
An official website of the United States government
The .gov means it’s official. Federal government websites often end in .gov or .mil. Before sharing sensitive information, make sure you’re on a federal government site.
The site is secure. The https:// ensures that you are connecting to the official website and that any information you provide is encrypted and transmitted securely.
- Publications
- Account settings
Preview improvements coming to the PMC website in October 2024. Learn More or Try it out now .
- Advanced Search
- Journal List
- Plants (Basel)

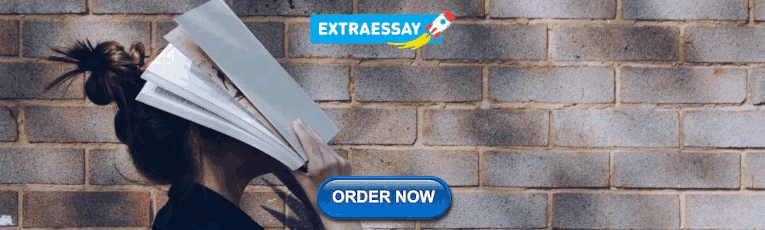
Integrated Nutrient Management Enhances Productivity and Nitrogen Use Efficiency of Crops in Acidic and Charland Soils
Mohammad mojibur rahman.
1 Department of Soil Science, Bangladesh Agricultural University, Mymensingh 2202, Bangladesh; moc.liamg@irabrubijom (M.M.R.); db.ude.uab@15134bahihs (S.U.); db.ude.uab@rignahajrmm (M.M.R.J.)
2 Bangladesh Agricultural Research Institute, Gazipur 1701, Bangladesh
Shihab Uddin
Mohammad mofizur rahman jahangir, zakaria m. solaiman.
3 UWA School of Agriculture and Environment, The UWA Institute of Agriculture, The University of Western Australia, Perth, WA 6009, Australia; [email protected]
Saud Alamri
4 Department of Botany and Microbiology, College of Science, King Saud University, Riyadh 2455, Saudi Arabia; as.ude.usk@irmalauas (S.A.); as.ude.usk@iuqiddishm (M.H.S.)
Manzer H. Siddiqui
Mohammad rafiqul islam, associated data.
The raw data supporting the conclusions of this article will be made available by the authors, without undue reservation.
Integrated Plant Nutrient System (IPNS) is practiced worldwide to maintain soil quality. Two field experiments were conducted in 2019 and 2020 in acidic and charland soils to assess the impact of different manures, viz., poultry manure (PM), vermicompost (VC), compost (OF), rice husk biochar (RHB), poultry manure biochar (PMB)-based IPNS, and dolomite over control on productivity and nitrogen use efficiency (NUE) of the Mustard-Boro-Transplanted Aman and Maize-Jute-Transplanted Aman cropping patterns, and on soil properties. The experiments were laid out in a randomized complete block design with four replications. The results showed that IPNS treatments significantly improved soil aggregate properties and total nitrogen in acidic soil, and bulk density in charland soil. In both years, IPNS treatments increased system productivity from 55.4 to 82.8% in acidic soil and from 43.3 to 115.4% in charland soil over that of control. IPNS and dolomite treatments increased nitrogen uptake from 35.5 to 105.7% over that of control and NUE in both soils in 2019 and 2020. PMB- and OF-based INPS treatments exhibited superior performances in both soils, and the impact was more prominent in 2020. Therefore, PMB- and OF-based IPNS can be recommended for maximizing system productivity and NUE with concurrent improvement of physicochemical properties of acidic and charland soils.
1. Introduction
The increasing growth rate of the world’s population will necessitate a significant increase in agricultural production to meet the demand by 2025 [ 1 ], which will increase land degradation due to intensive agricultural use and indiscriminate fertilizer application. Soil physical, chemical, and biological properties like organic carbon, hydraulic conductivity, BD, runoff and erosion, nutrient availability, microbial biomass, and enzyme activities are severely affected by land degradation [ 2 , 3 , 4 ]. Acidic and charland soils are degraded soils in Bangladesh with low organic matter and nutrient status, threatening crop production of about 36% of land [ 5 , 6 ], as well as 57% of the world’s land coverage [ 7 ]. Soil acidity affects plant growth by influencing soil microbes, nutrient leaching, and nutrient and toxic element availability. Charland soil is mainly a major concern in the Bengal Delta of India and Bangladesh for crop production because of its sandy type soil texture, low water holding capacity, and low nutrient status. Thus, these two soils need to be ameliorated to maintain soil health and quality for sustainable crops and increased crop production to ensure food security for the country.
Farmers were using organic manures such as poultry manure (PM), compost (OF), cowdung (CD), and others for their beneficial effects on soil health by improving soil physicochemical properties and by increasing macro- and micronutrient availability since ancient times [ 8 , 9 ]. Biochar, a carbon-rich compound, resulting from the pyrolysis process of different biomasses acts as an alternative or complementary organic amendment [ 10 , 11 ]. Positive effects of biochar application on soil physical, chemical, and biological properties and on crop yield were reported earlier [ 12 , 13 , 14 ], although no or adverse effects on soil properties and crop yield in sandy loam and Fort Collins loam soils having a pH of 6.15 and 8.70 were also reported [ 15 , 16 ]. The incorporation of biochar derived from rice husk into soils could significantly improve soil physicochemical properties, such as soil moisture content, water holding capacity, BD, available-N nutrients, etc., in paddy fields, [ 17 ] and thereby increase crop yield [ 18 , 19 ]. Biochar application decreased soil BD, whereas increased porosity, available soil water content, organic carbon (OC), soil pH, available P, cation exchange capacity (CEC), exchangeable K, and Ca were observed [ 20 ]. Biochar produced from animal origins may have higher nutrient content, but its agronomic value as a soil conditioner was not investigated [ 19 ]. Several authors reported that vermicompost (VC) amendment acts as a slow-release fertilizer and can directly increase crop production through increased availability of plant nutrients. It indirectly promotes soil quality by improving soil structure and stimulating microbial activity relative to conventional chemical fertilization [ 21 , 22 , 23 ]. Application of dolomite in combination with PM and chemical fertilizer increases crop productivity, N uptake, and NUE, as well as improves the physicochemical properties of acidic soil [ 7 , 24 , 25 ].
The Integrated Plant Nutrient System (IPNS) is a combination of organic and inorganic fertilizers that can be used to reduce the use of chemical fertilizers by establishing a balance between fertilizer inputs and crop nutrient requirements, thereby maintaining soil fertility, restoring soil health, and providing plants with nutrient requirements continuously [ 26 , 27 , 28 ]. Bilkis et al. [ 29 ] reported that PM and VC based INPS approaches increased yield and nutrient uptake of the Boro-Fallow-T. Aman cropping pattern as well as soil fertility. Saha et al. [ 30 ] observed that the addition of NPK fertilizers and organic manure, lime, and biofertilizers increased soil organic carbon (SOC) content, aggregate stability, moisture-retention capacity, and infiltration rate of the soil while reducing BD. Chaudhry et al. [ 31 ] reported that biochar and 50% of recommended dose of NPK was most effective for improving soil physico-chemical properties viz., BD, particle density, porosity, pH, EC, organic matter, SOC, total N, available P, K, soil microbial biomass C, and soil microbial biomass N at 0–30 cm depth. The efficiency of nutrients can be increased through the integrated use of organic manures and chemical fertilizers [ 32 ]. The slow and gradual release of N from organic manure is an advantage over sole chemical fertilization for achieving higher NUE, grain yield, and quality of rice [ 33 , 34 ].
Previous research in the acidic and charland soils primarily focused on the fertility assessment and the impact of combined application of manures (primarily cowdung and poultry manure) and chemical fertilizers, but not as an IPNS approach or in conjugation with conservation agriculture in other soil types [ 35 , 36 , 37 ]. The present study introduced biochar based IPNS as a means of soil nutrient management for diversified crops in these study areas for the first time. However, the present research knowledge on the impact of different IPNS approaches, especially biochar-based IPNS approaches, on crop yields, total system productivity, N uptake and use efficiency, and soil physicochemical properties under different cropping patterns is mostly limited to acidic and charland soils. We hypothesize that applying organic amendments, especially biochar, and chemical fertilizer as a means of IPNS will improve crop productivity, N uptake and use efficiency, and soil physicochemical properties. Thus, the research was conducted to (1) evaluate the effects of different IPNS approaches on soil aggregate properties; (2) determine the impacts of different IPNS approaches on soil physicochemical properties; and (3) measure the effects of different IPNS approaches on crop yields, system productivity, N uptake, and NUE.
2. Materials and Methods
2.1. description of the experimental site and initial soil properties.
In two farmers’ fields of Madhupur, Tangail (24°59.82′ N, 90°03.99′ E) and Islampur, Jamalpur (25°80.73′ N, 89°81.90′ E), the study concentrated on acid soils in Madhupur, Tangail (24°59.82′ N, 90°03.99′ E) and charland soils in Islampur, Jamalpur (25°80.73′ N, 89°81.90′ E). The climate in the region is subtropical monsoon, with an average annual temperature of 26 °C, rainfall of 1,800 mm and an average relative humidity of 85% (local weather station). The general soil type of acid soil in Madhupur, Tangail with a deep red to brown terrace soil [ 38 ] is located in agro-ecological zone 28 (AEZ-28; Madhupur Tract), while charland soil in Islampur, Jamalpur with a noncalcareous dark grey floodplain soil [ 38 ] is located in agro-ecological zone 9 (AEZ-9; Old Brahmaputra Floodplain). The soil texture of the Madhupur site was clay loam with a pH of 5.5, making it strongly acidic, whereas the texture of the Islampur site was sandy loam with a pH of 6.6, making it near neutral in nature. Table 1 shows the initial physicochemical properties of soil at the Madhupur and Islampur sites.
Initial soil properties for acid soil and charland soil.
2.2. Experimental Design and Crop Managements
At both locations, the experiment began in mid-October of 2018 and lasted through November of 2020. The experiment was set up in a randomized complete block design (RCBD, Table S1 in Supplementary Material ), with the experimental area divided into four blocks representing the replications, to reduce the heterogenic effects of soil. There was a total of 24 plots in each experimental site (i.e., six treatments with four replications). The unit plot size was 5 × 4 m at both locations, with 0.75 m of interplot space, and 1 m of interblock space.
On a farmer’s field at Madhupur site (acidic soil), the treatments included PM, RHB, PMB, and dolomite (at a rate of 1 t ha −1 at the beginning of the experiment), whereas PM, PMB, OF, and VC were used as treatments for a farmer’s field on the charland soils of Islampur site. At both locations, organic materials were applied at a rate of 3 t ha −1 for each crop, biochar at a rate of 2 t ha −1 for each crop, while the rest of the nutrients were applied as chemical fertilizers using the IPNS approach based on agro-ecological zone (AEZ) and following the fertilizer recommendation guide-2018 (FRG-2018). The other two treatments at both sites were recommended fertilizer doses only from chemical fertilizer (RD, following FRG-2018), and no organic or inorganic fertilizer (control). The total supply of all the nutrients in each treatment except control was equivalent to the standard dose, RD. The manures had a moisture level of 15% when they were applied, and the chemical properties of the organic amendments are presented in Table 2 .
Chemical properties of organic amendments used in experiments.
PM = Poultry Manure, VC = Vermicompost, OF = compost, RHB = Rice Husk Biochar, PMB = Poultry Manure Biochar.
At the Madhupur site (acidic soil), the cropping pattern was Mustard ( Brassica napus )–Boro rice (Winter rice- Oryza sativa L.)–Transplanted (T.) Aman rice (monsoon rice- Oryza sativa L.). The crop varieties were BARI Sarisa-14 for mustard, BRRI dhan28 for boro rice, and BRRI dhan71 for T. Aman rice, respectively. The seed rate of mustard was 6–7 kg ha −1 , and seeds were sown by line sowing with a distance of 25 cm between lines. Three rice seedlings were transplanted per hill with 20 × 20 cm spacing at both sites. Mustard seed was planted between mid-October and mid-November in 2018 and 2019, whereas seeds of boro rice were sown in the seedbed between 15 November and 29 November in 2018 and 2019 and transplanted in the main field from the last week of January to the first week of February in 2019 and 2020. Similarly, seeds of T. Aman rice were sown in the seedbed between 5 July and 15 July, 2019 and 2020, and transplanted by mid-August in 2019 and 2020. The recommended fertilizer doses at the Madhupur site were 90 kg N, 18 kg P, 40 kg K, 5 kg S, and 1 kg B per ha for mustard; 144 kg N, 9 kg P, 60 kg K, 4 kg S, and 1.5 kg Zn per ha for boro rice; 90 kg N, 7 kg P, 50 kg K, 4 kg S, and 1 kg Zn per ha for T. Aman rice, respectively.
Similarly, a Maize ( Zea mays )-Jute ( Corchorus capsularis )-T. Aman rice (monsoon rice- Oryza sativa L.) cropping pattern was used to carry out the experiment at the Islampur site (charland soil). The crop varieties were Kaveri 50 for maize, Tosha Paat for jute and BRRI dhan71 for T. Aman rice, respectively. Maize seed was sown in line at a rate of 25 kg ha −1 , with a spacing of 60 × 20 cm. Similarly, the jute seed rate was 6 kg ha −1 , and seeds were sown by line sowing with 25 × 5 cm spacing. Maize seed was sown between October and November 2018 and 2019, whereas jute seed was sown in April 2019 and 2020. T. Aman seeds were sown and transplanted at the same time as the Madhupur site. The recommended fertilizer doses at the Islampur site were 225 kg N, 40 kg P, 80 kg K, 30 kg S, 5 kg Mg, 2 kg Zn per ha for maize; 90 kg N, 8 kg P, 50 kg K, 8 kg S per ha for jute; 90 kg N, 8 kg P, 50 kg K, 4 kg S per ha for T. Aman rice, respectively.
The sources of N, P, K, S, Zn, and B were urea, triple superphosphate (TSP), muriate of potash (MoP), gypsum, zinc sulfate, and boric acid, respectively. All organic amendments, dolomite, and all chemical fertilizers except urea were applied during the land preparation for all crops. In the case of rice, urea was administered in three equal parts: 7–10 days after transplanting (DAT), 25–30 days after transplanting (DAT), and 50–55 days after transplanting (DAT). In the case of maize, one-third of the urea was applied during the land preparation and the remaining urea was separated into two equal halves. The first instalment was administered at the 8 to 10 leaf stage (30–35 days after sowing, or DAS), followed by the second instalment at 60–65 DAS. In contrast, half of the urea was provided during the final land preparation in jute and mustard. The remaining urea was applied 40–45 days after seeding in jute and during the flowering stage of mustard.
In mustard, the first irrigation was provided 20–25 days after sowing (before flowering) and the second one within 55 days of seed sowing (during fruit setting). The rice fields were irrigated a day before the final land preparation and then, when necessary, to maintain standing water at about 3 cm above the soil surface throughout the growing season. Depending on soil type and crop requirement, 4–5 irrigations were applied to maize and T. Aman, whereas boro rice required 8 irrigations during the cropping season. When the plants were 10 to 12 cm tall and produced 3–4 leaves, the jute was watered. Then, supplemental irrigation was applied to the crop in accordance with its needs.
A nonselective herbicide, glyphosate (Roundup ® ; Bayer crop science Ltd., Dhaka, Bangladesh), was sprayed across the area at a rate of 1.85 kg ha −1 3 days before land preparation. Furthermore, 7 days after transplanting rice seedlings, pretilachlor (Superhit ® , post-emergence herbicide) was applied at a rate of 450 g ha −1 . Brifer 5G and Cidial 5G (ACI Bangladesh Ltd., Dhaka, Bangladesh) were used to control rice insects as needed. To control Alternaria leaf blight of mustard, seeds were treated with Provax-200 before sowing, and Ripcord 10 EC was sprayed to control cutworm. Jute was sprayed with Diazinon 60 EC, Karate 2.5 EC, and Dithane M-45 to prevent major diseases and pests. Contact insecticides like Ripcord 10 EC and DCC 100 EC were sprayed to control maize insects.
2.3. Harvesting and Data Recording
Each crop was harvested at physiological maturity in a 2 m 2 microplot at the center of each replicated plot. For rice and mustard, grain yield was estimated at 14% moisture content and for maize it was 20%. The NUE was estimated for rice and maize only. Jute was harvested after 120 days of sowing when the flowers had been shed. During harvesting, plants were cut at ground level, and then retting, stripping, washing, sun-dried, and yield data were recorded.
2.4. Plant Analysis and Determination of Nitrogen Use Efficiency (NUE)
From each plot, all parts of cob (i.e., grain, outer sheath, and kernel) and stover of maize, as well as the grain and straw of rice, were chopped separately and dried in an oven at 65 °C until a constant weight was obtained. The oven-dried samples were ground and sieved in a 2-mm sieve [ 39 ]. About 0.1 g of oven dry ground plant sample was taken into a digestion flask to which 1.1 g catalyst mixture (K 2 SO 4 :CuSO 4 ·5H 2 O:Se = 100:10:1) and 5 mL H 2 SO 4 were added. The flasks were swirled and allowed to stand for about 10 min. Then heating (380 °C) was continued until the digest was clear and colorless. After cooling, the content was taken into 100 mL volumetric flask and the volume was made up to the mark with distilled water. A reagent blank was prepared in a similar manner. This digest was used for total N determination by semi micro-Kjeldahl method as described by Bremner and Mulvaney [ 40 ].
Total N uptake by crops was determined by the formula [ 41 ]:
The NUE expressed as a percentage (%) was determined as described by Moll et al. [ 42 ] as follow:
2.5. Soil Sampling and Analysis
After two years of testing with the cropping patterns, Mustard-Boro-T. Aman and Maize-Jute-T. Aman, geo-referenced (GPS—recorded X, Y coordinates, and altitude) composite soils were developed under six different treatments. Firstly, composite soil samples (a mixture of five core samples) at 0–15 cm depth were collected randomly from each replicated plot after harvesting in November 2020 (6th crop phase of each sequence). Then, the samples were air-dried in the shadow for 15 days at room temperature (~25 °C).
2.5.1. Soil Aggregate Properties
Soil aggregate size fractionations were performed using the wet sieving method to obtain water-stable aggregates [ 43 ] in 250 g soil over a sequence of sieves with mesh sizes 2.0, 0.85, 0.30, 0.15 and 0.053-mm. Soil aggregate fractions retained on each sieve after being dispersed in water on a planetary shaker at a rate of 31 rpm were transferred to a nickel cup and oven-dried at 65 °C until a constant weight was obtained. The respective mass of each aggregate size of soil was converted to the relative percentage (over the total mass of aggregates). Aggregate mean weight diameter (MWD) was estimated using Equation (3) below [ 44 , 45 ].
where, mi and di are weight and the mean diameter of aggregate fraction i, respectively.
Aggregate stability index (SI) was obtained by determining the MWD of air-dried soils and soils sieved after being dispersed in water on a planetary shaker [ 46 ] following Equation (4).
where, Instability Index = MWD (dry sieving) − MWD (wet sieving).
2.5.2. Total Nitrogen, pH and Bulk Density
Soil TN was measured using the semimicro Kjeldahl method [ 40 ]. A glass electrode pH meter was used to measure the pH of the samples in a 1:2.5 (soil:water ratio) [ 47 ]. Soil bulk density was determined by following the standard core method [ 48 ]. Antecedent soil moisture content was measured using the thermo-gravimetric method [ 49 ].
2.6. Statistical Analysis
A one-way analysis of variance (ANOVA) was performed using different treatments as a random variable. The distribution of data for normality was checked before ANOVA. Data were statistically analyzed to ascertain the significant differences in treatment effects using Statistix 10 software. A posthoc test was performed to separate differences between treatments using Tukey-Kamer’s multiple comparisons. All statistical analyses were considered significant at p < 0.05 unless otherwise mentioned.
3.1. Effect of Different Treatments on Soil Physicochemical Properties, Crop Productivity, N Uptake and NUE in Acidic Soil
3.1.1. effect of different treatments on soil physicochemical properties, aggregate size distribution.
The proportion of soil material in aggregate size of < 0.053 mm was higher in control, which was statistically similar to RD, whereas the lowest value was observed in PMB, which was identical to all the treatments except control. The aggregate size classes of 0.053–0.15, 0.15–0.30, 0.30–0.85, and 0.85–2 mm were statistically similar in all the treatments. The aggregate size of > 2.0 mm increased due to the application of treatments compared to the control, exhibiting the highest value in PMB, although it was similar to other treatments except for control ( Figure 1 ).

Distribution of different aggregate size fractions within 0–15 cm soil layer under different treatments in acidic soil (bars = average and line = standard error of mean, SEM; n = 10). (PM = poultry manure, RHB = rice husk biochar, PMB = poultry manure biochar, RD = recommended dose from only chemical fertilizer).
The proportion of microaggregates (<0.30 mm) was greater in all the treatments than macroaggregates (>0.30) ( Table 3 ). The highest microaggregate proportion (%) was observed in control, which was statistically similar to RD, whereas treatments except control were similar. In contrast, macroaggregate increased in all the treatments compared to the control. MWD, GMD, and SI increased due to the application of different treatments compared to the control treatment. All the treatments except control were similar to each other with respect these properties ( Table 3 ).
Effects of different treatments on soil aggregate properties of acidic soil.
PM = poultry manure, RHB = rice husk biochar, PMB = poultry manure biochar, RD = recommended dose from only chemical fertilizer, MaAS = proportional macroaggregate mass, MiAS = proportional microaggregate mass, SI = stability index, MWD = aggregate mean weight diameter, GMD = aggregate geometric mean diameter. Data are mean ± SE ( n = 4). Averaged values within a column, succeeded by different small letters (a, b) differ significantly, * indicates significant at 5% level of significance, ** indicates significant at 1% level of significance.
Total Nitrogen, pH, and Bulk Density
Different treatments had a significant influence on soil TN ( p < 0.001, Table 2 ). Except for RHB, all the treatments significantly increased TN content in the soil compared to that of the control. The highest TN increase over control was observed in PMB (33.3%), whereas the least increase was observed in RD (11.1%) ( Table 4 ). Likewise, soil pH was also significantly influenced by different treatments ( p < 0.001, Table 4 ). Soil pH ranged from 5.22 to 5.72 in various treatments. The highest soil pH was observed in PMB, which was identical to PM, whereas the lowest value was observed in Dolomite + RD and RD. Soil pH decreased by 3.3 and 3.5% in RD and Dolomite + RD, respectively, compared to that of the control, whereas soil pH increased by 2.4, 3.0, and 5.7%, respectively, in RHB, PM, and PMB, respectively. In contrast, different treatments had no significant effect on soil BD ( p > 0.05, Table 4 ).
Effects of different treatments on physicochemical properties of acidic soil.
PM = poultry manure, RHB = rice husk biochar, PMB = poultry manure biochar, RD = recommended dose from only chemical fertilizer. Data are mean ± SE ( n = 4). Averaged values within a column, succeeded by different small letters (a, b, c), differ significantly between different treatments at p < 0.05 significance level. *** indicates significant at the 0.001 probability level, ns = non-significant.
3.1.2. Effect of Different Treatments on Crop Yield and System Productivity of Mustard-Boro-T. Aman Rice Cropping Pattern
Different IPNS treatments significantly influenced grain yield of mustard in both 2019 and 2020, and straw yield in 2020 ( p < 0.001, Table 5 ). In 2019, the increase in grain yield of mustard over control varied from 66.7 to 116.7%. The highest increase was observed in PMB and RD, and the lowest increase in PM and Dolomite + RD. In 2020, the increases in grain and straw yields of mustard over control varied from 85.7 to 128.67% and 79.2 to 199.9%, respectively. The highest increases were observed in Dolomite + RD, and the lowest increases in PMB ( Table 5 ).
Effects of different treatments on crop yield and system productivity of Mustard-Boro-T. Aman rice cropping pattern in acidic soil.
PM = poultry manure, RHB = rice husk biochar, PMB = poultry manure biochar, RD = recommended dose from only chemical fertilizer, Data are mean ± SE ( n = 4). Averaged values within a column, succeeded by different small letters (a, b, c, d), differ significantly between different treatments at p < 0.05 significance level. * Significant at the 0.05 probability level. ** Significant at the 0.01 probability level. *** Significant at the 0.001 probability level, ns = nonsignificant.
Likewise, grain and straw yield of boro rice were also significantly influenced by different IPNS treatments in both 2019 and 2020 ( p < 0.001, Table 5 ). In 2019, the increases in grain and straw yields of boro rice over control ranged from 47.5 to 67.5% and 41.7 to 59.3%, respectively. The highest increases were observed in RHB, and the lowest increases in Dolomite + RD. In 2020, the increases in grain and straw yields of boro rice over control varied from 80.0 to 102.9% and 64.5 to 77.3%, respectively. The highest increases in grain and straw yields were observed in PMB and RHB, respectively, and the lowest increases in RHB and RD for grain and straw yields, respectively ( Table 5 ).
Similarly, grain and straw yield of T. Aman rice were also significantly influenced by different IPNS treatments in both 2019 and 2020 ( p < 0.001, Table 5 ). In 2019, the increases in grain and straw yields of T. Aman rice over control varied from 50.0 to 66.7% and 38.7 to 50.3%, respectively. The highest increases in grain and straw yields were observed in Dolomite + RD, and the lowest increase in PM. In 2020, the increases in grain and straw yields of T. Aman rice over control varied from 42.9 to 60.0% and 16.1 to 34.3%, respectively. The highest increases were observed in RHB, and the lowest increases in RD ( Table 5 ).
Different IPNS treatments significantly increased the total system productivity of the Mustard-Boro-T. Aman rice cropping pattern compared to that of the control in 2019 and 2020 ( p < 0.001, Table 5 ). In 2019, the increase in system productivity in different treatments over control ranged from 55.4 to 69.6%, exhibiting the highest value in PMB and the lowest in Dolomite + RD ( Table 5 ). In 2020, the increase over control varied from 74.7 to 82.8%. The highest increase was observed in Dolomite + RD and the lowest in RHB ( Table 5 ).
3.1.3. Effect of Different Treatments on Nitrogen Uptake and NUE by T. Aman and Boro Rice
N uptake and NUE by T. Aman rice were also significantly influenced by different IPNS treatments in both 2019 and 2020 ( Table 6 ). In 2019, the increase in N uptake over control from 45.1 to 63.4% exhibited the highest PMB value and the lowest RHB value ( Table 3 ). The increase in N uptake over control ranged from 54.1 to 65.9% in 2020. The highest increase was observed in PMB and the lowest increase was observed in PM and RD, respectively ( Table 6 ). The highest NUE was noted in PMB in both 2019 and 2020, whereas the lowest NUE was noted in RHB and RD, respectively, in 2019 and 2020 ( Table 6 ).
Effects of different treatments on nitrogen uptake and NUE in acidic soil.
PM = poultry manure, RHB = rice husk biochar, PMB = poultry manure biochar, RD = recommended dose from only chemical fertilizer, Data are mean ± SE ( n = 4). Averaged values within a column, succeeded by different small letters (a, b, c) differ significantly, ns indicates nonsignificant, * indicates significant at 5% level of significance, ** indicates significant at 1% level of significance, *** indicates significant at 0.1% level of significance.
Similarly, different IPNS treatments significantly influenced N uptake by boro rice in 2019 and 2020, and NUE in 2020 ( Table 6 ). In 2019, the increase in N uptake over that of control ranged from 65.7 to 74.7%, exhibiting the highest RHB value and the lowest RD value ( Table 3 ). The increase in N uptake over control ranged from 76.4 to 96.2% in 2020. The highest increase was observed in PMB and the lowest increase was observed in RHB ( Table 6 ). The highest NUE was noted in PMB in 2019 and 2020, whereas the lowest was noted in Dolomite + RD and RHB in 2019 and 2020 ( Table 6 ).
3.2. Effect of Different Treatments on Soil Physicochemical Properties, Crop Productivity, N Uptake and NUE in Charland Soil
3.2.1. effect of different treatments on soil physicochemical properties.
The proportion of soil material in aggregate size classes of < 0.053 was higher in control, which was statistically similar to RD, PM, and VC, whereas the lowest value was observed in PMB, which was identical to OF and VC. The aggregate size classes of 0.053-0.15, 0.15–0.30, and 0.30–0.85 mm were statistically similar in all the treatments ( Figure 2 ). The highest aggregate size of 0.85–2.0 mm was observed in PMB, which was statistically identical to OF ( Figure 2 ). Similarly, the proportion of aggregate size of > 2.0 mm also increased when the soil was treated with PMB based IPNS, which was statistically similar to PM ( Figure 2 ).

Distribution of different aggregate size fractions within 0–15 cm soil layer under different treatments in charland soil (bars = average and line = standard error of mean, SEM; n = 10). (PM = poultry manure, PMB = poultry manure biochar, OF = compost, VC = vermicompost, RD = recommended dose from only chemical fertilizer).
The proportion of micro aggregates (< 0.30 mm) were greater in all the treatments than macroaggregates (> 0.30) ( Table 7 ). The highest micro aggregate proportion (%) was observed in RD which was statistically similar to all the treatments except PMB. In contrast, macro aggregate increased in PMB compared to that of the control, other treatments were statistically identical. MWD, GMD, and SI increased by 32, 11, and 19% in PMB compared to that of the control treatment. All the treatments except PMB were similar to control in all these cases ( Table 7 ).
Effects of different treatments on soil aggregate properties of charland soil.
PM = poultry manure, PMB = poultry manure biochar, OF = compost, VC = vermicompost, RD = recommended dose from only chemical fertilizer, MaAS = proportional macro aggregate mass, MiAS = proportional micro aggregate mass, SI = stability index, MWD = aggregate mean weight diameter, GMD = aggregate geometric mean diameter. Data are mean ± SE ( n = 4). Averaged values within a column, succeeded by different small letters (a, b), differ significantly between different treatments at p < 0.05 significance level. *** Significant at 0.001 probability level.
Organic amendment based IPNS approaches had no significant effects on soil TN and pH after the completion of the experiment ( p > 0.05, Table 8 ). In contrast, different treatments in this study significantly influenced soil BD ( p < 0.05, Table 8 ). Soil BD ranged from 1.26 to 1.33 g cm −3 , exhibiting the highest value in control and RD, identical to other treatments except for PMB. The lowest value was observed in PMB, which was identical to PM and OF, respectively. Compared to that of control, BD decreased by about 0.8, 2.3, 3.0, and 5.3% in VC, PM, OF, and PMB, respectively.
Effects of different treatments on physicochemical properties of charland soil.
PM = poultry manure, PMB = poultry manure biochar, OF = compost, VC = vermicompost, RD = recommended dose from only chemical fertilizer, Data are mean ± SE ( n = 4). Averaged values within a column, succeeded by different small letters (a, b) differ significantly, * indicates significant at 5% level of significance, ns indicates nonsignificant.
3.2.2. Effect of Different Treatments on Crop Yield and System Productivity of Maize-Jute-T. Aman Rice Cropping Pattern
Different IPNS treatments significantly influenced grain and straw yields of maize in both 2019 and 2020 ( p < 0.001, Table 9 ). In 2019, the increases in grain and straw yields of maize over control varied from 60.9 to 89.1% and 99.1 to 138.9%, respectively. The highest increases in grain and straw yields were observed in PM, and the lowest increases in grain and straw yields were observed in VC and PMB, respectively. In 2020, the increases in grain and straw yields of maize over control varied from 106.1 to 130.3% and 53.5 to 83.1%, respectively. The highest increases in grain and straw yields were observed in PMB and PM, respectively, and the lowest increases in VC ( Table 9 ).
Effects of different treatments on crop yield and system productivity of Maize-Jute-T. Aman rice cropping pattern in charland soil.
PM = poultry manure, PMB = poultry manure biochar, OF = compost, VC = vermicompost, RD = recommended dose from only chemical fertilizer, Data are mean ± SE ( n = 4). Averaged values within a column, succeeded by different small letters (a, b, c, d), differ significantly between different treatments at p < 0.05 significance level. * Significant at 0.05 probability level. ** Significant at 0.01 probability level. *** Significant at 0.001 probability level.
Likewise, the fiber yield of jute was also significantly influenced by different IPNS treatments in both 2019 and 2020 ( p < 0.001, Table 9 ). In 2019, jute yield in different treatments ranged from 2.5 to 3.7 t ha −1 , exhibiting the highest yield in VC, which was identical to all the treatments except control, and the lowest yield in control. The increase in yield over control varied from 24.0 to 48.0%. The highest increase was observed in VC, and the lowest increase in RD. In 2020, jute yield in different treatments ranged from 1.2 to 2.6 t ha −1 , exhibiting the highest yield in PMB, which was similar to PM, and the lowest yield in control. The increase in yield over control varied from 33.3 to 116.7%. The highest increase was observed in PMB, and the lowest increase in OF and RD ( Table 9 ).
Similarly, grain and straw yields of T. Aman rice were also significantly influenced by different IPNS treatments in both 2019 and 2020 ( p < 0.001, Table 9 ). In 2019, the increases in grain and straw yield of T. Aman over control ranged from 24.3 to 45.9% and 22.1 to 31.7%, respectively. The highest increases in grain and straw yields were observed in OF, and the lowest increases in PM. In 2020, the increase in grain and straw yields of T. Aman rice over control varied from 77.8 to 138.9% and 94.9 to 124.3%, respectively. The highest increases in grain and straw yields were observed in OF and PMB, respectively, and the lowest increases in grain and straw yields were observed in VC and PM, respectively ( Table 9 ).
Different IPNS treatments significantly increased the total system productivity of the Maize-Jute-T. Aman rice cropping pattern compared to that of the control in 2019 and 2020 ( p < 0.001, Table 9 ). In 2019, the increase in system productivity in different treatments over control ranged from 43.3 to 51.1%, exhibiting the highest value in PMB and the lowest in RD ( Table 9 ). In 2020, the increase over control varied from 74.4 to 115.4%. The highest increase was observed in PMB and the lowest in VC.
3.2.3. Effect of Different Treatments on N Uptake and NUE by T. Aman Rice and Maize
N uptake and NUE by T. Aman rice were also significantly influenced by different IPNS treatments in both 2019 and 2020 ( Table 10 ). In 2019, the increase in N uptake over control from 35.5 to 47.2% exhibited the highest value in OF and the lowest value in PM ( Table 10 ). On the other hand, the increase in N uptake over control ranged from 87.1 to 109.3% in 2020. The highest increase was observed in OF and the lowest increase was observed in PM ( Table 10 ). The highest NUE was noted in PMB and the lowest NUE was noted in PM in both 2019 and 2020, respectively ( Table 10 ).
Effects of organic amendments on N uptake and N use efficiency by T. Aman rice and maize.
PM = poultry manure, PMB = poultry manure biochar, OF = compost, VC = vermicompost, RD = recommended dose from only chemical fertilizer, Data are mean ± SE ( n = 4). Averaged values within a column, succeeded by different small letters (a, b, c) differ significantly, ** indicates significant at 1% level of significance, *** indicates significant at 1% level of significance.
Similarly, different IPNS treatments significantly influenced N uptake and NUE by maize in both 2019 and 2020 ( Table 10 ). In 2019, the increase in N uptake over control ranged from 71.5 to 79.2%, exhibiting the highest value in OF and the lowest value in VC ( Table 10 ). The increase in N uptake over control ranged from 94.5 to 105.7% in 2020. The highest increase was observed in OF and the lowest increase was observed in PM ( Table 10 ). The highest NUE was noted in OF in both 2019 and 2020, whereas the lowest NUE was noted in VC and PM, in both 2019 and 2020 ( Table 10 ).
4. Discussion
4.1. effect of ipns on soil physicochemical properties.
IPNS is used worldwide to improve the physical, chemical, and biological properties of degraded soils. The IPNS treatments in the present study significantly influenced the soil physicochemical properties of the acidic and charland soils. Our results demonstrated that all the IPNS treatments, particularly biochar and compost-based IPNS, significantly increased water-soluble macroaggregate (WSA) groups in soils, stability index, MWD, and GMD ( Table 3 and Table 7 ), which were in accordance with previous studies [ 50 , 51 , 52 ]. Biochar and compost, according to Ibrahim et al. [ 53 ], significantly improved aggregate stability and water-soluble macroaggregate (>0.25 mm) in loamy sand soil. Mi et al. [ 54 ] found that NPK and organic matter (straw residue or cattle manure) significantly increased the water-soluble aggregate > 0.25 mm, MWD, and GMD, while reducing the proportion of 0.25 mm aggregates at two field experiment sites in Jinhua, Zhejiang province, and Jintan, Jiangsu province. According to Annabi et al. [ 55 ], adding organic matter to loam soils affects aggregate stability, and they ascribe this to increased microbial activity, which improves aggregate stability. In research conducted by Šimanský et al. [ 56 ], using N fertilizer in combination with biochar increased the macroaggregates. They observed that WSA of 3–2 mm and 5–3 mm sizes were 75 and 149% higher when biochar was applied along with N fertilizer (20 t biochar ha −1 + 80 kg N ha −1 ), whereas sole application of biochar (20 t ha −1 ) resulted in a comparatively small (0.5–0.25 mm) size aggregate. Similar results were also observed by Ma et al. [ 57 ], who reported that both the NPK fertilizer + maize straw and NPK + biochar treatment significantly enhanced the relative proportion of macroaggregates (> 2 mm) and the mean weight diameter while decreasing the relative ratio of microaggregates (< 0.25 mm). Organic matter is essential for repositioning soil particles so that aggregates can form [ 58 ]. Organic material incorporation into soil considerably increases soil organic matter content while also releasing organic acids, polysaccharides, and other by products that bind free primary particles into macroaggregates, resulting in the formation of macroaggregates with a larger (> 2 mm) size [ 59 ]. Another assumption is that organic manures function as stimulants for microbes [ 60 ], encouraging microbial development and increasing the density and efficacy of mycorrhizal hyphae, which enhances aggregate stability [ 61 , 62 , 63 ]. Adding nitrogen to the soil can speed up the biochar mineralization process, resulting in increased aggregation [ 56 , 64 ]. However, Xin et al. [ 65 ] reported that integrated application of half organic compost and half mineral fertilizer did not result in greater proportions of the > 0.25 mm aggregate than after using NPK for 23 years. Biochar made from a mixture of wood and straw, on the other hand, did not influence aggregate stability [ 55 ]. Furthermore, due to differences in parameters such as the quality, amount, and timing of organic matter addition, the research does not clearly show a link between aggregate stability and rates of organic input [ 66 ].
Addition of organic and inorganic fertilizers significantly increased soil TN in acidic soil ( Table 4 ), which aligns with Islam et al. [ 7 , 24 , 25 ] and Van Chuong [ 67 ]. Previous research showed that the C:N ratio of the manure is essential for mineralization and the build-up of SOC and TN, and that a low C:N ratio boosts the mineralization process, resulting in increased soil TN [ 68 , 69 ]. However, the effect of IPNS on soil TN in charland soil was nonsignificant ( Table 8 ). Our results suggest that IPNS is more efficient in acidic soil than in charland soil in terms of increasing soil TN.
Our results demonstrated that IPNS treatments significantly increased soil pH compared to the control, although sole application of chemical fertilizer decreased soil pH, as supported by previous studies [ 54 , 70 , 71 ]. Lin et al. [ 72 ] observed that organic fertilizer and chemical fertilizer significantly increased the tea orchard soil pH and microbial population. This is attributed to the increased soil organic matter content, which encourages soil maturation, improves soil structure, and raises the soil base saturation percentage by the organic manure, which can help to reduce soil acidity [ 73 , 74 ]. On the other hand, chemical fertilizer undergoes rapid hydrolysis and subsequent nitrification, resulting in the release of protons and a decrease in soil pH.
The results revealed that IPNS treatments had no significant effect on soil bulk density in acidic soil, although IPNS treatments significantly decreased soil bulk density in charland soil. Many studies have shown that including organic material in the soil reduces its bulk density [ 75 , 76 ]. The reason for the decreased soil bulk density is the addition of organic materials, which increases soil organic matter content, resulting in soils that are more friable, porous, and chemically active, reducing soil bulk density [ 77 ]. However, in acidic soil, the effect was not observed, which may be due to the clay type soil texture.
4.2. Effect of IPNS on Crop Yield, Nitrogen Uptake and Nitrogen Use Efficiency
Chemical fertilizers are widely used in the current agricultural farming system, which has a detrimental impact on soil health, the environment, and crop yield. Nitrogen is the most important yet limited nutrient among chemical fertilizers worldwide due to very low NUE due to gaseous losses such as denitrification, volatilization, and leaching from agricultural cropping systems [ 78 , 79 ]. Our results showed that the combined application of organic manure, inorganic fertilizer and dolomite significantly increased total crop yields (i.e., system productivity) of the cropping patterns ( Table 5 and Table 9 ), which is consistent with previous studies in acidic soil [ 7 , 24 , 25 ]. In the acidic soil of Nalitabari, Sherpur, Islam et al. [ 7 , 24 ] reported that combined application of lime, organic amendment, and chemical fertilizer increased yield and nutrient uptake of the T. Aman-Maize-Fallow and Wheat-Mungbean-T. Aman cropping patterns. In another study at the same site as the present study, Islam et al. [ 25 ] observed that combined application of lime, organic manure, and chemical fertilizer significantly improved the total system productivity and nutrient absorption of the T. Aman-Mustard-Boro cropping pattern with subsequent improvement in the soil quality. The authors postulated the rise in soil pH and other soil physicochemical properties as the cause of the increase in crop productivity and nutrient uptake. Iqbal et al. [ 80 ] reported that 30% PM + 70% chemical fertilizer increased rice grain yield by 95% and nutrient uptake and NUE. In a field experiment at the Agronomic Research Farm, University of Agriculture Faisalabad, Pakistan, Mahmood et al. [ 81 ] observed that NPK at 150-85-50 + 7 t ha −1 PM significantly increased maize yield with concurrent improvement in soil properties. Similarly, Bedada et al. [ 82 ] found that the combined application of compost and urea improved soil physicochemical properties and increased maize production. Improvement in soil physical conditions along with a sufficient amount of water enhanced crop nutrient uptake capacity, resulting in greater biological output at a specific quantity of fertilizer application [ 83 ]. Hossain et al. [ 84 ] similarly found that FYM treatment increased N absorption in rice compared to no fertilizer and inorganic fertilizer applications. Chemical fertilizer alone reduced nutrient absorption due to poor availability and more significant nutritional loss [ 85 ]. Crop N requirements must be synchronized with N supply to improve N usage efficiency. Crop type, availability of other nutrients, nutrient leaching, weather, and genotypic variations are all factors that influence crop NUE [ 86 , 87 ]. In rice, Dawe et al. [ 88 ] discovered that N losses are reduced as yield and NUE increase, while Fofana et al. [ 89 ] observed comparable effects in maize crops. In two-year field research, Rehim et al. [ 90 ] reported a 98% increase in N absorption of wheat grains and a 200% increase in NUE when 75% urea+25% farmyard manure was applied instead of urea alone. These findings showed that combining organic and inorganic sources to improve NUE in crops is more effective [ 91 ].
5. Conclusions
Two-year evaluation of IPNS indicates that it is potentially a good practice for sustainable crop production in acidic and charland soils. The present research results revealed that poultry manure biochar-based IPNS showed the best enrichments in terms of crop yields, system productivity, nitrogen uptake, and NUE of the Mustard-Boro-T. Aman cropping pattern in acidic soil. All the IPNS treatments improved soil aggregate properties, with increased macroaggregate formation, and also enhanced soil TN concentration and pH. Compost and poultry manure biochar based IPNS improved the crop yields, total system productivity, nitrogen uptake, and use efficiency of the Maize-Jute-T. Aman cropping pattern in charland soil. Poultry manure biochar based IPNS increased macroaggregate and decreased the soil bulk density of charland soil. Therefore, poultry manure biochar- and compost-based IPNS can be recommended for maximizing crop productivity, nitrogen uptake, and use efficiency, with subsequent improvement of the physicochemical properties of acidic and charland soils.
Acknowledgments
The authors are grateful to The World Bank for its partial support to conduct this research. The authors would like to extend their sincere appreciation to the Researchers Supporting Project number (RSP-2021/347), King Saud University, Riyadh, Saudi Arabia.
Supplementary Materials
The following are available online at https://www.mdpi.com/article/10.3390/plants10112547/s1 , Table S1: List of abbreviations.
Author Contributions
Conceptualization, M.M.R., M.M.R.J. and M.R.I.; methodology, M.M.R., M.M.R.J. and M.R.I.; software, M.M.R. and S.U.; validation, M.M.R., M.M.R.J. and M.R.I.; formal analysis, M.M.R. and S.U.; investigation, M.M.R., M.M.R.J. and M.R.I.; resources, M.M.R.J. and M.R.I.; data curation, M.M.R., S.U., M.M.R.J. and M.R.I.; writing—original draft preparation, M.M.R., S.U., M.M.R.J. and M.R.I.; writing—review and editing, M.M.R., S.U., M.M.R.J., Z.M.S., S.A., M.H.S. and M.R.I.; visualization, S.U., M.M.R.J. and M.R.I.; supervision, M.M.R.J. and M.R.I.; project administration, M.M.R.J. and M.R.I.; funding acquisition, M.M.R.J., S.A., M.H.S. and M.R.I. All authors have read and agreed to the published version of the manuscript.
The World Bank funded this research under National Agricultural Technology Program- Phase II Project (NATP-2) administered by Bangladesh Agricultural Research Council (BARC) (Project ID: 135). It was also partially supported by Researchers Supporting Project number (RSP-2021/347), King Saud University, Riyadh, Saudi Arabia.
Institutional Review Board Statement
Not applicable.
Informed Consent Statement
Data availability statement, conflicts of interest.
The authors declare that the research was conducted in the absence of any commercial or financial relationships that could be construed as a potential conflict of interest.
Publisher’s Note: MDPI stays neutral with regard to jurisdictional claims in published maps and institutional affiliations.
Thank you for visiting nature.com. You are using a browser version with limited support for CSS. To obtain the best experience, we recommend you use a more up to date browser (or turn off compatibility mode in Internet Explorer). In the meantime, to ensure continued support, we are displaying the site without styles and JavaScript.
- View all journals
- My Account Login
- Explore content
- About the journal
- Publish with us
- Sign up for alerts
- Open access
- Published: 15 January 2021
Crop nutrient management using Nutrient Expert improves yield, increases farmers’ income and reduces greenhouse gas emissions
- Tek B. Sapkota ORCID: orcid.org/0000-0001-5311-0586 1 ,
- Mangi L. Jat ORCID: orcid.org/0000-0003-0582-1126 2 ,
- Dharamvir S. Rana 3 ,
- Arun Khatri-Chhetri 4 ,
- Hanuman S. Jat 5 ,
- Deepak Bijarniya 6 ,
- Jhabar M. Sutaliya 7 ,
- Manish Kumar 8 ,
- Love K. Singh 8 ,
- Raj K. Jat 9 ,
- Kailash Kalvaniya 2 ,
- Gokul Prasad 2 ,
- Harminder S. Sidhu 8 ,
- Munmun Rai 2 ,
- T. Satyanarayana 10 &
- Kaushik Majumdar 11
Scientific Reports volume 11 , Article number: 1564 ( 2021 ) Cite this article
9658 Accesses
52 Citations
43 Altmetric
Metrics details
- Climate-change mitigation
- Environmental impact
Reduction of excess nutrient application and balanced fertilizer use are the key mitigation options in agriculture. We evaluated Nutrient Expert (NE) tool-based site-specific nutrient management (SSNM) in rice and wheat crops by establishing 1594 side-by-side comparison trials with farmers’ fertilization practices (FFP) across the Indo-Gangetic Plains (IGP) of India. We found that NE-based fertilizer management can lower global warming potential (GWP) by about 2.5% in rice, and between 12 and 20% in wheat over FFP. More than 80% of the participating farmers increased their crop yield and farm income by applying the NE-based fertilizer recommendation. We also observed that increased crop yield and reduced fertilizer consumption and associated greenhouse gas (GHG) emissions by using NE was significantly influenced by the crop type, agro-ecology, soil properties and farmers’ current level of fertilization. Adoption of NE-based fertilizer recommendation practice in all rice and wheat acreage in India would translate into 13.92 million tonnes (Mt) more rice and wheat production with 1.44 Mt less N fertilizer use, and a reduction in GHG of 5.34 Mt CO 2 e per year over farmers’ current practice. Our study establishes the utility of NE to help implement SSNM in smallholder production systems for increasing crop yields and farmers’ income while reducing GHG emissions.
Similar content being viewed by others
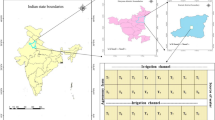
Boosting wheat yield, profitability and NUE with prilled and nano urea in conservation tillage
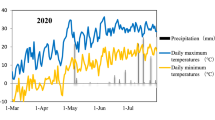
Optimizing nutrient inputs by balancing spring wheat yield and environmental effects in the Hetao Irrigation District of China

Global mean nitrogen recovery efficiency in croplands can be enhanced by optimal nutrient, crop and soil management practices
Introduction.
In recent years, the potential to mitigate climate change by improving nutrient use efficiency (NUE) in croplands has received considerable attention in the agricultural research and policy agendas 1 , 2 , 3 . The use of chemical fertilizers, nitrogen (N) in particular, in crop production is at the center of managing both food security and environmental problems 4 , 5 , 6 . Enhancing crop yields through increased use of nutrients is essential to meet current as well as future food demand 7 . On the other hand, because fertilizer application in croplands is a major source of anthropogenic nitrous oxide (N 2 O) emissions 8 , reducing greenhouse gas (GHG) emissions through proper fertilizer management is essential to address agriculture’s contribution to climate change 2 . Moreover, excess and improper use of nutrients in crop production have large cost implications for the farmers 9 . Therefore, improving NUE in croplands provides the opportunity to address the triple challenge of food security, farmers’ livelihood and environmental protection, globally.
Site-Specific Nutrient Management (SSNM) involves optimizing nutrient inputs considering demand (plant needs) and supply (from soils indigenous sources) of the nutrients according to their variation in time and space thereby ensuring field-specific nutrient management in a particular cropping season 10 , 11 . Various technologies and practices such as Chlorophyll Meter 12 , Leaf Color Chart 13 , GreenSeeker 14 and decision support systems for instance Nutrient Expert (NE) ( http://software.ipni.net ) and Rice Crop Manager ( http://cropmanager.irri.org/home ) are available for helping farmers to implement SSNM and improve NUE 9 , 15 . The NE tool was developed to implement crop nutrient management specific to farmers' fields with or without a soil test 16 . Although a few studies have evaluated the agronomic and environmental performance of NE 17 , 18 , 19 , it has not been evaluated on a large number of farms with varying agro-climatic conditions and across various levels of crop intensification. Farmers’ participatory trials are useful to assess the utility of the tool and also enable farmers to make informed decisions for crop nutrient management.
This study presents results from a large number of on-farm participatory trials (1594-paired data) comparing farmers’ fertilizer practices (FFP) vs NE-based nutrient management in terms of fertilizer inputs, yields, economic returns, and GHG emissions in rice and wheat fields in India. Rice and wheat are the major crops grown in India and consume 50% of the fertilizer used in the country 20 . The study in India was particularly important because India consumes 14% of total fertilizer use globally 20 but its NUE is one of the lowest in the world 21 . This is mainly driven by imbalanced and inadequate use of nutrients given the skewed government's subsidy on nitrogenous fertilizer than on other nutrients 22 . The results of this study provide rich information to the agriculture and fertilizer policymakers to enable them to design fertilizer use and distribution policies and farmers' support programs in the IGP and other parts of the country.
Fertilizer input and crop yield
The use of the NE tool significantly reduced the amount of nigrogen (N) use in both rice and wheat crops compared to the FFP (Fig. 1 a). Although potash (K 2 O) input was significantly higher under NE than under FFP (Fig. 1 b), phosphorus (P 2 O 5 ) input was also significantly lower under NE-based recommendations than FFP, except for rice in Western IGP (Fig. 1 c). The N, P 2 O, and K 2 O application rates in each comparison trial in rice and wheat fields under NE and FFP in the study areas are presented in Supplementary Figure S1 . Farmers in the Western IGP were either applying high P 2 O 5 rates in rice cultivation or none at all. We also observed that many farmers in the IGP region were not using K 2 O on rice and wheat crops.
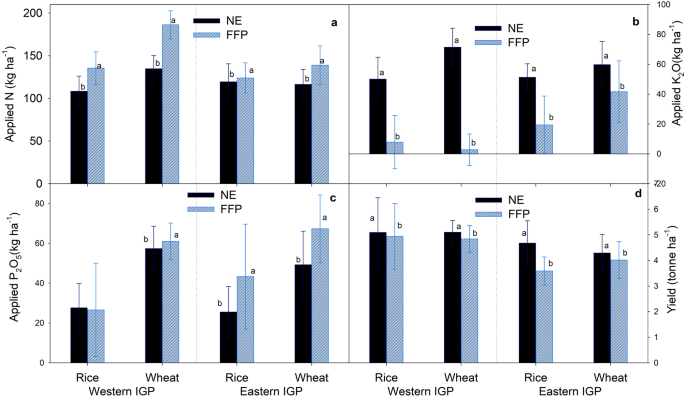
Rate of nitrogen ( a ), potash ( b ) and phosphorus ( c ) application and grain yield ( d ) from rice and wheat under Nutrient Expert (NE) and Farmers’ Fertilizer Practices (FFP) in the study areas. Values are average of all the comparison trials over the study period. Within each pair, bars bearing different lowercase letters are significantly different from each other based on the paired t-test (p = 0.05). The error bar shows the standard deviation. IGP = Indo-Gangetic Plains.
The NE-based recommendation significantly reduced N application over the FFP in the wheat crop more than in rice, and in the Western IGP more than in the Eastern IGP (Fig. 2 , left panel). The effect of NE in reducing N application over the FFP was influenced by farmers’ fertilizer application rates. The reduction in N rate by NE over FFP was higher in the cases where farmers’ N application rate was higher and the effect gradually reduced as the rate of farmers’ N application decreased (Fig. 2 , left panel). Similarly, the effect of NE in reducing the N application rate was higher where farmers were applying between 41 and 70 kg P 2 O 5 ha −1 than in the cases where farmers were not applying it or were applying smaller quantities, i.e., < 40 kg of P 2 O 5 ha −1 . N reduction was also higher in the cases where farmers were not applying K 2 O than in the cases where farmers were applying K 2 O (Fig. 2 , left panel).
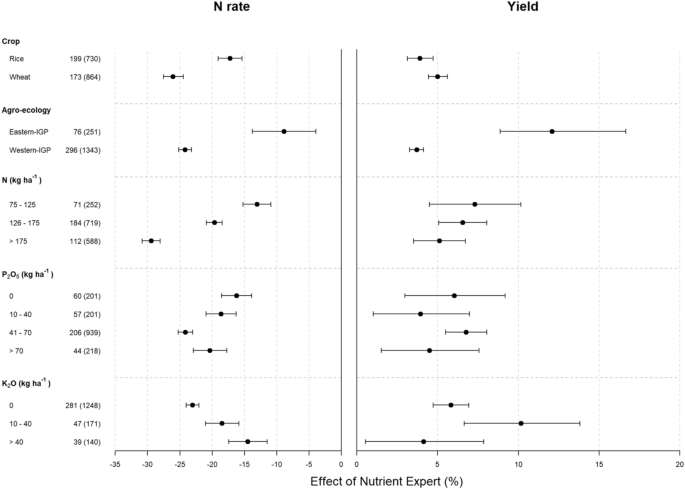
Percent change in nitrogen use rate (left panel) and grain yields (right panel) due to NE-based fertilizer management compared to FFP segregated by crop types, agro-ecological zones and farmers’ fertilizer application rates. In the Y-axis, the numbers outside parentheses denote the number of villages and the ones inside parentheses show the number of pairs analyzed. Error bars indicate 95% confidence intervals (CI). The changes are considered significant when 95% CI does not overlap zero, and the effects of categories within a parameter are significantly different when their 95% CI do not overlap with each other. IGP = Indo-Gangetic Plains.
Overall, NE-based fertilizer management significantly increased the yield of both rice and wheat in both agro-ecologies as compared to FFP (Fig. 1 d). However, the increment was highest in rice in Eastern IGP. When separated by agro-ecology, the yield increment due to NE was higher in Eastern IGP than in Western IGP (Fig. 2 , right panel). Although the yield response to NE-based fertilizer management was not significant under different rates of fertilizer (N, P 2 O 5 and K 2 O) application by farmers, yield improvement due to NE was comparatively high where farmers used less K 2 O (Fig. 2 , right panel).
Out of 135 pair comparisons in the rice fields of Eastern IGP, NE yielded higher than FFP in all cases, with less N input than FFP in 49% of cases and with more N input than FFP in 50% of cases (Supplementary Fig. S2 , upper left). In the case of wheat in this agro-ecology and compared to FFP, NE increased crop yield in 78% of cases out of 116 pair comparisons, in 65% of cases with reduced N input and in the remaining 13% of cases with increased N input (Supplementary Fig. S2 , lower left). Here, compared to FFP, NE reduced yield in 20% of cases mostly with reduced N input. In Western IGP, on the other hand, compared to FFP, NE increased rice and wheat yield in 83% out of 595 pair comparisons and in 91% out of 748 pair comparisons, respectively, mostly with reduced N input (Supplementary Fig. S2 , right panels).
Greenhouse gas emissions
The farm gate GWP from rice production in the study area ranged from 2463 to 5482 kgCO 2 e ha −1 whereas that from wheat production ranged from 287 to 2463 kgCO 2 e ha −1 . Similarly, GHG emission intensity of rice ranged from 509 to 1606 kgCO 2 e tonne −1 grain yield and that of wheat ranged from 71 to 769 kgCO 2 e tonne −1 grain yield. Total GWP and GHG emission intensity of rice and wheat production was significantly lower under NE-based fertilizer management than under FFP in both agro-ecologies (Fig. 3 A,B).
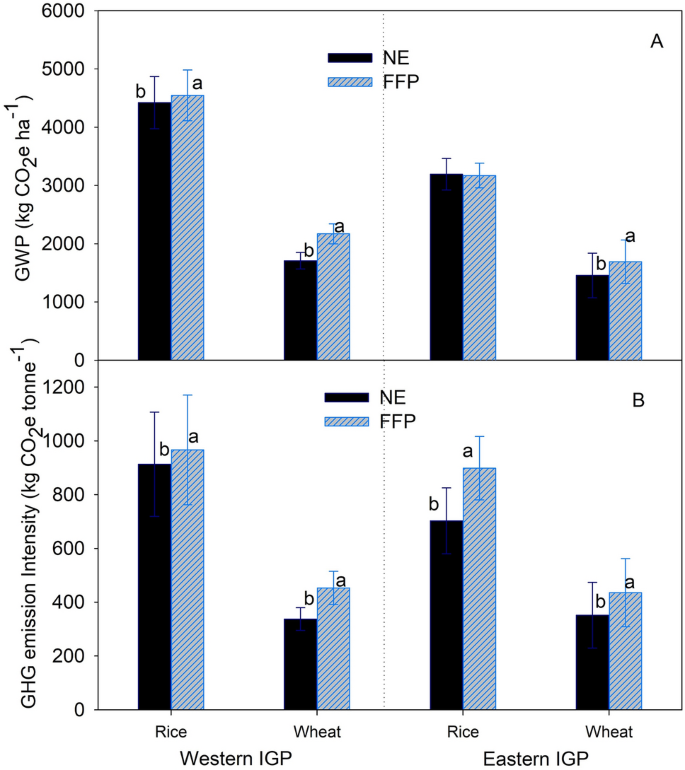
Total global warming potential ( A ) and emission intensity ( B ) from rice and wheat production under NE and FFP in the study areas. Values are average of all the comparison trials over the study period. Within each pair, bars bearing different lower case letters are significantly different from each other based on a paired t-test (p = 0.05). Error bar shows the standard deviation. IGP = Indo-Gangetic Plains.
The NE-based fertilizer management reduced more GWP in wheat than in rice and more in Western IGP than in Eastern IGP (Fig. 4 , left panel). When separated by farmers’ fertilizer rate, the effect of NE-based fertilizer recommendations in reducing total GWP was significantly smaller with lower applications of N and P 2 O 5 (< 40 kg P 2 O 5 ha −1 ) than in the cases where farmers were applying higher rates of these fertilizers (Fig. 4 , left panel). Similarly, the effect of NE in reducing GHG intensity was higher in wheat than in rice and higher in Eastern IGP than in Western IGP (Fig. 4 , right panel). The effect of NE in reducing total GHG emission intensity was significantly smaller with lower applications of N and P 2 O 5, (< 40 kg P 2 O 5 ha −1 ) than in cases where farmers were applying higher rates, i.e., > 175 kg N and > 40 kg P 2 O 5 ha −1 (Fig. 4 , right panel).
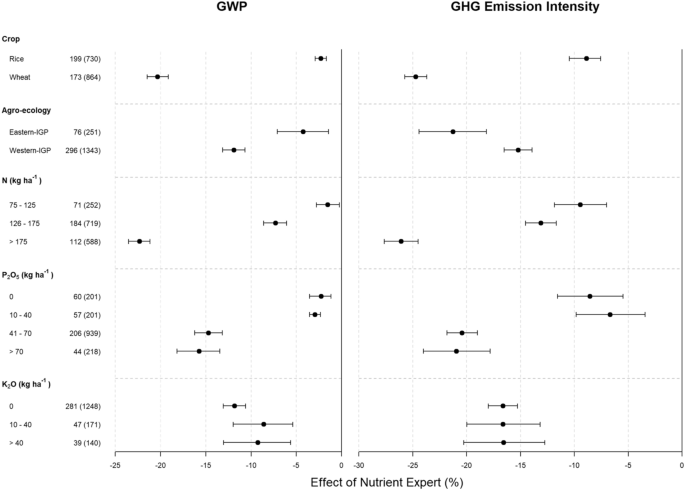
Percent change in global warming potential (GWP, left panel) and emission intensity (right panel) due to NE-based fertilizer management compared to FFP segregated by crop types, agro-ecological zones and farmers’ fertilizer application rates. In the Y-axis, the numbers outside parentheses denote the number of villages and the ones inside parentheses show the number of pairs analyzed. Error bars indicate 95% confidence intervals (CI). The changes are considered significant when 95% CI does not overlap zero, and the effects of categories within a parameter are significantly different when their 95% CI do not overlap with each other. IGP = Indo-Gangetic Plains.
Cost–benefit of change in fertilizer rate
The cost of fertilizer application under NE-based recommendations in rice and wheat crops was largely affected by the FFP in the different agro-ecological zones. NE significantly increased the cost of fertilizer for both rice and wheat in Western IGP (Fig. 5 a). In Eastern IGP, the cost of fertilizer for rice was not significantly different between NE and FFP, whereas that for wheat was significantly higher under FFP (Fig. 5 a). The percent increment in fertilizer cost due to NE over FFP was higher in rice than in wheat (Fig. 6 , left panel). When separated by agro-ecologies, compared to FFP, NE significantly increased cost of fertilizer in Western IGP but reduced it in Eastern IGP, although not significantly. Compared to FFP, NE slightly increased the total cost of fertilizer in cases where farmers’ N rate was < 175 compared to cases where farmers’ N rate was > 175 kg ha −1 (Fig. 6 , left panel). The total cost of fertilizer due to NE significantly increased in cases where farmers did not apply any P 2 O 5 or K 2 O, and the total cost actually decreased in cases where farmers applied > 70 kg P 2 O 5 and > 10 kg K 2 O ha −1 (Fig. 6 , left panel).
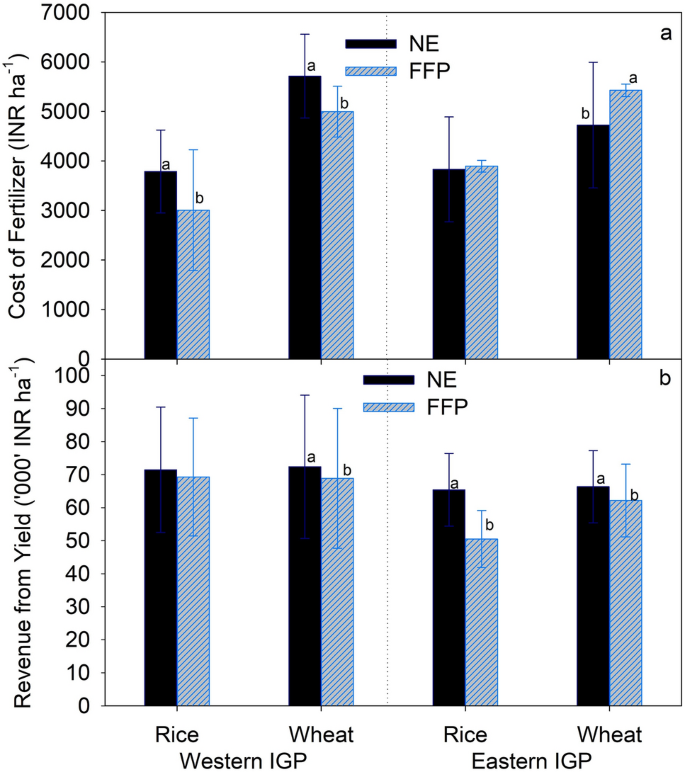
Cost of fertilizer and income from yield in rice and wheat production under Nutrient Expert (NE) and Farmers’ Fertilizer Practice (FFP) in the study areas. Values are average of all the comparison trials over the study period. Within each pair, bars bearing different lowercase letters are significantly different from each other based on paired t-test (p = 0.05). Error bar shows standard deviation. IGP = Indo-Gangetic Plains.
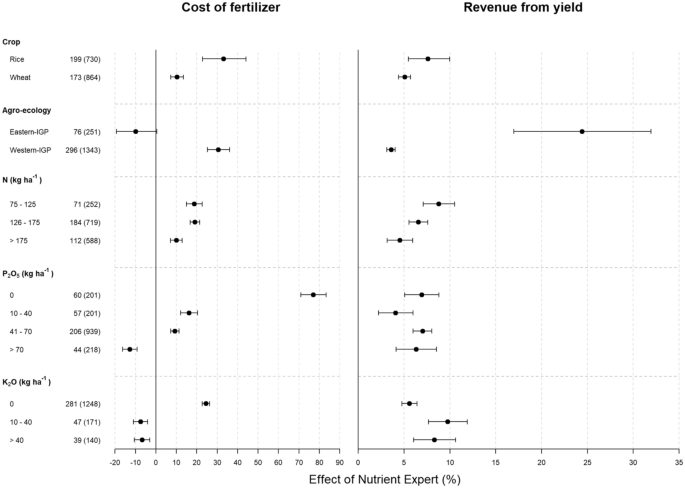
Percent in fertilizer cost (left panel) and revenue from yield (right panel) due to NE-based fertilizer management compared to FFP segregated by crop types, agro-ecological zones and fertilizer application rates. In the Y-axis, the numbers outside parentheses denote the number of villages and the ones inside parentheses show the number of pairs analyzed. Error bars indicate 95% confidence intervals (CI). The changes are considered significant when 95% CI does not overlap zero, and the effects of categories within a parameter are significantly different when their 95% CI do not overlap with each other. IGP = Indo-Gangetic Plains.
Except for rice in Western IGP, NE significantly increased the gross income from crop production compared to FFP (Fig. 5 b). When separated by agro-ecological zones, the revenue increase due to NE over FFP was significantly higher in Eastern IGP than in Western IGP (Fig. 6 , right panel). Similarly, the percentage of revenue increase from NE over FFP was higher in cases where farmers’ N application rate was lower (N < 125 kg N ha −1 ) than in cases where farmers’ N application rate was higher (N > 175 kg N ha −1 ).
Use of the nutrient expert tool for SSNM
This study presented the benefits of using the NE tool for SSNM in rice and wheat crops in two different agro-ecological zones in India. The NE-based nutrient management not only increased the grain yield of rice and wheat crops but also decreased the total N and P 2 O 5 applied in the fields (Fig. 1 ). The results of increased yields and decreased N rates through NE-based nutrient management are in agreement with other findings in the region 19 that also reported increased yield and reduced consumption of N and P 2 O 5 through NE-based fertilizer recommendation. Increased crop yield and reduced fertilizer consumption by NE can be attributed to increased NUE as NE gives dynamic fertilizer recommendation based on growing conditions, soil indigenous nutrient supply and residual nutrients from previous crops thereby minimizing the loss of nutrients. Through on-farm comparison of various nutrient management strategies, Sapkota et al. 19 reported significant improvement in the use efficiency of N and P 2 O 5 through NE based fertilizer management in wheat thereby increasing yield and profitability. In addition, a more balanced nutrition in NE might have led to increases in NUE through more vigorous plant growth and greater tolerance against biotic and abiotic stresses. In general, farmers do not apply K fertilizer resulting into imbalanced nutrients, which ultimately reduces the efficiency of other applied nutrients. Xu et al. 23 also reported increased NUE and crop yield due to NE over farmers’ practice of fertilizer management in spring maize in Northeast China.
The magnitude of the effect of NE in improving NUE thereby decreasing the use of fertilizers and/or increasing yields varied by crops, agro-ecology and farmers’ current fertilizer rates. The regional variations in N reduction and yield gains (Fig. 2 ) were largely influenced by the current level of crop intensification and yield. The net gain through NE-based fertilizer recommendations is probably due to the increased partial factor productivity of N, which can be attributed to the balanced use of nutrients in rice and wheat crops. The majority of rice and wheat farmers in the IGP and elsewhere in South Asia apply N fertilizer following blanket recommendations based upon crop response data averaged over large geographic areas 24 . Crop fertilization following such blanket recommendations results in under-fertilization in some areas and over-fertilization in others. The NE-based recommendations help to overcome this problem by considering field conditions, management practices and crop characteristics for nutrient application.
This study presented interesting results on N rate change and yield response across the agro-ecologies. Most farmers (64% of rice growers and 77% of wheat growers) realized yield increases despite the reduction in N application on both rice and wheat crops (Fig. S2 ). Only about 15% of rice growers and 12% of wheat growers experienced yield losses due to NE but with decreased N application, probably because these farmers were applying N beyond the economic optimum. Increased N application had positive yield impacts for 27% of rice and 7% of wheat growers mainly in Eastern IGP (Fig. S2 ). This shows an opportunity to close yield gaps in low-input areas by NE. Further, the percentage of N reduction by NE under high N rate in farmers’ fields (Fig. 2 , left panel) with equal yield improvement (Fig. 2 , right panel) suggests that the benefit of NE over FFP comes by both reducing N rate and improving yield in high-input areas, and mainly by increasing yield in low-input areas.
Overall, NE-based fertilizer management in rice–wheat systems can reduce N fertilizer by ca. 18% (ca. 10% in Eastern IGP and ca. 25% in Western IGP; Fig. 2 , left panel) without compromising yield (Fig. 2 , right panel). Our analysis further shows that SSNM through NE increased rice and wheat production by about 4–12% in India (Fig. 2 , right panel). These results reveal that NE-based SSNM has great potential for improving yields and NUE in rice and wheat crops to close the existing yield gaps and reduce excess N application. Moreover, efficient N fertilizer management strategies through adoption of NE-based recommendations could be one of the sustainable intensification pathways for the rice–wheat system in IGP and similar agro-ecologies in the region and beyond.
Cost implications of the NE use
This study showed that NE-based fertilizer recommendation has both positive and negative impacts on the total cost of fertilizer use. More than 50% of farmers (55% in rice and 51% in wheat) have experienced increase in total cost of fertilizer over FFP (Supplementary Fig. S3 ). This also varied across the agro-ecological zones, again mainly due to different levels of crop intensification. Many farmers in the study areas either avoid or practice low application of P 2 O 5 and K 2 O. NE balances the fertilizer use with adequate application of P 2 O 5 and K 2 O, which increased the total cost of fertilizer use despite the reduction in N application (Fig. 6 , left panel). However, we also observed that, compared to FFP, NE reduced the total fertilizer cost for many farmers (45% in rice and 49% in wheat). These farmers were already using some amount of P 2 O 5 and K 2 O, and therefore, the cost decrease came mainly from reduced N application under NE.
Most farmers experienced yield gain and, therefore, higher income from grain yield despite the increase or decrease in total fertilizer cost (Fig. 6 , right panel, and Fig. S2 ). In cases where yield gain was achieved due to increased fertilizer use, the increase in fertilizer cost was compensated by the yield gains. Few farmers in our study have received double gain i.e. decrease in fertilizer cost as well as yield gains. This is probably due to balanced fertilization with adequate application of potassium under NE-based fertilizer management that improved the NUE and increased the grain yields, thereby resulting in positive net returns. Larger revenue gain than the fertilizer cost indicates a large yield gap and huge potential to close this gap through better fertilizer management. Our results are in agreement with Xu et al. 23 who reported that increase in gross return above fertilizer cost due to NE in spring maize was mainly due to increase in grain yield.
GHG mitigation potential
This study showed there is a large potential for reducing excess N from rice and wheat fields with the use of NE-based fertilizer recommendations (Fig. 1 a). Application of N fertilizer is typically a main driver of N 2 O fluxes from rice–wheat systems 25 . The GHG emission reduction due to NE-based fertilizer management was higher in wheat than in rice (Fig. 4 ), mainly because of two reasons. Firstly, farmers generally apply higher doses of N fertilizer in wheat than in rice (Fig. 1 a) and N reduction due to NE-based fertilizer management was higher in wheat than in rice (Fig. 2 , left panel). This results in more GHG reduction in wheat. Secondly, the fertilizer-induced field emissions of N 2 O would be higher in upland crops such as wheat than in lowland crops such as rice 26 . Therefore, even with same level of fertilizer N reduction through NE, the percentage of GHG emission reduction would be higher in wheat than in rice. This emission reduction potential also varied spatially depending on the current level of fertilizer use (Fig. 4 , left panel). Reduction in both GWP as well as GHG emission intensity by NE was higher in cases where farmers were applying higher rates of N and P 2 O 5 (Fig. 4 ). This was mainly due to the reduction in fertilizer use by NE in these cases (Fig. 2 , left panel) and subsequently, the reduction in fertilizer-induced emissions of N 2 O and CO 2 .
The emission intensity in both crops decreased under NE-based recommendations (Fig. 3 , upper panel) due to the partial or combined effect of the reduction in N application and yield gain by NE (Figs. 1 and 2 ). NE reduced GHG emission intensity more in wheat than in rice (Fig. 4 , right panel). This is because NE resulted into higher reduction in N rates and higher yield increments in wheat than in rice (Fig. 2 ). Similarly, NE reduced GHG emission intensity more in Eastern IGP than in Western IGP (Fig. 4 , right panel) mainly because NE resulted in larger yield gains in Eastern IGP compared to Western IGP (Fig. 2 , right panel). These results demonstrate the importance of NE for closing yield gaps in low-input production systems. Magnitude of GHG reduction by NE in our study (ca. 2.5% in rice, and 12–20% in wheat) was lower than reported by Zhang et al. 27 (ca. 45%) from winter wheat in North-central China. This was mainly because farmers in North-Central China commonly apply higher dose of N (> 300 kg N ha −1 ) 27 , 28 . Thus, the magnitude of N reduction through adoption of NE is higher in such areas with over-fertilization and therefore higher magnitude of fertilizer-induced GHG savings.
Implications of NE-based nutrient management
Mineral fertilizers play an important role in increasing crop production and securing food security of growing population. However, excessive or imbalanced use of fertilizer not only increase the production cost to farmers but also contributes to the environmental pollution. Therefore, in intensive crop growing area such as Indian IGP, N fertilizer must be applied judiciously to balance optimum yield against the cost of fertilizer and the negative environmental effects of excess N application. Agriculture is the second largest source of GHG emissions in India, accounting for ~ 18% of gross national emissions. Identifying high-yield low-emission pathways for the country’s cereal production is key for reducing agriculture’s contribution to total GHG emissions 24 . India recently declared a voluntary goal of reducing the emission intensity of its gross domestic product by 35% over the 2005 level, by 2030 (India’s NDC submitted to UNFCCC). Reduction in excess nutrient application and balanced fertilizer use are the key mitigation options in Indian agriculture 29 , 30 . This option can contribute to reducing large amounts of GHG emissions from the agriculture sector including gains in crop yield and income for most farmers. Soil test-based fertilizer recommendations are difficult to use for smallholder farms in South Asia because of constraints such as available testing facilities, farmers’ access to such facilities, cost, and timeliness. Given the situation, a science-based, reliable and practically feasible site-specific fertilizer recommendation method is required to respond to the low NUE caused by imbalanced fertilization practices. Decision support systems (DSS) are nowadays progressively being used to facilitate application of improved nutrient management practices in the farmers’ fields.
Thus, scaling the use of NE-based SSNM can partially address the challenge of increasing food production to meet the growing food demand and reducing agricultural emissions particularly in the area where crop yield gaps and agricultural emissions are high. NE-based nutrient recommendation can be scaled-up through government extension systems and schemes (e.g. Soil Health Card Scheme of India: https://www.soilhealth.dac.gov.in/ ). Based on authors’ experience in the region, NE is easy to learn by farmers, can be used through their android cell phones. Many progressive farmers in the study area are already using NE not only on their farm but also in the farm of fellow farmers.
The implications of NE-based fertilizer management in terms of yield, N consumption and GHG emissions are tremendously high in countries like India. In 2016–2017, India produced 109.7 and 98.51 Mt of rice and wheat, respectively ( https://eands.dacnet.nic.in/ ). If our observed on-farm yield increases of rice and wheat through NE-based fertilizer management over FFP represent the total rice and wheat area in India, this will translate into the production of 8.5 and 5.4 Mt additional rice and wheat, respectively, without additional production costs. Annual N fertilizer consumption in India was about 17.4 Mt in 2016–2017 31 . Assuming 50% of this total N is used for rice and wheat production 20 , estimated N fertilizer savings due to NE-based fertilizer management in rice and wheat in India will be about 1.44 Mt with huge implications on costs and GHG savings. Through a bottom-up analysis using a large number of datasets in India, Sapkota et al. calculated fertilizer-related emissions from rice and wheat to be 558 and 775 kg CO 2 e per ha, respectively 29 . If our results of GHG emission savings of 2.5% in rice and 20% in wheat due to NE-based fertilizer management could be achieved in all rice and wheat areas in the country, this would translate into GHG savings of 5.2 Mt CO 2 e, i.e., 0.61 Mt CO 2 e from rice and 4.63 Mt CO 2 e from wheat. However, this would also increase the consumption of K 2 O with huge implications for the production and import of K fertilizer and associated costs and this trade-off warrants further study. We conducted this research both in high-input (Western IGP) and low-input (Eastern IGP) production systems in the major rice–wheat belt of India and covered a sufficiently large number of farmers (ca. 1600 pair comparisons) to make it representative of major rice–wheat growing areas in the region. Given the level of implications in terms of yields, total N, P 2 O 5 and K 2 O consumptions and GHG emissions, NE-based fertilizer management certainly merits further scientific investigation and policy consideration.
This study evaluated NE-based site-specific nutrient management vis-à-vis farmers’ fertilizer practice in rice and wheat in both high-input and low-input production systems across the rice–wheat belt of India through large numbers of on-farm comparison trials. Overall, NE-based recommendations reduced N input by 15–35%, increased grain yield by 4–8% and reduced global warming potential by 2–20%. The study also shows that NE-based SSNM is more important for closing the yield gap in low-intensive systems and decreasing nutrient input and minimizing nutrient loss high-intensive systems. Adoption of NE-based site-specific nutrient management across all rice and wheat growing areas in India would translate into additional grain production of 13.92 Mt, N consumption reduction of 1.44 Mt and total GHG savings of 5.24 Mt CO 2 e per year with some additional use of K fertilizer. In smallholder production systems, where soil testing of each field is nearly impossible, a simple decision support tool such as NE could be helpful to promote site-specific nutrient management contributing to both food security and environmental sustainability goals.
On-farm comparison trials
We conducted this study in farmers’ fields in the states of Punjab, Haryana and Bihar in India (Fig. S4 ). Punjab and Haryana represent high-input production systems typical of the Western Indo-Gangetic Plains (IGP), whereas Bihar represents relatively low-input production systems typical of the Eastern IGP. In general, intensive mechanized production makes the cereal systems of IGP GHG emission intensive. The Western IGP are characterized by semi-arid climate with mean annual rainfall varying from 544 to 970 mm. The climate in Eastern IGP is characterized by hot and humid summers and cold winters, with an average annual rainfall of 1350 mm, 70% of which falls between July to September. An overview of the agro-ecological conditions of the study sites are given in Supplementary Table 1 .
We conducted on-farm comparison trials for four years during the 2013–2014 to 2016–2017 cropping seasons. Altogether, we conducted 1594 pair comparison trials (1094 in Haryana, 245 in Punjab and 251 in Bihar), 730 trials for rice and 864 trials for wheat. Each trial had two paired plots–one with a fertilizer recommendation determined by NE and one with FFP. We used basic information about the plots, such as soil characteristics, yield and nutrients applied to previous crops, together with information about the present crop and the target yield to estimate nutrient recommendation from NE software ( http://software.ipni.net ). NE estimates the attainable yield utilizing the information provided by farmers about growing conditions, determines the nutrient balance in the cropping system based on yield and nutrients applied to previous crops, and combines such information with soil characteristics to predict the crop response to N, P and K, and generates nutrient recommendations specific to that field 16 . The plot size ranged from 1000 to 2000 m 2 in Haryana and Punjab, and from 500 to 1500 m 2 in Bihar. All practices, except fertilizer management, were similar for paired plots within the comparison trials. The participating farmers primarily managed the plots. The researchers consulted with farmers to calculate NE-based recommendations using the Nutrient Expert tool and collected relevant data from the trials.
Crop management in the field
Most farmers adopted intensive tillage practices, i.e., conventional tillage (CT: two harrowings/rotavator, two plowings using a tine cultivator, and one field leveling using a wooden plank), whereas some farmers adopted zero tillage (ZT) for growing rice and wheat. In the CT system, rice was established by transplanting 25–30-day-old seedlings in puddled (wet tillage) soil. In the CT system, wheat was established either by broadcasting the seeds in the field after land preparation or by drilling using a seed-cum-fertilizer drill. In the ZT system, both rice and wheat were seeded using a zero-till planter or a turbo Happy Seeder 32 without preparatory tillage. Depending upon water availability and farmers’ preference, some farmers kept their rice field continuously flooded, whereas some followed alternate wetting and drying cycles. In Punjab and Haryana, rice fields received 20–25 irrigations per season, whereas in Bihar, farmers applied only 4–8 irrigations depending upon rainfall. In general, wheat received four irrigations of 6–7 cm each at 20–25, 45–50, 75–80 and 110–120 days after sowing.
We calculated NE-based fertilizer recommendations using the farm management information provided by the farmers supplemented with soil and climatic condition of the field. NE-based fertilizer recommendations varied from farm to farm depending upon soil type, cropping history and management practices, whereas a farmer’s fertilizer practice was as per his/her prevailing practice. Farmers’ fertilizer management practices also varied from farm to farm depending upon farmers’ knowledge of fertilizer management, their purchasing power and so on. For both crops, the total amounts of P 2 O 5 and K 2 O and 15–20% of the N were applied as basal fertilizer using di-ammonium phosphate and muriate of potash. The remaining amount of N was top-dressed in two equal splits 20–25 and 40–50 days after seeding/transplanting using urea under both NE and FFP. Figure 1 shows the average N, P 2 O 5 and K 2 O rates under NE and FFP for both crops in both agro-ecologies and Supplementary Fig. S1 presents their pairwise distribution.
Data collection
We recorded and compiled all the management practices in each farmer’s field, such as tillage and residue management, nutrient and water management, as well as crop protection. We obtained climate information (temperature and rainfall) about the farm from the nearest agricultural science center. We obtained site specific soil data such as texture, soil organic carbon, soil pH and bulk density from the International Soil Reference and Information Centre ( https://www.isric.org/explore/soilgrids ) 33 . We also recorded the amount of fuel and electricity consumed for various farm operations during the entire crop cycle. At maturity, we recorded the grain yield (at 13% moisture content) by harvesting three 3 m 2 quadrates in each plot.
Estimation of GHG emissions and global warming potential
We estimated GHG emissions from each plot using the CCAFS Mitigation Options Tool 34 , hereafter referred to as CCAFS-MOT, which combines several empirical models to estimate GHG emissions from different land uses. The tool recognizes context specific factors that influence GHG emissions such as soil and climate, production inputs and management practices. To estimate total GHG emissions from the production systems, i.e., global warming potential (GWP), we converted all GHG emissions into CO 2 equivalents (CO 2 e) using the global warming potential (over 100 years) of 28 and 265 for CH 4 and N 2 O, respectively 35 . We then divided total GWP by grain yield to determine GHG emission intensity.
Estimation of fertilizer costs and income from crop yield
As everything except nutrient management was similar within the comparison pair, we only used the fertilizer cost and income from yield for comparison between NE and FFP. The year-wise fertilizer cost and price of grains that were used for the economic analysis is provided in Supplementary Table 2 . The fertilizer cost was estimated by using the market rate of the respective fertilizer for the respective years obtained from the Fertilizer Association of India ( https://www.faidelhi.org/ ; Supplementary Table 2 ). We calculated the income from grain yield by multiplying the total grain yield with minimum support price (MSP) for the respective years (Supplementary Table 2 ). The MSP is an agriculture product price set by the Food Corporation of India (FCI) to purchase directly from the farmers ( http://fci.gov.in ).
Statistical analysis
We conducted a paired t-test comparison of the variables of interest using Costat Software 36 . As we had pair comparisons of NE versus FFP in each farmer’s field, the paired t-test is appropriate for examining the difference in means. Once the effect of NE over FFP in terms of fertilizer rate, yield, GHG emissions, fertilizer cost and income were determined through the paired t-test, those variables were also subjected to meta-analysis to determine the influence of various agro-climatic conditions and management factors (e.g., crop types, agro-climatic zone, farmers’ fertilizer rate) on the effectiveness of NE over FFP. For this, we considered each of the on-farm comparison trials characterized by crop, location, year, soil properties, management information and so on, as a data point. All the trials within a location that were similar in the above-mentioned characteristics constituted a study (a village had one or more studies), and all pair-comparison trials within the specific study were considered replications and used to calculate the standard deviation and effect size in meta-analysis. We performed meta-analysis using MetaWin 2.1 in two stages 37 , 38 . At first, we calculated the effect size for each study as the natural log of the response ratio (lnR) using the following equation 39 :
where X NE is the mean of response variables (yield, N-rate, GHG emission intensity, global warming potential) due to NE, and X FFP is the mean of these variables in FFP. This ratio is comparable between the studies, while the logarithmic transformation ensures that variability in the ratio's denominator has no greater influence on the metric than variability in its numerator.
We then combined the effect sizes from the studies using a mixed-effect model to calculate the cumulative effect size and the 95% confidence intervals (CIs) through bootstrapping with 4999 iterations 40 . The mixed-effect model is a random-effect meta-analytic model for categorical data 37 , assuming random variation among studies within a group and fixed variation between groups. We considered the cumulative effect significant if the CIs did not overlap with zero and the effect sizes among the categories significantly different if their CIs did not overlap. For ease of interpretation, we back-transformed the results and reported them as the percentage change caused by NE in relation to FFP. We considered the difference significant only when p values were < 0.05.
Ross, K. et al. Enhancing NDCs: Opportunities in Agriculture (World Resources Institute and Oxfam, Washington DC, 2019).
Google Scholar
Carlson, K. M. et al. Greenhouse gas emissions intensity of global croplands. Nat. Clim. Change. 7 , 63–78 (2017).
Article ADS CAS Google Scholar
Kanter, D. R., Zhang, X., Mauzerall, D. L., Malyshev, S. & Shevliakova, E. The importance of climate change and nitrogen use efficiency for future nitrous oxide emissions from agriculture. Environ. Res. Lett. 11 , 094003 (2016).
Article ADS Google Scholar
IPCC. IPCC special report on climate change, desertification, land degradation, sustainable land management, food security, and greenhouse gas fluxes in terrestrial ecosystems. Summary for policymakers (Intergovernmental Panel on Climate Change, Geneva, Switzerland, 2019).
Reynolds, T. W. et al. Environmental impacts and constraints associated with the production of major food crops in Sub-Saharan Africa and South Asia. Food Secur. 7 , 795–822 (2015).
Article Google Scholar
Spiertz, J. H. J. Nitrogen, sustainable agriculture and food security: A review. Sustain. Agric. 30 , 635–651 (2009).
Garnett, T. et al. Sustainable intensification in agriculture: Premises and policies. Science 341 , 33–34 (2013).
Sutton, M. A. et al. Our nutrient world: the challenge to produce more food and energy with less pollution . (Global Overview of Nutrient Management. Centre for Ecology and Hydrology, Edinburgh on behalf of the Global Partnership on Nutrient Management and the International Nitrogen Initiative, 2013).
Good, A. G. & Beatty, P. H. Fertilizing nature: A tragedy of excess in the commons. PLoS Biol. 9 , 1–9 (2011).
Buresh, R. J. & Witt, C. Site-specific nutrient management. In Fertilizer Best Management Practices: Gneral principles, strategy for their adoption and voluntary initiatives vs regulations. Paper presented at IFA International Workshop on Fertilizer Best Management Practices, 7–9 March 2007, Brussels, Belgium (ed. IFIA) (International Fertilizer Industry Association, 2007).
Dobermann, A. & Witt, C. The evolution of site-specific nutrient management in irrigated rice systems of Asia. In Increasing productivity of intensive rice systems through site-specific nutrient management (eds. Dobermann, A., Witt, C. & Dawe, D.) 410 (Science Publisher Inc., and International Rice Research Institute (IRRI), 2004).
Shapiro, C. A. et al. Using a Chlorophyll Meter to Improve N Management (University of Nebraska-Lincoln Extension Bulletin G, Lincoln, 2013).
LCC. Leaf Color Chart (LCC). (2020). http://www.knowledgebank.irri.org/step-by-step-production/growth/soil-fertility/leaf-color-chart .
Bijay-Singh, et al. Site-specific fertilizer nitrogen management in irrigated transplanted rice ( Oryza sativa ) using an optical sensor. Precis. Agric. 16 , 455–475 (2015).
Sapkota, T. B. et al. Precision nutrient management under conservation agriculture-based cereal systems in South Asia. In Climate Change and Agricultural Development: Improving Resilience through Climate Smart Agriculture, Agroecology and Conservation (ed. Nagothu, U. S.) 322 (Taylor & Francis Group, Routledge, 2016).
Pampolino, M. F., Witt, C., Pasuquin, J. M., Johnston, A. & Fisher, M. J. Development approach and evaluation of the Nutrient Expert software for nutrient management in cereal crops. Comput. Electron. Agric. 88 , 103–110 (2012).
Xu, X. et al. Methodology of fertilizer recommendation based on yield response and agronomic efficiency for rice in China. Food Crop. Res. 206 , 33–42 (2017).
Jat, R. D. et al. Conservation agriculture and precision nutrient management practices in maize-wheat system: Effects on crop and water productivity and economic profitability. Food Crop. Res. 222 , 111–120 (2018).
Sapkota, T. B. et al. Precision nutrient management in conservation agriculture based wheat production of Northwest India: Profitability, nutrient use efficiency and environmental footprint. Food Crop. Res. 155 , 233–244 (2014).
Heffer, P., Gruere, A. & Roberts, T. Assessment of Fertilizer Use by Crop at the Global Level . International Fertilizer Association (IFA) and International Plant Nutrition Institute (IPNI) 5 , (2017).
Farnworth, C. R., Stirling, C., Sapkota, T. B., Jat, M. L. & Misiko, M. Gender and inorganic nitrogen: What are the implications of moving towards a more balanced use of nitrogen fertilizer in the tropics ?. Int. J. Agric. Sustain. 15 , 136–152 (2017).
Singh, V. K., Dwivedi, B. S., Shukla, A. K., Chauhan, Y. S. & Yadav, R. L. Diversification of rice with pigeonpea in a rice–wheat cropping system on a TypicUstochrept: Effect on soil fertility, yield and nutrient use efficiency. Food Crop. Res. 92 , 85–105 (2005).
Xu, X. et al. Narrowing yield gaps and increasing nutrient use efficiencies using the Nutrient Expert system for maize in Northeast China. Food Crop. Res. 194 , 75–82 (2016).
Sapkota, T. B. et al. Identifying optimum rates of fertilizer nitrogen application to maximize economic return and minimize nitrous oxide emission from rice–wheat systems in the Indo-Gangetic Plains of India. Arch. Agron. Soil Sci. 00 , 1–16 (2020).
CAS Google Scholar
Reay, D. S. et al. Global agriculture and nitrous oxide emissions. Nat. Clim. Change 2 , 410–416 (2012).
Albanito, F. et al. Direct nitrous oxide emissions from tropical and sub-tropical agricultural systems—A review and modelling of emission factors. Sci. Rep. 7 , 1–12 (2017).
Zhang, J. J. et al. Nutrient expert improves nitrogen efficiency and environmental benefits for winter wheat in China. Agron. J. 110 , 696–706 (2018).
Article CAS Google Scholar
Cui, Z. et al. On-farm evaluation of an in-season nitrogen management strategy based on soil Nmin test. Food Crop. Res. 105 , 48–55 (2008).
Sapkota, T. B. et al. Cost-effective opportunities for climate change mitigation in Indian agriculture. Sci. Total Environ. 655 , 1342–1354 (2019).
Bordoloi, N., Baruah, K. K. & Hazarika, B. Fertilizer management through coated urea to mitigate greenhouse gas (N 2 O) emission and improve soil quality in agroclimatic zone of Northeast India. Environ. Sci. Pollut. Res. 27 , 11919–11931 (2020).
Tewatia, R. K. & Chanda, T. K. Trends in fertilizer nitrogen proudction and consumption in India. in The Indian Nitrogen Assessment: Sources of Reactive Nitrogen, Environmental and Climate Effects, Management Options and Policies (eds. Abrol, Y. P. et al. ) 45–56 (Woodhead Publishing, Duxford, United Kingdom, 2017).
Chapter Google Scholar
Sidhu, H. S. et al. Development and evaluation of the Turbo Happy Seeder for sowing wheat into heavy rice residues in NW India. Food Crop. Res. 184 , 201–212 (2015).
Hengl, T. et al. SoilGrids250m: Global gridded soil information based on machine learning. PLoS ONE 12 , e0169748 (2017).
Feliciano, D., Nayak, D. R., Vetter, S. H. & Hillier, J. CCAFS-MOT—A tool for farmers, extension services and policy-advisors to identify mitigation options for agriculture. Agric. Syst. 154 , 100–111 (2017).
IPCC. Climate Change 2013. The Physical Science Basis. Working Group I contribuiton to the Fifth Assessment Report of the Intergovernmental Panel on Climate Change. Chapter 8: Anthropogenic and Natural RAdiative Forcing. Intergovernmental Panel on Climate Chang . (2013).
CoHort, S. CoHort Software. Monterey, CA. USA (2017). http://www.cohort.com . Accessed 17 July 2017.
Rosenberg, M. S., Adams, D. C. & Gurevitch, J. MetaWin: Statistical Software for Meta-analysis. Version 2.0 (Sinauer Associates, Sunderland, 2000).
Chakraborty, D. et al. A global analysis of alternative tillage and crop establishment practices for economically and environmentally efficient rice production. Sci. Rep. 7 , 1–11 (2017).
Hedges, L. V., Gurevitch, J. & Curtis, P. S. The meta-analysis of response ratios in experimental ecology. Ecology 80 , 1150–1156 (1999).
Adams, D. C., Gurevitch, J. & Rosenberg, S. Resampling tests for meta-analysis of ecological data. Ecology 78 , 1277–1283 (1997).
Download references
Acknowledgements
This work was carried out by the International Maize and Wheat Improvement Center (CIMMYT) in collaboration with farmers, and funded by the CGIAR research programs (CRPs) on Climate Change, Agriculture and Food Security (CCAFS) & Wheat Agri-Food System (WHEAT) and the Indian Council of Agricultural Research (ICAR) under window 3. CCAFS’ work is supported by CGIAR Fund Donors and through bilateral funding agreements. For details please visit https://ccafs.cgiar.org/donors . The views expressed in this paper cannot be taken to reflect the official opinions of these organizations. The dataset associated with this manuscript will be available together with the supplementary materials of this manuscript.
Author information
Authors and affiliations.
International Maize and Wheat Improvement Center (CIMMYT), El Batan, Mexico
Tek B. Sapkota
International Maize and Wheat Improvement Center (CIMMYT), New Delhi, India
Mangi L. Jat, Kailash Kalvaniya, Gokul Prasad & Munmun Rai
International Rice Research Institute (IRRI), NASC Complex, New Delhi, 110012, India
Dharamvir S. Rana
CGIAR Research Program on Climate Change, Agriculture and Food Security (CCAFS), CIAT-Bioversity Alliance, Cali, Colombia
Arun Khatri-Chhetri
ICAR-Central Soil Salinity Research Institute (CSSRI), Karnal, Haryana, India
Hanuman S. Jat
International Maize and Wheat Improvement Center (CIMMYT), CSSRI, Karnal, India
Deepak Bijarniya
CCS Haryana Agriculture University, Hisar, Haryana, India
Jhabar M. Sutaliya
International Maize and Wheat Improvement Center (CIMMYT), Borlaug Institute for South Asia (BISA), Ludhiana, Punjab, India
Manish Kumar, Love K. Singh & Harminder S. Sidhu
International Maize and Wheat Improvement Center (CIMMYT), Borlaug Institute for South Asia (BISA), Pusa, Samastipur, Bihar, India
International Plant Nutrition Institute (IPNI), Gurgaon, Haryana, 122001, India
T. Satyanarayana
African Plant Nutrition Institute (APNI), & Univerśite Mohammed VI Polytechnique, Benguérir, Morocco
Kaushik Majumdar
You can also search for this author in PubMed Google Scholar
Contributions
M.L.J. and T.B.S. conceptualized the idea. M.L.J designed the experiment and coordinated field work. H.S.J., D.B., J.M.S., M.K., L.K.S., R.K.J., K.K., H.S.S., implemented on-farm trials and collected data, T.B.S., D.S.R. and G.P. analyzed the data. T.B.S. and A.K.C. drafted the manuscript. All authors reviewed the manuscript and contributed.
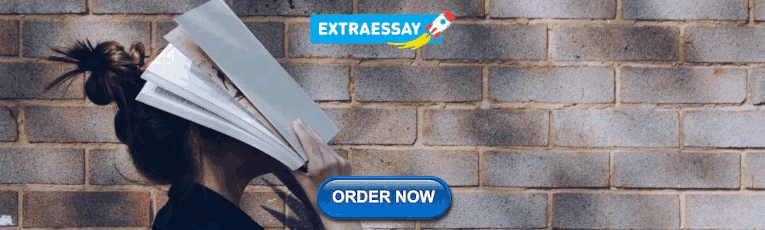
Corresponding author
Correspondence to Mangi L. Jat .
Ethics declarations
Competing interests.
The authors declare no competing interests.
Additional information
Publisher's note.
Springer Nature remains neutral with regard to jurisdictional claims in published maps and institutional affiliations.
Supplementary Information
Supplementary information., rights and permissions.
Open Access This article is licensed under a Creative Commons Attribution 4.0 International License, which permits use, sharing, adaptation, distribution and reproduction in any medium or format, as long as you give appropriate credit to the original author(s) and the source, provide a link to the Creative Commons licence, and indicate if changes were made. The images or other third party material in this article are included in the article's Creative Commons licence, unless indicated otherwise in a credit line to the material. If material is not included in the article's Creative Commons licence and your intended use is not permitted by statutory regulation or exceeds the permitted use, you will need to obtain permission directly from the copyright holder. To view a copy of this licence, visit http://creativecommons.org/licenses/by/4.0/ .
Reprints and permissions
About this article
Cite this article.
Sapkota, T.B., Jat, M.L., Rana, D.S. et al. Crop nutrient management using Nutrient Expert improves yield, increases farmers’ income and reduces greenhouse gas emissions. Sci Rep 11 , 1564 (2021). https://doi.org/10.1038/s41598-020-79883-x
Download citation
Received : 18 June 2020
Accepted : 09 December 2020
Published : 15 January 2021
DOI : https://doi.org/10.1038/s41598-020-79883-x
Share this article
Anyone you share the following link with will be able to read this content:
Sorry, a shareable link is not currently available for this article.
Provided by the Springer Nature SharedIt content-sharing initiative
This article is cited by
Diverse nutrient management strategies for achieving a sustainable energy-food-environment nexus in rice-rice production systems.
- Saheed Garnaik
- Prasanna Kumar Samant
- Hari Sankar Nayak
Environment, Development and Sustainability (2024)
A bibliographic review of climate change and fertilization as the main drivers of maize yield: implications for food security
- Akasairi Ocwa
- Endre Harsanyi
- Safwan Mohammed
Agriculture & Food Security (2023)
Spatially differentiated nitrogen supply is key in a global food–fertilizer price crisis
- Sieglinde Snapp
- Tek Bahadur Sapkota
- Bram Govaerts
Nature Sustainability (2023)
Nutrient Use Efficiency and Greenhouse Gas Emissions Affected by Fertilization and Farmyard Manure Addition in Rice–Wheat System
- Jagdeep-Singh
- Shahida Nisar
International Journal of Plant Production (2023)
Foliar nutrient supplementation with micronutrient-embedded fertilizer increases biofortification, soil biological activity and productivity of eggplant
- Ram Swaroop Bana
- Gograj Singh Jat
- Teekam Singh
Scientific Reports (2022)
By submitting a comment you agree to abide by our Terms and Community Guidelines . If you find something abusive or that does not comply with our terms or guidelines please flag it as inappropriate.
Quick links
- Explore articles by subject
- Guide to authors
- Editorial policies
Sign up for the Nature Briefing: Anthropocene newsletter — what matters in anthropocene research, free to your inbox weekly.

Soil Fertility, Integrated Management, and Sustainability
- Reference work entry
- First Online: 25 November 2020
- Cite this reference work entry
- Golda A. Edwin 6 &
- Nakkeeran Muthu 6
Part of the book series: Encyclopedia of the UN Sustainable Development Goals ((ENUNSDG))
130 Accesses
Definitions
According to the Soil Science Society of America ( 2019 ), soils are nothing but a complex mixture of decaying remains of once-living things. It is a product of complex interactions between several factors: that includes atmosphere, biosphere, climate, terrain, flora, fauna, and organic and inorganic materials interacting over time. The soil is considered an ecosystem and is composed of materials distributed in either solid, liquid, or gaseous forms that changes continually by way of physical, chemical, and biological processes (Baillie 2001 ; Lavelle and Spain 2001 ).
It takes 1000s of years for its natural formation and is also considered the “skin of the earth,” vital for sustaining all life forms. Soil fertility is the ability of the soil to provide plant habitat and sustain agricultural plant growth.
According to the Food and Agriculture Organization of the United Nations (FAO 2017 ), soil fertility is a component of soil productivity that deals with the capacity to...
This is a preview of subscription content, log in via an institution to check access.
Access this chapter
- Available as PDF
- Read on any device
- Instant download
- Own it forever
- Available as EPUB and PDF
- Durable hardcover edition
- Dispatched in 3 to 5 business days
- Free shipping worldwide - see info
Tax calculation will be finalised at checkout
Purchases are for personal use only
Institutional subscriptions
Abbott LK, Murphy DV (eds) (2003) Soil biological fertility: a key to sustainable land use in agriculture. Springer Science & Business Media, Berlin, Germany
Google Scholar
Adams JE (1966) Influence of mulches on runoff, erosion, and soil moisture depletion. Soil Sci Soc Am J 30(1):110–114. https://doi.org/10.2136/sssaj1966.03615995003000010036x
Article Google Scholar
Adhikari K, Hartemink AE (2016) Linking soils to ecosystem services – a global review. Geoderma 262:101–111
Article CAS Google Scholar
Allison FE (1957) Nitrogen and soil fertility, soils, yearbook of agriculture. United States Department of Agriculture, USA, pp 85–94
Ameeta S, Ronak C (2017) A review on the effect of organic and chemical fertilizers on plants. Int J Res Appl Sci Eng Technol 5(2):677–680
Arcurs C (2017) Safeguarding our soils. Nat Commun. https://doi.org/10.1038/s41467-017-02070-6
Baillie IC (2001) Soil survey staff 1999, soil taxonomy: a basic system of soil classification for making and interpreting soil surveys, agricultural handbook 436, Natural Resources Conservation Service, USDA, Washington DC, USA, pp. 869. Soil Use Manag 17(1):57–60
Baumhardt RL, Blanco-Canqui H (2014) Soil conservation practices. Enc Agric Food Syst 5:153–165
Bhattacharya A (2018) Changing Climate and Resource use Efficiency in Plants. Academic Press, London, UK
Bitew Y, Alemayehu M (2017) Impact of crop production inputs on soil health: a review. Asian J Plant Sci 16(3):109–131
Brundtland Commission Report (1987). Retrieved from https://sustainabledevelopment.un.org/content/documents/5987our-common-future.pdf Accessed on 10 Aug 2019
Cairns I, Handyside B, Harris M, Lambreschtsen N, Ngapo N (2001) Soil conservation technical handbook. The Ministry for the Environment, Wellington. Available at: https://www.mfe.govt.nz/sites/default/files/soil-conserv-handbook-jun01.pdf . Accessed 30 Aug 2019
California Environmental Protection Agency (2009) Soil toxicity and bioassessment test methods for ecological risk assessment. Office of Environmental Health Hazard Assessment. https://oehha.ca.gov/media/downloads/ecotoxicology/document/soiltox120208.pdf . Accessed 26 Sept 2019
Cameron KC, Di HJ, Moir JL (2013) Nitrogen losses from the soil/plant system: a review. Ann Appl Biol 162(2):145–173
De Vries W, Kros J, Dolman MA, Vellinga TV, De Boer HC, Gerritsen AL et al (2015) Environmental impacts of innovative dairy farming systems aiming at improved internal nutrient cycling: a multi-scale assessment. Sci Total Environ 536:432–442
Diacono M, Montemurro F (2010) Long-term effects of organic amendments on soil fertility. A review. Agron Sustain Dev 30:401–422
Dolman MA, Sonneveld MPW, Mollenhorst H, de Boer IJM (2014) Benchmarking the economic, environmental and societal performance of Dutch dairy farms aiming at internal recycling of nutrients. J Clean Prod 73:245–252. https://doi.org/10.1016/j.jclepro.2014.02.043
European Commission (EC) (2006) Communication from the Commission to the Council, the European Parliament, the European Economic, and Social Committee and the Committee of the Regions, Thematic Strategy for Soil Protection, COM 231 Final, Brussels
FAO (2015) The food and agriculture organization of the United Nations. The state of the world’s land and water resources for food and agriculture (SOLAW) – managing systems at risk. Food and Agriculture Organization of the United Nations/Earthscan, Rome/London, 2011. Available online http://www.fao.org/docrep/017/i1688e/i1688e.pdf . Accessed on 15 Sept 2015
FAO (2017) Food and agricultural organization of the United Nations. Africa regional overview of food security and nutrition. http://www.fao.org/publications/card/en/c/a0745c69-2412-4f56-afa9-6b011d2e0b71/
Ge S, Zhu Z, Jiang Y (2018) Long-term impact of fertilization on soil pH and fertility in an apple production system. J Soil Sci Plant Nutr 18(1):282–293. Online ISSN 0718-9516
Godfray HCJ, Beddington JR, Crute IR, Haddad L, Lawrence D, Muir JF et al (2010) Food security: the challenge of feeding 9 billion people. Science 327(5967):812–818
Green BW (2015) Fertilizers in aquaculture. In: Feed and feeding practices in aquaculture (2015). Food science, technology and nutrition. Woodhead Publishing Series, United Kingdom, pp 27–52
Gruhn P, Goletti F, Yudelman M (2000) Integrated nutrient management, soil fertility, and sustainable agriculture: current issues and future challenges. Food, agriculture, and the environment discussion paper 32. International Food Policy Research Institute, Washington, DC
Haight JM (1971) Franklin D. Roosevelt and a naval quarantine of Japan 1937. Pac Hist Rev 40(2):203–226
Henry B, Murphy B, Cowie A (2018) Sustainable land management for environmental benefits and food security; a synthesis report for the GEF. Global Environmental Facility, Washington, DC
IFSC (2000) International farmers suicide crisis. http://www.un.org/esa/sustdev/csd/csd16/PF/presentations/farmers_relief.pdf . Accessed 2 July 2018
Karmakar R, Das I, Dutta D, Rakshit A (2016) Potential effects of climate change on soil properties: a review. Sci Int 4(2):51–73
Kaur S, Kumar V, Chawla M, Cavallo L, Poater A, Upadhyay N (2017) Pesticides curbing soil fertility: effect of Complexation of free metal ions. Front Chem 5:1–9
Keesstra SD, Bouma J, Wallinga J, Tittonell P, Smith P, Cerdà A et al (2016) The significance of soils and soil science towards realization of the United Nations sustainable development goals. Soil 2(2):111–128
Laudicina VA, Novara A, Barbera V, Egli M, Badalucco L (2015) Long-term tillage and cropping system effects on chemical and biochemical characteristics of soil organic matter in a Mediterranean semiarid environment. Land Degrad Dev 26(1):45–53
Lavelle P, Spain AV (2001) Soil Ecology. Kluwer Academic Publishers, The Netherlands
Lejcuś K, Dąbrowska J, Garlikowski D, Kordas L (2015) Water loss from soil and water absorbing geocomposite. https://doi.org/10.7763/IPCBEE.2015.V84.21
Mahid A-K (2002) Soil erosion: effect on soil productivity ICM News Archive. https://crops.extension.iastate.edu/soil-erosion-effect-soil-productivity . Accessed 23 Sept 2019
Massah J, Azadegan B (2016) Effect of chemical fertilizers on soil compaction and degradation. Agric Mech Asia Afr Lat Am 47(1):44–50
Maximillian J, Brusseau ML, Glenn EP, Matthias AD (2019) Pollution and environmental perturbations in the global system. In: Brusseau ML, Pepper IL, Gerba CP (eds) Environmental and pollution science, 3rd edn. Academic, pp 457–476. https://doi.org/10.1016/B978-0-12-814719-1.00025-2
Meena H, Sharma R, Sankhyan N, Sepehya S (2017) Effect of continuous application of fertilizers, farm yard manure and lime on soil fertility and productivity of maize-wheat system in an acid Alfisol. Commun Soil Sci Plant Anal 48. https://doi.org/10.1080/00103624.2017.1373800
Montgomery HL (2010) How is soil made? Crabtree Publishing Company, New York
Murdock LW, Frye WW (1985) Erosion: its effect on soil properties, productivity and profit. AGR-University of Kentucky, Cooperative Extension Service, USA
Nathanail P (2011) Sustainable remediation: Quo vadis?. Rem J 21(4):35–44
Neina D (2019) The role of soil pH in plant nutrition and soil remediation. Appl Environ Soil Sci. https://doi.org/10.1155/2019/5794869
Nguemezi C, Tematio P, Yemefack M, Tsozue D, Silatsa T (2020) Soil quality and soil fertility status in major soil groups at the Tombel area, south-West Cameroon. Heliyon 6(2). https://doi.org/10.1016/j.heliyon.2020.e03432
Nield D (2017) Good news: soil could be a much larger carbon sink than we hoped. https://www.sciencealert.com/soil-minerals-could-be-huge-carbon-dioxide-sink . Accessed 2 July 2018
Novara A, Pisciotta A, Minacapilli M, Maltese A, Capodici F, Cerdà A, Gristina L (2018) The impact of soil erosion on soil fertility and vine vigor. A multidisciplinary approach based on field, laboratory and remote sensing approaches. Sci Total Environ:622, 474–623, 480. https://doi.org/10.1016/j.scitotenv.2017.11.272
Nugumbi E (2017) Continuing the wins in soil health restoration in Africa. World Policy Blog. http://www.worldpolicy.org/blog/2017/02/14/continuing-wins-soil-health-restoration-africa
Osman KT (2013) Soil resources and soil degradation. In: Soils. Springer, Dordrecht, pp 175–213
Chapter Google Scholar
Osman KT (2014) Physical deterioration of soil. In: Soil degradation, conservation and remediation. Springer, Dordrecht, pp 45–67
Panpatte D, Jhala Y (2019) Soil fertility management for sustainable development. Springer, Singapore
Book Google Scholar
Pereira P, Brevik EC, Oliva M, Estebaranz F, Depellegrin D, Novara A, Cerdà A, Menshov O (2017) Goal-oriented soil mapping: applying modern methods supported by local knowledge. In: Pereira P, Brevik EC, Muñoz-Rojas M, Miller BA (eds) Soil mapping and process modeling for sustainable land use management. Elsevier, pp 61–83. https://doi.org/10.1016/B978-0-12-805200-6.00003-7
Pimentel D, Burgess M (2013) Soil erosion threatens food production. Agriculture 3:443–463. https://doi.org/10.3390/agriculture3030443
Prashar P, Shah S (2016) Impact of fertilizers and pesticides on soil microflora in agriculture. Sustain Agric Rev 19:331–362. https://doi.org/10.1007/978-3-319-26777-7_8
Rajesh Kumar Mishra, Mohammad N, Royachoudhury N (2016) Soil pollution: causes, effects and control, vol 3(1). Tropical Forest Research Institute, Jabalpur
Rekacewicz P ( 2008) Global soil degradation. UNEP/GRID-Arendal – From collection: IAASTD – International assessment of agricultural science and technology for development. Available online http://www.grida.no/graphicslib/detail/global-soil-degradation_9aa7 . Accessed 15 Nov 2015
Rodríguez-Eugenio N, McLaughlin M, Pennock D (2018) Soil pollution: a hidden reality. FAO, Rome. 142 pp
Sanderman J, Hengl T, Fiske GJ (2017) Soil carbon debt of 12,000 years of human land use. Proc Natl Acad Sci 114(36):9575–9580
SSSA (2019) Soils overview. Retrieved from https://www.soils.org/files/about-soils/soils-overview.pdf . Accessed 10 Aug 2019
Tibbett M, Gil-Martínez M, Fraser T, Green ID, Duddigan S, De Oliveira VH, Raulund-Rasmussen K, Sizmur T, Diaz A (2019) Long-term acidification of pH neutral grasslands affects soil biodiversity, fertility and function in a heathland restoration. Catena 180:401–415. https://doi.org/10.1016/j.catena.2019.03.013
Tóth G, Hermann T, da Silva MR, Montanarella L (2018) Monitoring soil for sustainable development and land degradation neutrality. Environ Monit Assess 190(2):57
Trophyhunter (2015) How does pH affect soil fertility and how is this related to plant growth? eNotes Editorial, https://www.enotes.com/homework-help/how-ph-affects-soil-fertility-how-this-related-579022 . Accessed 23 Sept 2019
USDA (2019) Healthy soil for life. Retrieved from https://www.nrcs.usda.gov/wps/portal/nrcs/main/soils/health/ . Accessed 10 Aug 2019
Weingarten D (2017) Why are America’s farmers killing themselves in record numbers. The Guardian, 6, 2017. https://www.theguardian.com/us-news/2017/dec/06/why-are-americas-farmers-killing-themselves-in-record-numbers. Accessed 02 July 2018
World Health Organization (2018) Joint child malnutrition estimate: levels and trends (2017 edition). http://www.who.int/nutgrowth
Zhang YY, Wu W, Liu H (2019) Factors affecting variations of soil pH in different horizons in hilly regions. PLoS One 14(6), e0218563.
Download references
Author information
Authors and affiliations.
Association for Promoting Sustainability in Campuses and Communities (APSCC), Puducherry, India
Golda A. Edwin & Nakkeeran Muthu
You can also search for this author in PubMed Google Scholar
Corresponding author
Correspondence to Golda A. Edwin .
Editor information
Editors and affiliations.
European School of Sustainability Science and Research, Hamburg University of Applied Sciences, Hamburg, Germany
Walter Leal Filho
Center for Neuroscience and Cell Biology, Institute for Interdisciplinary Research, University of Coimbra, Coimbra, Portugal
Anabela Marisa Azul
Faculty of Engineering and Architecture, Passo Fundo University, Passo Fundo, Brazil
Luciana Brandli
HAW Hamburg, Hamburg, Germany
Amanda Lange Salvia
University of Chester, Chester, UK
Section Editor information
Green Campus Initiatives, Waste Management Rules & Green Protocol Compliance, Pondicherry University, Puducherry, India
Nandhivarman Muthu
Center for Food Security Studies, College of Development Studies, Addis Ababa University, Addis Ababa, Ethiopia
Desalegn Yayeh Ayal
Rights and permissions
Reprints and permissions
Copyright information
© 2021 Springer Nature Switzerland AG
About this entry
Cite this entry.
Edwin, G.A., Muthu, N. (2021). Soil Fertility, Integrated Management, and Sustainability. In: Leal Filho, W., Azul, A.M., Brandli, L., Lange Salvia, A., Wall, T. (eds) Life on Land. Encyclopedia of the UN Sustainable Development Goals. Springer, Cham. https://doi.org/10.1007/978-3-319-95981-8_11
Download citation
DOI : https://doi.org/10.1007/978-3-319-95981-8_11
Published : 25 November 2020
Publisher Name : Springer, Cham
Print ISBN : 978-3-319-95980-1
Online ISBN : 978-3-319-95981-8
eBook Packages : Earth and Environmental Science Reference Module Physical and Materials Science Reference Module Earth and Environmental Sciences
Share this entry
Anyone you share the following link with will be able to read this content:
Sorry, a shareable link is not currently available for this article.
Provided by the Springer Nature SharedIt content-sharing initiative
- Publish with us
Policies and ethics
- Find a journal
- Track your research
EDITORIAL article
Editorial: integrated nutrients management: an approach for sustainable crop production and food security in changing climates.
- 1 Department of Agronomy, Faculty of Crop Production Sciences, The University of Agriculture, Peshawar, Pakistan
- 2 Faculty of Agriculture, The University of Zagreb, Zagreb, Croatia
- 3 Department of Biological sciences, Al Hussein Bin Talal University Ma’an, Ma’an, Jordan
Editorial on the Research Topic Integrated nutrients management: an approach for sustainable crop production and food security in changing climates
Introduction
In an era of shifting climates and evolving agricultural paradigms, the need for sustainable approaches to crop production and food security has become paramount. This Research Topic, titled “Integrated Nutrients Management for Sustainable Crop Production and Food Security in Changing Climates,” presents a collection of pioneering research that addresses the intricate relationship between INM, soil health, and global agricultural sustainability in the context of changing climates ( Amanullah, 2017 ; Amanullah and Fahad, 2018 ). The issue has been meticulously curated under the editorial guidance of Amanullah, Gabrijel Ondrasek, and Abdel Rahman Al-Tawaha, with the aim of contributing to the advancement of agriculture in a changing world by emphasizing the critical role of INM in increasing crop productivity, reducing fertilizer costs, and solving food security challenges.
INM and soil health
Integrated Nutrient Management (INM) plays a pivotal role in enhancing soil health, particularly in the context of changing climates. INM's holistic approach involves the synergistic use of organic, chemical, and bio-fertilizers. By maintaining a balanced nutrient profile and fostering beneficial microbial communities in the soil, INM contributes to improved soil structure and fertility ( FAO and ITPS, 2016 ). This is essential for adapting to climate variability, as healthier soils are better equipped to withstand extreme weather events and support sustained crop production ( Krasilnikov et al., 2022 ).
INM and crop productivity
INM is intrinsically linked to increased crop productivity, a critical aspect of food security in a changing climate. By optimizing nutrient availability to crops, INM ensures that plants receive the essential elements they need for growth and development ( Amanullah et al., 2019a ; Amanullah et al., 2019b ). The judicious use of organic matter, bio-fertilizers, and targeted nutrient applications enhances crop yield and resilience, ultimately leading to higher agricultural productivity. This boost in productivity is vital to meet the growing global demand for food while mitigating the impacts of climate change on crop production.
INM and costs of chemical fertilizers
One of the primary benefits of INM is its potential to reduce the reliance on expensive chemical fertilizers. With climate change exacerbating resource constraints and increasing fertilizer costs, the adoption of INM practices can be cost-effective for farmers ( Amanullah et al., 2020 ; Amanullah et al., 2021 ). By incorporating organic materials and bio-fertilizers, INM allows for more efficient nutrient utilization, minimizing the need for excessive chemical inputs. This not only lowers production costs but also promotes sustainable agricultural practices, which are essential in a changing climate ( Khan et al., 2022 ; Imran and Amanullah, 2023 ).
INM and food security
Food security is inextricably linked to INM, especially in the face of climate change-induced challenges. INM practices contribute to higher crop yields, ensuring a stable food supply ( Khalid et al., 2022 ). By maintaining soil fertility, INM helps safeguard agricultural productivity against climate-related shocks, such as droughts and floods. Additionally, the sustainable nature of INM reduces the environmental impact of agriculture, preserving ecosystems and safeguarding the long-term availability of food resources ( Nadia et al., 2023 ).
INM and climate change adaptation
As climate change alters temperature and precipitation patterns, INM emerges as a valuable tool for climate change adaptation in agriculture. INM practices help crops better withstand environmental stressors like heat and water scarcity by enhancing their resilience ( Krasilnikov et al., 2022 ). Moreover, the reduced carbon footprint associated with INM aligns with global efforts to mitigate climate change. By sequestering carbon in soils and reducing greenhouse gas emissions from synthetic fertilizers, INM contributes to a more sustainable and climate-resilient agricultural system ( FAO and ITPS, 2016 ).
These integrated approaches are essential for addressing the complex challenges posed by changing climates and ensuring a resilient and food-secure future.
Articles and insights
This Research Topic features 12 articles that collectively illuminate various dimensions of INM’s impact on sustainable agriculture. The diverse research contributions delve into vital aspects of agricultural sustainability, exploring novel solutions that extend from nano-technological interventions to organic waste management.
Here are insights from all 12 articles published in the Research Topic on Integrated Nutrient Management (INM), along with their relevance to soil health, crop productivity, lower costs of chemical fertilizers, food security, and climate change, while also including information related to yield and food security:
Eco-friendly disease management
Khan et al. demonstrate the potential of eco-friendly IONPs synthesized from M. spicata in combatting late blight disease. This research offers an eco-conscious approach to disease control, reducing yield losses and contributing to food security. It also aligns with sustainable agriculture by minimizing chemical pesticide usage and preserving soil health.
Enhancing manure quality
Holatko et al. explore co-composting cattle manure with biochar and elemental sulfur. This strategy not only enhances soil microbiological characteristics but also improves manure quality. Better-quality manure means improved nutrient content for crops, higher yields, and reduced reliance on synthetic fertilizers, contributing to food security.
Transitioning to biological fertilizers
Abdo et al. advocate for shifting from chemical fertilizers to biological fertilizers and growth stimulants. Their research underscores the positive impact of these alternatives on crop yield and soil health, promoting higher productivity and food security while reducing environmental impact.
Alternative fertilization
Dombinov et al. investigate sugarcane bagasse ash (SCBA) as a potential fertilizer for soybeans. SCBA's nutrient-rich composition offers a sustainable alternative to traditional fertilizers, reducing costs, and ensuring a stable yield, contributing to food security.
Flooding stress tolerance
Yijun et al. explore strategies to enhance flooding stress tolerance in soybean. This research provides insights into mitigating climate-induced challenges, ensuring crop productivity, and safeguarding food security even in adverse environmental conditions.
Precision agriculture
Li et al. introduce unmanned aerial vehicle (UAV) multispectral imaging for efficient plant nutrient deficiency diagnosis. This technology enhances precision agriculture practices, optimizing nutrient management, and crop yield, ultimately supporting food security.
Phosphorus and manure integration
Jamal et al. emphasize the integrated use of phosphorus fertilizer and farmyard manure for improving wheat productivity. This approach can reduce reliance on synthetic phosphorus fertilizers, lower production costs, and ensure a stable wheat yield, contributing to food security.
Nanomaterials in agriculture
Hammerschmiedt et al. explore the effects of graphene oxide and elemental nano-sulfur on soil biological properties and lettuce plant biomass. This research showcases the potential of nanomaterials in sustainable agriculture, with implications for soil health, higher crop productivity, and food security.
Organic manure alternatives
Lee et al. highlight the value of Hanwoo manure as an organic alternative to chemical fertilizers. This research offers a sustainable pathway to maintaining soil fertility, increasing crop yield, and ensuring food security while reducing the environmental impact.
Tillage and mowing strategies
Du et al. uncover the impact of tillage methods and mowing time on Cyperus esculentus cultivation. This study provides insights into sustainable practices for specific crops, which are critical for climate-resilient agriculture, higher yields, and food security.
Improving soil microbial communities
Guo et al. focus on the transformative role of biochar from organic waste in enhancing soil fertility and citrus growth on acid red soil. Improved soil health and fertility contribute to increased crop yield and food security, while the use of organic waste aligns with sustainability.
Alleviating root rot
Tagele et al. investigate cow dung's potential as a soil amendment to alleviate ginseng root rot. By enhancing soil health and crop resilience, this research supports higher yields and food security in the face of changing climates.
These insights collectively underscore the multidimensional benefits of INM, ranging from improved soil health and higher crop productivity to reduced costs, enhanced food security, and resilience to climate change. Adopting INM practices is a crucial step towards achieving sustainable agriculture and ensuring a stable food supply in a changing world.
Conclusion and future prospects
This Research Topic underscores the pivotal role of INM in promoting sustainable agriculture, improving soil health, and ensuring global food security in the context of changing climates. The findings collectively support the notion that INM is a fundamental strategy to enhance soil fertility, health, and resilience, which is crucial in the face of climate variability. As research continues to evolve, precision agriculture technologies, genetic diversity preservation, and innovative practices promise to shape a future where sustainable agriculture is the cornerstone of food security, even amidst the challenges posed by changing climates.
Author contributions
A: Writing – original draft, Writing – review & editing. GO: Writing – review & editing. AA: Writing – review & editing.
Acknowledgments
We would like to extend our sincere gratitude to Frontier in Plant Science for entrusting us with the responsibility of guest editing the Research Topic titled “Integrated Nutrients Management for Sustainable Crop Production and Food Security: A Frontier in Changing Climates.” Our heartfelt thanks also go to the authors, reviewers, and contributors who have made this Research Topic a valuable and insightful resource.
Conflict of interest
The authors declare that the research was conducted in the absence of any commercial or financial relationships that could be construed as a potential conflict of interest.
Publisher’s note
All claims expressed in this article are solely those of the authors and do not necessarily represent those of their affiliated organizations, or those of the publisher, the editors and the reviewers. Any product that may be evaluated in this article, or claim that may be made by its manufacturer, is not guaranteed or endorsed by the publisher.
Amanullah. (2017). “Integrated use of organic carbon, plant nutrients and bio-fertilizers is key to improve field crops productivity under arid and semiarid climates,” in FAO (2017) Proceedings of the global symposium on soil organic carbon 2017 (Rome, Italy: Food and Agriculture Organization of the United Nations), 480–481.
Google Scholar
Amanullah, Fahad, S. (2018). “Integrated nutrient management in corn production: symbiosis for food security and grower’s income in arid and semiarid climates,” in Corn - production and human health in changing climate . Eds. Amanullah, Fahad, S. (London, UK: InTech), 03–12.
Amanullah, A., Inamullah, Alkahtani, J., Elshikh, M. S., Alwahibi, M. S., Muhammad, A., et al. (2020). Phosphorus and zinc fertilization improve productivity and profitability of rice cultivars under rice-wheat system. Agronomy 10, 1085. doi: 10.3390/agronomy10081085
CrossRef Full Text | Google Scholar
Amanullah, Khan, A., Khalid, S., Shah, A., Parmar, B., Muhammad, A., et al. (2019a). Integrated management of phosphorus, organic sources, and beneficial microbes improve dry matter partitioning of maize. Commun. Soil Sci. Plant Anal. 50 (20), 2544–2569. doi: 10.1080/00103624.2019.1667378
Amanullah, A., Khalid, S., Imran, Ali Khan, H., Arif, M., Altawaha, A. R., et al. (2019b). “Organic matter management in cereals based system: symbiosis for improving crop productivity and soil health,” in Sustainable agriculture reviews-29 , vol. 29 . Eds. Lal, R., Francaviglia, R. (Cham: Springer), 67–92.
Amanullah, Khalid, S., Muhammad, A., Yar, M., Elshikh, M. S., Akram, H. M., et al. (2021). Integrated use of biofertilizers with organic and inorganic phosphorus sources improve dry matter partitioning and yield of hybrid maize, Comm. Soil Sci. Plant Anal. 52 (21), 2732–2747. doi: 10.1080/00103624.2021.1956520
FAO, ITPS (2016). Voluntary guidelines for sustainable soil management (VGSSM) (Rome, Italy: Global Soil Partnership. FAO).
Imran, Amanullah. (2023). Integration of peach ( Prunus persica L) remnants in combination with beneficial microbes and phosphorus differ phosphorus use efficiency, agronomic efficiency and partial factor productivity in soybean vs. maize crops. J. Plant Nutr. 46 (8), 1745–1756. doi: 10.1080/01904167.2022.2099890
Khalid, S., Amanullah, Ahmed, I. (2022). Enhancing zinc biofortification of wheat through integration of zinc, compost, and zinc-solubilizing bacteria. Agriculture 12, 968. doi: 10.3390/agriculture12070968
Khan, I., Amanullah, Jamal, A., Mihoub, A., Farooq, O., Saeed, M. F., et al. (2022). Partial substitution of chemical fertilizers with organic supplements increased wheat productivity and profitability under limited and assured irrigation regimes. Agriculture 12, 1754. doi: 10.3390/agriculture12111754
Krasilnikov, P., Taboada, M. A., Amanullah (2022). Fertilizer use, soil health and agricultural sustainability. Agriculture 12, 462. doi: 10.3390/agriculture12040462
Nadia, Amanullah, Arif, M., Muhammad, D. (2023). Improvement in wheat productivity with integrated management of beneficial microbes along with organic and inorganic phosphorus sources. Agriculture 13, 1118. doi: 10.3390/agriculture13061118
Keywords: integrated nutrients management, chemical fertilizers, organic fertilizers, biofertilizers and nanofertilizers, yield and food security
Citation: Amanullah, Ondrasek G and Al-Tawaha AR (2023) Editorial: Integrated nutrients management: an approach for sustainable crop production and food security in changing climates. Front. Plant Sci. 14:1288030. doi: 10.3389/fpls.2023.1288030
Received: 03 September 2023; Accepted: 15 September 2023; Published: 09 October 2023.
Edited and Reviewed by:
Copyright © 2023 Amanullah, Ondrasek and Al-Tawaha. This is an open-access article distributed under the terms of the Creative Commons Attribution License (CC BY) . The use, distribution or reproduction in other forums is permitted, provided the original author(s) and the copyright owner(s) are credited and that the original publication in this journal is cited, in accordance with accepted academic practice. No use, distribution or reproduction is permitted which does not comply with these terms.
*Correspondence: Amanullah, [email protected]
Disclaimer: All claims expressed in this article are solely those of the authors and do not necessarily represent those of their affiliated organizations, or those of the publisher, the editors and the reviewers. Any product that may be evaluated in this article or claim that may be made by its manufacturer is not guaranteed or endorsed by the publisher.
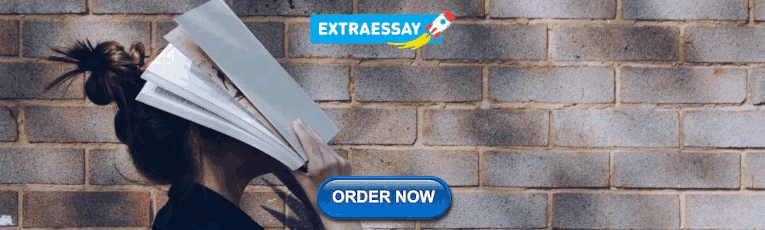
IMAGES
VIDEO
COMMENTS
This review explores the concepts, objectives, procedures, principles, and usage. of INM in agriculture. Several studies revealed that I NM enriches crop yields by 8 - 150%. compared with ...
This Research Topic, titled "Integrated Nutrients Management for Sustainable Crop Production and Food Security in Changing Climates," presents a collection of pioneering research that addresses the intricate relationship between INM, ... Integrated Nutrient Management (INM) plays a pivotal role in enhancing soil health, particularly in the ...
The work conducted by Nyamadzawo et al. (2017) in Zimbabwe in maize indicated practicing INM reduced about 17.1% GHGs over conventional nutrient management . Another ... Future research in integrated nutrient management can prioritize enhancing soil quality, a critical aspect of sustainable agriculture. ...
Integrated Plant Nutrient System (IPNS) is practiced worldwide to maintain soil quality. Two field experiments were conducted in 2019 and 2020 in acidic and charland soils to assess the impact of different manures, viz., poultry manure (PM), vermicompost (VC), compost (OF), rice husk biochar (RHB), poultry manure biochar (PMB)-based IPNS, and dolomite over control on productivity and nitrogen ...
Reduction of excess nutrient application and balanced fertilizer use are the key mitigation options in agriculture. We evaluated Nutrient Expert (NE) tool-based site-specific nutrient management ...
Integrated nutrients management (INM) refers to the maintenance of soil fertility and improvement in crop productivity with the application of plant nutrients through the combined application of organic fertilizers (animal manures and plant residues), chemical fertilizers (urea, SSP, DAP, etc.) and bio-fertilizers (beneficial microbes). The 4 ...
Integrated nutrient management determines long-term sustainable productivity levels as well as immediate nutrient availability in the soil. ... The research work was carried out by AK; DPS, BS, PV contributed to the morphological and biochemical work; US contributed to the plant and soil nutrition work; NSP, OAS and MM contributed to data and ...
Gruhn P, Goletti F, Yudelman M (2000) Integrated nutrient management, soil fertility, and sustainable agriculture: current issues and future challenges. Food, agriculture, and the environment discussion paper 32. International Food Policy Research Institute, Washington, DC. Google Scholar
Integrated nutrient management (INM) on yield trends and sustainability, nutrient balance and soil fertility in a long-term (30 years) rice-wheat system in the Indo-Gangetic plains of India ... The authors wish to acknowledge the financial assistance of the All India Co-ordinated Research Project on "Integrated Farming Systems" funded by ...
Here are insights from all 12 articles published in the Research Topic on Integrated Nutrient Management (INM), along with their relevance to soil health, crop productivity, lower costs of chemical fertilizers, food security, and climate change, while also including information related to yield and food security: Eco-friendly disease management
The aim is to provide a snapshot of some of the most exciting work published in the various research areas of the journal. Original Submission Date Received: . ... The results revealed that integrated nutrient management improved soil carbon stock, microbial biomass carbon, and soil fertility more than the other treatments.
Integrated nutrient management (INM) is a method that helps to sustain agricultural production and protect the environment for future generations. It may be defined as the application of soil fertility management practices that maximize fertilizer and organic resource use efficiency to enhance crop production [ 8 ].
The approach on integrated nutrient management (INM) with judicious use of organic and inorganic f crops, ... The recent years research work carried out on the integrated nutrient management in India and abroad with respect to growth, yield, nutrient uptake, quality parameters and economics of sweet corn has been critically reviewed in this ...
Integrated nutrient management exerts positive impact on biological, physical and chemical properties of soil. Combined application of N ... Yousufwala, for providing certified seed for this research work. This work was supported by the projects VEGA 1/0683/20 and APVV-18-0465. The current work was funded by Taif University Researchers ...
Integrated nutrient management (INM) has been a popular area of investigation in crop production research, with varied concepts and applications. Better responsiveness of soil microbial biomass over a chemically available nutrient pool to nutrient input has led to an increased interest in measuring the quantum of nutrients held microbially. This has advocated the possibility of using changes ...
nutrient management with emphasis on organics will help in attaining and sustaining higher yields. The present study was undertaken to assess the effect of integrated nutrient management on the growth, yield, nutritional quality and profitability of finger millet. The research work done in this area is reviewed in this chapter.
secondary nutrients. Integrated nutrient management which utilizes local resources in an optimum way maintaining the sustainability of the system and health of the environment is a good choice for these areas. Farmers are practicing with integrated nutrient management since ages by design or default according to soil and climatic conditions.
The experimental data presented in Table 4.2 indicated that the nitrogen content (%) in straw was significantly affected by different treatment combinations of integrated nutrient management applied during the summer season of the year 2020-21 and 2021-22 of mungbean. The nitrogen content (%) in straw was observed highest under T9 treatment ...
Wastewater treatment plants are transitioning from a sole focus on treatment objectives to integrated resource recovery and upcycling. Effective carbon management is critical for upcycling within a water resource recovery facility (WRRF) to produce energy or other usable products, which involves carbon diversion at primary treatment and waste activated sludge (WAS) from biological treatment ...