COVID-19: impact on Public Health and hypothesis-driven investigations on genetic susceptibility and severity
Affiliations.
- 1 Departamento de Genética Humana, Instituto Nacional de Saúde Doutor Ricardo Jorge (INSA,IP), Lisboa, Portugal. [email protected].
- 2 Instituto de Investigação do Medicamento (iMed.ULisboa), Faculdade de Farmácia, Universidade de Lisboa, Lisboa, Portugal. [email protected].
- 3 Atlántida Centro de Investigación y Desarrollo de Estudios Profesionales (CIDEP), Granada, Spain.
- 4 MED-Instituto Mediterrâneo para a Agricultura, Ambiente e Desenvolvimento, Escola de Ciências e Tecnologia, Universidade de Évora, Évora, Portugal.
- 5 Université Claude Bernard Lyon 1, Lyon, France.
- 6 Departamento de Doenças Infeciosas, INSA, IP, Lisboa, Portugal.
- 7 Host-Pathogen Interaction Unit, Instituto de Investigação do Medicamento (iMed.ULisboa), Faculdade de Farmácia, Universidade de Lisboa, Lisboa, Portugal.
- 8 Hospital Egas Moniz, Centro Hospitalar Lisboa Ocidental, Lisboa, Portugal.
- PMID: 35348847
- PMCID: PMC8961091
- DOI: 10.1007/s00251-022-01261-w
COVID-19 is a new complex multisystem disease caused by the novel coronavirus SARS-CoV-2. In slightly over 2 years, it infected nearly 500 million and killed 6 million human beings worldwide, causing an unprecedented coronavirus pandemic. Currently, the international scientific community is engaged in elucidating the molecular mechanisms of the pathophysiology of SARS-CoV-2 infection as a basis of scientific developments for the future control of COVID-19. Global exome and genome analysis efforts work to define the human genetics of protective immunity to SARS-CoV-2 infection. Here, we review the current knowledge regarding the SARS-CoV-2 infection, the implications of COVID-19 to Public Health and discuss genotype to phenotype association approaches that could be exploited through the selection of candidate genes to identify the genetic determinants of severe COVID-19.
Keywords: COVID-19; Candidate gene association studies (CGAS); Genetic determinants of severe disease; Genetic susceptibility to infection; Genome-wide association studies (GWAS); Public Health.
© 2022. The Author(s), under exclusive licence to Springer-Verlag GmbH Germany, part of Springer Nature.
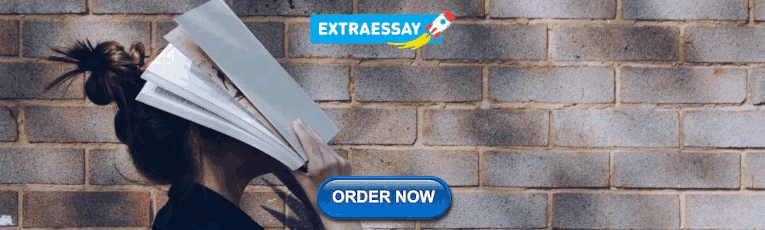
Publication types
- COVID-19* / genetics
- Genetic Predisposition to Disease
- Public Health
Click through the PLOS taxonomy to find articles in your field.
For more information about PLOS Subject Areas, click here .
Loading metrics
Open Access
Peer-reviewed
Research Article
Changing risk factors for developing SARS-CoV-2 infection from Delta to Omicron
Roles Conceptualization, Data curation, Formal analysis, Funding acquisition, Investigation, Methodology, Supervision, Visualization, Writing – original draft, Writing – review & editing
Affiliation Norwich Medical School, University of East Anglia, Norwich, United Kingdom

Roles Writing – original draft, Writing – review & editing
* E-mail: [email protected]
- Paul R. Hunter,
- Julii Brainard
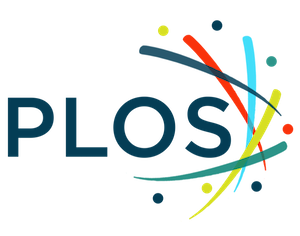
- Published: May 15, 2024
- https://doi.org/10.1371/journal.pone.0299714
- Peer Review
- Reader Comments
One of the few studies to estimate infection risk with SARS-CoV-2 in the general population was the UK Office of National Statistics Infection Survey. This survey provided data that allowed us to describe and interpret apparent risk factors for testing positive for SARS-CoV-2 in a period when variants and COVID-19 controls experienced large changes.
The ONS published estimates of likelihood of individuals testing positive in two week monitoring periods between 21 st November 2021 and 7 th May 2022, relating this positivity to social and behavioural factors. We applied meta-regression to these estimates of likelihood of testing positive to determine whether the monitored potential risk factors remained constant during the pandemic.
Some risk factors had consistent relationship with risk of infection (always protective or always linked to higher risk, throughout monitoring period). Other risk factors had variable relationship with risk of infection, with changes seeming to especially correlate with the emergence of Omicron BA.2 dominance. These variable factors were mask-wearing habits, history of foreign travel, household size, working status (retired or not) and contact with children or persons age over 70.
Relevance of some risk factors to likelihood of testing positive for SARS-CoV-2 may relate to reinfection risk, variant infectiousness and status of social distancing regulations.
Citation: Hunter PR, Brainard J (2024) Changing risk factors for developing SARS-CoV-2 infection from Delta to Omicron. PLoS ONE 19(5): e0299714. https://doi.org/10.1371/journal.pone.0299714
Editor: Marwan Osman, Yale University School of Medicine, UNITED STATES
Received: October 27, 2023; Accepted: February 14, 2024; Published: May 15, 2024
Copyright: © 2024 Hunter, Brainard. This is an open access article distributed under the terms of the Creative Commons Attribution License , which permits unrestricted use, distribution, and reproduction in any medium, provided the original author and source are credited.
Data Availability: The data used in this study are not owned by the authors and therefore cannot be directly publicly redistributed by the authors for instance, by placing the dataset in a public archive. However, the data are currently publicly available at: https://www.ons.gov.uk/peoplepopulationandcommunity/healthandsocialcare/conditionsanddiseases/datasets/coronaviruscovid19infectionsinthecommunityinengland/25may2022 .
Funding: PRH and JB were funded by the National Institute for Health Research Health Protection Research Unit (NIHR HPRU, grant NIHR200890) in Emergency Preparedness and Response at King’s College London in partnership with the UK Health Security Agency (UKHSA), in collaboration with the University of East Anglia. The views expressed are those of the authors and not necessarily those of the NHS, the NIHR, any of our employers, the Department of Health or the UKHSA. The funders had no role in study design, data collection and analysis, decision to publish, or preparation of the manuscript.
Competing interests: The authors have declared that no competing interests exist.
Introduction
Since the start of the COVID-19 pandemic in December 2019 there have been hundreds if not thousands of publications seeking to address the risk factors for deleterious COVID-19 outcomes. However, relatively few of those publications were well designed to identify risk factors for infection, whether or not symptomatic, rather than risk factors for symptomatic infection, hospitalisation and deaths. One of the more powerful studies was based on the UK Biobank cohort which analysed a cohort of almost a quarter of a million people. In that study, non-modifiable risk factors for confirmed infection in March to May 2020 (when testing in the UK was limited to persons with substantial medical need or occupational risk) included male sex, black ethnicity and socioeconomic deprivation [ 1 ]. Another comprehensive government agency early review of the evidence into disparities in risk and outcomes from COVID-19 infections in patients detected between February and April 2020 inclusive [ 2 ] found that age and ethnicity were major drivers of infection and severe outcome. These early pandemic studies and many other COVID-19 risk studies overwhelmingly related to patients with symptomatic illness if not actual severe disease.
As far as we are aware, the only community-based studies of infection as opposed to symptomatic infection that also reported on behavioural risk factors is the coronavirus infection survey in the UK conducted by the UK Office of National Statistics (CIS ONS). This was a cohort study that aimed to sample up to 150,000 individuals over the age of 2 years every fortnight [ 3 ]. The CIS ONS was the least biased epidemic tracker because it was designed to collect data on all cases, including very mild, pre-symptomatic and asymptomatic cases [ 4 ]. As such, the CIS ONS gave the most complete picture of infection spread at any one time, and risk of infection, at any severity.
ONS published its estimates of prevalence and incidence of COVID-19 weekly, the last report was in March 2023 (ONS 2023b). In addition, ONS published risk factor analyses in its “characteristics report” once or twice a month from June 2021 to November 2022 [ 5 ]. These analyses include estimates of risk by during two-week periods for amongst other things prior vaccination and infection, occupational factors, age and gender, overseas travel, and wearing of face coverings. Risk factors were presented for each of subsequent two-week periods enabling determination of association between specific factors and case status.
We report a study to determine whether risk factors for infection changed after the emergence of the omicron variant. We explored whether risk factors for infection remained the same as they were prior to the emergence of Omicron or if they changed. In addition, we wanted to know whether risk avoidance behaviours, such as mask wearing, continued to be associated with reduced risk.
All data included in this study come from publicly available analyses produced by the UK Office of National Statistics (ONS) and were published online [ 5 ]. The ONS covid survey recruited about 200,000 people and took throat swabs for COVID-19 using rt PCR every two weeks. Basic prevalence estimates were published every week and for a limited time period, the ONS published its COVID-19 characteristics analyses where they reported risk factors for testing positive in each two-week period. The outcome variable in the ONS analyses was whether or not someone tested positive and did not distinguish between whether someone had symptoms or not. The data for the analyses presented here were published on 25 th May 2022 [ 5 ]. After that date, analyses changed to presenting data on risk factors for reinfection only. The analyses used here presented estimated likelihood of testing positive along with the standard error for various risk factors in sequential fortnightly periods between 21 st November 2021 and 7 th May 2022, as this was the period when ONS published the relevant analyses. These likelihoods were calculated by ONS from models that controlled for age, region, sex, ethnicity, deprivation, household size, multi-generational household, and urban/rural classification as well as vaccination history and history of prior infection. The UK Census as undertaken by ONS defines a mutigenerational household as “ Households where people from across more than two generations of the same family live together . This includes households with grandparents and grandchildren whether or not the intervening generation also live in the household ” [ 6 ].
The primary data is not publicly available, and our analyses were done on the published summary data for each fortnightly time period. We consider separately the likelihood of testing positive over the whole period, as well as the likelihood of testing positive in any single report period (n = 12 in the monitoring period). Because we do not have access to the primary data, we used meta-regression, with the metareg tool in STATA 17.0. Initial analyses were the random effects pooled likelihood of testing positive for each risk factor over the included time periods. Then an analysis for trend was undertaken by adding period number (one to 12) as a fixed effect to the model. Those risk factors with a significant trend analysis were then used in fir tree graphs generated using STATSDirect 3.6. Significance threshold was set at p < 0.05.
We discuss the results with respect to concurrent dominant variant and government epidemic control policies. The time period covered by these analyses include the final few weeks when the Delta variant was dominant in the UK (until 19 December 2021), after which Omicron variant BA.1 was dominant until 1 March 2022 and Omicron variant BA.2 was dominant until 15 June 2022 [ 7 ]. The introduction and easing of non-pharmaceutical control measures varied between the four devolved administrations that comprise the UK, but the data presented here were most influenced by concurrent controls regime in England (the most populous administrative region). The end of all COVID-19 controls in England was announced on 21 Feb 2022 [ 8 ], which stated that the legal requirement to self-isolate stopped on 24 Feb and that guidelines to self-isolate as well as free testing would cease on 1 April 2022. Because this is a secondary analysis of published aggregate data, ethical approval was not required.
The meta-regression analyses are shown in Table 1 . Likelihoods of testing positive allowing for selected risk factors in specific periods are shown in Figs 1 – 4 . S1-S29 Figs in S1 Appendix show the pattern for those risk factors for which trend was not statistically significant at the p = 0.05 level. In this monitoring period, the risk factors not associated with any likelihood of testing positive and for which there was no significant trend were work in health or social care (p = 0.848), work in a patient facing role (p = 0.534), work in a care or nursing home (p = 0.468) and work in contact with others (p = 0.066). Variables that were associated with difference in the likelihood of testing positive but there was no significant change (p > 0.005 for trend over time) over the study period were:
- PPT PowerPoint slide
- PNG larger image
- TIFF original image
a. 2 people in household, b. 3 people in household, c. 4 people in household, d. 5 or more people in household.
https://doi.org/10.1371/journal.pone.0299714.g001
a. Adults living with someone age 16 years or younger, b. Adults aged under 70 years and living with someone age 70 years or older.
https://doi.org/10.1371/journal.pone.0299714.g002
a. School children in enclosed spaces, b. School children in school, c. Adults in enclosed spaces, d. Adults at school or at work.
https://doi.org/10.1371/journal.pone.0299714.g003
a. Living in urban city or town compared to major urban area, b. Being retired compared to being employed and going to work, c. Travelled abroad in recent 28 days compared to no foreign travel in last 28 days.
https://doi.org/10.1371/journal.pone.0299714.g004
https://doi.org/10.1371/journal.pone.0299714.t001
- Gender, with females consistently less likely to test positive (likelihood -0.051, p < 0.001)
- Being an ethnic minority was linked to less positivity (likelihood -0.119, p = 0.012)
- People from a multi-generational household were less likely to test positive (likelihood -0.055, p = 0.025)
- People were less likely to test positive if in the previous 28 days they had contact with a care home (likelihood –0.126, p = 0.008) or a hospital (likelihood -0.176, p < 0.001)
- Tobacco smokers were less likely to test positive (likelihood -0.170, p < 0.001)
- Living in a house with someone who was disabled was associated with lower risk (likelihood –0.126, p < 0.001)
- Being disabled meant lower risk of having infection (likelihood -0.075, p = 0.005)
- Increasing numbers of physical contacts and socially distanced contacts in the previous 7 days were also associated with increased risk. For physical contacts risk plateaued about 10 such contacts and for socially distanced contacts these plateaued about 20 such contacts.
Other risk factors for infection were not as simple to describe and were even variable during the monitoring period. There was a complex relationship between household size and positivity. People living with others, especially two or more others, had consistent increased risk of testing positive ( Table 1 , likelihood of testing positive increased with household size, p always < 0.001). Figs 1 – 4 show the likelihood of testing positive for selected risk factors with confidence intervals, in each of the 12 two week periods. Between the figures on each row in the figures is a chart indicating changing proportions of tested samples in each of the Delta and Omicron variants over the concurrent time period, earliest at top to latest at bottom. This may facilitate interpretation about how changes in variants changed or not with each risk factor. Fig 1 focuses on household size, with likelihoods shown compared to singleton households. The likelihoods of testing positive generally decreased for households with 3 or more members after 12 Feb 2022, but the likelihood of testing positive increased for households with size = 2 in the period from January to May 2022. The greatest decrease in likelihood of testing positive was for the largest households (n = 5+). By the end of the study period people in larger households (4 and above) had negligibly greater risk than people living in singleton households.
Although overall there was no difference in risk between people who lived with or did not live with someone aged ≤ 16 years (likelihood = 0.035, p = 0.504), there was evidence of significant change (trend over time p = 0.015). Fig 2A shows that there was a notable negative association with risk from about end February onwards. People aged under 70 years who lived with someone 70 years or older had overall lower likelihood of testing positive (likelihood = -0.097, p = 0.034), and this relationship also had a significant change over time (p = 0.021). However, detailed analysis ( Fig 2B ) is that those sharing households with older adults were actually only at reduced risk for the first half of the study period. By about mid-February there was little effect on risk of infection.
Compared to always wearing face coverings, school-aged children who never wore face coverings had an insignificantly higher likelihood of testing positive ( Table 1 : likelihood was 0.028 in enclosed spaces, p = 0.533; likelihood was 0.051 at school, p = 0.462). However, both of these comparisons had significance for trend over time. Fig 3A and 3B show that the likelihood of testing positive for never-wearers was higher (significantly so) in the early monitoring period (before end February 2022) in both settings, and much lower in the March-May period (although not usually significantly different from the always-wearers at p < 0.05).
Compared to always wearing face-coverings, adults had higher risk of testing positive ( Table 1 : 0.146 in enclosed spaces, p = 0.019; 0.077 at school or work, p = 0.019). Again, both of these comparisons had significance for trend over time. Fig 4C and 4D show that never wearing a face covering was much more strongly linked to positivity before early-mid January, and by end May not wearing a face covering in adults had no effect on risk for adults in these settings.
There was no evidence for trend over time for likelihood of infection in rural towns or villages (p > 0.005 in Table 1 ), but change over time was indicated for minor urban areas compared to major urban areas (p = 0.005). Compared to living in a major urban area, people in smaller urban locations had lower risk of testing positive during the first half of the monitored period ( Fig 4A ), and then greater risk of positivity which coincided with the emergence of BA.2 dominance and announcements about lifting of all COVID-19 restrictions on or around 1 March 2022.
Being retired was associated with reduced risk ( Table 1 , likelihood -0.127, p < 0.001) compared to those in work overall, but any protective effect had disappeared by 27 Feb 2022 ( Fig 4B ), which coincided with the start of the second Omicron wave.
Travel abroad was not associated any increased risk before 2 January, but after Omicron became dominant, travel abroad had a substantially increased association with risk of infection ( Fig 4C ), likelihood = 0.45 or 0.54 in January 2022. This elevated likelihood of testing positive linked to travel abroad subsequently declined somewhat in the Feb-May 2022 period.
We have shown that effect of many of the posited risk or protective factors for COVID-19 infection varied during the course of the pandemic in the UK. Some factors that were associated with lower risk for testing positive during the Delta and Omicron BA.1 periods (eg., such as being retired or wearing face coverings) were no longer associated with any risk difference or even an increased risk during the second Omicron wave and after it was announced that restrictions would be lifted. Some factors that were associated in our data with increased risk of testing positive early on, such as living with someone under age 16 or children wearing face-coverings, were associated with much reduced or even no risk later on. Multi-generational households had no time trend change in risk of infection, although size of household did have a changing relationship with risk of infection over time; this suggests that multi-generational households are not reliably also the largest households. Some risk factors such as working in health or social care or in contact with others, were often found to be important in the first year of the pandemic [by July 2020: 9 , 10 ], but were not associated with overall higher or changing risk of infection in our study period. Being an ethnic minority was strongly associated with increased risk in the first few months of the UK epidemic [by May 2020: 11 ], but was associated with lower risk and no significant trend change during our full monitoring period.
We are not the first investigators to find that risk factors for COVID-19 changed during the course of a within-country epidemic. In a large case-controlled study of symptomatic people with confirmed SARS-CoV-2 positivity in the USA, Hansen and colleagues (2022) [ 12 ] noted that reported contact with a known case was highly associated with infection during the Delta period but much less so for Omicron. Unlike our analysis, that study did not include any behavioural characteristics. In a study using international datasets, Sharma and colleagues (2021) [ 13 ] found that many of the non-pharmaceutical interventions that were effective in early 2020, such as school closures and gathering bans, were less effective in the winter 2020/21 wave.
The finding that wearing face coverings ceased being protective after the first Omicron wave is worthy of further discussion. It is possible that behavioural changes drove this change in part, as wearing face coverings became less expected after the announcements that COVID-19 restrictions were being lifted. Physical contact rates in all settings likely rose sharply in wake of the announcements about the plans for “Living with COVID-19”. However, behavioural changes may not explain all changes in risk factor association with infection. Biological changes such as increased infectivity with new variants or waxing and waning immunity in the population seem likely to also cause differences in risk or protective factors. The balance of evidence is that wearing face coverings reduces transmission of respiratory infections in community settings and did reduce transmission of COVID-19 (Chou et al. 2023). The question, however, is by how much. Systematic review of pre-pandemic evidence [ 14 ] and analysis of original survey data during the COVID-19 pandemic [ 15 ] both indicated that mask wearing could or did reduce transmission of SARS-CoV-2 by about 19%. But these conclusions were derived mainly from data prior to the emergence of Omicron variants.
From the data presented here, prior to Omicron BA.2, never wearing a mask was associated with an increased risk of around 30% in adults and 10% in children. But by the second Omicron wave (mid to late February 2022 onwards) there was no protective effect from mask wearing in adults and possibly an increased risk of infection in children. The need for children to wear masks in school has been a topic of considerable debate. Budzyn and colleagues [ 16 ] reported that in the summer of 2021, those US counties that did not have school mask mandates in place reported a greater increase in paediatric COVID-19 infections compared to those that did. However, this study only covered the first two weeks of the school term. In an analysis of the same data source but including data from many more counties and for a longer period of time. Chandra and Høeg (2022) [ 17 ] found that by the ninth week after school start, any association between mask mandates in schools and reduced risk of infection had disappeared. They also concluded that once confounding factors such as poverty and a Social Vulnerability Index were included, counties imposing mask mandates saw more paediatric infections.
The question remains what could be the mechanism(s) that help to explain why protective interventions that were associated with decreased risk early in the pandemic become less protective or even associated with increased risk. Similarly, we can ask why did some variables that were associated with increased risk early in the pandemic cease to be associated with risk of infection later.
We propose that two biological mechanisms were relevant. The first hypothesis is that because Omicron was more infectious than previous variants [ 18 ], interventions that had previously been able to suppress the effective reproduction number to keep it close to 1.0 were no longer as effective, thereby increasing risk of infection in circumstances where previously risk was relatively low. Our finding that the risk associated with international travel increased substantially after the appearance of Omicron supports this hypothesis. However, we note that the change in risk associated with foreign travel applies to a period when international travel was rapidly becoming easier (pandemic travel restrictions were being eased). This change in opportunity may have correlated with changes in other risk factors and behaviours not recorded. In any event, the first hypothesis does not seem likely to explain all of our findings, such as the shift from reduced to increased risk associated with wearing face coverings in school.
The second hypothesis is linked to immunity from prior infection. People who accept greater risk are more likely to have been infected and so more likely to be immune later in the pandemic and at lower risk of infection. Early in the pandemic most published epidemic models of the COVID-19 pandemic were of the SIR or SEIR form (Susceptible, Exposed, Infected and Recovered) [ 19 ]. However, these models perform badly after the first wave for infections like COVID-19 where immunity to reinfection is not long lasting [ 20 ]. As is now well known, COVID-19 infection and immunisation only provides immunity against further infection for a relatively limited period, of the order of just a few months [ 21 ]. A more appropriate epidemic model for COVID-19 at least after the first wave is the SEIRS model (Susceptible, Exposed, Infected, Recovered and Susceptible) [ 20 ]. In the SEIRS model, an epidemic in a previously naive population will pass through a series of diminishing waves until the infection becomes endemic. The COVID-19 pandemic has generally followed this path [ 22 ]. As the epidemic transitions to become endemic the drivers of infection change and then a major driver of infection rates is the rate at which immunity is lost. At this point, interventions that would have had a major impact on slowing transmission early in the epidemic phase have less influence. That non pharmaceutical interventions have value primarily in the early stages of a pandemic is something that has been known for some time. Their value lies largely in delaying most people’s infections until a suitable vaccine becomes available [ 23 ].
Ideally, we would have used the primary individual level data and undertaken some form of longitudinal panel or multilevel mixed effects regression analysis. However, we only had access to summary data for each time period. Thus, the data were effectively clustered by time period [ 24 ]. Calculating summary data for each cluster followed by standard analysis of that summary data is an acceptable approach [ 23 ]. The main weakness of this approach is that just using the central estimate does not take account of the precision of each summary value [ 23 ]. Meta-analysis is an approach that combines the results from different studies whilst accounting for the precision of each study [ 25 ]. Thus, our use of meta-regression allowed us to use the summary data from each time period whilst adjusting for the precision of each estimate.
In conclusion we have shown that risk factors for infection and effectiveness of public health interventions are not fixed but sometimes, and may commonly, change during the course of a pandemic. It is plausible and seems most likely that at least partial explanations for such changes are increased immunity in people at high risk of infection as the epidemic progressed, and the rate at which such immunity is lost or maintained by repeat exposure.
Supporting information
S1 appendix. changing covid risk factors..
https://doi.org/10.1371/journal.pone.0299714.s001
Acknowledgments
We acknowledge the essential contribution from the Office of National Statistics.
- View Article
- PubMed/NCBI
- Google Scholar
- 6. ONS. Census 2021 dictionary: Office for National Statistics; 2021 [cited 2024 Jan 29]. Available from: https://www.ons.gov.uk/census/census2021dictionary .
- 7. Office for National Statistics. Coronavirus (COVID-19) latest insights: Infections: ONS; 2023 [updated Mar 30; cited 2023 Sep 27]. Available from: https://www.ons.gov.uk/peoplepopulationandcommunity/healthandsocialcare/conditionsanddiseases/articles/coronaviruscovid19latestinsights/infections .
- 24. Kirkwood BR, Sterne JA. Essential medical statistics. 2nd ed: John Wiley & Sons; 2010.
- 25. Borenstein M, Hedges LV, Higgins JP, Rothstein HR. Introduction to Meta-analysis: John Wiley & Sons; 2021.
- - Google Chrome
Intended for healthcare professionals
- Access provided by Google Indexer
- My email alerts
- BMA member login
- Username * Password * Forgot your log in details? Need to activate BMA Member Log In Log in via OpenAthens Log in via your institution

Search form
- Advanced search
- Search responses
- Search blogs
- News & Views
- What do we know about...
What do we know about covid-19’s effects on the brain?
- Related content
- Peer review
- Katharine Lang , freelance journalist
- lang.kathj26{at}gmail.com
Katharine Lang investigates how SARS-CoV-2 infection can affect the brain, how it might have these effects, and what can be done to minimise the risk
What are the neurological symptoms of covid-19?
Covid may be primarily a respiratory infection, but a common symptom is “brain fog”—problems with memory or concentration—which can persist for weeks or months as part of long covid. 1 And it’s not the only neurological effect.
Giovanni Schifitto, professor of neurology at the University of Rochester Medical School in New York, explains that neurological symptoms are widespread. “In the acute phase, common things like lack of smell, changes in taste, increase in headaches, cognitive dysfunction, and strokes have been reported, and there are effects on the peripheral nervous system,” he says.
Although these complications are more likely in people with severe covid—a 2021 study found that 80% of people admitted to hospital with covid-19 experienced neurological symptoms 2 —they can also affect people who experience only mild covid symptoms. 3 These neurological symptoms were also found to be more likely in people who were older, male, or white and in people with pre-existing neurological disorders.
The most common neurological symptoms are loss of smell (anosmia) and loss of taste (ageusia). Studies have suggested that during a bout of covid as many as 68% of people experience loss of smell and 44% lose their sense of taste. 4 5 But no evidence suggests that these effects are due to the virus entering the brain.
Other studies have reported a range of neurological symptoms alongside general fatigue. These symptoms are much like those experienced after a “mild traumatic brain injury” (as defined in one study 6 ) or from concussion—including dizziness, headaches, seizures, stroke, delirium, and balance disturbances.
The UK Covid and Cognition study (Covcog, from the University of Cambridge) reported cognitive dysfunction in some 70% of people with signs of long covid (or “post-covid condition,” 7 although it’s worth noting that there are no agreed international diagnostic criteria for long covid), and brain fog and difficulty concentrating were found to be more common than lasting respiratory symptoms. 8 The Covcog study found that the severity of lasting symptoms was related to the severity of symptoms during acute covid.
Data from the UK Coronavirus (Covid-19) Infection Survey from March 2023 9 showed that difficulty concentrating was the second most common symptom in long covid (after fatigue) and was experienced by 51% of respondents. More recently, a community based study used online assessments to evaluate cognitive impairment after covid infection. 10 Participants who had been infected showed measurable long term cognitive deficits not evident in the no-covid group.
What causes these symptoms? Is it the virus entering the brain?
This is a complex issue and the subject of much debate. In vitro studies have found that the virus disrupts the blood-brain barrier, although this was seen only with the original wild-type SARS-CoV-2 and omicron variants. 11 This research also found that the virus may affect the function of cells in the central nervous system.
Because loss of smell is a common symptom, some researchers have suggested that the virus may enter the brain through the olfactory system. Stephen Griffin, virologist at the University of Leeds, notes that the UK Biobank study 12 recorded “reduced grey matter thickness—comprised of the main cellular ‘bodies’ of neurons, rather than their tendril-like projections—in parts of the brain linked with olfactory sensing, namely the limbic system and primary olfactory cortex,” which could be evidence for this route of entry.
However, there’s little other evidence that the virus enters cells in the brain. Schifitto says, “There’s been one report suggesting the virus gets into astrocytes 13 ; others have not really found the virus in specific cells.”
But he adds, “The virus can cause damage without infecting cells. If it’s activating other cells in the brain that cause inflammation, you don’t need the virus to be there to cause problems. The amount of cytokines in the blood could activate, for example, microglia, a classical cell type involved in chronic neuroinflammation.”
Does inflammation play a role?
The consensus is that inflammation, rather than invasion by the virus, is highly likely to be responsible for the neurological symptoms seen in both acute and long covid. One 2023 study found raised levels of brain injury markers and inflammatory mediators during the acute phase of covid. 14
Daniel Altmann, professor of immunology at Imperial College London, says, “Many ideas have been put forward, but just as there can be immunopathology in any other organ—for example, through excessive inflammatory cytokines—so it is for the brain.”
He cites a 2022 study in mice and people 15 that showed lasting post-covid cytokine activity, particularly CCL11 (eotaxin 1) in the central nervous system. CCL11 is known to play a role both in allergic conditions and in brain disorders. 16 Studies in mice revealed that, as well as elevated cytokines/chemokines in the cerebrospinal fluid, the myelin on nerve cells was damaged, which could be one explanation for cognitive issues. Little evidence suggests that demyelination has been seen in people after covid.
Another study, published in October 2023, has proposed an alternative theory: that peripheral serotonin reduction after covid impedes the vagus nerve—impairing the responses of the hippocampus, which is involved in learning, memory, and emotion. 17
What about microbleeds and strokes associated with covid-19?
Many people have reported microbleeds and strokes after infection. Schifitto says that the virus often infects endothelial cells anywhere in the body, including those in the brain vessels. He explains, “It’s not surprising, if something is affecting the endothelial cells, that this may predispose people to small strokes, large strokes, or dysregulation of the blood-brain barrier, which then could predispose to additional inflammation coming from the bloodstream into the brain.”
Altmann says, “There is massive evidence for this. Many mechanisms have been demonstrated for endothelial, prothrombotic effects of the virus, so the effect is fairly easily rationalised.”
What’s the effect of covid-19 on dementia?
Schifitto expresses concern that covid may lead to an increase in dementias, telling The BMJ that dementias are “almost always a mix with vascular disease included—so if SARS-CoV-2 has had an impact on the microcirculation, we may see an increase in vascular disease in the brain, which may contribute to earlier or more aggressive dementia. That’s a concern, but there’s no evidence so far.”
Benedict Michael, professor of neuroscience at the University of Liverpool, says that this risk may well not apply to the whole population but could potentially increase the likelihood of dementia in people already at increased risk of neurodegenerative disease.
Schifitto adds, “Most dementias do not progress rapidly, so it would be unlikely that we’d see a surge within a few years.” He’s currently investigating neuroimaging biomarkers to see if these might give early warning of small vessel disease or microcirculation problems in people who have had covid.
Can vaccination help reduce the neurological effects of covid-19?
The only way to avoid neurological symptoms during and after infection is to avoid catching covid in the first place, says Altmann. But vaccination could also reduce the risk.
Griffin points to preclinical studies 18 indicating that vaccination can prevent SARS-CoV-2 from damaging the brain in some experimental models. He adds, “Plus, we know that vaccination is one of the few things that reduce the risk of long covid.”
Griffin warns that the long term consequences of covid aren’t being considered enough when planning vaccination and other prevention strategies in the UK—“which would seem most unwise given the accumulating evidence of long term harm in neurological and indeed other tissues.”
Competing interests: None declared.
Provenance and peer review: Commissioned; peer reviewed.
This article is made freely available for personal use in accordance with BMJ's website terms and conditions for the duration of the covid-19 pandemic or until otherwise determined by BMJ. You may download and print the article for any lawful, non-commercial purpose (including text and data mining) provided that all copyright notices and trade marks are retained.
- McCorkell L ,
- GCS-NeuroCOVID Consortium and ENERGY Consortium
- Fastenberg JH ,
- Granholm AC
- ↵ World Health Organization. Post-covid 19 condition (long covid). 7 Dec 2022. https://www.who.int/europe/news-room/fact-sheets/item/post-covid-19-condition
- Benito Ballesteros A ,
- ↵ Office for National Statistics. Prevalence of ongoing symptoms following coronavirus (covid-19) infection in the UK: 30 March 2023. 30 Mar 2023. https://www.ons.gov.uk/peoplepopulationandcommunity/healthandsocialcare/conditionsanddiseases/bulletins/prevalenceofongoingsymptomsfollowingcoronaviruscovid19infectionintheuk/30march2023
- Hampshire A ,
- Atchison C ,
- Skorniewska Z ,
- PHOSP-COVID Study Collaborative Group
- Alfaro-Almagro F ,
- Michael BD ,
- Needham EJ ,
- ISARIC4C Investigators ,
- COVID-CNS Consortium
- Fernández-Castañeda A ,
- Geraghty AC ,
- Ivanovska M ,
- Murdjeva M ,
- Devason AS ,
- Villadiego J ,
- García-Arriaza J ,
- Ramírez-Lorca R ,
- Alzheimer's disease & dementia
- Arthritis & Rheumatism
- Attention deficit disorders
- Autism spectrum disorders
- Biomedical technology
- Diseases, Conditions, Syndromes
- Endocrinology & Metabolism
- Gastroenterology
- Gerontology & Geriatrics
- Health informatics
- Inflammatory disorders
- Medical economics
- Medical research
- Medications
- Neuroscience
- Obstetrics & gynaecology
- Oncology & Cancer
- Ophthalmology
- Overweight & Obesity
- Parkinson's & Movement disorders
- Psychology & Psychiatry
- Radiology & Imaging
- Sleep disorders
- Sports medicine & Kinesiology
- Vaccination
- Breast cancer
- Cardiovascular disease
- Chronic obstructive pulmonary disease
- Colon cancer
- Coronary artery disease
- Heart attack
- Heart disease
- High blood pressure
- Kidney disease
- Lung cancer
- Multiple sclerosis
- Myocardial infarction
- Ovarian cancer
- Post traumatic stress disorder
- Rheumatoid arthritis
- Schizophrenia
- Skin cancer
- Type 2 diabetes
- Full List »
share this!
May 20, 2024
This article has been reviewed according to Science X's editorial process and policies . Editors have highlighted the following attributes while ensuring the content's credibility:
fact-checked
peer-reviewed publication
trusted source
From infection to chronic illness: Learning from long COVID
by American Society for Microbiology
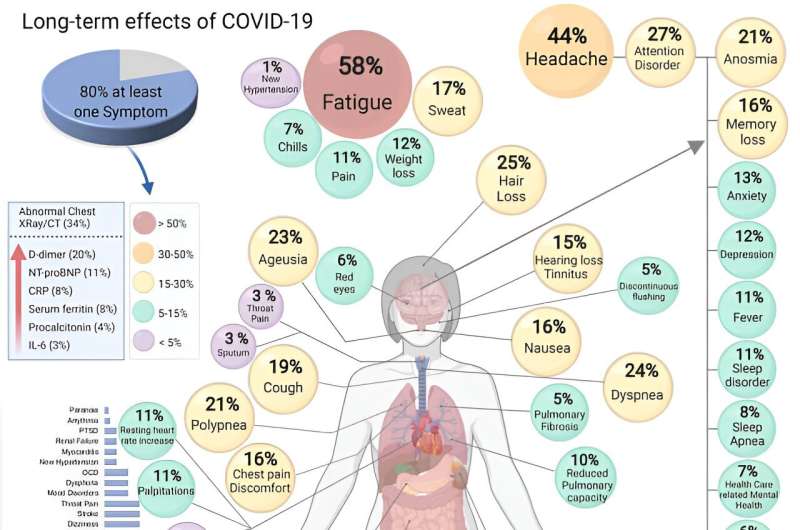
Since the COVID-19 pandemic, SARS-CoV-2 has caused unexplained, chronic, post-infection symptoms in survivors. The phenomenon, known as post-acute sequelae of SARS-CoV-2 infection or "long COVID," surfaced shortly after COVID-19 testing was introduced to the public, and doctors began noting a pattern of onset of chronic fatigue and dysautonomia in patients who had recovered from acute COVID-19 infection.
Yet, SARS-CoV-2 is not the only pathogen that has been linked to chronic symptoms. While there are known links between bacterial and viral infections with post-acute infection syndromes , much is still unknown about the mechanisms that underpin these illnesses or why they occur.
A silver lining of the COVID-19 pandemic is that it reignited research interest in this field. How can we better recognize and treat these chronic disabilities? We dive into the research to better understand the relationship between microbe and host, as well as the immunological landscape these pathogens leave behind.
What microbes can lead to post infection chronic illnesses?
Epstein-barr virus.
The Epstein-Barr Virus (EBV), one of nine known human herpesvirus types, and the most common viruses to infect humans, has long been associated with an array of different diseases and conditions, including systemic lupus erythematosus, Sjögren syndrome and MS.
This double stranded DNA virus is primarily transmitted through saliva, earning it the moniker "the kissing virus" and is the known etiological agent of infectious mononucleosis.
The principal targets of EBV infection are B cells, the type of white blood cells that make antibodies. EBV can directly infect B cells in the oral cavity or access them indirectly via oral epithelial cells. Following infection, EBV can establish lifelong latency in infected B cells. Reactivation of EBV can be triggered by stress or immunosuppressant treatment. Upon reactivation, the virus can be transmitted to new hosts.
Researchers have been focusing on pathogenic mechanisms that lead to post infection chronic stimulation of the immune system. One hypothesis is that pathogen remnants (i.e., viral RNA), which contain pathogen associated molecular patterns (PAMPs) stay in the host at undetectable levels and persistently activate host pathogen recognition receptors.
Constant activation of these receptors can cause continuous inflammation, subsequently damaging host tissue and potentially initiating autoimmune activation. This is not exclusive to EBV and applies to a wide range of post-acute infection syndromes.
The establishment of latency to later propagate throughout a host's lifespan may help explain the connection between EBV and autoimmune activation. One example is the link between Pityriasis lichenoides chronica (PLC) and EBV.
PLC is a rare skin disease that results in scaly, red or brown bumps across the trunk and extremities, which can come and go (relapsing and remitting) for months to years. Research indicates that PLC papules are remnants of different subsets of overactivated T cells that remain after viral infections.
While the cause of PLC ultimately remains unknown, it has been linked to EBV as a hypersensitivity reaction in patients with elevated EBV titers. It is still unknown whether recent infection alone is the cause of PLC, or if there is more to this immune dysregulation that gets repeatedly activated from previous EBV infection.
Similarly to EBV, post-infected COVID-19 patients have been diagnosed with the inflammatory rash Pityriasis Lichenoides et Varioliformis Acuta .
Another hypothesis on the cause of post-acute infection syndromes is that acute infections may induce autoimmune activation by impairing regulatory T cells or through molecular mimicry of self-antigens by the pathogen.
Studies have shown that EBV is linked to a >30-fold increased risk of developing MS, and an EBV protein (EBV nuclear antigen 1) has been found to elicit auto-antibody cross reactivity with central nervous glial cell adhesion molecules of the host.
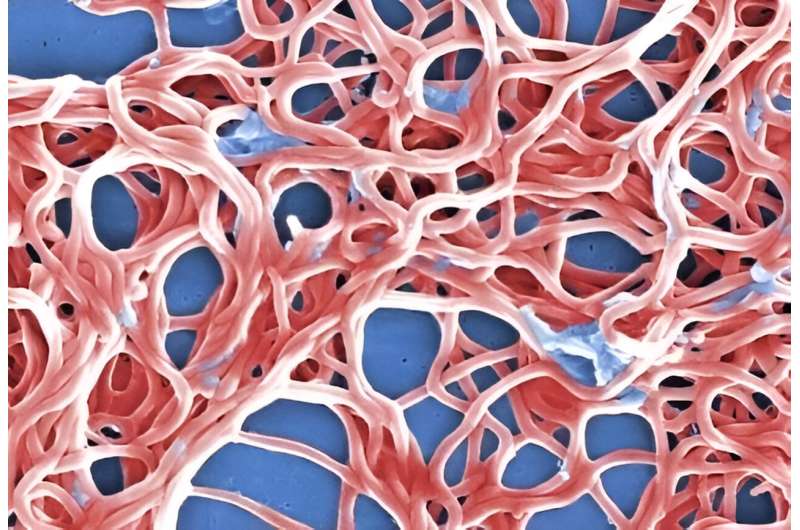
Borrelia burgdorferi
Borrelia burgdorferi is another example of a pathogen that appears to cause post-acute infection syndromes. B. burgdorferi is the bacterium that causes Lyme disease and is transmitted to humans by blacklegged ticks in different regions of the U.S. and abroad.
While Lyme disease can be treated with antibiotics, post-treatment Lyme disease syndrome (PTLDS) is a rising health concern. PTLDS is a condition where patients experience chronic fatigue, pain and cognitive dysfunction for at least six months following antibiotic treatment and clearance of infection.
There is debate in the Lyme disease research field about the plausibility of PTLDS as a syndrome caused by Lyme disease itself, as many symptoms associated with PTLDS are seen in other post-acute infection syndromes. The U.S. Center for Disease Control (CDC) does recognize long term symptoms associated with post Lyme disease clearance as PTLDS.
Potential causes of PTLDS may be permanent tissue damage following infection, an activated autoimmune response or an undetectable ongoing Borrelia infection. However, ongoing infections in the context of PTLDS have not been seen in clinical settings, and the CDC recommends that the term "Chronic Lyme disease" not be utilized as it implies that there is an ongoing bacterial infection causing PTLDS symptoms when the cause currently remains unknown.
One hypothesis on cause of PTLDS is loss of "tolerance building." In "tolerance building" carrier hosts show no symptoms of Lyme disease (i.e., arthritis, carditis) because an immune tolerance has been built to essentially ignore the bacteria, similar to how we mitigate inflammation in the gut microbiome with commensal bacteria.
Linden Hu, M.D., a professor of immunology at Tufts Medical school, describes in ASM's Meet the Microbiologist podcast how this loss of tolerance building in humans may contribute to increased inflammation detrimental to the host.
"If you knock out genes in animals that are involved in the innate immune pathway, you would have predicted that you'd get less inflammation, but what we actually see in many cases where you knock out components of the innate immune system is that you get increased inflammation," Hu explained.
Researchers have found that B. burgdorferi infected patients with single nucleotide polymorphisms (SNPs) in the pathogen recognition receptor Toll like receptor 1 have higher inflammatory responses and subsequent antibiotic-refractory Lyme arthritis .
Recently, Hu's group identified how a complex transcriptional network gets reprogrammed in macrophages during Borrelia infection to modulate long term inflammation in Lyme arthritis.
Excitingly, in 2023 the National Institute of Allergy and Infectious Diseases (NIAID) announced it awarded five project awards to fund PTLDS research citing the urgent need for more information as vector borne diseases are increasing in the U.S.
A COVID-19 infection tends to last about 4–14 days, while long COVID is defined as persistent symptoms lasting 4 weeks or longer following COVID-19 infection with no other infection diagnosis. Symptoms include fatigue, post-exertion malaise and dysautonomia.
Patients also may experience other debilitating symptoms, including dizziness, migraines and brain fog, and research indicates that COVID-19 can activate other post-acute infection syndromes, including postural orthostatic tachycardia syndrome (POTS).
POTS is a chronic disease that prohibits patients from standing for long periods of time and increases heart rate in sitting or standing positions due to autonomic nerve damage and subsequent functional dysregulation. Although these symptoms are present because of POTS, it is unknown whether permanent tissue damage itself further contributes to disease progression.
In some long COVID patients, extensive organ damage (lung, heart and cardiovascular tissue) and the inability to fully repair these tissues may contribute to continued chronic disability.
Interestingly, SARS-CoV-2 can also induce auto-antibodies against G-protein coupled receptors, (GPCRs) , which have been linked to different autoimmune disorders .
GPCRs play a role in modulating host immune responses in regulating effector cell trafficking and response to migration cues in the immunological environment. Therefore, GPCR auto-antibodies can cause microscopic damage to vascular tissue, which ultimately induces vascular remodeling and systemic autoimmunity by chronically targeting GPCRs and triggering a cascade of inflammatory cytokines.
These proposed mechanisms of post-acute infection syndromes are not necessarily exclusive and may activate each other in overlapping cycles. Questions about whether permanent tissue damage resulting from COVID-19 may be contributing to disease progression of long COVID remain unanswered.
What do these post-acute infection illnesses look like for patients?
The impact of post-acute infection syndromes on daily activities and overall quality of life can be devastating and, in some cases, lead to long-term disability. POTS and PASC were greatly highlighted as the COVID-19 pandemic progressed. Stories shared about the severity of PASC on relatively young and healthy individuals has helped promote advocacy for more research in this field.
Furthermore, these stories have helped break the stigma surrounding post-acute infection chronic disability health care. Multiple news and media outlets have have covered the toll long COVID has had on completely reordering patients' lives. These stories describe patients' loss of ability to work and perform basic cognitive abilities, such as remembering how to perform oral hygiene care.
How can we better detect and diagnose?
The onset of chronic disability associated with post-acute infection syndromes is not limited to the pathogens discussed in this article. Other pathogens, including Ebola virus , Coxiella burnetii , Chikungunya virus , West Nile virus and Camylobacter also have associations with long term chronic diseases. Yet their causes and pathology remain relatively unfamiliar to the medical community.
Since there is no standardization in diagnosis, post-acute infection syndromes are often dismissed as signs of other, more common, illnesses (chronic fatigue syndrome, fibromyalgia, depression, anxiety, etc.) or misinterpreted as cardiac or neurological abnormalities.
Furthermore, when these syndromes are recognized/diagnosed, patients often experience delays (or absence of) proper treatment, due to a lack of standardization in treatment plans for chronic illnesses. Patient health and well-being continues to diminish as a result.
Fortunately, in the aftermath of COVID-19, chronic illness has garnered more support, and biomedical research has received additional funding to further elucidate the causes of post-acute infection syndromes. Bettering our understanding of the mechanistic causes will allow us to create appropriate diagnosis standards and increase quality of life for those suffering with these chronic disabilities.
Explore further
Feedback to editors

Why nightmares and 'daymares' could be early warning signs of autoimmune disease
13 hours ago

Yoga and meditation-induced altered states of consciousness are common in the general population, study says
15 hours ago

Second Phase 3 clinical trial again shows dupilumab lessens disease in COPD patients with type 2 inflammation

Study sheds light on bacteria associated with pre-term birth

Researchers find intriguing connections between Alzheimer's disease and other common conditions
16 hours ago

Research shows linked biological pathways driving skin inflammation
17 hours ago

Bioluminescence and 3D-printed implants shed light on brain–spinal interactions

Researchers uncover biological trigger of early puberty

Social media-related nightmares: Study explores links between social media use, mental health and sleep quality

New device helps paraplegics regain partial use of hands
18 hours ago
Related Stories

Study finds potential new treatment path for lasting Lyme disease symptoms
Apr 18, 2024

Pre-clinical research indicates mRNA vaccine shows promise for reducing cases of Lyme disease
Sep 19, 2023

Long COVID-19 is linked to chronic pain conditions, says study
Feb 12, 2024

'Post-viral malaise': Why avoiding the term 'long-COVID' doesn't change anything for those affected
Mar 28, 2024

We can learn a lot about long COVID from years of diagnosing and treating chronic fatigue syndrome
Feb 23, 2023

Chronic fatigue syndrome may have a post-viral infection origin
Sep 6, 2023
Recommended for you

New AI algorithm may improve autoimmune disease prediction and therapies
19 hours ago

Research shows that ferroptosis, a form of cell death, occurs in severe COVID-19 patient lungs
22 hours ago

World-first trial shows benefits of finding and treating undiagnosed asthma and COPD
May 19, 2024
Let us know if there is a problem with our content
Use this form if you have come across a typo, inaccuracy or would like to send an edit request for the content on this page. For general inquiries, please use our contact form . For general feedback, use the public comments section below (please adhere to guidelines ).
Please select the most appropriate category to facilitate processing of your request
Thank you for taking time to provide your feedback to the editors.
Your feedback is important to us. However, we do not guarantee individual replies due to the high volume of messages.
E-mail the story
Your email address is used only to let the recipient know who sent the email. Neither your address nor the recipient's address will be used for any other purpose. The information you enter will appear in your e-mail message and is not retained by Medical Xpress in any form.
Newsletter sign up
Get weekly and/or daily updates delivered to your inbox. You can unsubscribe at any time and we'll never share your details to third parties.
More information Privacy policy
Donate and enjoy an ad-free experience
We keep our content available to everyone. Consider supporting Science X's mission by getting a premium account.
E-mail newsletter
Log in using your username and password
- Search More Search for this keyword Advanced search
- Latest content
- Supplements
- BMJ Journals More You are viewing from: Google Indexer
You are here
- Volume 5, Issue 6
- Hypothesis to explain the severe form of COVID-19 in Northern Italy
- Article Text
- Article info
- Citation Tools
- Rapid Responses
- Article metrics

- http://orcid.org/0000-0002-6285-7355 Luca Cegolon 1 , 2 ,
- Jennifer Pichierri 3 ,
- Giuseppe Mastrangelo 4 ,
- Sandro Cinquetti 1 ,
- Giovanni Sotgiu 5 ,
- Saverio Bellizzi 6 ,
- Giuseppe Pichierri 7
- 1 Public Health Department , Local Health Unit N. 2 "Marca Trevigiana" , Treviso , Veneto Region , Italy
- 2 IRCCS Materno Infantile Burlo Garofolo , Trieste , Friuli-Venezia Giulia Region , Italy
- 3 Children and Young People’s Diabetes , University College London Hospitals NHS Foundation Trust , London , UK
- 4 Cardiac, Thoracic and Vascular Sciences , Padua University Hospital , Padua , Veneto Region , Italy
- 5 Department of Medical, Surgical and Experimental Sciences , University of Sassari , Sassari , Sardinia Region , Italy
- 6 Partnership for Maternal, Newborn and Child Health , World Health Organization , Geneve , Switzerland
- 7 Microbiology Department , Kingston Hospital NHS Foundation Trust , Kingston upon Thames , London , UK
- Correspondence to Dr Luca Cegolon; l.cegolon{at}gmail.com
https://doi.org/10.1136/bmjgh-2020-002564
Statistics from Altmetric.com
Request permissions.
If you wish to reuse any or all of this article please use the link below which will take you to the Copyright Clearance Center’s RightsLink service. You will be able to get a quick price and instant permission to reuse the content in many different ways.
- public health
- prevention strategies
- epidemiology
Summary box
What is already known about this subject.
Human coronaviruses are known to cause respiratory re-infections, regardless of pre-existing humoural immunity.
There is evidence suggesting that severe acute respiratory syndrome coronavirus type 2 (SARS-CoV-2) had been circulating in Italy before the first COVID-19 case was detected in the country.
What are the new findings?
Prior infections with SARS-CoV-2 (or other viruses/coronaviruses) may arguably predispose to more severe forms of the disease following re-infection with SARS-CoV-2, with an immunological mechanism known as Antibody-Dependent-Enhancement, already observed with infections sustained by other coronaviruses (MERS-CoV and SARS-CoV) or other viruses such as the West Nile Virus and Dengue.
What are the recommendations for policy and practice?
If confirmed by in vivo studies, this hypothesis may have relevant implications for the treatment of severe forms of COVID-19, yet the possibility to produce an effective vaccine against SARS-CoV-2 might be hampered.
The ongoing COVID-19 pandemic, caused by the novel severe acute respiratory syndrome coronavirus type 2 (SARS-CoV-2), has affected 212 countries worldwide at various degrees as of 8 May 2020. 1
In this paper we discuss a hypothesis that prior viral infections—either by SARS-CoV-2 or different strains of coronaviruses, or potentially even other respiratory viruses—may predispose to more severe forms of COVID-19, following a secondary infection with SARS-CoV-2.
Most COVID-19 infections are asymptomatic or manifest with mild to moderate respiratory symptoms (fever, cough, sore throat, myalgia, fatigue and even non-severe pneumonia). Of patients with COVID-19, 14%–15% develop severe pneumonia and 5%–6% a critical condition requiring admission to intensive care unit (ICU). 2–4 Death may eventually occur after an average of 17.8 days since the onset of symptoms. 5
Among all countries, Italy (which was the first European COVID-19 cluster) presents a critical disease pattern as of 8 May 2020, having the third highest number of COVID-19 cases in the world after the USA and Spain, the fourth highest prevalence of the disease after Spain, Belgium and the USA, the third highest total number of deaths attributed to COVID-19 after the USA and the UK, and the third highest prevalence of COVID-19 mortality after Belgium and Spain, despite a current 1% rate of severe/critical disease among active cases, which has been progressively decreasing over time. 1
The cross-country discrepancies in the burden of COVID-19 observed so far across the globe cannot be explained only by differences in population age structures. 6–8 In fact, Japan has a population double that of Italy, with the proportion of subjects older than 65 being 28.8% in Japan vs 21.7% in Italy. 9 10 Nonetheless, as of 8 May 2020, the difference in COVID-19 prevalence between Japan (122 per million) and Italy (3570 per million) is massive. 1 Likewise, in Germany the percentage of individuals >65 is reportedly 22.1% (hence slightly higher than Italy), but the prevalence of COVID-19 is currently 2022 per million. 1 11 In Iran the proportion of people >65 is 5.5% (hence much younger than the Italian, German and Japanese populations), but the prevalence of COVID-19 is 1246 per million, as of 8 May 2020. 1 12
The mortality rate for COVID-19 is reportedly enhanced by 5.6%–10.5% in the presence of any comorbidities (hypertension, diabetes, cardiovascular diseases, cancer and/or chronic respiratory conditions) and becomes significantly and progressively higher after 50 years of age, 4 6 although the severe form of the disease increases linearly at any age stage. 5 Cold dry weather is a recognised risk factor for respiratory infections, rendering viruses as influenza more stable and individuals more susceptible. 13 14 This applies also to SARS-CoV-2, the viability and transmissibility of which reportedly reduce with hot and humid weather conditions. 14 Moreover, unfavourable disease progression and clinical outcomes of COVID-19 were found to be associated with cigarette smoking in a systematic review. 15
A number of factors may have contributed to enhancing the risk of infection with SARS-CoV-2 in Northern Italy. The age by which half of all young people leave parental home is higher in Italy than other European Union countries, 16 and such multigenerational cohabitation probably contributed to increase COVID-19 contagion among elderly individuals. The universal use of face masks was initially discouraged in Italy in order to preserve the limited supplies of personal protective equipment for professional use in healthcare settings; another argument initially was that face masks are ineffective in protecting against coronavirus infections. 17 Further major findings of the relevant literature have been summarised in figure 1 . An extraordinary elevated incidence of COVID-19 could have been the result of a perfect storm triggered by multiple interplaying factors. The affected areas in Northern Italy (regions of Lombardy, Emilia-Romagna, Piedmont and Veneto) are characterised by high population density 18 and recognised air pollution, 19 20 especially from fine particulate matter (PM2.5), which was found to increase the risk of death from COVID-19 in the USA. 21 Northern Italy includes several cities which, similarly to Philadelphia (USA) during the Spanish flu pandemic in 1918, 22 are historically important and densely populated, where social gatherings as well as business activities are certainly fundamental—the latter being vital to the economy of the entire country. These cultural and social dynamics might have influenced the initial resistance and reluctance of the general population to comply with the social restrictions progressively enforced by the Italian government (until full lockdown was established on 21 March). Moreover, the intense case finding in Italy was preceded by an initial overall underestimation of the COVID-19 threat by the Italian government and subsequently by the general population, who perceived the disease as just some sort of influenza, despite worrying news from the first affected country (China). 23 Thereafter SARS-CoV-2 was also going to spread to other European countries, which have also now been heavily affected by the disease. 1
- Download figure
- Open in new tab
- Download powerpoint
Conceptual framework explaining the relationships between various factors and incidence and severe/critical form of COVID-19. Established items: orange boxes; hypothetical items: blue boxes. ADE, antibody-dependent enhancement; ARDS, acute respiratory distress syndrome; COVID-19, coronavirus infectious disease 2019; IPC, infection prevention and control; SARS-CoV-2, severe acute respiratory syndrome coronavirus type.
The epidemiological investigations conducted by the Italian National Institute of Health ( Istituto Superiore di Sanità ) suggest that the vast majority of cases but the first three acquired the infection in Italy. It can therefore be reasonably argued that SARS-CoV-2 had been circulating in the country—especially in Lombardy, Emilia-Romagna, Piedmont and Veneto regions—for weeks before the first patient was found. 24 Human coronaviruses are known to cause respiratory reinfections regardless of pre-existing humoural immunity, both at the individual and community level. 25 At the same time, presumed hospital-associated transmission of SARS-CoV-2 has been reported since the initial stages of the COVID-19 outbreak in Wuhan (China) in 41% of the total number of patients, 70% of whom were healthcare staff. 26 This could have also occurred in Italy, where healthcare workers reportedly make up 9% out of all COVID-19 cases. 27 We therefore hypothesise (see blue boxes in figure 1 ) that repeated cycles of infection within a community (especially in older adults)—or even more worryingly in a healthcare setting—could have the potential to cause more severe forms of COVID-19, with acute respiratory distress syndrome (ARDS), the fundamental pathophysiology of severe viral pneumonia due to COVID-19, requiring admission to ICU. 28 ARDS associated with COVID-19 shares clinical features with the critical form of the 2003 severe acute respiratory syndrome coronavirus (SARS-CoV) epidemic, in particular lymphopaenia, massive infiltration of phagocytes and inflammation sustained by cytokines. 4 8 29
A plausible mechanistic hypothesis could be the antibody-dependent enhancement (ADE), sustained by prior exposure to SARS-CoV-2 or other viruses/coronaviruses. 8 Previous circulation and exposure to other coronaviruses similar to SARS-CoV-2 causing mild/asymptomatic cold-like symptoms shall in fact not be ruled out. 8 Binding and neutralising antibody response against other types of human coronaviruses was recently reported to increase with age in adult patients, 25 and this may explain the increased linear risk of severe COVID-19 with age, with death being significantly higher in patients older than 50. 5
Non-neutralising, subneutralising or even fully neutralising antibodies may play a key role in ADE. 30 Wan et al 30 have recently described a molecular mechanism for ADE involving the Middle East respiratory syndrome coronavirus (MERS-CoV), similar to what is already known for SARS-CoV and flaviviruses as Dengue and the West Nile virus. 31–35 While the entry of SARS-CoV into the phagocytes occurred principally through the human ACE2 receptor, the ADE mechanism was shown to be enhanced by antibodies specific for the spike (S) envelope glycoprotein binding with the macrophage receptor and subsequent enhancement of target cell infections. 33–35 Likewise, the antibody/opsonised SARS-CoV-2 particles may bind avidly with the IgFc receptors of target cells, increasing the virus yield as well as the production of cytokines. This may also explain the higher risk of thromboembolism reportedly associated with severe/critical COVID-19. 4 36 37
Anecdotal clinical reports of ‘biphasic infection’ and ‘cytokine storm’ seem to possibly point towards this direction, biphasic infection simply being the immunological result of a secondary infection by other coronaviruses or a reinfection due to SARS-CoV-2. 38–41 An early elevation of serum proinflammatory cytokines, suggesting a pathological mechanism mediated by cytokine storm, has been observed with both severe forms of SARS-CoV and MERS-CoV infections. The latter two viruses share a genomic similarity of about 79% and 50% with SARS-CoV-2, respectively. 41 42 Several Rhinolophus bats-related coronavirus strains have been found to share even higher sequence homology with SARS-CoV-2. 33 An abnormal humoural response due to ADE, in the early stages of a secondary infection by SARS-CoV-2, may delay the innate antiviral immune response relying on the production of type 1 interferon (IFN-1). This would compromise the initial antiviral response of the host, with subsequent elevated influx of proinflammatory cytokines, hyperinflammatory neutrophils and monocytes-macrophages and hypercoagulable state accountable for ARDS and typical pneumonia observed in patients affected by severe/critical COVID-19 ( figure 1 ). 4 41 43 44
If confirmed, this hypothesis would have relevant implications for the treatment of COVID-19 and the development of an effective vaccine. The licensing of a vaccine against human coronaviruses has failed thus far, partly because immunised individuals could potentially be at higher risk of ADE sustained by facilitated uptake of viral antigen-antibody complexes by target cells. 4 31 33 44 The approval of a vaccine against SARS-CoV-2 may encounter similar obstacles. Likewise, herd immunity would not be a possibility with COVID-19. WHO recommends passive immunotherapy when vaccine and antivirals are not available for emerging infections. 45 In a preliminary uncontrolled case study on five critically ill patients with COVID-19 who developed ARDS, the administration of convalescent plasma—drawn from five patients who recovered from COVID-19 and containing SARS-CoV-2-specific neutralising antibodies (IgG)—between 10 and 22 days since admission significantly improved the clinical status of all, resolving ARDS in four of them within 12 days since transfusion. 46 On the other hand, the treatment of severe COVID-19 may also benefit from monoclonal antibodies targeting proinflammatory cytokines 4 as well as supplements of IFN-1 in combination with other antiviral drugs. 47–50
Whether secondary infections from other coronaviruses or repeated community reinfections of SARS-CoV-2 may account for more severe presentations of COVID-19 observed in some countries compared with others, 8 and whether it is only a matter of time for the virus to circulate and infect a significant proportion of the population before causing reinfections and therefore more severe clinical features, are something which will require more indepth epidemiological and immunological/serological investigations. A better understanding of any underlying immunological mechanism or any additional risk factor which could explain cross-country differences in the rates of severe disease and mortality attributable to COVID-19 will help to guide international public health responses during this ongoing pandemic. It will be important to clarify if the ARDS mechanism responsible for the severe respiratory infection could potentially be attributable to ADE.
Two different in vivo strategies could be employed to endorse this hypothesis.
First (observational design), all healthcare workers and blood donors should undergo serum test for COVID-19. Individuals presenting SARS-CoV-2 IgG antibodies should be included in a local/regional/national ad-hoc register and monitored over time for the possible development of severe disease sustained by ADE, which would need to be confirmed by blood count, dosage of IFN and proinflammatory cytokines, in addition to chest CT scan. The risk of developing severe COVID-19 should be estimated and stratified by relevant risk factors, including baseline serum level of SARS-CoV-2-specific IgG antibodies, age, sex, potential occupational exposure, medical history (particularly previous infections and vaccination status), any comorbidities, area of residence and health status of household members, among others.
Second (experimental laboratory design), animal models (hamsters, rodents, palm civets, monkeys, ferrets) 33 51 52 could be infected by SARS-CoV-2 (or other viruses/coronaviruses) and subsequently re-exposed to SARS-CoV-2 to verify the possibility of onset of ARDS sustained by ADE.
- Worldometers
- World Health Organization
- Chan JF-W ,
- Kok K-H , et al
- Dorigatti I , et al
- Index Mundi
- Feng K , et al
- Vardavas CI ,
- Iacovou M ,
- Xia N , et al
- Office for National Statistics (ISTAT)
- Ghermandi G ,
- Harrison RM
- Liang S , et al
- Nethery RC ,
- Sabath BM , et al
- Hannigan T ,
- Steele C , et al
- Start Magazine
- National Institute of Health (ISS)
- Donovan MM ,
- Hu C , et al
- International Council of Nurses
- Xu J , et al
- Cheung CY ,
- Ng IHY , et al
- Sun S , et al
- Gollins SW ,
- Porterfield JS
- Urbina AN ,
- Chang MR , et al
- Yang Z-yong ,
- Werner HC ,
- Kong W-pui , et al
- Tseng S-P ,
- Yen C-H , et al
- Tirado SMC ,
- Zhao L , et al
- Thachil J ,
- Iba T , et al
- McAuley DF ,
- Brown M , et al
- Mahallawi WH ,
- Khabour OF ,
- Zhang Q , et al
- Wu AKL , et al
- Prompetchara E ,
- Li J , et al
- Pedersen SF ,
- Corapi WV ,
- Ngichabe CK , et al
- Zhao F , et al
- Sallard E ,
- Lescure F-X ,
- Yazdanpanah Y , et al
- Chen Q , et al
- Loutfy MR ,
- Siminovitch KA , et al
- Omrani AS ,
- Baig K , et al
- Cheung CY , et al
- Roberts A , et al
Handling editor Seye Abimbola
Contributors LC, JP, SB, GP and GM equally contributed to conceiving the idea and drafting the manuscript. SC and GS contributed to drafting the manuscript.
Funding The authors have not declared a specific grant for this research from any funding agency in the public, commercial or not-for-profit sectors.
Competing interests None declared.
Patient consent for publication Not required.
Provenance and peer review Not commissioned; externally peer reviewed.
Data availability statement There are no data in this work.
Read the full text or download the PDF:
- Ministry of Health
News Highlights

18th May 2024
Category: Highlights Press Releases
Thank you for visiting nature.com. You are using a browser version with limited support for CSS. To obtain the best experience, we recommend you use a more up to date browser (or turn off compatibility mode in Internet Explorer). In the meantime, to ensure continued support, we are displaying the site without styles and JavaScript.
- View all journals
- Explore content
- About the journal
- Publish with us
- Sign up for alerts
- Review Article
- Published: 13 January 2023
Long COVID: major findings, mechanisms and recommendations
- Hannah E. Davis ORCID: orcid.org/0000-0002-1245-2034 1 ,
- Lisa McCorkell ORCID: orcid.org/0000-0002-3261-6737 2 ,
- Julia Moore Vogel ORCID: orcid.org/0000-0002-4902-3540 3 &
- Eric J. Topol ORCID: orcid.org/0000-0002-1478-4729 3
Nature Reviews Microbiology volume 21 , pages 133–146 ( 2023 ) Cite this article
1.38m Accesses
1045 Citations
16683 Altmetric
Metrics details
- Research data
- Viral infection
An Author Correction to this article was published on 17 April 2023
This article has been updated
Long COVID is an often debilitating illness that occurs in at least 10% of severe acute respiratory syndrome coronavirus 2 (SARS-CoV-2) infections. More than 200 symptoms have been identified with impacts on multiple organ systems. At least 65 million individuals worldwide are estimated to have long COVID, with cases increasing daily. Biomedical research has made substantial progress in identifying various pathophysiological changes and risk factors and in characterizing the illness; further, similarities with other viral-onset illnesses such as myalgic encephalomyelitis/chronic fatigue syndrome and postural orthostatic tachycardia syndrome have laid the groundwork for research in the field. In this Review, we explore the current literature and highlight key findings, the overlap with other conditions, the variable onset of symptoms, long COVID in children and the impact of vaccinations. Although these key findings are critical to understanding long COVID, current diagnostic and treatment options are insufficient, and clinical trials must be prioritized that address leading hypotheses. Additionally, to strengthen long COVID research, future studies must account for biases and SARS-CoV-2 testing issues, build on viral-onset research, be inclusive of marginalized populations and meaningfully engage patients throughout the research process.
Similar content being viewed by others
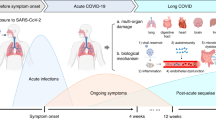
The long-term health outcomes, pathophysiological mechanisms and multidisciplinary management of long COVID
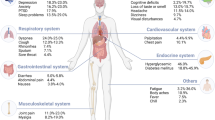
Epidemiology, clinical presentation, pathophysiology, and management of long COVID: an update
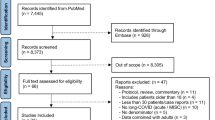
Long-COVID in children and adolescents: a systematic review and meta-analyses
Introduction.
Long COVID (sometimes referred to as ‘post-acute sequelae of COVID-19’) is a multisystemic condition comprising often severe symptoms that follow a severe acute respiratory syndrome coronavirus 2 (SARS-CoV-2) infection. At least 65 million individuals around the world have long COVID, based on a conservative estimated incidence of 10% of infected people and more than 651 million documented COVID-19 cases worldwide 1 ; the number is likely much higher due to many undocumented cases. The incidence is estimated at 10–30% of non-hospitalized cases, 50–70% of hospitalized cases 2 , 3 and 10–12% of vaccinated cases 4 , 5 . Long COVID is associated with all ages and acute phase disease severities, with the highest percentage of diagnoses between the ages of 36 and 50 years, and most long COVID cases are in non-hospitalized patients with a mild acute illness 6 , as this population represents the majority of overall COVID-19 cases. There are many research challenges, as outlined in this Review, and many open questions, particularly relating to pathophysiology, effective treatments and risk factors.
Hundreds of biomedical findings have been documented, with many patients experiencing dozens of symptoms across multiple organ systems 7 (Fig. 1 ). Long COVID encompasses multiple adverse outcomes, with common new-onset conditions including cardiovascular, thrombotic and cerebrovascular disease 8 , type 2 diabetes 9 , myalgic encephalomyelitis/chronic fatigue syndrome (ME/CFS) 10 , 11 and dysautonomia, especially postural orthostatic tachycardia syndrome (POTS) 12 (Fig. 2 ). Symptoms can last for years 13 , and particularly in cases of new-onset ME/CFS and dysautonomia are expected to be lifelong 14 . With significant proportions of individuals with long COVID unable to return to work 7 , the scale of newly disabled individuals is contributing to labour shortages 15 . There are currently no validated effective treatments.
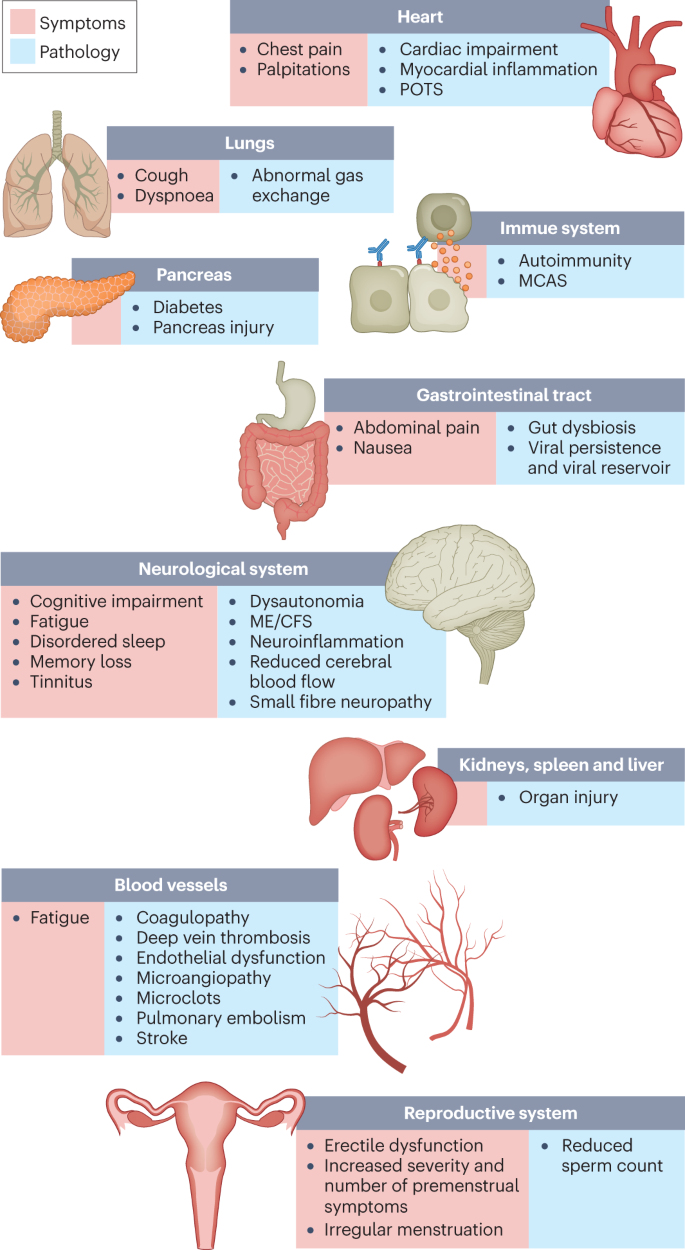
The impacts of long COVID on numerous organs with a wide variety of pathology are shown. The presentation of pathologies is often overlapping, which can exacerbate management challenges. MCAS, mast cell activation syndrome; ME/CFS, myalgic encephalomyelitis/chronic fatigue syndrome; POTS, postural orthostatic tachycardia syndrome.

Because diagnosis-specific data on large populations with long COVID are sparse, outcomes from general infections are included and a large proportion of medical conditions are expected to result from long COVID, although the precise proportion cannot be determined. One year after the initial infection, severe acute respiratory syndrome coronavirus 2 (SARS-CoV-2) infections increased the risk of cardiac arrest, death, diabetes, heart failure, pulmonary embolism and stroke, as studied with use of US Department of Veterans Affairs databases. Additionally, there is clear increased risk of developing myalgic encephalomyelitis/chronic fatigue syndrome (ME/CFS) and dysautonomia. Six months after breakthrough infection, increased risks were observed for cardiovascular conditions, coagulation and haematological conditions, death, fatigue, neurological conditions and pulmonary conditions in the same cohort. The hazard ratio is the ratio of how often an event occurs in one group relative to another; in this case people who have had COVID-19 compared with those who have not. Data sources are as follows: diabetes 9 , cardiovascular outcomes 8 , dysautonomia 12 , 201 , ME/CFS 10 , 202 and breakthrough infections 4 .
There are likely multiple, potentially overlapping, causes of long COVID. Several hypotheses for its pathogenesis have been suggested, including persisting reservoirs of SARS-CoV-2 in tissues 16 , 17 ; immune dysregulation 17 , 18 , 19 , 20 with or without reactivation of underlying pathogens, including herpesviruses such as Epstein–Barr virus (EBV) and human herpesvirus 6 (HHV-6) among others 17 , 18 , 21 , 22 ; impacts of SARS-CoV-2 on the microbiota, including the virome 17 , 23 , 24 , 25 ; autoimmunity 17 , 26 , 27 , 28 and priming of the immune system from molecular mimicry 17 ; microvascular blood clotting with endothelial dysfunction 17 , 29 , 30 , 31 ; and dysfunctional signalling in the brainstem and/or vagus nerve 17 , 32 (Fig. 3 ). Mechanistic studies are generally at an early stage, and although work that builds on existing research from postviral illnesses such as ME/CFS has advanced some theories, many questions remain and are a priority to address. Risk factors potentially include female sex, type 2 diabetes, EBV reactivation, the presence of specific autoantibodies 27 , connective tissue disorders 33 , attention deficit hyperactivity disorder, chronic urticaria and allergic rhinitis 34 , although a third of people with long COVID have no identified pre-existing conditions 6 . A higher prevalence of long Covid has been reported in certain ethnicities, including people with Hispanic or Latino heritage 35 . Socio-economic risk factors include lower income and an inability to adequately rest in the early weeks after developing COVID-19 (refs. 36 , 37 ). Before the emergence of SARS-CoV-2, multiple viral and bacterial infections were known to cause postinfectious illnesses such as ME/CFS 17 , 38 , and there are indications that long COVID shares their mechanistic and phenotypic characteristics 17 , 39 . Further, dysautonomia has been observed in other postviral illnesses and is frequently observed in long COVID 7 .
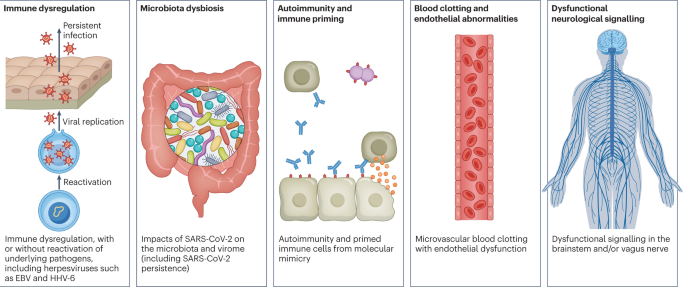
There are several hypothesized mechanisms for long COVID pathogenesis, including immune dysregulation, microbiota disruption, autoimmunity, clotting and endothelial abnormality, and dysfunctional neurological signalling. EBV, Epstein–Barr virus; HHV-6, human herpesvirus 6; SARS-CoV-2, severe acute respiratory syndrome coronavirus 2.
In this Review, we explore the current knowledge base of long COVID as well as misconceptions surrounding long COVID and areas where additional research is needed. Because most patients with long COVID were not hospitalized for their initial SARS-CoV-2 infection 6 , we focus on research that includes patients with mild acute COVID-19 (meaning not hospitalized and without evidence of respiratory disease). Most of the studies we discuss refer to adults, except for those in Box 1 .
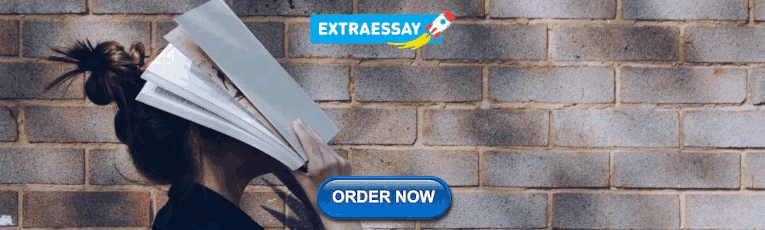
Box 1 Long COVID in children
Long COVID impacts children of all ages. One study found that fatigue, headache, dizziness, dyspnoea, chest pain, dysosmia, dysgeusia, reduced appetite, concentration difficulties, memory issues, mental exhaustion, physical exhaustion and sleep issues were more common in individuals with long COVID aged 15–19 years compared with controls of the same age 203 . A nationwide study in Denmark comparing children with a positive PCR test result with control individuals found that the former had a higher chance of reporting at least one symptom lasting more than 2 months 204 . Similarly to adults with long COVID, children with long COVID experience fatigue, postexertional malaise, cognitive dysfunction, memory loss, headaches, orthostatic intolerance, sleep difficulty and shortness of breath 204 , 205 . Liver injury has been recorded in children who were not hospitalized during acute severe acute respiratory syndrome coronavirus 2 (SARS-CoV-2) infections 206 , and although rare, children who had COVID-19 have increased risks of acute pulmonary embolism, myocarditis and cardiomyopathy, venous thromboembolic events, acute and unspecified renal failure, and type 1 diabetes 207 . Infants born to women who had COVID-19 during pregnancy were more likely to receive a neurodevelopmental diagnosis in the first year after delivery 208 . A paediatric long COVID centre’s experience treating patients suggests that adolescents with a moderate to severe form of long COVID have features consistent with myalgic encephalomyelitis/chronic fatigue syndrome 205 . Children experiencing long COVID have hypometabolism in the brain similar to the patterns found in adults with long COVID 209 . Long-term pulmonary dysfunction is found in children with long COVID and those who have recovered from COVID-19 (ref. 210 ). Children with long COVID were more likely to have had attention deficit hyperactivity disorder, chronic urticaria and allergic rhinitis before being infected 34 .
More research on long COVID in children is needed, although there are difficulties in ensuring a proper control group due to testing issues. Several studies have found that children infected with SARS-CoV-2 are considerably less likely to have a positive PCR test result than adults despite seroconverting weeks later, with up to 90% of cases being missed 189 , 190 . Additionally, children are much less likely to seroconvert and, if they develop antibodies, are more likely to have a waning response months after infection compared with adults 193 .
Major findings
Immunology and virology.
Studies looking at immune dysregulation in individuals with long COVID who had mild acute COVID-19 have found T cell alterations, including exhausted T cells 18 , reduced CD4 + and CD8 + effector memory cell numbers 18 , 19 and elevated PD1 expression on central memory cells, persisting for at least 13 months 19 . Studies have also reported highly activated innate immune cells, a lack of naive T and B cells and elevated expression of type I and type III interferons (interferon-β (IFNβ) and IFNλ1), persisting for at least 8 months 20 . A comprehensive study comparing patients with long COVID with uninfected individuals and infected individuals without long COVID found increases in the numbers of non-classical monocytes, activated B cells, double-negative B cells, and IL-4- and IL-6-secreting CD4 + T cells and decreases in the numbers of conventional dendritic cells and exhausted T cells and low cortisol levels in individuals with long COVID at a median of 14 months after infection 18 . The expansion of cytotoxic T cells has been found to be associated with the gastrointestinal presentation of long COVID 27 . Additional studies have found elevated levels of cytokines, particularly IL-1β, IL-6, TNF and IP10 (refs. 40 , 41 ), and a recent preprint has reported persistent elevation of the level of CCL11, which is associated with cognitive dysfunction 42 . It remains to be seen whether the pattern of cytokines in ME/CFS, where the levels of certain cytokines are elevated in the first 2–3 years of illness but decrease over time without a corresponding decrease in symptoms 43 , is similar in long COVID.
Multiple studies have found elevated levels of autoantibodies in long COVID 27 , including autoantibodies to ACE2 (ref. 28 ) (the receptor for SARS-CoV-2 entry), β 2 -adrenoceptor, muscarinic M2 receptor, angiotensin II AT 1 receptor and the angiotensin 1–7 MAS receptor 26 . High levels of other autoantibodies have been found in some patients with COVID-19 more generally, including autoantibodies that target the tissue (such as connective tissue, extracellular matrix components, vascular endothelium, coagulation factors and platelets), organ systems (including the lung, central nervous system, skin and gastrointestinal tract), immunomodulatory proteins (cytokines, chemokines, complement components and cell-surface proteins) 44 . A major comprehensive study, however, did not find autoantibodies to be a major component of long COVID 18 .
Reactivated viruses, including EBV and HHV-6, have been found in patients with long COVID 18 , 21 , 22 , 27 (and have been identified in ME/CFS 45 ), and lead to mitochondrial fragmentation and severely affect energy metabolism 46 . A recent preprint has reported that EBV reactivation is associated with fatigue and neurocognitive dysfunction in patients with long COVID 22 .
Several studies have shown low or no SARS-CoV-2 antibody production and other insufficient immune responses in the acute stage of COVID-19 to be predictive of long COVID at 6–7 months, in both hospitalized patients and non-hospitalized patients 47 , 48 . These insufficient immune responses include a low baseline level of IgG 48 , low levels of receptor-binding domain and spike-specific memory B cells, low levels of nucleocapsid IgG 49 and low peaks of spike-specific IgG 47 . In a recent preprint, low or absent CD4 + T cell and CD8 + T cell responses were noted in patients with severe long COVID 49 , and a separate study found lower levels of CD8 + T cells expressing CD107a and a decline in nucleocapsid-specific interferon-γ-producing CD8 + T cells in patients with long COVID compared with infected controls without long COVID 50 . High levels of autoantibodies in long COVID have been found to be inversely correlated with protective COVID-19 antibodies, suggesting that patients with high autoantibody levels may be more likely to have breakthrough infections 27 . SARS-CoV-2 viral rebound in the gut, possibly resulting from viral persistence, has also been associated with lower levels and slower production of receptor-binding domain IgA and IgG antibodies 51 . There are major differences in antibody creation, seroreversion and antibody titre levels across the sexes, with women being less likely to seroconvert, being more likely to serorevert and having lower antibody levels overall 52 , 53 , even affecting antibody waning after vaccination 54 .
Several reports have pointed towards possible viral persistence as a driver of long COVID symptoms; viral proteins and/or RNA has been found in the reproductive system, cardiovascular system, brain, muscles, eyes, lymph nodes, appendix, breast tissue, hepatic tissue, lung tissue, plasma, stool and urine 55 , 56 , 57 , 58 , 59 , 60 . In one study, circulating SARS-CoV-2 spike antigen was found in 60% of a cohort of 37 patients with long COVID up to 12 months after diagnosis compared with 0% of 26 SARS-CoV-2-infected individuals, likely implying a reservoir of active virus or components of the virus 16 . Indeed, multiple reports following gastrointestinal biopsies have indicated the presence of virus, suggestive of a persistent reservoir in some patients 58 , 61 .
Vascular issues and organ damage
Although COVID-19 was initially recognized as a respiratory illness, SARS-CoV-2 has capability to damage many organ systems. The damage that has been demonstrated across diverse tissues has predominantly been attributed to immune-mediated response and inflammation, rather than direct infection of cells by the virus. Circulatory system disruption includes endothelial dysfunction and subsequent downstream effects, and increased risks of deep vein thrombosis, pulmonary embolism and bleeding events 29 , 30 , 62 . Microclots detected in both acute COVID-19 and long COVID contribute to thrombosis 63 and are an attractive diagnostic and therapeutic target. Long-term changes to the size and stiffness of blood cells have also been found in long COVID, with the potential to affect oxygen delivery 64 . A long-lasting reduction in vascular density, specifically affecting small capillaries, was found in patients with long COVID compared with controls, 18 months after infection 65 . A study finding elevated levels of vascular transformation blood biomarkers in long COVID also found that the angiogenesis markers ANG1 and P-selectin both had high sensitivity and specificity for predicting long COVID status 66 .
An analysis of the US Department of Veterans Affairs databases (VA data) including more than 150,000 individuals 1 year after SARS-CoV-2 infection indicated a significantly increased risk of a variety of cardiovascular diseases, including heart failure, dysrhythmias and stroke, independent of the severity of initial COVID-19 presentation 8 (Fig. 2 ). Cardiac MRI studies revealed cardiac impairment in 78% of 100 individuals who had a prior COVID-19 episode (investigated an average of 71 days after infection 67 ) and in 58% of participants with long COVID (studied 12 months after infection 68 ), reinforcing the durability of cardiac abnormalities.
Multiple studies have revealed multi-organ damage associated with COVID-19. One prospective study of low-risk individuals, looking at the heart, lungs, liver, kidneys, pancreas and spleen, noted that 70% of 201 patients had damage to at least one organ and 29% had multi-organ damage 69 . In a 1-year follow-up study, conducted by the same research group with 536 participants, the study authors found that 59% had single-organ damage and 27% multi-organ damage 70 . A dedicated kidney study of VA data including more than 89,000 individuals who had COVID-19 noted an increased risk of numerous adverse kidney outcomes 71 . Another VA data analysis, including more than 181,000 individuals who had COVID-19, found that infection also increases the risk of type 2 diabetes 9 (Fig. 2 ). The organ damage experienced by patients with long COVID appears durable, and long-term effects remain unknown.
Neurological and cognitive systems
Neurological and cognitive symptoms are a major feature of long COVID, including sensorimotor symptoms, memory loss, cognitive impairment, paresthesia, dizziness and balance issues, sensitivity to light and noise, loss of (or phantom) smell or taste, and autonomic dysfunction, often impacting activities of daily living 7 , 32 . Audiovestibular manifestations of long COVID include tinnitus, hearing loss and vertigo 7 , 72 .
In a meta-analysis, fatigue was found in 32% and cognitive impairment was found in 22% of patients with COVID-19 at 12 weeks after infection 3 . Cognitive impairments in long COVID are debilitating, at the same magnitude as intoxication at the UK drink driving limit or 10 years of cognitive ageing 73 , and may increase over time, with one study finding occurrence in 16% of patients at 2 months after infection and 26% of patients at 12 months after infection 74 . Activation of the kynurenine pathway, particularly the presence of the metabolites quinolinic acid, 3-hydroxyanthranilic acid and kynurenine, has been identified in long COVID, and is associated with cognitive impairment 74 . Cognitive impairment has also been found in individuals who recovered from COVID-19 (ref. 75 ), and at higher rates when objective versus subjective measures were used 3 , suggesting that a subset of those with cognitive impairment may not recognize and/or report their impairment. Cognitive impairment is a feature that manifests itself independently of mental health conditions such as anxiety and depression 74 , 76 , and occurs at similar rates in hospitalized and non-hospitalized patients 74 , 76 . A report of more than 1.3 million people who had COVID-19 showed mental health conditions such as anxiety and depression returned to normal over time, but increased risks of cognitive impairment (brain fog), seizures, dementia, psychosis and other neurocognitive conditions persisted for at least 2 years 77 .
Possible mechanisms for these neuropathologies include neuroinflammation, damage to blood vessels by coagulopathy and endothelial dysfunction, and injury to neurons 32 . Studies have found Alzheimer disease-like signalling in patients with long COVID 78 , peptides that self-assemble into amyloid clumps which are toxic to neurons 79 , widespread neuroinflammation 80 , brain and brainstem hypometabolism correlated with specific symptoms 81 , 82 and abnormal cerebrospinal fluid findings in non-hospitalized individuals with long COVID along with an association between younger age and a delayed onset of neurological symptoms 83 . Multilineage cellular dysregulation and myelin loss were reported in a recent preprint in patients with long COVID who had mild infections, with microglial reactivity similar to that seen in chemotherapy, known as ‘chemo-brain’ 42 . A study from the UK Biobank, including brain imaging in the same patients before and after COVID-19 as well as control individuals, showed a reduction in grey matter thickness in the orbitofrontal cortex and parahippocampal gyrus (markers of tissue damage in areas connected to the primary olfactory cortex), an overall reduction in brain size and greater cognitive decline in patients after COVID-19 compared with controls, even in non-hospitalized patients. Although that study looked at individuals with COVID-19 compared with controls, not specifically long COVID, it may have an implication for the cognitive component of long COVID 84 . Abnormal levels of mitochondrial proteins as well as SARS-CoV-2 spike and nucleocapsid proteins have been found in the central nervous system 85 . Tetrahydrobiopterin deficiencies and oxidative stress are found in long COVID as well 86 .
In the eyes, corneal small nerve fibre loss and increased dendritic cell density have been found in long COVID 87 , 88 , as well as significantly altered pupillary light responses 89 and impaired retinal microcirculation 90 . SARS-CoV-2 can infect and replicate in retinal 59 and brain 91 organoids. Other manifestations of long COVID include retinal haemorrhages, cotton wool spots and retinal vein occlusion 92 .
Mouse models of mild SARS-CoV-2 infection demonstrated microglial reactivity and elevated levels of CCL11, which is associated with cognitive dysfunction and impaired neurogenesis 42 . Hamster models exhibited an ongoing inflammatory state, involving T cell and myeloid activation, production of pro-inflammatory cytokines and an interferon response that was correlated with anxiety and depression-like behaviours in the hamsters, with similar transcriptional signatures found in the tissue of humans who had recovered from COVID-19 (ref. 93 ). Infected non-human primates with mild illness showed neuroinflammation, neuronal injury and apoptosis, brain microhaemorrhages, and chronic hypoxaemia and brain hypoxia 94 .
Recent reports indicate low blood cortisol levels in patients with long COVID as compared with control individuals, more than 1 year into symptom duration 18 , 27 . Low cortisol production by the adrenal gland should be compensated by an increase in adrenocorticotropic hormone (ACTH) production by the pituitary gland, but this was not the case, supporting hypothalamus–pituitary–adrenal axis dysfunction 18 . This may also reflect an underlying neuroinflammatory process. Low cortisol levels have previously been documented in individuals with ME/CFS.
ME/CFS, dysautonomia and related conditions
ME/CFS is a multisystem neuroimmune illness with onset often following a viral or bacterial infection. Criteria include a “substantial reduction or impairment in the ability to engage in pre-illness levels of occupational, educational, social, or personal activities” for at least 6 months, accompanied by a profound fatigue that is not alleviated by rest, along with postexertional malaise, unrefreshing sleep and cognitive impairment or orthostatic intolerance (or both) 95 . Up to 75% of people with ME/CFS cannot work full-time and 25% have severe ME/CFS, which often means they are bed-bound, have extreme sensitivity to sensory input and are dependent on others for care 96 . There is a vast collection of biomedical findings in ME/CFS 97 , 98 , although these are not well known to researchers and clinicians in other fields.
Many researchers have commented on the similarity between ME/CFS and long COVID 99 ; around half of individuals with long COVID are estimated to meet the criteria for ME/CFS 10 , 11 , 29 , 100 , and in studies where the cardinal ME/CFS symptom of postexertional malaise is measured, a majority of individuals with long COVID report experiencing postexertional malaise 7 , 100 . A study of orthostatic stress in individuals with long COVID and individuals with ME/CFS found similar haemodynamic, symptomatic and cognitive abnormalities in both groups compared with healthy individuals 101 . Importantly, it is not surprising that ME/CFS should stem from SARS-CoV-2 infection as 27.1% of SARS-CoV infection survivors in one study met the criteria for ME/CFS diagnosis 4 years after onset 102 . A wide range of pathogens cause ME/CFS onset, including EBV, Coxiella burnetii (which causes Q fever), Ross River virus and West Nile virus 38 .
Consistent abnormal findings in ME/CFS include diminished natural killer cell function, T cell exhaustion and other T cell abnormalities, mitochondrial dysfunction, and vascular and endothelial abnormalities, including deformed red blood cells and reduced blood volume. Other abnormalities include exercise intolerance, impaired oxygen consumption and a reduced anaerobic threshold, and abnormal metabolic profiles, including altered usage of fatty acids and amino acids. Altered neurological functions have also been observed, including neuroinflammation, reduced cerebral blood flow, brainstem abnormalities and elevated ventricular lactate level, as well as abnormal eye and vision findings. Reactivated herpesviruses (including EBV, HHV-6, HHV-7 and human cytomegalovirus) are also associated with ME/CFS 97 , 98 , 103 , 104 .
Many of these findings have been observed in long COVID studies in both adults and children (Box 1 ). Long COVID research has found mitochondrial dysfunction including loss of mitochondrial membrane potential 105 and possible dysfunctional mitochondrial metabolism 106 , altered fatty acid metabolism and dysfunctional mitochondrion-dependent lipid catabolism consistent with mitochondrial dysfunction in exercise intolerance 107 , redox imbalance 108 , and exercise intolerance and impaired oxygen extraction 100 , 109 , 110 . Studies have also found endothelial dysfunction 29 , cerebral blood flow abnormalities and metabolic changes 81 , 111 , 112 , 113 (even in individuals with long COVID whose POTS symptoms abate 114 ), extensive neuroinflammation 42 , 80 , reactivated herpesviruses 18 , 21 , 27 , deformed red blood cells 64 and many findings discussed elsewhere. Microclots and hyperactivated platelets are found not only in individuals with long COVID but also in individuals with ME/CFS 115 .
Dysautonomia, particularly POTS, is commonly comorbid with ME/CFS 116 and also often has a viral onset 117 . POTS is associated with G protein-coupled adrenergic receptor and muscarinic acetylcholine receptor autoantibodies, platelet storage pool deficiency, small fibre neuropathy and other neuropathologies 118 . Both POTS and small fibre neuropathy are commonly found in long COVID 111 , 119 , with one study finding POTS in 67% of a cohort with long COVID 120 .
Mast cell activation syndrome is also commonly comorbid with ME/CFS. The number and severity of mast cell activation syndrome symptoms substantially increased in patients with long COVID compared with pre-COVID and control individuals 121 , with histamine receptor antagonists resulting in improvements in the majority of patients 19 .
Other conditions that are commonly comorbid with ME/CFS include connective tissue disorders including Ehlers–Danlos syndrome and hypermobility, neuro-orthopaedic spinal and skull conditions, and endometriosis 33 , 122 , 123 . Evidence is indicating these conditions may be comorbid with long COVID as well. The overlap of postviral conditions with these conditions should be explored further.
Reproductive system
Impacts on the reproductive system are often reported in long COVID, although little research has been done to document the extent of the impact and sex-specific pathophysiology. Menstrual alterations are more likely to occur in women and people who menstruate with long COVID than in women and people who menstruate with no history of COVID and those who had COVID-19 but not long COVID 124 . Menstruation and the week before menstruation have been identified by patients as triggers for relapses of long COVID symptoms 7 . Declined ovarian reserve and reproductive endocrine disorder have been observed in people with COVID-19 (ref. 125 ), and initial theories suggest that SARS-CoV-2 infection affects ovary hormone production and/or the endometrial response due to the abundance of ACE2 receptors on ovarian and endometrial tissue 126 . Individuals with both COVID-19 and menstrual changes were more likely to experience fatigue, headache, body ache and pain, and shortness of breath than those who did not have menstrual changes, and the most common menstrual changes were irregular menstruation, increased premenstrual symptoms and infrequent menstruation 127 .
Research on ME/CFS shows associations between ME/CFS and premenstrual dysphoric disorder, polycystic ovarian syndrome, menstrual cycle abnormalities, ovarian cysts, early menopause and endometriosis 128 , 129 , 130 . Pregnancy, postpartum changes, perimenopause and menstrual cycle fluctuations affect ME/CFS and influence metabolic and immune system changes 129 . Long COVID research should focus on these relationships to better understand the pathophysiology.
Viral persistence in the penile tissue has been documented, as has an increased risk of erectile dysfunction, likely resulting from endothelial dysfunction 131 . In one study, impairments to sperm count, semen volume, motility, sperm morphology and sperm concentration were reported in individuals with long COVID compared with control individuals, and were correlated with elevated levels of cytokines and the presence of caspase 8, caspase 9 and caspase 3 in seminal fluid 132 .
Respiratory system
Respiratory conditions are a common phenotype in long COVID, and in one study occurred twice as often in COVID-19 survivors as in the general population 2 . Shortness of breath and cough are the most common respiratory symptoms, and persisted for at least 7 months in 40% and 20% of patients with long COVID, respectively 7 . Several imaging studies that included non-hospitalized individuals with long COVID demonstrated pulmonary abnormalities including in air trapping and lung perfusion 133 , 134 . An immunological and proteomic study of patients 3–6 months after infection indicated apoptosis and epithelial damage in the airway but not in blood samples 135 . Further immunological characterization comparing individuals with long COVID with individuals who had recovered from COVID-19 noted a correlation between decreased lung function, systemic inflammation and SARS-CoV-2-specific T cells 136 .
Gastrointestinal system
Long COVID gastrointestinal symptoms include nausea, abdominal pain, loss of appetite, heartburn and constipation 137 . The gut microbiota composition is significantly altered in patients with COVID-19 (ref. 23 ), and gut microbiota dysbiosis is also a key component of ME/CFS 138 . Higher levels of Ruminococcus gnavus and Bacteroides vulgatus and lower levels of Faecalibacterium prausnitzii have been found in people with long COVID compared with non-COVID-19 controls (from before the pandemic), with gut dysbiosis lasting at least 14 months; low levels of butyrate-producing bacteria are strongly correlated with long COVID at 6 months 24 . Persisting respiratory and neurological symptoms are each associated with specific gut pathogens 24 . Additionally, SARS-CoV-2 RNA is present in stool samples of patients with COVID-19 (ref. 139 ), with one study indicating persistence in the faeces of 12.7% of participants 4 months after diagnosis of COVID-19 and in 3.8% of participants at 7 months after diagnosis 61 . Most patients with long COVID symptoms and inflammatory bowel disease 7 months after infection had antigen persistence in the gut mucosa 140 . Higher levels of fungal translocation, from the gut and/or lung epithelium, have been found in the plasma of patients with long COVID compared with those without long COVID or SARS-CoV-2-negative controls, possibly inducing cytokine production 141 . Transferring gut bacteria from patients with long COVID to healthy mice resulted in lost cognitive functioning and impaired lung defences in the mice, who were partially treated with the commensal probiotic bacterium Bifidobacterium longum 25 .
The onset and time course of symptoms differ across individuals and by symptom type. Neurological symptoms often have a delayed onset of weeks to months: among participants with cognitive symptoms, 43% reported a delayed onset of cognitive symptoms at least 1 month after COVID-19, with the delay associated with younger age 83 . Several neurocognitive symptoms worsen over time and tend to persist longer, whereas gastrointestinal and respiratory symptoms are more likely to resolve 7 , 74 , 142 . Additionally, pain in joints, bones, ears, neck and back are more common at 1 year than at 2 months, as is paresthesia, hair loss, blurry vision and swelling of the legs, hands and feet 143 . Parosmia has an average onset of 3 months after the initial infection 144 ; unlike other neurocognitive symptoms, it often decreases over time 143 .
Few people with long COVID demonstrate full recovery, with one study finding that 85% of patients who had symptoms 2 months after the initial infection reported symptoms 1 year after symptom onset 143 . Future prognosis is uncertain, although diagnoses of ME/CFS and dysautonomia are generally lifelong.
Diagnostic tools and treatments
Although diagnostic tools exist for some components of long COVID (for example, tilt table tests for POTS 145 and MRI scans to detect cardiovascular impairment 68 ), diagnostic tools for long COVID are mostly in development, including imaging to detect microclots 63 , corneal microscopy to identify small fibre neuropathy 87 , new fragmentation of QRS complex on electrocardiograms as indicative of cardiac injury 146 and use of hyperpolarized MRI to detect pulmonary gas exchange abnormalities 147 . On the basis of the tests that are offered as standard care, the results for patients with long COVID are often normal; many providers are unaware of the symptom-specific testing and diagnostic recommendations from the ME/CFS community 148 . Early research into biomarkers suggests that levels of extracellular vesicles 85 and/or immune markers indicating high cytotoxicity 149 could be indicative of long COVID. Intriguingly, dogs can identify individuals with long COVID on the basis of sweat samples 150 . Biomarker research in ME/CFS may also be applicable to long COVID, including electrical impedance blood tests, saliva tests, erythrocyte deformation, sex-specific plasma lipid profiles and variables related to isocapnic buffering 151 , 152 , 153 , 154 . The importance of developing and validating biomarkers that can be used for the diagnosis of long COVID cannot be adequately emphasized — they will not only be helpful in establishing the diagnosis but will also be helpful for objectively defining treatment responses.
Although there are currently no broadly effective treatments for long COVID, treatments for certain components have been effective for subsets of populations (Table 1 ). Many strategies for ME/CFS are effective for individuals with long COVID, including pacing 7 , 37 and symptom-specific pharmacological options (for example, β-blockers for POTS, low-dose naltrexone for neuroinflammation 155 and intravenous immunoglobulin for immune dysfunction) and non-pharmacological options (including increasing salt intake for POTS, cognitive pacing for cognitive dysfunction and elimination diets for gastrointestinal symptoms) 96 . Low-dose naltrexone has been used in many diseases, including ME/CFS 155 , and has also shown promise in treating long COVID 156 . H 1 and H 2 antihistamines, often following protocols for mast cell activation syndrome and particularly involving famotidine, are used to alleviate a wide range of symptoms 19 , 157 , although they are not a cure. Another drug, BC007, potentially addresses autoimmunity by neutralizing G protein-coupled receptor autoantibody levels 158 . Anticoagulant regimens are a promising way to address abnormal clotting 159 ; in one study, resolution of symptoms was seen in all 24 patients receiving triple anticoagulant therapy 31 . Apheresis has also shown promise to alleviate long COVID symptoms; it has been theorized to help remove microclots 160 and has been shown to reduce autoantibodies in ME/CFS 161 . However, it is quite expensive, and its benefits are uncertain. Some supplements have shown promise in treating both long COVID and ME/CFS, including coenzyme Q 10 and d -ribose 162 , and may deserve further study.
Of note, exercise is harmful for patients with long COVID who have ME/CFS or postexertional malaise 110 , 163 and should not be used as a treatment 164 , 165 , 166 ; one study of people with long COVID noted that physical activity worsened the condition of 75% of patients, and less than 1% saw improvement 109 .
Pilot studies and case reports have revealed additional treatment options worth exploring. A case report noted resolution of long COVID following treatment with the antiviral Paxlovid 167 , and a study investigating the treatment of acute COVID-19 with Paxlovid showed a 25% reduction in the incidence of long COVID 168 ; Paxlovid should be investigated further for prevention and treatment of long COVID. A small trial of sulodexide in individuals with endothelial dysfunction saw a reduction in symptom severity 169 . Pilot studies of probiotics indicated potential in alleviating gastrointestinal and non-gastrointestinal symptoms 170 , 171 . Two patients with long COVID experienced substantial alleviation of dysautonomia symptoms following stellate ganglion block 172 . An early study noted that Pycnogenol statistically significantly improved physiological measurements (for example, reduction in oxidative stress) and quality of life (indicated by higher Karnofsky Performance Scale Index scores) 173 , 174 , as hypothesized on the basis of success in other clinical studies.
Taken together, the current treatment options are based on small-scale pilot studies in long COVID or what has been effective in other diseases; several additional trials are in progress 175 . There is a wide range of possible treatment options from ME/CFS covering various mechanisms, including improving natural killer cell function, removing autoantibodies, immunosuppressants, antivirals for reactivated herpesviruses, antioxidants, mitochondrial support and mitochondrial energy generation 176 , 177 ; most need to be clinically trialled, which should happen urgently. Many newer treatment options remain underexplored, including anticoagulants and SARS-CoV-2-specific antivirals, and a lack of funding is a significant limitation to robust trials.
Impact of vaccines, variants and reinfections
The impact of vaccination on the incidence of long COVID differs across studies, in part because of differing study methods, time since vaccination and definitions of long COVID. One study indicated no significant difference in the development of long COVID between vaccinated individuals and unvaccinated individuals 178 ; other studies indicate that vaccines provide partial protection, with a reduced risk of long COVID between 15% and 41% 4 , 5 , with long COVID continuing to impact 9% of people with COVID-19.
The different SARS-CoV-2 variants and level of (and time since) vaccination may impact the development of long COVID. The UK’s Office for National Statistics found that long COVID was 50% less common in double-vaccinated participants with Omicron BA.1 than in double-vaccinated participants Delta, but that there was no significant difference between triple-vaccinated participants; it also found long COVID was more common after Omicron BA.2 infection than after BA.1 infection in triple-vaccinated participants, with 9.3% developing long COVID from infection with the BA.2 variant 179 .
The impact of vaccination on long COVID symptoms in people who had already developed long COVID differs among patients, with 16.7% of patients experiencing a relief of symptoms, 21.4% experiencing a worsening of symptoms and the remainder experiencing unchanged symptoms 180 .
Reinfections are increasingly common 181 . The impact of multiple instances of COVID-19, including the rate of long COVID in those who recovered from a first infection but developed long COVID following reinfection, and the impact of reinfection on those with pre-existing long COVID is crucial to understand to inform future policy decisions. Early research shows an increasing risk of long COVID sequelae after the second and third infection, even in double-vaccinated and triple-vaccinated people 182 . Existing literature suggests multiple infections may cause additional harm or susceptibility to the ME/CFS-type presentation 33 , 183 .
There is also early evidence that certain immune responses in people with long COVID, including low levels of protective antibodies and elevated levels of autoantibodies, may suggest an increased susceptibility to reinfection 27 .
Challenges and recommendations
Issues with PCR and antibody testing throughout the pandemic, inaccurate pandemic narratives and widespread lack of postviral knowledge have caused downstream issues and biases in long COVID research and care.
Testing issues
Most patients with COVID-19 from the first waves did not have laboratory-confirmed infection, with PCR tests being difficult to access unless individuals were hospitalized. Only 1–3% of cases to March 2020 were likely detected 184 , and the CDC estimates that only 25% of cases in the USA were reported from February 2020 to September 2021 (ref. 185 ); that percentage has likely decreased with the rise in use of at-home rapid tests.
Although PCR tests are our best tool for detecting SARS-CoV-2 infections, their false negative rates are still high 186 . Further bias is caused by false negative rates being higher in women and adults younger than 40 years 187 , those with a low viral load 188 and children (Box 1 ), with several studies showing 52–90% of cases in children missed by PCR tests 189 , 190 . The high false negative PCR rate results in symptomatic patients with COVID-19, who seek a COVID-19 test but receive a false negative result, being included as a control in many studies. Those who have a positive PCR test result (who are more likely to be included in research) are more likely to be male or have a higher viral load. Additionally, the lack of test accessibility as well as the false negative rates has created a significant barrier to care, as many long COVID clinics require PCR tests for admission.
Similarly, there is a broad misconception that everyone makes and retains SARS-CoV-2 antibodies, and many clinicians and researchers are unaware of the limited utility of antibody tests to determine prior infection. Between 22% and 36% of people infected with SARS-CoV-2 do not seroconvert, and many others lose their antibodies over the first few months, with both non-seroconversion and seroreversion being more likely in women, children and individuals with mild infections 52 , 53 , 191 , 192 , 193 . At 4 and 8 months after infection, 19% and 61% of patients, respectively, who had mild infections and developed antibodies were found to have seroreverted, compared with 2% and 29% of patients, respectively who had severe infections 191 . Still, many clinicians and researchers use antibody tests to include or exclude patients with COVID-19 from control groups.
Furthermore, during periods of test inaccessibility, tests were given on the basis of patients having COVID-19-specific symptoms such as loss of smell and taste, fever, and respiratory symptoms, resulting in a bias towards people with those symptoms.
Misinformation on PCR and antibody tests has resulted in the categorization of patients with long COVID into non-COVID-19 control groups, biasing the research output. Because low or no antibody levels and viral load may be related to long COVID pathophysiology, including a clinically diagnosed cohort will strengthen the research.
Important miscues
The narrative that COVID-19 had only respiratory sequelae led to a delayed realization of the neurological, cardiovascular and other multisystem impacts of COVID-19. Many long COVID clinics and providers still disproportionately focus on respiratory rehabilitation, which results in skewed electronic health record data. Electronic health record data are also more comprehensive for those who were hospitalized with COVID-19 than for those who were in community care, leading to a bias towards the more traditional severe respiratory presentation and less focus on non-hospitalized patients, who tend to have neurological and/or ME/CFS-type presentations.
The narrative that initially mild COVID-19 cases, generally defined as not requiring hospitalization in the acute phase, would not have long-term consequences has also had downstream effects on research. These so-called mild cases that result in long COVID often have an underlying biology different from acute severe cases, but the same types of tests are being used to evaluate patients. This is despite basic tests such as D-dimer, C-reactive protein (CRP) and antinuclear antibody tests and complete blood count being known to often return normal results in patients with long COVID. Tests that return abnormal results in patients with ME/CFS and dysautonomia, such as total immunoglobulin tests, natural killer cell function tests, the tilt table or NASA lean test, the four-point salivary cortisol test, reactivated herpesvirus panels, small fibre neuropathy biopsy, and tests looking for abnormal brain perfusion 96 , should instead be prioritized. Other recurring issues include studies failing to include the full range of symptoms, particularly neurological and reproductive system symptoms, and not asking patients about symptom frequency, severity and disability. Cardinal symptoms such as postexertional malaise are not widely known, and therefore are rarely included in study designs.
Widespread lack of postviral knowledge and misinformation
The widespread lack of knowledge of viral-onset illnesses, especially ME/CFS and dysautonomia, as well as often imperfect coding, prevents these conditions from being identified and documented by clinicians; this means that they are frequently absent from electronic health record data. Further, because ME/CFS and dysautonomia research is not widely known or comprehensively taught in medical schools 194 , long COVID research is often not built on past findings, and tends to repeat old hypotheses. Additionally, long COVID research studies and medical histories tend to document only the risk factors for severe acute COVID-19, which are different from the risk factors for conditions that overlap with long COVID such as ME/CFS and dysautonomia (for example, connective tissue disorders such as Ehlers–Danlos syndrome, prior illnesses such as infectious mononucleosis and mast cell involvement) 33 , 195 , 196 .
Clinicians who are not familiar with ME/CFS and dysautonomia often misdiagnose mental health disorders in patients; four in five patients with POTS receive a diagnosis with a psychiatric or psychological condition before receiving a POTS diagnosis, with only 37% continuing to have the psychiatric or psychological diagnosis once they have received their POTS diagnosis 117 . Researchers who are unfamiliar with ME/CFS and dysautonomia often do not know to use specific validated tools when conducting mental health testing, as anxiety scales often include autonomic symptoms such as tachycardia, and depression scales often include symptoms such as fatigue, both of which overestimate mental health disorder prevalence in these conditions 197 , 198 .
Recommendations
Although research into long COVID has been expansive and has accelerated, the existing research is not enough to improve outcomes for people with long COVID. To ensure an adequate response to the long COVID crisis, we need research that builds on existing knowledge and is inclusive of the patient experience, training and education for the health-care and research workforce, a public communication campaign, and robust policies and funding to support research and care in long COVID.
We need a comprehensive long COVID research agenda that builds on the existing knowledge from ME/CFS, dysautonomia and other viral-onset conditions, including but not limited to brain and brainstem inflammation, appropriate neuroimaging techniques, neuroimmunology, metabolic profiling, impaired endothelial function, mitochondrial fragmentation, antiviral and metabolic phenotypes, hypoperfusion/cerebral blood flow, nanoneedle diagnostic testing, overlaps with connective tissue disorders, autoimmunity and autoantibodies, viral/microbial persistence, intracranial hypertension, hypermobility, craniocervical obstructions, altered T and B cells, metabolomics and proteomics, elevated blood lactate level, herpesvirus reactivations, immune changes in the early versus late postviral years, and changes to the gut microbiota. The mechanisms of and overlaps between long COVID and connective tissue involvement, mast cells and inflammatory conditions such as endometriosis are particularly understudied and should be focused on. Because of the high prevalence of ME/CFS, POTS and other postinfectious illnesses in patients with long COVID, long COVID research should include people who developed ME/CFS and other postinfectious illnesses from a trigger other than SARS-CoV-2 in comparator groups to improve understanding of the onset and pathophysiology of these illnesses 113 . Additionally, there is a known immune exhaustion process that occurs between the second and third year of illness in ME/CFS, with test results for cytokines being different between patients who have been sick for shorter durations (less than 2 years) than for those who have been sick for longer durations 43 . Because of this, studies should implement subanalyses based on the length of time participants have been ill. Because ME/CFS and dysautonomia research is not widely known across the biomedical field, long COVID research should be led by experts from these areas to build on existing research and create new diagnostic and imaging tools.
Robust clinical trials must be a priority moving forward as patients currently have few treatment options. In the absence of validated treatment options, patients and physicians conduct individual experiments, which result in the duplication of efforts without generalizable knowledge and pose undue risks to patients. Robust study design and knowledge sharing must be prioritized by both funding institutions and clinician-researchers.
It is critical that research on long COVID be representative of (or oversample) the populations who had COVID-19 and are developing long COVID at high rates, which is disproportionately people of colour 35 . Medical research has historically under-represented these populations, and over-representation of white and socio-economically privileged patients has been common in long COVID research. Researchers must work within communities of colour, LGBTQ+ communities and low-income communities to build trust and conduct culturally competent studies that will provide insights and treatments for long COVID for marginalized populations.
As a subset of patients will improve over time, and others will have episodic symptoms, care should be taken to incorporate the possibility of alleviation of symptoms into the study design, and care should be taken not to ascribe improvement to a particular cause without proper modelling.
Finally, it is critical that communities of patients with long COVID and associated conditions are meaningfully engaged in long COVID research and clinical trials. The knowledge of those who experience an illness is crucial in identifying proper study design and key research questions and solutions, improving the speed and direction of research.
Training and education of the health-care and research workforce
To prepare the next generation of health-care providers and researchers, medical schools must improve their education on pandemics, viruses and infection-initiated illnesses such as long COVID and ME/CFS, and competency evaluations should include these illnesses. As of 2013, only 6% of medical schools fully cover ME/CFS across the domains of treatment, research and curricula, which has created obstacles to care, accurate diagnosis, research and treatment 194 . To ensure people with long COVID and associated conditions can receive adequate care now, professional societies and government agencies must educate the health-care and research workforce on these illnesses, including the history of and current best practices for ME/CFS to not repeat mistakes of the past, which have worsened patients’ prognoses. The research community has made a misstep in its efforts to treat ME/CFS 199 , and some physicians, poorly educated in the aetiology and pathophysiology of the disorder, still advise patients to pursue harmful interventions such as graded exercise therapy and cognitive behavioural therapy, despite the injury that these interventions cause 200 and the fact that they are explicitly not advised as treatments 163 , 164 , 166 .
Public communications campaign
In addition to providing education on long COVID to the biomedical community, we need a public communications campaign that informs the public about the risks and outcomes of long COVID.
Policies and funding
Finally, we need policies and funding that will sustain long COVID research and enable people with long COVID to receive adequate care and support. For instance, in the USA, the creation of a national institute for complex chronic conditions within the NIH would go a long way in providing a durable funding mechanism and a robust research agenda. Further, we need to create and fund centres of excellence, which would provide inclusive, historically informed and culturally competent care, as well as conduct research and provide medical education to primary care providers. Additionally, research and clinical care do not exist in silos. It is critical to push forward policies that address both the social determinants of health and the social support that is needed for disabled people.
Conclusions
Long COVID is a multisystemic illness encompassing ME/CFS, dysautonomia, impacts on multiple organ systems, and vascular and clotting abnormalities. It has already debilitated millions of individuals worldwide, and that number is continuing to grow. On the basis of more than 2 years of research on long COVID and decades of research on conditions such as ME/CFS, a significant proportion of individuals with long COVID may have lifelong disabilities if no action is taken. Diagnostic and treatment options are currently insufficient, and many clinical trials are urgently needed to rigorously test treatments that address hypothesized underlying biological mechanisms, including viral persistence, neuroinflammation, excessive blood clotting and autoimmunity.
Acknowledgements
We would like to thank the long COVID and associated conditions patient and research community and the entire team at Patient-Led Research Collaborative. E.J.T. was supported by National Center for Advancing Translational Sciences (NCATS) grant UL1TR002550.
Author information
Authors and affiliations.
Patient-Led Research Collaborative, New York, NY, USA
Hannah E. Davis
Patient-Led Research Collaborative, Oakland, CA, USA
Lisa McCorkell
Scripps Research Translational Institute, Scripps Research, La Jolla, CA, USA
Julia Moore Vogel & Eric J. Topol
You can also search for this author in PubMed Google Scholar
Contributions
The authors contributed equally to all aspects of the article.
Corresponding author
Correspondence to Eric J. Topol .
Ethics declarations
Competing interests.
The authors declare no competing interests.
Peer review
Peer review information.
Nature Reviews Microbiology thanks Akiko Iwasaki, Ziyad Al-Aly and the other, anonymous, reviewer(s) for their contribution to the peer review of this work.
Additional information
Publisher’s note Springer Nature remains neutral with regard to jurisdictional claims in published maps and institutional affiliations.
Rights and permissions
Springer Nature or its licensor (e.g. a society or other partner) holds exclusive rights to this article under a publishing agreement with the author(s) or other rightsholder(s); author self-archiving of the accepted manuscript version of this article is solely governed by the terms of such publishing agreement and applicable law.
Reprints and permissions
About this article
Cite this article.
Davis, H.E., McCorkell, L., Vogel, J.M. et al. Long COVID: major findings, mechanisms and recommendations. Nat Rev Microbiol 21 , 133–146 (2023). https://doi.org/10.1038/s41579-022-00846-2
Download citation
Accepted : 05 December 2022
Published : 13 January 2023
Issue Date : March 2023
DOI : https://doi.org/10.1038/s41579-022-00846-2
Share this article
Anyone you share the following link with will be able to read this content:
Sorry, a shareable link is not currently available for this article.
Provided by the Springer Nature SharedIt content-sharing initiative
This article is cited by
Long covid symptoms after 8-month recovery: persistent static lung hyperinflation associated with small airway dysfunction.
- Jia-Yih Feng
- Diahn-Warng Perng
Respiratory Research (2024)
Variations in respiratory and functional symptoms at four months after hospitalisation due to COVID-19: a cross-sectional study
- Monika Fagevik Olsén
- Louise Lannefors
- Hanna C. Persson
BMC Pulmonary Medicine (2024)
Post-recovery health domain scores among outpatients by SARS-CoV-2 testing status during the pre-Delta period
- Jennifer P. King
- Jessie R. Chung
- Edward A. Belongia
BMC Infectious Diseases (2024)
Transforming health care systems towards high-performance organizations: qualitative study based on learning from COVID-19 pandemic in the Basque Country (Spain)
- Ane Fullaondo
- Irati Erreguerena
- Esteban de Manuel Keenoy
BMC Health Services Research (2024)
Psychometric properties of the COVID-19 Yorkshire Rehabilitation Scale: Post-Covid-19 syndrome in Iranian elderly population
- Negar Tamadoni
- Afsaneh Bakhtiari
- Hossein-Ali Nikbakht
Quick links
- Explore articles by subject
- Guide to authors
- Editorial policies
Sign up for the Nature Briefing newsletter — what matters in science, free to your inbox daily.


An official website of the United States government
The .gov means it’s official. Federal government websites often end in .gov or .mil. Before sharing sensitive information, make sure you’re on a federal government site.
The site is secure. The https:// ensures that you are connecting to the official website and that any information you provide is encrypted and transmitted securely.
- Publications
- Account settings
Preview improvements coming to the PMC website in October 2024. Learn More or Try it out now .
- Advanced Search
- Journal List
- Elsevier - PMC COVID-19 Collection

A hypothesis on the role of the human immune system in covid-19
Associated data.
The COVID-19 pandemic has not spared any continent. The disease has affected more than 7,500,000 individuals globally and killed approximately 450,000 individuals. The disease is caused by a very small virus named severe acute respiratory syndrome coronavirus 2 (SARS-CoV-2), which is an enveloped single-stranded RNA virus with a spike-like structure on its envelope that can interact with the angiotensin-converting enzyme 2 (ACE2) receptor after cleavage. ACE2 receptors are present in the human lungs and other organs. SARS-CoV-2 is a new virus that belongs to the subgenus Sarbecovirus; viruses in this subgenus have spread widely in the previous years and caused outbreaks of severe acute respiratory syndromes.
This hypothesis provides insights into a possible human immune response to SARS-CoV-2. It is concise in the immune system that stimulates respiratory mucosa sensitivity, which increases mucus secretion and decreases clarity. Sex determining region Y box 2 (SOX2) is required to regulate epithelial cell proliferation by increasing the number of goblet cells and decreasing the number of other types of cells. SOX2 may be involved in the pathogenic process of anosmia, which is the loss of the sense of smell, and ageusia, which is the loss of taste functions of the tongue; both are symptoms of COVID-19. The most serious point in this cascade is mucus hypersecretion, which can be an underlying cause of hypoxia and therefore, a cause of acute respiratory distress syndrome in infected patients. Mucus hypersecretion leads to mucus plug formation. Additionally, airway, alveoli, and endotracheal tube blockages can occur and be complicated by emphysema and hypoxia, which are resistant to oxygenation. These symptoms may be more severe if a patient is especially sensitive to coronavirus. This letter provides suggestions for drugs that might be useful in the treatment of COVID-19; however, further analysis is needed.
Respiratory tissue hypersensitivity
SARS-CoV-2 RNA was detected with a higher load in nasal swabs than in throat swabs in both symptomatic and asymptomatic patients [1] . Sungnank et al. [2] believe that the nasal epithelium is an initial target of infection and serves as a reservoir of SARS-CoV-2 for transmission and spread to the lower respiratory tract. Pneumatization is the process of development of air-filled sinuses in the skull bone [3] , and paranasal sinus pneumatization is usually seen at birth; for the maxillary sinuses, pneumatization may occur at 9 months of age to ethmoid and sphenoid sinuses. After the age of 5 years, pneumatization may be seen in the frontal sinuses [4] . Sinuses are part of the upper respiratory system; however, there is currently no information regarding the effect of COVID-19 on them; imaging by a paranasal CT scan may clarify this point and explain the initiation of infection and delayed negative PCR results, especially in children with a mild clinical presentation of the disease.
The respiratory mucosa is an organ that acts as a defensive mechanism after exposure to foreign bodies, such as viruses. It traps the virus via sticky secretions and then moves it out via the cilia [5] . Transmission of SARS-CoV-2 is associated with air droplets; mucus traps virus particles inside the body and outside of the body. Arumugham suggests that the novel coronavirus hyperstimulates the mucosa through a type I hypersensitivity reaction that is similar to the pathophysiology of dengue virus and some other viruses, which trigger an elevation in inflammatory biomarkers as an immune response [6] . This is supported by other studies showing that both SARS-CoV-1 and SARS-CoV-2 excessively stimulate the human inflammatory response; therefore, disease severity cannot be predicted by the viral load [7] , [8] , [9] . Chronic obstructive pulmonary disease (COPD) is an inflammatory disease associated with a high risk of pneumonia-related mortality and a lower immune response. This disease causes a persistent increase in mucus production; some treatments such as inhaled steroids may mitigate this risk [10] . If SARS-CoV-2 infects COPD patients, it will increase the severity of the infection by up to five-fold compared to infection in normal individuals [11] . Indeed, COVID-19 pathological investigations showed that the inflammatory reaction manifested as hypersensitivity pneumonitis rather than viral pneumonitis, as shown in a study conducted by Sufang Tian et al. [12] . This pathological information encouraged clinicians to add a steroid as an anti-inflammatory drug for the treatment regime of COVID-19 [12] , [13] . These pathological findings consider SARS-CoV-2 as an allergic antigen for respiratory mucosa; it stimulates mucus secretion and alters its chemical structure to enhance the entry of the virus into cells.
Mucus hypersecretion induced by antigens or allergens is a characteristic response of many respiratory diseases, including cystic fibrosis, bronchitis, and asthma. In all of these diseases, secretory cells are enhanced by different mechanisms, such as hyperplasia, metaplasia, or changes in the chemical structure of the mucus. The mucus secreted is composed of water and mucin, which has the properties of both a deformable solid and a gooey fluid. Mucins are enormous glycoproteins that are rich in threonine and serine residues. They are covalently associated with sugar chains that are anionic because of carboxyl or sulfate bundles. Several genes such as MUC5AC and MUC5B are known to encode mucins that are cysteine-rich, which allow the establishment of disulfide bonds that contribute to the polymers being produced in the airways. This arrangement and the profundity of the periciliary layer appear to play a pivotal role in the control of transport via mucociliary clearance within the airway routes. [14] , [15] . Mucus gel can build a macromolecule, which is a polymer of a complex network removable by S:S bond cleavage [5] . Recently, others have examined the role of accumulation of mucus and mucin secretion in the progression of obstructive lung disease. Lack of MUC5B contributes to mucus aggregation in the nasal airways [16] .
The mechanism underlying the regulation of mucogenesis is still not clear; however, many studies have focused on this aspect. This paper reviews the mechanism most compatible with the COVID-19-related clinical presentation. SOX2 is a member of the sex determining region Y box gene family that encodes transcription factors. SOX2 belongs to the SOX B1 subgroup, which contains both SOX1 and SOX3 [17] . SOX2 is essential for the morphogenesis of the normal trachea and lungs and is exclusively expressed in the epithelium of the trachea and airways [18] .
Tompkins et. al. concluded that allergen-induced goblet cell differentiation and mucus production were absent in the respiratory epithelium without SOX2. They found that SOX2 is required to activate the proliferative process of the goblet, ciliated, and Clara cells to extend mucus production following exposure to allergens. [19] , [20] .
In patients with COVID-19, symptoms of olfactory and gustatory dysfunction were observed in 85.6% and 88% of patients, respectively [21] . A study by Packard and his colleagues revealed that retroviral infection can affect SOX2 in mice, which might disturb the production of the olfactory epithelium [22] . Studies on humans have revealed that SOX2 in the olfactory epithelium plays a role in suppressing neuronal differentiation [23] . After data analysis of ACE 2 receptor expression, Brann et al. suggested that SARS-CoV-2 suppresses olfactory function by affecting olfactory supporting cells, perivascular, and stem cells, while not affecting neurons [24] .
COVID-19 pneumonia presents an atypical form of atypical respiratory distress syndrome because, in COVID-19, there is a dissociation between the severity of hypoxemia and relatively high compliance of alveoli to ventilator pressure; additionally, lung mechanics indicate that there is a well-preserved lung gas volume in affected patients [25] .
According to Rancé et al. [26] , sensitivity to allergens is more frequent among children older than 6 years; sensitivity increases over time as dermatological changes affect respiratory symptoms and result in more severe anaphylaxis in older children [27] . Reviewing these hypersensitivity theories is suggested as the cause of mild symptoms of COVID-19 in children.
Suggested treatments based on the hypothesis
Bromhexine hydrochloride is a mucolytic agent that causes only a few mild side effects. It may serve as either a new therapeutic agent during the early stages of COVID-19 or as a preventive agent by blocking the entry of SARS-CoV-2 into alveolar cells via the TMPRSS2 receptor. Therefore, it can be used as an antiviral agent [28] . It plays a role in the proteolytic activation and invasion of the human airway epithelium by the influenza virus [29] as well as SARS and MERS coronaviruses [30] . N-acetylcysteine was also used for the prevention and treatment of COVID-19, but only in limited clinical trials [31] . In addition, this paper suggests that mucolytics may play a role in the treatment of SARS-CoV-2 infection by decreasing the viscosity of mucus, which allows the virus to be washed out of the body as quickly as possible.
Steroids are effective in controlling allergic respiratory diseases such as asthma; in contrast, nonsteroidal anti-inflammatory (NSAID) drugs may exacerbate asthma symptoms because their mechanism involves increasing leukotrienes.
A retrospective study showed that low doses of a steroidal anti-inflammatory drug early in the disease process were useful against COVID-19 and were associated with faster improvement of pneumonia [32] . Some clinical observational data revealed that symptoms of COVID-19 were exacerbated after the addition of an NSAID to the therapy; therefore, it was advised to avoid its usage [33]. If effective, the use of mucolytics and steroids in the treatment of SARS-CoV-2 infection may support our hypothesis of mucosal hypersensitivity.
SARS-CoV-2 is a small virus that is not able to cause all the COVID-19 symptoms. The response of the human body and its malfunction are responsible for the main pathogenesis of COVID-19. The immune system of the host may treat SARS-CoV-2 as an allergen and stimulate allergic immunity as a defensive mechanism. This paper suggests that mucosal hypersensitivity plays a role in COVID-19. SARS-CoV-2 may induce SOX2 to increase goblet cell proliferation, increase mucus hypersecretion, and decrease Clara and specialized epithelial cells, as seen in other allergic and inflammatory diseases. This analysis explains the differences in the severity of disease presentation. This review suggests drugs that might be useful in the treatment of COVID-19, but further investigation is required.
Funding source
This study did not receive any grant or funding from any department or institute.
Acknowledgement
Conflict of interest statement.
The authors declare they have no conflict of interest to report.
Appendix A Supplementary data to this article can be found online at https://doi.org/10.1016/j.mehy.2020.110066 .
Appendix A. Supplementary data
The following are the Supplementary data to this article:
Ottawa will stop providing COVID-19 rapid tests to regions
No plans to replenish inventory, set to expire in december, says health canada.
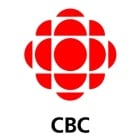
Social Sharing
The Canadian government plans to stop supplying provinces and territories with free COVID-19 rapid tests, which has an infection control epidemiologist worried about two-tiered health care, increased spread and increased health-care costs.
"The federal government continues to support Canada's rapid testing needs while the federal inventory remains," Health Canada spokesperson Nicholas Janveau told CBC News.
"That said, rapid test programming was and continues to be a provincial/territorial responsibility."
Ottawa currently has about 70 million of the tests, which people can use at home to screen for the virus. About 3.6 million of these have already expired and are ineligible for distribution.
The tests usually come in boxes of five or 10, reducing the inventory to 11.5 million test kits.
Given the current COVID-19 outlook, inventory levels, and indicated testing demands, the federal government does not anticipate the need for additional federal procurements at this time. - Nicholas Janveau, Health Canada spokesperson
Canada's estimated population, as of Jan. 1, is nearly 41 million, according to Statistics Canada.
More than three million of the tests are also already spoken for. A number of provinces and territories requested them and they are in various stages of deployment.
While Health Canada has authorized extending the shelf life of some rapid tests, all of the tests in the federal inventory will expire by December, said Janveau.
"Given the current COVID-19 outlook, inventory levels, and indicated testing demands, the federal government does not anticipate the need for additional federal procurements at this time," he said in an emailed statement.
Public health should not be based on 'ability to pay'
Infection control epidemiologist Colin Furness, a self-described "early and strong proponent of rapid tests," who has spent years saying more resources are needed to fight the pandemic, said he's not surprised Ottawa wants to "get out of the testing game" and doesn't blame the federal government since health care is a provincial and territorial responsibility.
The problem, said Furness, is that if the jurisdictions don't step up to provide free, or at least subsidized tests, people will be forced to buy them if they want to know whether they're COVID-positive and should take measures to prevent transmission. And this creates a divide.
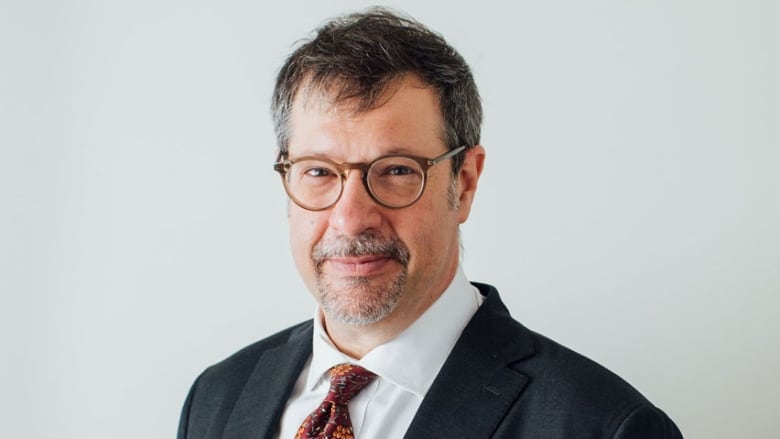
"Public health should not be based on your ability to pay," said Furness, an associate professor at the University of Toronto.
Some people can afford to buy rapid tests, available at some pharmacies, stores and online for about $7 plus tax per test, but "many can't."
"I think we should be very cognizant that rapid tests are part of what makes us healthy. It's part of health care. It's a diagnostic [tool] and it just doesn't make sense to commodify it," said Furness. "It's just going to create sickness and sickness is expensive for everybody."
N.B. to determine next steps for its program
At least one province is mulling the future of its COVID-19 rapid point-of-care testing program. Last week, New Brunswick said demand for the tests has declined steadily since last fall, and the province is "determining next steps."
New Brunswick has an adequate supply of the tests, which are all due to expire in September, said Department of Health spokesperson Sean Hatchard.
- New Brunswick mulls future of COVID-19 rapid tests, as virus kills 2, hospitalizes child under 4
He did not say how many, but the federal government's website shows New Brunswick had an estimated inventory of 147,000 tests from Ottawa, as of last June — the smallest stockpile in the country. The department has previously said it has an additional reserve of tests, however, beyond what is reported on the federal website.
Hatchard did not say if the province plans to order any more.
Lack of public health policies, messaging
Furness said it's no surprise demand for rapid tests has dropped because public health officials across the country aren't telling people to test.
"They're going along with the narrative that really there isn't any more COVID, or very little, and this is not something you need to worry about," he said.
Similarly, there are no COVID policies, said Furness.
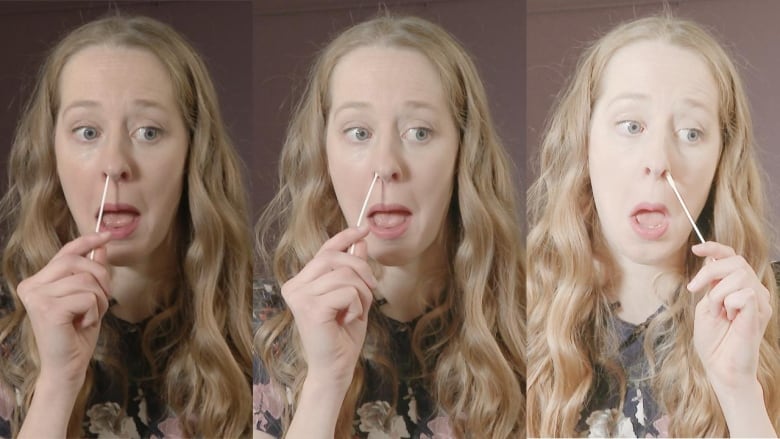
"I mean, what good is it to test positive if you still have to go to work because you need to feed your family and you don't have any paid sick days, right?
"What good is a rapid test when public health guidance says, 'Well, as long as you feel pretty good and you're not coughing too hard, you should go ahead and go to work?'"
Stay home when sick, regardless of testing
Testing is an important tool to limit the spread of COVID-19, along with personal protective measures and vaccination, the Health Canada spokesperson acknowledged.
"Rapid tests may be used to quickly identify if you have COVID-19, and isolate if the result is positive," said Janveau.
Still, the Public Health Agency of Canada recommends anyone who feels sick or has COVID-like symptoms stay home and limit their contact with others — regardless of whether they've tested positive or not, he said.
Federal strategic reserve no longer maintained
Ottawa ordered more than 811 million rapid tests throughout the pandemic, at a cost of about $5 billion. Of those, roughly 680 million went to provincial and territorial rapid testing programs.
A federal strategic reserve of rapid tests was maintained until Dec. 31, to ensure tests were readily available in Canada in case of future COVID-19 waves or an increase in demand, said Janveau.
While that reserve is no longer maintained, "provinces and territories have continued to receive rapid tests from the federal inventory upon request and while supplies last," he said.
To date, about 12.6 million expired tests have been disposed of from the federal inventory. Janveau did not respond to questions about the costs involved.
Related Stories
- Alberta is bringing in more rapid COVID-19 tests as millions destined to be tossed
- Q&A COVID-19 home testing as important as ever, infectious disease specialist says
- Q&A What an internist is saying about COVID-19 and rapid tests ahead of the holidays
- COVID-19 rapid tests now available without appointment at some N.B. sites
- Rapid tests are less accessible even as COVID wave ramps up across Canada, doctors say
- MyServiceNSW
- Manage account
- Logout of MyServiceNSW
Protecting yourself from COVID-19
How to help protect yourself and your community from COVID-19 infection.
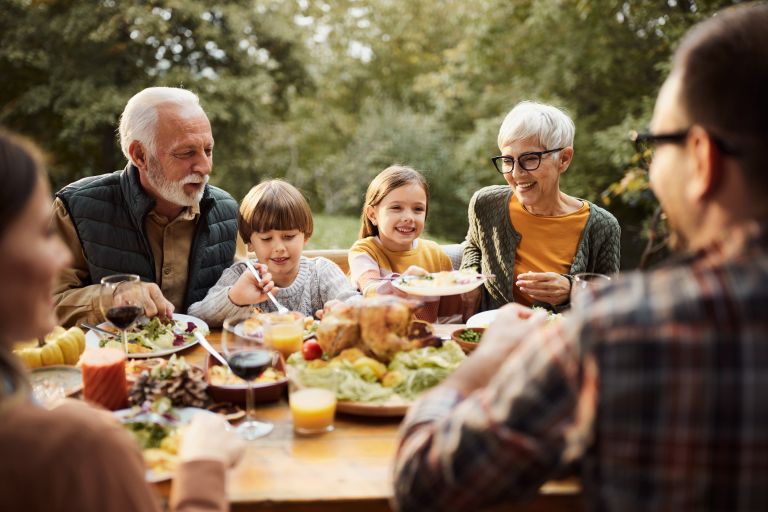
COVID-19 update – 16 May 2024
COVID-19 has increased to moderate levels.
Read the latest data from NSW Health .
Continue to protect other people. Please stay home if you have any cold or flu symptoms. Wear a mask if you need to leave home.
Steps to keep yourself safe

Stay home if you have cold or flu symptoms. Wear a mask if you need to leave home.

You may be required to wear a mask in high risk settings.

Stay up to date with your recommended COVID-19 vaccinations .

Don't visit people who are at higher risk if you have COVID-19 or symptoms.

Talk with your doctor now if you're at higher risk . You may need a PCR test and be eligible for antivirals.

Gather outdoors or in well-ventilated indoor spaces.
Guidance on protecting other people
Advice for parents of children in childcare and schools.
Find out what COVID-safe measures are in place at NSW early childhood education centres and schools.
Visiting people in high-risk settings
If you're visiting someone in aged care or disability care, find out what you can do to keep them safe.
Advice for workers
Guidance for workers affected by COVID-19.
Advice for people at higher risk of severe illness
Find the full list of people who are considered at higher risk .
People over 70
People with disability, pregnant women and new parents, aboriginal communities, easy read and translated resources, easy read information on covid-19, in-language and translated covid-19 support, contact us and find translation help.
- Service NSW – information and advice for NSW residents and businesses. Phone 13 77 88
- Healthdirect – government-funded 24-hour health advice. Phone 1800 022 222
- Disability Gateway – information for people with disability. Phone 1800 643 787
- Mental health support, services and programs
- Service NSW Savings Finder – find rebates and vouchers relevant to you
- Business Concierge – tailored advice from Business Connect advisors. Phone 13 77 88
- Translating and Interpreting Service (TIS National) – free service provided by the Australian Government. Phone 13 14 50
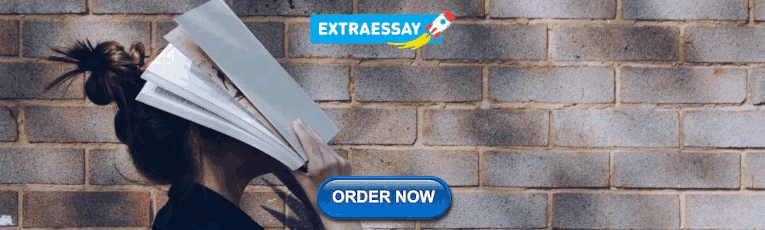
COMMENTS
Hypothesis generating for candidate gene studies of COVID-19 relies on our knowledge of the prepandemic CoV infections, our current understanding of the molecular mechanisms governing the pathophysiology of SARS-CoV-2 infection, and recent findings from genome wide studies. ... Berk M. Genetic gateways to COVID-19 infection: Implications for ...
On 26 May, US President Joe Biden tasked the US Intelligence Community to join efforts to find SARS-CoV-2's origins, whatever they might be, and report back in 90 days. Divisive COVID 'lab ...
Hypothesis. On January 30, 2020, the World Health Organization (WHO) announced a global public health emergency of severe acute respiratory syndrome-coronavirus-2 (SARS-CoV-2) causing illness of coronavirus disease-2019 (COVID-19) .As of October 1, 2020, worldwide 34,166,633 cases were reported and 1,018,876 have died with virus diagnosis.
The ongoing COVID-19 pandemic, caused by the novel severe acute respiratory syndrome coronavirus type 2 (SARS-CoV-2), has affected 212 countries worldwide at various degrees as of 8 May 2020. 1 In this paper we discuss a hypothesis that prior viral infections—either by SARS-CoV-2 or different strains of coronaviruses, or potentially even other respiratory viruses—may predispose to more ...
COVID19 pandemic. After last year's assembly, ... deeper investigation into the hypothesis that ... it dies or clears the infection. And, even if the animal tests positive, viruses found in ...
The severe acute respiratory syndrome coronavirus 2 (SARS-CoV-2) outbreak, which was first reported in Wuhan, China, in December, 2019, has had an enormous impact on China and the whole world. The disease caused by SARS-CoV-2 is named coronavirus disease 2019 (COVID-19). By March 19, 2020, the number of confirmed cases had increased to over 200 ...
The COVID-19 pandemic has shown a markedly low proportion of cases among children 1,2,3,4.Age disparities in observed cases could be explained by children having lower susceptibility to infection ...
The COVID-19 pandemic has the potential to affect the human microbiome in infected and uninfected individuals, having a substantial impact on human health over the long term. This pandemic intersects with a decades-long decline in microbial diversity and ancestral microbes due to hygiene, antibiotics, and urban living (the hygiene hypothesis).
This hypothesis was strengthened by direct study of mucosal responses at the time of COVID-19 diagnosis: ... A quarter of children experienced persistent symptoms months after hospitalization with acute COVID-19 infection, with almost 1 in 10 experiencing multisystem involvement . In total, over 200 different symptoms have been reported ...
Currently, the international scientific community is engaged in elucidating the molecular mechanisms of the pathophysiology of SARS-CoV-2 infection as a basis of scientific developments for the future control of COVID-19. Global exome and genome analysis efforts work to define the human genetics of protective immunity to SARS-CoV-2 infection.
The timeframe for reinfection is fundamental to numerous aspects of public health decision making. As the COVID-19 pandemic continues, reinfection is likely to become increasingly common. Maintaining public health measures that curb transmission—including among individuals who were previously infected with SARS-CoV-2—coupled with persistent efforts to accelerate vaccination worldwide is ...
Some COVID-19 patients progressed rapidly to ARDS and septic shock, which was eventually followed by multiple organ failure [7], [8]. Therefore, the effort on initial management of COVID-19 must be addressed to the early recognition of the suspect and contain the disease spread by immediate isolation and infection control measures [56].
Given the above-mentioned hypothesis that COVID-19 vaccine confers protective effect on COVID-19-indcuded ADs, the level of COVID-19 related risk could be attenuated by the high vaccination rate among COVID-19 infected patients in our study cohort (Table 1, 81.9% completed at least two dose vaccination). This assumption is further verified by ...
Background One of the few studies to estimate infection risk with SARS-CoV-2 in the general population was the UK Office of National Statistics Infection Survey. This survey provided data that allowed us to describe and interpret apparent risk factors for testing positive for SARS-CoV-2 in a period when variants and COVID-19 controls experienced large changes. Method The ONS published ...
COVID-19 infection is caused by the novel severe acute respiratory syndrome coronavirus 2 (SAR-CoV-2). This novel virus has transformed into different resistant variants (e.g., omicron; delta; alpha; epsilon) since its first emergence in 2019. The National Institutes of Health and Infectious Diseases Society of America guidelines currently recommend adding either baricitinib or tocilizumab to ...
Considerable evidence from other infectious diseases substantiates this hypothesis and recent evidence from COVID-19 points in the same direction. ... Recent studies using Syrian hamster models of COVID-19 infection have already shown that higher doses of administered SARS-CoV-2 virus led to more severe disease even though viral loads during ...
Katharine Lang investigates how SARS-CoV-2 infection can affect the brain, how it might have these effects, and what can be done to minimise the risk Covid may be primarily a respiratory infection, but a common symptom is "brain fog"—problems with memory or concentration—which can persist for weeks or months as part of long covid.1 And it's not the only neurological effect.
SARS-CoV-2. A COVID-19 infection tends to last about 4-14 days, while long COVID is defined as persistent symptoms lasting 4 weeks or longer following COVID-19 infection with no other infection ...
Abstract. Coronavirus disease 2019 (COVID-19), the current pandemic disease, is caused by severe acute respiratory syndrome coronavirus 2 (SARS-CoV-2) infection. Type I and III interferons (IFNs ...
In this commentary we argue that the hygiene hypothesis may apply to COVID-19 susceptibility and also that residence in low hygienic conditions acts to train innate immune defenses to minimize the severity of infection. We advocate that approaches, which elevate innate immune functions, should be used to minimize the consequences of COVID-19 ...
The most distinctive comorbidities of 32 non-survivors from a group of 52 intensive care unit patients with novel coronavirus disease 2019 (COVID-19) in the study by Xiaobo Yang and colleagues1 were cerebrovascular diseases (22%) and diabetes (22%). Another study2 included 1099 patients with confirmed COVID-19, of whom 173 had severe disease with comorbidities of hypertension (23·7% ...
The ongoing COVID-19 pandemic, caused by the novel severe acute respiratory syndrome coronavirus type 2 (SARS-CoV-2), has affected 212 countries worldwide at various degrees as of 8 May 2020.1 In this paper we discuss a hypothesis that prior viral infections—either by SARS-CoV-2 or different strains of coronaviruses, or potentially even other respiratory viruses—may predispose to more ...
One hypothesis to resolve this discrepancy is that repeated infections somehow alter the immune system to make infections more difficult. ... indicates that the average person in this combined population has a 0.92% chance of a COVID-19 infection every year; a 2252 case rate means the average individual will suffer from 2.3 COVID-19 infections ...
First, we respectfully disagree about the timing to define the transition from "usual" COVID-19 to pCOVID, that the Israelian colleagues set at 14 days without providing any specific reference to support this statement, only positing that the majority of patients resolve infection within this time frame
4. Globally, JN.1 and its sub-lineages, including KP.1 and KP.2, remain the predominant COVID-19 variants. Locally, the combined proportion of KP.1 and KP.2 currently accounts for over two-thirds of COVID-19 cases in Singapore. As of 3 May 2024, the World Health Organization has classified KP.2 as a Variant Under Monitoring.
Long COVID is an often debilitating illness that occurs in at least 10% of severe acute respiratory syndrome coronavirus 2 (SARS-CoV-2) infections. More than 200 symptoms have been identified with ...
Background and Aims: An increase in the number of cases of acute hepatitis of unknown origin (HUO) in children was observed in 2021. Adenovirus and adeno-associated virus 2 (AAV2) infections have been suggested as possible triggers. However, the potential etiology is still unclear. We aimed to characterize a cohort of children with HUO in Israel in view of the COVID-19 pandemic.
The COVID-19 pandemic has not spared any continent. The disease has affected more than 7,500,000 individuals globally and killed approximately 450,000 individuals. ... If effective, the use of mucolytics and steroids in the treatment of SARS-CoV-2 infection may support our hypothesis of mucosal hypersensitivity. Conclusion. SARS-CoV-2 is a ...
The federal government plans to stop supplying provinces and territories with free COVID-19 rapid tests, which has an infection control epidemiologist worried about two-tiered health care ...
Wear a mask if you need to leave home. You may be required to wear a mask in high risk settings. Stay up to date with your recommended COVID-19 vaccinations. Don't visit people who are at higher risk if you have COVID-19 or symptoms. Talk with your doctor now if you're at higher risk. You may need a PCR test and be eligible for antivirals.