “free radical theory of aging”, which states that free radicals play a central role in the aging process. A second era of free radical research began in 1969 by Mc Cord and Fridovich, who discovered superoxide dismutase, the first enzymatic defense system against superoxide anion [ 4 ]. For the first time in 1971, Loschen indicated that the Reactive oxygen species are generated in cellular metabolic respiration [ 5 ], which was supported by Nohl and Hegner (1978) [ 6 ]. In 1977, Mittal and Murad reported that the hydroxyl radical, OH˙ induces the formation of the second messenger cyclic GMP by activating the enzyme guanylate cyclase [ 7 ]. Later in 1989, Hallliwell and Gutteridge reported that reactive oxygen species (ROS) include both free radical and non radical derivatives of oxygen [ 8 ]. Since then a massive data has been documented on the role of free radicals in various pathophysiological conditions.
Introduction
Free radicals are the products of normal cellular metabolism. A free radical can be defined as an atom or molecule containing one or more unpaired electrons in valency shell or outer orbit and is capable of independent existence. The odd number of electron(s) of a free radical makes it unstable, short lived and highly reactive. Because of their high reactivity, they can abstract electrons from other compounds to attain stability. Thus the attacked molecule loses its electron and becomes a free radical itself, beginning a chain reaction cascade which finally damages the living cell [ 9 ]. Both ROS and RNS collectively constitute the free radicals and other non radical reactive species [ 10 ]. The ROS/RNS play a twofold job as both beneficial and toxic compounds to the living system. At moderate or low levels ROS/RNS have beneficial effects and involve in various physiological functions such as in immune function (i.e. defense against pathogenic microorganisms), in a number of cellular signaling pathways, in mitogenic response and in redox regulation [ 11 , 12 ]. But at higher concentration, both ROS as well as RNS generate oxidative stress and nitrosative stress, respectively, causing potential damage to the biomolecules. The oxidative stress and nitrosative stress are developed when there is an excess production of ROS/RNS on one side and a deficiency of enzymatic and non enzymatic antioxidants on the other side. Most importantly, the excess ROS can damage the integrity of various biomolecules including lipids [ 13 ], proteins [ 14 ] and DNA [ 15 ] leading to increased oxidative stress in various human diseases such as diabetes mellitus, neurodegenerative diseases, rheumatoid arthritis, cataracts, cardiovascular diseases, respiratory diseases as well as in aging process. This review deals with the chemistry, formation and sources, and molecular targets of free radicals, it highlights the implication of free radicals in various diseased conditions.
Reactive Oxygen Species (ROS) and Reactive Nitrogen Species (RNS)
In general pro-oxidants/oxidants are termed as ROS/RNS. The most important free radicals produced during metabolic reactions are radicals derived from oxygen, ROS. Both the ROS and RNS can be classified into two groups of compounds namely; radicals and non-radicals (see Table 1 ). Radicals are the species which contain at least one unpaired electron in the shells around the atomic nucleus and are capable of independent existence. The oxygen molecule itself is a radical, and because of the presence of two unpaired electrons it is referred as biradical. The examples for the radicals include Superoxide ( O 2 ∙ - ), Oxygen radical ( O 2 ∙ ∙ ), Hydroxyl ( OH ∙ ), Alkoxyradical ( RO ∙ ), Peroxyl radical ( ROO ∙ ), Nitric oxide (nitrogen monoxide) ( NO ∙ ) and nitrogen dioxide ( NO 2 ∙ ) [ 17 ]. The high reactivity of these radicals is due to the presence of one unpaired electron which tends to donate it or to obtain another electron to attain stability. The non radical species include hydrogen peroxide (H 2 O 2 ), hypochlorous acid (HOCl), hypobromous acid (HOBr), ozone (O 3 ), singlet oxygen ( 1 O 2 ), nitrous acid (HNO 2 ), nitrosyl cation (NO + ), nitroxyl anion (NO − ), dinitrogen trioxide (N 2 O 3 ), dinitrogen tetraoxide (N 2 O 4 ), nitronium (nitryl) cation (NO 2 + ), organic peroxides (ROOH), aldehydes (HCOR) and peroxynitrite (ONOOH) [ 16 , 17 ]. These non radical species are not free radicals but can easily lead to free radical reactions in living organisms [ 18 ].
Table 1
List of ROS and RNS produced during metabolism [ 16 , 17 , 19 ]
a The half life of some radicals depends on the environmental medium, for example the half life of NO • in an air saturated solution may be few minutes. S seconds, min minutes
Properties of Some Free Radicals
Superoxide ion radical ( o 2 ∙ - ).
Superoxide anion radical is the most important widespread ROS formed by the enzymatic process, autooxidation reaction and by a nonenzymatic electron transfer reactions in which an electron is transferred to molecular oxygen [ 20 ]. It is mostly produced within the mitochondria and its reactivity with the biomolecules is low. The enzymes that can produce superoxide include xanthine oxidase [ 21 ], lipooxygenase, cyclooxygenase [ 22 , 23 ] and NADPH dependent oxidase. It can exist in two forms such as O 2 •− or hydroperoxyl radical (HO 2 ) at low pH [ 24 ]. The hydroperoxyl radical is the most important form and can easily enter the phospholipid bilayer than the charged form (O 2 •− ). Under physiological pH the most occurring form is superoxide. It can act as reducing agent and it reduces iron complexes such as cytochrome-c and ferric-ethylene diaminetetraacetic acid (Fe + -EDTA), in which Fe +3 is reduced to Fe +2 . It can also act as oxidizing agent and oxidize ascorbic acid and tocopherol.
Superoxide radical react with another superoxide radical in a dismutation reaction (Eq. 1 ), in which one radical is oxidized to oxygen and other is reduced to hydrogen peroxide [ 25 ]. O 2 + O 2 ∙ - + 2 H 2 O → Cu , Zn , Mn - SOD H 2 O 2 + O 2 1
Hydroxyl Radical (OH ∙ )
Hydroxyl radical is the neutral form of hydroxide ion and is a highly reactive free radical [ 26 ]. It can strongly react with both organic and inorganic molecules including DNA, proteins, lipids, and carbohydrates and cause severe damage to the cells than any other ROS can do [ 27 ]. It is formed in a Fenton reaction (Eq. 2 ), in which H 2 O 2 react with metal ions (Fe +2 or Cu + ), often bound in complex with different proteins such as ferritin (an intracellular protein that stores iron) and ceruloplasmin (plasma copper carrying protein) or other molecules [ 28 ]. Under stress conditions, an excess of O 2 •− releases free iron from ferritin and the released free iron participates in Fenton reaction to form OH • . It is also formed by the reaction between superoxide radical and H 2 O 2 in a reaction called Haber–Weiss reaction (Eq. 3 ) [ 29 ].
Peroxyl Radical (ROO ∙ )
It is derived from oxygen in living systems. The simplest form of peroxyl radical is perhydroxyl radical (HOO • ) which is formed by the protonation of superoxide [ 30 ]. About 0.3 % of the total O 2 •− in the cytosol of a typical cell is in the protonated form. It initiates fatty acid peroxidation and also can promote tumor development [ 31 ].
Hydrogen Peroxide (H 2 O 2 )
Hydrogen peroxide is formed in vivo in a dismutation reaction catalyzed by the enzyme superoxide dismutase (SOD) (Eq. 1). It is not a free radical but it can cause damage to the cell at relatively low concentration (10 μM), but at higher levels, the cellular energy producing enzymes such as glyceraldehhyde-3-phosphate dehydrogenase are inactivated. It can easily penetrate the biological membranes. H 2 O 2 has no direct effect on DNA but can damage DNA by producing hydroxyl radical (OH − ) in the presence of transition metal ions [ 32 ]. The major antioxidant enzymes that can eliminate the H 2 O 2 include catalase, glutathione peroxidase and peroxiredoxins [ 33 , 34 ].
Singlet Oxygen ( 1 O 2 )
It is an electronically high excited, meta-stable state of molecular oxygen and is a highly reactive toxic reactive oxygen species [ 35 ]. Upon activation, the molecular oxygen is excited to first state 1 Δ g and then to next higher excited singlet state, 1εg. The first excited state, 1 Δ g , has two electrons with opposite spins in the same π* orbital whereas, the second excited state, 1εg, has one electron in each degenerated π* orbital with opposite spins [ 36 ]. The 1 Δ g state is extremely reactive, and compared to the other electronically excited states [ 36 ]. It is produced in vivo by the activation of neutrophils (Eq. 4 ) [ 37 ] and eosinophils [ 38 ]. It is also formed by some of the enzymatic reactions catalyzed by enzymes such as lipoxygenases [ 39 ], dioxygenases [ 40 ], and lactoperoxidase [ 41 ]. It is a highly potent oxidizing agent that can cause DNA damage [ 42 ] and tissue damage [ 38 ].
Ozone (O 3 )
Ozone is a powerful oxidant may be produced in vivo by antibody catalyzed water oxidation pathway which plays an important role in inflammation [ 43 ]. It can form free radicals and other reactive intermediates by oxidizing the biological molecules. It can cause lipid peroxidation [ 44 ] and oxidize different functional groups, for example, amine, alcohol, aldehyde and sulphydryl, present in proteins [ 45 , 46 ] and nucleic acids [ 47 ]. It can also cause chromosomal aberrations which may be due to direct attack by O 3 or by the free radicals generated by it [ 48 ].
Hypochlorous Acid (HOCl)
It is a major oxidant produced by the activated neutrophils at the site of inflammation from hydrogen peroxide and chloride in a reaction catalyzed by the enzyme myeloperoxidase [ 49 ].
HOCl is a strong reactive species involved in oxidation and chlorination reactions. It can oxidize thiols and other biological molecules including, ascorbate, urate, pyridine nucleotides, and tryptophan [ 50 , 51 ]. HOCl chlorinates several compounds such as amines to give chloramines; tyrosyl residues to give ring chlorinated products, cholesterol and unsaturated lipids to give chlorohydrins, and it can also chlorinate DNA [ 52 ].
Nitric Oxide or Nitrogen Monoxide (NO • )
It is a small molecule generated in tissues by different nitric oxide synthases (NOS)s which convert L-arginine to L-citrulline [ 53 ]. In this reaction one of the terminal guanido nitrogen atoms undergo oxidation and produces NO . . Three types of isoforms of NOS such as neuronal NOS (nNOS), endothelial NOS (eNOS) and inducible NOS (iNOS) are involved in the formation of the NO radical.
It is both aqueous and lipid soluble and therefore it readily diffuses through cytoplasm and plasma membrane [ 54 ]. The NO • is an important intracellular second messenger stimulates guanylate cyclase and protein kinases and helps in smooth muscle relaxation in blood vessels. It is identical to endothelium derived relaxing factor (EDRF) produced by vascular endothelial cells which is an important mediator of vascular responses [ 55 ]. It can also act as an important cellular redox regulator [ 56 ] and regulate enzymatic activity by nitrosylating the proteins [ 57 ]. Since it is involved in many biological activities like blood pressure regulation, smooth muscle relaxation, neurotransmission, defensive mechanisms and immune regulation, this molecule was regarded as molecule of the year 1992 [ 58 ].
Peroxynitrite (OONO − ) and Other Reactive Nitrogen Species
Peroxynitrite (OONO − ) is formed by the reaction between O 2 ∙ - and NO ∙ . It is highly toxic [ 59 ] and can directly react with CO 2 to form other highly reactive nitroso peroxo carboxylate (ONOOCO 2 − ) or peroxynitrous acid (ONOOH). The ONOOH further undergo homolysis to form both OH • and NO 2 or rearrange to form NO 3 . OONO - can oxidize lipids, oxidize methionine and tyrosine residues in proteins and oxidizes DNA to form nitroguanine [ 60 ]. The nitrotyrosine residues are considered as marker of peroxynitrite induced cellular damage [ 61 ].
NO reacts with O 2 and water to form nitrate and nitrite ions. One electron oxidation of NO • results in nitrosonium cation ( NO 2 ∙ + ) while on electron reduction results in nitroxyl anion (NO − ). These two ions can react with NO and form N 2 O and OH ∙ . NO ∙ can react with a variety of radicals such as H 2 O 2 and HOCl to form N 2 O 3 , NO 2 − and NO 3 − [ 62 ].
Sources of Free Radicals
The ROS can be produced from either endogenous or exogenous sources. The endogenous sources of ROS include different cellular organs such as mitochondria, peroxisomes and endoplasmic reticulum, where the oxygen consumption is high.
Mitochondria
Most of the intracellular ROS are derived from mitochondria (Fig. 1 ). The superoxide radicals are produced at two major sites in the electron transport chain, namely complex I (NADH dehydrogenase) and complex III (ubiquinone cytochrome c reductase). The transfer of electrons from complex I or II to coenzyme Q or ubiquinone (Q) results in the formation of reduced form of coenzyme Q (QH 2 ). The reduced form QH 2 regenerates coenzyme Q via an unstable intermediate semiquinone anion ( ∙ Q - ) in the Q-cycle. The formed ∙ Q - immediately transfers electrons to molecular oxygen leading to the formation of superoxide radical. The generation of superoxide is non-enzymatic and therefore higher the metabolic rate, the greater is the production of the ROS [ 63 ].

Mitochondrial ROS production
The superoxide anion is converted to hydrogen peroxide by the action of mitochondrial superoxide dismutase (MnSOD). H2O2 can be detoxified by the Catalase (CAT) and glutathione peroxidase (GPx).
The other mitochondrial components which contribute to the formation of ROS include monoamino oxidase, α-ketoglutarae dehydrogenase, glycerol phosphate dehydrogenase and p66shc [ 64 ].
The p66Shc is an important member of the ShcA protein family, which contains another two more proteins, p46Shc and p52 Shc. The mammalian p66Shc is a 66-kDa isoform of the growth factor adaptor protein involved in apoptosis. It mediates the production of ROS in mitochondria. Most of the p66Shc is located in cytoplasm with a small fraction localized in the mitochondrial intermembrane space. Upon oxidative stress, p66Shc translocates to mitochondrial intermembrane space, where it associates with cytochrome-c, thus inducing ROS generation [ 65 ].
Peroxisomes
In peroxisomes the respiratory pathway involves the transfer of electrons from various metabolites to the oxygen leads to H 2 O 2 formation [ 66 ], but is not coupled to oxidative phosphorylation to produce ATP instead free energy is released in the form of heat. The other free radicals produced in peroxisomes include H 2 O 2 , O 2 •− OH • and NO • . The β-oxidation of fatty acids is the major metabolic process producing H 2 O 2 in the peroxisomes. As reviewed elsewhere, the different peroxisomal enzymes such as acyl CoA oxidases, D-amino acid oxidase, L-α-hydroxy oxidase, urate oxidase, xanthine oxidase, D-aspartate oxidase have been shown to produce different ROS [ 67 ] [see Table 2 ].
Table 2
ROS producing enzymes in peroxisomes
Endoplasmic Reticulum
The enzymes of endoplasmic reticulum such as cytochrome p-450 and b5 enzymes and diamine oxidase contribute to the formation of ROS [ 68 ]. Another important thiol oxidase enzyme, Erop1p catalyses the transfer of electrons from dithiols to molecular oxygen results in the formation of H 2 O 2 [ 69 ].
The other endogenous sources of ROS include prostaglandin synthesis, auto-oxidation of adrenalin, phagocytic cells, reduced riboflavin, FMNH 2 , FADH 2 , cytochrome P 450, immune cell activation, inflammation, mental stress, excessive exercise, infection, cancer, aging, ischemia etc. [ 68 ].
On the other hand, ROS are also produced in the biological systems by various exogenous sources shown in Table 3 [ 10 ].
Table 3
ROS generated from exogenous sources
Molecular targets of free radicals
When there is an imbalance between the free radical production (ROS/RNS) and antioxidant defenses, the former will be produced in higher concentrations leading to oxidative stress and nitrosative stress. Since these free radicals are highly reactive, they can damage all the three important classes of biological molecules including nucleic acids, proteins, and lipids [ 70 ].
Deoxyribonucleic Acid (DNA)
Both ROS/RNS can oxidatively damage the nucleic acids. The mitochondrial DNA is more vulnerable to the ROS attack than the nuclear DNA, because it is located in close proximity to the ROS generated place. ROS, most importantly, the OH • radical directly reacts with all components of DNA such as purine and pyrimidine bases, deoxyribose sugar backbone [ 71 ] and causes a number of alternations including single and double stranded breaks in DNA. The OH ∙ radical abstracts hydrogen atoms to produce a number of modified puine as well as pyrimidine base by-products and DNA- protein cross links. The pyrimidine attack by OH • produces different pyrimidine adducts like thymine glycol, uracil glycol, 5-hydroxydeoxy uridine, 5-hydroxy deoxycytidine, hydantoin and others. The purine adducts formed by hydroxyl radical attack include, 8-hydroxydeoxy guanosine, 8-hydroxy deoxy adenosine, 2,6-diamino-4-hydroxy-5-formamidopyrimidine. The other free radical induced adducts of DNA bases include, 5-formyl uracil, cytosine glycol, 5,6-dihydrothyronine, 5-hydroxy-6-hydro-cytosine, 5-hydroxy-6-hydro uracil, uracil glycol, and alloxan [ 72 ]. The major free radical induced adducts of the sugar moiety in DNA include glycolic acid, 2-deoxytetrodialdose, erythrose, 2-deoxypentonic acid lactone, 2-deoxypentose-4-ulose [ 72 ]. 8-hydroxy deoxyguanosine is considered as the biomarker of oxidative DNA damage and is involved in mutagenesis, carcinogenesis and ageing. The levels of 8-OHdG are higher in mitochondrial DNA than in nuclear DNA [ 73 ].
On the other hand, the RNS, most importantly, peroxynitrite (OONO − ) interacts with guanine to produce nitrative and oxidative DNA lesions such as 8-nitroguanine and 8-oxodeoxyguanosine respectively [ 74 ]. 8-nitroguanine formed is unstable and can be spontaneously removed, resulting in the formation of an apurininc site [ 75 ]. Conversely adenine can be paired with 8-nitroguanine during DNA synthesis resulting in a G-T transversions [ 76 ]. Accordingly 8-nitroguanine is a mutagenic DNA lesion involved in carcinogenesis.
Ribonucleic acid (RNA)
ROS can attack different RNAs produced in the body. The RNA is more prone to oxidative damage than DNA, due to its single stranded nature, lack of an active repair mechanism for oxidized RNA, less protection by proteins than DNA and moreover these cytoplasmic RNAs are located in close proximity to the mitochondria where loads of ROS are produced. Indeed, RNA is subjected to more oxidative damage than DNA in humans [ 77 ]. 7, 8-dihydro-8-oxo-guanosine (8-oxoG) is the most extensively studied RNA damage product and its levels are raised in various pathological conditions like Alzheimer’s disease [ 78 ], Parkinson’s disease [ 79 ], atherosclerosis [ 80 ], hemochromastosis [ 81 ] and myopathies [ 82 ].
The membrane lipids, especially the polyunsaturated fatty acid residues of phospholipids are more susceptible to oxidation by free radicals [ 83 ]. The lipid peroxidation is very important in vivo because of its involvement in various pathological conditions. The lipid peroxidation results in the loss of membrane functioning, for example, decreased fluidity, inactivation of membrane bound enzymes and receptors [ 84 ]. The lipid peroxidation is initiated, when any free radical attacks and abstracts hydrogen from a methylene groups (CH 2 ) in a fatty acid (LH) which results in the formation of a carbon centered lipid radical (L • ). The lipid radical can react with molecular oxygen to form a lipid peroxyl radical (LOO • ). The resultant lipid peroxyl radical (LOO • ) undergo rearrangement via a cyclisation reaction to form endoperoxides, which finally form malondialdehyde (MDA) and 4-hydroxyl nonenal (4-HNA), the toxic end products of lipid peroxidation that cause damage to the DNA and proteins [ 85 ]. These lipid peroxyl radicals can further propagate the peroxidation process by abstracting hydrogen atoms from the other lipid molecules. Isoprostanes (prostaglandin like substances produced by in the body by the esterfication of arachidonic acid) constitute the important product of lipid peroxidation of arachidonic acid and are considered as the makers of the oxidative lipid damage [ 86 ].
The protein oxidation can be induced by radical species such as O 2 •− , OH • , peroxyl, alkoxyl, hydroperoxyl as well as by the non radical species such as H 2 O 2 , O 3 , HOCl, singlet oxygen, OONO - [ 87 ]. ROS oxidize different amino acids present in the proteins, causing formation of protein–protein cross linkages, results in the denaturing and loss of functioning of proteins, loss of enzyme activity, loss of function of receptors and transport proteins [ 88 ]. The sulphur containing amino acids such as methionine and cysteine are more susceptible to oxidation by ROS and are converted to disulphides and methionine sulphoxide [ 89 , 90 ] respectively. However in biological systems, only these two oxidized forms of proteins can be converted back to their native form by two different enzymes namely disulfide reductases and methionine sulfoxide reductases respectively [ 91 – 94 ].
The ROS mediated attack of different amino acids results in the formation of different oxidation products such as, tryptophan forms nitrotryptophan, kynurenine, formylkynurinine; Phenylalanine forms 2,3-Dihydroxyphenylalanine, 2-, 3-, and 4-hydroxyphenylalanine; Tyrosine forms 3,4-Dihydroxyphenylalanine, tyrosine–tyrosine cross-linkages, Tyr-O-Tyr, cross-linked nitrotyrosine; Histidine forms 2-Oxohistidine, asparagine, aspartic acid; Arginine forms glutamic semialdehyde; Lysine forms a-Aminoadipic semialdehyde; Proline forms 2-Pyrrolidone, 4- and 5-hydroxyproline pyroglutamic acid, glutamic semialdehyde; threonine forms 2-Amino-3-ketobutyric acid; leucine and valine residues form hydroxyl residues [ 91 ].
The ROS induced oxidative damage of amino acid residues such as lysine, proline, threonine and arginine yields carbonyl derivatives. The presence of carbonyl groups in proteins has been considered as the marker of ROS mediated protein oxidation [ 95 ]. The other specific markers of protein oxidation are O-tyrosine (a marker for hydroxyl radical) and 3-nitrotyrosine (a marker for RNS). An increase in the levels of protein carbonyls is observed in a number of pathological conditions such as, Alzheimer’s disease [ 96 ], parkinson’s disease [ 97 ], muscular dystrophy [ 98 ], cataractogenesis [ 99 ], Rheumatoid Arthritis [ 100 ], diabetes [ 101 ], progeria, atherosclerosis, respiratory dystrous syndrome, Werner’s syndrome [ 91 ], and ageing [ 96 , 102 ].
Free Radicals and Diseases
Free radicals are involved in many pathological conditions such as many types of diabetes, neurodegenerative diseases, cardiovascular diseases (CVDs), cancer, cataracts, asthma, rheumatoid arthritis, inflammation, burns, intestinal tract diseases, progerias and ischemic and post-ischemic pathologies. The role of free radicals in some of the important disease conditions (see Fig. 2 ) is discussed in this section.

Pathological role of free radicals
Diabetes Mellitus
Diabetes mellitus is heterogeneous group of chronic disorders characterized by enhanced blood glucose levels (hyperglycemia) resulting from defective insulin secretion (in type I diabetes), resistance to insulin action (in type II diabetes) or both [ 103 ]. The major symptoms are thirst, hunger, emaciation, and weakness, eventually lead to coma. DM is associated with the increased production of free radicals or decreased activity of the antioxidant systems, which leads to development of oxidative stress [ 104 , 105 ]. The hyperglycemic condition induces increased free radical production via four different routes namely, 1) increased glycolysis, results in increased ratio between the rate of oxidation of G3P to 1, 3-DPG, following increased NADH/NAD + ratio (redox imbalance) 2) activated sorbitol (or polyol) pathway, causes the accumulation of both sorbitol and fructose, results in decreased reduced GSH and increased NADH/NAD + ratio. 3) autoxidation of glucose, results in the generation of different free radicals such as H2O2, OH • , O 2 •− and ketoaldehydes and 4) non enzymatic protein glycation, results in the formation of AGEs which upon interacting with RAGEs generate oxidative stress [ 106 ].
Both mitochondrial and non-mitochondrial derived ROS contribute to oxidative stress during DM. Under normal conditions, the electron transport chain complexes I and III are the key sites of superoxide production [ 107 ]. However, the increased glucose levels in DM lead to increased glycolysis resulting in the augmented generation of pyruvate, thus raising the inner mitochondrial membrane potential upwards, followed by mitochondrial dysfunction and increased ROS production at electron transport chain complex II [ 108 ].
Neurodegenrative Diseases
The central nervous system (CNS) is particularly susceptible to the oxidants due to the presence of high lipid content, high consumption of oxygen, and low levels of antioxidant enzymes, for example, SOD is localized primarily in neurons, and GSH and GPx are localized in astrocytes [ 109 ]. The lipid peroxidation by ROS leads to progressive loss of membrane fluidity, decreases membrane potential, and increases permeability to ions such as Ca 2+ . The regions of the brain such as hippocampus, substantia nigra, and the striatum are particularly susceptible to attack by free radicals [ 110 , 111 ]. The oxidative-stress state has been also implicated in several neurodegenerative diseases such as Alzheimer’s [ 112 ], Parkinson’s [ 113 ], Huntington’s, lateral amyotrophic sclerosis [ 114 ], and multiple Sclerosis [ 115 ].
Parkinson’s Disease (PD)
Parkinson’s disease (PD) is characterized by the loss of dopaminergic neurons (involve in learning, memory and motor control), especially in the midbrain area called the substantia nigra, accompanied by deposition of inclusion bodies (Lewy bodies) of α-synuclein. The redox imbalance causes oxidative damage to these neurons and begins to alter the synthesis and metabolic pathway of dopamine leads to a further increase in oxidative stress because of quinine formation [ 111 ]. The characteristic clinical symptoms of PD include, jerky movements, trembling of the hands and lips, and tremors [ 116 ]. Dopamine, a neurotransmitter, can also act as a metal chelator, has the ability to generate H 2 O 2 via Fenton reaction. Ceruloplasmin (an extracellular ferroxidase required for regulating cellular iron load and transport) oxidation results in the decreased ferroxidase activity followed by the accumulation of intracellular iron in neurons in PD. The increased levels of Fe +3 mediate the production of hydroxyl radicals, results in the damage of dopaminergic neurons in PD [ 117 ].
Alzheimer’s Disease (AD)
Alzheimer’s disease (AD) is characterized by the accumulation of amyloid protein plaques (formed from the improper folding and processing of amyloid β precursor protein-ABPP) [ 168 ] and intracellular neurofibrillary tangles made up of abnormal and hyperphosphorylated tau protein [ 118 ]. The hyperphosphorylated tau protein aggregates binds to Fe 3+ , results in the production of neurofibrillary tangles [ 119 ]. The Amyloid-β peptide (Aβ) can chelate with transition metal ions (Cu 2+ , Zn 2+ and Fe 3+ ), and produce H 2 O 2 via transition metal mediated catlysis and finally gives toxic OH˙ radical [ 120 ]. The lipid peroxidation is also extensive in AD patients, which can induce neuronal death by multiple mechanisms such as impairment of function of ion pumps (both Na + /K + -ATPase and Ca +2 -ATPase), glucose transporters and glutamate transporters. The other oxidative markers of protein damage such as protein carbonyls and 3-nitrotyrosine have been also observed in AD patients [ 118 ].
Multiple Sclerosis (MS)
MS is an autoimmune neuronal disorder characterized by impaired nerve conduction due to demylination of central nervous system (CNS). The activated microglia/macrophages initiate the MS by the generation of ROS [ 121 ] that can induce lipid peroxidation, results in the demylination and damage of neurons. Elevated TBARS levels and reduced protein SH groups, the representatives of protein oxidation and slightly reduced SOD was reported in MS patients [ 122 ]. Apart from ROS generation, an impaired iron metabolism has been also considered to play a major role in pathogenesis of disease.
It is one of the leading causes of death in humans. Free radicals cause different types of chemical changes in DNA, thus they could be mutagenic and involved in the etiology of cancer [ 123 , 124 ]. Cancer cells in particular, in comparison to normal cells, have higher levels of ROS and are more susceptible to mitochondrial dysfunction due to their higher metabolic rate [ 125 ]. Cancer cells display elevated levels of oxidative stress due to activation of oncogenes and loss of tumor suppressors [ 126 ]. ROS by altering the growth signals and gene expression cause continuous proliferation of cancer cells [ 127 ]. ROS can damage DNA by inducing base modifications, deletions, strand breakage, chromosomal rearrangements and hyper- and hypo-methylation of DNA [ 128 ].
Colorectal Cancer
Colorectal cancer (CRC) is the third most common cancer worldwide, accounting for 608,000 deaths per year [ 129 ]. The gastrointestinal tract, particularly the colon and rectum, is continuously exposed to ROS originating from both endogenous and exogenous sources [ 130 ]. Colon cancer originates from the epithelial cells that line the bowel. These cells divide rapidly and have a high metabolic rate [ 131 ]. Since the intestinal mucosa is constantly confronting with diet and bacterial-derived oxidants and carcinogens, an unrestrained production of free radicals, redox imbalance, and DNA damage occurs, finally leads to an altered intestinal metabolic homeostasis with cancer as an endpoint [ 133 ]. The human colorectal tumors have increased levels of nitric oxide (NO) [ 132 ], 8-oxodG in DNA [ 133 ], and lipid peroxides [ 134 ]. Suzuki et al. 2004 [ 135 ] have reported increased serum levels of oxidized low density lipoprotein in patients with CRC compared to healthy individuals.
Breast Cancer
Damage to the breast epithelium by ROS can lead to fibroblasts proliferation, hyperplasia of epithelium, cellular atypia and breast cancer [ 136 ]. In majority of breast carcinomas the oxidative stress can be induced by the over expression of thymidine phosphorylase enzyme which catabolizes thymidine to thymine and 2-deoxy-D-ribose-1-phosphate; the latter is a powerful reducing sugar that rapidly glycates proteins, generating oxygen radicals within the carcinoma cell [ 137 ]. Another breast specific mechanism of oxidative stress induction involves a mammary gland specific lactoperoxidase enzyme catalyzed one electron oxidation of 17-β-oestradiol to a reactive phenoxyl radical [ 138 ].
Prostate Cancer
The ROS produced are responsible for the cellular proliferation of prostate cancer cells [ 139 ]. Overexpression of NADPH oxidase 1 (Nox1) protein is an early event in the development of prostate cancer. Prostrate Tumors have considerably higher levels of ROS and Nox1 levels [ 140 ]. The superoxide produced (by NOX) in prostate cancer cells facilitates cellular immortality through resistance to programmed cell death which results in cancer-promoting effect [ 141 ]. Kumar et al. 2008 [ 142 ] have reported elevated ROS levels in prostate cancer cells compared with normal cells. Veeramani et al. 2008 [ 143 ] have reported that elevated levels of ROS might also be formed by the simultaneous increased levels of p66Shc protein in prostate cancer cells.
Lung Cancer
Lung cancer has been the most commonly diagnosed cancer and is the central cause of cancer death in men worldwide [ 144 ]. Lung cancer mortality account 30 % of all cancer related deaths. Oxidative stress plays an important role in lung inflammation and lung cancer [ 145 ]. Cigarette Smoking is the most crucial environmental risk factor in lung cancer etiology. It is estimated that smoking accounts for ~80 % of global lung cancer burden in males and 50 % in females [ 146 ]. Cigarette smoke particulate matter contains complex mixture of numerous carcinogens and stable ROS with very long half-lives. These ROS can damage the tissues resulting in progressive transformation of cells into the malignant form, which leads to increased frequency of mutations by the oxidative damage to DNA and, eventually leading to lung cancer [ 147 ]. Smokers develop lung cancer a 10-fold higher than non-smokers. Lung cancer (LC) and chronic obstructive pulmonary disease (COPD) commonly coexist in smokers, and the presence of COPD increases the risk of developing LC [ 148 ].
Bladder Cancer
Bladder cancer is one of the most common cancers across the world, ranking the fourth and tenth in men and women, respectively [ 149 ]. The most common risk factors for bladder cancer are cigarette smoking, exposure to industrial carcinogens (aromatic amines), high levels of arsenic intake and diet [ 150 ]. Oxidative stress critically contributes to the development of bladder cancer [ 151 ]. Various lines of evidence reported an increased oxidative stress in patients with breast cancer [ 152 , 153 ]. Increased NO levels have been reported in bladder cancer patients [ 154 ]. This NO stimulates matrix metalloproteinases (MMPs), especially prolidase activity, which is involved in the terminal step of collagen degradation. Significantly higher serum prolidase activities were reported in patients with bladder cancer than healthy controls [ 155 ]. Therefore, increased prolidase activity may, in part, play a role in the pathogenesis of bladder cancer.
Epidemiological studies reveal that low levels of antioxidants are associated with an increased risk of cancer. Significant increase in total oxidant status levels and decrease in total antioxidant status were observed in patients with bladder cancer [ 156 ]. Significantly lower levels of plasma protein, total thiol groups and protein-bound thiol groups and elevated levels of Protein carbonyl groups were observed in bladder cancer patients than in healthy controls [ 157 ].
Cardiovascular Diseases (CVDs)
Cardiovascular diseases are a class of pathologies involving the heart and blood vessels (arteries, capillaries, and veins). They include cardiac diseases, vascular diseases of the brain and kidney, and peripheral arterial disease. Most of the people are dying due to CVDs compared to other diseases [ 158 ].
Atherosclerosis
Atherosclerosis is a condition commonly referred to as hardening of the arteries. Hyperlipidemia is a major risk factor for atherosclerosis. Elevated levels of oxidized low density lipoprotein (LDL), glucose and free fatty acids are found in patients with atherosclerosis, T2D, and obesity [ 159 ]. A profound imbalance of oxidants and antioxidants resulting in oxidative stress is observed in atherosclerosis. In the vessel wall, endothelial cells, smooth muscle cells (SMCs) and macrophages are sources of free radicals [ 160 ]. Endothelial dysfunction leads to increased endothelial permeability, up regulation of endothelial adhesion molecules, and inflammatory cell infiltration into the arterial wall [ 160 ]. A substantial data has been shown that ROS are involved in endothelial injury, dysfunction, and lesion progression [ 161 ]. The ROS dependent activation of the MMPs results in the degradation of intimal extracellular matrices and promotes smooth muscle cell migration [ 162 ]. Cigarette smoking contain large amount of free radicals and may down-regulate key exogenous and endogenous antioixdants such as vitamin-D, carotenes, GPx and SOD and can lead to the dysfunction of monocytes and vascular smooth muscle cells [ 163 ]. The proatherogenic agents such as oxidaised lipids, high glucose and cigarette constituents give rise to increased free radical production.
The endothelial nitric oxide synthase (eNOS) derived NO . plays an important role in maintaining vascular tone and vasoreactivity, vasodialation, platelet aggregation and in maintaining balance between smooth muscle cell growth and differentiation. Decreased NO bioavailabilty is the one of the major feature of the CVDs [249]. During reduced availability of BH4 or L-Arg, eNOS becomes uncoupled from a NO ∙ to a O 2 ∙ - state [ 164 ]. The O 2 •− formed can interact with NO • to produce ONOO - , a damaging and cytotoxic free radical with potential to disturb cardiovascular function. ONOO - reduces the bioavailability of NO leading to reduced endothelial vascular regulatory capacity and increased vascular dysfunction. ONOO − also inactivates the BH4 cofactor effectively amplifying the damaging effects of eNOS uncoupling the endothelium [ 164 ]. Mitochondrial DNA damage is frequently observed in human atherosclerosis in both circulating and vessel wall cells [ 165 ]. Oxidative stress mediated damaged mitochondrial DNA that escape autophagy induces a potent inflammatory response in atherosclerosis [ 166 ]. Malfunction of DNA repair leads to defects in cell proliferation, apoptosis, and mitochondrial dysfunction, which in turn leads to ketosis, hyperlipidemia, and increased fat storage, promoting atherosclerosis and the metabolic syndrome [ 167 ].
Hypertension (HT)
Hypertension (HT) is a major health problem worldwide account 40 % of the total adult population [ 168 ]. Persons with hypertension are at an increased risk for stroke, heart disease, kidney failure, and premature mortality. Free radical induced oxidative stress in part contributes to endothelial dysfunction and development of hypertension [ 169 ]. Increased ROS generation eliminates NO • by forming ONOO - , thus reducing NO • bioavailability which leads to decreased endothelium-dependent vasodilation resulting in hypertension [ 170 ]. A decrease in NO bioavailability and an increase in oxidative stress are present in human hypertension [ 171 ]. Oxidation-induced impairment of NO also results in reduced opposition to the vasoconstrictive and hypertensive effects of angiotensin II. Angiotensin II decreases NO bioavailability by promoting oxidative stress [ 172 ].
It is the most common cause of the visual impairment affecting about 25 million people throughout the world, with the highest incidence occurring in developing countries [ 173 , 174 ]. It is characterized by opacity of the eye lens that reduces the amount of incoming light and results in visual impairment [ 173 ]. Although a number of factors such as genetic factors, diabetes, aging, smoking, drugs, malnutrition, radiation (x-rays and UV rays) and alteration in both endocrine and enzymatic equilibrium have been implicated in cataract formation [ 175 ], the free radical induced oxidative stress is considered as one of the major underlying mechanism of cataract disorder [ 176 ]. Oxidation of proteins, lipids and DNA is seen in cataract lenses. Proteins lose sulfhydryl (–SH) groups become cross linked by non disulfide bonds, form high molecular aggregates and become insoluble [ 177 ]. The oxidative stress induced lipid peroxidation toxic product such as HNE induce the fragmentation of lens proteins contributing towards the opacity of the lens [ 178 ]. Oxidative stress has been shown to induce lens opacification both in experimental animal models and cultured lens systems [ 179 ].
The cornea absorbs the light in the range of above 300 nm results in the activation of tryptophan, to form N-formyl kynurenine, 3-hydroxy kynurenine and other photoproducts [ 180 , 181 ]. These photoproducts gradually accumulate in the centre of the lens are capable of generating singlet oxygen which induce protein damage leading to the loss of transparency [ 181 ]. Cataract lens have an intracellular ionic imbalance (i.e. altered ionic homeostasis) than normal lens. The ROS induced by UV rays in sunlight alters the ionic homeostasis results in the increased levels of Ca +2 and Na +2 , coupled with the decreased levels of Mg +2 and K +2 in the lens [ 182 ]. The increased calcium activates calpains, a family of calcium dependent non-lysosomal cysteine proteases [ 175 ], which degrade lens proteins such as both α and β crystalline proteins results in opaque lens characteristic of cataract [ 183 ]. Elevated levels of H 2 O 2 were observed in cataract lenses than normal lenses [ 178 , 184 ].
Rheumatoid Arthritis (RA)
RA is chronic multisystem disease of unknown cause which affects approximately 1–2 % of the total world population and women are affected more than men [ 185 ]. The disease is characterized by synovial and systemic inflammation with joint swelling, morning stiffness, destruction of articular tissues, joint deformities, fatigue, loss of appetite and weakness [ 186 – 189 ]. It is believed to be a T-lymphocyte driven disease in which a sudden influx of T-cells into the affected joints is followed by an increased number of macrophages and fibroblasts drawn the release of cytokines particularly IL-1 and TNF-alpha. This cytokine release and subsequent migration is thought to be responsible for the chronic inflammation and characteristic changes in RA [ 188 ].
Several lines of evidence suggest a role for oxidative stress in the pathogenesis of RA. Both ROS and RNS damage cartilage. Tissue injury in inflammation results in NO . production by articular chondrocytes and synovial fibroblasts and elevated levels of NO . are observed in the serum and synovial fluid of RA patients [ 190 ]. The free radicals, particularly NO ∙ and O 2 •− , inhibit the synthesis of matrix components like proteoglycans by chondrocytes and also damage the extracellular matrix through activation and up regulation of matrix metalloproteinases [ 191 ]. The HOCl, produced by myeloperoxidase (MPO) in neutrophils, chlorinate the tyrosine residues to form 3-chlorotyrosine and damage the collagen, thus implicated in arthritogensis. RA patients have increased plasma MPO concentrations [ 186 ].
Elevated levels of MDA, NO • , protein carbonyls, oxidized hyaluronic acid and oxidized LDL have been reported in RA patients [ 192 – 195 ]. These oxidized LDL can be ingested in large quantities by monocytes results in the formation of Foam cells that are present in atherosclerotic plaques of vessles and have also been found in RA synovial fluid [ 196 ]. Cigarette smoking is also considered as the most established environmental factor for RA. Both particulate and gaseous phases of smoke contain high concentrations of free radicals that can interact with DNA and could cause genetic mutations and activation responsible for the development of RA [ 197 ].
Free radicals are involved in various respiratory diseases such as respiratory distress syndrome, chronic obstructive pulmonary disease, chronic bronchitis, asthma [ 198 ]. Asthma is the most common disorders of the airways of the lungs and is one of the major global health problems [ 199 ]. It is characterized by chronic inflammation of the airways involving variable and recurrent airflow obstruction and bronchial hyperreactivity associated with airway remodelling [ 200 , 201 ]. Airway remodeling is a dynamic process involving mucous hypersecretion, collagen deposition, wall thickening, myocyte hypertrophy and hyperplasia, myofibroblast hyperplasia, vascular proliferation and alterations in airway elastic fibers, all of which culminate in persistent structural alterations of the airway [ 202 ]. NO is endogenously produced in mammalian airways by NOS and is known to regulate many aspects of human asthma, including modulation of airway and vascular smooth muscle tone and the inflammation. Increased production of airway NO is a key factor in the development of airway hyperresponsiveness [ 203 ].
ROS are produced both intracellularly by lung parenchymal cells and extracellularly by lung macrophages. Increased generation of oxidants have been reported in asthma patients than in healthy individuals [ 204 ] which provoked airway inflammation by inducing diverse pro-inflammatory mediators including macrophages, neutrophils and eosinophils [ 205 ]. Numerous studies have suggested that oxidative stress is caused by overproduction of various free radicals or by an insufficient antioxidant defense system in asthma and thus it contributes to the tissue damage which is induced by inflammatory cells [ 206 ]. Elevated levels of oxidative stress markers such as H 2 O 2 , 8-isoprostane, nitric oxide, and carbon monoxide were reported in exhaled air of asthmatic patients [ 204 ]. Increased MDA levels, and Protein carbonyls; decreased protein sulfhydryl and antioxidant activity were observed in plasma, bronchoalveolar lavage (BAL) fluid and exhaled air of asthamatic patients [ 207 – 209 ].
Free radicals (ROS/RNS) are produced by normal metabolism and are involved in various physiological and pathological conditions. When there is an imbalance between the antioxidants and oxidants, the fee radicals accumulate leading to vigorous damage to macromolecules such as nucleic acids, proteins and lipids. This leads to tissue damage in various disease conditions such as diabetes mellitus, neurodegenerative diseases, cancer, cardiovascular diseases, cataracts, rheumatoid arthritis, asthma etc. and thus severely hastening the disease progression.
Contributor Information
Alugoju Phaniendra, Email: [email protected] .
Dinesh Babu Jestadi, Email: [email protected] .
Latha Periyasamy, Email: [email protected] .
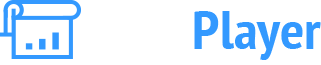
- My presentations
Auth with social network:
Download presentation
We think you have liked this presentation. If you wish to download it, please recommend it to your friends in any social system. Share buttons are a little bit lower. Thank you!
Presentation is loading. Please wait.
Free radicals and Antioxidants
Published by Kathleen Dixon Modified over 9 years ago
Similar presentations
Presentation on theme: "Free radicals and Antioxidants"— Presentation transcript:
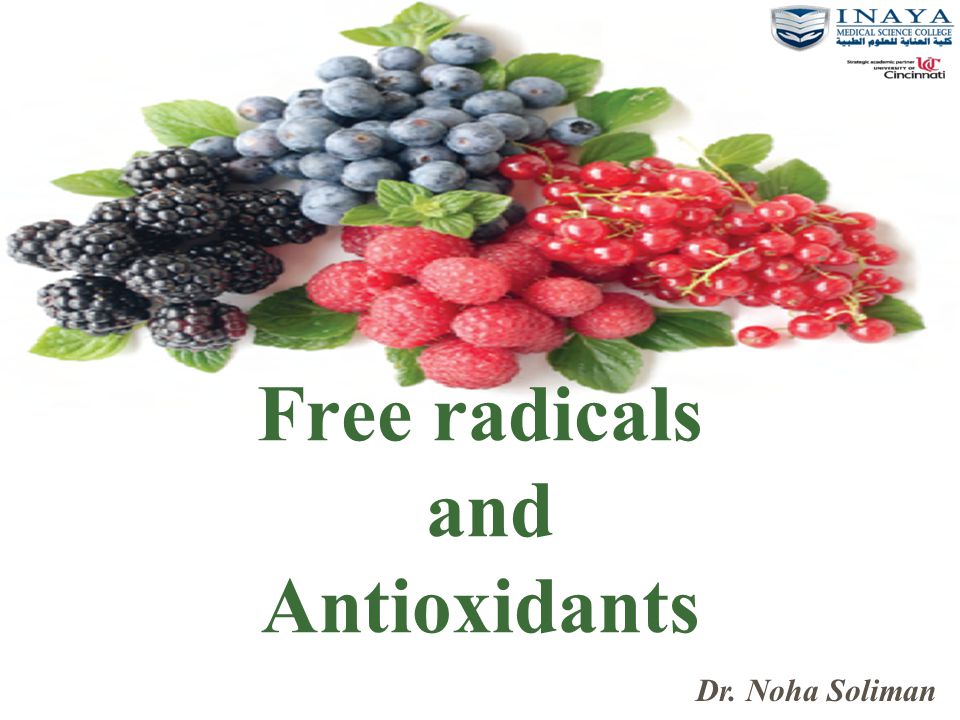
Lipid Peroxidation 1 st Year MBBS Lipid Peroxidation refers to the oxidative degradation of lipids.oxidativelipids It is the process in which.
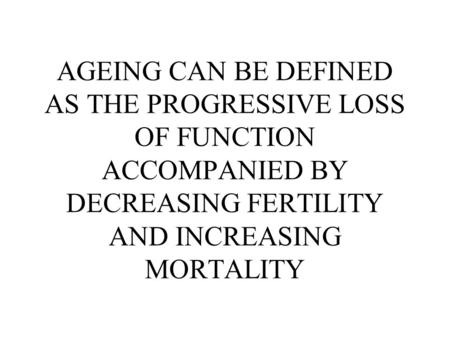
AGEING CAN BE DEFINED AS THE PROGRESSIVE LOSS OF FUNCTION ACCOMPANIED BY DECREASING FERTILITY AND INCREASING MORTALITY.
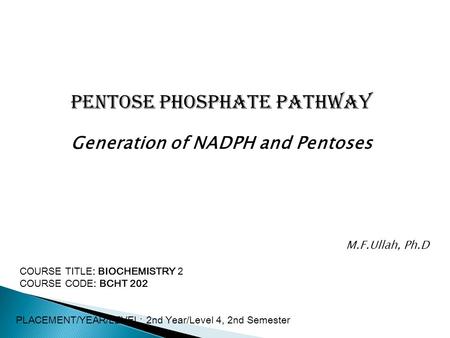
Pentose Phosphate Pathway Generation of NADPH and Pentoses COURSE TITLE: BIOCHEMISTRY 2 COURSE CODE: BCHT 202 PLACEMENT/YEAR/LEVEL: 2nd Year/Level 4, 2nd.
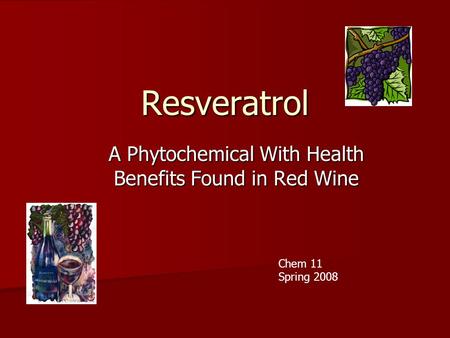
Resveratrol A Phytochemical With Health Benefits Found in Red Wine Chem 11 Spring 2008.
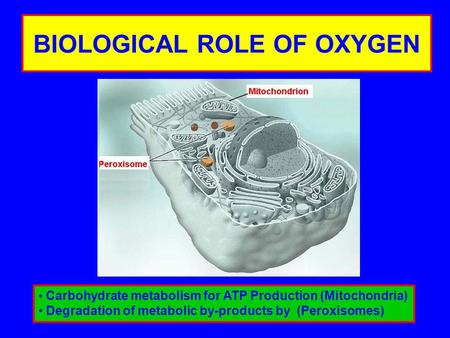
BIOLOGICAL ROLE OF OXYGEN
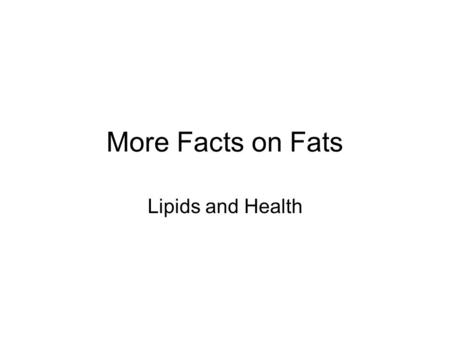
More Facts on Fats Lipids and Health.
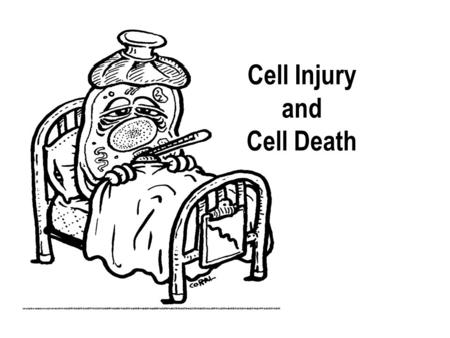
Cell Injury and Cell Death

A Concentrated Energy Source
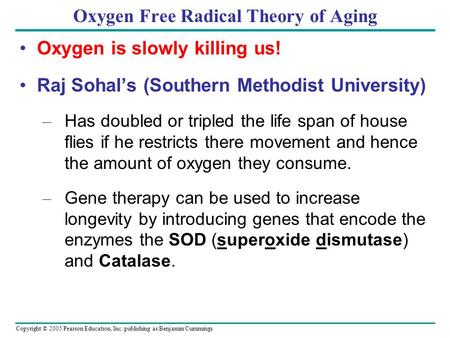
Copyright © 2005 Pearson Education, Inc. publishing as Benjamin Cummings Oxygen Free Radical Theory of Aging Oxygen is slowly killing us! Raj Sohal’s (Southern.
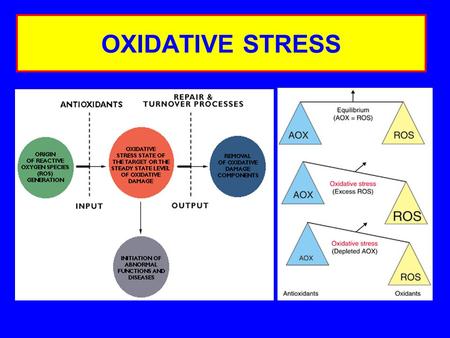
OXIDATIVE STRESS. INDUCTION OF OXIDATIVE STRESS Glutathione conjugation MalnutritionMutation Phagocytic activation Tissue damageSmoking.
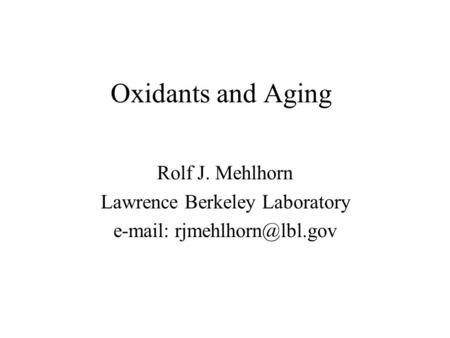
Oxidants and Aging Rolf J. Mehlhorn Lawrence Berkeley Laboratory
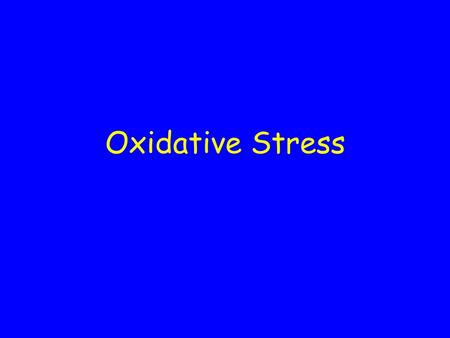
Oxidative Stress.
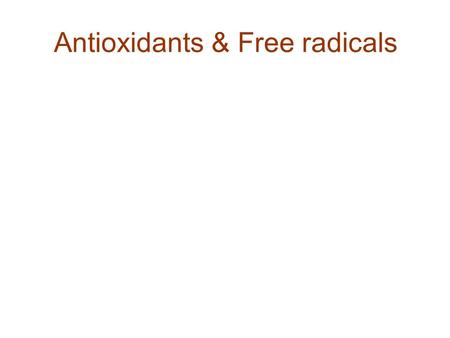
Antioxidants & Free radicals. What are Reactive Oxygen Species? ROS also known as Free oxygen radicals Any molecule with an unpaired electron
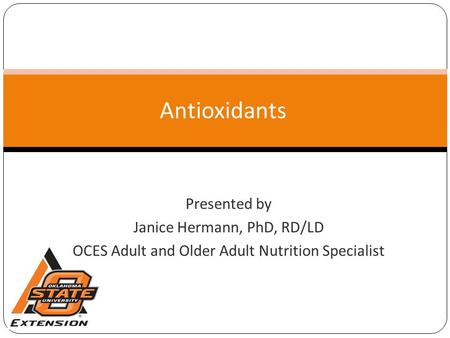
Antioxidants Presented by Janice Hermann, PhD, RD/LD OCES Adult and Older Adult Nutrition Specialist.
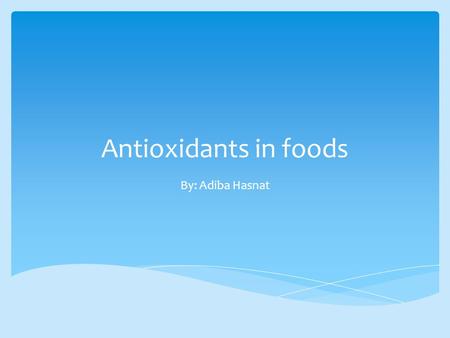
Antioxidants in foods By: Adiba Hasnat.
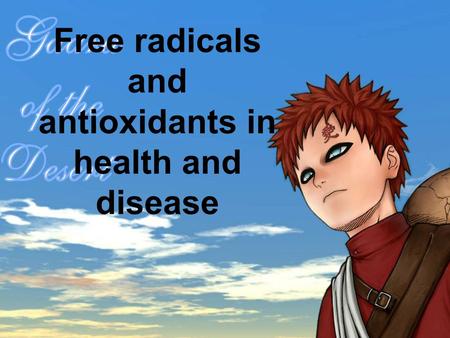
Free radicals and antioxidants in health and disease
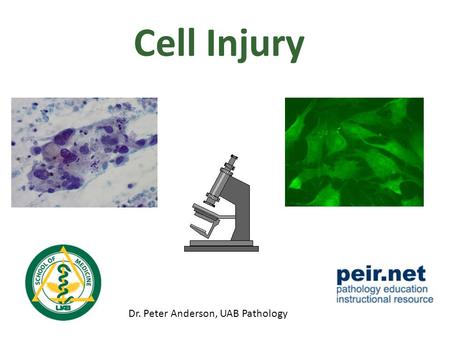
Cell Injury Dr. Peter Anderson, UAB Pathology.
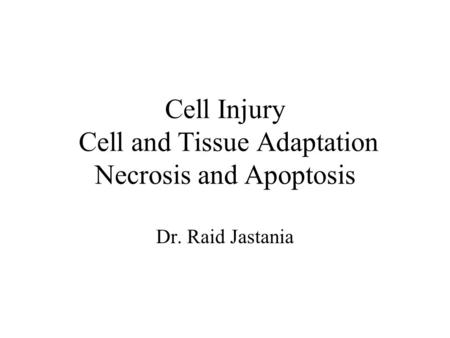
Cell Injury Cell and Tissue Adaptation Necrosis and Apoptosis Dr. Raid Jastania.
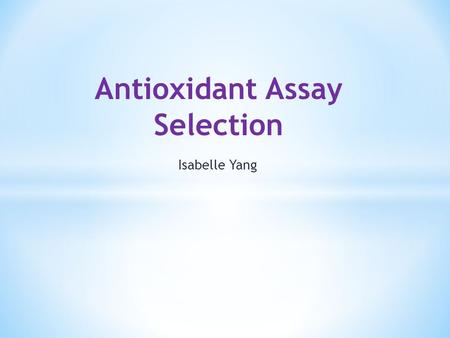
Isabelle Yang Antioxidant Assay Selection. Generally: Oxidation is loss of electrons.
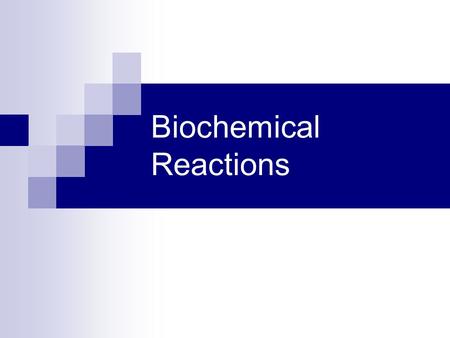
Biochemical Reactions. First… a Summary Macromolecules Monomers + functional groups Four types of macromolecules of interest to us: Carbohydrates.
About project
© 2024 SlidePlayer.com Inc. All rights reserved.
Advertisement
Free Radicals: Properties, Sources, Targets, and Their Implication in Various Diseases
- Review Article
- Published: 15 July 2014
- Volume 30 , pages 11–26, ( 2015 )
Cite this article
- Alugoju Phaniendra 1 ,
- Dinesh Babu Jestadi 1 &
- Latha Periyasamy 1
14k Accesses
1532 Citations
226 Altmetric
51 Mentions
Explore all metrics
Free radicals and other oxidants have gained importance in the field of biology due to their central role in various physiological conditions as well as their implication in a diverse range of diseases. The free radicals, both the reactive oxygen species (ROS) and reactive nitrogen species (RNS), are derived from both endogenous sources (mitochondria, peroxisomes, endoplasmic reticulum, phagocytic cells etc.) and exogenous sources (pollution, alcohol, tobacco smoke, heavy metals, transition metals, industrial solvents, pesticides, certain drugs like halothane, paracetamol, and radiation). Free radicals can adversely affect various important classes of biological molecules such as nucleic acids, lipids, and proteins, thereby altering the normal redox status leading to increased oxidative stress. The free radicals induced oxidative stress has been reported to be involved in several diseased conditions such as diabetes mellitus, neurodegenerative disorders (Parkinson’s disease-PD, Alzheimer’s disease-AD and Multiple sclerosis-MS), cardiovascular diseases (atherosclerosis and hypertension), respiratory diseases (asthma), cataract development, rheumatoid arthritis and in various cancers (colorectal, prostate, breast, lung, bladder cancers). This review deals with chemistry, formation and sources, and molecular targets of free radicals and it provides a brief overview on the pathogenesis of various diseased conditions caused by ROS/RNS.
This is a preview of subscription content, log in via an institution to check access.
Access this article
Price includes VAT (Russian Federation)
Instant access to the full article PDF.
Rent this article via DeepDyve
Institutional subscriptions
Similar content being viewed by others
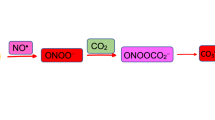
Several lines of antioxidant defense against oxidative stress: antioxidant enzymes, nanomaterials with multiple enzyme-mimicking activities, and low-molecular-weight antioxidants
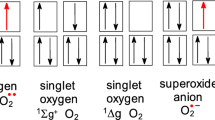
Reactive oxygen species, toxicity, oxidative stress, and antioxidants: chronic diseases and aging
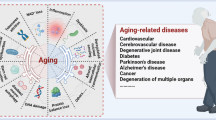
Molecular mechanisms of aging and anti-aging strategies
Gomberg M. An Incidence of Trivalent Carbon Trimethylphenyl. J Am Chem Soc. 1900;22:757–71.
Google Scholar
Gerschman R, Gilbert DL, Nye SW, Dwyer P, Fenn WO. Oxygen poisoning and x-irradiation-A mechanism in common. Science. 1954;119:623–6.
CAS PubMed Google Scholar
Commoner B, Townsend J, Pake GE. Free radicals in biological materials. Nature. 1954;174(4432):689–91.
McCord JM, Fridovich I. Superoxide dismutase an enzymatic function for erythrocuprein (chemocuprein). J Biol Chem. 1969;244(22):6049–55.
Loschen G, Flohe L, chance B. Respiratory chain linked H O production in pigeon heart mitochondria. FEBS Lett. 1971;18(2):261–4.
Nohl H, Hegner D. Do mitochondria produce oxygen radicals in vivo? Eur J Biochem. 1978;82:563–7.
Mittal CK, Murad F. Activation of guanylate cyclase by superoxide-dismutase and hydroxyl radical-Physiological regulator of guanosine 3′,5′-monophosphate formation. Proc Natl Acad Sci USA. 1977;74(10):4360–4.
CAS PubMed Central PubMed Google Scholar
Halliwell B, Gutteridge JMC. Free radicals in biology and medicine. 2nd ed. Oxford: Clarendon Press; 1989.
Mukherji SM, Singh SP. Reaction mechanism in organic chemistry. Madras: Macmillan IndiaPress; 1986.
Pham-Huy LA, Hua He, Pham-Huy C. Free Radicals, Antioxidants in Disease and Health. Int J Biomed Sci. 2008;4(2):89–96.
Valko M, Leibfritz D, Moncola J, Cronin MT, Mazura M, Telser J. Review Free radicals and antioxidants in normal physiological functions and human disease. Int J Biochem Cell Biol. 2007;39(1):44–84.
Nordberg J, Arner EJ. Reactive oxygen species, antioxidants, and the mammalian Thioredoxin system. Free Radical Biol Med. 2001;31(11):1287–312.
CAS Google Scholar
Yla-Herttuala S. Oxidized LDL and atherogenesis. Ann N Y Acad Sci. 1999;874:134–7.
Stadtman ER, Levine RL. Protein oxidation. Ann N Y Acad Sci. 2000;899:191–208.
Marnett LJ. Oxyradicals and DNA damage. Carcinogenesis. 2000;21(3):361–70.
Kohen R, Nyska A. Invited review Oxidation of Biological Systems: Oxidative Stress Phenomena, Antioxidants, Redox Reactions, and Methods for Their Quantification. Toxicol Pathol. 2002;30(6):620–50.
Halliwell B. Free Radicals and other reactive species in disease. Nature Encyclopedia of life sciences. 2001. p. 1–7.
Genestra M. Oxyl radicals, redox-sensitive signalling cascades and antioxidants. Review Cell Signal. 2007;19(9):1807–19.
Mugoni V, Santoro MM. Manipulating redox signaling to block tumor angiogenesis, research directions in tumor angiogenesis, Dr. Jianyuan Chai (Ed.), ISBN: 978-953-51-0963-1, InTech, 2013. doi: 10.5772/54593 .
Michelson AM, McCord JM, Fridovich I. Superoxide and Superoxide Dismutases. London: Academic Press; 1977. p. 320.
Kuppusamy P, Zweier JL. Characterization of free radical generation by xanthine oxidase. Evidence for hydroxyl radical generation. J Biol Chem. 1989;264(17):9880–4.
Kontos HA, Wei EP, Ellis EF, Jenkins LW, Povlishock JT, Rowe GT, et al. Appearance of superoxide anion radical in cerebral extracellular space during increased prostaglandin synthesis in cats. Circ Res. 1985;57(1):142–51.
McIntyre M, Bohr DF, Dominiczak AF. Endothelial function in hypertension. Hypertension. 1999;34:539–45.
Bielski BHJ, Cabelli DE. Superoxide and hydroxyl radical chemistry in aqueous solution. Active Oxygen in Chemistry. 1996;66–104.
Bielski BHJ, Cabelli BH, Arudi RL, Ross AB. Reactivity of RO2/O2. Radicals in aqueous solution. J Phys Chem Ref Data. 1985;14:1041–100.
Bedwell S, Dean RT, Jessup W. The action of defined oxygen centred free radicals on human low density lipoprotein. Biochem J. 1989;262(3):707–12.
Halliwell B. Oxidants and human disease: some new concepts. FASEB J. 1987;1(5):358–64.
Fenton HJH. Oxidation of tartaric acid in the presence of iron. J Chem Soc Trans. 1894;65:899–910.
Haber F, Weiss J. The catalytic decomposition of hydrogen peroxide by iron salts. Proc R Soc London (A). 1934;147:332–51.
De Grey ADNJ. HO 2 ˙: the forgotten radical. DNA Cell Biol. 2002;21:251–7.
PubMed Google Scholar
Cerruti PA. Pro-oxidant states and tumor activation. Science. 1985;227:375–81.
Halliwell B, Clement MV, Long LH. Hydrogen peroxide in the human body. FEBS Lett. 2000;486(1):10–3.
Mates JM, Perez-Gomez C, Nunez de Castro I. Antioxidant enzymes and human diseases. Clin Biochem. 1999;32(8):595–603.
Chae HZ, Kang SW, Rhee SG. Isoforms of mammalian peroxiredoxin that reduce peroxides in presence of thioredoxin. Methods Enzymol. 1999;300:219–26.
Hojo Y, Okado A, kawazoe S, Mizutani T. In vivo singlet-oxygen generation in blood of chromium(VI)-treated mice an electron spin resonance spin-trapping study. Biol Trace Elem Res. 2000;76(1):85–93.
Agnez-Lima LF, Melo JT, Silva AE, Oliveira AH, Timoteo AR, Lima-Bessa KM, et al. Review DNA damage by singlet oxygen and cellular protective mechanisms. Mutat Res. 2012;751(1):1–14.
Hampton MB, Kettle AJ, Winterbourn CC. Inside the neutrophil phagosome: oxidants, myeloperoxidase, and bacterial killing. Blood. 1998;92(9):3007–17.
Kanovasky JR. Singlet oxygen production by biological systems. Chem Biol Interact. 1989;70(1–2):1–28.
Chan HWS. Singlet oxygen analogs in biological systems: coupled oxygenation of 1,3-dienes by soybean lipoxidase. J Am Chem Soc. 1971;93(9):2357–8.
Hayaishi O, Nozaki M. Nature and mechanisms of oxygenases. Science. 1969;164:389–96.
Kanofsky JR. Singlet oxygen production by lactoperoxidase. J Biol Chem. 1983;258(10):5991–3.
Sies H, Menck CF. Singlet oxygen induced DNA damage. Mutat Res. 1992;275:367–75.
Lerner RA, Eschenmoser A. Ozone in biology. Proc Natl Acad Sci USA. 2003;100(6):3013–5.
Goldstein BD, Lodi C, Collinson C, Balchum OJ. Ozone and lipid peroxidation. Arch Environ Heath. 1969;18:631–5.
Freeman BA, Mudd JB. Reaction of ozone with sulfhydryls of human erythrocytes. Arch Biochem Biophys. 1981;208(1):212–20.
Mudd JB, Leavitt R, Ongun A, McManus TT. Reaction of ozone with amino acids and proteins. Atmos Environ. 1969;3:669–81.
Mustafa MG. Biochemical Basis of Ozone Toxicity. Free Radical Biol Med. 1990;9:245–65.
Fetner RH. Ozone induced chromosome breakage in human cell culture. Nature. 1962;194:793–4.
Winterbourn CC, Kettle AJ. Biomarkers of myeloperoxidase-derived hypochlorous acid. Free Radic Biol Med. 2000;29(5):403–9.
Albrich JM, McCarthy CA, Hurst JK. Biological reactivity of hypochlorous acid: implications for microbicidal mechanisms of leukocyte myeloperoxidase. Proc Natl Acad Sci USA. 1981;78(1):210–4.
Winterbourn CC. Comparative reactivities of various biological compounds with myeloperoxidase-hydrogen peroxide-chloride, and similarity of the oxidant to hypochlorite. Biochim Biophys Acta. 1985;840(2):204–10.
Prutz WA. Hypochlorous acid interactions with thiols, nucleotides, DNA, and other biological substrates. Arch Biochem Biophys. 1996;332(1):110–20.
Andrew PJ, Mayer B. Enzymatic function of nitric oxide synthases. Cardiovasc Res. 1999;43(3):521–31.
Chiueh CC. Neuroprotective properties of nitric oxide. Ann N Y Acad Sci. 1999;890:301–11.
Ignarro LJ, Buga GM, Wood KS, Byrns RE, Chandhuri G. Endothelium-derived relaxing factor produced and released from artery and vein is nitric oxide. Proc Natl Acad Sci USA. 1987;84:9265–9.
Wink DA, Mitchell JB. Chemical biology of nitric oxide: insights into regulatory, cytotoxic, and cytoprotective mechanisms of nitric oxide. Free Radic Biol Med. 1998;25(4–5):434–56.
Stamler JS. Redox signaling: nitrosylation and related target interactions of nitric oxide. Cell. 1994;78(6):931–6.
Koshland DE Jr. The molecule of the year. Science. 1992;258(5090):1861.
Beckman JS, Koppenol WH. Nitric oxide, superoxide, and peroxynitrite: the good, the bad, and ugly. Am J Physiol. 1996;271:C1424–37.
Douki H, Cadet J. Peroxynitrite mediated oxidation of purine bases of nucleosides and isolated DNA. Free Rad Res. 1996;24(5):369–80.
Ischiropoulos H, Al-Mehdi AB. Peroxynitrite mediated oxidative protein modifications. FEBS Lett. 1995;364(3):279–82.
Czapski G, Goldstein S. The role of the reactions of NO with superoxide and oxygen in biological systems: a kinetic approach. Free Radic Biol Med. 1995;19(6):785–94.
Finkel T, Holbrook NJ. Oxidants, oxidative stress and the biology of ageing. Nature. 2000;408:239–47.
Starkov AA. The role of mitochondria in reactive oxygen species metabolism and signaling. Ann NY Acad Sci. 2008;1147:37–52.
Giorgio M, Migliaccio E, Orsini F, Paolucci D, Moroni M, Contursi C, et al. Electron transfer between cytochrome c and p66Shc generates reactive oxygen species that trigger mitochondrial apoptosis. Cell. 2005;122(2):221–33.
De Duve C, Bauduhuin P. peroxisomes (microbodies and related particles). Physiol Rev. 1966;46:323–57.
Schrader M, Fahimi HD. Review Peroxisomes and oxidative stress. Biochim Biophys Acta. 2006;1763(12):1755–66.
Cheeseman KH, Slater TF. An introduction to free radical biochemistry. Br Med Bull. 1993;49(3):481–93.
Gross E, Sevier CS, Heldman N, Vitu E, Bentzur M, Kaiser CA, et al. Generating disulfides enzymatically: reaction products and electron acceptors of the endoplasmic reticulum thiol oxidase Ero1p. Proc Nat Acad Sci USA. 2006;103(2):299–304.
Droge W. Review Free radicals in the physiological control of cell function. Physiol Rev. 2002;82(1):47–95.
Halliwell B, Gutteridge JM. Free radicals in biology and medicine, vol. Third edition. Midsomer Norton: Oxford University Press; 1999.
Dizdaroglu M, Jaruga P, Birincioglu M, Rodriguez H. Free radical-induced damage to DNA: mechanisms and measurement. Free Radical Biol Med. 2002;32(11):1102–15.
Barja G. The flux of free radical attack through mitochondrial DNA is related to aging rate. Aging (Milano). 2000;12(5):342–55.
Hiraku Y, Kawanishi S, Ichinose T, Murata M. The role of iNOS-mediated DNA damage in infection- and asbestos-induced carcinogenesis. Ann NY Acad Sci. 2010;1203:15–22.
Yermilov V, Rubio J, Ohshima H. Formation of 8-nitroguanine in DNA treated with peroxynitrite in vitro and its rapid removal from DNA by depurination. FEBS Lett. 1995;376(3):207–10.
Loeb LA, Preston BD. Mutagenesis by apurinic/apyrimidinic sites. Annu Rev Genet. 1986;20:201–30.
Hofer T, Badouard C, Bajak E, Ravanat JL, Mattsson A, Cotgreave IA. Hydrogen peroxide causes greater oxidation in cellular RNA than in DNA. Biol Chem. 2005;386(4):333–7.
Abe T, Tohgi H, Isobe C, Murata T, Sato C. Remarkable increase in the concentration of 8-hydroxyguanosine in cerebrospinal fluid from patients with Alzheimer’s disease. J Neurosci Res. 2002;70(3):447–50.
Kikuchi A, Takeda A, Onodera H, Kimpara T, Hisanaga K, Sato N, et al. Systemic increase of oxidative nucleic acid damage in Parkinson’s disease and multiple system atrophy. Neurobiol Dis. 2002;9(2):244–8.
Martinet W, de Meyer GR, Herman AG, Kockx MM. Reactive oxygen species induce RNA damage in human atherosclerosis. Eur J Clin Invest. 2004;34(5):323–7.
Broedbaek K, Poulsen HE, Weimann A, Kom GD, Schwedhelm E, Nielsen P, et al. Urinary excretion of biomarkers of oxidatively damaged DNA and RNA in hereditary hemochromatosis. Free Radical Biol Med. 2009;47(8):1230–3.
Tateyama M, Takeda A, Onodera Y, Matsuzaki M, Hasegawa T, Nunomura A, et al. Oxidative stress and predominant Abeta 42 (43) deposition in myopathies with rimmed vacuoles. Acta Neuropathol. 2003;105(6):581–5.
Siems WG, Grune T, Esterbauer H. 4-Hydroxynonenal formation during ischemia and reperfusion of rat small-intestine. Life Sci. 1995;57(8):785–9.
Bast A. Oxidative stress and calcium homeostasis. In: Halliwell B, Aruoma OI, editors. DNA and free radicals. London: Ellis Horwood; 1993. p. 95–108.
Marnett LJ. Lipid peroxidation—DNA damage by malondialdehyde. Mutat Res. 1999;424(1–2):83–95.
Aruoma OI. Free radicals, oxidative stress, and antioxidants in human health and disease. J Am Oil chem Soc. 1998;75(2):199–212.
Dean RT, Fu S, Stocker R, Davies MJ. Biochemistry and pathology of radical-mediated protein oxidation. Biochem J. 1997;324:1–18.
Butterfield DA, Koppal T, Howard B, Subramaniam R, Hall N, Hensley K, et al. Structural and functional changes in proteins induced by free radical-mediated oxidative stress and protective action of the antioxidants N-tert-butyl-alpha-phenylnitrone and vitamin E. Ann N Y Acad Sci. 1998;854:448–62.
Brodie E, Reed DJ. Cellular recovery of glyceraldehyde-3-phosphate dehydrogenase activity and thiol status after exposure to hydroperoxide. Arch Biochem Biophys. 1990;276(1):210–2.
Pryor WA, Jin X, Squadrito GL. One- and two-electron oxidations of methionine by peroxynitrite. Proc Natl Acad Sci USA. 1994;91(23):11173–7.
Berlett BS, Stadtman E. Protein oxidation in aging, disease, and oxidative stress. J Bio Chem. 1997;272(33):20313–6.
Kikugawa K, Kato T, Okamoto Y. Damage of amino acids and proteins induced by nitrogen dioxide, a free radical toxin, in air. Free Rad Biol Med. 1994;16(3):373–82.
Uchida K, Kawakishi S. 2-oxohistidine as a novel biological marker for oxidatively modified proteins. FEBS Lett. 1993;332(3):208–10.
Garrison WM. Reaction mechanisms in radiolysis of peptides, polypeptides, and proteins. Chem Rev. 1987;8792:381–98.
Chevion M, Berenshtein E, Stadtman ER. Human studies related to protein oxidation: protein carbonyl content as a marker of damage. Free Radic Res. 2000;33:S99–108.
Smith CD, Carney JM, Starke-Reed PE, Oliver CN, Stadtman ER, Floyd RA, et al. Excess brain protein oxidation and enzyme dysfunction in normal aging and in Alzheimer disease. Proc Natl Acad Sci USA. 1991;88(23):10540–3.
Floor E, Wetzel MG. Increased protein oxidation in human substantia nigra pars compacta in comparison with basal ganglia and prefrontal cortex measured with an improved dinitrophenylhydrazine assay. J Neurochem. 1998;7091:268–75.
Murphy ME, Kehrer JP. Oxidation state of tissue thiol groups and content of protein carbonyl groups in chickens with inherited muscular dystrophy. Biochem J. 1989;260(2):359–64.
Garland D, Russell P, Zigler JS. Oxidative modification of lens proteins. Basic Life Sci. 1988;49:347–53.
Chapman ML, Rubin BR, Gracy RW. Increased carbonyl content of proteins in synovial fluid from patients with rhematoid arthritis. J Rheumatol. 1989;16(1):15–8.
Jones RH, Hothersall JS. The effect of diabetes and dietary ascorbate supplementation on the oxidative modification of rat lens beta L crystallin. Biochem Med Metab Biol. 1993;50(2):197–209.
Oliver CN, Ahn BW, Moerman EJ, Goldstein S, Stadtman ER. Age-related changes in oxidized proteins. J Biol Chem. 1987;262(12):5488–91.
Gavin JR, Alberti KGMM, Davidson MB, DeFronzo RA, Drash A, Gabbe SG, et al. Report of the expert committee on the diagnosis and classification of diabetes mellitus. Diabetes Care. 1997;20:1183–97.
Oberley LW. Free radicals and diabetes. Free Radic Biol Med. 1988;5(2):113–24.
Bashan N, Kovsan J, Kachko I, Ovadia H, Rudich A. Positive and negative regulation of insulin signaling by reactive oxygen and nitrogen species. Physiol Rev. 2009;89(1):27–71.
Ahmed RG. The physiological and biochemical Effects of diabetes on the balance between oxidative stress and Antioxidant defense system. Med J Islam World Acad Sci. 2005;15(1):31–42.
Kwong LK, Sohal RS. Substrate and site specificity of hydrogen peroxide generation in mouse mitochondria. Arch Biochem Biophys. 1998;350(1):118–26.
Bajaj S, Khan A. Antioxidants and diabetes. Indian J Endocrinol Metab. 2012;2:S267–71.
Pollack M, Leeuwenburgh C. Molecular mechanisms of oxidative stress in aging: free radicals, aging, antioxidants and disease. Elsevier Science B.V. Handbook of Oxidants and Antioxidants in Exercise. 1999;881–923.
Rivas-Arancibia S, Guevara-Guzmán R, López-Vidal Y, Rodríguez-Martínez E, Zanardo-Gomes M, Angoa-Pérez M, et al. Oxidative stress caused by ozone exposure induces loss of brain repair in the hippocampus of adult rats. Toxicol Sci. 2010;113(1):187–97.
Santiago-López JA, Bautista-Martínez CI, Reyes-Hernandez M, Aguilar-Martínez S, Rivas- Arancibia S. Oxidative stress, progressive damage in the substantia nigra and plasma dopamine oxidation, in rats chronically exposed to ozone. Toxicol Lett. 2010;197(3):193–200.
Pan XD, Zhu YG, Lin N, Zhang J, Ye QY, Huang HP, Chen XC. Microglial phagocytosis induced by fibrillar β-amyloid is attenuated by oligomeric β-amyloid: implications for Alzheimer’s disease. Mol Neurodegener. 2011;6(45):1–17.
Sevcsik E, Trexler AJ, Dunn JM, Rhoades E. Allostery in a disordered protein: oxidative modifications to α-synuclein act distally to regulate membrane binding. J Am Chem Soc. 2011;133(18):7152–8.
Zhao W, Varghese M, Yemul S, Pan Y, Cheng A, Marano P, et al. Peroxisome proliferator activator receptor gamma coactivator-1alpha (PGC-1α) improves motor performance and survival in a mouse model of amyotrophic lateral sclerosis. Mol Neurodegener. 2011;6(1):1–8.
Witherick J, Wilkins A, Scolding N, Kemp K. Mechanisms of oxidative damage in multiple sclerosis and a cell therapy approach to treatment. Autoimmune Dis. 2010;1–11.
Fisher LJ, Gage FH. Radical directions in Parkinson’s disease. Nat Med. 1995;1(3):201–3.
Olivieri S, Conti A, Iannaccone S, Cannistraci CV, Campanella A, Barbariga M, et al. Ceruloplasmin oxidation, a feature of Parkinson’s disease CSF, inhibits ferroxidase activity and promotes cellular iron retention. J Neurosci. 2011;31:18568–77.
Butterfield DA, Perluigi M, Sultana R. Oxidative stress in Alzheimer’s disease brain: new insights from redox proteomics. Eur J Pharmacol. 2006;545(1):39–50.
Chang Y, Kong Q, Shan X, Tian G, Ilieva H, Cleveland DW, et al. Messenger RNA oxidation occurs early in disease pathogenesis and promotes motor neuron degeneration in ALS. PLoS ONE. 2008;3(8):1–19.
Uttara B, Singh AV, Zamboni P, Mahajan RT. Oxidative stress and neurodegenerative diseases. A Review of upstream and downstream antioxidant therapeutic options. Curr Neuropharmacol. 2009;7(1):65–74.
Gonsette RE. Neurodegeneration in multiple sclerosis: the role of oxidative stress and excitotoxicity. J Neurol Sci. 2008;274(1–2):48–53.
Mitosek-Szewczyk K, Gordon-Krajcer W, Walendzik P, Stelmasiak Z. Free radical peroxidation products in cerebrospinal fluid and serum of patients with multiple sclerosis after glucocorticoid therapy. Folia Neuropathol. 2010;48(2):116–22.
Goldstein BD, Witz G. Free radicals and carcinogenesis. Free Radic Res Commum. 1990;11(1–3):3–10.
Dreher D, Junod AF. Role of oxygen free radicals in cancer development. Eur J Cancer. 1996;32A(1):30–8.
Acuna UM, Wittwer J, Ayers S, Pearce CJ, Oberlies NH, De Blanco EJ. Effects of (5Z)-7-Oxozeaenol on the Oxidative pathway of cancer cells. Anticancer Res. 2012;32(7):2665–71.
Cairns RA, Harris I, McCracken S, Mak TW. Cancer cell metabolism. Cold Spring Harb Symp Quant Biol. 2011;76:299–311.
Valko M, Leibfritz D, Moncol J, Cronin MT, Mazur M, Telser J. Free radicals and antioxidants in normal hitological functions and human disease. Int J Biochem Cell Biol. 2007;39(1):44–84.
Valko M, Izakovic M, Mazur M, Rhodes CJ, Telser J. Role of oxygen radicals in DNA damage and cancer incidence. Mol Cell Biochem. 2004;266(1–2):37–56.
Jemal A, Bray F, Center MM, Ferlay J, Ward E, Forman D. Global cancer statistics. CA Cancer J Clin. 2011;61(2):69–90.
Blau S, Rubinstein A, Bass P, Singaram C, Kohen R. Differences in the reducing power along the rat GI tract: lower antioxidant capacity of the colon. Mol Cell Biochem. 1999;194(1–2):185–91.
Foksinski M, Rozalski R, Guz J, Ruszkowska B, Sztukowska P, Piwowarski M, et al. Urinary excretion of DNA repair products correlates with metabolic rates as well as with maximum life spans of different mammalian species. Free Radic Biol Med. 2004;37(9):1449–54.
Haklar G, Sayin-Ozveri E, Yuksel M, Aktan AO, Yalcin AS. Different kinds of reactive oxygen and nitrogen species were detected in colon and breast tumors. Cancer Lett. 2001;165(2):219–24.
Guz J, Foksinski M, Siomek A, Gackowski D, Rozalski R, Dziaman T, et al. The relationship between 8-oxo-7,8-dihydro-2-deoxyguanosine level and extent of cytosine methylation in leukocytes DNA of healthy subjects and in patients with colon adenomas and carcinomas. Mutat Res. 2008;640(1–2):170–3.
Rainis T, Maor I, Lanir A, Shnizer S, Lavy A. Enhanced oxidative stress and leucocyte activation in neoplastic tissues of the colon. Dig Dis Sci. 2007;52(2):526–30.
Suzuki K, Ito Y, Wakai K, Kawado M, Hashimoto S, Toyoshima H, et al. Serum oxidized low-density lipoprotein levels and risk of colorectal cancer: a case-control study nested in the Japan Collaborative Cohort Study. Cancer Epidemiol Biomark Prev. 2004;13(11):1781–7.
Murrell TG. Epidemiological and biochemical support for a theory on the cause and prevention of breast cancer. Med Hypotheses. 1991;36(4):389–96.
Brown NS, Jones A, Fujiyama C, Harris AL, Bicknell R. Thymidine phosphorylase induces carcinoma cell oxidative stress and promotes secretion of angiogenic factors. Cancer Res. 2000;60(22):6298–302.
Sipe HJ Jr, Jordan SJ, Hanna PM, Mason RP. The metabolism of 17 beta-estradiol by lactoperoxidase: a possible source of oxidative stress in breast cancer. Carcinogenesis. 1994;15(11):2637–43.
Arnold RS, He J, Remo A, Ritsick D, Yin-Goen Q, Lambeth JD, et al. Nox1 expression determines cellular reactive oxygen and modulates c-fos-induced growth factor, interleukin-8, and Cav-1. Am J Pathol. 2007;171(6):2021–32.
Lim SD, Sun C, Lambeth JD, Marshall F, Amin M, Chung L, et al. Increased Nox1 and hydrogen peroxide in prostate cancer. Prostate. 2005;62(2):200–7.
Brar SS, Corbin Z, Kennedy TP, Hemendinger R, Thornton L, Bommarius B, et al. NOX5 NAD(P)H oxidase regulates growth and apoptosis in DU 145 prostate cancer cells. Am J Physiol Cell Physiol. 2003;285(2):C353–69.
Kumar B, Koul S, Khandrika L, Measchan RB, Koul HK. Oxidative stress is inherent in prostate cancer cells and is required for aggressive phenotype. Cancer Res. 2008;68:1777–85.
Veeramani S, Yuan TC, Lin FF, Lin MF. Mitochondrial redox signaling by p66Shc in involved in regulating androgenic growth stimulation of human prostate cancer cell. Oncogene. 2008;27(37):5057–68.
WHO World cancer Report 2008. In: Boyle P, Levin B, editors. Lung cancer, 12. Chapter 5.10.
Azad N, Rojanasakul Y, Vallyathan V. Inflammation and lung cancer: roles of reactive oxygen/nitrogen species. J Toxicol Environ Health. 2008;11(1):1–15.
Hoagland LF 4th, Campa MJ, Gottlin EB, Herndon 2nd JE, Patz Jr EF. Haptoglobin and posttranslational glycan-modified derivatives as serum biomarkers for the diagnosis of nonsmall cell lung cancer. Cancer. 2007;110(10):2260–2268.
Pastora MD, Nogala A, Molina-Pineloa S, Meléndeza R, Salinasa A, González De la Penaa M, et al. Identification of proteomic signatures associated with lung cancer and COPD. J Proteomics. 2013;89:227–37.
Jemal A, Siegel R, Ward E, Murray T, Xu J, Thun MJ. Cancer statistics. CA Cancer J Clin. 2007;57:43–6.
Wynder EL, Goldsmith R. The epidemiology of bladder cancer: a second look. Cancer. 1977;40:1246–68.
Opanuraks J, Boonla C, Saelim C, Kittikowit W, Sumpatanukul P, Honglertsakula C, et al. Elevated urinary total sialic acid and increased oxidative stress in patients with bladder cancer. Asian Biomedicine. 2010;4(5):703–10.
Opanuraks J, Boonla C, Saelim C, Kittikowit W, Sumpatanukul P, Honglertsakula C, Tosukhowong P. Elevated urinary total sialic acid and increased oxidative stress in patients with bladder cancer. Asian Biomedicine. 2010;4(5):703–10.
Soini Y, Haapasaari KM, Vaarala MH, Turpeenniemi-Hujanen T, Karja V, Karihtala P. 8-hydroxydeguanosine and nitrotyrosine are prognostic factors in urinary bladder carcinoma. Int J Clin Exp Pathol. 2011;4(3):267–75.
PubMed Central PubMed Google Scholar
Eijan AM, Piccardo I, Riveros MD, Sandes EO, Porcella H, Jasnis MA, et al. Nitric oxide in patients with transitional bladder cancer. J Surg Oncol. 2002;81:203–8.
Gecit I, Aslan M, Gunes M, Pirincci N, Esen R, Demir H, et al. Serum prolidase activity, oxidative stress, and nitric oxide levels in patients with bladder cancer. J Cancer Res Clin Oncol. 2012;138(5):739–43.
Ellidag HY, Eren E, Aydın O, Akgol E, Yalcınkaya S, Sezer C, et al. Ischemia Modified Albumin Levels and Oxidative Stress in Patients with Bladder Cancer. Asian Pacific J Cancer Prev. 2013;14(5):2759–63.
Yılmaz IA, Akçay T, Çakatay U, Telci A, Ataus S, Yalcin V. Relation between bladder cancer and protein oxidation. ˙. Int Urol Nephrol. 2003;35(3):345–50.
DeMarchi E, B Faldassari, Bononi A, Wieckowski MR, Pinton P. Oxidative Stress in Cardiovascular Diseases and Obesity: Role of p66Shc and Protein Kinase C Oxidative Medicine and Cellular Longevity. 2013;1-11. Review.
Yin Y, Pastrana JL, Li X, Huang X, Mallilankaraman K, Choi ET, et al. Inflammasomes: sensors of metabolic stresses for vascular inflammation. Front Biosci. 2013;18:638–49.
Ross R. Atherosclerosis—an inflammatory disease. N Engl J Med. 1999;340(2):115–26.
Papaharalambus CA, Griendling KK. Basic mechanisms of oxidative stress and reactive oxygen species in cardiovascular injury. Trends Cardiovasc Med. 2007;17(2):48–54.
Rajagopalan S, Meng XP, Ramasamy S, Harrison DG, Galis ZS. Reactive oxygen species produced by macrophage-derived foam cells regulate the activity of vascular matrix metalloproteinases in vitro. Implications for atherosclerotic plaque stability. J Clin Invest. 1996;98(11):2572–9.
Barnoya J, Glantz SA. Cardiovascular effects of secondhand smoke: nearly as large as smoking. Circulation. 2005;111(20):2684–98.
Yang Z, Ming XF. Recent advances in understanding endothelial dysfunction in atherosclerosis. Clin Med Res. 2006;4(1):53–65.
Botto N, Rizza A, Colombo MG, Mazzone AM, Manfredi S, Masetti S, et al. Evidence for DNA damage in patients with coronary artery disease. Mutat Res. 2001;493(1–2):23–30.
Ding Z, Liu S, Wang X, Khaidakov M, Dai Y, Mehta JL. Oxidant stress in mitochondrial DNA damage, autophagy and inflammation in atherosclerosis. Sci Rep. 2013;3:1–6.
Mercer JR, Cheng KK, Figg N, Gorenne I, Mahmoudi M, Griffin J, et al. DNA damage links mitochondrial dysfunction to atherosclerosis and the metabolic syndrome novelty and significance. Circ Res. 2010;107:1021–31.
A global brief on hypertension. World health day 2013. WHO.
Zalba G, Jose GS, Moreno MU, Fortuno MA, Fortuno A, Beaumont FJ, et al. Oxidative stress in arterial hypertension role of NAD(P)H oxidase. Hypertension. 2001;38(6):1395–9.
Dzau VJ. Tissue angiotensin and pathobiology of vascular disease: a unifying hypothesis. Hypertension. 2001;37:1047–52.
Touyz RM. Reactive oxygen species, vascular oxidative stress, and redox signaling in hypertension: what is the clinical significance? Hypertension. 2004;44(3):248–52.
Touyz RM. Reactive oxygen species and angiotensin II signaling in vascular cells: implications in cardiovascular disease. Braz J Med Biol Res. 2004;37(8):1263–73.
Hashim Z, Zarina S. Osmotic stress induced oxidative damage: Possible mechanism of cataract formation in diabetes. J Diabetes Complicat. 2012;26(4):275–9.
Thylefors B, Negrel AD, Pararajasegaram R, Dadzie KY. Global data on blindness. Bull World Health Organ. 1995;73(1):115–21.
Nagai N, Fukuhata T, Ito Y. Effect of magnesium deficiency on intracellular ATP Levels in human lens epithelial cells. Biol Pharm Bull. 2007;30(1):6–10.
Beebe DC, Holekamp NM, Shui YB. Oxidative damage and the prevention of age-related cataracts. Ophthalmic Res. 2010;44(3):155–65.
Berthoud VM, Beyer EC. Forum review article oxidative stress, lens gap junctions, and cataracts. Antioxid Redox Signal. 2009;11(2):339–53.
Bhuyan KC, Bhuyan DK, Podos SM. Lipid peroxidation in cataract of the human. Life Sci. 1986;38(16):1463–71.
Gupta SK, Trivedi D, Srivastava S, Joshi S, Halder N, Verma SD. Lycopene attenuates oxidative stress induced experimental cataract development: an in vitro and in vivo study. Nutrition. 2003;19(9):794–9.
Boettner EH, Walter JR. Transmission of the ocular media. GPO Invest Ophthalmol Vis Sci. 1962;1:776–83.
Krishna CM, Uppuluri S, Riesz P, Zigler JS Jr, Balasubramian D. A study of the photodynamic efficiencies of some eye lens constituents. Photochem Photobiol. 1991;54(1):51–8.
Dilsiz N, Olcucu A, Atas M. Determination of calcium, sodium, potassium and magnesium concentrations in human senile cataractous lenses. Cell Biochem Funct. 2000;18(4):259–62.
David LL, Azuma M, Shearer TR. Cataract and the acceleration of calpain-induced beta-crystallin insolubilization occurring during normal maturation of rat lens. Invest Ophthalmol Vis Sci. 1994;35(3):785–93.
Spector A, Garner WH. Hydrogen peroxide and human cataract. Exp Eye Res. 1981;33(6):673–81.
Hapeta B, Koczy B, Fitowska A, Dobrakowski M, Kasperczyk A, Ostałowska A, et al. Metabolism and protein transformations in synovial membrane of a knee joint in the course of rheumatoid arthritis and degenerative arthritis. Pol Orthop Traumatol. 2012;77:53–8.
Stamp LK, Khalilova I, Tarr JM, Senthilmohan R, Turner R, Haigh RC, et al. Myeloperoxidase and oxidative stress in rheumatoid arthritis. Rheumatology (Oxford). 2012;51(10):1796–803.
Desai PB, Manjunath S, Kadi S, Chetana K, Vanishree J. Oxidative stress and enzymatic antioxidant status in rheumatoid arthritis: a case control study. Eur Rev Med Pharmacol Sci. 2010;14(11):959–67.
Grover HS, Gaba N, Gupta A, Marya CM. Rheumatoid arthritis: a review and dental care considerations. Nepal Med Coll J. 2011;13(2):74–6.
De Pablo P, Dietrich T, McAlindon TE. Association of periodontal disease and tooth loss with rheumatoid arthritis in the US population. J Rheumatol. 2008;35(1):70–6.
Vasanthi P, Nalini G, Rajasekhar G. Status of oxidative stress in rheumatoid arthritis. Int J Rheum Dis. 2009;12(1):29–33.
Hitchon CA, El-Gabalawy HS. Review Oxidation in rheumatoid arthritis. Arthritis Res Ther. 2004;6(6):265–78.
Grootveld M, Henderson EB, Farell A, Blake DR, Parkes HG, Haycock P. Oxidative damage to hyaluronate and glucose in synovial fluid during exercise of the inflamed rheumatoid joint. Detection of abnormal lowmolecular-mass metabolites by proton-n.m.r. spectroscopy. Biochem J. 1991;273:459–67.
Rowley D, Gutteridge JM, Blake D, Farr M, Halliwell B. Lipid peroxidation in rheumatoid arthritis: thiobarbituric acid-reactive material and catalytic iron salts in synovial fluid from rheumatoid patients. Clin Sci (London). 1984;66(6):691–5.
Dai L, Lamb DJ, Leake DS. Evidence for oxidized low density lipoprotein in synovial fluid from rheumatoid arthritis patients. Free Radic Res. 2000;32(6):479–86.
Dalle-Donne I, Rossi R, Giustarini D, Milzani A, Colombo R. Protein carbonyl groups as biomarkers of oxidative stress. Clin Chim Acta. 2003;329(1–2):23–38.
Dai L, Lamb DJ, Leake DS, Kus ML, Jones HW, Morris CJ, et al. Evidence for oxidised low density lipoprotein in synovial fluid from rheumatoid arthritis patients. Free Radic Res. 2000;32(6):479–86.
Costenbader KH, Karlson EW. Cigarette smoking and autoimmune disease: what can we learn from epidemiology? Lupus. 2006;15(11):737–45.
Kottova M, Pourova J, Voprsalova M. Oxidative stress and its role in respiratory diseases. Ceska Slov Farm. 2007;56(5):215–9.
Masoli M, Fabian D, Holt S, Beasley R. The global burden of asthma: Executive summary of the GINA Dissemination Committee report. Allergy. 2004;59(5):469–78.
Chung KF. Role of inflammation in the hyper reactivity of the airways in asthma. Thorax. 1986;41:657–62.
Barnes PJ. Reactive oxygen species and airway inflammation. Free Radic Biol Med. 1990;9(3):235–43.
Xiao M, Zhu T, Wang T, Wen FQ. Hydrogen-rich saline reduces airway remodeling via inactivation of NF-κB in a murine model of asthma. Eur Rev Med Pharmacol Sci. 2013;17(8):1033–43.
Tohyama Y, Kanazawa H, Fujiwara F, Hirata K, Fujimoto S, Yoshikawa J. Role of nitric oxide on airway microvascular permeability in patients with asthma. Osaka City Med J. 2005;51(1):1–9.
Rahman I, Biswas SK, Kode A. Oxidant and antioxidant balance in the airways and airway diseases. Eur J Pharmacol. 2006;533(1–3):222–39.
Terada LS. Specificity in reactive oxidant signaling: Think globally, act locally. J Cell Biol. 2006;174(5):615–23.
Fujisawa T. Role of oxygen radicals on bronchial asthma. Curr Drug Targets Inflamm Allergy. 2005;4(4):505–9.
Ozaras R, Tahan V, Turkmen S, Talay F, Besirli K, Aydin S, et al. Changes in malondialdehyde levels in bronchoalveolar fluid and serum by the treatment of asthma with inhaled steroid and beta2-agonist. Respirology. 2000;5(3):289–92.
Ahmad A, Shameem M, Husain Q. Relation of oxidant-antioxidant imbalance with disease progression in patients with asthma. Ann Thorac Med. 2012;7(4):226–32.
Pobed’onna HP. Antioxidant protection, metabolites of nitrogen oxide on the forming of oxidative stress in patients with bronchial asthma. Lik Sprava. 2005;(5–6):36–40.
Download references
Author information
Authors and affiliations.
Department of Biochemistry and Molecular Biology, Pondicherry University, Pondicherry, 605 014, India
Alugoju Phaniendra, Dinesh Babu Jestadi & Latha Periyasamy
You can also search for this author in PubMed Google Scholar
Corresponding author
Correspondence to Latha Periyasamy .
Rights and permissions
Reprints and permissions
About this article
Phaniendra, A., Jestadi, D.B. & Periyasamy, L. Free Radicals: Properties, Sources, Targets, and Their Implication in Various Diseases. Ind J Clin Biochem 30 , 11–26 (2015). https://doi.org/10.1007/s12291-014-0446-0
Download citation
Received : 02 October 2013
Accepted : 14 May 2014
Published : 15 July 2014
Issue Date : January 2015
DOI : https://doi.org/10.1007/s12291-014-0446-0
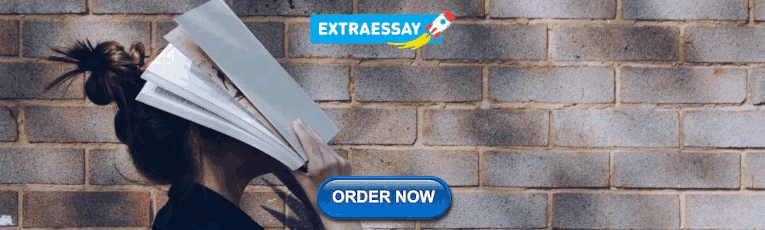
Share this article
Anyone you share the following link with will be able to read this content:
Sorry, a shareable link is not currently available for this article.
Provided by the Springer Nature SharedIt content-sharing initiative
- Free radicals
- Reactive oxygen species (ROS)
- Reactive nitrogen species (RNS)
- Oxidative stress
- Find a journal
- Publish with us
- Track your research
- Type 2 Diabetes
- Heart Disease
- Digestive Health
- Multiple Sclerosis
- Diet & Nutrition
- Supplements
- Health Insurance
- Public Health
- Patient Rights
- Caregivers & Loved Ones
- End of Life Concerns
- Health News
- Thyroid Test Analyzer
- Doctor Discussion Guides
- Hemoglobin A1c Test Analyzer
- Lipid Test Analyzer
- Complete Blood Count (CBC) Analyzer
- What to Buy
- Editorial Process
- Meet Our Medical Expert Board
Free Radicals: Definition, Cause, and Role in Cancer
What Exactly Are Free Radicals and Why Are They Important?
What Are Free Radicals?
- Causes and Sources
- Free Radicals and Cancer
- Antioxidants
- Reducing Free Radicals
Free radicals are highly reactive and unstable molecules that are made by the body naturally as a byproduct of normal metabolism . Free radicals can also be made by the body after exposure to toxins in the environment such as tobacco smoke and ultraviolet (UV) light .
Free radicals have a lifespan of only a fraction of a second, but during that time can damage DNA , sometimes causing mutations that can increase your risk of getting health conditions like heart disease and cancer .
Antioxidants in the foods we eat can neutralize the unstable molecules and reduce the chances of them causing damage.
This article will explain what free radicals are. You will also learn the kind of harm free radicals cause, which supplements or vitamins may protect against free radicals, and what foods to include in your diet to reduce oxidative stress .
Free radicals are atoms that have one electron that is not paired. Sometimes they have more than one unpaired electron.
Electrons need to be paired to be stable. Free radicals constantly look to bind with another atom or molecule to become stable.
As they're looking around, free radicals can damage human cells. The consequences of that damage include effects like speeding up the aging process and even playing a role in the development of cancer and other diseases.
Types of Free Radicals
There are many types of free radicals. The oxygen free radicals ( reactive oxygen species ) are the most important in humans.
Examples of oxygen free radicals include:
- Singlet oxygen (when oxygen is "split" into single atoms with unpaired electrons)
- Hydrogen peroxide
- Superoxides
- Hydroxyl anions
Sources of Free Radicals
Free radicals can come from normal metabolic processes in the body or from exposure to cancer-causing substances ( carcinogens ) or other harmful substances in the environment.
Free Radicals From Normal Body Processes
The body makes free radicals while breaking down nutrients to give us the energy we need to function.
The production of free radicals during these normal processes in the body is one reason why our risk for many diseases goes up as we age even if we haven't been exposed to toxic substances.
Free Radicals From Carcinogens
Free radicals can also be made when we're exposed to carcinogens such as:
- Tobacco smoke
- Ultraviolet radiation
- Environmental and occupational substances and chemicals (e.g., asbestos and vinyl chloride )
- Some viruses
- Medical radiation
- Air pollution
Effect of Free Radicals on the Body: Oxidative Stress
Once free radicals are made, they're free to do damage to the body—whether they came from exposure to a carcinogen or the normal processes of the body.
The availability of free radicals creates something called oxidative stress in the body. It's called "stress" because the chemical reactions that let free radicals get an electron occur in the presence of oxygen.
There are several parts to this process. When one free radical "steals" an electron from a molecule, that molecule becomes a free radical because it's missing an electron. That cycle continues and makes more free radicals.
Free radicals can damage the body's DNA . Our DNA contains our genes, proteins, lipids, cell membranes, and other important substances. Damaged DNA can lead to disease.
The Free Radical Theory of Aging
There are several theories about why our bodies age and free radicals are a key player in many of them. Free radicals are not considered fully responsible for aging-related changes, though; it's more likely that normal aging is related to many processes in the body.
How Free Radicals Can Cause Cancer
Damage to genes in the DNA can cause them to make ineffective proteins. Some of those proteins are an important part of making sure the DNA is working right.
A key area where damage can cause problems is in tumor suppressor genes . These genes direct the proteins that repair damaged DNA or cause cells that are damaged so badly they can't be fixed to be removed through "programmed cell death" ( apoptosis ).
Usually, it's a series of mutations in tumor suppressor genes and other genes that lead a cancer cell to form.
Antioxidants and Free Radicals
Many of the plant chemicals ( phytochemicals ) in our foods are antioxidants. These nutrients stop the formation of free radicals and may reduce the damage they would cause in the body.
The power of antioxidants to fight free radicals is one reason why a diet rich in vegetables and fruits has been linked with a lower risk of many diseases.
Examples of antioxidants that may help combat free radicals and oxidative stress include:
- Beta-carotene
- Anthocyanidins
- Epigallacatechin-3-gallate (EGCG)
Many foods and drinks are good sources of different antioxidants , like berries and green tea .
Do Antioxidant Supplements Fight Free Radicals?
Studies have shown that a diet rich in antioxidants is associated with a lower risk of many chronic diseases, including cancer. However, using antioxidant supplements does not appear to have the same effect.
For example, research had shown that people who had a higher intake of foods rich in beta-carotene and vitamin E had a lower risk of developing lung cancer.
To find out why this might be the case, researchers did a study where one group of people took a daily supplement of beta-carotene, and the other did not to see if their risk of lung cancer would be affected.
The results were a bit surprising: the men in the study who smoked and took beta-carotene had a higher risk of developing lung cancer, not a lower risk.
Can People With Cancer Take Antioxidant Supplements?
If you're having treatment for cancer, you might be worried about free radicals and wonder if you should up your antioxidant intake to fight off more damage.
Always talk to your oncologist about any supplement you're thinking about trying. They will guide you on what is safe to take (or not) while you are having treatment.
However, taking antioxidant supplements may worsen the prognosis for some cancers, and certain vitamin supplements may make cancer treatments less effective.
In one study, postmenopausal women with breast cancer who used antioxidant supplements during chemotherapy and radiation had a poorer prognosis. Two other, separate studies found that antioxidant supplements (such as vitamin E) may promote the growth and spread of lung cancer.
While antioxidant supplements are often not recommended, your oncologist will likely encourage you to eat a balanced, nutritious diet that naturally contains antioxidants.
How to Reduce Free Radicals in Your Body
You can't completely avoid free radicals because they're part of a natural process in your body that you don't control. You also can't always avoid being exposed to toxins—for example, you might run into them at your job.
That said, you can do your best to avoid exposures and consider safety when you can't avoid them.
You can also arm your body with antioxidants to fight free radicals. While your body does make antioxidants, it doesn't make enough. For example, eating a "rainbow of foods" that will supply you with them is key.
That said, even when people "do everything right"—like avoiding carcinogens and eating an antioxidant-rich diet—they can still get cancer or other diseases.
Free radicals are unstable molecules in the body that can damage DNA in cells. In turn, this can increase your risk for disease, including cancer.
The body naturally makes some free radicals as a byproduct of the processes it normally does, but you can also get more free radicals by exposure to certain toxic substances.
Antioxidants, like those found naturally in fruits and vegetables, are a key way to "fight" free radicals and the oxidative stress they cause in your body. However, antioxidant supplements are less likely to help and may even do more harm than good.
Phaniendra A, Jestadi DB, Periyasamy L. Free radicals: properties, sources, targets, and their implication in various diseases . Indian J Clin Biochem . 2015;30(1):11-26. doi:10.1007/s12291-014-0446-0
Michigan State University. What you need to know about antioxidants .
Lobo V, Patil A, Phatak A, Chandra N. Free radicals, antioxidants and functional foods: Impact on human health . Pharmacogn Rev . 2010;4(8):118-126. doi:10.4103/0973-7847.70902
Jiang D, Rusling JF. Oxidation Chemistry of DNA and p53 Tumor Suppressor Gene . ChemistryOpen . 2019;8(3):252-265. Published 2019 Feb 22. doi:10.1002/open.201800292
Neha K, Haider MR, Pathak A, Yar MS. Medicinal prospects of antioxidants: A review . European Journal of Medicinal Chemistry. 2019. 178:687-704. doi:10.1016/j.ejmech.2019.06.010
Choi Y, Larson N, Steffen LM, et al. Plant‐Centered Diet and Risk of Incident Cardiovascular Disease During Young to Middle Adulthood . Journal of the American Heart Association . 2021;10(16). doi:10.1161/jaha.120.020718
Alsharairi N. The Effects of Dietary Supplements on Asthma and Lung Cancer Risk in Smokers and Non-Smokers: A Review of the Literature . Nutrients. 2019. 11(4): 725. doi:10.3390/nu11040725
Jung A, Cai X, Thoene K, et al. Antioxidant Supplementation and Breast Cancer Prognosis in Postmenopausal Women Undergoing Chemotherapy and Radiation Therapy . The American Journal of Clinical Nutrition. 2019. 109(1):69-78. doi:10.1093/ajcn/nqy223
Lignitto L, LeBoeuf SE, Hamer H, et al. Nrf2 Activation Promotes Lung Cancer Metastasis by Inhibiting the Degradation of Bach1 . Cell. 2019. doi: 10.1016/j.cell.2019.06.003
By Lynne Eldridge, MD Lynne Eldrige, MD, is a lung cancer physician, patient advocate, and award-winning author of "Avoiding Cancer One Day at a Time."
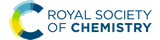
Free radicals, natural antioxidants, and their reaction mechanisms
Satish Balasaheb Nimse * a and Dilipkumar Pal b a Institute for Applied Chemistry, Department of Chemistry, Hallym University, Chuncheon, 200-702, Korea. E-mail: [email protected] ; Fax: +82-33-256-3421; Tel: +82-33-248-2076 b Institute of Pharmaceutical Sciences, Guru Ghasidas Vishwavidyalaya (A Central University), Koni, Bilaspur, Chhattisgarh-495009, India
First published on 12th March 2015
The normal biochemical reactions in our body, increased exposure to the environment, and higher levels of dietary xenobiotic's result in the generation of reactive oxygen species (ROS) and reactive nitrogen species (RNS). The ROS and RNS create oxidative stress in different pathophysiological conditions. The reported chemical evidence suggests that dietary antioxidants help in disease prevention. The antioxidant compounds react in one-electron reactions with free radicals in vivo / in vitro and prevent oxidative damage. Therefore, it is very important to understand the reaction mechanism of antioxidants with the free radicals. This review elaborates the mechanism of action of the natural antioxidant compounds and assays for the evaluation of their antioxidant activities. The reaction mechanisms of the antioxidant assays are briefly discussed (165 references). Practical applications: understanding the reaction mechanisms can help in evaluating the antioxidant activity of various antioxidant compounds as well as in the development of novel antioxidants.
1. Introduction and background
Normal biochemical reactions, increased exposure to the environment, and higher levels of dietary xenobiotics result in the generation of reactive oxygen species (ROS) and reactive nitrogen species (RNS). 2 ROS and RNS are responsible for the oxidative stress in different pathophysiological conditions. 3 Cellular constituents of our body are altered in oxidative stress conditions, resulting in various disease states. The oxidative stress can be effectively neutralized by enhancing cellular defenses in the form of antioxidants. 4,5 Certain compounds act as in vivo antioxidants by raising the levels of endogenous antioxidant defenses. Expression of genes encoding the enzymes such as superoxide dismutase (SOD), catalase (CAT), glutathione peroxidase (GSHPx) increases the level of endogenous antioxidants. 6
Antioxidants can be categorized in multiple ways. Based on their activity, they can be categorized as enzymatic and non-enzymatic antioxidants. Enzymatic antioxidants work by breaking down and removing free radicals. The antioxidant enzymes convert dangerous oxidative products to hydrogen peroxide (H 2 O 2 ) and then to water, in a multi-step process in presence of cofactors such as copper, zinc, manganese, and iron. Non-enzymatic antioxidants work by interrupting free radical chain reactions. Few examples of the non-enzymatic antioxidants are vitamin C, vitamin E, plant polyphenol, carotenoids, and glutathione. 7
The other way of categorizing the antioxidants is based on their solubility in the water or lipids. The antioxidants can be categorized as water-soluble and lipid-soluble antioxidants. The water-soluble antioxidants ( e.g. vitamin C) are present in the cellular fluids such as cytosol, or cytoplasmic matrix. The lipid-soluble antioxidants ( e.g. vitamin E, carotenoids, and lipoic acid) are predominantly located in cell membranes.
The antioxidants can also be categorized according to their size, the small-molecule antioxidants and large-molecule antioxidants. The small-molecule antioxidants neutralize the ROS in a process called radical scavenging and carry them away. The main antioxidants in this category are vitamin C, vitamin E, carotenoids, and glutathione (GSH). The large-molecule antioxidants are enzymes (SOD, CAT, and GSHPx) and sacrificial proteins (albumin) that absorb ROS and prevent them from attacking other essential proteins.
To understand the mechanism of action of antioxidants, it is necessary to understand the generation of free radicals and their damaging reactions. This review elaborates the generation and damages that free radicals create, mechanism of action of the natural antioxidant compounds and assays for the evaluation of their antioxidant properties. The reaction mechanisms of the antioxidant assays are discussed. The scope of this article is limited to the natural antioxidants and the in vitro assays for evaluation of their antioxidant properties.
2. Generation of free radicals
Peroxynitrite reacts with the aromatic amino acid residues in the enzyme resulting in the nitration of the aromatic amino acids. Such a change in the aminoacid residue can result in the enzyme inactivation. However, nitric oxide is an important cytotoxic effector molecule in the defense against tumor cells, various protozoa, fungi, helminthes, and mycobacteria. 11,12 The other sources of free radical reactions are cyclooxygenation, lipooxygenation, lipid peroxidation, metabolism of xenobiotics, and ultraviolet radiations. 13
3. Damaging reactions of free radicals
Lipid hydroperoxides always break down to aldehydes. Many of these aldehydes are biologically active compounds, which can diffuse from the original site of attack and spread the attack to the other parts of the cell. 18,19 Lipid peroxidation has been widely associated with the tissue injuries and diseases. 20
Oxygen metabolism generates ˙OH, O 2 ˙ − , and the non-radical H 2 O 2 . The ˙OH is highly reactive and reacts with biological molecules such as DNAs, proteins, and lipids, which results in the chemical modifications of these molecules. There are several research reports on the oxidative damage of DNA due to the ˙OH. 21–23
The ˙OH reacts with the basepairs of DNA, resulting in the oxidative damage of the heterocyclic moiety and the sugar moiety in the oligonucleotides by a variety of mechanisms. This type of oxidative damage to DNA is highly correlated to the physiological conditions such as mutagenesis, carcinogenesis, and aging. 24,25 The addition reactions yield OH-adduct radicals of DNA bases ( Scheme 1 ), whereas the allyl radical of thymine and carbon-centered sugar radicals ( Scheme 2 ) are formed from the abstraction reactions.
As shown in the Scheme 1 , the ˙OH reacts with the guanine of the DNA to produce the C-8-hydroxy-adduct radical of guanine, which is converted to the 2,6-diamino-4-hydroxy-5-formamidopyrimidine upon reduction and ring opening reactions. However, the C-8-hydroxy-adduct radical of guanine is converted to the 8-hydroxyguanine upon oxidation reaction. The ˙OH radical reacts with the heterocyclic moiety of the thymine and cytosine at C5- and C6-positions, resulting in the C5–OH and C6–OH adduct radicals, respectively. The oxidation reaction of these adduct radicals with water (followed by deprotonation) results in the formation of the cytosine glycol and thymine glycol, respectively. 26 Overall, the reactions of the ˙OH with the DNA bases result in the impaired dsDNA.
As shown in the Scheme 2 , the ˙OH reacts with the sugar moiety of DNA by abstracting an H-atom from rom C5 carbon atom. One unique reaction of the C5′-centered radical of the sugar moiety in DNA is the addition to the C8-position of the purine ring in the same nucleoside ( e.g. guanine). This intramolecular cyclization results in the formation of the 8,5′-cyclopurine-2′-deoxynucleosides. The reactions of carbon-centered sugar radicals result in the DNA strand breaks and base-free sites by a variety of mechanisms.
Proteins are oxidatively damaged by the combined action of activated oxygen species and the trace metal ions such as Fe 2+ and Cu 2+ . The amino acid's lysine, proline, histidine, and arginine have been found to be the most sensitive to oxidative damage. Recent studies indicate that, a wide range of residue modifications can occur including formation of peroxides, 27,28 and carbonyls. 29 Generation of the carbonyl residue is a useful measure of oxidative damage to proteins. Thus, the oxidative damage to tissue results in the increased amount of oxidized protein. A detailed review by Cooke et al. provides important informations on the oxidative DNA damage, mechanisms, mutations, and related diseases. 30
Low levels of antioxidants have been associated with the heart disease and cancer. 31,32 Antioxidants provide protection against a number of disease processes such as aging, allergies, algesia, arthritis, asthma, atherosclerosis, autoimmune diseases, bronchopulmonary dyspepsia, cancer. The other disorders to which antioxidants provide protection are cataract, cerebral ischemia, diabetes mellitus, eczema, gastrointestinal inflammatory diseases, genetic disorders. 33 Following section elaborates the mechanism of action of the radical scavenging activities of various natural antioxidant molecules.
4. Modulation of free radicals by natural antioxidants
SOD's located in the cytosol and mitochondria, catalytically convert the O 2 ˙ − into oxygen and H 2 O 2 in presence of the metal ion cofactors such as copper (Cu), zinc (Zn), or manganese (Mn). 35 The enzyme CAT present in the peroxisome, converts H 2 O 2 to water and oxygen ( eqn (12) ). 36,37 GSHPx are found both in the cytoplasm and extracellularly in almost every human tissue. GSHPx convert the H 2 O 2 into the water ( Table 2 ). The enzyme GSHPx has strong activity towards both H 2 O 2 and fatty acid hydroperoxides ( eqn (13) ). 38,39 The enzyme peroxyredoxin catalyze the reduction of H 2 O 2 , organic hydroperoxides and the peroxynitrite (ONOO − ). The different expression profiles, subcellular locations, and substrates of the antioxidant enzymes reveal the complex nature of the ROS biology. Clearly, the antioxidant enzymes play a major role in the prevention of oxidative damage. As demonstrated in the Scheme 3 , CAT, GSHPx, and SOD show synergistic effect in the scavenging of O 2 ˙ − .
The enzymatic antioxidants and their mechanism of antioxidant activity has been explained in details in several review articles. 40–42 Therefore, this article focuses mainly on the nonenzymatic antioxidants of natural origin.
The nonenzymatic antioxidants are of two types, the natural antioxidants and the synthetic antioxidants. However, the scope of this article is limited to the natural antioxidants; hence the synthetic antioxidants will not be considered for the discussion.
4.1. Vitamins
The resultant tocopheroxyl radical is relatively stable and in normal circumstances, insufficiently reactive to initiate lipid peroxidation itself, which is an essential criterion of a good antioxidant. 45–47 It should be noted that, vitamin E exerts antioxidant effects by scavenging lipid peroxyl radicals in vivo as well as in vitro systems. However, vitamin E is not an efficient scavenger of ˙OH and alkoxyl radicals (˙OR) in vivo . 48
Vitamin C or ascorbic acid 2 , is a water-soluble free radical scavenger. Moreover, it regenerates vitamin E in cell membranes in combination with GSH or compounds capable of donating reducing equivalents. 49–51 Vitamin C, changes to the ascorbate radical ( Scheme 4 ) by donating an electron to the lipid radical in order to terminate the lipid peroxidation chain reaction. The pairs of ascorbate radicals react rapidly to produce one molecule of ascorbate and one molecule of dehydroascorbate. The dehydroascorbate does not have any antioxidant capacity. Hence, dehydroascorbate is converted back into the ascorbate by the addition of two electrons. The last stage of the addition of two electrons to the dehydroascorbate has been proposed to be carried out by oxidoreductase.
Antioxidant potential of vitamin A 3 was first described by Monaghan and Schmitt, 52 who reported that vitamin A can protect lipids against rancidity. Several reviews have appeared to outline the basic structural and metabolic characteristics of vitamin A and information about its potential as antioxidants in relation to the heart diseases. 53,54 Vitamin A has a vital antioxidant contribution in protecting human LDL against copper-stimulated oxidation ( Scheme 5 ). 55,56
4.2. Bioflavonoids
Bioflavonoids are a group of natural benzo-γ-pyran derivatives ( 4–23 ) and are found to possess strong antioxidant activities. 59,60 Bioflavonoids widely distributed in fruits and vegetables, are reported to exert multiple biological effects including free radical-scavenging activity. It has been reported that the bioflavonoids have a protective effect on the DNA damage induced by the hydroxyl radicals. 61 One of the mechanisms that explains the protective effect of the flavonoids on the DNA is the involvement of the chelating metal ions, such as copper or iron. The flavonoids complexed with the copper or iron prevent the generation of the ROS. 62–64
Quercetin 5 is a flavonol, known to protect DNA from oxidative damage resulting from the attack of ˙OH, H 2 O 2 , and O 2 ˙ − on the DNA oligonucleotides ( Scheme 6 ). 65 On the contrary, quercetin is also reported to be carcinogenic agent. 66,67 According to the reports, quercetin has opposite effects on DNA damage induced by cupric ion depending on the concentration of cupric ion ( Scheme 7 ). 68,69 At the low concentration of cupric ions (≤25 μM), quercetin exhibit a protective role. While, at higher concentration of cupric ion (≥25 μM), quercetin enhances the damage to DNA by ROS. Therefore, it is very important to consider the concentration of the chelating metal ions, such as copper or iron while evaluating the protective or degenerative effects of quercetin and other bioflavonoids.
Anthocynidine, a class of flavonoids are potential antioxidants and their effectiveness in the inhibition of the lipid oxidation is related to their metal ion-chelating activity ( Scheme 8 ) and free-radical scavenging activity ( Scheme 9 ). Three structural groups are important determinants of the radical-scavenging activity of anthocynidines 18–20 . 70 First, the ortho -dihydroxy structure in the B-ring. Second, the 2,3 double bond in conjugation. Third, the 4-oxofunction in the C-ring. Flavonoids form complexes with the metal ions by using the 3- or 5-hydroxyl and 4-ketosubstituents or hydroxyl groups in ortho position in the B-ring. 71
As shown in the Scheme 9 , the anthocynidins (cynidin 19 ) can donate an electron (accompanied by a hydrogen nucleus) to a free radical from –OH groups attached to the phenolic rings. 72–74 This electron stabilizes and inactivates the free radical. In this process, the polyphenolic reducing agent changes to an aroxyl radical, which is comparatively more stable due to resonance than the free radical that it has reduced. The overall result is the termination of damaging oxidative chain reactions.
4.3. Carotenoids 75,76
(ii) Xanthophyll: zeaxanthine 28 , lutein 29 .
Carotenoids are among the most common lipid soluble phytonutrients. Lycopene 24 and β-carotene 25 are the prominent carotenoids among other 600 different compounds. 77 The biosynthetic pathway as shown in Scheme 10 demonstrates the synthesis of carotenoids 26–29 from phytoene 25 , which is synthesized from two molecules of geranylgeranyl pyrophosphate 24 . Carotenoids are well known to scavenge the peroxyl radicals more efficiently as compared to any other ROS. The peroxyl radicals generated in the process of lipid peroxidation can damage the lipids in the cell wall. Scavenging of peroxyl radicals can disrupt the reaction sequence and prevent the damage to cellular lipids. The long unsaturated alkyl chains in carotenoids make them highly lipophilic. Carotenoids are known to play an important role in the protection of cellular membranes and lipoproteins against the ROS due to their peroxyl radical scavenging activity. 78,79 Carotenoids deactivate the peroxyl radicals by reacting with them to form resonance stabilized carbon-centered radical adducts.
Lycopene 24 , is the most potent antioxidant naturally present in many fruits and vegetables. The high number of conjugated double bonds in lycopen endows it the singlet oxygen quenching ability. Lycopene demonstrate the strong singlet oxygen quenching ability as compared to the α-tocopherol 1 or β-carotene 25 . 80 β-Carotene 12 is a naturally occurring orange-colored carotenoid, abundantly found in the yellow-orange fruits and in dark-green leafy vegetables. 81,82 β-Carotene demonstrates potential antioxidant property due to its chemical structure and the interaction with biological membranes. 83 It is well-known that, the β-carotene quenches singlet oxygen with higher efficiency as compared to the α-tocopherol. 84 In addition, it is also known that the ( Z )-isomers of the β-carotene possess antioxidant activity in vitro . 85,86 Furthermore, the β-carotene can be converted into the two molecules of vitamin A by the β-carotene-15,15′-dioxygenase catalyzed cleavage.
4.4. Hydroxycinnamates
It is widely accepted that, the dietary antioxidants that protect LDL from oxidation can prevent the atherosclerosis and coronary heart disease. Hydroxycinnamic acids 30–33 and their conjugates prevent oxidative damage to the LDL. 87 The in vitro studies involving human LDL as the oxidizing substrate showed that the hydroxycinnamic acids have higher antioxidant activity as compared to the corresponding hydroxybenzoic acids. 88 The antioxidant activity of the derivatives of the hydroxycinnamates is clearly correlated with the hydroxylation and methylation patterns of the aromatic ring. The antioxidant efficiency of the free hydroxycinnamates on the human LDL oxidation in vitro , decreases in the order of caffeic acid 31 > sinapic acid 32 > ferulic acid 30 > p -coumaric acid 33 .
The presence of the o -dihydroxy group in the phenolic ring (as in caffeic acid) enhances the antioxidant activity of hydroxycinnamic acids toward human LDL oxidation in vitro . 89 The radical scavenging antioxidant mechanism of the hydroxycinnamic acids are similar to that of the flavanoids because of their ability to donate an hydroxyl hydrogen and resonance stabilization of the resulting antioxidant radicals. The o -dihydroxy substituents also allow the metal ion chelation similar to that of flavanoids.
4.5. Other natural antioxidants
Theaflavin 34 and theaflavin-3-gallate 35 possesses in vitro antioxidative properties against lipid peroxidation in the erythrocyte membranes and microsomes. They also suppress the mutagenic effects induced by H 2 O 2 . 92 Theaflavins inhibit the H 2 O 2 induced cleavage and mutagenicity of the DNA single-strand. 93,94 In general, theaflavins scavenge the free radicals to produce antioxidative and antimutagenic effects. Apart from the aromatic hydroxyl groups of theaflavins, the gallic acid moiety is essential for their antioxidant activity. The theaflavin-3-gallate 35 is a stronger antioxidant than that of theaflavin 34 . Moreover, the digallate derivatives of theaflavin demonstrate the increased antioxidant activity.
Allicin (diallyl thiosulfinate) 36 is the biologically active compound mainly found in the garlic extracts. Allicin is known to possess various biological activities including the antibacterial, antifungal, and inhibition of cancer promotion. 95 Moreover, allicin is known to reduce serum cholesterol and triglyceride levels as well as atherosclerotic plaque formation and platelet aggregation. 96 Until now, a variety of biological effects of allicin were attributed to antioxidant activity. 97 However, recently it has been found that the active ingredients responsible for the antioxidant property of garlic is 2-propenesulfenic acid and not the allicin. 98 Thiosulfinates undergoes Cope elimination to form sulfenic acids, thioaldehydes or thioketones. The S–S bond in the thiosulfinate is much weaker than the S–C bond in a sulfoxide. Hence, this process can occur at room temperature. Cope elimination is even more susceptible for the allyl (and benzyl) thiosulfinates, such as allicin 36 , because of the weak β C–H bond of the allyl moiety. Allicin is known to undergo Cope elimination at room temperature to give 2-propenesulfenic acid and thioacrolein as shown in the Scheme 11 . 99,100
The Scheme 12a demonstrate the mechanism of the radical-scavenging activity of the allicin. The radical-scavenging activity of allicin involves H-atom transfer to a peroxyl radical from the methylene of the allyl group on the divalent sulfur. Scheme 12b demonstrate an alternative mechanism, where the radical-scavenging activity of allicin can be accounted for 2-propenesulfenic acid, which is produced from allicin by Cope elimination. 101 2-Propenesulfenic acid is reported to be over 1000 times more reactive toward ˙OOH radicals than allicin (2.60 × 10 7 vs. 7.38 × 10 3 L mol −1 s −1 , at 298 K). 102
Piperine (1-piperoylpiperidine) 37 , is an alkaloid present in fruits of black pepper ( Piper nigrum ), long pepper ( Piper longum ), and other piper species (family: Piperaceae). Piperine possesses many pharmacological activities, including anti-inflammatory and analgesic effect, 103 anti-ulcer activities, 104 antidepressant effect, 105 cognitive enhancing effect, 106 cytoprotective effect, and antioxidant activity. 107 It is interesting to notice that, the higher concentration of piperine results in the increased production of the ˙OH. Whereas, in low concentrations piperine acts as an antioxidant. 108 Piperine demonstrates synergistic antioxidant activity by doubling the absorption of dietary curcumin 38 . 109
Curcumin 38 , a lipid soluble active principle of turmeric is a bis-α, β-unsaturated β-diketone that exhibit's keto–enol tautomerism. 110 Curcumin 38 , shows remarkable antioxidant activity, and it has been found to be an excellent free radical scavenger. 111 Curcumin has a chain breaking antioxidant ability comparable to that of the vitamin E.
As shown in the Schemes 13 and 14 , the free radical scavenging activity of curcumin is correlated to the phenolic OH group and the CH 2 group of the β-diketone moiety. The free radical can undergo electron transfer or abstract H-atom from either of these two sites. However, pulse radiolysis and other biochemical methods credited the antioxidant activity of curcumin to its phenolic OH group. 112
The Scheme 14 depicts the mechanism for the autoxidation of curcumin initiated by hydrogen abstraction from one of the phenolic hydroxyl groups. 113 The phenoxyl radical moves into the carbon chain leaving a quinone methide that is eventually quenched by the water molecules. The methide radical performs a 5-exo-cyclization with the double bond to give the cyclopentadione ring and generating the carbon-centered radical.
The reaction of curcumin with the molecular oxygen (O 2 ) results in the peroxyl radical. The peroxyl radical is then reduced to the hydroperoxide by abstracting a hydrogen atom from another curcumin molecule, propagating the autoxidation chain reaction. Subsequently, the hydroperoxide loses water and rearranges into the spiro-epoxide. The hydrolysis of the epoxide by the (water-derived) hydroxyl group results in the formation of the final bicyclopentadione product. 114 It has been found that the copper complex of curcumin (curcumin–Cu( II )) show promising SOD activity, with improved antioxidant efficacy. 115,116
The mechanism of the O 2 ˙ − scavenging activity of the curcumin–Cu( II ) complex is depicted in the Scheme 15 . When O 2 ˙ − are allowed react with the curcumin–Cu( II ) complex, a major fraction of O 2 ˙ − reacts with Cu 2+ moiety, while only a small fraction reacts with curcumin. The reaction causes reduction of Cu 2+ to Cu + . The Cu + undergoes subsequent oxidation by another molecule of O 2 ˙ − , thereby regenerating the parent complex.
Therefore, the catalytic activity comes mainly from the reversible redox reactions within the Cu 2+ /Cu + couple in the complex. However, in presence of the excess O 2 ˙ − , the phenolic moiety undergoes oxidation resulting in the production of the phenoxyl radicals. Then these phenoxyl radicals can generate new products or react with reduced copper ions of the complex resulting in the regeneration of the complex.
4.6. Physisological antioxidants
Uric acid 39 in plasma possesses strong radical scavenging activity. 117,118 Uric acid is the most abundant aqueous antioxidant found in humans. It contributes for as much as two-thirds of all free radical scavenging activities in the plasma. 119 Uric acid is a powerful scavenger of carbon-centered radicals and peroxyl radicals in the hydrophilic environment. However, it loses it's radical scavenging activity within lipid membranes. 120
Uric acid is an exceptional scavenger of peroxynitrite (ONOO − ) in the extracellular fluid. 121 However, it is important to note that the uric acid cannot scavenge the O 2 ˙ − . Moreover, uric acid requires the presence of ascorbic acid ( Scheme 16 ) and thiols for the complete scavenging of peroxynitrites. Neither of these antioxidants (ascorbic acid, thiols) alone can prevent reaction of peroxynitrite with tetrahydrobiopterin, which leads to uncoupling of nitric oxide (NO˙) synthase. 122 This indicates that the uric acid plays a crucial role in the scavenging of the peroxynitrite.
GSH 40 in cell cytosol, together with its related enzymes, comprises a system that maintains the intracellular reducing environment, which acts as primary defense against excessive generation of harmful ROS. 123,124 The oxygen radical scavenging activity of GSH directly expedites the ROS neutralization and the repair of ROS-induced damage. 125
As shown in the Scheme 17 , three groups of enzymes can be identified in the GSH catalytic cycle: glutathione oxidase, glutathione reductase, and GSHPx. Glutathione oxidase and GSHPx catalyze the oxidation of GSH to GSH disulfide (GSSG). Whereas, glutathione reductase is responsible for the regeneration of GSH from GSSG in an NADPH-dependent process. 126 Cells can produce GSSG or convert it to GSH by using NADPH in the presence of the glutathione reductase. However, the de nova synthesis of glutathione from its amino acid constituents is required for the elevation of glutathione as an adaptive response to oxidative stress. The presence of the sulfhydryl group in glutathione allows it to serve as an antioxidant.
4.7. Fungal antioxidants
5. in vitro methods for evaluation of antioxidant activity, 5.1. assay of superoxide anion radical scavenging activity.
The method for the evaluation of the O 2 ˙ − scavenging activity of antioxidants is explained here by using PMS–NADH–NBT system, which is composed of N -methylphenazine methosulphate (PMS), nitroblue tetrazolium chloride (NBT), and NADH (a reduced form of nicotineamide-adenine-dinucleotide).
As shown in the Scheme 18 , the O 2 ˙ − produced in the coupling reaction of PMS–NADH in presence of dissolved oxygen reduces NBT. The decrease of absorbance at 560 nm with antioxidant indicates the consumption of O 2 ˙ − in the reaction mixture. The O 2 ˙ − scavenging activity can be measured as described by Robak and Gryglewski. 140 Gallic acid, BHA, ascorbic acid, α-tocopherol, and curcumin can be used as positive controls in this assay.
5.2. Assay of 2,2-diphenyl-1-picrylhydrazyl (DPPH) free radical scavenging activity
Due to its odd electron, the methanolic solution of DPPH shows a strong absorption band at 517 nm. As shown in the Scheme 19 , the DPPH radical reacts with suitable reducing agent producing new bond, thus changing the color of solution. The solution loses color with the increase in the concentration of antioxidant as the electrons taken up by DPPH radical from the antioxidant. 143,144 Such reactivity has been used to test the ability of compounds/plant extracts to act as free radical scavengers. 145 Reduction of the DPPH radicals can be monitored spectrophotometrically by the decrease in absorbance at 517 nm.
5.3. Assay for total reactive oxygen potential (TRAP) and total antioxidant reactivity (TAR)
As shown in the Scheme 20 , the AAPH undergoes thermal decomposition in solution to produce two carbon-centered amidino propane (AP) radicals, which can add O 2 to form peroxyl radicals. However, the carbon-centered radicals usually predominate. 151 The amidino propane (AP) radical takes up a proton from luminol to produce a luminol radical. The luminol radical reacts with de-protonated H 2 O 2 to yield a short-lived hydroperoxide intermediate (LO 2 H − ), which rapidly decomposes into the excited state 3-aminophthalic acid (AP*). The AP* loses energy in the form of chemiluminescence to give ground state 3-aminophthalic acid. 152
5.4. In vitro antioxidant evaluation by phospholipids peroxidation
The activity of test compound to inhibit peroxidation of membrane lipids at pH 7.4 is tested using phospholipids. The interference of the test drug with color development is determined by adding a previously determined concentration of the test compound to the TBA reagents and used to determine the extent of peroxidation of animal phospholipids. 156,157 In this assay, the antioxidant activity is a measure of concentration-dependent inhibition of a phospholipid peroxidation.
5.5. In vitro antioxidant evaluation by deoxyribose assay
The reaction of deoxyribose and ˙OH has been discussed extensively in the literature. 158,159 The ˙OH attack deoxyribose to form products that react with TBA upon heating at low pH and yield a pink chromogen. Scheme 22 depicts the proposed mechanism of chromogen formation from reaction of deoxyribose and ˙OH followed by reaction with TBARS.
In general, the in vivo assays for testing potential antioxidants are more expensive because they require complex cellular testing systems or full clinical trials. However, it is very important to proceed to cellular assays after screening antioxidant activity with an in vitro method in order to obtain information on some aspects like uptake, bioavailability, and metabolism. 160 The new definition of an antioxidant, a redox-active compound or mixture able to modulate the redox status of the cell, makes it critical to use in vivo assays in order to evaluate the antioxidant activity of a compound. 161 There are several reports on the in vivo assays for the evaluation of the antioxidant activity. 162,163 However, we have limited the scope of this review to the in vitro assays for the evaluation of the antioxidant activities of natural antioxidants. There are several other reports, which elaborate the advantages and disadvantages of various methods for the evaluation of antioxidant activity. 164,165
6. Current trends and future directions
Many investigators found that, increasing the level of defense mechanisms against oxidative stress could extend an organism's health span. Therefore, few setbacks in the antioxidant research with the molecules showing strong antioxidant activity in vitro and non-antioxidant effects in cells and tissues should not discourage the important research in this field. Finally, the collective effort is must be undertaken for the understanding of the mechanisms in the free radical scavenging activities of known antioxidants to derive the potent antioxidants.
7. Conclusion
Conflicts of interest, abbreviations, acknowledgements, notes and references.
- I. S. Young and J. V. Woodside, J. Clin. Pathol. , 2001, 54 , 176–186 CrossRef CAS .
- K. Bagchi and S. Puri, East. Meditrr. Health. J. , 1998, 4 , 350–360 Search PubMed .
- Y. W. Kim and T. V. Byzova, Blood , 2014, 123 , 625–631 CrossRef CAS PubMed .
- H. Sies, Exp. Physiol. , 1997, 82 , 291–295 CrossRef CAS .
- D. K. Pal and S. B. Nimse, Asian J. Chem. , 2006, 13 , 3004–3008 Search PubMed .
- C. E. Thomas and B. Kalyanaraman, in Oxygen Radical and the Disease Process , Harvard Academic Publishers, Netherlands, 1997 Search PubMed .
- F. Shahidi and Y. Zhong, Eur. J. Lipid Sci. Technol. , 2010, 112 , 930–940 CrossRef CAS .
- B. M. Babior, Blood , 1999, 93 , 1464–1476 CAS .
- J. A. Knight, in Free radicals, antioxidants, aging and disease , AACC Press, Washington, 1999 Search PubMed .
- L. Zhu, C. Gunn and J. S. Beckman, Arch. Biochem. Biophys. , 1992, 298 , 452–457 CrossRef CAS .
- S. Moncanda, R. M. J. Palmer and E. A. Higgs, Pharmacol. Rev. , 1991, 43 , 109–142 Search PubMed .
- C. F. Nathan and J. B. Hibbs Jr, Curr. Opin. Immunol. , 1991, 3 , 65–70 CrossRef CAS .
- F. Shahidi and Y. Zhong, Chem. Soc. Rev. , 2010, 39 , 4067–4079 RSC .
- A. V. Rao and S. Agarwal, Nutr. Res. , 1999, 19 , 305–326 CrossRef CAS .
- A. M. Jenkinson, A. R. Collins, S. J. Duthie, K. W. J. Wahle and G. G. Duthie, FASEB J. , 1999, 13 , 2138–2142 CAS .
- Y. Park, S. Nam, H. J. Yi, H. J. Hong and M. Lee, Nutr. Res. , 2009, 29 , 812–818 CrossRef CAS PubMed .
- H. Esterbauer, M. Dieber-Rotheneder, G. Waeg, G. Striegl and G. Juergens, Chem. Res. Toxicol. , 1990, 3 , 77–92 CrossRef CAS .
- W. A. Pryor and N. A. Porter, Free Radical Biol. Med. , 1990, 8 , 541–543 CrossRef CAS .
- T. P. A. Devasgayam, K. K. Boloor and T. Ramasarma, Indian J Biochem. Biophys. , 2003, 40 , 300–308 Search PubMed .
- H. Esterbauer, R. J. Schanr and H. Zollen, Free Radical Biol. Med. , 1991, 11 , 81–121 CrossRef CAS .
- M. Dizdaroglu, P. Jaruga, M. Birincioglu and H. Rodriguez, Free Radical Biol. Med. , 2002, 32 , 1102–1115 CrossRef CAS .
- B. Halliwell and J. M. C. Gutteridge, in Free radicals in biology and medicine , Oxford University Press, Oxford, 4th edn, 2007 Search PubMed .
- C. von Sonntag, in Free-radical-induced DNA damage and its repair , Springer, Hiedelberg, 2006 Search PubMed .
- M. Dizdaroglu, Mutat. Res. , 1992, 275 , 331–342 CAS .
- A. P. Breen and J. A. Murphy, Free Radical Biol. Med. , 1995, 18 , 1033–1077 CrossRef CAS .
- M. Dizdaroglu and P. Jaruga, Free Radical Res. , 2012, 46 , 382–419 CrossRef CAS PubMed .
- J. A. Simpson, S. Narita, S. Gieseg, S. Gebicki, J. M. Gebicki and R. T. Dean, Biochem. J. , 1992, 282 , 621–624 CAS .
- S. Gieseg, S. Duggan and J. M. Gebicki, Biochem. J. , 2000, 350 , 215–218 CrossRef CAS .
- E. R. Stadtman, Free Radical Biol. Med. , 1990, 9 , 315–325 CrossRef CAS .
- M. S. Cooke, M. D. Evans, M. Dizdaroglu and J. Lunec, FASEB J. , 2003, 17 , 1195–1214 CrossRef CAS PubMed .
- P. M. Kris-Etherton, K. D. Hecker, A. Bonanome, S. M. Coval, A. E. Binkoski, K. F. Hilpert, A. E. Griel and T. D. Etherton, Am. J. Med. , 2002, 113 , 71–88 CrossRef .
- D. Pal, S. Banerjee and A. Ghosh, J. Adv. Pharm. Technol. Res. , 2012, 3 , 16–24 CAS .
- E. A. Lissi, M. Salim-Hanna, C. Pascual and M. Del-Castillo, Free Radical Biol. Med. , 1995, 18 , 153–158 CrossRef CAS .
- M. Koruk, S. Taysi, M. C. Savas, O. Yilmaz, F. Akcay and M. Karakok, Ann. Clin. Lab. Sci. , 2004, 34 , 57–62 CAS .
- D. R. Gough and T. G. Cotter, Cell Death Dis. , 2011, 2 , e213 CrossRef CAS PubMed .
- C. D. Zhan, R. K. Sindhu, J. Pang, A. Ehdaie and N. D. Vaziri, J. Hypertens. , 2004, 22 , 2025–2033 CrossRef CAS PubMed .
- J. R. Stone and S. Yang, Antioxid. Redox Signaling , 2006, 8 , 243–270 CrossRef CAS PubMed .
- E. Cabiscol, J. Tamarit and J. Ros, Int. Microbiol. , 2000, 3 , 3–8 CAS .
- J. R. Arthur, Cell Mol. Life Sci. , 2000, 57 , 1825–1835 CrossRef CAS .
- J. M. Matés, Toxicology , 2000, 153 , 83–104 CrossRef .
- M. Valko, C. J. Rhodes, J. Moncol, M. Izakovic and M. Mazur, Chem.–Biol. Interact. , 2006, 160 , 1–40 CrossRef CAS PubMed .
- J. M. Matés, C. Perez-Gomez and I. N. De Castro, Clin. Biochem. , 1999, 32 , 595–603 CrossRef .
- S. Tafazoli, J. S. Wright and P. J. O'Brien, Chem. Res. Toxicol. , 2005, 18 , 1567–1574 CrossRef CAS PubMed .
- A. Vojdani, M. Bazargan, E. Vojdani and J. Wright, Cancer. Detect. Prev. , 2000, 24 , 508–523 CAS .
- P. K. Witting, J. M. Upston and R. Stocker, Biochemistry , 1997, 36 , 1251–1258 CrossRef CAS PubMed .
- P. Morlière, L. K. Patterson, C. M. M. Santos, A. M. S. Silva, J. Mazière, P. Filipe, A. Gomes, E. Fernandes, M. B. Q. Garciah and R. Santusc, Org. Biomol. Chem. , 2012, 10 , 2068–2076 Search PubMed .
- R. Stocker, V. W. Boury and B. Frei, Proc. Natl. Acad. Sci. U. S. A. , 1991, 88 , 1646–1650 CrossRef CAS .
- E. Niki, Free Radical Biol. Med. , 2014, 66 , 3–12 CrossRef CAS PubMed .
- E. Niki, Am. J. Clin. Nutr. , 1991, 54 , 1119S–1124S CAS .
- K. L. Retsky, M. W. Freeman and B. Frei, J. Biol. Chem. , 1993, 268 , 1304–1309 CAS .
- C. Oh, M. Li, E. Kim, J. S. Park, J. Lee and S. W. Ham, Bull. Korean Chem. Soc. , 2010, 31 , 3513–3514 CrossRef CAS .
- B. R. Monaghan and F. O. Schmitt, J. Biol. Chem. , 1932, 96 , 387–395 CAS .
- R. S. Parker, FASEB J. , 1996, 10 , 542–551 CAS .
- A. V. Vieira, W. J. Schneider and P. M. Vieira, J. Endocrinol. , 1995, 146 , 201–207 CrossRef CAS .
- M. A. Livrea, L. Tesoriere, A. Bongiorno, A. M. Pintaudi, M. Ciaccio and A. Riccio, Free Radical Biol. Med. , 1995, 18 , 401–409 CrossRef CAS .
- L. Tesoriere, D. D'arpa, R. Re and M. A. Livrea, Arch. Biochem. Biophys. , 1997, 343 , 13–18 CrossRef CAS PubMed .
- P. G. Pietta, J. Nat. Prod. , 2000, 63 , 1035–1042 CrossRef CAS PubMed .
- C. A. Rice-Evans, N. J. Miller and G. Paganga, Free Radical Biol. Med. , 1996, 20 , 933–956 CrossRef CAS .
- P. Pietta, J. Nat. Prod. , 2000, 63 , 1035–1042 CrossRef CAS PubMed .
- R. J. Nijveldt, E. van Nood, D. E. C. van Hoorn, P. G. Boelens, K. van Norren and P. A. van Leeuwen, Am. J. Clin. Nutr. , 2001, 74 , 418–425 CAS .
- A. Russo, R. Acquaviva, A. Campisi, V. Sorrenti, C. D. Giacomo, G. Virgata, M. L. Barcellona and A. Vanella, Cell Biol. Toxicol. , 2000, 16 , 91–98 CrossRef CAS .
- F. V. Rubens de Souza and F. W. De Giovani, Redox Rep. , 2004, 9 , 97–104 CrossRef PubMed .
- T. Armida, T. Maurizio, T. Andrea and B. Sergio, J. Mol. Struct. , 2005, 44 , 759–766 Search PubMed .
- J. Zhou, L. F. Wang, J. Y. Wang and N. Tang, Transition Met. Chem. , 2001, 26 , 57–93 CrossRef CAS .
- V. Krishnamachari, L. H. Levine and P. W. Pareá, J. Agric. Food Chem. , 2002, 50 , 4357–4363 CrossRef CAS PubMed .
- A. Das, J. H. Wang and E. J. Lien, Prog. Drug Res. , 1994, 42 , 133–166 CrossRef CAS .
- S. J. Duthie, J. W. ohnson and V. L. Dobson, Mutat. Res. , 1997, 390 , 141–151 CAS .
- T. Jun, Z. Liancai and W. Bochu, Int. J. Pharmacol. , 2007, 3 , 19–26 Search PubMed .
- D. Galaris and A. Evangelou, Crit. Rev. Oncol. Hematol. , 2002, 42 , 93–103 CrossRef .
- S. S. Pekkarinen, I. M. Heinonen and A. I. Hopia, J. Sci. Food Agric. , 1999, 79 , 499–506 CrossRef CAS .
- M. G. Miguel, J. Appl. Pharm. Sci. , 2011, 01 , 07–15 Search PubMed .
- A. Castañeda-Ovando, M. L. Pacheco-Hernández, M. E. Páez-Hernández, J. A. Rodríguez and G.-C. Vidal, Food Chem. , 2009, 113 , 859–871 CrossRef PubMed .
- R. J. Nijveldt, E. van Nood, D. E. C. van Hoorn, P. G. Boelens, K. van Norren and P. A. M. van Leeuwen, Am. J. Clin. Nutr. , 2001, 74 , 418–425 CAS .
- S. A. van Acker, D. J. van den Berg, M. N. Tromp, D. H. Griffioen, W. P. van Bennekom, W. J. van der Vijgh and A. Bast, Free Radical Biol. Med. , 1996, 20 , 331–342 CrossRef CAS .
- W. Stahl and H. Sies, Mol. Aspects Med. , 2003, 24 , 345–351 CrossRef CAS .
- L. Mueller and V. Boehm, Molecules , 2011, 16 , 1055–1069 CrossRef PubMed .
- J. A. Olson and N. I. Krinsky, FASEB J. , 1995, 9 , 1547–1550 CAS .
- H. Sies and W. Stahl, Am. J. Clin. Nutr. , 1995, 62 , 1315S–1321S CAS .
- J. G. Erhardt, C. Meisner, J. C. Bode and C. Bode, Am. J. Clin. Nutr. , 2003, 78 , 1219–1224 CAS .
- A. V. Rao and L. G. Rao, Pharmacol. Res. , 2007, 55 , 207–216 CrossRef CAS PubMed .
- N. I. Krinsky and E. J. Johnson, Mol. Aspects Med. , 2005, 26 , 459–516 CrossRef CAS PubMed .
- G. Riccioni, Curr. Atheroscler. Rep. , 2009, 11 , 434–439 CrossRef CAS .
- P. Di Mascio, S. Kaiser and H. Sies, Arch. Biochem. Biophys. , 1989, 274 , 532–538 CrossRef CAS .
- G. Levin, M. Yeshurun and S. Mokady, Nutr. Cancer , 1997, 27 , 293–297 CrossRef CAS PubMed .
- V. Böhm, N. L. Puspitasari-Nienaber, M. G. Ferruzzi and S. J. Schwartz, J. Agric. Food Chem. , 2002, 50 , 221–226 CrossRef PubMed .
- M. F. Andreasen, A. Landbo, L. P. Christensen, Å. Hansen and A. S. Meyer, J. Agric. Food Chem. , 2001, 49 , 4090–4096 CrossRef CAS PubMed .
- F. Natella, M. Nardini, M. Di Filici and C. Scaccini, J. Agric. Food Chem. , 1999, 47 , 1453–1459 CrossRef CAS PubMed .
- A. S. Meyer, J. L. Donovan, D. A. Pearson, A. L. Waterhouse and E. N. Frankel, J. Agric. Food Chem. , 1998, 46 , 1783–1787 CrossRef CAS .
- V. P. Menon and A. R. Sudheer, Adv. Exp. Med. Biol. , 2007, 595 , 105–125 CrossRef .
- J. S. Wright, J. Mol. Struct.: THEOCHEM , 2002, 59 , 207–217 CrossRef .
- N. J. Miller, C. Castelluccio, L. Tijburg and C. Rice-Evans, FEBS Lett. , 1996, 392 , 40–44 CrossRef CAS .
- M. Shiraki, Y. Hara, T. Osawa, H. Kumon, T. Nakayama and S. Kawakishi, Mutat. Res. , 1994, 323 , 29–34 CAS .
- L. K. Leung, Y. Su, R. Chen, Z. Zhang, Y. Huang and Z. Y. Chen, J. Nutr. , 2001, 131 , 2248–2251 CAS .
- S. Ankri, T. Miron, A. Rabinkov, M. Wilchek and D. Mirelman, Antimicrob. Agents Chemother. , 1997, 41 , 2286–2288 CAS .
- K. C. Agarwal, Med. Res. Rev. , 1996, 16 , 111–124 CrossRef CAS .
- K. Prasad, V. A. Laxdal, M. Yu and B. L. Raney, Mol. Cell. Biochem. , 1995, 148 , 183–189 CrossRef CAS .
- V. Vaidya, K. U. Ingold and D. A. Pratt, Angew. Chem., Int. Ed. , 2009, 48 , 157–159 CrossRef CAS PubMed .
- E. Block, Angew. Chem., Int. Ed. , 1992, 31 , 1135–1178 CrossRef .
- P. T. Lynett, K. Butts, V. Vaidya, G. E. Garretta and D. A. Pratt, Org. Biomol. Chem. , 2011, 9 , 3320–3330 CAS .
- R. Amorati, P. T. Lynett, L. Valgimigli and D. A. Pratt, Chem.–Eur. J. , 2012, 18 , 6370–6379 CrossRef CAS PubMed .
- A. Galano and M. Francisco-Marquez, J. Phys. Chem. B , 2009, 113 , 16077–16081 CrossRef CAS PubMed .
- S. K. Gupta, P. Bansal, R. K. Bhardwaj and T. Velpandian, Pharmacol. Res. , 2000, 41 , 657–662 CrossRef CAS PubMed .
- Y. F. Bai and H. Xu, Acta Pharmacol. Sin. , 2000, 21 , 357–359 CAS .
- S. A. Lee, S. S. Hong, X. H. Han, J. S. Hwang, G. J. Oh, K. S. Lee, M. K. Lee, B. Y. Hwang and J. S. Ro, Chem. Pharm. Bull. , 2005, 53 , 832–835 CrossRef CAS .
- J. Wattanathorn, P. Chonpathompikunlert, S. Muchimapura, A. Priprem and O. Tankamnerdthai, Food Chem. Toxicol. , 2008, 46 , 3106–3110 CrossRef CAS PubMed .
- K. Selvendiran, J. P. Singh, K. B. Krishnan and D. Sakthisekaran, Fitoterapia , 2003, 74 , 109–115 CrossRef CAS .
- G. Shoba, D. Joy, T. Joseph, M. Majeed, R. Rajendran and P. S. Srinivas, Planta Med. , 1998, 64 , 353–356 CrossRef CAS PubMed .
- P. Anand, A. B. Kunnumakkara, R. A. Newman and B. B. Aggarwal, Mol. Pharm. , 2007, 4 , 807–818 CrossRef CAS PubMed .
- P. Ananda, S. G. Thomas, A. B. Kunnumakkara, C. Sundaram, K. B. Harikumar, B. Sung, S. T. Tharakan, K. Misra, I. K. Priyadarsini, K. N. Rajasekharan and B. B. Aggarwal, Biochem. Pharmacol. , 2008, 76 , 1590–1611 CrossRef PubMed .
- K. I. Priyadarsini, D. K. Maity, G. H. Naik, M. Sudheer, M. K. Unnikrishnan, J. G. Satav and H. Mohan, Free Radical Biol. Med. , 2003, 35 , 475–484 CrossRef CAS .
- M. Salem, S. Rohanib and E. R. Gillies, RSC Adv. , 2014, 4 , 10815–10829 RSC .
- O. N. Gordon and C. Schneider, Trends Mol. Med. , 2012, 18 , 361–363 CrossRef CAS PubMed .
- M. Griesser, V. Pistis, T. Suzuki, N. Tejera, D. A. Pratt and C. Schneider, J. Biol. Chem. , 2011, 286 , 1114–1124 CrossRef CAS PubMed .
- N. Sreejayan, M. N. A. Rao, K. I. Priyadarsini and T. P. A. Devasagayam, Int. J. Pharmacol. , 1997, 151 , 127–130 CrossRef CAS .
- A. Barik, B. Mishra, L. Shen, H. Mohan, R. M. Kadam, S. Dutta, H. Zhang and K. I. Priyadarsini, Free Radical Biol. Med. , 2005, 39 , 811–822 CrossRef CAS PubMed .
- B. Stinefelt, S. S. Leonard, K. P. Blemings, X. Shi and H. Klandorf, Ann. Clin. Lab. Sci. , 2005, 35 , 37–45 CAS .
- A. Galano and J. R. Alvarez-Idaboy, RSC Adv. , 2011, 1 , 1763–1771 RSC .
- W. S. Warning, QJM: An International Journal of Medicine , 2002, 95 , 691–693 CrossRef .
- S. Muraoka and T. Miura, Pharmacol. Toxicol. , 2003, 93 , 284–289 CAS .
- G. L. Squadrito, R. Cueto, A. E. Splenser, A. Valavanidis, H. Zhang, R. M. Uppu and W. A. Pryor, Arch. Biochem. Biophys. , 2000, 376 , 333–337 CrossRef CAS PubMed .
- N. Kuzkaya, N. Weissmann, D. G. Harrison and S. Dikalov, Biochem. Pharmacol. , 2005, 70 , 343–354 CrossRef CAS PubMed .
- P. Ahmadpoor, E. Eftekhar, J. Nourooz-Zadeh, H. Servat, K. Makhdoomi and A. Ghafari, Iran J. Kidney Dis. , 2009, 3 , 22–27 Search PubMed .
- R. B. Pereira, C. Sousa, A. Costa, P. B. Andrade and P. Valentão, Molecules , 2013, 18 , 8858–8872 CrossRef CAS PubMed .
- A. Pastore, G. Federici, E. Bertini and F. Piemonte, Clin. Chim. Acta , 2003, 333 , 19–39 CrossRef CAS .
- P. C. Huber and W. P. Almeida, Quim. Nova , 2008, 31 , 1170–1179 CrossRef CAS PubMed .
- M. A. Karaman, N. M. Mimica-Dukić and M. N. Matavulj, Arch. Biol. Sci. , 2005, 57 , 93–100 CrossRef .
- R. B. Jalgaonwala, B. V. Mohite and R. T. Mahajan, J. Microbiol. Biotechnol. Res. , 2011, 1 , 21–32 Search PubMed .
- N. Sadrati, H. Daoud, A. Zerroug, S. Dahamna and S. Bouharati, J. Plant Prot. Res. , 2013, 53 , 128–136 Search PubMed .
- F. Vélez-González, D. Ortegón-Reyna, A. A. Ramos-Organillo, A. L. Peraza-Campos, M. T. Sumaya-Martínez, R. Tapia-Benavides and F. J. Martínez-Martíneza, ARKIVOC , 2008, V , 55–64 Search PubMed .
- S. Lee, J. Han, H. Kim, E. Kim, T. Jeong, W. S. Lee and K. Cho, Bioorg. Med. Chem. Lett. , 2004, 14 , 4677–4681 CrossRef CAS PubMed .
- F. Natella, M. Nardini, M. D. Felice and C. Scaccini, J. Agric. Food Chem. , 1999, 47 , 1453–1459 CrossRef CAS PubMed .
- D. Point, P. Coudert, F. Leal, C. Rubat, V. Sautou-Miranda, J. Chopineau and J. Couquelet, Il Farmaco , 1998, 53 , 85–88 CrossRef CAS .
- D. Krishnaiah, R. Sarbatly and R. Nithyanandam, Food Bioprod. Process. , 2011, 89 , 217–233 CrossRef CAS PubMed .
- D. Pal and S. Mitra, J. Adv. Pharm. Technol. Res. , 2010, 1 , 268–272 Search PubMed .
- M. N. Alam, N. J. Bristi and M. Rafiquzzaman, Saudi Pharm. J. , 2013, 21 , 143–152 CrossRef PubMed .
- M. Carocho and I. C. F. R. Ferreira, Food Chem. Toxicol. , 2013, 51 , 15–25 CrossRef CAS PubMed .
- J. A. Imlay, Nat. Rev. Microbiol. , 2013, 11 , 443–454 CrossRef CAS PubMed .
- A. Meyer-Isaksen, Trends Food Sci. Technol. , 1995, 6 , 300–304 CrossRef .
- J. Robak and R. J. Gryglewski, Flavonoids are scavengers of superoxide anions, Biochem. Pharmacol. , 1988, 37 , 837–841 CrossRef CAS .
- C. W. Choi, S. C. Kim, S. S. Hwang, B. K. Choi, H. J. Ahn, M. Y. Lee, S. H. Park and S. K. Kim, Plant Sci. , 2002, 163 , 1161–1168 CrossRef CAS .
- C. Sánchez-Moreno, Food Sci. Technol. Int. , 2002, 8 , 121–137 CrossRef PubMed .
- C. A. Calliste, P. Trouillas, D. P. Allais, A. Simon and J. L. Duroux, J. Agric. Food Chem. , 2001, 49 , 3321–3327 CrossRef CAS PubMed .
- C. A. Calliste, D. Kozlowski, J. L. Duroux, Y. Champavier, A. J. Chulia and P. Trouillas, Food Chem. , 2010, 118 , 489–496 CrossRef CAS PubMed .
- H. Chang, Y. Ho, M. Sheu, Y. Lin, M. Tseng, S. Wu, G. Huang and Y. Chang, Bot. Stud. , 2007, 48 , 407–417 CAS .
- P. Evelson, M. Travacio, M. Repetto, J. Escobar, S. Llesuy and E. A. Lissi, Arch. Biochem. Biophys. , 2001, 388 , 261–266 CrossRef CAS PubMed .
- H. Leontowicz, S. Gorinstein, A. Lojek, M. Leontowicz, M. Ciz, R. Soliva-Fortuny, Y. Park and S. Jung, J. Nutr. Biochem. , 2002, 13 , 603–610 CrossRef CAS .
- M. G. Repetto and S. F. Llesuy, Braz. J. Med. Biol. Res. , 2002, 35 , 523–534 CAS .
- P. Miciński, K. Pawlicki, E. Wielgus, M. Bochenek, P. Gogol and B. Ficek, Reprod. Biol. , 2011, 11 , 135–144 CrossRef .
- R. He, Y. Li, X. Li, R. Yi, X. Wang, B. Tsoi, K. K. H. Lee, K. Abe, X. Yang and H. Kurihara, PLoS One , 2013, 8 , e57732 CAS .
- F. S. Hosseinian, A. D. Muir, N. D. Westcott and E. S. Krol, Org. Biomol. Chem. , 2007, 5 , 644–654 CAS .
- S. Kohtani, K. Yoshida, T. Maekawa, A. Iwase, A. Kudo, H. Miyabe and R. Nakagaki, Phys. Chem. Chem. Phys. , 2008, 10 , 2986–2992 RSC .
- H. Esterbauer, J. H. Gebicki and G. Jürgens, Free Radical Biol. Med. , 1992, 13 , 341–390 CrossRef CAS .
- E. Niki, Y. Yoshida, Y. Saito and N. Noguchi, Biochem. Biophys. Res. Commun. , 2005, 338 , 668–676 CrossRef CAS PubMed .
- T. A. Dix and J. Aikens, Chem. Res. Toxicol. , 1993, 6 , 2–18 CrossRef CAS .
- V. Mišík, K. Ondriaš and P. Balgavý, Gen. Physiol. Biophys. , 1992, 11 , 317–325 Search PubMed .
- K. Marshall, R. J. Reiter, B. Poeggeler, O. I. Aruoma and B. Halliwell, Free Radical Biol. Med. , 1996, 21 , 307–315 CrossRef CAS .
- O. I. Aruoma, in Free radicals in tropical diseases , Harwood Academic Publishers, 1993 Search PubMed .
- O. I. Aruoma, Free Radical Biol. Med. , 1996, 20 , 675–705 CrossRef CAS .
- C. L. Alarcón and A. Denicola, Anal. Chim. Acta , 2013, 763 , 1–10 CrossRef PubMed .
- K. L. Wolfe and R. H. Liu, J. Agric. Food Chem. , 2007, 55 , 8896–8907 CrossRef CAS PubMed .
- D. Honzel, S. G. Carter, K. A. Redman, A. G. Schauss, J. R. Endres and G. S. Jensen, J. Agric. Food Chem. , 2008, 56 , 8319–8325 CrossRef CAS PubMed .
- J. A. Royall and H. Ischiropoulos, Arch. Biochem. Biophys. , 1993, 302 , 348–355 CrossRef CAS PubMed .
- M. Antolovich, P. D. Prenzler, E. Patsalides, S. McDonald and K. Robards, Analyst , 2002, 127 , 183–198 RSC .
- R. Apak, S. Gorinstein, V. Böhm, K. M. Schaich, M. Özyürek and K. Güçlü, Pure Appl. Chem. , 2013, 85 , 957–998 CrossRef CAS .
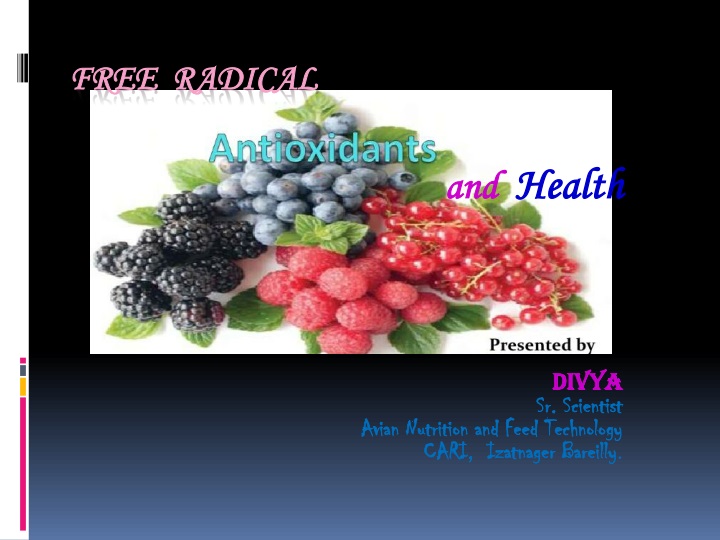
Free radical
Jul 03, 2014
1.41k likes | 3.82k Views
and Health. Divya Sr. Scientist Avian Nutrition and Feed Technology CARI, Izatnager Bareilly. Free radical. Structure of Atom. An atom consists of an small, positively charged nucleus surrounded by a cloud of negatively charged electrons.
Share Presentation
- natural antioxidants level maximum
- tbars value
- nitrogenoxide
- molecular weight antioxidants e
- repair mechanisms
- tissue damage
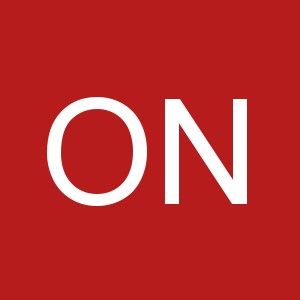
Presentation Transcript
and Health Divya Sr. Scientist Avian Nutrition and Feed Technology CARI, Izatnager Bareilly. Free radical
Structure of Atom • An atom consists of an small, positively charged nucleus surrounded by a cloud of negatively charged electrons. • Electrons in every orbital must be paired and these paired electrons spin in the opposite direction. • It is called stable form of an atom Stable atoms contain an even number of paired electrons.
Free radical - what itis? • particles withunpaired electron spinning around the nucleus. (can be atom, ions, molecule). • Any atom or molecule capable of independent existence (hence the term ‘free’) that contains one or more unpaired electrons • Free radicals are highly reactive and can cause damage to molecules in the cell
Formation of Free Radical • Free radical are ionized particle in human body • Free radicals produced in NORMAL cellular metabolism are vital to certain body functions, such as fighting disease or injury. When tissue is diseased or damaged, the body’s immune system sends disease fighting cells to the site, where they produce free radicals in an effort to destroy foreign invaders.
Free radicals are also produced by • Pollution • Ultraviolet light and radiation • Toxic substances • cigarette smoke, overexposure to sunlight
3. H 2 O 2 Hydrogen peroxide 1 O 2 Singlet oxygen NO • Types of Free radicals • There are two major types of free radical species: • reactive oxygen species (ROS) • reactive nitrogen species (RNS).
Free radicals superoxide, O2 · - hydroxyl radical, OH · peroxyl, ROO · alkoxyl, RO · hydroperoxyl, HO2 · Particals, which are not free radicals hydrogen peroxide, H2O2 hypochlorous acid, HClO ozone, O3 singlet oxygen, 1O2 ROS (reactive oxygen species)
Free radicals nitrogen oxide, NO . Nitrogenoxide, NO2. Particals, which are not free radicals nitrosyl, NO+ nitrous acid, HONO nitogen(III) oxide, N2O3 peroxynitrite, ONOO - alkylperoxinitrite, ROONO RNS (reactive nitrogen species)
Mechanism of radical reactions Radicals are highly reactive species Three distinc steps • initiation (homolytic covalent bonds cleavage) formation of radicals • propagation (chain propagation) in this step required free radical is regenerated repeatedly as a result of chain reaction • termination
Free Radical Oxidation Stable antioxidant radical breaks the cycle (unstable peroxyl radical) R* + O2 ROO* ROO* + RH ROOH + R* RO* + RH ROH + R* Aldehydes, organic acids, alcohols, ketones (hydroperoxide breakdown products contribute off flavors)
Free radicals damage • Steal electrons from cells, DNA, enzymes and cell membranes. • Damage to cell structures, nucleic acids, lipids and proteins. Lipids in cell membranes are very prone to oxidative damage because some free radicals tend to concentrate in the membrane and cause oxidative damage, known as lipid peroxidation
Free radical and diseases Number of human degenerative diseases. • Oxidative stress is a harmful condition that occurs when there is an excess of ROS and/or a decrease in antioxidant levels, this may caused tissue damage by physical, chemical, psychological factors that lead to tissue injury in human and causes different diseases Free radical and ageing Aging pigments (lipofusin granules) are accumulated in the subsarcolmal region of the muscle fibers and becomes more abundant with increasing age. • Other diseases such as atherosclerosis, Parkinson's disease and Alzheimer's are also attributed to free radicals.
Need of antioxidant • Cell produce defense against excessive free radicals by their preventative mechanisms, repair mechanisms, physical defenses and antioxidant defenses. • Antioxidants prevent cell and tissue damage as they act as Scavenger. • Food plants including fruits, vegetables and spices are the primary sources of naturally occurring antioxidants for humans
Antioxidant Antioxidant means "against oxidation." Antioxidants are an inhibitor of the process of oxidation, even at relatively small concentration and thus have diverse physiological role in the body . Antioxidants are any substance that delay or inhibits oxidative damage to a target molecule. At a time one antioxidant molecule can react with single free radicals and are capable to neutralize free radicals by donating one of their own electrons, ending the carbon-stealing reaction.
CLASSIFICATION Non-Enzymatic antioxidants Enzymatic antioxidants 1. Minerals e.g.-Zinc, Selenium 2. Vitamins e.g.-VitaminA, Vitamin C, Vitamin E, Vitamin F 3. Carotenoidse.g.--carotene, Lycopene, Lutein, Zeaxanthin 4. Low molecular weight Antioxidants e.g.-glutathione, uric acid 5. Organosulfur compounds e.g-Allium, Allylsulfide,Indoles 6. Polyphenols Glutathione reductase, Glucose 6-phosphate dehydrogenase. • superoxide dismutase
Types of natural antioxidant Photochemical Stilbenes/ Lignans Phenolic Acids Flavonoids Anthocyanins Flavones,Flavanones Isoflavones Flavanols Flavonols Epicatechins Catechins
sources of Natural antioxidant Polyphenolic compounds Carotenoids Vitamin-A Vitamin-C Vitamin E . Organosulfur compounds
Mechanism of action of antioxidants • Two principle mechanisms- chain- breaking mechanism by which the primary antioxidant donates an electron to the free radical present in the systems--as beta-carotene and vitamins C and E, or it simply decays into a harmless product. • Preventive - Antioxidant enzymes like superoxide dismutase, catalase and glutathione peroxidase prevent oxidation by reducing the rate of chain initiation. They can also prevent oxidation by stabilizing transition metal radicals such as copper and iron.
Three major levels of antioxidant defense in the cell Free radicals First level of defense Free radicals Second level of defense Third level of defense Se-GSH-Px Glutathione Uric acid Lipases, proteases, etc Vitamins A, E, C, carotenoids Metal-binding proteins , Free radicals Catalase Free radicals
Levels of antioxidant defense in the cell • The first line of defense is the preventive antioxidants, which suppress the formation of free radicals. • The second line of defense is the antioxidants that scavenge the active radicals to suppress chain initiation and/or break the chain propagation reactions • The third line of defense is the repair and de novo antioxidants. The proteolytic enzymes, proteinases, proteases, and peptidases, present in the cytosol and in the mitochondria of mammalian cells
Function • Destroying the free radicals that damage cells. • Promoting the growth of healthy cells. • Protecting cells against premature, abnormal ageing. • Help fight age-related macular degeneration. • Provide excellent support for the body’s immune system. • prevent the oxidation of unsaturated fats
Antioxidant defense system • Antioxidants act as radical scavenger, hydrogen donor, electron donor, peroxide decomposer, singlet oxygen quencher, enzyme inhibitor, metal-chelating agents.
Antioxidants in Human health • Considerable research demonstrates the human health benefits of naturally occurring antioxidant compounds. • Claims of Various properties • anti-viral, • anti-inflammatory, • anti-cancer, • anti-mutagenic, • anti-tumour, • hepatoprotective
Natural extracts with antioxidant properties The rate of lipid oxidation can be effectively retarded by the use of antioxidants. TBARS value of chicken nuggets
………….Natural extracts with antioxidant properties • The antimicrobial properties of essential oils extracted from plants used against a wide range of microorganisms have been considered as an alternative to antibiotics in livestock. Therefore, essential oils are promising as feed additives to improve feed efficiency and control the spread of pathogens in livestock • The human intake of green tea decreases total cholesterol, increases the high-density lipoprotein (HDL) fraction, and decreases lipoprotein oxidation.
Antioxidants in Poultry health • Hatching timeis an environmental stress for the chick. At this time stress caused by environment is very height. • Though natural antioxidants level maximum at this stage but in the cycle process enhance lipid unsaturation in tissues. • Low level concentrations of ascorbic acid (can limit vitamin E recycling) and high temperature and humidity increase risk of lipid peroxidation
……..Antioxidants in Poultry health • Effect on egg production • In the production system time between the egg laid and cooling is variable. Whatever effort may be made some changes are bound to happen in composition of egg due to high temperature and time gap. • Free radical is responsible accelerating these compositional change and damage to lipids and proteins. Addition of antioxidant in feed may retard such changes ultimately improve egg production.
……Antioxidants in Poultry health Transport of chicks: • Transportation from hatchery to farmis another source of stress. For breeding companies where chicken transportation could involve several thousand miles, a very high degree of stress would be associated with temperature fluctuation and dehydration. • Oxidation of fat in feed: Oxidized fat in the dietcan cause oxidative stress in the intestine increasing antioxidant nutrient requirements. When a chicken diet includes spent fat after its high temperature treatment, the fat usually contains peroxides and hydroperoxides that can contribute substantially to oxidative stress. It is necessary to evaluate benefits vs disadvantages of using such fat sources.
……..Antioxidants in Animal health • Vaccination stress: Vaccinationis also a substantial stress; and in some cases using vitamin E, for example, as a vaccine adjuvant can help improve vaccination efficiency. • Exposure to Mycotoxin: Mycotoxinscan substantially decrease antioxidant assimilation from the feed and increase their requirement to prevent damaging effects of free radicals produced as a result of mycotoxin exposure.
Applications of some antioxidant in poultry production • Increased antioxidant supplementation for improvement of meat quality during storage. • It is recorded that vitamin E and selenium combination is very effective to reduce drip loss. • Decline in egg production due to heat related stress is increased due to less Vitamin E in feed. • Increased antioxidant supplementation decreased mycotoxin toxicity.
continue-- • Poultry has become a very competitive business and any addition on coast or change in feed ingredient will affect the cost of production and quality of the products. • While adding antioxidant in the feed one has to be careful about coast, age, feed and food laws of the country. • Certainly there are reports available for beneficial effect of antioxidants improving the immunity system, health management and quality of the poultry products but all of them cannot be introduced in poultry feed. • Most precise study has been undertaken on available antioxidants and their scope of application in poultry feed.
continue-- • On the other hand, under conditions of oxidative stress where free radical production dramatic increases, then without external help it is difficult to prevent damage to major organs and systems. This external help takes the form of increased dietary supplementation of natural antioxidants. For the poultry business it need to understand at the time of feed formulator when and how much fed additive is needed and economically justified.
- More by User
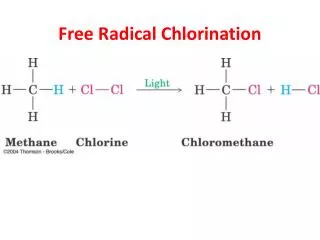
Free Radical Chlorination
Free Radical Chlorination. Experimental Evidence Helps to Determine Mechanism. Chlorination does not occur at room temperature in the dark . The most effective wavelength of light is blue that is strongly absorbed by Cl 2 gas.
1.08k views • 15 slides
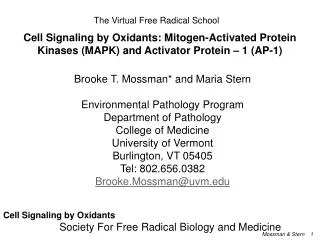
The Virtual Free Radical School
The Virtual Free Radical School. Cell Signaling by Oxidants: Mitogen-Activated Protein Kinases (MAPK) and Activator Protein – 1 (AP-1). Brooke T. Mossman* and Maria Stern Environmental Pathology Program Department of Pathology College of Medicine University of Vermont Burlington, VT 05405
429 views • 10 slides
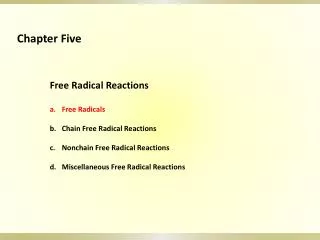
Free Radical Reactions Free Radicals Chain Free Radical Reactions Nonchain Free Radical Reactions Miscellaneous Free R
Chapter Five. Free Radical Reactions Free Radicals Chain Free Radical Reactions Nonchain Free Radical Reactions Miscellaneous Free Radical Reactions. Free Radicals. Discovery of free radicals Prof. Gomberg was the first to characterize a free radical in 1900.
1.44k views • 32 slides
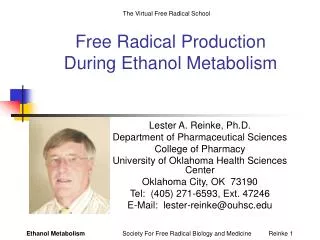
Free Radical Production During Ethanol Metabolism
The Virtual Free Radical School. Free Radical Production During Ethanol Metabolism. Lester A. Reinke, Ph.D. Department of Pharmaceutical Sciences College of Pharmacy University of Oklahoma Health Sciences Center Oklahoma City, OK 73190 Tel: (405) 271-6593, Ext. 47246
698 views • 17 slides
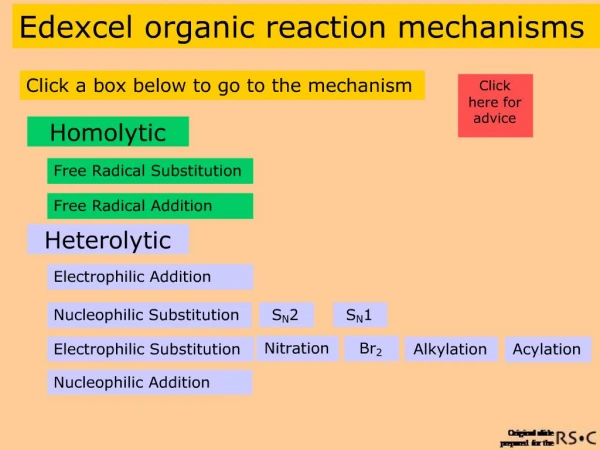
Free Radical Substitution
725 views • 17 slides
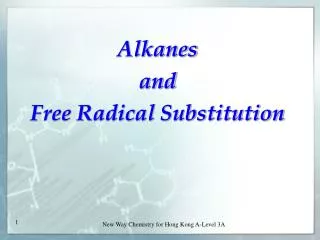
Alkanes and Free Radical Substitution
Alkanes and Free Radical Substitution. Preparation of Alkanes. Crude Oil as a Source of Alkanes. Petroleum Refining. The first step is fractional distillation separate petroleum into fractions based on the volatility of its components performed in a petroleum refinery
1.39k views • 98 slides
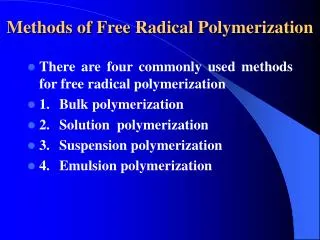
Methods of Free Radical Polymerization
Methods of Free Radical Polymerization. There are four commonly used methods for free radical polymerization 1. Bulk polymerization 2. Solution polymerization 3. Suspension polymerization 4. Emulsion polymerization. BULK POLYMERIZATION.
1.19k views • 10 slides
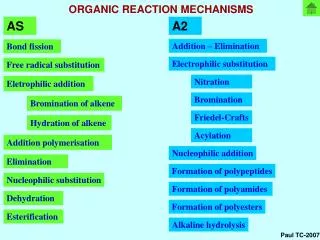
Free radical substitution
ORGANIC REACTION MECHANISMS. AS. A2. Addition – Elimination. Bond fission. Electrophilic substitution. Free radical substitution. Nitration. Eletrophilic addition. Bromination. Bromination of alkene. Friedel-Crafts. Hydration of alkene. Acylation. Addition polymerisation.
499 views • 20 slides
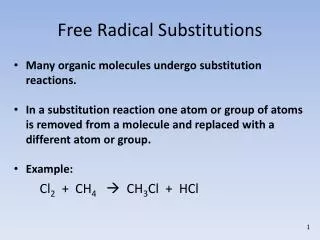
Free Radical Substitutions
Free Radical Substitutions. Many organic molecules undergo substitution reactions . In a substitution reaction one atom or group of atoms is removed from a molecule and replaced with a different atom or group . Example: Cl 2 + CH 4 CH 3 Cl + HCl. 1.
966 views • 9 slides
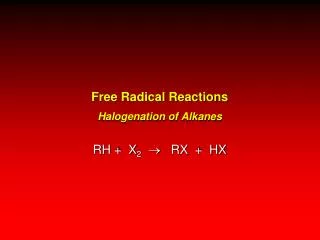
Free Radical Reactions Halogenation of Alkanes
Free Radical Reactions Halogenation of Alkanes. RH + X 2 RX + HX. Reactive Intermediates. O rbital hybridization in carbocations and carbanions :. Reactive Intermediates: Free Radicals.
3.18k views • 62 slides
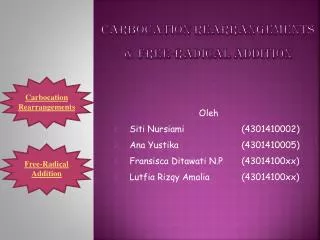
CARBOCATION REARRANGEMENTS & FREE-RADICAL ADDITION
CARBOCATION REARRANGEMENTS & FREE-RADICAL ADDITION . Carbocation Rearrangements. Oleh Siti Nursiami (4301410002) Ana Yustika (4301410005) Fransisca Ditawati N.P (43014100xx) Lutfia Rizqy Amalia (43014100xx). Free-Radical Addition.
717 views • 15 slides
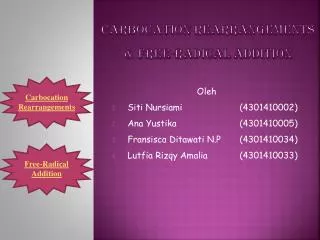
CARBOCATION REARRANGEMENTS & FREE-RADICAL ADDITION . Carbocation Rearrangements. Oleh Siti Nursiami (4301410002) Ana Yustika (4301410005) Fransisca Ditawati N.P (43014100 34 ) Lutfia Rizqy Amalia (43014100 33 ). Free-Radical Addition.
347 views • 10 slides
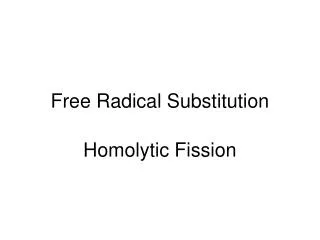
Free Radical Substitution. Homolytic Fission. Substitution Rxn (free radical substitution). Is a chemical reaction in which an atom or group of atoms in a molecule is replaced by another atom or group of atoms. Mechanism of reaction.
2.36k views • 75 slides
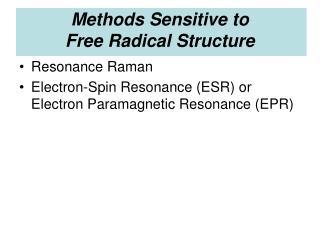
Methods Sensitive to Free Radical Structure
Methods Sensitive to Free Radical Structure. Resonance Raman Electron-Spin Resonance (ESR) or Electron Paramagnetic Resonance (EPR). Motivation. Absorption spectra of free radical and excited states are generally broad and featureless Conductivity is not species specific
433 views • 25 slides
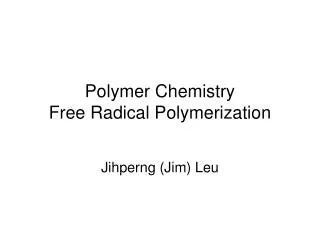
Polymer Chemistry Free Radical Polymerization
Polymer Chemistry Free Radical Polymerization. Jihperng (Jim) Leu. Chain Polymerization. Free-radical polymerization What is free-radical Each addition reproduces the reactive group. most widely practised method of polymerization Three-Stage Initiation Propagation Termination.
4.02k views • 22 slides
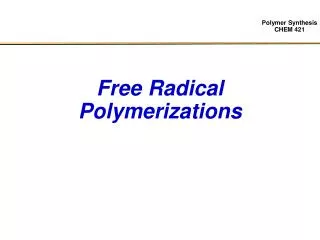
Free Radical Polymerizations
Free Radical Polymerizations. Reading assignments: Chapter 3-3, 3-4, 3-5, 3-8 (Odian). Free Radical Solution Polymerizations. Initiation Propagation Termination. d [ R • ] / dt = 2 k d [ I ]. = 2 f k d [ I ]. - d [ R • ] / dt = k i [R•] [M]. r p = k p [ M •] [M].
703 views • 25 slides
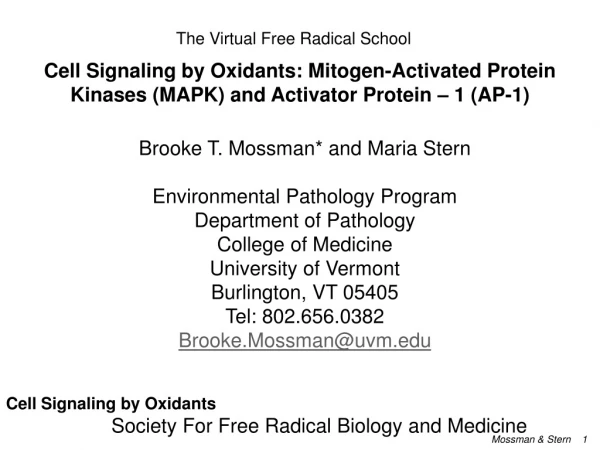
122 views • 10 slides
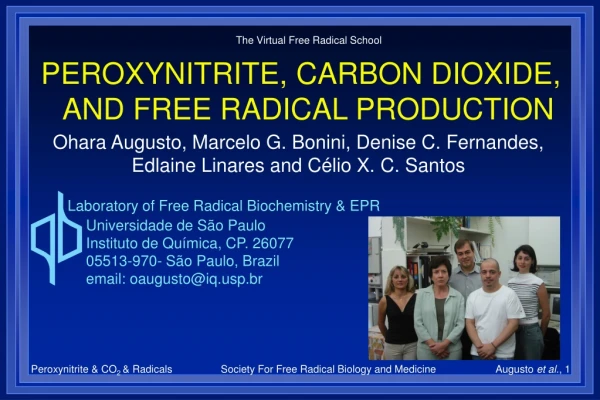
The Virtual Free Radical School. PEROXYNITRITE, CARBON DIOXIDE, AND FREE RADICAL PRODUCTION. Ohara Augusto, Marcelo G. Bonini, Denise C. Fernandes, Edlaine Linares and Célio X. C. Santos. Laboratory of Free Radical Biochemistry & EPR. Universidade de São Paulo
201 views • 19 slides
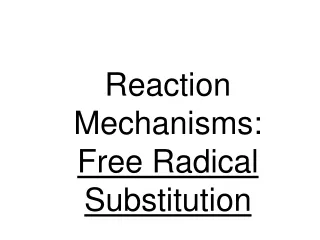
Reaction Mechanisms: Free Radical Substitution
Reaction Mechanisms: Free Radical Substitution. Monochlorination of methane. CH 4 + Cl 2 → CH 3 Cl + HCl methane chloromethane. Initiation. Ultraviolet light causes splitting of chlorine molecules into chlorine atoms Cl - Cl → Cl ● + Cl ●
166 views • 11 slides
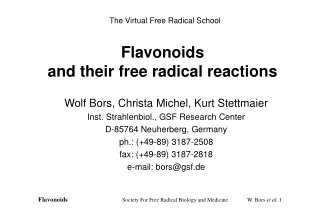
Flavonoids and their free radical reactions
The Virtual Free Radical School. Flavonoids and their free radical reactions. Wolf Bors, Christa Michel, Kurt Stettmaier Inst. Strahlenbiol., GSF Research Center D-85764 Neuherberg, Germany ph.: (+49-89) 3187-2508 fax: (+49-89) 3187-2818 e-mail: [email protected].
180 views • 15 slides
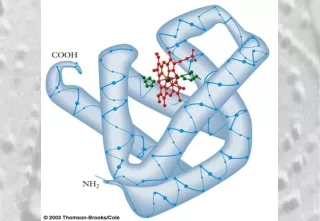
Introduction to free-radical polymerization
Introduction to free-radical polymerization. Polymerization mechanisms. - Step-growth polymerization. Polymerization mechanisms. - Chain-growth polymerization. Advantages of free-radical polymerization. very robust technique wide range of vinyl monomers and functionalities
223 views • 11 slides
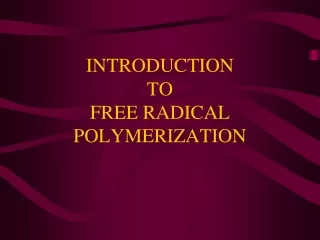
INTRODUCTION TO FREE RADICAL POLYMERIZATION
INTRODUCTION TO FREE RADICAL POLYMERIZATION. Polymerization mechanisms. - Step-growth polymerization. Polymerization mechanisms. - Chain-growth polymerization. Advantages of free-radical polymerization. very robust technique wide range of vinyl monomers and functionalities
450 views • 16 slides
The best free cultural &
educational media on the web
- Online Courses
- Certificates
- Degrees & Mini-Degrees
- Audio Books
The Radical Artistic & Philosophical World of William Blake: A Short Introduction
in Art , History | June 4th, 2024 Leave a Comment
Over the years, we’ve featured the work of William Blake fairly often here on Open Culture: his own illuminated books ; his illustrations for everything from the Divine Comedy to Mary Wollstonecraft’s Original Stories from Real Life to the Book of Job ; pairs of Doc Martens made out of his paintings Satan Smiting Job with Sore Boils and The House of Death . Blake continues to capture our imaginations, despite having lived in the very different world of the mid-eighteenth century to the mid-nineteenth — but then, he also lived in a world well apart from his contemporaries.
“Blake belonged to the Romantic age, but stands utterly alone in that age, both as an artist and as a poet,” says gallerist-Youtuber James Payne in his new Great Art Explained video above . “He is someone who invented his very own form of graphic art, which organically fused beautiful images with powerful poetry, while he also forged his own distinctive philosophical worldview and created an original cosmology of gods and spirits designed to express his ideas about love, freedom, nature, and the divine.” It wouldn’t be an exaggeration to call him a visionary, not least since he experienced actual visions throughout almost his entire life.
Not just a visual artist but “one of the greatest poets in the English language,” Blake produced a body of work in which word and image are inseparable. Though it “addresses contemporary subjects like social inequality and poverty, child exploitation, racial discrimination, and religious hypocrisy,” its worldliness is exceeded by its otherworldliness. What compels us is as much the power of art itself as the “vast and complicated mythology” underlying the project on which Blake worked until the very end of his life. His ideal was “liberty from tyranny in all forms,” political, religious, scientific, and any other kind besides; in pursuing it, he could hardly have limited himself to just one plane of existence.
Related content:
The Otherworldly Art of William Blake: An Introduction to the Visionary Poet and Painter
William Blake: The Remarkable Printing Process of the English Poet, Artist & Visionary
Enter an Archive of William Blake’s Fantastical “Illuminated Books”: The Images Are Sublime, and in High Resolution
William Blake’s Hallucinatory Illustrations of John Milton’s Paradise Lost
Great Art Explained: Watch 15 Minute Introductions to Great Works by Warhol, Rothko, Kahlo, Picasso & More
Based in Seoul, Colin M a rshall writes and broadcas ts on cities, language, and culture. His projects include the Substack newsletter Books on Cities and the book The Stateless City: a Walk through 21st-Century Los Angeles. Follow him on Twitter at @colinm a rshall or on Facebook .
by Colin Marshall | Permalink | Comments (0) |
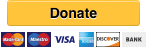
Related posts:
Comments (0).
Be the first to comment.
Add a comment
Leave a reply.
Name (required)
Email (required)
XHTML: You can use these tags: <a href="" title=""> <abbr title=""> <acronym title=""> <b> <blockquote cite=""> <cite> <code> <del datetime=""> <em> <i> <q cite=""> <s> <strike> <strong>
Click here to cancel reply.
- 1,700 Free Online Courses
- 200 Online Certificate Programs
- 100+ Online Degree & Mini-Degree Programs
- 1,150 Free Movies
- 1,000 Free Audio Books
- 150+ Best Podcasts
- 800 Free eBooks
- 200 Free Textbooks
- 300 Free Language Lessons
- 150 Free Business Courses
- Free K-12 Education
- Get Our Daily Email
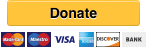
Free Courses
- Art & Art History
- Classics/Ancient World
- Computer Science
- Data Science
- Engineering
- Environment
- Political Science
- Writing & Journalism
- All 1500 Free Courses
- 1000+ MOOCs & Certificate Courses
Receive our Daily Email
Free updates, get our daily email.
Get the best cultural and educational resources on the web curated for you in a daily email. We never spam. Unsubscribe at any time.
FOLLOW ON SOCIAL MEDIA
Free Movies
- 1150 Free Movies Online
- Free Film Noir
- Silent Films
- Documentaries
- Martial Arts/Kung Fu
- Free Hitchcock Films
- Free Charlie Chaplin
- Free John Wayne Movies
- Free Tarkovsky Films
- Free Dziga Vertov
- Free Oscar Winners
- Free Language Lessons
- All Languages
Free eBooks
- 700 Free eBooks
- Free Philosophy eBooks
- The Harvard Classics
- Philip K. Dick Stories
- Neil Gaiman Stories
- David Foster Wallace Stories & Essays
- Hemingway Stories
- Great Gatsby & Other Fitzgerald Novels
- HP Lovecraft
- Edgar Allan Poe
- Free Alice Munro Stories
- Jennifer Egan Stories
- George Saunders Stories
- Hunter S. Thompson Essays
- Joan Didion Essays
- Gabriel Garcia Marquez Stories
- David Sedaris Stories
- Stephen King
- Golden Age Comics
- Free Books by UC Press
- Life Changing Books
Free Audio Books
- 700 Free Audio Books
- Free Audio Books: Fiction
- Free Audio Books: Poetry
- Free Audio Books: Non-Fiction
Free Textbooks
- Free Physics Textbooks
- Free Computer Science Textbooks
- Free Math Textbooks
K-12 Resources
- Free Video Lessons
- Web Resources by Subject
- Quality YouTube Channels
- Teacher Resources
- All Free Kids Resources
Free Art & Images
- All Art Images & Books
- The Rijksmuseum
- Smithsonian
- The Guggenheim
- The National Gallery
- The Whitney
- LA County Museum
- Stanford University
- British Library
- Google Art Project
- French Revolution
- Getty Images
- Guggenheim Art Books
- Met Art Books
- Getty Art Books
- New York Public Library Maps
- Museum of New Zealand
- Smarthistory
- Coloring Books
- All Bach Organ Works
- All of Bach
- 80,000 Classical Music Scores
- Free Classical Music
- Live Classical Music
- 9,000 Grateful Dead Concerts
- Alan Lomax Blues & Folk Archive
Writing Tips
- William Zinsser
- Kurt Vonnegut
- Toni Morrison
- Margaret Atwood
- David Ogilvy
- Billy Wilder
- All posts by date
Personal Finance
- Open Personal Finance
- Amazon Kindle
- Architecture
- Artificial Intelligence
- Beat & Tweets
- Comics/Cartoons
- Current Affairs
- English Language
- Entrepreneurship
- Food & Drink
- Graduation Speech
- How to Learn for Free
- Internet Archive
- Language Lessons
- Most Popular
- Neuroscience
- Photography
- Pretty Much Pop
- Productivity
- UC Berkeley
- Uncategorized
- Video - Arts & Culture
- Video - Politics/Society
- Video - Science
- Video Games
Great Lectures
- Michel Foucault
- Sun Ra at UC Berkeley
- Richard Feynman
- Joseph Campbell
- Jorge Luis Borges
- Leonard Bernstein
- Richard Dawkins
- Buckminster Fuller
- Walter Kaufmann on Existentialism
- Jacques Lacan
- Roland Barthes
- Nobel Lectures by Writers
- Bertrand Russell
- Oxford Philosophy Lectures
Receive our newsletter!
Open Culture scours the web for the best educational media. We find the free courses and audio books you need, the language lessons & educational videos you want, and plenty of enlightenment in between.
Great Recordings
- T.S. Eliot Reads Waste Land
- Sylvia Plath - Ariel
- Joyce Reads Ulysses
- Joyce - Finnegans Wake
- Patti Smith Reads Virginia Woolf
- Albert Einstein
- Charles Bukowski
- Bill Murray
- Fitzgerald Reads Shakespeare
- William Faulkner
- Flannery O'Connor
- Tolkien - The Hobbit
- Allen Ginsberg - Howl
- Dylan Thomas
- Anne Sexton
- John Cheever
- David Foster Wallace
Book Lists By
- Neil deGrasse Tyson
- Ernest Hemingway
- F. Scott Fitzgerald
- Allen Ginsberg
- Patti Smith
- Henry Miller
- Christopher Hitchens
- Joseph Brodsky
- Donald Barthelme
- David Bowie
- Samuel Beckett
- Art Garfunkel
- Marilyn Monroe
- Picks by Female Creatives
- Zadie Smith & Gary Shteyngart
- Lynda Barry
Favorite Movies
- Kurosawa's 100
- David Lynch
- Werner Herzog
- Woody Allen
- Wes Anderson
- Luis Buñuel
- Roger Ebert
- Susan Sontag
- Scorsese Foreign Films
- Philosophy Films
- February 2024
- January 2024
- December 2023
- November 2023
- October 2023
- September 2023
- August 2023
- February 2023
- January 2023
- December 2022
- November 2022
- October 2022
- September 2022
- August 2022
- February 2022
- January 2022
- December 2021
- November 2021
- October 2021
- September 2021
- August 2021
- February 2021
- January 2021
- December 2020
- November 2020
- October 2020
- September 2020
- August 2020
- February 2020
- January 2020
- December 2019
- November 2019
- October 2019
- September 2019
- August 2019
- February 2019
- January 2019
- December 2018
- November 2018
- October 2018
- September 2018
- August 2018
- February 2018
- January 2018
- December 2017
- November 2017
- October 2017
- September 2017
- August 2017
- February 2017
- January 2017
- December 2016
- November 2016
- October 2016
- September 2016
- August 2016
- February 2016
- January 2016
- December 2015
- November 2015
- October 2015
- September 2015
- August 2015
- February 2015
- January 2015
- December 2014
- November 2014
- October 2014
- September 2014
- August 2014
- February 2014
- January 2014
- December 2013
- November 2013
- October 2013
- September 2013
- August 2013
- February 2013
- January 2013
- December 2012
- November 2012
- October 2012
- September 2012
- August 2012
- February 2012
- January 2012
- December 2011
- November 2011
- October 2011
- September 2011
- August 2011
- February 2011
- January 2011
- December 2010
- November 2010
- October 2010
- September 2010
- August 2010
- February 2010
- January 2010
- December 2009
- November 2009
- October 2009
- September 2009
- August 2009
- February 2009
- January 2009
- December 2008
- November 2008
- October 2008
- September 2008
- August 2008
- February 2008
- January 2008
- December 2007
- November 2007
- October 2007
- September 2007
- August 2007
- February 2007
- January 2007
- December 2006
- November 2006
- October 2006
- September 2006
©2006-2024 Open Culture, LLC. All rights reserved.
- Advertise with Us
- Copyright Policy
- Privacy Policy
- Terms of Use

- Share full article
Advertisement
Supported by
Lawyers to Plastics Makers: Prepare for ‘Astronomical’ PFAS Lawsuits
At an industry presentation about dangerous “forever chemicals,” lawyers predicted a wave of lawsuits that could dwarf asbestos litigation, audio from the event revealed.

By Hiroko Tabuchi
The defense lawyer minced no words as he addressed a room full of plastic-industry executives. Prepare for a wave of lawsuits with potentially “astronomical” costs. Speaking at a conference earlier this year, the lawyer, Brian Gross, said the coming litigation could “dwarf anything related to asbestos,” one of the most sprawling corporate-liability battles in United States history.
Mr. Gross was referring to PFAS, the “forever chemicals” that have emerged as one of the major pollution issues of our time. Used for decades in countless everyday objects — cosmetics, takeout containers, frying pans — PFAS have been linked to serious health risks including cancer. Last month the federal government said several types of PFAS must be removed from the drinking water of hundreds of millions of Americans .
“Do what you can, while you can, before you get sued,” Mr. Gross said at the February session, according to a recording of the event made by a participant and examined by The New York Times. “Review any marketing materials or other communications that you’ve had with your customers, with your suppliers, see whether there’s anything in those documents that’s problematic to your defense,” he said. “Weed out people and find the right witness to represent your company.”
A spokesman for Mr. Gross’s employer, MG+M The Law Firm, which defends companies in high-stakes litigation, didn’t respond to questions about Mr. Gross’s remarks and said he was unavailable to discuss them.
A wide swath of the chemicals, plastics and related industries are gearing up to fight a surge in litigation related to PFAS, or per- and polyfluoroalkyl substances, a class of nearly 15,000 versatile synthetic chemicals linked to serious health problems.
PFAS chemicals have been detected almost everywhere scientists have looked: in drinking water, in rain falling over the Great Lakes , even in Antarctic snow . They are thought to be present in the blood of nearly every American . Researchers have linked exposure to PFAS to testicular and kidney cancers, developmental delays in children, decreased fertility, liver damage and thyroid disease. The man-made chemicals are so long-lasting that scientists haven’t been able to reliably identify how long it might take for them to break down.
PFAS-related lawsuits have already targeted manufacturers in the United States, including DuPont, its spinoff Chemours, and 3M. Last year, 3M agreed to pay at least $10 billion to water utilities across the United States that had sought compensation for cleanup costs. Thirty state attorneys general have also sued PFAS manufacturers, accusing the manufacturers of widespread contamination.
But experts say the legal battle is just beginning. Under increasing scrutiny are a wider universe of companies that use PFAS in their products. This month, plaintiffs filed a class-action lawsuit against Bic, accusing the razor company for failing to disclose that some of its razors contained PFAS.
Bic said it doesn’t comment on pending litigation, and said it had a longstanding commitment to safety.
The Biden administration has moved to regulate the chemicals, for the first time requiring municipal water systems to remove six types of PFAS . Last month, the Environmental Protection Agency also designated two of those PFAS chemicals as hazardous substances under the Superfund law, shifting responsibility for their cleanup at contaminated sites from taxpayers to polluters.
Both rules are expected to prompt a new round of litigation from water utilities, local communities and others suing for cleanup costs.
“To say that the floodgates are opening is an understatement,” said Emily M. Lamond, an attorney who focuses on environmental litigation at the law firm Cole Schotz. “Take tobacco, asbestos, MTBE, combine them, and I think we’re still going to see more PFAS-related litigation,” she said, referring to methyl tert-butyl ether, a former harmful gasoline additive that contaminated drinking water. Together, the trio led to claims totaling hundreds of billions of dollars.
PFAS were an industrial marvel when chemists at Dupont in the 1940s synthesized the material, a remarkably durable compound resistant to water, stains, heat and grease. It quickly became a mainstay in DuPont’s Teflon nonstick pans and 3M’s Scotchgard fabric protector. A powerful fire suppressant, it helped firefighters battle flames. Today, they are used for everyday items as varied as microwave popcorn bags, shampoos, raincoats and firefighting foam.
But the very qualities that have made PFAS so valuable have also prevented them from breaking down naturally in the environment. As PFAS entered the environment from factories, products and landfills, the chemicals have started to accumulate in water, air and soil.
Industry documents released through litigation show that manufacturers found adverse health effects from PFAS exposure as early as 1961 . But it wasn’t until the early 2000s that questions increasingly emerged in public about their safety. In 2005, the E.P.A. fined DuPont $10 million , at that time the largest administrative fine ever levied by the agency, for failing to disclose PFAS’s adverse effects.
All that has set the stage for a potential legal storm. Unlike tobacco, used by only a subset of the public, “pretty much every one of us in the United States is walking around with PFAS in our bodies,” said Erik Olson, senior strategic director for environmental health at the Natural Resources Defense Council. “And we’re being exposed without our knowledge or consent, often by industries that knew how dangerous the chemicals were, and failed to disclose that,” he said. “That’s a formula for really significant liability.”
Sandy Wynn-Stelt of Belmont, Mich., brought one early case. A year after she lost her husband to liver cancer in 2016, she discovered that the Christmas tree farm in front of her home, which had seemed such an idyllic setting, had been a dumping ground for PFAS-laden tannery waste from Wolverine World Wide, the maker of Hush Puppies shoes.
Wolverine had been among the first to license 3M’s Scotchgard for its waterproof footwear. Mrs. Wynn-Stelt got her blood tested, and found PFAS levels hundreds of times the norm. In 2020, she was diagnosed with thyroid cancer.
She sued Wolverine and 3M, and reached a settlement in 2021. Separately, nearly 2,000 local residents settled a class-action lawsuit against Wolverine. The region’s water source remains polluted with PFAS.
“Those lawyers are exactly right. This is going to be huge, now that people are starting to hold companies accountable,” Mrs. Wynn-Stelt said.
Wolverine declined to comment. 3M said it continued to “address PFAS litigation by defending itself in court or through negotiated resolutions.”
Much of the course of future litigation hinges on the evidence over PFAS’s health risks. There is broad scientific consensus that certain PFAS chemicals are harmful. “There’s a weight of evidence,” said Linda Birnbaum, a toxicologist and the former director of the National Institute of Environmental Health Sciences. “Multiple studies by different investigators, and in different populations.”
Max Swetman, another MG+M partner who presented with Mr. Gross at the February industry conference, addressed the research in his remarks to the group. “There’s a whole lot of new science being created,” he said. “It’s not the best for us.”
Still, some of the research could be vulnerable to criticism, he said. Getting the right experts to testify was crucial, he said. “Epidemiologists, if you get the right one, is always going to be your best expert in trial.”
Mr. Swetman was unavailable to comment on his remarks, according to his law firm.
One challenge facing medical research lies in the sheer number of different PFAS chemicals that have now entered the environment, each of which can have slightly different health effects, said Steph Tai, associate dean at the University of Wisconsin’s Nelson Institute for Environmental Studies and an expert in the use of science in environmental protection and litigation.
“The other thing, too, is that it takes a long time for health effects to show up,” Dr. Tai said, so the only way that scientists have been able to assess those effects is through long-term studies. Researchers must essentially look for what is referred to as “natural experiments,” according to Dr. Tai, comparing people who are naturally less exposed to PFAS with those who are more exposed. That inevitably leads to some uncertainties.
The industry has scored some major victories. Last November, the United States Court of Appeals for the Sixth Circuit tossed out a lawsuit that would have covered every Ohio citizen in a major case over exposure to PFAS, ruling that a firefighter who brought the lawsuit failed to prove that the PFAS found in his blood specifically came from the companies he sued.
3M phased out most uses of two of the most widely used PFAS chemicals, PFOS and PFOA, in the early 2000s, and DuPont in 2015 stopped using PFOA. 3M has said it will phase out the manufacturing of PFAS chemicals by the end of next year. The company will also work to discontinue their use in products, though that’s contingent on the company finding substitutes.
“As the science and technology of PFAS, societal and regulatory expectations, and our expectations of ourselves have evolved, so has how we manage PFAS,” 3M said.
DuPont referred inquiries to Chemours, the company that was spun off in 2015. Chemours declined to comment.
A long and difficult cleanup is beginning. President Biden’s 2021 infrastructure law provides $9 billion to help communities address PFAS contamination, and the E.P.A. has said $1 billion of that money would be set aside to help states with initial testing and treatment. Meantime, new kinds of PFAS are still being released into the environment. Scientists are working to learn more about them.
Hiroko Tabuchi covers the intersection of business and climate for The Times. She has been a journalist for more than 20 years in Tokyo and New York. More about Hiroko Tabuchi
The Proliferation of ‘Forever Chemicals’
Pfas, or per- and polyfluoroalkyl substances, are hazardous compounds that pose a global threat to human health..
For the first time, the U.S. government is requiring municipal water systems to detect and remove PFAS from drinking water .
A global study found harmful levels of PFAS in water samples taken far from any obvious source of contamination.
Virtually indestructible, PFAS are used in fast-food packaging and countless household items .
PFAS lurk in much of what we eat, drink and use, but scientists are only beginning to understand how they affect our health .
Though no one can avoid forever chemicals entirely, Wirecutter offers tips on how to limit your exposure .
Scientists have spent years searching for ways to destroy forever chemicals. In 2022, a team of chemists found a cheap, effective method to break them down .

Press Release Details
Nvidia nim revolutionizes model deployment, now available to transform world’s millions of developers into generative ai developers.
- 150+ Partners Across Every Layer of AI Ecosystem Embedding NIM Inference Microservices to Speed Enterprise AI Application Deployments From Weeks to Minutes
- NVIDIA Developer Program Members Gain Free Access to NIM for Research, Development and Testing
TAIPEI, Taiwan, June 02, 2024 (GLOBE NEWSWIRE) -- COMPUTEX -- NVIDIA today announced that the world’s 28 million developers can now download NVIDIA NIM ™ — inference microservices that provide models as optimized containers — to deploy on clouds, data centers or workstations, giving them the ability to easily build generative AI applications for copilots, chatbots and more, in minutes rather than weeks.
These new generative AI applications are becoming increasingly complex and often utilize multiple models with different capabilities for generating text, images, video, speech and more. NVIDIA NIM dramatically increases developer productivity by providing a simple, standardized way to add generative AI to their applications.
NIM also enables enterprises to maximize their infrastructure investments. For example, running Meta Llama 3-8B in a NIM produces up to 3x more generative AI tokens on accelerated infrastructure than without NIM. This lets enterprises boost efficiency and use the same amount of compute infrastructure to generate more responses.
Nearly 200 technology partners — including Cadence, Cloudera , Cohesity , DataStax , NetApp , Scale AI and Synopsys — are integrating NIM into their platforms to speed generative AI deployments for domain-specific applications, such as copilots, code assistants and digital human avatars. Hugging Face is now offering NIM — starting with Meta Llama 3 .
“Every enterprise is looking to add generative AI to its operations, but not every enterprise has a dedicated team of AI researchers,” said Jensen Huang, founder and CEO of NVIDIA. “Integrated into platforms everywhere, accessible to developers everywhere, running everywhere — NVIDIA NIM is helping the technology industry put generative AI in reach for every organization.”
Enterprises can deploy AI applications in production with NIM through the NVIDIA AI Enterprise software platform. Starting next month, members of the NVIDIA Developer Program can access NIM for free for research, development and testing on their preferred infrastructure.
40+ NIM Microservices Power Gen AI Models Across Modalities NIM containers are pre-built to speed model deployment for GPU-accelerated inference and can include NVIDIA CUDA ® software, NVIDIA Triton Inference Server ™ and NVIDIA TensorRT™-LLM software.
Over 40 NVIDIA and community models are available to experience as NIM endpoints on ai.nvidia.com , including Databricks DBRX , Google’s open model Gemma, Meta Llama 3, Microsoft Phi-3, Mistral Large, Mixtral 8x22B and Snowflake Arctic.
Developers can now access NVIDIA NIM microservices for Meta Llama 3 models from the Hugging Face AI platform. This lets developers easily access and run the Llama 3 NIM in just a few clicks using Hugging Face Inference Endpoints, powered by NVIDIA GPUs on their preferred cloud.
Enterprises can use NIM to run applications for generating text, images and video, speech and digital humans. With NVIDIA BioNeMo ™ NIM microservices for digital biology, researchers can build novel protein structures to accelerate drug discovery.
Dozens of healthcare companies are deploying NIM to power generative AI inference across a range of applications, including surgical planning, digital assistants, drug discovery and clinical trial optimization.
With new NVIDIA ACE NIM microservices , developers can easily build and operate interactive, lifelike digital humans in applications for customer service, telehealth, education, gaming and entertainment.
Hundreds of AI Ecosyst em Partners Embedding NIM Platform providers including Canonical , Red Hat , Nutanix and VMware (acquired by Broadcom) are supporting NIM on open-source KServe or enterprise solutions. AI application companies Hippocratic AI , Glean , Kinetica and Redis are also deploying NIM to power generative AI inference.
Leading AI tools and MLOps partners — including Amazon SageMaker, Microsoft Azure AI, Dataiku, DataRobot, deepset , Domino Data Lab, LangChain , Llama Index , Replicate , Run.ai, Saturn Cloud , Securiti AI and Weights & Biases — have also embedded NIM into their platforms to enable developers to build and deploy domain-specific generative AI applications with optimized inference.
Global system integrators and service delivery partners Accenture, Deloitte, Infosys, Latentview , Quantiphi , SoftServe, TCS and Wipro have created NIM competencies to help the world’s enterprises quickly develop and deploy production AI strategies.
Enterprises can run NIM-enabled applications virtually anywhere, including on NVIDIA-Certified Systems ™ from global infrastructure manufacturers Cisco, Dell Technologies , Hewlett-Packard Enterprise , Lenovo and Supermicro, as well as server manufacturers ASRock Rack , ASUS , GIGABYTE , Ingrasys , Inventec , Pegatron , QCT, Wistron and Wiwynn. NIM microservices have also been integrated into Amazon Web Services , Google Cloud , Azure and Oracle Cloud Infrastructure .
Titans of Industry Amp Up Gener ative AI With NIM Industry leaders Foxconn, Pegatron, Amdocs , Lowe’s, ServiceNow and Siemens are among the businesses using NIM for generative AI applications in manufacturing, healthcare, financial services, retail, customer service and more:
- Foxconn — the world’s largest electronics manufacturer — is using NIM in the development of domain-specific LLMs embedded into a variety of internal systems and processes in its AI factories for smart manufacturing, smart cities and smart electric vehicles.
- Pegatron — a Taiwanese electronics manufacturing company — is leveraging NIM for Project TaME, a Taiwan Mixtral of Experts model designed to advance the development of local LLMs for industries.
- Amdocs — a leading global provider of software and services to communications and media companies — is using NIM to run a customer billing LLM that significantly lowers the cost of tokens, improves accuracy by up to 30% and reduces latency by 80%, driving near real-time responses.
- Lowe’s — a FORTUNE ® 50 home improvement company — is using generative AI for a variety of use cases. For example, the retailer is leveraging NVIDIA NIM inference microservices to elevate experiences for associates and customers.
- ServiceNow — the AI platform for business transformation — announced earlier this year that it was one of the first platform providers to access NIM to enable fast, scalable and more cost-effective LLM development and deployment for its customers. NIM microservices are integrated within the Now AI multimodal model and are available to customers that have ServiceNow’s generative AI experience, Now Assist, installed.
- Siemens — a global technology company focused on industry, infrastructure, transport and healthcare — is integrating its operational technology with NIM microservices for shop floor AI workloads. It is also building an on-premises version of its Industrial Copilot for Machine Operators using NIM.
Availability Developers can experiment with NVIDIA microservices at ai.nvidia.com at no charge. Enterprises can deploy production-grade NIM microservices with NVIDIA AI Enterprise running on NVIDIA-Certified Systems and leading cloud platforms. Starting next month, members of the NVIDIA Developer Program will gain free access to NIM for research and testing.
Watch Huang’s COMPUTEX keynote to learn more about NVIDIA NIM.
About NVIDIA NVIDIA (NASDAQ: NVDA) is the world leader in accelerated computing.
For further information, contact: Anna Kiachian NVIDIA Corporation +1-650-224-9820 [email protected]
Certain statements in this press release including, but not limited to, statements as to: the benefits, impact, performance, features, and availability of NVIDIA’s products and technologies, including NVIDIA NIM, NVIDIA CUDA, NVIDIA Triton Inference Server, NVIDIA TensorRT-LLM software, NVIDIA Developer program, NVIDIA BioNeMo, NVIDIA-Certified Systems, and NVIDIA AI Enterprise; our collaborations and partnerships with third parties and the benefits and impacts thereof; third parties using or adopting our products or technologies; every enterprise looking to add generative AI to its operations; and NVIDIA NIM helping the technology industry put generative AI in reach for every organization are forward-looking statements that are subject to risks and uncertainties that could cause results to be materially different than expectations. Important factors that could cause actual results to differ materially include: global economic conditions; our reliance on third parties to manufacture, assemble, package and test our products; the impact of technological development and competition; development of new products and technologies or enhancements to our existing product and technologies; market acceptance of our products or our partners' products; design, manufacturing or software defects; changes in consumer preferences or demands; changes in industry standards and interfaces; unexpected loss of performance of our products or technologies when integrated into systems; as well as other factors detailed from time to time in the most recent reports NVIDIA files with the Securities and Exchange Commission, or SEC, including, but not limited to, its annual report on Form 10-K and quarterly reports on Form 10-Q. Copies of reports filed with the SEC are posted on the company's website and are available from NVIDIA without charge. These forward-looking statements are not guarantees of future performance and speak only as of the date hereof, and, except as required by law, NVIDIA disclaims any obligation to update these forward-looking statements to reflect future events or circumstances.
© 2024 NVIDIA Corporation. All rights reserved. NVIDIA, the NVIDIA logo, BioNeMo, CUDA, NVIDIA NIM, NVIDIA Triton Inference Server and TensorRT are trademarks and/or registered trademarks of NVIDIA Corporation in the U.S. and other countries. Other company and product names may be trademarks of the respective companies with which they are associated. Features, pricing, availability and specifications are subject to change without notice.
A photo accompanying this announcement is available at: https://www.globenewswire.com/NewsRoom/AttachmentNg/4fe99b19-66e7-4473-b9ff-f1380eae0ff8
NVIDIA NIM revolutionizes model deployment for every layer of the AI ecosystem.
Quick links.
- Email Alerts
- Request Printed Materials
- Download Library
To receive notifications via email, enter your email address and select at least one subscription below. After submitting your information, you will receive an email. You must click the link in the email to activate your subscription. You can sign up for additional subscriptions at any time.
Email Alert Sign Up Confirmation
Investor contact.
2788 San Tomas Expressway Santa Clara, CA 95051
- Contact Investor Relations
Investor Resources
- Request Information
- Stock Quote & Chart
- Historical Price Lookup
- Investment Calculator
- Fundamentals
- Analyst Coverage
- Management Team
- Board of Directors
- Governance Documents
- Committee Composition
- Contact the Board
- Corporate Sustainability
- Events & Presentations
Financial Info
- Financial Reports
- SEC Filings
- Quarterly Results
- Annual Reports and Proxies
Investors and others should note that we announce material financial information to our investors using our investor relations website, press releases, SEC filings and public conference calls and webcasts. We intend to use our @NVIDIA Twitter account, NVIDIA Facebook page, NVIDIA LinkedIn page and company blog as a means of disclosing information about our company, our services and other matters and for complying with our disclosure obligations under Regulation FD. The information we post through these social media channels may be deemed material. Accordingly, investors should monitor these accounts and the blog, in addition to following our press releases, SEC filings and public conference calls and webcasts. This list may be updated from time to time.

- Privacy Policy
- Manage My Privacy
- Do Not Sell or Share My Data
- Terms of Service
- Accessibility
- Corporate Policies
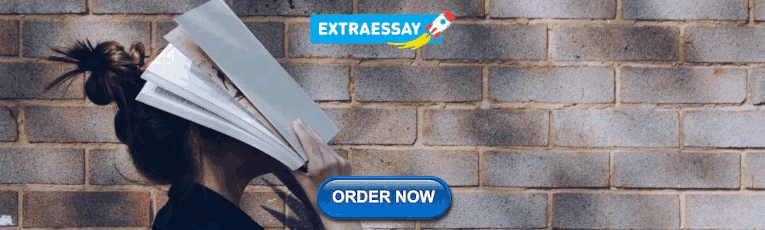
IMAGES
VIDEO
COMMENTS
Free radicals play an important role in combustion, atmospheric chemistry, polymerization, plasma chemistry, biochemistry, and many other chemical processes. In living organisms, the free radicals superoxide and nitric oxide and their reaction products regulate many processes, such as control of vascular tone and thus blood pressure.
Examples of Free Radicals. Consider three reactive species: a methyl anion, methyl cation and methyl radical. These radicals are shown below. Ethane is composed of two methyl groups connected by a covalent bond and is a very stable compound. The methyl anion and methyl cation have an ionic bond mainly between carbons and counter ions ...
Free radicals are atoms, molecules, or ions with unpaired electrons that make them highly reactive. They are formed through processes like homolysis and oxidation-reduction reactions. Free radical stability is determined by factors like conjugation, hybridization, and hyperconjugation which disperse and stabilize the unpaired electron.
Figure 8.1.1 8.1. 1: Superoxide: A molecule with one unpaired electron, which makes it a free radical. ( CC BY-SA 3.0 ; by DoSiDo via Wikimedia Commons) Figure 8.1.2 8.1. 2: Normal oxygen is converted to an oxygen free radical by losing one electron in its outer orbital, leaving one unpaired electron.
Free radicals and other oxidants have gained importance in the field of biology due to their central role in various physiological conditions as well as their implication in a diverse range of diseases. The free radicals, both the reactive oxygen species (ROS) and reactive nitrogen species (RNS), are derived from both endogenous sources ...
Free radicals are highly reactive molecules that are produced through normal cell metabolism and environmental exposures. They can damage cells by reacting with lipids, proteins, and DNA if produced in excess. The body has antioxidant defenses like enzymes and nutrients that neutralize free radicals. However, oxidative stress occurs when there ...
Free radicals thus adversely alter lipids, proteins, and DNA and trigger a number of human diseases. Free radicals are a main cause of cardiovascular diseases, cancer, aging and immune defense disorders. Foods like berries and carrot protect us against free radicals. 1.
Matthew Belliveau. 11 years ago. Free radical halogenation is one of the main ways to produce chloromethane, dichloromethane, trichloromethane, and tetrachloromathane. They have different boiling points and are therefore separated into different fractions. These chemicals are important in many industrial and commercial uses.
Presentation on theme: "Free radicals and Antioxidants"— Presentation transcript: ... 5 Free Radical: A free radical is an atom capable of independent existence (hence the term "free") that contains one or more unpaired electrons in its outer orbital. It is an electron-deficient species. ...
Free radicals may be defined as the species that contain one or more unpaired electrons. They are generally less stable and react in fraction of seconds with another species. 9.1.1 Formation of Free Radicals Three general methods are used for the generation of free radicals. 9.1.1.1 Thermal Generation Two types of compounds dissociate to give ...
Free radicals and other oxidants have gained importance in the field of biology due to their central role in various physiological conditions as well as their implication in a diverse range of diseases. The free radicals, both the reactive oxygen species (ROS) and reactive nitrogen species (RNS), are derived from both endogenous sources (mitochondria, peroxisomes, endoplasmic reticulum ...
Free radicals create oxidative stress. If you don't have enough antioxidants to keep free radicals in check, they go rogue and steal electrons from wherever they can get them. When you have too ...
Free radicals are unstable molecules in the body that can damage DNA in cells. In turn, this can increase your risk for disease, including cancer. The body naturally makes some free radicals as a byproduct of the processes it normally does, but you can also get more free radicals by exposure to certain toxic substances.
Free Radicals. Page ID. In chemistry, a radical (more precisely, a free radical) is an atom, molecule, or ion that has unpaired valence electrons or an open electron shell, and therefore may be seen as having one or more "dangling" covalent bonds. With some exceptions, these "dangling" bonds make free radicals highly chemically reactive towards ...
DNA oxidative damage 8-hydroxyguanine Free radicals can react with structural units of DNA and can damage purine and pyrimidine bases and deoxyribose as well. Reactive nitrogen species can cause deamination and nitration of purine bases. 8-hydroxyguanine is produced after additionof OH• to C8 of guanine.
Free radicals - Download as a PDF or view online for free. Free radicals - Download as a PDF or view online for free ... This presentation was provided by William Mattingly of the Smithsonian Institution, during the closing segment of the NISO training series "AI & Prompt Design." Session Eight: Limitations and Potential Solutions, was held on ...
1. Introduction and background Antioxidants are molecules that inhibit or quench free radical reactions and delay or inhibit cellular damage. 1 Though the antioxidant defenses are different from species to species, the presence of the antioxidant defense is universal. Antioxidants exists both in enzymatic and non-enzymatic forms in the intracellular and extracellular environment.
Free radicals have a habit of stealing electrons from any molecule they come across to make themselves stable and it's what causes all the trouble and potentially can cause cellular injury. Now, a free radical is formed when any molecule gains or loses an electron. In the body, free radicals can be generated physiologically, which means as a ...
Harmful effects of free radicals A. Free Radical and biomolecules 3. Carbohydrates • Glycation increases the susceptibility of proteins to the attack by free radicals. 4. Nucleic acid • cause DNA strand breaks, fragmentation of bases and deoxyribose results in cytotoxicity and mutations.
Presentation Transcript. and Health Divya Sr. Scientist Avian Nutrition and Feed Technology CARI, Izatnager Bareilly. Free radical. Structure of Atom • An atom consists of an small, positively charged nucleus surrounded by a cloud of negatively charged electrons. • Electrons in every orbital must be paired and these paired electrons spin in ...
Over the past decade, the merger of copper catalysis with free-radical chemistry emerged as a general approach to bypass the challenge of copper(I) oxidative addition. Specifically, it was demonstrated that copper(III) intermediates can be accessed by adding free radicals onto copper(II) species. Regarding amination chemistry, this merger of ...
Alcohols are among the most abundant chemical feedstocks, yet they remain vastly underutilized as coupling partners in transition metal catalysis. Herein, we describe a copper metallaphotoredox manifold for the open shell deoxygenative coupling of alcohols with N-nucleophiles to forge C(sp3)-N bonds, a linkage of high value in pharmaceutical agents that is challenging to access via ...
These include 1. Oxygen in its triplet state (3O2) or singlet state (O2), hydroxyl radical (OH), nitric oxide (NO), hypochlorous acid (HOCl), hydrogen peroxide (H2O2) and the superoxide radical (O2_). 2. Others are carbon-centered free radicals that arise from the attack of an oxidizing radical on an organic molecule. 3.
Great Art Explained: Watch 15 Minute Introductions to Great Works by Warhol, Rothko, Kahlo, Picasso & More. Based in Seoul, Colin M a rshall writes and broadcasts on cities, language, and culture. His projects include the Substack newsletter Books on Cities and the book The Stateless City: a Walk through 21st-Century Los ...
At an industry presentation about dangerous "forever chemicals," lawyers predicted a wave of lawsuits that could dwarf asbestos litigation, audio from the event revealed.
2. 2 Pharmacology of Free Radicals Free Radicals A free radical is defined as any chemical species that contains unpaired electron (s) in its outer orbit. Because of these unpaired electrons, free radicals are highly reactive and readily take part in chemical reactions with virtually all cell components (lipids, proteins, complex carbohydrates and nucleic acids) in the body.
150+ Partners Across Every Layer of AI Ecosystem Embedding NIM Inference Microservices to Speed Enterprise AI Application Deployments From Weeks to Minutes NVIDIA Developer Program Members Gain Free Access to NIM for Research, Development and Testing TAIPEI, Taiwan, June 02, 2024 (GLOBE NEWSWIRE) - COMPUTEX - NVIDIA today announced that the world's 28 million developers can now download ...
You need to enable JavaScript to run this app.
5. EXAMPLES OF FREE RADICALS EXAMPLE S OF FREE RADICALS : Superoxide anion Singlet oxygen Hydrogen peroxyl radical Hydroxyl radical Hydrogen peroxide Nitric Oxide PeroxyNitrite Lipid peroxide radical. Hypochlorous acid Out of these H2O2 and Singlet oxygen are not free radicals but due to extreme reactivity they are included in Reactive oxygen ...