Thank you for visiting nature.com. You are using a browser version with limited support for CSS. To obtain the best experience, we recommend you use a more up to date browser (or turn off compatibility mode in Internet Explorer). In the meantime, to ensure continued support, we are displaying the site without styles and JavaScript.
- View all journals
- ADVERTISEMENT FEATURE Advertiser retains sole responsibility for the content of this article
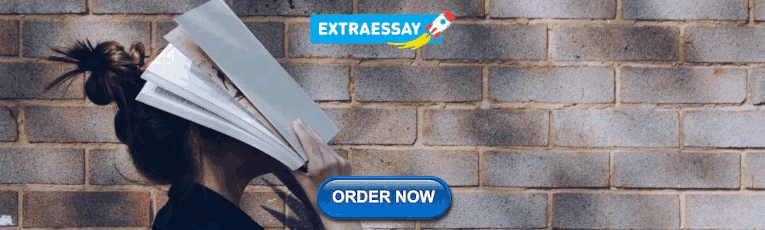
A case study in development of material science
Produced by
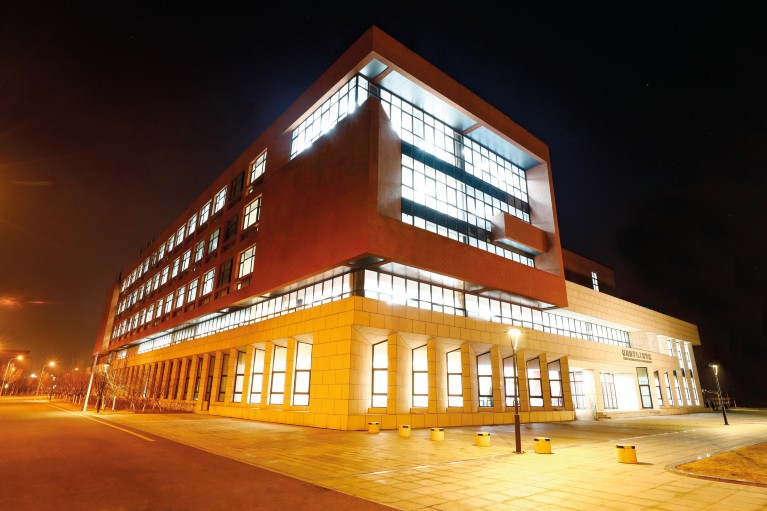
Credit: Nankai University
China’s significant increase in research investment has already brought a boom in the country’s research output. The gains are probably best illustrated in the field of material science, in which the number of publications has grown from almost none in the early 1980s to the largest in the world now. With applications ranging from manufacturing and electronics, to energy and biomedicine, material science is of profound interest to government funders and a field on which national economies rely heavily.
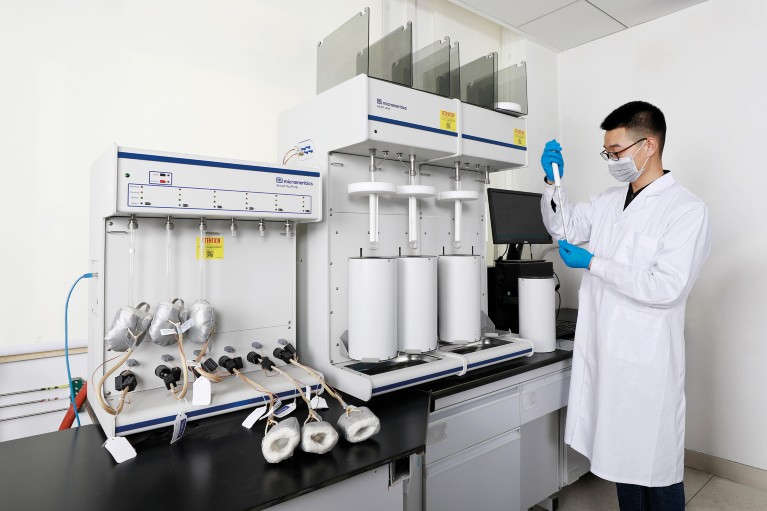
Data from the Essential Science Indicators (ESI) in 2018 show that Nankai is ranked among the global top 1.2‰, or 97th, in material science, up from 116th in mid-2017. It leads among Chinese universities in the number of citations per publication in journals listed in the Science Citation Index (SCI). Two MSE faculty members were listed among the 2018 Global Highly Cited Researchers by Clarivate Analytics.
Booming research results
One area of leading research performance at MSE is rare earth functional materials, with 30 highly cited papers tracked by ESI. “China has abundant rare earth resources, but a comprehensive scheme for using them is yet to be developed,” said Yan Chunhua, a member of the Chinese Academy of Sciences (CAS), and head of the MSE’s Centre for Rare Earth and Inorganic Functional Materials. The centre’s researchers develop novel technological processes of rare earth products and explore new applications of rare earth materials, ranging from energy and catalysis, to high-precision manufacturing and sensors. Their results have led to more than 10 patents.
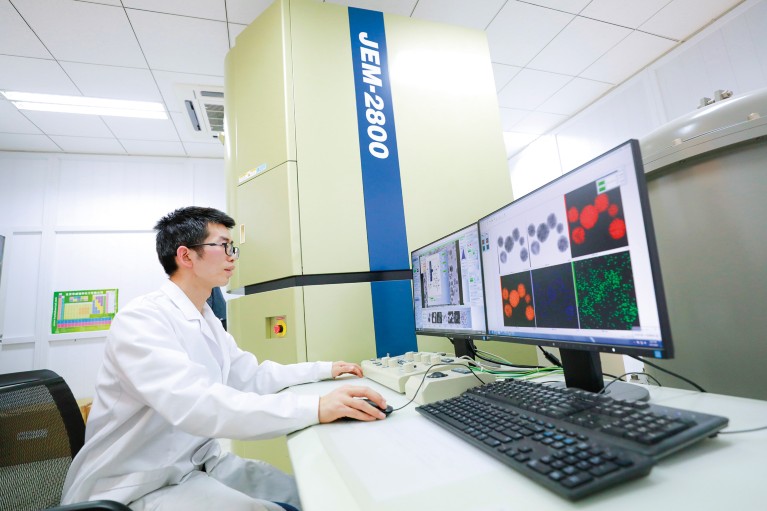
Prize-winning science
At the MSE Centre for Optical, Electrical, and Magnetic Materials, Bu Xian-He, the centre head and dean of MSE, leads a group that focuses on constructing inorganic solid materials for energy storage and conversion. They won the second prize of the National Natural Science Award in 2014 for their work on the synthesis, structure modulation, and properties of coordination polymers. Researchers at the centre also work on inorganic-organic hybrid magnetic and polymer materials, and construction of functional molecular aggregate materials.
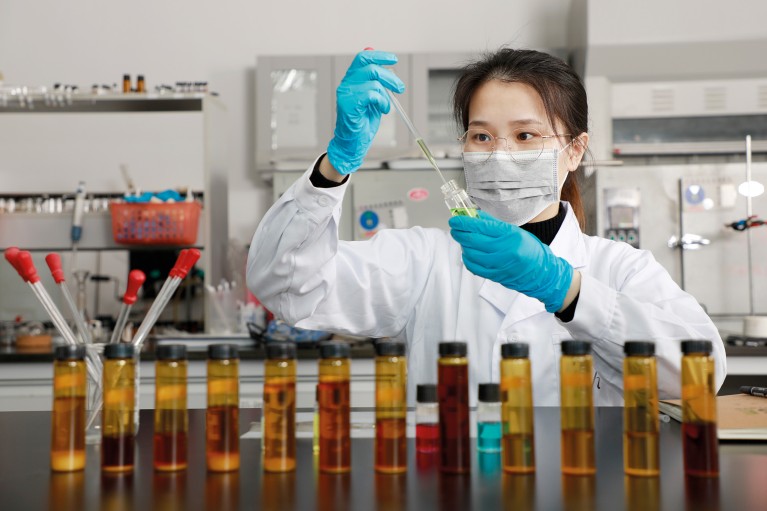
MSE researchers on new catalytic materials have established a new methodology for characterizing highly active intermediates in catalytic reactions by integrating technologies of chemical capture, spectroscopic analysis and computational simulation, which has facilitated the study of molecular sieve materials. They have also developed the AlPO-34 molecular sieve, a crystalline material with regular pores the size of a molecule for catalysis. Their results have gained 20 national patents.
To meet growing needs for new materials and devices for information and energy, researchers at the MSE Centre for Photonics/Electronic Materials have made progress in silicon-based illumination devices. They have designed nano-layered compound films with specific photoelectric properties and developed their applications in sensors, supercapacitors and other devices.
Outlook to the future
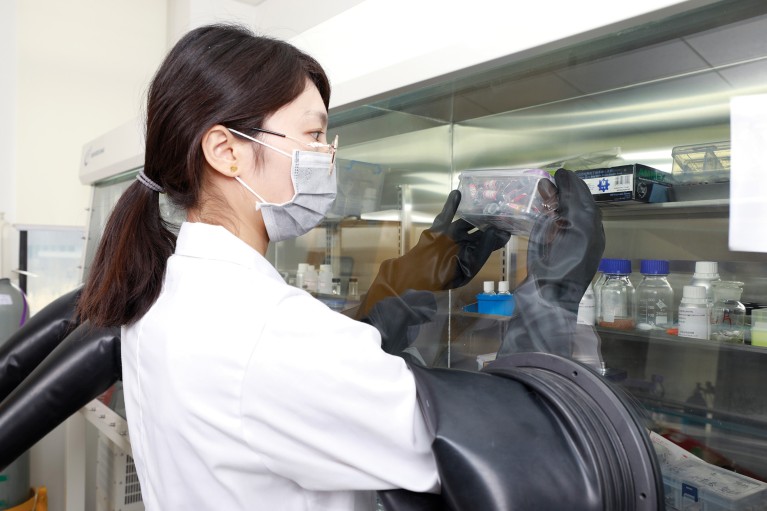
In an effort to develop cross-disciplinary and application-based research, the school is growing its computational materials science and materials genome research. Investments are also being made to advance research on biomedical, environmental, and intelligent materials and devices to better meet societal demands. In educational programmes, MSE encourages the integration of natural science and engineering to form interdisciplinary teams.
As Nankai University has received government support for building a world-class university, MSE is leveraging university and government resources to expand its current provincial and national key research platforms. Continual investment and infrastructure support will ensure a vibrant future for MSE.
+86 (0)22 85358786
http://mse.nankai.edu.cn
Quick links
- Explore articles by subject
- Guide to authors
- Editorial policies
- MSE Strategic Plan 2023
- Undergraduate Programs
- Graduate Programs
Research Topics
- Research Groups
- Research Videos
- Research Professionals
- Graduate Students
- MSE Advisory Council
- Awards and Honors
- Position Openings in MSE
- Collaborative Facilities Across Campus
- Alumni Spotlights
- Distinguished Alumni Award
- Class Photos
- Giving Opportunities
- Recruit Students
- MSE Newsletters
- Experience and Employment
- Graduate Services and Activities
- Forms & Checklists
- Identity, Health, Wellness
The field of Materials Science & Engineering is evolving dramatically as we enter the 21st Century. What began as the study of metals and ceramics in the 1960s has broadened in recent years to include semiconductors and soft materials. With this evolution and broadening of the discipline, current research projects span multiple materials classes and build on expertise in many different fields. As a result, current research in Materials Science and Engineering is increasingly defined by materials systems rather than materials classes.
At Cornell, the Department of Materials Science & Engineering (MS&E) has adopted this new systems-based vision of the field by defining four strategic areas which are considered to be critical for today’s emerging research. The four strategic research areas are Energy Production and Storage, Electronics and Photonics, Bioinspired Materials and Systems, and Green Technologies.
Materials Science & Engineering is an exciting and vibrant interdisciplinary research field. Cornell MS&E draws upon its world-class faculty, innovative researchers, state-of-the-art facilities and highly collaborative research environment to respond to challenging technological and societal demands both in the present and the future.

Energy Production and Storage
Energy research will prove to be the most prosperous growth area for the department, the College and the University. The inevitability of an energy crisis and global climate change has intensified efforts in alternative energy research around the world. The excitement building around this sector is reminiscent of the early years of the information technology revolution. Among the many possible sources of alternative energy, the following areas are particularly aligned with the current materials research at Cornell as they play to our existing strengths: photocatalysis, photovoltaics, thermoelectrics, phononics, batteries and supercapacitors .
Relevant Research Areas:
- Energy Systems
- Advanced Materials Processing
- Materials Synthesis and Processing
- Nanotechnology
- Nonlinear Dynamics
- Polymers and Soft Matter
- Semiconductor Physics and Devices
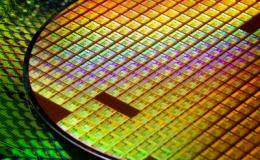
Electronics & Photonics
The use of semiconductor devices and circuits will continue to play a major role in modern life. Therefore electronics and photonics are considered premier growth areas. As feature sizes decrease, incremental research based on current methods and materials is unlikely to enable Moore's Law to continue. New materials and processing techniques are needed. Advances in nanoscale fabrication have led to recent advances in this field. We have targeted the following areas: oxide semiconductors, 3D integration, materials beyond silicon, high K and low K dielectrics, plasmonics, spintronics, and multiferroics.
- Computational Mechanics
- Computational Solid Mechanics
- Condensed Matter and Material Science
- Surface Science

Bioinspired Materials and Systems
Scientists and engineers are increasingly turning to nature for inspiration. The solutions arrived at by natural selection are often a good starting point in the search for answers to scientific and technical problems. Designing and building bioinspired devices or systems can tell us more about the original animal or plant model. The following areas are particularly aligned with the current materials research at Cornell: bioinspired composites, engineered protein films for adhesion, lubrication and sensing applications , molecular tools for in-vitro and in-vivo imaging (C-Dots, FRET), as well as biomaterials for tissue engineering and drug delivery.
- Biomedical Engineering
- Biomechanics and Mechanobiology
- Biomedical Imaging and Instrumentation
- Biotechnology
- Drug Delivery and Nanomedicine
- Mechanics of Biological Materials
- Nanobio Applications
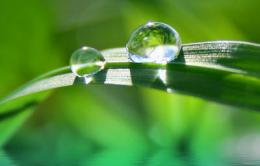
Green Technologies
The 21st century has been called the "century of the environment." Neither governments nor individual citizens can any longer assume that social challenges such as pollution, dwindling natural resources and climate change can be set aside for future generations. Strategies for clean and sustainable communities need to be established now, community by community. A dawning era of creativity and innovation in "green technology" (also known as "clean technology") is bringing the promise of a healthier planet (as well as the prospect of growing businesses) that can sustain its health. We have targeted green composites and new systems for CO2 capture and conversion as areas of future growth .
MSE 5090: Case Studies in Material Selection
Case study topics.
Browse Course Material
Course info.
- Prof. Lionel C. Kimerling
Departments
- Materials Science and Engineering
As Taught In
- Electrical Engineering
- Electronic Materials
Learning Resource Types
Principles of engineering practice, final project.
Case Study and Project Reports will be presented by the assigned Teams. The process is as follows:
Prof. Kimerling will lead a short in-class discussion on the approach for each Case Study or Project. Q&A is encouraged to clarify specific details.
The Instructors and TAs will moderate online Discussion Forums: within this Forum students should post their team’s tentative outline, develop concepts, discuss sources and preliminary findings. Instructors and TA will provide feedback within this Discussion Forum.
An optional office meeting with Prof. Kimerling is available if desired by any Group.
On the day of presentations, each Group must present a 20 minute presentation of 5-6 slides. Each member of the Group must present one slide from this presentation. Slides must be posted to the Web site the night before.
Students are expected to bring hard copies of all presentations to class.
Corrected slides and a final 2-page report must be posted to the Web site two days after presentation.
Grade assignment for the Case Studies and Projects will account for the following:
- presentation and writing skills
- clarity and rationality of the design execution
- presentation of background, issues, alternatives and conclusions
Project 1: Engineering the Future of Solar Electricity
All student work is presented with permission of the authors.

You are leaving MIT OpenCourseWare
Bridging the Gap Between Theory and Active Learning: A Case Study of Project-Based Learning in Introduction to Materials Science and Engineering
Ieee account.
- Change Username/Password
- Update Address
Purchase Details
- Payment Options
- Order History
- View Purchased Documents
Profile Information
- Communications Preferences
- Profession and Education
- Technical Interests
- US & Canada: +1 800 678 4333
- Worldwide: +1 732 981 0060
- Contact & Support
- About IEEE Xplore
- Accessibility
- Terms of Use
- Nondiscrimination Policy
- Privacy & Opting Out of Cookies
A not-for-profit organization, IEEE is the world's largest technical professional organization dedicated to advancing technology for the benefit of humanity. © Copyright 2024 IEEE - All rights reserved. Use of this web site signifies your agreement to the terms and conditions.
- The University Library
Case studies: Embedding IDL as part of a programme level approach
Case study of the MEng Materials Science and Engineering (MATU39)
Overview and outcomes of the MEng Materials Science and Engineering project to embed IDL.
The Library’s support for Materials Science and Engineering undergraduate students had, in the past, predominantly taken the form of face-to-face induction sessions at the start of each academic year.
The aim of this project was to collaboratively explore how and where Library content and information and digital literacy (IDL) could be effectively embedded in the virtual learning environment for Materials Science and Engineering students.
After exploring the IDL resources and Library content available to Materials Science and Engineering students and learning about the different digital learning tools that could be used to embed these in the programme, James reflected upon each of the modules in his programme to identify where, when, and how it would be most effective and appropriate to embed IDL and Library content.
The project team met several times throughout the project with representatives from the department, which provided further insight into the programme level approach being developed for the Materials Science and Engineering programme and gaining staff input into how Library content and IDL could most effectively be embedded within this.
There have been a number of positive outcomes from the work undertaken:
Raised awareness of information and digital literacy (IDL) support available to department to support their work on programme level approach.
Materials Science and Engineering Library subject guide has been linked from every Blackboard module for the Materials Science and Engineering programme.
IDL resources have been embedded in specific Blackboard courses at the point of student need, specifically:
MAT320/405 tutorials: using StarPlus and databases; understanding plagiarism ; Harvard and IEEE referencing guides; Discovering images , Creating and using images , How to attribute images and Adobe Photoshop guidance via LinkedIn Learning .
MAT1820 tutorials: Using StarPlus and Using Web of Science , and Harvard and IEEE referencing guides.
Work will continue in the coming academic year to explore embedding the following IDL resources into the department's UG tutorials with personal tutors: Understanding plagiarism ; Harvard and IEEE referencing guides; How to attribute images .
The Library and Digital Learning Team will work with colleagues in Materials Science and Engineering to explore using the IDL reflection template and reflective portfolio during UG tutorial sessions.
Staff within Materials Science and Engineering are piloting the IDL Quickmarks set in Turnitin to enrich assessment feedback and enable students to further develop their IDL.
Library IDL resources have been added in the Blackboard content collection and Kaltura shared repository.
Reflections
James nohl, library student associate.
The way in which reading had been delivered to us was varied between modules. Some reading lists were populated with the core reading, others did not utilise the reading lists, [but] consistently reading was referred to in lecture slides.
We saw that the reading lists could reflect what was given in lecture slides and perhaps students would utilise or follow up on reading more often if it was accessible [via a reading list] and took them straight through the StarPlus service which we are very acquainted with.
Giving [the subject guide] more visibility might help students explore the resources offered by the Library and also it gives them more consistency between the modules in how the Library content is embedded. Students will see the value of resources that are provided at the right time and the right place.
The reflective portfolio will make students more aware of their IDL skills, the university’s IDL framework and prompt them to use these resources to develop their IDL skills.
Pete Mella, Digital Learning Advisor
This project showed that students find the Library's IDL resources very useful, but they often don't know about them at the right time.
They can be surfaced much more effectively at the point when the respective skills are required during their courses, and work that came out of this project, such as QuickMarks and the IDL area of the Content Collection, can help tutors find and deploy them more easily.
Tutors also provide very good information, but in inconsistent ways on the VLE that often do not utilise useful features such as the reading lists.
Consistency within a programme on core information can reduce students' time and confusion when navigating their Blackboard courses.
Related information
Ask a question.
Email: [email protected]
Phone: +44 114 222 7200
Sheffield is a research university with a global reputation for excellence. We're a member of the Russell Group: one of the 24 leading UK universities for research and teaching.
- Skip to Content
- Bulletin Home
2024-25 General Bulletin

Department of Materials Science and Engineering
White Building (7204) Phone: 216.368.4230 Department Chair: Frank Ernst [email protected]
Materials science and engineering is a discipline that extends from understanding the microscopic structure and properties of materials to designing materials in engineering systems and evaluating their performance. Achievements in materials engineering underpin the revolutionary advances in technology that define the modern standard of living. Materials scientists and engineers understand how the properties of materials relate to their microscopic structure and composition and engineer the synthesis and microstructure of materials to advance their performance in conventional and innovative technical applications.
The Department of Materials Science and Engineering of the Case School of Engineering offers programs leading to the degrees of Bachelor of Science in Engineering, Master of Science, and Doctor of Philosophy. The technological challenges that materials engineers face demand knowledge across a broad spectrum of materials. The Department conducts academic and research activities with metals, ceramics, semiconductors, polymers, and composites. Timely research and education respond to the demands for new materials and improved materials performance in existing applications, often transcending the traditional materials categories.
While a discipline of engineering, the field brings basic science to bear on the technological challenges related to the performance of industrial products and their manufacture. Materials science draws on chemistry in its concern for bonding, synthesis, and composition of engineering materials and their chemical interactions with the environment. Physics provides a basis for understanding the atomistic and electronic structure of materials and how they determine mechanical, thermal, optical, magnetic, and electrical properties. Mathematics, computation, and data science provide quantitative physical theories and modeling of the atomistic and electronic structure and provide advances in methods for microstructural analysis, materials design, and manufacturing processes.
The Department of Materials Science and Engineering engages faculty, students, postdoctoral researchers, engineers, and staff in developing and understanding relationships between processing, structure, properties, and the performance of materials in engineering applications. The Department provides a research-intensive environment that encourages collaboration and underpins modern education of undergraduate and graduate students as well as professionals in the field. This environment provides a strong foundation for advancing the frontiers of materials research, developing important technical innovations, and preparing engineers and scientists for challenging leadership careers.
Research Areas
Deformation and fracture.
Stress–strain relations during elastic and anelastic deformation. Plastic deformation mechanisms controlled by dislocation activity, twinning, or transformation-induced shear mechanisms, as well as by creep and viscous flow mechanisms under uniaxial, biaxial, and triaxial stress states, in particular in plane-strain and/or plane-stress conditions. Relationships between structure (atomistic structure and microstructure) and mechanical behavior of crystalline and glassy materials, including metals, intermetallics, semiconductors, ceramics, and composites. State-of-the-art facilities are available for testing mechanical properties over a range of strain rates, test temperatures, stress states, and size scales under monotonic and cyclic loading and under stress–corrosion conditions.
Materials Processing
Phase-transformation- and thermo-mechanical processing of alloys, including solution-, precipitation-, recovery-and-recrystallization- and stress-relief heat-treatments, also for intentional generation of residual-stresses. Deformation processing of materials. Surface engineering, crystal growth, sputter-, vapor- and laser-ablation synthesis of films. Melting and casting of metal alloys into sand/ceramic molds, injection into metallic molds, and by rapid solidification to form crystalline or (metallic-) glass ribbons. Ceramic- and metal powder synthesis. Consolidation processing by cold-pressing and sintering, electric-field-assisted compaction, or hot-pressing. Composite materials by forming of layered materials, electroplated metals, diffusion-bonding, brazing, and welding. Electrochemical- and thermo-chemical conversion processing, e.g. oxide-film growth by anodizing or thermochemical conversion. Synthesis of micro-to-nano-porous metal/oxide structures, e.g. for battery and capacitor electrodes or for catalyst support.
Environmental Effects
Durability and lifetime extension of structural, energy-conversion-, and energy-storage materials, including materials for solar energy conversion. Corrosion, oxidation, stress-corrosion, low- and high-cycle fatigue, adhesion, decohesion, friction, and wear. Surface modification and coatings, adhesion, bonding, and dis-bonding of dissimilar materials, reliability of electronics, photonics, and sensors.
Surfaces and Interfaces
Material surfaces in vacuum, ambient-, and chemical environments, grain- and phase boundaries, hetero-interfaces (interfaces between different metals, ceramics, carbon/graphite, polymers, and combinations thereof).
Electronic, Magnetic, and Optical Materials
Materials for energy conversion technologies, such as photovoltaics, organic and inorganic light-emitting diodes and displays, fuel cells, electrolytic capacitors, solid-state Li-ion batteries, and building-envelope materials. Processing, properties, and characterization of magnetic, ferroelectric, and piezoelectric materials.
Microcharacterization of Materials
Facilities for high-resolution imaging, spatially resolved chemical analysis and spectrometry, and diffractometry. Conventional, analytical, and high-resolution transmission electron microscopy, scanning electron microscopy, focused ion beam techniques, scanning probe microscopy, light-optical microscopy, optical and electron spectroscopies, surface analysis, and X-ray diffractometry.
Materials Data Science
Rapid qualification of alloys, data science applications in polymers and coatings. Distributed computing, informatics, statistical analytics, exploratory data analysis, statistical modeling, and prediction. Hadoop, cloud computing, and computationally intensive research are supported through the operation of a scalable high-performance computing (HPC) system.
Frank Ernst, Dr. rer. nat. habil. (University of Göttingen) Leonard Case Jr. Professor of Engineering https://goo.gl/OWsF9K Microstructure and microcharacterization, alloy surface engineering, defects in crystalline materials, interface- and stress-related phenomena.
Laura S. Bruckman, PhD (University of South Carolina) Associate Professor Materials data science, lifetime and degradation science, study protocol development, spatiotemporal data integration
Jennifer W. Carter, PhD (The Ohio State University) Associate Professor Processing–structure–property relationships of crystalline and amorphous materials. Multi-scale material characterization methods for correlating local microstructural features with mechanical and environmental responses.
Roger H. French, PhD (Massachusetts Institute of Technology) Kyocera Professor Optical properties and electronic structure of polymers, ceramics, optical and biomolecular materials. These determine the vdW interactions which drive wetting of interfaces and mesoscale assembly biomolecular and inorganic systems including CNTs, proteins and DNA. Energy research focused on lifetime and degradation science. Including developing CRADLE, a Hadoop/Hbase/Spark-based distributed computing environment, for data science and analytics of complex systems such as photovoltaics and outdoor exposed materials. This allows multi-factor real-world performance to be integrated with lab-based datasets to identify mechanisms and pathways activated over lifetime using statistical and machine learning.
Hyeji Im, PhD (Korea Advanced Institute of Science and Technology) Assistant Professor Development and manufacturing of structural materials, extreme environment materials, metal additive manufacturing, sustainable alloy design and processing, materials characterization and metallurgical phenomena, and nano-microstructure control.
Neamul Khansur (UNSW Sydney, Australia) Assistant Professor Processing-structure-properties relationships in multi-functional materials. Powder aerosol deposition for fabricating protective and functional ceramic coatings. In situ X-ray and neutron diffraction characterization methods for non-linear dielectric ceramics
John J. Lewandowski, PhD (Carnegie Mellon University) Arthur P. Armington Professor of Engineering Mechanical behavior of materials. Fracture and fatigue. Micromechanisms of deformation and fracture. Composite materials. Bulk metallic glasses and composites. Refractory metals. Toughening of brittle materials. High-pressure deformation and fracture studies. Hydrostatic extrusion.
James D. McGuffin-Cawley, PhD (Case Western Reserve University) Arthur S. Holden Professor of Engineering Powder processing of ceramics. Manufacturing and materials. Additive manufacturing and rapid prototyping. Aggregation phenomena. Defects, diffusion, and solid state reactions. Materials for optical devices.
Alp Sehirlioglu, PhD (University of Illinois at Urbana Champaign) Associate Professor Energy conversion materials, including piezoelectrics and thermoelectrics. Bulk and film electro-ceramics. Epitaxial oxide films.
Gerhard E. Welsch, PhD (Case Western Reserve University) Professor High-temperature materials. Materials for capacitive energy storage. Metals, metal sponges, oxides. Mechanical and electrical properties. Synthesis.
Matthew A. Willard, PhD (Carnegie Mellon University) Associate Professor Magnetic materials: properties, microstructure evolution, phase formation, and processing conditions. Rapid solidification processing. Soft magnetic materials. Permanent magnet materials. Magnetic shape memory alloys, magnetocaloric effects, magnetic nanoparticles, and multiferroics.
Research Faculty
Erika Barcelos, PhD (Case Western Reserve University) Research Assistant Professor
Janet L. Gbur, PhD (Case Western Reserve University) Research Assistant Professor Fatigue and fracture of medical materials, mechanical behavior of superelastic Nitinol; development of microscale medical devices for rehabilitation; and flexible circuit fabrication using aerosol jet printing.
Hoda Amani Hamedani, PhD (Georgia Institute of Technology) Research Assistant Professor Nanomaterials synthesis and characterization for electrochemical energy harvesting, conversion (solar cells, fuel cells). Nanostructured platforms for biomedical applications including flexible bioelectronics and implantable microdevices, localized drug delivery, neural interfacing, sensing and in vivo power generation.
Pawan Tripathi, PhD (Indian Institute of Technology) Research Assistant Professor Materials Data Science, Advanced Manufacturing, Synchrotron Data Analysis, Image Processing, Deep Learning, Artificial Intelligence, Uncertainty Quantification, Atomistic Simulation
Jeffrey Yarus, PhD (University of South Carolina) Research Professor Applications of data science and statistics in materials science, materials engineering, and geology.
Secondary Faculty
Clemens Burda, PhD Professor Chemistry
Sunniva Collins, PhD Associate Professor Mechanical Engineering
Liming Dai, PhD Kent Hale Smith Professor Macromolecular Science and Engineering
Walter Lambrecht, PhD Professor Physics
Clare Rimnac, PhD Professor Mechanical Engineering
Mohan Sankaran, PhD Goodrich Professor of Engineering Innovation Chemical Engineering
Russell Wang, DDS Associate Professor Dentistry
Xiong (Bill) Yu, PhD, PE Professor Civil Engineering
Adjunct Faculty
Jennifer Braid, PhD (Colorado School of Mines) Adjunct Professor Developing data science and computer vision techniques for PV module and system research
Arnon Chait, PhD (The Ohio State University) Adjunct Professor NASA Lewis Research Center
Mark DeGuire, PhD (Massachusetts Institute of Technology) Adjunct Associate Professor
George Fisher, PhD Adjunct Professor Ion Vacuum Technologies Corporation
N.J. Henry Holroyd, PhD (Newcastle University) Adjunct Professor Luxfer Gas Cylinders
Jeffrey J. Hoyt, PhD (University of California, Berkeley) Adjunct Professor McMaster University
Jennie S. Hwang, PhD (Case Western Reserve University) Adjunct Professor H-Technologies Group
Peter Lagerlof, PhD (Case Western Reserve University) Adjunct Associate Professor
Ina Martin, PhD (Colorado State University) Adjunct Assistant Professor Case Western Reserve University
Farrel Martin, PhD Adjunct Professor United States Naval Research Laboratory
David Matthiesen, PhD (Massachusetts Institute of Technology) Adjunct Associate Professor
Terence Mitchell, PhD (University of Cambridge) Adjunct Professor Los Alamos National Laboratory
Erik Mueller, PhD (University of Florida) Adjunct Assistant Professor
Badri Narayanan, PhD (The Ohio State University) Adjunct Assistant Professor Lincoln Electric
Joe H. Payer, PhD Adjunct Professor University of Akron
Timothy Peshek, PhD (Case Western Reserve University) Adjunct Assistant Professor NASA Glenn Research Center
Rudolph Podgornik, PhD (University of Ljubljana) Adjunct Professor University of Ljubljana
Gary Ruff, PhD (Case Western Reserve University) Adjunct Professor Ruff Associates
Ali Sayir, PhD (Case Western Reserve University) Adjunct Professor Air Force Office of Scientific Research
Mohsen Seifi, PhD (Case Western Reserve University) Adjunct Assistant Professor ASTM International
Emeritus Faculty
William A. "Bud" Baeslack III, PhD (Rensselaer Polytechnic Institute) Professor Emeritus Welding, joining of materials, and titanium and aluminum metallurgy
Mark De Guire, PhD (Massachusetts Institute of Technology) Associate Professor Emeritus Synthesis and properties of ceramics in bulk and thin-film form, including fuel cell materials, gas sensors, coatings for biomedical applications, photovoltaics, and ferrites. Testing and microstructural characterization of materials for alternative energy applications. High-temperature phase equilibria. Defect chemistry.
Arthur H. Heuer Professor Emeritus
Peter Lagerlof, PhD (Case Western Reserve University) Associate Professor Emeritus Mechanical properties of ceramics and metals. Low-temperature deformation twinning. Light-induced plasticity of semiconductors. Methodology of transmission electron microscopy and diffractometry.
David Matthiesen, PhD (Massachusetts Institute of Technology) Associate Professor Emeritus Nitride-based ferromagnetic materials. Applied atomistic simulation of materials. Materials for use in wind turbines. Wind resource measurements onshore and offshore. Materials interactions with ice. Bulk crystal growth processing. Process engineering in manufacturing. Heat, mass, and momentum transport.
Pirouz Pirouz Professor Emeritus
- Applied Data Science, Graduate Certificate
- Applied Data Science, Minor
- Materials Science and Engineering, BSE
- Materials Science and Engineering, Minor
- Materials Science and Engineering, MS
- Materials Science and Engineering, PhD
Dual Degrees
- Programs Toward Graduate or Professional Degrees
Related Majors in Other Departments
- Data Science and Analytics, BS (administered by the Department of Computer and Data Sciences )
Advanced Manufacturing and Mechanical Reliability Center (AMMRC)
White Building 115, 211, 216, 222, 300, 338
Deformation Processing Laboratory: White Building 115 Nitonol Commercialization Accelerator: White Building 300, 338 Mechanical Testing Laboratories: White Building 211, 216, 222
Contact: John Lewandowski 216-368-4234 [email protected]
The AMMRC (Advanced Manufacturing and Mechanical Reliability Center) permits the determination of mechanical behavior of materials over loading rates ranging from static to impact, with the capability of testing under a variety of stress states under either monotonic or cyclic conditions. A variety of furnaces and environmental chambers are available to enable testing at temperatures ranging from -196 °C to 1800 °C. The facility is operated under the direction of a faculty member and under the guidance of a full-time engineer. The facility contains one of the few laboratories in the world for high-pressure deformation and processing, enabling experimentation under a variety of stress states and temperatures. This state-of-the-art facility includes the following equipment:
- High-Pressure Deformation Apparatus: Th is unit enables tension or compression testing to be conducted under conditions of high hydrostatic pressure and consists of a pressure vessel and diagnostics for measurement of load and displacement on deforming specimens, as well as instantaneous pressure in the vessel. Pressures up to 1.0 GPa loads up to 10 kN, and displacements of up to 25 mm are possible. This oil-based apparatus can be operated at temperatures up to 300 °C.
- Hydrostatic-Extrusion Apparatus: Hydrostatic extrusion (e.g. pressure-to-air, pressure-to-pressure) can be conducted at temperatures up to 300 °C on manually operated equipment interfaced with a computer data acquisition package. Pressures up to 2.0 GPa are possible, with reduction ratios up to 6 to 1, while various diagnostics provide real time monitoring of extrusion pressure and ram displacement.
- Advanced Forging-Simulation Rig: A multi-actuator MTS machine based on 1.5 MN, four post frame, enables sub-scale forging simulations over industrially relevant strain rates. A 490 kN forging actuator is powered by five nitrogen accumulators enabling loading rates up to 3.0 m/s on large specimens. A 980 kN indexing actuator provides precise deformation sequences for either single, or multiple, deformation sequences. Dat a acquisition at rates sufficient for analysis is available. Testing with heated dies is possible.
- Advanced Metal-Forming Rig: A four-post frame with separate control of punch actuator speed and blank hold down pressure enables determination of forming limit diagrams. Dynamic control of blank hold down pressure is possible, with maximum punch actuator speeds of 30.0 cm/s. A variety of die sets are available.
- Servo-hydraulic Machines: Four MTS Model 810 computer-controlled machines with load capacities of 13 kN, 90 kN, 220 kN, and 220 kN, permit tension, compression, and fatigue studies to be conducted under load-, strain-, or stroke control. Fatigue crack growth may be monitored via a DC potential drop technique as well as via KRAK gauges applied to the specimen surfaces. Fatigue studies may be conducted at frequencies up to 30 Hz. In addition, an Instron Model 1331 90 kN Servo-hydraulic machine is available for both quasi-state and cyclic testing.
- Universal Testing Machines: Three INSTRON screw-driven machines, including two INSTRON Model 1125 units permit tension, compression, and torsion testing.
- Electromechanical Testing Machine: A computer-controlled INSTRON Model 1361 can be operated under load-, strain-, or stroke control. Stroke rates as slow as 0.3 nm/s are possible.
- Fatigue Testing Machines: Three Sonntag fatigue machines and two R. R. Moore rotating-bending fatigue machines are available for producing fatigue-life (S–N) data. The Sonntag machines may be operated at frequencies up to 60 Hz.
- Creep Testing Machines: Three constant load frames with temperature capabilities up to 800 °C permit creep testing, while recently modified creep frames permit thermal cycling experiments as well as slow cyclic creep experiments.
- Impact Testing Machines: Two Charpy impact machines with capacities ranging from 20 ft-lbs to 240 ft-lbs are available. Accessories include a Dynatup instrumentation package interfaced with an IBM PC, which enables recording of load vs. time traces on bend specimens as well as on tension specimens tested under impact conditions.
- Instrumented Microhardness Tester: A Nikon Model QM High-Temperature Microhardness Tester permits indentation studies on specimens tested at temperatures ranging from -196 °C to 1 200 °C under vacuum and inert gas atmospheres. This unit is complemented by a Zwick Model 3212 Microhardness Tester as well as a variety of Rockwell Hardness and Brinell Hardness Testing Machines.
Swagelok Center for Surface Analysis of Materials
Glennan Building 101
Contact: Jennifer Carter, 216-368-4214, [email protected] Jeffrey Pigott, 216-368-6012, [email protected] Website: https://engineering.case.edu/centers/scsam/
SCSAM, the Swagelok Center for Surface Analysis of Materials, is a multi-user facility providing cutting-edge major instrumentation for microcharacterization of materials. SCSAM is administered by the CSE (Case School of Engineering) and is central to much of the research carried out by CSE's seven departments. The facility is also extensively used by the CAS (College of Arts and Sciences) Departments of Physics, Chemistry, Biology, and Earth, Environmental, and Planetary Sciences, as well as many departments within the School of Medicine and the School of Dental Medicine. Typically, more than 200 users, mostly academic, utilize the facility per year.
SCSAM's instruments encompass a wide and complementary range of characterization techniques, which provide a comprehensive resource for high-resolution imaging, diffractometry, and spatially-resolved compositional analysis.
Current capabilities for high-resolution imaging include: an AFM (atomic force microscope) which can optionally be operated with an imaging nanoindenter scan head or a stand-alone automated nanoindenter; a Keyence optical microscope providing the next-generation of optical microscopy with a large depth-of-field and advanced measurement capabilities for inspection and failure analysis.; two scanning electron microscopes, one equipped for FIB (focused ion beam) micromachining, and both equipped with XEDS (X-ray energy-dispersive spectrometry), TSEM (transmission scanning electron microscopy), and EBSD (electron backscatter diffraction) detectors.
For XRD (X-ray diffractometry), SCSAM provides two diffractometers with 1D and 2D detectors to allow for phase identification, phase fraction determination, crystal structure refinements, as well as stress and strain measurements of crystalline solids.
SCSAM's surface analysis suite of instruments includes an instrument for ToF-SIMS (time-of-flight secondary-ion mass spectrometry), a SAM (scanning Auger microprobe) for spatially resolved AES (Auger electron spectroscopy), and an instrument for XPS (X-ray photoelectron spectroscopy, also known as ESCA, electron spectrometry for chemical analysis), that accomplishes high spatial resolution by operating with a focused X-ray beam.
SCSAM’s instruments are housed in a centralized area allowing users convenient access to state-of-the-art tools for their research.
Magnetometry Laboratory
Contact: Matthew Willard 216-368-5070
The Magnetometry Laboratory has facilities used to investigate the magnetic properties of materials. This laboratory has the following instruments:
Lake Shore Cryotronics Model 7410 Vibrating Sample Magnetometer This instrument serves for measurement of hysteresis loops (at constant temperature) and thermomagnetic measurements (at constant magnetic field). The maximum applied field at room temperature (without furnace in place) is 3.1 T. For high temperature measurements, the maximum applied field is 2.5 T over the temperature range from room temperature to 1000 °C.
Home-Built Magnetostriction Measurement System This system has been designed and built to measure the shape change of magnetic materials under applied magnetic fields. Better than 1 ppm sensitivity is possible by this strain gauge technique. An applied field of ≈0.2 T is used to saturate samples.
SDLE Research Center
White Building 538
Contact: Roger French
216-368-3655
The SDLE Research Center was established in 2011 as a Wright Project Center with funding from Ohio Third Frontier. Initially it was dedicated to advancing the fields of lifetime and degradation science using data science. The research center activities have expanded to include research focused on the durability and degradation of environmentally exposed, long-lived materials and technologies such as photovoltaics (PV), coatings, energy efficient lighting, and building envelope applications, as well as broad-based collaborations in materials data science in reliability and degradation, carbon capture and storage, geothermal energy applications.
Today the SDLE Research Center is advancing the development of Materials Data Science for use in Materials Reliability, Materials Science and broader application areas.
The SDLE Research Center, also includes the SDLE Core Facility, which is a CWRU User Facility, which provides both reliability and Materials Data Science tools and capabilities available to the CWRU community, other academic researchers and industrial and national laboratory researchers.
A data science approach is needed to handle large-scale data on materials, components, systems, modules, commercial power plants, and the grid. These approaches involve data ingestion into nonrelational data warehouses and data-driven modeling with a foundation in the underlying physics and chemistry of degradation and lifetime performance. Assembling FAIR (Findable, Accessible, Interoperable, Reusable) data and other data, developing and sharing codes and tools, and reporting research results along a materials value chain is a key component of the Center. The SDLE Research Center facilitates complex data-driven modeling, including geostatistical, geospatiotemporal modeling, graph network modeling, and degradation network models. The data analytics platform (CRADLE), an integrated distributed and high performance computing cluster, was developed in the center to facilitate large data storage and analysis with ease of access to team members enabling fleets of high performance computing jobs for improved data analytics.
The SDLE Center has developed a method to enable large-scale distributed analysis of commercial fleet scale photovoltaic (PV) power plants for both performance loss rate (PLR) determination and power forecasting. This study includes a set of timeseries datasets for 100,000 PV plant inverters and determines the data quality of these plants in relation to prediction of PLR. Additionally, a multi-year benchmarking and review of the impact of data quality and filtering, power prediction algorithms, and PLR determination methods has defined the challenges in PLR determination. The data quality, data gaps, and filtering of timeseries data of commercial fleets of PV plants restrict which algorithms analyses can use and can bias results and reduce their accuracy. Data quality and data gaps can be improved with spatio-temporal graph neural network (st-GNN) models of PV power plant data including satellite weather data and autoencoders for data imputation of missing data. FAIR data principles are used to make FAIR data and models in order to improve transferability of data and models.
The SDLE Center has a focus on materials data science in relation to long-lived materials. This work determines the degradation mechanisms in material systems, which can be mitigated to optimize lifetime performance of materials, components, and devices. Understanding these key degradation mechanisms in relation to the stress and stress level is fundamental to lifetime and degradation science (L&DS). By encompassing the knowledge from the experimental insights of the degradation of materials, the lifetime of materials can be predicted under multiple different stress conditions. Thus far traditional materials reliability has been flawed with costly failures in applications such as polyamide backsheet failure in photovoltaic (PV) modules. The Center has developed an epidemiological approach to understanding materials degradation which provides more scientific value by giving information on the standard deviation within a population. Additionally, by combining standard and modified accelerated exposures with real-world exposures, degradation can be more accurately predicted on a variety of different grades of materials or component structure. Then data-driven or network modeling provides insights into the impact of stress conditions on degradation and performance. Real-world degradation gives the information on the complex and synergistic nature of materials degradation compared to single or even combinational accelerated stressors. The unique environment that a material exists in the real world or in-use conditions is varied due to specific microstressors as well as the impact of climate change on climate zones.
Geostatistical geospatiotemporal modeling is an active area of research within SDLE which is a quantitative method for mapping phenomena that are inherently tied to geographic and/or temporal space. The method provides for estimating at unsampled locations and for simulating multiple equally probable realizations to assess the space of uncertainty in the subsurface, surface, or near surface environment. Applications include environmental, mineral resources, geothermal, hydrology, agriculture, climate, forestry, soil, air, and more.
The SDLE Research Center’s Core Facility has capabilities and equipment including:
Outdoor solar exposures: SunFarm with 14 dual-axis solar trackers with multi-sun concentrators, and power degradation monitoring
Solar simulators for 1-1000X solar exposures
Multi-factor environmental test chambers with temperature, humidity, freeze/thaw, and cycling
A full suite of optical, interfacial, thermo-mechanical, and electrical evaluation tools for materials, components, and systems
CRADLE: 800 Terabytes of nonrelational data warehouses based on Cloudera’s distribution of Apache’s Hadoop, Hbase, and Spark
High Performance Compute Cluster for data science and analytics
The Center for Materials Data Science for Reliability and Degradation (MDS-Rely)
The Center for Materials Data Science for Reliability and Degradation (MDS-Rely) is a National Science Foundation (NSF) Industry-University Cooperative Research Center (IUCRC) which CWRU leads in partnership with the University of Pittsburgh. Center Members from both industry and government join the Center as Members and directly fund center research. MDS-Rely seeks to apply data science-informed research to better understand the reliability and lifetime of essential materials, while creating code packages, models, and other materials-agnostic deliverables that are jointly owned by Member organizations .
Through a series of competencies, research thrusts, and materials value chains, MDS-Rely focuses on transferable research outputs while training a data-enabled workforce of students and graduates that enjoy strong, collaborative relationships with our Member organizations. Through a diverse research portfolio, MDS-Rely provides insight into the following areas:
1. Competencies: Standards & Reliability Protocols; Materials Data Science; Reliability, Performance, & Degradation Solutions 2. Research Thrusts: Weathering & Performance; Subtractive & Additive Manufacturing; Energy Technologies. 3. Materials Value Chains: Polymers, Elastomers, & Coatings; Metals & Alloys; Semiconductors & Optoelectronics; and an evolving focus on circular economy, sustainability, & climate.
MDS-Rely works in close partnership with the SDLE Research Center. Interested parties should reach out to Jonathan Steirer, Managing Director, at [email protected] or Roger French, Center Director, at [email protected]
Applied Data Science (DSCI)
Materials science and engineering (emse).
DSCI 330. Cognition and Computation. 3 Units.
An introduction to (1) theories of the relationship between cognition and computation; (2) computational models of human cognition (e.g. models of decision-making or concept creation); and (3) computational tools for the study of human cognition. All three dimensions involve data science: theories are tested against archives of brain imaging data; models are derived from and tested against datasets of e.g., financial decisions (markets), legal rulings and findings (juries, judges, courts), legislative actions, and healthcare decisions; computational tools aggregate data and operate upon it analytically, for search, recognition, tagging, machine learning, statistical description, and hypothesis testing. Offered as COGS 330 , COGS 430 , DSCI 330 and DSCI 430 .
DSCI 332. Spatial Statistics for Near Surface, Surface, and Subsurface Modeling. 3 Units.
This course is on spatial modeling of near surface, surface, and subsurface data, also known as geostatistical modeling. Spatial modeling has its origins in predictive modeling of minerals in subsurface formations, from which many examples are used in this class. Students will learn the basics of spatial models in order to understand how they are built from various data types and how their uncertainties are assessed and risk reduced. Students will be expected to learn the rudimentary navigation of R Studio, execute pre-written publically available R code (provided), and make simple modifications. Graduate students will be expected to learn the above and develop a 10 week modeling project focused on the use of spatial modeling methods with R using data relevant to their specific discipline or interest. These projects will include preparing datasets to be executed in R code scripts. Resulting scripts will be placed in a git repository for use by other students as open source resources along with documentation demonstrating the reproducible spatial modeling science and analyses for these problems. Geostatistical (spatial) mapping is applicable across many disciplines. Examples of graduate projects from previous classes include subsurface modeling (geology), earthquake mapping (geophysics/civil engineering), soil stability modeling (civil engineering), aquifer characterization (hydrology), and pollution/contaminant mapping (environmental studies/medicine). Offered as DSCI 332 and DSCI 432 .
DSCI 351. Exploratory Data Science. 3 Units.
In this course, we will learn data science and analysis approaches to identify statistically significance relationships and better model and predict the behavior of these systems. We will assemble and explore real-world datasets, perform clustering and pair plot analyses to investigate correlations, and logistic regression will be employed to develop associated predictive models. Results will be interpreted, visualized and discussed. We will introduce basic elements of statistical analysis using R Project open source software for exploratory data analysis and model development. R is an open-source software project with broad abilities to access machine-readable open-data resources, data cleaning and munging functions, and a rich selection of statistical packages, used for data analytics, model development and prediction. This will include an introduction to R data types, reading and writing data, looping, plotting and regular expressions, so that one can start performing variable transformations for linear fitting and developing structural equation models, while exploring for statistically significant relationships. The M section of DSCI 351 is for students focusing on Materials Data Science. Offered as DSCI 351 , DSCI 351M and DSCI 451 . Prereq: ( ENGR 130 or ENGR 131 or CSDS 132 or ECSE 132 or DSCI 134) and ( STAT 312R or STAT 201R or SYBB 310 or PQHS 431 ).
DSCI 351M. Exploratory Data Science. 3 Units.
DSCI 352. Applied Data Science Research. 3 Units.
This is a project based data science research class, in which project teams identify a research project under the guidance of a domain expert professor. The research is structured as a data analysis project including the 6 steps of developing a reproducible data science project, including 1: Define the ADS question, 2: Identify, locate, and/or generate the data 3: Exploratory data analysis 4: Statistical modeling and prediction 5: Synthesizing the results in the domain context 6: Creation of reproducible research, Including code, datasets, documentation and reports. During the course special topic lectures will include Ethics, Privacy, Openness, Security, Ethics. Value. The M section of DSCI 352 is for students focusing on Materials Data Science. Offered as DSCI 352 , DSCI 352M and DSCI 452 . Prereq: ( CSDS 133 or DSCI 134 or ENGR 130 or ENGR 131 or CSDS 132 or ECSE 132 ) and ( STAT 312R or STAT 201R or SYBB 310 or PQHS 431 or OPRE 207 ) and ( DSCI 351 or ( SYBB 311A and SYBB 311B and SYBB 311C and SYBB 311D) or SYBB 321 or MKMR 201 ).
DSCI 352M. Applied Data Science Research. 3 Units.
DSCI 353. Data Science: Statistical Learning, Modeling and Prediction. 3 Units.
In this course, we will use an open data science tool chain to develop reproducible data analyses useful for inference, modeling and prediction of the behavior of complex systems. In addition to the standard data cleaning, assembly and exploratory data analysis steps essential to all data analyses, we will identify statistically significant relationships from datasets derived from population samples, and infer the reliability of these findings. We will use regression methods to model a number of both real-world and lab-based systems producing predictive models applicable in comparable populations. We will assemble and explore real-world datasets, use pair-wise plots to explore correlations, perform clustering, self-similarity, and logistic regression develop both fixed-effect and mixed-effect predictive models. We will introduce machine-learning approaches for classification and tree-based methods. Results will be interpreted, visualized and discussed. We will introduce the basic elements of data science and analytics using R Project open source software. R is an open-source software project with broad abilities to access machine-readable open-data resources, data cleaning and assembly functions, and a rich selection of statistical packages, used for data analytics, model development, prediction, inference and clustering. With this background, it becomes possible to start performing variable transformations for linear regression fitting and developing structural equation models, fixed-effects and mixed-effects models along with other statistical learning techniques, while exploring for statistically significant relationships. The class will be structured to have a balance of theory and practice. We'll split class into Foundation and Practicum a) Foundation: lectures, presentations, discussion b) Practicum: coding, demonstrations and hands-on data science work. The M section of DSCI 353 is for students focusing on Materials Data Science. Offered as DSCI 353 , DSCI 353M and DSCI 453 .
DSCI 353M. Data Science: Statistical Learning, Modeling and Prediction. 3 Units.
DSCI 354. Data Visualization and Analytics. 3 Units.
Data Visualization and Analytics students will learn data visualization and analytics techniques focused on different types of data such as time-series, spectral, or image data science problems. This class will focus on increasing analysis of complex data sets through visualization by enhancing exploratory data analysis and data cleaning. This class will focus on creating effective data visualizations to communicate data analytics results to different audiences. Different datasets will be provided to develop different types of visualizations and analytics. Types of data visualizations include in interactive plots (e.g., bar graphs change over time), applications that allow users to adjust the visualizations based on their decisions (e.g., shiny applications), interactive maps, 3-D plots of data, etc. Discussing how an audience understands information and brings in data as well as the ethics of making data visualizations will be discussed. The class will also include ways to increase modeling and analysis with effective visualizations for credible, data-driven decision making. This will include a git repository for other students to use these codes as open source resources and the preparation of reproducible data science analyses for different types of problems. Offered as DSCI 354 , DSCI 354M , and DSCI 454 . Prereq: ( DSCI 351 or DSCI 351M ) and ( DSCI 353 or DSCI 353M ).
DSCI 354M. Data Visualization and Analytics. 3 Units.
DSCI 430. Cognition and Computation. 3 Units.
DSCI 432. Spatial Statistics for Near Surface, Surface, and Subsurface Modeling. 3 Units.
DSCI 451. Exploratory Data Science. 3 Units.
In this course, we will learn data science and analysis approaches to identify statistically significance relationships and better model and predict the behavior of these systems. We will assemble and explore real-world datasets, perform clustering and pair plot analyses to investigate correlations, and logistic regression will be employed to develop associated predictive models. Results will be interpreted, visualized and discussed. We will introduce basic elements of statistical analysis using R Project open source software for exploratory data analysis and model development. R is an open-source software project with broad abilities to access machine-readable open-data resources, data cleaning and munging functions, and a rich selection of statistical packages, used for data analytics, model development and prediction. This will include an introduction to R data types, reading and writing data, looping, plotting and regular expressions, so that one can start performing variable transformations for linear fitting and developing structural equation models, while exploring for statistically significant relationships. The M section of DSCI 351 is for students focusing on Materials Data Science. Offered as DSCI 351 , DSCI 351M and DSCI 451 .
DSCI 452. Applied Data Science Research. 3 Units.
This is a project based data science research class, in which project teams identify a research project under the guidance of a domain expert professor. The research is structured as a data analysis project including the 6 steps of developing a reproducible data science project, including 1: Define the ADS question, 2: Identify, locate, and/or generate the data 3: Exploratory data analysis 4: Statistical modeling and prediction 5: Synthesizing the results in the domain context 6: Creation of reproducible research, Including code, datasets, documentation and reports. During the course special topic lectures will include Ethics, Privacy, Openness, Security, Ethics. Value. The M section of DSCI 352 is for students focusing on Materials Data Science. Offered as DSCI 352 , DSCI 352M and DSCI 452 .
DSCI 453. Data Science: Statistical Learning, Modeling and Prediction. 3 Units.
DSCI 454. Data Visualization and Analytics. 3 Units.
Data Visualization and Analytics students will learn data visualization and analytics techniques focused on different types of data such as time-series, spectral, or image data science problems. This class will focus on increasing analysis of complex data sets through visualization by enhancing exploratory data analysis and data cleaning. This class will focus on creating effective data visualizations to communicate data analytics results to different audiences. Different datasets will be provided to develop different types of visualizations and analytics. Types of data visualizations include in interactive plots (e.g., bar graphs change over time), applications that allow users to adjust the visualizations based on their decisions (e.g., shiny applications), interactive maps, 3-D plots of data, etc. Discussing how an audience understands information and brings in data as well as the ethics of making data visualizations will be discussed. The class will also include ways to increase modeling and analysis with effective visualizations for credible, data-driven decision making. This will include a git repository for other students to use these codes as open source resources and the preparation of reproducible data science analyses for different types of problems. Offered as DSCI 354 , DSCI 354M , and DSCI 454 . Prereq: DSCI 451 and DSCI 453 .
EMSE 102. Materials for Current and Future Technologies. 1 Unit.
Open to all students discussing the importance of materials on current and future technologies. The course will be a series of seminars by the faculty at the Department of Materials Science and Engineering covering important topics such as materials processing, use of materials in a variety of technologically important areas; e.g., construction, energy related technologies, biomedical applications and space applications.
EMSE 110. Transitioning Ideas to Reality I - Materials in Service of Industry and Society. 1 Unit.
In order for ideas to impact the lives of individuals and society they must be moved from "blue sky" to that which is manufacturable. Therein lies true creativity - design under constraint. Greater Cleveland is fortunate to have a diverse set of industries that serve medical, aerospace, electric, and advanced-materials technologies. This course involves trips to an array of work sites of leading companies to witness first-hand the processes and products, and to interact directly with practitioners. Occasional in-class speakers with demonstrations will be used when it is not logistically reasonable to visit off-site.
EMSE 120. Transitioning Ideas to Reality II - Manufacturing Laboratory. 2 Units.
This course complements EMSE 110 . In that class students witness a diverse array of processing on-site in industry. In this class students work in teams and as individuals within processing laboratories working with an array of "real materials" to explore the potential of casting, machining, and deformation processes to produce real parts and/or components. An introduction to CAD as a means of communication is provided. The bulk of the term is spent in labs doing hands-on work. Planned work is carried out to demonstrate techniques and potential. Students have the opportunity to work independently or in teams to produce articles as varied as jewelry, electronics, transportation vehicles, or novel components or devices of the students' choosing.
EMSE 125. First Year Research in Materials Science and Engineering. 1 Unit.
First year students conduct independent research in the area of material science and engineering, working closely with graduate student(s) and/or postdoctoral fellow(s), and supervised by an EMSE faculty member. An average of 5-6 hr/wk in the laboratory, periodic updates, and an end of semester report is required. Prereq: Limited to first year undergraduate students..
EMSE 220. Materials Laboratory I. 2 Units.
Experiments designed to introduce processing, microstructure and property relationships of metal alloys and ceramics. Solidification of a binary alloy and metallography by optical and scanning electron microscopy. Synthesis of ceramics powders, thermal analysis using thermogravimetric analysis and differential thermal analysis, powder consolidation. Kinetics of high-temperature sintering, grain growth, and metal oxidation. Statistical analysis of experimental results. Recommended preparation or recommended co-requisite: EMSE 276 . This course satisfies the GER Disciplinary Communication requirement only in combination with EMSE 320 . Counts as a Disciplinary Communication course.
EMSE 228. Mathematical and Computational Methods for Materials Science and Engineering. 3 Units.
The course combines fundamental topics of material science and engineering with underlying mathematical methods and coding for computation. Focusing on the mathematics of vectors and using Mathematica as computational framework, the course teaches how to solve problems drawn from crystallography, diffraction, imaging of materials, and image processing. Students will develop a fundamental understanding of the basis for solving these problems including understanding the constituent equations, solution methods, and analysis and presentation of results. Prereq: ( ENGR 130 or ENGR 131 or CSDS 132 or ECSE 132 ) and ( ENGR 145 or CHEM 106 or PHYS 221 ).
EMSE 276. Materials Properties: Composition and Structure. 3 Units.
Relation of crystal structure, microstructure, and chemical composition to the selected properties of materials. The role materials processing has in controlling structure so as to obtain desired properties, using examples from metals, semiconductors, ceramics, and composites. Demonstration of properties determined by type and strength of interatomic bonding; presence of crystallographic defects; and microstructural control. Use of diagrams, frameworks, and mathematical relationships to evaluate and predict properties. A systematic review of characterization methods for assessing the state of different classes of materials will be provided throughout the course. Prereq: MATH 121 and ( CHEM 106 or PHYS 221 or ENGR 145 ). Prereq or Coreq: PHYS 122 or PHYS 124 .
EMSE 308. Welding Metallurgy. 3 Units.
Introduction to arc welding and metallurgy of welding. The course provides a broad overview of different industrial applications requiring welding, the variables controlling critical property requirements of the weld and a survey of the different types of arc welding processes. The course details the fundamental concepts that govern the different aspects of arc welding including the welding arc, weld pool solidification, precipitate formation and solid state phase transformations. Offered as EMSE 308 and EMSE 408 . Coreq: EMSE 327 .
EMSE 319. Processing and Manufacturing of Materials. 3 Units.
Introduction to processing technologies by which materials are manufactured into engineering components. Discussion of how processing methods are dependent on desired composition, structure, microstructure, and defects, and how processing affects material performance. Emphasis will be placed on processes and treatments to achieve or improve chemical, mechanical, physical performance and/or aesthetics, including: casting, welding, forging, cold-forming, powder processing of metals and ceramics, and polymer and composite processing. Coverage of statistics and computational tools relevant to materials manufacturing. Prereq: EMSE 276 .
EMSE 320. Materials Laboratory II. 1 Unit.
Introduce the design of microstructural characterization approaches to elucidate the structure and/or microstructure. It will include demonstrations of appropriate techniques for different material classes. The characterization is the enabler of the materials science and engineering triad: processing-structure-properties-performance. Students will reflect on how professional standards, statistical analysis, and instrument limitations require that engineers and scientists conduct failure analysis and interpret conclusions. Recommended preparation: EMSE 276 . Recommended corequisite: EMSE 372 . This course satisfies the GER Disciplinary Communication requirement only in combination with EMSE 220 . Counts as a Disciplinary Communication course.
EMSE 325. Undergraduate Research in Materials Science and Engineering. 1 - 3 Units.
Undergraduate laboratory research in materials science and engineering. Students will undertake an independent research project alongside graduate student(s) and/or postdoctoral fellow(s), and will be supervised by an EMSE faculty member. Written and oral reports will be given on a regular basis, and an end of semester report is required. The course can be repeated up to four (4) times for a total of six (6) credit hours. Prereq: Sophomore or Junior standing and consent of instructor.
EMSE 327. Thermodynamic Stability and Rate Processes. 3 Units.
An introduction to thermodynamics of materials as applied to metals, ceramics, polymers and optical/radiant heat transfer for photovoltaics. The laws of thermodynamics are introduced and the general approaches used in the thermodynamic method are presented. Systems studied span phase stability and oxidation in metals and oxides; nitride ceramics and semiconductors; polymerization, crystallization and block copolymer domain formation; and the thermodynamics of systems such as for solar power collection and conversion. Recommended preparation: EMSE 228 and ENGR 225 or equivalent. Prereq: EMSE 276 or EMSE 201.
EMSE 328. Mesoscale Structural Control of Functional Materials. 3 Units.
The course focuses on mesoscale structure of materials and their interrelated effects on properties, mostly in electrical in nature. The mesoscale science covers the structures varying from electronic- to micro-structure. In each scale, fundamental science will be complimented by examples of applications and how the structure is exploited both to modify and enable function. The student will develop an understanding of how the structure across multiple scales are interrelated and how to tailor them for desired outcomes. Offered as: EMSE 328 and EMSE 428 . Prereq: ( MATH 223 or MATH 227 ) and ( EMSE 276 or EMSE 201).
EMSE 330. Materials Laboratory III. 2 Units.
Experiments designed to characterize and evaluate different microstructures produced by variations in processing. Hardenability of steels, TTT and CT diagrams, precipitation hardening of alloys and fracture of brittle materials. Statistical analysis of experimental results. Recommended preparation: EMSE 276 . Recommended preparation or co-requisites: EMSE 327 .
EMSE 335. Strategic Metals and Materials for the 21st Century. 3 Units.
This course seeks to create an understanding of the role of mineral-based materials in the modern economy focusing on how such knowledge can and should be used in making strategic choices in an engineering context. The history of the role of materials in emerging technologies from a historical perspective will be briefly explored. The current literature will be used to demonstrate the connectedness of materials availability and the development and sustainability of engineering advances with examples of applications exploiting structural, electronic, optical, magnetic, and energy conversion properties. Processing will be comprehensively reviewed from source through refinement through processing including property development through application of an illustrative set of engineering materials representing commodities, less common metals, and minor metals. The concept of strategic recycling, including design for recycling and waste stream management will be considered. Offered as EMSE 335 and EMSE 435 . Prereq: Senior standing or graduate student.
EMSE 343. Processing of Electronic Materials. 3 Units.
The class will focus on the processing of materials for electronic applications. Necessary background into the fundamentals and applications will be given at the beginning to provide the basis for choices made during processing. MOSFET will be used as the target application. However, the processing steps covered are related to many other semiconductor based applications. The class will include both planar and bulk processing. Offered as: EMSE 343 and EMSE 443 . Prereq: ( PHYS 122 or PHYS 124 ) and EMSE 276 .
EMSE 345. Engineered Materials for Biomedical Applications. 3 Units.
A survey of synthetic biomedical materials from the perspective of materials science and engineering, focusing on how processing/synthesis, structure, and properties determine materials performance under the engineering demands imposed by physiological environments. Comparisons and contrasts between engineered metals, ceramics, and polymers, versus the biological materials they are called on to replace; consequences for materials and device design. Biomedical materials in applications such as orthopedic implants, dental restorations, wound healing, ophthalmic materials, and biomedical microelectromechanical systems (bioMEMS). Additive manufacturing of biomedical materials. Prereq: ENGR 200 and ENGR 145 .
EMSE 349. Role of Materials in Energy and Sustainability. 3 Units.
This course has two parts: engineered materials as consumers of resources (raw materials, energy); and as key contributors to energy efficiency and sustainable energy technologies. Topics covered include: Energy usage in the U.S. and the world. Availability of raw materials, including strategic materials; factors affecting global reserves and annual world production. Resource demand of materials production, fabrication, and recycling. Design strategies, and how the inclusion of environmental impacts in design criteria can affect design outcomes and material selection. Roles of engineered materials in energy technologies: photovoltaics, solar thermal, fuel cells, wind, batteries, capacitors. Materials in energy-efficient lighting. Energy return on energy invested. Semester projects will allow students to explore related topics (e.g. geothermal; biomass; energy-efficient manufacturing and transportation). Offered as EMSE 349 and EMSE 449 . Prereq: (ENGR 225 or EMAE 251 or EMAC 351 ) and ENGR 145 and ( PHYS 122 or PHYS 124 ) or Requisites Not Met permission.
EMSE 368. Thesis/Article Writing for Scientists and Engineers. 3 Units.
For writing a publication, such as a thesis or a journal article, in a field of science or engineering, students need a diverse set of skills in addition to mastering the scientific content. Generally, scientific writing requires proficiency in document organization, professional presentation of numerical and graphical data, literature retrieval and management, text processing, version control, graphical illustration, the English language, elements of style, etc. For scientists and engineers, specifically, writing a publication requires additional knowledge about e.g. conventions of numerical precision, error limits, mathematical typesetting, proper use of units, proper digital processing of micrographs, etc. Having to acquire these essential skills at the beginning of writing a thesis or a journal article may compromise the outcome by distracting from the most important task of composing the best possible scientific content. This course properly prepares students for scientific writing with a comprehensive spectrum of knowledge, skills, and tools enabling them to fully focus on the scientific content of their thesis or publication when the time has come to start writing. Similar to artistic drawing, where the ability to "see" is as (or more!) important as skills of the hand, the ability of proper scientific writing is intimately linked to the ability of critically reviewing scientific texts. Therefore, students will practice both authoring and critical reviewing of scientific texts. To sharpen students' skills of reviewing, examples of good and less good scientific writing will be taken from published literature in science and engineering and analyzed in the context of knowledge acquired in the course. At the end of the course, students will have set up skills and a highly functional work environment to start writing their role thesis or article with full focus on the scientific content. Students of all disciplines of science and engineering will benefit from the course material. Offered as EMSE 368 and EMSE 468 .
EMSE 372. Structural Materials by Design. 4 Units.
Materials selection and design of mechanical and structural elements with respect to static failure, elastic stability, residual stresses, stress concentrations, impact, fatigue, creep, and environmental conditions. Mechanical behavior of engineering materials (metals, polymers, ceramics, composites). Influence of ultrastructural and microstructural aspects of materials on mechanical properties. Mechanical test methods covered. Models of deformation behavior of isotropic and anisotropic materials. Methods to analyze static and fatigue fracture properties. Rational approaches to materials selection for new and existing designs of structures. Failure analysis methods and examples, and the professional ethical responsibility of the engineer. Four mandatory laboratories, with reports. Offered as EMAE 372 and EMSE 372 . Prereq: ENGR 200 .
EMSE 379. Design for Lifetime Performance. 3 Units.
The roles of processing and properties of a material on its performance, cost, maintenance, degradation and end-of-life treatment. Corrosion and oxidation, hydrogen and transformation-induced degradation mechanisms. Defects from prior processing. New defects generated during use/operation, e.g. generated from radiation, stress, heat etc. Accumulation and growth of defects during use/operation; crack nucleation, propagation, failure. Impact, abrasion, wear, corrosion/oxidation/reduction, stress-corrosion, fatigue, fretting, creep. Evaluation of degradation: Non-destructive versus destructive methods, estimation of remaining lifetime. Mitigation of degradation mechanisms. Statistical tools for assessing a material's lifetime performance. Capstone design project. Prereq: EMSE 372 . Coreq: EMSE 319 .
EMSE 396. Special Project or Thesis. 1 - 18 Units.
Special research projects or undergraduate thesis in selected material areas.
EMSE 398. Senior Project in Materials I. 1 Unit.
Independent Research project. Projects selected from those suggested by faculty; usually entail original research. The EMSE 398 and 399 sequence form an approved SAGES capstone. Counts as a SAGES Senior Capstone course.
EMSE 399. Senior Project in Materials II. 2 Units.
Independent Research project. Projects selected from those suggested by faculty; usually entail original research. Requirements include periodic reporting of progress, plus a final oral presentation and written report. Counts as a SAGES Senior Capstone course. Prereq: EMSE 398 .
EMSE 400T. Graduate Teaching I. 0 Unit.
To provide teaching experience for all Ph.D.-bound graduate students. This will include preparing exams/quizzes, homework, leading recitation sessions, tutoring, providing laboratory assistance, and developing teaching aids that include both web-based and classroom materials. Graduate students will meet with supervising faculty member throughout the semester. Grading is pass/fail. Students must receive three passing grades and up to two assignments may be taken concurrently. Recommended preparation: Ph.D. student in Materials Science and Engineering.
EMSE 408. Welding Metallurgy. 3 Units.
Introduction to arc welding and metallurgy of welding. The course provides a broad overview of different industrial applications requiring welding, the variables controlling critical property requirements of the weld and a survey of the different types of arc welding processes. The course details the fundamental concepts that govern the different aspects of arc welding including the welding arc, weld pool solidification, precipitate formation and solid state phase transformations. Offered as EMSE 308 and EMSE 408 .
EMSE 409. Deformation Processing. 3 Units.
Flow stress as a function of material and processing parameters; yielding criteria; stress states in elastic-plastic deformation; forming methods: forging, rolling, extrusion, drawing, stretch forming, composite forming.
EMSE 413. Fundamentals of Materials Engineering and Science. 3 Units.
Provides a background in materials for graduate students with undergraduate majors in other branches of engineering and science: reviews basic bonding relations, structure, and defects in crystals. Lattice dynamics; thermodynamic relations in multi-component systems; microstructural control in metals and ceramics; mechanical and chemical properties of materials as affected by structure; control of properties by techniques involving structure property relations; basic electrical, magnetic and optical properties.
EMSE 414. Electrical, Magnetic, Optical, and Thermal Properties of Materials. 3 Units.
Reviews quantum mechanics as applied to materials, energy bands, and density of states; Electrical properties of metals, semiconductors, insulators, and superconductors; Optical properties of materials, including: metallic luster, color, and optoelectronics; Magnetic properties of materials, including: Types of magnetic behavior, theory, and applications; Thermal properties of materials, including: heat capacity, thermal expansion, and thermal conductivity. Prereq: Graduate Standing in Materials Science and Engineering or Requisites Not Met permission.
EMSE 417. Properties of Materials in Extreme Environments. 3 Units.
Fundamentals of degradation pathways of materials under extreme conditions; thermodynamic stability of microstructures, deformation mechanisms, and failure mechanisms. Extreme conditions that will typically be addressed include: elevated temperatures, high-strain rates (ballistic), environmental effects, nuclear radiation, and small scales. Examples will be drawn from recent events as appropriate.
EMSE 421. Fracture of Materials. 3 Units.
Micromechanisms of deformation and fracture of engineering materials. Brittle fracture and ductile fracture mechanisms in relation to microstructure. Strength, toughness, and test techniques. Review of predictive models. Recommended preparation: ENGR 200 and EMSE 427 ; or consent.
EMSE 422. Failure Analysis. 3 Units.
Methods and procedures for determining the basic causes of failures in structures and components. Recognition of fractures and excessive deformations in terms of their nature and origin. Development and full characterization of fractures. Review of essential mechanical behavior concepts and fracture mechanics concepts applied to failure analyses in inorganic, organic, and composite systems. Legal, ethical, and professional aspects of failures from service. Prereq: EMSE 372 or EMAE 372 or Requisites Not Met permission.
EMSE 427. Defects in Solids. 3 Units.
Defects in solids control many properties of interest to the materials scientist or engineer. This course focuses on point, line, and interfacial defects in crystals and their interactions, including calculations of defect energies and interaction forces. Crystallographic defects presented include point defects (e.g., vacancies, interstitials, substitutional and interstitial impurities), line defects (e.g., dislocations), and planar defects (e.g., grain boundaries). The consequence of point defects on diffusion as well as on optical and electronic properties is discussed. Dislocation motion and dislocation dissociation are treated, and the influence of dislocation dynamics on yield phenomena, work hardening, and other mechanical properties are discussed. The role of grain boundaries and inter-phase boundaries in determining the physical properties of the material are presented. Experimental techniques for characterizing defects are integrated throughout the course. Recommended preparation: MATH 223 (or equivalent) and EMSE 276 (or equivalent).
EMSE 428. Mesoscale Structural Control of Functional Materials. 3 Units.
The course focuses on mesoscale structure of materials and their interrelated effects on properties, mostly in electrical in nature. The mesoscale science covers the structures varying from electronic- to micro-structure. In each scale, fundamental science will be complimented by examples of applications and how the structure is exploited both to modify and enable function. The student will develop an understanding of how the structure across multiple scales are interrelated and how to tailor them for desired outcomes. Offered as: EMSE 328 and EMSE 428 .
EMSE 435. Strategic Metals and Materials for the 21st Century. 3 Units.
EMSE 443. Processing of Electronic Materials. 3 Units.
EMSE 449. Role of Materials in Energy and Sustainability. 3 Units.
This course has two parts: engineered materials as consumers of resources (raw materials, energy); and as key contributors to energy efficiency and sustainable energy technologies. Topics covered include: Energy usage in the U.S. and the world. Availability of raw materials, including strategic materials; factors affecting global reserves and annual world production. Resource demand of materials production, fabrication, and recycling. Design strategies, and how the inclusion of environmental impacts in design criteria can affect design outcomes and material selection. Roles of engineered materials in energy technologies: photovoltaics, solar thermal, fuel cells, wind, batteries, capacitors. Materials in energy-efficient lighting. Energy return on energy invested. Semester projects will allow students to explore related topics (e.g. geothermal; biomass; energy-efficient manufacturing and transportation). Offered as EMSE 349 and EMSE 449 . Prereq: ENGR 225 and ( ENGR 145 or EMSE 146) and ( PHYS 122 or PHYS 124 ) or requisites not met permission.
EMSE 463. Magnetism and Magnetic Materials. 3 Units.
This course covers the fundamentals of magnetism and application of modern magnetic materials especially for energy and data storage technologies. The course will focus on intrinsic and extrinsic magnetic properties, processing of magnetic materials to achieve important magnetic performance metrics, and the state-of-the-art magnetic materials used today. The topics related to intrinsic properties, include: magnetic dipole moments, magnetization, exchange coupling, magnetic anisotropy and magnetostriction. Topics related to extrinsic properties, include: magnetic hysteresis, frequency dependent magnetic response and magnetic losses. Technologically important permanent magnets (including rare earth containing alloys and magnetic oxides), soft magnets (including electrical steels, amorphous, ferrites, and nanocrystalline alloys), and thin film materials (including iron platinum) will be discussed in the context of their technological interest. Throughout the course, experimental techniques and data analysis will be discussed. The course is suitable for most graduate students and advanced undergraduates in engineering and science.
EMSE 468. Thesis/Article Writing for Scientists and Engineers. 3 Units.
EMSE 499. Materials Science and Engineering Colloquium. 0 Unit.
Invited speakers deliver lectures on topics of active research in materials science. Speakers include researchers at universities, government laboratories, and industry. Course is offered only for 0 credits. Attendance is required.
EMSE 500T. Graduate Teaching II. 0 Unit.
To provide teaching experience for all Ph.D.-bound graduate students. This will include preparing exams/quizzes/homework, leading recitation sessions, tutoring, providing laboratory assistance, and developing teaching aids that include both web-based and classroom materials. Graduate students will meet with supervising faculty member throughout the semester. Grading is pass/fail. Students must receive three passing grades and up to two assignments may be taken concurrently. Recommended preparation: Ph.D. student in Materials Science and Engineering.
EMSE 503. Structure of Materials. 3 Units.
The structure of materials and physical properties are explored in terms of atomic bonding and the resulting crystallography. The course will cover basic crystal chemistry, basic crystallography (crystal symmetries, point groups, translation symmetries, space lattices, and crystal classes), basic characterization techniques and basic physical properties related to a materials structure.
EMSE 504. Thermodynamics of Solids. 3 Units.
Review of the first, second, and third laws of thermodynamics and their consequences. Stability criteria, simultaneous chemical reactions, binary and multi-component solutions, phase diagrams, surfaces, adsorption phenomena.
EMSE 505. Phase Transformations, Kinetics, and Microstructure. 3 Units.
Phase diagrams are used in materials science and engineering to understand the interrelationships of composition, microstructure, and processing conditions. The microstructure and phases constitution of metallic and nonmetallic systems alike are determined by the thermodynamic driving forces and reaction pathways. In this course, solution thermodynamics, the energetics of surfaces and interfaces, and both diffusional and diffusionless phase transformations are reviewed. The development of the laws of diffusion and its application for both melts and solids are covered. Phase equilibria and microstructure in multicomponent systems will also be discussed.
EMSE 515. Analytical Methods in Materials Science. 3 Units.
Microcharacterization techniques of materials science and engineering: SPM (scanning probe microscopy), SEM (scanning electron microscopy), FIB (focused ion beam) techniques, SIMS (secondary ion mass spectrometry), EPMA (electron probe microanalysis), XPS (X-ray photoelectron spectrometry), and AES (Auger electron spectrometry), ESCA (electron spectrometry for chemical analysis). The course includes theory, application examples, and laboratory demonstrations.
EMSE 599. Critical Review of Materials Science and Engineering Colloquium. 1 - 2 Units.
Invited speakers deliver lectures on topics of active research in materials science. Speakers include researchers at universities, government laboratories, and industry. Each course offering is for 1 or 2 credits but the course can be taken multiple times totaling up to a maximum of six credits. Attendance is required. Graded coursework is in the form of a term paper per credit. The topic for the term paper(s) should be chosen from seminar topics. The term paper will be graded by the advisor of the graduate student.
EMSE 600T. Graduate Teaching III. 0 Unit.
To provide teaching experience for all Ph.D.-bound graduate students. This will include preparing exam/quizzes/homework, leading recitation sessions, tutoring, providing laboratory assistance, and developing teaching aids that include both web-based and classroom materials. Graduate students will meet with supervising faculty member throughout the semester. Grading is pass/fail. Students must receive three passing grades and up to two assignments may be taken concurrently. Recommended preparation: Ph.D. student in Materials Science and Engineering.
EMSE 601. Independent Study. 1 - 18 Units.
EMSE 634. Special Topics of Materials Science. 1 - 3 Units.
This course introduces graduate students to specific topics of material science, tailored to individual interests of the students. For example, students with interest in specific techniques for microcharacterization of materials may be educated in the physical background of these techniques by studying literature under the guidance of the instructor, presenting and discussing the learned material with the instructor and other students, and being trained in practical experimentation in laboratory sessions demonstrating these techniques on instruments of SCSAM, the Swagelok Center for Surface Analysis of Materials.
EMSE 649. Special Projects. 1 - 18 Units.
EMSE 651. Thesis M.S.. 1 - 18 Units.
Required for Master's degree. A research problem in metallurgy, ceramics, electronic materials, biomaterials or archeological and art historical materials, culminating in the writing of a thesis.
EMSE 695. Project M.S.. 1 - 9 Units.
Research course taken by Plan B M.S. students. Prereq: Enrolled in the EMSE Plan B MS Program.
EMSE 701. Dissertation Ph.D.. 1 - 9 Units.
Required for Ph.D. degree. A research problem in metallurgy, ceramics, electronic materials, biomaterials or archeological and art historical materials, culminating in the writing of a thesis. Prereq: Predoctoral research consent or advanced to Ph.D. candidacy milestone.
Print Options
Print this page.
The PDF will include all information unique to this page.
- Biomedical Engineering
- Chemical & Biomolecular Engineering
- Civil and Environmental Engineering
- Computer & Data Sciences
- Electrical, Computer and Systems Engineering
- Macromolecular Science & Engineering
- Materials Science & Engineering
- Mechanical & Aerospace Engineering
- Intranet Login
Search form
Case school of engineering.
- Global Opportunities
- Outside The Classroom
Advanced Materials
- Big Data and Digital Solutions
- Energy and Sustainability
- High-Performance Manufacturing
- Human Health
- Micro-/Nano-electronics and Sensors
- Robotics and Motion Control
- Smart Systems and Cities
- Take Your Idea to Market
- Faculty Achievements
- Peer-Reviewed Publications
- Research News
Recent Grants
$155k | dod office of naval research | | "", $110k | american chemical society | mark de guire | "chemical expansivity in ceramic oxygen transport materials", $100k | nsf | gary wnek | "planning grant: engineering research center for advanced films & packaging", recent publications, through-thickness inhomogeneity of sensitization on environmentally assisted cracking in aa5083-h128 alloy, 3d interpenetrated graphene foam/58s bioactive glass scaffolds for electrical-stimulation-assisted differentiation of rabbit mesenchymal stem cells to enhance bone regeneration, facile synthesis of nanostructural high-performance cu-pb electrocatalysts for co2 reduction, gecko-inspired adhesive.
Gecko-inspired adhesive holds firm in extreme temperatures
Knotty polymers
Unraveling the secrets of knotty polymers
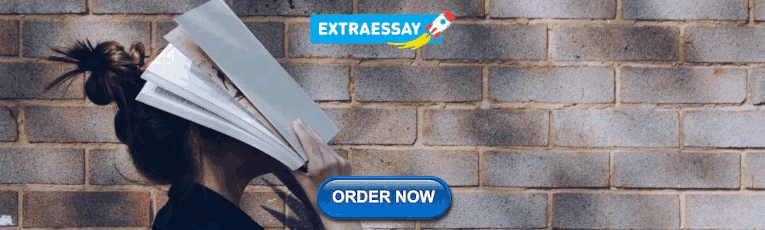
Materials Branch
Explore the materials branch
The range of elements available for device production has exploded in the past 20 years, enabling entirely new classes of electronic and mechanical products, biomedical implants, tissues, coatings and more.
We’re leaders in the world of advanced materials, with three of our school’s seven departments moving this field forward: Materials, Macromolecular Science and Engineering and Chemical and Biomolecular Engineering. And with our home in Northeast Ohio—a region brimming with material and chemical industry leaders—we collaborate on moving the latest materials technologies quickly from research to use.
Centers and institutes that conduct research in Advanced Materials
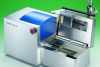
Center for Layered Polymeric Systems (CLiPS)
An NSF Science and Technology Center advancing the nation's science and technology agenda through the development of new materials and material systems
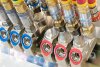
Advanced Manufacturing and Mechanical Reliability Center
Develops and provides mechanical testing for materials using an array of equipment.
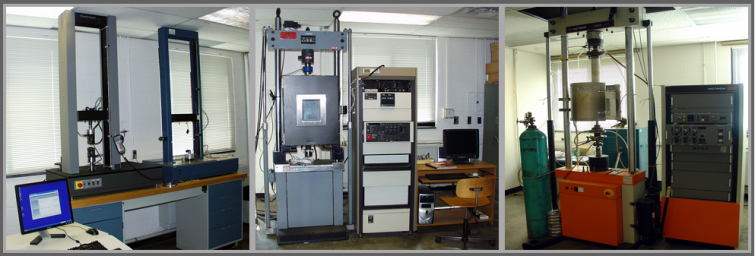
Swagelok Center for Surface Analysis of Materials
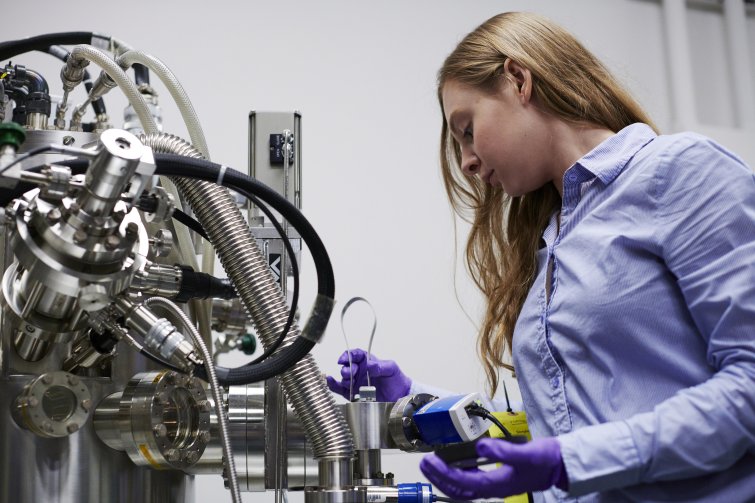
Provides expert staff and specialized analytical equipment to solve materials characterization challenges encountered in education, research and industry.
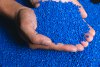
Center for Advanced Polymer Processing
Develops new advanced and functional multiphase complex materials and optimizes the performance of existing ones by integrating the most advanced experimental and computational capabilities.
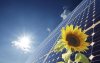
SDLE Research Center
Studies solar photovoltaic and other outdoor exposed technologies using degradation science, data science and analytics.
Faculty who conduct research in Advanced Materials
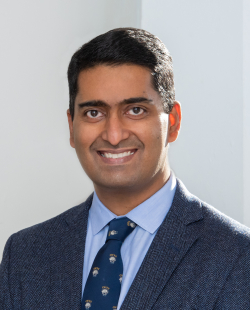
Rohan Akolkar
Develops new electrochemical processes for applications including nano-material fabrication, energy storage, electrometallurgy and sensors
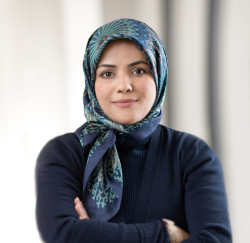
Hoda Amani Hamedani
Develops multifunctional materials and flexible nanostructured platforms for electrochemical and biomedical devices, localized drug delivery, neural interfacing, and electrochemical sensing; studies nanomaterials evolution/interactions in controlled (liquid) environments using in-situ characterization techniques
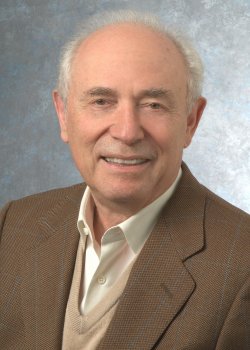
Develops processing-structure-property relationships in polymeric systems; creates micro- and nano-layered films; and produces biomimetic hierarchical structures of soft materials
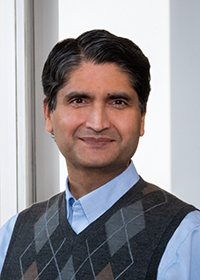
Harihara Baskaran
Understands and solves problems in biology and medicine using transport principles
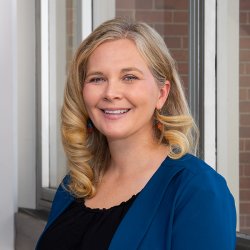
Laura Bruckman
Develops predictive lifetime models for materials degradation related to stress conditions and induced degradation mechanisms evaluated by quantitative spectroscopic characterization of materials
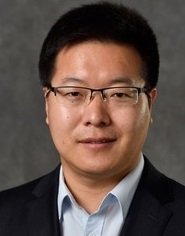
Changyong (Chase) Cao
Study the mechanics, designs, and manufacturing of smart multifunctional materials, soft robotics, soft electronics, and self-powered sensing systems.
Soft Machines & Electronics Lab
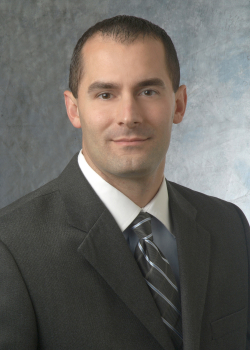
Jeff Capadona
Engineers biomaterials-based strategies to improve the performance and longevity of central nervous system implanted devices
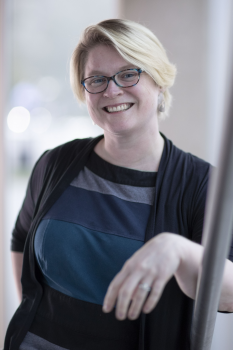
Jennifer Carter
deformation mechanisms of metals and metal-matrix composites; fatigue, fracture, and creep; failure analysis; electron microscopy; 3D microscopy; novel methodologies for multi-scale material characterization; data science and analytics; open science
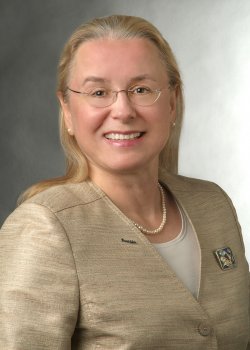
Sunniva Collins
Engineers metallic surfaces for improved performance; selects materials and designs manufacturing processes for innovative outcomes
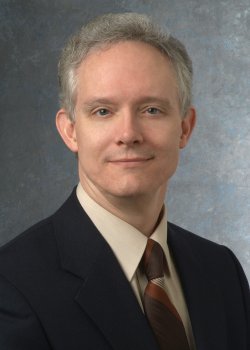
Mark De Guire
Analyzes performance of ceramics in energy applications, including fuel cells and oxygen transport membranes
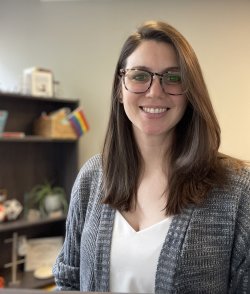
Christine Duval
Develops separation materials and processes to benefit nuclear medicine, environmental protection, and nuclear waste recycling and remediation.
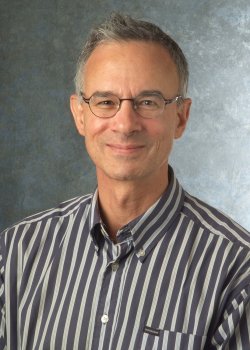
Steven Eppell
Designs, synthesizes and tests orthopedic biomaterials using biomimetic strategies emphasizing nanoscale structures and self-assembly
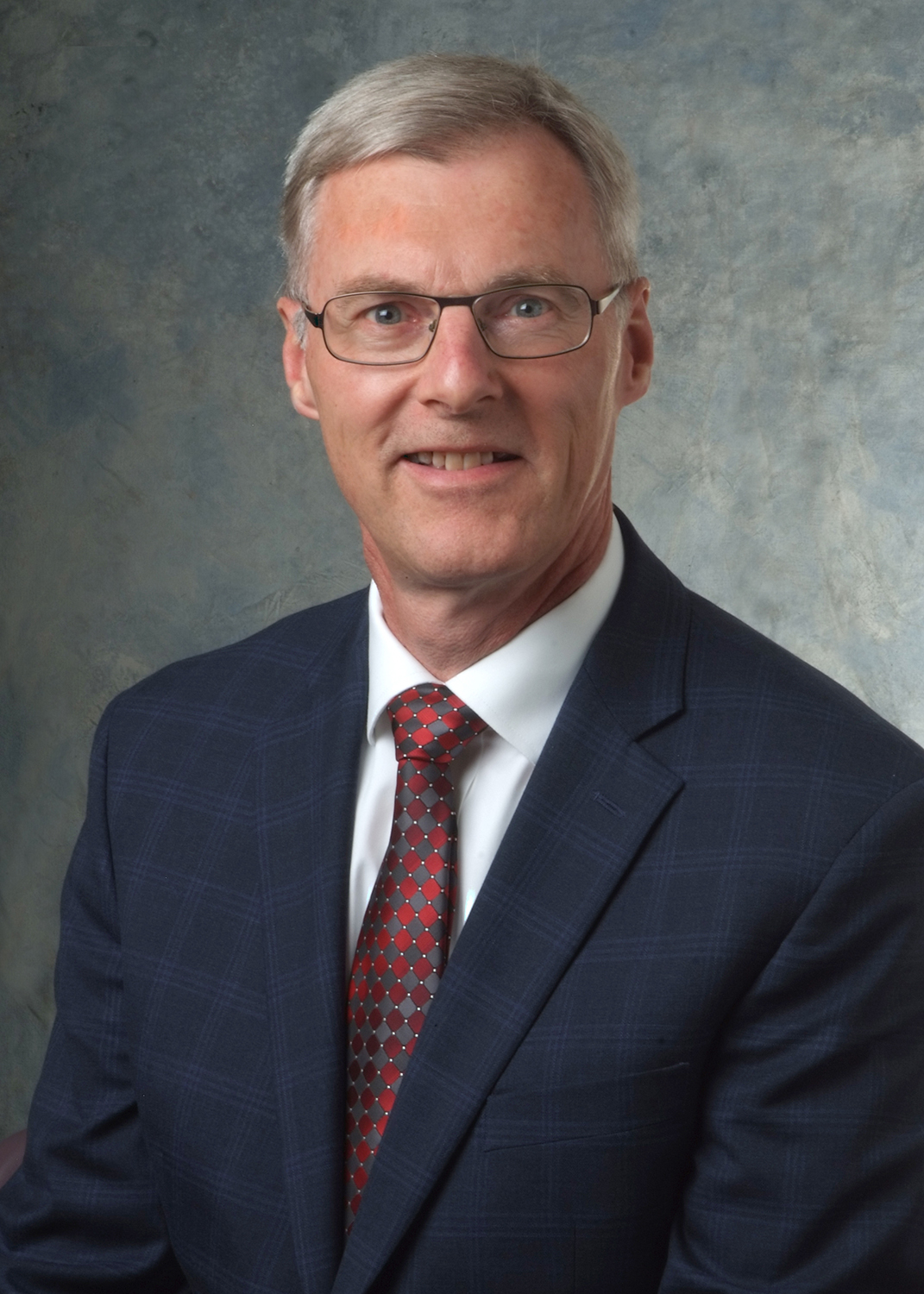
Frank Ernst
Studies and engineers microstructures, interfaces and surfaces of metallic materials by novel methods of processing and microcharacterization
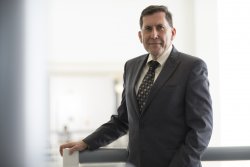
Donald Feke
Develops novel polymeric materials and ultrasonic-based separation processes for nano- and microscale multi-phase systems
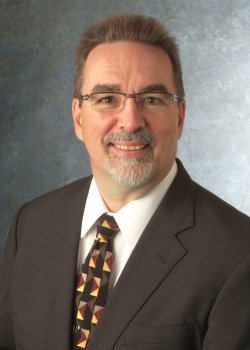
Roger French
Applies data science and analytics to energy and materials science research problems
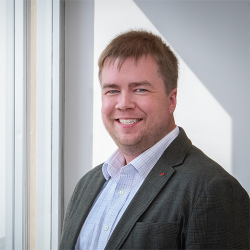
Michael Hore
Combines experiments, theory and simulation to study fundamental problems in the polymer physics of grafted and non-linear polymers
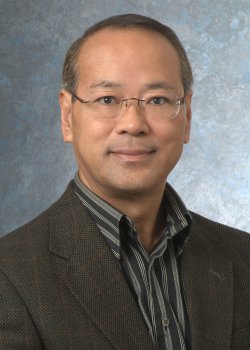
Hatsuo Ishida
Develops advanced benzoxazine resins, thermosetting resins based on natural renewable materials and green flame retarding systems
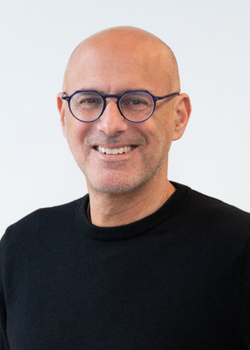
Daniel Lacks
Develops first-principles molecular-scale theories of chemical processes and materials properties
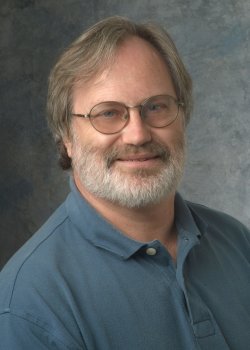
Peter Lagerlof
Develops a unified theory for plastic deformation via slip and deformation twinning
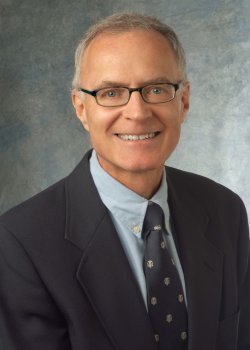
John Lewandowski
Researches material reliability for biomedical and structural applications, advanced materials manufacturing and processing/microstructure/property relationships. Hybrid Autonomous Manufacturing.
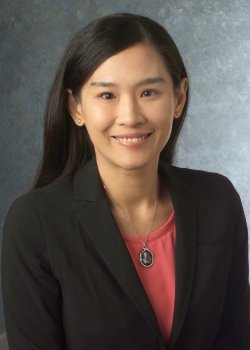
Ya-Ting Liao
Creates computational models of combustion, fire and fire behavior and develops fire-resistant structures
Computational Fire Dynamics Lab
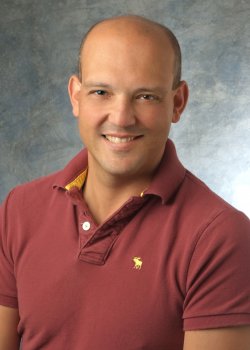
Develops multi-scale, complex polymer-based materials, using both experimental and computational-based tools
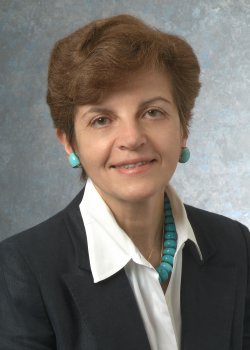
Ica Manas-Zloczower
Engineering new materials and technologies for industrial applications
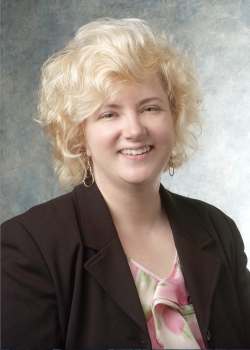
Heidi Martin
Develops diamond electrodes for electrochemical and neural device applications
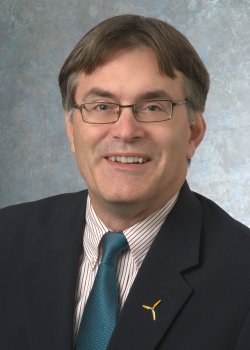
David Matthiesen
Develops process engineering solutions for the manufacturing of new magnetic materials
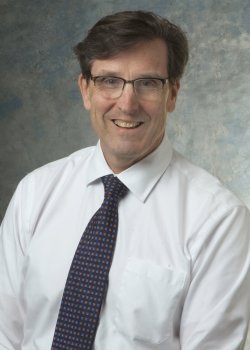
Jim McGuffin-Cawley
Develops new methodologies for material processing, characterizes processing-property relationships, studies high-temperature diffusion and solid-state reactions, fosters industry-university relationships
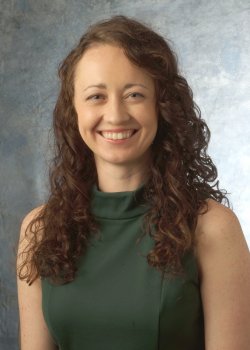
Julie Renner
Develops biomolecular platforms to control solid-liquid interfaces and enable a new generation of advanced technologies
Publications
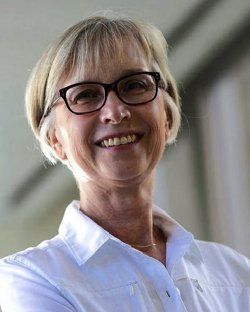
Clare Rimnac
Advances the long-term performance of implants and structural bone allografts through material analysis and characterization
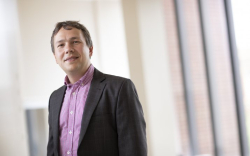
Valentin Rodionov
Investigates catalysis with soft materials: catalytic surfactants and polymers; complex macromolecular architectures for bio-inspired catalysis; and ligand-mediated nanocatalysis
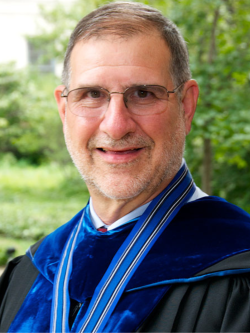
Robert Savinell
Develops high-performance electrochemical energy conversion and storage technologies through fundamental and applied studies of interfacial and transport processes
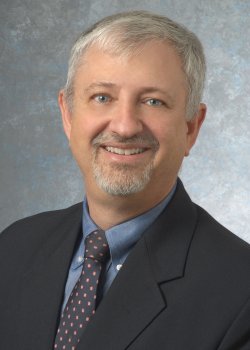
David Schiraldi
Develops bio-based, flame-retarded plastics, polymer aerogels and packaging materials; and studies properties of polyesters
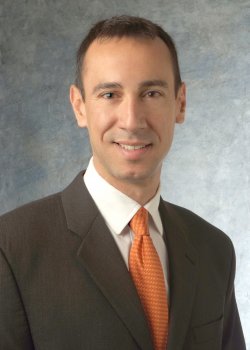
Alp Sehirlioglu
Develops new materials through exploitation of interfaces to control functionality and exploration of multi-functionality for energy-related applications
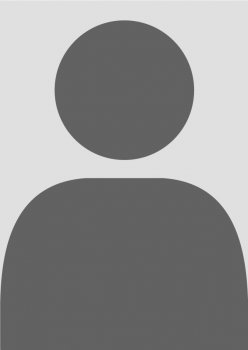
Tina Vrabec
Develops novel waveforms and electrodes for downregulation of the nervous system for clinical applications including peripheral, autonomic and pain
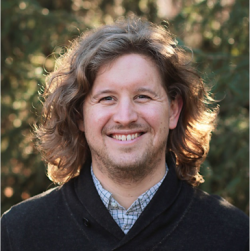
Robert Warburton
Develops computational models of interfacial chemical reactions relevant to applications in catalysis and energy storage
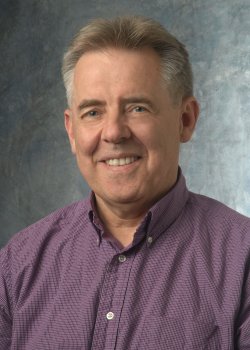
Gerhard Welsch
Develops new processing methods and designs for energy storage and optimized materials
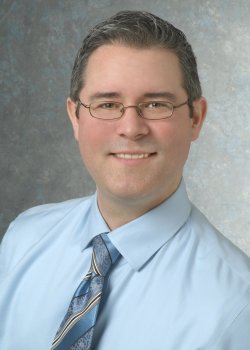
Matthew Willard
Investigates phase transformations and materials processing, especially their impact on structure and properties of materials
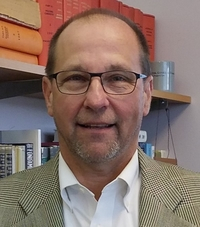
Develops advanced polymers for packaging, biomaterial applications and more.
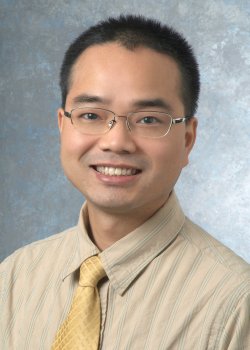
Multiscale multiphysics processes in geosystems and infrastructure, interdisciplinary innovations in intelligent infrastructure technologies towards resilience and sustainability.
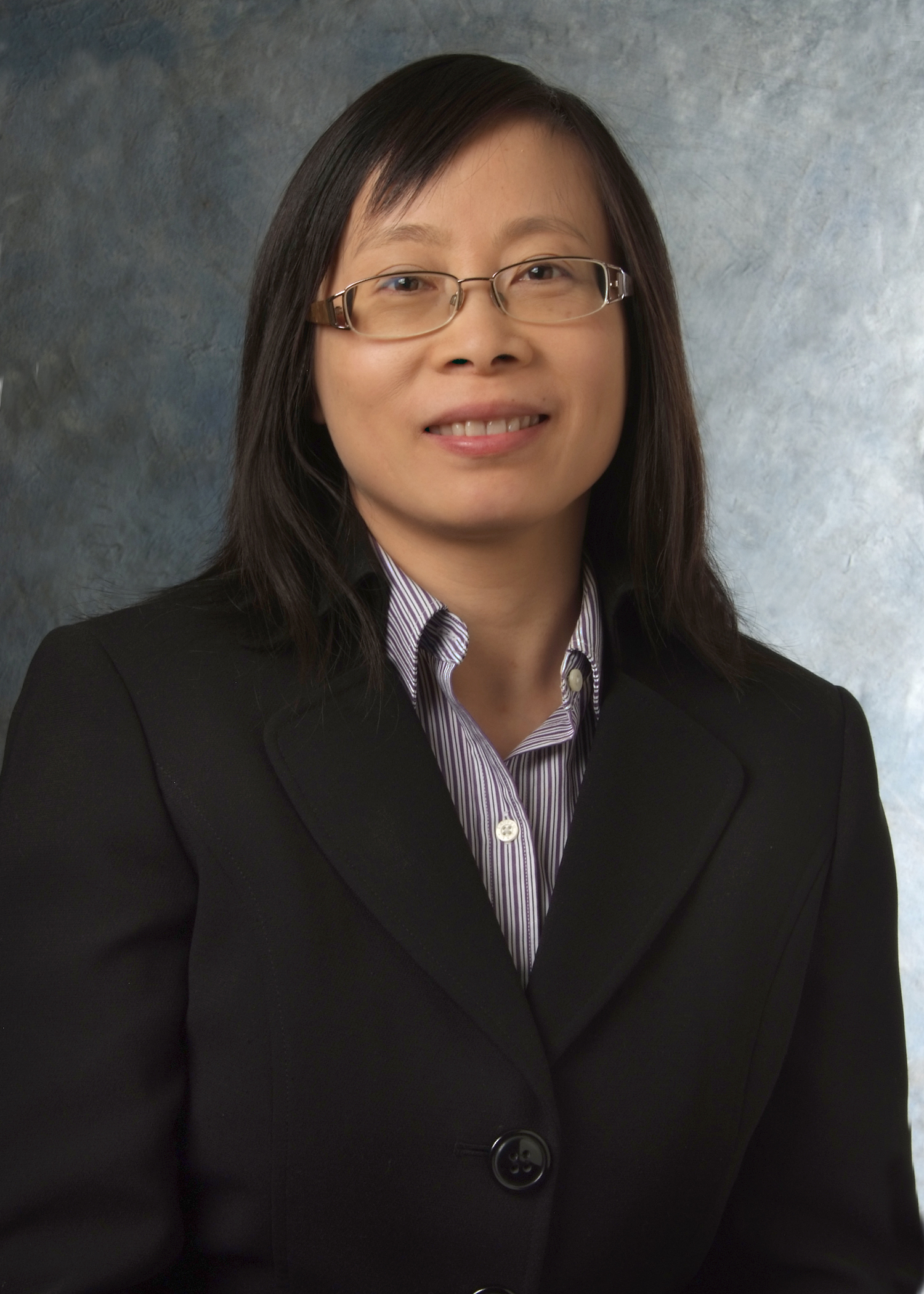
Huichun (Judy) Zhang
Examines fate and transformation of environmental contaminants in natural and engineered environments and develops advanced water/wastewater treatment technologies
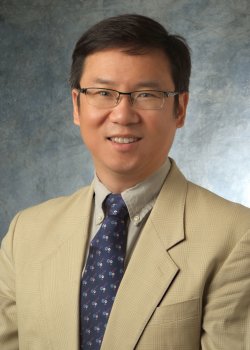
Develops high-energy and high-performance polymers based on close relationships among structure, property and processing
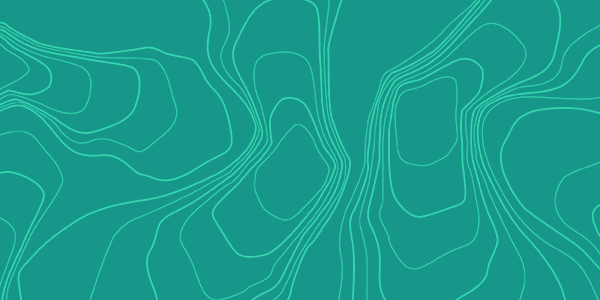
Engineering and Physical Sciences Research Council (EPSRC)
EPSRC creates knowledge in engineering and physical sciences for UK capability to benefit society and the economy.
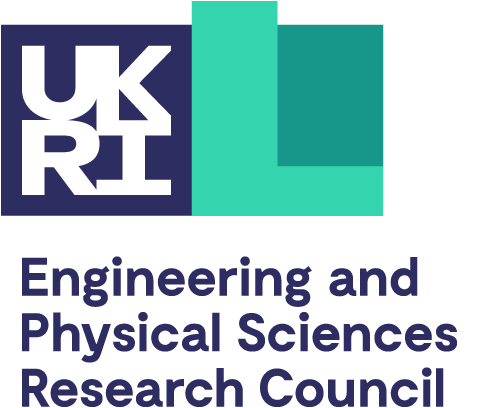
EPSRC content
- About EPSRC
- Funding, assessment and award management
- Strategy, remit and programmes
- People, skills and fellowships
- Innovation and collaboration
- Investments, impacts and engagement
- Institutes, facilities and resources
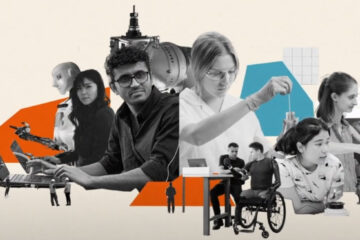
YouTube: We are the Engineering and Physical Sciences Research Council
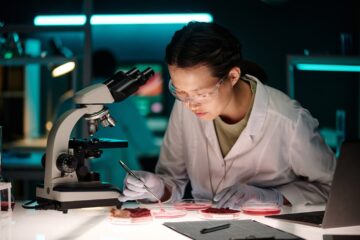
YouTube: Culturing meat for a sustainable food system
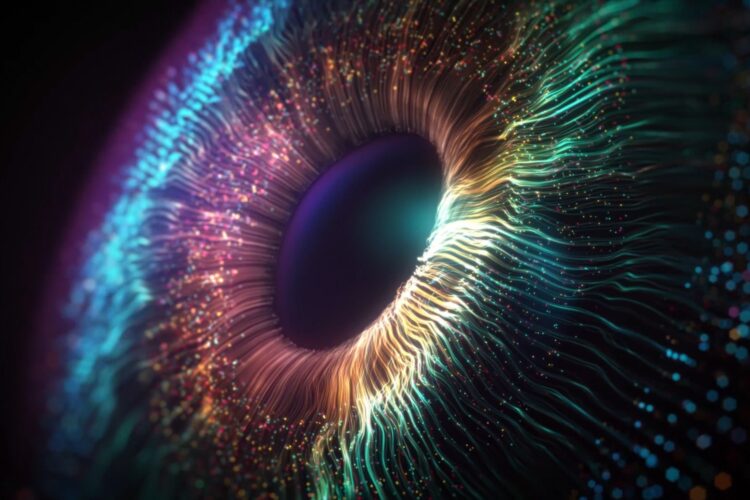
23 October 2024
World-leading researchers named as Turing AI fellows
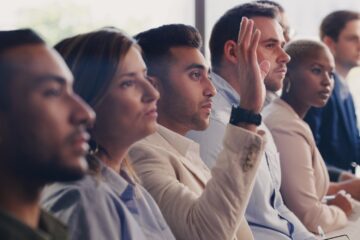
22 October 2024
UKRI seeks your views in our 2024 stakeholder perception survey
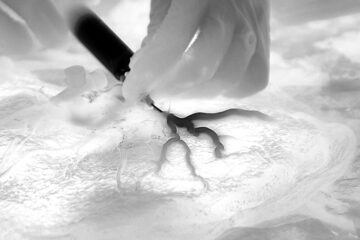
6 October 2024
Robotic clothing and ‘listening’ for cancer among new projects
View all EPSRC news
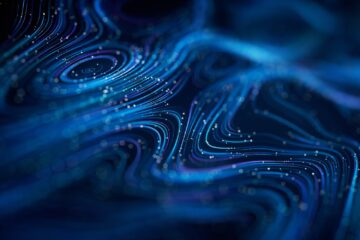
30 September 2024
Transforming biology with generative AI
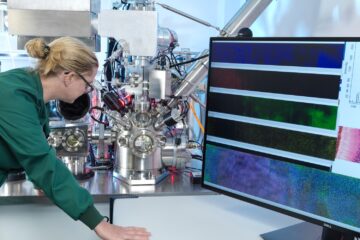
7 August 2024
My first 100 days as Director of the Rosalind Franklin Institute
View all EPSRC blog posts
Funding opportunities
Pre-announcement: future leaders fellowships: round 10.
Funding to support ambitious research and innovation across UK Research and Innovation (UKRI)’s remit. You must be an early career researcher or innovator who is either:
- looking to establish or transition to independence
- developing your own original and ambitious plans within a commercial setting
UKRI Future Leaders Fellowships Renewal Meeting 11 (Invite only)
Future Leaders Fellowship (FLF) award holders have the opportunity to extend their four-year fellowship by up to a further three years. The renewal funding will continue to support the development of a fellow and further the fellow’s work to undertake adventurous, ambitious programmes that tackle difficult and novel challenges.
View all EPSRC opportunities
Engineering and Physical Sciences Research Council (EPSRC) Polaris House, North Star Avenue, Swindon, SN2 1ET
Connect with EPSRC
Subscribe to ukri emails.
Keep up to date with funding, news and events, and a weekly newsletter.
UK and Japan quantum technology networking fair 2024
6 December 2024
View all EPSRC events
This is the website for UKRI: our seven research councils, Research England and Innovate UK. Let us know if you have feedback or would like to help improve our online products and services .
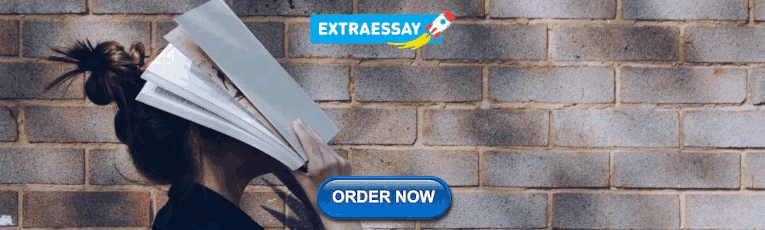
IMAGES
VIDEO
COMMENTS
Project-based classes, Engineering pedagogy, Materials Science Education, Technical Communications pedagogy, Teamwork and management education INTRODUCTION Materials science plays a role in many industries and is crucial to innovation and new product development. From a technical standpoint, materials scientists need a strong background in
Learn how Nankai University's School of Material Science and Engineering has grown its research capacities and achievements in various fields of material science, such as rare earth, energy ...
Prof. Kimerling will lead a short in-class discussion on the approach for each Case Study or Project. Q&A is encouraged to clarify specific details. The Instructors and TAs will moderate online Discussion Forums: within this Forum students should post their team's tentative outline, develop concepts, discuss sources and preliminary findings.
Learn from internationally renowned faculty and access world-class equipment in materials science and engineering at Case Western Reserve University. Choose from three formats of master's degree: accelerated, professional or research, and explore cutting-edge topics like data science, additive manufacturing and energy conversion.
Learn about the four strategic areas of research in Materials Science and Engineering at Cornell: Energy Production and Storage, Electronics and Photonics, Bioinspired Materials and Systems, and Green Technologies. Explore the relevant research topics and areas for each strategic area and the interdisciplinary nature of the field.
This study takes a material engineering approach to choosing a material and compares it to the actual steps that were taken to reach a solution." Skateboard Wheels: Reserve desk - Lewis, G., Selection of Engineering Materials, Skateboard Wheels, Chapter 16, Prentice Hall, Englewood Cliffs, New Jersey, 1990. 143 - 148. This case study focuses on ...
Research-informed Education in Materials Science and Engineering: A Case Study 3 Journal of Materials Education Vol. 38 (1-2) achieved due to the disparate X-ray absorption
Grade assignment for the Case Studies and Projects will account for the following: presentation and writing skills; clarity and rationality of the design execution; presentation of background, issues, alternatives and conclusions; Project 1: Engineering the Future of Solar Electricity. All student work is presented with permission of the authors.
Aimed at both graduate and undergraduate students as well as non-specialist researchers in materials science and engineering, including ceramics, metallurgy, and chemistry, this is an ideal introductory and reference book. ... Case studies on energy materials design 13. Summary and future development of materials design Appendix A Appendix B ...
The current investigation is a case study of project-based learning methodology (PBL) applied to one of the most widespread engineering basic courses: introduction to materials science and engineering. The project was conducted with first year undergraduate students of Mechanical Engineering. For this, some of the theory-based class lecture was reduced to fit 40% of a multidisciplinary project ...
Advanced Materials Science and Engineering Concentration. Students may satisfy the concentration requirement by taking 15 credit hours of courses from engineering, math, statistics, or natural sciences departments (beyond those specifically required in the curriculum) at the 300 level or above, plus a course to satisfy the Mathematics/Natural Sciences/Statistics requirement in the Engineering ...
The Library's support for Materials Science and Engineering undergraduate students had, in the past, predominantly taken the form of face-to-face induction sessions at the start of each academic year. The aim of this project was to collaboratively explore how and where Library content and ...
Learn how to create and improve materials for various applications in this ABET-accredited bachelor's degree program. Explore five areas of concentration, research opportunities and career paths in materials science and engineering.
Congratulations to Anuvi Gupta, a sophomore in the Department of Materials Science and Engineering, on being selected as a Case School of Engineering at Case Western Reserve University SOURCE - STEM 2023 Summer Research Scholar. Tuesday, August 1, 2023
This case study when presented on the website should peak some interest, give understanding and encourage growth in the material science knowledge of its readers. Case Study. The following brief case study outlines bicycle component design through materials selection. In Material Science Engineering understanding the material selection process ...
Within science, technology, engineering, and mathematics (STEM) disciplines, James B. Conant at Harvard University was the first science educator in the late 1940s to teach an entire course using case studies via the lecture format (Conant & Van Deventer, 1951).
The Department of Materials Science and Engineering of the Case School of Engineering offers programs leading to the degrees of Bachelor of Science in Engineering, Master of Science, and Doctor of Philosophy. ... (PLR) determination and power forecasting. This study includes a set of timeseries datasets for 100,000 PV plant inverters and ...
Materials Science Battery Case Study : Project Introduction : Topic Introduction . What is a Battery? Components of Cells and Batteries : How do Batteries Work? How many Different Types of Batteries are there? Classification of Cells or Batteries ...
A peer-reviewed journal that publishes studies on the load-bearing capacity of materials influenced by their properties, microstructure and environment. Find the latest articles, aims and scope, editorial board, and special issues on topics such as nanomechanical testing and additive manufacturing.
The range of elements available for device production has exploded in the past 20 years, enabling entirely new classes of electronic and mechanical products, biomedical implants, tissues, coatings and more. We're leaders in the world of advanced materials, with three of our school's seven departments moving this field forward: Materials, Macromolecular Science and Engineering and Chemical ...
EPSRC funds research and innovation in engineering and physical sciences to benefit UK society and economy. Find out about EPSRC's latest news, funding opportunities, programmes and contact details.
Frank Ernst is the department chair and a professor of materials science and engineering at Case Western Reserve University. He studies and engineers microstructures, interfaces and surfaces of metallic materials by novel methods of processing and microcharacterization.